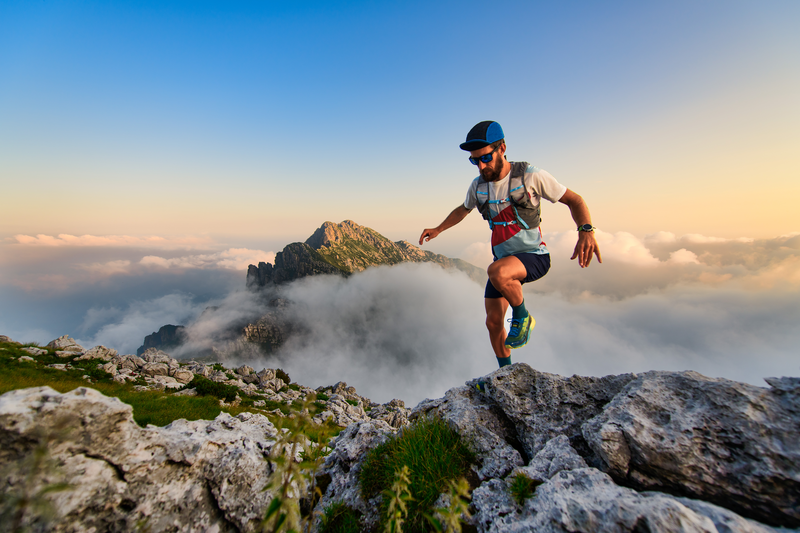
94% of researchers rate our articles as excellent or good
Learn more about the work of our research integrity team to safeguard the quality of each article we publish.
Find out more
ORIGINAL RESEARCH article
Front. Pharmacol. , 11 February 2025
Sec. Experimental Pharmacology and Drug Discovery
Volume 16 - 2025 | https://doi.org/10.3389/fphar.2025.1513105
Background: Aflatoxins are highly potent mycotoxins that can seriously harm the health of humans and a variety of animal species. On the other hand, camel milk and silymarin offer a variety of positive effects for many animal species. In addition, camel milk and silymarin reduce the impact of AFB1 on the hematology, serum biochemical markers, histopathology of the liver and testes, and expression of the inflammatory, antioxidant, and male reproductive genes.
Methods: 40 rats were used to evaluate the beneficial effect of silymarin and camel milk against aflatoxin B1 (AFB1) toxicity in rats. The classified treatments were the control negative (no treatment) and the control positive (supplied with 1.4 mg aflatoxin/kg diet) for 28 days. Camel milk group (supplied with 1.4 mg aflatoxin/kg diet) for 28 days and camel milk (1 milliliter of camel milk per kilogram of body weight) orally, from day 29 to day 43). Silymarin (supplied with 1.4 mg aflatoxin/kg diet) for 28 days and silymarin (20 mg silymarin/kg b.wt), orally, from day 29 to day 43). The evaluation was done through measuring leukocyte count, liver function tests, carcinoembryonic antigen (CEA), alpha-fetoprotein (AFP), ferritin, and testosterone. Moreover, the histopathology of the liver and testes was done along with expression levels of specific genes in the liver and testes.
Results: The outcomes showed that the post-treatment with silymarin and camel milk improved biochemical markers in serum and ability to reproduce.
Conclusion: In conclusion, post-treatment with camel milk and silymarin could mitigate the negative effect of AFB1 on rats.
Aspergillus flavus and A. parasiticus are two species of Aspergillus that generate mycotoxin as their secondary metabolite (Ali et al., 2022; Saleemi et al., 2023). Aflatoxin B1 (AFB1), the most dangerous aflatoxin, is a key contributor to hepatocellular cancer (Rotimi et al., 2021). A. flavus-produced mycotoxins are frequently found on grains that have been harvested and stored damp. It can also be detected on grains that have been grown under stressful conditions, such as high moisture and temperature and on cereals like wheat, corn, beans, and rice (Kudayer et al., 2019). AFB1 can result in oxidative stress, growth inhibition, and liver damage (Schrenk et al., 2020). It has significant DNA mutagenicity and carcinogenicity, and the International Agency for Research on Cancer (IARC) has categorized it as a group-1 carcinogen (Kilic et al., 2022). Moreover, AFB1 has been widely reported to be hepatotoxic during mammalian growth and development (Wu et al., 2022). It is well recognized that aflatoxins are strong mutagenic, hepatotoxic, hepatocarcinogenic, nephrotoxic, teratogenic, genotoxic, and immunosuppressive and that they also impede several metabolic processes, thus harming the heart, liver, and kidneys (Yilmaz et al., 2018; Altyar et al., 2023; 2024). These poisons have been implicated as the cause of some human deaths and excessive livestock mortality (Ijaz et al., 2022). Excessively vacuolated cells and spermatogenesis suppression were observed in the testicles of aflatoxicated rats (Kudayer et al., 2019).
Milk is crucial to maintaining health and preventing disease (Meisel, 2005; Yahya et al., 2018). Milk from camels and cows is a vital source of crucial nutrients (Saeed et al., 2022). Many diabetic people require camel milk for medical help, and it is also employed as an anti-microbial hepatoprotective medication due to its unique properties (Althnaian et al., 2013). In addition to minerals and vitamins, camel milk also contains several useful biological components (Albarrak, 2023). The treatment of various immunological abnormalities and metabolic dysfunctions, particularly those linked to some kinds of diabetes, has shown positive medical results (Agrawal et al., 2002; 2007; Mohamad et al., 2009). Additionally, camel milk is used to treat several chronic illnesses (Alorainy et al., 2023). Numerous studies claim that camel milk protects against the harmful effects of toxic substances like cadmium chloride, aluminum chloride, paracetamol, carbon tetrachloride, and cisplatin (Al-Asmari et al., 2017; Al-Fartosi et al., 2011; Afifi, 2010; Al-Hashem et al., 2009; Dallak, 2009; Al-Hashem, 2009).
Natural remedies made from plants are being used more frequently across the world because they are seen to be potentially safe and effective alternatives to conventional therapies for liver problems (Wang et al., 2018). Silybum marianum (Asteraceae) has a long history of use as a treatment for a variety of hepatic illnesses, including cirrhosis and hepatitis, as well as preventing liver damage arising from toxins and chemicals in the environment (Abenavoli et al., 2010; Pascual et al., 1993). Silymarin, a hepatoprotective substance that has demonstrated protective properties against inflammation, oxidation, and apoptosis, has been used clinically for centuries, either by itself or as a main component of a variety of medicinal formulations (Arafa, 2009; Shuppan et al., 1999). Silymarin’s stabilizing impact on cytoplasmic membranes is linked to its anti-hepatotoxic action (Wang et al., 2018).
Previous studies (Hassaneen et al., 2023; Hassaneen et al., 2024) have discussed the therapeutic effect of camel milk and silymarin on the harmful effect of aflatoxin in rats from the onset of poisoning. Therefore, this experiment was conducted to evaluate post-treatment with camel milk and silymarin for 14 days on a male rat model exposed to aflatoxin toxicity for 28 days in the diet.
The Faculty of Veterinary Medicine, Alexandria University, Egypt, gave its ethical approval to this work. We acquired 40 mature (4 weeks old) male (Wister white) rats (84.7 g; Hassaneen et al. (2024)) from the Abdo farm for laboratory animals located in Alexandria, Egypt, for this study (Figure 1). We were granted permission to use the rats after formally applying to the Faculty of Veterinary Medicine, Matrouh University. During the acclimatization (15 days) and experimental periods (43 days), water was available at all times. The aflatoxicated diet was prepared using autoclaved crushed yellow corn, where 10 mL of the toxigenic strain’s spore suspension (106 spores/mL) was added. After that, the treated corn was incubated for 21 days at 28–30°C to ferment it. After 21 days, the corn was incubated for 3–4 days at 50°C to kill the fungus. The crushed corn was then ground into a powder using the grinder. Next, AFB1 was calculated using a representative sample of 25 g powdered corn (AOAC, 1980). To obtain the required dosage of 1.4 mg of aflatoxin/kg of feed, the tainted corn was then mixed with commercially crushed corn previously measured for the presence of mycotoxins. All rats were given free access to this prepared feed. They were classified into four groups of ten rats each. The classified groups were the control negative (no medical attention) and control positive (administered aflatoxin 1.4 mg/kg feed for 28 days, as per El-Nekeety et al. (2014)). The camel milk group was administered aflatoxin 1.4 mg/kg feed for 28 days and 1 mL of camel milk per kilogram of body weight administered orally as per Al-Hashem et al. (2009) from days 29 to 43. The silymarin group was administered aflatoxin 1.4 mg/kg feed for 28 days and oral silymarin (20 mg/kg b.wt) suspension, as per Rastogi et al. (2001), from days 29 to 43.
After 43 days (end of the trial), whole blood with EDTA and serum samples were collected from the medial canthus of the eye of all groups after isoflurane anesthesia. The whole blood was used to count leukocytes and deferential. Meanwhile, serum was stored at −20°C to measure aminotransferases (AST and ALT) and the proteins’ calorimetric and carcinoembryonic antigen (CEA), alpha-fetoprotein (AFP), ferritin, and testosterone by a Microplate Immunosymmetric assay. Liver and testis tissue samples (n = 5) were collected after cervical dislocation for histopathological examination in formalin. The sections of the liver and testes were collected and immediately fixed in 10% buffered formalin and processed for histopathological evaluation using routine paraffin sections. Sections 5 μm thick were cut and stained with hematoxylin and eosin (H&E) as per Bancroft and Gamble (2008). To pathologically score the liver and testes, eight X200 power fields with a total area of roughly 11–12 mm2 were examined. On a three-point rating system, lesions of the liver and testes were evaluated by adding the following factors: necrosis, degenerative and inflammatory alterations, and the diffusion of the lesions. In accordance with normal tissue, mild, moderate, severe (multi focused), and more serious lesions (diffuse), the scores for each parameter ranged from 0 to 4. Moreover, the tissue samples from liver and testes were collected for gene expression and stored at −08°C until RNA isolation.
We employed quantitative real-time PCR (qRT-PCR) to obtain the expression of: NQO 1, NAD(P)H quinone dehydrogenase 1; TNFα, tumor necrosis factor α; APE1, apurinic/apyrimidinic endodeoxyribonuclease 1; OGG1, 8-oxoguanine DNA glycosylase; LHR, luteinizing hormone receptor; StAR, steroidogenic acute regulatory protein. The liver and testicular samples were obtained, frozen in liquid nitrogen, and kept at −80°C to extract RNA. Using an RNA Purification Kit from Thermo Scientific (USA), RNA was isolated from the frozen samples in accordance with the manufacturer’s instructions. To produce cDNA from a fixed concentration of RNA, Thermo Scientific USA’s Intron-Power cDNA synthesis kit was utilized. Using the β-actin housekeeping gene as a normalization, the negative control group was the calibrator. Specific primers (Table 1) were utilized to amplify NQO 1, TNFα, APE1, LHR, and StAR in rats to assay the qRT-PCR. The 2−ΔΔCt approach was utilized to analyze the data acquired (Livak and Schmittgen, 2001).
Data were analyzed by one way analysis of variance (ANOVA), where all results are reported as means ± SEM. Shapiro–Wilk and Levene testing confirmed normal distribution and variance homogeneity. GraphPad Prism version 7.00 for Windows, GraphPad Software, La Jolla, California, United States, www.graphpad.com, was used to perform statistical analysis and create graphs. The significance level was P ≤ 0.05.
Table 2 illustrates the impact of camel milk and silymarin post-treatment on leukocytic count in rats given diets containing 1.4 mg of aflatoxin B1/kg diet for 43 days. The counts of WBC, lymphocytic, monocyte, and basophil decreased in the control positive group as opposed to the control negative; these decreases were significant (P ≤ 0.05). Meanwhile, the neutrophil count significantly (P ≤ 0.05) increased in the control positive as opposed to the negative. On the other hand, the eosinophil count increased in the control positive group as opposed to the negative control without significance (P ≤ 0.05). Both post-treatments (camel milk and silymarin) increased counts of WBC, lymphocyte, and basophil compared to the positive control group. Meanwhile, the results for eosinophils and monocytes were significantly (P ≤ 0.05) increased only in the silymarin group, as opposed to the positive control. On the other hand, the neutrophil count significantly (P ≤ 0.05) decreased in both post-treatment groups compared to the positive control.
Table 3 illustrates the impact of post-treatment camel milk and silymarin on liver function in rats given diets containing 1.4 mg aflatoxin B1/kg for 43 days. There was a significant (P ≤ 0.05) increase in the serum concentration of AST and ALT in the control positive group compared with the control negative. Meanwhile, serum proteins decreased in the control positive groups without significance (P ≤ 0.05) as opposed to the negative. The post-treatment outcomes showed that the serum ALT and AST significantly (P ≤ 0.05) decreased in the group that received camel milk as opposed to the positive control. Meanwhile, AST only in the silymarin group significantly (P ≤ 0.05) decreased as opposed to the positive control. Serum proteins increased in the camel milk and silymarin groups as opposed to the positive control without significance (P ≤ 0.05), except that serum albumin significantly (P ≤ 0.05) increased in the camel milk group.
Table 4 illustrates the impact of camel milk and silymarin post-treatment on serum biochemical measurements in rats given diets containing 1.4 mg aflatoxin B1/kg for 43 days. The concentrations of AFP and CEA increased significantly (P ≤ 0.05) in the control positive group as opposed to the control negative, while serum ferritin decreased in the control positive group without significance (P ≤ 0.05) compared to control negative. On the other hand, the testosterone result decreased significantly (P ≤ 0.05) in the control positive group as opposed to the control negative. For post-treatment, the concentrations of AFP and CEA of the post-treatment groups (camel milk and silymarin) significantly (P ≤ 0.05) decreased as opposed to the positive control. Moreover, testosterone significantly (P ≤ 0.05) increased in the camel milk and silymarin groups compared to the control positive. Meanwhile, serum ferritin significantly (P ≤ 0.05) increased in the camel milk group as opposed to the positive control one, while it increased without significance (P ≤ 0.05) in the silymarin group.
The quantitative assessment of the lesion scores in the liver and testis tissues regarding aflatoxin supplementation and administration of camel milk and silymarin is shown in Figure 2. The rats supplemented with a control diet had normal tissue. Rats supplemented with the aflatoxicated diet showed the most severe damage to their liver and testis tissues. However, the lesion scores significantly decreased in rats administered camel milk and silymarin.
Figure 2. Lesion score of the liver and testis tissues in different groups. (A) Lesion score of the liver tissue. (B) Lesion score of the testis tissues.
The result of the liver and testicular tissue histopathology is shown in Figures 3 and 4 at 15 days post-treatment. The control group’s liver displayed normal hepatic histology, with a normal portal region and normal hepatocyte arrangement in cords (PA) (Figure 3A). The control positive group demonstrated significant portal area fibrosis and a high level of mononuclear cell infiltration in the portal area (Figure 3B). However, camel and silymarin helped lessen these adverse effects, the liver of the camel milk group showing decreased vacuolar degeneration of the hepatocytes (Figures 3C, D, respectively).
Figure 3. Photomicrograph of the liver (n = 5) of the experimental groups (H&E, X200, Scale bar = 50 μ). (A) Liver of the control negative group showing normal hepatic histology featuring normal hepatocytes arranging in cords and normal portal area (PA). (B) Liver of the control positive group showing marked fibrosis of the portal area and a high level of mononuclear cell infiltration in the portal area. (C) Liver of the camel milk group showing decrease the vacuolar degeneration of the hepatocytes (arrows). (D) Liver of the silymarin group showing marked decrease the vacuolar degenerative changes of the hepatocytes (arrows).
Figure 4. Photomicrograph of the testes (n = 5) of the experimental groups (H&E, X200, Scale bar 50p). (A) Testes of the control negative group showing normal testicular histology with the seminiferous tubule lined with multilayer of spermatogenic cells (SC) and impacted with sperm (arrow) with interstitial Leydig cells (LC). (B) Testes of the control positive group showing arrest of spermatogenesis in some seminiferous tubules with degenerative changes of spermatogenic cells and interstitial edema. (C) Testes of the camel milk group showing decrease in the vacuolar degeneration of the spermatogenic cells (arrows) with decrease in the oedema within the interstitial tissues (SC indicates spermatogenic cells and LC indicates Leydig cells). (D) Testis of the silymarin group showing a marked decrease in the degenerative changes and mostly revealing normal testicular histology as the seminiferous tubule lined with a multilayer of spermatogenic cells (SC) and impacted with sperms (SP) with interstitial Leydig cells (LC).
The histopathology of the testes of the treatment groups at 15 days post-treatment of the experiment is shown in Figure 4. The testes of the negative group showed typical testicular histology, with sperm affected by interstitial Leydig cells (LC) and spermatogenic cells (SC) lining the seminiferous tubule (Figure 4A). The testes of the control positive group showed spermatogenesis arrest in certain seminiferous tubules accompanied by interstitial edema and spermatogenic cell degenerative alterations (Figure 4B). The testes of the camel milk group showed decreased vacuolar degeneration of the spermatogenic cells with decreased oedema within the interstitial tissues (SC indicates spermatogenic cells and LC indicates Leydig cells) (Figure 4C). The testes of the silymarin group showed a marked decrease in the degenerative changes and mostly revealed typical testicular histology, with sperm affected by interstitial Leydig cells (LC) and spermatogenic cells (SC) lining the seminiferous tubule (Figure 4D).
As demonstrated in Figure 5, the findings of gene expression were assessed by qRT-PCR, normalized to β-actin mRNA of the liver tissue of the experimental groups at 15 days post-treatment. Aflatoxin administration produced a significant (control positive group) downregulation of TNFα, APE1, and NQO 1 and a non-significant downregulation of OGG1, as opposed to the levels in the control negative group. The treatments (camel milk and silymarin) significantly upregulated the gene expression of APE1 and NQO 1 compared to the levels in the positive control group. OGG1 was insignificantly upregulated, as opposed to the levels in the control negative group. TNFα was significantly upregulated in the silymarin group compared to the levels in the control negative and positive groups.
Figure 5. Ameliorative effects of camel milk and silymarin on gene expression in liver tissues. The gene expression (fold change) was calculated relative to the control negative group (calibrator). (A) Fold change of APE1 gene, (B) Fold change of TNFα gene, (C) Fold change of OGG1 gene, and (D) Fold change of NQA gene. AFB: Aflatoxin, CM: camel milk, SILY: Silymarin.
As demonstrated in Figure 6, the findings of gene expression were assessed by qRT-PCR, normalized to β-actin mRNA of the testis tissue of the experimental groups at 15 days post-treatment. As opposed to the control negative group, the injection of aflatoxin resulted in a substantial upregulation of TNFα mRNA expression. Camel milk and silymarin administration produced significant downregulation of LHR1, StAR1, and TNFα mRNA expression in comparison with the control negative group.
Figure 6. Ameliorative effects of camel milk and silymarin on gene expression in testicular tissues. The gene expression (fold change) was calculated relative to the control negative group (calibrator). (A) Fold change of StAR1 gene, (B) Fold change of TNFα gene, and (C) Fold change of LHR gene. AFB: Aflatoxin, CM: camel milk, SILY: Silymarin.
Previous research has identified the harmful effects of AFB1 on the liver and kidneys, suppression of regular growth, modifications to developmental and reproductive factors, immunotoxicity, and alterations to the gut microbiome of rodents (Li et al., 2022; Sobral et al., 2022). Adverse alterations in renal and hepatic biochemical markers frequently point to liver damage linked to aflatoxin in animals (Aleissa et al., 2020; Hernandez-Valdivia et al., 2021). The present study demonstrated the adverse effects of AF on leukocytic count, which align with Uluişik et al. (2020), Kılıç et al. (2022), and Hassaneen et al. (2023), who reported that compared to the control group, the aflatoxicosis group had a significantly decreased WBC, lymphocyte, and monocyte count. However, these findings could be explained by aflatoxin’s harm to hematopoietic tissue (Pepeljnjak et al., 2003).
There are numerous documented benefits of camel milk, including antiviral, antibacterial, antitumor, antifungal, antioxidant, anti-inflammatory, hypoglycemic, and anti-cancer properties (Behrouz et al., 2022). When given orally for 15 days following treatment, camel milk helped to mitigate the detrimental impact of AFB1 on leukocytic numbers. These findings were in line with Khan (2017), who found that feeding camel milk to mice reduced the leukopenic effect of cyclophosphamide. These results may be attributed to the antioxidant properties of camel milk (Kumarappan et al., 2010). Moreover, Rahman et al. (2005) reported that camel milk contains high levels of vitamins C, A, E, zinc, and magnesium (Abu Zeid et al., 2019). These vitamins and minerals have an antioxidant effect. Several enzymes in the body have been found to be associated with zinc, which can prevent cell damage by strengthening the antioxidant system and its various functions (Ozdemir and Inanc, 2005; Abu Zeid et al., 2019).
Numerous studies have documented the antiviral, anti-fibrotic, anti-proliferative, immunomodulatory, and antioxidant qualities of silymarin. It also has an impact on DNA and RNA synthesis. Moreover, silymarin keeps the hepatocyte membrane intact and prevents harmful compounds or xenobiotics from entering the cell (Karimi et al., 2011). Its supplementation has increased the WBC count in quail (Khazaei et al., 2022). The curative and preventive benefits of silymarin on rat immunological toxicity caused by chlorpyrifos were described by El Elaimy et al. (2013). Since silymarin can stop the generation of free radicals during the metabolism of harmful substances, it possesses hepatoprotective and antioxidant properties (El-Gendy et al., 2024; Zalat et al., 2021).
The liver’s ability to detoxify the body and eliminate contaminants and impurities makes it one of the most important organs in the body (Khan, 2006). The serum biochemical markers, particularly the levels of serum proteins and AST and ALT activities, show how AFB1 affects the liver. Because serum AST and ALT are located in the cytoplasm and are consequently discharged into circulation following cellular damage, they are the most sensitive markers used in the diagnosis of liver damage. The extracellular turnover of these marker enzymes, their subsequent release of proteins, methods of cellular injury, and mechanisms of neoplastic processes are all reflected in the analysis of Jahan et al. (2011). These serum enzyme activity findings are consistent with El-Bahr (2015), Abdel-Wahhab et al. (2016), Abdel-Daim et al. (2021), and Hassaneen et al. (2023), who demonstrated that rats given AFB1 showed significantly higher liver enzymes (ALT and AST) than control. Increases in serum ALT and AST activity are known to be indicative of liver injury.
In this investigation, post-treatment 15 days with camel milk or silymarin to rats was observed to reduce the rise in serum AST and ALT activity brought on by AFB1 treatment. Our findings corroborated those of Althnaian et al. (2013), who observed that camel milk administration to rats reduced the rise in serum AST and ALT activity brought on by CCl4 treatment. This might be due to their antioxidant and anti-inflammatory properties. Legrand et al. (2005) reported that the distinctive anti-inflammatory and antioxidant properties of CM can be explained by its high lactoferrin content. El Mesallamy et al. (2011) demonstrated that in comparison to mice treated with NDEA, silymarin administration significantly decreased blood ALT, AST, and GGT activity. These results may be attributed to the antioxidant effect of silymarin. Silymarin may help the liver’s antioxidant defense by increasing hepatic glutathione (Vargas-Mendoza et al., 2014). In addition, the body weight, histological damage, serum ALT, AST, BUN, SCr, and tissue NO levels were dramatically returned to normal following 7-day post-treatment with silymarin administered 1 h after APAP injection (Bektur et al., 2016). Pradeep et al. (2007) reported that after receiving a single dose of diethyl nitrosamine via injection, the elevated activity of AST and ALT in serum was considerably reduced after 30 days of silymarin treatment.
This study’s results that serum proteins decrease albumin by administration of AFB1 agree with Abdel-Wahhab and Aly (2005), Abdel-Daim et al. (2020a), and Abdel-Daim et al. (2020b), who found that whereas aflatoxin therapy significantly decreased total protein, albumin, and cholesterol, it significantly increased ALT, AST, alkaline phosphatase, total bilirubin, and urea. Total protein levels were significantly lower in rats exposed to AFB1 than in the negative group (El-Bahr, 2015). These could be brought on by AFB1, which disrupts the process of protein biosynthesis by creating adducts with proteins, DNA, and RNA, suppressing the production of RNA and DNA-dependent RNA polymerase activity and inducing degranulation of the endoplasmic reticulum (Mohajeri et al., 2018). In this study, rats given camel milk as a post-treatment for 15 days showed an increase in their serum albumin concentration. These outcomes were accepted by Althnaian et al. (2013), who demonstrated that when compared to rats that got CCl4 alone, rats that received camel milk either alone or in combination showed a significant rise in serum albumin concentration. When camel milk is administered to both humans and animals, there is a potential correlation between the rise in plasma protein thiol activities and the reduction in lipid peroxidation processes, which leads to an increase in albumin concentration (Al-Hashem et al., 2009; Al-Fartosi et al., 2012). Moreover, this might be because of their dietary standards or because of their higher plasma protein thiol activities and lower lipid peroxidation (Sadek et al., 2016; Al-Fartosi et al., 2012). On the other hand, it has been documented that silymarin’s hepatoprotective mechanisms include an increase in hepatic protein synthesis (Popoola et al., 2022; Bijak et al., 2014). Moreover, silymarin’s capacity to lower inflammation (Bahmani et al., 2015) and its antioxidant qualities (Shaker et al., 2010), which actively lower reactive oxygen species and prevent cellular damage, are responsible for the decrease in the concentration of liver enzymes in the blood. It has also been demonstrated that silymarin stimulates RNA polymerase I activity, which in turn promotes protein production in hepatocytes (Vargas-Mendoza et al., 2014).
The aflatoxin group showed a significant decline according to the serum ferritin data. The results of Stoltzfus et al. (2004) affirm these results, stating that a drop in serum ferritin leads to a decrease in serum iron. AFB1 caused a decrease in serum iron, total iron-binding capacity, utilized iron-binding capacity, and transferrin levels (Hassan et al., 2020). However, CEA and AFP are specific markers for liver cancer. Reactive oxygen species are produced more frequently by AFB1, which damages DNA and causes cancer (Albadrani et al., 2024a and b; Huang et al., 2020). The rise in AFP and CEA concentrations is in line with a previous investigation that discovered rats which consumed AFB1 might elevate these levels (Abdel-Wahhab et al., 2006; Abdel-Wahhab et al., 2010; Abdel-Wahhab et al., 2020). According to the results of the current investigation, camel milk and silymarin lower the AFP and CEA levels in rat serum. This agrees with Hassaneen et al. (2023), who reported a substantial drop in AFP and CEA in groups given silymarin and camel milk.
Leydig cells create testosterone, which is necessary for the maintenance of testicular function and the control of spermatogenesis (Payne and Youngblood, 1995; Walker, 2011). The testosterone level analysis results from this study were astonishingly compatible with those of several other researchers. Owumi et al. (2020) revealed that in comparison to control rats, exposure to AFB1 alone caused a considerable drop in the serum levels of testosterone, FSH, and LH. It also caused a concurrent dip in the activities of G6PD, LDH, ACP, and ALP in the testicular tissue. This reduction in testosterone level might be attributed to decreased testes weights reported by Salem et al. (2001) in rabbits. The ameliorative AFB1 effect on testosterone levels is amplified by camel milk and silymarin in this study, which raises the hormone’s serum level. These outcomes concurred with Zakaria et al. (2016) and Gad et al. (2018) that testicular and serum testosterone levels significantly increased in male rats fed camel milk. Rats fed CM produced increased reproductive hormones, had more sperm, and gained weight in their reproductive organs (Zakaria et al., 2016). The high zinc content of camel milk (Zakaria et al., 2016) may be the cause for these outcomes, as zinc is necessary to sustain serum testosterone levels (Shahraki et al., 2015). Zinc deficiency prevents the pituitary gland releasing follicular stimulating and luteinizing hormones, which stimulate the production of testosterone (Egwurugwu et al., 2013). Furthermore, zinc also contributes to the release and operation of the male hormone testosterone through the enzymes that control the arachidonic acid cascade (Boland and Lonergan, 2003). Abedi et al. (2016) discovered that silymarin increased rat testosterone, ascribing this increase to silymarin’s antioxidant properties. Additionally, milk thistle seeds greatly increased the quality and fertility of semen and the concentration of testosterone in rabbit bucks (Attia et al., 2017). Moreover, Faraji et al. (2019) reported that reversing testosterone levels in mice was more possible with silymarin than the cadmium chloride group.
The serum biochemical markers were corroborated by the liver’s histopathology research findings. The findings of the liver pathological changes in the group treated with aflatoxin are consistent with El-Bahr (2015), who found that rats fed AFB1 showed modified lobular architecture and moderate-to-severe degenerative changes in their livers, marked by hepatocytes that seemed to be in vacuoles and edema. In most hepatocytes, there were a significant number of dispersed, isolated necrotic cells (apoptotic cells). Histopathological and ultrastructural analyses of the liver cells of aflatoxicated rats revealed extensive vacuolar degeneration and necrosis signals (Ali et al., 2021; Khatoon et al., 2024).
The liver pathological changes in the silymarin post-treated group parallel the findings of Ahmad et al. (2002), who demonstrated that thioacetamide therapy was significantly reversed in rat livers after receiving post-treatment with Jigreen and silymarin for 21 days. This was indicated by normal central vein, hepatic cells with well-preserved cytoplasm, and a conspicuous nucleus and nucleolus. Rat liver sections treated with silymarin showed a modest improvement in hepatocytes compared to the NDEA-treated group, with fewer cytomegalic, vacuolated hepatocytes and reduced prominence and nuclear vesiculation (El Mesallamy et al., 2011). This improvement might be explained by silymarin’s ability to effectively lower hepatocytes’ intracellular ROS levels, reducing oxidative stress-induced cellular damage. Additionally, it was discovered that silymarin therapy increased hepatic cell proliferation, indicating that improved liver regeneration might aid in replacing the injured liver cells (Wu et al., 2008). On the other hand, the pathological liver changes in the camel milk post-treated group resemble the findings of Ibrahim et al. (2017), who reported that hepatocytes in treated EVOO and camel milk groups were fully protected and that the hepatic architecture was more normal than acetaminophen (APAP)-induced liver toxicity group in mice. Hepatic tissues from rabbits given camel milk exhibited a notable build-up of macrophages and lymphocytes in the parenchyma, granulomatous lesion, and fibrosis (Saleem et al., 2021).
The results of testicular pathological changes at different stages of life are consistent with Laciakova et al. (1995), who demonstrated how long-term exposure to aflatoxins in pigs and rats results in spermatogenic epithelial cell dystrophy and other histological abnormalities in the male reproductive system. Additionally, anomalies in sperm morphology, testis histology, and meiotic chromosomes have been connected to aflatoxin exposure (Sinha and Prasad, 1990). Aboelhassan et al. (2018) revealed that AFB1 treatment resulted in some seminiferous tubules in the testis degenerating compared to the control group. The seminiferous tubule basement membrane buckled, and the quantity of spermatogenic cells decreased, indicating this degeneration. The histological architectural framework of treated rats exhibited significant alterations, as evidenced by necrosis of the tubules in seminiferous and inadequate sperm in the testis, lumen, or epididymis of AFB1 (Owumi et al., 2022). The testicular pathological changes in the camel milk post-treated group parallel the findings of Mohamed et al. (2019), who reported that using CM reversed the toxic effects of fenpropathrin by blocking the action of apoptosis via the caspase 3 and P53 pathways—crucial for the repair of tissue in the testes and other organs. CM group male rats dominated the entirely typical structure of the germinal epithelium in the seminiferous tubules and interstitial tissues, which showed active spermatogenesis and consisted of a regular arrangement of all forms of germ and Sertoli cells (Mohammad et al., 2023). The testicular pathological changes in the silymarin post-treated group resemble the findings of Faraji et al. (2019), who reported that, when comparing the silymarin + cadmium chloride group to the cadmium chloride group in mice, these histological alterations were significantly recovered. Comparing the silymarin-only group to the control group, measurements were made of the diameter of the seminiferous tubule wall, the spermatogonial nucleus, the density of sperm in the lumen, and the regularity of the germ. Additionally, in comparison to the control group, there was a decrease in the interstitial tissue and germinal epithelium vacuolation in the silymarin group. Following the administration of CDDP, silymarin corrected a significant decrease in serum levels of testosterone as well as a significant injury to the testicles and epididymis (El-Hameed et al., 2021).
TNF-α is the first and most important inflammatory mediator in the genesis of inflammation (Cruceriu et al., 2020; Jang et al., 2021). TNF-α and NF-B/p65 pathways produce pro-inflammatory cytokines such as IL-6 and iNOS, as well as adhesion molecules that encourage leukocyte migration to the site of inflammation (Arbab et al., 2017; Giridharan and Srinivasan, 2018). TNF-α gene expression in liver tissue was downregulated in the present investigation as a result of aflatoxin treatment. However, TNF-α gene expression was markedly elevated in camel milk and silymarin after a 15-day post-treatment. These results are consistent with Jiang et al. (2015), who revealed that the expression of IL-2, IL-4, IL-6, IL-10, IL17, IFN- γ, and TNF- α mRNA was commonly downregulated in the duodenum, jejunum, and ileum of broilers in the AFB1 group. The expression of TNF-α, IFN-γ, and IL-4 was decreased in rats exposed to AFB1, in accordance with Qian et al. (2014).
The aflatoxin-treated group exhibited considerable downregulation of the OGG1 and APE1 base excision repair genes, with OGG1 showing just a little downregulation compared to the control group. These findings support the conclusions of Liu et al. (2018), who showed that AFB1 significantly downregulated the expression of BER genes OGG1 and XRCC1.
In order to protect wholesome cells from oxidative stress and malignancy, NQO1 is essential. Despite the biological activities of this “cell protector,” NQO1’s antioxidant role was paradoxically demonstrated by the evidence demonstrating that genetic variation or disruption of the gene increased the likelihood of chemical-induced toxicity and cancer (Su et al., 2012; Yang et al., 2014; Aboubakr et al., 2021). NQO1 was notably downregulated in the group treated with aflatoxin in contrast to the other groups. These results are in line with Wang et al. (2022), who demonstrated that the livers of aflatoxicated mice had considerably lower mRNA levels of downstream target genes, including SOD, CAT, HO-1, and NQO1. The livers of aflatoxicated mice showed lower expression of the Nrf2, HO-1, GCLC, NQO1, and SOD1 genes (Rajput et al., 2021). In addition, in this study, silymarin post-treated to aflatoxicated rats for 15 days showed significant upregulation of NQO1 gene expression. Owumi et al. (2020) concluded that exposure to AFB1 alone obviously enhanced NO, TNF-a, and IL-1b levels in addition to MPO activity and also decreased the anti-inflammatory cytokine, IL-10, in the epididymis, testes, and hypothalamus of the treated rats. This result suggests the induction of inflammation. It is commonly known that uncontrolled testis production of cytokines promote inflammatories including TNF-a and IL-1b, which are detrimental to sperm formation and cause male infertility (Fijak et al., 2018). Nonetheless, TNF-α gene expression was significantly downregulated in the groups that received post-treatment camel milk and silymarin for 15 days. These findings agree with Belhan et al. (2021), who found that the testicular tissue’s expression levels of 8-hydroxy-2-deoxyguanosine (8-OHdG) and tumor necrosis factor alpha (TNF-α) greatly rose in the torsion/detorsion groups, and that these levels decreased when silymarin was administered.
StAR is usually recognized as the steroidogenesis’ rate-limiting stage and is required for the transport of cholesterols into mitochondria (Hasegawa et al., 2000). The LHR-mediated steroidogenic pathway regulates both the expression and activation of StAR in Leydig cells (Adedara et al., 2014; Zeng et al., 2018). The downregulation of StAR1 resulting from the administration of camel milk and silymarin for 15 days following aflatoxin exposure was statistically significant compared to the negative group. Additionally, after 15 days with both treatments, there was a considerable downregulation of LHR1 compared to the negative group.
The results of this study indicate that camel milk and silymarin reduce the adverse effects of AFB1 on the rat model’s leukogram, AST and ALT activity, serum proteins, ferritin, alpha-fetoprotein, carcinoembryonic antigen, liver pathology, and gene expression of tumor necrosis factor (TNF-α), antioxidant gene [NAD (P) H quinone oxidoreductase 1 (NQO1)], and base excision repair genes (APE1 and OGG1) in the liver tissue, as well as testosterone and the expression of genes related to luteinizing hormone receptor, steroidogenic acute regulatory protein, and tumor necrosis factor α with normal testicular architecture. Thus, it is possible to conclude that consuming camel milk and silymarin lessened the detrimental effects of AFB1 on the rat model’s leukocytic count, blood biochemistry, liver and testes pathology, and the expression of certain genes.
The original contributions presented in the study are included in the article/supplementary material; further inquiries can be directed to the corresponding author.
The Faculty of Veterinary Medicine at Alexandria University’s Ethical Committee gave its approval for the current study to use laboratory animals for sampling. The study was conducted in accordance with local legislation and institutional requirements.
NH: conceptualization, investigation, methodology, and writing–original draft. SH: conceptualization, data curation, formal analysis, investigation, methodology, software, supervision, writing–original draft, and writing–review and editing. AE: conceptualization, data curation, formal analysis, investigation, methodology, software, supervision, writing–original draft, and writing–review and editing. GA: conceptualization, formal analysis, funding acquisition, and writing–original draft. MA-G: conceptualization, data curation, formal analysis, funding acquisition, and writing–original draft. ZM: conceptualization, data curation, formal analysis, and writing–original draft. SF: conceptualization, data curation, formal analysis, investigation, methodology, software, supervision, writing–original draft, and writing–review and editing. EE-D: conceptualization, data curation, formal analysis, and writing–original draft. HS: conceptualization, data curation, formal analysis, and writing–original draft.
The authors declare that financial support was received for the research, authorship, and/or publication of this article. This study was supported by Princess Nourah bint Abdulrahman University Researchers Supporting Project number (PNURSP2025R30), Princess Nourah bint Abdulrahman University, Riyadh, Saudi Arabia. This research was funded by the Researchers Supporting Project number (RSPD2025R811), King Saud University, Riyadh, Saudi Arabia.
The authors declare that the research was conducted in the absence of any commercial or financial relationships that could be construed as a potential conflict of interest.
The reviewer EH declared a shared affiliation with the author HS to the handling editor at the time of review.
The authors declare that no Generative AI was used in the creation of this manuscript.
All claims expressed in this article are solely those of the authors and do not necessarily represent those of their affiliated organizations, or those of the publisher, the editors and the reviewers. Any product that may be evaluated in this article, or claim that may be made by its manufacturer, is not guaranteed or endorsed by the publisher.
Abdel-Daim, M. M., Abdeen, A., Jalouli, M., Abdelkader, A., Megahed, A., Alkahtane, A., et al. (2021). Fucoidan supplementation modulates hepato-renal oxidative stress and DNA damage induced by aflatoxin B1 intoxication in rats. Sci. Total Env. 768, 144781. doi:10.1016/j.scitotenv.2020.144781
Abdel-Daim, M. M., Dawood, M. A., Aleya, L., and Alkahtani, S. (2020a). Effects of fucoidan on the hematic indicators and antioxidative responses of Nile tilapia (Oreochromis niloticus) fed diets contaminated with aflatoxin B1. Env. Sci. Poll. Res. 27 (11), 12579–12586. doi:10.1007/s11356-020-07854-w
Abdel-Daim, M. M., Dawood, M. A., AlKahtane, A. A., Abdeen, A., Abdel-Latif, H. M., Senousy, H. H., et al. (2020b). Spirulina platensis mediated the biochemical indices and antioxidative function of Nile tilapia (Oreochromis niloticus) intoxicated with aflatoxin B1. Toxicon 184, 152–157. doi:10.1016/j.toxicon.2020.06.001
Abdel-Wahhab, M., and Aly, S. (2005). Antioxidant property of Nigella sativa (black cumin) and Syzygium aromaticum (clove) in rats during aflatoxicosis. J. Appl. Toxicol. Int. J. 25 (3), 218–223. doi:10.1002/jat.1057
Abdel-Wahhab, M. A., Ahmed, H. H., and Hagazi, M. M. (2006). Prevention of aflatoxin B1-initiated hepatotoxicity in rat by marine algae extracts. J. Appl. Toxicol. Int. J. 26 (3), 229–238. doi:10.1002/jat.1127
Abdel-Wahhab, M. A., El-Nekeety, A. A., Hathout, A. S., Salman, A. S., Abdel-Aziem, S. H., Sabry, B. A., et al. (2020). Bioactive compounds from Aspergillus niger extract enhance the antioxidant activity and prevent the genotoxicity in aflatoxin B1-treated rats. Toxicon 181, 57–68. doi:10.1016/j.toxicon.2020.04.103
Abdel-Wahhab, M. A., Hassan, N. S., El-Kady, A. A., Khadrawy, Y. A., El-Nekeety, A. A., Mohamed, S. R., et al. (2010). Red ginseng extract protects against aflatoxin B1 and fumonisins-induced hepatic pre-cancerous lesions in rats. Food Chem. Toxicol. 48 (2), 733–742. doi:10.1016/j.fct.2009.12.006
Abdel-Wahhab, M. A., Salman, A. S., Ibrahim, M. I., El-Kady, A. A., Abdel-Aziem, S. H., Hassan, N. S., et al. (2016). Curcumin nanoparticles loaded hydrogels protects against aflatoxin B1-induced genotoxicity in rat liver. Food Chem. Toxicol. 94, 159–171. doi:10.1016/j.fct.2016.06.005
Abedi, H., Jahromi, H. K., Hashemi, S. A., Jashni, H. K., Jahromi, Z. K., and Pourahmadi, M. (2016). The effect of silymarin on spermatogenesis process in rats. Int. Sci. J. Med. Res. Health Sci. 5 (6), 146–150.
Abenavoli, L., Capasso, R., Milic, N., and Capasso, F. (2010). Milk thistle in liver diseases: past, present, future. Phytother. Res. 24 (10), 1423–1432. doi:10.1002/ptr.3207
Aboelhassan, D. M., Hafiz, N. A., Ghaly, I. S., Shabana, M. E., Farag, I. M., Abdalla, A. M., et al. (2018). The role of Moringa Oleifera extract in maintenance of brain and testis tissues against Aflatoxin B1 (Afb1) toxicity on light of molecular genetic and histological studies in rats. World J. Pharm. Res. 7, 109–140. doi:10.20959/wjpr20188-11943
Aboubakr, M., Elshafae, S. M., Abdelhiee, E. Y., Fadl, S. E., Soliman, A., Abdelkader, A., et al. (2021). Antioxidant and anti-inflammatory potential of thymoquinone and lycopene mitigate the chlorpyrifos-induced toxic neuropathy. Pharmaceuticals 14 (9), 940. doi:10.3390/ph14090940
Abu Zeid, E. H., El Sharkawy, N. I., Moustafa, G. G., Anwer, A. M., and Al Nady, A. G. (2019). Camel milk mitigates hemato-immunotoxic impacts of fenpropathrin oral intoxication in male rat. Alex. J. Vet. Sci. 63 (2), 77. doi:10.5455/ajvs.66559
Adedara, I. A., Nanjappa, M. K., Farombi, E. O., and Akingbemi, B. T. (2014). Aflatoxin B1 disrupts the androgen biosynthetic pathway in rat Leydig cells. Food Chem. Toxicol. 65, 252–259. doi:10.1016/j.fct.2013.12.027
Afifi, M. E. (2010). Effect of camel's milk on Cisplatin-induced nephrotoxicity in Swiss Albino mice. Am. J. Biochem. Biotechnol. 6 (2), 141–147. doi:10.3844/ajbbsp.2010.141.147
Agrawal, R. P., Budania, S., Sharma, P., Gupta, R., Kochar, D. K., Panwar, R. B., et al. (2007). Zero prevalence of diabetes in camel milk consuming Raica community of north-west Rajasthan, India. Diabetes Res. Clin. Pract. 76 (2), 290–296. doi:10.1016/j.diabres.2006.09.036
Agrawal, R. P., Swami, S. C., Beniwal, R., Kochar, D. K., and Kothari, R. P. (2002). Effect of CM on glycemic control, risk factors and diabetes quality of life in type-1 diabetes: a randomized prospective controlled study. Int. J. Diabetes Develop. Counties 22, 70–74.
Ahmad, A., Pillai, K., Najmi, A. K., Ahmad, S. J., Pal, S., and Balani, D. (2002). Evaluation of hepatoprotective potential of jigrine post-treatment against thioacetamide induced hepatic damage. J. Ethnopharmacol. 79 (1), 35–41. doi:10.1016/S0378-8741(01)00349-X
Al-Asmari, A. K., Abbasmanthiri, R., Al-Elawi, A. M., Al-Horaib, G., Al-Sadoon, K., and Al-Asmari, B. A. (2017). Effect of camel milk against renal toxicity in experimental rats. Pak. J. Pharm. Sci. 30, 561–565.
Albadrani, G. M., Altyar, A. E., Kensara, O. A., Haridy, M. A., Zaazouee, M. S., Elshanbary, A. A., et al. (2024a). Effects of alfa lipoic acid and coenzyme Q10 treatment on AFB1-induced oxidative, inflammatory, and DNA damages in rats. Toxicon 249, 108083. doi:10.1016/j.toxicon.2024.108083
Albadrani, G. M., Altyar, A. E., Kensara, O. A., Haridy, M. A., Zaazouee, M. S., Elshanbary, A. A., et al. (2024b). Antioxidant, anti-inflammatory, and anti-DNA damage effects of carnosic acid against aflatoxin B1-induced hepatic, renal, and cardiac toxicities in rats. Toxicol. Res. 13 (3), tfae083. doi:10.1093/toxres/tfae083
Albarrak, S. M. (2023). Evaluation of somatic cells count, antioxidants and antimicrobial proteins in milk samples obtained from different breeds of the dromedary camel. Int. J. Vet. Sci. 12 (3), 462–469. doi:10.47278/journal.ijvs/2022.221
Aleissa, M. S., Alkahtani, S., Abd Eldaim, M. A., Ahmed, A. M., Bungău, S. G., Almutairi, B., et al. (2020). Fucoidan ameliorates oxidative stress, inflammation, DNA damage, and hepatorenal injuries in diabetic rats intoxicated with aflatoxin B1. Oxid. Med. Cell. Longev. (1). doi:10.1155/2020/9316751
Al-Fartosi, K. G., Khuon, O. S., and Al-Tae, H. I. (2011). Protective role of camel’s milk against paracetamol induced hepatotoxicity in male rats. Int. J. Res. Pharm. Biomed. Sci. 2, 1795–1799.
Al-Fartosi, K. G., Majid, A., Auda, M. A., and Hussein, M. H. (2012). The role of Camel’s milk against some oxidant-antioxidant markers of male rats treated with CCl4. Int. J. Res. Pharm. Biomed. Sci. 3 (1), 385–389.
Al-Hashem, F. (2009). Camel’s milk protects against aluminum chloride-induced toxicity in the liver and kidney of white albino rats. Am. J. Biochem. Biotechnol. 5 (3), 98–108. doi:10.3844/ajbbsp.2009.98.108
Al-Hashem, F., Mohammad, D., Bashir, N., Mohammad, A., Riyadh, E., Mohammad, K., et al. (2009). Camel's milk protects against cadmium chloride induced toxicity in white albino rats. Am. J. Pharmacol. Toxicol. 4 (3), 107–117.
Ali, F. A. Z., Abdel-Maksoud, F. M., Abd Elaziz, H. O., Al-Brakati, A., and Elmahallawy, E. K. (2021). Descriptive histopathological and ultrastructural study of hepatocellular alterations induced by aflatoxin B1 in rats. Animals 11 (2), 509. doi:10.3390/ani11020509
Ali, M. Z., Sana, S., Sheikh, A. A., and Maheen, Z. (2022). Molecular characterization of toxigenic Aspergillus flavus isolated from sick broiler lungs and risk factors analysis. Pak. Vet. J. 42 (2), 194–200. doi:10.29261/pakvetj/2022.037
Alorainy, M. S., Alhajuj, A. M., and Fathi, S. M. (2023). Biochemical identification, direct molecular detection, and antimicrobial agent resistance of Staphylococcus aureus isolated from raw camel milk in Al Madinah Region, Saudi Arabia. Int. J. Vet. Sci. 12 (5), 740–745. doi:10.47278/journal.ijvs/2023.048
Althnaian, T., Albokhadaim, I., and El-Bahr, S. M. (2013). Biochemical and histopathological study in rats intoxicated with carbontetrachloride and treated with camel milk. Springer Plus 2(1), 57–7. doi:10.1186/2193-1801-2-57
Altyar, A. E., Kensara, O. A., Noreldin, A. E., Albadrani, G. M., El-Demerdash, F. M., Sayed, A. A., et al. (2024). Spirulina platensis ameliorates hepatic oxidative stress and DNA damage induced by aflatoxin B1 in rats. Toxicon 237, 107553. doi:10.1016/j.toxicon.2023.107553
Altyar, A. E., Kensara, O. A., Sayed, A. A., Aleya, L., Almutairi, M. H., Zaazouee, M. S., et al. (2023). Acute aflatoxin B1-induced hepatic and cardiac oxidative damage in rats: ameliorative effects of morin. Heliyon 9 (11), e21837. doi:10.1016/j.heliyon.2023.e21837
Arafa, H. M. (2009). Uroprotective effects of curcumin in cyclophosphamide-induced haemorrhagic cystitis paradigm. Basic Clin. Pharmacol. Toxicol. 104 (5), 393–399. doi:10.1111/j.1742-7843.2009.00379.x
Arbab, M., Tahir, S., Niazi, M. K., Ishaq, M., Hussain, A., Siddique, P. M., et al. (2017). TNF-α genetic predisposition and higher expression of inflammatory pathway components in keratoconus. Investig. Ophthalmol. Vis. Sci. 58 (9), 3481–3487. doi:10.1167/iovs.16-21400
Attia, Y. A., Hamed, R. S., Bovera, F., Abd El, A. E. H. E., Al-Harthi, M. A., and Shahba, H. A. (2017). Semen quality, antioxidant status and reproductive performance of rabbits bucks fed milk thistle seeds and rosemary leaves. Anim. Reprod. Sci. 184, 178–186. doi:10.1016/j.anireprosci.2017.07.014
Bahmani, M., Shirzad, H., Rafieian, S., and Rafieian-Kopaei, M. (2015). Silybum marianum: beyond hepatoprotection. Altern. Med. 20 (4), 292–301. doi:10.1177/2156587215571116
Bancroft, J. D., and Gamble, M. (2008). Theory and practice of histological techniques. Elsevier health sciences.
Behrouz, S., Saadat, S., Memarzia, A., Sarir, H., Folkerts, G., and Boskabady, M. H. (2022). The antioxidant, anti-inflammatory and immunomodulatory effects of camel milk. Front. Immunol. 13, 855342. doi:10.3389/fimmu.2022.855342
Bektur, N. E., Sahin, E., Baycu, C., and Unver, G. (2016). Protective effects of silymarin against acetaminophen-induced hepatotoxicity and nephrotoxicity in mice. Toxicol. Ind. Health 32 (4), 589–600. doi:10.1177/0748233713502841
Belhan, S., Yildirim, S., Kayikci, C., Kömüroğlu, A. U., Özdek, U., and Kuşçu, Y. (2021). Investigation of the effect of silymarin on oxidative DNA damageand inflammatory markers in ischemia/reperfusion injuryfollowing experimental testicular torsion/detorsion in rats. Turk. J. Zool. 45 (4), 267–276. doi:10.3906/zoo-2103-5
Bijak, M., Ponczek, M. B., and Nowak, P. (2014). Polyphenol compounds belonging to flavonoids inhibit activity of coagulation factor X. Int. J. Biol. Macromol. 65, 129–135. doi:10.1016/j.ijbiomac.2014.01.023
Boland, M. P., and Lonergan, P. (2003). Trace minerals in production and reproduction in dairy cows. Adv. Dairy Technol. 15, 319–330.
Cruceriu, D., Baldasici, O., Balacescu, O., and Berindan-Neagoe, I. (2020). The dual role of tumor necrosis factor-alpha (TNF-α) in breast cancer: molecular insights and therapeutic approaches. Cell. Oncol. 43, 1–18. doi:10.1007/s13402-019-00489-1
Dallak, M. (2009). Camel's milk protects against cadmium chloride-induced hypocromic microcytic anemia and oxidative stress in red blood cells of white albino rats. Am. J. Pharmacol. Toxicol. 4 (4), 136–143. doi:10.3844/ajptsp.2009.136.143
Egwurugwu, J. N., Ifedi, C. U., Uchefuna, R. C., Ezeokafor, E. N., and Alagwu, E. A. (2013). Effects of zinc on male sex hormones and semen quality in rats. J. Physiol. Sci. 28 (1), 17–22.
El-Bahr, S. (2015). Effect of curcumin on hepatic antioxidant enzymes activities and gene expressions in rats intoxicated with aflatoxin B1. Phytother. Res. 29 (1), 134–140. doi:10.1002/ptr.5239
Elblehi, S. S., El Euony, O. I., and El-Nahas, A. F. (2019). Partial ameliorative effect of Moringa leaf ethanolic extract on the reproductive toxicity and the expression of steroidogenic genes induced by subchronic cadmium in male rats. Environ. Sci. Poll. Res. 26, 23306–23318. doi:10.1007/s11356-019-05607-y
El Elaimy, I. A., Ibrahim, H. M., Abdel Gafaar, F. R., and Al-Awthan, Y. S. (2013). Protective and curative effect of silymarin against chlorpyrifos-induced immunotoxicity in rats. Delta J. Sci. 36 (2), 106–115. doi:10.21608/djs.2013.139662
El-Gendy, H. F., Khalifa, H. K., Omran, A., Korany, R. M., Selim, S., Hussein, E., et al. (2024). Unveiling the potential of silymarin, spirulina platensis, and chlorella vulgaris towards cardiotoxicity via modulating antioxidant activity, inflammation, and apoptosis in rats. Life 14 (10), 1289. doi:10.3390/life14101289
El-Hameed, A., Mahgoub, H. A., Awadin, W., and Elshaieb, A. (2021). The in vivo ameliorative effect of silymarin on cisplatin-associated ovarian and testicular histopathological and biochemical alterations. Mansoura Vet. Med. J. 22 (2), 65–71. doi:10.21608/mvmj.2021.56846.1025
El Mesallamy, H. O., Metwally, N. S., Soliman, M. S., Ahmed, K. A., and Abdel Moaty, M. M. (2011). The chemopreventive effect of Ginkgo biloba and Silybum marianum extracts on hepatocarcinogenesis in rats. Cancer Cell. Int. 11, 38–12. doi:10.1186/1475-2867-11-38
El-Nekeety, A. A., Abdel-Azeim, S. H., Hassan, A. M., Hassan, N. S., Aly, S. E., and Abdel-Wahhab, M. A. (2014). Quercetin inhibits the cytotoxicity and oxidative stress in liver of rats fed aflatoxin-contaminated diet. Toxicol. Rep. 1, 319–329. doi:10.1016/j.toxrep.2014.05.014
Faraji, T., Momeni, H. R., and Malmir, M. (2019). Protective effects of silymarin on testis histopathology, oxidative stress indicators, antioxidant defence enzymes and serum testosterone in cadmium-treated mice. Andrologia 51 (5), e13242. doi:10.1111/and.13242
Fijak, M., Pilatz, A., Hedger, M. P., Nicolas, N., Bhushan, S., Michel, V., et al. (2018). Infectious, inflammatory and ‘autoimmune’male factor infertility: how do rodent models inform clinical practice? Hum. Reprod. Update 24 (4), 416–441. doi:10.1093/humupd/dmy009
Gad, S. B., Fayed, A.-H. H., Hafez, M. H., and Abdel-Hafeiz, A. H. (2018). Does camel milk have a positive impact on rat reproductive functions? Alex. J. Vet. Sci. 59 (1), 134. doi:10.5455/ajvs.4340
Giridharan, S., and Srinivasan, M. (2018). Mechanisms of NF-κB p65 and strategies for therapeutic manipulation. J. Inflamm. Res. 11, 407–419. doi:10.2147/JIR.S140188
Gong, Z., Ye, H., Huo, Y., Wang, L., Huang, Y., Huang, M., et al. (2018). S-allyl-cysteine attenuates carbon tetrachloride-induced liver fibrosis in rats by targeting STAT3/SMAD3 pathway. Am. J. Transl. Res. 10 (5), 1337–1346.
Hasegawa, T., Zhao, L., Caron, K. M., Majdic, G., Suzuki, T., Shizawa, S., et al. (2000). Developmental roles of the steroidogenic acute regulatory protein (StAR) as revealed by StAR knockout mice. Mol. Endocrinol. 14 (9), 1462–1471. doi:10.1210/mend.14.9.0515
Hassan, A., Mansour, M., Sayed-ElAhl, R., El-Din, H. T., Awad, M., and Younis, E. (2020). Influence of selenium nanoparticles on the effects of poisoning with aflatoxins. Adv. Anim. Vet. Sci. 8(s2), 64–73. doi:10.17582/journal.aavs/2020/8.s2.64.73
Hassaneen, N. H., Hemeda, S. A., El Nahas, A. F., Fadl, S. E., and El-Diasty, E. M. (2023). Ameliorative effects of camel milk and silymarin upon aflatoxin B1 induced hepatic injury in rats. Sci. Rep. 13 (1), 15092. doi:10.1038/s41598-023-41586-4
Hassaneen, N. H., Hemeda, S. A., El Nahas, A. F., Fadl, S. E., and El-Diasty, E. M. (2024). Camel milk or silymarin could improve the negative effects that experimentally produced by aflatoxin B1 on rat’s male reproductive system. BMC Vet. Res. 20 (1), 108. doi:10.1186/s12917-024-03965-5
Hernandez-Valdivia, E., Valdivia-Flores, A., Cruz-Vazquez, C., Martinez-Saldaña, M., Quezada-Tristan, T., Rangel-Muñoz, E., et al. (2021). Diagnosis of subclinical aflatoxicosis by biochemical changes in dairy cows under field conditions. Pak. Vet. J. 41 (1), 33–38. doi:10.29261/pakvetj/2020.075
Huang, B., Chen, Q., Wang, L., Gao, X., Zhu, W., Mu, P., et al. (2020). Aflatoxin B1 induces neurotoxicity through reactive oxygen species generation, DNA damage, apoptosis, and S-phase cell cycle arrest. Int. J. Mol. Sci. 21 (18), 6517. doi:10.3390/ijms21186517
Ibrahim, M. A. B., Wani, F. A., and Rahiman, S. (2017). Hepatoprotective effect of olive oil and camel milk on acetaminophen-induced liver toxicity in mice. Int. J. Med. Sci. Public Health 6 (1), 186–194. doi:10.5455/ijmsph.2017.07092016614
Ijaz, M. U., Ishtiaq, A., Ehsan, N., Imran, M., and Zhu, G. (2022). Hepatoprotective potential of genkwanin against aflatoxin b1-induced biochemical, inflammatory and histopathological toxicity in rats. Pak. Vet. J. 42 (4), 493–498. doi:10.29261/pakvetj/2022.048
Jahan, M., Vani, G., and Shyamaladevi, C. (2011). Anti-carcinogenic effect of solanum trilobatum in diethylnitrosamine induced and phenobarbital promoted heaptocarcinogenesis in rats. Asian J. biochem. 6 (1), 74–81. doi:10.3923/ajb.2011.74.81
Jang, D.-i., Lee, A.-H., Shin, H.-Y., Song, H.-R., Park, J.-H., Kang, T.-B., et al. (2021). The role of tumor necrosis factor alpha (TNF-α) in autoimmune disease and current TNF-α inhibitors in therapeutics. Int. J. Mol. Sci. 22 (5), 2719. doi:10.3390/ijms22052719
Jiang, M., Peng, X., Fang, J., Cui, H., Yu, Z., and Chen, Z. (2015). Effects of aflatoxin B1 on T-cell subsets and mRNA expression of cytokines in the intestine of broilers. Int. J. Mol. Sci. 16 (4), 6945–6959. doi:10.3390/ijms16046945
Karimi, G., Vahabzadeh, M., Lari, P., Rashedinia, M., and Moshiri, M. (2011). Silymarin, a promising pharmacological agent for treatment of diseases. J. Basic Med. Sci. 14 (4), 308–317.
Khan, M. A. (2017). Immune potentiating and antitoxic effects of camel milk against cyclophosphamide-induced toxicity in BALB/C mice. Int. J. Health Sci. 11 (4), 18–22.
Khan, S. (2006). Evaluation of hyperbilirubinemia in acute inflammation of appendix: a prospective study of 45 cases. Kathmandu Univ. Med. J. 4 (3), 281–289.
Khatoon, A. A., Amin, S., Majeed, S. T., Gul, M. I., Arshad, M. K., Saleemi, A., et al. (2024). Dietary Chlorella vulgaris mitigates aflatoxin B1 toxicity in broiler chicks: toxicopathological, hematobiochemical and immunological perspectives. Toxicon 251, 108127. doi:10.1016/j.toxicon.2024.108127
Khazaei, R., Seidavi, A., and Bouyeh, M. (2022). A review on the mechanisms of the effect of silymarin in milk thistle (Silybum marianum) on some laboratory animals. Vet. Med. Sci. 8 (1), 289–301. doi:10.1002/vms3.641
Kılıç, K. D., Gökhan, A., Sözmen, E. Y., and Uysal, A. (2022). Liver histology and biochemistry of exposed newborn and infant rats with experimental aflatoxicosis. Pak. Vet. J. 42 (4), 453–460. doi:10.29261/pakvetj/2022.066
Kudayer, A. M., Alsandaqchi, A. T., Saleh, F. M., and Alwan, N. A. (2019). Toxic effect of aflatoxin B1 on heart, lung, and testis of male albino rats: histopathology study. IOP Conf. Ser. Mater. Sci. Eng. 571 (1), 012055. doi:10.1088/1757-899X/571/1/012055
Kumarappan, C. T., Vhand, B. S., Mandal, S. C., and Sengottuvel, T. (2010). Hepatoprotective effect of the poly phenolic extract from ichnocarpus fruits scens leaves. Deccan J. Pharmacol. 1, 1–16.
Laciakova, A., Vargova, M., and Laciak, V. (1995). Some mycotoxins causing health-problems in farm=animals. Biologia 50 (3), 293–295.
Legrand, D., Elass, E., Carpentier, M., and Mazurier, J. (2005). Lactoferrin: a modulator of immune and inflammatory responses. Cell. Mol. Life Sci. 62, 2549–2559. doi:10.1007/s00018-005-5370-2
Li, C., Liu, X., Wu, J., Ji, X., and Xu, Q. (2022). Research progress in toxicological effects and mechanism of aflatoxin B1 toxin. J. 10, e13850. doi:10.7717/peerj.13850
Liu, W., Wang, L., Zheng, C., Liu, L., Wang, J., Li, D., et al. (2018). Microcystin-LR increases genotoxicity induced by aflatoxin B1 through oxidative stress and DNA base excision repair genes in human hepatic cell lines. Env. Pollut. 233, 455–463. doi:10.1016/j.envpol.2017.10.067
Livak, K. J., and Schmittgen, T. D. (2001). Analysis of relative gene expression data using real-time quantitative PCR and the 2(-Delta Delta C(T)) Method. Methods 25 (4), 402–408. doi:10.1006/meth.2001.1262
Luceri, C., Bigagli, E., Femia, A. P., Caderni, G., Giovannelli, L., and Lodovici, M. (2018). Aging related changes in circulating reactive oxygen species (ROS) and protein carbonyls are indicative of liver oxidative injury. Toxicol. Rep. 5, 141–145. doi:10.1016/j.toxrep.2017.12.017
Meisel, H. (2005). Biochemical properties of peptides encrypted in bovine milk proteins. Curr. Med. Chem. 12 (16), 1905–1919. doi:10.2174/0929867054546618
Mohajeri, M., Behnam, B., Cicero, A. F., and Sahebkar, A. (2018). Protective effects of curcumin against aflatoxicosis: a comprehensive review. J. Cell. Physiol. 233 (4), 3552–3577. doi:10.1002/jcp.26212
Mohamad, R. H., Zekry, H. A., Al-Mehdar, O., Salama, S. E., El-Shaieb, A. A., El-Basmy, M. G., et al. (2009). Camel milk as an adjuvant therapy for the treatment of type 1 diabetes: verification of a traditional ethnomedical practice. J. Med. Food 12 (2), 461–465. doi:10.1089/jmf.2008.0009
Mohamed, A. A.-R., Abdellatief, S. A., Khater, S. I., Ali, H., and Al-Gabri, N. A. (2019). Fenpropathrin induces testicular damage, apoptosis, and genomic DNA damage in adult rats: protective role of camel milk. Ecotoxicol. Environ. Saf. 181, 548–558. doi:10.1016/j.ecoenv.2019.06.047
Mohammad, B. J., Al-Saaidi, J. A., and Al Zwean, D. H. (2023). The protective role of Camel milk against reprotoxicity, hepatotoxicity, and nephrotoxicity in aflatoxic-induced male rats. Res. J. Pharm. Technol. 16 (3), 1072–1078. doi:10.52711/0974-360X.2023.00179
Owumi, S. E., Adedara, I. A., Akomolafe, A. P., Farombi, E. O., and Oyelere, A. K. (2020). Gallic acid enhances reproductive function by modulating oxido-inflammatory and apoptosis mediators in rats exposed to aflatoxin-B1. Exp. Biol. Med. 245 (12), 1016–1028. doi:10.1177/1535370220936206
Owumi, S. E., Irozuru, C. E., Arunsi, U. O., Faleke, H. O., and Oyelere, A. K. (2022). Caffeic acid mitigates aflatoxin B1-mediated toxicity in the male rat reproductive system by modulating inflammatory and apoptotic responses, testicular function, and the redox-regulatory systems. J. Food Biochem. 46 (5), e14090. doi:10.1111/jfbc.14090
Ozdemir, G., and Inanc, F. (2005). Zinc may protect remote ocular injury caused by intestinal ischemia reperfusion in rats. Tohoku J. Exp. Med. 206 (3), 247–251. doi:10.1620/tjem.206.247
Pascual, C., Armesto, J., and Muriel, P. (1993). Effect of silymarin and silybinin on oxygen radicals. Drug Dev. Res. 29 (1), 73–77. doi:10.1002/ddr.430290109
Payne, A. H., and Youngblood, G. L. (1995). Regulation of expression of steroidogenic enzymes in Leydig cells. Biol. Reprod. 52 (2), 217–225. doi:10.1095/biolreprod52.2.217
Pepeljnjak, S., Petrinec, Z., Kovacic, S., and Segvic, M. (2003). Screening toxicity study in young carp (Cyprinus carpio L.) on feed amended with fumonisin B 1. Mycopathologia 156, 139–145. doi:10.1023/A:1022944927493
Popoola, A. B., Ademilusi, E. O., Adedeji, T. G., and Fasanmade, A. A. (2022). Effect of silymarin on blood coagulation profile and osmotic fragility in carbon tetrachloride induced hepatotoxicity in male Wistar rats. Toxicol. Rep. 9, 1325–1330. doi:10.1016/j.toxrep.2022.06.005
Pradeep, K., Mohan, C. V. R., Gobianand, K., and Karthikeyan, S. (2007). Silymarin modulates the oxidant–antioxidant imbalance during diethylnitrosamine induced oxidative stress in rats. Eur. J. Pharmacol. 560 (2-3), 110–116. doi:10.1016/j.ejphar.2006.12.023
Qian, G., Tang, L., Guo, X., Wang, F., Massey, M. E., Su, J., et al. (2014). Aflatoxin B1 modulates the expression of phenotypic markers and cytokines by splenic lymphocytes of male F344 rats. J. Appl. Toxicol. 34 (3), 241–249. doi:10.1002/jat.2866
Rahman, I., Biswas, S. K., Jimenez, L. A., Torres, M., and Forman, H. J. (2005). Glutathione, stress responses, and redox signaling in lung inflammation. Antioxid. Redox Signal. 7 (1-2), 42–59. doi:10.1089/ars.2005.7.42
Rajput, S. A., Shaukat, A., Wu, K., Rajput, I. R., Baloch, D. M., Akhtar, R. W., et al. (2021). Luteolin alleviates aflatoxinB1-induced apoptosis and oxidative stress in the liver of mice through activation of Nrf2 signaling pathway. Antioxidants 10 (8), 1268. doi:10.3390/antiox10081268
Rastogi, R., Srivastava, A. K., and Rastogi, A. K. (2001). Long term effect of aflatoxin B1 on lipid peroxidation in rat liver and kidney: effect of picroliv and silymarin. Phytother. Res. 15 (4), 307–310. doi:10.1002/ptr.722
Rotimi, O. A., Onuzulu, C. D., Dewald, A. L., Ehlinger, J., Adelani, I. B., Olasehinde, O. E., et al. (2021). Early life exposure to aflatoxin B1 in rats: alterations in lipids, hormones, and DNA methylation among the offspring. Int. J. Environ. Res. Public Health 18 (2), 589. doi:10.3390/ijerph18020589
Sadek, K., Beltagy, D., Saleh, E., and Abouelkhair, R. (2016). Camel milk and bee honey regulate profibrotic cytokine gene transcripts in liver cirrhosis induced by carbon tetrachloride. Can. J. Physiol. Pharmacol. 94 (11), 1141–1150. doi:10.1139/cjpp-2015-0596
Saeed, E., Amer, A. A., Keshta, H. G., and Khalifa, E. (2022). Prevalence and antibiogram of Salmonella enterica isolated from raw dromedary camel milk in Matrouh governorate, Egypt. Int. J. Vet. Sci. 11 (2), 168–174. doi:10.47278/journal.ijvs/2021.088
Saleem, H. D., Al-Obaidi, A. H., and Al-Tmemy, W. B. (2021). Histopathological changes due to toxic effect of aflatoxin B1 on liver, kidney and therapeutic/preventive role of camel milk. Biochem. Cell. Arch. 21 (1), DocID.
Saleemi, M. K., Raza, A., Khatoon, A., Zubair, M., Yongping, X., Murtaza, B., et al. (2023). Toxic effects of aflatoxin B1 on hematobiochemical and histopathological parameters of juvenile white leghorn male birds and their amelioration with vitamin E and Moringa oleifera. Pak. Vet. J. doi:10.29261/pakvetj/2023.053
Salem, M. H., Kamel, K. I., Yousef, M. I., Hassan, G. A., and El-Nouty, F. D. (2001). Protective role of ascorbic acid to enhance semen quality of rabbits treated with sublethal doses of aflatoxin B1. Toxicol 162 (3), 209–218. doi:10.1016/S0300-483X(01)00366-3
Schrenk, D., Bignami, M., Bodin, L., Chipman, J. K., del Mazo, J., Grasl-Kraupp, B., et al. (2020). Risk assessment of aflatoxins in food. Efsa J. 18 (3), e06040. Article e6040. doi:10.2903/j.efsa.2020.6040
Shahraki, M. R., Forghani, T., Mohammadi, M., and Khazaei-Feizalabad, A. (2015). The effect of intraventricular administration of zinc on serum LH, FSH, prolactin, and testosterone in male rats. Zahedan J. Res. Med. Sci. 17 (9). doi:10.17795/zjrms-1059
Shaker, E., Mahmoud, H., and Mnaa, S. (2010). Silymarin, the antioxidant component and Silybum marianum extracts prevent liver damage. Food Chem. Toxicol. 48, 803–806. doi:10.1016/j.fct.2009.12.011
Shi, Z., Zhang, H., Liu, Y., Xu, M., and Dai, J. (2007). Alterations in gene expression and testosterone synthesis in the testes of male rats exposed to perfluorododecanoic acid. Tox. Sci. 98 (1), 206–215. doi:10.1093/toxsci/kfm070
Shuppan, D., Ji-Dong, J., Brinkhaus, B., and Hahn, E. G. (1999). Herbal products for liver diseases: a therapeutic challenge for the new millennium. Hepatology 30, 1099–1104. doi:10.1002/hep.510300437
Sinha, S., and Prasad, V. (1990). Effect of dietary concentration of crude aflatoxin on meiotic chromosomes, sperm morphology and sperm count in mice, Mus musculus. Proc. Natl. Acad. Sci. India Sect. B. Biol. Sci. 56 (3), 269–276.
Sobral, M. M. C., Gonçalves, T., Martins, Z. E., Bäuerl, C., Cortés-Macías, E., Collado, M. C., et al. (2022). Mycotoxin interactions along the gastrointestinal tract: in vitro semi-dynamic digestion and static colonic fermentation of a contaminated meal. Toxins 14 (1), 28. doi:10.3390/toxins14010028
Stoltzfus, R. J., Mullany, L., and Black, R. E. (2004). Iron deficiency anaemia. Comp. quantification health risks Glob. regional Burd. Dis. attributable Sel. major risk factors 1, 163–209.
Su, X.-L., Yan, M.-R., and Yang, L.Qimuge-Suyila (2012). NQO1 C609T polymorphism correlated to colon cancer risk in farmers from western region of Inner Mongolia. CJCR 24, 317–322. doi:10.3978/j.issn.1000-9604.2012.08.01
Uluişik, D., Keskin, E., and Hatipoğlu, D. (2020). Effects of curcumin on hematological parameters in aflatoxin B1 applied rats. TJSE 22 (2), 265–270. doi:10.15314/tsed.735620
Vargas-Mendoza, N., Madrigal-Santillán, E., Morales-González, Á., Esquivel-Soto, J., Esquivel-Chirino, C., y González-Rubio, M. G. L., et al. (2014). Hepatoprotective effect of silymarin. World J. Hepatol. 6 (3), 144–149. doi:10.4254/wjh.v6.i3.144
Walker, W. H. (2011). Testosterone signaling and the regulation of spermatogenesis. Spermatogenesis 1 (2), 116–120. doi:10.4161/spmg.1.2.16956
Wang, L., Huang, Q. H., Li, Y. X., Huang, Y. F., Xie, J. H., Xu, L. Q., et al. (2018). Protective effects of silymarin on triptolide-induced acute hepatotoxicity in rats. Mol. Med. Rep. 17 (1), 789–800. doi:10.3892/mmr.2017.7958
Wang, Y., Liu, F., Liu, M., Zhou, X., Wang, M., Cao, K., et al. (2022). Curcumin mitigates aflatoxin B1-induced liver injury via regulating the NLRP3 inflammasome and Nrf2 signaling pathway. Food Chem. Toxicol. 161, 112823. doi:10.1016/j.fct.2022.112823
Wu, K., Jia, S., Xue, D., Rajput, S. A., Liu, M., Qi, D., et al. (2022). Dual effects of zearalenone on aflatoxin B1–induced liver and mammary gland toxicity in pregnant and lactating rats. Ecotoxicol. Environ. Saf. 245, 114115. doi:10.1016/j.ecoenv.2022.114115
Wu, Y.-F., Fu, S.-L., Kao, C.-H., Yang, C.-W., Lin, C.-H., Hsu, M.-T., et al. (2008). Chemopreventive effect of silymarin on liver pathology in HBV X protein transgenic mice. Cancer Res. 68 (6), 2033–2042. doi:10.1158/0008-5472.CAN-07-2450
Xie, Z., Wu, B., Shen, G., Li, X., and Wu, Q. (2018). Curcumin alleviates liver oxidative stress in type 1 diabetic rats. Mol. Med. Rep. 17 (1), 103–108. doi:10.3892/mmr.2017.7911
Yahya, M. A., Alhaj, O. A., Al-Khalifah, A. S., and Almnaizel, A. T. (2018). Hypocholesterolemic effect of camel milk on rats fed a high-cholesterol diet. Emir J. Food Agric. 288-294, 288. doi:10.9755/ejfa.2018.v30.i4.1664
Yang, Y., Zhang, Y., Wu, Q., Cui, X., Lin, Z., Liu, S., et al. (2014). Clinical implications of high NQO1 expression in breast cancers. J. Exp. Clin. Cancer Res. 33 (1), 14–19. doi:10.1186/1756-9966-33-14
Yilmaz, S., Kaya, E., Karaca, A., and Karatas, O. (2018). Aflatoxin B1 induced renal and cardiac damage in rats: protective effect of lycopene. Res. Vet. Sci. 119, 268–275. doi:10.1016/j.rvsc.2018.07.007
Zakaria, A. D., Bayad, A. E. S., Abdel-Raheem, S., Al-Busadah, K., and Albokhadaim, I. (2016). Camel's milk improves the semen characteristic in immobilization stressed rats. Asian J. Anim. Sci. 10 (2), 139–146. doi:10.3923/ajas.2016.139.146
Zalat, Z., Kohaf, N., Alm El-Din, M., Elewa, H., and Abdel-Latif, M. M. M. (2021). Silymarin: a promising cardioprotective agent. Azhar Int. J. Pharm. Med. Sci. 1(1), 13–21. doi:10.21608/aijpms.2021.52962.1014
Keywords: aflatoxin B1, post-treatment, camel milk, silymarin, serum biochemistry, gene expression
Citation: Hassaneen NH, Hemeda SA, El Nahas AF, Albadrani GM, Al-Ghadi MQ, Mohammedsaleh ZM, Fadl SE, El-Diasty EM and Sakr HI (2025) Post-treatment of rat aflatoxicosis by camel milk and silymarin. Front. Pharmacol. 16:1513105. doi: 10.3389/fphar.2025.1513105
Received: 17 October 2024; Accepted: 10 January 2025;
Published: 11 February 2025.
Edited by:
Ahmed Esmat Abdel Moneim, Helwan University, EgyptReviewed by:
Sekena Hassanien Abdel-Aziem, National Research Centre, EgyptCopyright © 2025 Hassaneen, Hemeda, El Nahas, Albadrani, Al-Ghadi, Mohammedsaleh, Fadl, El-Diasty and Sakr. This is an open-access article distributed under the terms of the Creative Commons Attribution License (CC BY). The use, distribution or reproduction in other forums is permitted, provided the original author(s) and the copyright owner(s) are credited and that the original publication in this journal is cited, in accordance with accepted academic practice. No use, distribution or reproduction is permitted which does not comply with these terms.
*Correspondence: Sabreen E. Fadl, bm91cm1hbGxha0BtYXUuZWR1LmVn
Disclaimer: All claims expressed in this article are solely those of the authors and do not necessarily represent those of their affiliated organizations, or those of the publisher, the editors and the reviewers. Any product that may be evaluated in this article or claim that may be made by its manufacturer is not guaranteed or endorsed by the publisher.
Research integrity at Frontiers
Learn more about the work of our research integrity team to safeguard the quality of each article we publish.