- 1Department of Pharmacy, Sichuan Academy of Medical Sciences and Sichuan Provincial People’s Hospital, School of Medicine, University of Electronic Science and Technology of China, Chengdu, China
- 2Personalized Drug Therapy Key Laboratory of Sichuan Province, Sichuan Academy of Medical Sciences and Sichuan Provincial People’s Hospital, School of Medicine, University of Electronic Science and Technology of China, Chengdu, China
- 3School of Pharmacy, North Sichuan Medical Collage, Nanchong, China
- 4Power China Chengdu Engineering Corporation Limited, Chengdu, China
- 5Department of Pharmacy, Chengdu Women’s and Children’s Central Hospital, School of Medicine, University of Electronic Science and Technology of China, Chengdu, China
- 6Department of Pharmacy, The Fourth People’s Hospital of Chengdu, Chengdu, China
Ethnopharmacological Relevance: The dried rhizome of Ligusticum chuanxiong S.H.Qiu, Y.Q.Zeng, K.Y.Pan, Y.C.Tang and J.M.Xu (Apiaceae; including the horticultural variety Ligusticum chuanxiong Hort.) [synonym: Conioselinum anthriscoides (H.Boissieu) Pimenov and Kljuykov (The taxonomic classification has been adopted by the World Checklist of Vascular Plants)] is a traditional Chinese botanical drug renowned for its anti-inflammatory and antioxidant properties. It has been widely used to treatment various diseases, particularly cardio-cerebral vascular diseases (CCVDs).
Aim of the review: This review aims to summarize recent advances in Ligusticum chuanxiong (CX) research, including its chemical composition and pharmacological effects, and modern clinical applications.
Materials and methods: A systematic literature search was conducted using keywords such as “Chuanxiong,” “traditional Chinese medicine,” “chemical components,” “metabolites,” “CCVDs,” and “pharmacological effects” to identify relevant literature published between 2014 and 2025. Databases including PubMed, Web of Science, Google Scholar, and CNKI were utilized. Chemical structures in SMILES format were retrieved from the PubChem, and two-dimensional chemical structures were generated using ChemDraw Ultra 8.0. Classical prescriptions of chuanxiong were obtained from authoritative traditional Chinese medicine databases.
Results: Over 100 metabolites have been isolated and identified from CX, classified into nine major classes. Key bioactive compounds include senkyunolide A, ligustilide, tetramethylpyrazine (TMP), and ligusticum CX polysaccharides (LCP). CX demonstrates significant pharmacological effects in treating CCVDs, such as atherosclerosis (AS), myocardial and cerebral ischemia-reperfusion injury, and hypertension. Its therapeutic mechanisms include antiplatelet activity, endothelial cell protection, anti-inflammatory, antioxidant, and anti-apoptotic properties. CX can be administered alone or in combination with other traditional Chinese medicines (TCMs) or chemical drugs, showing efficacy in cardiovascular, nervous system, digestive system disorders, as well as analgesia and anticancer activities.
Conclusion: CX holds substantial clinical value for treating multi-system diseases, with extensive evidence supporting its use in CCVDs. Further research and clinical exploration of CX are warranted to fully harness its therapeutic potential.
1 Introduction
The dried rhizome of Ligusticum chuanxiong S.H.Qiu, Y.Q.Zeng, K.Y.Pan, Y.C.Tang and J.M.Xu (Apiaceae; including the horticultural variety Ligusticum chuanxiong Hort.) [synonym: Conioselinum anthriscoides (H.Boissieu) Pimenov and Kljuykov (The taxonomic classification of this species has been adopted by the World Checklist of Vascular Plants (WCVP))] (CX, Figure 1) is a Chinese herbal medicine first documented in the Shennong Materia Medica and further referenced in ancient books such as the Compendium of Materia Medica and the Synopsis of Prescriptions of the Golden Chamber (Qiu et al., 1979). Table 1 summarizes the definitions and descriptions of Ligusticum chuanxiong as outlined in various national or regional pharmacopoeias.
CX is typically harvested during the summer when stem nodes are visibly swollen and exhibit a slight purple hue. After removal of soil and sand, the rhizomes are sun-dried, roasted, and then cleaned of root hairs. The identification of CX involves morphological, microscopic, and physicochemical analyses. Morphologically, CX appears as irregular, nodular masses with a yellow-brown, rough surface marked by parallel raised nodes and small, tumor-like root scars. The rhizome is firm, emitting a fragrant aroma, and possesses a bitter, spicy taste with a mild numbing sensation. Microscopically, CX is characterized by its cellular structure: the cork layer consists of more than ten rows of cells, with a narrow cortex containing scattered vascular bundles and a distinct cambium. The phloem is broad, and the cambium forms wavy rings or irregular polygons. The xylem vessels are polygonal or round, and the pith is notably large. Parenchyma cells contain oil cells, starch grains, and calcium oxalate crystals. The powdered form of CX is light yellow-brown or gray-brown, with oval or elliptical starch grains and round or cluster-like calcium oxalate crystals. Physicochemical identification involves specific chemical reactions, for instance, when CX powder is treated with petroleum ether and methanol, a red-purple color reaction is observed (Commission, 2020).
According to TCM theory, CX is characterized by a spicy and warm nature and is associated with the liver, gallbladder, and pericardium meridians. Its therapeutic effects extend upwards to the head and downwards to the xuehai point, located in the depression posterior to the ankle joint. CX facilitates blood circulation (活血通络huo xue tong luo), dispels wind-cold (祛风散寒qu feng san han), and exhibits properties such as invigorating blood (活血huo xue), promoting qi flow (行气xing qi), expelling wind (祛风qu feng), and alleviating pain (止痛zhi tong). CX can be used alone or in combination with other Chinese botanical drugs to treat various conditions, including stroke, angina pectoris, bruises, swelling, and menstrual irregularities (Figures 2, 3). Table 2 provides a list of some classic botanical drugs formulas incorporating CX (Chen et al., 2018; Commission, 2020; Shao et al., 2021). Beyond its medicinal applications, CX also holds culinary value, particularly its leaves. The leaves of CX possess a flavor profile reminiscent of celery and yeast, making them suitable for use in soups, stews, or salads. Additionally, the aromatic aerial parts of the plant are often utilized as fragrant botanical drugs (Li Q. et al., 2024; Zhou et al., 2024).
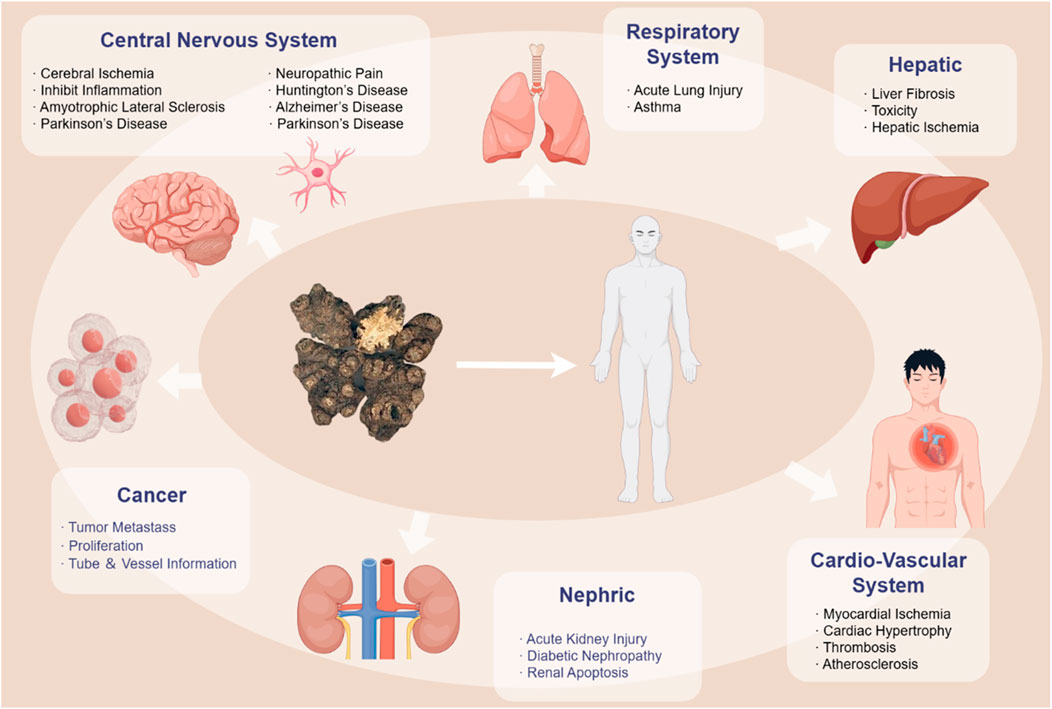
Figure 2. Pharmacological Effects of Ligusticum chuanxiong. In TCM theory, CX is a kind of Qi medicine that can reach and work in multiple tissues and organs with the blood. This figure is based on the main affecting parts of the body.
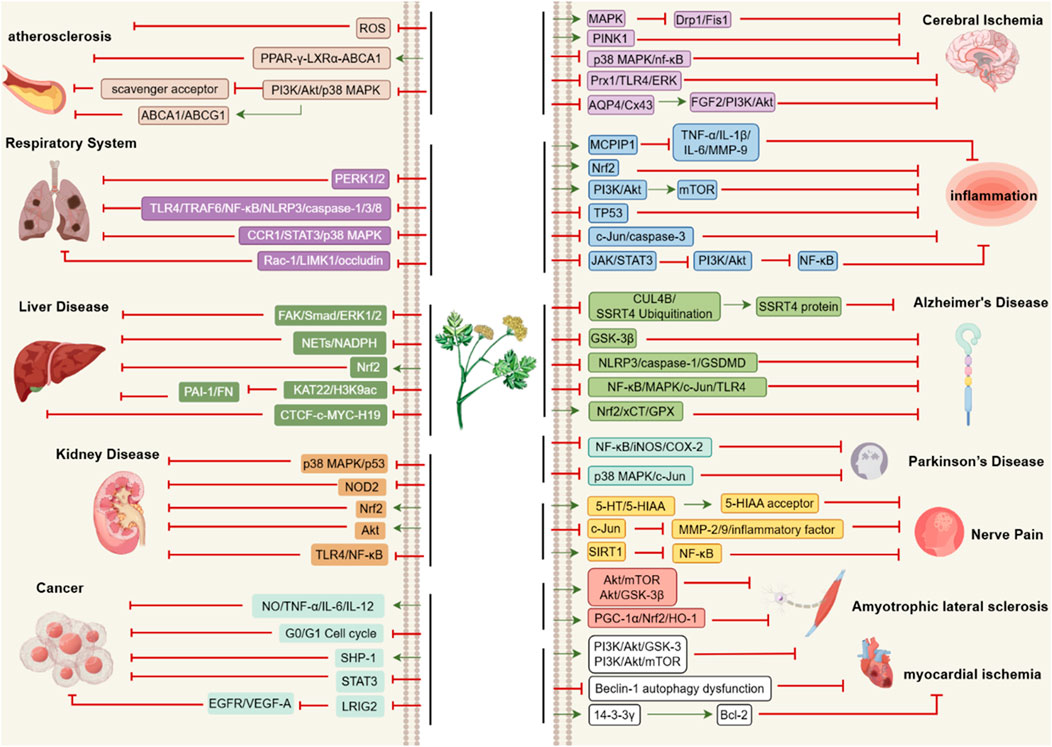
Figure 3. Pharmacological Targets of Ligusticum chuanxiong. The specific pharmacological effects of CX are realized through the above pathways. The arrows represent the promoting effects while the dash lines represent the inhibitory effects.
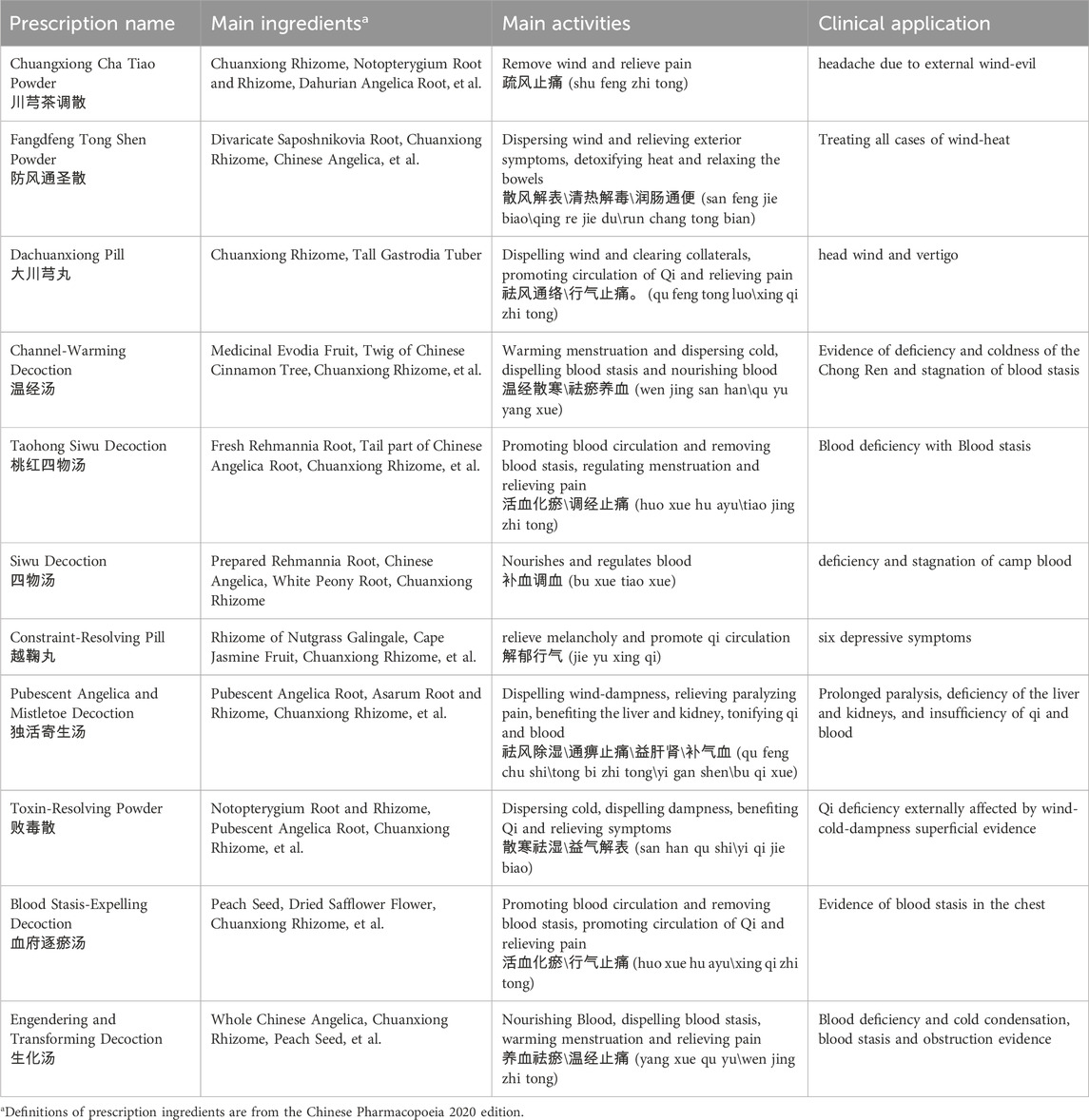
Table 2. Application of Ligusticum chuanxiong in Classical Prescriptions of traditional Chinese medicine.
China faces a high incidence of CCVDs, which rank among the leading causes of mortality in the population (Liberale et al., 2021; Xu et al., 2022). In recent years, the onset of CCVDs such as hypertension, stroke, and coronary heart disease has shifted significantly to younger age groups, with a notable rise in incidence and prevalence among young and middle-aged individuals. Pharmacotherapy plays a pivotal role in managing CCVDs, with ongoing research and development of new drugs and therapeutic approaches. Conventional Western medications, including nitrates, statins, β-blockers, and antiplatelet agents, have effectively treated these conditions (Sikora et al., 2022). However, patients with CCVDs often face significant residual risks, and Western drugs are associated with varying degrees of adverse effects. Consequently, healthcare professionals are increasingly integrating TCM formulations with conventional Western medications to improve therapeutic outcomes (Li H.-q. et al., 2015; Liu X.-t. et al., 2016).
Chinese herbal medicines that promote blood circulation and resolve blood stasis offer unique advantages in enhancing platelet function and hemodynamics, making them valuable in treating CCVDs (Guo et al., 2020; Li et al., 2022a). As a prominent Chinese herbal medicine, CX has been used in China for centuries. Through the integration of TCM theory and modern pharmacological research, the diverse pharmacological effects of CX have been elucidated, particularly its anti-inflammatory and antioxidant properties. Recent studies have made significant progress in understanding its chemical metabolites, pharmacological effects, pharmacokinetics, and safety profiles. Over 100 chemical metabolites have been isolated from CX, including phthalides, terpenes and their enol derivatives, alkaloids, polysaccharides, and organic acids and their ester derivatives. These metabolites exhibit multiple pharmacological activities, such as vasodilation, blood circulation enhancement, antiplatelet aggregation, antioxidant, and anti-inflammatory effects. Notably, some metabolites, such as ligustilide, have been clinically applied in the treatment of CCVDs (Han, 2017; Chen et al., 2018).
This study conducted a systematic literature search using keywords such as Chuanxiong, traditional Chinese medicine, chemical components, metabolites, CCVDs, and pharmacological effects. Relevant literature published between 2014 and 2025 was retrieved from databases including PubMed, Web of Science, Google Scholar, and CNKI. The inclusion criteria comprised English articles with an impact factor greater than 3.0 and Chinese articles from “China’s Core Science and Technology Journals” or “Peking University Library Chinese Core Journals.” A total of 8,534 publications were considered to retrospectively review the progress of modern research on CX, summarize its chemical composition and pharmacological effects, and provide a foundation for its contemporary clinical applications.
2 Metabolites of Ligusticum chuanxiong
To elucidate the pharmacological activities and clinical applications of CX, a comprehensive analysis of its chemical metabolites is essential. Research on the pharmacodynamic basis of CX has progressed since the 1970s, with over 100 metabolites identified to date. Key bioactive metabolites include phthalides, alkaloids, and polysaccharides. Chemical structure in SMILES format were retrieved from PubChem, and two-dimensional chemical structures representations were generated using ChemDraw Ultra 8.0. A detailed summary of the metabolites and their derivatives is presented in Table 3, with corresponding chemical structures depicted in Figures 4–8.
2.1 Volatile oils
Volatile oils are characteristic metabolites of plants in the Apiaceae family, contributing significantly to the aroma of CX. Low-polarity metabolites, such as pathalides, terpenes, and their enol derivatives are typically volatile and are often present in volatile oils (Tang et al., 2022). Currently, several phthalide metabolites have been identified and isolated, which can be categorized into three main types based on their core structures: monomeric phthalides (1–8), hydroxylated phthalides (9–23), and dimeric phthalides (24–29). Representative metabolites include cnidilide, senkyunolide A, and ligustilide. Previous studies have demonstrated the efficacy of phthalides in improving blood circulation, reducing blood stasis, and providing progressive stimulation, particularly for cardiovascular and cerebrovascular diseases, as well as dysmenorrhea (Chen et al., 2011; Li J.-h. et al., 2015; Li et al., 2017). Research on terpenes remains limited; however, terpenes can be classified into monoterpenes (30–46), sesquiterpenes (47–63), triterpenes (64), and terpene derivatives (65–72).
2.2 Organic acids
Organic acids are primarily found in the volatile oil of CX and to a lesser extent in CX extracts, with 19 types identified. These are categorized into phenolic acids (84–90), fatty acids (91–93), and organic esters (94–102), among which ferulic acid and caffeic acid have been extensively studied. Ferulic acid, one of the most active metabolites in CX, has clinical applications, demonstrating anti-inflammatory properties, enhancement of blood flow, inhibition of platelet aggregation, prevention of thrombosis, and neuroprotective effects (Mathew and Abraham, 2004; Lin et al., 2009; Luo et al., 2019). Ferulic acid has shown antioxidant properties through its interaction with 1,1-diphenyl-2-picryl-hydrazyl radical, acting as a key antioxidant metabolite in CX (Wang et al., 2015). Meanwhile, due to its abundance, ferulic acid is frequently used as a quality control marker for CX. According to the 2020 edition of the Chinese Pharmacopoeia, the ferulic acid content in dried CX should not be less than 0.01% (Commission, 2020).
2.3 Alkaloids
Alkaloids are among the most important metabolites of CX; yet only a limited number of alkaloids have been isolated from CX, including organic amines (73,74), pyrazines (75–77), pyrimidines (78), purines (79,80), pyridines (81), carbolines (82), and scopolamine types (83) (Pu et al., 2019a). Contemporary research has confirmed the various pharmacological activities of alkaloids (Li et al., 2022b). In 1977, Chinese researchers identified ligustilide, also known as TMP, a pyrazine metabolite widely present in Ligusticum species. TMP is a representative alkaloid in CX (Chen et al., 2018; Yue et al., 2024).
2.4 Others
LCPs are another active metabolite of CX with multiple bioactivities, including antioxidant, immunomodulatory, antitumor, and antibacterial effects. In addition to these metabolites, CX also contains aldehydes (103), phenols (104–109), ketones (110–114), anhydrides (115), and aromatic hydrocarbons (116). This study collects and summarizes pharmacological studies of CX extracts from the past 5 years in Table 4.
3 Pharmacological properties of Ligusticum chuanxiong
3.1 Central nervous system
3.1.1 Cerebral ischemia
Cerebral ischemia/reperfusion (I/R) injury is commonly associated with high rates of disability and mortality due to its complex pathological mechanisms. CX, a traditional medicine, is widely used in the treatment of ischemic stroke. Several metabolites of CX have demonstrated efficacy in managing cerebral ischemia by modulating oxidative stress, protecting blood vessels, and regulating inflammation. For instance, the National Medical Products Administration (NMPA) recognized butylphthalide as a therapeutic agent for ischemic stroke in 2005 (Zou et al., 2018). TMP inhibits platelet adhesion and the release of inflammatory factors by suppressing the P38 mitogen-activated protein kinase (MAPK) and nuclear factor kappa-B (NF-κB) signaling pathways, thereby protecting microvascular endothelial cells in the brain (Zhang H. et al., 2020). Ligustilide activates adenosine 5‘-monophosphate (AMP)-activated protein kinase (AMPK) signaling and PTEN induced putative kinase 1 (PINK1)/Parkin expression, which helps alleviate ischemic stroke damage (Mao et al., 2022; Wu et al., 2022). In a mouse model of induced intracerebral hemorrhage, ligustilide and senkyunolide H were shown to exert potent neuroprotective effects against hemorrhagic stroke by inhibiting the Paired Related Homeobox 1 (Prx1)/Toll-like receptor 4 (TLR4)/NF-κB pathway, and subsequent immune and neuroinflammatory damage (Han et al., 2018). In a subacute ischemic rat model, TMP was found to inhibit the overexpression of aquaporin 4 (AQP4) and connexin 43 (Cx43) in astrocytes by altering A1-A2 reactivity. TMP also activates the fibroblast growth factor (FGF) 2/phosphoinositide 3-kinase (PI3K)/Akt pathway, which may contribute to its promotion of neurovascular repair following ischemic stroke (Feng X. F. et al., 2023). Additional studies have reported that CX and Binpian [Cinnamomum camphora(L.)Presl] exhibit significant synergistic effects in promoting neurogenesis and reducing astrocyte generation, with CX playing a central role and Binpian enhancing its effects. Binpian is frequently combined with CX to improve the treatment of ischemic stroke (Song et al., 2023; Zhang X. et al., 2024).
3.1.2 Inflammation inhibition
Senkyunolide A and Z-ligustilide, two isolated metabolites, demonstrate inhibitory effects on neuroinflammation (Or et al., 2011). In microsphere-embolized (ME) rats, CX promotes neurogenesis and reduces inflammatory factors, thereby protecting neurons from cerebral ischemia (Wang et al., 2020). TMP has been shown to decrease the levels of inflammatory factors, such as tumor necrosis factor (TNF)-α, interleukin (IL)-1β, IL-6, and matrix metalloproteinase (MMP)-9, in ischemic brain tissue, exerting neuroprotective effects in mice subjected to I/R injury. This neuroprotective effect may be mediated by the upregulation of monocyte chemoattractant protein-induced protein 1 (MCPIP1), as the neuroprotection and anti-inflammatory effect were inhibited upon knockdown of MCPIP1 using specific small interfering RNA (siRNA) (Jin et al., 2021). TMP also protects retinal ganglion cells by inhibiting apoptosis and autophagy via the PI3K/Akt/mammalian target of rapamycin (mTOR) pathway. Additionally, Senkyunolide I has been found to protect mouse neuroblastoma cells from glutamate-induced injury by downregulating the c-Jun N-terminal kinase (c-JUN)/cysteinyl aspartate specific proteinase 3 (caspase-3) pathway and attenuating apoptosis (Wang M. et al., 2021). Furthermore, ligustilide exerts protective effects against oxygen-glucose deprivation/reperfusion (OGD/R) injury in PC12 cells by inhibiting apoptosis through the mitochondrial pathway and promoting autophagy via the Liver kinase B1 (LKB1)-AMPK-mTOR signaling pathway (Zhao et al., 2020). Ma et al. (2024) found that QBT, a novel phthalein metabolite, significantly reduced cognitive dysfunction and neuronal damage in rats with vascular dementia (VaD). It also inhibited VaD-induced hyperactivation of microglia and astrocytes, along with associated inflammatory responses. Moreover, QBT exerts anti-inflammatory effects by inhibiting the chemokine C-X-C motif ligand 12 (CXCL12)/chemokine C-X-C motif receptor 4 (CXCR4) axis and its downstream Janus kinase 2 (JAK2)/signal transducer and activator of transcription 3 (STAT3) and PI3K/Akt/NF-κB pathways, thereby attenuating neuroinflammatory responses both in vivo and in vitro.
3.1.3 Alzheimer’s disease
Alzheimer’s disease (AD) is the most common neurodegenerative disorder, leading to various functional impairments, including memory loss, dementia, and aphasia. It is the third leading cause of disability and death in the elderly, following cardiovascular diseases and malignant tumors, and is considered a major societal burden (Jeremic et al., 2021). Several hypotheses have been proposed regarding the pathogenesis of AD, with the most widely accepted mechanisms involving the excessive accumulation of beta-amyloid (Aβ) outside neurons and the aggregation of hyperphosphorylated tau protein within neurons, resulting in the formation of neurofibrillary tangles (NFTs) (Du et al., 2018; Scheltens et al., 2021). Despite extensive efforts to develop therapeutic strategies for AD, only a few drugs have been approved for treatment, all of which merely provide symptomatic relief and are associated with significant side effects. Effective cures or preventive treatments remain scarce.
In the context of tau pathology, CX has been reported to target 70 key pathways associated with tau-related pathological mechanisms, including interactions with astrocytes, endothelial cells, and microglia. This action effectively reverses tau hyperphosphorylation and promotes its degradation through the lysosomal pathway, thereby mitigating tau-mediated intracellular transport impairment, axonal and synaptic damage, and neuronal death. The primary active metabolites identified in this process include neocnidilide, ferulic acid, Z-ligustilide, and butylidene phthalide, which have demonstrated neuroprotective effects against AD. In contrast, TMP, a widely used therapeutic agent for I/R-induced brain injury, has been associated with only two tau-related targets, suggesting limited therapeutic relevance in tau pathology-based AD (Zeng et al., 2022). However, compared to the control group, the TMP-related group exhibited significant improvements in learning and memory abilities in transgenic AD mice, along with reduced levels of Aβ and tau phosphorylation in the brain (Huang et al., 2021). These effects may be attributed to TMP’s ability to regulate mitochondrial function, maintain synaptic integrity and cytoskeletal stability, and modulate proteins associated with adenosine triphosphate (ATP) and guanosine triphosphate (GTP) binding. Another potential mechanism underlying TMP’s anti-AD’s effects involves the downregulation of Cullin 4B (CUL4B) in the brain tissue of AD mice, which inhibits the ubiquitination of somatostatin receptor 4 (SSRT4) and upregulates SSRT4 protein levels. This mechanism has been confirmed in mice with CUL4B overexpression or SSRT4 silencing (Weng et al., 2021). Additionally, TMP may partially restore cholinergic neuronal function in streptozotocin (STZ)-induced AD rats by inhibiting glycogen synthase kinase (GSK)-3β activity, thereby improving learning and memory deficits (Lu F. et al., 2017).
One study demonstrated that QBT exhibits strong biological activity against cerebrovascular diseases. In VaD rats, QBT reversed aberrant changes associated with ferroptosis and pyroptosis by upregulating the nuclear factor erythroid 2-related factor 2 (Nrf2)/cystine-glutamate antiporter (xCT)/glutathione peroxidase 4 (GPX4) pathway, downregulating the nucleotide-binding oligomerization domain-like receptor family pyrin domain-containing 3 (NLRP3)/cysteine-requiring aspartate protease-1 (Caspase-1)/gasdermin D (GSDMD) pathway, and inhibiting both ferroptosis and pyroptosis in neuronal cells. These actions exerted neuroprotective effects, significantly reducing neuronal damage and cognitive dysfunction in VaD rats (Feng et al., 2024). Furthermore, the methanol extract of CX effectively mitigated cognitive impairment caused by bilateral common carotid artery stenosis, primarily through the inhibition of astrocytes and microglia (Lim et al., 2024).
3.1.4 Others
Parkinson’s disease (PD) is a progressive neurodegenerative disorder characterized by a gradual loss of motor function, with the primary pathological hallmarks being the sustained degeneration of dopaminergic neurons in the substantia nigra and the intracellular accumulation of α-synuclein in the form of Lewy bodies and Lewy neurites (Kouli et al., 2018). CX may play a role in PD biology, as 53 metabolites in CX have been found to affect the biological compartments of human neuronal oxidative stress (hNOS) cells derived from PD patients. These metabolites may interfere with the biological functions of cellular organelles, including early endosomes, lysosomes, and autophagosomes. Additionally, TMP downregulates neuroinflammation markers such as NF-κB, inducible nitric oxide synthase (iNOS), and cyclooxygenase-2 (COX2) (Michel et al., 2017). Canhui and Mengchan (2023) found that ligustilide improved the survival rate of PD cell models, reduced cell cycle arrest in the G0/G1 phase, and inhibited apoptosis, primarily by suppressing the activation of the p38 MAPK/c-JUN signaling pathway.
The antimigraine effects of total alkaloids in CX have been confirmed to be mediated by the upregulation of 5-hydroxytryptamine (5-HT) and its metabolite 5-hydroxyindole acetic acid (5-HIAA), achieved through increased expression of the 5-Hydroxytryptamine Receptor 1B (5-HT1B) and suppression of c-Jun (Pu et al., 2019b). Furthermore, TMP selectively inhibits c-Jun phosphorylation and reduces the expression of MMP-2/9 and pro-inflammatory cytokines secreted by astrocytes, thereby alleviating neuropathic pain caused by chronic compression injury (CCI). TMP also inhibits the activation of the NF-κB pathway in microglia by upregulating the expression of silent information regulator 1 (SIRT-1), thereby reducing neuroinflammatory responses and alleviating migraine (Chen et al., 2024).
Huntington’s disease (HD) is a neurodegenerative disorder with an autosomal dominant inheritance pattern, primarily characterized by motor dysfunction, ataxia, and cognitive decline. 3-Nitropropionic acid (3-NP) is commonly used to induce HD-like symptoms in animal models. Treatment with TMP significantly improves behavioral outcomes in 3-NP-induced HD rats, with biochemical analyses indicating a marked reduction in oxidative stress parameters (Danduga et al., 2018).
3.2 Cadio-vascular system
3.2.1 Myocardial ischemia
Myocardial ischemia improvement is one of the most significant pharmacological actions of CX and its most widely applied use in clinical practice. Research indicates that CX exerts anti-inflammatory, antioxidative, and anti-apoptotic effects on cardiomyocytes subjected to I/R injury, while also promoting coronary blood flow and myocardial metabolism, thereby limiting the extent of myocardial infarction (Zheng et al., 2018). Additionally, studies have shown that CX protects against acute myocardial ischemia induced by isoproterenol through activation of the PI3K/Akt/GSK-3 signaling pathway (Yang et al., 2019).
Wang et al. (2024) demonstrated that TMP intervention reduced cardiomyocyte apoptosis induced by OGD/R and I/R, enhanced cellular viability and autophagy, and improved myocardial tissue necrosis in I/R mice in a dose-dependent manner. Ligustilide has been shown to attenuate myocardial injury caused by inflammation, reduce fibrosis and hypertrophy of myocardial cells, and inhibit ventricular dilation following myocardial infarction. Furthermore, ligustilide provides protective effects in familial dilated cardiomyopathy. When combined with other Chinese herbal medicines, ligustilide can enhance therapeutic efficacy synergistically; for example, its combination with berberine has demonstrated more effective antiplatelet and anti-inflammatory effects in rats with acute myocardial infarction (Zhang et al., 2016).
In addition to inflammation and oxidative stress, autophagic dysfunction plays a significant role in various cardiovascular diseases. Studies have demonstrated that the lactone metabolite of ligustilide can activate the PI3K/Akt/mTOR signaling pathway, restore autophagic flux, and exert cardioprotective effects (Wang et al., 2018). Moreover, CX protects the myocardium from I/R injury by inhibiting Beclin-1-related autophagic dysfunction and enhancing the translocation of B-cell lymphoma-2 (Bcl-2) through increased expression of 14-3-3γ(Zuo et al., 2019).
3.2.2 Atherosclerosis
AS is characterized by the accumulation of lipids, complex carbohydrates, and/or thrombus formation, accompanied by a progressive fibrotic response and calcium deposition, ultimately leading to arterial thickening, hardening, and luminal narrowing (Libby, 2021). Zhang et al. (2024b) explored the mechanisms through which Zhizi (Gardenia jasminoides Ellis) and CX botanical drugs attenuate the progression of AS via Deoxyribonucleic Acid (DNA) methylation. The results indicate that both Zhizi and CX effectively slow AS progression by inhibiting MAPK/extracellular signal-regulated kinase (ERK)-mediated apoptosis through hypermethylation of the fibroblast growth factor receptor 3 (FGFR3) promoter region. Additionally, they alleviate AS by inhibiting the TNF/NF-κB axis and M1 macrophage polarization (Zhang et al., 2024c). Liu et al. (2024) demonstrated for the first time the potential of oral Z-ligustilide treatment to alleviate AS by reducing inflammation and enhancing intestinal barrier function. TMP exerts therapeutic effects on AS primarily through its anti-inflammatory, antioxidant, and lipid-lowering actions, effectively inhibiting lipid accumulation and enhancing intracellular cholesterol efflux. Key targets implicated in these mechanisms include peroxisome proliferator-activated receptors (PPAR), sterol regulatory element-binding proteins (SREBPs)/cleavage activating protein (SCAP), progesterone and adiponectin receptors 3, scavenger receptors (SR-A, CD36), ATP-binding cassette transporters (ABCA1 and ABCG1), and the PI3K/Akt and p38/MAPK signaling pathways (Chen et al., 2017; Duan et al., 2017).
3.2.3 Qthers
In the management of hypertension, TMP has been reported to exert dual actions: it blocks the entry of extracellular Ca2+ through calcium channels and inhibits the release of Ca2+ stored within vascular smooth muscle cells (SMCs) (Ren et al., 2012). The Kcnma1 gene encodes the large conductance calcium-activated potassium channels (BKCa), which are critical for treating various cardiovascular, muscular, and nervous system disorders. Research has shown that TMP helps maintain BKCa β1 protein expression by inhibiting endoplasmic reticulum stress, restoring BKCa currents in porcine coronary artery SMCs, and improving BKCa-mediated vasodilation in coronary arteries (Sun et al., 2019; Szteyn and Singh, 2020).
Liguzinediol exhibits a dose-dependent positive inotropic effect in doxorubicin-induced heart failure mice, indicating its potential as a treatment for heart failure without causing adverse reactions such as arrhythmias or hypotension. This beneficial effect is primarily mediated by an increase in the bcl-2/Bcl-2 associated X protein (Bax) ratio and the suppression of caspase-3 expression and NK-κB activation (Li et al., 2014a). Sodium ferulate has been shown to mitigate myocardial hypertrophy induced by abdominal aorta coarctation by inhibiting protein kinase C beta (PKC-β) and activating the MAPK signaling pathways (Luo et al., 2019).
TMP also demonstrates inhibitory effects on thrombosis. It exerts its antithrombotic effects by reducing inflammatory cytokines and adhesion molecules, modulating MAPK signaling, attenuating oxidative stress, preventing apoptosis, inhibiting platelet aggregation, and suppressing the expression of fibrinogen and von Willebrand factor (Yang et al., 2023). In addition to TMP and ferulic acid, four other effective metabolites have been identified: neochlorogenic acid, 1-H-benzimidazole-2-amine, 3,8-dihydroxyacyl lactone, and CX triterpenes. These metabolites interact with the core amino acids of target proteins, effectively inhibiting blood clot formation.
3.3 Effects of Ligusticum chuanxiong on other systems or organs
3.3.1 Respiratory system
Studies have found that CX adjunctive therapy can significantly improve the clinical symptoms of patients with chronic idiopathic pulmonary fibrosis (Shupei, 2010). The use of microemulsion formulations encapsulating the volatile oils of Danggui [Angelica sinensis (Oliv.) Diels] and CX has been shown to enhance their ability to alleviate acute lung injury (ALI) (Zhang et al., 2022). TMP is primarily regarded as an active medicinal metabolite of CX that targets the respiratory system. In vivo studies have demonstrated that TMP inhibits the proliferation of airway SMCs by reducing p-ERK 1/2 levels, a process associated with the MAPK signaling pathway (Li et al., 2018). Jiang et al. confirmed that TMP exhibits both anti-inflammatory and anti-apoptotic activities in macrophages, which have been validated in vivo and in vitro. These effects involve the TLR4/Tumor necrosis factor receptor-associated factor 6 (TRAF6)/NF-κB/NLRP3/caspase-1 and TLR4/cysteine-requiring aspartate protease-8 (caspase-8)/caspase-3 signaling pathways (Jiang R. et al., 2021). Furthermore, TMP has been shown to alleviate lung inflammation in an ovalbumin-induced asthma mouse model by downregulating C-C Motif Chemokine Receptor 1 (CCR1), STAT3, and p38 MAPK proteins, thereby inhibiting airway hyperresponsiveness and the release of inflammatory and chemotactic factors (Wei et al., 2016). These findings suggest that TMP may have potential in treating ALI and acute respiratory distress syndrome (ARDS) primarily through reducing inflammatory cell infiltration and lowering pro-inflammatory factor secretion in the alveoli. Furthermore, TMP has shown efficacy in alleviating ALI caused by sepsis, reducing inflammatory cell infiltration, and mitigating lipopolysaccharide-induced lung tissue damage. TMP significantly inhibits the Rac Family Small GTPase 1 (Rac1)/LIM Domain Kinase 1 (LIMK1)/Zonula occludens-1 (ZO-1)/occludin signaling pathway (Min et al., 2022).
3.3.2 Hepatic protection
Ferulic acid demonstrates anti-hepatic stellate cell (HSC) activity by inhibiting focal adhesion kinase (FAK) and blocking the Smad and ERK1/2 signaling pathways, highlighting its potential in treating liver fibrosis (Xu et al., 2015). In a rat model of thioacetamide-induced liver fibrosis, butylidene phthalide exhibited significant anti-fibrotic effects by inhibiting epithelial-mesenchymal transition, reducing inflammatory responses, and promoting hepatocyte proliferation (Chuang et al., 2016). Additionally, CX combined with Danggui has been shown to prevent liver fibrosis (Wu et al., 2021). TMP has been reported to effectively attenuate hepatic I/R injury by inhibiting neutrophil extracellular trap formation and nicotinamide adenine dinucleotide phosphate (NADPH) oxidase (Liu et al., 2021). In the study by Lu et al., TMP was also found to confer hepatoprotective effects and improve hepatic steatosis via Nrf2 activation (Lu C. et al., 2017). Furthermore, Lu et al. (2023) reported that the organic acids in CX can alleviate liver injury through multiple signaling pathways, primarily involving the positive regulation of nitric oxide (NO) biosynthesis, Toll-like receptor signaling, NOD-like receptor signaling, and TNF signaling pathways. Additionally, the phthalate ester extract from CX exhibits stronger anti-cholestatic activity, both in vivo and in vitro, compared to other CX extracts. Its protective effect on bile duct cells and HSCs is mediated by reducing the transcription and release of plasminogen activator inhibitor-1 (PAI-1) and fibronectin through lysine acetyltransferase 2A (KAT2A)/histone 3 lysine 9 acetylation (H3K9ac) (Li Y. et al., 2023).
3.3.3 Nephric protection
Studies have shown that CX and Dahuang(Rheum officinale Baill.) may inhibit renal tubular epithelial cell apoptosis and improve acute kidney injury (AKI) and renal fibrosis by suppressing the p38 MAPK/p53 signaling pathway (Li J. et al., 2023). Xia et al. (2024) found that ligustilide alleviates oxidative stress during renal I/R injury by maintaining Sirt3-dependent mitochondrial homeostasis. Qi et al. (2024) identified potential effective metabolites of CX in renal protection, revealing that phthalides improve hyperglycemia-induced renal dysfunction by enhancing Nrf2 activation, reducing collagen deposition, and alleviating inflammation and oxidative stress. Levistolide A mitigates AKI through its antioxidant properties and modulation of the TLR4/NF-κB signaling pathway (Shi J. et al., 2024). TMP exhibits anti-apoptotic and therapeutic effects in diabetic nephropathy (DN) rats by activating the Akt signaling pathway and reducing oxidative stress (Rai et al., 2019). Its renal protective effects include attenuation of renal pathological changes, reduction in blood urea nitrogen, serum creatinine, 24-h urinary albumin, and glycated hemoglobin (HbA1c) levels, as well as an improvement in creatinine clearance (Zhuang et al., 2020). Additionally, TMP plays a significant protective role in AKI, primarily by downregulating the expression of nucleotide-binding oligomerization domain 2 (NOD2), which reduces renal cell apoptosis in a rat ischemia-reperfusion injury model (Jiang G. et al., 2021). TMP has been applied clinically to mitigate AKI. Senkyunolide I alleviates renal I/R injury by inhibiting oxidative stress, endoplasmic reticulum stress, and apoptosis (Zhu et al., 2022).
3.3.4 Cytotoxic effects
Tumor metastasis plays a critical role in the progression of malignant tumors, inhibiting autophagy and apoptosis in cancer cells, thereby promoting uncontrolled cell division and proliferation. CX, a natural plant extract, contains various metabolites with multi-targeted antitumor activities, including the promotion of tumor cell apoptosis and the inhibition of metastasis (Wang K. et al., 2021). Perlolyrine has been identified as a metabolite with antiproliferative effects on human gastric cancer cell lines (Lee et al., 2016); metabolites CX0, CX1, and CX2 exhibit inhibitory effects on the growth of HepG2, SMMC7721, A549, and HCT-116 cells (Hu et al., 2016a); metabolites CXP-1a and CXP-3a stimulate macrophages to produce NO, TNF-α, IL-6, and IL-12, while inhibiting the growth of HepG2 and Hep3B cells through immune modulation by blocking the cell cycle in the G0/G1 phase, thus promoting cancer cell apoptosis (Zhong et al., 2021). Jung et al. (2025) elucidated the molecular mechanisms underlying TMP’s anticancer effects: TMP induces apoptosis in HCCLM3 and Hep3B cells by activating key apoptosis factors and inhibiting proteins associated with cell survival and angiogenesis. It enhances autophagy by promoting the formation of autophagosomes and stimulating autophagy-related proteins, while upregulating SHP-1 to inhibit the activation of the STAT3 signaling pathway, thereby suppressing tumorigenesis and activating cell death pathways. TMP significantly inhibits tumor growth and triggers apoptosis and autophagy in tumor tissues.
4 Clinical practice of Ligusticum chuanxiong
Seventeen Chinese patent medicines containing CX as the main ingredient were retrieved from the NMPA, including CX Cha Tiao Power, CX Cha Tiao Granules, CX Cha Tiao Pills, Compound CX Capsules, DaCX Tablets, CX Cha Tiao Tablets, CX Qingnao Granules, CX Cha Tiao Dai Pao Ji, CX Cha Tiao Dripping Pills, CX Cha Tiao Oral Liquid, CX Cha Tiao Chong Ji, Shiquan Dabu Pills, Compound CX Tablets, DaCX Oral Liquid, CX Cha Tiao Dai Pao Cha, and DaCX Granules.
Qunfang (2024) found that the combining of CX Cha Tiao Power and acupuncture significantly improved clinical efficacy, alleviated headache symptoms, and positively influenced prognosis. Lihua et al. (2023) study on 224 patients with chronic cerebral ischemia and headache using CX Qingnao Granules showed a significantly higher total clinical efficacy rate than the control group (P < 0.05), with reductions in headache frequency and protection of cognitive function. Ran and Qingfang (2024) used DaCX Oral Liquid to treat 192 stroke sequela patients, finding a clinical efficacy rate of 94.79% in the treatment group (DaCX Oral Liquid + conventional therapy), significantly higher than the 84.38% in the control group. Jianfang and Wenlong (2022) investigated the clinical effect of Compound CX Capsules combined with Arorol hydrochloride tablets in treating angina pectoris, reporting a significantly higher total effective rate in the treatment group (98.31% vs. 86.44%, P < 0.05). This combination therapy not only improved symptoms but also promoted cytokine level improvements and enhanced quality of life. CX-based formulations have been widely used in clinical practice for related diseases, but most research is still focused on Chinese literature, with limited studies published in English. Additionally, these studies often combine CX with other clinical treatments (e.g., acupuncture and Western medicines). The effectiveness of CX-based formulations in clinical applications still requires further validation through multicenter, large-sample studies.
5 Safety
As a traditional Chinese botanical drug, CX is not classified as highly toxic, moderately toxic, or low-toxicity in the 2020 edition of the Chinese Pharmacopoeia. To date, no significant organ toxicity has been reported in relation to its use, distinguishing it from other botanical drugs known to have toxic effects. For example, Baihuasheshecao (Hedyotis diffusa Willd.) has been linked to toxicity in the cardiac, digestive, neurological, urinary, and respiratory systems; Maqianzi (Strychnos nux-vomica Linn.) is toxic to the digestive, hepatic, renal, and nervous systems; and Feiyangcao(Euphorbia hirta Linn.) exhibits corrosive toxicity to the digestive system (Li N. et al., 2022).
In a study by Zhang et al., the acute toxicity and dermal sensitivity of essential oil (EO) extracted from CX were evaluated. The results showed that the EO caused mild irritation to rabbit skin, but no significant signs of pigmentation, bleeding, roughness, or skin thinning were observed in guinea pigs after 7 days of treatment. The median lethal doses (LD50) were determined to be 7.23 g/kg and 2.25 g/kg for oral and intraperitoneal administration, respectively, equivalent to approximately 14,606 and 5,091 times the clinical dosage (Zhang et al., 2012). Furthermore, CX demonstrated low embryotoxicity, as indicated by the median inhibitory proliferation values of mouse embryonic stem cells (9.39 mg/mL) and mouse embryonic fibroblasts (18.78 mg/mL), suggesting a weak potential for embryonic developmental toxicity based on the embryonic stem cell test linear discriminant formula (Wang et al., 2019).
6 Critical discussion on the taxonomic status of Ligusticum chuanxiong
Based upon more recent botanical literature, WCVP recognises the plant employed as CX as C. anthriscoides ‘Chuanxiong’ a variety of the species C. anthriscoides (H.Boissieu) Pimenov and Kljuykov. It cites a single scientific synonym for this variety: Ligusticum chuanxiong S.H.Qiu, Y.Q.Zeng, K.Y.Pan, Y.C.Tang and J.M.Xu. Medicinal Plant Names Services records 18 non-scientific names in use in the medical literature for drugs derived from this plant drawn from 24 different medical references (https://mpns.science.kew.org/mpns-portal/plantDetail?plantId=2934837&query=conioselinum+anthriscoides&filter=&fuzzy=false&nameType=all&dbs=wcsCmp).
According to WCVP, the species C. anthriscoides belongs to the Genus Conioselinum which is in the Apiaceae family (APG IV classification). WCVP lists 11 scientific synonyms for this species (https://powo.science.kew.org/taxon/urn:lsid:ipni.org:names:77146159-1#synonyms). Globally WCVP lists 18 species belonging to the Genus Conioselinum Fisch. ex Hoffm. and a further 37 species belonging to the Genus Ligusticum L (in the same family). Regardless of the name used for this species, the 18 species placed by WCVP into the same Genus indicate where the closer evolutionary relationships exist and therefore where there is a greater likelihood of shared chemical pathways. The understanding of taxonomic relationships evolves as more evidence (chemical or molecular) becomes available and will reflect regional access and disciplinary tradition. As a consequence the names employed in different disciplines or at different times will also vary.
Currently, there remain certain discrepancies among researchers regarding the classification of CX whole plant. Yuan et al. (2021) were the first to reveal that the phylogenetic relationship between Ligusticum chuanxiong and Ligusticum jeholense is closer than that with Ligusticum sinense. Consequently, they endorsed the original nomenclature proposed by Qiu for Ligusticum chuanxiong, rather than the revision suggested by Pu. Similarly, a 2009 Master’s thesis noted that a comparative study of Ligusticum chuanxiong from Sichuan, Gansu, and Yunnan, and its closely related species Ligusticum sinense Oliv., revealed morphological, chemical, and chromosomal differences. The study suggested that the Ligusticum chuanxiong from Gansu and Yunnan closely resembles the medicinal CX, and the differences in plant morphology and medicinal properties might be attributed to regional, ecological, and anthropogenic factors. In contrast, Ligusticum sinense Oliv. showed substantial differences and should be classified as a separate species (Ma, 2009). Furthermore, Ren et al.'s chloroplast phylogenomic analysis emphasized the need to narrow the classification scope of Chinese Ligusticum chuanxiong, calling for urgent revisions to its taxonomy (Ren et al., 2022).
In this study, the scientific name used is classified as “unplaced” in authoritative plant taxonomy literature (Govaerts et al., 2021). However, the authors still consider its application in this research to be reasonable. Firstly, the authors focus on the clinical application of CX in China, particularly based on its plant source from the Chinese Pharmacopoeia. The pharmacological effects and clinical applications of CX in CCVDs are summarized and analyzed in detail. CX is a medicinal botanical drug native to Sichuan, China, which is considered a “authentic” medicinal material (authentic medicinal materials refer to those that have been long selected and optimized through clinical application in TCM, grown in specific regions, and distinguished from other materials of the same species by superior quality, efficacy, and stability, and are well-recognized) (Linying et al., 2021). The scientific name Ligusticum chuanxiong S.H.Qiu, Y.Q.Zeng, K.Y.Pan, Y.C.Tang and J.M.Xu also appears in the United States Pharmacopeia, with a specific reference to its origin from “Sichuan,” confirming it as the plant referred to in this study. Moreover, in March 2024, the International Organization for Standardization (ISO) established a standard for Chinese CX (ISO 8071:2024) (Standardization, 2024), which defines the scope as Ligusticum chuanxiong rhizome (rhizome of Ligusticum chuanxiong Hort.), further validating the legitimacy of this scientific name.
However, it is important to note that the taxonomic status of CX whole plant remains an area worthy of further investigation, particularly regarding its relationship with wild populations in terms of morphological, chemical, and genetic characteristics, which has yet to be fully elucidated. Although this study has drawn significant conclusions based on existing literature and experimental data, the complexity of taxonomic classification suggests that the term Ligusticum chuanxiong used in different studies may refer to distinct species, varieties, or even different Genus. This potential heterogeneity may influence the comparability and reproducibility of the research results. Additionally, some of the referenced studies did not provide voucher specimen [13 pharmacological studies did provide such data (Yan et al., 2022; Qi et al., 2024; Tang et al., 2021; Yang et al., 2020; ManLi et al., 2023; Feng R. et al., 2023; Juan et al., 2022; Zhong-Hui et al., 2019; Shaojie et al., 2021; Wang et al., 2020; Lim et al., 2024; Pu et al., 2019b; Wu et al., 2021)], highlighting the need for greater emphasis on the standardization and traceability of materials in future research. The conclusions of this study are based on available data, but due to the ongoing taxonomic controversy, future investigations could further validate and refine these findings through more rigorous taxonomic identification and material standardization. Overall, this study provides an important foundation for understanding the pharmacological effects of CX and points the way for future improvements in research.
7 Conclusion and prospects
CX, a traditional Chinese medicinal botanical drug, exhibits multiple pharmacological effects, including promoting blood circulation (活血 huoxue), anti-inflammatory, antioxidant, and analgesic activities, and its active components and modern formulations have made it an important agent for the prevention and treatment of cardiovascular diseases and tumors. However, CX also presents the following research limitations: (1) Unclear Taxonomic Status: The distinction between Ligusticum chuanxiong and its wild populations (which may be considered different species or subspecies) has not been fully explored. We advocate for multidisciplinary collaboration, involving botanists, chemists, and pharmacologists, to conduct a comprehensive morphological, chemical, and genetic comparative study of Ligusticum chuanxiong and its closely related species. This also highlights the need to full describe from where the material was sourced, who authenticated it and where voucher specimens have been consulted. Aubtors should consult https://ga-online.org/best-practice/. Additionally, we recommend that future studies adhere to the standards outlined in the ConPhyMP guidelines to further enhance the scientific rigor and reliability of the research (Heinrich et al., 2022). Only by clarifying its taxonomic status can the scientific validity and reproducibility of future studies be ensured, thereby providing a more reliable theoretical foundation for the clinical application of this medicinal botanical drug. (2) Limitations of Animal Models: The therapeutic effects of CX in disease treatment have primarily been investigated in animal or cell models. These models do not accurately reflect the pathophysiological impact of multiple comorbidities in humans, and translating laboratory findings into clinical practice remains a critical unresolved issue. Consequently, advancing large-scale clinical trials is an essential step to further research and validate the effects of CX. (3) Lack of Bioinformatics Research: Although studies on the metabolites and pharmacological effects of CX have progressively increased, bioinformatics research remains relatively underdeveloped. Bioinformatics, an emerging research methodology, can integrate diverse biological data through computational analysis to elucidate the mechanisms, targets, and relationships between drugs and diseases. When confronted with the limitations of clinical or animal research, network pharmacology studies of CX may provide valuable insights. By constructing the interaction network between drugs and diseases, network pharmacology helps identify potential targets of CX metabolites, explore their pathways in complex biological systems, and further enrich and broaden the scope of traditional pharmacological research. (4) Unresolved Mechanisms of Action: Although several studies have explored the mechanisms of action of CX, a unified consensus has yet to emerge within the academic community. Elucidating the mechanisms of CX will enable a more accurate assessment of its safety and efficacy. (5) Delayed Product Development: Currently, CX products remain limited. The CX-related drugs marketed in China primarily consist of CX tea powder, with limited products developed through the compatibility of CX and its active metabolites with other drugs, such as Danggui and Huangqi [Astragalus membranaceus (Fisch.) Bge. var.mongholicus (Bge.) Hsiao]. The national government has yet to prioritize the development of relevant innovative products. (6) Neuropharmacological Potential Yet to Be Explored: Although some literature suggests limited pharmacological properties of CX in the nervous system, the research is still in its preliminary stages. Nevertheless, CX holds significant research potential in neuroprotection and antidepressant effects. In conclusion, CX is a multifunctional compound with vast research potential. Future research will facilitate the advancement of its development in broader applications, particularly in the treatment of neurological diseases.
Author contributions
YW: Investigation, Methodology, Writing – review and editing. LW: Investigation, Methodology, Software, Supervision, Writing – original draft, Writing – review and editing. HW: Data curation, Writing – original draft. MJ: Data curation, Writing – original draft. YC: Conceptualization, Resources, Visualization, Writing – review and editing. XZ: Methodology, Project administration, Writing – review and editing. LL: Formal Analysis, Project administration, Writing – review and editing. QY: Formal Analysis, Investigation, Project administration, Writing – review and editing. LH: Formal Analysis, Investigation, Supervision, Writing – review and editing. LB: Formal Analysis, Validation, Visualization, Writing – review and editing. YB: Conceptualization, Funding acquisition, Investigation, Methodology, Resources, Writing – review and editing.
Funding
The author(s) declare that financial support was received for the research and/or publication of this article. This work was supported by Research Project established by Chinese Pharmaceutical Association Hospital Pharmacy department (NO.CPA-Z05-ZC-2023002), China International Medical Foundation (No. Z-2021-46-2101-2023), the Project of Sichuan Provincial Drug Administration (No. 2024012), the Sichuan Pharmaceutical Association New Quality Pharmacy Start-up Plan Project (NO.scyxh20240701), the Sichuan Provincial Administration of Traditional Chinese Medicine (2023MS222), Scientific research project of Sichuan Medical Association (No. S22084), the Open Research Fund of State Key Laboratory of Southwestern Chinese Medicine Resources (No. SKLTCM2022019).
Conflict of interest
Author YC was employed by Power China Chengdu Engineering Corporation Limited.
The remaining authors declare that the research was conducted in the absence of any commercial or financial relationships that could be construed as a potential conflict of interest.
Generative AI statement
The author(s) declare that no Generative AI was used in the creation of this manuscript.
Publisher’s note
All claims expressed in this article are solely those of the authors and do not necessarily represent those of their affiliated organizations, or those of the publisher, the editors and the reviewers. Any product that may be evaluated in this article, or claim that may be made by its manufacturer, is not guaranteed or endorsed by the publisher.
References
Asgary, S., Naderi, G. A., Shams Ardekani, M. R., Sahebkar, A., Airin, A., Aslani, S., et al. (2013). Chemical analysis and biological activities of Cupressus sempervirens var. horizontalis essential oils. Pharm. Biol. 51 (2), 137–144. doi:10.3109/13880209.2012.715168
Bai, L., Kee, H. J., Han, X., Zhao, T., Kee, S. J., and Jeong, M. H. (2021). Protocatechuic acid attenuates isoproterenol-induced cardiac hypertrophy via downregulation of ROCK1-Sp1-PKCγ axis. Sci. Rep. 11 (1), 17343. doi:10.1038/s41598-021-96761-2
Canhui, T., and Mengchan, W. (2023). Protective effect of ligustilide on a cellular model of Parkinson's disease and its mechanism study. Chin. J. Clin. Pharmacol. 39 (06), 795–799. doi:10.13699/j.cnki.1001-6821.2023.06.008
Cao, F., Liu, W., Wen, Y., He, Z., and Qin, W. (1983). Studies on the chemical constituents of Ligusticum chuanxiong Hort. Chin. Tradit. Herb. Drugs 14, 1–2.
Cao, Y., Yao, W., Yang, T., Yang, M., Liu, Z., Luo, H., et al. (2024). Elucidating the mechanisms of Buyang Huanwu Decoction in treating chronic cerebral ischemia: a combined approach using network pharmacology, molecular docking, and in vivo validation. Phytomedicine 132, 155820. doi:10.1016/j.phymed.2024.155820
Chang, X. L., Ma, Y. B., Zhang, X. M., Jiang, Z. Y., and Chen, J. J. (2007). Studies on chemical constituents of rhizomes of Ligusticum chuanxiong. Zhongguo Zhong Yao Za Zhi 32 (15), 1533–1536.
Charakida, A., Charakida, M., and Chu, A. C. (2007). Double-blind, randomized, placebo-controlled study of a lotion containing triethyl citrate and ethyl linoleate in the treatment of acne vulgaris. Br. J. Dermatol 157 (3), 569–574. doi:10.1111/j.1365-2133.2007.08083.x
Chen, C., Wang, D., Bo, C., and Du, L. (2003). Studies on the chemical constituents of ligusticum chuanxiong hort in yanbian. Chin. Tradit. Herb. Drugs (24-25).
Chen, J., Tian, J., Ge, H., Liu, R., and Xiao, J. (2017). Effects of tetramethylpyrazine from Chinese black vinegar on antioxidant and hypolipidemia activities in HepG2 cells. Food Chem. Toxicol. 109, 930–940. doi:10.1016/j.fct.2016.12.017
Chen, L., Peng, C., Liu, Y., Chen, H., and Xiang, C. (2011). Discussion on forming pattern of dao-di herbs Ligusticum chuanxiong. China J. Chin. Mater Med. 36 (16), 2303–2305.
Chen, Y., Mo, S., Li, J., and Ge, F. (2004). Study on constituents of volatile oil of Ligusticum chuanxiong Hort. J. Chin. Med., 580–582. doi:10.13863/j.issn1001-4454.2004.08.017
Chen, Y., Xing, Z., Chen, J., Sun, C., Liu, Y., Peng, C., et al. (2024). SIRT1 activation by Ligustrazine ameliorates migraine via the paracrine interaction of microglia and neurons. Phytomedicine 135, 156069. doi:10.1016/j.phymed.2024.156069
Chen, Z., Zhang, C., Gao, F., Fu, Q., Fu, C., He, Y., et al. (2018). A systematic review on the rhizome of Ligusticum chuanxiong Hort. (Chuanxiong). Food Chem. Toxicol. 119, 309–325. doi:10.1016/j.fct.2018.02.050
Cheng, J., Zhengzhong, Z., Lihua, C., Haiting, Z., Shihua, F., Weifeng, Z., et al. (2024). Effect of volatile oil of Ligusticum chuanxiong on transdermal properties and cytotoxicity of triptolide in vitro. Chin. Pharm. 35 (4), 413–418. doi:10.6039/j.issn.1001-0408.2024.04.06
Cheng, Z., Jiang, J., Yang, X., Chu, H., Jin, M., Li, Y., et al. (2017). The research of genetic toxicity of β-phellandrene. Environ. Toxicol. Pharmacol. 54, 28–33. doi:10.1016/j.etap.2017.06.011
Chruszcz-Lipska, K. (2022). Probing the stereochemical structure of carenes using Raman and Raman optical activity spectroscopy. Spectrochim. Acta A Mol. Biomol. Spectrosc. 276, 121176. doi:10.1016/j.saa.2022.121176
Chuang, H.-M., Su, H.-L., Li, C., Lin, S.-Z., Yen, S.-Y., Huang, M.-H., et al. (2016). The role of butylidenephthalide in targeting the microenvironment which contributes to liver fibrosis amelioration. Front. Pharmacol. 7, 112. doi:10.3389/fphar.2016.00112
Commission, N. P. (2020). Chinese pharmacopoeia (2020 edition I). Bei Jing: China Medical Science and Technology Press.
Cui, S., Feng, X., and Xia, Z. (2024). Ligusticum chuanxiong hort. Ameliorates neuropathic pain by regulating microglial M1 polarization: a study based on network pharmacology. J. Pain Res. 17, 1881–1901. doi:10.2147/jpr.S446137
Danduga, R. C. S. R., Dondapati, S. R., Kola, P. K., Grace, L., Tadigiri, R. V. B., and Kanakaraju, V. K. (2018). Neuroprotective activity of tetramethylpyrazine against 3-nitropropionic acid induced Huntington's disease-like symptoms in rats. Biomed. Pharmacother. 105, 1254–1268. doi:10.1016/j.biopha.2018.06.079
Deng, S., Chen, S. N., Yao, P., Nikolic, D., van Breemen, R. B., Bolton, J. L., et al. (2006). Serotonergic activity-guided phytochemical investigation of the roots of Angelica sinensis. J. Nat. Prod. 69 (4), 536–541. doi:10.1021/np050301s
Du, X., Wang, X., and Geng, M. (2018). Alzheimer's disease hypothesis and related therapies. Transl. Neurodegener. 7, 2. doi:10.1186/s40035-018-0107-y
Duan, J., Xiang, D., Luo, H., Wang, G., Ye, Y., Yu, C., et al. (2017). Tetramethylpyrazine suppresses lipid accumulation in macrophages via upregulation of the ATP-binding cassette transporters and downregulation of scavenger receptors. Oncol. Rep. 38 (4), 2267–2276. doi:10.3892/or.2017.5881
Feng, L., Wu, Y. J., Yang, Y. R., Yue, B. J., Peng, C., Chen, C., et al. (2024). QBT improved cognitive dysfunction in rats with vascular dementia by regulating the Nrf2/xCT/GPX4 and NLRP3/Caspase-1/GSDMD pathways to inhibit ferroptosis and pyroptosis of neurons. Int. Immunopharmacol. 142 (Pt A), 113070. doi:10.1016/j.intimp.2024.113070
Feng, R., Liu, J., Guo, L., Shu, H.-Z., Zhou, Q.-M., Liu, L., et al. (2023a). A pair of new enantiomeric hybrid phthalide–adenines with a rare 5-oxa-1-azaspiro[3,4]octane moiety and two pairs of new enantiomeric hybrid paraethyl phenol–adenines from Ligusticum chuanxiong. Arabian J. Chem. 16 (5), 104696. doi:10.1016/j.arabjc.2023.104696
Feng, X. F., Li, M. C., Lin, Z. Y., Li, M. Z., Lu, Y., Zhuang, Y. M., et al. (2023b). Tetramethylpyrazine promotes stroke recovery by inducing the restoration of neurovascular unit and transformation of A1/A2 reactive astrocytes. Front. Cell Neurosci. 17, 1125412. doi:10.3389/fncel.2023.1125412
Gang, W. (2018). “StudyonStructural characteristics of ligusticum CHUANXIONG in the prevention and treatment of myocardial ischemia injury and biopharmaceutical properries of CHUANXIONG lactone components,” in master (Chengdu: Anhui University of traditional Chinese Medicine).
Govaerts, R., Nic Lughadha, E., Black, N., Turner, R., and Paton, A. (2021). The World Checklist of Vascular Plants, a continuously updated resource for exploring global plant diversity. Sci. Data 8, 215. doi:10.1038/s41597-021-00997-6
Guo, R., Li, L., Su, J., Li, S., Duncan, S. E., Liu, Z., et al. (2020). Pharmacological activity and mechanism of tanshinone IIA in related diseases. Drug Des. Devel Ther. 14, 4735–4748. doi:10.2147/dddt.S266911
Han, L., Liu, D.-L., Zeng, Q.-K., Shi, M.-Q., Zhao, L.-X., He, Q., et al. (2018). The neuroprotective effects and probable mechanisms of Ligustilide and its degradative products on intracerebral hemorrhage in mice. Int. Immunopharmacol. 63, 43–57. doi:10.1016/j.intimp.2018.06.045
Han, W. (2017). Research progress on chemical composition and pharmacological effects of chuanxiong. China J. Chin. Mater Med. 19 (09), 1341–1349. doi:10.13313/j.issn.1673-4890.2017.9.027
He, X., Zhao, L., Zhong, W., Chen, H. Y., Shan, X., Tang, N., et al. (2020). Insight into potent leads for alzheimer's disease by using several artificial intelligence algorithms. Biomed. Pharmacother. 129, 110360. doi:10.1016/j.biopha.2020.110360
Heinrich, M., Jalil, B., Abdel-Tawab, M., Echeverria, J., Kulić, Ž., McGaw, L. J., et al. (2022). Best Practice in the chemical characterisation of extracts used in pharmacological and toxicological research-The ConPhyMP-Guidelines. Front. Pharmacol. 13, 953205. doi:10.3389/fphar.2022.953205
Hongmei, Z., Aijun, Z., and Shuai, L. (2024). Chuanxiong rhizoma and angelicase sinensis radix volatile oil study and transdermal effect based on the concept of unification of drugs and excipients. Chin. J. Pharm. 59 (23), 2249–2257.
Hu, J., Jia, X., Fang, X., Li, P., He, C., and Chen, M. (2016a). Ultrasonic extraction, antioxidant and anticancer activities of novel polysaccharides from Chuanxiong rhizome. Int. J. Biol. Macromol. 85, 277–284. doi:10.1016/j.ijbiomac.2015.12.046
Hu, J., Jia, X., Fang, X., Li, P., He, C., and Chen, M. (2016b). Ultrasonic extraction, antioxidant and anticancer activities of novel polysaccharides from Chuanxiong rhizome. Int. J. Biol. Macromol. 85, 277–284. doi:10.1016/j.ijbiomac.2015.12.046
Huang, X., Yang, J., Huang, X., Zhang, Z., Liu, J., Zou, L., et al. (2021). Tetramethylpyrazine improves cognitive impairment and modifies the hippocampal proteome in two mouse models of alzheimer's disease. Front. Cell Dev. Biol. 9, 632843. doi:10.3389/fcell.2021.632843
Huang, Y., and Pu, F. (1990). Chemical components of the essential oil from Ligusticum brachylobum Franch. Zhongguo Zhong Yao Za Zhi 15 (7), 422–423.
Huang, Y. Z., and Pu, F. D. (1988). Studies on the chemical components of the essential oil from the rhizome of Ligusticum sinense Oliv. cv. Chuanxiong hort. Yao Xue Xue Bao 23 (6), 426–429.
Industry, B. I. o.t.P. (1979). Studyies on the structuer of a phthalide compound. Acta Pharm. Sin. 14, 670–675.
International, B. R. (2024). Retracted: molecular mechanism of palmitic acid on myocardial contractility in hypertensive rats and its relationship with neural nitric oxide synthase protein in cardiomyocytes. Biomed. Res. Int. 2024, 9809026. doi:10.1155/2024/9809026
Islam, A. U. S., Hellman, B., Nyberg, F., Amir, N., Jayaraj, R. L., Petroianu, G., et al. (2020). Myrcene attenuates renal inflammation and oxidative stress in the adrenalectomized rat model. Molecules 25 (19), 4492. doi:10.3390/molecules25194492
Jang, H., Song, G., Lim, W., and Park, S. (2025). Toxic effects of dibutyl phthalate on trophoblast through mitochondria mediated cellular dysfunction. Toxicol. Appl. Pharmacol. 495, 117186. doi:10.1016/j.taap.2024.117186
Jeong, H. U., Kwon, S. S., Kong, T. Y., Kim, J. H., and Lee, H. S. (2014). Inhibitory effects of cedrol, β-cedrene, and thujopsene on cytochrome P450 enzyme activities in human liver microsomes. J. Toxicol. Environ. Health A 77 (22-24), 1522–1532. doi:10.1080/15287394.2014.955906
Jeremic, D., Jimenez-Diaz, L., and Navarro-Lopez, J. D. (2021). Past, present and future of therapeutic strategies against amyloid-beta peptides in Alzheimer's disease: a systematic review. Ageing Res. Rev. 72, 101496. doi:10.1016/j.arr.2021.101496
Jianfang, W., and Wenlong, L. (2022). Clinical study on the treatment of angina pectoris with compound Chuanxiong capsule combined with Arolol. Mod. Drugs Clin. 37 (03), 531–534.
Jiang, G., Xin, R., Yuan, W., Zhang, L., Meng, X., Sun, W., et al. (2021a). Ligustrazine ameliorates acute kidney injury through downregulation of NOD2-mediated inflammation. Int. J. Mol. Med. 45 (3), 731–742. doi:10.3892/ijmm.2020.4464
Jiang, R., Xu, J., Zhang, Y., Zhu, X., Liu, J., and Tan, Y. (2021b). Ligustrazine alleviate acute lung injury through suppressing pyroptosis and apoptosis of alveolar macrophages. Front. Pharmacol. 12, 680512. doi:10.3389/fphar.2021.680512
Jin, L., Sun, S., Ryu, Y., Piao, Z. H., Liu, B., Choi, S. Y., et al. (2018). Gallic acid improves cardiac dysfunction and fibrosis in pressure overload-induced heart failure. Sci. Rep. 8 (1), 9302. doi:10.1038/s41598-018-27599-4
Jin, Z., Liang, J., and Kolattukudy, P. E. (2021). Tetramethylpyrazine preserves the integrity of blood-brain barrier associated with upregulation of MCPIP1 in a murine model of focal ischemic stroke. Front. Pharmacol. 12, 710358. doi:10.3389/fphar.2021.710358
Juan, L., Rui, F., Ou, D., Hong, N., Si, L. L., Zhen, S. H., et al. (2022). Isoindolines and phthalides from the rhizomes of Ligusticum chuanxiong and their relaxant effects on the uterine smooth muscle. Phytochem. (prepublish) 198, 113159. doi:10.1016/j.phytochem.2022.113159
Jung, Y. Y., Hong, Y., Nam, D., Deivasigamani, A., Narula, A. S., Chinnathambi, A., et al. (2025). TMP: a dual modulator of apoptosis and autophagy via SHP-1 regulation in hepatocellular carcinoma. Life Sci. 361, 123316. doi:10.1016/j.lfs.2024.123316
Kobayashi, M., and Mitsuhashi, H. (1987). Studies on the constituents of umbelliferae plantx. XVII. Structures of three new ligustilide derivatives from Ligusticum wallichii. Chem. and Pharm. Bull. 35 (12), 4789–4792. doi:10.1248/cpb.35.4789
Kouli, A., Torsney, K. M., and Kuan, W. L. (2018). “Parkinson's disease: etiology, neuropathology, and pathogenesis,” in Parkinson's disease: pathogenesis and clinical aspects. Editors T. B. Stoker,, and J. C. Greenland (Brisbane (AU): Codon Publications).
Lee, S. H., Jeong, S. J., Jang, G. Y., Kim, M. Y., Hwang, I. G., Kim, H. Y., et al. (2016). Isolation and identification of an antiproliferative compound from fructose-tryptophan maillard reaction products. J. Agric. Food Chem. 64 (15), 3041–3047. doi:10.1021/acs.jafc.6b00157
Li, D., Li, Y., Yang, S., Yu, Z., Xing, Y., and Wu, M. (2022a). Mechanism and potential target of blood-activating Chinese botanical drugs combined with anti-platelet drugs: prevention and treatment of atherosclerotic cardiovascular diseases. Front. Pharmacol. 13, 811422. doi:10.3389/fphar.2022.811422
Li, D., Long, Y., Yu, S., Shi, A., Wan, J., Wen, J., et al. (2022b). Research advances in cardio-cerebrovascular diseases of ligusticum chuanxiong hort. Front. Pharmacol. 12, 832673. doi:10.3389/fphar.2021.832673
Li, H., Hu, S., Zhou, Y., Xu, D., and Ma, Y. (2018). Research progress on the pharmacology of the main pharmacologically active ingredients of Chuanxiong. Clin. Pharmacol. Ther. 23, 1302–1308. doi:10.12092/j.issn.1009-2501.2018.11.018
Li, H.-q., Wei, J.-j., Xia, W., Li, J.-h., Liu, A.-j., Yin, S.-b., et al. (2015a). Promoting blood circulation for removing blood stasis therapy for acute intracerebral hemorrhage: a systematic review and meta-analysis. Acta Pharmacol. Sin. 36 (6), 659–675. doi:10.1038/aps.2014.139
Li, H. X., Ding, M. Y., and Yu, J. Y. (2002). Separation and identification of the phthalic anhydride derivatives of Liqusticum Chuanxiong Hort by GC-MS, TLC, HPLC-DAD, and HPLC-MS. J. Chromatogr. Sci. 40 (3), 156–161. doi:10.1093/chromsci/40.3.156
Li, J., Li, T., Li, Z., Song, Z., and Gong, X. (2023a). Nephroprotective mechanisms of Rhizoma Chuanxiong and Radix et Rhizoma Rhei against acute renal injury and renal fibrosis based on network pharmacology and experimental validation. Front. Pharmacol. 14, 1154743. doi:10.3389/fphar.2023.1154743
Li, J., Yu, J., Ma, H., Yang, N., Li, L., Zheng, D.-d., et al. (2017). Intranasal pretreatment with Z-ligustilide, the main volatile component of rhizoma chuanxiong, confers prophylaxis against cerebral ischemia via Nrf2 and HSP70 signaling pathways. J. Agric. Food Chem. 65 (8), 1533–1542. doi:10.1021/acs.jafc.6b04979
Li, J.-h., Cao, X.-p., Wei, J.-j., Song, L., Liao, F.-j., Zheng, G.-q., et al. (2015b). Chuanxiong chadiao powder, a famous Chinese herbal prescription, for headache: a systematic review and meta-analysis. Complement. Ther. Med. 23 (4), 577–590. doi:10.1016/j.ctim.2015.06.012
Li, N., Miao, M., and Bai, L. (2022c). Clinical toxicity mechanism and rescue measures of large poisonous traditional Chinese medicine. J. Tradit. Chin. Med. 37, 659–664.
Li, Q., He, P., Zhang, D., Pei, Z., Chen, Y., Li, Z., et al. (2024a). Current situation and development strategy of Chuanxiong Rhizoma industry. Chin. Herb. Med. 55, 2771–2783.
Li, S. L., Chan, S. S., Lin, G., Ling, L., Yan, R., Chung, H. S., et al. (2003). Simultaneous analysis of seventeen chemical ingredients of Ligusticum chuanxiong by on-line high performance liquid chromatography-diode array detector-mass spectrometry. Planta Med. 69 (5), 445–451. doi:10.1055/s-2003-39709
Li, Y., Li, F., Ding, M., Ma, Z., Li, S., Qu, J., et al. (2024b). Chuanxiong Rhizoma extracts prevent liver fibrosis via targeting CTCF-c-MYC-H19 pathway. Chin. Herb. Med. 16 (1), 82–93. doi:10.1016/j.chmed.2023.07.003
Li, Y., Li, F., Ding, M., Ma, Z., Li, S., Qu, J., et al. (2024c). Chuanxiong Rhizoma extracts prevent liver fibrosis via targeting CTCF-c-MYC-H19 pathway. Chin. Herb. Med. 16 (01), 82–93. doi:10.1016/j.chmed.2023.07.003
Li, Y., Ma, Z., Ding, M., Jia, K., Xu, B., Zhou, F., et al. (2023b). Chuanxiong Rhizoma extracts prevent cholestatic liver injury by targeting H3K9ac-mediated and cholangiocyte-derived secretory protein PAI-1 and FN. Chin. J. Nat. Med. 21 (9), 694–709. doi:10.1016/s1875-5364(23)60416-9
Li, Y., Song, P., Zhu, Q., Yin, Q.-y., Ji, J.-w., Li, W., et al. (2014a). Liguzinediol improved the heart function and inhibited myocardial cell apoptosis in rats with heart failure. Acta Pharmacol. Sin. 35 (10), 1257–1264. doi:10.1038/aps.2014.75
Li, Y., Song, P., Zhu, Q., Yin, Q. Y., Ji, J. W., Li, W., et al. (2014b). Liguzinediol improved the heart function and inhibited myocardial cell apoptosis in rats with heart failure. Acta Pharmacol. Sin. 35 (10), 1257–1264. doi:10.1038/aps.2014.75
Libby, P. (2021). The changing landscape of atherosclerosis. Nature 592 (7855), 524–533. doi:10.1038/s41586-021-03392-8
Liberale, L., Ministrini, S., Carbone, F., Camici, G. G., and Montecucco, F. (2021). Cytokines as therapeutic targets for cardio- and cerebrovascular diseases. Basic Res. Cardiol. 116 (1), 23. doi:10.1007/s00395-021-00863-x
Lihua, W., Yi, Y., Desheng, Z., Yamei, T., and Huimin, L. (2023). Study on the efficacy of Chuanxiong Qingbao Granules in the treatment of chronic cerebral ischemia with headache. Chin. J. Pract. Intern. Med. 43 (01), 74–77. doi:10.19538/j.nk2023010115
Lim, S., Lim, C., and Cho, S. (2024). Methanol extract of Ligusticum chuanxiong Hort. Rhizome ameliorates bilateral common carotid artery stenosis-induced cognitive deficit in mice by altering microglia and astrocyte activation. Front. Pharmacol. 15, 1329895. doi:10.3389/fphar.2024.1329895
Lin, J., Wang, Q., Zhou, S., Xu, S., and Yao, K. (2022). Tetramethylpyrazine: a review on its mechanisms and functions. Biomed. Pharmacother. 150, 113005. doi:10.1016/j.biopha.2022.113005
Lin, L., Jingyue, X., Sisi, Z., Shijing, D., Fengjuan, Z., Qinshan, Z., et al. (2024). Study on the mechanism of prevention of arterial thrombosis by volatile oil of Rhizoma Ligusticum chuanxiong. Chin. Med. Pharmacol. Clin. 40 (6), 67–71.
Lin, Z., Zhu, D., Yan, Y., and Yu, B. (2009). Neuroprotection by herbal formula FBD and its active compounds. Pharm. Biol. 47 (7), 608–614. doi:10.1080/13880200902913403
Linying, N., Tiezhu, C., and Qingmao, F. (2021). Evolution of the distribution of genuine medicinal materials in sichuan province in Materia Medica works in different historical periods. China J. Chin. Materia Medica 46, 1564–1573. doi:10.19540/j.cnki.cjcmm.20201214.602
Liu, H., Zhou, X. M., Zheng, T., Wu, M. Z., Feng, S., Lin, Y., et al. (2025). Chemical constituents of butyl-phthalides from Ligusticum sinense. Zhongguo Zhong Yao Za Zhi 50 (2), 439–443. doi:10.19540/j.cnki.cjcmm.20241101.202
Liu, S. J., Fu, J. J., Liao, Z. Y., Liu, Y. X., He, J., He, L. Y., et al. (2024). Z-ligustilide alleviates atherosclerosis by reconstructing gut microbiota and sustaining gut barrier integrity through activation of cannabinoid receptor 2. Phytomedicine 135, 156117. doi:10.1016/j.phymed.2024.156117
Liu, X., Lian, Y., Gao, B., Lan, Z., and Lu, X. (2014). GC-MS detection of essential oil of Chuanxiong Rhizoma extracted using different methods. Pharm. Clin. Chin. Materia Medica 5 (17-19).
Liu, X.-t., Ren, P.-w., Peng, L., Kang, D.-y., Zhang, T.-l., Wen, S., et al. (2016a). Effectiveness and safety of ShenXiong glucose injection for acute ischemic stroke: a systematic review and GRADE approach. Bmc Complem Altern. M. 16, 68. doi:10.1186/s12906-016-1038-8
Liu, Y., Luo, Z. Q., Lv, B. R., Zhao, H. Y., and Dong, L. (2016b). Absorption and metabolism of Chuanxiong Rhizoma decoction with multi-component sequential metabolism method. Zhongguo Zhong Yao Za Zhi 41 (7), 1178–1182. doi:10.4268/cjcmm20160703
Liu, Y., Qin, X., Lei, Z., Chai, H., Huang, Z., and Wu, Z. (2021). Tetramethylpyrazine inhibits neutrophil extracellular traps formation and alleviates hepatic ischemia/reperfusion injury in rat liver transplantation. Expe Cell Res. 406 (1), 112719. doi:10.1016/j.yexcr.2021.112719
Long, Y., Yu, S., Li, D., Shi, A., Ma, Y., Deng, J., et al. (2023). Preparation, characterization and safety evaluation of Ligusticum chuanxiong essential oils liposomes for treatment of cerebral ischemia-reperfusion injury. Food Chem. Toxicol. 175, 113723. doi:10.1016/j.fct.2023.113723
Lu, C., Xu, W., Shao, J., Zhang, F., Chen, A., and Zheng, S. (2017a). Nrf2 activation is required for ligustrazine to inhibit hepatic steatosis in alcohol-preferring mice and hepatocytes. Toxicol. Sci. 155 (2), 432–443. doi:10.1093/toxsci/kfw228
Lu, F., Li, X., Li, W., Wei, K., Yao, Y., Zhang, Q., et al. (2017b). Tetramethylpyrazine reverses intracerebroventricular streptozotocin-induced memory deficits by inhibiting GSK-3β. Acta Bioch Bioph Sin. 49 (8), 722–728. doi:10.1093/abbs/gmx059
Lu, L., Lu, T., Wu, Y., Wang, Y., Ke, X., and Yang, R. (2023). Research on the effectiveness and material basis of Ligusticum chuanxiong in alleviating acute liver injury. J. Ethnopharmacol. 314, 116643. doi:10.1016/j.jep.2023.116643
Luo, M., Chen, P.-p., Yang, L., Wang, P., Lu, Y.-l., Shi, F.-g., et al. (2019). Sodium ferulate inhibits myocardial hypertrophy induced by abdominal coarctation in rats: involvement of cardiac PKC and MAPK signaling pathways. Biomed. Pharmacother. 112, 108735. doi:10.1016/j.biopha.2019.108735
Luo, S., Li, J., Zhou, Y., Zhai, Z., Li, Q., Huang, Z., et al. (2025). Diisooctyl phthalate (DIOP) exposure leads to cell apoptosis to HUVEC cells and toxicity to Caenorhabditis elegans through increasing the oxidative stress. Ecotoxicol. Environ. Saf. 290, 117594. doi:10.1016/j.ecoenv.2024.117594
Ma, D. (2009). “Comparative study between Ligusticum Chuanxiong and its close relative Ligusticum sinense Oliv. from three different origins,” in Master (Chengdu Chengdu University of Traditional Chinese Medicine).
Ma, K. T., Wu, Y. J., Yang, Y. X., Wu, T., Chen, C., Peng, F., et al. (2024). A novel phthalein component ameliorates neuroinflammation and cognitive dysfunction by suppressing the CXCL12/CXCR4 axis in rats with vascular dementia. J. Ethnopharmacol. 328, 118117. doi:10.1016/j.jep.2024.118117
ManLi, Y., HaoDong, Y., ZhiShu, T., XiaoHui, H., Rui, Z., TaoTao, X., et al. (2023). Lignan and phthalide derivatives from the rhizome of ligusticum chuanxiong (rhizoma chuanxiong) and evaluation of their anti-xanthine oxidase activities. ACS omega 8 (42), 39855–39864. doi:10.1021/acsomega.3c06172
Mao, Z., Tian, L., Liu, J., Wu, Q., Wang, N., Wang, G., et al. (2022). Ligustilide ameliorates hippocampal neuronal injury after cerebral ischemia reperfusion through activating PINK1/Parkin-dependent mitophagy. Phytomedicine 101, 154111. doi:10.1016/j.phymed.2022.154111
Mathew, S., and Abraham, T. E. (2004). Ferulic acid: an antioxidant found naturally in plant cell walls and feruloyl esterases involved in its release and their applications. Crit. Rev. Biotechnol. 24 (2-3), 59–83. doi:10.1080/07388550490491467
Michel, H. E., Tadros, M. G., Esmat, A., Khalifa, A. E., and Abdel-Tawab, A. M. (2017). Tetramethylpyrazine ameliorates rotenone-induced Parkinson’s disease in rats: involvement of its anti-inflammatory and anti-apoptotic actions. Mol. Neurobiol. 54 (7), 4866–4878. doi:10.1007/s12035-016-0028-7
Min, S., Tao, W., Ding, D., Zhang, X., Zhao, S., Zhang, Y., et al. (2022). Tetramethylpyrazine ameliorates acute lung injury by regulating the Rac1/LIMK1 signaling pathway. Front. Pharmacol. 13, 1005014. doi:10.3389/fphar.2022.1005014
Ming, Y., Zhaoping, W., Zhiqiang, L., Bo, L., Hua, Z., and Jing, L. (2024). Network pharmacological study and experimental validationof Rhizoma Chuanxiong volatile oil in the treatment of angina pectoris. Chin. J. Laboratory Animals 32 (07), 867–878.
Mingyan, L., Lirong, L., Menghan, F., Zhu, M., Zhang, Y., Yan, X., et al. (2024). Effect and mechanism of Chuanxiong Rhizoma on permeability of blood-brain barrier. Chinese herbal medicine. 55 (01), 138–147.
Naito, T., Ikeya, Y., Okada, M., Mistuhashi, H., and Maruno, M. (1996). Two phthalides from Ligusticum chuanxiong. Phytochemistry 41 (1), 233–236. doi:10.1016/0031-9422(95)00524-2
Or, T. C. T., Yang, C. L. H., Law, A. H. Y., Li, J. C. B., and Lau, A. S. Y. (2011). Isolation and identification of anti-inflammatory constituents from Ligusticum chuanxiong and their underlying mechanisms of action on microglia. Neuropharmacology 60 (6), 823–831. doi:10.1016/j.neuropharm.2010.12.002
Pu, Z.-H., Liu, J., Peng, C., Luo, M., Zhou, Q.-M., Xie, X.-F., et al. (2019a). Nucleoside alkaloids with anti-platelet aggregation activity from the rhizomes of Ligusticum striatum. Nat. Prod. Res. 33 (10), 1399–1405. doi:10.1080/14786419.2017.1416382
Pu, Z.-H., Peng, C., Xie, X.-F., Luo, M., Zhu, H., Feng, R., et al. (2019b). Alkaloids from the rhizomes of Ligusticum striatum exert antimigraine effects through regulating 5-HT1B receptor and c-Jun. J. Ethnopharmacol. 237, 39–46. doi:10.1016/j.jep.2019.03.026
Qi, X. Y., Peng, G. C., Han, Q. T., Yan, J., Chen, L. Z., Wang, T., et al. (2024). Phthalides from the rhizome of Ligusticum chuanxiong Hort. attenuate diabetic nephropathy in mice. J. Ethnopharmacol. 319 (Pt 2), 117247. doi:10.1016/j.jep.2023.117247
Qiu, S. H., Zeng, Y. Q., Pan, K. Y., Tang, Y. C., and Xu, J. M. (1979). Ligusticum chuanxiong acta phytotax. Sin. 17, 102.
Qunfang, R. (2024). Effects of Chuanxiong Cha Tiao San plus flavor combined with acupuncture on patients with chronic headache. Pract. Integr. Chin. West. Med. Clin. 24 (02), 98–100+116. doi:10.13638/j.issn.1671-4040.2024.02.029
Rai, U., Kosuru, R., Prakash, S., Tiwari, V., and Singh, S. (2019). Tetramethylpyrazine alleviates diabetic nephropathy through the activation of Akt signalling pathway in rats. Eur. J. Pharmacol. 865, 172763. doi:10.1016/j.ejphar.2019.172763
Ran, X., and Qingfang, W. (2024). Observation on the therapeutic effect of Da Chuanxiong Oral Liquid in the treatment of post-stroke sequelae. Syst. Med. 9 (21), 177–180. doi:10.19368/j.cnki.2096-1782.2024.21.177
Ren, D., Xie, N., Yang, N., Qian, S., and Duan, J. (2008). Studies on the chemical constituents of aerial parts of Ligusticum chuanxiong Hort. China J. Chin. Mat. Med., 2417–2418.
Ren, D. C., Yang, N. Y., Qian, S. H., Xie, N., Zhou, X. M., and Duan, J. A. (2007). Chemical study on aerial parts of Ligusticum chuanxiong. Zhongguo Zhong Yao Za Zhi 32 (14), 1418–1420.
Ren, T., Xie, D., Peng, C., Gui, L., Price, M., Zhou, S., et al. (2022). Molecular evolution and phylogenetic relationships of Ligusticum (Apiaceae) inferred from the whole plastome sequences. BMC Ecol. Evol. 22 (1), 55. doi:10.1186/s12862-022-02010-z
Ren, Z., Ma, J., Zhang, P., Luo, A., Zhang, S., Kong, L., et al. (2012). The effect of ligustrazine on L-type calcium current, calcium transient and contractility in rabbit ventricular myocytes. J. Ethnopharmacol. 144 (3), 555–561. doi:10.1016/j.jep.2012.09.037
Ruan, Q., Zhang, Y., Hu, Y., and He, X. (2003). Effects of different preparation methods on chemical constituents of volatile oil of Ligusticum chuanxiong Hort. China J. Chin. Mat. Med., 95–97.
Scheltens, P., De Strooper, B., Kivipelto, M., Holstege, H., Chetelat, G., Teunissen, C. E., et al. (2021). Alzheimer's disease. Lancet 397 (10284), 1577–1590. doi:10.1016/S0140-6736(20)32205-4
Shanshan, L., Qin, Z., Pengfei, Q., Ming, Y., Shuyuan, S., Xiaojin, L., et al. (2021). Effect and mechanism of phthalides from Ligusticum chuanxiong on oxygen-glucose deprivation/reperfusion induced MDCK-MDR1 cell injury. Chin. Herb. Med. 52 (10), 2958–2966.
Shao, H., He, X., Zhang, L., Du, S., Yi, X., Cui, X., et al. (2021). Efficacy of ligustrazine injection as adjunctive therapy in treating acute cerebral infarction: a systematic review and meta-analysis. Front. Pharmacol. 12, 761722. doi:10.3389/fphar.2021.761722
Shaojie, Z., Lijun, A., Zhengguo, L., Xuelian, W., Honglin, W., Lijuan, S., et al. (2021). Structural elucidation of an immunological arabinan from the rhizomes of Ligusticum chuanxiong, a traditional Chinese medicine. Int. J. Biol. Macromol. 170, 42–52. doi:10.1016/j.ijbiomac.2020.12.069
Sharawy, M. H., El-Agamy, D. S., Shalaby, A. A., and Ammar el, S. M. (2013). Protective effects of methyl palmitate against silica-induced pulmonary fibrosis in rats. Int. Immunopharmacol. 16 (2), 191–198. doi:10.1016/j.intimp.2013.04.007
Shi, J., Li, S., Yi, L., Gao, M., Cai, J., Yang, C., et al. (2024a). Levistolide a attenuates acute kidney injury in mice by inhibiting the TLR-4/NF-κB pathway. Drug Des. Devel Ther. 18, 5583–5597. doi:10.2147/dddt.S476548
Shi, Y., Li, H., Lin, Y., Wang, S., and Shen, G. (2024b). Effective constituents and protective effect of Mudan granules against Schwann cell injury. J. Ethnopharmacol. 323, 117692. doi:10.1016/j.jep.2023.117692
Shuai, S. Y., Liu, S. S., Liu, X. J., Zhang, G. S., Zheng, Q., Yue, P. F., et al. (2022). Essential oil of Ligusticum chuanxiong Hort. Regulated P-gp protein and tight junction protein to change pharmacokinetic parameters of temozolomide in blood, brain and tumor. J. Ethnopharmacol. 298, 115646. doi:10.1016/j.jep.2022.115646
Shupei, B. (2010). Clinical observation on 36 cases of chronic idiopathic pulmonary fibrosis treated with Chuanxiong auxiliary therapy. China Med. Front. 5 (04), 26+29.
Sikora, J., Karczmarska-Wodzka, A., Bugieda, J., and Sobczak, P. (2022). The importance of platelets response during antiplatelet treatment after ischemic stroke-between benefit and risk: a systematic review. Int. J. Mol. Med. 23 (3), 1043. doi:10.3390/ijms23031043
Siqueira, H. D. S., Neto, B. S., Sousa, D. P., Gomes, B. S., da Silva, F. V., Cunha, F. V. M., et al. (2016). α-Phellandrene, a cyclic monoterpene, attenuates inflammatory response through neutrophil migration inhibition and mast cell degranulation. Life Sci. 160, 27–33. doi:10.1016/j.lfs.2016.07.008
Song, X., Xing, W., Zhang, X., Wang, X., Ji, J., Lu, J., et al. (2023). Exploring the synergic mechanism of Ligusticum striatum DC. and borneol in attenuating BMECs injury and maintaining tight junctions against cerebral ischaemia based on the HIF-1α/VEGF signalling pathway. J. Ethnopharmacol. 301, 115764. doi:10.1016/j.jep.2022.115764
Srimagal, A., Mishra, S., and Pradhan, R. C. (2017). Effects of ethyl oleate and microwave blanching on drying kinetics of bitter gourd. J. Food Sci. Technol. 54 (5), 1192–1198. doi:10.1007/s13197-017-2518-7
Standardization, I. O. f. (2024). Traditional Chinese medicine — ligusticum chuanxiong rhizome. Available online at: https://www.iso.org/obp/ui/en/#iso:std:iso:8071:ed-1:v1:en.
Sun, W.-T., Wang, X.-C., Novakovic, A., Wang, J., He, G.-W., and Yang, Q. (2019). Protection of dilator function of coronary arteries from homocysteine by tetramethylpyrazine: role of ER stress in modulation of BKCa channels. Vasc. Pharmacol. 113, 27–37. doi:10.1016/j.vph.2018.10.009
Suručić, R., Kundaković, T., Lakušić, B., Drakul, D., Milovanović, S. R., and Kovačević, N. (2017). Variations in chemical composition, vasorelaxant and angiotensin I-converting enzyme inhibitory activities of essential oil from aerial parts of seseli pallasii besser (Apiaceae). Chem. Biodivers. 14 (5). doi:10.1002/cbdv.201600407
Szteyn, K., and Singh, H. (2020). BK(Ca)Channels as targets for cardioprotection. Antioxidants 9 (8), 760. doi:10.3390/antiox9080760
Takashi, N., Takao, K., Kazuaki, N., Yukinobu, I., Minoru, O., and Hiroshi, M. (1992). Two phthalides from Ligusticum chuangxiong. Phytochemistry 31 (2), 639–642. doi:10.1016/0031-9422(92)90051-Q
Tan, J., Yang, J., Aobulikasimu, N., Zhang, C., Cao, B., Lv, H., et al. (2023). Senkyunolide B exhibits broad-spectrum antifungal activity against plant and human pathogenic fungi via inhibiting spore germination and destroying the mature biofilm. Pest Manag. Sci. 79 (12), 4952–4963. doi:10.1002/ps.7696
Tang, F., Yan, Y. M., Yan, H. L., Wang, L. X., Hu, C. J., Wang, H. L., et al. (2021). Chuanxiongdiolides R4 and R5, phthalide dimers with a complex polycyclic skeleton from the aerial parts of Ligusticum chuanxiong and their vasodilator activity. Bioorg Chem. 107, 104523. doi:10.1016/j.bioorg.2020.104523
Tang, G. H., Jiang, G. H., and Zheng, L. F. (2000). Pharmacokinetics of perlolyrine in rats by stable isotope dilution in conjunction with GC-MS. Acta Pharmacol. Sin. 21 (7), 660–662.
Tang, Y., Li, Q., Yuan, C., Chen, S., Luo, S., Guo, J., et al. (2022). Profiling complex volatile components by HS-GC-MS and entropy minimization software: an example on Ligusticum chuanxiong Hort. J. Pharm. Biomed. 218, 114854. doi:10.1016/j.jpba.2022.114854
Tang, Y., Zhu, M., Yu, S., Hua, Y., Duan, J. A., Su, S., et al. (2010). Identification and comparative quantification of bio-active phthalides in essential oils from si-Wu-tang, fo-shou-san, radix angelica and rhizoma chuanxiong. Molecules 15 (1), 341–351. doi:10.3390/molecules15010341
Tianhao, Z., Lu, H., Ou, D., Cheng, P., Jinfeng, C., Yuan, P., et al. (2022). Study on the anti-migraineeffect of volatile oil of rhizoma chuanxiong based on 5-HT1B receptor. Chin. J. Traditional Chin. Med. 40 (03), 174–177+271. doi:10.13193/j.issn.1673-7717.2022.03.040
Türkez, H., Celik, K., and Toğar, B. (2014). Effects of copaene, a tricyclic sesquiterpene, on human lymphocytes cells in vitro. Cytotechnology 66 (4), 597–603. doi:10.1007/s10616-013-9611-1
Wang, G., Dai, G., Song, J., Zhu, M., Liu, Y., Hou, X., et al. (2018). Lactone component from ligusticum chuanxiong alleviates myocardial ischemia injury through inhibiting autophagy. Front. Pharmacol. 9, 301. doi:10.3389/fphar.2018.00301
Wang, H., Bao, Q., Yi, H., and Xia, Q. (2019). The evaluation of embryotoxicity of Ligusticum chuanxiong on mice and embryonic stem cells. J. Ethnopharmacol. 239, 111895. doi:10.1016/j.jep.2019.111895
Wang, K., Chen, Q., Shao, Y., Yin, S., Liu, C., Liu, Y., et al. (2021a). Anticancer activities of TCM and their active components against tumor metastasis. Biomed. Pharmacother. 133, 111044. doi:10.1016/j.biopha.2020.111044
Wang, K., Zhou, Y., Wen, C., Du, L., Li, L., Cui, Y., et al. (2024). Protective effects of tetramethylpyrazine on myocardial ischemia/reperfusion injury involve NLRP3 inflammasome suppression by autophagy activation. Biochem. Pharmacol. 229, 116541. doi:10.1016/j.bcp.2024.116541
Wang, L.-Y., Tang, Y.-P., Liu, X., Zhu, M., Tao, W.-W., Li, W.-X., et al. (2015). Effects of ferulic acid on antioxidant activity in Angelicae Sinensis Radix, Chuanxiong Rhizoma, and their combination. Chin. J. Nat. Med. 13 (6), 401–408. doi:10.1016/s1875-5364(15)30032-7
Wang, M., Hayashi, H., Horinokita, I., Asada, M., Iwatani, Y., Liu, J.-x., et al. (2021b). Neuroprotective effects of Senkyunolide I against glutamate-induced cells death by attenuating JNK/caspase-3 activation and apoptosis. Biomed. Pharmacother. 140, 111696. doi:10.1016/j.biopha.2021.111696
Wang, M., Yao, M., Liu, J., Takagi, N., Yang, B., Zhang, M., et al. (2020). Ligusticum chuanxiong exerts neuroprotection by promoting adult neurogenesis and inhibiting inflammation in the hippocampus of ME cerebral ischemia rats. J. Ethnopharmacol. 249, 112385. doi:10.1016/j.jep.2019.112385
Wang, N., Zhang, Y., Li, X., Tong, Y., Kong, H., and Xu, G. (2010). Analysis of volatile oils of Ligusticum chuanxiong Hort. from different geographical origins by comprehensive two-dimensional gas chromatography/time-of-flight mass spectrometry. Se Pu 28 (4), 329–335. doi:10.3724/sp.j.1123.2010.00329
Wang, Y., Gao, X., Wang, P., Fushan, A., Shunei, Y., and Shanxia, X. (1985). Adenine and adenosine, another group of active ingredients in chuanxiong rhizome. Chin. Tradit. Herb. Drugs 16, 17.
Wei, Y., Liu, J., Zhang, H., Du, X., Luo, Q., Sun, J., et al. (2016). Ligustrazine attenuates inflammation and the associated chemokines and receptors in ovalbumine-induced mouse asthma model. Environ. Toxicol. Phar 46, 55–61. doi:10.1016/j.etap.2016.07.005
Weng, G., Zhou, B., Liu, T., Huang, Z., and Huang, S. (2021). Tetramethylpyrazine improves cognitive function of alzheimer's disease mice by regulating SSTR4 ubiquitination. Drug Des. Devel Ther. 15, 2385–2399. doi:10.2147/DDDT.S290030
Whelan, J. (2008). The health implications of changing linoleic acid intakes. Prostagl. Leukot. Essent. Fat. Acids 79 (3-5), 165–167. doi:10.1016/j.plefa.2008.09.013
Wu, J.-Z., Li, Y.-J., Huang, G.-R., Xu, B., Zhou, F., Liu, R.-P., et al. (2021). Mechanisms exploration of Angelicae Sinensis Radix and Ligusticum Chuanxiong Rhizoma herb-pair for liver fibrosis prevention based on network pharmacology and experimental pharmacologylogy. Chin. J. Nat. Med. 19 (4), 241–254. doi:10.1016/s1875-5364(21)60026-2
Wu, Q., Liu, J., Mao, Z., Tian, L., Wang, N., Wang, G., et al. (2022). Ligustilide attenuates ischemic stroke injury by promoting Drp1-mediated mitochondrial fission via activation of AMPK. Phytomedicine 95, 153884. doi:10.1016/j.phymed.2021.153884
Wu, Q., and Yang, X. W. (2008). GC-MS analysis of essential oil from rhizomes of Ligusticum chuanxiong cultivated in GAP base for Chinese medicinal materials of China. Zhongguo Zhong Yao Za Zhi 33 (3), 276–280.
Xia, K., Jin, Z., Qiu, Q., Zhou, Y., Lu, Y., Qiu, T., et al. (2024). Ligustilide alleviates oxidative stress during renal ischemia-reperfusion injury through maintaining Sirt3-dependent mitochondrial homeostasis. Phytomedicine 134, 155975. doi:10.1016/j.phymed.2024.155975
Xie, Y., Liu, H., Lin, L., Zhao, M., Zhang, L., Zhang, Y., et al. (2019). Application of natural deep eutectic solvents to extract ferulic acid from Ligusticum chuanxiong Hort with microwave assistance. RSC Adv. 9 (39), 22677–22684. doi:10.1039/c9ra02665g
Xu, J., Zhang, X., Jin, A., Pan, Y., Li, Z., Meng, X., et al. (2022). Trends and risk factors associated with stroke recurrence in China, 2007-2018. Jama Netw. Open 5 (6), e2216341. doi:10.1001/jamanetworkopen.2022.16341
Xu, T., Pan, Z., Dong, M., Yu, C., and Niu, Y. (2015). Ferulic acid suppresses activation of hepatic stellate cells through ERK1/2 and Smad signaling pathways in vitro. Biochem. Pharmacol. 93 (1), 49–58. doi:10.1016/j.bcp.2014.10.016
Xuhui, L., Lin, Z., and Yumei, H. (2023). Exploring the inhibitory effect of Chuanxiong volatile oil on glioma angiogenesis based on EGFR/VEGF-A signaling pathway. Mod. J. Integr. Chin. West. Med. 32 (05), 633–636+679.
Yajing, L., Zhi, M., Mingning, D., Kexin, J., Bing, X., Fei, Z., et al. (2023). Chuanxiong Rhizoma extracts prevent cholestatic liver injury by targeting H3K9ac-mediated and cholangiocyte-derived secretory protein PAI-1 and FN. Chin. J. Nat. Med. 21 (9), 694–709. doi:10.1016/S1875-5364(23)60416-9
Yan, H., Zhou, Y., Tang, F., Wang, C., Wu, J., Hu, C., et al. (2022). A comprehensive investigation on the chemical diversity and efficacy of different parts of Ligusticum chuanxiong. Food Funct. 13 (3), 1092–1107. doi:10.1039/d1fo02811a
Yan, X., Lin, J., Liu, Z., David, S. D., Liang, D., Nie, S., et al. (2024). The recent progress of tricyclic aromadendrene-type sesquiterpenoids: biological activities and biosynthesis. Biomolecules 14 (9), 1133. doi:10.3390/biom14091133
Yang, C. K., Pan, Q. Q., Ji, K., Luo, C. C., Tian, Z., Zhou, H. Y., et al. (2023). Protective mechanism of tetramethylpyrazine on cardiovascular system. Zhongguo Zhong Yao Za Zhi 48 (6), 1446–1454. doi:10.19540/j.cnki.cjcmm.20221012.402
Yang, L., Liu, J., Li, Y., and Qi, G. (2018). Bornyl acetate suppresses ox-LDL-induced attachment of THP-1 monocytes to endothelial cells. Biomed. Pharmacother. 103, 234–239. doi:10.1016/j.biopha.2018.03.152
Yang, Q., Huang, D. D., Li, D. G., Chen, B., Zhang, L. M., Yuan, C. L., et al. (2019). Tetramethylpyrazine exerts a protective effect against injury from acute myocardial ischemia by regulating the PI3K/Akt/GSK-3 signaling pathway. Cell Mol. Biol. Lett. 24, 17. doi:10.1186/s11658-019-0141-5
Yang, Y.-Y., Wu, Z.-Y., Xia, F.-B., Zhang, H., Wang, X., Gao, J.-L., et al. (2020). Characterization of thrombin/factor Xa inhibitors in Rhizoma Chuanxiong through UPLC-MS-based multivariate statistical analysis. Chin. Med. 15 (1), 93. doi:10.1186/s13020-020-00376-0
Yao, X., Jiao, S., Qin, M., Hu, W., Yi, B., and Liu, D. (2020). Vanillic acid alleviates acute myocardial hypoxia/reoxygenation injury by inhibiting oxidative stress. Oxid. Med. Cell Longev. 2020, 8348035. doi:10.1155/2020/8348035
Yeo, D., Hwang, S. J., Song, Y. S., and Lee, H. J. (2021). Humulene inhibits acute gastric mucosal injury by enhancing mucosal integrity. Antioxidants (Basel) 10 (5), 761. doi:10.3390/antiox10050761
Yiyu, Z., Dechao, M., Yongcheng, H., Jie, W., Jie, Y., Chaowen, Z., et al. (2023). Studies on the volatile oil fractions and in vitro anti-inflammatory activity of Rhizoma Ligustici Chuanxiong. Sichuan Agric. Sci. Technol. (04), 116–120.
Yoon, B. H., Jung, J. W., Lee, J. J., Cho, Y. W., Jang, C. G., Jin, C., et al. (2007). Anxiolytic-like effects of sinapic acid in mice. Life Sci. 81 (3), 234–240. doi:10.1016/j.lfs.2007.05.007
Yuan, C., Sha, X., Xiong, M., Zhong, W., Wei, Y., Li, M., et al. (2021). Uncovering dynamic evolution in the plastid genome of seven Ligusticum species provides insights into species discrimination and phylogenetic implications. Sci. Rep. 11 (1), 988. doi:10.1038/s41598-020-80225-0
Yuan, Y., Zhou, J., and Zheng, X. (2000). Studies on volatile oil in Ligusticum chuanxiong by supercritical fluid extraction. Chin. Pharm. J. 35, 84–87.
Yue, Y., Chunyan, M., Zixin, S., Yingxin, Z., and Gaoqi, L. (2024). Progress in the study of chemical composition and pharmacological effects of chuanxiong rhizome. Glob. Chin. Med., 1–9.
Yumei, H., Ruibin, L., Jie, Y., and Lin, Z. (2022). Impacts of volatile oil of ligusticum chuanxiong on glioma angiogenesis by regulating EGFR/VEGF-A pathway via LRlG2. Chin. Pharm. 25 (11), 1873–1877+1903. doi:10.19962/j.cnki.issn1008-049X.2022.11.001
Yun, H., Shuiqing, G., Xianjian, H., and Dongliang, Z. (2021). Effect of dl-3-n-butylphthalide on the lmprovement of neurological function in rats with traumatic brain lnjury. Chin. Pharm. Ind. 30 (08), 32–35.
Zeng, P., Su, H. F., Ye, C. Y., Qiu, S. W., Shi, A., Wang, J. Z., et al. (2022). A tau pathogenesis-based network pharmacology approach for exploring the protections of chuanxiong rhizoma in alzheimer's disease. Front. Pharmacol. 13, 877806. doi:10.3389/fphar.2022.877806
Zhai, J., Ren, Z., Wang, Y., Han, M., Han, N., Liu, Z., et al. (2020). Traditional Chinese patent medicine Zhixiong Capsule (ZXC) alleviated formed atherosclerotic plaque in rat thoracic artery and the mechanism investigation including blood-dissolved-component-based network pharmacology analysis and biochemical validation. J. Ethnopharmacol. 254, 112523. doi:10.1016/j.jep.2019.112523
Zhang, F., Xu, Y., Shen, L., Huang, J., Xu, S., Li, J., et al. (2021). GuanXinNing tablet attenuates alzheimer's disease via improving gut microbiota, host metabolites, and neuronal apoptosis in rabbits. Evid. Based Complement. Altern. Med. 2021, 9253281. doi:10.1155/2021/9253281
Zhang, H., Han, T., Yu, C.-H., Jiang, Y.-P., Peng, C., Ran, X., et al. (2012). Analysis of the chemical composition, acute toxicity and skin sensitivity of essential oil from rhizomes of Ligusticum chuanxiong. J. Ethnopharmacol. 144 (3), 791–796. doi:10.1016/j.jep.2012.10.010
Zhang, H., Tang, W., Wang, S., Zhang, J., and Fan, X. (2020a). Tetramethylpyrazine inhibits platelet adhesion and inflammatory response in vascular endothelial cells by inhibiting P38 MAPK and NF-kappa B signaling pathways. Inflammation 43 (1), 286–297. doi:10.1007/s10753-019-01119-6
Zhang, L., Yan, Q., Zhang, W., Li, X., Zhang, X., Du, S., et al. (2022). Enhancement of the functionality of attenuating acute lung injury by a microemulsion formulation with volatile oil of Angelicae Sinensis Radix and Ligusticum Chuanxiong Rhizoma encapsulated. Biomed. Pharmacother. 156, 113888. doi:10.1016/j.biopha.2022.113888
Zhang, X., Deng, F., Wang, X., Liu, F., Zhu, Y., Yu, B., et al. (2024a). Synergistic amelioration between Ligusticum striatum DC and borneol against cerebral ischemia by promoting astrocytes-mediated neurogenesis. J. Ethnopharmacol. 327, 118062. doi:10.1016/j.jep.2024.118062
Zhang, X., Yan, H. W., Feng, Z. M., Yang, Y. N., Jiang, J. S., and Zhang, P. C. (2020b). Neophathalides A and B, two pairs of unusual phthalide analog enantiomers from Ligusticum chuanxiong. Org. Biomol. Chem. 18 (28), 5453–5457. doi:10.1039/d0ob01014f
Zhang, Y., Li, D., Jia, Z., Mei, J., Wang, Y., Zhang, Y., et al. (2024b). Zhizi-Chuanxiong herb pair alleviates atherosclerosis progression in ApoE(-/-) mice by promoting the methylation of FGFR3 to inhibit MAPK/ERK-mediated apoptosis. J. Ethnopharmacol. 319 (Pt 1), 117188. doi:10.1016/j.jep.2023.117188
Zhang, Y., Ma, X.-j., Guo, C.-y., Wang, M.-m., Kou, N., Qu, H., et al. (2016). Pretreatment with a combination of ligustrazine and berberine improves cardiac function in rats with coronary microembolization. Acta Pharmacol. Sin. 37 (4), 463–472. doi:10.1038/aps.2015.147
Zhang, Y., Qi, Y., Jia, Z., Li, Y., Wu, L., Zhou, Q., et al. (2024c). Effects and mechanisms of Zhizi Chuanxiong herb pair against atherosclerosis: an integration of network pharmacology, molecular docking, and experimental validation. Chin. Med. 19 (1), 8. doi:10.1186/s13020-023-00874-x
Zhao, D.-Y., Yu, D.-D., Ren, L., and Bi, G.-R. (2020). Ligustilide protects PC12 cells from oxygen-glucose deprivation/reoxygenation-induced apoptosis via the LKB1-AMPK-mTOR signaling pathway. Neural Regen. Res. 15 (3), 473–481. doi:10.4103/1673-5374.266059
Zheng, Q., Huang, Y.-y., Zhu, P.-c., Tong, Q., Bao, X.-y., Zhang, Q.-h., et al. (2018). Ligustrazine exerts cardioprotection in animal models of myocardial ischemia/reperfusion injury: preclinical evidence and possible mechanisms. Front. Pharmacol. 9, 729. doi:10.3389/fphar.2018.00729
Zhong, C., Liu, Z., Zhang, X., Pu, Y., Yang, Z., and Bao, Y. (2021). Physicochemical properties of polysaccharides from Ligusticum chuanxiong and analysis of their anti-tumor potential through immunoregulation. Food Funct. 12 (4), 1719–1731. doi:10.1039/d0fo02978e
Zhong-Hui, P., Peng, C., Xie, X. F., Min, L., Huan, Z., Rui, F., et al. (2019). Alkaloids from the rhizomes of Ligusticum striatum exert antimigraine effects through regulating 5-HT1B receptor and c-Jun. J. Ethnopharmacol. 237, 39–46. doi:10.1016/j.jep.2019.03.026
Zhou, G., Huang, H., Jiang, Z., Jiang, X., and Wang, Q. (2024). Research progress on chemical constituents of ligusticum chuanxiong hort and their application in prevention and treatment of cardiovascular diseases. Tradit. Chin. Med. 13, 2841–2849. doi:10.12677/tcm.2024.1311419
Zhu, L., and Sheng, H. (2013). GC-MS analysis of volatile components in Ligusticumv chuanxiong Hort. J. Shandong Univ. Trad. Chin. Med. 37, 164–165. doi:10.16294/j.cnki.1007-659x.2013.02.031
Zhu, Y. L., Huang, J., Chen, X. Y., Xie, J., Yang, Q., Wang, J. F., et al. (2022). Senkyunolide I alleviates renal Ischemia-Reperfusion injury by inhibiting oxidative stress, endoplasmic reticulum stress and apoptosis. Int. Immunopharmacol. 102, 108393. doi:10.1016/j.intimp.2021.108393
Zhuang, Z., Wang, Z.-H., Huang, Y.-Y., Zheng, Q., and Pan, X.-D. (2020). Protective effect and possible mechanisms of ligustrazine isolated from Ligusticum wallichii on nephropathy in rats with diabetes: a preclinical systematic review and meta-analysis. J. Ethnopharmacol. 252, 112568. doi:10.1016/j.jep.2020.112568
Zou, J., Chen, G.-D., Zhao, H., Huang, Y., Luo, X., Xu, W., et al. (2018). Triligustilides A and B: two pairs of phthalide trimers from angelica sinensis with a complex polycyclic skeleton and their activities. Org. Lett. 20 (3), 884–887. doi:10.1021/acs.orglett.8b00017
Zuo, A., Wang, L., Xiao, H., Li, L., Liu, Y., and Yi, J. (2011). Identification of the absorbed components and metabolites in rat plasma after oral administration of Rhizoma Chuanxiong decoction by HPLC-ESI-MS/MS. J. Pharm. Biomed. Anal. 56 (5), 1046–1056. doi:10.1016/j.jpba.2011.08.010
Zuo, Z., Zuo, P.-f., Sheng, Z.-l., Wang, X., Ding, J.-d., and Ma, G.-s. (2019). Tetramethylprazine attenuates myocardial ischemia/reperfusion injury through modulation of autophagy. Life Sci. 239, 117016. doi:10.1016/j.lfs.2019.117016
Glossary
Aβ amyloid-β protein
AD Alzheimer’s Disease
AKI acute kidney injury
ALI acute lung injury
ALS Amyotrophic lateral sclerosis
ARDS acute respiratory distress syndrome
AS atherosclerosis
ATP adenosine triphosphate
AMPK adenosine 5‘-monophosphate (AMP)-activated protein kinase
AQP aquaporin 4
AK2 Janus kinase 2
Bcl-2 B-cell lymphoma-2
BKCa big potassium calcium-activated channels
Bax Bcl-2 associated X protein
CX dried rhizome of Ligusticum chuanxiong
CCVD cardio-cerebral vascular diseases
CUL4B Cullin 4B
CCI chronic compression injury
c-JUN c-Jun N-terminal kinase
Cx43 connexin43
CXCL12 chemokine C-X-C motif ligand 12
CXCR4 chemokine C-X-C motif receptor 4
Caspase-1 cysteine-requiring aspartate protease-1
caspase-3 cysteinyl aspartate specific proteinase 3
COX2 cyclooxygenase-2
caspase-8 cysteine-requiring aspartate protease-8
CCR1 C-C Motif Chemokine Receptor 1
DN diabetic nephropathy
DNA deoxyriboNucleic Acid
EO essential oil
ERK extracellular signal-regulated kinase
FAK focal adhesion kinase
FGF fibroblast growth factor
FGFR3 fibroblast growth factor receptor 3
GSK Glycogen Synthase Kinase
GTP guanosine triphosphate
GPX4 glutathione peroxidase 4
GSDMD gasdermin D
HD Huntington’s disease
HO-1 heme oxygenase-1
HSC hepatic stellate cell
H3K9ac histone 3 lysine 9 acetylation
hNOS human neuronal oxidative stress
IL Interleukin
I/R ischemia/reperfusion
iNOS inducible nitric oxide synthase
ISO International Organization for Standardization
KAT2A lysine acetyltransferase 2A
LCP ligusticum CX polysaccharides
LKB1 liver kinase B1
LIMK1 LIM Domain Kinase 1
LD50 median lethal dose
MAPK mitogen-activated protein kinase
MCPIP1 monocyte chemotactic protein-induced protein 1
ME microsphere-embolized
MMP matrix metalloprotein
mTOR mammalian target of rapamycin
NFTs neurofibrillary tangles
NMPA National Medical Products Administration
NO nitric oxide
Nrf2 nuclear factor erythroid 2-related factor 2
NFκB nuclear factor kappa-B
NADPH nicotinamide adenine dinucleotide phosphate
NOD2 nucleotide-binding oligomerization domain 2
OGD/R oxygen-glucose deprivation/reperfusion
NLRP3 Nod-like receptor family pyrin domain-containing 3
PD Parkinson’s disease
PKC protein kinase C
PI3K phosphoinositide 3-kinase
PPAR peroxisome proliferator-activated receptors
PINK1 PTEN induced putative kinase 1
Prx1 paired related homeobox 1
PGC-1α peroxisome proliferator-activated receptor-γ coactivator-1α
PKC-β protein kinase C beta
PAI-1 plasminogen activator inhibitor-1
QBT a novel phthalein metabolite
Rac1 Rac Family Small GTPase 1
SMCs smooth muscle cells
SR scavenger receptor
SREBPs sterol regulatory element-binding proteins
STZ streptozotocin
SOD superoxide dismutase
siRNA small interfering RNA
STAT3 signal transducer and activator of transcription 3
SSRT4 somatostatin receptor 4
SIRT-1 silent information regulator 1
SCAP sterol regulatory element-binding proteins leavage activating protein
TBN tetramethylpyrazine nitrone
TCM traditional Chinese medicine
TMP tetramethylpyrazine
TNF tumor necrosis factor
TRAF6 tumor necrosis factor receptor-associated factor 6
TLR4 Toll-like receptor 4
TDP-43 TAR DNA-binding protein of 43 kDa;
WCVP World Checklist of Vascular Plants
xCT cystine-glutamate antiporter
ZO-1 Zonula occludens-1
3-NP 3-Nitropropionic acid
5-HIAA 5-hydroxyindole acetic acid
5-HT 5-hydroxytryptamine
5-HT1B 5-Hydroxytryptamine Receptor 1B
Keywords: ethnopharmacology, Ligusticum chuanxiong, traditional Chinese medicine, metabolite, pharmacological effects, cardio-cerebral vascular diseases
Citation: Wang Y, Wu L, Wang H, Jiang M, Chen Y, Zheng X, Li L, Yin Q, Han L, Bai L and Bian Y (2025) Ligusticum chuanxiong: a chemical, pharmacological and clinical review. Front. Pharmacol. 16:1523176. doi: 10.3389/fphar.2025.1523176
Received: 05 November 2024; Accepted: 21 March 2025;
Published: 01 April 2025.
Edited by:
Michael Heinrich, University College London, United KingdomReviewed by:
Ruyu Yao, Chinese Academy of Sciences (CAS), ChinaDiego Rivera, University of Murcia, Spain
Bin Yu, Nanjing University of Chinese Medicine, China
Bob Allkin, Royal Botanic Gardens, Kew, United Kingdom
Copyright © 2025 Wang, Wu, Wang, Jiang, Chen, Zheng, Li, Yin, Han, Bai and Bian. This is an open-access article distributed under the terms of the Creative Commons Attribution License (CC BY). The use, distribution or reproduction in other forums is permitted, provided the original author(s) and the copyright owner(s) are credited and that the original publication in this journal is cited, in accordance with accepted academic practice. No use, distribution or reproduction is permitted which does not comply with these terms.
*Correspondence: Yuan Bian, Ymlhbnl1YW41NjdAMTI2LmNvbQ==; Lan Bai, YmxjaUAxNjMuY29t; Lizhu Han, eXVtbXlsaWxhQDE2My5jb20=
†These authors share first authorship