- 1Department of Biomedical Sciences, College of Osteopathic Medicine of the Pacific Northwest, Western University of Health Sciences, Lebanon, OR, United States
- 2Department of Behavioral Neuroscience, Oregon Health and Science University, Portland, OR, United States
- 3Departments of Behavioral Neuroscience, Neurology, Psychiatry, and Radiation Medicine, Division of Neuroscience ONPRC, Oregon Health and Science University, Portland, OR, United States
The goal of this review is to highlight the role of second-hand smoke (SHS) or environmental tobacco smoke (ETS) and e-cigarette (EC) vapors on brain integrity and function during development and adulthood, including how it relates to increasing the risk for age-related neurodegenerative disorders. A systematic review of the literature of the effect of SHS or ETS and e-cigarette vapors on the brain revealed a total of 284 or 372 publications and 312 publications, respectively. After taking into account duplicate publications or publications focused on policy, surveys or other organs than brain, there are limited studies on the effects of SHS, ETS or EC vapors on brain structure and function. In this review, we examine the major constituents in SHS or EC vapors and their effects on brain health, mechanisms by which SHS or vapors alters brain integrity and function, including behavioral and cognitive performance. We hope that this review will encourage investigators to explore further the short-as well long-term effects of SHS or vapor exposure on the developing and adult brain to better understand its role in neurodevelopmental disorders and neurodegenerative diseases and ultimately to develop therapeutic modalities to reduce or even prevent the short- and long-term detrimental effects on brain health.
1 Introduction
Please see Figure 1 for the Prisma statement.
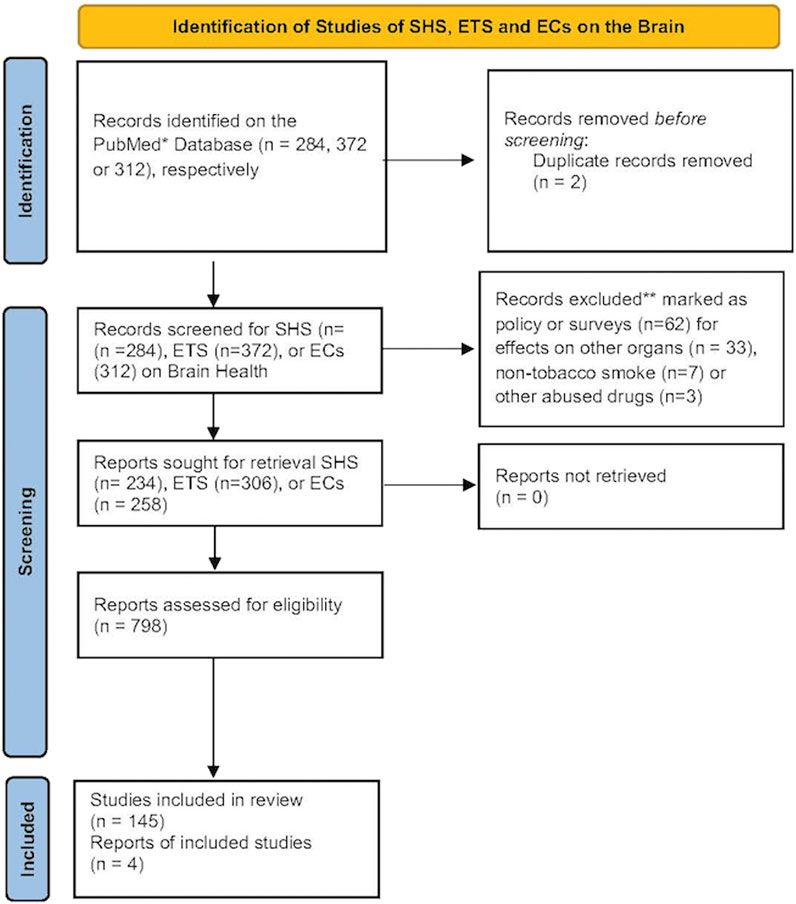
Figure 1. The number of records identified from the PubMed database was searched using the terms SHS, ETS or EC and brain. Records that were excluded from this review were those focusing primarily on policy or surveys, effects on non-nervous tissues, non-tobacco smoke or from other abused drugs. These reduced the number of records for each term that focused primarily on brain health. A document from the CDC on a visual dictionary of electronic cigarettes or vaping was also included in this review (Centers for Disease Control and Prevention, 2024).
Tobacco use is one of the leading risk factors for disease burden and mortality worldwide, contributing to 229.8 million (95% uncertainty interval: 213.1–246.4 million) disability-adjusted life years and 8.7 million (8.1–9.3 million) deaths in 2019 (Flor et al., 2024). Second-hand smoke (SHS) exposure, also referred to as passive or environmental tobacco smoke (ETS), is a major tobacco-related public health concern for nonsmokers. SHS increases the risk of nine health outcomes, including ischemic heart disease stroke, diabetes and lung cancer, but the effects on the nervous system have not been extensively examined (Flor et al., 2024). Although smoking rates have gradually declined over the past 50 years, ∼37% of the global population is still being exposed to the smoke emitted from the burning end of tobacco products (sidestream smoke, SS) or exhaled from smokers (mainstream smoke, MS), with higher rates of exposure reported among women and children compared to men (Flor et al., 2024). This is concerning, since tobacco smoke is composed of thousands of toxic chemicals and compounds, including many carcinogens, which when inhaled can lead to disease and death, especially among vulnerable populations.
SHS or ETS consists of sidestream (∼85%) and mainstream smoke (∼15%) (Soleimani et al., 2022). Mainstream smoke is exhaled from a smoker, while sidestream smoke is smoke emitted from a burning cigarette. Indoors, SHS can persist for hours to become more toxic with time and duration, a process known as aging (Schick and Glantz, 2005; Schick and Glantz, 2007). When second-hand smoke is released into the open air, it changes both chemically and physically. Human exposure to SHS depends on airflow patterns, dilution volume, the distance between smokers and non-smokers, and smoking prevalence (Public Health Service, 2006). The toxic chemicals in SHS can also react with atmospheric air to generate other toxins that can be inhaled (Centers for Disease Control and Prevention, 2010; Fu et al., 2012). SHS contains >7,000 chemicals with at least 70 of them being carcinogenic (Li and Hecht, 2022). Nicotine, polycyclic aromatic hydrocarbons (PAHs) and aldehydes (formaldehyde, acetaldehyde and propionaldehyde) are the most abundant chemical constituents commonly found in SHS. SHS also contains smaller amounts of metals and nitrosamines (Soleimani et al., 2022). However, aldehyde concentrations in SHS can exceed those of nicotine, depending upon exposure conditions (e.g., indoor vs outdoor places). PAHs, formaldehyde, and acetaldehyde all have the potential to damage DNA (genotoxins) associated with neurodevelopmental and neurological disorders (Perera et al., 2014; Joshi et al., 2019; Rana et al., 2021; Kou et al., 2022; Yuan et al., 2023). These genotoxic effects might also vary among different ethnic populations. For example, recent studies indicate that a mutation of the enzyme that metabolizes formaldehyde and acetaldehyde (aldehyde dehydrogenase 2, ALDH2) is highly prevalent (30%–50%) among the East Asian population (Chen et al., 2015; Wang X. et al., 2024), which can increase the susceptibility to second-hand exposure to toxins (Ma et al., 2024).
Electronic cigarettes (e.g., e-cigarettes, ECs, vape pens, etc.) or electronic nicotine delivery systems (ENDS) contain a liquid solution (“pods”) composed of various amounts of nicotine, flavoring substances, and other chemicals that is vaporized upon activation of an electronic heating element that is triggered by inhalation (Grana et al., 2014; Lopez-Ojeda and Hurley, 2024). Using ECs, is commonly known as ‘vaping. Details about the different types of ECs, their components and aerosols can be found in recent reviews by the Center for Disease Control (Centers for Disease Control and Prevention, 2024) and other investigators (Lopez-Ojeda and Hurley, 2024; Omaiye et al., 2022; Heywood et al., 2024). ECs have been promoted as containing fewer toxic chemicals than conventional cigarettes suggesting that these type of cigarettes are less harmful (Heywood et al., 2024; Hamann et al., 2023; Izquierdo-Condoy et al., 2024). However, the exhaled vapors from e-cigarettes also contains toxic chemicals and carcinogens (e.g., acrolein, benzene, diacetyl, formaldehyde) following second-hand exposure of individuals (Lopez-Ojeda and Hurley, 2024; Armendariz-Castillo et al., 2019; Yan et al., 2021). These findings suggest that e-cigarettes may be less harmful for smokers, but they are not safe for non-smokers (Izquierdo-Condoy et al., 2024). While there are many studies examining the neurological effects of e-cig vapors in humans (Hamann et al., 2023), there have been limited studies to investigate the direct effects of e-cigarette vapors on brain function and structure using animal models (Siegel et al., 2022; Ruszkiewicz et al., 2020). The vapors from e-cigarettes also contain significant amounts of nicotine, as well as other constituents found in SHS, but at lower concentrations (Ebersole et al., 2020) (Table 1). Formaldehyde can also be formed during the partial combustion of propylene glycol and glycerol liquids in e-cigarettes (Strongin et al., 2024), which reaches more deeply into the lungs than gaseous formaldehyde (Pankow, 2017).
Exposure to second-hand smoke (SHS) at different times during brain development (fetal, infant, and adolescence) can produce short- or long-term effects on brain structure and function leading to neurodevelopmental disorders (NDDs) (Slotkin et al., 2015; Lin et al., 2021; Ou et al., 2024). Children exposed from pregnancy to childhood have a higher risk of developing Attention Deficit Hyperactivity Disorder (ADHD) during school-aged years and this risk is somewhat stronger for SHS exposure during the prenatal and postnatal periods (Lin et al., 2021). A more recent study of SHS exposure and neurodevelopmental disorders also revealed that exposure is associated with higher risk of ADHD and other learning disabilities (Ou et al., 2024). Collectively, these studies demonstrate that SHS can induce short- and long-term structural effects on the developing brain to cause permanent functional changes. The contribution of individual toxins in cigarette on both brain development and function appears to be greater when they are combined (Slotkin et al., 2019). Combined exposure of pregnant rats to both a PAH (i.e., benzo [a]pyrene) and nicotine impairs acetylcholine presynaptic activity and upregulates acetylcholine and serotonin receptors in adolescent rats when compared with exposure to either agent alone. These studies demonstrate that exposure to combinations of SHS chemicals is more detrimental to the developing brain than single exposure to SHS chemicals. Since SHS contains more than 4,000 chemicals (Arfaeinia et al., 2023), two or more of them may be more detrimental to the developing brain, but studies assessing combinations of SHS chemicals have yet to been conducted.
2 Pharmacology and pathology of nicotine in the brain
Nicotine is one of the most abundant toxins in SHS (mg to µg quantities), next to aldehydes and polycyclic aromatic hydrocarbons (Arfaeinia et al., 2023), and is also produced after the heating of tobacco products (e.g., e-cigarettes) (Upadhyay et al., 2023). EC cigarette pods contain approximately 59.2–66.7 mg/mL of nicotine, which is comparable to one pack of 20 conventional cigarettes (Lopez-Ojeda and Hurley, 2024). Nicotine levels in e-cigarette aerosols can range anywhere from 0–50 mg/mL of liquid (Yan et al., 2021; Goniewicz et al., 2018; Prochaska et al., 2022) and are reportedly lower following exposure of individuals to vapors vs SHS (Tattan-Birch et al., 2024). This difference may be explained by the 99% retention of nicotine by vapors following inhalation (Czogala et al., 2014; St Helen et al., 2016). SHS inhaled from conventional cigarettes or e-cigarette vapors is absorbed into the pulmonary circulation where it binds to neuronal nicotinic acetylcholine receptors (nAChRs) that mediate fast neurotransmission in both the central and peripheral nervous system (Wells and Lotfipour, 2023). The inhaled nicotine causes the release of multiple neurotransmitters (e.g., dopamine, norepinepinephrine, acetylcholine, GABA and glutamate) in the reward/addiction pathways and involved in cognition, as well as activation of nicotinic receptors at the neuromuscular junction (Figure 2). Cholinergic receptors are located in several brain regions notably the midbrain tegmentum, the striatum, nucleus accumbens and the ventral tegmentum (VTA). The addictive properties of nicotine are reportedly due to activation of nACHRs in the brain to cause the release acetylcholine and dopamine in the nucleus acccumbens (Tiwari et al., 2020). GABAergic, serotonergic, noradrenergic, and brain stem cholinergic may also mediate the actions of nicotine on the brain. The addictive properties of nicotine may also be related to its activation of both dopaminergic neurons of VTA as well the GABA-ergic neurons (Varani et al., 2018). The activation of nicotinic receptors at the neuromuscular junction by SHS can also cause degeneration, consistent with a key role for smoke exposure causing denervation in patients with chronic pulmonary disease (Kapchinsky et al., 2018). In the CNS, nicotine modulates the reward/addiction pathways and cognition through activation of nAChRs in the mesocortical and mesolimbic dopaminergic (DA) pathways (Ikemoto and Bonci, 2014). The rewarding and cognitive effects of nicotine are mediated through the activation of mesocortical DA receptors in the prefrontal cortex and anterior cingulate cortex, while the activation of DA receptors by nicotine in the nucleus accumbens and amygdala modulate synaptic plasticity and long-term potentiation that are more important in addiction.
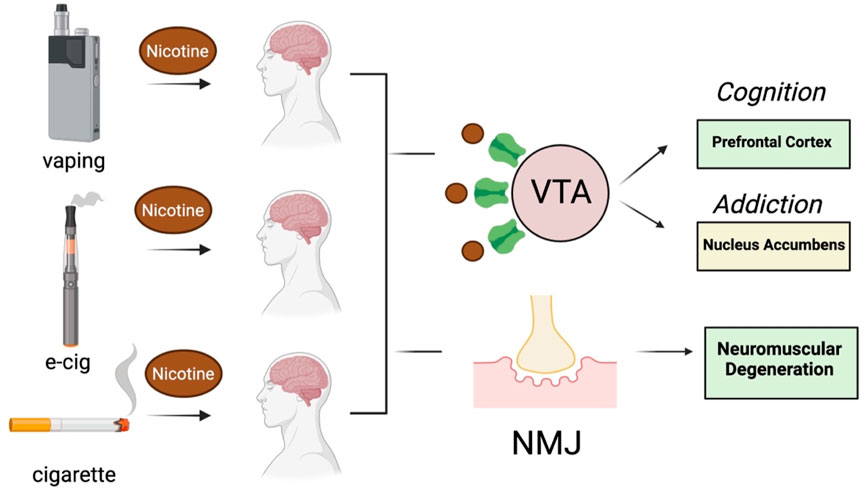
Figure 2. Effect of nicotine on brain and neuromuscular function following exposure to vapors or SHS generated by e-cigarettes and conventional cigarettes (respectively). VTA, ventral tegmentum area, NMJ, neuromuscular junction. Images were created with BioRender.
Inhaled nicotine can also have long-term effects on brain development, because nicotinic acetylcholine receptors (nAChRs) play a very important role in modulating the release of neurotransmitters during key stages of neurodevelopment (England et al., 2017). Nicotinic receptors regulate critical aspects of brain maturation during the prenatal, early postnatal, and adolescent periods (Dwyer et al., 2009). Nicotine interferes with catecholamine and brainstem autonomic nuclei development during the rodent prenatal period (first and second trimester in humans), alters the neocortex, hippocampus, and cerebellum during the early rodent postnatal period (third trimester in humans) and influences the limbic system and later monoamine-containing neuron maturation during adolescence (Dwyer et al., 2009). SHS exposure during fetal (prenatal) or neonatal (postnatal) brain development can also produce long-term effects on the developing brain to disrupt brain plasticity and overall brain structure (e.g., volume, thinning) to lead to neurodevelopmental disorders (e.g., ADHD, schizophrenia, ASD, and anxiety) (Ou et al., 2024; Herrmann et al., 2008; Colyer-Patel et al., 2023; Greenwood et al., 2024).
Prenatal exposure to nicotine from tobacco products might also produce neurodevelopmental delay through epigenetic changes (Buck et al., 2020; Gould, 2023; Hoang et al., 2024). DNA methylation changes are observed in mothers who are exposed to cigarette smoke during pregnancy (Markunas et al., 2014). Markunas and colleagues (Markunas et al., 2014) showed that DNA methylation is changed in 110 gene regions and notably FRMD4A, a gene associated with Alzheimer disease (AD) and nicotine dependence and CNTNAP2, a gene associates with neural development, autism spectrum disorder, schizophrenia, and language impairment. Another study (Rauschert et al., 2019) also revealed that tobacco use is associated with differential DNA methylation of both FRMD4A and CNTNAP2. More recently, Hoang and colleagues (Hoang et al., 2024) reported that in utero exposure to environmental tobacco smoke (ETS) alters DNA methylation of both CNTNAP2 and FRMD4a that might persist into adulthood. Collectively, these studies provide evidence that prenatal exposure to nicotine in tobacco smoke can alter brain processes involved in neural development, age-related neurodegenerative conditions like Alzheimer’s disease, and addiction.
Neurotrophins, like nerve growth factor (NGF) and brain-derived neurotrophic factor (BDNF), play important roles in neuronal development, function, and survival during early stages of both the central and peripheral nervous system (Ferraguti et al., 2023). In general, the brain peptide system plays an important role in nicotine addiction and drugs that target this system prevent the activation of reward systems (Bruijnzeel, 2017; Boiangiu et al., 2023). Exposure of the fetus to SHS during pregnancy appears to alter the expression of neuropeptides (BDNF, PCAP) by the region-specific activation of nicotinic receptors (Machaalani et al., 2019). Nicotine also increases brain-derived neurotrophic factor (BDNF) levels in the hippocampus and neocortex. Mice exposed to environmental tobacco smoke (ETS) during the first two postnatal weeks show lower locomotor activity, anxiety-like behavior and corresponding reduced levels of synaptic proteins and BDNF in the cerebellum, striatum and prefrontal cortex (Torres et al., 2018). Exposure to tobacco smoke during various periods of brain development also alters synaptic and neurotrophin levels in different brain regions that appear to last long after early life exposure to SHS.
3 Pharmacology and pathology of other SHS chemicals on the brain
As discussed above, smoke from cigarettes or e-cigarettes contains many chemicals at levels comparable to nicotine (see Table 1). SHS also contains aldehydes and polycyclic aromatic hydrocarbons (PAHs), as well as lower concentrations of other constituents (Arfaeinia et al., 2023), which have also been detected in e-cigarette vapors or even after the heating (versus combustion) of tobacco products (HTP) (Upadhyay et al., 2023; Vivarelli et al., 2022). HTPs are a newer category of tobacco products that generate nicotine and other chemicals, but at lower amounts than conventional cigarettes. Sufficient evidence supports that pre-natal PAH exposure negatively impacts cognitive development with specific regard to child intelligence (Humphreys and Valdes Hernandez, 2022). PAH during childhood and as an adult also was associated with an increase in biomarkers of neuroinflammation found in neurodegenerative diseases like AD and PD (Humphreys and Valdes Hernandez, 2022). There are ethnicity differences in SHS exposure that need to be considered as well. Smoking and SHS exposure accounts for the largest exposure to PAHs of non-Hispanic Whites vs other age-matched ethnic groups as well as older age groups (Gearhart-Serna et al., 2021). This large study indicates that there are vulnerable subpopulations with high PAH intake as a result of different smoking behaviors and potentially other exposures. Prenatal exposure to PAHs might alter mitochondrial copy number as a mechanism to explain its ability to impair neurodevelopment in children (Cao et al., 2020). Cord blood levels of benzo [a]pyrene DNA adducts (marker of PAH exposure) are inversely associated with the development of infants born to pregnant mothers who are exposed to PAHs from a Chinese coal burning power plant (Kalia et al., 2017). Benzo [a]pyrene DNA adducts levels are also negatively associated with BDNF levels in cord blood, suggesting that PAHs might cause neurodevelopmental delay through a DNA damage-mediated mechanism. The PAH concentration in cord blood after exposure to SHS would be expected to be much lower than after exposure of mothers to PAHs from a coal-burning plant.
Reactive aldehydes like acetaldehyde, acrolein and formaldehyde are formed during the combustion of tobacco products (Tulen et al., 2022). Acetaldehyde induces cytotoxicity by disrupting mitochondrial function to cause oxidative stress in neural cells (Yan et al., 2022), while acrolein causes DNA damage and oxidative stress in non-neural cells (Bellamri et al., 2022; Hikisz and Jacenik, 2023) and is elevated in the brain of patients with neurodegenerative diseases (Chang et al., 2022). Acrolein and formaldehyde induce Alzheimer-like disease pathology when administered to rodents (Liu et al., 2018; Chen et al., 2022) or primates (Zhai et al., 2018). Adult male rats treated for 3 months with acrolein show neurobehavioral alterations and cognitive impairments that are associated with electrophysiological disturbances (Khoramjouy et al., 2021). Endogenous acrolein plays a significant role in the pathogenesis of various neurodegenerative diseases, including Alzheimer’s disease (Chang et al., 2022), possibly through inducing oxidative stress reducing brain antioxidant levels and activating the MAPK pathway resulting in the hyperphosphorylation of tau and increasing amyloid-β levels, both biomarkers of neuropathology (Dhapola et al., 2023; Jallow et al., 2024). Thus, environmental exposure of humans to tobacco smoke and endogenous antioxidant levels could be important risk factors for the developing as well as adult brain (Chang et al., 2022) (Figure 3).
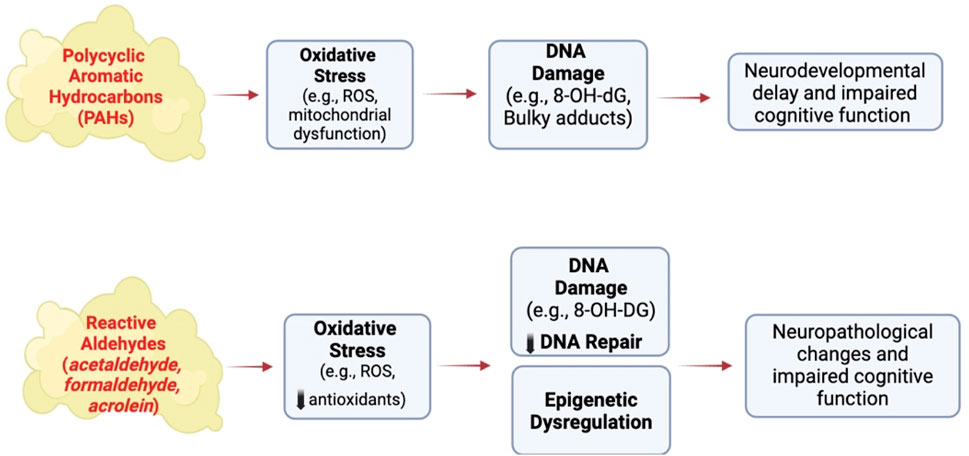
Figure 3. Effect of other SHS chemicals on brain structure and function following exposure to conventional cigarettes. ROS, reactive oxygen species, 8-OH-dG, 8-hydroxy-2′-deoxyguaonsine,. Images were created using BioRender software.
Formaldehyde (FA) is as environmental contaminant with toxic potential that also serves as an indispensable and thus normal physiological metabolite in the healthy brain, where it is hypothesized to regulate learning and memory via the N-methyl-D-aspartate receptor (Ai et al., 2019). FA is also a product of various metabolic pathways that participate in the one-carbon cycle, which provides carbon for the synthesis and modification of bio-compounds, such as DNA, RNA, and amino acids (Li et al., 2021). Endogenous FA plays a role in epigenetic regulation by regulating the methylation and demethylation of DNA, histones, and RNA (Li et al., 2021). At high levels, FA can pose a significant threat to genomic stability (Reingruber and Pontel, 2018), DNA repair (Nadalutti et al., 2021; Weng et al., 2018; Tang et al., 2022) and impede transcription, with negative physiological consequences (Mulderrig et al., 2021), through epigenetic alteration (Li et al., 2021), including neuronal and endothelial damage, (Chen et al., 2024). Notably, impaired memory is observed in mice with elevated endogenous FA, induced by knock-out of the gene coding for aldehyde dehydrogenase-2, a key mitochondrial enzyme for the effective metabolism of alcohol and acetaldehyde (Ai et al., 2019). Exposure to high levels of FA by inhalation (3.0 mg/m3) impairs cognitive function, including memory, in humans, causing neuronal damage and oxidative stress in the cerebellum of experimental animals, and inducing the misfolding of neuronal tau and related proteins in vitro (Rana et al., 2021). The balance between genotoxins and benign metabolites is presumed to depend on concentration, localization, pH and redox state, features that are or potentially altered during disease progression (Hopkinson and Schofield, 2018).
4 Pathway changes associated with cellular damage and inflammation in brain
A systematic review of the effects of active and passive smoking of conventional cigarettes, electronic cigarettes and tobacco heating products indicated that active and passive smoking induce oxidative stress and inflammatory responses in peripheral tissues (Kopa-Stojak and Pawliczak, 2024; Zieba et al., 2024), but the nervous system was not examined (Kopa-Stojak and Pawliczak, 2024). Oxidative stress-induced DNA damage and inflammation are also emerging as key triggers of dementia and related neurological disorders (Houldsworth, 2024; Giri et al., 2024; Neven et al., 2024; Firdous et al., 2024), as well as neurodevelopmental disorders (Qing et al., 2023; Lubrano et al., 2024; Xu et al., 2024). Since 90% of neurodegenerative diseases (e.g., MCI, dementia) are sporadic, this suggests that environmental factors like tobacco smoke might play an important, but undefined, role in their etiology (Ourry et al., 2024; Pandics et al., 2023). Early life exposure to SHS may also be an important risk factor for dementia (Chen, 2012; Zhou and Wang, 2021; Wan et al., 2024), as well as neurodevelopmental disorders (Hall et al., 2016; Julvez et al., 2021; Mukhopadhyay et al., 2010; Wade et al., 2023). Studies with animal models suggest that the effect of SHS on the brain may be due to increased oxidative stress and inflammation during brain development, leading to increased brain cell apoptosis in adulthood (Vivarelli et al., 2024; Raber et al., 2021; Raber et al., 2023; Lopes et al., 2023). Short-term exposure of 2-month-old mice (6h/day x 5 days/wk x four or 8 weeks) to a mixture of sidestream/mainstream cigarette smoke impairs brain insulin signaling and induces the accumulation of neuropathological proteins (Deochand et al., 2015; Deochand et al., 2016). Shorter durations of mainstream/sidestream smoke (1h/day x 1 month) induces lipid peroxides, DNA damage, and tau dysregulation (tau isomers, phosphotau) in the brain of neonatal mice (La Maestra et al., 2011), markers of neuropathology frequently observed in MCI and patients with dementia (Lovell and Markesbery, 2007; Simpson et al., 2016; Wirz et al., 2014). Longer exposures of 2-month-old rats or 3-month-old APP/PS1 transgenic mice to sidestream cigarette smoke (1h/day x 5 days/wk x two or 4 months) induces tau and amyloid pathology like that reported in MCI and patients with dementia (Ho et al., 2012; Moreno-Gonzalez et al., 2013). These studies strongly suggest that SHS increases the risk of developing MCI and dementia by perturbing brain metabolism (i.e., insulin signaling, oxidative stress) and the accumulation of neuropathological proteins (i.e., tau, amyloid). SHS induces a distinct brain metabolic profile characterized by oxidative stress (Raber et al., 2021; Raber et al., 2023; Neal et al., 2016) and inflammation (Lopes et al., 2023; D et al., 2019; Chan et al., 2020). The cortex and hippocampus in the brains from the offspring of female C57BL/6 mice exposed to air or SHS (50 μg/m3; 5h/day, 5 days/week for 5 weeks and 2 days) were examined by untargeted metabolomics. Insulin signaling, which regulates an abundance of metabolic proteins, is altered in the hippocampus of the offspring exposed throughout development to SHS. An increase in glutathione-S-transferase is also detected, and a trend towards increased glutathione reductase activity, increases GSSG, and a decreased GSH/GSSG ratio is observed. In a systematic review of the literature, it was also reported that cigarette smoking (active and passive) induces oxidative stress and an inflammatory response in peripheral (i.e., non-neurological) tissues (Kopa-Stojak and Pawliczak, 2024; Kanithi et al., 2022; Prasad and Bondy, 2022; Mukharjee et al., 2020). Thus, exposure to SHS (passive smoking) on the brain and non-neurological tissues induces both oxidative stress and inflammation.
A multi-center study of six European countries that examined the relationship between early life environmental exposures and child cognitive function found that ETS exposures adversely and cross sectionally associate with cognitive function (Julvez et al., 2021). In human studies, maternal exposure to SHS is also closely linked to small brain size and changes in brain structure that associated with a higher risk of cognitive impairments (Colyer-Patel et al., 2023; Greenwood et al., 2024; Chan et al., 2020) and psychotic experiences (Wang D. et al., 2024). SHS also induces oxidative stress and inflammation in the developing brain (D et al., 2019; Chan et al., 2020; Lobo Torres et al., 2012; Mohamed et al., 2022; Church et al., 2020). Acute exposure to SHS on postnatal day 18 increased GST activity and malondialdehyde (MDA levels in the hippocampus, GPx and SOD activity in the prefrontal cortex and GST activity and MDA levels in the striatum and cerebellum of postnatal mice (Lobo Torres et al., 2012). Three hours later, SOD activity and MDA levels increased in the hippocampus and the activity of all enzymes decreased in the prefrontal cortex. This study shows that SHS induces oxidative stress by perturbing antioxidant enzymes in distinct brain regions during early brain development like that reported in older animals. Thus, oxidative stress appears to be an early event following exposure to ETS or SHS from tobacco products. Pregnant mice were exposed to e-cig vapor (2.4% nicotine) from GD five until postnatal day 7 (PD7) and the brain of mice at PD7 and PD 90 examined for reactive oxygen species (ROS) and pro-inflammatory cytokines (Archie et al., 2023). E-cig vapor reduced antioxidant marker expression and increased the expression of pro-inflammatory and cytokine markers in the PD7 brain, but not the PD 90 brain. Pregnant mice were also exposed daily to e-cigarette chemicals (propylene glycol, vegetable glycol) and 16 mg/mL of nicotine for 3 h/d, 7 days a week from gestational day (GD) 0.5 until GD 17.5 (Church et al., 2020). Male and female offspring of e-cigarette exposed mice had lower scores on the novel object recognition task and reduced inflammatory markers in the diencephalon (IL-4, IFNγ) and hippocampus (IFNγ; females only). This experimental study demonstrates that e-cigarette vapors can also persistently alter the neuroimmunology and behavior following maternal exposure. Thus, oxidative stress and inflammatory markers are also increased in the brain of mice after in utero exposure to SHS from both conventional cigarettes and e-cigarettes (Figure 4).
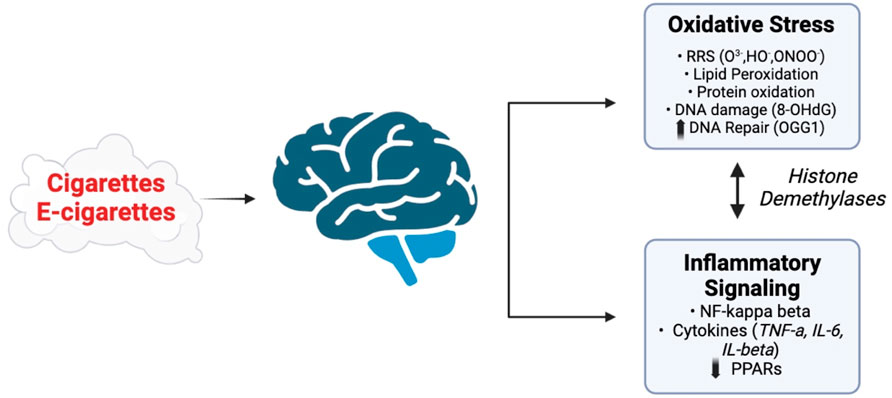
Figure 4. Potential pathways affected by exposure of the CNS to vapors or SHS generated by e-cigarettes and conventional cigarettes. Adapted from Vivarelli et al. (2024), Arfaeinia et al. (2023), Addissouky et al. (2024), and Auschwitz et al. (2023). Images were created using BioRender software.
5 MCI, neurodegeneration, and other neurological disorders
As described earlier, there is growing evidence that non-smokers exposed to SHS are at an increased risk of developing MCI (Chen, 2012; Llewellyn et al., 2009; Yolton et al., 2005; Murphy et al., 2020; Akhtar et al., 2013; Cataldo et al., 2010; Chang et al., 2012) and dementia (Chen, 2012; Cataldo et al., 2010; Chang et al., 2012), as well as neurodevelopmental disorders (Ou et al., 2024; Chan et al., 2020; Pagani, 2014). Exposure to SHS increases the risk for dementia among individuals who never smoked (Zhou and Wang, 2021; Wan et al., 2024; He et al., 2020) and it is 2–6 times more toxic and tumorigenic to humans than mainstream smoke (Schick and Glantz, 2005; Akhtar et al., 2013). Cholinergic dysfunction of the nucleus basalis of Meynert (NBM) is hypothesized to be an important factor for the increased risk of AD (Slotkin et al., 2015; Slotkin et al., 2019; Toro et al., 2008). Chronic exposure to nicotine through smoking may lead to atrophy of cholinergic input areas of the basal forebrain. Chronic exposure to nicotine through smoking disrupts the functional connectivity between the NBM and precuneus in MCI patients (Qiu et al., 2022). The ability of cigarette smoke to disrupt the connectivity in both non-smokers and those with MCI suggests that exposure to cigarette smoke disrupts cognition. Yet, 6 months of transdermal nicotine administration (16 mg/day) to MCI patients improves primary and secondary cognitive measures of attention, memory, and mental processing (Heffernan and O'Neill, 2013). These studies demonstrate that exposure to nicotine through cigarette smoke can either disrupt cholinergic function or be protective. Early life exposure to nicotine and other SHS constituents might be the key to understanding how the toxins in cigarette smoke induce their long-term effects on learning and memory, executive function, and the reward circuitry (Hall et al., 2016; Cauley et al., 2018; Ponzoni et al., 2020).
Base excision repair (BER) is the primary cellular pathway for repairing oxidative DNA damage (e.g., 8-oxo-deoxyguanosine, 8-oxodG) (Oka et al., 2021) that is reportedly impaired in both MCI individuals and those with AD (Chang et al., 2022; Cherbuin et al., 2024). Furthermore, the brain of dementia subjects also exhibits activation of the DNA damage response (DDR) pathway, inflammatory changes and cellular senescence (Schwab et al., 2021). Exposing mice for 4 months to active (La Maestra et al., 2011) or passive (Moreno-Gonzalez et al., 2013) cigarette smoke induces oxidative stress, DNA damage and neuropathology in mice. Chronic exposure of mice to SHS (90% side stream, 10% mainstream smoke x 2.8 h/day x 7 days/wk x 10.4 mos) induces dark, shrunken cells, hippocampal thinning, and the presence of activated astrocytes and prominent 8-oxoG staining in the prefrontal cortex (PFC) and hippocampus (HIPP) (Raber et al., 2021; Lopes et al., 2023). 8-oxoguanine DNA glycosylase (Ogg1) staining is also reduced in the PFC and CA3 hippocampal neurons of SHS chronically exposed mice. Apurinic/apyrimdinic endonuclease (Ape1) staining is more prominent in the PFC and the HIPP in SHS chronically exposed mice. These studies demonstrate that oxidative DNA damage (8-oxoG) is elevated and oxidative DNA repair (Ogg1 and Ape1) is altered in the brain of SHS exposed mice, as well as activation of reactive astrocytes. The percentage of 8-OHdG-labeled cells in the CA1 region of the hippocampus is associated with performance in the novel object recognition test, consistent with urine and serum levels of 8-OHdG serving as a biomarker of cognitive performance in humans. Therefore, SHS induces both oxidative DNA damage and repair, as well as inflammation as possible underlying mechanism(s) of the behavioral and cognitive function and metabolic changes that were observed in chronically exposed mice (Raber et al., 2021). These findings suggest that human exposure to cigarette smoke induces oxidative stress, genomic stress (i.e., DNA damage and repair) and enhances neuroinflammation like that in age-related neurodegenerative diseases (Simpson et al., 2016; Shadfar et al., 2023) (Figure 5).
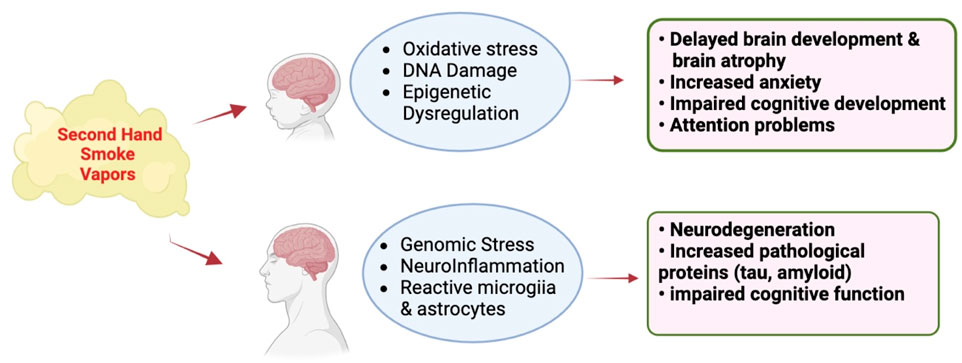
Figure 5. Effect of vapors or SHS generated by e-cigarettes and conventional cigarettes (respectively) on the developing and mature CNS. Adapted from Merecz-Sadowska et al. (2020), Prasedya et al. (2020), Chan et al. (2020), and Garza et al. (2023). Images were generated using BioRender software.
With regard to neurodevelopmental disorders, a recent META analysis involving 54 studies revealed associations between SHS exposure and the risks of developing attention deficit hyperactivity disorder (ADHD) and learning disabilities (LD) (Ou et al., 2024). There is also an association between cotinine exposure and ADHD. Consistent with the increased ADHD risk, prenatal SHS and postnatal maternal distress alter the efficiency of the cingulo-opercular (CO) network, which is involved in task control and executive function (Freedman et al., 2020). In addition to SHS, third hand smoke, consisting of residual tobacco smoke pollutants that remain on surfaces and in dust after tobacco has been smoked, which are re-emitted into the gas phase or react with oxidants and other compounds in the environment to yield secondary pollutants and include nicotine, three-ethenylpyridine (3-EP), phenol, cresols, naphthalene, formaldehyde, and tobacco- specific nitrosamines that have detrimental effects on the developing brain (Matt et al., 2011). Third hand smoke can be inhaled through inhalation, ingestion, or dermal uptake from the air, dust, and from surfaces. Consistent with the human data, third hand smoke for 4 weeks in mice increased inflammatory cytokines in plasma and increased epinephrine and aspartate aminotransferase, a biomarker of liver damage (Adhami et al., 2017). These detrimental effects are more pronounced when the mice were chronically exposed to third hand smoke for 8, 16, and 24 weeks. With longer third hand smoke exposure, mice become hyperglycemic and hyperinsulinimic, indicating an important role for impaired insulin sensitivity after third hand smoke exposure. Pre- and post-natally, there can also be a combination of SHS and third hand smoke.
6 Conclusion
The above studies of SHS exposure in animals and humans demonstrate that the brain is a key target of nicotine and other constituents following exposure to tobacco products. Early life exposure to SHS disrupts brain development to increase the risk for neurodevelopmental disorders (ADHD, learning disabilities). On the other hand, exposure of the mature brain to SHS is considered a risk factor mild cognitive impairment and dementia. A common target for SHS in the developing and adult brain is oxidative stress, inflammation responses and genomic stress that might be responsible for triggering both neurodevelopmental disorders as well as dementia (Figure 6). Given the world-wide exposure of pregnant women, children and adults to SHS, additional research will be required to pinpoint the mechanism by which SHS is a risk factor for both neurodevelopmental and neurodegenerative disorders with the goal of protecting the most vulnerable to these environmental exposures.
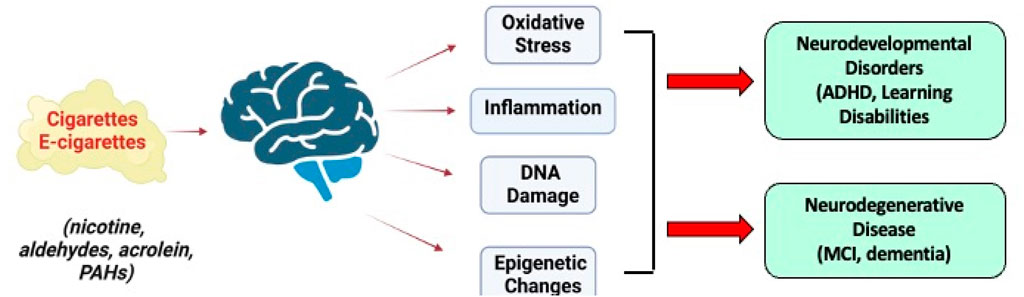
Figure 6. Long-term effects of SHS constituents from conventional and e-cigarettes on the brain and their role in neurodevelopmental disorders and neurodegenerative disease. Images were created with BioRender.
Author contributions
JR: Conceptualization, Funding acquisition, Writing–original draft, Writing–review and editing. GK: Conceptualization, Funding acquisition, Writing–original draft, Writing–review and editing.
Funding
The author(s) declare that financial support was received for the research, authorship, and/or publication of this article. This research was partially funded by NIEHS R21 ES027943, NIA R21 AG079158, a pilot award from the Western University of Health Sciences, and the development account of JR at OHSU.
Conflict of interest
The authors declare that the research was conducted in the absence of any commercial or financial relationships that could be construed as a potential conflict of interest.
The author(s) declared that they were an editorial board member of Frontiers, at the time of submission. This had no impact on the peer review process and the final decision.
The reviewer KL declared a shared affiliation with the author GK to the handling editor at the time of review.
Publisher’s note
All claims expressed in this article are solely those of the authors and do not necessarily represent those of their affiliated organizations, or those of the publisher, the editors and the reviewers. Any product that may be evaluated in this article, or claim that may be made by its manufacturer, is not guaranteed or endorsed by the publisher.
References
Addissouky, T. A., El Sayed, I. E. T., Ali, M. M. A., Wang, Y., El Baz, A., Elarabany, N., et al. (2024). Oxidative stress and inflammation: elucidating mechanisms of smoking-attributable pathology for therapeutic targeting. Bull. Natl. Res. Centre 48, 16. doi:10.1186/s42269-024-01174-6
Adhami, N., Chen, Y., and Martins-Green, M. (2017). Biomarkers of disease can be detected in mice as early as 4 weeks after initiation of exposure to third-hand smoke levels equivalent to those found in homes of smokers. Clin. Sci. (Lond) 131, 2409–2426. doi:10.1042/CS20171053
Ai, L., Tan, T., Tang, Y., Yang, J., Cui, D., Wang, R., et al. (2019). Endogenous formaldehyde is a memory-related molecule in mice and humans. Commun. Biol. 2, 446. doi:10.1038/s42003-019-0694-x
Akhtar, W. Z., Andresen, E. M., Cannell, M. B., and Xu, X. (2013). Association of blood cotinine level with cognitive and physical performance in non-smoking older adults. Environ. Res. 121, 64–70. doi:10.1016/j.envres.2012.10.013
Archie, S. R., Sifat, A. E., Mara, D., Ahn, Y., Akter, K. A., Zhang, Y., et al. (2023). Impact of in-utero electronic cigarette exposure on neonatal neuroinflammation, oxidative stress and mitochondrial function. Front. Pharmacol. 14, 1227145. doi:10.3389/fphar.2023.1227145
Arfaeinia, H., Ghaemi, M., Jahantigh, A., Soleimani, F., and Hashemi, H. (2023). Secondhand and thirdhand smoke: a review on chemical contents, exposure routes, and protective strategies. Environ. Sci. Pollut. Res. Int. 30, 78017–78029. doi:10.1007/s11356-023-28128-1
Armendariz-Castillo, I., Guerrero, S., Vera-Guapi, A., Cevallos-Vilatuna, T., Garcia-Cardenas, J. M., Guevara-Ramirez, P., et al. (2019). Genotoxic and carcinogenic potential of compounds associated with electronic cigarettes: a systematic review. Biomed. Res. Int. 2019, 1386710. doi:10.1155/2019/1386710
Auschwitz, E., Almeda, J., and Andl, C. D. (2023). Mechanisms of E-cigarette vape-induced epithelial cell damage. Cells 12, 2552. doi:10.3390/cells12212552
Bellamri, M., Walmsley, S. J., Brown, C., Brandt, K., Konorev, D., Day, A., et al. (2022). DNA damage and oxidative stress of tobacco smoke condensate in human bladder epithelial cells. Chem. Res. Toxicol. 35, 1863–1880. doi:10.1021/acs.chemrestox.2c00153
Boiangiu, R. S., Brinza, I., Honceriu, I., Mihasan, M., and Hritcu, L. (2023). Insights into pharmacological activities of nicotine and 6-Hydroxy-L-nicotine, a bacterial nicotine derivative: a systematic review. Biomolecules 14, 23. doi:10.3390/biom14010023
Bruijnzeel, A. W. (2017). Neuropeptide systems and new treatments for nicotine addiction. Psychopharmacol. Berl. 234, 1419–1437. doi:10.1007/s00213-016-4513-5
Buck, J. M., O'Neill, H. C., and Stitzel, J. A. (2020). Developmental nicotine exposure engenders intergenerational downregulation and aberrant posttranslational modification of cardinal epigenetic factors in the frontal cortices, striata, and hippocampi of adolescent mice. Epigenetics Chromatin 13, 13. doi:10.1186/s13072-020-00332-0
Cao, X., Li, J., Cheng, L., Deng, Y., Li, Y., Yan, Z., et al. (2020). The associations between prenatal exposure to polycyclic aromatic hydrocarbon metabolites, umbilical cord blood mitochondrial DNA copy number, and children's neurobehavioral development. Environ. Pollut. 265, 114594. doi:10.1016/j.envpol.2020.114594
Cataldo, J. K., Prochaska, J. J., and Glantz, S. A. (2010). Cigarette smoking is a risk factor for Alzheimer's Disease: an analysis controlling for tobacco industry affiliation. J. Alzheimers Dis. 19, 465–480. doi:10.3233/JAD-2010-1240
Cauley, M., Hall, B. J., Abreu-Villaca, Y., Junaid, S., White, H., Kiany, A., et al. (2018). Critical developmental periods for effects of low-level tobacco smoke exposure on behavioral performance. Neurotoxicology 68, 81–87. doi:10.1016/j.neuro.2018.07.012
Centers for Disease Control and Prevention (2010). How tobacco smoke causes disease: the biology and behavioral basis for smoking-attributable disease: a report of the surgeon general. Rockville, MD Washington, DC: U.S. Dept. Of health and human services, public health Service for sale by the supt. of Docs., U.S. G.P.O.
Centers for Disease Control and Prevention (2024). E-Cigarettes, or vaping, products visual dictionary, Centers for Disease Control and Prevention: Atlanta, GA.
Chan, Y. L., Oliver, B. G., and Chen, H. (2020). What lessons have we learnt about the impact of maternal cigarette smoking from animal models? Clin. Exp. Pharmacol. Physiol. 47, 337–344. doi:10.1111/1440-1681.13182
Chang, C. C., Zhao, Y., Lee, C. W., and Ganguli, M. (2012). Smoking, death, and Alzheimer disease: a case of competing risks. Alzheimer Dis. Assoc. Disord. 26, 300–306. doi:10.1097/WAD.0b013e3182420b6e
Chang, X., Wang, Y., Zheng, B., Chen, Y., Xie, J., Song, Y., et al. (2022). The role of acrolein in neurodegenerative diseases and its protective strategy. Foods 11, 3203. doi:10.3390/foods11203203
Chen, C. H., Cruz, L. A., and Mochly-Rosen, D. (2015). Pharmacological recruitment of aldehyde dehydrogenase 3A1 (ALDH3A1) to assist ALDH2 in acetaldehyde and ethanol metabolism in vivo. Proc. Natl. Acad. Sci. U. S. A. 112, 3074–3079. doi:10.1073/pnas.1414657112
Chen, C., Lu, J., Peng, W., Mak, M. S., Yang, Y., Zhu, Z., et al. (2022). Acrolein, an endogenous aldehyde induces Alzheimer's disease-like pathologies in mice: a new sporadic AD animal model. Pharmacol. Res. 175, 106003. doi:10.1016/j.phrs.2021.106003
Chen, J., Chen, W., Zhang, J., Zhao, H., Cui, J., Wu, J., et al. (2024). Dual effects of endogenous formaldehyde on the organism and drugs for its removal. J. Appl. Toxicol. 44, 798–817. doi:10.1002/jat.4546
Chen, R. (2012). Association of environmental tobacco smoke with dementia and Alzheimer's disease among never smokers. Alzheimers Dement. 8, 590–595. doi:10.1016/j.jalz.2011.09.231
Cherbuin, N., Patel, H., Walsh, E. I., Ambikairajah, A., Burns, R., Brustle, A., et al. (2024). Cognitive function is associated with the genetically determined efficiency of DNA repair mechanisms. Genes (Basel) 15, 153. doi:10.3390/genes15020153
Church, J. S., Chace-Donahue, F., Blum, J. L., Ratner, J. R., Zelikoff, J. T., and Schwartzer, J. J. (2020). Neuroinflammatory and behavioral outcomes measured in adult offspring of mice exposed prenatally to E-cigarette aerosols. Environ. Health Perspect. 128, 47006. doi:10.1289/EHP6067
Colyer-Patel, K., Kuhns, L., Weidema, A., Lesscher, H., and Cousijn, J. (2023). Age-dependent effects of tobacco smoke and nicotine on cognition and the brain: a systematic review of the human and animal literature comparing adolescents and adults. Neurosci. Biobehav Rev. 146, 105038. doi:10.1016/j.neubiorev.2023.105038
Czogala, J., Goniewicz, M. L., Fidelus, B., Zielinska-Danch, W., Travers, M. J., and Sobczak, A. (2014). Secondhand exposure to vapors from electronic cigarettes. Nicotine Tob. Res. 16, 655–662. doi:10.1093/ntr/ntt203
D, A. Q., Witt Hansen, A., Bellaver, B., Bobermin, L. D., R, R. P., Bandiera, S., et al. (2019). Combined exposure to alcohol and tobacco smoke changes oxidative, inflammatory, and neurotrophic parameters in different areas of the brains of rats. ACS Chem. Neurosci. 10, 1336–1346. doi:10.1021/acschemneuro.8b00412
Deochand, C., Tong, M., Agarwal, A. R., Cadenas, E., and de la Monte, S. M. (2015). Tobacco smoke exposure impairs brain insulin/IGF signaling: potential Co-factor role in neurodegeneration. J. Alzheimers Dis. 50, 373–386. doi:10.3233/JAD-150664
Deochand, C., Tong, M., Agarwal, A. R., Cadenas, E., and de la Monte, S. M. (2016). Tobacco smoke exposure impairs brain insulin/IGF signaling: potential Co-factor role in neurodegeneration. J. Alzheimers Dis. 50, 373–386. doi:10.3233/JAD-150664
Dhapola, R., Kumari, S., Sharma, P., and HariKrishnaReddy, D. (2023). Insight into the emerging and common experimental in-vivo models of Alzheimer's disease. Lab. Anim. Res. 39, 33. doi:10.1186/s42826-023-00184-1
Dwyer, J. B., McQuown, S. C., and Leslie, F. M. (2009). The dynamic effects of nicotine on the developing brain. Pharmacol. Ther. 122, 125–139. doi:10.1016/j.pharmthera.2009.02.003
Ebersole, J., Samburova, V., Son, Y., Cappelli, D., Demopoulos, C., Capurro, A., et al. (2020). Harmful chemicals emitted from electronic cigarettes and potential deleterious effects in the oral cavity. Tob. Induc. Dis. 18, 41. doi:10.18332/tid/116988
England, L. J., Aagaard, K., Bloch, M., Conway, K., Cosgrove, K., Grana, R., et al. (2017). Developmental toxicity of nicotine: a transdisciplinary synthesis and implications for emerging tobacco products. Neurosci. Biobehav Rev. 72, 176–189. doi:10.1016/j.neubiorev.2016.11.013
Farsalinos, K. E., and Gillman, G. (2017). Carbonyl emissions in E-cigarette aerosol: a systematic review and methodological considerations. Front. Physiol. 8, 1119. doi:10.3389/fphys.2017.01119
Ferraguti, G., Terracina, S., Micangeli, G., Lucarelli, M., Tarani, L., Ceccanti, M., et al. (2023). NGF and BDNF in pediatrics syndromes. Neurosci. Biobehav Rev. 145, 105015. doi:10.1016/j.neubiorev.2022.105015
Firdous, S. M., Khan, S. A., and Maity, A. (2024). Oxidative stress-mediated neuroinflammation in Alzheimer's disease. Naunyn Schmiedeb. Arch. Pharmacol. 397, 8189–8209. doi:10.1007/s00210-024-03188-3
Flor, L. S., Anderson, J. A., Ahmad, N., Aravkin, A., Carr, S., Dai, X., et al. (2024). Health effects associated with exposure to secondhand smoke: a Burden of Proof study. Nat. Med. 30, 149–167. doi:10.1038/s41591-023-02743-4
Freedman, L., Zivan, M., Farah, R., and Horowitz-Kraus, T. (2020). Greater functional connectivity within the cingulo-opercular and ventral attention networks is related to better fluent reading: a resting-state functional connectivity study. Neuroimage Clin. 26, 102214. doi:10.1016/j.nicl.2020.102214
Fu, P. P., Xia, Q., Sun, X., and Yu, H. (2012). Phototoxicity and environmental transformation of polycyclic aromatic hydrocarbons (PAHs)-light-induced reactive oxygen species, lipid peroxidation, and DNA damage. J. Environ. Sci. Health C Environ. Carcinog. Ecotoxicol. Rev. 30, 1–41. doi:10.1080/10590501.2012.653887
Garza, A. P., Morton, L., Pallinger, E., Buzas, E. I., Schreiber, S., Schott, B. H., et al. (2023). Initial and ongoing tobacco smoking elicits vascular damage and distinct inflammatory response linked to neurodegeneration. Brain Behav. Immun. Health 28, 100597. doi:10.1016/j.bbih.2023.100597
Gearhart-Serna, L. M., Tacam, M., Slotkin, T. A., and Devi, G. R. (2021). Analysis of polycyclic aromatic hydrocarbon intake in the US adult population from NHANES 2005-2014 identifies vulnerable subpopulations, suggests interaction between tobacco smoke exposure and sociodemographic factors. Environ. Res. 201, 111614. doi:10.1016/j.envres.2021.111614
Giri, P. M., Banerjee, A., Ghosal, A., and Layek, B. (2024). Neuroinflammation in neurodegenerative disorders: current knowledge and therapeutic implications. Int. J. Mol. Sci. 25, 3995. doi:10.3390/ijms25073995
Goniewicz, M. L., Smith, D. M., Edwards, K. C., Blount, B. C., Caldwell, K. L., Feng, J., et al. (2018). Comparison of nicotine and toxicant exposure in users of electronic cigarettes and combustible cigarettes. JAMA Netw. Open 1, e185937. doi:10.1001/jamanetworkopen.2018.5937
Gould, T. J. (2023). Epigenetic and long-term effects of nicotine on biology, behavior, and health. Pharmacol. Res. 192, 106741. doi:10.1016/j.phrs.2023.106741
Grana, R., Benowitz, N., and Glantz, S. A. (2014). E-cigarettes: a scientific review. Circulation 129, 1972–1986. doi:10.1161/CIRCULATIONAHA.114.007667
Greenwood, P. B., DeSerisy, M., Koe, E., Rodriguez, E., Salas, L., Perera, F. P., et al. (2024). Combined and sequential exposure to prenatal second hand smoke and postnatal maternal distress is associated with cingulo-opercular global efficiency and attention problems in school-age children. Neurotoxicol Teratol. 102, 107338. doi:10.1016/j.ntt.2024.107338
Hall, B. J., Cauley, M., Burke, D. A., Kiany, A., Slotkin, T. A., and Levin, E. D. (2016). Cognitive and behavioral impairments evoked by low-level exposure to tobacco smoke components: comparison with nicotine alone. Toxicol. Sci. 151, 236–244. doi:10.1093/toxsci/kfw042
Hamann, S. L., Kungskulniti, N., Charoenca, N., Kasemsup, V., Ruangkanchanasetr, S., and Jongkhajornpong, P. (2023). Electronic cigarette harms: aggregate evidence shows damage to biological systems. Int. J. Environ. Res. Public Health 20, 6808. doi:10.3390/ijerph20196808
He, F., Li, T., Lin, J., Li, F., Zhai, Y., Zhang, T., et al. (2020). Passive smoking exposure in living environments reduces cognitive function: a prospective cohort study in older adults. Int. J. Environ. Res. Public Health 17, 1402. doi:10.3390/ijerph17041402
Heffernan, T. M., and O'Neill, T. S. (2013). Everyday prospective memory and executive function deficits associated with exposure to second-hand smoke. J. Addict. 2013, 160486. doi:10.1155/2013/160486
Herrmann, M., King, K., and Weitzman, M. (2008). Prenatal tobacco smoke and postnatal secondhand smoke exposure and child neurodevelopment. Curr. Opin. Pediatr. 20, 184–190. doi:10.1097/MOP.0b013e3282f56165
Heywood, J., Abele, G., Langenbach, B., Litvin, S., Smallets, S., and Paustenbach, D. (2024). Composition of e-cigarette aerosols: a review and risk assessment of selected compounds. J. Appl. Toxicol. 45, 364–386. doi:10.1002/jat.4683
Hikisz, P., and Jacenik, D. (2023). The tobacco smoke component, acrolein, as a major culprit in lung diseases and respiratory cancers: molecular mechanisms of acrolein cytotoxic activity. Cells 12, 879. doi:10.3390/cells12060879
Ho, Y. S., Yang, X., Yeung, S. C., Chiu, K., Lau, C. F., Tsang, A. W., et al. (2012). Cigarette smoking accelerated brain aging and induced pre-Alzheimer-like neuropathology in rats. PLoS One 7, e36752. doi:10.1371/journal.pone.0036752
Hoang, T. T., Lee, Y., McCartney, D. L., Kersten, E. T. G., Page, C. M., Hulls, P. M., et al. (2024). Comprehensive evaluation of smoking exposures and their interactions on DNA methylation. EBioMedicine 100, 104956. doi:10.1016/j.ebiom.2023.104956
Hopkinson, R. J., and Schofield, C. J. (2018). Deciphering functions of intracellular formaldehyde: linking cancer and aldehyde metabolism. Biochemistry 57, 904–906. doi:10.1021/acs.biochem.7b01304
Houldsworth, A. (2024). Role of oxidative stress in neurodegenerative disorders: a review of reactive oxygen species and prevention by antioxidants. Brain Commun. 6, fcad356. doi:10.1093/braincomms/fcad356
Humphreys, J., and Valdes Hernandez, M. D. C. (2022). Impact of polycyclic aromatic hydrocarbon exposure on cognitive function and neurodegeneration in humans: a systematic review and meta-analysis. Front. Neurol. 13, 1052333. doi:10.3389/fneur.2022.1052333
Ikemoto, S., and Bonci, A. (2014). Neurocircuitry of drug reward. Neuropharmacol 76, 329–341. doi:10.1016/j.neuropharm.2013.04.031
Izquierdo-Condoy, J. S., Naranjo-Lara, P., Morales-Lapo, E., Hidalgo, M. R., Tello-De-la-Torre, A., Vasconez-Gonzales, E., et al. (2024). Direct health implications of e-cigarette use: a systematic scoping review with evidence assessment. Front. Public Health 12, 1427752. doi:10.3389/fpubh.2024.1427752
Jallow, A. W., Nguyen, D. P. Q., Sanotra, M. R., Hsu, C. H., Lin, Y. F., and Lin, Y. F. (2024). A comprehensive bibliometric analysis of global research on the role of acrolein in Alzheimer's disease pathogenesis: involvement of amyloid-beta. Front. Aging Neurosci. 16, 1378260. doi:10.3389/fnagi.2024.1378260
Joshi, A. U., Van Wassenhove, L. D., Logas, K. R., Minhas, P. S., Andreasson, K. I., Weinberg, K. I., et al. (2019). Aldehyde dehydrogenase 2 activity and aldehydic load contribute to neuroinflammation and Alzheimer's disease related pathology. Acta Neuropathol. Commun. 7, 190. doi:10.1186/s40478-019-0839-7
Julvez, J., Lopez-Vicente, M., Warembourg, C., Maitre, L., Philippat, C., Gutzkow, K. B., et al. (2021). Early life multiple exposures and child cognitive function: a multi-centric birth cohort study in six European countries. Environ. Pollut. 284, 117404. doi:10.1016/j.envpol.2021.117404
Kalia, V., Perera, F., and Tang, D. (2017). Environmental pollutants and neurodevelopment: review of benefits from closure of a coal-burning power plant in tongliang, China. Glob. Pediatr. Health 4, 2333794X17721609. doi:10.1177/2333794X17721609
Kanithi, M., Junapudi, S., Shah, S. I., Matta Reddy, A., Ullah, G., and Chidipi, B. (2022). Alterations of mitochondrial network by cigarette smoking and E-cigarette vaping. Cells 11, 1688. doi:10.3390/cells11101688
Kapchinsky, S., Vuda, M., Miguez, K., Elkrief, D., de Souza, A. R., Baglole, C. J., et al. (2018). Smoke-induced neuromuscular junction degeneration precedes the fibre type shift and atrophy in chronic obstructive pulmonary disease. J. Physiol. 596, 2865–2881. doi:10.1113/JP275558
Khoramjouy, M., Naderi, N., Kobarfard, F., Heidarli, E., and Faizi, M. (2021). An intensified acrolein exposure can affect memory and cognition in rat. Neurotox. Res. 39, 277–291. doi:10.1007/s12640-020-00278-x
Kopa-Stojak, P. N., and Pawliczak, R. (2024). Comparison of the effects of active and passive smoking of tobacco cigarettes, electronic nicotine delivery systems and tobacco heating products on the expression and secretion of oxidative stress and inflammatory response markers. A systematic review. Inhal. Toxicol. 36, 75–89. doi:10.1080/08958378.2024.2319315
Kou, Y., Zhao, H., Cui, D., Han, H., and Tong, Z. (2022). Formaldehyde toxicity in age-related neurological dementia. Ageing Res. Rev. 73, 101512. doi:10.1016/j.arr.2021.101512
La Maestra, S., Kisby, G. E., Micale, R. T., Johnson, J., Kow, Y. W., Bao, G., et al. (2011). Cigarette smoke induces DNA damage and alters base-excision repair and tau levels in the brain of neonatal mice. Toxicol. Sci. 123, 471–479. doi:10.1093/toxsci/kfr187
Li, Y., and Hecht, S. S. (2022). Carcinogenic components of tobacco and tobacco smoke: a 2022 update. Food Chem. Toxicol. 165, 113179. doi:10.1016/j.fct.2022.113179
Li, T., Wei, Y., Qu, M., Mou, L., Miao, J., Xi, M., et al. (2021). Formaldehyde and de/methylation in age-related cognitive impairment. Genes (Basel) 12, 913. doi:10.3390/genes12060913
Lin, L. Z., Xu, S. L., Wu, Q. Z., Zhou, Y., Ma, H. M., Chen, D. H., et al. (2021). Association of prenatal, early postnatal, or current exposure to secondhand smoke with attention-deficit/hyperactivity disorder symptoms in children. JAMA Netw. Open 4, e2110931. doi:10.1001/jamanetworkopen.2021.10931
Liu, X., Zhang, Y., Wu, R., Ye, M., Zhao, Y., Kang, J., et al. (2018). Acute formaldehyde exposure induced early Alzheimer-like changes in mouse brain. Toxicol. Mech. Methods 28, 95–104. doi:10.1080/15376516.2017.1368053
Llewellyn, D. J., Lang, I. A., Langa, K. M., Naughton, F., and Matthews, F. E. (2009). Exposure to secondhand smoke and cognitive impairment in non-smokers: national cross sectional study with cotinine measurement. BMJ 338, b462. doi:10.1136/bmj.b462
Lobo Torres, L. H., Moreira, W. L., Tamborelli Garcia, R. C., Annoni, R., Nicoletti Carvalho, A. L., Teixeira, S. A., et al. (2012). Environmental tobacco smoke induces oxidative stress in distinct brain regions of infant mice. J. Toxicol. Environ. Health A 75, 971–980. doi:10.1080/15287394.2012.695985
Lopes, L. A., Davenport, C., Ramos Torres, E., Chlebowski, A., Mikami, A., Raber, J., et al. (2023). Neuropathological examination of mice chronically exposed to secondhand smoke. Mil. Med. 188, 575–583. doi:10.1093/milmed/usad247
Lopez-Ojeda, W., and Hurley, R. A. (2024). Vaping and the brain: effects of electronic cigarettes and E-liquid substances. J. Neuropsychiatry Clin. Neurosci. 36, A41–A45. doi:10.1176/appi.neuropsych.20230184
Lovell, M. A., and Markesbery, W. R. (2007). Oxidative DNA damage in mild cognitive impairment and late-stage Alzheimer's disease. Nucleic Acids Res. 35, 7497–7504. doi:10.1093/nar/gkm821
Lubrano, C., Parisi, F., and Cetin, I. (2024). Impact of maternal environment and inflammation on fetal neurodevelopment. Antioxidants (Basel) 13, 453. doi:10.3390/antiox13040453
Ma, H., Lou, K., Shu, Q., Song, X., and Xu, H. (2024). Aldehyde dehydrogenase 2 deficiency reinforces formaldehyde-potentiated pro-inflammatory responses and glycolysis in macrophages. J. Biochem. Mol. Toxicol. 38, e23518. doi:10.1002/jbt.23518
Machaalani, R., Thawley, M., Huang, J., and Chen, H. (2019). Effects of prenatal cigarette smoke exposure on BDNF, PACAP, microglia and gliosis expression in the young male mouse brainstem. Neurotoxicology 74, 40–46. doi:10.1016/j.neuro.2019.05.009
Markunas, C. A., Xu, Z., Harlid, S., Wade, P. A., Lie, R. T., Taylor, J. A., et al. (2014). Identification of DNA methylation changes in newborns related to maternal smoking during pregnancy. Environ. Health Perspect. 122, 1147–1153. doi:10.1289/ehp.1307892
Matt, G. E., Quintana, P. J., Destaillats, H., Gundel, L. A., Sleiman, M., Singer, B. C., et al. (2011). Thirdhand tobacco smoke: emerging evidence and arguments for a multidisciplinary research agenda. Environ. Health Perspect. 119, 1218–1226. doi:10.1289/ehp.1103500
Merecz-Sadowska, A., Sitarek, P., Zielinska-Blizniewska, H., Malinowska, K., Zajdel, K., Zakonnik, L., et al. (2020). A summary of in vitro and in vivo studies evaluating the impact of E-cigarette exposure on living organisms and the environment. Int. J. Mol. Sci. 21, 652. doi:10.3390/ijms21020652
Mohamed, A. A., Bohy, K. M. E., Moustafa, G. G., Mohammed, H. H., Metwally, M. M. M., Mohammed, H. E. D., et al. (2022). Sustained functioning impairments and oxidative stress with neurobehavioral dysfunction associated with oral nicotine exposure in the brain of a murine model of ehrlich ascites carcinoma: modifying the antioxidant role of chlorella vulgaris. Biol. (Basel) 11, 279. doi:10.3390/biology11020279
Moreno-Gonzalez, I., Estrada, L. D., Sanchez-Mejias, E., and Soto, C. (2013). Smoking exacerbates amyloid pathology in a mouse model of Alzheimer's disease. Nat. Commun. 4, 1495. doi:10.1038/ncomms2494
Mukharjee, S., Bank, S., and Maiti, S. (2020). Chronic tobacco exposure by smoking develops insulin resistance. Endocr. Metab. Immune Disord. Drug Targets 20, 869–877. doi:10.2174/1871530320666200217123901
Mukhopadhyay, P., Horn, K. H., Greene, R. M., and Michele Pisano, M. (2010). Prenatal exposure to environmental tobacco smoke alters gene expression in the developing murine hippocampus. Reprod. Toxicol. 29, 164–175. doi:10.1016/j.reprotox.2009.12.001
Mulderrig, L., Garaycoechea, J. I., Tuong, Z. K., Millington, C. L., Dingler, F. A., Ferdinand, J. R., et al. (2021). Aldehyde-driven transcriptional stress triggers an anorexic DNA damage response. Nature 600, 158–163. doi:10.1038/s41586-021-04133-7
Murphy, P. J., Guo, J., Jenkins, T. G., James, E. R., Hoidal, J. R., Huecksteadt, T., et al. (2020). NRF2 loss recapitulates heritable impacts of paternal cigarette smoke exposure. PLoS Genet. 16, e1008756. doi:10.1371/journal.pgen.1008756
Nadalutti, C. A., Prasad, R., and Wilson, S. H. (2021). Perspectives on formaldehyde dysregulation: mitochondrial DNA damage and repair in mammalian cells. DNA Repair (Amst) 105, 103134. doi:10.1016/j.dnarep.2021.103134
Neal, R. E., Jagadapillai, R., Chen, J., Webb, C., Stocke, K., Greene, R. M., et al. (2016). Developmental cigarette smoke exposure II: Hippocampus proteome and metabolome profiles in adult offspring. Reprod. Toxicol. 65, 436–447. doi:10.1016/j.reprotox.2016.05.007
Neven, J., Issayama, L. K., Dewachter, I., and Wilson, D. M. (2024). Genomic stress and impaired DNA repair in Alzheimer disease. DNA Repair (Amst) 139, 103678. doi:10.1016/j.dnarep.2024.103678
Oka, S., Leon, J., Sakumi, K., Abolhassani, N., Sheng, Z., Tsuchimoto, D., et al. (2021). MTH1 and OGG1 maintain a low level of 8-oxoguanine in Alzheimer's brain, and prevent the progression of Alzheimer's pathogenesis. Sci. Rep. 11, 5819. doi:10.1038/s41598-021-84640-9
Olmedo, P., Goessler, W., Tanda, S., Grau-Perez, M., Jarmul, S., Aherrera, A., et al. (2018). Metal concentrations in e-cigarette liquid and aerosol samples: the contribution of metallic coils. Environ. Health Perspect. 126, 027010. doi:10.1289/EHP2175
Omaiye, E. E., Luo, W., McWhirter, K. J., Pankow, J. F., and Talbot, P. (2022). Disposable puff bar electronic cigarettes: chemical composition and toxicity of E-liquids and a synthetic coolant. Chem. Res. Toxicol. 35, 1344–1358. doi:10.1021/acs.chemrestox.1c00423
Ou, X. X., Wang, X., Zhan, X. L., Shen, S. L., Karatela, S., Jing, J., et al. (2024). The associations of secondhand smoke exposure with neurodevelopmental disorders and critical time window identification: a systematic review and meta-analysis. Sci. Total Environ. 913, 169649. doi:10.1016/j.scitotenv.2023.169649
Ourry, V., Binette, A. P., St-Onge, F., Strikwerda-Brown, C., Chagnot, A., Poirier, J., et al. (2024). How do modifiable risk factors affect alzheimer's disease pathology or mitigate its effect on clinical symptom expression? Biol. Psychiatry 95, 1006–1019. doi:10.1016/j.biopsych.2023.09.003
Pagani, L. S. (2014). Environmental tobacco smoke exposure and brain development: the case of attention deficit/hyperactivity disorder. Neurosci. Biobehav Rev. 44, 195–205. doi:10.1016/j.neubiorev.2013.03.008
Pandics, T., Major, D., Fazekas-Pongor, V., Szarvas, Z., Peterfi, A., Mukli, P., et al. (2023). Exposome and unhealthy aging: environmental drivers from air pollution to occupational exposures. Geroscience 45, 3381–3408. doi:10.1007/s11357-023-00913-3
Pankow, J. F. (2017). Calculating compound dependent gas-droplet distributions in aerosols of propylene glycol and glycerol from electronic cigarettes. J. Aerosol Sci. 107, 9–13. doi:10.1016/j.jaerosci.2017.02.003
Perera, F. P., Chang, H. W., Tang, D., Roen, E. L., Herbstman, J., Margolis, A., et al. (2014). Early-life exposure to polycyclic aromatic hydrocarbons and ADHD behavior problems. PLoS One 9, e111670. doi:10.1371/journal.pone.0111670
Ponzoni, L., Braida, D., Carboni, L., Moretti, M., Viani, P., Clementi, F., et al. (2020). Persistent cognitive and affective alterations at late withdrawal stages after long-term intermittent exposure to tobacco smoke or electronic cigarette vapour: behavioural changes and their neurochemical correlates. Pharmacol. Res. 158, 104941. doi:10.1016/j.phrs.2020.104941
Prasad, K. N., and Bondy, S. C. (2022). Electronic cigarette aerosol increases the risk of organ dysfunction by enhancing oxidative stress and inflammation. Drug Chem. Toxicol. 45, 2561–2567. doi:10.1080/01480545.2021.1972680
Prasedya, E. S., Ambana, Y., Martyasari, N. W. R., Aprizal, Y., Nurrijawati, , and Sunarpi, (2020). Short-term E-cigarette toxicity effects on brain cognitive memory functions and inflammatory responses in mice. Toxicol. Res. 36, 267–273. doi:10.1007/s43188-019-00031-3
Prochaska, J. J., Vogel, E. A., and Benowitz, N. (2022). Nicotine delivery and cigarette equivalents from vaping a JUULpod. Tob. Control 31, e88–e93. doi:10.1136/tobaccocontrol-2020-056367
Public Health Service (2006). The Health Consequences of Involuntary Exposure to Tobacco Smoke. Atlanta (GA): A Report of the Surgeon General.
Qing, X., Zhang, G., and Wang, Z. Q. (2023). DNA damage response in neurodevelopment and neuromaintenance. FEBS J. 290, 3300–3310. doi:10.1111/febs.16535
Qiu, T., Xie, F., Zeng, Q., Shen, Z., Du, G., Xu, X., et al. (2022). Interactions between cigarette smoking and cognitive status on functional connectivity of the cortico-striatal circuits in individuals without dementia: a resting-state functional MRI study. CNS Neurosci. Ther. 28, 1195–1204. doi:10.1111/cns.13852
Quintana, P. J. E., Lopez-Galvez, N., Dodder, N. G., Hoh, E., Matt, G. E., Zakarian, J. M., et al. (2021). Nicotine, cotinine, and tobacco-specific nitrosamines measured in children's silicone wristbands in relation to secondhand smoke and E-cigarette vapor exposure. Nicotine Tob. Res. 23, 592–599. doi:10.1093/ntr/ntaa140
Raber, J., Perez, R., Torres, E. R. S., Krenik, D., Boutros, S., Patel, E., et al. (2021). Effects of chronic secondhand smoke (SHS) exposure on cognitive performance and metabolic pathways in the Hippocampus of wild-type and human tau mice. Environ. Health Perspect. 129, 57009. doi:10.1289/EHP8428
Raber, J., Stagaman, K., Kasschau, K. D., Davenport, C., Lopes, L., Nguyen, D., et al. (2023). Behavioral and cognitive performance following exposure to second-hand smoke (SHS) from tobacco products associated with oxidative-stress-induced DNA damage and repair and disruption of the gut microbiome. Genes (Basel) 14, 1702. doi:10.3390/genes14091702
Rana, I., Rieswijk, L., Steinmaus, C., and Zhang, L. (2021). Formaldehyde and brain disorders: a meta-analysis and bioinformatics approach. Neurotox. Res. 39, 924–948. doi:10.1007/s12640-020-00320-y
Rauschert, S., Melton, P. E., Burdge, G., Craig, J. M., Godfrey, K. M., Holbrook, J. D., et al. (2019). Maternal smoking during pregnancy induces persistent epigenetic changes into adolescence, independent of postnatal smoke exposure and is associated with cardiometabolic risk. Front. Genet. 10, 770. doi:10.3389/fgene.2019.00770
Reingruber, H., and Pontel, L. B. (2018). Formaldehyde metabolism and its impact on human health. Curr. Opin. Toxicol. 9, 28–34. doi:10.1016/j.cotox.2018.07.001
Ruszkiewicz, J. A., Zhang, Z., Goncalves, F. M., Tizabi, Y., Zelikoff, J. T., and Aschner, M. (2020). Neurotoxicity of e-cigarettes. Food Chem. Toxicol. 138, 111245. doi:10.1016/j.fct.2020.111245
Schick, S., and Glantz, S. (2005). Philip Morris toxicological experiments with fresh sidestream smoke: more toxic than mainstream smoke. Tob. Control 14, 396–404. doi:10.1136/tc.2005.011288
Schick, S. F., and Glantz, S. (2007). Concentrations of the carcinogen 4-(methylnitrosamino)-1-(3-pyridyl)-1-butanone in sidestream cigarette smoke increase after release into indoor air: results from unpublished tobacco industry research. Cancer Epidemiol. Biomarkers Prev. 16, 1547–1553. doi:10.1158/1055-9965.EPI-07-0210
Schober, W., Szendrei, K., Matzen, W., Osiander-Fuchs, H., Heitmann, D., Schettgen, T., et al. (2014). Use of electronic cigarettes (e-cigarettes) impairs indoor air quality and increases FeNO levels of e-cigarette consumers. Int. J. Hyg. Environ. Health 217, 628–637. doi:10.1016/j.ijheh.2013.11.003
Schwab, N., Ju, Y., and Hazrati, L. N. (2021). Early onset senescence and cognitive impairment in a murine model of repeated mtbi. Acta. Neuropathol. Commun. 82. doi:10.1186/s40478-021-01190-x
Shadfar, S., Parakh, S., Jamali, M. S., and Atkin, J. D. (2023). Redox dysregulation as a driver for DNA damage and its relationship to neurodegenerative diseases. Transl. Neurodegener. 12, 18. doi:10.1186/s40035-023-00350-4
Siegel, J., Patel, S. H., Mankaliye, B., and Raval, A. P. (2022). Impact of electronic cigarette vaping on cerebral ischemia: what we know so far. Transl. Stroke Res. 13, 923–938. doi:10.1007/s12975-022-01011-w
Simpson, J. E., Ince, P. G., Minett, T., Matthews, F. E., Heath, P. R., Shaw, P. J., et al. (2016). Neuronal DNA damage response-associated dysregulation of signalling pathways and cholesterol metabolism at the earliest stages of Alzheimer-type pathology. Neuropathol. Appl. Neurobiol. 42, 167–179. doi:10.1111/nan.12252
Slotkin, T. A., Skavicus, S., Card, J., Stadler, A., Levin, E. D., and Seidler, F. J. (2015). Developmental neurotoxicity of tobacco smoke directed toward cholinergic and serotonergic systems: more than just nicotine. Toxicol. Sci. 147, 178–189. doi:10.1093/toxsci/kfv123
Slotkin, T. A., Skavicus, S., Ko, A., Levin, E. D., and Seidler, F. J. (2019). The developmental neurotoxicity of tobacco smoke can Be mimicked by a combination of nicotine and benzo[a]Pyrene: effects on cholinergic and serotonergic systems. Toxicol. Sci. 167, 293–304. doi:10.1093/toxsci/kfy241
Soleimani, F., Dobaradaran, S., De-la-Torre, G. E., Schmidt, T. C., and Saeedi, R. (2022). Content of toxic components of cigarette, cigarette smoke vs cigarette butts: a comprehensive systematic review. Sci. Total Environ. 813, 152667. doi:10.1016/j.scitotenv.2021.152667
St Helen, G., Havel, C., Dempsey, D. A., Jacob, P., and Benowitz, N. L. (2016). Nicotine delivery, retention and pharmacokinetics from various electronic cigarettes. Addiction 111, 535–544. doi:10.1111/add.13183
Strongin, R. M., Sharma, E., Erythropel, H. C., Kassem, N. O. F., Noel, A., Peyton, D. H., et al. (2024). Chemical and physiological interactions between e-liquid constituents: cause for concern? Tob Control.
Tang, M. S., Lee, H. W., Weng, M. W., Wang, H. T., Hu, Y., Chen, L. C., et al. (2022). DNA damage, DNA repair and carcinogenicity: tobacco smoke versus electronic cigarette aerosol. Mutat. Res. Rev. Mutat. Res. 789, 108409. doi:10.1016/j.mrrev.2021.108409
Tattan-Birch, H., Brown, J., Jackson, S. E., Jarvis, M. J., and Shahab, L. (2024). Secondhand nicotine absorption from E-cigarette vapor vs tobacco smoke in children. JAMA Netw. Open 7, e2421246. doi:10.1001/jamanetworkopen.2024.21246
Tiwari, R. K., Sharma, V., Pandey, R. K., and Shukla, S. S. (2020). Nicotine addiction: neurobiology and mechanism. J. Pharmacopuncture 23, 1–7. doi:10.3831/KPI.2020.23.001
Toro, R., Leonard, G., Lerner, J. V., Lerner, R. M., Perron, M., Pike, G. B., et al. (2008). Prenatal exposure to maternal cigarette smoking and the adolescent cerebral cortex. Neuropsychopharmacology 33, 1019–1027. doi:10.1038/sj.npp.1301484
Torres, S., Merino, C., Paton, B., Correig, X., and Ramirez, N. (2018). Biomarkers of exposure to secondhand and thirdhand tobacco smoke: recent advances and future perspectives. Int. J. Environ. Res. Public Health 15, 2693. doi:10.3390/ijerph15122693
Tulen, C. B. M., Duistermaat, E., Cremers, J., Klerx, W. N. M., Fokkens, P. H. B., Weibolt, N., et al. (2022). Smoking-associated exposure of human primary bronchial epithelial cells to aldehydes: impact on molecular mechanisms controlling mitochondrial content and function. Cells 11, 3481. doi:10.3390/cells11213481
Upadhyay, S., Rahman, M., Johanson, G., Palmberg, L., and Ganguly, K. (2023). Heated tobacco products: insights into composition and toxicity. Toxics 11, 667. doi:10.3390/toxics11080667
Varani, A. P., Pedron, V. T., Aon, A. J., Hocht, C., Acosta, G. B., Bettler, B., et al. (2018). Nicotine-induced molecular alterations are modulated by GABA(B) receptor activity. Addict. Biol. 23, 230–246. doi:10.1111/adb.12506
Vivarelli, F., Granata, S., Rullo, L., Mussoni, M., Candeletti, S., Romualdi, P., et al. (2022). On the toxicity of e-cigarettes consumption: focus on pathological cellular mechanisms. Pharmacol. Res. 182, 106315. doi:10.1016/j.phrs.2022.106315
Vivarelli, F., Morosini, C., Rullo, L., Losapio, L. M., Lacorte, A., Sangiorgi, S., et al. (2024). Effects of unburned tobacco smoke on inflammatory and oxidative mediators in the rat prefrontal cortex. Front. Pharmacol. 15, 1328917. doi:10.3389/fphar.2024.1328917
Wade, N. E., McCabe, C. J., Wallace, A. L., Gonzalez, M. R., Hoh, E., Infante, M. A., et al. (2023). Clouding up cognition? Secondhand cannabis and tobacco exposure related to cognitive functioning in youth. Biol. Psychiatry Glob. Open Sci. 3, 233–242. doi:10.1016/j.bpsgos.2022.01.010
Wan, Z., Zhang, X., He, H., Zhang, Y., Chen, G. C., Qin, L. Q., et al. (2024). Secondhand smoke exposure and risk of dementia in nonsmokers: a population-based cohort study. Neuroepidemiology 58, 166–173. doi:10.1159/000535828
Wang, X., Wang, J., Chen, Y., Qian, X., Luo, S., Wang, X., et al. (2024a). The aldehyde dehydrogenase 2 rs671 variant enhances amyloid β pathology. Nat. Commun. 15, 2594. doi:10.1038/s41467-024-46899-0
Wang, D., Ma, Z., Fan, Y., Chen, H., Sun, M., and Fan, F. (2024b). Tobacco smoking, second-hand smoking exposure in relation to psychotic-like experiences in adolescents. Early Interv. Psychiatry 18, 102–112. doi:10.1111/eip.13439
Wells, A. C., and Lotfipour, S. (2023). Prenatal nicotine exposure during pregnancy results in adverse neurodevelopmental alterations and neurobehavioral deficits. Adv. Drug Alcohol Res. 3, 11628. doi:10.3389/adar.2023.11628
Weng, M. W., Lee, H. W., Park, S. H., Hu, Y., Wang, H. T., Chen, L. C., et al. (2018). Aldehydes are the predominant forces inducing DNA damage and inhibiting DNA repair in tobacco smoke carcinogenesis. Proc. Natl. Acad. Sci. U. S. A. 115, E6152–E6161. doi:10.1073/pnas.1804869115
Wirz, K. T., Keitel, S., Swaab, D. F., Verhaagen, J., and Bossers, K. (2014). Early molecular changes in Alzheimer disease: can we catch the disease in its presymptomatic phase? J. Alzheimers Dis. 38, 719–740. doi:10.3233/JAD-130920
Xu, P., Liu, B., Chen, H., Wang, H., Guo, X., and Yuan, J. (2024). PAHs as environmental pollutants and their neurotoxic effects. Comp. Biochem. Physiol. C Toxicol. Pharmacol. 283, 109975. doi:10.1016/j.cbpc.2024.109975
Yan, B., Zagorevski, D., Ilievski, V., Kleiman, N. J., Re, D. B., Navas-Acien, A., et al. (2021). Identification of newly formed toxic chemicals in E-cigarette aerosols with Orbitrap mass spectrometry and implications on E-cigarette control. Eur. J. Mass Spectrom. (Chichester) 27, 141–148. doi:10.1177/14690667211040207
Yan, T., Zhao, Y., Jiang, Z., and Chen, J. (2022). Acetaldehyde induces cytotoxicity via triggering mitochondrial dysfunction and overactive mitophagy. Mol. Neurobiol. 59, 3933–3946. doi:10.1007/s12035-022-02828-0
Yolton, K., Dietrich, K., Auinger, P., Lanphear, B. P., and Hornung, R. (2005). Exposure to environmental tobacco smoke and cognitive abilities among U.S. children and adolescents. Environ. Health Perspect. 113, 98–103. doi:10.1289/ehp.7210
Yuan, Y., Wu, Y., Zhao, H., Ren, J., Su, W., Kou, Y., et al. (2023). Tropospheric formaldehyde levels infer ambient formaldehyde-induced brain diseases and global burden in China, 2013-2019. Sci. Total Environ. 883, 163553. doi:10.1016/j.scitotenv.2023.163553
Zhai, R., Rizak, J., Zheng, N., He, X., Li, Z., Yin, Y., et al. (2018). Alzheimer's disease-like pathologies and cognitive impairments induced by formaldehyde in non-human primates. Curr. Alzheimer Res. 15, 1304–1321. doi:10.2174/1567205015666180904150118
Zhou, S., and Wang, K. (2021). Childhood secondhand smoke exposure and risk of dementia, alzheimer's disease and stroke in adulthood: a prospective cohort study. J. Prev. Alzheimers Dis. 8, 345–350. doi:10.14283/jpad.2021.10
Zieba, S., Blachnio-Zabielska, A., Maciejczyk, M., Pogodzinska, K., Szuta, M., Lo Giudice, G., et al. (2024). Impact of smoking on salivary lipid profile and oxidative stress in young adults: a comparative analysis between traditional cigarettes, E-cigarettes, and heat-not-burn products. Med. Sci. Monit. 30, e942507. doi:10.12659/msm.942507
Keywords: environmental tobacco smoke (ETS), nicotine, oxidative stress, e-cigarettes, DNA damage/repair
Citation: Kisby G and Raber J (2025) World no-tobacco: effects of second-hand smoke (SHS) and vapors on the developing and adult brain. Front. Pharmacol. 16:1466332. doi: 10.3389/fphar.2025.1466332
Received: 17 July 2024; Accepted: 05 February 2025;
Published: 06 March 2025.
Edited by:
Nicholas M. Barnes, University of Birmingham, United KingdomReviewed by:
Qihai Gu, Mercer University, United StatesVijayapandi Pandy, MIT World Peace University, India
Kabirullah Lutfy, Western University of Health Sciences, United States
Intan Suhana Munira Mat Azmi, Sultan Zainal Abidin University, Malaysia
Copyright © 2025 Kisby and Raber. This is an open-access article distributed under the terms of the Creative Commons Attribution License (CC BY). The use, distribution or reproduction in other forums is permitted, provided the original author(s) and the copyright owner(s) are credited and that the original publication in this journal is cited, in accordance with accepted academic practice. No use, distribution or reproduction is permitted which does not comply with these terms.
*Correspondence: Jacob Raber, cmFiZXJqQG9oc3UuZWR1; Glen Kisby, Z2tpc2J5QHdlc3Rlcm51LmVkdQ==