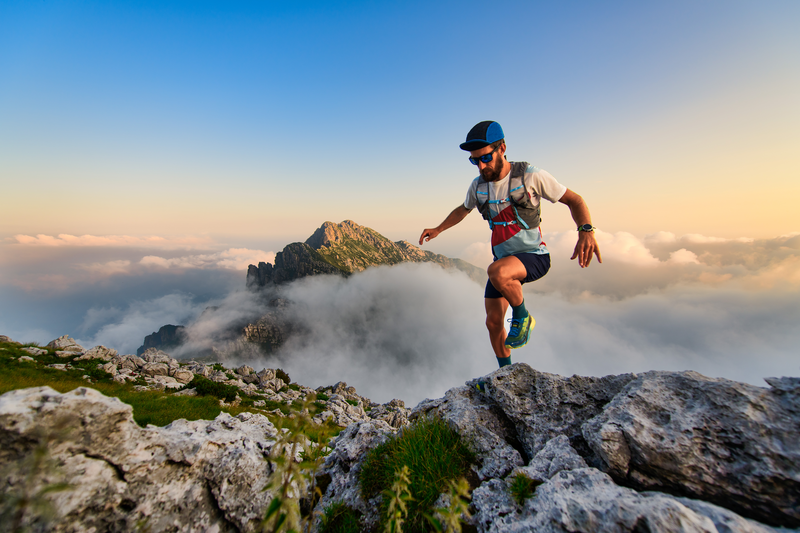
95% of researchers rate our articles as excellent or good
Learn more about the work of our research integrity team to safeguard the quality of each article we publish.
Find out more
REVIEW article
Front. Pharmacol. , 28 February 2025
Sec. Cardiovascular and Smooth Muscle Pharmacology
Volume 16 - 2025 | https://doi.org/10.3389/fphar.2025.1523727
This article is part of the Research Topic Innovative Approaches and Molecular Mechanisms in Cardiovascular Pharmacology View all 8 articles
Coronary microvascular disease (CMVD) has emerged as a new target for the occurrence and development of heart failure treatment. Various indicators such as Index of Microvascular Resistance, Coronary Flow Reserve, Microvascular Resistance Reserve, Hyperemic Microvascular Resistance and Coronary Flow Velocity Reserve can be used to assess CMVD. Coronary microcirculation dysfunction is one of the important pathogenic mechanisms of heart failure. Sodium-Glucose Co-Transporter 2 (SGLT2) Inhibitors have been widely used in the treatment of various types of heart failure, but their specific pharmacological mechanisms are not yet fully understood. Studies have shown that SGLT2 inhibitors may be involved in the pathophysiology of CMVD by regulating cellular pathophysiological processes such as oxidative stress, mitochondrial function, energy metabolism, vascular genesis, and signalling pathways. Therefore, coronary microvascular dysfunction may be one of the treatment targets of using SGLT2 inhibitors in heart failure. Several animal experiments have found that SGLT2 inhibitors can improve microcirculatory dysfunction. However, the results of several clinical trials on the effects of SGLT2 inhibitors on coronary microcirculation have been different. Therefore, it is still lack of conclusive evidence on the effects of SGLT2 inhibitors on microcirculatory dysfunction. This review aims to summarize the completed and ongoing experiments regarding the effects of SGLT2 inhibitors on coronary microcirculation, in order to better elucidate the impact of SGLT2 inhibitors on microcirculation. It seeks to provide valuable information for the pharmacological mechanisms of SGLT2 inhibitors, the study of diseases related to coronary microcirculation disorders, and the treatment of heart failure.
Heart failure is the end stage of various heart diseases and has become an important public health issue affecting the health of residents. Taking China as an example, the standardized prevalence rate of heart failure in the population aged 25 and above is 1.1%, which is approximately 12.1 million patients. The crude incidence rate is 248 cases per 100,000 people, with about 3 million new cases annually. The prognosis of heart failure patients is poor, with a mortality rate exceeding 50% in 5 years and a readmission rate of up to 69% within 1 year. Of those, 40.5% of the patients had more than 3 hospitalizations with an average hospitalization cost of 29,746 yuan and an average outpatient cost of 6,023 yuan per year (Wang et al., 2021; Mamas et al., 2017; Huang et al., 2017). In recent years, significant progress has been made in the treatment of management in heart failure, with the use of SGLT2 inhibitors being an important discovery in treatment (Zinman et al., 2015a; Neal et al., 2017a; Wiviott et al., 2019a; McMurray et al., 2019; Packer et al., 2020; Anker et al., 2021; Solomon et al., 2022). SGLT2 inhibitors has shown significant efficiency in several landmark heart failure randomized clinical trials and is recommended by major guidelines (Tsutsui, 2022; Heidenreich et al., 2022; Moghaddam et al., 2023; Patel et al., 2023). The simplicity, safety, and tolerability of SGLT2 inhibitors effectively combat clinical inertia in heart failure GDMT treatment (Khan et al., 2020), and it is widely used in the treatment of various types of heart failure. However, the full benefits of SGLT2 inhibitors on cardiovascular outcomes are not yet fully understood. In recent years, a series of studies have been conducted on the benefits of SGLT2 inhibitors in coronary microcirculation; however, the conclusions of these studies lack definitive opinions. This review will summarize the basic and clinical research on the effects of SGLT2 inhibitors on coronary microcirculation, which will help the development of heart failure treatment.
The coronary circulation is composed of epicardial coronary arteries (vessel diameter 0.5 mm–5 mm), arterioles (vessel diameter approximately 0.1 mm–0.5 mm), small arteries (vessel diameter <0.1 mm), and capillaries (vessel diameter <10um) (anatomy as shown in Figure 1). The arterioles, small arteries, and capillaries are the main components of the coronary microcirculation, which is responsible for regulating pressure and blood flow of the coronary arterioles. The arterioles are sensitive to pressure changes, while the distal arterioles are sensitive to flow changes. Capillaries provide 90% of the blood supply to the myocardium and serve as the sites for exchange of oxygen, nutrients, and metabolic substances in the myocardium. The coronary microcirculation plays an important role in regulating coronary perfusion pressure and blood flow through mechanisms of flow-mediated vasodilation, autoregulation of coronary blood flow, and regulation of myocardial oxygen consumption and metabolic substances (Chilian, 1997; Paulus and Tschope, 2013).
Coronary Flow Reserve (CFR) denotes the ratio of coronary blood flow (CBF) or myocardial blood flow (MBF) to the corresponding index at rest at or near maximal coronary artery dilation. It serves as a comprehensive indicator of the reserve function of the entire coronary artery system. CFR can be measured through PET, SPECT, cardiac magnetic resonance imaging (CMR), and coronary angiography. CFR is an independent predictor of major adverse cardiovascular events in patients with known or suspected coronary artery disease (CAD) (Indorkar et al., 2019). CFR is influenced by multiple factors, including coronary blood flow at rest (increased resting blood flow can lead to a decrease in CFR), the cross-sectional area of resistance vessels within a unit volume of myocardium (thickening of the vessel wall can lead to a decrease in CFR), the pressure outside the coronary vessels (increased wall tension can lead to a decrease in CFR), and coronary perfusion pressure (a decrease in blood pressure can lead to a decrease in CFR) (Marcus et al., 1987). Research findings indicate that in patients with coronary artery disease, if CFR <2.0, the 10-year major adverse cardiovascular event rate and cardiovascular mortality rate increase (Lee et al., 2022).
The index of microvascular resistance (IMR) in the coronary arteries is a new indicator proposed in recent years to evaluate the function of distal microvasculature in coronary artery stenosis. IMR is defined as the product of the pressure measured inside the coronary artery and the inverse of the mean transit time (Tm) in a hyperemic state. It reflects the specificity of myocardial microcirculation. Microvascular resistance is directly proportional to the pressure gradient across the myocardium (Pd-Pv) and inversely proportional to the blood flow (f) (Eshtehardi et al., 2011). In the absence of significant epicardial coronary artery stenosis, IMR has higher repeatability than CFR. IMR can specifically evaluate the function of microvasculature distal to the stenotic lesion and accurately predict myocardial tissue perfusion levels, ventricular remodeling, and recovery of cardiac function after acute myocardial infarction reperfusion therapy (Fearon et al., 2008; Ito et al., 2010). An IMR ≥25 is considered the threshold for diagnosing microvascular dysfunction (Lee et al., 2022).
The MBF refers to the small blood vessel network in the heart muscle, which is responsible for providing oxygen and nutrients to the myocardium. It responds to changes in the body’s metabolism and oxygen demand to meet the needs of the myocardial cells (Pelletier-Galarneau et al., 2019). MBF can be measured using Single-photon emission CT, PET, Transthoracic Doppler Echocardiography (TTDE), and CMR. The results of the above measurement are indicators of the quantity and velocity of the myocardial blood flow. Higher MBF usually indicates that the myocardial tissue is adequately supplied with blood to meet its metabolic demands, which is crucial for maintaining myocardial function. Conversely, lower MBF may indicate presence of coronary microvascular dysfunction or coronary artery disease.
Microvascular resistance reserve (MRR) is defined as the ratio of actual resting microvascular resistance to hyperemic microvascular resistance (HMR). The calculation is as follows: MRR = (Qmax/Qrest) × (Pa,rest/Pd,hyper). Pa,rest represents the aortic pressure at rest, Pd,hyper represents the distal coronary artery pressure measured during hyperemia, while Qrest and Qmax represents the actual blood flow measured at rest and during hyperemia, respectively. MRR can be invasively measured using coronary artery doppler, continuous thermodilution, or bolus thermodilution (De Bruyne et al., 2021).
HMR can measure the average flow velocity and pressure during the maximum hyperemic phase of the cardiac cycle in the distal coronary artery (or in the absence of stenotic lesions, in the distal coronary artery) using a doppler transducer and a pressure sensor mounted on a coronary guidewire. The calculation of HMR is as follows: HMR = pressure/flow rate (Xaplanteris et al., 2018). CFR <2.5 and HMR >1.7 mmHg/cm/s suggest coronary microvascular dysfunction. HMR is not affected by resting coronary blood flow.
Coronary Flow Velocity Reserve (CFVR) is typically assessed through TTDE or coronary angiography. CFVR represents the ratio of peak diastolic coronary artery blood flow velocity during hyperemia to the velocity at rest. Elevated CFVR value signals superior coronary microcirculation function and increased blood flow reserve, while decreased in CFVR may indicate presence of coronary microcirculation abnormalities, particularly prevalent in diabetic individuals (Jurgens et al., 2021).
CMVD refers to the comprehensive effect of multiple pathogenic factors that lead to structural and/or functional abnormalities in the coronary pre-small arteries and small arteries resulting in clinical syndrome of effort angina or objective evidence of myocardial ischemia. Initially CMVD was named X Syndrome by Kemp HG in 1973, it was later referred to as microvascular dysfunction by Camici PG in 2007. In 2013, the European Society of Cardiology officially recognized it as microvascular dysfunction in stable coronary artery disease treatment guidelines, incorporated it into the spectrum of clinical coronary heart disease, and provided initial diagnostic and therapeutic recommendations (Montalescot et al., 2013). In 2017, the Chinese experts formally consented and designated it as “coronary microvascular disease” (Wenqiang et al., 2013).
The main pathophysiology of CMVD involves structural or functional abnormalities in the microvesses of the heart. Contributing factors include endothelial dysfunction, inflammatory responses, microvascular constriction, and platelet activation. These factors collectively result in compromised contraction and relaxation capabilities of the coronary microvessels, which impacts myocardial perfusion and metabolism. The aberrant microvascular function observed in CMVD patients can lead to myocardial ischemia and hypoxia with symptoms such as angina, myocardial ischemia, and potential myocardial damage. Furthermore, CMVD is closely linked to the occurrence of cardiovascular events and the progression of heart failure. Its involvement in the pathogenesis and progression of heart failure, diabetes, and coronary artery disease makes it a novel therapeutic target in these disease states.
Taking Heart Failure with Preserved Ejection Fraction (HFpEF) as an example, the PROMIS-HFpEF study confirmed the correlation between CMVD and HFpEF. The PROMIS-HFpEF study is a prospective, multicenter, multinational study aimed at investigating the prevalence and related factors of coronary microvascular dysfunction in patients with HFpEF (Shah et al., 2018). The study utilized echocardiography and adenosine-based transthoracic doppler echocardiography to measure CFR. The results showed that of the 202 HFpEF patients, 151 individuals (74.8%) had coronary microvascular dysfunction (CFR <2.5). Furthermore, in HFpEF patients, the prevalence of CMVD was high and was associated with atrial fibrillation, smoking, and abnormalities in certain physiological markers (i.e., urine albumin/creatinine ratio, troponin, and NT-proBNP levels). This study was recognized as one of the top ten cardiovascular disease advancements in 2018.
The pathogenesis of HFpEF is intricate with systemic inflammation playing a crucial role in its development (Fraction, 2023). Various conditions such as obesity, diabetes, chronic obstructive pulmonary disease, and salt-sensitive hypertension can trigger a systemic pro-inflammatory state leading to inflammation in the coronary microvascular endothelium. The above pathogenesis mechanism in turn, reduces the bioavailability of nitric oxide in neighboring myocardial cells, as well as the levels of cyclic guanosine monophosphate and protein kinase G (PKG) activity. The decrease in NO bioavailability and PKG activity promotes hypertrophic changes and increases resting tension in myocardial cells, contributing to myocardial stiffness and interstitial fibrosis, and ultimately leading to diastolic left ventricular (LV) stiffness and the progression of heart failure (Paulus and Tschope, 2013). Coronary microvascular dysfunction is initiated by a low-grade inflammatory response to cardiovascular risk factors, which results in local myocardial ischemia and hypoxia, and further exacerbate dysfunction in the coronary microvascular endothelium. Impaired coronary endothelial function leads to reduced microvascular NO bioavailability, as well as decreased levels of cGMP, PKG, and transforming growth factor (TGF)-β in myocardial cells, and ultimately promotes myocardial hypertrophy and fibrosis that contributes to the development of HFpEF (Paulus and Tschope, 2013; Fraction, 2023; van Heerebeek et al., 2012; Liu et al., 2017; Harvey et al., 2016; Forrester et al., 2018; Zile et al., 2015; Paulus, 2020; Schulz et al., 2008; Nikolic et al., 2022). The significant role of coronary microvascular dysfunction in the pathogenesis of HFpEF was highlighted in numerous studies (Table 1). (Paulus and Tschope, 2013; Shah et al., 2018; Lee et al., 2016; Taqueti et al., 2018; Mohammed et al., 2015; Sucato et al., 2015; Kato et al., 2016; Srivaratharajah et al., 2016; Dryer et al., 2018; Löffler et al., 2019; Kato et al., 2021; Ozcan et al., 2021; Ratchford et al., 2023; Arnold et al., 2022).
Table 1. Study on coronary microvascular dysfunction in patients with ejection fraction preserved heart failure.
In conclusion, CMVD is involved in the occurrence and development of various diseases, making it an important therapeutic target. Therefore, effective intervention in coronary microvascular dysfunction is crucial for the prevention and treatment of cardiovascular diseases and their related complications.
SGLT2 inhibitors are a class of anti-diabetic drugs. With ongoing research, the benefits of SGLT2 inhibitors in heart failure are becoming increasingly significant. Clinical studies have accumulated evidence from patients with diabetes and without diabetes, from HFrEF to HFpEF, and from chronic to acute heart failure. This shift from being primarily antidiabetic agents to heart failure treatment drugs has been incorporated into guidelines, providing a new tool for improving the prognosis of heart failure patients. Studies such as EMPA-REG OUTCOME, CANVAS, and DECLARE-TIMI58 have shown the cardiovascular safety of SGLT2 inhibitors in diabetic patients (Zinman et al., 2015b; Neal et al., 2017b; Wiviott et al., 2019b), particularly in reducing the risk of heart failure hospitalization. Trials like DAPA-HF, EMPEROR-Reduced, EMPEROR Preserved, and DELIVER have demonstrated the ability of SGLT2 inhibitors to reduce the risk of heart failure hospitalization and cardiovascular death in HFrEF, HFpEF, and HFmrEF (McMurray et al., 2019; Packer et al., 2020; Anker et al., 2021; Solomon et al., 2022). The SOLOIST-WHF study confirmed the benefits of SGLT2 inhibitors in patients with worsening of heart failure. SGLT2 inhibitors have become the first class of heart failure treatment drugs to span across all ejection fractions (Bhatt et al., 2021).
The 2021 ESC included SGLT2 inhibitors for the first time in the international authoritative heart failure guidelines, listing them as cornerstone medications for heart failure with reduced ejection fraction. It emphasized that SGLT2 inhibitors, as one of the four cornerstone drugs, should be used in combination early on. The 2022 ACC guidelines recommended SGLT2 inhibitors as a Class 2A recommendation for treating patients with heart failure with preserved ejection fraction. In August 2022, the Chinese Heart Failure Center Alliance Expert Committee established the Chinese expert consensus on the clinical application of SGLT2 inhibitors in heart failure to standardize their rational use. The consensus recommended a clinical application pathway for using SGLT2 inhibitors in heart failure. The 2023 update of the Chinese National Heart Failure Guidelines highlighted SGLT2 inhibitors as a first-line choice for treating HFpEF and HFmrEF (Fraction, 2023).
The expression of SGLT2 is highly specific to renal tissue and is expressed in pancreatic alpha cells, but not in human heart. Only SGLT-1 is expressed at a low level (Di Franco et al., 2017). Therefore, the potential effects of SGLT-2 inhibitors on cardiac function may be indirect, mainly mediated by systemic hemodynamics and metabolism (Lee et al., 2017). In the rat model of myocardial infarction, SGLT-2 receptor seems to reduce the release of myocardial superoxide, the presence of myofibroblasts and inflammatory macrophages, and subsequent myocardial fibrosis. In diabetic mice, SGLT-2 inhibition decreased the expression of transforming growth factor-β, the levels of type I and III collagen and overall cardiac fibrosis (Li et al., 2019). It is not clear whether this effect also occurs in humans.
Studies in structural and functional research have shown that SGLT2 inhibitors can improve cardiac structure progression by reducing left ventricular mass, inhibiting left ventricular wall thickness, and slowing the progression of left ventricular wall thickness and cavity radius (Verma et al., 2019; Soga et al., 2018; Brown et al., 2020; Matsutani et al., 2018). SGLT2 inhibitors mainly improve cardiac function by increasing LVEF and reducing left ventricular end-diastolic volume, left ventricular end-systolic volume, left atrial volume index, and left ventricular mass index (Lee et al., 2021; Lan et al., 2021; von Lewinski et al., 2022; Ersbøll et al., 2022).
Diastolic dysfunction is the core hemodynamic feature of ejection fraction retention (HFpEF) in heart failure, which involves many physiological processes, including left ventricular remodeling, cardiac metabolic dysfunction and extracellular matrix fibrosis. In animal models and patients of HFpEF, reduced bioavailability of endothelium-dependent nitric oxide (NO) has been shown to promote the proliferation of fibroblasts and myofibroblasts and affect the diastolic function of energy-dependent cardiomyocytes by regulating the low phosphorylation of cytoskeletal protein Titin (Borbély et al., 2009). Studies from endomyocardial biopsy samples from patients with HFpEF have shown that inflammatory microvascular endothelial cells contribute to the migration of monocytes and promote the release of transforming growth factor-β (TGF--β), which promotes the transformation, collagen production and cross-linking of fibroblasts to myofibroblasts (Mohammed et al., 2015; Borbély et al., 2009; Kirkman et al., 2020). In addition, this pro-inflammatory and pro-oxidative environment may make dysfunctional coronary microvessels more prone to recurrent myocardial ischemia and microinfarction, further leading to interstitial fibrosis, changes in substrate metabolism and decreased systolic reserves, resulting in the occurrence of HFpEF (Mohammed et al., 2015). Research in pathophysiology has shown that the effects of SGLT2 inhibitors on cardiomyocyte hypertrophy and cardiac fibroblast fibrosis are mainly achieved through the regulation of multiple signaling pathways, including the AMPK/mTOR pathway, JAK/STAT pathway, SGK1 pathway, and sGC/cGMP/PKG pathway, leading to a reduction in cardiomyocyte volume increase; lowering of ventricular mass index; reduction in the extent of cardiac fibrosis and decrease in the deposition of extracellular matrix in cardiomyocytes, thereby inhibiting cardiomyocyte hypertrophy and non-cardiomyocyte proliferation, reducing ventricular mass increase and cardiac fibrosis (Yurista et al., 2019; Li et al., 2021; Hsieh et al., 2022; Lin et al., 2022; Xue et al., 2019). Additionally, SGLT2 inhibitors also improve cardiac structure and function by regulating cellular pathophysiological processes such as autophagy, apoptosis, oxidative stress, mitochondrial function, energy metabolism, angiogenesis, and signaling pathway dysregulation (Li et al., 2021; Nah et al., 2020; Liu et al., 2021; Long et al., 2022; Xing et al., 2021; Bugga et al., 2022). These regulatory effects help alleviate inflammation and cellular dysfunction during the process of cardiac remodeling (Figure 1).
SGLT2i has shown a wide range of benefits in cardiovascular treatment, including its potential effects on coronary microcirculation function. Coronary microcirculation disturbance plays an important role in the development of diabetes and cardiovascular disease. However, the effect of SGLT2 inhibitors on coronary microcirculation has not been determined. Several animal experiments and clinical studies have explored the effects of SGLT2 inhibitors on Coronary microcirculation disturbance, but the results are different and lack of a conclusive opinion. This review aims to summarize the current research and provide valuable insights for the pharmacological mechanism of SGLT2 inhibitors and the pathological mechanism of CMVD.
L-arginine is known to be the physiological precursor of NO formation in endothelium-dependent vasodilation. NO has a variety of intracellular effects, which can lead to vasodilation, endothelial regeneration, inhibition of leukocyte chemotaxis and platelet adhesion. In a study conducted by Adingupu et al., in 2020, db/db mice were treated with Englergin for 10 weeks and then used high-resolution ultrasound imaging to measure CFVR to evaluate coronary microcirculation. Among them, SGLT 2i increased the bioavailability of NO by increasing L-arginine/ADMA ratio, and then improved CFVR and myocardial flow reserve (Adingupu et al., 2019). In 2022, Yimin Tu et al. randomly divided db/db mice into db/db group, db/db + EMPA group, and db/m mice as control group. After 8 weeks of treatment, it was found that EMPA could inhibit the loss of cardiac pericytes and increase the coverage of pericytes to coronary microvessels in type 2 diabetic mice (Tu et al., 2022). In previous studies, it has been found that the decrease of the number and coverage of cardiac pericytes may be involved in the progress of CMD (Ferland-McCollough et al., 2017), in which pericytes play an important role in maintaining vascular stability, and the improvement of their number and function is helpful to improve the function and structure of cardiac microvessels (Trost et al., 2016). These animal experimental results support the view that SGLT2 inhibitors improve coronary microcirculation in animal models (Table 2).
Multiple clinical trials, however, have found a lack of conclusive evidence on the impact of SGLT2 inhibitors on coronary microcirculation.
In 2021, Katrine M et al. conducted a randomized double-blind, placebo-controlled crossover trial on 12 patients with a 4-week treatment of empagliflozin and placebo. Evaluation using 11C-acetate PET/CT after the experiment showed that empagliflozin reduced resting MBF by 13%, which remained significant even after adjusting for cardiac workload, but had no significant effect on stress MBF. On the whole, SGLT2 inhibitors is a benign result for coronary microcirculation disturbance (Lauritsen et al., 2021). In 2022, a preliminary report by Leccisotti et al. on the DAPAHEART Trial demonstrated the random allocation of 16 patients to receive dapagliflozin (n = 8) or placebo (n = 8) for 4 weeks in a double-blind, placebo-controlled treatment. Evaluation using FDG PET/CT after the experiment showed a significant improvement in myocardial flow reserve in patients receiving dapagliflozin treatment. Resting rate-pressure product correction confirmed an increase in myocardial flow reserve in the dapagliflozin group. Resting MBF in the dapagliflozin group was significantly lower than in the placebo group, even after correcting for resting rate-pressure product (Leccisotti et al., 2022). The above studies have found that SGLT2 inhibitors can improve the disturbance of coronary microcirculation.
However, a randomized, placebo-controlled crossover study conducted by Lauritsen et al., in 2021 found that after 12 weeks of treatment with empagliflozin 25 mg and placebo, there was no significant impact on CFVR assessed by TTDE (Suhrs et al., 2022). Additionally, a randomized study consistent with this result investigated 90 patients with Type 2 Diabetes and cardiovascular disease or high risk, and found that empagliflozin treatment for 13 weeks had no effect on myocardial flow reserve measured by Rubidium-82 positron emission tomography (Jurgens et al., 2021). In 2022, Magnus Lundin et al. conducted a randomized trial on sodium-glucose cotransporter inhibition (SOCOGAMI) in patients with newly discovered glucose abnormalities and recent myocardial infarction, where 42 patients were randomized to receive empagliflozin 25 mg (n = 20) or placebo (n = 22), and after 7 months of treatment, measurements using CMR and echocardiography showed that empagliflozin did not affect coronary flow reserve (Lundin et al., 2022).
Based on current clinical research, the conclusions regarding the efficacy of SGLT2 inhibitors on coronary microcirculation in actual clinical populations are not consistent. This phenomenon reflects that SGLT2 inhibitors may exhibit differentiated effects in different patient populations and disease characteristics. Therefore, more large-scale, long-term clinical trials are needed in the future to further validate their efficacy on coronary microcirculation in different patient groups, in order to comprehensively assess their true value in clinical practice. A prospective, single-center, randomized LUCENT-J study is currently underway in Japan (Tamanaha et al., 2024), which is a prospective, single-center, randomized, two-arm, parallel-level, active-controlled study. Forty Type 2 Diabetes patients are being randomly assigned in a 1:1 ratio to receive dapagliflozin or the control group and treated for 24 weeks. Changes in myocardial flow reserve are measured using 13N-ammonia positron emission tomography computed tomography scans, and the experimental results will provide more clinical evidence for us to observe the efficacy of SGLT2 inhibitors on coronary microcirculation (Table 3).
The current clinical studies on the efficacy of SGLT2 inhibitors on coronary microcirculation in real clinical populations yield inconsistent conclusions. This may be related to the design of the study, the differences among the subjects and the diversity of measurement methods. Therefore, further large-scale, long-term clinical trials are needed to comprehensively evaluate the exact effect of SGLT2 inhibitors on coronary artery microcirculation in different patient groups, so as to determine the best clinical application and potential benefits. These results are expected to provide useful clues for the pharmacological study of SGLT2 inhibitors, especially for the mechanism of coronary microcirculation.
SC: Writing–original draft, Writing–review and editing, Conceptualization, Software. WO: Funding acquisition, Methodology, Writing–review and editing. SG: Funding acquisition, Conceptualization, Writing–review and editing. LC: Funding acquisition, Methodology, Writing–review and editing. BL: Conceptualization, Funding acquisition, Project administration, Writing–review and editing. ZZ: Funding acquisition, Methodology, Conceptualization, Data curation, Supervision, Writing–review and editing.
The author(s) declare that financial support was received for the research, authorship, and/or publication of this article. This study was supported by Medical Scientific Research Foundation of Guangdong Province, China (B2023089); Self-financing scientific and technological innovation project of Foshan (2220001004375); and Project of The Second People’s Hospital of Foshan (2022B02).
The authors declare that the research was conducted in the absence of any commercial or financial relationships that could be construed as a potential conflict of interest.
The author(s) declare that no Generative AI was used in the creation of this manuscript.
All claims expressed in this article are solely those of the authors and do not necessarily represent those of their affiliated organizations, or those of the publisher, the editors and the reviewers. Any product that may be evaluated in this article, or claim that may be made by its manufacturer, is not guaranteed or endorsed by the publisher.
Adingupu, D. D., Göpel, S. O., Grönros, J., Behrendt, M., Sotak, M., Miliotis, T., et al. (2019). SGLT2 inhibition with empagliflozin improves coronary microvascular function and cardiac contractility in prediabetic ob/ob(-/-) mice. Cardiovasc Diabetol. 18 (1), 16. doi:10.1186/s12933-019-0820-6
Anker, S. D., Butler, J., Filippatos, G., Ferreira, J. P., Bocchi, E., Bohm, M., et al. (2021). Empagliflozin in heart failure with a preserved ejection fraction. N. Engl. J. Med. 385 (16), 1451–1461. doi:10.1056/NEJMoa2107038
Arnold, J. R., Kanagala, P., Budgeon, C. A., Jerosch-Herold, M., Gulsin, G. S., Singh, A., et al. (2022). Prevalence and prognostic significance of microvascular dysfunction in heart failure with preserved ejection fraction. JACC Cardiovasc Imaging 15 (6), 1001–1011. doi:10.1016/j.jcmg.2021.11.022
Bhatt, D. L., Szarek, M., Steg, P. G., Cannon, C. P., Leiter, L. A., McGuire, D. K., et al. (2021). Sotagliflozin in patients with diabetes and recent worsening heart failure. N. Engl. J. Med. 384 (2), 117–128. doi:10.1056/NEJMoa2030183
Borbély, A., Falcao-Pires, I., van Heerebeek, L., Hamdani, N., Edes, I., Gavina, C., et al. (2009). Hypophosphorylation of the Stiff N2B titin isoform raises cardiomyocyte resting tension in failing human myocardium. Circ. Res. 104 (6), 780–786. doi:10.1161/CIRCRESAHA.108.193326
Brown, A. J. M., Gandy, S., McCrimmon, R., Houston, J. G., Struthers, A. D., and Lang, C. C. (2020). A randomized controlled trial of dapagliflozin on left ventricular hypertrophy in people with type two diabetes: the DAPA-LVH trial. Eur. Heart J. 41 (36), 3421–3432. doi:10.1093/eurheartj/ehaa419
Bugga, P., Mohammed, S. A., Alam, M. J., Katare, P., Meghwani, H., Maulik, S. K., et al. (2022). Empagliflozin prohibits high-fructose diet-induced cardiac dysfunction in rats via attenuation of mitochondria-driven oxidative stress. Life Sci. 307, 120862. doi:10.1016/j.lfs.2022.120862
Chilian, W. M. (1997). Coronary microcirculation in health and disease. Summary of an NHLBI workshop. Circulation 95 (2), 522–528. doi:10.1161/01.cir.95.2.522
De Bruyne, B., Pijls, N. H. J., Gallinoro, E., Candreva, A., Fournier, S., Keulards, D. C. J., et al. (2021). Microvascular resistance reserve for assessment of coronary microvascular function: JACC technology corner. J. Am. Coll. Cardiol. 78 (15), 1541–1549. doi:10.1016/j.jacc.2021.08.017
Di Franco, A., Cantini, G., Tani, A., Coppini, R., Zecchi-Orlandini, S., Raimondi, L., et al. (2017). Sodium-dependent glucose transporters (SGLT) in human ischemic heart: a new potential pharmacological target. Int. J. Cardiol. 243, 86–90. doi:10.1016/j.ijcard.2017.05.032
Dryer, K., Gajjar, M., Narang, N., Lee, M., Paul, J., Shah, A. P., et al. (2018). Coronary microvascular dysfunction in patients with heart failure with preserved ejection fraction. Am. J. Physiol. Heart Circ. Physiol. 314 (5), H1033–h1042. doi:10.1152/ajpheart.00680.2017
Ersbøll, M., Jürgens, M., Hasbak, P., Kjær, A., Wolsk, E., Zerahn, B., et al. (2022). Effect of empagliflozin on myocardial structure and function in patients with type 2 diabetes at high cardiovascular risk: the SIMPLE randomized clinical trial. Int. J. Cardiovasc Imaging 38 (3), 579–587. doi:10.1007/s10554-021-02443-5
Eshtehardi, P., Luke, J., McDaniel, M. C., and Samady, H. (2011). Intravascular imaging tools in the cardiac catheterization laboratory: comprehensive assessment of anatomy and physiology. J. Cardiovasc Transl. Res. 4 (4), 393–403. doi:10.1007/s12265-011-9272-4
Fearon, W. F., Shah, M., Ng, M., Brinton, T., Wilson, A., Tremmel, J. A., et al. (2008). Predictive value of the index of microcirculatory resistance in patients with ST-segment elevation myocardial infarction. J. Am. Coll. Cardiol. 51 (5), 560–565. doi:10.1016/j.jacc.2007.08.062
Ferland-McCollough, D., Slater, S., Richard, J., Reni, C., and Mangialardi, G. (2017). Pericytes, an overlooked player in vascular pathobiology. Pharmacol. Ther. 171, 30–42. doi:10.1016/j.pharmthera.2016.11.008
Forrester, S. J., Kikuchi, D. S., Hernandes, M. S., Xu, Q., and Griendling, K. K. (2018). Reactive oxygen species in metabolic and inflammatory signaling. Circ. Res. 122 (6), 877–902. doi:10.1161/CIRCRESAHA.117.311401
Fraction, C. E. (2023). Diagnosis and treatment of heart failure with preserved ejection fraction: Chinese expert consensus 2023. Chin. Circulation J. 38 (4), 375–393. doi:10.3760/cma.j.cn112148-20230907-00135
Harvey, A., Montezano, A. C., Lopes, R. A., Rios, F., and Touyz, R. M. (2016). Vascular fibrosis in aging and hypertension: molecular mechanisms and clinical implications. Can. J. Cardiol. 32 (5), 659–668. doi:10.1016/j.cjca.2016.02.070
Heidenreich, P. A., Bozkurt, B., Aguilar, D., Allen, L. A., Byun, J. J., Colvin, M. M., et al. (2022). 2022 AHA/ACC/HFSA guideline for the management of heart failure: a report of the American college of Cardiology/American heart association joint committee on clinical practice guidelines. J. Am. Coll. Cardiol. 79 (17), e263–e421. doi:10.1016/j.jacc.2021.12.012
Hsieh, P. L., Chu, P. M., Cheng, H. C., Huang, Y. T., Chou, W. C., Tsai, K. L., et al. (2022). Dapagliflozin mitigates doxorubicin-caused myocardium damage by regulating AKT-mediated oxidative stress, cardiac remodeling, and inflammation. Int. J. Mol. Sci. 23 (17), 10146. doi:10.3390/ijms231710146
Huang, J., Yin, H., Zhang, M., Ni, Q., and Xuan, J. (2017). Understanding the economic burden of heart failure in China: impact on disease management and resource utilization. J. Med. Econ. 20 (5), 549–553. doi:10.1080/13696998.2017.1297309
Indorkar, R., Kwong, R. Y., Romano, S., White, B. E., Chia, R. C., Trybula, M., et al. (2019). Global coronary flow reserve measured during stress cardiac magnetic resonance imaging is an independent predictor of adverse cardiovascular events. JACC Cardiovasc Imaging 12 (8 Pt 2), 1686–1695. doi:10.1016/j.jcmg.2018.08.018
Ito, N., Nanto, S., Doi, Y., Sawano, H., Masuda, D., Yamashita, S., et al. (2010). High index of microcirculatory resistance level after successful primary percutaneous coronary intervention can be improved by intracoronary administration of nicorandil. Circ. J. 74 (5), 909–915. doi:10.1253/circj.cj-09-0943
Jurgens, M., Schou, M., Hasbak, P., Kjaer, A., Wolsk, E., Zerahn, B., et al. (2021). Effects of empagliflozin on myocardial flow reserve in patients with type 2 diabetes mellitus: the SIMPLE trial. J. Am. Heart Assoc. 10 (15), e020418. doi:10.1161/JAHA.120.020418
Kato, S., Fukui, K., Kodama, S., Azuma, M., Nakayama, N., Iwasawa, T., et al. (2021). Cardiovascular magnetic resonance assessment of coronary flow reserve improves risk stratification in heart failure with preserved ejection fraction. J. Cardiovasc Magn. Reson 23 (1), 112. doi:10.1186/s12968-021-00807-3
Katrine, M. L., Bent, R. R. N., Lars, P. T., Mogens, J., Jakob, H., Troels, K. H., et al. (2020). SGLT2 inhibition does not affect myocardial fatty acid oxidation or uptake, but reduces myocardial glucose uptake and blood flow in individuals with type 2 diabetes: A randomized double-blind, placebo-controlled crossover trial. Diabetes 70 (3), 800–808. doi:10.2337/db20-0921
Kato, S., Saito, N., Kirigaya, H., Gyotoku, D., Iinuma, N., Kusakawa, Y., et al. (2016). Impairment of coronary flow reserve evaluated by phase Contrast cine-magnetic resonance imaging in patients with heart failure with preserved ejection fraction. J. Am. Heart Assoc. 5 (2), e002649. doi:10.1161/JAHA.115.002649
Khan, M. S., Butler, J., and Greene, S. J. (2020). The time is now for sodium glucose Co-transporter 2 inhibitors for heart failure: a call to overcome clinical inertia. Circ. Heart Fail 13 (12), e008030. doi:10.1161/CIRCHEARTFAILURE.120.008030
Kirkman, D. L., Bohmke, N., Billingsley, H. E., and Carbone, S. (2020). Sarcopenic obesity in heart failure with preserved ejection fraction. Front. Endocrinol. (Lausanne) 11, 558271. doi:10.3389/fendo.2020.558271
Lan, N. S. R., Yeap, B. B., Fegan, P. G., Green, G., Rankin, J. M., and Dwivedi, G. (2021). Empagliflozin and left ventricular diastolic function following an acute coronary syndrome in patients with type 2 diabetes. Int. J. Cardiovasc Imaging 37 (2), 517–527. doi:10.1007/s10554-020-02034-w
Lauritsen, K. M., Nielsen, B. R. R., Tolbod, L. P., Johannsen, M., Hansen, J., Hansen, T. K., et al. (2021). SGLT2 inhibition Does not affect myocardial fatty Acid oxidation or Uptake, but reduces myocardial glucose Uptake and blood flow in individuals with type 2 diabetes: a randomized double-blind, placebo-controlled crossover trial. Diabetes 70 (3), 800–808. doi:10.2337/db20-0921
Leccisotti, L., Cinti, F., Sorice, G. P., D'Amario, D., Lorusso, M., Guzzardi, M. A., et al. (2022). Dapagliflozin improves myocardial flow reserve in patients with type 2 diabetes: the DAPAHEART Trial: a preliminary report. Cardiovasc Diabetol. 21 (1), 173. doi:10.1186/s12933-022-01607-4
Lee, J. F., Barrett-O'Keefe, Z., Garten, R. S., Nelson, A. D., Ryan, J. J., Nativi, J. N., et al. (2016). Evidence of microvascular dysfunction in heart failure with preserved ejection fraction. Heart 102 (4), 278–284. doi:10.1136/heartjnl-2015-308403
Lee, M. M. Y., Brooksbank, K. J. M., Wetherall, K., Mangion, K., Roditi, G., Campbell, R. T., et al. (2021). Effect of empagliflozin on left ventricular volumes in patients with type 2 diabetes, or prediabetes, and heart failure with reduced ejection fraction (SUGAR-DM-HF). Circulation 143 (6), 516–525. doi:10.1161/CIRCULATIONAHA.120.052186
Lee, S. H., Shin, D., Lee, J. M., van de Hoef, T. P., Hong, D., Choi, K. H., et al. (2022). Clinical relevance of ischemia with nonobstructive coronary arteries according to coronary microvascular dysfunction. J. Am. Heart Assoc. 11 (9), e025171. doi:10.1161/JAHA.121.025171
Lee, T. M., Chang, N. C., and Lin, S. Z. (2017). Dapagliflozin, a selective SGLT2 Inhibitor, attenuated cardiac fibrosis by regulating the macrophage polarization via STAT3 signaling in infarcted rat hearts. Free Radic. Biol. Med. 104, 298–310. doi:10.1016/j.freeradbiomed.2017.01.035
Li, C., Zhang, J., Xue, M., Li, X., Han, F., Liu, X., et al. (2019). SGLT2 inhibition with empagliflozin attenuates myocardial oxidative stress and fibrosis in diabetic mice heart. Cardiovasc Diabetol. 18 (1), 15. doi:10.1186/s12933-019-0816-2
Li, X., Lu, Q., Qiu, Y., do Carmo, J. M., Wang, Z., da Silva, A. A., et al. (2021). Direct cardiac actions of the sodium glucose Co-transporter 2 inhibitor empagliflozin improve myocardial oxidative phosphorylation and attenuate pressure-overload heart failure. J. Am. Heart Assoc. 10 (6), e018298. doi:10.1161/JAHA.120.018298
Lin, K., Yang, N., Luo, W., Qian, J. F., Zhu, W. W., Ye, S. J., et al. (2022). Direct cardio-protection of Dapagliflozin against obesity-related cardiomyopathy via NHE1/MAPK signaling. Acta Pharmacol. Sin. 43 (10), 2624–2635. doi:10.1038/s41401-022-00885-8
Liu, G., Ma, C., Yang, H., and Zhang, P. Y. (2017). Transforming growth factor β and its role in heart disease. Exp. Ther. Med. 13 (5), 2123–2128. doi:10.3892/etm.2017.4246
Liu, Y., Wu, M., Xu, J., Xu, B., and Kang, L. (2021). Empagliflozin prevents from early cardiac injury post myocardial infarction in non-diabetic mice. Eur. J. Pharm. Sci. 161, 105788. doi:10.1016/j.ejps.2021.105788
Löffler, A. I., Pan, J. A., Balfour, P. C., Shaw, P. W., Yang, Y., Nasir, M., et al. (2019). Frequency of coronary microvascular dysfunction and Diffuse myocardial fibrosis (measured by cardiovascular magnetic resonance) in patients with heart failure and preserved left ventricular ejection fraction. Am. J. Cardiol. 124 (10), 1584–1589. doi:10.1016/j.amjcard.2019.08.011
Long, Q., Li, L., Yang, H., Lu, Y., Yang, H., Zhu, Y., et al. (2022). SGLT2 inhibitor, canagliflozin, ameliorates cardiac inflammation in experimental autoimmune myocarditis. Int. Immunopharmacol. 110, 109024. doi:10.1016/j.intimp.2022.109024
Lundin, M., Ferrannini, G., Mellbin, L., Johansson, I., Norhammar, A., Näsman, P., et al. (2022). SOdium-glucose CO-transporter inhibition in patients with newly detected Glucose Abnormalities and a recent Myocardial Infarction (SOCOGAMI). Diabetes Res. Clin. Pract. 193, 110141. doi:10.1016/j.diabres.2022.110141
Mamas, M. A., Sperrin, M., Watson, M. C., Coutts, A., Wilde, K., Burton, C., et al. (2017). Do patients have worse outcomes in heart failure than in cancer? A primary care-based cohort study with 10-year follow-up in Scotland. Eur. J. Heart Fail 19 (9), 1095–1104. doi:10.1002/ejhf.822
Marcus, M. L., Wilson, R. F., and White, C. W. (1987). Methods of measurement of myocardial blood flow in patients: a critical review. Circulation 76 (2), 245–253. doi:10.1161/01.cir.76.2.245
Matsutani, D., Sakamoto, M., Kayama, Y., Takeda, N., Horiuchi, R., and Utsunomiya, K. (2018). Effect of canagliflozin on left ventricular diastolic function in patients with type 2 diabetes. Cardiovasc Diabetol. 17 (1), 73. doi:10.1186/s12933-018-0717-9
McMurray, J. J. V., Solomon, S. D., Inzucchi, S. E., Kober, L., Kosiborod, M. N., Martinez, F. A., et al. (2019). Dapagliflozin in patients with heart failure and reduced ejection fraction. N. Engl. J. Med. 381 (21), 1995–2008. doi:10.1056/NEJMoa1911303
Moghaddam, N., Hawkins, N. M., McKelvie, R., Poon, S., Joncas, S. X., MacFadyen, J., et al. (2023). Patient eligibility for established and novel guideline-directed medical therapies after acute heart failure hospitalization. JACC Heart Fail 11 (5), 596–606. doi:10.1016/j.jchf.2022.10.013
Mohammed, S. F., Hussain, S., Mirzoyev, S. A., Edwards, W. D., Maleszewski, J. J., and Redfield, M. M. (2015). Coronary microvascular rarefaction and myocardial fibrosis in heart failure with preserved ejection fraction. Circulation 131 (6), 550–559. doi:10.1161/CIRCULATIONAHA.114.009625
Montalescot, G., Sechtem, U., Achenbach, S., Andreotti, F., Arden, C., Budaj, A., et al. (2013). 2013 ESC guidelines on the management of stable coronary artery disease: the Task Force on the management of stable coronary artery disease of the European Society of Cardiology. Eur. Heart J. 34 (38), 2949–3003. doi:10.1093/eurheartj/eht296
Nah, J., Zhai, P., Huang, C. Y., Fernández Á, F., Mareedu, S., Levine, B., et al. (2020). Upregulation of Rubicon promotes autosis during myocardial ischemia/reperfusion injury. J. Clin. Invest. 130 (6), 2978–2991. doi:10.1172/JCI132366
Neal, B., Perkovic, V., Mahaffey, K. W., de Zeeuw, D., Fulcher, G., Erondu, N., et al. (2017b). Canagliflozin and cardiovascular and renal events in type 2 diabetes. N. Engl. J. Med. 377 (7), 2099–2657. doi:10.1056/NEJMc1712572
Neal, B., Perkovic, V., Mahaffey, K. W., de Zeeuw, D., Fulcher, G., Erondu, N., et al. (2017a). Canagliflozin and cardiovascular and renal events in type 2 diabetes. N. Engl. J. Med. 377 (7), 2099–2657. doi:10.1056/NEJMc1712572
Nikolic, M., Zivkovic, V., Jovic, J. J., Sretenovic, J., Davidovic, G., Simovic, S., et al. (2022). SGLT2 inhibitors: a focus on cardiac benefits and potential mechanisms. Heart Fail Rev. 27 (3), 935–949. doi:10.1007/s10741-021-10079-9
Ozcan, C., Allan, T., Besser, S. A., de la Pena, A., and Blair, J. (2021). The relationship between coronary microvascular dysfunction, atrial fibrillation and heart failure with preserved ejection fraction. Am. J. Cardiovasc Dis. 11 (1), 29–38.
Packer, M., Anker, S. D., Butler, J., Filippatos, G., Pocock, S. J., Carson, P., et al. (2020). Cardiovascular and renal outcomes with empagliflozin in heart failure. N. Engl. J. Med. 383 (15), 1413–1424. doi:10.1056/NEJMoa2022190
Patel, J., Rassekh, N., Fonarow, G. C., Deedwania, P., Sheikh, F. H., Ahmed, A., et al. (2023). Guideline-directed medical therapy for the treatment of heart failure with reduced ejection fraction. Drugs 83 (9), 747–759. doi:10.1007/s40265-023-01887-4
Paulus, W. J. (2020). Unfolding discoveries in heart failure. N. Engl. J. Med. 382 (7), 679–682. doi:10.1056/NEJMcibr1913825
Paulus, W. J., and Tschope, C. (2013). A novel paradigm for heart failure with preserved ejection fraction: comorbidities drive myocardial dysfunction and remodeling through coronary microvascular endothelial inflammation. J. Am. Coll. Cardiol. 62 (4), 263–271. doi:10.1016/j.jacc.2013.02.092
Pelletier-Galarneau, M., Martineau, P., and El Fakhri, G. (2019). Quantification of PET myocardial blood flow. Curr. Cardiol. Rep. 21 (3), 11. doi:10.1007/s11886-019-1096-x
Ratchford, S. M., Bunsawat, K., Alpenglow, J. K., Zhao, J., Wright, J. B., Ryan, J. J., et al. (2023). Improved vascular function and functional capacity following l-citrulline administration in patients with heart failure with preserved ejection fraction: a single-arm, open-label, prospective pilot study. J. Appl. Physiol. 134 (2), 328–338. doi:10.1152/japplphysiol.00445.2022
Schulz, E., Jansen, T., Wenzel, P., Daiber, A., and Münzel, T. (2008). Nitric oxide, tetrahydrobiopterin, oxidative stress, and endothelial dysfunction in hypertension. Antioxid. Redox Signal 10 (6), 1115–1126. doi:10.1089/ars.2007.1989
Shah, S. J., Lam, C. S. P., Svedlund, S., Saraste, A., Hage, C., Tan, R. S., et al. (2018). Prevalence and correlates of coronary microvascular dysfunction in heart failure with preserved ejection fraction: PROMIS-HFpEF. Eur. Heart J. 39 (37), 3439–3450. doi:10.1093/eurheartj/ehy531
Soga, F., Tanaka, H., Tatsumi, K., Mochizuki, Y., Sano, H., Toki, H., et al. (2018). Impact of dapagliflozin on left ventricular diastolic function of patients with type 2 diabetic mellitus with chronic heart failure. Cardiovasc Diabetol. 17 (1), 132. doi:10.1186/s12933-018-0775-z
Solomon, S. D., McMurray, J. J. V., Claggett, B., de Boer, R. A., DeMets, D., Hernandez, A. F., et al. (2022). Dapagliflozin in heart failure with mildly reduced or preserved ejection fraction. N. Engl. J. Med. 387 (12), 1089–1098. doi:10.1056/NEJMoa2206286
Srivaratharajah, K., Coutinho, T., deKemp, R., Liu, P., Haddad, H., Stadnick, E., et al. (2016). Reduced myocardial flow in heart failure patients with preserved ejection fraction. Circ. Heart Fail 9 (7), e002562. doi:10.1161/CIRCHEARTFAILURE.115.002562
Stephen, M. R., Kanokwan, B., Jeremy, K. A., Jia, Z., Josephine, B. W., John, J. R., et al. (2022). Improved vascular function and functional capacity following l-citrulline administration in patients with heart failure with preserved ejection fraction: a single-arm, open-label, prospective pilot study. J. Appl. Physiol. 134 (2), 328–338. doi:10.1152/japplphysiol.00445.2022
Sucato, V., Evola, S., Novo, G., Sansone, A., Quagliana, A., Andolina, G., et al. (2015). Angiographic evaluation of coronary microvascular dysfunction in patients with heart failure and preserved ejection fraction. Microcirculation 22 (7), 528–533. doi:10.1111/micc.12223
Suhrs, H. E., Nilsson, M., Bové, K. B., Zander, M., and Prescott, E. (2022). Effect of empagliflozin on coronary microvascular function in patients with type 2 diabetes mellitus-A randomized, placebo-controlled cross-over study. PLoS One 17 (2), e0263481. doi:10.1371/journal.pone.0263481
Tamanaha, T., Makino, H., Son, C., Koezuka, R., Tochiya, M., Omura-Ohata, Y., et al. (2024). Effect of Luseogliflozin on myocardial flow reserve in patients with type 2 diabetes mellitus (LUCENT-J study). Diabetes Ther. 15 (5), 1245–1254. doi:10.1007/s13300-024-01571-0
Taqueti, V. R., Solomon, S. D., Shah, A. M., Desai, A. S., Groarke, J. D., Osborne, M. T., et al. (2018). Coronary microvascular dysfunction and future risk of heart failure with preserved ejection fraction. Eur. Heart J. 39 (10), 840–849. doi:10.1093/eurheartj/ehx721
Trost, A., Lange, S., Schroedl, F., Bruckner, D., Motloch, K. A., Bogner, B., et al. (2016). Brain and retinal pericytes: origin, function and role. Front. Cell Neurosci. 10, 20. doi:10.3389/fncel.2016.00020
Tsutsui, H. (2022). Recent advances in the pharmacological therapy of chronic heart failure: evidence and guidelines. Pharmacol. Ther. 238, 108185. doi:10.1016/j.pharmthera.2022.108185
Tu, Y., Li, Q., Zhou, Y., Ye, Z., Wu, C., Xie, E., et al. (2022). Empagliflozin inhibits coronary microvascular dysfunction and reduces cardiac pericyte loss in db/db mice. Front. Cardiovasc Med. 9, 995216. doi:10.3389/fcvm.2022.995216
van Heerebeek, L., Hamdani, N., Falcao-Pires, I., Leite-Moreira, A. F., Begieneman, M. P., Bronzwaer, J. G., et al. (2012). Low myocardial protein kinase G activity in heart failure with preserved ejection fraction. Circulation 126 (7), 830–839. doi:10.1161/CIRCULATIONAHA.111.076075
Verma, S., Mazer, C. D., Yan, A. T., Mason, T., Garg, V., Teoh, H., et al. (2019). Effect of empagliflozin on left ventricular mass in patients with type 2 diabetes mellitus and coronary artery disease: the EMPA-HEART CardioLink-6 randomized clinical trial. Circulation 140 (21), 1693–1702. doi:10.1161/CIRCULATIONAHA.119.042375
von Lewinski, D., Kolesnik, E., Tripolt, N. J., Pferschy, P. N., Benedikt, M., Wallner, M., et al. (2022). Empagliflozin in acute myocardial infarction: the EMMY trial. Eur. Heart J. 43 (41), 4421–4432. doi:10.1093/eurheartj/ehac494
Wang, H., Chai, K., Du, M., Wang, S., Cai, J. P., Li, Y., et al. (2021). Prevalence and incidence of heart failure among urban patients in China: a national population-based analysis. Circ. Heart Fail 14 (10), e008406. doi:10.1161/circheartfailure.121.008406
Wenqiang, C., Mei, N., He, H., Hongliang, C., Xianghua, F., Wei, G., et al. (2023). Chinese expert consensus on the diagnosis and treatment of coronary microvascular diseases (2023 Edition). MedComm 4 (6), e438. doi:10.1002/mco2.438
Wiviott, S. D., Raz, I., Bonaca, M. P., Mosenzon, O., Kato, E. T., Cahn, A., et al. (2019b). Dapagliflozin and cardiovascular outcomes in type 2 diabetes. N. Engl. J. Med. 380 (4), 347–357. doi:10.1056/NEJMoa1812389
Wiviott, S. D., Raz, I., Bonaca, M. P., Mosenzon, O., Kato, E. T., Cahn, A., et al. (2019a). Dapagliflozin and cardiovascular outcomes in type 2 diabetes. N. Engl. J. Med. 380 (4), 347–357. doi:10.1056/NEJMoa1812389
Xaplanteris, P., Fournier, S., Keulards, D. C. J., Adjedj, J., Ciccarelli, G., Milkas, A., et al. (2018). Catheter-based measurements of absolute coronary blood flow and microvascular resistance: feasibility, safety, and reproducibility in humans. Circ. Cardiovasc Interv. 11 (3), e006194. doi:10.1161/CIRCINTERVENTIONS.117.006194
Xing, Y. J., Liu, B. H., Wan, S. J., Cheng, Y., Zhou, S. M., Sun, Y., et al. (2021). A SGLT2 inhibitor dapagliflozin alleviates diabetic cardiomyopathy by suppressing high glucose-induced oxidative stress in vivo and in vitro. Front. Pharmacol. 12, 708177. doi:10.3389/fphar.2021.708177
Xue, M., Li, T., Wang, Y., Chang, Y., Cheng, Y., Lu, Y., et al. (2019). Empagliflozin prevents cardiomyopathy via sGC-cGMP-PKG pathway in type 2 diabetes mice. Clin. Sci. (Lond) 133 (15), 1705–1720. doi:10.1042/CS20190585
Yurista, S. R., Silljé, H. H. W., Oberdorf-Maass, S. U., Schouten, E. M., Pavez Giani, M. G., Hillebrands, J. L., et al. (2019). Sodium-glucose co-transporter 2 inhibition with empagliflozin improves cardiac function in non-diabetic rats with left ventricular dysfunction after myocardial infarction. Eur. J. Heart Fail 21 (7), 862–873. doi:10.1002/ejhf.1473
Zile, M. R., Baicu, C. F., Ikonomidis, J. S., Stroud, R. E., Nietert, P. J., Bradshaw, A. D., et al. (2015). Myocardial stiffness in patients with heart failure and a preserved ejection fraction: contributions of collagen and titin. Circulation 131 (14), 1247–1259. doi:10.1161/CIRCULATIONAHA.114.013215
Zinman, B., Wanner, C., Lachin, J. M., Fitchett, D., Bluhmki, E., Hantel, S., et al. (2015b). Empagliflozin, cardiovascular outcomes, and mortality in type 2 diabetes. N. Engl. J. Med. 373 (22), 2117–2128. doi:10.1056/NEJMoa1504720
Keywords: sodium-glucose Co-transporter 2 inhibitors, coronary microvascular disease, heart failure, SGLT2 inhibitor, HFpEF, heart failure with preserved ejection fraction
Citation: Chen S, Ou W, Gan S, Chen L, Liu B and Zhang Z (2025) Effect of sodium-glucose Co-transporter 2 inhibitors on coronary microcirculation. Front. Pharmacol. 16:1523727. doi: 10.3389/fphar.2025.1523727
Received: 06 November 2024; Accepted: 10 February 2025;
Published: 28 February 2025.
Edited by:
Yuli Huang, Southern Medical University, ChinaReviewed by:
Akiyuki Nishimura, National Institute for Physiological Sciences (NIPS), JapanCopyright © 2025 Chen, Ou, Gan, Chen, Liu and Zhang. This is an open-access article distributed under the terms of the Creative Commons Attribution License (CC BY). The use, distribution or reproduction in other forums is permitted, provided the original author(s) and the copyright owner(s) are credited and that the original publication in this journal is cited, in accordance with accepted academic practice. No use, distribution or reproduction is permitted which does not comply with these terms.
*Correspondence: Shaoxin Chen, YTEzNzI5MTY0OTg5QDE2My5jb20=; Zhenhong Zhang, MTMyMTY3MDUwNDdAMTYzLmNvbQ==
†These authors share first authorship
Disclaimer: All claims expressed in this article are solely those of the authors and do not necessarily represent those of their affiliated organizations, or those of the publisher, the editors and the reviewers. Any product that may be evaluated in this article or claim that may be made by its manufacturer is not guaranteed or endorsed by the publisher.
Research integrity at Frontiers
Learn more about the work of our research integrity team to safeguard the quality of each article we publish.