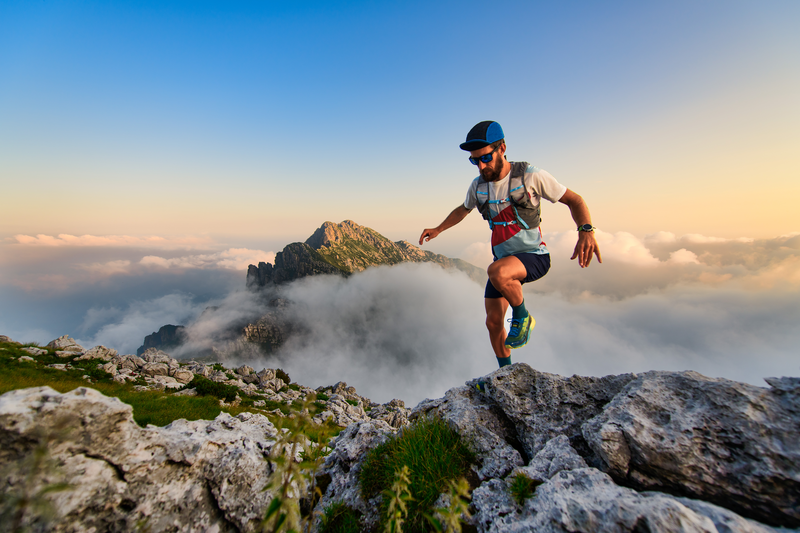
94% of researchers rate our articles as excellent or good
Learn more about the work of our research integrity team to safeguard the quality of each article we publish.
Find out more
ORIGINAL RESEARCH article
Front. Pharmacol.
Sec. Pharmacogenetics and Pharmacogenomics
Volume 16 - 2025 | doi: 10.3389/fphar.2025.1519383
The final, formatted version of the article will be published soon.
You have multiple emails registered with Frontiers:
Please enter your email address:
If you already have an account, please login
You don't have a Frontiers account ? You can register here
Background: Aspirin is a cornerstone medication for acute ischemic stroke (AIS), but its efficacy varies significantly among individuals. This study aimed to develop a pharmacogenetic polygenic response score (PgxRS) to predict the incidence of adverse outcomes in aspirin-treated AIS patients.Methods: We conducted a retrospective study involving 828 AIS patients who received aspirin therapy. Fifteen candidate single nucleotide variants (SNPs) in genes related to aspirin's mechanism of action, transport, metabolism, and platelet function were genotyped. The association between SNPs and the risk of unfavorable prognosis (defined as modified Rankin Scale score >1 at 90 days) was assessed using logistic regression analysis. Multivariable models incorporating SNPs and clinical factors were developed to predict adverse outcomes.Results: The rs1045642 GG genotype in the ABCB1 gene was significantly associated with a lower risk of unfavorable prognosis, while the rs1371097 T allele in the P2Y1 gene was linked to a higher risk. A prediction model incorporating these two SNPs along with clinical variables demonstrated moderate diagnostic accuracy for predicting unfavorable prognosis (AUC=0.78, 95% CI: 0.74-0.81).Our findings suggest that rs1045642 and rs1371097 genotypes contribute to variability in aspirin response among AIS patients. The developed PgxRS, incorporating these SNPs and clinical factors, can potentially aid in risk stratification and guide personalized antiplatelet therapy decisions. However, further validation in larger, diverse cohorts is warranted.
Keywords: Aspirin, Acute ischemic stroke, Single nucleotide variant, Pharmacogenetics, prognosis, personalized medicine
Received: 29 Oct 2024; Accepted: 19 Feb 2025.
Copyright: © 2025 Ma, Zhang, Li, Ding, Zhang, Xue, Ci, Bai, Hu, Zhou and Yue. This is an open-access article distributed under the terms of the Creative Commons Attribution License (CC BY). The use, distribution or reproduction in other forums is permitted, provided the original author(s) or licensor are credited and that the original publication in this journal is cited, in accordance with accepted academic practice. No use, distribution or reproduction is permitted which does not comply with these terms.
* Correspondence:
Ruinan Ma, Department of Neurology, Yangpu Hospital, Tongji University, Shanghai, China
Liang Hu, Department of Neurology, Yangpu Hospital, Tongji University, Shanghai, China
Daizhan Zhou, Department of Neurology, Yangpu Hospital, Tongji University, Shanghai, China
Yunhua Yue, Department of Neurology, Yangpu Hospital, Tongji University, Shanghai, China
Disclaimer: All claims expressed in this article are solely those of the authors and do not necessarily represent those of their affiliated organizations, or those of the publisher, the editors and the reviewers. Any product that may be evaluated in this article or claim that may be made by its manufacturer is not guaranteed or endorsed by the publisher.
Research integrity at Frontiers
Learn more about the work of our research integrity team to safeguard the quality of each article we publish.