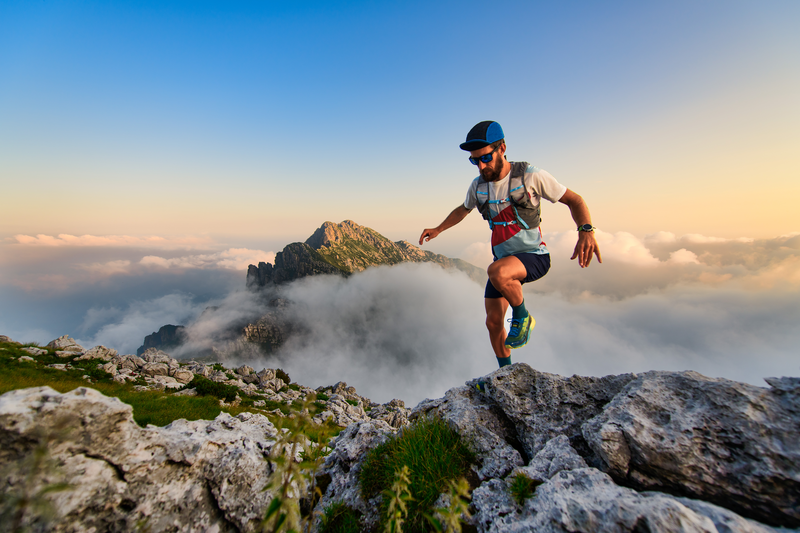
95% of researchers rate our articles as excellent or good
Learn more about the work of our research integrity team to safeguard the quality of each article we publish.
Find out more
SYSTEMATIC REVIEW article
Front. Pharmacol.
Sec. Ethnopharmacology
Volume 16 - 2025 | doi: 10.3389/fphar.2025.1510657
This article is part of the Research Topic Efficacy and Mechanism of Herbal Medicines and Their Functional Compounds in Preventing and Treating Cardiovascular Diseases and Cardiovascular Disease Risk Factors - Volume II View all 12 articles
The final, formatted version of the article will be published soon.
You have multiple emails registered with Frontiers:
Please enter your email address:
If you already have an account, please login
You don't have a Frontiers account ? You can register here
Background: Hydroxysafflor yellow A (HSYA) possesses a variety of pharmacological activities which has been demonstrated to be effective against ischemic heart disease (IHD). This study aimed to comprehensively examine the efficacy and summarize the potential mechanisms of HSYA against IHD in animal models.Methods: We conducted electronic searches for preclinical studies on PubMed, Embase, Web of Science, Cochrane Library, CNKI, SinoMed, Wanfang, and Chinese VIP databases from inception to January 31, 2024. The CAMARADES checklist was chosen to assess the quality of evidence. STATA 14.0 software was utilized to analyze the data. The underlying mechanisms were categorized and summarized.Results: Twenty-eight studies involving 686 rodents were included and the mean score of methodology quality was 5.04 (range from 4-7). Meta-analysis observed that HSYA could decrease myocardial infarction size (SMD: –2.82, 95%CI: –3.56 to –2.08, p < 0.001) and reduce the levels of biomarkers of myocardial injury including cTnI (SMD: –3.82, 95%CI: –5.20 to –2.44, p < 0.001) and CK-MB (SMD: –2.74, 95%CI: –3.58 to –1.91, p < 0.001). HSYA displayed an improvement in cardiac function indicators including LVEF, LVSP, +dp/dt max and -dp/dt max. Furthermore, HSYA was able to reduce the levels of MDA, TNF-α and IL-6, while increasing SOD and NO levels. Mechanistically, the protective effect of HSYA in alleviating myocardial injury after ischemia may be associated with NLRP3 inflammasome, Bcl-2, Bax, caspase-3, eNOS proteins, and TLR/NF-κB, Nrf2/HO-1, JAK/STAT, PI3K/Akt, AMPK/mTOR, VEGFA pathways.Conclusions: This study demonstrates that HSYA exerts cardioprotective effects in decreasing infarct size, reducing myocardial enzymes and improving cardiac function, which may be mediated by anti-inflammatory, antioxidant, anti-apoptotic, regulation of autophagy, improvement of microcirculation and promotion of angiogenesis. However, the absence of safety assessment, lack of animal models of co-morbidities, and inconsistency between timing of administration and clinical practice are limitations of preclinical studies.
Keywords: Hydroxysafflor yellow A, Myocardial Ischemia, Myocardial Infarction, Myocardial ischemia/reperfusion injury, Meta-analysis
Received: 13 Oct 2024; Accepted: 28 Mar 2025.
Copyright: © 2025 Mao, Jiang, Pang, Pan, Jia, Gao and Lin. This is an open-access article distributed under the terms of the Creative Commons Attribution License (CC BY). The use, distribution or reproduction in other forums is permitted, provided the original author(s) or licensor are credited and that the original publication in this journal is cited, in accordance with accepted academic practice. No use, distribution or reproduction is permitted which does not comply with these terms.
* Correspondence:
Qun Gao, Dongzhimen Hospital, Beijing University of Chinese Medicine, Beijing, China
Qian Lin, Dongzhimen Hospital, Beijing University of Chinese Medicine, Beijing, China
Disclaimer: All claims expressed in this article are solely those of the authors and do not necessarily represent those of their affiliated organizations, or those of the publisher, the editors and the reviewers. Any product that may be evaluated in this article or claim that may be made by its manufacturer is not guaranteed or endorsed by the publisher.
Research integrity at Frontiers
Learn more about the work of our research integrity team to safeguard the quality of each article we publish.