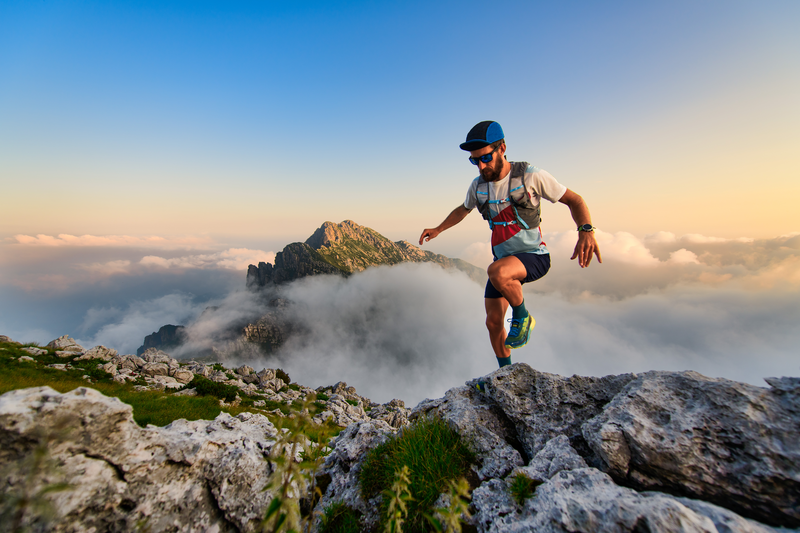
94% of researchers rate our articles as excellent or good
Learn more about the work of our research integrity team to safeguard the quality of each article we publish.
Find out more
REVIEW article
Front. Pharmacol. , 24 January 2025
Sec. Drug Metabolism and Transport
Volume 16 - 2025 | https://doi.org/10.3389/fphar.2025.1522814
This article is part of the Research Topic Liver Diseases and Their Impact on Drug Metabolism View all articles
Monosodium glutamate (MSG) is an odorless white solid crystalline derived from the amino acid glutamic acid. It is widely used as a flavor enhancer, but its excessive consumption has been associated with toxicity to various organs. In MSG-induced liver injury, few mechanisms have been identified, which started with the generation of reactive oxygen species that leads to oxidative stress which further causes liver injury. In response to this health concern, there is growing interest in various plant products such as plant extracts, flavonoids and phenolic compounds that were able to minimize oxidative stress, serum transaminases and scavenge free radicals in the liver after MSG administration. This review explores the potential of various plant products as dietary supplements to MSG-induced liver injury, focusing on their antioxidant activities, modulatory effects on liver function markers, and histological outcomes. By compiling this evidence, this review provides insights into their potential as preventive strategies against MSG-related liver toxicity, supporting their inclusion in dietary regimens for the maintenance of liver function.
In this modern era, urban communities increasingly rely on commercially processed foods and drugs, as a consequence of industrialization, urbanization, and the rapid expansion of the working class. Both food additives and drugs undergo extensive testing and regulation to ensure safety, efficacy, and compliance with established standards. Whether it is a pharmaceutical compound designed to treat a medical condition (Syed et al., 2023; Abdullah et al., 2024; Sari et al., 2024) or a food additive aimed to enhance food quality and safety (Pressman et al., 2017; Alemu, 2022), rigorous protocols govern their development, testing, and approval processes, reflecting a shared commitment to consumer health and risk minimization.
In Asia, monosodium glutamate (MSG) is a widely used food additive, primarily due to the increased demand in the food processing industry (Naveen Kumar et al., 2020). It imparts a special flavor known as umami, one of the five basic tastes, alongside sweetness, sourness, bitterness, and saltiness, MSG enhances the savory depth of foods. The term umami is derived from Japanese, meaning “pleasant savory taste.” This distinctive flavor leaves a subtle yet lasting aftertaste, inducing salivation and a slight sensation of furriness on the tongue, while stimulating areas across the throat, roof, and back of the mouth (Green and Nachtigal, 2012). Contrary to the misconception of a taste map specific for each flavor, umami is detected throughout the tongue, from its tip to its back, and across various regions of the mouth (Chamma et al., 2018). Moreover, studies show that umami receptors, including mGluR4, mGluR1, and taste receptor type 1 (T1R1 + T1R3) (Chaudhari et al., 2009), are widely distributed in almost every region of the tongue, debunking the myth of distinct taste regions in the tongue (Wijayasekara and Wansapala, 2017).
MSG typically appears as an odorless white crystalline solid, often in the form of monohydrate (Oluwole et al., 2024). It is highly water-soluble (∼740 g/L) but practically insoluble in organic solvents (Nguyen Thuy et al., 2020). The chemical formula of MSG is C5H8NNaO4 and its IUPAC name is sodium; (2S)-2-amino-5-hydroxy-5-oxopentanoate. Figure 1 illustrates the chemical structure of MSG. MSG is not hygroscopic or light-sensitive, but it has a high melting point, allowing it to maintain quality even during extended storage at room temperature (Nguyen Thuy et al., 2020). Its stability at high temperatures (decomposed above 350°C) ensures resilience during food processing. In solution, MSG acts as an ampholyte, functioning as either an acid or a base depending on the pH (Nahok et al., 2019). When glutamate is released from the weak ionic bond between sodium and glutamate, it can participate in various reactions under specific conditions, such as Maillard reaction in the presence of reducing sugars and elevated temperatures (Nguyen Thuy et al., 2020).
MSG is derived from glutamic acid, an amino acid common found in proteins and peptides found in nature. Commercially, it is produced through the fermentation of molasses and is present in various protein-rich fermented products, such as soy sauce and hydrolyzed vegetable protein (Peica et al., 2007). The free form of glutamic acid, known as glutamate, is a naturally occurring amino acid in the body, and plays a key role in human metabolism. It can be found abundantly in high-protein foods such as meat, fish, milk, and some vegetables (Loi and Cynober, 2022). Glutamate interacts with glutamate receptors, contributing to the characteristic taste of many processed foods (Yamamoto and Inui-Yamamoto, 2023). Notably, over 99.6% of MSG consists of naturally predominant L-glutamate enantiomer, which is responsible for its flavor-enhancing properties. Interestingly, while MSG contains both L- and D-glutamate isomers, only the L-glutamate enantiomer has flavor-enhancing properties (Bera et al., 2017).
In Chinese and Japanese cuisine, MSG is commonly used to enhance flavor in various ready-to-serve foods like soups and sauces. Although food safety agencies largely consider MSG safe, several studies have raised concerns about potential long-term health risks. A growing body of research suggests correlations between MSG and various health issues (Zanfirescu et al., 2019). Previous research indicates that MSG may exert toxic effects on fetal development (Shosha et al., 2023). Physiological complications linked to MSG toxicity include hypertension, obesity, gastrointestinal disturbances, and impairments in the nervous, reproductive and endocrine systems.
The average daily intake of MSG in developed countries is estimated to be 0.3–1.0 g/day, it has been reported to be 0.58 g/day and 10.0 g/day in the United Kingdom (Sahin et al., 2023). Additionally, MSG taken by Asian is estimated ranging of 10 to 20 fold than the European and American average daily intake (ADI) (Jubaidi et al., 2019). The consumption of MSG has been associated with symptoms such as numbness, weakness, flushing, sweating, dizziness, and headaches, typically occurring within 10 min to 2 h after eating and lasting up to 4 h (Zanfirescu et al., 2019). Additionally, MSG intake has been linked to increased risks of hyperlipidemia, hyperglycemia, and oxidative stress (Singh and Ahluwalia, 2012). Studies in adult mice after MSG treatment revealed alterations in levels of thiobarbituric acid reactive substances (TBARS) and antioxidant activities such as reduced glutathione (GSH), catalase (CAT), and superoxide dismutase (SOD), as well as disruption in biochemical parameters of carbohydrates, lipids, and proteins (Elbessoumy et al., 2010). Therefore, the convenience of processed foods must be weighed against these potential health concerns, prompting a closer examination of our dietary choices amid urbanized lifestyles (Chakraborty, 2019).
Studies on MSG-induced liver injury reveal significant adverse effects on hepatotoxicity markers, including decreased albumin and total protein activities, with prolonged MSG consumption contributing to liver dysfunction and insulin resistance (Niaz et al., 2018). Oxidative stress plays a pivotal role, calling for a re-evaluation of the MSG safety profile. Comprehensive research of various dosages, administration routes, durations, and experimental models provides valuable insights into MSG-induced liver damage. The adverse effects on hepatotoxicity markers, oxidative stress, and potential protective measures underscore the complexity of MSG’s impact on liver health, necessitating ongoing research and regulatory scrutiny.
Oxidative stress is a key factor in MSG-induced liver damage, primarily due to the harmful effects of reactive oxygen species (ROS), which reduce levels of GSH and activities ofantioxidant enzymes such as glutathione peroxidase (GPx), SOD and CAT, while increasing hepatic malondialdehyde (MDA) levels (Banerjee et al., 2021). Additionally, serum transaminases, alkaline phosphatase (ALP) and total bilirubin were also increased in MSG-induced liver damage (Elbessoumy et al., 2010; Abbas and Abbas, 2016; Sahin et al., 2023).
Bilirubin is a product of hemoglobin which is used as a biomarker to identify the injury of liver function. This is due to it’s the involvement in uptake, conjugation, and excretion function in the liver. Bilirubin levels in the plasma increase in severe liver injury. Then unconjugated ‘‘indirect’’ bilirubin is produced and is bound to albumin to be transported to the liver. Albumin synthesis is decreased in liver diseases (Sahin et al., 2023).
Previous studies have demonstrated that MSG increased the expression of pro-inflammatory cytokines such as tumor necrosis factor alpha (TNF-α) and interleukin (IL)-6 (Eid et al., 2019; Banerjee et al., 2021; Asejeje et al., 2023). MSG consumption increases ROS generation, leading to the damage of lipids, proteins and DNA, through free radicals’ activity. Lipid peroxidation damages polyunsaturated fatty acids in cell membranes. Ultimately, all these events cause cell death through apoptosis (Sahin et al., 2023). Figure 2 illustrates the overview of the mechanism of MSG-induced liver injury.
Dietary intervention is one of the anticipated strategies for preventing MSG toxicity, which has been mostly evaluated in preclinical studies. Plant products or natural remedies with a wide range of medicinal properties, such as antioxidant, anti-inflammatory and anti-apoptotic effects, have attracted researchers’ interest as potential countermeasures against MSG toxicity. For instance, various types of traditional medicine have shown efficacy in reducing MSG toxicity (Hajihasani et al., 2020). However, most studies on MSG-induced toxicity have been conducted only at the preclinical level without advancing to further stages. This section will discuss currently available plant products that have demonstrated hepatoprotective effects against MSG-induced liver injury (Table 1). By examining these prospects, this review aims to highlight the potential of plant products as dietary interventions for maintaining liver health in the context of MSG exposure.
Annona muricata Linn. (Annonaceae), commonly known as soursop or graviola, originates from tropical African countries. The plant’s leaves and root bark are traditionally used as anthelmintic and antiphlogistic medicines, while its flowers and fruit pods are used to treat catarrh (Mohammed et al., 2016). A. muricata contains tannins as its predominant compound, which exhibit liver protection against MSG-induced liver injury by reducing AST, ALT and ALP. Additionally, A. muricata restored antioxidant enzymes, namely, SOD, CAT, GST and GSH. Histopathological analysis of the A. muricata-treated group revealed only a few pyknotic nuclei of hepatocytes and a mild degree of hepatocyte degeneration. Furthermore, limited centrilobular hepatic vacuolation and mononuclear cell infiltration were observed in the A. muricata-treated group (Mohammed et al., 2016; Yousef, 2022). A. muricata treatment was also reported to mitigate MSG-induced cellular apoptosis, by significantly decreasing the higher levels of Bax, caspase 3, and P53 and increasing Bcl2 in MSG-treated rats (Shukry et al., 2020).
Camellia sinensis L. or green tea, belongs to the Theaceae family and originates from Southeast Asia (Southern China, North India, Myanmar and Cambodia) (Shivashankara et al., 2014). It is a natural source of antioxidants, containing high levels of polyphenols, such as catechins, gallic acid and ellagic acid. Ershad (2020) reported that oral gavage of 0.015 g/day of green tea extract for 30 days provided protection against MSG-induced liver injury. Green tea extracts lowered ALT and reduced hepatocyte diameter. Furthermore, another study by Al-Salmi et al. (2019) showed that both green tea extract-treated and zinc oxide/green tea extract nanoparticles-treated rats exhibited reduced lipid peroxidation, increased GPx and GSH activities, and restored liver architecture following MSG-induced liver damage, such as liver tissue necrotic and inflammatory cell infiltration into liver cords.
Eruca sativa Mill. is globally distributed and frequently eaten raw (leaves or sprouts) due to its characteristic peppery flavor (Testai et al., 2022). The seeds contain carotenoids, vitamin C, flavonoids (such as appiin and luteolin) and glucosinolates (El-Badawy et al., 2018). E. sativa has demonstrated several biological activities, including anticarcinogenic and antioxidant properties (Tousson et al., 2019). Oral administration of E. sativa at a dose of 30 mg/kg improved liver functions by reducing ALT, AST and ALP, as well as increasing total protein and albumin levels. Furthermore, E. sativa seeds were able to blunt the elevation of MDA and SOD. In MSG-treated livers, which showed hepatocyte derangement, central vein congestion and inflammatory cell infiltration, which were improved by E. sativa seed treatment.
Grape seed oil has gained interest among researchers worldwide due to its medicinal properties, such as high antioxidant, anti-inflammatory, anticancer and antimicrobial effects (Garavaglia et al., 2016). Additionally, grape seed oil contains a wide range of active pharmacological compounds, such as flavonoids, phenolic compounds, lipophilic constituents (such as vitamin E), minerals, unsaturated fatty acids and phytosterols (Garavaglia et al., 2016; Alshubaily et al., 2018). Linoleic acid, catechins, epicatechins, trans-resveratrol and procyanidin B1 were obtained from cold-pressing method of grape seed oil (Garavaglia et al., 2016). Oral pre-treatment with grape seed oil before MSG administration provides better protection against liver injury. Grape seed oil reduced the serum transaminases and ALP activities. Additionally, grape seed oil was able to reverse abnormal values of antioxidant biomarkers, such as GSH, SOD and CAT (Alshubaily et al., 2018).
Lagerstroemia speciosa (L.) Pers. belongs to the Lythraceae family (Al-Snafi, 2019). It is abundantly found in Asia’s tropical and subtropical regions, including India, Sri Lanka, Cambodia, Myanmar, Thailand, Vietnam, Indonesia, Malaysia and Philippines (Pal et al., 2020). L. speciosa has been associated with various medicinal properties, including anti-inflammatory and antioxidant effects against ROS in the liver (Tiwary et al., 2017). Major phytochemical compounds found in L. speciosa include palmitic acid (1.16%), octadecanoic acid (1.94%), 5-methyluridine (29.48%), catechine (2.41%), epigallocatechin (40.06%), and norgestrel (2.59%) (Pal et al., 2020). Studies have shown that L. speciosa provides liver protection against MSG-induced liver injury by lowering the serum transaminases (ALT and AST) and other liver biomarkers (ALP and GGT). Additionally, L. speciosa was able to restore the antioxidant enzymes, namely, GPx, GST, CAT and SOD. Histopathological analysis in the L. speciosa treated group also revealed reduced necrosis with decreased inflammation and improved hepatocyte architecture (Pal et al., 2020).
Lepidium sativum or garden cress, is a small perennial edible herb that belongs to the Brassicaceae family. This fast-growing plant originates from Egypt and Southwest Asia (Gupta and Gupta, 2024). Due to its unique mixture of sulforaphane and flavonol, L. sativum exerts antioxidant activity and serves as an effective free radical scavenger (Gupta and Gupta, 2024). It contains gallic acid, chlorogenic acid, catechin and pyrocatechol as its highest phenolic compounds (El-Gendy et al., 2023). In MSG-induced liver injury rats, administration of L. sativum for 30 days decreased MDA levels and increased SOD and CAT. Furthermore, L. sativum prevented elevations in transaminases enzymes and other liver biomarkers, such as ALP, total bilirubin, direct bilirubin and indirect bilirubin, while protecting the microscopic hepatic architecture from distortion (El-Gendy et al., 2023).
Linum usitatissimum (Linn.), commonly known as flaxseed or linseed, is widely distributed in West Asia and Mediterranean coastal lands and belongs to the family Linaceae (Badole et al., 2013). Flaxseed consists of polyunsaturated fatty acids such as oleic acid, linoleic acid, palmitic acid and stearic acid (Goyal et al., 2014), which have demonstrated various pharmacological effects, such as anticancer, anticonvulsant and antioxidant activities (Chera et al., 2022). Mushatet and Jawad (2020) demonstrated that a 30-day flaxseed treatment at 400 mg/kg prevented liver injury by restoring transaminase enzymes and ALP following MSG administration. Similarly, mixture of flaxseed oil and canola oil at different ratios (1:3, 1:1, and 3:1) to rat food given to MSG-treated rats for 30 days mitigated MSG-induced elevated transaminase enzymes activites and total bilirubin levels (Anwar and Mohamed, 2010).
Moringa oleifera (Moringaceae), also known as horseradish tree, native to India and Bangladesh, is widely cultivated worldwide, including in regions such as the Pacific Islands, the Caribbean, Latin America, and Asia (Islam et al., 2021). Various medicinal properties have been attributed to M. oleifera including anticancer, anti-inflammatory and antioxidant activities (Ramamurthy et al., 2021; Azlan et al., 2022; Abd Rashid et al., 2023). The aqueous extract of M. oleifera contains myricetin, quercetin, kaempferol, isorhamnetin, rutin, and phenolic acids (Niziol-Lukaszewska et al., 2020). Oral administration of M. oleifera at a dose of 200 mg/kg for 4 weeks reduced the detrimental effects of MSG in the liver, as evidenced by a reduction in transaminase enzymes, ALP and GGT. Furthermore, the activities of GST, SOD and CAT were also increased. Histologically, M. oleifera preserved hepatic architecture and attenuated the liver injury, exhibiting mild vacuolated hepatocytes and diffuse Kupffer cell proliferation (Albrahim and Binobead, 2018; El-Gharabawy et al., 2019).
Murraya koenigii, belonging to the botanical family Rutaceae, is commonly known as curry leaves (Balakrishnan et al., 2020). M. koenigii is widely distributed in tropical and subtropical regions. Its aqueous extract contains bioactive compounds such as mahanine, mahanimbine, koenimbine and koenigine. Additionally, M. koenigii exhibits high scavenging activity (74.16%), effectively converting free radicals into more stable products, which leads to the termination of free radical chain reactions (Shinde and Mohan, 2022). Consumption of M. koenigii aqueous extract in an MSG-induced liver injury rat model significantly restored abnormal activities of transaminase enzymes, ALP, total bilirubin and albumin. Histologically, M. koenigii aqueous extract exhibited mild cytoplasmic vacuolation, sinusoidal congestion and cellular aggregates around the portal area (Shinde and Mohan, 2022).
Myrtus communis L. (myrtle), from the Myrtaceae family, can grow spontaneously as a small tree. It is abundantly found in the coastal areas of the Mediterranean regions, such as North Africa and Southern Europe, as well as in South America, Australia, and the Himalayas (Giampieri et al., 2020). Traditionally, myrtle has been used to treat cough, gastrointestinal disorders, urinary diseases and skin ailments (Alipour et al., 2014). Previous research has proven that myrtle contains bioactive compounds such as polyphenols, flavonoids, anthocyanins, phenolic acids, lignans, tannins, organic acids, fatty acids and minerals, which contribute to its anti-inflammatory, anticancer and antimicrobial properties (Giampieri et al., 2020). El-Kholy et al. (2018) identified timolol and carvacrol as the major compounds in the aqueous extract of myrtle. Oral intake of myrtle demonstrated liver protection against MSG-induced liver injury, with treatment over 7 days significantly lowered serum transminases and ALP. Additionally, myrtle restored the antioxidant enzymes such as GSH, GPx, SOD and CAT (El-Kholy et al., 2018). Further study demonstrated that administration of myrtle extracts significantly improved cell viability by mitigating MSG-induced decrease in live cell percentage and increase in early and late apoptotic cell percentage (Hassan et al., 2020).
Newbouldia laevis, originating from Nigeria, belongs to the Bignoniaceae family. N. laevis has been traditionally used as medicine to treat malaria, diabetes and epilepsy (Ukwubile et al., 2023). The methanol extract of N. laevis contains chrysoeriol, quinones, 2-acetylfuro-1,4-naphathoquinone, 2-hydroxy-3 methoxy-9,10-dioxo-9,10-dihydroanthracene-1-carbaldehyde, lapachol, sterols, oleanolic acid, canthic acid and ceramide as major bioactive compounds (Kuete et al., 2007). After MSG administration, orally given N. laevis methanol extract for 14 days improved biochemical and histological parameters associated with liver injury. Biochemically, N. laevis reduced ALT, AST and ALP activities while increasing total protein and albumin activities. Histopathological findings revealed N. laevis improved liver architecture, with radially arranged hepatocytes, normal-sized nuclei and no observed histopathological lesions (Paul et al., 2020).
Nigella sativa, belonging to the Ranunculaceae family, is commonly known as black seed and grows in Eastern Europe, the Middle East, and Western Asia (Tavakkoli et al., 2017). N. sativa seeds have been extensively used in the treatment of various diseases worldwide. N. sativa exerts multiple medicinal activities, including anticancer, anti-inflammatory, antioxidant, hepatoprotective and renal protective properties (Al-Seeni et al., 2016; Bordoni et al., 2019; Hannan et al., 2021; Mehraj et al., 2022). Previous studies have shown that the major constituents of N. sativa seeds include 9,12-octadecadienoic acid, hexadecanoic acid, and thymoquinone (Abd-Elkareem et al., 2022). Oral administration of MSG and N. sativa seeds for 21 days improved biochemical and histological parameters associated with acute liver injury. Biochemically, N. sativa seeds reduced AST, ALP, total bilirubin, direct bilirubin and albumin activites. Histopathological findings showed that N. sativa seeds restored the normal arrangement of hepatic cords in parenchyma cells, with minimal congestion of hepatic sinusoids. Additionally, only a few degenerated necrotic hepatocytes and minor lymphoid cell infiltrations in the portal area were observed (Abd-Elkareem et al., 2022).
Opuntia ficus-indica, belonging to the Cactaceae family, originates from Mexico, Latin America, South Africa and Mediterranean countries. The flowers and fruits of O. ficus-indica are used to treat gastrointestinal tract diseases such as ulcers and diarrhea (El-Mostafa et al., 2014). O. ficus-indica contains a high polyphenol content, contributing to its antioxidant and anti-inflammatory properties (Kuti, 2004). Simultaneous treatment with O. ficus-indica fruit juice and MSG provided significant protection against liver injury. Treatment with O. ficus-indica fruit juice reduced the body weight of the mother rat and their offspring, likely due to its anti-inflammatory properties, which mitigated MSG-induced inflammation associated with increased body weight (Ghanem et al., 2023). Additionally, O. ficus-indica treatment reduced serum transaminases, total bilirubin and LDH levels. It also reduced MDA levels and caspase 3 activities, while increasing SOD and CAT activities. Histological analysis was found parallel to be with biochemical results, showing that O. ficus-indica markedly improved liver architecture (Ghanem et al., 2023).
Phoenix dactilefera L., commonly known as Ajwa date palm tree, is one of the oldest and primary staple crops in Southwest Asia and North Africa (Al-Alawi et al., 2017). Studies have shown that Ajwa date variety is an ancient herbal remedy with antioxidant, anti-inflammatory, anti-mutagenic and anticancer medicinal properties (Khalid et al., 2017). Ajwa dates contain dodeca-1,6-dien-12-ol, 6,10 dimethyl; 13-tetradece-11-yn-1-ol; 12-methyl-E,E-2,13-octadecadien1-ol and 1-(cyclopropyl-nitro-methyl)-cyclopentanol as major compounds (Eldeen et al., 2022). Oral administration of Ajwa dates improved liver functions by reducing AST and ALT to near normal values. Ajwa dates also mitigated the elevation of SOD and CAT while increasing MDA levels. Additionally, administration of Ajwa dates in MSG-induced liver injury led to a normal arrangement of hepatic cords with normal-sized hepatocytes (Shamim et al., 2020).
Rhodiola rosea, belonging to the Crassulaceae family, is commonly known as roseroot, goldenroot or articroot. It can be found in the mountainous and arctic areas in Asia, Europe and North America (Durazzo et al., 2022). Previous studies identified rosavins (phenylpropanoids), salidroside (phenylethanoid derivatives), flavonoids, rosiridol (monoterpene), daucosterol (triterpenes), gallic acid (phenolic acid) as the major bioactive compounds in R. rosea (Ivanova Stojcheva and Quintela, 2022). Oral administration of MSG and R. rosea for 10 days improved biochemical and histological parameters associated with liver injury. Biochemically, R. rosea reduced AST, ALT and GGT activities. Additionally, R. rosea restored antioxidant properties, such as GSH, CAT, SOD and MDA in MSG-induced liver injury. Histopathological findings showed that R. rosea resulted in moderate congestion of the liver’s central vein, intact hepatocytes and mild dilation of blood sinusoids in MSG-induced liver injury (Soliman et al., 2023).
Millet or guinea corn is derived from the leaves of S. bicolor L. Moench. Due to its composition of phenolic compounds (e.g., phenolic acid, flavonoids, stilbenes, and tannins), vitamins (e.g., B-complex, A, D, E, and K), and minerals (e.g., potassium, phosphorus, magnesium, and zinc), S. bicolor exhibits anti-inflammatory, antioxidant, anti-colon cancer, and immune modulator functions (Vanamala et al., 2018; Khalid et al., 2022). When administered for 14 days in an MSG-induced liver injury model, Jobelyn® (JB, commercialized millet) significantly decreased MDA and GSH levels, and increased GST, CAT and SOD activities. Furthermore, JB prevented elevations in AST, ALT and ALP activities and protected the hepatic microscopic architecture from distortion (Omogbiya et al., 2021).
Solanum melongena, commonly known as eggplant, belongs to the Solanaceae family and originates from Asian countries, the Middle East, and the Mediterranean basin (Cericola et al., 2013). It has been traditionally used to treat asthma, bronchitis, diabetes, arthritis and hypercholesterolemia (Yarmohammadi et al., 2021). When co-administered with MSG, eggplant extract provided enhanced protection against liver injury, increasing antioxidant markers such as GSH, CAT and SOD, while lowering MDA, a biomarker for lipid peroxidation (Mbah and Egbuonu, 2017).
Solanum torvum, also known as Turkey berry, is from the family of Solanaceae. Turkey berries methanolic extract contain alkaloids, flavonoids, tannins, saponins, solasonine, solamargine, torvanol A and torvoside H, which exhibit anti-inflammatory, antibacterial, antifungal and antidiabetic effects (Darkwah et al., 2020; Govender et al., 2022). Turkey berry is reported to have higher total phenolic content, compared to its other close relatives like S. melongena and S. ferrugineum (Weremfo et al., 2022). In MSG-induced liver injury, turkey berry reduced body weight and liver weight relative to body weight, attributed to its anti-inflammatory properties (Ogwu et al., 2023). Turkey berry extract significantly restored abnormal activities of transaminase enzyme, ALP, total bilirubin, total protein and albumin. Histologically, turkey berry extract exhibited mild centrilobular cytoplasmic vacuolation, sinusoidal congestion, nuclear pyknosis, and cellular aggregates of lymphocytes and macrophages around the portal area, while maintaining normal liver architecture (Kadam et al., 2019).
Uncaria gambir Roxb., commonly known as gambir, is a plant native to Southeast Asia and is widely used in alternative medicine with various applications. Gambir contains secondary metabolites such as alkaloids, tannins, saponins and catechins, which are attributed to its antioxidants, anti-inflammatory, anticancer and antimicrobial properties (Pane et al., 2021; Munggari et al., 2022). Oral intake of gambir for 4 weeks exhibited liver protection against MSG-induced liver injury, with only minimal mononuclear aggregates observed in some portal tracts (Pane et al., 2021).
Zingiber officinale, or ginger, belongs to the Zingiberaceae family, contains high levels of bioactive compounds, particularly phenolic and terpene compounds. Adesola et al. (2021) demonstrated that the aqueous extract of Z. officinale contains alkaloids, saponins, steroids and cardiac glycoside. These bioactive compounds contribute to ginger’s high antioxidant, anti-inflammatory, antimicrobial, anticancer and neuroprotective properties (Mao et al., 2019; Adesola et al., 2021). Ginger also exhibited liver protection against MSG-induced liver injury by decreasing AST, ALT and albumin activities. Additionally, ginger was found to restore hepatocytes of nearly normal hepatic lobular architecture, with only slightly dilated and congested central veins, blood sinusoids, and few cellular infiltration (Mustafa and Qader, 2016; Abd El Hady Mousa et al., 2021).
Phenolic compounds or polyphenols are secondary metabolites present in most plant tissues, including fruits, vegetables and other plant food sources (de la Rosa et al., 2019). Phenolic compounds are mainly localized in cells vacuoles, chloroplasts and nuclei (Khlestkina, 2013). They are synthesized through the phenylpropanoid pathway and possess an aromatic ring with one or more hydroxyl groups derived from the aromatic amino acid phenylalanine (Tura and Robards, 2002). Phenolic compounds are involved in chemical interactions between organisms, acting as reducing agents, hydrogen donors and singlet oxygen quenchers (Tura and Robards, 2002; Fattahi et al., 2014), which promote various biological activities, such as antioxidant, anti-inflammatory, antibacterial, antifungal and antihepatotoxicity effects.
Tannic acid (TA) is a natural tannin from the polyphenolic group that can be efficiently isolated from herbaceous and woody plants (Kaczmarek, 2020). A previous study revealed the protective effect of TA in MSG-toxified rat liver (Mohamed et al., 2021). Rats orally administered with 100 mg/kg TA were compared with rats that received MSG (2 g/kg) and those received both TA and MSG. The administration of the designated treatments to all rats for 4 weeks. Biomarkers of liver function (ALT and AST) indicated a significant reduction in serum activities in the TA + MSG-treated groups compared to the MSG-treated group, which had increased activities relative to the TA-treated group. Histopathological examination also showed significant improvement in the hepatic architecture of the TA + MSG-treated rats, with nearly normal hepatocytes, milder vacuolated cytoplasm and less prominent Kupffer cells, along with normal blood sinusoids. In contrast, MSG-treated rats showed severe hepatic tissue damage, such as congestion and dilatation of blood vessels (Mohamed et al., 2021). Interestingly, treatment with TA attenuated MSG-induced upregulation of Aurora kinase A (Aurka) and Cyclin B2 (Ccnb2), and downregulation of Coagulation Factor IX (F9) and Cytochrome P450 2E1 (Cyp2e1) gene expressions, in which these genes are hepatocellular carcinoma-associated key genes (Tosun et al., 2024).
In another study, apocyanin (4-hydroxy-3-methoxyacetophenone) (APO), isolated from Apocynum cannabinum roots, was investigated for its healing effects against MSG-induced liver damage in Sprague-Dawley rats. Four groups of rats (APO-treated, MSG-treated, APO + MSG-treated, control) were examined for histopathological, ultrastructural and biochemical analyses. APO administration significantly improved histopathological damage in MSG-induced liver injury, with decreased vacuolated hepatocytes, increased glycogen distribution and normal connective tissue distribution. The APO + MSG-treated group showed lower activities of ALT, AST, ALP, total bilirubin, MDA and MPO, along with higher albumin, GSH levels, and SOD activities, indicating APO’s prominent ameliorating effect on MSG-induced liver damage (Sahin et al., 2023).
Lycopene (LYC), another sub-polyphenolic, has been reported to have hepatoprotective effects against MSG-induced hepatic toxicity in rats at different doses (15 and 35 mg/kg/day)(Elwan et al., 2021). LYC supplementation (10 mg/kg/day) before MSG administration for 10 days and co-administration with MSG for 30 days partially restored hepatic enzymes. At lower MSG toxicity (induced with 15 mg/kg/day), portal tracts showed only congested vessels and the hepatocytes exhibited mild hydropic degeneration. In contrast, LYC-treated rats at higher MSG toxicity (induced with 35 mg/kg/day), there was congestion in the central veins, and most portal tracts were mildly expanded with fibrous tissue, chronic inflammation and bile ductuli proliferation. LYC-supplemented rats at both MSG doses also exhibited promising cytoprotective activities, as evidenced by reduced ALT and AST activities, increased SOD activities and GSH levels, and improved GPx activities, along with decreased MDA and growth factor beta1 (TGF-β1) levels (Elwan et al., 2021).
The ameliorating effects of vitamin C and propolis on MSG-induced liver dysfunction in rats were also documented. Diab and Hamza (2016) identified that supplementation of combined vitamin C and propolis significantly reduced liver function biomarkers (ALT, AST and LDH) compared to individual treatment. Similarly, liver homogenates antioxidant parameters showed decreased MDA levels and increased CAT, SOD and GPx activities. Additional studies on propolis alone at doses of 100 mg/kg (El-Alfy et al., 2020) and 90 mg/kg (Ibrahim et al., 2019) demonstrated protection against MSG-induced liver toxicity in mice and rats. Propolis treatment improved liver architecture and mitigated tissue degeneration in MSG-induced liver injury, consistent with previous findings (Diab and Hamza, 2016).
Flavonoids are a subclass of phenolic compounds that act as naturally occurring antioxidants in various diseases (Hor et al., 2019). There are six major classes of flavonoids consisting of flavanol, flavone, flavanone, flavanol (catechins), isoflavone, and anthocyanidin, each differing in molecular structure. The individual structure of these classes, their sub-compounds, and their food sources are well documented (Anand David et al., 2016).
Quercetin (QU) belongs to the flavanol class and is found in various foods, including apples, cherries, grapes, onion peel, stink bean, tea, wine, etc (Adeyemi et al., 2020; Siti et al., 2022). QU has been reported to exhibit strong antioxidant effects through free radical scavenging activity, inhibition of lipid peroxidation, metal ion chelation, and modulation of cellular antioxidant responses (Xu et al., 2019). The potential of QU to modulate liver function and reduce oxidative stress induced by MSG was investigated in a previous study (Ahmed et al., 2019). Albino rats administered MSG and supplemented with QU at 14 mg/kg for 30 days showed an alleviation of hepatic lesions, with central veins returning to a normal structure, and both hepatocytes and sinusoids appearing similar to those in the control group (Ahmed et al., 2019). Biochemical analysis further supported the ameliorating effect, showing decreased activities of AST, ALT, lipid peroxidation and GSH, along with increased GPx and SOD activities (Youssef et al., 2023).
Rutin is another potent antioxidant flavonoid that is present in buckwheat, tomato leaves, apples, tea, passion flower, spinach and onions (Hosseinzadeh and Nassiri-Asl, 2014). The hepatoprotective effects of rutin have been shown in several studies (Abdel-Ghaffar et al., 2017; Rakshit et al., 2021), which can be attributed to its ability to increase antioxidant enzyme activity (Samir et al., 2019). The protective role of rutin was evaluated by supplementing different doses of rutin (25 and 50 mg/kg) in rats induced with MSG. Results showed that rutin administration at both low and high doses significantly decreased MDA level and enhanced antioxidant activity in the tested rats (Samir et al., 2019).
Various types of plant provide phytochemicals, which show numerous biological activities such as antiulcerogenic, antioxidant, anticancer, antimicrobial, anti-inflammatory, and hepatoprotective properties. Among the plant parts employed for ethnopharmacological use, leaves were highly utilized, followed by whole plants, roots, fruits, seeds, bark, rhizomes, and flowers. In this review article, the most commonly used form of extraction was alcoholic (ethanolic and methanolic), followed by aqueous, petroleum ether and ethyl acetate. Through a variety of cellular and molecular mechanisms, phenolic compound and flavonoids such as tannic acids, lycopene, apocyanin, quercetin, rutin and other plant extracts exhibit exceptional preventive and therapeutic actions against MSG-induced liver injury. These processes include ameliorating cellular responses to oxidative stress, such as the production of SOD, CAT, GSH, GPx, and GR, as well as decreasing proinflammatory cytokines and lipid peroxidation products. These plant products work as a free radical scavenger to inhibit the effects of various ROS. Additionally, the mentioned plant products above can reduce blood lipid levels, enhance liver function, and preserve liver architecture. Figure 3 provides an overview of the mechanism of action of plant products against MSG-induced liver injury.
Figure 3. Overview mechanism of action of food supplement from plants against MSG-induced liver injury.
The Food and Drug Administration (FDA) has exempted herbal and dietary supplements from detailed review, as they are considered low-risk products (Alostad et al., 2018; Pauzi et al., 2022). For example, administrating Cistus ladaniferus aqueous extract orally at doses of 500, 700 and 1,000 mg/kg body weight daily to Wistar rats for 90 days showed no mortality or treatment-related adverse effects in terms of body weight, general behavior, relative organ weights, and urine, hematological and biochemical parameters (El Kabbaoui et al., 2017). However, safety concerns about herbal product use need to be addressed, especially for prolonged or high dose uses. Currently, there is a lack of safety assessment regarding the long-term effects of herbal products on liver health and overall metabolism. Additionally, certain plant products may interact with prescribed medications, potentially leading to unforeseen side effects. For example, garlic and ginseng may interact with warfarin, increasing the risk of post-operative bleeding, while primrose oil may induce undiagnosed epilepsy, particularly in patients undergoing drug therapy for epilepsy (Palaian et al., 2007). Therefore, rigorous safety evaluations are essential to address the potential adverse effects and toxicity of plant products, which is crucial for patient safety.
Another limitation is the lack of standardization in herbal products, which can result in inconsistencies in the concentration and potency of bioactive compounds. Variability in growing conditions, initial processing and storage of plant materials, different extraction methods, storage conditions, and contamination with exogenous substances (e.g., pesticides and heavy metals) can significantly impact the composition of herbal products, affecting their therapeutic potential (Heinrich et al., 2022; Amirah et al., 2023; Yadav et al., 2024). For instance, the polyphenolic content in three Mediterranean wild species: Phyllirea latifolia, Cistus incanus and Pistacia lentiscus, showed seasonal (monthly) and diurnal (time-of-day) variations (Gori et al., 2020). Additionally, the antioxidant activity of herbs and spice extracts, measured by three methods (DPPH, ABTS and FRAP), varies depending on the time of extraction (30 min or 24 h) and solvent used (water, 50% ethanol in water or ethanol) (Muzolf-Panek and Stuper-Szablewska, 2021). To achieve standardized herbal products, plant authentication may require DNA barcoding alongside botanical and chemical tests. In addition to using chromatographic or spectroscopic techniques to generate chemical fingerprints of plant extracts, DNA-based methods can provide genomic information, adding valuable tools for quality control (Klein-Junior et al., 2021).
The bioavailability and metabolism of plant products in the human body present another challenge. Many bioactive compounds found in plants, such as polyphenols and flavonoids, have low bioavailability due to rapid metabolism or poor absorption attributed to their low absorption in the gastrointestinal tract. For instance, only 1%–2% of anthocyanins retain their structure after ingestion (Tena et al., 2020). This limitation means that the doses effective in animal studies may not yield comparable results in humans, unless the compounds are chemically modified or paired with bioenhancers. Without overcoming these bioavailability issues, the efficacy of plant-based supplements could be diminished, even if their protective effects are evident in laboratory settings. Advanced delivery systems, such as encapsulation technologies (e.g., biopolymer nanoparticles, emulsions, liposomes, solid lipid nanoparticles, and nanostructure lipid carriers), have improved the stability, bioavailability and bioactivity of phytochemicals (Yang et al., 2020; Woon et al., 2022). For example, encapsulation of curcumin into a novel natural turmeric matrix increased its absorption, with blood levels 6 times higher than commercially available curcumin with volatile oil formulations, and 5 times higher than curcumin with phospholipids (Gopi et al., 2017).
Lastly, the lack of robust clinical data limits the establishment of clear dosage guidelines and treatment protocols. The majority of the studies on plant products for MSG-induced liver injury focus on animal models, making it difficult to determine the appropriate dosage, frequency, and duration of treatment, which can hinder the integration of these supplements into standardized healthcare practices. To address this gap, future research should focus on well-designed clinical trials to assess the efficacy and safety of plant-based supplements in human populations.
The mechanism of MSG-induced liver injury is markedly demonstrated through histopathological analysis, liver function profiles and oxidative stress (antioxidant status). In this review, we found that MSG dose ranging from 5 mg/kg up to 400 g/kg able to produce liver damage with prolonged excessive intake. Some of the studies also used extremely high dose of MSG which is 300–400 g/kg of MSG, which is rarely to be taken by human at any equivalent dose. Plant products offer a significant advantage as supplementary therapeutic agents against MSG-induced liver injury. Our review concludes that various plant products provide protection against MSG-induced liver injury, as indicated by promising preclinical findings. However, the clinical applications of these plant products, which restrict their potential use for the treatment and management of MSG-induced liver injury. Further research is warranted to explore the underlying mechanism of each plant product listed to mitigate MSG-induced liver injury. Additionally, researchers should investigate the bioactive compounds in each plant extract. This is necessary to establish a relationship between the antioxidant properties of each bioactive compound and the protective effects of the plant.
DK: Conceptualization, Investigation, Project administration, Writing–original draft, Writing–review and editing. EA: Conceptualization, Investigation, Project administration, Writing–original draft, Writing–review and editing. ZH: Writing–original draft, Writing–review and editing. FS: Writing–original draft, Writing–review and editing. NA: Conceptualization, Investigation, Project administration, Writing–original draft, Writing–review and editing. ST: Writing–original draft, Writing–review and editing.
The author(s) declare that no financial support was received for the research, authorship, and/or publication of this article.
The authors declare that the research was conducted in the absence of any commercial or financial relationships that could be construed as a potential conflict of interest.
The author(s) declared that they were an editorial board member of Frontiers, at the time of submission. This had no impact on the peer review process and the final decision.
The authors declare that Gen AI was used in the creation of this manuscript. During the preparation of this work the authors used the Chat Generative Pre-trained Transformer (ChatGPT) only to correct grammar in certain parts of the work. After using this tool/service, the authors reviewed and edited the content as needed and take full responsibility for the content of the publication.
All claims expressed in this article are solely those of the authors and do not necessarily represent those of their affiliated organizations, or those of the publisher, the editors and the reviewers. Any product that may be evaluated in this article, or claim that may be made by its manufacturer, is not guaranteed or endorsed by the publisher.
Abbas, M. F., and Abbas, A. H. (2016). Hepatotoxicity induced by monosodium glutamatee (MSG) in rats andd the possible hepatoprotective role of n-acetylceisteine. Egypt. J. Forensic Sci. Appl. Toxicol. 16 (1), 159–178. doi:10.21608/ejfsat.2016.39959
Abdel-Ghaffar, O., Mahmoud, S. T., Said, A. A., and Sanad, FAAY (2017). Hepatoprotective effect of rutin against oxidative stress of isoniazid in albino rats. Int. J. Pharmacol. 13 (6), 516–528. doi:10.3923/ijp.2017.516.528
Abd El Hady Mousa, M., Mansour, H., Eid, F., and Mashaal, A. (2021). Anti-inflammatory activity of ginger modulates macrophage activation against the inflammatory pathway of monosodium glutamate. J. Food Biochem. doi:10.1111/jfbc.13819
Abd-Elkareem, M., Soliman, M., Abd El-Rahman, M. A. M., and Abou Khalil, N. S. (2022). The protective effect of Nigella sativa seeds against monosodium glutamate-induced hepatic dysfunction in rats. Toxicol. Rep. 9, 147–153. doi:10.1016/j.toxrep.2022.01.014
Abd Rashid, N., Mohamad Najib, N. H., Abdul Jalil, N. A., and Teoh, S. L. (2023). Essential oils in cervical cancer: narrative review on current insights and future prospects. Antioxidants 12 (12), 2109. doi:10.3390/antiox12122109
Abdullah, E., Amirullah, N. A., Vijayan, H., Abd Rashid, N., Abdullah, N., and Zainal Abidin, N. (2024). Chemopreventive role of proteins and polysaccharides from Pleurotus tuber-regium. Food Biosci. 60, 104311. doi:10.1016/j.fbio.2024.104311
Adesola, R. O., Ogbole, E., Itodo, A. E., Salami, O., and Abdulazeez, M. D. (2021). Aqueous extracts of ginger (Zingiber officinale Roscoe) and garlic (Allium satium L.) bulbs: phytochemical screening and in vivo antitrypanosomal effect. World News Nat. Sci. 37, 135–150.
Adeyemi, O. S., Ebugosi, C., Akpor, O. B., Hetta, H. F., Al-Rashed, S., Otohinoyi, D. A., et al. (2020). Quercetin caused redox homeostasis imbalance and activated the kynurenine pathway (running title: quercetin caused oxidative stress). Biology 9 (8), 219. doi:10.3390/biology9080219
Ahmed, R. R., Abdul-Hamid, M., Galaly, S. R., and Hamdalla, H. M. (2019). Monosodium glutamate-induced liver microscopic and biochemical changes in male rats, and the possible amendment of quercetin. Egypt. J. Zoology 71 (71), 44–55. doi:10.21608/ejz.2019.37158
Al-Alawi, R. A., Al-Mashiqri, J. H., Al-Nadabi, J. S. M., Al-Shihi, B. I., and Baqi, Y. (2017). Date palm tree (Phoenix dactylifera l.): natural products and therapeutic options. Front. Plant Sci. 8, 845. doi:10.3389/fpls.2017.00845
Albrahim, T., and Binobead, M. A. (2018). Roles of Moringa oleifera leaf extract in improving the impact of high dietary intake of monosodium glutamate-induced liver toxicity, oxidative stress, genotoxicity, DNA damage, and PCNA alterations in male rats. Oxidative Med. Cell. Longev. 2018, 4501097. doi:10.1155/2018/4501097
Alemu, T. T. (2022). Effect of food additives on the food quality and safety: a review. Int. J. Diabetes and Metabolic Disord. 7 (2), 229–237. doi:10.33140/ijdmd.07.02.06
Alipour, G., Dashti, S., and Hosseinzadeh, H. (2014). Review of pharmacological effects of Myrtus communis L. and its active constituents. Phytotherapy Res. 28 (8), 1125–1136. doi:10.1002/ptr.5122
Alostad, A. H., Steinke, D. T., and Schafheutle, E. I. (2018). International comparison of five herbal medicine registration systems to inform regulation development: United Kingdom, Germany, United States of America, United Arab Emirates and kingdom of Bahrain. Pharm. Med. 32 (1), 39–49. doi:10.1007/s40290-018-0223-0
Al-Salmi, F. A., Hamza, R. Z., and El-Shenawy, N. S. (2019). The interaction of zinc oxide/green tea extract complex nanoparticles and its effect on monosodium glutamate toxicity in liver of rats. Curr. Pharm. Biotechnol. 20 (6), 465–475. doi:10.2174/1389201020666190408120532
Al-Seeni, M. N., El Rabey, H. A., Zamzami, M. A., and Alnefayee, A. M. (2016). The hepatoprotective activity of olive oil and Nigella sativa oil against CCl4 induced hepatotoxicity in male rats. BMC Complementary Altern. Med. 16 (1), 438. doi:10.1186/s12906-016-1422-4
Alshubaily, F. A., Jambi, E. J., Khojah, S. M., Balgoon, M. J., and Alzahrani, M. H. (2018). Prospective capability of grape seed oil in face with the inverse influence of monosodium glutamate on liver and kidneys tasks. Aust. J. Basic Appl. Sci. 12 (6), 48–52. doi:10.22587/ajbas.2018.12.6.9
Al-Snafi, A. E. (2019). Medicinal value of Lagerstroemia speciosa: an updated review. Int. J. Curr. Pharm. Res. 11 (5), 18–26. doi:10.22159/ijcpr.2019v11i5.35708
Amirah, S. S., Khairil, M., Murdiono, W. E., Halmi, M. I. E., Amalina, N. R., Yong, J. W. H., et al. (2023). Edaphic influences on the nutrient concentrations and antioxidant activity of different tea clones (Camellia sinensis (O.) Kuntze) grown at the lowland tea plantation, Bukit Cheeding, Selangor, Malaysia. Malays. J. Soil Sci. 27, 147–163.
Anand David, A. V., Arulmoli, R., and Parasuraman, S. (2016). Overviews of biological importance of quercetin: a bioactive flavonoid. Pharmacogn. Rev. 10 (20), 84–89. doi:10.4103/0973-7847.194044
Anwar, M. M., and Mohamed, N. E. (2010). Impact of flax seed and canola oils mixture supplementation on the physiological and biochemical changes induced by monosodium glutamate in rats. J. Radiat. Res. Appl. Sci. 3 (3B), 943–964.
Asejeje, F. O., Gabriel, G. O., and Abiola, M. A. (2023). Monosodium glutamate aggravates lipopolysaccharide-induced liver injury via inflammation and oxidative stress in rats. Nutrire 48 (1), 5. doi:10.1186/s41110-023-00188-w
Azlan, U. K., Khairul Annuar, N. A., Mediani, A., Aizat, W. M., Damanhuri, H. A., Tong, X., et al. (2022). An insight into the neuroprotective and anti-neuroinflammatory effects and mechanisms of Moringa oleifera. Front. Pharmacol. 13, 1035220. doi:10.3389/fphar.2022.1035220
Badole, S. L., Zanwar, A. A., and Bodhankar, S. L. (2013). “Antihyperglycemic potential of secoisolaricinol diglucoside,” in Bioactive food as dietary interventions for diabetes. Editors R. R. Watson, and V. R. Preedy (Boston: Academic Press), 53–57. doi:10.1016/B978-0-12-397153-1.00005-6
Balakrishnan, R., Vijayraja, D., Jo, S. H., Ganesan, P., Su-Kim, I., and Choi, D. K. (2020). Medicinal profile, phytochemistry, and pharmacological activities of Murraya koenigii and its primary bioactive compounds. Antioxidants 9 (2), 101. doi:10.3390/antiox9020101
Banerjee, A., Mukherjee, S., and Maji, B. K. (2021). Monosodium glutamate causes hepato-cardiac derangement in male rats. Hum. and Exp. Toxicol. 40 (12_Suppl. l), S359–S369. doi:10.1177/09603271211049550
Bera, T. K., Kar, S. K., Yadav, P. K., Mukherjee, P., Yadav, S., and Joshi, B. (2017). Effects of monosodium glutamate on human health: a systematic review. World J. Pharm. Sci. 5 (5), 78–202.
Bordoni, L., Fedeli, D., Nasuti, C., Maggi, F., Papa, F., Wabitsch, M., et al. (2019). Antioxidant and anti-inflammatory properties of Nigella sativa oil in human pre-adipocytes. Antioxidants 8 (2), 51. doi:10.3390/antiox8020051
Cericola, F., Portis, E., Toppino, L., Barchi, L., Acciarri, N., Ciriaci, T., et al. (2013). The population structure and diversity of eggplant from Asia and the Mediterranean Basin. PLoS One 8 (9), e73702. doi:10.1371/journal.pone.0073702
Chakraborty, S. P. (2019). Patho-physiological and toxicological aspects of monosodium glutamate. Toxicol. Mech. Methods 29 (6), 389–396. doi:10.1080/15376516.2018.1528649
Chamma, H., Jebai, F., Abdel Sater, F., Ezzedine, M., Mortada, M., Kchour, G., et al. (2018). Taste mapping: a new approach for the taste regions. Asian J. Sci. Technol. 9 (9), 8710–8713.
Chaudhari, N., Pereira, E., and Roper, S. D. (2009). Taste receptors for umami: the case for multiple receptors. Am. J. Clin. Nutr. 90 (3), 738S–742S. doi:10.3945/ajcn.2009.27462H
Chera, E. I., Pop, R. M., Parvu, M., Soritau, O., Uifalean, A., Catoi, F. A., et al. (2022). Flaxseed ethanol extracts’ antitumor, antioxidant, and anti-inflammatory potential. Antioxidants 11 (5), 892. doi:10.3390/antiox11050892
Darkwah, W. K., Koomson, D. A., Miwornunyuie, N., Nkoom, M., and Puplampu, J. B. (2020). Review: phytochemistry and medicinal properties of Solanum torvum fruits. All Life 13 (1), 498–506. doi:10.1080/26895293.2020.1817799
de la Rosa, L. A., Moreno-Escamilla, J. O., Rodrigo-García, J., and Alvarez-Parrilla, E. (2019). “Phenolic compounds,” in Postharvest physiology and biochemistry of fruits and vegetables. Editor E. M. Yahia (Sawston, United Kingdom: Woodhead Publishing), 253–271. doi:10.1016/B978-0-12-813278-4.00012-9.Duxford
Diab, A., and Hamza, R. (2016). Monosodium glutamate induced hepatotoxicity and the possible mitigating effect of vitamin C and propolis. J. Adv. Med. Pharm. Sci. 7 (4), 1–10. doi:10.9734/JAMPS/2016/24196
Durazzo, A., Lucarini, M., Nazhand, A., Coêlho, A. G., Souto, E. B., Arcanjo, D. D. R., et al. (2022). Rhodiola rosea: main features and its beneficial properties. Rendiconti Lincei Sci. Fis. Nat. 33, 71–82. doi:10.1007/s12210-022-01055-y
Eid, R. A., Al-Shraim, M., Zaki, M. S., Kamar, S. S., Abdel Latif, N. S., Negm, S., et al. (2019). Vitamin E protects against monosodium glutamate-induced acute liver injury and hepatocyte ultrastructural alterations in rats. Ultrastruct. Pathol. 43 (4-5), 199–208. doi:10.1080/01913123.2019.1673860
El-Alfy, N. Z., Mahmoud, M. F., Eissa, M. I., and Emam, A. A. (2020). Role of propolis against toxic effects of monosodium glutamate on histology and expression lipid metabolism genes of mice liver. Indian J. Public Health Res. and Dev. 11 (5), 867–873. doi:10.37506/ijphrd.v11i5.9548
El-Badawy, M., Youssef, H., Hafez, Y., El-Sanafawy, H., El-Maghraby, M., and El-Giziry, A. (2018). Effect of rocket oil addition on productive and reproductive performance of growing ram lambs under hot climate condition. J. Animal Poult. Prod. 9 (1), 9–15. doi:10.21608/jappmu.2018.35742
Elbessoumy, A. M., Ghareeb, D. A., and Khalil, A. A. (2010). Toxic effects of monosodium glutamate. on the hepatic tissue damage of rats and the beneficial role of garlic extract: involvement of oxidative stress, hyperlipidemia and hyperglycemia. J. Biochem. Biotechnol. 1 (1), 19–25.
Eldeen, RSEF, ElNaggar, S. A., El-Said, K. S., Elwan, M., and Sarhan, F. W. (2022). Date (Phoenix dactylifera L.) seeds extract mitigates the hepato-renal toxicities induced by monosodium glutamate in male albino mice. J. Fac. Specif. Educ. 8 (42), 1507–1531. doi:10.21608/jedu.2022.170217.1766
El-Gendy, M. S., El-Gezawy, E. S., Saleh, A. A., Alhotan, R. A., Al-Badwi, M. A. A., Hussein, E. O. S., et al. (2023). Investigating the chemical composition of Lepidium sativum seeds and their ability to safeguard against monosodium glutamate-induced hepatic dysfunction. Foods 12 (22), 4129. doi:10.3390/foods12224129
El-Gharabawy, R. M., Ahmed, A. S., and Al-Adhadh, T. I. (2019). Ameliorating effect of Moringa against liver and kidney injury induced by monosodium glutamate. Annu. Res. and Rev. Biol. 33 (3), 1–10. doi:10.9734/arrb/2019/v33i330124
El Kabbaoui, M., Chda, A., El-Akhal, J., Azdad, O., Mejrhit, N., Aarab, L., et al. (2017). Acute and sub-chronic toxicity studies of the aqueous extract from leaves of Cistus ladaniferus L. in mice and rats. J. Ethnopharmacol. 209, 147–156. doi:10.1016/j.jep.2017.07.029
El-Kholy, W. M., El-Sawi, M. R. F., and Galal, N. A. (2018). Effect of Myrtus communis extract against hepatotoxicity induced by monosodium glutamate and acrylamide in male rats. Egypt. J. Hosp. Med. 70 (9), 1576–1581. doi:10.12816/0044686
El-Mostafa, K., El Kharrassi, Y., Badreddine, A., Andreoletti, P., Vamecq, J., El Kebbaj, M. S., et al. (2014). Nopal cactus (Opuntia ficus-indica) as a source of bioactive compounds for nutrition, health and disease. Molecules 19 (9), 14879–14901. doi:10.3390/molecules190914879
Elwan, S., Bahr, H., Hassan, A., El Ghannam, A. E. R., and Mahmoud, Y. (2021). Anti-oxidant and hepatoprotective possibilities of lycopene against monosodium glutamate induced detrimental effects in rats. Suez Canal Veterinary Med. J. 26 (2), 341–354. doi:10.21608/scvmj.2021.217936
Ershad, B. (2020). Potential of green tea (Camellia sinensis L.) in improving the function of the liver in mice induced by monosodium glutamate (MSG). Int. J. Sci. Soc. 2 (4), 56–62. doi:10.54783/ijsoc.v2i4.192
Fattahi, S., Zabihi, E., Abedian, Z., Pourbagher, R., Motevalizadeh Ardekani, A., Mostafazadeh, A., et al. (2014). Total phenolic and flavonoid contents of aqueous extract of stinging nettle and in vitro antiproliferative effect on Hela and BT-474 cell lines. Int. J. Mol. Cell. Med. 3 (2), 102–107.
Garavaglia, J., Markoski, M. M., Oliveira, A., and Marcadenti, A. (2016). Grape seed oil compounds: biological and chemical actions for health. Nutr. Metabolic Insights 9, 59–64. doi:10.4137/NMI.S32910
Ghanem, R., El-beltagy, A., Kamel, K., Brakat, E., and Elsayyad, H. (2023). Adverse effects of MSG and aspartame on the liver of female albino rats and their offspring and the possible ameliorative role Opuntia ficus-indica fruit. Delta Univ. Sci. J. 6 (2), 1–32. doi:10.21608/dusj.2023.318628
Giampieri, F., Cianciosi, D., and Forbes-Hernández, T. Y. (2020). Myrtle (Myrtus communis L.) berries, seeds, leaves, and essential oils: new undiscovered sources of natural compounds with promising health benefits. Food Front. 1 (3), 276–295. doi:10.1002/fft2.37
Gopi, S., Jacob, J., Varma, K., Jude, S., Amalraj, A., Arundhathy, C. A., et al. (2017). Comparative oral absorption of curcumin in a natural turmeric matrix with two other curcumin formulations: an open-label parallel-arm study. Phytotherapy Res. 31 (12), 1883–1891. doi:10.1002/ptr.5931
Gori, A., Nascimento, L. B., Ferrini, F., Centritto, M., and Brunetti, C. (2020). Seasonal and diurnal variation in leaf phenolics of three medicinal mediterranean wild species: what is the best harvesting moment to obtain the richest and the most antioxidant extracts? Molecules 25 (4), 956. doi:10.3390/molecules25040956
Govender, N., Zulkifli, N. S., Badrul Hisham, N. F., Ab Ghani, N. S., and Mohamed-Hussein, Z. A. (2022). Pea eggplant (Solanum torvum Swartz) is a source of plant food polyphenols with SARS-CoV inhibiting potential. PeerJ 10, e14168. doi:10.7717/peerj.14168
Goyal, A., Sharma, V., Upadhyay, N., Gill, S., and Sihag, M. (2014). Flax and flaxseed oil: an ancient medicine and modern functional food. J. Food Sci. Technol. 51 (9), 1633–1653. doi:10.1007/s13197-013-1247-9
Green, B. G., and Nachtigal, D. (2012). Somatosensory factors in taste perception: effects of active tasting and solution temperature. Physiology and Behav. 107 (4), 488–495. doi:10.1016/j.physbeh.2012.05.010
Gupta, S., and Gupta, R. (2024). Research update on the therapeutic potential of garden cress (Lepidium sativum Linn.) with threatened status. Curr. Drug Res. Rev. 16 (3), 369–380. doi:10.2174/0125899775273877231023102011
Hajihasani, M. M., Soheili, V., Zirak, M. R., Sahebkar, A., and Shakeri, A. (2020). Natural products as safeguards against monosodium glutamate-induced toxicity. Iran. J. Basic Med. Sci. 23 (4), 416–430. doi:10.22038/IJBMS.2020.43060.10123
Hannan, M. A., Zahan, M. S., Sarker, P. P., Moni, A., Ha, H., and Uddin, M. J. (2021). Protective effects of black cumin (Nigella sativa) and its bioactive constituent, thymoquinone against kidney injury: an aspect on pharmacological insights. Int. J. Mol. Sci. 22 (16), 9078. doi:10.3390/ijms22169078
Hassan, H. A., El-Kholy, W. M., El-Sawi, M. R. F., Galal, N. A., and Ramadan, M. F. (2020). Myrtle (Myrtus communis) leaf extract suppresses hepatotoxicity induced by monosodium glutamate and acrylamide through obstructing apoptosis, DNA fragmentation, and cell cycle arrest. Environ. Sci. Pollut. Res. 27 (18), 23188–23198. doi:10.1007/s11356-020-08780-7
Heinrich, M., Jalil, B., Abdel-Tawab, M., Echeverria, J., Kulic, Z., McGaw, L. J., et al. (2022). Best practice in the chemical characterisation of extracts used in pharmacological and toxicological research-The ConPhyMP-Guidelines. Front. Pharmacol. 13, 953205. doi:10.3389/fphar.2022.953205
Hor, S. L., Teoh, S. L., and Lim, W. L. (2019). Plant polyphenols as neuroprotective agents in Parkinson's disease targeting oxidative stress. Curr. Drug Targets 21 (5), 458–476. doi:10.2174/1389450120666191017120505
Hosseinzadeh, H., and Nassiri-Asl, M. (2014). Review of the protective effects of rutin on the metabolic function as an important dietary flavonoid. J. Endocrinol. Investigation 37 (9), 783–788. doi:10.1007/s40618-014-0096-3
Ibrahim, M., Khalifa, A., Saleh, A., and Tammam, H. (2019). Histopathological and histochemical assessment of monosodium glutamate-induced hepatic toxicity and the amelioration with propolis. Ain Shams J. Forensic Med. Clin. Toxicol. 33 (2), 24–36. doi:10.21608/ajfm.2019.36572
Islam, Z., Islam, S. M. R., Hossen, F., Mahtab-Ul-Islam, K., Hasan, M. R., and Karim, R. (2021). Moringa oleifera is a prominent source of nutrients with potential health benefits. Int. J. Food Sci. 2021, 6627265. doi:10.1155/2021/6627265
Ivanova Stojcheva, E., and Quintela, J. C. (2022). The effectiveness of Rhodiola rosea L. preparations in alleviating various aspects of life-stress symptoms and stress-induced conditions-encouraging clinical evidence. Molecules 27 (12), 3902. doi:10.3390/molecules27123902
Jubaidi, F. F., Mathialagan, R. D., Noor, M. M., Taib, I. S., and Budin, S. B. (2019). Monosodium glutamate daily oral supplementation: study of its effects on male reproductive system on rat model. Syst. Biol. Reproductive Med. 65 (3), 194–204. doi:10.1080/19396368.2019.1573274
Kaczmarek, B. (2020). Tannic acid with antiviral and antibacterial activity as A promising component of biomaterials-A minireview. Mater. (Basel) 13 (14), 3224. doi:10.3390/ma13143224
Kadam, V., Gangiirde, S., and Mohan, M. (2019). Protective effect of Solanum torvum on monosodium glutamate-induced hepatotoxicity and nephrotoxicity in rats. Indian J. Nat. Prod. Resour. 10 (1), 31–42. doi:10.56042/ijnpr.v10i1.19023
Khalid, S., Khalid, N., Khan, R. S., Ahmed, H., and Ahmad, A. (2017). A review on chemistry and pharmacology of Ajwa date fruit and pit. Trends Food Sci. and Technol. 63, 60–69. doi:10.1016/j.tifs.2017.02.009
Khalid, W., Ali, A., Arshad, M. S., Afzal, F., Akram, R., Siddeeg, A., et al. (2022). Nutrients and bioactive compounds of Sorghum bicolor L. used to prepare functional foods: a review on the efficacy against different chronic disorders. Int. J. Food Prop. 25 (1), 1045–1062. doi:10.1080/10942912.2022.2071293
Khlestkina, E. K. (2013). The adaptive role of flavonoids: emphasis on cereals. Cereal Res. Commun. 41 (2), 185–198. doi:10.1556/CRC.2013.0004
Klein-Junior, L. C., de Souza, M. R., Viaene, J., Bresolin, T. M. B., de Gasper, A. L., Henriques, A. T., et al. (2021). Quality control of herbal medicines: from traditional techniques to state-of-the-art approaches. Planta Medica 87 (12-13), 964–988. doi:10.1055/a-1529-8339
Kuete, V., Eyong, K. O., Folefoc, G. N., Beng, V. P., Hussain, H., Krohn, K., et al. (2007). Antimicrobial activity of the methanolic extract and of the chemical constituents isolated from Newbouldia laevis. Pharmazie 62 (7), 552–556. doi:10.1691/ph.2007.7.6757
Kuti, J. O. (2004). Antioxidant compounds from four Opuntia cactus pear fruit varieties. Food Chem. 85 (4), 527–533. doi:10.1016/S0308-8146(03)00184-5
Loi, C., and Cynober, L. (2022). Glutamate: a safe nutrient, not just a simple additive. Ann. Nutr. Metabolism 78 (3), 133–146. doi:10.1159/000522482
Mao, Q. Q., Xu, X. Y., Cao, S. Y., Gan, R. Y., Corke, H., Beta, T., et al. (2019). Bioactive compounds and bioactivities of ginger (Zingiber officinale Roscoe). Foods 8 (6), 185. doi:10.3390/foods8060185
Mbah, U., and Egbuonu, A. (2017). Ethanolic extract of Solanum melongena Linn fruit mitigated monosodium glutamate-induced oxidative stress. Int. J. Biochem. Res. and Rev. 18 (2), 1–8. doi:10.9734/ijbcrr/2017/35055
Mehraj, T., Elkanayati, R. M., Farooq, I., and Mir, T. M. (2022). “A review of Nigella sativa and its active principles as anticancer agents,” in Black seeds (Nigella sativa): pharmacological and therapeutic applications. Editors A. Khan, and M. U. Rehman (Amsterdam: Elsevier), 91–118. doi:10.1016/B978-0-12-824462-3.00012-3
Mohamed, P., Radwan, R., Mohamed, S. A., and Mohamed, S. (2021). Toxicity of monosodium glutamate on liver and body weight with the protective effect of tannic acid in adult male rats. Mansoura J. Forensic Med. Clin. Toxicol. 29 (2), 0–32. doi:10.21608/mjfmct.2021.58908.1028
Mohammed, E., Abd-Ella, M., Mohammed, A. E., and Mohammed, A. L. (2016). Attenuation of monosodium glutamate-induced hepatic and testicular toxicity in albino rats by Annona muricata Linn. (Annonaceae) leaf extract. IOSR J. Pharm. Biol. Sci. 11 (6), 61–69. doi:10.9790/3008-1106046169
Munggari, I. P., Kurnia, D., Deawati, Y., and Julaeha, E. (2022). Current research of phytochemical, medicinal and non-medicinal uses of Uncaria gambir Roxb.: a review. Molecules 27 (19), 6551. doi:10.3390/molecules27196551
Mushatet, M. K., and Jawad, R. A. (2020). Protective effect of Linum usitatissimum aqueous extract against hepatotoxicity induced by monosodium glutamate in male rats. Trop. J. Nat. Prod. Res. 4 (7), 322–325. doi:10.26538/tjnpr/v4i7.13
Mustafa, S. J., and Qader, G. I. (2016). Effect of L-glutamic acid on histology and functions of liver and kidney of rats and protective role of Zingiber officionale. Diyala J. Med. 11 (2), 51–59.
Muzolf-Panek, M., and Stuper-Szablewska, K. (2021). Comprehensive study on the antioxidant capacity and phenolic profiles of black seed and other spices and herbs: effect of solvent and time of extraction. J. Food Meas. Charact. 15, 4561–4574. doi:10.1007/s11694-021-01028-z
Nahok, K., Li, J. V., Phetcharaburanin, J., Abdul, H., Wongkham, C., Thanan, R., et al. (2019). Monosodium glutamate (MSG) renders alkalinizing properties and its urinary metabolic markers of MSG consumption in rats. Biomolecules 9 (10), 542. doi:10.3390/biom9100542
Naveen Kumar, R., Uday Kumar, P., and Hemalatha, R. (2020). Monosodium glutamate (MSG) - a food additive. The Indian Journal of Nutrition and Dietetics 57 (1), 98–107. doi:10.21048/ijnd.2020.57.1.23998
Nguyen Thuy, L., Salanta, L. C., Tofana, M., Socaci, S. A., Farcas, A. C., and Pop, C. R. (2020). Mini review about monosodium glutamate. Bull. UASVM Food Sci. Technol. 77 (1), 1–12. doi:10.15835/buasvmcn-fst:2019.0029
Niaz, K., Zaplatic, E., and Spoor, J. (2018). Extensive use of monosodium glutamate: a threat to public health? EXCLI J. 17, 273–278. doi:10.17179/excli2018-1092
Niziol-Lukaszewska, Z., Furman-Toczek, D., Bujak, T., Wasilewski, T., and Hordyjewicz-Baran, Z. (2020). Moringa oleifera L. extracts as bioactive ingredients that increase safety of body wash cosmetics. Dermatology Res. Pract. 2020, 8197902. doi:10.1155/2020/8197902
Ogwu, M. C., Dunkwu-Okafor, A., Omakor, I. A., and Izah, S. C. (2023). “Turkey berry (Solanum torvum Sw. [Solanaceae]): an overview of the phytochemical constituents, nutritional characteristics, and ethnomedicinal values for sustainability,” in Herbal medicine phytochemistry: applications and trends. Editors S. C. Izah, M. C. Ogwu, and M. Akram (Cham: Springer International Publishing), 1–28. doi:10.1007/978-3-031-21973-3_73-1
Oluwole, D. T., Ebiwonjumi, O. S., Ajayi, L. O., Alabi, O. D., Amos, V., Akanbi, G., et al. (2024). Disruptive consequences of monosodium glutamate on male reproductive function: a review. Curr. Res. Toxicol. 6, 100148. doi:10.1016/j.crtox.2024.100148
Omogbiya, A. I., Ben-Azu, B., Eduviere, A. T., Eneni, A. O., Nwokoye, P. O., Ajayi, A. M., et al. (2021). Monosodium glutamate induces memory and hepatic dysfunctions in mice: ameliorative role of Jobelyn® through the augmentation of cellular antioxidant defense machineries. Toxicol. Res. 37 (3), 323–335. doi:10.1007/s43188-020-00068-9
Pal, L. C., Kumar, A., Pande, V., and Rao, C. V. (2020). Hepatoprotective effect of bioactive fraction of Lagerstroemia speciosa (L.) Pers. bark against monosodium glutamate-induced liver toxicity. Pharmacogn. J. 12 (6), 1630–1640. doi:10.5530/pj.2020.12.223
Palaian, S., Mishra, P., Bista, D., Rajan, S., and Shankar, P. R. (2007). Safety profile of herbal drugs: urgent need for monitoring. J. Inst. Med. Nepal 28 (2), 57–61. doi:10.59779/jiomnepal.253
Pane, Y. S., Machrina, Y., Hasan, S., Lumongga, F., and Yunanda, Y. (2021). Natural product of gambier (Uncaria gambier Roxb) extracts to counter against hepatotoxicity effects due to monosodium glutamate induction in male mice. J. Adv. Pharm. Technol. Res. 12 (1), 104–108. doi:10.4103/japtr.JAPTR_268_20
Paul, I., Aloh, G. S., Osuocha, K. U., Iwueke, A. V., and Chukwu, E. C. (2020). Ameliorative potentials of methanol leaf extract of Newbouldia laevis on monosodium glutamate induced toxicity in female albino rats. Afr. J. Biol. Sci. 2 (3), 37–50. doi:10.33472/AFJBS.2.3.2020.37-50
Pauzi, N. A. M., Cheema, M. S., Ismail, A., Ghazali, A. R., and Abdullah, R. (2022). Safety assessment of natural products in Malaysia: current practices, challenges, and new strategies. Rev. Environ. Health 37 (2), 169–179. doi:10.1515/reveh-2021-0072
Peica, N., Lehene, C., Leopold, N., Schlucker, S., and Kiefer, W. (2007). Monosodium glutamate in its anhydrous and monohydrate form: differentiation by Raman spectroscopies and density functional calculations. Spectrochimica Acta Part A Mol. Biomol. Spectrosc. 66 (3), 604–615. doi:10.1016/j.saa.2006.03.037
Pressman, P., Clemens, R., Hayes, W., and Reddy, C. (2017). Food additive safety: a review of toxicologic and regulatory issues. Toxicol. Res. Appl. 1, 2397847317723572. doi:10.1177/2397847317723572
Rakshit, S., Shukla, P., Verma, A., Kumar Nirala, S., and Bhadauria, M. (2021). Protective role of rutin against combined exposure to lipopolysaccharide and D-galactosamine-induced dysfunctions in liver, kidney, and brain: hematological, biochemical, and histological evidences. J. Food Biochem. 45 (2), e13605. doi:10.1111/jfbc.13605
Ramamurthy, S., Varghese, S., Sudarsan, S., Muruganandhan, J., Mushtaq, S., Patil, P. B., et al. (2021). Moringa oleifera: antioxidant, anticancer, anti-inflammatory, and related properties of extracts in cell lines: a review of medicinal effects, phytochemistry, and applications. J. Contemp. Dent. Pract. 22 (12), 1483–1492.
Sahin, B., Acikel Elmas, M., Bingol Ozakpinar, O., and Arbak, S. (2023). The effects of apocynin on monosodium glutamate induced liver damage of rats. Heliyon 9 (7), e17327. doi:10.1016/j.heliyon.2023.e17327
Sari, F. N., Mohamed Said, M. S., and Abd Rashid, N. (2024). Antioxidant activity of ginger, lemongrass, and mixture of ginger and lemongrass. Afr. J. Biol. Sci. 6 (16), 2505–2510. doi:10.48047/AFJBS.6.16.2024.2504-2511
Samir, A., Kmel, A. H., Abdul, H., Mohamed, R., and Amer, A. S. (2019). The possible protective effect of rutin against hepatotoxicity induced by mono-sodium glutamate on male rats. Wulfenia J. 26 (4), 42–62.
Shamim, R., Iqbal, Z., Tariq, A., Zulfiqar, S., Ara, N., and Khan, F. (2020). Protective effects of Phoenix dactyliferaon monosodium glutamate induced histomorphological changes in hepatocytes of adult male albino rats: a light microscopic study. Med. Forum Mon. 31 (1), 87–90.
Shinde, M., and Mohan, M. (2022). Protective efficacy of Murraya koenigii aqueous extract against monosodium glutamate-induced hepatotoxicity in Wistar rats. Indian J. Nat. Prod. Resour. 13 (2), 188–196. doi:10.56042/ijnpr.v13i2.48407
Shivashankara, A. R., Kumar, A., Ravi, R., Simon, P., Rai, P., Francis, A., et al. (2014). “Hepatoprotective effects of green tea and its polyphenols: preclinical observations,” in Polyphenols in human health and disease. Editors R. R. Watson, V. R. Preedy, and S. Zibadi (Amsterdam: Academic Press), 715–721. doi:10.1016/B978-0-12-398456-2.00055-4
Shosha, H. M., Ebaid, H. M., Toraih, E. A., Abdelrazek, H. M. A., and Elrayess, R. A. (2023). Effect of monosodium glutamate on fetal development and progesterone level in pregnant Wistar Albino rats. Environ. Sci. Pollut. Res. 30 (17), 49779–49797. doi:10.1007/s11356-023-25661-x
Shukry, M., El-Shehawi, A. M., El-Kholy, W. M., Elsisy, R. A., Hamoda, H. S., Tohamy, H. G., et al. (2020). Ameliorative effect of graviola (Annona muricata) on mono sodium glutamate-induced hepatic injury in rats: antioxidant, apoptotic, anti-inflammatory, lipogenesis markers, and histopathological studies. Animals 10 (11), 1996. doi:10.3390/ani10111996
Singh, K., and Ahluwalia, P. (2012). Effect of monosodium glutamate on lipid peroxidation and certain antioxidant enzymes in cardiac tissue of alcoholic adult male mice. J. Cardiovasc Dis. Res. 3 (1), 12–18. doi:10.4103/0975-3583.91595
Siti, H. N., Jalil, J., Asmadi, A. Y., and Kamisah, Y. (2022). Effects of quercetin on cardiac function in pressure overload and postischemic cardiac injury in rodents: a systematic review and meta-analysis. Cardiovasc. Drugs Ther. 36 (1), 15–29. doi:10.1007/s10557-020-07100-y
Soliman, M. M., Althobaiti, S. A., and Sayed, S. (2023). Ameliorative impacts of Rhodiola rosea against hepatic toxicity induced by monosodium glutamate: role of inflammation-oxidative-stress-and apoptosis-associated markers. J. Taibah Univ. Sci. 17 (1). doi:10.1080/16583655.2023.2244749
Syed, A. H. S. A., Abd Rashid, N., Woon, C. K., and Abdul Jalil, N. A. (2023). Natural products targeting PI3K/AKT in myocardial ischemic reperfusion injury: a scoping review. Pharmaceuticals 16 (5), 739. doi:10.3390/ph16050739
Tavakkoli, A., Ahmadi, A., Razavi, B. M., and Hosseinzadeh, H. (2017). Black seed (Nigella sativa) and its constituent thymoquinone as an antidote or a protective agent against natural or chemical toxicities. Iran. J. Pharm. Res. 16, 2–23.
Tena, N., Martin, J., and Asuero, A. G. (2020). State of the art of anthocyanins: antioxidant activity, sources, bioavailability, and therapeutic effect in human health. Antioxidants 9 (5), 451. doi:10.3390/antiox9050451
Testai, L., Pagnotta, E., Piragine, E., Flori, L., Citi, V., Martelli, A., et al. (2022). Cardiovascular benefits of Eruca sativa mill. defatted seed meal extract: potential role of hydrogen sulfide. Phytotherapy Res. 36 (6), 2616–2627. doi:10.1002/ptr.7479
Tiwary, B. K., Dutta, S., Dey, P., Hossain, M., Kumar, A., Bihani, S., et al. (2017). Radical scavenging activities of Lagerstroemia speciosa (L.) Pers. petal extracts and its hepato-protection in CCl4-intoxicated mice. BMC Complementary Med. Ther. 17 (1), 55. doi:10.1186/s12906-016-1495-0
Tosun, H., Karadas, H., and Ceylan, H. (2024). Bioinformatics-based identification of hepatocellular carcinoma-associated hub genes and assessment of the restorative effect of tannic acid in rat liver exposed to monosodium glutamate. Cancer Med. 13, e7404. doi:10.1002/cam4.7404
Tousson, E., El-Atrash, A., and Karson, Y. (2019). Protective role of rocket seed (Eruca sativa) extract against monosodium glutamate-induced hepato-renal toxicity in male rats. Asian J. Res. Med. Pharm. Sci. 8 (3-4), 1–10. doi:10.9734/ajrimps/2019/v8i3-430136
Tura, D., and Robards, K. (2002). Sample handling strategies for the determination of biophenols in food and plants. J. Chromatogr. 975 (1), 71–93. doi:10.1016/s0021-9673(02)00879-8
Ukwubile, C. A., Ikpefan, E. O., Dibal, M. Y., Umeano, V. A., Menkiti, D. N., Kaosi, C. C., et al. (2023). Pharmacognostic profiles, evaluation of analgesic, anti-inflammatory and anticonvulsant activities of Newbouldia laevis (P. Beauv.) Seem. ex Bureau leaf and root extracts in Wistar rats. J. Ethnopharmacol. 314, 116632. doi:10.1016/j.jep.2023.116632
Vanamala, J. K. P., Massey, A. R., Pinnamaneni, S. R., Reddivari, L., and Reardon, K. F. (2018). Grain and sweet sorghum (Sorghum bicolor L. Moench) serves as a novel source of bioactive compounds for human health. Crit. Rev. Food Sci. Nutr. 58 (17), 2867–2881. doi:10.1080/10408398.2017.1344186
Weremfo, A., Adulley, F., Dabie, K., Abassah-Oppong, S., and Peprah-Yamoah, E. (2022). Optimization of ultrasound-assisted extraction of phenolic antioxidants from Turkey berry (Solanum torvum Sw) fruits using response surface methodology. J. Appl. Res. Med. Aromatic Plants 30, 100387. doi:10.1016/j.jarmap.2022.100387
Wijayasekara, K., and Wansapala, J. (2017). Uses, effects and properties of monosodium glutamate (MSG) on food and nutrition. Int. J. Food Sci. Nutr. 2 (3), 132–143.
Woon, C. K., Hui, W. K., Abas, R., Haron, M. H., Das, S., and Teoh, S. L. (2022). Natural product-based nanomedicine: recent advances and issues for the treatment of Alzheimer's disease. Curr. Neuropharmacol. 20 (8), 1498–1518. doi:10.2174/1570159X20666211217163540
Xu, D., Hu, M. J., Wang, Y. Q., and Cui, Y. L. (2019). Antioxidant activities of quercetin and its complexes for medicinal application. Molecules 24 (6), 1123. doi:10.3390/molecules24061123
Yadav, N., Singh, C. S., Venkatachalam, T., and Fathima, S. N. (2024). “Herbal medicine formulation, standardization, and commercialization challenges and sustainable strategies for improvement,” in Herbal medicine phytochemistry Reference series in phytochemistry. Editors S. C. Izah, M. C. Ogwu, and M. Akram (Cham: Springer). doi:10.1007/978-3-031-43199-9_40
Yamamoto, T., and Inui-Yamamoto, C. (2023). The flavor-enhancing action of glutamate and its mechanism involving the notion of kokum. NPJ Sci. Food 7 (1), 3. doi:10.1038/s41538-023-00178-2
Yang, B., Dong, Y., Wang, F., and Zhang, Y. (2020). Nanoformulations to enhance the bioavailability and physiological functions of polyphenols. Molecules 25 (20), 4613. doi:10.3390/molecules25204613
Yarmohammadi, F., Ghasemzadeh Rahbardar, M., and Hosseinzadeh, H. (2021). Effect of eggplant (Solanum melongena) on the metabolic syndrome: a review. Iran. J. Basic Med. Sci. 24 (4), 420–427. doi:10.22038/ijbms.2021.50276.11452
Yousef, S. M. (2022). Antioxidant effects of soursop extract and Nigella sativa seeds against oxidative stress induced by mono sodium glutamate in male rats. J. Res. Fields Specif. Educ. 8 (42), 1483–1505. doi:10.21608/jedu.2022.168095.1759
Youssef, G. S., El-Rebey, H. S., Abdel-Razek, H., Adel, M. M., Donia, S. S., and Motawea, S. M. (2023). Effect of diltiazem and quercetin on monosodium glutamate-induced hepatic dysfunction in rats. Menoufia Med. J. 35 (2), 1872–1878. doi:10.4103/mmj.mmj_268_22
Keywords: monosodium glutamate, liver injury, oxidative stress, herbal medicine, plant products, complementary medicine
Citation: Kasmara DP, Abdullah E, Harun Z, Sari FN, Abd Rashid N and Teoh SL (2025) Mini review of plant products as food supplement against MSG-induced liver injury: antioxidant, oxidative stress and histological prospects. Front. Pharmacol. 16:1522814. doi: 10.3389/fphar.2025.1522814
Received: 05 November 2024; Accepted: 02 January 2025;
Published: 24 January 2025.
Edited by:
Petr Pavek, Charles University, CzechiaReviewed by:
Mikhail Olugbemiro Nafiu, University of Ilorin, NigeriaCopyright © 2025 Kasmara, Abdullah, Harun, Sari, Abd Rashid and Teoh. This is an open-access article distributed under the terms of the Creative Commons Attribution License (CC BY). The use, distribution or reproduction in other forums is permitted, provided the original author(s) and the copyright owner(s) are credited and that the original publication in this journal is cited, in accordance with accepted academic practice. No use, distribution or reproduction is permitted which does not comply with these terms.
*Correspondence: Norhashima Abd Rashid, bm9yaGFzaGltYUBsaW5jb2xuLmVkdS5teQ==; Seong Lin Teoh, dGVvaHNlb25nbGluQHVrbS5lZHUubXk=
Disclaimer: All claims expressed in this article are solely those of the authors and do not necessarily represent those of their affiliated organizations, or those of the publisher, the editors and the reviewers. Any product that may be evaluated in this article or claim that may be made by its manufacturer is not guaranteed or endorsed by the publisher.
Research integrity at Frontiers
Learn more about the work of our research integrity team to safeguard the quality of each article we publish.