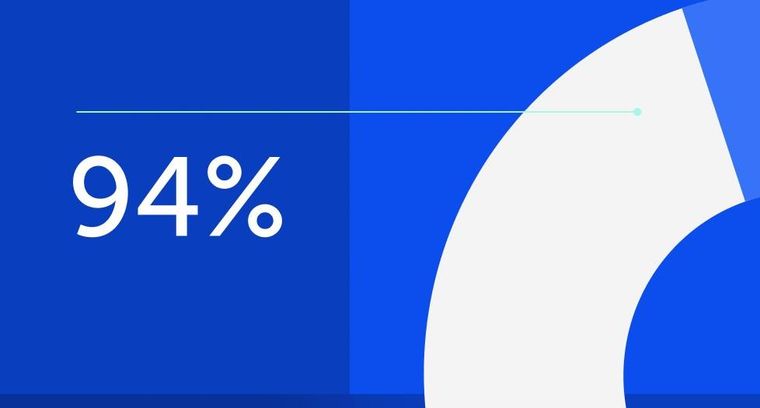
94% of researchers rate our articles as excellent or good
Learn more about the work of our research integrity team to safeguard the quality of each article we publish.
Find out more
REVIEW article
Front. Pharmacol., 12 December 2024
Sec. Inflammation Pharmacology
Volume 15 - 2024 | https://doi.org/10.3389/fphar.2024.1502298
CAR-T cell therapy, a cutting-edge cellular immunotherapy with demonstrated efficacy in treating hematologic malignancies, also exhibits significant promise for addressing autoimmune diseases. This innovative therapeutic approach holds promise for achieving long-term remission in autoimmune diseases, potentially offering significant benefits to affected patients. Current targets under investigation for the treatment of these conditions include CD19, CD20, and BCMA, among others. However, CAR-T therapy faces difficulties such as time-consuming cell manufacturing, complex and expensive process, and the possibility of severe adverse reactions complicating the treatment, etc. This article examines CAR-T therapy across various rheumatic autoimmune diseases, including systemic lupus erythematosus (SLE), rheumatoid arthritis (RA), Sjögren’s syndrome (SS), systemic sclerosis (SSc), antisynthetase syndrome (ASS), and ANCA-associated vasculitis (AAV), highlighting both therapeutic advancements and ongoing challenges.
Autoimmune diseases are typically chronic conditions characterized by a wide range of clinical manifestations affecting multiple organs (Ameer et al., 2022; Radu and Bungau, 2021; Manfrè et al., 2022; Bongartz et al., 2007; Adami et al., 2019). The pathogenic mechanisms underlying these diseases remain largely unresolved, and they are often incurable. These conditions frequently result in high rates of disability and mortality. The status quo of high medical costs associated with long-term medication use, in addition to the fact that some patients are ineffective or intolerant to conventional medications (antirheumatic drugs, nonsteroidal anti-inflammatory drugs, and glucocorticosteroids) (Lin et al., 2020; Huang et al., 2021), makes the treatment of autoimmune diseases an important clinical issue.
It is now well established that B cells play a crucial role in various autoimmune diseases. Several commonly used biologics exert their therapeutic effects by targeting and inhibiting B cell activation or proliferation. For instance, rituximab, an anti-CD20 agent frequently used for B cell-mediated diseases (Lee et al., 2020), is costly, necessitates frequent injections, and does not fully deplete B cells to achieve complete remission (Cataldi et al., 2017). Thus, there is a clear need to investigate alternative treatment modalities.
CAR-T cell therapy has demonstrated remarkable efficacy in treating leukemia and malignant tumors. Leveraging its therapeutic principle—where CAR-T cells are engineered to target and eliminate pathological immune cells—offers promising insights for its application in autoimmune diseases (Kansal et al., 2019; Jin et al., 2021). Several clinical studies of CAR-T for the treatment of autoimmunity have been conducted with encouraging results (Qin et al., 2023; Lundberg et al., 2023). This article provides an overview of the advancements in utilizing CAR-T therapy for treating autoimmune diseases. Our center has also conducted a clinical trial registered with the NCT for patients with relapsed and refractory systemic lupus erythematosus (SLE), where CAR-T therapy has demonstrated promising efficacy in treating autoimmune conditions.
Pathologic cells associated with autoimmune diseases include autoantibody-producing B cells, activated T cells, and antigen-presenting cells (antigen-presenting cells, APCs) (Boulch et al., 2021). Among them, B cells are mainly responsible for the humoral immune response and play an important role in a variety of autoimmune diseases by producing antibodies, releasing pro-inflammatory cytokines and activating T cells as APCs (Oh and Payne, 2022) (Figure 1B).
Figure 1. (A): The manufacturing process of CAR-T; (B): The mechanism of action of CAR-T in vivo; (C): Rheumatic autoimmune diseases related to abnormal B cell function; (D):5 generations of CAR-T therapy development.
Systemic lupus erythematosus is a complex disease characterized by antibody production and heterogeneity of clinical manifestations, with disruption of B-cell tolerance being a critical early event in the course of the disease (Krustev et al., 2023; Möckel et al., 2021). Rheumatoid arthritis is a chronic, progressive autoimmune disease in which defects in B-cell tolerance checkpoints, autoreactive B cells acting as antigen-presenting cells (APCs) to stimulate the activation of autoreactive T cells, and the production of proinflammatory and anti-inflammatory cytokines, are functions that, either individually or in combination, contribute to the pathogenesis of RA ((Finnegan et al., 2012)). In addition, multiple B cell subpopulations have been found in the salivary glands of patients with desiccation syndrome (Daridon et al., 2006; Qin et al., 2022; Verstappen et al., 2021; Rivière et al., 2021), and BAFF plays a prominent role in its pathogenesis as an essential survival factor for both transitional and mature B cells (Zhan et al., 2023). Systemic sclerosis is also a systemic autoimmune disease, mainly characterized by autoimmune abnormalities, especially B cells play an important role in the development of systemic sclerosis. Patients with this disease often present with hypergammaglobulinemia and the presence of various autoantibodies (Fukasawa et al., 2024). Anti-synthetase syndrome is a distinct subtype of idiopathic inflammatory myopathy, characterized by the presence of autoantibodies—particularly anti-Jo-1 antibodies—which are frequently associated with pathological manifestations such as interstitial lung disease, Raynaud’s phenomenon, and arthritis. Given these associations, deep depletion of B cells may offer therapeutic benefits. Anti-neutrophil cytoplasmic autoantibody (ANCA)-associated vasculitis (AAV) is a life-threatening systemic autoimmune disease, driven by autoantigens such as myeloperoxidase (MPO) or protease 3. Therapeutic strategies have been explored to achieve clinical benefits by depleting B cells that produce MPO-ANCA((McClure et al., 2018)) (Figure 1C).
Current treatments for autoimmune diseases focus on suppressing the immune system through small molecules that inhibit immune cell activation or proliferation, such as the B-cell depleting drug anti-CD20 monoclonal antibody rituximab, which was initially approved for use in certain types of B-cell malignancies and is now used to treat B-cell mediated autoimmune disease. However, some patients do not show remission, and even when effective, frequent injections are required and there is a risk of chronic B-cell failure and infection due to chronic immunosuppression (Tony et al., 2011). The drug has also been found to struggle with the complete elimination of B cells, as evidenced by the persistence of B cells within the synovium of rheumatoid arthritis patients treated with rituximab. This suggests that tissue-resident B cells may evade depletion by rituximab, in contrast to circulating B cells (Thurlings et al., 2010).
Chimeric antigen receptor T-cell (CAR-T) immunotherapy is a novel therapeutic approach that involves the extraction of T cells, either autologous or allogeneic, followed by in vitro expansion and subsequent infusion into the patient (Figure 1A). Genetic material with specific antigen recognition domains and T-cell activation signals are transferred into T cells by retroviruses or lentiviruses, so that T cells no longer require APCs for activation, and these CAR-T cells initially bind to antigen-expressing target cells and subsequently exert their cytotoxic effects, leading to the lysis of the target cells. In addition, CAR-T cells can differentiate into long-lived memory cells in vivo, facilitating more sustained remission. Leveraging the cytotoxicity and tissue infiltration capabilities of T cells, CAR-T cells can also effectively target difficult-to-reach, tissue-resident tumor cells. In comparison, conventional drugs are ineffective or intolerable for many patients, and biologic anti-cd20 antibodies require repeated administration to maintain therapy (Reddy et al., 2015). And over time, the host can become resistant to treatment, all of which further limits the use of conventional drugs.
A classic CAR has three main portions: ecto-domain, transmembrane domain and endo-domain. The ecto-domain includes a recognition domain of the antigen and a hinge domain, and the endodomain is characterized as a costimulatory and intracellular signaling domain. The first generation of CARs only consisted of three simple parts, there was no co-stimulation part, which could not secrete enough cytokines and led to the failure of amplification. Therefore, co-stimulation signals were added on the basis of the first generation, and the second generation of CARs was born. The co-stimulation signal can induce the proliferation of T cells and the release of cellular factors. The subsequent generations were designed on the basis of the second generation, the third generation added a stimulating factor on the basis of the second generation, and the fourth and fifth generations added cytokines to regulate the tumor microenvironment to enhance efficacy (Figure 1D) (Chen et al., 2023; Baker et al., 2023; Adabi et al., 2023).
CAR-T cells are genetically engineered T cells designed to express synthetic receptors on their surface, enabling them to identify and eliminate cancer cells by recognizing specific tumor antigens independently of human major histocompatibility complex (MHC) molecules (Chen et al., 2023). Initially conceived as a “living drug” to target and destroy tumors, CAR-T cells have shown promising efficacy in this regard. Natural killer (NK) cells eliminate target cells through two primary mechanisms: the release of cytolytic granules and the induction of apoptosis via Fas ligand (FasL). The incorporation of chimeric antigen receptors (CARs) enables both T cells and natural killer (NK) cells to selectively and effectively target and eliminate cancer cells. And it was observed that CAR NK has a better safety profile (Obstfeld et al., 2017). It also paves the way for the development of off-the-shelf CAR products that can be cryopreserved for long-term storage (Siegler et al., 2018). However, CAR-NK cells suffer from limited durability. A significant challenge for both CAR-T and CAR-NK cells in the treatment of solid tumors is their difficulty in effectively infiltrating the tumor’s interior. So researchers began introducing CARs into macrophages, which are able to infiltrate solid tumors naturally and effectively (Morrissey et al., 2018; Sternlicht and Werb, 2001; Klichinsky et al., 2020). To overcome these drawbacks, other cell therapies are being developed, and currently there are NK cells, macrophages, Treg cells, neutrophils, and pluripotent stem cell-derived CARs cell therapies (Table 1).
CD19 is a surface antigen predominantly expressed on B-lineage malignancies. It has become a prominent target for CAR-T therapy, beginning with the approval of Kymriah as the first anti-CD19 CAR product in 2017. Since then, CD19 CAR-T therapy has demonstrated success in clinical trials across various B-cell cancers (Wei et al., 2019; Kochenderfer et al., 2015). And gradually more targets such as BCMA, CD22, CD7, CD20, etc., have also been introduced in order to deeply deplete B cells (Table 1).
The manufacturing process for autologous CAR-T cells takes approximately 3 weeks, during which patients may experience disease progression. To address this issue, universal CAR-T cells derived from allogeneic sources have been proposed as an alternative. It is expected to be produced on a large scale as an “off-the-shelf” drug. Recently, Wang et al. reported the first use of allogeneic CAR-T cells in patients with autoimmune diseases. All three patients showed significant clinical improvement. And they showed significant clinical improvement and remained in remission for the 6 months of follow-up to date (Wang X. et al., 2024; Baker and June 2024). A major adverse event of allogeneic CAR-T therapy is the risk of GvHD due to attack of host organs by the injected T cells. No signs of GvHD have been reported in these patients, and another important safety issue in CAR-T therapy is CRS. However, Wang et al. did not observe any clinical signs of severe CRS. This may be due to the fact that the target burden of autoimmune diseases is much smaller compared to cancer. Alternatively, new approaches are being explored to eliminate in vitro manufacturing time through gene editing, namely in vivo cell reprogramming for in situ cell therapy (Nicolai et al., 2024). Currently, in situ reprogramming of target cells includes both viral and non-viral approaches. Viral vectors can mediate effective delivery, but are accompanied by immunogenicity and safety issues. Synthetic nanomaterials, such as lipid nanoparticles (LNPs), which have smaller immunogenicity, are easy to produce and low-cost, are promising alternatives to viral vectors, although they are still in the initial stages of exploration (Table 1).
Systemic lupus erythematosus (SLE) is a highly heterogeneous autoimmune disease that affects almost all organs and tissues (Tsokos et al., 2016). Disruption of immune tolerance and persistent autoantibody production are the two main features of SLE. Current CAR-T cell therapy targets for the treatment of systemic lupus erythematosus (SLE) include CD19, CD20, and BCMA. Administering anti-CD19 CAR-T cells in a mouse model of severe lupus nephritis led to improved disease outcomes and prolonged lifespan, indicating the efficacy of this therapy in SLE and underscoring its potential for clinical application (Jin et al., 2021). Elevated numbers of follicular helper CD4+ T cells (TFH) have been observed in patients with SLE, particularly during disease flares, with TFH counts correlating with levels of programmed cell death protein 1 (PD-1) expression (Crotty, 2011). Seth D. Reighard et al. designed and evaluated an innovative CAR-NK cell that targets PD-1-expressing cells to eliminate TFH. In short-term in vitro co-cultures, these PD-1-targeting CAR-NK cells selectively cleared TFH cells. These results bring new ideas for the treatment of SLE ((Reighard et al., 2020)). CAR Treg cells theoretically hold promise for restoring Treg numbers and function as a treatment for SLE. However, IL-21 secreted by CD4+ T cells compromises Treg survival and function, and a highly pro-inflammatory environment can drive Treg cells to convert into IL-17-producing or other inflammatory factor-secreting cells. These challenges underscore the complexities of using CAR Treg cells in SLE treatment, necessitating further investigation (Kim et al., 2015).
So far, 25cases of SLE treated with CAR-T have been reported, including 10cases in Germany (Krickau et al., 2024), 10 cases in China, and five cases in the US [(Boulougoura et al., 2023)]. In August 2021, Georgschett’s team reported the first patient treated with CAR-T for systemic lupus erythematosus, in which dsDNA autoantibody titers and C3 and C4 complement levels returned to normal after 5 weeks of CAR-T cell injections, proteinuria was virtually eradicated, and the Disease Activity Index was reduced from a score of 16 to 0, with no significant side effects (Mougiakakos et al., 2021). The team later reported on nine additional lupus patients treated with CAR-T, all of whom improved significantly (Mackensen et al., 2022; Subklewe et al., 1990; Li et al., 2024; Krickau et al., 2024).
Our center has also conducted a clinical study of CD19 CAR-T for the treatment of systemic lupus erythematosus (NCT06056921), which has been administered to two patients, both of whom have demonstrated improvement and a favorable safety profile.
Sjögre’s syndrome (SS) is a chronic autoimmune disease with multiple manifestations, the main manifestations of which include dryness of the mouth and eyes, as well as systemic complications (Zhan et al., 2023). B-cell overactivity is evidenced by the presence of various autoantibodies, including RF and anti-SSA/SSB antibodies, as well as by hypergammaglobulinemia. Additionally, some patients with systemic sclerosis may develop malignant B-cell lymphomas. B-cell activating factor (BAFF) is a key driver of systemic sclerosis (SS) development, playing a crucial role in promoting B-cell survival and hyperactivity (Nocturne and Mariette, 2018). Certain targeted therapies, such as rituximab, exert their therapeutic effects by depleting B cells. Anti-CD19 CAR-T cell therapy is similar in principle to CD20 monoclonal antibody therapy, which also treats SS through B-cell depletion. However, it has been observed that long-lived plasma cells in many SS patients do not express or express very low levels of CD20, and thus CD20 monoclonal antibodies cannot target them. In contrast to CD20, which is expressed primarily on mature B cells, CD19 is widely expressed throughout the maturation process of pre-B cells into plasma cells. This broader expression profile suggests that CD19 may be a more effective target for immunotherapy. Currently, two Phase I studies (NCT05085431 and NCT05859997) are investigating the safety and efficacy of CD19/BCMA CAR-T cells for the treatment of systemic sclerosis. The results of these studies have yet to be reported. To date, only a single case has been reported from Germany, involving a patient with a 10-year history of dry syndrome and secondary interstitial lung disease, who was subsequently diagnosed with diffuse large B-cell lymphoma (Sheng et al., 2023). Following treatment with anti-CD19 CAR-T cells, the patient achieved complete remission (CR). Notably, by day 90 post-therapy, the patient tested negative for antinuclear antibodies and anti-Ro-52 for the first time. Early in the treatment, the patient exhibited grade 2 cytokine release syndrome and grade 1 neurotoxicity, but the adverse effects were completely controlled after aggressive treatment, proving that they were tolerated reasonably well. As for more long-term efficacy, long-term follow-up is needed to confirm this.
Systemic sclerosis (SSc) is a connective tissue disease marked by autoimmunity, vasculopathy, excessive extracellular matrix deposition, and fibrosis, leading to atrophy of the skin, subcutaneous tissues, muscles, and internal organs, including the digestive system, lungs, heart, kidneys, and central nervous system (Yoshizaki, 2018). Multiple lines of evidence suggest that B cells play a significant role in the pathophysiology of severe systemic sclerosis. And CD19 was found to be significantly increased in expression in memory B cells and naive B cells in SSc (Beesley et al., 2022), so the current target of CAR-T clinical trials for the treatment of SSc is CD19.
The efficacy of the CD20 antibody-targeted drug rituximab (RTX) in the treatment of SSc remains controversial, with the use of rituximab demonstrating significant skin improvement in one randomized controlled trial, but it led to a decrease in forceful lung capacity (FVC) in another study (Ebata et al., 2021).
Changes in Treg populations were also observed in SSc patients, with a disruption of the balance between Treg and Th17 cells, an immune imbalance that has been implicated as a cause of the disease (Fenoglio et al., 2011; Frantz et al., 2018). As potential treatments for systemic sclerosis (SSc), therapies targeting Treg or Th17 cells are being explored with the aim of restoring the balance between these cell types (Liu et al., 2021). In systemic sclerosis (SSc), myelin basic protein (MBP) and myelin oligodendrocyte glycoprotein (MOG) can act as potential targets within the central nervous system (CNS). Human Tregs have been designed to express the Ob2F3 TCR V region to target myelin basic protein (MBP), and experiments suggest that these ob2f3-expressing Treg cells ameliorate experimental autoimmune encephalitis in transgenic mice (Ferreira et al., 2019). These significant findings indicate that engineered CAR-Tregs targeting the CNS have the potential to be developed for cellular therapy in patients with systemic sclerosis (SSc).
Reports have documented six cases of systemic sclerosis treated with CAR-T therapy, all originating from Germany (Wang X. et al., 2024; Bergmann et al., 2023; Müller et al., 2024; Subklewe et al., 1990). After CAR-T cell infusion, none of them experienced serious adverse effects, only mild cytokine release syndrome (CRS) grade 1, no signs of neurotoxicity, and all of them demonstrated autoantibody turnaround and rapid improvement of multisystemic manifestations of cardiac, joint, and cutaneous involvement in subsequent short-term follow-up, these data offer the initial evidence that targeted CD19 CAR-T cell therapy may be effective in treating severe cases of systemic sclerosis (Feng et al., 2022), but they do not fully clarify whether it is only a short-term effect of CAR-T cell therapy, and further observation of the extent of drug-free remission in SSc is warranted.
Idiopathic inflammatory myopathies (IIM) are a heterogeneous group of autoimmune disorders. Muscle weakness is usually the typical clinical presentation, but other organs may also be affected, including the skin, joints, lungs, heart, etc. Different myositis-specific auto-antibodies have been identified and IIM can be classified into several subgroups - dermatomyositis (including amyopathic dermatomyositis), antisynthetase syndrome, immune-mediated necrotizing myopathy, inclusion body myositis, polymyositis and overlap myositis (Lundberg et al., 2021). According to its pathogenesis, B-cell depletion shows promise in controlling the progression of the disease. Four patients with antisynthetase syndrome treated with CAR-T have been reported and all have shown favorable outcomes (Müller et al., 2023; Taubmann et al., 2024). Three of them developed low-grade CRS, including one with ICANS, and symptoms improved with tolizumab treatment. Longer-term follow-up and additional clinical trials are needed to assess the longer-term therapeutic profile of CAR-T in antisynthetase syndrome.
ANCA-associated vasculitis (AAV) encompasses a group of systemic autoimmune disorders marked by inflammation of small blood vessels and endothelial dysfunction. This condition can impact various organs and tissues, including the skin, lungs, kidneys, heart, gastrointestinal tract, and central nervous system. Based on clinical features and pathological manifestations, ANCA-associated vasculitis (AAV) can be categorized into three subgroups: granulomatosis with polyangiitis (GPA), microscopic polyangiitis (MPA), and eosinophilic granulomatosis with polyangiitis (EGPA) (Geetha and Jefferson, 2020). The pathogenesis of ANCA-associated vasculitis (AAV) remains not fully elucidated. However, Lodka et al. have demonstrated the feasibility and efficacy of CD19 CAR-T cell therapy for AAV treatment using a mouse model of MPO-AAV. Although myeloperoxidase (MPO)-ANCA was not completely eliminated, its levels were significantly reduced and demonstrated a strong protective effect against autoantibody-induced glomerulonephritis (Lodka et al., 2024). The application of this therapy for ANCA-associated vasculitis is currently restricted to vivo studies, and further data are needed to confirm its safety and efficacy.
Rheumatoid arthritis (RA) is a chronic, inflammatory, systemic autoimmune disease that affects the joints with severity that varies from patient to patient (Cush, 2022), with risk factors including age, gender, genetics, and environmental exposures (smoking, air pollutants, and occupation).
RA is presently incurable, and the goal of treatment is to reduce pain and stop or slow further damage. The pathogenesis and etiology of rheumatoid arthritis (RA) remain largely unknown. However, protein citrullination has long been identified as a key feature that triggers the immune response associated with this condition. The presence of anti-citrullinated protein antibodies (ACPAs) in serum is one of the most specific serological markers for rheumatoid arthritis (RA) and is closely linked to the disease’s development (Curran et al., 2020; Scherer et al., 2020). Previous studies have shown that RA patients are significantly heterogeneous and different patients can exhibit different types of autoantibodies. Zhang et al. demonstrated that anti-FITC CAR-Ts can be specifically targeted to tumor cells or autoreactive B cells of RA patients with high levels of autoantibodies by citrullinated peptide epitopes labeled with FITC (fluorescein isothiocyanate), and that this approach can be used according to the individual characteristics of a given RA patient to eliminate the respective specific subpopulation of autoreactive cells. This opens up a new field of targeted therapy for autoimmune diseases (Zhang et al., 2021). However, the main limitation of this study is that it only demonstrated that it is possible to eliminate auto-reactive B cells in vitro and lacks evidence of therapeutic efficacy in vivo. Therefore, its feasibility and safety in vivo are unknown and need to be further explored in a large number of experiments.
In addition, citrullinated waveform protein (CV) has been identified as a specific antigen found exclusively in the extracellular matrix of inflamed synovial tissues in RA patients and has been suggested as a potential target for CAR-T cell therapy (Van Steendam et al., 2010). Preliminary data suggest that CAR-Tregs targeting citrullinated waveform protein (CV) may play a role in RA, but preclinical manifestations are not yet clear (Orvain et al., 2021).
A search of clinicaltrials.gov was performed for currently ongoing clinical trials of CAR-T for the treatment of SLE, SS, SSc, AAV, and RA. (Search date 2024.7.15, with no results found for ASS) (Table 2).
While CAR-T therapy has demonstrated significant efficacy in treating both hematologic and solid tumors, it continues to encounter several challenges (Figure 2).
Figure 2. Current challenges and strategies for CAR-T treatment of autoimmune diseases at various stages of treatment.
Autologous CAR-T cell therapy involves a highly complex process of customizing CAR-T cells following the isolation of each patient’s white blood cells. This procedure typically requires approximately 3 weeks to complete (Iyer et al., 2018; Köhl et al., 2018). In response to the problem of long in vitro manufacturing time, universal CAR-T (UCAR-T) has been proposed, which is taken from T cells of healthy donors, exempted from the action of heterologous reactive T cells by knocking out genes such as TCR, CD52, CD7, etc., and can be infused back in the spot without waiting, and the large-scale production can significantly reduce the price, which greatly improves the accessibility of CAR-T products (Lin et al., 2021), and this approach is expected to break the dilemma.
During the time that autologous CAR-T is being manufactured in vitro, a number of patients will experience disease activity as a result of discontinuation of the drug and thus become ineligible for treatment. It may be possible to continue maintenance therapy with low-dose drugs during this period, and in addition, cyclophosphamide and fudarabine, which are used to clear lymphocytes, may have some therapeutic effect [(Green and Schultz, 2023; Srivastava et al., 2021)].
Immune cells from healthy donors are usually unaffected by cancer effects or exposure to drugs, whereas in tumor patients, which may be related to immunosuppression, the tumor microenvironment, or having undergone multiple lines of therapy, T-cell dysfunction has been observed in tumor patients in the past, and these aberrant T-cells may affect the efficacy of CAR-T therapy (Park et al., 2018; Thommen and Schumacher, 2018). Given that patients with autoimmune diseases are frequently treated with glucocorticoids and other medications such as mycophenolate mofetil or cyclophosphamide, which can impact the quality and quantity of T cells, it is crucial to consider these factors when evaluating CAR-T therapy (Schulze-Koops, 2004). In a clinical study involving CAR-T therapy for five patients with severe, treatment-resistant systemic lupus erythematosus, stable and reproducible production of CD19CAR-T cells from peripheral blood was successfully achieved. This outcome occurred despite the patients having been pre-treated with T-cell-targeting medications, including mycophenolate mofetil and glucocorticoids, as part of their disease management (Mackensen et al., 2022).
The high cost of CAR-T therapy is a significant barrier for many patients (de Lima Lopes and Nahas, 2018), largely due to the complexity of the manufacturing process, which involves genetically modifying T cells, tailoring the treatment to each patient, implementing stringent quality-control measures, and requiring specialized facilities for cell production and processing (Levine et al., 2017; Choi et al., 2022). Back in 2017, there were two approved CAR-T drugs available in the United States: Kymriah and Yescarta. Kymriah has a listed price of US$475,000 and Yescarta has a listed price of US$373,000, and that doesn't even include the cost of possible side effects of the treatment, which is why it's currently only used to treat patients with particularly severe autoimmune diseases. Ideally, however, if long-term drug-free remission can be achieved with CAR-T therapy, the cost may be balanced against the cost of long-term repeated use of hormones, immunosuppressants, and biologics to control the disease. In addition to UCAR-T mentioned above, in situ cellular reprogramming, a potentially groundbreaking technology, also opens up possibilities to reduce costs and simplify the acquisition process (Parayath and Stephan, 2021). It targets and reprograms T cells in vivo to express CARs without the need for any in vitro manufacturing, thus greatly reducing costs, simplifying the acquisition process, and increasing availability. T-cell-targeted mRNA lipid nanoparticles (LNPs) have recently been found to deliver in vivo coding in mice, allowing the generation of functional CAR-T cells in vivo.
Rurik et al. (2022). This study demonstrated that a single injection of an off-the-shelf product can effectively reprogram the immune system, a technology that takes CAR-T cell therapy another step toward reducing costs and increasing accessibility.
Although autoimmune diseases can be severe enough to cause death, the mortality rate is significantly lower than that of patients with malignant hematologic tumors. Therefore, the safety of the new treatment modality is the most concerned point for patients and doctors besides the efficacy. After the administration of CAR-T cells, their recognition of target cells triggers activation and proliferation, leading to the destruction of these target cells and the release of various cytokines, including IL-1, IL-2, IL-6, IL-8, and IL-10. The released cytokines further activate the patient’s immune cells, including T cells, B cells, monocytes, and macrophages, as well as non-immune cells such as endothelial cells. This cascade results in the release of additional cytokines, establishing a positive feedback loop known as cytokine release syndrome (CRS), which can escalate into a systemic inflammatory response with the potential to cause significant tissue and organ damage. Experiments have demonstrated that IL-6 plays a pivotal role in mediating cytokine release syndrome (CRS) (Morris et al., 2022; Garcia et al., 2021; Porter et al., 2011). The incidence of CRS is notably high in clinical trials targeting hematologic malignancies (Bouchkouj et al., 2019; Leahy et al., 2018). However, in CAR-T cell therapy trials for autoimmune diseases, no reports of grade 3 or higher CRS have been observed (Table 3). The good tolerability of CAR-T cell therapy in autoimmune diseases may be due to the fact that the amount of b-cells in patients with autoimmune diseases is many times lower than in patients with tumors. Therefore, cells carrying the target antigen (CD19) are quickly removed.
Additionally, some patients with acute lymphoblastic leukemia experience severe neurotoxicity, referred to as immune effector cell-associated neurotoxicity syndrome (ICANS), following CAR-T cell therapy (Santomasso et al., 2018). However, in the limited number of patients with autoimmune diseases currently undergoing CAR-T cell therapy, we have observed a relatively favorable tolerability profile, with no serious adverse effects reported (Table 3). Although some patients experienced a mild cytokine storm, this was effectively managed with prompt symptomatic treatment and tolizumab administration.
Hematotoxicity, which includes manifestations such as anemia, leukopenia, and thrombocytopenia (Fried et al., 2019), may occur early due to lymphocyte depletion before CAR-T therapy. Its late onset, however, is often associated with the severity of cytokine release syndrome (CRS) during treatment. This phenomenon has been observed in the management of both oncological and autoimmune diseases (Schett et al., 2023). Therefore, we need to closely monitor the changes in blood routine during the treatment period, and promptly transfuse component blood when necessary.
Off-target effects, where CAR-T cells either cross-react with non-target molecules or target antigens expressed on normal cells, are more commonly observed in tumor therapies (Castellarin et al., 2020; Chohan et al., 2023). These phenomena have not been reported in clinical trials of CAR-T therapy for autoimmune diseases. However, careful monitoring is essential to detect their potential occurrence during treatment.
Patients undergoing CAR-T cell therapy face an elevated risk of infection due to several factors, including immunodeficiency from their underlying disease, prior cytotoxic treatments, lymphodepleting therapy, potential complications such as CRS and ICANS, as well as neutropenia and hypogammaglobulinemia. Infectious complications, both early and late, are commonly observed following CAR-T cell administration in the treatment of hematologic malignancies (An et al., 2024). However, such complications have not been specifically reported in the context of autoimmune diseases. Evidence-based guidelines for anti-infective prophylaxis following CAR-T cell therapy are lacking, and routine use of fungal and bacterial prophylaxis is uncommon. However, it should be actively considered in patients with neutropenia.
A critical feature of CAR-T therapy is its capacity to identify target antigens and exert cytotoxic effects. When B cells proliferate again it may lead to disease relapse. However, a recent study found that after CAR-T treatment for autoimmune disease, newly emerged B cells predominantly expressed IgM and IgD, while memory B cells and plasmablasts were nearly absent. IgD is often expressed in relatively naïve B cells, including naïve B cells (IgD + CD27−), unswitched memory B cells (IgD+CD27+), So the phenomenon may be related to inflammatory suppression, deep B-cell clearance and subsequent reconstitution. (Schett et al., 2023; Shah et al., 2024).
CAR transgenes are integrated into the genome of T cells primarily through viruses, a process that may lead to tumors. Secondary malignancies have been reported after CAR T-cell therapy, including myelodysplastic syndromes, bladder cancer, or nonmelanoma skin cancers (Locke et al., 2019; Si Lim et al., 2021), therefore, there have been attempts to use vectors and gene editing techniques other than retroviruses and lentiviruses to create a safer product, with adeno-associated viruses and targeted nucleases offering promise (Moço et al., 2020).
Current clinical trials using CAR-T therapies have focused on removing B-cell markers such as CD19 or BCMA, and prolonged low levels of B-cells are often observed after CAR-T treatment of blood disorders, leaving patients susceptible to symptoms such as infections (An et al., 2024), and targeting infections that occur as a result of this B-cell dysplasia can be achieved with intravenous immune globulin. However, this has not been observed in autoimmune diseases for the moment (Schett et al., 2023) (Table 3). This regeneration of B cells is also a major point of difference in comparison to CAR-T therapy for tumors, the mechanism of which is currently unclear. Possible reasons for this include the fact that, unlike cancer patients, patients with autoimmune diseases receive fewer cytotoxic drugs, so stem cells and immune cells may be less affected (Schett et al., 2023). This may also be related to the distinct cellular niches for memory.
CAR T cells. The location of memory CAR-T cells is importantly linked to their persistence and B-cell reset. The earlier appearance of B-cell proliferation in lupus patients may be due to the fact that CAR T cells are located in secondary lymphoid organs, whereas in leukaemia memory CAR T cells are located in the bone marrow (Baker and June 2022). However, the proliferation of B-cells is theoretically incompatible with the long-term efficacy of CAR-t therapy, so a large number of clinical trials are needed to assess the long-term efficacy of CAR-T in autoimmune diseases.
As mentioned above, CAR-T has been explored for the treatment of a variety of autoimmune diseases, with current clinical trials showing good efficacy and a fair safety profile compared to tumors. However, there are some challenges that we need to continue to work to overcome before, during and after its treatment.
CAR-T therapy has shown remarkable success in the treatment of hematological diseases and solid tumors, and as an emerging therapy in autoimmune diseases, it has also demonstrated certain potential. However, the number of patients with autoimmune diseases who have received this therapy is not large enough, the follow-up period is short, and the relevant clinical data are still scarce. So the longer-term efficacy and safety are still unclear. We expect that it will become a mature therapy for autoimmune diseases in the future.
We searched PubMed and Web of science for articles published from From 1 January 2010 to 30 September 2024, using the search terms ‘CAR T cell’ and ‘autoimmune disease’ or ‘Rheumatic Autoimmune Disease’. We also searched for articles linked to the terms ‘B cell depletion’. We restricted our search to articles published in English. We included clinical studies, mechanistic studies, case reports, and case series, as well as reviews on CAR T-cell therapy into our references. Articles related to autologous and allogenic stem cell transplantation in autoimmune diseases, were also included.
QG: Writing–original draft, Writing–review and editing. JL: Writing–original draft. JW: Writing–review and editing. LL: Writing–original draft. JW: Writing–review and editing. LZ: Funding acquisition, Supervision, Writing–review and editing.
The author(s) declare that financial support was received for the research, authorship, and/or publication of this article. This article was funding by Health Commission of Shanxi Province research project (project number: 2018014); Shanxi Province Clinical Research Center for Dermatologic and Immunologic Diseases (Rheumatic diseases) (project number: LYZX-202301).
We thank all authors who participated in the study of The Advent of Chimeric Antigen Receptor T Cell Therapy in Recalibrating Immune balance for Autoimmune Disease Treatment.
The authors declare that the research was conducted in the absence of any commercial or financial relationships that could be construed as a potential conflict of interest.
The author(s) declare that no Generative AI was used in the creation of this manuscript.
All claims expressed in this article are solely those of the authors and do not necessarily represent those of their affiliated organizations, or those of the publisher, the editors and the reviewers. Any product that may be evaluated in this article, or claim that may be made by its manufacturer, is not guaranteed or endorsed by the publisher.
Adabi, N., Pordel, S., Rezaee, M. A., Shobeiri, F. S., and Shobeiri, S. S. (2023). Application of CAR-T cell technology in autoimmune diseases and human immunodeficiency virus infection treatment. J. Gene Med. 25 (5), e3484. doi:10.1002/jgm.3484
Adami, G., Fassio, A., Rossini, M., Caimmi, C., Giollo, A., Orsolini, G., et al. (2019). Osteoporosis in rheumatic diseases. Int. J. Mol. Sci. 20 (23), 5867. doi:10.3390/ijms20235867
Ameer, M. A., Chaudhry, H., Mushtaq, J., Khan, O. S., Babar, M., Hashim, T., et al. (2022). An overview of systemic lupus erythematosus (SLE) pathogenesis, classification, and management. Cureus 14 (10), e30330. doi:10.7759/cureus.30330
An, J., Zhao, J., Zou, P., Zhang, Y., Wei, J., Tian, W., et al. (2024). Infections associated with CAR-T cell therapy in patients with relapsed refractory multiple myeloma: risks and prevention strategies. Cancer Med. 13 (12), e7372. doi:10.1002/cam4.7372
Baker, D. J., Arany, Z., Baur, J. A., Epstein, J. A., and June, C. H. (2023). CAR T therapy beyond cancer: the evolution of a living drug. Nature 619 (7971), 707–715. doi:10.1038/s41586-023-06243-w
Baker, D. J., and June, C. H. (2022). CAR T therapy extends its reach to autoimmune diseases. Cell. 185 (24), 4471–4473. doi:10.1016/j.cell.2022.10.026
Baker, D. J., and June, C. H. (2024). Off-the-shelf CAR-T cells could prove paradigm shifting for autoimmune diseases. Cell 187 (18), 4826–4828. doi:10.1016/j.cell.2024.07.056
Beesley, C. F., Goldman, N. R., Taher, T. E., Denton, C. P., Abraham, D. J., Mageed, R. A., et al. (2022). Dysregulated B cell function and disease pathogenesis in systemic sclerosis. Front. Immunol. 13, 999008. doi:10.3389/fimmu.2022.999008
Bergmann, C., Müller, F., Distler, J. H. W., Györfi, A. H., Völkl, S., Aigner, M., et al. (2023). Treatment of a patient with severe systemic sclerosis (SSc) using CD19-targeted CAR T cells. Ann. Rheum. Dis. 82 (8), 1117–1120. doi:10.1136/ard-2023-223952
Bongartz, T., Cantaert, T., Atkins, S. R., Harle, P., Myers, J. L., Turesson, C., et al. (2007). Citrullination in extra-articular manifestations of rheumatoid arthritis. Rheumatology. 46 (1), 70–75. doi:10.1093/rheumatology/kel202
Bouchkouj, N., Kasamon, Y. L., de Claro, R. A., George, B., Lin, X., Lee, S., et al. (2019). FDA approval summary: axicabtagene ciloleucel for relapsed or refractory large B-cell lymphoma. Clin. Cancer Res. Off. J. Am. Assoc. Cancer Res. 25 (6), 1702–1708. doi:10.1158/1078-0432.CCR-18-2743
Boulch, M., Cazaux, M., Loe-Mie, Y., Thibaut, R., Corre, B., Lemaître, F., et al. (2021). A cross-talk between CAR T cell subsets and the tumor microenvironment is essential for sustained cytotoxic activity. Sci. Immunol. 6 (57), eabd4344. doi:10.1126/sciimmunol.abd4344
Boulougoura, A., Gendelman, H., Surmachevska, N., and Kyttaris, V. C. (2023). Journal club: anti-CD19 chimeric antigen receptor T cell therapy for refractory systemic lupus erythematosus. ACR Open Rheumatol. 5 (11), 624–628. doi:10.1002/acr2.11614
Castellarin, M., Sands, C., Da, T., Scholler, J., Graham, K., Buza, E., et al. (2020). A rational mouse model to detect on-target, off-tumor CAR T cell toxicity. JCI Insight 5 (14), e136012. doi:10.1172/jci.insight.136012
Cataldi, M., Vigliotti, C., Mosca, T., Cammarota, M., and Capone, D. (2017). Emerging role of the spleen in the pharmacokinetics of monoclonal antibodies, nanoparticles and exosomes. J. Turbul. 18 (6), 1249. doi:10.3390/ijms18061249Available at: https://www.zhangqiaokeyan.com/journal-foreign-detail/0704025951512.html Accessed May 6, 2024.
Chen, Y. J., Abila, B., and Mostafa Kamel, Y. (2023). CAR-T: what is next? Cancers (Basel) 15 (3), 663. doi:10.3390/cancers15030663
Chohan, K. L., Siegler, E. L., and Kenderian, S. S. (2023). CAR-T cell therapy: the efficacy and toxicity balance. Curr. Hematol. Malig. Rep. 18 (2), 9–18. doi:10.1007/s11899-023-00687-7
Choi, G., Shin, G., and Price, B. S. (2022). Prejudice? The value of chimeric antigen receptor (CAR) T-cell therapy. Int. J. Environ. Res. Public Health 19 (19), 12366. doi:10.3390/ijerph191912366
Crotty, S. (2011). Follicular helper CD4 T cells (TFH). Annu. Rev. Immunol. 29, 621–663. doi:10.1146/annurev-immunol-031210-101400
Curran, A. M., Naik, P., Giles, J. T., and Darrah, E. (2020). PAD enzymes in rheumatoid arthritis: pathogenic effectors and autoimmune targets. Nat. Rev. Rheumatol. 16 (6), 301–315. doi:10.1038/s41584-020-0409-1
Cush, J. J. (2022). Rheumatoid arthritis: early diagnosis and treatment. Rheum. Dis. Clin. North Am. 48 (2), 537–547. doi:10.1016/j.rdc.2022.02.010
Daridon, C., Pers, J. O., Devauchelle, V., Martins-Carvalho, C., Hutin, P., Pennec, Y. L., et al. (2006). Identification of transitional type II B cells in the salivary glands of patients with Sjögren’s syndrome. Arthritis Rheum. 54 (7), 2280–2288. doi:10.1002/art.21936
de Lima Lopes, G., and Nahas, G. R. (2018). Chimeric antigen receptor T cells, a savior with a high price. Chin. Clin. Oncol. 7 (2), 21. doi:10.21037/cco.2018.04.02
Ebata, S., Yoshizaki, A., Oba, K., Kashiwabara, K., Ueda, K., Uemura, Y., et al. (2021). Safety and efficacy of rituximab in systemic sclerosis (DESIRES): a double-blind, investigator-initiated, randomised, placebo-controlled trial. Lancet Rheumatol. 3 (7), e489–e497. doi:10.1016/S2665-9913(21)00107-7
Feng, J., Hu, Y. X., Zhang, M., Cao, H., Ke, Y., Xu, H., et al. (2022). Safety and efficacy of CD19 CAR-T cells for refractory systemic sclerosis: a phase I clinical trial. Blood 140, 10335–10336. doi:10.1182/blood-2022-169265Available at: http://www.semanticscholar.org/paper/df4ddbc94efe55fa1aa86a36cf7bfeb1433a5386 Accessed December 21, 2023.
Fenoglio, D., Battaglia, F., Parodi, A., Stringara, S., Negrini, S., Panico, N., et al. (2011). Alteration of Th17 and Treg cell subpopulations co-exist in patients affected with systemic sclerosis. Clin. Immunol. Orl. Fla 139 (3), 249–257. doi:10.1016/j.clim.2011.01.013
Ferreira, L. M. R., Muller, Y. D., Bluestone, J. A., and Tang, Q. (2019). Next-generation regulatory T cell therapy. Nat. Rev. Drug Discov. 18 (10), 749–769. doi:10.1038/s41573-019-0041-4
Finnegan, A., Ashaye, S., and Hamel, K. M. (2012). B effector cells in rheumatoid arthritis and experimental arthritis. Autoimmunity 45 (5), 353–363. doi:10.3109/08916934.2012.665526
Frantz, C., Auffray, C., Avouac, J., and Allanore, Y. (2018). Regulatory T cells in systemic sclerosis. Front. Immunol. 9, 2356. doi:10.3389/fimmu.2018.02356
Fried, S., Avigdor, A., Bielorai, B., Meir, A., Besser, M. J., Schachter, J., et al. (2019). Early and late hematologic toxicity following CD19 CAR-T cells. Bone Marrow Transpl. 54 (10), 1643–1650. doi:10.1038/s41409-019-0487-3
Fukasawa, T., Yoshizaki-Ogawa, A., Sato, S., and Yoshizaki, A. (2024). The role of B cells in systemic sclerosis. J. Dermatol 51 (7), 904–913. doi:10.1111/1346-8138.17134
Garcia, B. J., Heindel, K., Kochanek, M., Warnke, C., Stemmler, J., von Bergwelt-Baildon, M., et al. (2021). The critically ill CAR T-cell patient: relevant toxicities, their management and challenges in critical care. Med. Klin. Intensivmed. Notfallmedizin 116 (2), 121–128.
Geetha, D., and Jefferson, J. A. (2020). ANCA-associated vasculitis: core curriculum 2020. Am. J. Kidney Dis. Off. J. Natl. Kidney Found. 75 (1), 124–137. doi:10.1053/j.ajkd.2019.04.031
Green, S., and Schultz, L. (2023). Rational alternatives to fludarabine and cyclophosphamide-based pre-CAR lymphodepleting regimens in the pediatric and young adult B-all setting. Curr. Oncol. Rep. 25 (8), 841–846. doi:10.1007/s11912-023-01404-6
Huang, J., Fu, X., Chen, X., Li, Z., Huang, Y., and Liang, C. (2021). Promising therapeutic targets for treatment of rheumatoid arthritis. Front. Immunol. 12, 686155. doi:10.3389/fimmu.2021.686155
Iyer, R. K., Bowles, P. A., Kim, H., and Dulgar-Tulloch, A. (2018). Industrializing autologous adoptive immunotherapies: manufacturing advances and challenges. Front. Med. 5, 150. doi:10.3389/fmed.2018.00150
Jin, X., Xu, Q., Pu, C., Zhu, K., Lu, C., Jiang, Y., et al. (2021). Therapeutic efficacy of anti-CD19 CAR-T cells in a mouse model of systemic lupus erythematosus. Cell Mol. Immunol. 18 (8), 1896–1903. doi:10.1038/s41423-020-0472-1
Kansal, R., Richardson, N., Neeli, I., Khawaja, S., Chamberlain, D., Ghani, M., et al. (2019). Sustained B cell depletion by CD19-targeted CAR T cells is a highly effective treatment for murine lupus. Sci. Transl. Med. 11 (482), eaav1648. doi:10.1126/scitranslmed.aav1648Available at: http://www.socolar.com/Article/Index?aid=100019748401&jid=100000008294 Accessed September 6, 2024.
Kim, Y. C., Zhang, A. H., Su, Y., Rieder, S. A., Rossi, R. J., Ettinger, R. A., et al. (2015). Engineered antigen-specific human regulatory T cells: immunosuppression of FVIII-specific T- and B-cell responses. Blood 125 (7), 1107–1115. doi:10.1182/blood-2014-04-566786
Klichinsky, M., Ruella, M., Shestova, O., Lu, X. M., Best, A., Zeeman, M., et al. (2020). Human chimeric antigen receptor macrophages for cancer immunotherapy. Nat. Biotechnol. 38 (8), 947–953. doi:10.1038/s41587-020-0462-y
Kochenderfer, J. N., Dudley, M. E., Kassim, S. H., Somerville, R. P. T., Carpenter, R. O., Stetler-Stevenson, M., et al. (2015). Chemotherapy-refractory diffuse large B-cell lymphoma and indolent B-cell malignancies can be effectively treated with autologous T cells expressing an anti-CD19 chimeric antigen receptor. J. Clin. Oncol. Off. J. Am. Soc. Clin. Oncol. 33 (6), 540–549. doi:10.1200/JCO.2014.56.2025
Köhl, U., Arsenieva, S., Holzinger, A., and Abken, H. (2018). CAR T cells in trials: recent achievements and challenges that remain in the production of modified T cells for clinical applications. Hum. Gene Ther. 29 (5), 559–568. doi:10.1089/hum.2017.254
Krickau, T., Naumann-Bartsch, N., Aigner, M., Kharboutli, S., Kretschmann, S., Spoerl, S., et al. (2024). CAR T-cell therapy rescues adolescent with rapidly progressive lupus nephritis from haemodialysis. Lancet Lond Engl. 403 (10437), 1627–1630. doi:10.1016/S0140-6736(24)00424-0
Krustev, E., Clarke, A. E., and Barber, M. R. W. (2023). B cell depletion and inhibition in systemic lupus erythematosus. Expert Rev. Clin. Immunol. 19 (1), 55–70. doi:10.1080/1744666X.2023.2145281
Leahy, A. B., Elgarten, C. W., Grupp, S. A., Maude, S. L., and Teachey, D. T. (2018). Tisagenlecleucel for the treatment of B-cell acute lymphoblastic leukemia. Expert Rev. Anticancer Ther. 18 (10), 959–971. doi:10.1080/14737140.2018.1512411
Lee, D. S. W., Rojas, O. L., and Gommerman, J. L. (2020). B cell depletion therapies in autoimmune disease: advances and mechanistic insights. Nat. Rev. Drug Discov. (Suppl. 16), 179–199. doi:10.1038/s41573-020-00092-2Available at: https://www.nstl.gov.cn/paper_detail.html?id=ba32794c0308a6cece8dd7b9b3b721ae Accessed August 11, 2024.
Levine, B. L., Miskin, J., Wonnacott, K., and Keir, C. (2017). Global manufacturing of CAR T cell therapy. Mol. Ther. Methods Clin. Dev. 4, 92–101. doi:10.1016/j.omtm.2016.12.006
Li, M., Zhang, Y., Jiang, N., Ning, C., Wang, Q., Xu, D., et al. (2024). Anti-CD19 CAR T cells in refractory immune thrombocytopenia of SLE. N. Engl. J. Med. 391 (4), 376–378. doi:10.1056/NEJMc2403743
Lin, H., Cheng, J., Mu, W., Zhou, J., and Zhu, L. (2021). Advances in universal CAR-T cell therapy. Front. Immunol. 12, 744823. doi:10.3389/fimmu.2021.744823
Lin, Y. J., Anzaghe, M., and Schülke, S. (2020). Update on the pathomechanism, diagnosis, and treatment options for rheumatoid arthritis. Cells 9 (4), 880. doi:10.3390/cells9040880
Liu, X., Wu, Y., Li, M., Hao, J., and Zeng, X. (2021). Plasticity of Treg and imbalance of Treg/Th17 cells in patients with systemic sclerosis modified by FK506. Int. J. Immunopathol. Pharmacol. 35, 2058738421998086. doi:10.1177/2058738421998086
Locke, F. L., Ghobadi, A., Jacobson, C. A., Miklos, D. B., Lekakis, L. J., Oluwole, O. O., et al. (2019). Long-term safety and activity of axicabtagene ciloleucel in refractory large B-cell lymphoma (ZUMA-1): a single-arm, multicentre, phase 1-2 trial. Lancet Oncol. 20 (1), 31–42. doi:10.1016/S1470-2045(18)30864-7
Lodka, D., Zschummel, M., Bunse, M., Rousselle, A., Sonnemann, J., Kettritz, R., et al. (2024). CD19-targeting CAR T cells protect from ANCA-induced acute kidney injury. Ann. Rheum. Dis. 83 (4), 499–507. doi:10.1136/ard-2023-224875
Lundberg, I. E., Fujimoto, M., Vencovsky, J., Aggarwal, R., Holmqvist, M., Christopher-Stine, L., et al. (2021). Idiopathic inflammatory myopathies. Nat. Rev. Dis. Primer 7 (1), 86. doi:10.1038/s41572-021-00321-x
Lundberg, I. E., Galindo-Feria, A. S., and Horuluoglu, B. (2023). CD19-Targeting CAR T-cell therapy for antisynthetase syndrome. JAMA 329 (24), 2130–2131. doi:10.1001/jama.2023.7240
Mackensen, A., Müller, F., Mougiakakos, D., Böltz, S., Wilhelm, A., Aigner, M., et al. (2022). Anti-CD19 CAR T cell therapy for refractory systemic lupus erythematosus. Nat. Med. 28 (10), 2124–2132. doi:10.1038/s41591-022-02017-5
Manfrè, V., Chatzis, L. G., Cafaro, G., Fonzetti, S., Calvacchi, S., Fulvio, G., et al. (2022). Sjögren’s syndrome: one year in review 2022. Clin. Exp. Rheumatol. 40 (12), 2211–2224. doi:10.55563/clinexprheumatol/43z8gu
McClure, M., Gopaluni, S., Jayne, D., and Jones, R. (2018). B cell therapy in ANCA-associated vasculitis: current and emerging treatment options. Nat. Rev. Rheumatol. 14 (10), 580–591. doi:10.1038/s41584-018-0065-x
Möckel, T., Basta, F., Weinmann-Menke, J., and Schwarting, A. (2021). B cell activating factor (BAFF): structure, functions, autoimmunity and clinical implications in Systemic Lupus Erythematosus (SLE). Autoimmun. Rev. 20 (2), 102736. doi:10.1016/j.autrev.2020.102736
Moço, P. D., Aharony, N., and Kamen, A. (2020). Adeno-associated viral vectors for homology-directed generation of CAR-T cells. Biotechnol. J. 15 (1), e1900286. doi:10.1002/biot.201900286
Morris, E. C., Neelapu, S. S., Giavridis, T., and Sadelain, M. (2022). Cytokine release syndrome and associated neurotoxicity in cancer immunotherapy. Nat. Rev. Immunol. 22 (2), 85–96. doi:10.1038/s41577-021-00547-6
Morrissey, M. A., Williamson, A. P., Steinbach, A. M., Roberts, E. W., Kern, N., Headley, M. B., et al. (2018). Chimeric antigen receptors that trigger phagocytosis. eLife 7, e36688. doi:10.7554/eLife.36688
Mougiakakos, D., Krönke, G., Völkl, S., Kretschmann, S., Aigner, M., Kharboutli, S., et al. (2021). CD19-Targeted CAR T cells in refractory systemic lupus erythematosus. N. Engl. J. Med. 385 (6), 567–569. doi:10.1056/NEJMc2107725
Müller, F., Taubmann, J., Bucci, L., Wilhelm, A., Bergmann, C., Völkl, S., et al. (2024). CD19 CAR T-cell therapy in autoimmune disease - a case series with follow-up. N. Engl. J. Med. 390 (8), 687–700. doi:10.1056/NEJMoa2308917
Müller, F., Boeltz, S., Knitza, J., Aigner, M., Völkl, S., Kharboutli, S., et al. (2023). CD19-targeted CAR T cells in refractory antisynthetase syndrome. Lancet Lond Engl. 401 (10379), 815–818. doi:10.1016/s0140-6736(23)00023-5Available at: https://pubmed.ncbi.nlm.nih.gov/36930673/ Accessed September 3, 2024.
Nicolai, C. J., Parker, M. H., Qin, J., Tang, W., Ulrich-Lewis, J. T., Gottschalk, R. J., et al. (2024). In vivo CAR T-cell generation in nonhuman primates using lentiviral vectors displaying a multidomain fusion ligand. Blood 144 (9), 977–987. doi:10.1182/blood.2024024523
Nocturne, G., and Mariette, X. (2018). B cells in the pathogenesis of primary Sjögren syndrome. Nat. Rev. Rheumatol. 14 (3), 133–145. doi:10.1038/nrrheum.2018.1
Obstfeld, A. E., Frey, N. V., Mansfield, K., Lacey, S. F., June, C. H., Porter, D. L., et al. (2017). Cytokine release syndrome associated with chimeric-antigen receptor T-cell therapy: clinicopathological insights. Blood 130 (23), 2569–2572. doi:10.1182/blood-2017-08-802413
Oh, S., and Payne, A. S. (2022). Engineering cell therapies for autoimmune diseases: from preclinical to clinical proof of concept. Immune Netw. 22 (5), e37. doi:10.4110/in.2022.22.e37
Orvain, C., Boulch, M., Bousso, P., Allanore, Y., and Avouac, J. (2021). Is there a place for chimeric antigen receptor-T cells in the treatment of chronic autoimmune rheumatic diseases? Arthritis Rheumatol. Hob. N. J. 73 (11), 1954–1965. doi:10.1002/art.41812
Parayath, N. N., and Stephan, M. T. (2021). In situ programming of CAR T cells. Annu. Rev. Biomed. Eng. 23, 385–405. doi:10.1146/annurev-bioeng-070620-033348
Park, J. H., Rivière, I., Gonen, M., Wang, X., Sénéchal, B., Curran, K. J., et al. (2018). Long-term follow-up of CD19 CAR therapy in acute lymphoblastic leukemia. N. Engl. J. Med. 378 (5), 449–459. doi:10.1056/NEJMoa1709919
Pecher, A. C., Hensen, L., Klein, R., Schairer, R., Lutz, K., Atar, D., et al. (2023). CD19-Targeting CAR T cells for myositis and interstitial lung disease associated with antisynthetase syndrome. JAMA 329 (24), 2154–2162. doi:10.1001/jama.2023.8753
Porter, D. L., Levine, B. L., Kalos, M., Bagg, A., and June, C. H. (2011). Chimeric antigen receptor-modified T cells in chronic lymphoid leukemia. N. Engl. J. Med. 365 (8), 725–733. doi:10.1056/NEJMoa1103849
Qin, C., Dong, M. H., Zhou, L. Q., Wang, W., Cai, S. B., You, Y. F., et al. (2024). Single-cell analysis of refractory anti-SRP necrotizing myopathy treated with anti-BCMA CAR-T cell therapy. Proc. Natl. Acad. Sci. U. S. A. 121 (6), e2315990121. doi:10.1073/pnas.2315990121
Qin, C., Tian, D. S., Zhou, L. Q., Shang, K., Huang, L., Dong, M. H., et al. (2023). Anti-BCMA CAR T-cell therapy CT103A in relapsed or refractory AQP4-IgG seropositive neuromyelitis optica spectrum disorders: phase 1 trial interim results. Signal Transduct. Target Ther. 8 (1), 5. doi:10.1038/s41392-022-01278-3
Qin, L., Zhang, Y., Yang, X., Luo, Q., and Wang, H. (2022). Cardiac involvement in primary Sjӧgren's syndrome. Rheumatol. Int. 42 (2), 179–189. doi:10.1007/s00296-021-04970-9
Radu, A. F., and Bungau, S. G. (2021). Management of rheumatoid arthritis: an overview. Cells 10 (11), 2857. doi:10.3390/cells10112857
Reddy, V., Cambridge, G., Isenberg, D. A., Glennie, M. J., Cragg, M. S., and Leandro, M. (2015). Internalization of rituximab and the efficiency of B Cell depletion in rheumatoid arthritis and systemic lupus erythematosus. Arthritis Rheumatol. 67 (8), 2046–2055. doi:10.1002/art.39167
Reighard, S. D., Cranert, S. A., Rangel, K. M., Ali, A., Gyurova, I. E., de la Cruz-Lynch, A. T., et al. (2020). Therapeutic targeting of follicular T cells with chimeric antigen receptor-expressing natural killer cells. Cell Rep. Med. 1 (1), 100003. doi:10.1016/j.xcrm.2020.100003
Rivière, E., Pascaud, J., Virone, A., Dupré, A., Ly, B., Paoletti, A., et al. (2021). Interleukin-7/Interferon Axis drives T cell and salivary gland epithelial cell interactions in sjögren’s syndrome. Arthritis Rheumatol. Hob. N. J. 73 (4), 631–640. doi:10.1002/art.41558
Rurik, J. G., Tombácz, I., Yadegari, A., Méndez Fernández, P. O., Shewale, S. V., Li, L., et al. (2022). CAR T cells produced in vivo to treat cardiac injury. Science 375 (6576), 91–96. doi:10.1126/science.abm0594
Santomasso, B. D., Park, J. H., Salloum, D., Riviere, I., Flynn, J., Mead, E., et al. (2018). Clinical and biological correlates of neurotoxicity associated with CAR T-cell therapy in patients with B-cell acute lymphoblastic leukemia. Cancer Discov. 8 (8), 958–971. doi:10.1158/2159-8290.CD-17-1319
Scherer, H. U., Häupl, T., and Burmester, G. R. (2020). The etiology of rheumatoid arthritis. J. Autoimmun. 110, 102400. doi:10.1016/j.jaut.2019.102400
Schett, G., Mackensen, A., and Mougiakakos, D. (2023). CAR T-cell therapy in autoimmune diseases. Lancet Lond Engl. 402 (10416), 2034–2044. doi:10.1016/S0140-6736(23)01126-1
Schulze-Koops, H. (2004). Lymphopenia and autoimmune diseases. Arthritis Res. Ther. 6 (4), 178–180. doi:10.1186/ar1208
Shah, K., Leandro, M., Cragg, M., Kollert, F., Schuler, F., Klein, C., et al. (2024). Disrupting B and T-cell collaboration in autoimmune disease: T-cell engagers versus CAR T-cell therapy? Clin. Exp. Immunol. 217 (1), 15–30. doi:10.1093/cei/uxae031
Sheng, L., Zhang, Y., Song, Q., Jiang, X., Cao, W., Li, L., et al. (2023). Concurrent remission of lymphoma and Sjögren’s disease following anti-CD19 chimeric antigen receptor-T cell therapy for diffuse large B-cell lymphoma: a case report. Front. Immunol. 14, 1298815. doi:10.3389/fimmu.2023.1298815
Siegler, E. L., Zhu, Y., Wang, P., and Yang, L. (2018). Off-the-Shelf CAR-NK cells for cancer immunotherapy. Cell Stem Cell 23 (2), 160–161. doi:10.1016/j.stem.2018.07.007
Si Lim, S. J., Grupp, S. A., and DiNofia, A. M. (2021). Tisagenlecleucel for treatment of children and young adults with relapsed/refractory B-cell acute lymphoblastic leukemia. Pediatr. Blood Cancer 68 (9), e29123. doi:10.1002/pbc.29123
Srivastava, S., Furlan, S. N., Jaeger-Ruckstuhl, C. A., Sarvothama, M., Berger, C., Smythe, K. S., et al. (2021). Immunogenic chemotherapy enhances recruitment of CAR-T cells to lung tumors and improves antitumor efficacy when combined with checkpoint blockade. Cancer Cell 39 (2), 193–208.e10. doi:10.1016/j.ccell.2020.11.005
Sternlicht, M. D., and Werb, Z. (2001). How matrix metalloproteinases regulate cell behavior. Annu. Rev. Cell Dev. Biol. 17, 463–516. doi:10.1146/annurev.cellbio.17.1.463
Subklewe, M., Magno, G., Gebhardt, C., Bücklein, V., Szelinski, F., Arévalo, H. J. R., et al. (1990). Application of blinatumomab, a bispecific anti-CD3/CD19 T-cell engager, in treating severe systemic sclerosis: a case study. Eur. J. Cancer Oxf Engl. 204, 114071. doi:10.1016/j.ejca.2024.114071
Taubmann, J., Knitza, J., Müller, F., Völkl, S., Aigner, M., Kleyer, A., et al. (2024). Rescue therapy of antisynthetase syndrome with CD19-targeted CAR-T cells after failure of several B-cell depleting antibodies. Rheumatol. Oxf Engl. 63 (1), e12–e14. doi:10.1093/rheumatology/kead330
Thommen, D. S., and Schumacher, T. N. (2018). T cell dysfunction in cancer. Cancer Cell 33 (4), 547–562. doi:10.1016/j.ccell.2018.03.012
Thurlings, R. M., Teng, O., Vos, K., Gerlag, D. M., Aarden, L., Stapel, S. O., et al. (2010). Clinical response, pharmacokinetics, development of human anti-chimaeric antibodies, and synovial tissue response to rituximab treatment in patients with rheumatoid arthritis. Ann. Rheum. Dis. 69 (2), 409–412. doi:10.1136/ard.2009.109041
Tony, H. P., Burmester, G., Schulze-Koops, H., Grunke, M., Henes, J., Kötter, I., et al. (2011). Safety and clinical outcomes of rituximab therapy in patients with different autoimmune diseases: experience from a national registry (GRAID). Arthritis Res. Ther. 13 (3), R75. doi:10.1186/ar3337
Tsokos, G. C., Lo, M. S., Costa Reis, P., and Sullivan, K. E. (2016). New insights into the immunopathogenesis of systemic lupus erythematosus. Nat. Rev. Rheumatol. 12 (12), 716–730. doi:10.1038/nrrheum.2016.186
Van Steendam, K., Tilleman, K., De Ceuleneer, M., De Keyser, F., Elewaut, D., and Deforce, D. (2010). Citrullinated vimentin as an important antigen in immune complexes from synovial fluid of rheumatoid arthritis patients with antibodies against citrullinated proteins. Arthritis Res. Ther. 12 (4), R132. doi:10.1186/ar3070
Verstappen, G. M., Pringle, S., Bootsma, H., and Kroese, F. G. M. (2021). Epithelial-immune cell interplay in primary Sjögren syndrome salivary gland pathogenesis. Nat. Rev. Rheumatol. 17 (6), 333–348. doi:10.1038/s41584-021-00605-2
Wang, V., Gauthier, M., Decot, V., Reppel, L., and Bensoussan, D. (2023). Systematic review on CAR-T cell clinical trials up to 2022: academic center input. Cancers 15 (4), 1003. doi:10.3390/cancers15041003
Wang, W., He, S., Zhang, W., Zhang, H., DeStefano, V. M., Wada, M., et al. (2024b). BCMA-CD19 compound CAR T cells for systemic lupus erythematosus: a phase 1 open-label clinical trial. Ann. Rheum. Dis. 83 (10), 1304–1314. doi:10.1136/ard-2024-225785
Wang, X., Wu, X., Tan, B., Zhu, L., Zhang, Y., Lin, L., et al. (2024a). Allogeneic CD19-targeted CAR-T therapy in patients with severe myositis and systemic sclerosis. Cell 187 (18), 4890–4904.e9. doi:10.1016/j.cell.2024.06.027
Wei, J., Han, X., Bo, J., and Han, W. (2019). Target selection for CAR-T therapy. J. Hematol. OncolJ Hematol. Oncol. 12 (1), 62. doi:10.1186/s13045-019-0758-x
Yoshizaki, A. (2018). Pathogenic roles of B lymphocytes in systemic sclerosis. Immunol. Lett. 195, 76–82. doi:10.1016/j.imlet.2018.01.002
Zhan, Q., Zhang, J., Lin, Y., Chen, W., Fan, X., and Zhang, D. (2023). Pathogenesis and treatment of Sjogren’s syndrome: review and update. Front. Immunol. 14, 1127417. doi:10.3389/fimmu.2023.1127417
Keywords: CAR-T cell, autoimmune disease, systemic lupus erythematosus, rheumatoid arthritis, Sjögren’s syndrome, systemic sclerosis, idiopathic inflammatory myopathies, ANCA-associated vasculitis
Citation: Guo Q, Li J, Wang J, Li L, Wei J and Zhang L (2024) The advent of chimeric antigen receptor T Cell therapy in recalibrating immune balance for rheumatic autoimmune disease treatment. Front. Pharmacol. 15:1502298. doi: 10.3389/fphar.2024.1502298
Received: 26 September 2024; Accepted: 25 November 2024;
Published: 12 December 2024.
Edited by:
Sergey V. Ryzhov, Maine Medical Center, United StatesReviewed by:
Daniel Baker, University of Pennsylvania, United StatesCopyright © 2024 Guo, Li, Wang, Li, Wei and Zhang. This is an open-access article distributed under the terms of the Creative Commons Attribution License (CC BY). The use, distribution or reproduction in other forums is permitted, provided the original author(s) and the copyright owner(s) are credited and that the original publication in this journal is cited, in accordance with accepted academic practice. No use, distribution or reproduction is permitted which does not comply with these terms.
*Correspondence: Jia Wei, amlhd2VpQHRqaC50am11LmVkdS5jbg==; Liyun Zhang, emhhbmdseUBzeGJxZWguY29tLmNu
†These authors have contributed equally to this work and share first authorship
Disclaimer: All claims expressed in this article are solely those of the authors and do not necessarily represent those of their affiliated organizations, or those of the publisher, the editors and the reviewers. Any product that may be evaluated in this article or claim that may be made by its manufacturer is not guaranteed or endorsed by the publisher.
Research integrity at Frontiers
Learn more about the work of our research integrity team to safeguard the quality of each article we publish.