- 1Department of Pharmacological and Pharmaceutical Sciences, College of Pharmacy, University of Houston, Houston, TX, United States
- 2Department of Pharmaceutical Sciences, Irma Lerma College of Pharmacy, Texas A&M University, Kingsville, TX, United States
Introduction: Cigarette smoking is a well-established risk factor for renal dysfunction. Smoking associated with renal damage bears distinct physiological correlations in conditions such as diabetic nephropathy and obesity-induced glomerulopathy. However, the cellular and molecular basis of such an association remains poorly understood. High mobility group box 1(HMGB1) is a highly conserved non-histone chromatin associated protein that largely contributes to the pathogenesis of chronic inflammatory and autoimmune diseases such as sepsis, atherosclerosis, and chronic kidney diseases. Hence, the present study tested whether HMGB1 contributes to nicotine-induced podocyte injury.
Methods and Results: Biochemical analysis showed that nicotine treatment significantly increased the HMGB1 expression and release compared to vehicle treated podocytes. However, prior treatment with glycyrrhizin (Gly), a HMGB1 binder, abolished the nicotine-induced HMGB1 expression and release in podocytes. Furthermore, immunofluorescent analysis showed that nicotine treatment significantly decreased the expression of podocyte functional proteins- podocin and nephrin as compared to control cells. However, prior treatment with Gly attenuated the nicotine-induced nephrin and podocin reduction. In addition, nicotine treatment significantly increased desmin expression and cell permeability compared to vehicle treated podocytes. However, prior treatment with Gly attenuated the nicotine-induced desmin expression and cell permeability. Mechanistic elucidation revealed that nicotine treatment augmented the expression of toll like receptor 4 (TLR4) and pre-treatment with Gly abolished nicotine induced TLR4 upregulation. Pharmacological inhibition of TLR4 with Resatorvid, a TLR4 specific inhibitor, also attenuated nicotine induced podocyte damage.
Conclusion: HMGB1 is one of the important mediators of nicotine-induced podocyte injury through TLR4 activation.
1 Introduction
Cigarette smoking is a well-established cause for varied physiological dysfunctions viz cancer, atherosclerosis, thrombogenesis and vascular occlusion (Salonen and Salonen, 1993; United States. Dept. of Health and Human Services. et al., 1983; Passarelli et al., 2016; Jacobs et al., 2015; Lv et al., 2024; Datta et al., 2024a). In fact, cigarette smoking is responsible for close to 8 million mortalities globally every year- which also includes around 1.3 million non-smokers exposed to second-hand smoke (Organization, 2024). Cigarette smoking induces sympathetic stimulation, functional alterations in the endothelium, smooth muscle cell proliferation and dysfunction of vascular tone regulators- all of which play a significant role towards the onset and progression of arterial damage (Cryer et al., 1976; Ross, 1993). However, the exact mechanism involved remains largely unclear.
Studies over the years have suggested that cigarette smoking contributes to significant hemodynamic alterations and culminates to renal dysfunction (Franek et al., 1996; Mühlhauser, 1994). Meta-analysis patient-based studies and mechanistic investigations reveal that cigarette smoking is a significant risk factor that leads to both acute and chronic kidney injury onset and worsens conditions viz diabetic nephropathy, glomerulosclerosis, glomerulonephritis, and obesity associated glomerulopathy over the long term (Ito et al., 2020; Ataka et al., 2023; Wang et al., 2021; McDermott et al., 2020; Pesce et al., 2021; Liao et al., 2019; Jaimes et al., 2021; Gündoğdu and Anaforoğlu, 2022). Cigarette smoke is an aerosol comprising of both vapour and particulate phase materials (Smith and Fischer, 2001; Thielen et al., 2008; Borgerding and Klus, 2005; Osborne et al., 1956). Vapor phase constituents chiefly include carbon monoxide, acetaldehyde, formaldehyde, and nitrogen oxides (Moldoveanu and Charles, 2007; Pang and Lewis, 2011). Nicotine, biologically one of the most stable and active components of the particulate phase, is central to most of the pathophysiological dysfunctions associated with cigarette smoking (Benowitz and Burbank, 2016; West, 2017; Schweitzer et al., 2015). Active or passive forms of nicotine exposure enhances renal oxidative stress through mitochondrial reactive oxygen species (ROS) upregulation, transcriptional activation of the pro-apoptotic and pro-oxidant p66shc in renal proximal tubule cells, and NLRP3 inflammasome activation (Arany et al., 2016; Wu et al., 2018; Singh et al., 2019a; Wu et al., 2020; Datta et al., 2024b). Oxidative stress drives inflammatory cascades and renal fibrosis and culminates to chronic kidney injury and end-stage renal diseases (ESRD) (Arany et al., 2016; Wu et al., 2018; Singh et al., 2019a; Ramalingam et al., 2019; Akkoyun and Karadeniz, 2016; Harwani et al., 2016; Zheng et al., 2020; Mishra et al., 2015; Jha et al., 2018; Mayyas and Alzoubi, 2019). Although nicotine has been identified as a major risk factor for the onset and progression of CKI, clear mechanistic understanding of nicotine induced kidney damage remains largely unclear.
High Mobility Group Box 1 (HMGB1) is a highly conserved non-histone chromatin-associated protein widely recognized for its regulatory impact on vital cellular processes like autophagy, apoptosis, and cell survival (Datta et al., 2024a; Narumi et al., 2015; Tang et al., 2010a; Singh et al., 2019b). HMGB1 exhibits dual functionality based upon its localization- as a non-histone protein in the nucleus and as a prototypic damage associated molecular pattern (DAMP) molecule upon extracellular release (Gazzar et al., 2009; Yu and Spring, 1977; Zhang et al., 2019; Andersson et al., 2018). Upon extracellular release, HMGB1 orchestrates inflammatory cascades, immunological responses and drives conditions viz cancer, neurodegeneration, and cardiovascular complications (Tang et al., 2010b; Festoff et al., 2016; Huang et al., 2016). Pathophysiological insights reveal that HMGB1 also plays a significant role in the onset and progression of kidney damage (Good et al., 2015; Ito et al., 2007). Extracellular HMGB1 drives cellular damage and inflammatory cascades in renal ischemic reperfusion injury (IRI) and plays a pivotal role in acute kidney injury (AKI) onset via Tumor Necrosis Factor (TNF)-α/HMGB1 inflammatory signalling (Wu et al., 2010; Wang et al., 2020). Hence, in the current study we tested whether HMGB1 mediates nicotine-induced podocyte injury.
2 Materials and methods
2.1 Cell culture
A conditionally immortalized murine podocyte cell line (Division of Nephrology, Department of Medicine, Mount Sinai School of Medicine, NY, United States) was cultured undifferentiated with 10 U/mL recombinant mouse interferon-γ at 33°C on collagen I-coated flasks in RPMI-1640 media (Thermo Fisher Scientific, United States) containing 10% foetal bovine serum (R&D Systems, United States), 100 U/mL penicillin and 100 mg/mL streptomycin (Life Technologies Corporation, NY, United States). The podocytes were allowed to differentiate at 37°C for 10–14 days without interferon–γ. These differentiated podocytes were subsequently utilized for the experiments (Koka et al., 2019). Podocytes were pretreated with Glycyrrhizin (Gly, 120 μM) (Tokyo Chemical Industry Co. Ltd., Tokyo, Japan; dissolved in water for 30 min) (Mollica et al., 2007; Palumbo et al., 2004) or Resatorvid (TAK-242; 100 nM, Med Chem Express; United States; dissolved in dimethyl sulfoxide for 15 min) (Kashani et al., 2020; Kashani et al., 2019; Matsunaga et al., 2011) prior to nicotine treatment for overnight (8 μM; dissolved in water) (Singh et al., 2019a).
2.2 Immunofluorescence staining
The podocytes were grown on eight-well chamber slides (Thermo Fisher Scientific, United States) and treated as experimentally designed. The cells were then fixed with 4% paraformaldehyde for 15 min. Cells were then washed in phosphate-buffer saline (PBS) followed by blocking with 1% bovine serum albumin (BSA) for 1 h at room temperature. This was followed by primary antibody incubation at 4°C overnight against podocin (1:200, Sigma-Aldrich, United States; catalogue number P0372), desmin (1:200, Abcam, Cambridge, CA, United States; catalogue number ab15200), HMGB1 (1:200, Abcam, Cambridge, CA, United States; catalogue number ab18256) and nephrin (1:200; Santa Cruz Biotechnology, Inc., United States; catalogue number sc-377246). Subsequently, the slides were incubated at room temperature with Alexa Fluor 555-labeled secondary antibody (1:500, Invitrogen; catalogue numbers A32732 and A-31570) for 1 h. The slides were washed with PBS and mounted with DAPI containing mounting medium (Vector Laboratories, Inc., United States). The slides were sequentially scanned and imaged using confocal microscopy (Leica SP8 STED Confocal Microscope). ImageJ software was used for the quantification of mean fluorescence intensity of the images developed and statistical analysis was done using GraphPad Prism 9.2.0.
2.3 Extracellular HMGB1 quantification
The cultured murine podocytes were treated with nicotine with and without Gly (120 µM) overnight. The supernatants were collected and the concentration of the released extracellular HMGB1 was measured using commercial enzyme-linked immunosorbent assay (ELISA) kit (MyBioSource, San Diego, CA, United States) as per the manufacturer’s instructions.
2.4 Cell permeability assay
The monolayer permeability of podocytes in culture was measured according to previously optimized method (Boini et al., 2018; Boini et al., 2010). In summary, the podocytes were seeded in the upper chambers of 0.4 μm polycarbonate trans-well filters of a 24-well filtration microplate (Corning Inc., United States). After optimum confluence, the culture medium was replaced with fresh serum free RPMI 1640 media in presence of nicotine (8 μM) with or without Gly (30 min pretreatment) or Resatorvid (15 min pretreatment) and incubated overnight. Subsequently, the serum free media was discarded, fresh phenol red-free RPMI-1640 with 70 kDa fluorescein isothiocyanate (FITC)-dextran (2.5 μM) was added in the upper chambers and incubated for 3 h. Then, the filtration microplate was removed and the medium in the lower compartment was collected. The fluorescence intensity was measured in a spectrofluorometer (BioTek Instruments, Inc., Winooski, VT, United States) at 494 nm excitation and 521 nm emission wavelengths. The relative permeable fluorescence intensity was used as a measure of cell permeability.
2.5 Western blot
After being treated as experimentally designed, the cultured podocytes were washed with ice-cold PBS twice followed by homogenization in cell lysis buffer (BioVision, United States). After homogenization, they were centrifuged at 1,500 × g for 15 min at 4°C. The supernatants were collected and stored at −80°C until use. Cell homogenates were denatured with reducing Laemmli sodium dodecyl sulphate (SDS)-sample buffer and boiled for 5 min at 95°C. Homogenates were run on SDS-PAGE gel, transferred into a polyvinylidene difluoride (PVDF) membrane (Thermo Fisher Scientific, United States), and blocked with 5% BSA. The membranes were probed with primary antibodies for podocin (1:1000, Sigma-Aldrich, United States; catalogue number P0372), TLR4 (1:1000, Santa Cruz Biotechnology, Inc., United States; catalogue number sc-293072), RAGE (1:1000; Sigma-Aldrich, United States; catalogue number R5278), TLR2 (1:1000; Santa Cruz Biotechnology, Inc., United States; catalogue number sc-21759),and β-actin (1:1000; Santa Cruz Biotechnology, Inc., United States; catalogue number sc-47778) overnight at 4°C. The membranes were subsequently washed with 1X tris-buffered saline (TBS) and 0.5% tween, incubated with secondary antibody (catalogue numbers sc-2357 and 1706516) for 1 h, and then conjugated to horseradish peroxidase (HRP)-labelled immunoglobulin G. The bands on the membrane were enhanced by chemiluminescence. The membranes were scanned using Licor chemiluminescence system.
2.6 Statistical analysis
Quantification data for all the experiments were analysed using GraphPad Prism 9.2.0. Data was plotted as arithmetic mean ± standard error of mean (SEM); n represents the number of independent experiments. All data were tested for significance using Student’s unpaired t-test or one way ANOVA followed by a post hoc test. Results with p < 0.05 were considered statistically significant.
3 Results
3.1 Nicotine upregulates expression and extracellular release of HMGB1 in podocytes
To investigate the influence of nicotine on HMGB1 expression and extracellular release in podocytes, the cultured murine podocytes were treated with increasing concentrations of nicotine for 16 h. Our results show that nicotine dose dependently increases the HMGB1 expression (Figures 1A, B) and HMG1 extracellular release (Figure 1C) in podocytes.
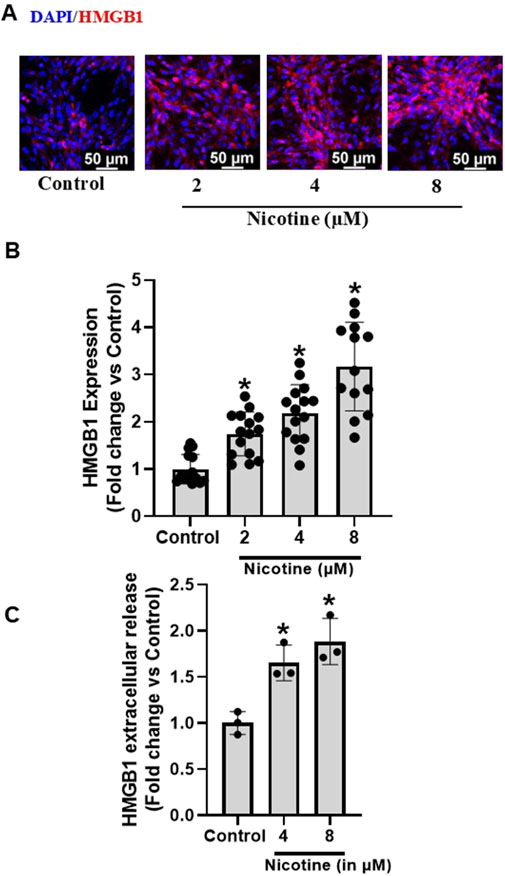
Figure 1. Effect of Nicotine on expression and extracellular release of HMGB1 in murine podocytes. Representative immunofluorescence images (Scale- 50 µm) (A) and summarized quantification data (B) depict HMGB1 expression in podocytes subjected to increasing concentrations of nicotine. ELISA quantification data (C) shows extracellular release pattern of HMGB1 as influenced by increasing nicotine concentrations. The immunofluorescence images were quantified using ImageJ software and statistical analysis of the quantified data was accomplished using GraphPad Prism 9.2.0. *p < 0.05 vs. control group.
3.2 HMGB1 targeted inhibition attenuates nicotine-induced HMGB1 expression and extracellular localization
Studies over the years have identified Gly, obtained from liquorice (Glycyrrhiza glabra) plant, as a potent inhibitor of HMGB1 and associated pro-inflammatory cascades (Mollica et al., 2007; Palumbo et al., 2004). Herein, we tested the influence of Gly on nicotine-induced HMGB1 expression and extracellular release. Our investigations reveal that Gly attenuates nicotine induced increase in extracellular release of HMGB1 (Figure 2A). Additionally, our immunofluorescence results show that Gly attenuates nicotine induced HMGB1 expression upregulation (Figures 2B, C). Together, these results confirm that HMGB1 binder Gly interferes with and attenuates nicotine induced increase in HMGB1 expression and extracellular release.
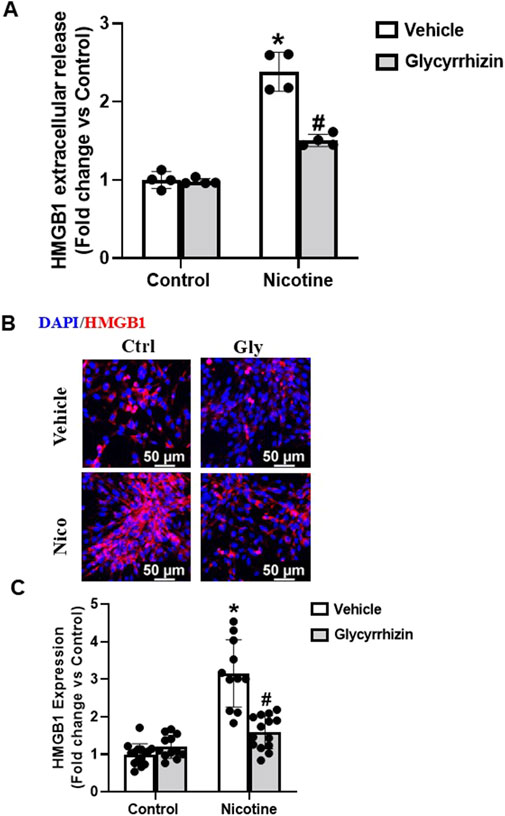
Figure 2. HMGB1 specific inhibition attenuates nicotine induced HMGB1 expression and extracellular release. ELISA quantification data (A), representative immunofluorescence images (Scale- 50 µm) (B) and summarized quantification (C) outlines the influence of Gly (HMGB1 specific binder) on nicotine induced extracellular release and expression of HMGB1. The immunofluorescence images were quantified using ImageJ software and statistical analysis of the quantified data was accomplished using GraphPad Prism 9.2.0. *p < 0.05 vs. control group, #p < 0.05 vs. nicotine treated group; Ctrl- Control, Gly- Glycyrrhizin, Nico- Nicotine.
3.3 Nicotine mediates podocyte damage in a HMGB1-dependent manner
Podocyte specific proteins podocin and nephrin are central to podocyte function and are downregulated upon podocyte damage (Saleem et al., 2002; Perico et al., 2016). In coherence with our former investigations (Singh et al., 2019a), our immunofluorescence analysis showed that nicotine-induced decrease in nephrin and podocin expression (Figures 3A–D). However, pre-treatment with Gly attenuated nicotine induced nephrin and podocin downregulation (Figures 3A–D). In addition, our immunofluorescence analysis studies also reveal that nicotine upregulates cytoskeletal desmin levels and mediates podocyte damage (Figures 3E, F). However, prior treatment with Gly prevents nicotine induced desmin upregulation (Figures 3E, F). Our results confirm that nicotine drives podocyte damage through HMGB1 activation and prior attenuation of HMGB1 extracellular release protects against nicotine induced podocyte injury.
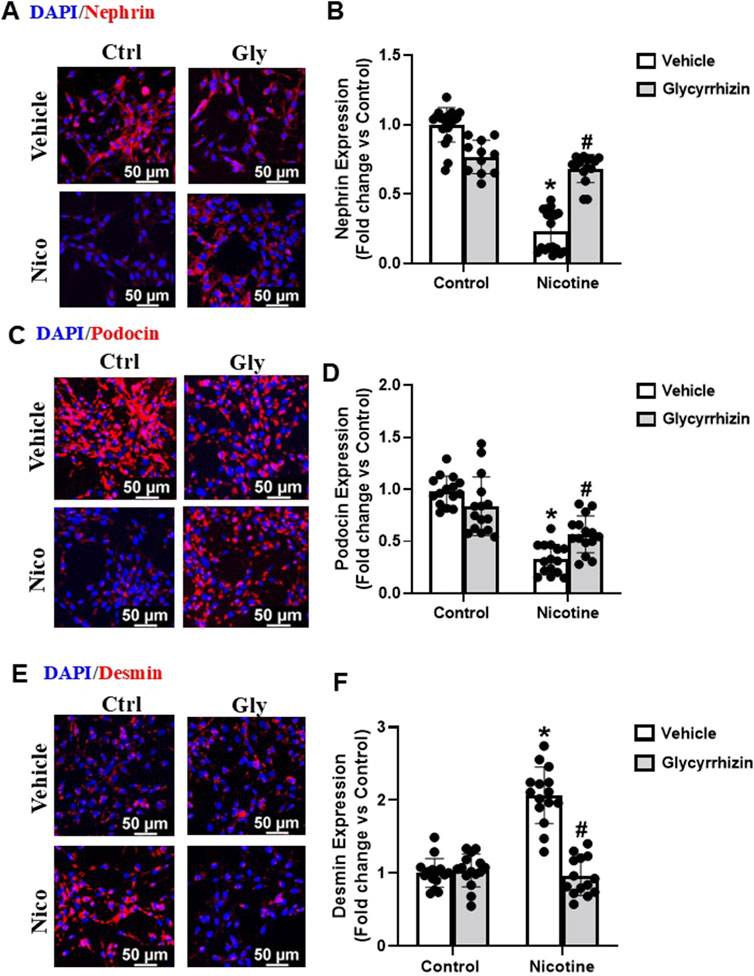
Figure 3. HMGB1 specific inhibition attenuates nicotine induced functional and structural damage in podocytes. Representative immunofluorescence images (Scale- 50 µm) and summarized quantification outlines the influence of Gly on nephrin (A, B), podocin (C, D) and cytoskeletal desmin (E, F) expression in podocytes treated with nicotine. ImageJ software was used for quantification of the immunofluorescence images and statistical analysis of the quantified data was accomplished using GraphPad Prism 9.2.0. *p < 0.05 vs. control group, #p < 0.05 vs. nicotine treated group; Ctrl- Control, Gly- Glycyrrhizin, Nico- Nicotine.
3.4 HMGB1 targeted inhibition attenuates nicotine induced podocyte permeability
Next, in functional studies we tested how monolayer permeability of podocytes was affected by nicotine in the presence and absence of Gly. Nicotine augments podocyte permeability relative to their control cells (Figure 4). However, prior treatment with Gly attenuates nicotine induced podocyte permeability upsurge (Figure 4). Our results confirm that inhibition of HMGB1 nucleus/cytoplasm translocation prevents nicotine associated upsurge in podocyte permeability.
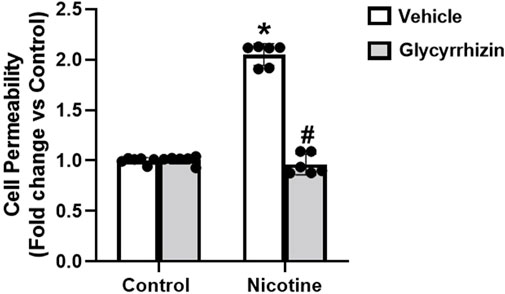
Figure 4. HMGB1 inhibition attenuates nicotine induced podocyte permeability. The influence of Gly on nicotine induced podocyte permeability was assessed using cell permeability assay. Statistical analysis of the quantified data was accomplished using GraphPad Prism 9.2.0. *p < 0.05 vs. control group, #p < 0.05 vs. nicotine treated group.
3.5 HMGB1 inhibition prevents nicotine induced toll-like receptor (TLR)4 upregulation in podocytes
Further we tested the influence of nicotine on cultured podocytes with and without HMGB1 inhibition. Our results reveal that nicotine increases TLR4 expression in podocytes as compared to the control counterparts (Figures 5A, B). However, prior treatment with Gly attenuated nicotine induced TLR4 upsurge (Figures 5A, B). We also tested for the influence of nicotine on TLR2 and receptor for advanced glycation end products (RAGE) in the presence and absence of Gly. TLR2 and RAGE levels exhibited no significant difference for nicotine with or without Gly relative to control podocytes (data not shown here). These results outline that HMGB1 mediates nicotine induced podocyte damage potentially via pro-inflammatory TLR4 upregulation and HMGB1 inhibition attenuates nicotine- induced TLR4 upregulation.
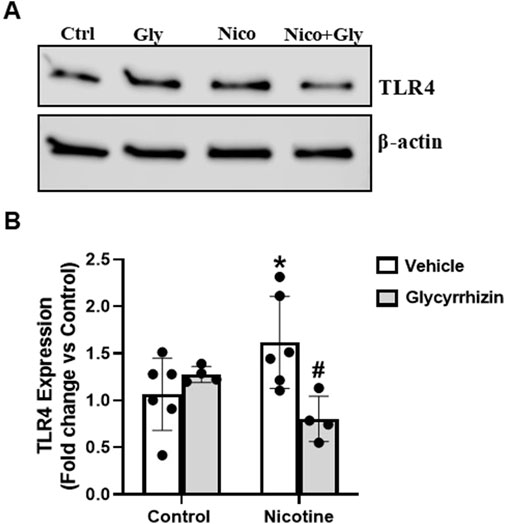
Figure 5. HMGB1 inhibition attenuates nicotine induced Toll-like receptor (TLR)-4 upregulation in podocytes. Western blot images (A) and associated quantification data (B) summarize the influence of Gly on nicotine induced TLR4 upregulation in podocytes. Western blot band intensity values were obtained using Image Studio Lite 5.2 and statistical analysis of the quantified data was done using GraphPad Prism 9.2.0. *p < 0.05 vs. control group, #p < 0.05 vs. nicotine treated group; Ctrl- Control, Gly- Glycyrrhizin, Nico- Nicotine, Nico + Gly- Nicotine + Glycyrrhizin.
3.6 TLR4 pharmacological inhibition lessens nicotine induced podocyte damage
In this study, we utilized Resatorvid (TAK-242), a small molecule inhibitor of TLR4 (Kashani et al., 2020; Kashani et al., 2019; Matsunaga et al., 2011), to investigate its influence on nicotine-induced podocyte injury. Dose-dependent studies were carried out to determine the optimum concentration of Resatorvid for our experiments (data not shown). 100 nM was found to be the optimum concentration for Resatorvid and utilized for further experiments in this study. Our immunofluorescence studies showed that Resatorvid protects against nicotine associated decrease in nephrin and podocin levels (Figures 6A–D). Additionally, Resatorvid attenuates nicotine induced desmin upregulation (Figures 6E, F). Together, these results confirm that nicotine-induced podocyte damage is mediated via TLR4 activation and TLR4 specific inhibition protects against nicotine associated podocyte damage.
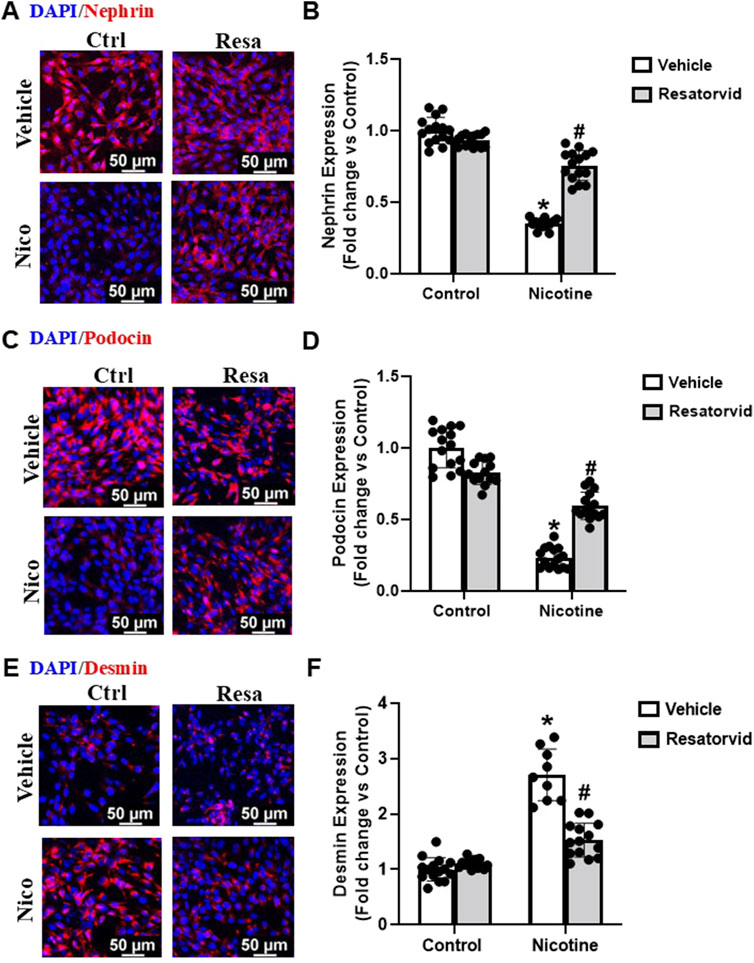
Figure 6. Pharmacological TLR4 inhibition lessens nicotine associated functional and structural injury in podocytes. Representative immunofluorescence images (Scale- 50 µm) and summarized quantification outlines the influence of Resatorvid (TLR4 specific inhibitor) on nephrin (A, B), podocin (C, D) and desmin (E, F) expression in podocytes treated with nicotine. ImageJ software was used for quantification of the immunofluorescence images and statistical analysis of the quantified data was accomplished using GraphPad Prism 9.2.0. *p < 0.05 vs. control group, #p < 0.05 vs. nicotine treated group; Ctrl- Control, Resa- Resatorvid (100 nM), Nico- Nicotine (8 µM).
3.7 TLR4 specific inhibition attenuates nicotine induced podocyte permeability
Furthermore, we tested the influence of Resatorvid mediated TLR4 inhibition on nicotine induced upsurge in podocyte permeability. Our results show that TLR4 inhibition protects against nicotine associated rise in podocyte monolayer permeability (Figure 7). These results further consolidate our understanding that TLR4 inhibition protects against nicotine-induced podocyte damage.
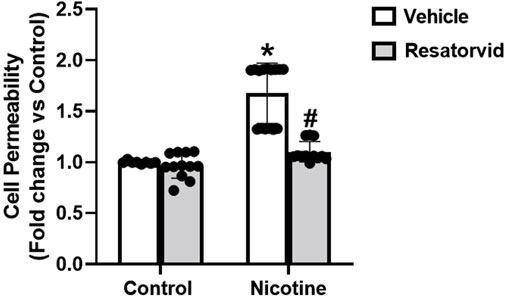
Figure 7. Pharmacological TLR4 inhibition attenuates nicotine induced podocyte permeability. The impact of Resatorvid on nicotine induced podocyte permeability was assessed using cell permeability assay. Statistical analysis of the quantified data was accomplished using GraphPad Prism 9.2.0. *p < 0.05 vs. control group, #p < 0.05 vs. nicotine treated group.
4 Discussion
The goal of the present study is to determine whether HMGB1 is implicated in the development of nicotine-induced podocyte injury. Our findings demonstrate that nicotine upregulates TLR4 expression and HMGB1 expression and extracellular release in mediating podocyte injury. However, pre-treatment with HMGB1 inhibiting Gly attenuated nicotine induced HMGB1 and TLR4 upregulation and associated podocyte damage. To our understanding, this is the first study to establish the pathophysiological role of HMGB1 in nicotine induced podocyte injury, primarily via TLR4 upregulation.
Nicotine, one of the most stable and active components of cigarette smoke, plays a pivotal role towards the onset and progression of proteinuria, diabetic nephropathy and subsequently, CKD (Briganti et al., 2002; Rossing et al., 2002; Hallan and Orth, 2011). Chronic exposure to nicotine augments mitochondrial ROS levels and exacerbates mitochondrial depolarization and renal cell apoptosis and/or necrosis- chiefly through the inhibition of epidermal growth factor receptor/Ras/Mitogen activated protein kinase (MAPK)/Extracellular signal-regulated kinase (ERK) cascade (Arany et al., 2010; Arany et al., 2008). Nicotine augments cyclooxygenase (COX)-2 expression and ERK1/2 phosphorylation and mediates proliferation and fibronectin generation in kidney-derived mesangial cells (Jaimes et al., 2009; Hua et al., 2010). Nicotine induced oxidative stress upregulates c-Jun N-terminal kinase (JNK) driven activator protein (AP)-1 activation and attributes to the tubular effects of nicotine (Arany et al., 2011). Renal function evaluation studies reveal that nicotine significantly reduces renal plasma flow rate and augments microalbuminuria risk (Gambaro et al., 1998; Gerstein et al., 2000). Smoking associated nicotine exposure downregulates estimated glomerular filtration rate (eGFR) and augments progression of proteinuria and autosomal polycystic kidney disease (Ozkok et al., 2013; Chase et al., 1991). However, exact mechanistic understanding of nicotine induced renal damage remains poorly understood and largely limits draggability in this regard.
High Mobility Group Box 1 (HMGB1) is a highly conserved non-histone chromatin-associated protein across species (Gazzar et al., 2009; Štros, 2010). It functions as a non-histone protein in the nucleus and as an inducer of inflammatory cytokines upon extracellular release (Gazzar et al., 2009; Andersson et al., 2018; Genschel and Modrich, 2009). Existing paradigm of studies show that HMGB1 is central to the onset and progression of renal dysfunctions (Poston and Koyner, 2019; Wang et al., 2004; Zhao et al., 2020). Studies outline that HMGB1 exhibits a regulatory role in driving onset and progression of secondary renal damage like glomerulonephritis, diabetic nephropathy and lupus nephritis (Tachibana et al., 2019; Andersen et al., 2014). Extracellularly released HMGB1 activates NF-ƘB signalling and mediates release of pro-inflammatory cytokines like TNF-α, Interleukin (IL)-6 and IL-1β in serum (Anders et al., 2018; Chen et al., 2018; Shen et al., 2024). However, the pathophysiological role of HMGB1 in smoking associated renal damage remains poorly understood. In this regard, our present study confirms that nicotine augments intracellular expression and extracellular release of HMGB1 in murine podocytes in a dose-dependent manner. Prior treatment with Glycyrrhizin (Gly), a HMGB1 binder (Mollica et al., 2007; Palumbo et al., 2004), attenuates nicotine associated HMGB1 upregulation in podocytes.
Podocin and Nephrin are podocyte specific proteins that are central to structural and functional integrity maintenance in podocytes (Saleem et al., 2002; Perico et al., 2016). Podocin governs structural organization of the slit diaphragm via interaction with podocyte specific nephrin and CD2 associated protein (CD2AP) (Saleem et al., 2002; Huber et al., 2003; Schwarz et al., 2001). On the other hand, nephrin is central to podocyte maturation during glomerular development and development of the slit diaphragm junctional complex (Done et al., 2008; Li et al., 2015). Our results confirm that nicotine downregulates podocin and nephrin expression and mediates podocyte damage. However, pre-treatment with HMGB1 binder Gly prevents nicotine induced podocin and nephrin downregulation. Cytoskeletal protein desmin is central to intermediate filament formation and maintenance of structural and mechanical integrity of podocytes (Schell and Huber, 2017a; Nagata, 2016). Dysregulation of the podocyte cytoskeletal framework attributes to anomalies chiefly foot process retraction and proteinuria (Fuchshofer et al., 2011; Schell and Huber, 2017b). In fact, upregulation of desmin constitutes one of the key features of podocyte injury associated glomerular diseases (Zou et al., 2006). Our investigations show that nicotine upregulates desmin expression in mediating podocyte damage. However, prior Gly treatment attenuates nicotine induced desmin upsurge. To further assess the functional significance of HMGB1 in nicotine induced podocyte injury, we examined the impact of nicotine on podocyte permeability with and without Gly treatment. Our results showed that nicotine augments podocyte permeability to FITC-dextran through HMGB1 activation and this was prevented via prior treatment with HMGB1 inhibitor Gly. These findings postulate that nicotine induced structural and functional decadence in podocytes potentially occurs via HMGB1 activation.
TLR4, a member of pattern recognition receptor (PRR) family, is central to intrarenal inflammatory response initiation- chiefly characterized by increase in proinflammatory cytokine and chemokine expression, neutrophil and monocyte influx and urinary elimination of cytokines and chemokines (Ramesh and Reeves, 2002; Ramesh et al., 2007; Ramesh and Reeves, 2003; Majumder et al., 2024). The cytokine-like proinflammatory properties of HMGB1 have been established to be primarily TLR4 dependent (Kim et al., 2013). HMGB1, upon activation, interacts with TLR4/myeloid differentiation protein 2 (MD2) which engages coreceptor CD14 (Yang et al., 2015; He et al., 2018). This, in turn, promotes the release of monocyte chemoattractant protein (MCP)-1, IF-induced protein 10 (IP-10) and macrophage inflammatory protein 1α (MIP-1α) (Nano et al., 2013; Rabadi et al., 2012). Pharmacological inhibition of TLR4 and/or associated pro-inflammatory signalling has been vastly undertaken to better understand the signalling mechanisms driving kidney damage (Niu et al., 2019; González-Guerrero et al., 2017; Shi et al., 2017). Existing paradigm of studies establish a strong correlation between HMGB1 activation and TLR4 signalling. However, it remains unknown whether TLR4 signalling is involved in nicotine induced renal damage. Our study confirms that nicotine upregulates TLR4 levels and drives podocyte damage. However, prior treatment with HMGB1 inhibiting Gly attenuates nicotine induced TLR4 upregulation. These findings postulate that nicotine upregulates TLR4 levels in podocytes potentially via HMGB1 activation. To further validate our hypothesis, we advocated pharmacological inhibition of TLR4 using Resatorvid to investigate nicotine-induced podocyte damage from functional and structural perspectives. Resatorvid mediated TLR4 inhibition recovers podocin and nephrin downregulation induced by nicotine associated podocyte damage. TLR4 inhibition also attenuates nicotine associated upregulation of desmin expression and podocyte permeability. Together, these findings confirm that HMGB1 activation and extracellular release drives TLR4 signalling and mediates nicotine induced podocyte injury.
In conclusion, our results show that HMGB1 is an important mediator of nicotine induced podocyte damage potentially via TLR4 activation. The amelioration of podocyte injury by inhibition of HMGB1 during nicotine stimulation implicates the pivotal role of HMGB1 in smoking-induced podocyte injury.
Data availability statement
The raw data supporting the conclusions of this article will be made available by the authors, without undue reservation.
Author contributions
SD: Data curation, Formal Analysis, Investigation, Methodology, Writing–original draft. MR: Investigation, Methodology, Writing–review and editing. SK: Conceptualization, Funding acquisition, Resources, Supervision, Writing–review and editing. KB: Conceptualization, Funding acquisition, Project administration, Validation, Writing–review and editing.
Funding
The author(s) declare that financial support was received for the research, authorship, and/or publication of this article. This research was funded by the National Institute of Health grant number R01HL148711 and American Heart Association grant number 24IPA127635.
Conflict of interest
The authors declare that the research was conducted in the absence of any commercial or financial relationships that could be construed as a potential conflict of interest.
The author(s) declared that they were an editorial board member of Frontiers, at the time of submission. This had no impact on the peer review process and the final decision.
Generative AI statement
The author(s) declare that no Generative AI was used in the creation of this manuscript.
Publisher’s note
All claims expressed in this article are solely those of the authors and do not necessarily represent those of their affiliated organizations, or those of the publisher, the editors and the reviewers. Any product that may be evaluated in this article, or claim that may be made by its manufacturer, is not guaranteed or endorsed by the publisher.
References
Akkoyun, H., and Karadeniz, A. (2016). Investigation of the protective effect of ellagic acid for preventing kidney injury in rats exposed to nicotine during the fetal period. Biotech. and Histochem. 91 (2), 108–115. doi:10.3109/10520295.2015.1078910
Anders, H.-J., Huber, T. B., Isermann, B., and Schiffer, M. (2018). CKD in diabetes: diabetic kidney disease versus nondiabetic kidney disease. Nat. Rev. Nephrol. 14 (6), 361–377. doi:10.1038/s41581-018-0001-y
Andersen, K., Eltrich, N., Lichtnekert, J., Anders, H.-J., and Vielhauer, V. (2014). The NLRP3/ASC inflammasome promotes T-cell-dependent immune complex glomerulonephritis by canonical and noncanonical mechanisms. Kidney Int. 86 (5), 965–978. doi:10.1038/ki.2014.161
Andersson, U., Yang, H., and Harris, H. (2018). “High-mobility group box 1 protein (HMGB1) operates as an alarmin outside as well as inside cells,” Seminars in immunology (Elsevier).
Arany, I., Faisal, A., Clark, J. S., Vera, T., Baliga, R., and Nagamine, Y. (2010). p66SHC-mediated mitochondrial dysfunction in renal proximal tubule cells during oxidative injury. Am. J. Physiol.-Renal Physiol. 298 (5), F1214–F1221. doi:10.1152/ajprenal.00639.2009
Arany, I., Faisal, A., Nagamine, Y., and Safirstein, R. L. (2008). p66shc inhibits pro-survival epidermal growth factor receptor/ERK signaling during severe oxidative stress in mouse renal proximal tubule cells. J. Biol. Chem. 283 (10), 6110–6117. doi:10.1074/jbc.M708799200
Arany, I., Grifoni, S., Clark, J. S., Csongradi, E., Maric, C., and Juncos, L. A. (2011). Chronic nicotine exposure exacerbates acute renal ischemic injury. Am. J. Physiol.-Renal Physiol. 301 (1), F125–F133. doi:10.1152/ajprenal.00041.2011
Arany, I., Hall, S., Reed, D. K., Reed, C. T., and Dixit, M. (2016). Nicotine enhances high-fat diet-induced oxidative stress in the kidney. Nicotine and Tob. Res. 18 (7), 1628–1634. doi:10.1093/ntr/ntw029
Ataka, E., Matsukuma, Y., Ueki, K., Tsuchimoto, A., Okabe, Y., Masutani, K., et al. (2023). Cumulative smoking dose is associated with subclinical renal injury: a pathological study in individuals without chronic kidney disease. Nephrol. Dial. Transplant. 38 (12), 2799–2808. doi:10.1093/ndt/gfad124
Benowitz, N. L., and Burbank, A. D. (2016). Cardiovascular toxicity of nicotine: implications for electronic cigarette use. Trends Cardiovasc. Med. 26 (6), 515–523. doi:10.1016/j.tcm.2016.03.001
Boini, K. M., Hussain, T., Li, P.-L., and Koka, S. S. (2018). Trimethylamine-N-oxide instigates NLRP3 inflammasome activation and endothelial dysfunction. Cell. Physiology Biochem. 44 (1), 152–162. doi:10.1159/000484623
Boini, K. M., Zhang, C., Xia, M., Han, W.-Q., Brimson, C., Poklis, J. L., et al. (2010). Visfatin-induced lipid raft redox signaling platforms and dysfunction in glomerular endothelial cells. Biochim. Biophys. Acta. 1801 (12), 1294–1304. doi:10.1016/j.bbalip.2010.09.001
Borgerding, M., and Klus, H. (2005). Analysis of complex mixtures–cigarette smoke. Exp. Toxicol. Pathology 57, 43–73. doi:10.1016/j.etp.2005.05.010
Briganti, E. M., Branley, P., Chadban, S. J., Shaw, J. E., McNeil, J. J., Welborn, T. A., et al. (2002). Smoking is associated with renal impairment and proteinuria in the normal population: the AusDiab kidney study. Australian Diabetes, Obesity and Lifestyle Study. Am. J. Kidney Dis. 40 (4), 704–712. doi:10.1053/ajkd.2002.35677
Chase, H. P., Garg, S. K., Marshall, G., Berg, C. L., Harris, S., Jackson, W. E., et al. (1991). Cigarette smoking increases the risk of albuminuria among subjects with type I diabetes. Jama 265 (5), 614–617. doi:10.1001/jama.1991.03460050068022
Chen, X., Ma, J., Kwan, T., Stribos, E. G., Messchendorp, A. L., Loh, Y. W., et al. (2018). Blockade of HMGB1 attenuates diabetic nephropathy in mice. Sci. Rep. 8 (1), 8319. doi:10.1038/s41598-018-26637-5
Cryer, P. E., Haymond, M. W., Santiago, J. V., and Shah, S. D. (1976). Norepinephrine and epinephrine release and adrenergic mediation of smoking-associated hemodynamic and metabolic events. N. Engl. J. Med. 295 (11), 573–577. doi:10.1056/NEJM197609092951101
Datta, S., Pasham, S., Inavolu, S., Boini, K. M., and Koka, S. (2024b). Role of gut microbial metabolites in cardiovascular diseases-current insights and the road ahead. Int. J. Mol. Sci. 25 (18), 10208. doi:10.3390/ijms251810208
Datta, S., Rahman, M. A., Koka, S., and Boini, K. M. (2024a). High mobility group box 1 (HMGB1): molecular signaling and potential therapeutic strategies. Cells 13 (23), 1946. doi:10.3390/cells13231946
Done, S., Takemoto, M., He, L., Sun, Y., Hultenby, K., Betsholtz, C., et al. (2008). Nephrin is involved in podocyte maturation but not survival during glomerular development. Kidney Int. 73 (6), 697–704. doi:10.1038/sj.ki.5002707
Festoff, B. W., Sajja, R. K., van Dreden, P., and Cucullo, L. (2016). HMGB1 and thrombin mediate the blood-brain barrier dysfunction acting as biomarkers of neuroinflammation and progression to neurodegeneration in Alzheimer’s disease. J. neuroinflammation 13 (1), 194–212. doi:10.1186/s12974-016-0670-z
Franek, E., Benk, U., Reinbold, F., Fliser, D., Clorius, J., and Ritz, E. (1996). Acute influence of smoking on renal function. Nephrol. Dial. Transpl. 11, 65A.
Fuchshofer, R., Ullmann, S., Zeilbeck, L. F., Baumann, M., Junglas, B., and Tamm, E. R. (2011). Connective tissue growth factor modulates podocyte actin cytoskeleton and extracellular matrix synthesis and is induced in podocytes upon injury. Histochem. cell Biol. 136, 301–319. doi:10.1007/s00418-011-0844-9
Gambaro, G., Verlato, F., Budakovic, A., Casara, D., Saladini, G., Del Prete, D., et al. (1998). Renal impairment in chronic cigarette smokers. J. Am. Soc. Nephrol. 9 (4), 562–567. doi:10.1681/ASN.V94562
Gazzar, M. E., Yoza, B. K., Chen, X., Garcia, B. A., Young, N. L., and McCall, C. E. (2009). Chromatin-specific remodeling by HMGB1 and linker histone H1 silences proinflammatory genes during endotoxin tolerance. Mol. Cell. Biol. 29 (7), 1959–1971. doi:10.1128/MCB.01862-08
Genschel, J., and Modrich, P. (2009). Functions of MutLalpha, replication protein A (RPA), and HMGB1 in 5'-directed mismatch repair. J. Biol. Chem. 284 (32), 21536–21544. doi:10.1074/jbc.M109.021287
Gerstein, H. C., Mann, J. F., Pogue, J., Dinneen, S. J., Hallé, J. P., Hoogwerf, B., et al. (2000). Prevalence and determinants of microalbuminuria in high-risk diabetic and nondiabetic patients in the heart outcomes prevention evaluation study. The HOPE study investigators. Diabetes care 23, B35–B39.
González-Guerrero, C., Cannata-Ortiz, P., Guerri, C., Egido, J., Ortiz, A., and Ramos, A. M. (2017). TLR4-mediated inflammation is a key pathogenic event leading to kidney damage and fibrosis in cyclosporine nephrotoxicity. Archives Toxicol. 91, 1925–1939. doi:10.1007/s00204-016-1830-8
Good, D. W., George, T., and Watts, I. I. I. B. A. (2015). High-mobility group box 1 inhibits HCO3− absorption in medullary thick ascending limb through a basolateral receptor for advanced glycation end products pathway. Am. J. Physiol.-Renal Physiol. 309 (8), F720–F730. doi:10.1152/ajprenal.00227.2015
Gündoğdu, Y., and Anaforoğlu, İ. (2022). Effects of smoking on diabetic nephropathy. Front. Clin. Diabetes Healthc. 3, 826383. doi:10.3389/fcdhc.2022.826383
Hallan, S. I., and Orth, S. R. (2011). Smoking is a risk factor in the progression to kidney failure. Kidney Int. 80 (5), 516–523. doi:10.1038/ki.2011.157
Harwani, S. C., Ratcliff, J., Sutterwala, F. S., Ballas, Z. K., Meyerholz, D. K., Chapleau, M. W., et al. (2016). Nicotine mediates CD161a+ renal macrophage infiltration and premature hypertension in the spontaneously hypertensive rat. Circulation Res. 119 (10), 1101–1115. doi:10.1161/CIRCRESAHA.116.309402
He, M., Bianchi, M. E., Coleman, T. R., Tracey, K. J., and Al-Abed, Y. (2018). Exploring the biological functional mechanism of the HMGB1/TLR4/MD-2 complex by surface plasmon resonance. Mol. Med. 24, 21–29. doi:10.1186/s10020-018-0023-8
Hua, P., Feng, W., Ji, S., Raij, L., and Jaimes, E. A. (2010). Nicotine worsens the severity of nephropathy in diabetic mice: implications for the progression of kidney disease in smokers. Am. J. Physiol.-Renal Physiol. 299 (4), F732–F739. doi:10.1152/ajprenal.00293.2010
Huang, Y.-Y., Su, W., Zhu, Z.-W., Tang, L., Hu, X.-Q., Zhou, S.-H., et al. (2016). Elevated serum HMGB1 in pulmonary arterial hypertension secondary to congenital heart disease. Vasc. Pharmacol. 85, 66–72. doi:10.1016/j.vph.2016.08.009
Huber, T. B., Simons, M., Hartleben, B., Sernetz, L., Schmidts, M., Gundlach, E., et al. (2003). Molecular basis of the functional podocin–nephrin complex: mutations in the NPHS2 gene disrupt nephrin targeting to lipid raft microdomains. Hum. Mol. Genet. 12 (24), 3397–3405. doi:10.1093/hmg/ddg360
Ito, K., Maeda, T., Tada, K., Takahashi, K., Yasuno, T., Masutani, K., et al. (2020). The role of cigarette smoking on new-onset of chronic kidney disease in a Japanese population without prior chronic kidney disease: iki epidemiological study of atherosclerosis and chronic kidney disease (ISSA-CKD). Clin. Exp. Nephrol. 24, 919–926. doi:10.1007/s10157-020-01914-8
Ito, T., Kawahara, K., Nakamura, T., Yamada, S., Abeyama, K., Hashiguchi, T., et al. (2007). High-mobility group box 1 protein promotes development of microvascular thrombosis in rats. J. Thromb. Haemost. 5 (1), 109–116. doi:10.1111/j.1538-7836.2006.02255.x
Jacobs, E. J., Newton, C. C., Carter, B. D., Feskanich, D., Freedman, N. D., Prentice, R. L., et al. (2015). What proportion of cancer deaths in the contemporary United States is attributable to cigarette smoking? Ann. Epidemiol. 25 (3), 179–182. doi:10.1016/j.annepidem.2014.11.008
Jaimes, E. A., Tian, R.-X., Joshi, M. S., and Raij, L. (2009). Nicotine augments glomerular injury in a rat model of acute nephritis. Am. J. Nephrol. 29 (4), 319–326. doi:10.1159/000163593
Jaimes, E. A., Zhou, M.-S., Siddiqui, M., Rezonzew, G., Tian, R., Seshan, S. V., et al. (2021). Nicotine, smoking, podocytes, and diabetic nephropathy. Am. J. Physiol.-Renal Physiol. 320 (3), F442–F453. doi:10.1152/ajprenal.00194.2020
Jha, J. C., Ho, F., Dan, C., and Jandeleit-Dahm, K. (2018). A causal link between oxidative stress and inflammation in cardiovascular and renal complications of diabetes. Clin. Sci. 132 (16), 1811–1836. doi:10.1042/CS20171459
Kashani, B., Zandi, Z., Bashash, D., Zaghal, A., Momeny, M., Poursani, E. M., et al. (2020). Small molecule inhibitor of TLR4 inhibits ovarian cancer cell proliferation: new insight into the anticancer effect of TAK-242 (Resatorvid). Cancer Chemother. Pharmacol. 85, 47–59. doi:10.1007/s00280-019-03988-y
Kashani, B., Zandi, Z., Karimzadeh, M. R., Bashash, D., Nasrollahzadeh, A., and Ghaffari, S. H. (2019). Blockade of TLR4 using TAK-242 (resatorvid) enhances anti-cancer effects of chemotherapeutic agents: a novel synergistic approach for breast and ovarian cancers. Immunol. Res. 67, 505–516. doi:10.1007/s12026-019-09113-8
Kim, S., Kim, S. Y., Pribis, J. P., Lotze, M., Mollen, K. P., Shapiro, R., et al. (2013). Signaling of high mobility group box 1 (HMGB1) through toll-like receptor 4 in macrophages requires CD14. Mol. Med. 19, 88–98. doi:10.2119/molmed.2012.00306
Koka, S., Xia, M., Zhang, C., Zhang, Y., Li, P. L., and Boini, K. M. (2019). Podocyte NLRP3 inflammasome activation and formation by adipokine visfatin. Cell Physiol. Biochem. 53 (2), 355–365. doi:10.33594/000000143
Li, X., Chuang, P. Y., D’Agati, V. D., Dai, Y., Yacoub, R., Fu, J., et al. (2015). Nephrin preserves podocyte viability and glomerular structure and function in adult kidneys. J. Am. Soc. Nephrol. 26 (10), 2361–2377. doi:10.1681/ASN.2014040405
Liao, D., Ma, L., Liu, J., and Fu, P. (2019). Cigarette smoking as a risk factor for diabetic nephropathy: a systematic review and meta-analysis of prospective cohort studies. PLoS One 14 (2), e0210213. doi:10.1371/journal.pone.0210213
Lv, G., Yang, M., Gai, K., Jia, Q., Wang, Z., Wang, B., et al. (2024). Multiple functions of HMGB1 in cancer. Front. Oncol. 14, 1384109. doi:10.3389/fonc.2024.1384109
Majumder, S., Pushpakumar, S. B., Almarshood, H., Ouseph, R., Gondim, D. D., Jala, V. R., et al. (2024). Toll-like receptor 4 mutation mitigates gut microbiota-mediated hypertensive kidney injury. Pharmacol. Res. 206, 107303. doi:10.1016/j.phrs.2024.107303
Matsunaga, N., Tsuchimori, N., Matsumoto, T., and Ii, M. (2011). TAK-242 (resatorvid), a small-molecule inhibitor of toll-like receptor (TLR) 4 signaling, binds selectively to TLR4 and interferes with interactions between TLR4 and its adaptor molecules. Mol. Pharmacol. 79 (1), 34–41. doi:10.1124/mol.110.068064
Mayyas, F., and Alzoubi, K. H. (2019). Impact of cigarette smoking on kidney inflammation and fibrosis in diabetic rats. Inhal. Toxicol. 31 (2), 45–51. doi:10.1080/08958378.2019.1597219
McDermott, G., Fu, X., Stone, J. H., Wallwork, R., Zhang, Y., Choi, H. K., et al. (2020). Association of cigarette smoking with antineutrophil cytoplasmic antibody–associated vasculitis. JAMA Intern. Med. 180 (6), 870–876. doi:10.1001/jamainternmed.2020.0675
Mishra, A., Chaturvedi, P., Datta, S., Sinukumar, S., Joshi, P., and Garg, A. (2015). Harmful effects of nicotine. Indian J. Med. Paediatr. Oncol. 36 (01), 24–31. doi:10.4103/0971-5851.151771
Moldoveanu, S. C., and Charles, F. S. (2007). Differences in the chemical composition of the particulate phase of inhaled and exhaled cigarette mainstream smoke. Contributions Tob. and Nicotine Res. 22 (4), 290–302.
Mollica, L., De Marchis, F., Spitaleri, A., Dallacosta, C., Pennacchini, D., Zamai, M., et al. (2007). Glycyrrhizin binds to high-mobility group box 1 protein and inhibits its cytokine activities. Chem. and Biol. 14 (4), 431–441. doi:10.1016/j.chembiol.2007.03.007
Mühlhauser, I. (1994). Cigarette smoking and diabetes: an update. Diabet. Med. 11 (4), 336–343. doi:10.1111/j.1464-5491.1994.tb00283.x
Nagata, M. (2016). Podocyte injury and its consequences. Kidney Int. 89 (6), 1221–1230. doi:10.1016/j.kint.2016.01.012
Nano, R., Racanicchi, L., Melzi, R., Mercalli, A., Maffi, P., Sordi, V., et al. (2013). Human pancreatic islet preparations release HMGB1:(ir) relevance for graft engraftment. Cell Transplant. 22 (11), 2175–2186. doi:10.3727/096368912X657783
Narumi, T., Shishido, T., Otaki, Y., Kadowaki, S., Honda, Y., Funayama, A., et al. (2015). High-mobility group box 1-mediated heat shock protein beta 1 expression attenuates mitochondrial dysfunction and apoptosis. J. Mol. Cell. Cardiol. 82, 1–12. doi:10.1016/j.yjmcc.2015.02.018
Niu, X., Yao, Q., Li, W., Zang, L., Li, W., Zhao, J., et al. (2019). Harmine mitigates LPS-induced acute kidney injury through inhibition of the TLR4-NF-κB/NLRP3 inflammasome signalling pathway in mice. Eur. J. Pharmacol. 849, 160–169. doi:10.1016/j.ejphar.2019.01.062
Organization, W. H. (2024) Tobacco. Available at: https://www.who.int/news-room/fact-sheets/detail/tobacco.
Osborne, J. S., Adamek, S., and Hobbs, M. E. (1956). Some components of gas phase of cigarette smoke. Anal. Chem. 28 (2), 211–215. doi:10.1021/ac60110a020
Ozkok, A., Akpinar, T. S., Tufan, F., Kanitez, N. A., Uysal, M., Guzel, M., et al. (2013). Clinical characteristics and predictors of progression of chronic kidney disease in autosomal dominant polycystic kidney disease: a single center experience. Clin. Exp. Nephrol. 17, 345–351. doi:10.1007/s10157-012-0706-3
Palumbo, R., Sampaolesi, M., De Marchis, F., Tonlorenzi, R., Colombetti, S., Mondino, A., et al. (2004). Extracellular HMGB1, a signal of tissue damage, induces mesoangioblast migration and proliferation. J. cell Biol. 164 (3), 441–449. doi:10.1083/jcb.200304135
Pang, X., and Lewis, A. C. (2011). Carbonyl compounds in gas and particle phases of mainstream cigarette smoke. Sci. Total Environ. 409 (23), 5000–5009. doi:10.1016/j.scitotenv.2011.07.065
Passarelli, M. N., Newcomb, P. A., Hampton, J. M., Trentham-Dietz, A., Titus, L. J., Egan, K. M., et al. (2016). Cigarette smoking before and after breast cancer diagnosis: mortality from breast cancer and smoking-related diseases. J. Clin. Oncol. 34 (12), 1315–1322. doi:10.1200/JCO.2015.63.9328
Perico, L., Conti, S., Benigni, A., and Remuzzi, G. (2016). Podocyte–actin dynamics in health and disease. Nat. Rev. Nephrol. 12 (11), 692–710. doi:10.1038/nrneph.2016.127
Pesce, F., Stea, E. D., Rossini, M., Fiorentino, M., Piancone, F., Infante, B., et al. (2021). Glomerulonephritis in AKI: from pathogenesis to therapeutic intervention. Front. Med. 7, 582272. doi:10.3389/fmed.2020.582272
Poston, J. T., and Koyner, J. L. (2019). Sepsis associated acute kidney injury. Bmj 364, k4891. doi:10.1136/bmj.k4891
Rabadi, M. M., Ghaly, T., Goligorksy, M. S., and Ratliff, B. B. (2012). HMGB1 in renal ischemic injury. Am. J. Physiology-Renal Physiology 303 (6), F873–F885. doi:10.1152/ajprenal.00092.2012
Ramalingam, A., Santhanathas, T., Shaukat Ali, S., and Zainalabidin, S. (2019). Resveratrol supplementation protects against nicotine-induced kidney injury. Int. J. Environ. Res. public health 16 (22), 4445. doi:10.3390/ijerph16224445
Ramesh, G., and Reeves, W. B. (2002). TNF-alpha mediates chemokine and cytokine expression and renal injury in cisplatin nephrotoxicity. J. Clin. investigation 110 (6), 835–842. doi:10.1172/JCI15606
Ramesh, G., and Reeves, W. B. (2003). TNFR2-mediated apoptosis and necrosis in cisplatin-induced acute renal failure. Am. J. Physiology-Renal Physiology 285 (4), F610–F618. doi:10.1152/ajprenal.00101.2003
Ramesh, G., Zhang, B., Uematsu, S., Akira, S., and Reeves, W. B. (2007). Endotoxin and cisplatin synergistically induce renal dysfunction and cytokine production in mice. Am. J. Physiol.-Renal Physiol. 293 (1), F325–F332. doi:10.1152/ajprenal.00158.2007
Ross, R. (1993). The pathogenesis of atherosclerosis: a perspective for the 1990s. Nature 362 (6423), 801–809. doi:10.1038/362801a0
Rossing, P., Hougaard, P., and Parving, H.-H. (2002). Risk factors for development of incipient and overt diabetic nephropathy in type 1 diabetic patients: a 10-year prospective observational study. Diabetes care 25 (5), 859–864. doi:10.2337/diacare.25.5.859
Saleem, M. A., Ni, L., Witherden, I., Tryggvason, K., Ruotsalainen, V., Mundel, P., et al. (2002). Co-localization of nephrin, podocin, and the actin cytoskeleton: evidence for a role in podocyte foot process formation. Am. J. Pathology 161 (4), 1459–1466. doi:10.1016/S0002-9440(10)64421-5
Salonen, J. T., and Salonen, R. (1993). Ultrasound B-mode imaging in observational studies of atherosclerotic progression. Circulation 87 (3 Suppl. l), II56–65.
Schell, C., and Huber, T. B. (2017a). The evolving complexity of the podocyte cytoskeleton. J. Am. Soc. Nephrol. 28 (11), 3166–3174. doi:10.1681/ASN.2017020143
Schell, C., and Huber, T. B. (2017b). The evolving complexity of the podocyte cytoskeleton. J. Am. Soc. Nephrol. JASN 28 (11), 3166–3174. doi:10.1681/ASN.2017020143
Schwarz, K., Simons, M., Reiser, J., Saleem, M. A., Faul, C., Kriz, W., et al. (2001). Podocin, a raft-associated component of the glomerular slit diaphragm, interacts with CD2AP and nephrin. J. Clin. investigation 108 (11), 1621–1629. doi:10.1172/JCI12849
Schweitzer, K. S., Chen, S. X., Law, S., Van Demark, M., Poirier, C., Justice, M. J., et al. (2015). Endothelial disruptive proinflammatory effects of nicotine and e-cigarette vapor exposures. Am. J. Physiol.-Lung Cell. Mol. Physiol. 309 (2), L175–L187. doi:10.1152/ajplung.00411.2014
Shen, P., Zhang, L., Jiang, X., Yu, B., and Zhang, J. (2024). Targeting HMGB1 and its interaction with receptors: challenges and future directions. J. Med. Chem. doi:10.1021/acs.jmedchem.4c01912
Shi, M., Zeng, X., Guo, F., Huang, R., Feng, Y., Ma, L., et al. (2017). Anti-inflammatory pyranochalcone derivative attenuates LPS-induced acute kidney injury via inhibiting TLR4/NF-κB pathway. Molecules 22 (10), 1683. doi:10.3390/molecules22101683
Singh, G. B., Kshirasagar, N., Patibandla, S., Puchchakayala, G., Koka, S., and Boini, K. M. (2019a). Nicotine instigates podocyte injury via NLRP3 inflammasomes activation. Aging (Albany NY) 11 (24), 12810–12821. doi:10.18632/aging.102611
Singh, G. B., Zhang, Y., Boini, K. M., and Koka, S. (2019b). High mobility group box 1 mediates TMAO-induced endothelial dysfunction. Int. J. Mol. Sci. 20 (14), 3570. doi:10.3390/ijms20143570
Smith, C. J., and Fischer, T. H. (2001). Particulate and vapor phase constituents of cigarette mainstream smoke and risk of myocardial infarction. Atherosclerosis 158 (2), 257–267. doi:10.1016/s0021-9150(01)00570-6
Štros, M. (2010). HMGB proteins: interactions with DNA and chromatin. Biochimica Biophysica Acta (BBA)-Gene Regul. Mech. 1799 (1-2), 101–113. doi:10.1016/j.bbagrm.2009.09.008
Tachibana, S., Iyoda, M., Matsumoto, K., Wada, Y., Suzuki, T., Iseri, K., et al. (2019). Recombinant human soluble thrombomodulin attenuates anti-glomerular basement membrane glomerulonephritis in Wistar–Kyoto rats through anti-inflammatory effects. Nephrol. Dial. Transplant. 34 (5), 774–782. doi:10.1093/ndt/gfy201
Tang, D., Kang, R., Cheh, C.-W., Livesey, K. M., Liang, X., Schapiro, N. E., et al. (2010b). HMGB1 release and redox regulates autophagy and apoptosis in cancer cells. Oncogene 29 (38), 5299–5310. doi:10.1038/onc.2010.261
Tang, D., Kang, R., Livesey, K. M., Cheh, C.-W., Farkas, A., Loughran, P., et al. (2010a). Endogenous HMGB1 regulates autophagy. J. Cell Biol. 190 (5), 881–892. doi:10.1083/jcb.200911078
Thielen, A., Klus, H., and Müller, L. (2008). Tobacco smoke: unraveling a controversial subject. Exp. Toxicol. Pathol. 60 (2-3), 141–156. doi:10.1016/j.etp.2008.01.014
United States. Dept. of Health and Human Services., United States. Public Health Service. Office of the Surgeon General., United States. Office on Smoking and Health (1983). The health consequences of smoking: cardiovascular disease a report of the surgeon general. Rockville (MD): US Department of Health and Human Services. Public Health Service, Office on Smoking and Health.
Wang, H., Yang, H., and Tracey, K. (2004). Extracellular role of HMGB1 in inflammation and sepsis. J. Intern. Med. 255 (3), 320–331. doi:10.1111/j.1365-2796.2003.01302.x
Wang, S., Qin, A., Pei, G., Jiang, Z., Dong, L., Tan, J., et al. (2021). Cigarette smoking may accelerate the progression of IgA nephropathy. BMC Nephrol. 22 (1), 239–248. doi:10.1186/s12882-021-02453-4
Wang, Y., Zhang, H., Chen, Q., Jiao, F., Shi, C., Pei, M., et al. (2020). TNF-α/HMGB1 inflammation signalling pathway regulates pyroptosis during liver failure and acute kidney injury. Cell Prolif. 53 (6), e12829. doi:10.1111/cpr.12829
West, R. (2017). Tobacco smoking: health impact, prevalence, correlates and interventions. Psychol. and health 32 (8), 1018–1036. doi:10.1080/08870446.2017.1325890
Wu, H., Ma, J., Wang, P., Corpuz, T. M., Panchapakesan, U., Wyburn, K. R., et al. (2010). HMGB1 contributes to kidney ischemia reperfusion injury. J. Am. Soc. Nephrol. JASN 21 (11), 1878–1890. doi:10.1681/ASN.2009101048
Wu, X., Zhang, H., Qi, W., Zhang, Y., Li, J., Li, Z., et al. (2018). Nicotine promotes atherosclerosis via ROS-NLRP3-mediated endothelial cell pyroptosis. Cell death and Dis. 9 (2), 171. doi:10.1038/s41419-017-0257-3
Wu, Z., Liu, Q., Zhu, K., Liu, Y., Chen, L., Guo, H., et al. (2020). Cigarette smoke induces the pyroptosis of urothelial cells through ROS/NLRP3/caspase-1 signaling pathway. Neurourol. Urodyn. 39 (2), 613–624. doi:10.1002/nau.24271
Yang, H., Wang, H., Ju, Z., Ragab, A. A., Lundbäck, P., Long, W., et al. (2015). MD-2 is required for disulfide HMGB1–dependent TLR4 signaling. J. Exp. Med. 212 (1), 5–14. doi:10.1084/jem.20141318
Yu, S.-H., and Spring, T. G. (1977). The interaction of nonhistone chromosomal proteins HMG1 and HMG2 with subfractions of H1 histone immobilized on agarose. Biochimi. Biophys. Acta. 492 (1), 20–28. doi:10.1016/0005-2795(77)90210-0
Zhang, Y.-G., Zhu, X., Lu, R., Messer, J. S., Xia, Y., Chang, E. B., et al. (2019). Intestinal epithelial HMGB1 inhibits bacterial infection via STAT3 regulation of autophagy. Autophagy 15 (11), 1935–1953. doi:10.1080/15548627.2019.1596485
Zhao, Z., Hu, Z., Zeng, R., and Yao, Y. (2020). HMGB1 in kidney diseases. Life Sci. 259, 118203. doi:10.1016/j.lfs.2020.118203
Zheng, C.-M., Lee, Y.-H., Chiu, I.-J., Chiu, Y.-J., Sung, L.-C., Hsu, Y.-H., et al. (2020). Nicotine causes nephrotoxicity through the induction of nlrp6 inflammasome and alpha7 nicotinic acetylcholine receptor. Toxics 8 (4), 92. doi:10.3390/toxics8040092
Keywords: podocytes, HMGB1, nicotine, TLR4, smoking
Citation: Datta S, Rahman MA, Koka S and Boini KM (2025) High mobility group box 1 (HMGB1) mediates nicotine-induced podocyte injury. Front. Pharmacol. 15:1540639. doi: 10.3389/fphar.2024.1540639
Received: 06 December 2024; Accepted: 18 December 2024;
Published: 07 January 2025.
Edited by:
Utpal Sen, University of Louisville, United StatesReviewed by:
Sivareddy Challa, University of Illinois at Peoria, United StatesKameswara Badri, Morehouse School of Medicine, United States
Copyright © 2025 Datta, Rahman, Koka and Boini. This is an open-access article distributed under the terms of the Creative Commons Attribution License (CC BY). The use, distribution or reproduction in other forums is permitted, provided the original author(s) and the copyright owner(s) are credited and that the original publication in this journal is cited, in accordance with accepted academic practice. No use, distribution or reproduction is permitted which does not comply with these terms.
*Correspondence: Krishna M. Boini, a21ib2luaUB1aC5lZHU=