- 1Liyuan Cardiovascular Center, Liyuan Hospital, Tongji Medical College, Huazhong University of Science and Technology, Wuhan, China
- 2Department of Pharmacy, Tianmen Hospital of Traditional Chinese Medicine Affiliated to Hubei University of Chinese Medicine, Tianmen, China
- 3Department of Pharmacy, Renmin Hospital, Wuhan University, Wuhan, China
- 4Department of Pharmacy, Liyuan Hospital, Tongji Medical College, Huazhong University of Science and Technology, Wuhan, China
This review provides an in-depth exploration of the potential of Ginsenoside Rg1 in the treatment of Parkinson’s disease (PD). The emphasis of this article was the therapeutic mechanisms of Rg1, which involved the reduction of inflammation, antioxidant properties, support for neuronal survival and regeneration, regulation of cellular energy processes, and enhancement of autophagic pathways. Rg1 may protect neurons and improve both motor and cognitive impairments associated with PD through multiple mechanisms. However, challenges exist in the clinical application of Rg1, such as low bioavailability as well as a lack of comprehensive long-term safety and efficacy data. This article also reviewed network pharmacology analyses published previously to identify and explore the potential molecular targets of Rg1 in PD treatment, while evaluating strategies such as drug delivery technologies, optimizing administration routes, and combination therapies. Ultimately, this review highlights the necessity for large-scale clinical trials to validate the clinical efficacy of Rg1 and discusses its potential for PD treatment clinically.
Introduction
Parkinson’s disease (PD) is a long-lasting neurodegenerative condition that manifests mainly as motor dysfunction (Ragothaman et al., 2023). The disease is characterized by the degeneration of dopaminergic neurons in the substantia nigra and the formation of Lewy bodies, which are linked to the abnormal accumulation of α-synuclein protein (Becerra-Calixto et al., 2023). Patients with PD may experience resting tremor, bradykinesia, muscle rigidity, and postural instability in most cases (Asci et al., 2023). The occurrence and prevalence of PD are on the rise in the context of the global increase in the elderly population (Ou et al., 2021). PD may affect about 1% of individuals aged 60 and older, with the prevalence increasing to 3%–4% in those aged 80 and above, showing a higher prevalence in men than women as well (Song et al., 2022). Current therapeutic option usually includes dopamine replacement therapy to provide the effect of symptom relief. However, this therapy is impossible to prevent disease progression and may result in side effects such as dyskinesia and the “wearing-off” phenomenon after long-term use (Mishima et al., 2023), causing serious impact on the quality of life of patients (Simonet et al., 2022). The potential of natural products has been highly concerned in treating neurodegenerative diseases recently. Panax ginseng, a well-known herbal remedy, has been confirmed to have active constituents called ginsenosides, supporting its various pharmacological benefits. Based on their chemical structure, ginsenosides are divided into two main categories: protopanaxadiol-type (e.g., Rb1, Rb2, Rc, and Rd) and protopanaxatriol-type (e.g., Re, Rg1, Rg2, and Rh1) (Zhang et al., 2024; Roos et al., 2022; Jin et al., 2019). These compounds display a broad range of biological activities (Table 1). Among these, ginsenoside Rg1 has gained particular attention due to its distinct neuroprotective effects. Rg1 stands out from other ginsenosides because it exhibits an extensive mechanism of preventing inflammation and oxidation as well as its modulation of key signaling pathways. Based on the specific properties of Rg1, it may be feasible for alleviating or retarding PD symptoms. The efficacy of Rg1 in experimental settings together with its safe application profile generates scientific interest in this compound. The variety and strength of the mechanisms of Rg1 establish it as the perfect candidate for additional research as a PD therapy.
Rg1 represents a triterpenoid saponin compound which falls under the protopanaxatriol group of ginsenosides. The molecule Rg1 consists of C42H72O14 atoms and has a molecular weight of 801.01 (Choi et al., 2022). Rg1 features a sugar chain bonded to the 3-hydroxy location on its triterpenoid core structure, which usually includes glucose and rhamnose units (Yang et al., 2022; Zhang H. et al., 2021). Rg1 demonstrates outstanding water solubility and effective compatibility with polar organic solvents such as ethanol, owing to the existence of multiple hydroxyl groups in its structure. The compound maintains chemical stability, yet with thermal and acidic resistance, while being susceptible to alkaline hydrolysis (He et al., 2021; Wang et al., 2024). Rg1 stands out in scientific research because of its powerful capabilities to fight neuroinflammation, produce antioxidant effects, and support neuronal survival, while promoting regeneration and energy metabolism regulation as well as its impact on autophagic processes (Li et al., 2023; Ji et al., 2023; Yao et al., 2021). Rg1 achieves neuroprotection by blocking pro-inflammatory cytokine release while activating signaling pathways that support synaptic plasticity and simultaneously promoting both neuronal survival and regeneration. Rg1 has been reported to effectively reduce PD pathology across different biological levels, with confirmed therapeutic potential in animal experiments. The underlying mechanisms of Rg1 have proven essential to reduce symptoms and delay disease development while enhancing life quality for PD patients. Accordingly, the present article reviews current findings about the effectiveness of Rg1 in PD treatment, as well as its anti-inflammatory and antioxidative properties along with neuroprotective mechanisms. This study aims to establish a research framework and suggest clinical usage improvements.
Inhibition of neuroinflammation
Neuroinflammation may be triggered by the excessive activation of microglia and astrocytes, serving as a fundamental characteristic of PD. The excessive activation of immune cells in the brain produces inflammatory molecules TNF-α, IL-1β and IL-6, worsening damage to dopamine-producing neurons and speed up PD development (Chiu et al., 2023; Zhang et al., 2022). Rg1 can suppress inflammatory responses by blocking essential inflammatory pathways such as NF-κB, MAPK, JAK2/STAT3, and TLR4/NF-κB, leading to the production and release of decreased inflammatory mediators (Im, 2020). Rg1 demonstrates anti-inflammatory properties, which can protect nerve cells and significantly improve PD motor symptoms such as bradykinesia and rigidity, as well as inflammatory-related symptoms (Zhou et al., 2025).
The management of PD relies heavily on anti-inflammatory effects, highlighting the necessity for deciphering the mechanism of oxidative stress substantially driving disease progression. We will analyze the therapeutic mechanism of Rg1 in PD treatment through its strong antioxidant properties, which extend therapeutic advantages beyond basic protection.
Antioxidation
Oxidative stress plays a central role in the development of PD, primarily through the excessive accumulation of free radicals and reactive oxygen species (ROS), thereby leading to neuronal damage and cell death (Mittal et al., 2023; Jiang and Bai, 2022). Rg1, recognized for its strong antioxidant properties, exerts its protective effects via several molecular mechanisms (Boas et al., 2021). Specifically, it effectively counteracts the harmful effects of free radicals by enhancing the activity of antioxidant enzymes, including superoxide dismutase (SOD) and glutathione peroxidase (GSH-Px), thus alleviating neurotoxicity induced by oxidative stress (Sun et al., 2022). Rg1 boosts cellular antioxidant defenses through activating Nrf2/ARE pathway, further promoting the expression of key antioxidant genes such as heme oxygenase-1 (HO-1) and NAD(P)H: quinone oxidoreductase 1 (NQO1) (Zhang et al., 2016). Moreover, Rg1 can also prolong its neuroprotective effects by preventing lipid peroxidation, particularly the formation of malondialdehyde (MDA) to protect cell membrane integrity (Zhang et al., 2023; Hong et al., 2022). Through the synergistic actions of these antioxidant and anti-inflammatory pathways, Rg1 plays a pivotal role in alleviating motor symptoms (e.g., bradykinesia and resting tremors), which may contribute to slowing the progression of PD.
The treatment of PD must emphasize on neuron survival and regeneration promotion after defending neurons against oxidative and inflammatory damage. Rg1 demonstrates strong potential to encourage neuronal healing by activating multiple signaling pathways, which will be subjected to in-depth investigations in the next section.
Promotion of neuronal survival and regeneration
By activating the Brain-derived neurotrophic factor/tropomyosin receptor kinase B (BDNF-TrkB) signaling pathway, Rg1 can protect neurons against degeneration while controlling synaptic plasticity (Zhong et al., 2020). The increased BDNF levels may further trigger the activation of phosphoinositide 3-kinase/protein kinase B (PI3K/Akt) pathway, serving as a key mechanism in neuronal development and survival (Hannan et al., 2020). The pathway promotes cell survival while preventing apoptosis. Simultaneously, the Mitogen-activated protein kinase/extracellular signal-regulated kinase (MAPK/ERK) pathway controls cell growth and differentiation (Ma et al., 2016). Rg1 exhibits neuroprotective properties by blocking neuronal apoptosis through its suppression of Caspase-3 activity (Fan et al., 2018). The neuroprotective properties of Rg1 extend to neuronal repair considering its modulatory role in Glycogen synthase kinase 3 beta (GSK-3β) and activation of the Wnt signaling pathway, thereby improving motor function and decelerating PD progression (Yang et al., 2022).
The therapeutic results for PD treatment improve when neuronal survival and regeneration receive support through optimized energy metabolism and enhanced autophagic processes. Here, we will elucidate the mechanism of action of Rg1 in PD through cellular energy balance improvement and autophagy stimulation to achieve better treatment results.
Regulation of energy metabolism and enhancement of autophagy
Beyond its previously identified benefits, Rg1 may be useful for treating PD through its regulation of energy metabolism and autophagic processes. In addition to protecting energy metabolism from high glucose disruption, this compound can also reduce mitochondrial damage and maintain mitochondrial membrane potential through ROS suppression and mitophagy stimulation (Yang et al., 2022; Ren Y. et al., 2023). On this basis, it can sustain neuronal survival and functional maintenance, while boosting cognitive performance, leading to improved quality of life for PD patients (Walter et al., 2016; Wang W. et al., 2023). Furthermore, Rg1 activates autophagy through the AMPK/mTOR signaling pathway to reduce neurodegenerative effects from metabolic disorders. The essential process of autophagy targets damaged cellular parts for degradation and removal, while maintaining neuronal health by eliminating damaged organelles and proteins (Ktena et al., 2022). Consequently, Rg1can support cellular homeostasis to prevent neuronal damage and death from progressing. The mechanism functions to protect motor abilities, which may benefit the alleviation of motor impairments in patients with PD (Shen et al., 2021). Rg1 demonstrates significant therapeutic promise for PD, explained by its multiple biological mechanisms, as shown in Figure 1. The use of Rg1 can integrate anti-inflammatory and antioxidative neuroprotection with metabolic regulation and autophagy enhancement, demonstrating therapeutic effectiveness. Meanwhile, Rg1 exhibits multiple effects cover a broad spectrum, thus revealing potential for PD treatment. These mechanisms improve motor functions while delivering significant benefits to cognitive performance. Our subsequent section examines the multiple pathways through which Rg1 improves learning and memory functions in PD patients.
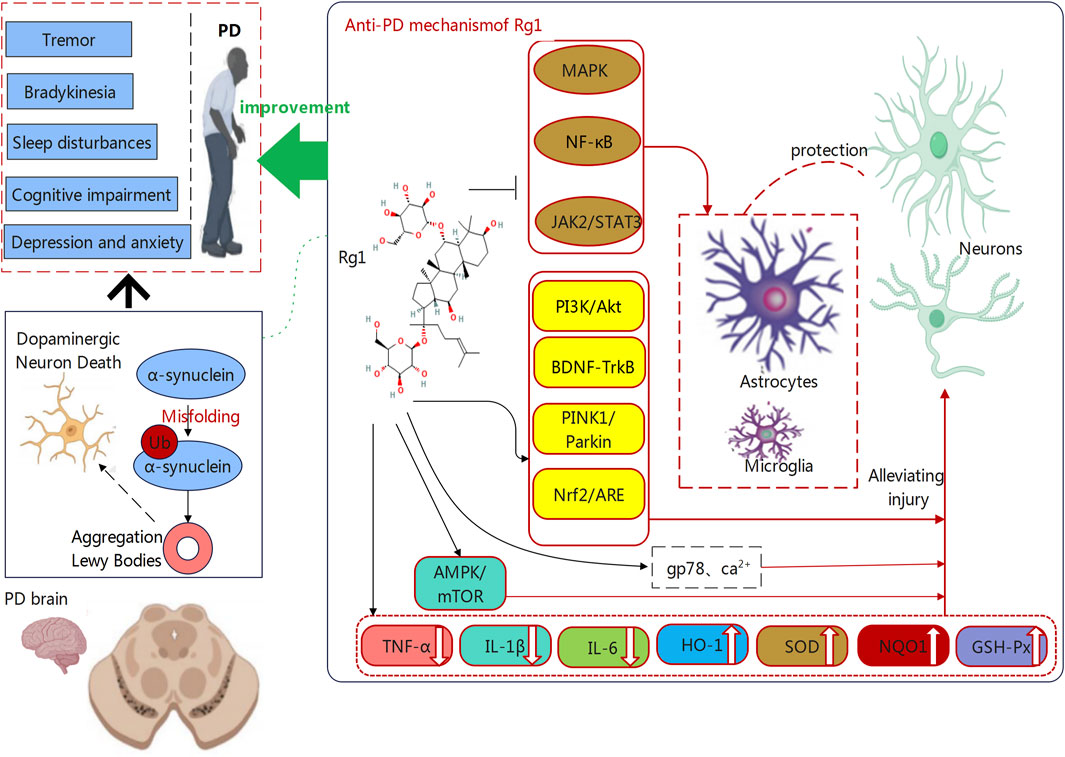
Figure 1. The mechanisms through which Ginsenoside Rg1 counteracts Parkinson’s Disease (PD). PD is characterized by α-synuclein aggregation and dopaminergic neuron degeneration. Rg1 protects neurons by reducing neuroinflammation and oxidative stress via the regulation of pathways such as NF-κB, MAPK, and Nrf2/ARE. It also enhances neuronal survival through PI3K/Akt, BDNF-TrkB, and PINK1/Parkin pathways. On these basis, Rg1 may alleviate PD symptoms such as tremor, bradykinesia, and cognitive impairment.
Improvement of learning and memory function in PD by Rg1
In the treatment of PD patients, Rg1 produces substantial improvements in cognitive functions (including learning and memory) through the activation of multiple biological pathways. Generally, PD shows major pathology through dopaminergic neuron degeneration in the substantia nigra, causing an approximately 80% decline of dopamine levels in the striatum (Liu J. Q. et al., 2020; Choi et al., 2021; Baudonnat et al., 2013). Dopamine loss may disrupt the motor control, while inducing severe cognitive function impairment, particularly disturbance of learning and memory functions (Silveira et al., 2019). Rg1 has been shown to support dopaminergic neuron survival and functional recovery, also increasing dopamine secretion and cognitive function improvement (Hernández-Vara et al., 2020). Its effect on increasing dopamine production and release can be explained by the activation of the PI3K/Akt signaling pathway to prevent neuronal apoptosis (Zhou et al., 2016). Consistently, this mechanism has been verified in multiple PD models, demonstrating Rg1’s capability to both maintain and elevate brain dopamine levels (Park et al., 2021).
Glutamate represents the dominant excitatory neurotransmitter across central nervous system pathways. As a result, excessive glutamate release may promote its role as a neurotoxin, causing damage to the function of neurons. PD patients commonly show cognitive deficiencies when glutamate levels rise above normal levels (Pourhamzeh et al., 2022). Rg1 protects neuronal functions from neurotoxicity by blocking excessive glutamate receptor activity and lessening glutamate release (Bacqué-Cazenave et al., 2020). Simultaneously, by targeting NMDA receptor activities, Rg1 improves memory-related tasks, while reducing glutamate discharge (Wang et al., 2016). Rg1 defends neurons against damage by reducing oxidative stress alongside glutamate-induced inflammation (Neshan et al., 2020).
Furthermore, Rg1 has been confirmed to raise BDNF levels, while triggering TrkB receptor activation to initiate PI3K/Akt and MAPK/ERK signal transmission. This agent affects the expression levels of essential synaptic plasticity-related genes and proteins. The behaviors at hand can strengthen synaptic interactions, while boosting connections in networks of neurons alongside both neurogenesis and synaptogenesis (Magistrelli et al., 2020; Thiruvengadam et al., 2021). Neural plasticity, or neural plasticity, is a process that involves structural and functional brain changes which occur due to learning experiences to support essential memory formation and storage processes. Prior experimental research has revealed the properties of Rg1 in amplifying neural plasticity capabilities (Feng et al., 2022).
A previous animal research has documented significant effect of Rg1 in improving cognitive function for PD models (Zhang W. et al., 2021). In another 6-OHDA-induced rat model with PD, Rg1 successfully elevated dopamine levels, leading to improved spatial learning and memory with enhanced synaptic plasticity effects (Massari et al., 2021). Animal study related to PD has proven the role of Rg1 as a potential therapeutic for neuroprotective and cognitive function improvements (Jiang et al., 2023). In AF64A memory deficit experiment, Rg1 profoundly improved cognitive functionality, exhibiting therapeutic potential for improving cognitive symptoms of PD. Y. R. Yan et al. also demonstrated in their study that PD patients experienced appreciable cognitive enhancements when treated with Rg1, accompanied by marked benefits in memory function and attention capabilities (Jagaran and Singh, 2022; Yan et al., 2021). Scientists connect the therapeutic improvement to Rg1 since this compound can increase dopamine and BDNF levels, whiledecreasing neuroinflammation, as well as supporting neuronal healing and growth (Huang et al., 2023). In addition, J. Prasuhn et al. reported that Rg1 increased spine levels of main neurotransmitters and neurotrophic factors, leading to both cognitive aid to PD patients and extensive brain protection (Prasuhn et al., 2021; Cifuentes et al., 2023).
Besides dopamine and glutamate targets, Rg1 interacts with multiple neurotransmitter pathways, including both acetylcholine and serotonin, which improves brain abilities, particularly learning and memory. Rg1 can promote the release of acetylcholine, a neurotransmitter maintaining critical cognitive functions, to enhance the cholinergic system, further bolstering cognitive capability (Liu Q. Q. et al., 2020; Singh et al., 2024; Sun et al., 2023). Through its effect on serotonin receptors, Rg1 brings two beneficial results, featuring both advanced cognitive performance and improved emotional health alongside mood (Gupta et al., 2021; Kiran et al., 2022; Khatri et al., 2023). Rg1 combats learning and memory deficits in PD by balancing dopaminergic and glutamatergic pathways, as well as raising BDNF amounts, and improving synaptic plasticity. Both animal and human investigations have shown that Rg1 significantly increases brain function, making it a feasible option for PD treatment.
So far, clinical trials on Rg1 are required to confirm its positive effects discovered during preclinical testing. The following section will analyze clinical research status on Rg1, while addressing difficulties and recommending strategies for moving beyond experimental research toward clinical practice.
Clinical applications of Rg1 in PD
Current clinical research remains inadequate to determine the value of Rg1 in the treatment of PD. Research to date shows Rg1 safety and general tolerance. However, extended clinical trials are still needed to examine its therapeutic potential and functional mechanisms. Recently, the medical utility of Rg1 is expected to be upgraded through nanotechnology integration, so as to increase its bioavailability while targeting destinations more precisely. Nanomedicine techniques are beneficial for extending medicinal stability by enhancing the physicochemical characteristics of drugs, while improving their therapeutic effectiveness in real-world conditions. The design of Rg1 nanoparticles enables enhanced blood-brain barrier permeability, facilitating targeted treatment to affected regions, thus improving the therapeutic outcomes (McLoughlin et al., 2024). Current studies focus mainly on the immediate effect, while presenting scarce information about Rg1 as well as its long-term safety and effectiveness. In the future, extended observational studies are necessary to assess long-term side effects and benefits together with risk factors. Future long-term clinical trials should investigate effects of Rg1 on PD patients’ motor capabilities and cognitive functions as well as their non-motor symptoms (Lyu et al., 2022; Khattab et al., 2021). Subsequent scientific exploration needs to target advanced delivery systems to maximize Rg1 bioavailability. Moreover, additional attention can be paid to delivery methods using polymer microsphere and gel systems alongside transdermal patches together with other bypass for first-pass metabolism systems, thus enhancing both the absorption and efficacy for Rg1 treatment. An ongoing study proposes a notable method of using intranasal delivery to target brain tissues, where it appears to both increase CNS drug levels and improve medical outcomes (Parkash et al., 2024; Pires et al., 2022). Meanwhile, it is important to decipher the mechanism of Rg1 when used with other treatments to increase its effectiveness as a feasible therapy for PD. Rg1, combined with dopamine replacement therapies delivers, was found to enhance neuroprotection, while protecting remaining dopaminergic neurons through the ability to reduce L-DOPA doses, thereby minimizing treatment-related side effects (García-Cárceles et al., 2022). Rg1 presents promising clinical outcomes for PD, although its long-duration safety and effects should be established through further research. Collectively, future wide-ranging clinical trials and multiple center research endeavors are essential to confirm the therapeutic benefit of Rg1 and its potential combined treatments for PD, which can facilitate the formulation of better treatment options for patients with PD.
Network pharmacology analysis
There exists a substantial obstacle in bringing Rg1 to clinical practice due to the requirement to demonstrate consistent research results that apply to the broad patient population. Animal trials and initial human clinical data form the basis for current research, generating doubts about their relevance when applied to large human clinical trials. The intricate study on the mechanism of action of Rg1 reveals valuable therapeutic possibilities, yet constituting obstacles for researchers trying to design success studies. Ironically, there is still an absence of complete assessments around potential unintended side effects related to the extensive target diversity of the action. Upcoming studies on well-controlled randomized trials are needed to profile the effectiveness of Rg1 among different populations, and develop dose and treatment guidelines. Network pharmacology can be employed to identify related pathways explaining the operational mechanism of Rg1, functioning as an effective tool. Through this approach, potential molecular targets can be identified systematically, which grants researchers better insight into therapeutic opportunities of Rg1. We will continue to elaborate network pharmacology outcomes, aiming at creating stronger scientific support for employing Rg1 in clinical practice. Network pharmacology techniques enable the location of possible targets of Rg1 within PD (PD). First, each possible target for Rg1 could be determined by using the SwissTargetPrediction tool (Our study used SwissTargetPrediction (http://www.swisstargetprediction.ch/). Next, genes related to PD could be filtered using the GeneCards database (https://www.genecards.org/). These targets were then imported into Cytoscape 3.10 to establish a drug-target-disease network, followed by the construction of a protein-protein interaction (PPI) network using String database (https://cn.string-db.org/). The PPI network was useful for the selection of targets for further analysis, representing areas with established experimental support. Furthermore, to verify the reliability of these targets, molecular docking was performed using AutoDockTools 1.5 and PyMOL, in combination with the PDB database (https://www.rcsb.org/). Finally, Rg1 presented 100 potential targets, which matched with a set of 9,366 PD-related targets to produce 72 shared targets for examination. Among these, HSP90AA1 and MMP9 were enrolled for thorough molecular docking analysis. AutoDockTools determined the binding energies to be −8.6 kcal/mol for HSP90AA1 and −7.1 kcal/mol for MMP9, as depicted in Figure 2. Our study demonstrated definitive binding relationships between targets, validating both their trustworthiness and potential use in therapy. We deployed network pharmacology to systematically map potential targets and biological pathways of Rg1, while developing interconnected drug-target-disease networks, for a purpose of better understanding molecular multi-target actions. Significantly, merging bioinformatics data with this technique can speed up target validation, resulting in more efficient research progress. Molecular docking analysis validates the identified targets, delivering strong scientific proof of the neuroprotective abilities of Rg1.
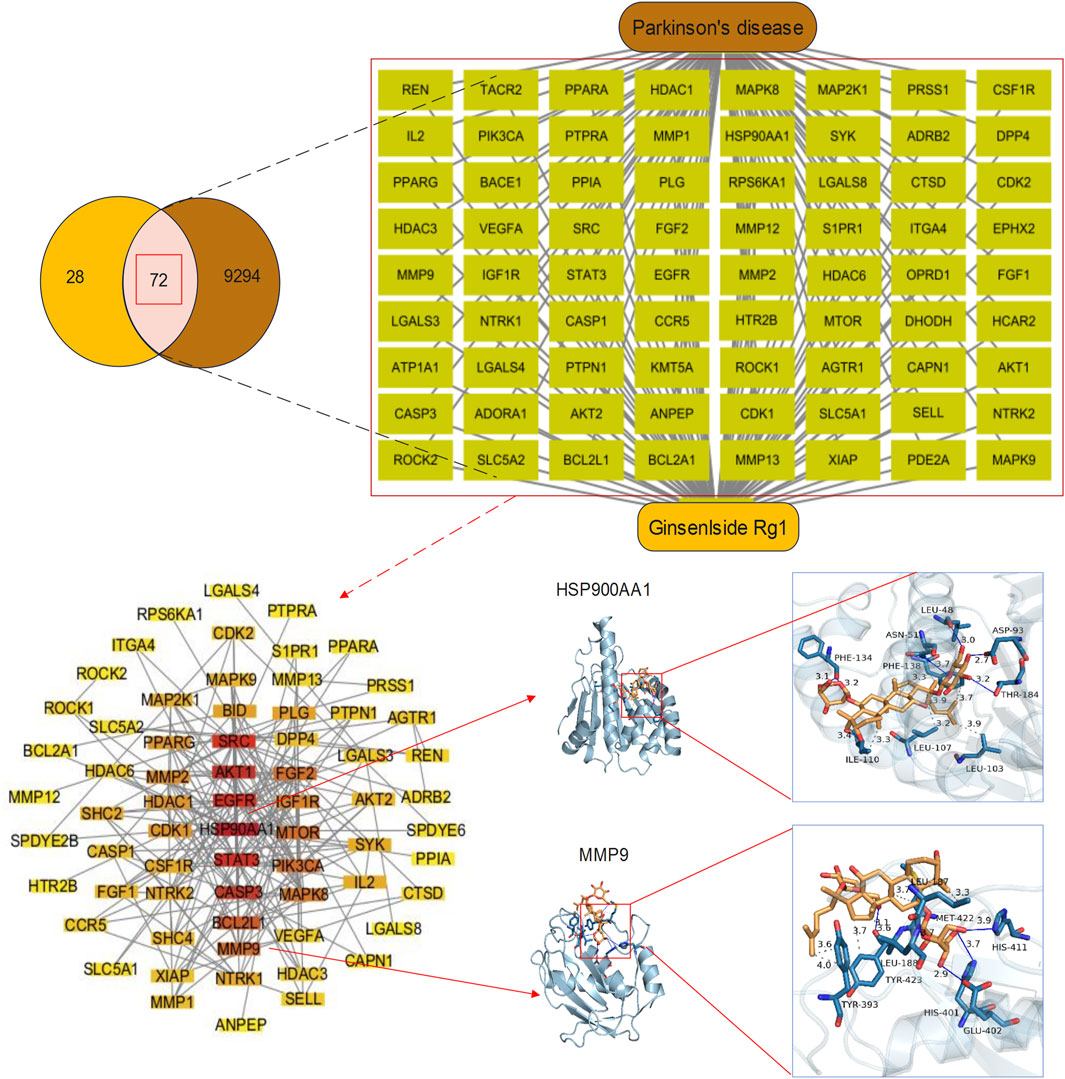
Figure 2. Potential targets and molecular docking of Rg1 against PD. Venn diagram and drug-targets-disease network of Rg1 targets and PD-related genes. There were 100 Rg1 targets and 9,366 PD-related genes, 72 of which were common targets. PPI network and molecular docking of HSP900AA1 and MMP9. The PPI network contained 67 key targets. The binding energy of HSP900AA1 and MMP9 were −8.6 kcal/mol and −7.1 kcal/mol, respectively, indicating a good docking effect.
Conclusion
Rg1 has shown significant promise for the treatment of PD in both foundational and clinical research, primarily attributed to its robust neuroprotective effects, such as its anti-inflammatory and antioxidant properties. Rg1 can effectively alleviate PD-associated motor and cognitive dysfunctions through various mechanisms, including the inhibition of neuroinflammation, reduction of oxidative stress, promotion of neuronal survival, and enhancement of synaptic plasticity. At this stage, the therapeutic efficacy of Rg1 remains to be established by further clinical validation, although the initial findings are encouraging. In terms of future direction of investigation, we can continue to comprehensively evaluate the long-term safety and effectiveness of Rg1 through large-scale, multi-center clinical trials; improve the bioavailability and targeting accuracy of Rg1 using advanced technologies like nanotechnology; explore potential synergies with current treatments to optimize therapeutic outcomes and minimize adverse effects; and conduct a deeper analysis of the molecular pathways that mediate the effects of Rg1 to fully understand its role in neurodegenerative disease treatment. Continued research can position Rg1 as a valuable therapeutic option for PD, offering improved therapeutic solutions for patients.
Author contributions
QW: Data curation, Investigation, Writing – original draft. LW: Formal analysis, Conceptualization, Writing–review and editing GC: Methodology, Software, Visualization, Writing – original draft. QC: Funding acquisition, Project administration, Supervision, Writing – review and editing.
Funding
The author(s) declare that financial support was received for the research and/or publication of this article. The present study was supported by the grant from Natural Science Foundation of Hubei Province (No. 2024AFB578), and The Intramural Research Program of Liyuan Hospital, Tongji Medical College, Huazhong University of Science and Technology (No. 2023LYYYSZRP0001).
Conflict of interest
The authors declare that the research was conducted in the absence of any commercial or financial relationships that could be construed as a potential conflict of interest.
Publisher’s note
All claims expressed in this article are solely those of the authors and do not necessarily represent those of their affiliated organizations, or those of the publisher, the editors and the reviewers. Any product that may be evaluated in this article, or claim that may be made by its manufacturer, is not guaranteed or endorsed by the publisher.
References
Asci, F., Falletti, M., Zampogna, A., Patera, M., Hallett, M., Rothwell, J., et al. (2023). Rigidity in Parkinson’s disease: evidence from biomechanical and neurophysiological measures. Brain 146, 3705–3718. doi:10.1093/brain/awad114
Bacqué-Cazenave, J., Bharatiya, R., Barrière, G., Delbecque, J. P., Bouguiyoud, N., Di Giovanni, G., et al. (2020). Serotonin in animal cognition and behavior. Int. J. Mol. Sci., 21. doi:10.3390/ijms21051649
Baudonnat, M., Huber, A., David, V., and Walton, M. E. (2013). Heads for learning, tails for memory: reward, reinforcement and a role of dopamine in determining behavioral relevance across multiple timescales. Front. Neurosci. 7, 175. doi:10.3389/fnins.2013.00175
Becerra-Calixto, A., Mukherjee, A., Ramirez, S., Sepulveda, S., Sinha, T., Al-Lahham, R., et al. (2023). Lewy body-like pathology and loss of dopaminergic neurons in midbrain organoids derived from familial Parkinson’s disease patient. Cells 12, 625. doi:10.3390/cells12040625
Boas, S. M., Joyce, K. L., and Cowell, R. M. (2021). The NRF2-dependent transcriptional regulation of antioxidant defense pathways: relevance for cell type-specific vulnerability to neurodegeneration and therapeutic intervention. Antioxidants (Basel) 11, 8. doi:10.3390/antiox11010008
Chiu, Y. J., Lin, C. H., Lin, C. Y., Yang, P. N., Lo, Y. S., Chen, Y. C., et al. (2023). Investigating therapeutic effects of indole derivatives targeting inflammation and oxidative stress in neurotoxin-induced cell and mouse models of Parkinson’s disease. Int. J. Mol. Sci. 24, 2642. doi:10.3390/ijms24032642
Choi, H. S., Koo, H. B., Jeon, S. W., Han, J. Y., Kim, J. S., Jun, K. M., et al. (2022). Modification of ginsenoside saponin composition via the CRISPR/Cas9-mediated knockout of protopanaxadiol 6-hydroxylase gene in Panax ginseng. J. Ginseng Res. 46, 505–514. doi:10.1016/j.jgr.2021.06.004
Choi, Y. J., Kim, D. W., Shin, M. J., Yeo, H. J., Yeo, E. J., Lee, L. R., et al. (2021). PEP-1-GLRX1 reduces dopaminergic neuronal cell loss by modulating MAPK and apoptosis signaling in Parkinson’s disease. Molecules 26, 3329. doi:10.3390/molecules26113329
Cifuentes, J., Cifuentes-Almanza, S., Puentes, P. R., Quezada, V., Barrios, A. F. G., Calderón-Peláez, M. A., et al. (2023). Multifunctional magnetoliposomes as drug delivery vehicles for the potential treatment of Parkinson’s disease. Front. Bioeng. Biotech. 11, 11. doi:10.3389/fbioe.2023.1181842
Ding, Y., Botchway, B. O. A., Zhang, Y., and Liu, X. (2023). Ginsenosides can target brain-derived neurotrophic factor to improve Parkinson’s disease. Food Funct. 14, 5537–5550. doi:10.1039/d2fo03484k
Fan, C., Song, Q., Wang, P., Li, Y., Yang, M., and Yu, S. Y. (2018). Neuroprotective effects of ginsenoside-Rg1 against depression-like behaviors via suppressing glial activation, synaptic deficits, and neuronal apoptosis in rats. Front. Immunol. 9, 2889. doi:10.3389/fimmu.2018.02889
Feng, H., Xue, M., Deng, H., Cheng, S., Hu, Y., and Zhou, C. (2022). Ginsenoside and its therapeutic potential for cognitive impairment. Biomolecules 12, 1310. doi:10.3390/biom12091310
García-Cárceles, J., Vázquez-Villa, H., Brea, J., De Guevara-Miranda, D. L., Cincilla, G., Sánchez-Martínez, M., et al. (2022). 2-(Fluoromethoxy)-4′-(S-methanesulfonimidoyl)-1,1′-biphenyl (UCM-1306), an orally bioavailable positive allosteric modulator of the human dopamine D1 receptor for Parkinson’s disease. J. Med. Chem. 65, 12256–12272. doi:10.1021/acs.jmedchem.2c00949
Gupta, S., Wang, M. Y., Azuma, Y., and Muma, N. A. (2021). Regulation of serotonin 1A receptor SUMOylation by SENP2 and PIASxα. Int. J. Mol. Sci. 22, 13176. doi:10.3390/ijms222413176
Hannan, M. A., Dash, R., Sohag, A. A. M., Haque, M. N., and Moon, I. S. (2020). Neuroprotection against oxidative stress: phytochemicals targeting TrkB signaling and the Nrf2-ARE antioxidant system. Front. Mol. Neurosci. 13, 116. doi:10.3389/fnmol.2020.00116
He, Y. B., Liu, Y. L., Yang, Z. D., Lu, J. H., Song, Y., Guan, Y. M., et al. (2021). Effect of ginsenoside-Rg1 on experimental Parkinson’s disease: a systematic review and meta-analysis of animal studies. Exp. Ther. Med. 21, 552. doi:10.3892/etm.2021.9984
Hernández-Vara, J., Sáez-Francàs, N., Lorenzo-Bosquet, C., Corominas-Roso, M., Cuberas-Borròs, G., Lucas-Del Pozo, S., et al. (2020). BDNF levels and nigrostriatal degeneration in “drug naive” Parkinson’s disease patients. An “in vivo” study using I-123-FP-CIT SPECT. Park. Relat. D. 78, 31–35. doi:10.1016/j.parkreldis.2020.06.037
Hong, D. G., Lee, S., Kim, J., Yang, S., Lee, M., Ahn, J., et al. (2022). Anti-inflammatory and neuroprotective effects of morin in an MPTP-induced Parkinson’s disease model. Int. J. Mol. Sci. 23, 10578. doi:10.3390/ijms231810578
Huang, Z. X., Hu, B. B., Xiang, B. H., Fang, H. Q., Zhang, B. Z., Wang, Y., et al. (2023). Biomimetic biomembrane encapsulation and targeted delivery of a nitric Oxide Release platform for therapy of Parkinson?s disease. Acs Biomater. Sci. Eng. 9, 2545–2557. doi:10.1021/acsbiomaterials.3c00146
Im, D. S. (2020). Pro-resolving effect of ginsenosides as an anti-inflammatory mechanism of panax ginseng. Biomolecules 10, 444. doi:10.3390/biom10030444
Jagaran, K., and Singh, M. (2022). Lipid nanoparticles: promising treatment approach for Parkinson’s disease. Int. J. Mol. Sci. 23, 9361. doi:10.3390/ijms23169361
Ji, Y. J., Kim, H. D., Lee, E. S., Jang, G. Y., and Seong, H. A. (2023). Heat treatment enhances the neuroprotective effects of crude ginseng saponin by increasing minor ginsenosides. Int. J. Mol. Sci. 24, 7223. doi:10.3390/ijms24087223
Jiang, H., and Bai, X. (2022). Apolipoprotein A-I mimetic peptides (ApoAI MP) improve oxidative stress and inflammatory responses in Parkinson’s disease mice. Front. Pharmacol. 13, 966232. doi:10.3389/fphar.2022.966232
Jiang, N., Lv, J. W., Zhang, Y. W., Sun, X. R., Yao, C. H., Wang, Q., et al. (2023). Protective effects of ginsenosides Rg1 and Rb1 against cognitive impairment induced by simulated microgravity in rats. Front. Pharmacol. 14, 1167398. doi:10.3389/fphar.2023.1167398
Jin, S., Jeon, J. H., Lee, S., Kang, W. Y., Seong, S. J., Yoon, Y. R., et al. (2019). Detection of 13 ginsenosides (Rb1, Rb2, Rc, Rd, Re, Rf, Rg1, Rg3, Rh2, F1, compound K, 20(S)-Protopanaxadiol, and 20(S)-Protopanaxatriol) in human plasma and application of the analytical method to human pharmacokinetic studies following two week-repeated administration of red ginseng extract. Molecules 24, 2618. doi:10.3390/molecules24142618
Khatri, D. K., Preeti, K., Tonape, S., Bhattacharjee, S., Patel, M., Shah, S. R., et al. (2023). Nanotechnological advances for nose to brain delivery of therapeutics to improve the Parkinson therapy. Curr. Neuropharmacol. 21, 493–516. doi:10.2174/1570159x20666220507022701
Khattab, A., Ahmed-Farid, O. A., and Nasr, S. A. (2021). Enhanced brain biodistribution of Ginsenoside Rg1 based self-nanoemulsifying drug delivery system to ameliorate metabolic syndromes and keep homeostatic balance. J. Drug Deliv. Sci. Tec. 61, 102276. doi:10.1016/j.jddst.2020.102276
Kiran, P., Debnath, S. K., Neekhra, S., Pawar, V., Khan, A., Dias, F., et al. (2022). Designing nanoformulation for the nose-to-brain delivery in Parkinson’s disease: advancements and barrier. Wires Nanomed Nanobi 14, e1768. doi:10.1002/wnan.1768
Ktena, N., Kaplanis, S. I., Kolotuev, I., Georgilis, A., Kallergi, E., Stavroulaki, V., et al. (2022). Autophagic degradation of CNS myelin maintains axon integrity. Cell Stress 6, 93–107. doi:10.15698/cst2022.12.274
Li, X., Huang, L., Kong, L., Su, Y., Zhou, H., Ji, P., et al. (2023). Ginsenoside Rg1 alleviates learning and memory impairments and Abeta disposition through inhibiting NLRP1 inflammasome and autophagy dysfunction in APP/PS1 mice. Mol. Med. Rep., 27. doi:10.3892/mmr.2022.12893
Liang, W., Liu, Y., Zhou, K., Jian, P., Zhang, Q., Chang, Z., et al. (2022). Ginsenoside Rb1 prevents lipopolysaccharide-induced depressive-like behavior by inhibiting inflammation and neural dysfunction and F2 elicits a novel antidepressant-like effect: a metabolite-based network pharmacology study. J. Ethnopharmacol. 282, 114655. doi:10.1016/j.jep.2021.114655
Liu, J. Q., Zhao, M., Zhang, Z., Cui, L. Y., Zhou, X., Zhang, W., et al. (2020). Rg1 improves LPS-induced Parkinsonian symptoms in mice via inhibition of NF-κB signaling and modulation of M1/M2 polarization. Acta Pharmacol. Sin. 41, 523–534. doi:10.1038/s41401-020-0358-x
Liu, Q. Q., Zhang, S. Z., Zhu, D. S., Tang, X. Y., Che, Y. Z., and Feng, X. Z. (2020). The parthenolide derivative ACT001 synergizes with low doses of L-DOPA to improve MPTP-induced Parkinson’s disease in mice. Behav. Brain Res. 379, 112337. doi:10.1016/j.bbr.2019.112337
Liu, S., Chen, W., Zhao, Y., Zong, Y., Li, J., and He, Z. (2023). Research progress on effects of ginsenoside Rg2 and Rh1 on nervous system and related mechanisms. Molecules 28, 7935. doi:10.3390/molecules28237935
Lu, J., Wang, X., Wu, A., Cao, Y., Dai, X., Liang, Y., et al. (2022). Ginsenosides in central nervous system diseases: pharmacological actions, mechanisms, and therapeutics. Phytother. Res. 36, 1523–1544. doi:10.1002/ptr.7395
Lyu, T. J., Zhang, Z. X., Chen, J., and Liu, Z. J. (2022). Ginsenoside Rg1 ameliorates apoptosis, senescence and oxidative stress in ox-LDL-induced vascular endothelial cells via the AMPK/SIRT3/p53 signaling pathway. Exp. Ther. Med. 24, ARTN 545. doi:10.3892/etm.2022.11482
Ma, B., Herzog, E. L., Moore, M., Lee, C. M., Na, S. H., Lee, C. G., et al. (2016). RIG-like helicase regulation of chitinase 3-like 1 Axis and pulmonary metastasis. Sci. Rep. 6, 26299. doi:10.1038/srep26299
Madhi, I., Kim, J. H., Shin, J. E., and Kim, Y. (2021). Ginsenoside Re exhibits neuroprotective effects by inhibiting neuroinflammation via CAMK/MAPK/NF-kappaB signaling in microglia. Mol. Med. Rep., 24. doi:10.3892/mmr.2021.12337
Magistrelli, L., Storelli, E., Rasini, E., Contaldi, E., Comi, C., Cosentino, M., et al. (2020). Relationship between circulating CD4+ T lymphocytes and cognitive impairment in patients with Parkinson’s disease. Brain Behav. Immun. 89, 668–674. doi:10.1016/j.bbi.2020.07.005
Massari, C. M., Constantino, L. C., and Tasca, C. I. (2021). Adenosine A1 and A2A receptors are involved on guanosine protective effects against oxidative burst and mitochondrial dysfunction induced by 6-OHDA in striatal slices. Purinerg Signal 17, 247–254. doi:10.1007/s11302-021-09765-y
McLoughlin, C. D., Nevins, S., Stein, J. B., Khakbiz, M., and Lee, K. B. (2024). Overcoming the blood–brain barrier: multifunctional nanomaterial-based strategies for targeted drug delivery in neurological disorders. Small Sci. 4, 4. doi:10.1002/smsc.202400232
Mishima, T., Chiu, S. W., Saiki, H., Yamaguchi, T., Shimo, Y., Maeda, T., et al. (2023). Risk factors for developing dyskinesia among Parkinson’s disease patients with wearing-off: J-FIRST. J. Neurol. Sci. 448, 120619. doi:10.1016/j.jns.2023.120619
Mittal, P., Dhankhar, S., Chauhan, S., Garg, N., Bhattacharya, T., Ali, M., et al. (2023). A review on natural antioxidants for their role in the treatment of Parkinson’s disease. Pharm. (Basel) 16, 908. doi:10.3390/ph16070908
Neshan, M., Campbell, A., Malakouti, S. K., Zareii, M., and Ahangari, G. (2020). Gene expression of serotonergic markers in peripheral blood mononuclear cells of patients with late-onset Alzheimer’s disease. Heliyon 6, e04716. doi:10.1016/j.heliyon.2020.e04716
Ou, Z., Pan, J., Tang, S., Duan, D., Yu, D., Nong, H., et al. (2021). Global trends in the incidence, prevalence, and years lived with disability of Parkinson’s disease in 204 countries/territories from 1990 to 2019. Front. Public Health 9, 776847. doi:10.3389/fpubh.2021.776847
Park, M., Kim, S.-H., and Lee, H.-J. (2021). Ginsenoside Rh1 exerts neuroprotective effects by activating the PI3K/Akt pathway in amyloid-β induced SH-SY5Y cells. Appl. Sci. 11, 5654. doi:10.3390/app11125654
Parkash, M., Shoaib, M. H., Sikandar, M., Yousuf, R. I., Saleem, M. T., Ahmed, F. R., et al. (2024). Formulation development, characterization, and mechanistic PBPK modeling of metoclopramide loaded halloysite nanotube (HNT) based drug-in-adhesive type transdermal drug delivery system. Sci. Rep. 14, 28512. doi:10.1038/s41598-024-80089-8
Pires, P. C., Rodrigues, M., Alves, G., and Santos, A. O. (2022). Strategies to improve drug strength in nasal preparations for brain delivery of low aqueous solubility drugs. Pharmaceutics 14, 588. doi:10.3390/pharmaceutics14030588
Pourhamzeh, M., Moravej, F. G., Arabi, M., Shahriari, E., Mehrabi, S., Ward, R., et al. (2022). The roles of serotonin in neuropsychiatric disorders. Cell. Mol. Neurobiol. 42, 1671–1692. doi:10.1007/s10571-021-01064-9
Prasuhn, J., Kasten, M., Vos, M., König, I. R., Schmid, S. M., Wilms, B., et al. (2021). The use of vitamin K2 in patients with Parkinson’s disease and mitochondrial dysfunction (PD-K2): a theranostic pilot study in a placebo-controlled parallel group design. Front. Neurol. 11, 592104. doi:10.3389/fneur.2020.592104
Ragothaman, A., Mancini, M., Nutt, J. G., Wang, J. P., Fair, D. A., Horak, F. B., et al. (2023). Motor networks, but also non-motor networks predict motor signs in Parkinson’s disease. Neuroimage-Clin. 40, 103541. doi:10.1016/j.nicl.2023.103541
Ren, Y., Ye, D., Ding, Y., and Wei, N. (2023). Ginsenoside Rk1 prevents 1-methyl-4-phenyl-1,2,3,6-tetrahydropyridine-induced Parkinson’s disease via activating silence information regulator 3-mediated Nrf2/HO-1 signaling pathway. Hum. Exp. Toxicol. 42, 9603271231220610. doi:10.1177/09603271231220610
Ren Z., Z., Yang, H., Zhu, C., Deng, J., and Fan, D. (2023). Ginsenoside Rh4 alleviates amyloid β plaque and Tau hyperphosphorylation by regulating neuroinflammation and the glycogen synthase kinase 3β signaling pathway. J. Agric. Food Chem. 71, 13783–13794. doi:10.1021/acs.jafc.3c02550
Roos, D. S., Klein, M., Deeg, D. J. H., Doty, R. L., and Berendse, H. W. (2022). Prevalence of prodromal symptoms of Parkinson’s disease in the late middle-aged population. J. Park. Dis. 12, 967–974. doi:10.3233/JPD-213007
Sarhene, M., Ni, J. Y., Duncan, E. S., Liu, Z., Li, S., Zhang, J., et al. (2021). Ginsenosides for cardiovascular diseases; update on pre-clinical and clinical evidence, pharmacological effects and the mechanisms of action. Pharmacol. Res. 166, 105481. doi:10.1016/j.phrs.2021.105481
Shen, F., Wang, J., Gao, F., Wang, J., and Zhu, G. (2021). Ginsenoside Rg1 prevents cognitive impairment and hippocampal neuronal apoptosis in experimental vascular dementia mice by promoting GPR30 expression. Neural Plast. 2021, 2412220. doi:10.1155/2021/2412220
Shi, Z., Chen, H., Zhou, X., Yang, W., and Lin, Y. (2022). Pharmacological effects of natural medicine ginsenosides against Alzheimer’s disease. Front. Pharmacol. 13, 952332. doi:10.3389/fphar.2022.952332
Silveira, C. R. A., MacKinley, J., Coleman, K., Li, Z., Finger, E., Bartha, R., et al. (2019). Ambroxol as a novel disease-modifying treatment for Parkinson’s disease dementia: protocol for a single-centre, randomized, double-blind, placebo-controlled trial. BMC Neurol. 19, 20. doi:10.1186/s12883-019-1252-3
Simonet, C., Bestwick, J., Jitlal, M., Waters, S., Ben-Joseph, A., Marshall, C. R., et al. (2022). Assessment of risk factors and early presentations of Parkinson disease in primary care in a diverse UK population. JAMA Neurol. 79, 359–369. doi:10.1001/jamaneurol.2022.0003
Singh, S., Agrawal, N., and Goyal, A. (2024). Role of alpha-7-nicotinic acetylcholine receptor in Alzheimer’s disease. Cns Neurol. Disord-Dr 23, 384–394. doi:10.2174/1871527322666230627123426
Song, Z., Liu, S., Li, X., Zhang, M., Wang, X., Shi, Z., et al. (2022). Prevalence of Parkinson’s disease in adults aged 65 Years and older in China: a multicenter population-based survey. Neuroepidemiology 56, 50–58. doi:10.1159/000520726
Sun, Y., Yang, Y., Liu, S., Yang, S., Chen, C., Lin, M., et al. (2022). New therapeutic approaches to and mechanisms of ginsenoside Rg1 against neurological diseases. Cells 11, 2529. doi:10.3390/cells11162529
Sun, Y. T., Kong, J. L., Ge, X. H., Mao, M. R., Yu, H. R., and Wang, Y. (2023). An antisense oligonucleotide-loaded blood-brain barrier penetrable nanoparticle mediating recruitment of endogenous neural stem cells for the treatment of Parkinson’s disease. Acs Nano 17, 4414–4432. doi:10.1021/acsnano.2c09752
Thiruvengadam, M., Venkidasamy, B., Subramanian, U., Samynathan, R., Ali Shariati, M., Rebezov, M., et al. (2021). Bioactive compounds in oxidative stress-mediated diseases: targeting the NRF2/ARE signaling pathway and epigenetic regulation. Antioxidants (Basel) 10, 1859. doi:10.3390/antiox10121859
Walter, C., Clemens, L. E., Muller, A. J., Fallier-Becker, P., Proikas-Cezanne, T., Riess, O., et al. (2016). Activation of AMPK-induced autophagy ameliorates Huntington disease pathology in vitro. Neuropharmacology 108, 24–38. doi:10.1016/j.neuropharm.2016.04.041
Wang, H., Yang, Y., Yang, S., Ren, S., Feng, J., Liu, Y., et al. (2024). Corrigendum: ginsenoside Rg1 ameliorates neuroinflammation via suppression of connexin43 ubiquitination to attenuate depression. Front. Pharmacol. 15, 1418824. doi:10.3389/fphar.2024.1418824
Wang, J., Zeng, L., Zhang, Y., Qi, W., Wang, Z., Tian, L., et al. (2022). Pharmacological properties, molecular mechanisms and therapeutic potential of ginsenoside Rg3 as an antioxidant and anti-inflammatory agent. Front. Pharmacol. 13, 975784. doi:10.3389/fphar.2022.975784
Wang, L., Zhang, Y., Song, Z., Liu, Q., Fan, D., and Song, X. (2023). Ginsenosides: a potential natural medicine to protect the lungs from lung cancer and inflammatory lung disease. Food Funct. 14, 9137–9166. doi:10.1039/d3fo02482b
Wang, W., Wang, L., Wang, L., Li, Y., Lan, T., Wang, C., et al. (2023). Ginsenoside-Rg1 synergized with voluntary running exercise protects against glial activation and dysregulation of neuronal plasticity in depression. Food Funct. 14, 7222–7239. doi:10.1039/d3fo00496a
Wang, Y., Liu, Q., Xu, Y., Zhang, Y., Lv, Y., Tan, Y., et al. (2016). Ginsenoside Rg1 protects against oxidative stress-induced neuronal apoptosis through myosin IIA-actin related cytoskeletal reorganization. Int. J. Biol. Sci. 12, 1341–1356. doi:10.7150/ijbs.15992
Yan, Y. R., Chen, Y., Liu, Z. X., Cai, F. Y., Niu, W. T., Song, L. M., et al. (2021). Brain delivery of curcumin through low-intensity ultrasound-induced blood-brain barrier opening via lipid-PLGA nanobubbles. Int. J. Nanomed 16, 7433–7447. doi:10.2147/Ijn.S327737
Yang, Y., Wang, L., Zhang, C., Guo, Y., Li, J., Wu, C., et al. (2022). Ginsenoside Rg1 improves Alzheimer’s disease by regulating oxidative stress, apoptosis, and neuroinflammation through Wnt/GSK-3β/β-catenin signaling pathway. Chem. Biol. Drug Des. 99, 884–896. doi:10.1111/cbdd.14041
Yao, Y., Li, C., Qian, F., Zhao, Y., Shi, X., Hong, D., et al. (2021). Ginsenoside Rg1 inhibits microglia pyroptosis induced by lipopolysaccharide through regulating STAT3 signaling. J. Inflamm. Res. 14, 6619–6632. doi:10.2147/JIR.S326888
Zhang, H., Su, Y., Sun, Z., Chen, M., Han, Y., Li, Y., et al. (2021). Ginsenoside Rg1 alleviates Aβ deposition by inhibiting NADPH oxidase 2 activation in APP/PS1 mice. J. Ginseng Res. 45, 665–675. doi:10.1016/j.jgr.2021.03.003
Zhang, L., Gao, X., Yang, C., Liang, Z., Guan, D., Yuan, T., et al. (2024). Structural characters and pharmacological activity of protopanaxadiol-type saponins and protopanaxatriol-type saponins from ginseng. Adv. Pharmacol. Pharm. Sci. 2024, 9096774. doi:10.1155/2024/9096774
Zhang, W., Chen, H., Ding, L., Gong, J., Zhang, M., Guo, W., et al. (2021). Trojan horse delivery of 4,4′-dimethoxychalcone for parkinsonian neuroprotection. Adv. Sci. (Weinh) 8, 2004555. doi:10.1002/advs.202004555
Zhang, Y., Zhang, Z., Wang, H., Cai, N., Zhou, S., Zhao, Y., et al. (2016). Neuroprotective effect of ginsenoside Rg1 prevents cognitive impairment induced by isoflurane anesthesia in aged rats via antioxidant, anti-inflammatory and anti-apoptotic effects mediated by the PI3K/AKT/GSK-3β pathway. Mol. Med. Rep. 14, 2778–2784. doi:10.3892/mmr.2016.5556
Zhang, Y. J., Zhu, W. K., Qi, F. Y., and Che, F. Y. (2023). CircHIPK3 promotes neuroinflammation through regulation of the miR-124-3p/STAT3/NLRP3 signaling pathway in Parkinson’s disease. Adv. Clin. Exp. Med. 32, 315–329. doi:10.17219/acem/154658
Zhang, Z., Yang, K., Mao, R., Zhong, D., Xu, Z., Xu, J., et al. (2022). Ginsenoside Rg1 inhibits oxidative stress and inflammation in rats with spinal cord injury via Nrf2/HO-1 signaling pathway. Neuroreport 33, 81–89. doi:10.1097/WNR.0000000000001757
Zhong, S. J., Wang, L., Gu, R. Z., Zhang, W. H., Lan, R., and Qin, X. Y. (2020). Ginsenoside Rg1 ameliorates the cognitive deficits in D-galactose and AlCl(3)-induced aging mice by restoring FGF2-Akt and BDNF-TrkB signaling axis to inhibit apoptosis. Int. J. Med. Sci. 17, 1048–1055. doi:10.7150/ijms.43979
Zhou, T., Zu, G., Zhang, X., Wang, X., Li, S., Gong, X., et al. (2016). Neuroprotective effects of ginsenoside Rg1 through the Wnt/β-catenin signaling pathway in both in vivo and in vitro models of Parkinson’s disease. Neuropharmacology 101, 480–489. doi:10.1016/j.neuropharm.2015.10.024
Zhou, T., Zu, G., Zhang, X., Wang, X., Li, S., Gong, X., et al. (2025). Corrigendum to “Neuroprotective effects of ginsenoside Rg1 through the Wnt/β-catenin signaling pathway in both in vivo and in vitro models of Parkinson’s disease” [Neuropharmacology 101 (2016) 480-489 NEUROPHARM-D-15-00626]. Neuropharmacology 265, 110260. doi:10.1016/j.neuropharm.2024.110260
Keywords: ginsenoside Rg1, Parkinson’s disease, anti-inflammatory, antioxidative, neuroprotection
Citation: Wang Q, Wei L, Chen G and Chen Q (2025) Ginsenoside Rg1 in Parkinson’s disease: from basic research to clinical applications. Front. Pharmacol. 16:1490480. doi: 10.3389/fphar.2025.1490480
Received: 03 September 2024; Accepted: 01 April 2025;
Published: 16 April 2025.
Edited by:
Jae-hyeon Park, National Institute on Aging (NIH), United StatesReviewed by:
Rajbir Singh, Patanjali Research Institute, IndiaNaveen Sharma, Chung-Ang University, Republic of Korea
Copyright © 2025 Wang, Wei, Chen and Chen. This is an open-access article distributed under the terms of the Creative Commons Attribution License (CC BY). The use, distribution or reproduction in other forums is permitted, provided the original author(s) and the copyright owner(s) are credited and that the original publication in this journal is cited, in accordance with accepted academic practice. No use, distribution or reproduction is permitted which does not comply with these terms.
*Correspondence: Qiang Chen, bHljaGVucWlhbmdAaHVzdC5lZHUuY24=
†These authors share first authorship