- The Key Laboratory of Environmental Pollution Monitoring and Disease Control, Ministry of Education, Department of Toxicology, School of Public Health, Guizhou Medical University, Guiyang, Guizhou, China
Arsenic is an environmental metalloid contaminant known to induce multi-system and multi-organ damage, yet the precise toxicological mechanisms remain unclear. Moreover, effective low-toxicity interventions or treatments are lacking. This study aims to investigate the potential ameliorative effects of Rosa roxburghii Tratt juice (RRTJ) on metabolic disorders in arsenicosis patients, with a focus on plasma metabolite profiles. Using ultra-high-performance liquid chromatography-tandem mass spectrometry (UHPLC-MS/MS), we analyzed the plasma metabolic profiles of arsenicosis patients before and after RRTJ intervention. After RRTJ intervention, significant alterations were observed in the plasma levels of 61 metabolites, with 30 metabolites upregulated and 31 downregulated. These metabolites were predominantly involved in six key biological pathways, including taurine and hypotaurine metabolism, histidine metabolism, β-alanine metabolism, glycine, serine, and threonine metabolism, pentose and glucuronate interconversions, as well as cysteine and methionine metabolism. In conclusion, RRTJ intervention may effectively alleviate metabolic disorders associated with arsenic toxicity, potentially through its antioxidant, anti-inflammatory effects and regulation of methylation pathways.
1 Introduction
Arsenic is an environmental metalloid contaminant that is widely present in water, food, and the environment (Kang et al., 2011). Ingestion of excessive arsenic can lead to its accumulation in vital organs such as the lungs, liver, kidneys, and skin, causing a variety of diseases, including cardiovascular diseases (Domingo-Relloso et al., 2022), disorders of the nervous system (Thakur et al., 2021), ailments affecting the digestive system (Zhong et al., 2021) and respiratory conditions (Wang W. et al., 2020). Research has demonstrated that the pathogenesis of systemic damage induced by arsenic primarily encompasses immune dysregulation, oxidative stress, epigenetic alterations and metabolic disturbances (Rahaman et al., 2021; Jamal et al., 2020; Chakraborty et al., 2022).
In recent years, food and drug dual-use substances have been widely recognized for their ability to regulate metabolic activities and alleviate disease processes (Khan et al., 2022; Bhambri et al., 2022). Rosa roxburghii Tratt (RRT), a fruit species endemic to Guizhou Province, is known for its rich nutrients and bioactive compounds, including vitamin C, flavonoids, polyphenolic compounds and triterpenoids (Xu et al., 2022). The presence of these natural constituents endows RRT with exceptional nutritional value and potential health benefits (Huang et al., 2022). We have previously demonstrated that the juice derived from freshly squeezed Rosa roxburghii Tratt exhibits immunoregulatory potential by significantly reducing the population of Th17 cells and modulating the Th17-related inflammatory cytokines IL-17 and IL-6 (Dong et al., 2022). Rosa roxburghii Tratt juice (RRTJ) can also reverse elemental imbalances caused by arsenic, such as elevated levels of Al, As, and Fe, and decreased levels of Cu, Zn, Se, etc., thereby regulating the body’s elemental balance (Xu et al., 2022). In vivo studies have demonstrated that RRTJ effectively counteracts liver oxidative damage in a rat model of arsenic poisoning by inhibiting the acetylation of histone H3K18 in the liver (Ma et al., 2023). Moreover, RRTJ polyphenols may indirectly mitigate lung injury by reducingphenylalanine levels and increasing tryptophan levels (Tang et al., 2022). Despite the various potential disease-alleviating mechanisms demonstrated by Rosa roxburghii Tratt preparations in in vitro experiments and animal models, the capacity of these preparations to modulate systemic health damage in arsenicosis patients remains to be explored through further epidemiological studies and omics analysis.
With the rapid development of omics technology, metabolomics has been extensively applied in various domains, including disease diagnosis, drug toxicity assessment, nutritional interventions and drug mechanism research, due to its high resolution, high sensitivity, and high throughput capabilities (Rispoli et al., 2021). Metabolomics is the scientific investigation of metabolites produced through endogenous reactions within the body, which closely reflect disease phenotypes. Most biological processes such as cell signaling, energy transfer and intercellular communication are complexly regulated by metabolites. Under specific physiological and pathological conditions, metabolites can serve as distinctive molecular markers while offering a comprehensive perspective on endogenous fluctuations in response to exogenous stimuli (Wang R. et al., 2020). Plasma metabolites can reflect changes in metabolic pathways associated with endogenous metabolites in a system, organ or whole organism. By detecting variations in plasma metabolites, a more precise understanding of the relationship between disease aetiology, drugs and disease development can be achieved (Qiu et al., 2023). Untargeted metabolomics can detect hundreds of small-molecule metabolites to find sensitive markers of disease. In toxicology research, the use of untargeted metabolomics technology can more quickly and effectively screen the targets of exogenous chemicals so as to adopt targeted treatment measures (Szeremeta et al., 2021). Our previous research has shown that arsenicosis patients exhibit metabolic disorders, which are associated with the severity of arsenic poisoning symptoms (Zhang et al., 2023). However, the potential of RRTJ to reverse the metabolic profile of arsenicosis patients remains uncertain. Therefore, this study employs untargeted metabolomics techniques to assess the impact of RRTJ on plasma metabolites in arsenicosis patients, aiming to provide a reference for subsequent mechanistic investigations.
To investigate the impact of RRTJ on metabolic disorders in arsenicosis patients, we initially conducted a comprehensive analysis of the plasma metabolic profiles of arsenicosis patients before and after an RRTJ intervention. Subsequently, we further scrutinized the differential metabolites before and after the RRTJ intervention to elucidate the influence of RRTJ on plasma metabolites. The ultimate goal of this research was to provide scientific evidence from a metabolomics perspective to support the potential application value of RRTJ in alleviating arsenic poisoning.
2 Materials and methods
2.1 Study design
A total of 46 arsenicosis patients (arsenicosis patients before the RRTJ intervention group, RRTJ-B) in Yuzhang Town, Xingyi City, Guizhou Province, China, participated in this study. Inclusion criteria: (1) all participants were permanent residents of Jiaole village; (2) have a history of arsenic exposure in coal-burning arsenic area; (3) were diagnosed with arsenicosis according to the Endemic Arsenic Poisoning Diagnostic Criteria (WS/T 211–2015). Exclusion criteria: (1) a history of occupational arsenic exposure; (2) a heritage metabolic disease or inborn metabolic deficiency; (3) a recent history of drugs that could affect metabolic function (Medications for Hypertension, Diabetes, and Cardiovascular Diseases). (4) individuals who themselves or their family members were allergic to RRTJ. Additionally, A total of 60 healthy villagers were randomly enrolled from Shang Batian village, an arsenic-free area, to form the reference group (RF). The reference group shared similar dietary habits, economic status, nutritional status, and lifestyle as the arsenicosis group, but did not have a history of using arsenic-rich coal or any signs of arsenic poisoning. Met the exclusion criteria for the arsenicosis group.
RRTJ was purchased from Sinopharm Group Guizhou Health Industry Development Co., LTD., National Health Commission, PRC Health Food License No. [2002]0004. RF did not receive any intervention measures. Arsenicosis patients were given 20 mL RRTJ per day (arsenicosis patients after the RRTJ intervention group, RRTJ-A), according to the recommended dosage in the health food instructions, for 3 months (Xu et al., 2022) (Figure 1).
Peripheral blood was collected into vacuum tubes containing ethylenediamine tetraacetic acid (EDTA). The tubes were centrifuged immediately, and the plasma was separated from the other blood components. The plasma was then dispensed and stored at −80°C.
Ethical approval for this study was obtained from the Ethics Committee of Guizhou Medical University (No. 201403001). Written informed consent was received from all participants before experimentation.
2.2 Metabolomics processing
The metabolomics analysis utilized the Agilent 1,290 Infinity LC system (Agilent Technologies, United States) in conjunction with the AB SCIEX Triple TOF 6600 system (AB SCIEX, United States), interfaced with an electrospray ionization source, and followed a non-targeted metabolomics protocol based on established procedures. Blood plasma samples were preprocessed by combining them with an equal volume of cold methanol/acetonitrile/water (2:2:1), then centrifuged at 14,000 g and 4°C for 15 min. Each quality control (QC) sample was assessed for measurement stability and reproducibility using a volume of 10 μL. Chromatographic separation occurred on an ACQUITY UPLC BEH column (2.1 × 100 mm, 2.1 μm particle size, Waters, United States). Prior to injection onto the column, blood plasma samples were dissolved in a mixture of methanol and ethanol (1:1) at a volume of 100 μL. The mobile phase consisted of water containing ammonium acetate and ammonia along with acetonitrile running at a flow rate of500 μL/min in a gradient elution program. Gas pressures for elution were set as follows: gas-1:60 psi; gas-2:60 psi; curtain gas:30 psi; spray voltages used for positive and negative modes were +5.5 kV and −5.5 kV respectively; source temperature maintained at600°C; precursor ion mass-to-charge ratio range from MS1 was between60-1,000 Da while product ions from MS2 ranged between25-1,000 Da; collision energy applied was35 ± 15 eV; desolvation voltage utilizedwas60 V.A window width exclusionof4Dafor isotopeswas also implemented. Once mass spectra measurementswere obtained, XCMS softwarewas employedfor metabolite extractionand annotation. The peak selection process involvedthe useofCentWave algorithm,and Metabolite Profile Annotationalgorithm suite (CAMERA)was utilizedfor annotatingisotopesand complexes.
2.3 Statistical analyses
The data were statistically analysed using MetaboAnalyst. T-tests and fold change (FC) analysis were used to compare two groups. Metabolites with a variable importance in projection (VIP) > 1.0, P < 0.05 and FC > 1.5 or FC < 0.67 (Zhu et al., 2022; Wang et al., 2023) were selected as potential biomarkers. MetaboAnalyst was also used for principal component analysis (PCA), partial least squares-discriminant analysis (PLS-DA), orthogonal partial least squares discriminant analysis (OPLS-DA) and heat map analysis to reflect the changes in each metabolite. Metabolic pathway analysis was performed using the Kyoto Encyclopaedia of Genes and Genomes (KEGG) database.
The general participant data were analysed using SPSS 22.0 software. Graphs were plotted using GraphPad Prism software, version 9.0. Quantitative data were tested for normality and homogeneity of variance. Normally distributed data are presented as the mean ± standard deviation (SD), and independent samples t-tests were used for two-group comparisons. The Chi-square test was used to compare rates between groups. Differences were considered statistically significant at P < 0.05.
3 Results
3.1 Demographic data of the study population
The sample comprised 106 participants, including 46 arsenicosis patients and 60 reference individuals. The reference residents and arsenicosis patients exhibited similar characteristics in terms of age, gender, as well as smoking and alcohol consumption history, indicating a fundamental consistency in the demographic structure and lifestyle among between the two groups (all P > 0.05, Table 1).
3.2 RRTJ ameliorated plasma metabolic spectrum disorders in arsenicosis patients
To investigate the effect of RRTJ on the metabolics of arsenicosis patients, we comprehensively analysed the plasma metabolic profiles and metabolites of the RF, RRTJ-B and RRTJ-A groups using ultra-high performance liquid chromatography-tandem mass spectrometer (UHPLC-MS/MS). A total of 1,264 metabolites were identified in the plasma samples, with 807 detected in positive ion mode and 457 detected in negative ion mode. Subsequently, PCA score plots were employed to examine the differences between the RF, RRTJ-B and RRTJ-A groups. The PCA plots are shown in Figures 2A,B. The separation between the RF and RRTJ-B groups was significant in the PCA score plot, indicating that the plasma metabolites of the arsenicosis patients had changed significantly. In addition, the samples in the RRTJ-A group were closer to those in the RF group, indicating that RRTJ reversed the metabolic disorder of arsenicosis patients to some extent.
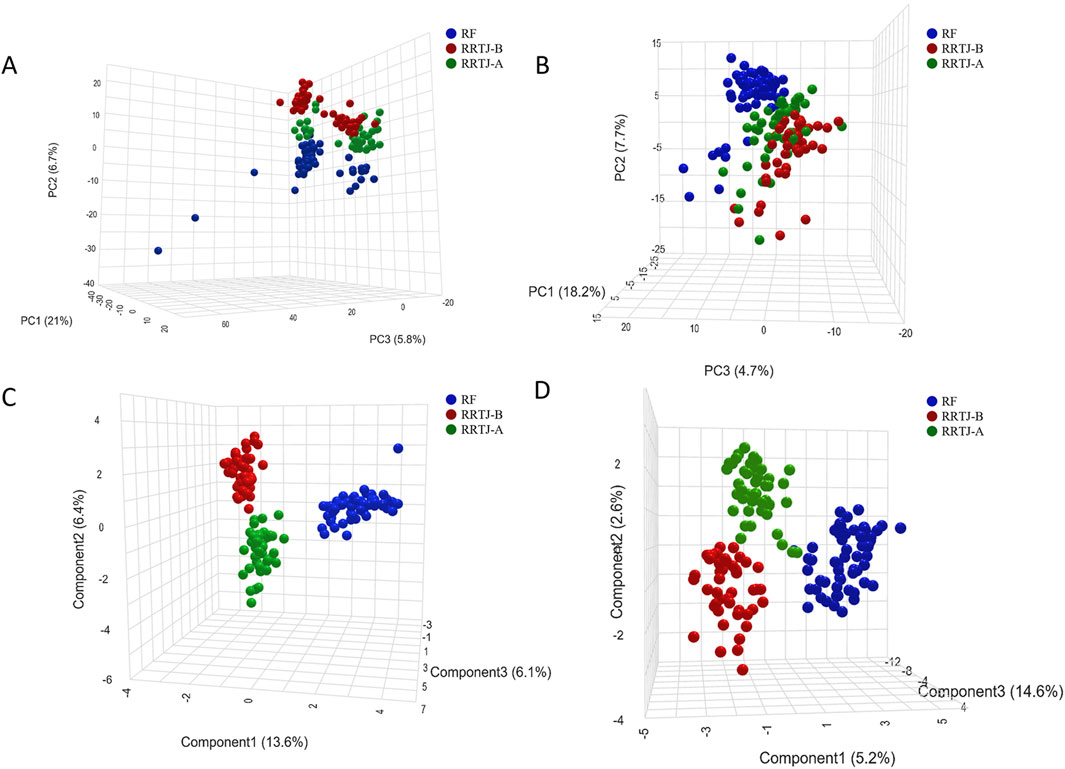
Figure 2. Metabolite changes in the plasma across groups were analyzed using PCA (A,B) and PLS-DA (C,D) score plot.
To further investigate the effect of RRTJ on the plasma metabolic profile of arsenicosis patients, a supervised partial least squares discriminant analysis (PLS-DA) model was applied. The PLS-DA score plots (Figures 2C,D) showed clear separation among the RF, RRTJ-B and RRTJ-A groups, and clustered distinctly in both the positive and negative ion modes, indicating that the group differences were more remarkable than the individual differences. The plasma metabolic profile of the arsenicosis patients after the RRTJ intervention was significantly separated from that of the arsenicosis patients before the RRTJ intervention, indicating that RRTJ affected the metabolic profile of arsenicosis patients and improved the endogenous metabolite disorder. These findings highlight the substantial alterations in the plasma metabolic profile of arsenicosis patients following an RRTJ intervention.
3.3 RRTJ restored multiple plasma metabolite levels in arsenicosis patients
To reveal the effect of RRTJ on metabolites in arsenicosis patients, supervised multivariate analysis was performed to further compare the plasma metabolites of the RF and RRTJ-B groups, as well as the RRTJ-B and RRTJ-A groups. OPLS-DA is a supervised method of multivariate analysis. As shown in Figure 3, OPLS-DA revealed good separation of the RF and RRTJ-B groups in both positive and negative ion modes. Similarly, the RRTJ-A group was clearly separated from the RRTJ-B group. This verifies the stability of the OPLS-DA model. These data suggest that the between-group differences are much larger than the within-group differences.
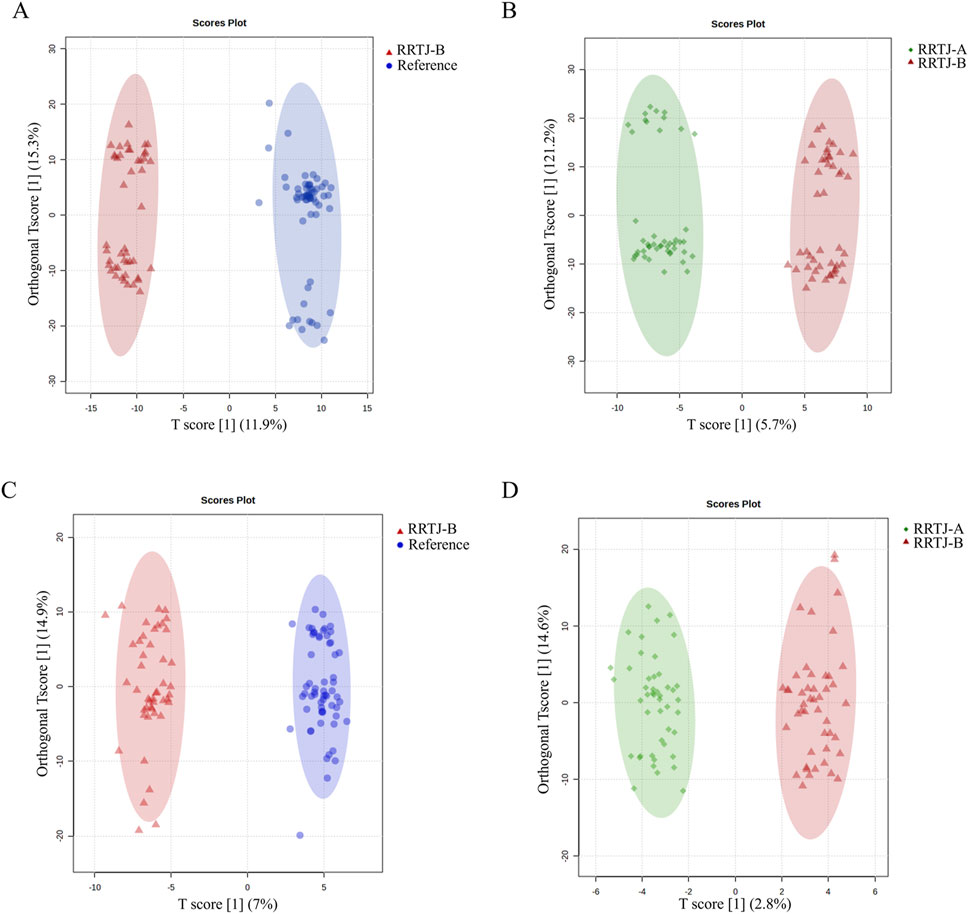
Figure 3. The changes in plasma metabolites among groups were analyzed using OPLS-DA in both positive ion mode (A,B) and negative ion mode (C,D).
A VIP>1 indicates that a metabolite contributes significantly to the differentiation between the two groups and serve as a potential metabolite biomarker of RRTJ. In addition, based on previous research, P < 0.05, FC > 1.5 or FC < 0.67 were also used to identify potential metabolites of RRTJ to alleviate arsenic toxicity (Fu et al., 2023; Zhou et al., 2023). The volcano plot (Figure 4A) revealed 394 differential metabolites between the RF and RRTJ-B groups. Among them, 196 were upregulated and 198 were downregulated. Figure 4B shows that there were 159 differential metabolites between the RRTJ-B and RRTJ-A groups. Of these, 76 were upregulated and 83 were downregulated.
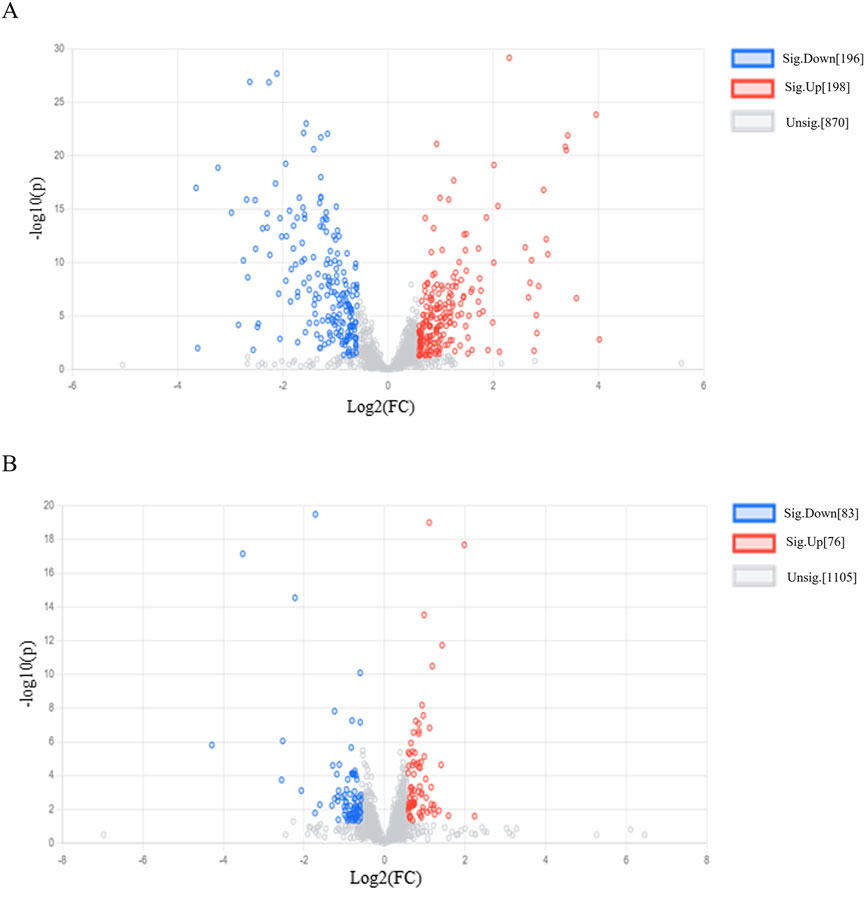
Figure 4. Volcanic plots of plasma metabolite. (A) RRTJ-B group vs. RF group, (B) RRTJ-A group vs. RRTJ-B group.
A Venn diagram was further constructed to identify intersecting metabolites. As shown in the Venn diagram (Figure 5), a total of 61 metabolites were significantly modulated by RRTJ (Table 2). Among them, 30 metabolites, including L-carnosine, 2-isopropylmalic acid, arg-gly and D-xylose, were upregulated (P < 0.05), and 31 metabolites, including dimethylglycine, taurine, Dl-homocysteine, hypoxanthine and prostaglandin f2. α, were downregulated in the RRTJ-A group compared to the RRTJ-B group (P < 0.05, Figure 6). To simultaneously visualize the contents of all potential targeted metabolites in the different groups, a heat map was constructed (Figure 7). In this heat map, red to blue represents a reduced metabolite content. The heatmap results indicated that RRTJ significantly reversed the abnormal levels of 61 metabolites and returned their expression levels to normal.
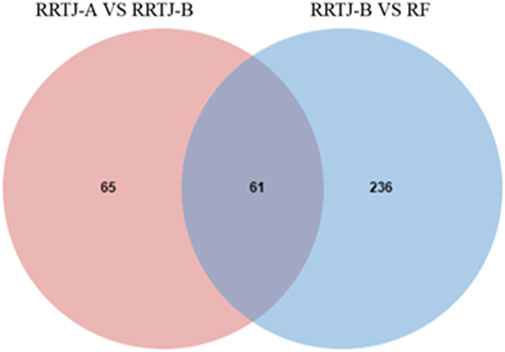
Figure 5. Venn diagram of differential metabolite between RRTJ-B group vs. RF group and RRTJ-A group vs. RRTJ-B group.
3.4 RRTJ ameliorates metabolic disorder in arsenicosis patients through various metabolic pathways
Metabolomics pathway analysis was conducted using MetaboAnalyst 6.0 to identify and visualize the potential metabolic pathways impacted by RRTJ. The identified 61 biomarkers were found to be involved in six metabolic pathways in which the pathway impact was greater than 0.05. The six pathways were taurine and hypotaurine metabolism, histidine metabolism, β-alanine metabolism, glycine, serine and threonine metabolism, pentose and glucuronate interconversions, and cysteine and methionine metabolism (Figure 8). These findings suggest a significant relationship between metabolite abnormalities and these metabolic pathways in arsenicosis patients. In particular, RRTJ may exert its effects through antioxidant, anti-inflammatory, and regulatory dysmethylation mechanisms, thereby alleviating arsenic poisoning.
4 Discussion
Based on untargeted metabolomics, this study analyzed the protective effect of RRTJ on metabolic disorders in arsenicosis patients, and initially explored the key metabolites and associated metabolic pathways involved in the potential mechanisms. The findings of the study demonstrate that RRTJ intervention has affected 61 differential metabolites. These metabolites were mainly enriched in six metabolic pathways, including taurine and hypotaurine metabolism, histidine metabolism, β-alanine metabolism, glycine, serine and threonine metabolism, pentose and glucuronate interconversions, and cysteine and methionine metabolism. These pathways are closely associated with antioxidant defence, anti-inflammatory responses, and the synthesis of methyl donors.
RRTJ exhibits a wide range of biological activities, mainly due to its high content of active compounds including flavonoids, vitamin C, polyphenols and polysaccharides (Jain et al., 2024). After these ingredients into the body, can adjust the variety of metabolites play a role of healthcare (Ji et al., 2022). Among them, polyphenols and flavonoids can significantly increase the content of glutathione (GSH) in plasma, and enhance the body’s antioxidant defense and immune capacity (Lapenna, 2023; Tang et al., 2023; Grabez et al., 2022). In addition, vitamin C and polyphenols may reduce plasma homocysteine (Hcy) levels by regulating the activity of enzymes in the methionine cycle (Ammar et al., 2020; Wu et al., 2024).
Studies have shown that individuals with arsenic poisoning exhibit severe oxidative and antioxidant imbalances (Hu et al., 2020). Enhancing antioxidant capacity is considered an effective approach to alleviate arsenic poisoning. RRT is a natural product known to have antioxidant capacity. On one hand, RRTJ can reduce the generation of ROS. Arsenic exposure leads to elevated levels of hypoxanthine, which induces oxidative stress by generating excessive ROS, resulting in cellular dysfunction and ultimately apoptosis or necrosis (Ryu et al., 2016; Biasibetti-Brendler et al., 2018). This process promotes multi-organ damage in arsenic poisoning (Kim et al., 2017; Toledo-Ibelles et al., 2021). Following RRTJ intervention, plasma hypoxanthine levels significantly decreased, thus diminishing ROS production. On the other hand, RRTJ also functions to scavenge ROS. L-carnosine levels are reduced in arsenic poisoning and can serve as a marker to distinguish the severity of arsenicosis (Zhang et al., 2023). L-carnosine contains numerous polyphenolic molecules that can scavenge ROS free radicals, thereby reducing malondialdehyde (MDA), superoxide dismutase (SOD), glutathione peroxidase (GPx), and catalase, and thus preventing cellular oxidative damage (Caruso et al., 2019; Park et al., 2022; Mousa and Aldebasi, 2021). In this study, RRTJ significantly increased the plasma concentration of L-carnosine, thereby promoting its ROS-scavenging and antioxidant effects. Additionally, we found that the levels of the oxidative stress biomarker prostaglandin F2-α and the antioxidant taurine were significantly reduced following RRTJ intervention (Nagui et al., 2022). Arsenic exposure induces oxidative stress, leading to a compensatory increase in taurine levels to exert antioxidant functions. After RRTJ intervention, oxidative stress in arsenicosis patients was partially alleviated, with levels of prostaglandin F2-α and taurine gradually decreasing.
Dysregulated inflammation responses is the crucial mechanisms that cause the health hazard of arsenism patients (Liu et al., 2022; Wang et al., 2021). In this study, we found that the effects of RRTJ on metabolites are closely associated with the regulation of imbalanced inflammatory responses. 2-isopropylmalate is a metabolite that protects cells from damage. Interestingly, our findings showed that RRTJ increased the concentration of 2-isopropylmalate in the plasma of arsenicosis patients. An increase in the 2-isopropylmalate content can inhibit the expression of the pro-inflammatory cytokines IL-6 and TNF-α, and the activation of NF-κB in cells, thereby exerting an anti-inflammatory effect (Bae et al., 2021; Choi et al., 2021). The other metabolite, D-xylose, is a product of the direct metabolism of xylitol by xylose reductase and the coenzyme NAD(P)+. The significant increase in D-xylose after the RRTJ intervention may further prevent injury and inflammation by stimulating the synthesis and secretion of proteoglycan (PG) and glycosaminoglycan (GAG) in cells and preventing the degradation of heparan sulphate (Cheudjeu, 2020). Together, these results suggest that RRTJ exerts an anti-inflammatory effect by increasing the contents of 2-isopropylmalate and D-xylose.
Arsenic can deplete S-adenosylmethionine (SAM) through As3MT, resulting in an insufficient supply of SAM. This blocks the methylation modification, disturbing the normal physiological function of genes, resulting in a toxic effect (Li et al., 2023). The glycine, serine and threonine cycle as well as the methionine cycle are closely related to one-carbon metabolism. The findings of the current study revealed a significant decrease in the plasma glycine level and a significant increase in the homocysteine level in arsenicosis patients. A reduction in the glycine level leads to decreased SAM synthesis while an elevation of the homocysteine level reflects increased SAM consumption. Both factors affect proper body methylation modification. Glycine contributes carbon units for one-carbon metabolism and participates in glutathione and SAM synthesis. Our previous in vivo experiments demonstrated that an RRTJ intervention can increase the SAM supply, regulate the H3K36me3 methylation level, and improve arsenic-induced liver injury in rats (Ma et al., 2023). By comparing the plasma glycine and homocysteine levels before and after the RRTJ intervention among arsenic poisoning patients, the current study observed a significant increase in the glycine content accompanied by a notable decrease in the homocysteine content. RRTJ intervention promotes an elevated SAM content by supplementing the body with glycine (Ling et al., 2022). Simultaneously, it binds to methyltransferases using SAM as a cofactor for protein, small-molecule, lipid and nucleic acid methylation to maintain normal physiological function within the body (Winter et al., 2022). The reduction in the homocysteine level following the RRTJ intervention in this study can be attributed to its conversion into SAM through binding with ingested methyl groups, thereby providing the body with necessary methyl donors (Mohammad and Kowluru, 2020). Therefore, it is speculated that RRTJ can restore arsenic-induced SAM deficiency by promoting glycine synthesis and reducing the homocysteine content in the body to alleviate arsenic-induced methylation disorder.
We have previously explored the antagonistic effects of RRTJ on immune imbalance, oxidative stress and methylation disorder induced by arsenic. On this basis, this study conducted an RRTJ intervention in arsenicosis patients, further supported the aforementioned viewpoints at the plasma metabolite level, and obtained 61 kinds of metabolites with possible effects such as L-carnosine, Dl-homocysteine, prostaglandin f2. α. This provides insights for subsequent research on the mechanism of alleviating arsenic poisoning from a metabolic perspective. Despite its strengths, this study still has some limitations. Firstly, this was an observational study, and the mechanism by which potential metabolites targeted by RRTJ to alleviate arsenicosis still need to be further verified in future in vitro experiments. Secondly, RRTJ contains a variety of active components, and subsequent research could explore which specific components play key roles in inducing changes in metabolites.
5 Conclusion
This study demonstrated that RRTJ can regulating metabolic disorder in arsenicosis patients. The restoration of 61 different metabolites may exert antioxidant, anti-inflammatory, and methylation donor synthesis functions through multiple metabolic pathways. These findings provide new insights into the potential mechanisms by which RRTJ may protect against arsenic poisoning.
Data availability statement
The raw data supporting the conclusions of this article will be made available by the authors, without undue reservation.
Ethics statement
Ethical approval for this study was obtained from the Ethics Committee of Guizhou Medical University (No. 201403001). The studies were conducted in accordance with the local legislation and institutional requirements. The participants provided their written informed consent to participate in this study.
Author contributions
LY: Visualization, Writing – original draft, Writing – review and editing. KZ: Investigation, Writing – original draft, Writing – review and editing. WW: Visualization, Writing – review and editing. BS: Data curation, Investigation, Writing – review and editing. PL: Investigation, Writing – review and editing. AZ: Conceptualization, Data curation, Formal Analysis, Funding acquisition, Investigation, Methodology, Project administration, Resources, Supervision, Writing – review and editing.
Funding
The author(s) declare that financial support was received for the research and/or publication of this article. This work was supported by the Key project of National Natural Science Foundation of China (No. 81430077).
Conflict of interest
The authors declare that the research was conducted in the absence of any commercial or financial relationships that could be construed as a potential conflict of interest.
Publisher’s note
All claims expressed in this article are solely those of the authors and do not necessarily represent those of their affiliated organizations, or those of the publisher, the editors and the reviewers. Any product that may be evaluated in this article, or claim that may be made by its manufacturer, is not guaranteed or endorsed by the publisher.
References
Ammar, A., MounaTurki, Trabelsi, K., Bragazzi, N. L., Boukhris, O., Bouaziz, M., et al. (2020). Effects of natural polyphenol-rich pomegranate juice on the acute and delayed response of Homocysteine and steroidal hormones following weightlifting exercises: a double-blind, placebo-controlled trial. J. Int. Soc. Sports Nutr. 17 (1), 15. doi:10.1186/s12970-020-00345-w
Bae, J. E., Min, D., Choi, J. Y., Choi, H., Kim, J. B., Park, N. Y., et al. (2021). Primary ciliogenesis by 2-isopropylmalic acid prevents pm2.5-induced inflammatory response and MMP-1 activation in human dermal fibroblasts and a 3-D-skin model. Int. J. Mol. Sci. 22 (20), 10941. doi:10.3390/ijms222010941
Bhambri, A., Srivastava, M., Mahale, V. G., Mahale, S., and Karn, S. K. (2022). Mushrooms as potential sources of active metabolites and medicines. Front. Microbiol. 13, 837266. doi:10.3389/fmicb.2022.837266
Biasibetti-Brendler, H., Schmitz, F., Pierozan, P., Zanotto, B. S., Prezzi, C. A., de Andrade, R. B., et al. (2018). Hypoxanthine induces neuroenergetic impairment and cell death in striatum of young adult wistar rats. Mol. Neurobiol. 55 (5), 4098–4106. doi:10.1007/s12035-017-0634-z
Caruso, G., Fresta, C. G., Fidilio, A., O'Donnell, F., Musso, N., Lazzarino, G., et al. (2019). Carnosine decreases PMA-induced oxidative stress and inflammation in murine macrophages. Antioxidants (Basel) 8 (8), 281. doi:10.3390/antiox8080281
Chakraborty, A., Ghosh, S., Biswas, B., Pramanik, S., Nriagu, J., and Bhowmick, S. (2022). Epigenetic modifications from arsenic exposure: a comprehensive review. Sci. Total Environ. 810, 151218. doi:10.1016/j.scitotenv.2021.151218
Cheudjeu, A. (2020). Correlation of D-xylose with severity and morbidity-related factors of COVID-19 and possible therapeutic use of D-xylose and antibiotics for COVID-19. Life Sci. 260, 118335. doi:10.1016/j.lfs.2020.118335
Choi, J. Y., Bae, J. E., Kim, J. B., Jo, D. S., Park, N. Y., Kim, Y. H., et al. (2021). 2-IPMA ameliorates PM2.5-induced inflammation by promoting primary ciliogenesis in RPE cells. Molecules 26 (17), 5409. doi:10.3390/molecules26175409
Domingo-Relloso, A., Makhani, K., Riffo-Campos, A. L., Tellez-Plaza, M., Klein, K. O., Subedi, P., et al. (2022). Arsenic exposure, blood DNA methylation, and cardiovascular disease. Circ. Res. 131 (2), e51–e69. doi:10.1161/CIRCRESAHA.122.320991
Dong, L., Xia, S., Sun, B., Ma, L., Chen, X., Wei, S., et al. (2022). Potential value and mechanism of Rosa roxburghii tratt juice on pro-inflammatory responses in peripheral blood of patients with arsenic poisoning. Hum. Exp. Toxicol. 41, 9603271221121313. doi:10.1177/09603271221121313
Fu, J., Li, J., Sun, Y., Liu, S., Song, F., and Liu, Z. (2023). An integrated study on the comprehensive mechanism of Schisandra chinensis polysaccharides mitigating Alzheimer's disease in rats using a UPLC-Q-TOF-MS based serum and urine metabolomics strategy. Food Funct. 14 (2), 734–745. doi:10.1039/d2fo02842e
Grabez, M., Skrbic, R., Stojiljkovic, M. P., Vučić, V., Rudić Grujić, V., Jakovljević, V., et al. (2022). A prospective, randomized, double-blind, placebo-controlled trial of polyphenols on the outcomes of inflammatory factors and oxidative stress in patients with type 2 diabetes mellitus. Rev. Cardiovasc Med. 23 (2), 57. doi:10.31083/j.rcm2302057
Huang, D., Li, C., Chen, Q., Xie, X., Fu, X., Chen, C., et al. (2022). Identification of polyphenols from Rosa roxburghii Tratt pomace and evaluation of in vitro and in vivo antioxidant activity. Food Chem. 377, 131922. doi:10.1016/j.foodchem.2021.131922
Hu, Y., Xiao, T., Wang, Q., Liang, B., and Zhang, A. (2020). Effects of essential trace elements and oxidative stress on endemic arsenism caused by coal burning in PR China. Biol. Trace Elem. Res. 198 (1), 25–36. doi:10.1007/s12011-020-02047-5
Jain, A., Sarsaiya, S., Gong, Q., Wu, Q., and Shi, J. (2024). Chemical diversity, traditional uses, and bioactivities of Rosa roxburghii Tratt: a comprehensive review. Pharmacol. Ther. 259, 108657. doi:10.1016/j.pharmthera.2024.108657
Jamal, Z., Das, J., Ghosh, S., Gupta, A., Chattopadhyay, S., and Chatterji, U. (2020). Arsenic-induced immunomodulatory effects disorient the survival-death interface by stabilizing the Hsp90/Beclin1 interaction. Chemosphere 238, 124647. doi:10.1016/j.chemosphere.2019.124647
Ji, J., Zhang, S., Yuan, M., Zhang, M., Tang, L., Wang, P., et al. (2022). Fermented Rosa roxburghii tratt juice alleviates high-fat diet-induced hyperlipidemia in rats by modulating gut microbiota and metabolites. Front. Pharmacol. 13, 883629. doi:10.3389/fphar.2022.883629
Kang, Y., Liu, G., Chou, C. L., Wong, M. H., Zheng, L., and Ding, R. (2011). Arsenic in Chinese coals: distribution, modes of occurrence, and environmental effects. Sci. Total Environ. 412-413, 1–13. doi:10.1016/j.scitotenv.2011.10.026
Khan, T. J., Xu, X., Xie, X., Dai, X., Sun, P., Xie, Q., et al. (2022). Tremella fuciformis crude polysaccharides attenuates steatosis and suppresses inflammation in diet-induced NAFLD mice. Curr. Issues Mol. Biol. 44 (3), 1224–1234. doi:10.3390/cimb44030081
Kim, Y. J., Ryu, H. M., Choi, J. Y., Cho, J. H., Kim, C. D., Park, S. H., et al. (2017). Hypoxanthine causes endothelial dysfunction through oxidative stress-induced apoptosis. Biochem. Biophys. Res. Commun. 482 (4), 821–827. doi:10.1016/j.bbrc.2016.11.119
Lapenna, D. (2023). Glutathione and glutathione-dependent enzymes: from biochemistry to gerontology and successful aging. Ageing Res. Rev. 92, 102066. doi:10.1016/j.arr.2023.102066
Li, H., Wu, L., Ye, F., Wang, D., Wang, L., Li, W., et al. (2023). As3MT via consuming SAM is involved in arsenic-induced nonalcoholic fatty liver disease by blocking m(6)A-mediated miR-142-5p maturation. Sci. Total Environ. 892, 164746. doi:10.1016/j.scitotenv.2023.164746
Ling, H., Li, M., Yang, C., Sun, S., Zhang, W., Zhao, L., et al. (2022). Glycine increased ferroptosis via SAM-mediated GPX4 promoter methylation in rheumatoid arthritis. Rheumatol. Oxf. 61 (11), 4521–4534. doi:10.1093/rheumatology/keac069
Liu, Y., Wang, W., Zou, Z., Sun, B., Liang, B., and Zhang, A. (2022). Association and risk of circulating inflammatory markers with hyperglycemia in coal-burning arsenicosis. Ecotoxicol. Environ. Saf. 247, 114208. doi:10.1016/j.ecoenv.2022.114208
Ma, L., Hou, T., Zhu, K., and Zhang, A. (2023). Inhibition of histone H3K18 acetylation-dependent antioxidant pathways involved in arsenic-induced liver injury in rats and the protective effect of Rosa roxburghii tratt juice. Toxics 11 (6), 503. doi:10.3390/toxics11060503
Mohammad, G., and Kowluru, R. A. (2020). Homocysteine disrupts balance between MMP-9 and its tissue inhibitor in diabetic retinopathy: the role of DNA methylation. Int. J. Mol. Sci. 21 (5), 1771. doi:10.3390/ijms21051771
Mousa, A. M., and Aldebasi, Y. H. (2021). L-carnosine mitigates interleukin-1α-induced dry eye disease in rabbits via its antioxidant, anti-inflammatory, antiapoptotic, and antifibrotic effects. Cutan. Ocul. Toxicol. 40 (3), 241–251. doi:10.1080/15569527.2021.1935995
Nagui, N. A., EL-Tartoushy, S. A., Rashed, L. A., and Elmasry, M. F. (2022). Assessment of prostaglandin F2-alpha (PGF2α) in lesional and nonlesional skin of vitiligo patients. Int. J. Dermatol 61 (11), 1390–1396. doi:10.1111/ijd.16307
Park, J., Jang, J., Cha, S. R., Baek, H., Lee, J., Hong, S. H., et al. (2022). L-Carnosine attenuates bleomycin-induced oxidative stress via NFκB pathway in the pathogenesis of pulmonary fibrosis. Antioxidants (Basel) 11 (12), 2462. doi:10.3390/antiox11122462
Qiu, S., Cai, Y., Yao, H., Lin, C., Xie, Y., Tang, S., et al. (2023). Small molecule metabolites: discovery of biomarkers and therapeutic targets. Signal Transduct. Target Ther. 8 (1), 132. doi:10.1038/s41392-023-01399-3
Rahaman, M. S., Rahman, M. M., Mise, N., Sikder, M. T., Ichihara, G., Uddin, M. K., et al. (2021). Environmental arsenic exposure and its contribution to human diseases, toxicity mechanism and management. Environ. Pollut. 289, 117940. doi:10.1016/j.envpol.2021.117940
Rispoli, M. G., Valentinuzzi, S., DE Luca, G., Del Boccio, P., Federici, L., Di Ioia, M., et al. (2021). Contribution of metabolomics to multiple sclerosis diagnosis, prognosis and treatment. Int. J. Mol. Sci. 22 (20), 11112. doi:10.3390/ijms222011112
Ryu, H. M., Kim, Y. J., Oh, E. J., Oh, S. H., Choi, J. Y., Cho, J. H., et al. (2016). Hypoxanthine induces cholesterol accumulation and incites atherosclerosis in apolipoprotein E-deficient mice and cells. J. Cell Mol. Med. 20 (11), 2160–2172. doi:10.1111/jcmm.12916
Szeremeta, M., Pietrowska, K., Niemcunowicz-Janica, A., Kretowski, A., and Ciborowski, M. (2021). Applications of metabolomics in forensic toxicology and forensic medicine. Int. J. Mol. Sci. 22 (6), 3010. doi:10.3390/ijms22063010
Tang, L., Zhang, S., Zhang, M., Wang, P. J., Liang, G. Y., and Gao, X. L. (2022). Analysis of protective effects of Rosa Roxburghii Tratt fruit polyphenols on lipopolysaccharide-induced acute lung injury through network pharmacology and metabolomics. Food Sci. Nutr. 10 (12), 4258–4269. doi:10.1002/fsn3.3019
Tang, L., Zhang, S., Zhang, M., Wang, P. J., Liang, G. Y., and Gao, X. L. (2023). Integrated proteomics and metabolomics analysis to explore the amelioration mechanisms of Rosa roxburghii tratt fruit polyphenols on lipopolysaccharide-induced acute lung injury mice. J. Agric. Food Chem. 71 (6), 3079–3092. doi:10.1021/acs.jafc.2c04344
Thakur, M., Rachamalla, M., Niyogi, S., Datusalia, A. K., and Flora, S. J. S. (2021). Molecular mechanism of arsenic-induced neurotoxicity including neuronal dysfunctions. Int. J. Mol. Sci. 22 (18), 10077. doi:10.3390/ijms221810077
Toledo-Ibelles, P., Gutierrez-Vidal, R., Calixto-Tlacomulco, S., Delgado-Coello, B., and Mas-Oliva, J. (2021). Hepatic accumulation of hypoxanthine: a link between hyperuricemia and nonalcoholic fatty liver disease. Arch. Med. Res. 52 (7), 692–702. doi:10.1016/j.arcmed.2021.04.005
Wang, X. B., Wang, M. L., Chu, Y. J., Zhou, P. P., Zhang, X. Y., Zou, J., et al. (2023). Integrated pharmacokinetics and pharmacometabolomics to reveal the synergistic mechanism of a multicomponent Chinese patent medicine, Mailuo Shutong pills against thromboangiitis obliterans. Phytomedicine 112, 154709. doi:10.1016/j.phymed.2023.154709
Wang, R., Li, B., Lam, S. M., and Shui, G. (2020b). Integration of lipidomics and metabolomics for in-depth understanding of cellular mechanism and disease progression. J. Genet. Genomics 47 (2), 69–83. doi:10.1016/j.jgg.2019.11.009
Wang, W., Zheng, F., Lin, C., and Zhang, A. (2020a). Changes in energy metabolism and macrophage polarization: potential mechanisms of arsenic-induced lung injury. Ecotoxicol. Environ. Saf. 204, 110948. doi:10.1016/j.ecoenv.2020.110948
Wang, W., Zheng, F., and Zhang, A. (2021). Arsenic-induced lung inflammation and fibrosis in a rat model: contribution of the HMGB1/RAGE, PI3K/AKT, and TGF-β1/SMAD pathways. Toxicol. Appl. Pharmacol. 432, 115757. doi:10.1016/j.taap.2021.115757
Winter, A. D., Tjahjono, E., Beltran, L. J., Johnstone, I. L., Bulleid, N. J., and Page, A. P. (2022). Dietary-derived vitamin B12 protects Caenorhabditis elegans from thiol-reducing agents. BMC Biol. 20 (1), 228. doi:10.1186/s12915-022-01415-y
Wu, P., Ma, J., Yang, S., Wu, H., Ma, X., Chen, D., et al. (2024). Association between homocysteine and blood pressure in the NHANES 2003-2006: the mediating role of Vitamin C. Front. Nutr. 11, 1379096. doi:10.3389/fnut.2024.1379096
Xu, Y., Sun, B., Zeng, Q., Wei, S., Yang, G., and Zhang, A. (2022). Assessing the association of element imbalances with arsenism and the potential application value of Rosa roxburghii tratt juice. Front. Pharmacol. 13, 819472. doi:10.3389/fphar.2022.819472
Zhang, J., Ma, L., Li, B., Chen, X., Wang, D., and Zhang, A. (2023). Identification of biomarkers for risk assessment of arsenicosis based on untargeted metabolomics and machine learning algorithms. Sci. Total Environ. 870, 161861. doi:10.1016/j.scitotenv.2023.161861
Zhong, G., Wan, F., Lan, J., Jiang, X., Wu, S., Pan, J., et al. (2021). Arsenic exposure induces intestinal barrier damage and consequent activation of gut-liver axis leading to inflammation and pyroptosis of liver in ducks. Sci. Total Environ. 788, 147780. doi:10.1016/j.scitotenv.2021.147780
Zhou, S., Gao, X., Chen, C., Zhang, J., Zhang, Y., Zhang, L., et al. (2023). Porcine cardiac blood - Salvia miltiorrhiza root alleviates cerebral ischemia reperfusion injury by inhibiting oxidative stress induced apoptosis through PI3K/AKT/Bcl-2/Bax signaling pathway. J. Ethnopharmacol. 316, 116698. doi:10.1016/j.jep.2023.116698
Keywords: arsenic, arsenicosis patients, metabolic disorder, Rosa roxburghii Tratt juice, untargeted metabolomics
Citation: Yang L, Zhu K, Wang W, Sun B, Luo P and Zhang A (2025) Rosa roxburghii Tratt juice ameliorates metabolic disorder in arsenicosis patients based on the analysis of untargeted plasma metabolomics. Front. Pharmacol. 16:1488113. doi: 10.3389/fphar.2025.1488113
Received: 29 August 2024; Accepted: 11 April 2025;
Published: 22 April 2025.
Edited by:
Michael Heinrich, University College London, United KingdomReviewed by:
Jianqiang Xu, Dalian University of Technology, ChinaRuiwen Zhang, University of Houston, United States
Copyright © 2025 Yang, Zhu, Wang, Sun, Luo and Zhang. This is an open-access article distributed under the terms of the Creative Commons Attribution License (CC BY). The use, distribution or reproduction in other forums is permitted, provided the original author(s) and the copyright owner(s) are credited and that the original publication in this journal is cited, in accordance with accepted academic practice. No use, distribution or reproduction is permitted which does not comply with these terms.
*Correspondence: Aihua Zhang, YWlodWFnenlrZEAxNjMuY29t
†These authors have contributed equally to this work