- 1Department of Cardiology, China-Japan Union Hospital of Jilin University, Jilin Provincial Cardiovascular Research Institute, Changchun, China
- 2Department of Critical Care Medicine, Beijing Anzhen Hospital, Capital Medical University, Beijing, China
- 3Norman Bethune Health Science Center of Jilin University, Changchun, China
- 4The Second Affiliated Hospital of Xi’an Jiaotong University, Xi’an, China
- 5National Key Discipline in Hematology of China, Department of Hematology, The First Hospital of Jilin University, Changchun, China
- 6The Hull Family Cardiac Fibrillation Management Laboratory, Toronto General Hospital, Toronto, ON, Canada
The Bruton’s Tyrosine Kinase Inhibitor, ibrutinib, has been widely employed due to its significant efficacy in B-cell lymphoma. However, the subsequent cardiac complications, notably atrial fibrillation (AF) and ventricular arrhythmias (VAs),associated with ibrutinib treatment have emerged as a major concern in cardio-oncology and hematology. Ibrutinib-induced AF has been well described, whereas mechanisms of ibrutinib-induced VAs are still under-investigation. The incidence of ibrutinib-induced VAs can vary vastly due to under-recognition and limitations of the retrospective studies. Recent investigations, including our previous work, have proposed several potential mechanisms contributing to this adverse event, necessitating further validation. The development of effective strategies for the prevention and treatment of ibrutinib-induced VAs still requires in-depth exploration. This review aims to establish a comprehensive framework encompassing the epidemiology, mechanistic insights, and clinical considerations related to ibrutinib-induced VAs. This article outlines potential strategies for the clinical management of patients undergoing ibrutinib therapy based on suggested mechanisms.
1 Introduction
Chronic activation of the B-cell receptor signaling pathway and its downstream overexpression as well as activation of Bruton’s Tyrosine Kinase (BTK) characterizes the pathogenesis of various B-cell non-Hodgkin’s lymphomas. Ibrutinib, the first covalent kinase inhibitor targeting BTK and downstream signaling cascades, has demonstrated remarkable clinical efficacy in extending the survival and enhancing the quality of life for affected patients (Byrd et al., 2013; Woyach et al., 2018; Wang et al., 2013; Treon et al., 2021). Due to its ability to cause significant improvement in disease prognosis, it was approved as a “breakthrough therapy” for the treatment of chronic lymphocytic leukemia (CLL), chronic myeloid leukemia, marginal zone lymphoma, and waldenstrom macroglobulinemia (Lee et al., 2016; Dreyling et al., 2016; Shanafelt et al., 2019; Treon et al., 2018). However, in addition to the typical adverse reactions neutropenia and bleeding, accumulating evidences suggest ibrutinib treatment can lead to cardiac arrhythmias (Burger et al., 2015; Lampson et al., 2017; Tuomi et al., 2018; Castillo et al., 2024; Gambril et al., 2024; Moslehi et al., 2024; Sherazi et al., 2023). This review aims to comprehensively address the epidemiology, pathogenesis, and clinical characteristics of ibrutinib-induced ventricular arrhythmias (VAs), drawing upon the latest findings in the literature. Furthermore, it seeks to present innovative strategies for clinical management founded upon an understanding of the underlying mechanisms.
2 Epidemiology of ibrutinib-induced VAs
Ibrutinib is the first BTK Inhibitor (BTKi) approved for clinical use and is effective against various B-cell malignancies. Currently, it is being widely used as a first-line treatment for managing CLL, chronic myeloid leukemia, marginal zone lymphoma, and waldenstrom macroglobulinemia (Brown, 2018; Aw and Brown, 2017; Burger et al., 2014; Advani et al., 2013). In several large-scale randomized controlled clinical trials, patients treated with ibrutinib exhibited significant improvements in progression-free survival, overall response rate, and overall survival compared to those in the control group (Byrd et al., 2013; Burger et al., 2015).
Nevertheless, it has been documented that ibrutinib can cause various arrhythmias, including supraventricular tachycardia, atrial fibrillation (AF), and VAs, among which AF was identified as the most prevalent manifestation. Ibrutinib-induced arrhythmias have limited its application in clinical utility (Wang et al., 2015; Herman et al., 2011; Li et al., 2023). It is essential to note that while ibrutinib-induced VAs is infrequent, they represent critical and potentially life-threatening side effects associated with ibrutinib therapy (Lampson et al., 2017; Tomcsányi et al., 2016).
2.1 Increased risk and incidence
Ibrutinib-induced AF was first found in the Phase III RESONATE study back in 2014 (Byrd et al., 2014). The emergence of ibrutinib-related polymorphic ventricular tachycardia (VT) and ventricular fibrillation (VF) was initially documented in a patient undergoing ibrutinib treatment in 2016 (Wallace et al., 2016). Subsequently, a spectrum of VAs, encompassing short-run VT, VF, electrical storm, and torsade de points (TdPs), have been reported in association with ibrutinib administratio n in ten documented cases (Table 1) (Tomcsányi et al., 2016; Beyer et al., 2017). A series of retrospective studies of the databases on adverse reactions have been conducted for concerns related to the safety of ibrutinib (Table 2).
Lampson et al. (2017) conducted an analysis of the Food and Drug Administration (FDA) Adverse Event Reporting System (FAERS) data ranging from the 4th quarter of 2013 to the 4th quarter of 2015, they identified 13 patients who developed various VAs after ibrutinib treatment. The calculated weighted incidence rate of ibrutinib-induced VAs was 788 people/100,000 person-years, significantly higher (2.0 to 3.9-fold) than the age-matched incidence in the general population (200–400/100,000 person-years) (Lampson et al., 2017; Becker et al., 1993; Chugh et al., 2004; Rothwell et al., 2005). Additionally, in a retrospective study by Guha et al. (2018), 582 patients who were administered ibrutinib, 11 patients developed symptomatic VAs presumably related to ibrutinib therapy. Notably, six patients without pre-existing cardiac disease developed VAs, resulting in a calculated weighted incidence of 596 per 100,000 person-years, which was significantly higher (expected Relative Risk 12.4) than the average population (48.1 per 100,000 person-years) (Sirichand et al., 2017). In 2018, an updated query of FAERS (Data from November 2013- November 2017) by Cheng et al. (2018) identified 33 cases of VAs (based on the WHO/UMC causality assessment scale) possibly associated with ibrutinib treatment. The causal relationship between ibrutinib and VAs was determined as probable in 8 patients without established confounding factors, such as electrolyte imbalance or other QT-prolongation medication. Although the rest of the 25 patients were identified as possible casualties, it is noteworthy that a positive dechallenge concerning ibrutinib discontinuation or positive rechallenge regarding ibrutinib reinitiating indicated that ibrutinib was directly associated with VAs. An extensive analysis of the Vigibase, the international pharmacovigilance database conducted by Salem et al. (2019), delved into the cardiotoxic effects of ibrutinib. This investigation identified 70 patients, comprising 31 cases of VT and 20 instances of VF, where ibrutinib was linked to the induction of VAs. Ibrutinib was found to be closely associated with a calculated higher incidence of VAs (reported odds ratio [ROR]: 4.7; 95% CI: 3.7–5.9; p < 0.0001). A recent study led by Zheng et al. (2023) conducted a comprehensive analysis of the FAERS database covering 2014 to 2021. This investigation shed light on the frequent occurrence of cardiotoxic events, specifically VT and VF, associated with using ibrutinib. The analysis yielded a substantial information component value of 1.18 and a noteworthy ROR of 2.27 for VT. Within the spectrum of cardiovascular toxicities, torsade de pointes/QT prolongation (TdP/QTp) represented the category with the highest fatalities. The information component and ROR values for TdP/QTp were 0.17 and 1.12, respectively.
Early evidence from the literature provides us with a preliminary estimation of the incidence of this critical arrhythmia. Insights gleaned from prescription data of ibrutinib and results from a small randomized controlled trial with IMBRUVICA provide informative. In this trial, spanning a median follow-up of 19.5 months, the incidence of VAs, including ventricular premature beats, VT, ventricular flutter, and VF, among all Common Terminology Criteria for Adverse Events (CTCAE) grades, was 1.0% in the ibrutinib group (n = 1,157) and 0.4% in the control group (n = 958). The incidence rates for CTCAE grade 3 and above were 0.3% and 0%, respectively (IMBRUVICA, 2022). In a phase Ⅲ clinical trial conducted by Woyach et al. (2018), unexpected mortality was observed in 1.1% of patients in the chemoimmunotherapy group and 3% of those treated with ibrutinib. This discrepancy in mortality rates could potentially be attributed to life-threatening VF.
Interestingly, in contrast to the relatively low reported incidence ranging from 0.3% to 3%, a recent retrospective study employing ambulatory electrocardiogram (ECG) monitoring during ibrutinib treatment revealed a notably higher incidence of VAs among treated patients (Fazal et al., 2021). This retrospective study found that 43% of the 72 patients monitored had experienced non-sustained VT during ibrutinib treatment, with most cases asymptomatic. Notably, the study also highlighted the possibility of significant underestimation of asymptomatic non-sustained VT occurrences due to the absence of continuous monitoring. However, it is essential to acknowledge that this study had limitations, including a lack of information regarding patients’ arrhythmia history before ibrutinib treatment. The presence of an extraordinary higher pre-existing AF history (incidence 13%) might have contributed to an overestimation of VAs in this small-scale study.
These studies have consistently reaffirmed a significant increase in the risk of VAs following exposure to ibrutinib. However, it is crucial to note that these studies did not provide a comprehensive depiction of the incidences of ibrutinib-induced VAs, as they did not encompass the entire population exposed to the drug. Due to the under-recognition of this side effect and limitations inherent in reporting systems, the perceived risk was initially assumed to be relatively low. Subsequently, with the establishment of cohorts based on real-world data and clinical trial findings, Health Canada took action by posting information on 26 July 2018, regarding the results of an assessment of the risk of severe and life-threatening arrhythmias, particularly ventricular tachycardia, induced by ibrutinib. This information was disseminated to medical professionals and patients via InfoWatch (Canada, 2018). In May 2022, the FDA revised the instructions and guidance for utilizing ibrutinib. Notably, the updated guidance explicitly underscores the occurrence of severe and fatal cardiac arrhythmias as a prominent and significant adverse outcome associated with this medication. This heightened emphasis reflects the growing awareness within the medical community of the cardiac safety concerns linked to ibrutinib use (Canada, 2018).
2.2 Age, pre-existing cardiac disease, and time to event
Lampson’s study examined 13 cases of ibrutinib-induced VAs, reporting a median time from the start of ibrutinib treatment to the occurrence of VAs as 65 days, with a range spanning from 6 to 698 days (Lampson et al., 2017). The median age of patients in this study was 61 years, ranging from 49 to 85 years. Importantly, comprehensive cardiac evaluations revealed no pre-existing cardiac causes for VAs in these cases.
Similarly, Cheng et al. (2018) conducted a study involving 33 patients, identifying a median onset time of 115 days for VAs, with a range from 9 to 791 days. Notably, nine patients developed VAs within the first month of initiating ibrutinib therapy. Coronary angiography and cardiac function assessments for these patients showed no severe ischemic heart disease, and eight of these patients maintained normal left ventricular ejection fractions.
In addition, Guha et al. (2018) reported a median time to VA onset of 16 months, with a range from 0.7 to 57.6 months, in their study. Over a 32-month follow-up period, symptomatic VAs were typically observed within 11 months. Notably, seven patients showed a presumed association with ibrutinib, and six of these patients had no prior history of coronary artery disease or heart failure with reduced ejection fraction.
Synthesizing these findings, it appears that ibrutinib-induced VAs are more likely to occur in older patients, particularly those over 60. However, the timing of VA onset varies considerably, ranging from early occurrence (within 65 days) to much later detection (up to 16 months in long-term follow-up). Additionally, a significant portion of patients experiencing VAs had no prior cardiovascular disease (CVD), emphasizing the multifactorial and complex nature of these events. Despite these findings, the potential influence of factors such as region, race, or other demographic variables on VA risk remains unclear due to the limited diversity in the current body of research.
2.3 Dose
In C57BL/6 mice, it has been demonstrated that a single high dose of ibrutinib is more prone to induce arrhythmias than a low dose. However, no dose association has been observed in cases of chronic administration. Instead, the incidence of arrhythmias may be more related to plasma concentration (Tuomi et al., 2018). Moreover, in clinical data, no significant correlation has been identified between the incidence of arrhythmias and the administered dose of ibrutinib.
Cheng et al. (2018) reported the contributory role of ibrutinib dose on VAs in 33 patients. They observed that whether patients were treated with the FDA-recommended doses of 420 mg/day, 560 mg/day, or even a reduced dose of 280 mg/day, there was no clear correlation between the dose administered and the occurrence of VAs. This assessment considered hepatic metabolism parameters, and no evidence of hepatic dysfunction or cytochrome P450 3A inhibition was found, as indicated in the primary drug safety evaluation report (IMBRUVICA, 2022). Similarly, an analysis of VigiBase data by Salem et al. (2021) revealed that the administration of 420 mg/day or 560 mg/day doses accounted for a significant portion of total ibrutinib-induced VAs, with 71.4% and 17.8%, respectively. This observation further suggests a loose association between different doses of ibrutinib and the occurrence of ibrutinib-induced VAs. These findings underscore the complex nature of ibrutinib-associated arrhythmias, where factors other than the specific dose may exert a more substantial influence.
2.4 Comparison with ibrutinib-induced AF
Ibrutinib was approved for treating relapsed/refractory CLL by the FDA in 2014 (Mato et al., 2022). Extensive research has explored the association between ibrutinib and AF. It has been firmly established that AF is the most prevalent cardiac complication associated with ibrutinib (Christensen et al., 2022; Buske et al., 2023). In contrast, VAs represent are rare but represent one of the most severe types of arrhythmias induced by ibrutinib. These two cardiac complications can be differentiated from each other based on numerous similarities, including age, incidence, and their relationship to the primary disease (Figure 1).
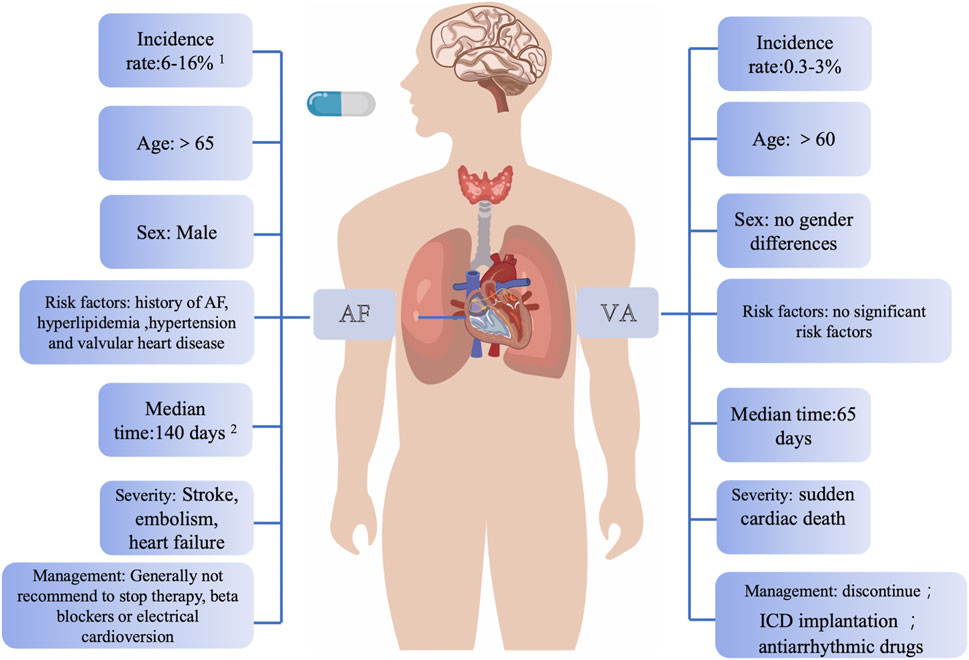
Figure 1. Main clinical differences between ibrutinib-associated AF and VAs. This figure highlights that AF typically occurs in older patients with extended ibrutinib use and is associated with male gender and a history of AF, whereas VAs are more acute, life-threatening, and often arise in patients without pre-existing cardiovascular disease. VAs generally necessitate discontinuing ibrutinib, while AF may allow dose adjustments. Data sources: 1. Characterization of AF events in ibrutinib trials; 2. Long-term follow-up of MCL patients on single-agent ibrutinib. Abbreviations: AF, atrial fibrillation; VAs, ventricular arrhythmias; ICD, implantable cardioverter defibrillator.
In various epidemiological studies on AF caused by ibrutinib, advanced age (>65 years) was identified as a risk factor for AF after medication, and the incidence of AF was positively correlated with the duration of medication (Wang et al., 2013; Burger et al., 2014; Tang et al., 2018; Brown et al., 2017; O'Brien et al., 2014; Byrd et al., 2015; Shanafelt et al., 2017). The available evidence from various case reports and retrospective studies regarding VAs induced by ibrutinib therapy does not provide conclusive support for a significant difference in the age of affected patients. Some retrospective studies have reported a median age of over 60 for individuals experiencing ibrutinib-associated VAs. Our previous basic research also suggested a higher occurrence of VAs in older individuals (Du et al., 2020). However, it is crucial to consider the potential inclusion bias associated with the age at the onset of CLL, which typically occurs in individuals with a median age ranging between 67 and 72 (Yang et al., 2021; Strati and Shanafelt, 2015). This inherent age factor in CLL patients may influence the observed age distribution in ibrutinib-related VAs cases. In summary, while existing evidence suggests a possible increased risk of VAs in older individuals, drawing definitive conclusions regarding age-related differences requires further investigation through large-scale clinical studies that carefully account for CLL onset age and other potential confounding factors.
Various studies have pinpointed several potential risk factors for developing AF, including age, male, a prior history of AF, hyperlipidemia, hypertension, and valvular heart disease (Brown et al., 2017; Shanafelt et al., 2017; Wiczer et al., 2017; Thompson et al., 2016). In the context of ibrutinib-induced VAs, limited evidence suggested that male gender, wide QRS, a history of AF, diabetes, heart failure, coronary artery disease, and valvular heart disease could be associated with these arrhythmias (Guha et al., 2018). It is noteworthy that a substantial body of evidence contradicts these findings. A significant proportion of patients who develop VAs after ibrutinib treatment have been reported to have no pre-existing CVD or traditional cardiovascular risk factors.
AF can lead to impaired functional capacity and increase the risk of stroke. However, it is essential to emphasize that this clinical challenge can often be effectively managed and even resolved through a collaborative and multidisciplinary approach involving hematologists and cardiologists. International retrospective analyses have also suggested that patients who experience the onset of AF while on ibrutinib treatment and have their ibrutinib dose either reduced without interruption or continue with the total amount may achieve better progression-free survival compared to those who have their ibrutinib treatment interrupted (Thompson et al., 2016). In contrast, the symptoms associated with VAs can be more acute, carry a higher risk, and are potentially life-threatening compared to AF (Fazal et al., 2023; Wang and Guo, 2022). Due to the substantial mortality rate associated with VAs, it is recommended that patients experiencing VAs should immediately discontinue ibrutinib therapy (Tang et al., 2022). This highlights the critical need for vigilant monitoring and rapid intervention in cases of VAs to ensure patient safety.
3 Clinical characteristics of ibrutinib-induced VAs
3.1 Symptoms and severity
The symptoms of ibrutinib-induced VAs can vary from palpitation, dizziness, lightheadedness, and chest discomfort to loss of consciousness and sudden cardiac death (SCD) (Tang et al., 2022). A high SCD rate has been reported in several prior cases and studies. Lampson et al. (2017) study identified that the SCD incidence rate of ibrutinib was 788 events per 100,000 person-years. In the HELIOS study, this rate reached 1991 events per 100,000 person-years. One of the key challenges in assessing VAs is the absence of clear and standardized definitions and indicators (Fazal et al., 2023; Chanan-Khan et al., 2016). Cardiac monitors can detect non-persistent ventricular tachycardia, irregular ventricular premature beats, and polymorphic ventricular tachycardia such as Tdp, which can evolve into VF in severe cases (Fazal et al., 2021; Fazal et al., 2023). Once these arrhythmias occur, they often arise continuously during ibrutinib treatment and induce fatal adverse prognosis. Hence, early monitoring and identification of the occurrence of VAs is vital to improve the prognosis.
3.2 QT interval changes associated with ibrutinib exposure
Several tyrosine kinase inhibitors have been associated with QT interval prolongation, including nilotinib, dasatinib, and sunitinib (Lu et al., 2012). However, the potential of ibrutinib to prolong the QT interval, leading to VAs, has not been definitively established.
Beyer et al. (2017) reported that a patient undergoing treatment with ibrutinib was found to have a prolonged QT interval of 590 ms and subsequently experienced pleomorphic VAs and electrical storms. QT interval prolongation has also been reported in cardiovascular events in standardized MedDRA Query with disproportionality analysis in the FDA FAERS. (Zheng et al., 2023). However, In a small-size retrospective study, Fradley et al. (2020) found that after applying ibrutinib, both the QT interval and the corrected QT were significantly shortened. Indeed, several studies have suggested that ibrutinib may not significantly prolong the QT interval (Essa et al., 2022). In a study on the application of ibrutinib in healthy subjects, short-term administration of conventional and high-dose ibrutinib did not produce a clinically meaningful prolongation of the QT interval (de Jong et al., 2017). Even in patients monitored with non-persistent ventricular tachycardia and other VA events, no substantial effect of ibrutinib on QT interval has been detected (Lampson et al., 2017; Fazal et al., 2021).
Overall, several factors, such as enrolled patient cohort and drug combination, can significantly affect the change of QT interval. The prevailing consensus among studies is that ibrutinib therapy is not typically associated with QT interval prolongation (Salem et al., 2021).
3.3 Management of ibrutinib-induced VAs
During clinical treatment, patients who experience symptoms such as palpitations, dizziness, or syncope associated with VAs during ibrutinib therapy are commonly recommended to undergo ambulatory ECG monitoring and implantable ECG monitoring as diagnostic tools. (Guha et al., 2018; Bhat et al., 2022; Solbiati et al., 2017; Merner et al., 2008; Sen-Chowdhry et al., 2007). Rivera et al. (2021) reported that a patient undergoing ibrutinib treatment for CLL developed hemodynamically unstable tachyarrhythmias after 4 months of therapy. Following the implantation loop recorder and an adjustment to the treatment regimen, the arrhythmia episodes were effectively terminated, and the CLL achieved complete remission. This underscores the critical role of advanced monitoring techniques and tailored treatment adjustments in managing ibrutinib-induced arrhythmias and optimizing patient outcomes.
Ibrutinib is mainly metabolized by cytochrome P450 3A4 (CYP3A4) enzymes (IMBRUVICA, 2022). When combined with CYP3A4 inducers or inhibitor, significant inter-drug interactions may occur, potentially altering drug toxicity and efficacy (de Jong et al., 2015; Scheers et al., 2015). Combining ibrutinib with duvelisib, a moderate CYP3A4 inhibitor, can increase the concertation of ibrutinib, leading to potential unexpected adverse outcomes, including fatalities (Paul et al., 2023). As a precautionary measure, it is strongly recommended to avoid the concurrent use of ibrutinib with drugs that interact with CYP3A4. If such combinations are deemed necessary, the ibrutinib dose should be reduced, and its blood concentrations closely monitored.
VAs is often characterized by insidious onset, rapid progression, and high mortality. Arrhythmias, including VAs, constitute one of the primary reasons for discontinuing ibrutinib therapy. In a study that investigated various types of arrhythmias induced by ibrutinib, it was discovered that among the patients who experienced VAs, 25% had to discontinue ibrutinib due to arrhythmias (Tuomi et al., 2018). By current guidelines, patients who develop signs and symptoms of VAs while on ibrutinib therapy are strongly advised to discontinue the drug. Following discontinuation, a comprehensive clinical risk/benefit assessment should be conducted before potentially considering the reinitiation of the same therapy. Clinicians should maintain a high degree of vigilance when patients on ibrutinib exhibit suspected symptoms of VAs, such as dizziness, palpitations, syncope (Koplan and Stevenson, 2009). Furthermore, for patients with a confirmed diagnosis of VAs, efforts should be made to identify and address any underlying predisposing factors for VAs. In cases of VAs without hemodynamic disturbance, treatment options may include administering medications such as lidocaine, beta-blockers, or intravenous amiodarone as a bolus injection. It is important to note that antiarrhythmic drugs can potentially cause or exacerbate arrhythmias, so close monitoring of vital signs is essential during medication administration (Jamshidi et al., 2012; Kudenchuk et al., 2017; Rajan et al., 2017; Zeppenfeld et al., 2022). For patients with VAs accompanied by hemodynamic disturbances, rapid electrical cardioversion should be implemented when necessary, following administration of drugs like Amiodarone or lidocaine, which can prevent the recurrence of VAs in a short time. Implantable cardioverter defibrillator implantation therapy can be an option for patients diagnosed with VAs. In cases of recurrent VAs or repeated shocks following implantable cardioverter defibrillator implantation, catheter ablation therapy should be considered (Al-Khatib et al., 2017). These strategies are customized to the severity context of each patient’s condition, presenting a valuable contribution to the management and treatment of VAs induced by ibrutinib.
New generation BTKi, including acalabrutinib and zanubrutinib, have shown promising outcomes in CLL treatment. Preliminary cohort studies have indicated a lower incidence of AF associated with these newer BTKi options (Byrd et al., 2021; Hillmen et al., 2020). Consequently, acalabrutinib has been considered a viable alternative for patients who need to discontinue treatment with ibrutinib due to adverse events (Ferrajoli et al., 2021; Furman et al., 2021). Although the newer generation of BTKi is generally believed to have a reduced occurrence of cardiovascular toxicities such as AF and VT, recent studies has demonstrated that acalabrutinib can also induce VT and SCD (Bhat et al., 2022; Brown et al., 2022; Zhai et al., 2023). Our recent publication also indicated increased ventricular vulnerability in in vivo SD rat model treated with acalabrutinib (Zhao et al., 2024a). Pirtobrutinib, a noncovalent BTKi, has shown impression efficacy in relapsed CLL and fewer adverse cardiovascular events (De, 2023; Gomez et al., 2023; Mato et al., 2021). However, due to the limited time since their introduction to the market and insufficient data from clinical trials, ongoing investigations are needed to determine whether the newer generation BTKi effectively reduces the incidence of VAs. Comprehensive research and extensive clinical studies are essential to establish the safety profile of these agents concerning cardiovascular toxicities.
Awan et al. (2022) convened an International Steering Committee of 12 physicians to design practical recommendations for optimal treatment strategies in patients receiving BTKi and effective ways to mitigate cardiovascular toxicity. They recommended that the caregivers thoroughly assess a patient’s level of cardiovascular risk before initiating treatment. This assessment should encompass not only established CVD but also potential risk factors. It should be tailored based on the patient’s preexisting conditions, risk factors, a baseline ECG and a detailed cardiac history. For those with a significant cardiac history or risk factors, echocardiography is also recommended as part of the baseline evaluation. Patients should be informed beforehand about the potential risk of rare but fatal VAs and should be vigilant for symptoms. Regular monitoring, including ECG and rhythm monitoring, should be conducted to screen for VAs (Tang et al., 2022; Quartermaine et al., 2023; Paydas, 2019). Some researchers have proposed that patients who develop VAs or SCD while on therapy should not be re-challenged, as there are prior case reports of recurrent ventricular events in patients in whom the medication was reintroduced (Boriani et al., 2023).
Overall, the symptoms of VAs can vary. In instances where patients are asymptomatic or experience only palpitations, increased vigilance becomes imperative. Holter and implantable cardiac monitors can significantly enhance monitoring capabilities in such cases, aiding in the detection of subtle or intermittent arrhythmias. For VAs leading to hemodynamic compromise or induce syncope, discontinuing ibrutinib is crucial. Whether the newer BTKi can serve as an effective alternative requires further research and evaluation. Close attention should be paid to potential interactions and appropriate monitoring when using BTKi with drugs metabolized by CYP3A4. The occurrence of VAs is a complex and severe clinical issue. Thus, a multidisciplinary approach involving hematologists and cardiologists is required to facilitate optimal treatment and care for affected patients. This collaborative approach ensures the best possible management and outcomes for individuals receiving BTKi therapy.
4 Potential mechanism of ibrutinib-induced VAs
Structural changes in the myocardium induced by ibrutinib may constitute the basis of electrophysiology remodeling and VAs. In an in vivo mice study, echocardiography after 4 weeks of ibrutinib administration showed a significant increase in left ventricular (LV) diameter, indicating a trend of LV enlargement (Jiang et al., 2019). In a cohort of 33 patients treated with ibrutinib who underwent cardiac magnetic resonance imaging (MRI), 54.8% displayed late gadolinium enhancement, a marker of myocardial fibrosis. Remarkably, this subgroup of patients experienced disproportionately higher rates of subsequent cardiac events. In one typical case, a patient undergoing ibrutinib treatment exhibited myocardium inflammation and discrete fibrosis on cardiac MRI just 2 months into therapy, which was closely linked to the patient’s SCD (Buck et al., 2023).
Electrophysiological and molecular mechanisms of ibrutinib-induced VAs in preclinical models are summarized as follows.
4.1 Electrophysiological mechanisms
Increased autonomicity and repolarization disorders are two possible electrophysiological mechanisms. An ex-vivo study on cardiomyocytes found that ibrutinib induced abnormal action potentials and increased late sodium currents, leading to enhanced cardiomyocyte automaticity (Yang and Roden, 2015). In our previous study using rat models, we investigated the electrophysiological mechanisms behind VAs triggered by acute ibrutinib administration (Du et al., 2020). In the Langendorff-perfused rat model, acute exposure to ibrutinib induced more prominent action potential duration (APD) alternans and APD alternans spatial discordance, both of which are markers of membrane electrophysiological instability strongly associated with the onset of VAs. Additionally, calcium mapping in this model revealed dysregulated calcium handling, characterized by increased calcium release (shortened time-to-peak and a lower calcium amplitude alternans ratio) and impaired calcium uptake (prolonged calcium transient duration 50). Further in vivo study also confirmed membrane repolarization heterogeneity and calcium dynamics dysfunction (Zhao et al., 2024b). Voltage mapping revealed more prevalent epicardial discordant APD alternans, a key marker of repolarization heterogeneity. Additionally, significant alterations in calcium dynamics were observed, including prolonged decay time constants of calcium transients, reduced amplitude alternans ratios, and increased spontaneous intracellular calcium elevations. These changes in calcium handling resulted in elevated diastolic intracellular calcium levels and heightened ventricular vulnerability.
In summary, membrane repolarization heterogeneity and dysregulated calcium dynamics constitutes the electrophysiological mechanism of ibrutinib induced VAs.
4.2 Molecular mechanisms
With evidence of impaired calcium dynamics, the investigation of the underlying molecular mechanisms centered on calcium-handling proteins and the upstream kinases that may be regulated by ibrutinib.
The dysfunction of calcium dynamics represents a critical downstream link that cannot be ignored in the context of Ibrutinib-related arrhythmias, whether atrial or ventricular. In a study conducted by Jiang et al. where ibrutinib was administered to C57BL/6 mice for 4 weeks, increased activity of calmodulin-dependent protein kinase II and the inhibition of ryanodine receptor 2-ser2814 and phospholamban-Thr17 were identified as potential contributors to ibrutinib-induced atrial structural remodeling and calcium dysregulation (Jiang et al., 2019).
For the upstream kinase affected by ibrutinib, various study explored different pathways. Early-stage studies ruled out the phosphatidylinositol 3-kinase-protein kinase B (PI3K-Akt) signaling pathway, a critical downstream effector of B-cell receptor signaling in the treatment of blood malignancies, as the underlying mechanism for ibrutinib-related AF (McMullen et al., 2014; Pretorius et al., 2009). Notably, the PI3K inhibitor idelalisib, which explicitly targets PI3K, has not exhibited cardiotoxic side effects (Nair and Cheson, 2016; Chung and Lee, 2014). Furthermore, in another in vivo study on C57BL/6 mice treated with ibrutinib/acalabrutinib (Xiao et al., 2020), researchers observed an increased susceptibility to atrial fibrosis and AF in the ibrutinib group, but not in the more specific BTKi, acalabrutinib, thus reaffirming the previously reported findings that off-target effects primarily caused ibrutinib-related arrhythmias. This in vivo research suggested that CSK is a critical molecule that inhibits and acts as a mediator for ibrutinib-related AF.
The energy metabolism pathway is another critical aspect to consider. The therapeutic effect of ibrutinib in lymphoma is closely associated with mitochondrial dysfunction, impacting the AMP-activated protein kinase (AMPK) signaling pathway, altered mitochondrial redox status, and cellular energy depletion (Sharif-Askari et al., 2019). Metabolic stress levels have also been linked with abnormal calcium release, diastolic calcium overload, and the influence of glycolytic intermediates on ryanodine receptor 2 calcium release. Additionally, ATP deficiency can result in decreased sarcoplasmic/endoplasmic reticulum Ca2⁺-ATPase 2a (SERCA2a) activity. Several previous studies have also established the role of metabolic stress levels in arrhythmias, particularly AF (Wiersma et al., 2019; Ozcan et al., 2019; Chakraborty et al., 2020). While molecular changes were not evident in the acute exposure study, our in -vivo findings confirmed that ibrutinib inhibits AMPK phosphorylation (Du et al., 2020; Zhao et al., 2024b). Since AMPK enhances SERCA2a expression, this inhibition significantly decreases SERCA2a levels (Morissette et al., 2019). The reduction in SERCA2a serves as a critical link between AMPK inhibition and impaired calcium handling. This dysregulation in calcium handling further contributes to membrane repolarization discordance, creating a substrate for electrical instability and increasing the risk of ibrutinib-induced VAs (Figure 2). Further validation has been provided by us using 5-Aminoimidazole-4-carboxamide ribonucleotide, an AMPK activator, which has been shown to reduce the vulnerability to ibrutinib-induced VAs (Zhao et al., 2024b). These findings highlight the potential of AMPK as a therapeutic target for managing ibrutinib-induced VAs in the future.
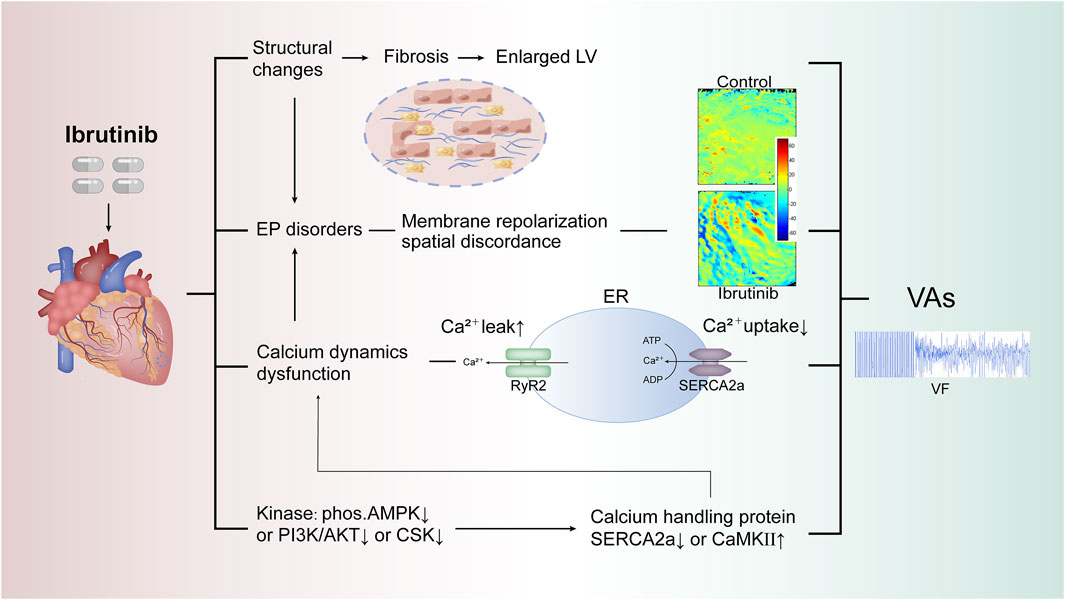
Figure 2. Proposed mechanisms of ibrutinib-induced VAs. This figure illustrates potential mechanisms of ibrutinib-induced VAs, focusing on key pathways involved in calcium dysregulation and electrophysiological instability. Dysregulated calcium handling, impaired SERCA2a function, and abnormal RyR2 activation are central to arrhythmogenesis. The role of upstream kinases (e.g., AMPK, PI3K/AKT) in modulating these processes and the impact of endoplasmic reticulum stress on calcium dynamics are also highlighted, increasing susceptibility to VAs. Abbreviations: VAs, ventricular arrhythmias; LV, left ventricle; EP, electrophysiological; RyR2, ryanodine receptor 2; SERCA2a, sarcoplasmic/endoplasmic reticulum calcium ATPase 2a; ER, endoplasmic reticulum; AMPK, AMP-activated protein kinase; PI3K/AKT, phosphoinositide 3-kinase/protein kinase B; CSK, C-terminal Src kinase; CaMKII, calcium/calmodulin-dependent protein kinase II; VF, ventricular fibrillation.
5 Conclusion
Among the cardiovascular complications arising from ibrutinib therapy, VAs are the most severe, yet not uncommon, and are associated with a poor prognosis. High-risk patients should undergo periodic screening to enable early detection of related symptoms. Timely intervention and improved clinical management are crucial for altering the prognosis. Effective collaboration between hematologists and cardiologists is essential to developing individualized treatment plans, including decisions regarding ibrutinib continuation or discontinuation, as well as arrhythmia management. Impaired AMPK activity leading to calcium handling dysfunction and ventricular repolarization discordance suggests increased vulnerability to ibrutinib-induced VAs.
Author contributions
YP: Writing–original draft, Writing–review and editing. YZ: Writing–original draft, Writing–review and editing. HR: Writing–review and editing. XW: Writing–review and editing. CL: Writing–review and editing. BD: Writing–original draft, Writing–review and editing. KN: Writing–review and editing. PY: Writing–review and editing.
Funding
The author(s) declare that financial support was received for the research, authorship, and/or publication of this article. This study was supported by National Natural Science Foundation of China (No. 82100337, 82300222), Jilin Provincial Natural Science Foundation (No. YDZJ202201ZYTS097) and the Bethune Program of Jilin University (No. 419161900105).
Conflict of interest
The authors declare that the research was conducted in the absence of any commercial or financial relationships that could be construed as a potential conflict of interest.
Generative AI statement
The author(s) declare that no Generative AI was used in the creation of this manuscript.
Publisher’s note
All claims expressed in this article are solely those of the authors and do not necessarily represent those of their affiliated organizations, or those of the publisher, the editors and the reviewers. Any product that may be evaluated in this article, or claim that may be made by its manufacturer, is not guaranteed or endorsed by the publisher.
Abbreviations
AF: atrial fibrillation; AMPK: AMP-activated protein kinase; APD: action potential duration; BTK: Bruton’s Tyrosine Kinase; BTKi: Bruton’s Tyrosine Kinase Inhibitor; CLL: chronic lymphocytic leukemia; CTCAE: Common Terminology Criteria for Adverse Events; CVD: cardiovascular disease; CYP3A4: cytochrome P450 3A4; ECG: electrocardiogram; FAERS: FDA Adverse Event Reporting System; FDA: Food and Drug Administration; LV: left ventricular; MRI: magnetic resonance imaging; PI3K: phosphatidylinositol 3-kinase; PI3K-Akt: phosphatidylinositol 3-kinase-protein kinase B; ROR: reported odds ratio; SCD: sudden cardiac death; SERCA2a: sarcoplasmic/endoplasmic reticulum Ca2⁺-ATPase 2a; TdPs: torsade de points; VAs: ventricular arrhythmias; VF: ventricular fibrillation; VT: ventricular tachycardia.
References
Advani, R. H., Buggy, J. J., Sharman, J. P., Smith, S. M., Boyd, T. E., Grant, B., et al. (2013). Bruton tyrosine kinase inhibitor ibrutinib (PCI-32765) has significant activity in patients with relapsed/refractory B-cell malignancies. J. Clin. Oncol. 31 (1), 88–94. doi:10.1200/JCO.2012.42.7906
Al-Khatib, S. M., Stevenson, W. G., Ackerman, M. J., Bryant, W. J., Callans, D. J., Curtis, A. B., et al. (2017). AHA/ACC/HRS guideline for management of patients with ventricular arrhythmias and the prevention of sudden cardiac death: a report of the American college of cardiology/American heart association task force on clinical practice guidelines and the heart rhythm society. J. Am. Coll. Cardiol. 72 (14), e91–e220. doi:10.1016/j.hrthm.2017.10.036
Aw, A., and Brown, J. R. (2017). Current status of Bruton's tyrosine kinase inhibitor development and use in B-cell malignancies. Drugs Aging 34 (7), 509–527. doi:10.1007/s40266-017-0468-4
Awan, F. T., Addison, D., Alfraih, F., Baratta, S. J., Campos, R. N., Cugliari, M. S., et al. (2022). International consensus statement on the management of cardiovascular risk of Bruton's tyrosine kinase inhibitors in CLL. Blood Adv. 6 (18), 5516–5525. doi:10.1182/bloodadvances.2022007938
Becker, L. B., Han, B. H., Meyer, P. M., Wright, F. A., Rhodes, K. V., Smith, D. W., et al. (1993). Racial differences in the incidence of cardiac arrest and subsequent survival. The CPR Chicago Project. N. Engl. J. Med. 329 (9), 600–606. doi:10.1056/NEJM199308263290902
Bernardeschi, P., Pirrotta, M. T., Del Rosso, A., Fontanelli, G., and Milandri, C. (2019). Sudden ventricular fibrillation and death during ibrutinib therapy-A case report. Eur. J. Haematol. 103 (4), 442–443. doi:10.1111/ejh.13290
Beyer, A., Ganti, B., Majkrzak, A., and Theyyunni, N. (2017). A perfect storm: tyrosine kinase inhibitor-associated polymorphic ventricular tachycardia. J. Emerg. Med. 52 (4), e123–e127. doi:10.1016/j.jemermed.2016.10.019
Bhat, S. A., Gambril, J. A., Azali, L., Chen, S. T., Rosen, L., Palettas, M., et al. (2022). Ventricular arrhythmias and sudden death events following acalabrutinib initiation. Blood 140, 2142–2145. doi:10.1182/blood.2022016953
Boriani, G., Menna, P., Morgagni, R., Minotti, G., and Vitolo, M. (2023). Ibrutinib and Bruton's tyrosine kinase inhibitors in chronic lymphocytic leukemia: focus on atrial fibrillation and ventricular tachyarrhythmias/sudden cardiac death. Chemotherapy 68 (2), 61–72. doi:10.1159/000528019
Brown, J. R. (2018). How I treat CLL patients with ibrutinib. Blood 131 (4), 379–386. doi:10.1182/blood-2017-08-764712
Brown, J. R., Byrd, J. C., Ghia, P., Sharman, J. P., Hillmen, P., Stephens, D. M., et al. (2022). Cardiovascular adverse events in patients with chronic lymphocytic leukemia receiving acalabrutinib monotherapy: pooled analysis of 762 patients. Haematologica 107 (6), 1335–1346. doi:10.3324/haematol.2021.278901
Brown, J. R., Eichhorst, B., Hillmen, P., Jurczak, W., Kaźmierczak, M., Lamanna, N., et al. (2023). Zanubrutinib or ibrutinib in relapsed or refractory chronic lymphocytic leukemia. N. Engl. J. Med. 388 (4), 319–332. doi:10.1056/NEJMoa2211582
Brown, J. R., Moslehi, J., O'Brien, S., Ghia, P., Hillmen, P., Cymbalista, F., et al. (2017). Characterization of atrial fibrillation adverse events reported in ibrutinib randomized controlled registration trials. Haematologica 102 (10), 1796–1805. doi:10.3324/haematol.2017.171041
Buck, B., Chum, A. P., Patel, M., Carter, R., Nawaz, H., Yildiz, V., et al. (2023). Cardiovascular magnetic resonance imaging in patients with ibrutinib-associated cardiotoxicity. JAMA Oncol. 9 (4), 552–555. doi:10.1001/jamaoncol.2022.6869
Burger, J. A., Keating, M. J., Wierda, W. G., Hartmann, E., Hoellenriegel, J., Rosin, N. Y., et al. (2014). Safety and activity of ibrutinib plus rituximab for patients with high-risk chronic lymphocytic leukaemia: a single-arm, phase 2 study. Lancet Oncol. 15 (10), 1090–1099. doi:10.1016/S1470-2045(14)70335-3
Burger, J. A., Tedeschi, A., Barr, P. M., Robak, T., Owen, C., Ghia, P., et al. (2015). Ibrutinib as initial therapy for patients with chronic lymphocytic leukemia. N. Engl. J. Med. 373 (25), 2425–2437. doi:10.1056/NEJMoa1509388
Buske, C., Jurczak, W., Salem, J. E., and Dimopoulos, M. A. (2023). Managing Waldenström's macroglobulinemia with BTK inhibitors. Leukemia 37 (1), 35–46. doi:10.1038/s41375-022-01732-9
Byrd, J. C., Brown, J. R., O'Brien, S., Barrientos, J. C., Kay, N. E., Reddy, N. M., et al. (2014). Ibrutinib versus ofatumumab in previously treated chronic lymphoid leukemia. N. Engl. J. Med. 371 (3), 213–223. doi:10.1056/NEJMoa1400376
Byrd, J. C., Furman, R. R., Coutre, S. E., Burger, J. A., Blum, K. A., Coleman, M., et al. (2015). Three-year follow-up of treatment-naïve and previously treated patients with CLL and SLL receiving single-agent ibrutinib. Blood 125 (16), 2497–2506. doi:10.1182/blood-2014-10-606038
Byrd, J. C., Furman, R. R., Coutre, S. E., Flinn, I. W., Burger, J. A., Blum, K. A., et al. (2013). Targeting BTK with ibrutinib in relapsed chronic lymphocytic leukemia. N. Engl. J. Med. 369 (1), 32–42. doi:10.1056/NEJMoa1215637
Byrd, J. C., Hillmen, P., Ghia, P., Kater, A. P., Chanan-Khan, A., Furman, R. R., et al. (2021). Acalabrutinib versus ibrutinib in previously treated chronic lymphocytic leukemia: results of the first randomized phase III trial. J. Clin. Oncol. 39 (31), 3441–3452. doi:10.1200/JCO.21.01210
Canada, H. (2018). “Summary Safety Review:IMBRUVICA (ibrutinib) - assessing the potential risk of a serious and life-threatening abnormal heart rhythm (ventricular tachyarrhythmia),” in Drug and Health product portal.
Castillo, J. J., Branagan, A. R., Sermer, D., Flynn, C. A., Meid, K., Little, M., et al. (2024). Ibrutinib and venetoclax as primary therapy in symptomatic, treatment-naïve Waldenström macroglobulinemia. Blood 143 (7), 582–591. doi:10.1182/blood.2023022420
Chakraborty, P., Nattel, S., and Nanthakumar, K. (2020). Linking cellular energy state to atrial fibrillation pathogenesis: potential role of adenosine monophosphate-activated protein kinase. Heart rhythm. 17 (8), 1398–1404. doi:10.1016/j.hrthm.2020.03.025
Chanan-Khan, A., Cramer, P., Demirkan, F., Fraser, G., Silva, R. S., Grosicki, S., et al. (2016). Ibrutinib combined with bendamustine and rituximab compared with placebo, bendamustine, and rituximab for previously treated chronic lymphocytic leukaemia or small lymphocytic lymphoma (HELIOS): a randomised, double-blind, phase 3 study. Lancet Oncol. 17 (2), 200–211. doi:10.1016/S1470-2045(15)00465-9
Cheng, C., Woronow, D., Nayernama, A., Wroblewski, T., and Jones, S. C. (2018). Ibrutinib-associated ventricular arrhythmia in the FDA adverse event reporting system. Leuk. Lymphoma 59 (12), 3016–3017. doi:10.1080/10428194.2018.1457149
Christensen, B. W., Zaha, V. G., and Awan, F. T. (2022). Cardiotoxicity of BTK inhibitors: ibrutinib and beyond. Expert Rev. Hematol. 15 (4), 321–331. doi:10.1080/17474086.2022.2067526
Chugh, S. S., Jui, J., Gunson, K., Stecker, E. C., John, B. T., Thompson, B., et al. (2004). Current burden of sudden cardiac death: multiple source surveillance versus retrospective death certificate-based review in a large U.S. community. J. Am. Coll. Cardiol. 44 (6), 1268–1275. doi:10.1016/j.jacc.2004.06.029
Chung, C., and Lee, R. (2014). Ibrutinib, obinutuzumab, idelalisib, and beyond: review of novel and evolving therapies for chronic lymphocytic leukemia. Pharmacotherapy 34 (12), 1298–1316. doi:10.1002/phar.1509
De, S. K. (2023). Pirtobrutinib: first non-covalent tyrosine kinase inhibitor for treating relapsed or refractory mantle cell lymphoma in adults. Curr. Med. Chem. doi:10.2174/0109298673251030231004052822
de Jong, J., Hellemans, P., Jiao, J. J., Huang, Y., Mesens, S., Sukbuntherng, J., et al. (2017). Ibrutinib does not prolong the corrected QT interval in healthy subjects: results from a thorough QT study. Cancer Chemother. Pharmacol. 80 (6), 1227–1237. doi:10.1007/s00280-017-3471-x
de Jong, J., Skee, D., Murphy, J., Sukbuntherng, J., Hellemans, P., Smit, J., et al. (2015). Effect of CYP3A perpetrators on ibrutinib exposure in healthy participants. Pharmacol. Res. Perspect. 3 (4), e00156. doi:10.1002/prp2.156
Dreyling, M., Jurczak, W., Jerkeman, M., Silva, R. S., Rusconi, C., Trneny, M., et al. (2016). Ibrutinib versus temsirolimus in patients with relapsed or refractory mantle-cell lymphoma: an international, randomised, open-label, phase 3 study. Lancet 387 (10020), 770–778. doi:10.1016/S0140-6736(15)00667-4
Du, B., Chakraborty, P., Azam, M. A., Massé, S., Lai, P. F. H., Niri, A., et al. (2020). Acute effects of ibrutinib on ventricular arrhythmia in spontaneously hypertensive rats. JACC CardioOncol 2 (4), 614–629. doi:10.1016/j.jaccao.2020.08.012
Essa, H., Dobson, R., and Lip, G. Y. H. (2022). Chemotherapy-induced arrhythmias. J. Cardiovasc Pharmacol. 80 (4), 531–539. doi:10.1097/FJC.0000000000001216
Fazal, M., Kapoor, R., Cheng, P., Rogers, A. J., Narayan, S. M., Wang, P., et al. (2021). Arrhythmia patterns in patients on ibrutinib. Front. Cardiovasc Med. 8, 792310. doi:10.3389/fcvm.2021.792310
Fazal, M., Wei, C., Chuy, K. L., Hussain, K., Gomez, S. E., Ba, S. S., et al. (2023). Tyrosine kinase inhibitor-associated ventricular arrhythmias: a case series and review of literature. J. Interv. Card. Electrophysiol. 66 (5), 1165–1175. doi:10.1007/s10840-022-01400-z
Ferrajoli, A., Byrd, J. C., Ghia, P., Sharman, J. P., Hillmen, P., Stephens, D. M., et al. (2021). CLL-354: pooled analysis of cardiovascular events from clinical trials evaluating acalabrutinib monotherapy in patients with chronic lymphocytic leukemia (CLL). Clin. Lymphoma Myeloma Leuk. 21, S323. doi:10.1016/s2152-2650(21)01760-2
Fradley, M. G., Welter-Frost, A., Gliksman, M., Emole, J., Viganego, F., Lee, D. H., et al. (2020). Electrocardiographic changes associated with ibrutinib exposure. Cancer control. 27 (1), 1073274820931808. doi:10.1177/1073274820931808
Furman, R. R., Byrd, J. C., Owen, R. G., O'Brien, S. M., Brown, J. R., Hillmen, P., et al. (2021). Pooled analysis of safety data from clinical trials evaluating acalabrutinib monotherapy in mature B-cell malignancies. Leukemia 35 (11), 3201–3211. doi:10.1038/s41375-021-01252-y
Gambril, J. A., Ghazi, S. M., Sansoterra, S., Ferdousi, M., Kola-Kehinde, O., Ruz, P., et al. (2024). Atrial fibrillation burden and clinical outcomes following BTK inhibitor initiation. Leukemia 38, 2141–2149. doi:10.1038/s41375-024-02334-3
Gomez, E. B., Ebata, K., Randeria, H. S., Rosendahl, M. S., Cedervall, E. P., Morales, T. H., et al. (2023). Preclinical characterization of pirtobrutinib, a highly selective, noncovalent (reversible) BTK inhibitor. Blood 142 (1), 62–72. doi:10.1182/blood.2022018674
Guha, A., Derbala, M. H., Zhao, Q., Wiczer, T. E., Woyach, J. A., Byrd, J. C., et al. (2018). Ventricular arrhythmias following ibrutinib initiation for lymphoid malignancies. J. Am. Coll. Cardiol. 72 (6), 697–698. doi:10.1016/j.jacc.2018.06.002
Herman, S. E., Gordon, A. L., Hertlein, E., Ramanunni, A., Zhang, X., Jaglowski, S., et al. (2011). Bruton tyrosine kinase represents a promising therapeutic target for treatment of chronic lymphocytic leukemia and is effectively targeted by PCI-32765. Blood 117 (23), 6287–6296. doi:10.1182/blood-2011-01-328484
Hillmen, P., Brown, J. R., Eichhorst, B. F., Lamanna, N., O'Brien, S. M., Qiu, L., et al. (2020). ALPINE: zanubrutinib versus ibrutinib in relapsed/refractory chronic lymphocytic leukemia/small lymphocytic lymphoma. Future Oncol. 16 (10), 517–523. doi:10.2217/fon-2019-0844
IMBRUVICA (2022). Highlights of prescribing information. Available at: https://www.accessdata.fda.gov/drugsatfda_docs/label/2022/217003s000lbl.pdf.
Jamshidi, Y., Nolte, I. M., Dalageorgou, C., Zheng, D., Johnson, T., Bastiaenen, R., et al. (2012). Common variation in the NOS1AP gene is associated with drug-induced QT prolongation and ventricular arrhythmia. J. Am. Coll. Cardiol. 60 (9), 841–850. doi:10.1016/j.jacc.2012.03.031
Jiang, L., Li, L., Ruan, Y., Zuo, S., Wu, X., Zhao, Q., et al. (2019). Ibrutinib promotes atrial fibrillation by inducing structural remodeling and calcium dysregulation in the atrium. Heart rhythm. 16 (9), 1374–1382. doi:10.1016/j.hrthm.2019.04.008
Koplan, B. A., and Stevenson, W. G. (2009). Ventricular tachycardia and sudden cardiac death. Mayo Clin. Proc. 84 (3), 289–297. doi:10.1016/S0025-6196(11)61149-X
Kudenchuk, P. J., Leroux, B. G., Daya, M., Rea, T., Vaillancourt, C., Morrison, L. J., et al. (2017). Antiarrhythmic drugs for nonshockable-turned-shockable out-of-hospital cardiac arrest: the ALPS study (amiodarone, lidocaine, or placebo). Circulation 136 (22), 2119–2131. doi:10.1161/CIRCULATIONAHA.117.028624
Lampson, B. L., Yu, L., Glynn, R. J., Barrientos, J. C., Jacobsen, E. D., Banerji, V., et al. (2017). Ventricular arrhythmias and sudden death in patients taking ibrutinib. Blood 129 (18), 2581–2584. doi:10.1182/blood-2016-10-742437
Lee, C. S., Rattu, M. A., and Kim, S. S. (2016). A review of a novel, Bruton's tyrosine kinase inhibitor, ibrutinib. J. Oncol. Pharm. Pract. 22 (1), 92–104. doi:10.1177/1078155214561281
Li, Y., Peng, X., Lin, R., Wang, X., Liu, X., Meng, F., et al. (2023). Tyrosine kinase inhibitor antitumor therapy and atrial fibrillation: potential off-target effects on mitochondrial function and cardiac substrate utilization. Cardiovasc. Innovations Appl. 8 (1). doi:10.15212/cvia.2023.0070
Lu, Z., Wu, C. Y., Jiang, Y. P., Ballou, L. M., Clausen, C., Cohen, I. S., et al. (2012). Suppression of phosphoinositide 3-kinase signaling and alteration of multiple ion currents in drug-induced long QT syndrome. Sci. Transl. Med. 4 (131), 131ra50. doi:10.1126/scitranslmed.3003623
Madgula, A. S., Singh, M., Almnajam, M., Pickett, C. C., and Kim, A. S. (2020). Ventricular tachycardia storm in a patient treated with ibrutinib for Waldenstrom macroglobulinemia. JACC Case Reports 2 (3), 523–526. doi:10.1016/j.jaccao.2020.06.006
Mato, A. R., Shah, N. N., Jurczak, W., Cheah, C. Y., Pagel, J. M., Woyach, J. A., et al. (2021). Pirtobrutinib in relapsed or refractory B-cell malignancies (BRUIN): a phase 1/2 study. Lancet 397 (10277), 892–901. doi:10.1016/S0140-6736(21)00224-5
Mato, A. R., Tang, B., Azmi, S., Yang, K., Zhang, X., Stern, J. C., et al. (2022). A clinical practice comparison of patients with chronic lymphocytic leukemia with and without deletion 17p receiving first-line treatment with ibrutinib. Haematologica 107 (11), 2630–2640. doi:10.3324/haematol.2021.280376
McMullen, J. R., Boey, E. J., Ooi, J. Y., Seymour, J. F., Keating, M. J., and Tam, C. S. (2014). Ibrutinib increases the risk of atrial fibrillation, potentially through inhibition of cardiac PI3K-Akt signaling. Blood 124 (25), 3829–3830. doi:10.1182/blood-2014-10-604272
Merner, N. D., Hodgkinson, K. A., Haywood, A. F., Connors, S., French, V. M., Drenckhahn, J. D., et al. (2008). Arrhythmogenic right ventricular cardiomyopathy type 5 is a fully penetrant, lethal arrhythmic disorder caused by a missense mutation in the TMEM43 gene. Am. J. Hum. Genet. 82 (4), 809–821. doi:10.1016/j.ajhg.2008.01.010
Moreno, C., Greil, R., Demirkan, F., Tedeschi, A., Anz, B., Larratt, L., et al. (2019). Ibrutinib plus obinutuzumab versus chlorambucil plus obinutuzumab in first-line treatment of chronic lymphocytic leukaemia (iLLUMINATE): a multicentre, randomised, open-label, phase 3 trial. Lancet Oncol. 20 (1), 43–56. doi:10.1016/S1470-2045(18)30788-5
Morissette, M. P., Susser, S. E., Stammers, A. N., Moffatt, T. L., Wigle, J. T., Wigle, T. J., et al. (2019). Exercise-induced increases in the expression and activity of cardiac sarcoplasmic reticulum calcium ATPase 2 is attenuated in AMPKα(2) kinase-dead mice. Can. J. Physiol. Pharmacol. 97 (8), 786–795. doi:10.1139/cjpp-2018-0737
Moslehi, J. J., Furman, R. R., Tam, C. S., Salem, J. E., Flowers, C. R., Cohen, A., et al. (2024). Cardiovascular events reported in patients with B-cell malignancies treated with zanubrutinib. Blood Adv. 8 (10), 2478–2490. doi:10.1182/bloodadvances.2023011641
Nair, K. S., and Cheson, B. (2016). The role of idelalisib in the treatment of relapsed and refractory chronic lymphocytic leukemia. Ther. Adv. Hematol. 7 (2), 69–84. doi:10.1177/2040620715625966
O'Brien, S., Furman, R. R., Coutre, S. E., Sharman, J. P., Burger, J. A., Blum, K. A., et al. (2014). Ibrutinib as initial therapy for elderly patients with chronic lymphocytic leukaemia or small lymphocytic lymphoma: an open-label, multicentre, phase 1b/2 trial. Lancet Oncol. 15 (1), 48–58. doi:10.1016/S1470-2045(13)70513-8
Ozcan, C., Li, Z., Kim, G., Jeevanandam, V., and Uriel, N. (2019). Molecular mechanism of the association between atrial fibrillation and heart failure includes energy metabolic dysregulation due to mitochondrial dysfunction. J. Card. Fail 25 (11), 911–920. doi:10.1016/j.cardfail.2019.08.005
Paul, S. R., Rosing, D. R., Haigney, M. C., Peer, C. J., Figg, W. D., Wiestner, A., et al. (2023). Cardiac toxicity in a pilot study of duvelisib and ibrutinib combination therapy for chronic lymphocytic leukaemia. Br. J. Haematol. 200 (2), 261–263. doi:10.1111/bjh.18558
Paydas, S. (2019). Management of adverse effects/toxicity of ibrutinib. Crit. Rev. Oncol. Hematol. 136, 56–63. doi:10.1016/j.critrevonc.2019.02.001
Pretorius, L., Du, X. J., Woodcock, E. A., Kiriazis, H., Lin, R. C., Marasco, S., et al. (2009). Reduced phosphoinositide 3-kinase (p110alpha) activation increases the susceptibility to atrial fibrillation. Am. J. Pathol. 175 (3), 998–1009. doi:10.2353/ajpath.2009.090126
Quartermaine, C., Ghazi, S. M., Yasin, A., Awan, F. T., Fradley, M., Wiczer, T., et al. (2023). Cardiovascular toxicities of BTK inhibitors in chronic lymphocytic leukemia: JACC: CardioOncology state-of-the-art review. JACC CardioOncol 5 (5), 570–590. doi:10.1016/j.jaccao.2023.09.002
Rajan, S., Folke, F., Hansen, S. M., Hansen, C. M., Kragholm, K., Gerds, T. A., et al. (2017). Incidence and survival outcome according to heart rhythm during resuscitation attempt in out-of-hospital cardiac arrest patients with presumed cardiac etiology. Resuscitation 114, 157–163. doi:10.1016/j.resuscitation.2016.12.021
Rivera, D., Takahashi, K., Durand, J. B., and Ferrajoli, A. (2021). Ibrutinib-associated cardiovascular events in a patient wearing an implanted loop recorder. Mediterr. J. Hematol. Infect. Dis. 13 (1), e2021044. doi:10.4084/MJHID.2021.044
Rothwell, P. M., Coull, A. J., Silver, L. E., Fairhead, J. F., Giles, M. F., Lovelock, C. E., et al. (2005). Population-based study of event-rate, incidence, case fatality, and mortality for all acute vascular events in all arterial territories (Oxford Vascular Study). Lancet 366 (9499), 1773–1783. doi:10.1016/S0140-6736(05)67702-1
Salem, J. E., Manouchehri, A., Bretagne, M., Lebrun-Vignes, B., Groarke, J. D., Johnson, D. B., et al. (2019). Cardiovascular toxicities associated with ibrutinib. J. Am. Coll. Cardiol. 74 (13), 1667–1678. doi:10.1016/j.jacc.2019.07.056
Salem, J. E., Nguyen, L. S., Moslehi, J. J., Ederhy, S., Lebrun-Vignes, B., Roden, D. M., et al. (2021). Anticancer drug-induced life-threatening ventricular arrhythmias: a World Health Organization pharmacovigilance study. Eur. Heart J. 42 (38), 3915–3928. doi:10.1093/eurheartj/ehab362
Scheers, E., Leclercq, L., de Jong, J., Bode, N., Bockx, M., Laenen, A., et al. (2015). Absorption, metabolism, and excretion of oral 1⁴C radiolabeled ibrutinib: an open-label, phase I, single-dose study in healthy men. Drug Metab. Dispos. 43 (2), 289–297. doi:10.1124/dmd.114.060061
Sen-Chowdhry, S., Syrris, P., and McKenna, W. J. (2007). Role of genetic analysis in the management of patients with arrhythmogenic right ventricular dysplasia/cardiomyopathy. J. Am. Coll. Cardiol. 50 (19), 1813–1821. doi:10.1016/j.jacc.2007.08.008
Shanafelt, T. D., Parikh, S. A., Noseworthy, P. A., Goede, V., Chaffee, K. G., Bahlo, J., et al. (2017). Atrial fibrillation in patients with chronic lymphocytic leukemia (CLL). Leuk. Lymphoma 58 (7), 1630–1639. doi:10.1080/10428194.2016.1257795
Shanafelt, T. D., Wang, X. V., Kay, N. E., Hanson, C. A., O'Brien, S., Barrientos, J., et al. (2019). Ibrutinib-Rituximab or chemoimmunotherapy for chronic lymphocytic leukemia. N. Engl. J. Med. 381 (5), 432–443. doi:10.1056/NEJMoa1817073
Sharif-Askari, B., Doyon, D., Paliouras, M., and Aloyz, R. (2019). Bruton's tyrosine kinase is at the crossroads of metabolic adaptation in primary malignant human lymphocytes. Sci. Rep. 9 (1), 11069. doi:10.1038/s41598-019-47305-2
Sherazi, S., Schleede, S., McNitt, S., Casulo, C., Moore, J. E., Storozynsky, E., et al. (2023). Arrhythmogenic cardiotoxicity associated with contemporary treatments of lymphoproliferative disorders. J. Am. Heart Assoc. 12 (6), e025786. doi:10.1161/JAHA.122.025786
Sirichand, S., Killu, A. M., Padmanabhan, D., Hodge, D. O., Chamberlain, A. M., Brady, P. A., et al. (2017). Incidence of idiopathic ventricular arrhythmias: a population-based study. Circ. Arrhythm. Electrophysiol. 10 (2), e004662. doi:10.1161/CIRCEP.116.004662
Solbiati, M., Casazza, G., Dipaola, F., Barbic, F., Caldato, M., Montano, N., et al. (2017). The diagnostic yield of implantable loop recorders in unexplained syncope: a systematic review and meta-analysis. Int. J. Cardiol. 231, 170–176. doi:10.1016/j.ijcard.2016.12.128
Strati, P., and Shanafelt, T. D. (2015). Monoclonal B-cell lymphocytosis and early-stage chronic lymphocytic leukemia: diagnosis, natural history, and risk stratification. Blood 126 (4), 454–462. doi:10.1182/blood-2015-02-585059
Tang, C. P. S., Lip, G. Y. H., McCormack, T., Lyon, A. R., Hillmen, P., Iyengar, S., et al. (2022). Management of cardiovascular complications of bruton tyrosine kinase inhibitors. Br. J. Haematol. 196 (1), 70–78. doi:10.1111/bjh.17788
Tang, C. P. S., McMullen, J., and Tam, C. (2018). Cardiac side effects of bruton tyrosine kinase (BTK) inhibitors. Leuk. Lymphoma 59 (7), 1554–1564. doi:10.1080/10428194.2017.1375110
Thompson, P. A., Lévy, V., Tam, C. S., Al Nawakil, C., Goudot, F. X., Quinquenel, A., et al. (2016). Atrial fibrillation in CLL patients treated with ibrutinib. An international retrospective study. Br. J. Haematol. 175 (3), 462–466. doi:10.1111/bjh.14324
Tomcsányi, J., Nényei, Z., Mátrai, Z., and Bózsik, B. (2016). Ibrutinib, an approved tyrosine kinase inhibitor as a potential cause of recurrent polymorphic ventricular tachycardia. JACC Clin. Electrophysiol. 2 (7), 847–849. doi:10.1016/j.jacep.2016.07.004
Treon, S. P., Gustine, J., Meid, K., Yang, G., Xu, L., Liu, X., et al. (2018). Ibrutinib monotherapy in symptomatic, treatment-naïve patients with waldenström macroglobulinemia. J. Clin. Oncol. 36 (27), 2755–2761. doi:10.1200/JCO.2018.78.6426
Treon, S. P., Meid, K., Gustine, J., Yang, G., Xu, L., Liu, X., et al. (2021). Long-term follow-up of ibrutinib monotherapy in symptomatic, previously treated patients with waldenström macroglobulinemia. J. Clin. Oncol. 39 (6), 565–575. doi:10.1200/JCO.20.00555
Tuomi, J. M., Xenocostas, A., and Jones, D. L. (2018). Increased susceptibility for atrial and ventricular cardiac arrhythmias in mice treated with a single high dose of ibrutinib. Can. J. Cardiol. 34 (3), 337–341. doi:10.1016/j.cjca.2017.12.001
Wallace, N., Wong, E., Cooper, D., and Chao, H. (2016). A case of new-onset cardiomyopathy and ventricular tachycardia in a patient receiving ibrutinib for relapsed mantle cell lymphoma. Clin. Case Rep. 4 (12), 1120–1121. doi:10.1002/ccr3.719
Wang, M. L., Blum, K. A., Martin, P., Goy, A., Auer, R., Kahl, B. S., et al. (2015). Long-term follow-up of MCL patients treated with single-agent ibrutinib: updated safety and efficacy results. Blood 126 (6), 739–745. doi:10.1182/blood-2015-03-635326
Wang, M. L., Rule, S., Martin, P., Goy, A., Auer, R., Kahl, B. S., et al. (2013). Targeting BTK with ibrutinib in relapsed or refractory mantle-cell lymphoma. N. Engl. J. Med. 369 (6), 507–516. doi:10.1056/NEJMoa1306220
Wang, X., and Guo, X. (2022). A rare case of a primary cardiac tumor presenting as fatal ventricular tachycardia. Cardiovasc. Innovations Appl. 7 (1). doi:10.15212/cvia.2022.0014
Wiczer, T. E., Levine, L. B., Brumbaugh, J., Coggins, J., Zhao, Q., Ruppert, A. S., et al. (2017). Cumulative incidence, risk factors, and management of atrial fibrillation in patients receiving ibrutinib. Blood Adv. 1 (20), 1739–1748. doi:10.1182/bloodadvances.2017009720
Wiersma, M., van Marion, D. M. S., Wüst, R. C. I., Houtkooper, R. H., Zhang, D., Groot, N. M. S., et al. (2019). Mitochondrial dysfunction underlies cardiomyocyte remodeling in experimental and clinical atrial fibrillation. Cells 8 (10), 1202. doi:10.3390/cells8101202
Woyach, J. A., Ruppert, A. S., Heerema, N. A., Zhao, W., Booth, A. M., Ding, W., et al. (2018). Ibrutinib regimens versus chemoimmunotherapy in older patients with untreated CLL. N. Engl. J. Med. 379 (26), 2517–2528. doi:10.1056/NEJMoa1812836
Xiao, L., Salem, J. E., Clauss, S., Hanley, A., Bapat, A., Hulsmans, M., et al. (2020). Ibrutinib-mediated atrial fibrillation attributable to inhibition of C-terminal Src kinase. Circulation 142 (25), 2443–2455. doi:10.1161/CIRCULATIONAHA.120.049210
Yang, S., Varghese, A. M., Sood, N., Chiattone, C., Akinola, N. O., Huang, X., et al. (2021). Ethnic and geographic diversity of chronic lymphocytic leukaemia. Leukemia 35 (2), 433–439. doi:10.1038/s41375-020-01057-5
Yang, T. M. J., and Roden, D. M. (2015). Abstract 14587: proarrhythmic effects of ibrutinib, a clinically approved inhibitor of bruton’S tyrosine kinase (BTK) used in cancer therapy. Circulation 132. doi:10.1161/circ.132.suppl_3.14587
Zeppenfeld, K., Tfelt-Hansen, J., de Riva, M., Winkel, B. G., Behr, E. R., Blom, N. A., et al. (2022). 2022 ESC Guidelines for the management of patients with ventricular arrhythmias and the prevention of sudden cardiac death. Eur. Heart J. 43 (40), 3997–4126. doi:10.1093/eurheartj/ehac262
Zhai, Y., Hu, F., Shi, W., Ye, X., Xu, J., Guo, X., et al. (2023). Pharmacovigilance analysis of cardiac risks associated with Bruton tyrosine kinase inhibitors. Expert Opin. Drug Saf. 22 (9), 857–869. doi:10.1080/14740338.2023.2204226
Zhao, Y., Chakraborty, P., Tomassetti, J., Subha, T., Massé, S., Thavendiranathan, P., et al. (2024a). Arrhythmogenic ventricular remodeling by next-generation Bruton's tyrosine kinase inhibitor acalabrutinib. Int. J. Mol. Sci. 25 (11), 6207. doi:10.3390/ijms25116207
Zhao, Y., Du, B., Chakraborty, P., Denham, N., Massé, S., Lai, P. F. H., et al. (2024b). Impaired cardiac AMPK (5'-adenosine monophosphate-activated protein kinase) and Ca(2+)-handling, and action potential duration heterogeneity in ibrutinib-induced ventricular arrhythmia vulnerability. J. Am. Heart Assoc. 13 (12), e032357. doi:10.1161/JAHA.123.032357
Keywords: ibrutinib, ventricular arrythmias, atrial fibrillation, Bruton’s tyrosine kinase inhibitor, cardio-oncology
Citation: Pan Y, Zhao Y, Ren H, Wang X, Liu C, Du B, Nanthakumar K and Yang P (2024) Epidemiology, clinical characteristics and potential mechanism of ibrutinib-induced ventricular arrhythmias. Front. Pharmacol. 15:1513913. doi: 10.3389/fphar.2024.1513913
Received: 19 October 2024; Accepted: 07 November 2024;
Published: 19 November 2024.
Edited by:
Zhi-Ren Zhang, Harbin Medical University, ChinaReviewed by:
Tong Liu, Tianjin Medical University, ChinaRan Zhang, Chinese PLA General Hospital, China
Copyright © 2024 Pan, Zhao, Ren, Wang, Liu, Du, Nanthakumar and Yang. This is an open-access article distributed under the terms of the Creative Commons Attribution License (CC BY). The use, distribution or reproduction in other forums is permitted, provided the original author(s) and the copyright owner(s) are credited and that the original publication in this journal is cited, in accordance with accepted academic practice. No use, distribution or reproduction is permitted which does not comply with these terms.
*Correspondence: Beibei Du, YmVpYmVpZHUyMDEyQGpsdS5lZHUuY24=
†These authors have contributed equally to this work