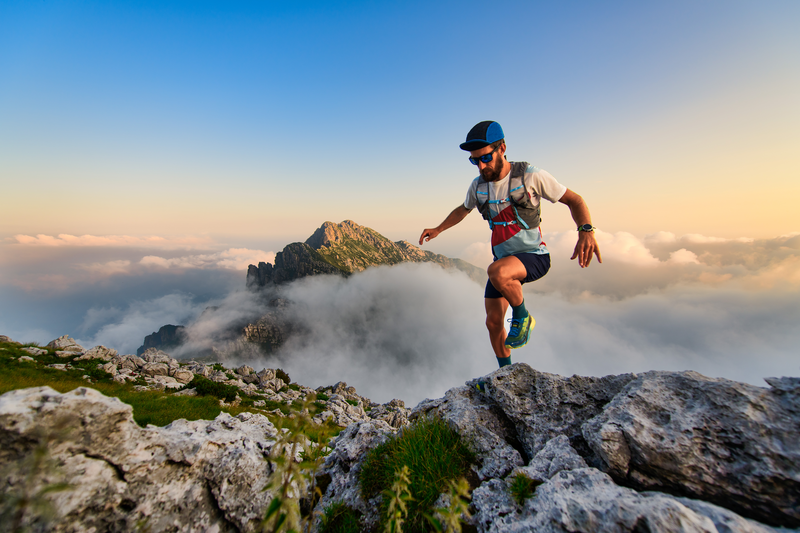
94% of researchers rate our articles as excellent or good
Learn more about the work of our research integrity team to safeguard the quality of each article we publish.
Find out more
ORIGINAL RESEARCH article
Front. Pharmacol.
Sec. Ethnopharmacology
Volume 16 - 2025 | doi: 10.3389/fphar.2025.1508762
This article is part of the Research Topic Bioactive Compounds from Medicinal Mushrooms and Plants - Extraction and Potential Application in Foods View all 8 articles
The final, formatted version of the article will be published soon.
You have multiple emails registered with Frontiers:
Please enter your email address:
If you already have an account, please login
You don't have a Frontiers account ? You can register here
The current study focuses on evaluating the biological activity and analysis of phytochemical content of extracts and extract-loaded nanoparticles from Sideritis germanicopolitana subsp. viridis (endemic, SGV) and S. libanotica subsp. linearis (SLL). It was found that the 70% ethanol extracts of SGV and SLL exhibited the highest antioxidant activity in terms of DPPH, FRAP and CUPRAC compared to other extracts. The findings showed that both 70% ethanol extract-loaded nanoparticles obtained from SGV and SLL showed lower DPPH radical scavenging, iron (III) reducing and copper (II) reducing activities compared to crude extracts. It was determined that the 70% extracts of SGV and SLL exhibited a higher potential to inhibit the enzyme urease than other extracts. The anti-urease activity of the nanoparticle loaded with SLL 70% ethanol extract was found to be greater than that of the nanoparticle made with SGV 70% ethanol extract. Furthermore, an analysis of the acetylcholinesterase enzyme inhibition capacity of various extracts from both plants revealed that the 70% ethanol extracts of each plant species had a greater potential for enzyme inhibition than the other extracts. The anticholinesterase activity of the nanoparticle loaded with SLL 70% extract was found to be higher than that of the nanoparticle loaded with SGV 70% ethanol extract. In this study the phenolic metabolites were examined, luteolin (27.44 µg/mg extract) and p-coumaric acid (20.03 µg/mg extract) were found at the highest concentration in the SGV plant while rosmarinic acid (8.70 µg/mg extract), caffeic acid (6.46 µg/mg extract) and p-coumaric acid (4.42 µg/mg extract) were found at the highest concentration in the SLL plant. However, the data demonstrated that the nanoparticles had lesser biological activity potential than crude extracts. The substantial biological activities of the nanoparticles developed as a result of this work are thought to use these formulations suitable for use as antioxidant, antiurease and anticholinesterase medicines in the future due to the benefits of using nanoparticles in the therapeutics such as the controlled release of the active agents and the diminished side effects.
Keywords: Nanoparticles, Biological activity, medicinal plants, Sideritis germanicopolitana subsp. viridis, S. libanotica subsp. linearis, HPLC-DAD
Received: 09 Oct 2024; Accepted: 14 Feb 2025.
Copyright: © 2025 Taşkın, Yılmaz, Hasan, Ermanoğlu, Taşkın, Senkardes, Şahin, Salihi, Sezer, Kerimoğlu and Kübra Elçioğlu. This is an open-access article distributed under the terms of the Creative Commons Attribution License (CC BY). The use, distribution or reproduction in other forums is permitted, provided the original author(s) or licensor are credited and that the original publication in this journal is cited, in accordance with accepted academic practice. No use, distribution or reproduction is permitted which does not comply with these terms.
* Correspondence:
Turgut Taşkın, Marmara University, Kadikoy, Türkiye
Disclaimer: All claims expressed in this article are solely those of the authors and do not necessarily represent those of their affiliated organizations, or those of the publisher, the editors and the reviewers. Any product that may be evaluated in this article or claim that may be made by its manufacturer is not guaranteed or endorsed by the publisher.
Research integrity at Frontiers
Learn more about the work of our research integrity team to safeguard the quality of each article we publish.