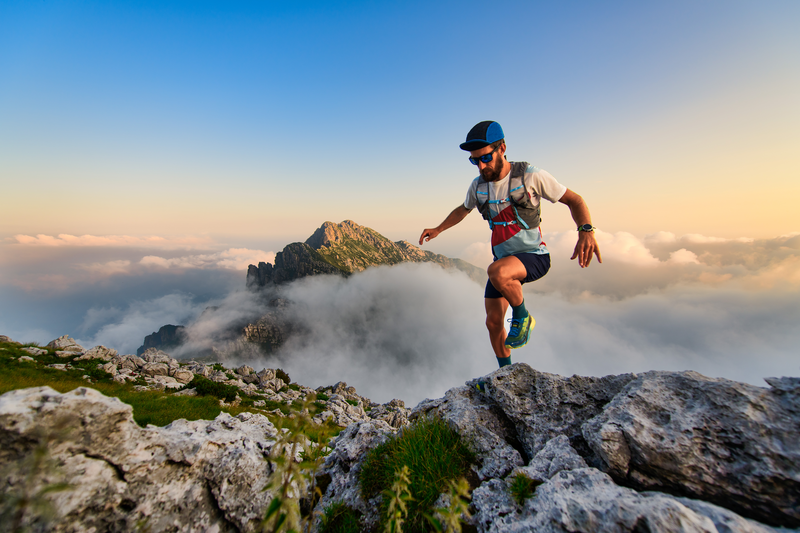
94% of researchers rate our articles as excellent or good
Learn more about the work of our research integrity team to safeguard the quality of each article we publish.
Find out more
REVIEW article
Front. Pharmacol.
Sec. Experimental Pharmacology and Drug Discovery
Volume 16 - 2025 | doi: 10.3389/fphar.2025.1504245
The final, formatted version of the article will be published soon.
You have multiple emails registered with Frontiers:
Please enter your email address:
If you already have an account, please login
You don't have a Frontiers account ? You can register here
Solanesol, identified as Nonaprenol alcohol, predominates in the Solanaceae family. This compound exists as a white to pale yellow solid at room temperature, characterized by low polarity and water insolubility. Its unique chemical structure-featuring nine non-conjugated double bonds and low polarity-confers remarkable biological activities. Recent studies have demonstrated that solanesol exhibits polypharmacological properties, including antimicrobial, antioxidant, anti-inflammatory, and membrane-stabilizing effects. Mechanistically, solanesol suppresses ROS generation and inhibits proinflammatory cytokines (IL-1β, TNF-α). Preclinical studies highlight its therapeutic potential in inflammatory disorders (periodontitis, neuropathic pain) and neurodegenerative diseases (Alzheimer's, Parkinson's). However, current research still faces critical bottlenecks, such as a lack of in vivo pharmacokinetic data, unclear molecular targets, and insufficient toxicity assessments. Future studies urgently need to integrate experimental approaches, including target screening, nanotechnology-based delivery systems, and multi-omics analysis, to elucidate its mechanisms of action and promote clinical translation. As a compound that combines natural safety with multi-target effects, solanesol is not only a research focus for the development of novel drugs but also a bridge connecting natural products and precision medicine, poised to lead the innovation of next-generation biocompatible therapies.
Keywords: solanesol1, Antimicrobial2, antioxidant3, anti-inflammatory4, membrane stabilization5
Received: 30 Sep 2024; Accepted: 04 Mar 2025.
Copyright: © 2025 Ma, Wei, Dong, Wang, Zhai, Liu, Chen, Fu, Hongwei, Hu and Chu. This is an open-access article distributed under the terms of the Creative Commons Attribution License (CC BY). The use, distribution or reproduction in other forums is permitted, provided the original author(s) or licensor are credited and that the original publication in this journal is cited, in accordance with accepted academic practice. No use, distribution or reproduction is permitted which does not comply with these terms.
* Correspondence:
Hou Hongwei, Beijing Life Science Academy, Beijing, China
Qingyuan Hu, Beijing Life Science Academy, Beijing, China
Ming Chu, Beijing Life Science Academy, Beijing, China
Disclaimer: All claims expressed in this article are solely those of the authors and do not necessarily represent those of their affiliated organizations, or those of the publisher, the editors and the reviewers. Any product that may be evaluated in this article or claim that may be made by its manufacturer is not guaranteed or endorsed by the publisher.
Research integrity at Frontiers
Learn more about the work of our research integrity team to safeguard the quality of each article we publish.