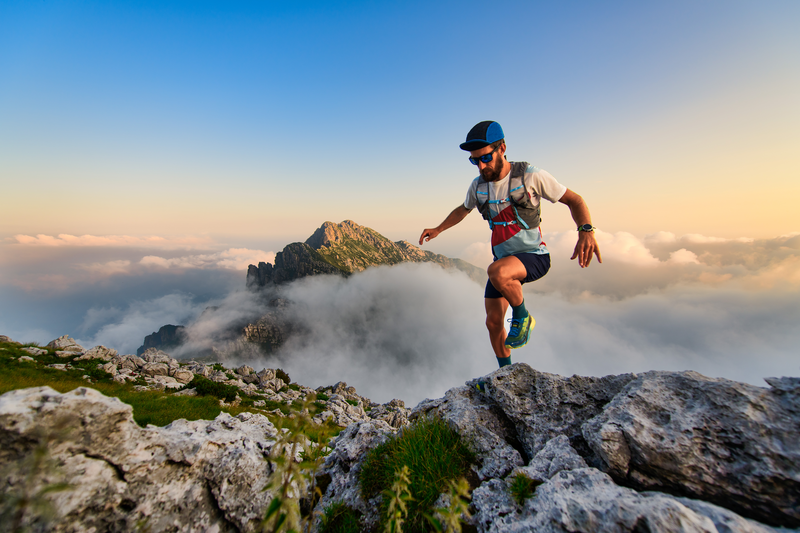
95% of researchers rate our articles as excellent or good
Learn more about the work of our research integrity team to safeguard the quality of each article we publish.
Find out more
REVIEW article
Front. Pharmacol.
Sec. Renal Pharmacology
Volume 16 - 2025 | doi: 10.3389/fphar.2025.1517047
This article is part of the Research Topic Reviews in Renal Pharmacology: 2024 View all 9 articles
The final, formatted version of the article will be published soon.
You have multiple emails registered with Frontiers:
Please enter your email address:
If you already have an account, please login
You don't have a Frontiers account ? You can register here
Uncontrolled hyperuricemia contributes to chronic kidney disease, characterized by renal inflammatory cell infiltration and tubulointerstitial fibrosis, eventually leading to renal failure. In addition to liver and kidney, the intestine tract plays a vital role in the development and progression of hyperuricemia and hyperuricemic nephropathy (HN) through various mechanisms. The conventional therapeutic strategy for HN is uric acid-lowering therapy (ULT) and renal protection; however, unsatisfactory results are often obtained in clinical practice. Growing evidence has demonstrated that traditional Chinese medicines (TCMs) achieve an anti-HN effect by modulating multiple targets and approaches with fewer side effects. Therefore, this paper reviews the pathogenesis of HN, including the role of soluble and insoluble urates in kidney and intestine, and the role of intestinal tract in the progression of HN. Meanwhile, the recent advancements in TCMs for the treatment of HN are summarized and analyzed, with a focus on their modulation of intestinal flora and metabolites, urate-related transporters, immuno-inflammation and barrier function in the intestines. Notably, for the first time, we propose the perspective that TCMs treat HN through a dualregulatory effect on the intestines and kidneys. Additionally, the problems existing in current research and the feasible research strategies combined with emerging technologies such as fermentation and nanotechnology are discussed, thus providing novel ideas for HN management.
Keywords: Hyperuricemic nephropathy, Gut Microbiota, Urate transporters, Immuno-inflammatory response, Traditional Chinese Medicine
Received: 25 Oct 2024; Accepted: 28 Mar 2025.
Copyright: © 2025 Wang, Li, Liu, Tan, Wu, Zhu, Hua, Dai, Li, Mao, Zhao and Yin. This is an open-access article distributed under the terms of the Creative Commons Attribution License (CC BY). The use, distribution or reproduction in other forums is permitted, provided the original author(s) or licensor are credited and that the original publication in this journal is cited, in accordance with accepted academic practice. No use, distribution or reproduction is permitted which does not comply with these terms.
* Correspondence:
Jun-Ning Zhao, College of Pharmacy, Southwest Medical University, Luzhou, China
Zhujun Yin, Sichuan Academy of Traditional Chinese Medicine, Chengdu, 610041, Sichuan Province, China
Disclaimer: All claims expressed in this article are solely those of the authors and do not necessarily represent those of their affiliated organizations, or those of the publisher, the editors and the reviewers. Any product that may be evaluated in this article or claim that may be made by its manufacturer is not guaranteed or endorsed by the publisher.
Research integrity at Frontiers
Learn more about the work of our research integrity team to safeguard the quality of each article we publish.