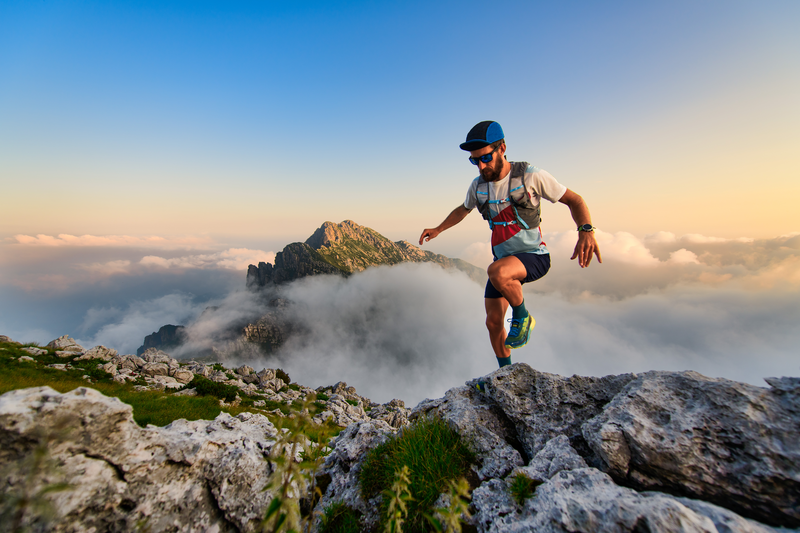
94% of researchers rate our articles as excellent or good
Learn more about the work of our research integrity team to safeguard the quality of each article we publish.
Find out more
REVIEW article
Front. Pharmacol. , 13 November 2024
Sec. Ethnopharmacology
Volume 15 - 2024 | https://doi.org/10.3389/fphar.2024.1499602
This article is part of the Research Topic Preventing and Treating Liver Diseases: Medicinal and Food Plants, their metabolites as potential options View all 16 articles
Non-alcoholic fatty liver disease (NAFLD) is a chronic liver condition closely associated with metabolic syndrome, with its incidence rate continuously rising globally. Recent studies have shown that the development of NAFLD is associated with insulin resistance, lipid metabolism disorder, oxidative stress and endoplasmic reticulum stress. Therapeutic strategies for NAFLD include lifestyle modifications, pharmacological treatments, and emerging biological therapies; however, there is currently no specific drug to treat NAFLD. However Chinese herb medicine (CHM) has shown potential in the treatment of NAFLD due to its unique therapeutic concepts and methods for centuries in China. This review aims to summarize the pathogenesis of NAFLD and some CHMs that have been shown to have therapeutic effects on NAFLD, thus enriching the scientific connotation of TCM theories and facilitating the exploration of TCM in the treatment of NAFLD.
Non-alcoholic fatty liver disease (NAFLD) is a growing public health issue worldwide, encompassing a spectrum of liver conditions ranging from simple fatty liver to nonalcoholic steatohepatitis (NASH), which may progress to cirrhosis and hepatocellular carcinoma (Diehl and Day, 2017). In certain parts of the world, the prevalence of NAFLD is thought to reach up to 26.5% (Younossi et al., 2023). Moreover, NAFLD prevalence in China is 32.5% (Teng et al., 2023). NAFLD is closely associated with components of the metabolic syndrome, such as obesity, type 2 diabetes and hyperlipidemia, and its prevalence is rising in tandem with the increasing prevalence of these metabolic disorders (Younossi et al., 2016).
NAFLD presents itself as a syndrome characterized by the accumulation of fat along with hepatocellular steatosis, ballooning degeneration, lobar inflammation, and predominantly fibrosis (Chalasani et al., 2018). The pathogenesis of NAFLD is complex, involving lipid metabolism disorders (Anwar et al., 2023), insulin resistance (Muzurović et al., 2021), inflammatory responses (Tilg and Moschen, 2010), mitochondrial dysfunction (Mansouri et al., 2018), oxidative stress (Karkucinska-Wieckowska et al., 2022), and endoplasmic reticulum stress (Ajoolabady et al., 2023). Currently, the treatment of NAFLD primarily relies on lifestyle modifications, including dietary adjustments and increased physical activity (El-Agroudy et al., 2019; Golabi et al., 2016). Contemporary medical approaches to treat NAFLD include the improvement of insulin resistance and the use of lipid-lowering drugs, antioxidants, and hepatocyte-protective agents, all promoting liver lipid metabolism and accelerating intrahepatic fat transport (Nassir, 2022). Although some drugs have entered clinical trials, to date, no specific drug has been approved for the treatment of NAFLD (Friedman et al., 2018). Therefore, it is urgent to develop specific drugs for the treatment of NAFLD, which will generate significant social and economic benefits.
Chinese herb medicine (CHM), as an integral part of traditional Chinese medicine (TCM), has unique theories and practical experiences in treating chronic diseases (Shi et al., 2020). The CHM approach to treating NAFLD is usually based on the principle of syndrome differentiation and treatment, aiming to harmonize the body’s yin-yang balance. CHM has been used extensively and safely for millennia to treat liver disorders. In recent years, numerous studies have indicated that certain components of CHM have effects such as modulating insulin resistance (Dai et al., 2022), regulating lipid metabolism (Yang J. M. et al., 2019), anti-inflammation (Lan T. et al., 2021) and antioxidant (Fan et al., 2023), offering new perspectives for the treatment of NAFLD.
With “NAFLD” and “Chinese herb medicine” as key words, we searched CNKI, WanFang, VIP, SinoMed, and PubMed database for relevant literature in the last 10 years. By systematically reviewing relevant literature, this review aims to provide a comprehensive analysis of existing research on the treatment of NAFLD with CHM, explore its mechanisms of action, assess its clinical efficacy, and propose future research directions.
The traditional “two hit” hypothesis has gradually transitioned to “multiple hit” theory (Fang et al., 2018). In the “two hit” hypothesis, the first hit is represented by insulin resistance associated with obesity, type 2 diabetes, hyperlipidemia, and other conditions, leading to excessive lipid deposition within hepatocytes. The second hit refers to the occurrence of lipid peroxidation and oxidative stress in hepatocytes with excessive lipid deposition, leading to mitochondrial dysfunction, production of inflammatory mediators, and activation of hepatic stellate cells, thereby resulting in NASH and fibrosis (James and Day, 1998). However, as it is inadequate to explain the several molecular and metabolic changes that take place in NAFLD, the “two-hit” hypothesis is now obsolete. “Multiple hit” theory includes various factors such as insulin resistance, lipid metabolism disorder, mitochondrial dysfunction, oxidative stress, and endoplasmic reticulum (ER) stress, etc (Buzzetti et al., 2016) (Figure 1).
Figure 1. The mechanisms of NAFLD. “Multiple hit” theory includes various factors such as insulin resistance, lipid metabolism disorder, mitochondrial dysfunction, oxidative stress, and endoplasmic reticulum (ER) stress. Abbreviations: IRS, insulin receptor substrate; PI3K, phosphatidylinositol 3-kinase; AKT, protein kinase B; SREBP1C, sterol regulatory element binding protein 1c; DNL, de novo lipogenesis; ChREBP, carbohydrate regulatory element-binding protein; ACC, acetyl-CoA carboxylase; FAS, fatty acid synthase; TCA, tricarboxylic acid cycle; ETC, electron transport chain; CPT1, carnitine palmitoyltransferase 1; ROS, reactive oxygen species; UPR, unfolded protein response; PERK, RNA-like ER kinase; eIF2α, phosphorylation of eukaryotic initiation factor-2α; ATF4, activation of transcription factor 4; ATF6, activation of transcription factor 6; CHOP, CCAAT-enhancer-binding protein homologous protein; IRE1, inositol-requiring enzyme 1; XBP1, X-box binding protein-1; JNK, c-Jun N-terminal kinase; TRAF2, TNF receptor-related factor 2.
Insulin resistance (IR) is one of the “multiple hits” predisposing to the development of NAFLD and progression to NASH (Peverill et al., 2014). Dietary factors are crucial for the development of NAFLD. Typical western diet, which has high consumption of fat, has been associated with IR (Fan and Cao, 2013). During the intake of calories, the insulin reduces the production of glucose in the liver by inhibiting glycogenolysis and limiting the postprandial rise in glucose. However, this feedback mechanism is impaired in individuals with IR, resulting in the continued elevation of hepatic glucose production despite the postprandial glucose increases (Fujii et al., 2020). The status of IR leads adipose tissue unresponsive to the antilipolytic effect of insulin, resulting in triglyceride (TG) hydrolysis and the ultimate formation of free fatty acids (FFAs) and glycerol (Schweiger et al., 2006). Increased lipolysis in adipocytes leads to an increase in circulating FFAs, which further exacerbates steatosis and IR in muscle tissue (Zhang et al., 2014).
The molecular mechanism of IR refers to the impairment of the appropriate downstream effects of insulin signaling in target tissues, such as the liver, muscle, and adipose tissue. Insulin exerts its effects on all cells by binding to its specific receptor, thereby initiating a cascade of intracellular signaling (Khan et al., 2019). Upon insulin binding, the insulin receptor phosphorylates itself and several members of the insulin receptor substrate (IRS) family. The typical IRS signalling pathways include those that are dependent on IRS1 or IRS2, which utilise the activities of phosphatidylinositol 3-kinase (PI3K), phosphoinositide-dependent kinase (PDK) and protein kinase B (AKT), as well as the RAS-extracellular-signal regulated kinase (ERK) pathway (Eckstein et al., 2017). Activation of IRS2 has been demonstrated to function as a regulator of sterol regulatory element binding protein 1c (SREBP-1c), thereby influencing de novo lipogenesis (DNL) (Schreuder et al., 2008). In states of IR, there is a reduction in the expression of IRS-2, which results in an increase in the expression of SREBP-1c and a corresponding elevation in the rate of DNL (Stefan et al., 2008). Additionally, the β-oxidation of FFAs is suppressed in states of insulin resistance, thereby further promoting the accumulation of hepatic lipids (Postic and Girard, 2008). Thus, disorders of lipid metabolism due to dysregulation of insulin signalling are key factors in the development and progression of NAFLD.
The liver plays a distinctive role in lipid metabolism, acting as a site for lipid uptake, synthesis, oxidation, and distribution of lipids to peripheral tissues (Nguyen et al., 2008). Hepatic fat accumulation results from an imbalance between lipid acquisition and lipid disposal. When energy intake is higher than consumption, excess energy is stored in the form of lipids. In patients with NAFLD, fat accumulates primarily in the form of TG within the liver (Jacome-Sosa and Parks, 2014). The formation of TG is the result of the esterification of glycerol and FFAs. Hepatic steatosis is initiated by an increase in the synthesis of TG in hepatocytes. This synthesis is dependent on the supply of a substrate originating from white adipose tissue (WAT), DNL, and the consumption of a high fat and/or high sugar diet (Donnelly et al., 2005; Heeren and Scheja, 2021; Machado and Cortez-Pinto, 2014).
Dysregulation of DNL is a central feature of liver lipid accumulation in NAFLD patients (Ipsen et al., 2018). The transcriptional regulation of DNL is mainly orchestrated by two key transcription factors: sterol regulatory element-binding protein 1c (SREBP1c) and carbohydrate regulatory element-binding protein (ChREBP) (Eberlé et al., 2004; Sanders and Griffin, 2016). SREBP1c expression is enhanced in NAFLD patients, with higher levels of hepatic triglyceride and upregulating genes coding for acetyl-CoA carboxylase (ACC) and fatty acid synthase (FAS) (Higuchi et al., 2008; Kohjima et al., 2007). In addition, SREBP1c indirectly contributes to the development of hepatic insulin resistance, since enhanced lipogenesis and subsequent accumulation of harmful lipid species, such as diacylglycerides, may interfere with insulin signaling (Kumashiro et al., 2011; Ter Horst et al., 2017). ChREBP is a key transcription factor for enzymes in the fructolysis, glycolysis, gluconeogenesis, and DNL pathways (Iizuka et al., 2004; Iizuka and Horikawa, 2008; Kim et al., 2016). Increased glucose concentration activates ChREBP to regulate the expression of ACC1 and FAS, thereby promoting DNL in hepatocytes (Denechaud et al., 2008; Dentin et al., 2006). Thus, A high fat and/or high sugar diet directly affects the DNL pathways, leading to the accumulation of lipid and the development of NAFLD.
A number of factors contribute to the occurrence of “multiple hits”, with oxidative stress being considered the primary cause of liver injury and disease progression in NAFLD (Friedman et al., 2018). An increase in FFAs in the liver, which may result from a number of different causes, can lead to the damage of β-oxidation and mitochondrial dysfunction, resulting in inflammation, which leads to oxidative stress (Chen et al., 2020). Reactive oxygen species (ROS) are important mediators of the inflammatory response (Begriche et al., 2006). ROS, which includes superoxide anion radicals (O2·-) and hydrogen peroxide (H2O2), are continuously produced intracellularly as byproducts of energetic metabolism in different types of liver cells (Masarone et al., 2018). Normal levels of reactive ROS act as signalling molecules that regulate a number of essential cellular processes, including metabolism, survival, immune defence, proliferation and differentiation through the modulation of transcription factors and epigenetic pathways (Forrester et al., 2018). A reduction in ROS generation leads to a decline in redox status and, consequently, impairs the ability of cells to perform physiological redox signaling (Zhang et al., 2019). In the case of oxidative stress, however, excessive ROS induce oxidative modifications to macromolecules, including DNA, lipids, and proteins, leading to the accumulation of damaged macromolecules and subsequent liver injury (Serviddio et al., 2013). In most cells, mitochondria are considered the most quantitatively relevant ROS generators (Mansouri et al., 2018). The liver contains between 500 and 4,000 mitochondria per hepatocyte, which collectively occupy approximately 18% of the cell volume (Degli Esposti et al., 2012). In addition to the mechanisms for the pathophysiology of NAFLD described above, multiple mitochondria-associated factors contribute to the development and progression of NAFLD. Such factors include reduced β-oxidation, impaired, ETC and ATP depletion, over-production of ROS, oxidative stress-mediated cell damage, and ultra-structural mitochondrial changes (Begriche et al., 2006; Begriche et al., 2013; Pessayre et al., 2002). These changes in mitochondrial function and structure exacerbate hepatic lipid accumulation and trigger inflammatory and fibrogenic processes, thereby contributing to the development and progression of NAFLD.
The main metabolic pathway affected by endoplasmic reticulum (ER) stress is lipogenesis (Flamment et al., 2010). ER stress is implicated in both the development of hepatic steatosis and the progression of NASH. Disruption of ER homeostasis has been observed in liver and adipose tissue of humans with NAFLD (Gentile et al., 2011; Puri et al., 2008). ER stress represents a protective response that restores protein homeostasis through the activation of the unfolded protein response (UPR) (Li W. et al., 2020). The UPR has been linked to lipid biosynthesis, insulin action, inflammation, and apoptosis (Glimcher and Lee, 2009; Hotamisligil, 2010; Kim et al., 2008).
UPR is mediated by three typical ER-resident stress sensors, protein kinase RNA-like ER kinase (PERK), inositol-requiring enzyme 1 (IRE1), and activating transcription factor 6 (ATF6) (Gong J. et al., 2017). These three proximal UPR sensors all regulate lipid storage in the liver (Donnelly et al., 2005). ER stress has been demonstrated to induce apoptosis through the activation of these three sensor dimers and autophosphorylation (Shao et al., 2022). The IRE1-XBP1 and PERK-peIF2α pathways upregulate the adipogenic gene program (Kaufman, 1999). Conversely, the interaction between ATF6, sterol regulatory element-binding protein 2 (SREBP2) and histone deacetylase 1 (HDAC1) can limit adipogenesis (Zeng et al., 2004). In addition, ATF6 upregulates the expression of X-box binding protein-1 (XBP1), which is one of the main regulators of UPR and interacts with the PI3K insulin signaling pathway, with increased nuclear translocation induced by insulin (Park et al., 2010). XBP1 is a crucial transcription factor that regulates the expression of genes encoding the adaptive UPR. The interaction between PI3K and XBP-1 is subject to modulation by both the cellular response to ER stress and the interaction itself (Winnay et al., 2010). Another consequence of UPR is the activation of SREBP-1c pathways, which results in the maintenance of liver fat accumulation and a further exacerbation of ER stress and UPR (Ferré and Foufelle, 2010). Thus, all three proximal UPR sensors, PERK, IRE1α, and ATF6α, can regulate lipid stores in the liver.
NAFLD is a complex metabolic disorder that often co-occurs with other metabolic conditions such as dyslipidemia, hypertension, and diabetes mellitus (Zhao et al., 2018). In TCM, while there is no direct equivalent term for NAFLD, the concept of “dampness” and “phlegm” is often used to describe conditions that resemble the symptoms and pathophysiological mechanisms associated with NAFLD. NAFLD can be recognized as hepatic syndromes like distention and fullness, phlegm syndrome like turbidity, hypochondriac pain, lump at the left hypochondrium and damp obstruction disease based on its symptoms and pathogenesis (Zhang and Li, 2017).
In long-term clinical practice, CHM has unique advantages in the treatment of NAFLD. Characterized by a multi-herbal composition and multi-target pharmacological effects, CHM is compatible with the complex pathogenesis of NAFLD. As a result, the majority of NAFLD patients have availed themselves of TCM therapies.
Considering the varying progression of NAFLD, it is imperative to select appropriate CHM for treatment at different stages. Initially, therapeutic interventions for NAFLD focus on soothing the liver, regulating the flow of qi, and bolstering the functions of the spleen and stomach. Xiao Yao San is often used to achieve the effect of soothing the liver and strengthening the spleen in clinical practice. As the condition progresses into the middle and later stages, the primary treatment strategies shift towards strengthening the spleen and nourishing the kidneys, invigorating blood circulation to disperse blood stasis, and additionally incorporating measures to clear heat and resolve dampness. Gexiazhuyu Tang with Erchen Tang are used to activate blood circulation to remove blood stasis, and resolve phlegm to disperse nodules. Sijunzi Tang with Jinguishenqi Pillsis are used to tonify the spleen and kidneys (Zhang and Li, 2017). Meanwhile, the selection of clinical prescriptions should be based on the primary symptom, with consideration given to the other symptoms. It is recommended that a slight variation be made in the selected prescriptions, and that a “differentiation treatment” be employed. Based on clinical experience, the common diagnostic treatment of NAFLD is shown in Table 1.
CHM emphasize on the importance of individualized therapy based on syndrome differentiation, and a variety of CHM formulas are used to alleviate specific symptoms associated with the condition. The selection of formulas is dependent upon the four properties of TCM (cold, hot, warm, cool), the five flavours (sour, bitter, sweet, spicy, salty), and the efficiency. We have assembled a diverse set of CHM formulas that are specifically designed to treat NAFLD and clarified the therapeutic mechanisms by which these CHM formulas ameliorate the disease (Table 2). For example, formulas such as Lingguizhugan decoction, Shenling Baizhu San, Chaihu Shugan powder are commonly used in clinical for the treatment of NAFLD.
In China, some TCM compounds have been approved as commercial Chinese polyherbal preparation (CCPP), while more TCM compounds are already in clinical trials (Table 3). Such as Dang Fei Li Gan Ning Capsules are primarily indicated for the treatment of patients with non-alcoholic simple fatty liver disease characterized by internal retention of damp-heat (Xiaoling et al., 2022). Hua Zhi Rou Gan Granules are primarily used for the treatment of patients with NAFLD characterized by damp-heat obstructing the middle burner syndrome (Cao et al., 2024; Liu W. et al., 2022). Each type of CCPP is selected based on the specific symptoms and underlying causes identified by a TCM practitioner, ensuring a personalized approach to treatment.
Currently, many clinical workers combined disease differentiation with syndrome differentiation and used proper prescriptions to effectively improve liver function and clinical symptoms in patients with NAFLD, achieving a satisfactory clinical effect.
In fact, in traditional medicine, botanical drugs play a crucial role in the prevention and mitigation of different human diseases. Due to the complicated metabolites found in botanical drugs, studying the active metabolites became the mainstream of TCM research. According to the literature and clinical experience, the Chinese medicinal herbs commonly used for treatment of NAFLD in clinical practice can be divided into the following types (Table 4). Here, we summarize the pharmacological effects of active metabolites from high-frequency single herbs.
Crataegus pinnatifida Bunge and its subspecies C. pinnatifida var. pubescens Nakai and C. pinnatifida var. Pinnatifida [syn.: Crataegus cuneata Siebold & Zucc.; Rosaceae] yield Shan Zha (crataegi fructus), is recognized for its ability to enhance digestion and alleviate bloating in TCM. Research has shown that C. pinnatifida Bunge possesses potent antioxidant and free radical scavenging activities, which can be attributed to its content of various bioactive metabolites, including chlorogenic acid, epicatechin, hyperoside, vitexin, quercetin, rutin, and procyanidins (Barros et al., 2011; Tadić et al., 2008; Zhang et al., 2001). These metabolites are reported to have many pharmacological effects, which are involved in the treatment of various diseases, including hypertension, cardiovascular, anti-oxidative, atherosclerosis, and hyperlipidemiawhich (Bahorun et al., 2003; Kirakosyan et al., 2003; Yoo et al., 2016). Among them, vitexin and quercetin are reported to intervene NAFLD.
The study showed that 5-week vitexin administration (40 mg/kg, i. g.) could obviously reduce hepatic fat deposition, alleviate lipid metabolism, and inhibit liver inflammation in NAFLD mice. In addition, vitexin significantly reduced hepatic macrophage infiltration, obviously downregulated the mRNA and protein expressions of hepatic SREBP-1c, FAS, ACC, and could significantly inhibit the expressions of TLR4/NF-κB signaling in NAFLD mice (Li C. et al., 2020).
Another metabolite, quercetin is also reported to protect the liver. The finding suggested that antiinflammatory responses, antioxidant, and improve ment of lipid metabolism via farnesoid X receptor 1 (FXR1)/Takeda G protein-coupled receptor 5 (TGR5) signaling pathways played key role in the hepatoprotective effects of quercetin (oral gavaged with the quercetin (100 mg/kg) once a day for 8 weeks) in T2DM-induced NAFLD db/db mice (Yang H. et al., 2019). In additional, quercetin revertes the balance of the gut microbiota and counteracted endotoxemia-induced activation of the TLR-4 pathway, subsequently inhibiting the inflammasome response and the activation of the reticulum stress pathway, which resulted in the prevention of deregulation in the expression of lipid metabolism genes (Porras et al., 2017).
In conclusion, C. pinnatifida Bunge can exert therapeutic effects on NAFLD through its anti-inflammatory properties, regulation of lipid metabolism, and modulation of the gut microbiota.
The rhizome of Alisma plantago-aquatica L. and its subspecies A. plantago-aquatica subsp. orientale (Sam.) Sam. [syn.: Alisma orientale (Sam.) Juz.; Alismataceae] yield Ze Xie (alismatis rhizoma), which has been used to treat various ailments, such as dysuria, edema, nephropathy, hyperlipidemia, and diabetes. A wide range of metabolites, mainly triterpenoids, sesquiterpenoids, and diterpenoids, have been isolated from A. plantago-aquatica L.; among which the protostane-type triterpenoids, termed alisol A and B have been proved to be effective on NAFLD (Gao et al., 2024; Li and Qu, 2012; Zhang et al., 2017).
Alisol A (100 mg/kg/day, intraperitoneal injection once daily for 4 weeks) effectively attenuated HFD-induced obesity, suppressed hepatic steatosis and improved lipid and glucose metabolism, and improved damaged β-oxidation in DIO mice. The pharmacologic action may be mediated through AMPK/ACC/SREBP-1c pathway activation (Ho et al., 2019). In another study, the MCD-induced mice were simultaneously treated with a daily dose of Alisol A (15, 30, and 60 mg·kg−1, ig) for 4 weeks. The results showed that Alisol A can also ameliorate steatohepatitis by inhibiting oxidative stress and stimulating autophagy through the AMPK/mTOR pathway (Wu et al., 2018).
A study indicated that treated with 100 mg/kg Alisol B once daily for 8 weeks showed significant therapeutic effects on DIO + CCl4 and CDA diet-induced murine NASH models. In this study, Alisol B was found to alleviate hepatocyte lipid accumulation and lipotoxicity in NASH mice via regulating RARα-PPARγ-CD36 cascade. Alisol B was also found to relieve cellular ROS level and decrease inflammatory cytokines expression in mouse primary hepatocytes, along with a robust blockade of JNK/NF-κB pathway (Zhao et al., 2022). In another study, NASH was induced in mice fed a MCD diet for 4 weeks. The mice were simultaneously treated with AB23A (15, 30, and 60 mg·kg−1·d−1, ig) for 4 weeks. The study suggested that Alisol B 23A protects against MCD-induced NASH in mice via activating the FXR signaling pathway, thus decreasing the accumulation of lipids in the liver, hepatic lobular inflammation and pericellular fibrosis (Meng et al., 2017).
In summary, A. plantago-aquatica L. may treat NAFLD by regulating pathways related to inflammation and lipid metabolism.
Salvia miltiorrhiza Bunge and its subspecies S. miltiorrhiza var. miltiorrhiza and S. miltiorrhiza var. charbonnelii (H.Lév.) C.Y.Wu [syn.: Salvia przewalskii Maxim.; Lamiaceae] yield Dan Shen (Radix et rhizoma salviae miltiorrhizae), is a traditional and folk medicine in Asian countries, especially in China and Japan (MEIm et al., 2019). Salvia miltiorrhiza Bunge is rich in bioactive metabolites such as tanshinones and salvianolic acids, which are believed to exert hepatoprotective, anti-inflammatory, and antioxidant effects (Shi et al., 2019; Wang Y. C. et al., 2015).
It has been demonstrated that tanshinones can modulate multiple targets, including PPARα, CYP1A2, and MMP2, thereby exerting regulatory effects on lipid metabolism, providing antioxidant benefits, and inhibiting fibrogenesis (Hong et al., 2017). Following intraperitoneal injection of tanshinone IIA (10 mg/kg/day) for 2 month, liver steatosis was significantly inhibited in mice on a high-fat diet. This study suggested that tanshinones IIA attenuates oxidative stress by decreasing ROS malondialdehyde (MDA) production and enhancing the activity of total superoxide dismutase (T-SOD) and glutathione peroxidase (GSH-PX), which may contribute to the inhibition of apoptosis and amelioration of liver steatosis (Yang et al., 2017). In addition, HFD-induced rats received 10 mg/kg tanshinone IIA by daily intraperitoneal injection for 3 months, the lipid deposition in the livers of hyperlipidemic rats and modulated the expression of miR-33a and SREBP-2/Pcsk9 signaling pathway proteins was attenuated (Jia et al., 2016).
Administration of Salvianolic acid A (20 and 40 mg/kg BW, respectively) via intraperitoneal injection ameliorates liver steatosis and hepatic damage in high-fat and high-carbohydrate diet-fed mice (Li S. et al., 2020). Additionally, Salvianolic acid A protects the liver from NAFLD caused by a high-fat diet by reducing both hepatic lipid accumulation and inflammation. This anti-inflammatory action may be attributed in part to the modulation of the TXNIP/NLRP3 inflammatory pathways (Ding et al., 2016). Salvianolic acid B is capable of modulating the SIRT1-dependent deacetylation of HMGB1, thereby offering protection against hepatic steatosis and inflammation induced by either a high-fat diet or palmitic acid (Zeng et al., 2015).
Thus, S. miltiorrhiza Bunge seemed to play its anti-oxidation role in the treatment of NAFLD.
Bupleurum chinense DC. [syn.: Bupleurum scorzonerifolium Willd. ; Apiaceae] yield Chai Hu (Bupleuri radix), is a herbal medicine for harmonizing and soothing liver qi stagnation (Law et al., 2014). Saikosaponins, especially Saikosaponina and Saikosaponind, as the major bioactive metabolites in B. chinense DC., represent anti-inflammatory, anti-oxidant, and hepatoprotective effects to treat NAFLD (Li et al., 2018).
Studies suggest that Saikosaponin a may influence key regulatory pathways involved in lipid metabolism, such as the PI3K/Akt/NF-κB/NLRP3 pathway, which is central to the homeostasis of lipid profiles within the liver (He et al., 2016). Additionally, Saikosaponin d improved lipid homeostasis by coordinately regulating PPARα activation-mediated both inhibition of SREBP1c-dependent FA biosynthesis and induction of FA degradation (Gu et al., 2022). Moreover, Saikosaponin d can induce improvement of fatty liver by decreasing ER-stress-related protein expression (Chang et al., 2021).
Overall, for the effects of B. chinense DC. on NAFLD, primary mechanisms are anti-inflammation and oxidative stress.
Senna tora (L.) Roxb. [syn.: Senna obtusifolia (L.). H.S.Irwin & Barneby; Fabaceae] yield Jue Ming Zi (Cassiae semen) has long been used for relieving constipation, improving liver function as well as preventing myopia. Anthraquinones, naphthopyranones, naphthalenes, flavones, polysaccharides and other metabolites, have been isolated and identified from Senna tora (L.) Roxb. (Chen et al., 2023).
It was found that S. tora (L.) Roxb. ethanol extract effectively inhibited de novo lipogenesis and ameliorated hepatic steatosis by promoting AMPK-mediated autophagy (Ding et al., 2023). Another study found that treatment with S. tora (L.) Roxb. extract lessened the effects of HFD-induced NAFLD rats, possibly by increasing the activity of antioxidant enzyme, inhibiting the MDA in liver and upregulated the expression of LDL-R to regulate the lipid metabolism process (Meng et al., 2019).
Naphthalenes can reduce lipid accumulation, liver injury and inflammation, gut microbiota disorders, intestinal barrier injury, and metabolic endotoxemia in HFD induced liver injury (Luo et al., 2021).
Oral administration of rhein (150 mg/kg in water) for 40 days significantly increased energy expenditure, reduced body weight, particularly body fat content, improved insulin resistance, and lowered circulating cholesterol levels in DIO mice. Rhein treatment also reduced liver triglyceride levels, reversed hepatic steatosis, and normalized ALT levels in these mice (Sheng et al., 2011). Rhein can reduce the expression of fat mass and obesity-associated protein and simultaneously alleviating oxidative stress and dysregulation in lipid metabolism within HFD rats. This beneficial impact might be linked to the downregulation of key inflammatory markers, such as TLR4, MYD88, and Cyr61 (Lin and Yu, 2021; Qin et al., 2013).
In conclusion,the mechanisms of S. tora (L.) Roxb. treating on NAFLD may relate to alleviate oxidative stress, reduce lipid accumulation, anti-inflammation, and regulate gut microbiota disorders.
The rising global prevalence of NAFLD has become a significant public health issue, necessitating the development of effective and comprehensive treatment strategies. Lifestyle modification with healthy eating and regular exercise represent the primary therapeutic approach for NAFLD (Neuschwander-Tetri, 2017). At present, there are no pharmacological treatments that have been approved by the relevant regulatory authorities for the specific treatment of NAFLD. It is, however, the case that certain pharmaceuticals are efficacious in the treatment of NAFLD, such as insulin sensitizer, lipid-lowering drugs, antioxidants, and weight-loss drugs. These drugs aim to improve metabolic imbalance and liver injury occurred in NAFLD (Dai et al., 2021; van Stee et al., 2018). Vitamin E has been found to correlate with a considerable decrease in liver steatosis, ballooning degeneration of hepatocytes, and pericellular fibrosis (Ekhlasi et al., 2016; Polyzos et al., 2017; Sanyal et al., 2010; Sanyal et al., 2004). The GLP-1 receptor agonist Liraglutide has demonstrated its effectiveness in diminishing the accumulation of fat in the liver and in lowering the levels of liver enzymes among patients diagnosed with nonalcoholic steatohepatitis (NASH) (Newsome et al., 2021; Seino et al., 2010; Yu et al., 2019). Statins can be used in the treatment of dyslipidemia in patients with NAFLD and NASH (Ciardullo and Perseghin, 2021; Pessayre et al., 2002). As a PPARγ agonist, pioglitazone is thought to improve insulin sensitivity in the liver, reduce hepatic fat accumulation, and potentially mitigate liver inflammation and injury through anti-inflammatory and antioxidant actions (Chalasani et al., 2018; Cusi et al., 2016). Obeticholic acid (OCA), a potent ligand for the Farnesoid X receptor (FXR), has been investigated as a therapeutic agent for NASH. Studies have demonstrated that OCA can lead to improvements in the clinical parameters of NASH, including the reduction of fibrosis and the amelioration of liver damage markers. Obeticholic acid (OCA), a potent ligand for the Farnesoid X receptor (FXR), has been investigated as a therapeutic agent for NASH. Studies have demonstrated that OCA can lead to improvements in the clinical parameters of NASH, including the reduction of fibrosis and the amelioration of liver damage markers (Neuschwander-Tetri et al., 2015; Stofan and Guo, 2020). In Europe, Silymarin has been historically uesed as a complementary therapy for the management of hepatic disorders. Silymarin is a key ingredient in the management of liver diseases including chronic hepatitis, cirrhosis, steatosis, alcoholic liver disorders and toxic liver injury, due to its antioxidant, anti-inflammatory and antifibrotic properties (Abenavoli et al., 2010; Schrieber et al., 2011; Wang M et al., 2015) (Figure 2).
Figure 2. NAFLD treatments with biomedicine. Vitamin E, Liraglutide, Statins, Pioglitazone, Obeticholic acid, Silymarin, etc. are used in the treatment of NAFLD.
CHM, with its principle of “holism” and “individualized treatment,” has demonstrated potential as a multifaceted treatment for the complex pathophysiology of NAFLD. However, CHM has the characteristics of “multicomponents, multitargets, and multipathways,” which also makes it difficult to study. In this review, we summarize the mechanisms of frequently used CCPPs, traditional Chinese formula and single herbs in the treatment of NAFLD. CHM has shown promising therapeutic effects in the treatment of NAFLD by regulating lipid metabolism, reducing inflammation, improving liver function and enhancing antioxidant defenses, with fewer adverse reactions. Thus, the use of CHM in the prevention and treatment of NAFLD has a broad development prospect.
Despite the promising therapeutic effects, there are inherent challenges in CHM treatment for NAFLD. Firstly, most patients with NAFLD do not have obvious symptoms and signs, making the diagnosis of the condition based only on the four diagnostic methods of TCM without objective evidence. Secondly, the CHM in registered clinical trials are limited, because long term effects of CHM are difficult to evaluate. In contrast to numerous synthetic pharmaceuticals, the gradual and nuanced actions of CHM may not manifest immediate outcomes. Consequently, it is essential to conduct comprehensive and prolonged observation periods to fully assess the efficacy and safety profiles. This presents a significant challenge in the design of clinical trials, as they must be sufficiently long-term to accurately assess the true benefits and potential adverse effects of CHM, while also being feasible in terms of resources and patient compliance. Lastly, a comprehensive understanding of the mechanism of CHM on NAFLD are not fully elucidated. The complex interplay of multiple bioactive compounds in CHM presents a great challenge in accurately discerning its precise mode of action. While this complexity is a defining feature of CHM’s holistic approach to treatment, it also necessitates advanced research methodologies to elucidate the complex interactions between CHM components and the biological systems with which they interact. Thus, the application of advanced scientific techniques, including metabolomics, transcriptomics, network pharmacology and microbiome analyses, is essential tol to fully elucidate the therapeutic activities of CHM and to substantiate its mechanisms of action in the context of NAFLD treatment. These approaches can facilitate a more systematic understanding of the underlying mechanisms of TCM, paving the way for future investigations.
YL: Writing–original draft, Resources, Methodology, Investigation, Formal Analysis, Conceptualization. YF: Writing–original draft, Visualization, Software, Formal Analysis, Data curation. JL: Writing–review and editing, Project administration, Conceptualization. XL: Writing–original draft, Visualization, Validation, Resources. XL: Writing–original draft, Methodology, Conceptualization. JH: Writing–review and editing, Supervision, Methodology, Funding acquisition.
The author(s) declare that financial support was received for the research, authorship, and/or publication of this article. This study was funded by the National Natural Science Foundation of China (No. 82074316) and Sichuan Science and Technology Program (No. 2024NSFSC1829).
The authors express their gratitude to Quanshen Feng and Dong Wang of the School of Basic Medical Sicience at Chengdu University of TCM for their writing guidance. This study also thanks the editors and reviewers for helping us to improve this manuscript.
The authors declare that the research was conducted in the absence of any commercial or financial relationships that could be construed as a potential conflict of interest.
All claims expressed in this article are solely those of the authors and do not necessarily represent those of their affiliated organizations, or those of the publisher, the editors and the reviewers. Any product that may be evaluated in this article, or claim that may be made by its manufacturer, is not guaranteed or endorsed by the publisher.
Abenavoli, L., Capasso, R., Milic, N., and Capasso, F. (2010). Milk thistle in liver diseases: past, present, future. Phytother. Res. 24 (10), 1423–1432. doi:10.1002/ptr.3207
Ajoolabady, A., Kaplowitz, N., Lebeaupin, C., Kroemer, G., Kaufman, R. J., Malhi, H., et al. (2023). Endoplasmic reticulum stress in liver diseases. Hepatology 77 (2), 619–639. doi:10.1002/hep.32562
Anwar, S. D., Foster, C., and Ashraf, A. (2023). Lipid disorders and metabolic-associated fatty liver disease. Endocrinol. Metab. Clin. North Am. 52 (3), 445–457. doi:10.1016/j.ecl.2023.01.003
Bahorun, T., Aumjaud, E., Ramphul, H., Rycha, M., Luximon-Ramma, A., Trotin, F., et al. (2003). Phenolic constituents and antioxidant capacities of Crataegus monogyna (Hawthorn) callus extracts. Nahrung 47 (3), 191–198. doi:10.1002/food.200390045
Barros, L., Carvalho, A. M., and Ferreira, I. C. (2011). Comparing the composition and bioactivity of Crataegus Monogyna flowers and fruits used in folk medicine. Phytochem. Anal. 22 (2), 181–188. doi:10.1002/pca.1267
Begriche, K., Igoudjil, A., Pessayre, D., and Fromenty, B. (2006). Mitochondrial dysfunction in NASH: causes, consequences and possible means to prevent it. Mitochondrion 6 (1), 1–28. doi:10.1016/j.mito.2005.10.004
Begriche, K., Massart, J., Robin, M. A., Bonnet, F., and Fromenty, B. (2013). Mitochondrial adaptations and dysfunctions in nonalcoholic fatty liver disease. Hepatology 58 (4), 1497–1507. doi:10.1002/hep.26226
Buzzetti, E., Pinzani, M., and Tsochatzis, E. A. (2016). The multiple-hit pathogenesis of non-alcoholic fatty liver disease (NAFLD). Metabolism 65 (8), 1038–1048. doi:10.1016/j.metabol.2015.12.012
Cao, L., Xu, E., Zheng, R., Zhangchen, Z., Zhong, R., Huang, F., et al. (2022a). Traditional Chinese medicine Lingguizhugan decoction ameliorate HFD-induced hepatic-lipid deposition in mice by inhibiting STING-mediated inflammation in macrophages. Chin. Med. 17 (1), 7. doi:10.1186/s13020-021-00559-3
Cao, Y., Chen, M. L., Yu, S. Y., Zhang, L., and Ji, G. (2024). Study on the mechanism of Huazhi Rougan Granules in alleviating non-alcoholic steatohepatitis by inhibiting endoplasmic reticulum stress. China J. Traditional Chin. Med. Pharm. 39 (05), 2195–2203.
Cao, Y., Shi, J., Song, L., Xu, J., Lu, H., Sun, J., et al. (2022b). Multi-omics integration analysis identifies lipid disorder of a non-alcoholic fatty liver disease (NAFLD) mouse model improved by zexie-baizhu decoction. Front. Pharmacol. 13, 858795. doi:10.3389/fphar.2022.858795
Cao, Y., Shu, X., Li, M., Yu, S., Li, C., Ji, G., et al. (2022c). Jiangzhi granule attenuates non-alcoholic steatohepatitis through modulating bile acid in mice fed high-fat vitamin D deficiency diet. Biomed. Pharmacother. 149, 112825. doi:10.1016/j.biopha.2022.112825
Chalasani, N., Younossi, Z., Lavine, J. E., Charlton, M., Cusi, K., Rinella, M., et al. (2018). The diagnosis and management of nonalcoholic fatty liver disease: practice guidance from the American Association for the Study of Liver Diseases. Hepatology 67 (1), 328–357. doi:10.1002/hep.29367
Chang, G. R., Lin, W. L., Lin, T. C., Liao, H. J., and Lu, Y. W. (2021). The ameliorative effects of Saikosaponin in thioacetamide-induced liver injury and non-alcoholic fatty liver disease in mice. Int. J. Mol. Sci. 22 (21), 11383. doi:10.3390/ijms222111383
Chen, D., Wang, Y., Yang, J., Ou, W., Lin, G., Zeng, Z., et al. (2024). Shenling Baizhu San ameliorates non-alcoholic fatty liver disease in mice by modulating gut microbiota and metabolites. Front. Pharmacol. 15, 1343755. doi:10.3389/fphar.2024.1343755
Chen, Y., Chen, X., Yang, X., Gao, P., Yue, C., Wang, L., et al. (2023). Cassiae Semen: a comprehensive review of botany, traditional use, phytochemistry, pharmacology, toxicity, and quality control. J. Ethnopharmacol. 306, 116199. doi:10.1016/j.jep.2023.116199
Chen, Z., Tian, R., She, Z., Cai, J., and Li, H. (2020). Role of oxidative stress in the pathogenesis of nonalcoholic fatty liver disease. Free Radic. Biol. Med. 152, 116–141. doi:10.1016/j.freeradbiomed.2020.02.025
Ciardullo, S., and Perseghin, G. (2021). Statin use is associated with lower prevalence of advanced liver fibrosis in patients with type 2 diabetes. Metabolism 121, 154752. doi:10.1016/j.metabol.2021.154752
Cui, C. X., Deng, J. N., Yan, L., Liu, Y. Y., Fan, J. Y., Mu, H. N., et al. (2017). Silibinin Capsules improves high fat diet-induced nonalcoholic fatty liver disease in hamsters through modifying hepatic de novo lipogenesis and fatty acid oxidation. J. Ethnopharmacol. 208, 24–35. doi:10.1016/j.jep.2017.06.030
Cusi, K., Orsak, B., Bril, F., Lomonaco, R., Hecht, J., Ortiz-Lopez, C., et al. (2016). Long-term pioglitazone treatment for patients with nonalcoholic steatohepatitis and prediabetes or type 2 diabetes mellitus: a randomized trial. Ann. Intern Med. 165 (5), 305–315. doi:10.7326/m15-1774
Dai, L., Xu, J., Liu, B., Dang, Y., Wang, R., Zhuang, L., et al. (2022). Lingguizhugan Decoction, a Chinese herbal formula, improves insulin resistance in overweight/obese subjects with non-alcoholic fatty liver disease: a translational approach. Front. Med. 16 (5), 745–759. doi:10.1007/s11684-021-0880-3
Dai, X., Feng, J., Chen, Y., Huang, S., Shi, X., Liu, X., et al. (2021). Traditional Chinese Medicine in nonalcoholic fatty liver disease: molecular insights and therapeutic perspectives. Chin. Med. 16 (1), 68. doi:10.1186/s13020-021-00469-4
Dang, Y., Xu, J., Yang, Y., Li, C., Zhang, Q., Zhou, W., et al. (2020). Ling-gui-zhu-gan decoction alleviates hepatic steatosis through SOCS2 modification by N6-methyladenosine. Biomed. Pharmacother. 127, 109976. doi:10.1016/j.biopha.2020.109976
Degli Esposti, D., Hamelin, J., Bosselut, N., Saffroy, R., Sebagh, M., Pommier, A., et al. (2012). Mitochondrial roles and cytoprotection in chronic liver injury. Biochem. Res. Int. 2012, 387626. doi:10.1155/2012/387626
Denechaud, P. D., Dentin, R., Girard, J., and Postic, C. (2008). Role of ChREBP in hepatic steatosis and insulin resistance. FEBS Lett. 582 (1), 68–73. doi:10.1016/j.febslet.2007.07.084
Deng, Y., Pan, M., Nie, H., Zheng, C., Tang, K., Zhang, Y., et al. (2019). Lipidomic analysis of the protective effects of shenling Baizhu san on non-alcoholic fatty liver disease in rats. Molecules 24 (21), 3943. doi:10.3390/molecules24213943
Dentin, R., Benhamed, F., Hainault, I., Fauveau, V., Foufelle, F., Dyck, J. R., et al. (2006). Liver-specific inhibition of ChREBP improves hepatic steatosis and insulin resistance in ob/ob mice. Diabetes 55 (8), 2159–2170. doi:10.2337/db06-0200
Diehl, A. M., and Day, C. (2017). Cause, pathogenesis, and treatment of nonalcoholic steatohepatitis. N. Engl. J. Med. 377 (21), 2063–2072. doi:10.1056/NEJMra1503519
Ding, C., Zhao, Y., Shi, X., Zhang, N., Zu, G., Li, Z., et al. (2016). New insights into salvianolic acid A action: regulation of the TXNIP/NLRP3 and TXNIP/ChREBP pathways ameliorates HFD-induced NAFLD in rats. Sci. Rep. 6, 28734. doi:10.1038/srep28734
Ding, M., Zhou, F., Li, Y., Liu, C., Gu, Y., Wu, J., et al. (2023). Cassiae Semen improves non-alcoholic fatty liver disease through autophagy-related pathway. Chin. Herb. Med. 15 (3), 421–429. doi:10.1016/j.chmed.2022.09.006
Donnelly, K. L., Smith, C. I., Schwarzenberg, S. J., Jessurun, J., Boldt, M. D., and Parks, E. J. (2005). Sources of fatty acids stored in liver and secreted via lipoproteins in patients with nonalcoholic fatty liver disease. J. Clin. Invest 115 (5), 1343–1351. doi:10.1172/jci23621
Eberlé, D., Hegarty, B., Bossard, P., Ferré, P., and Foufelle, F. (2004). SREBP transcription factors: master regulators of lipid homeostasis. Biochimie 86 (11), 839–848. doi:10.1016/j.biochi.2004.09.018
Eckstein, S. S., Weigert, C., and Lehmann, R. (2017). Divergent roles of IRS (insulin receptor substrate) 1 and 2 in liver and skeletal muscle. Curr. Med. Chem. 24 (17), 1827–1852. doi:10.2174/0929867324666170426142826
Ekhlasi, G., Kolahdouz Mohammadi, R., Agah, S., Zarrati, M., Hosseini, A. F., Arabshahi, S. S., et al. (2016). Do symbiotic and Vitamin E supplementation have favorite effects in nonalcoholic fatty liver disease? A randomized, double-blind, placebo-controlled trial. J. Res. Med. Sci. 21, 106. doi:10.4103/1735-1995.193178
El-Agroudy, N. N., Kurzbach, A., Rodionov, R. N., O'Sullivan, J., Roden, M., Birkenfeld, A. L., et al. (2019). Are lifestyle therapies effective for NAFLD treatment? Trends Endocrinol. Metab. 30 (10), 701–709. doi:10.1016/j.tem.2019.07.013
Fan, C., Wang, G., Chen, M., Li, Y., Tang, X., and Dai, Y. (2023). Therapeutic potential of alkaloid extract from Codonopsis Radix in alleviating hepatic lipid accumulation: insights into mitochondrial energy metabolism and endoplasmic reticulum stress regulation in NAFLD mice. Chin. J. Nat. Med. 21 (6), 411–422. doi:10.1016/s1875-5364(23)60403-0
Fan, J. G., and Cao, H. X. (2013). Role of diet and nutritional management in non-alcoholic fatty liver disease. J. Gastroenterol. Hepatol. 28 (Suppl. 4), 81–87. doi:10.1111/jgh.12244
Fang, Y. L., Chen, H., Wang, C. L., and Liang, L. (2018). Pathogenesis of non-alcoholic fatty liver disease in children and adolescence: from “two hit theory” to “multiple hit model”. World J. Gastroenterol. 24 (27), 2974–2983. doi:10.3748/wjg.v24.i27.2974
Ferré, P., and Foufelle, F. (2010). Hepatic steatosis: a role for de novo lipogenesis and the transcription factor SREBP-1c. Diabetes Obes. Metab. 12, 83–92. doi:10.1111/j.1463-1326.2010.01275.x
Flamment, M., Kammoun, H. L., Hainault, I., Ferré, P., and Foufelle, F. (2010). Endoplasmic reticulum stress: a new actor in the development of hepatic steatosis. Curr. Opin. Lipidol. 21 (3), 239–246. doi:10.1097/MOL.0b013e3283395e5c
Forrester, S. J., Kikuchi, D. S., Hernandes, M. S., Xu, Q., and Griendling, K. K. (2018). Reactive oxygen species in metabolic and inflammatory signaling. Circ. Res. 122 (6), 877–902. doi:10.1161/circresaha.117.311401
Friedman, S. L., Neuschwander-Tetri, B. A., Rinella, M., and Sanyal, A. J. (2018). Mechanisms of NAFLD development and therapeutic strategies. Nat. Med. 24 (7), 908–922. doi:10.1038/s41591-018-0104-9
Fujii, H., Kawada, N., and Japan Study Group Of Nafld, J.-N. (2020). The role of insulin resistance and diabetes in nonalcoholic fatty liver disease. Int. J. Mol. Sci. 21 (11), 3863. doi:10.3390/ijms21113863
Gao, G., Zhao, J., Ding, J., Liu, S., Shen, Y., Liu, C., et al. (2024). Alisol B regulates AMPK/mTOR/SREBPs via directly targeting VDAC1 to alleviate hyperlipidemia. Phytomedicine 128, 155313. doi:10.1016/j.phymed.2023.155313
Gentile, C. L., Frye, M., and Pagliassotti, M. J. (2011). Endoplasmic reticulum stress and the unfolded protein response in nonalcoholic fatty liver disease. Antioxid. Redox Signal 15 (2), 505–521. doi:10.1089/ars.2010.3790
Glimcher, L. H., and Lee, A. H. (2009). From sugar to fat: how the transcription factor XBP1 regulates hepatic lipogenesis. Ann. N. Y. Acad. Sci. 1173, E2–E9. doi:10.1111/j.1749-6632.2009.04956.x
Golabi, P., Locklear, C. T., Austin, P., Afdhal, S., Byrns, M., Gerber, L., et al. (2016). Effectiveness of exercise in hepatic fat mobilization in non-alcoholic fatty liver disease: systematic review. World J. Gastroenterol. 22 (27), 6318–6327. doi:10.3748/wjg.v22.i27.6318
Gong, J., Wang, X. Z., Wang, T., Chen, J. J., Xie, X. Y., Hu, H., et al. (2017). Molecular signal networks and regulating mechanisms of the unfolded protein response. J. Zhejiang Univ. Sci. B 18 (1), 1–14. doi:10.1631/jzus.B1600043
Gong, M., Wu, S., Yue, H., Liang, S., and Zou, Z. (2017). Study on the hepatoprotective effects of hugan tablets based on serum and liver metabonomics. China Pharm. 28 (34), 4776–4780. doi:10.6039/j.issn.1001-0408.2017.34.06
Gu, Y., Duan, S., Ding, M., Zheng, Q., Fan, G., Li, X., et al. (2022). Saikosaponin D attenuates metabolic associated fatty liver disease by coordinately tuning PPARα and INSIG/SREBP1c pathway. Phytomedicine 103, 154219. doi:10.1016/j.phymed.2022.154219
Guo, S., Chen, L., Chen, M., Li, Z., and Fan, Q. (2023). Study on the protective effect and mechanism of compound yiganling on nonalcoholic fatty liver based on network pharmacology and zebrafish model. Chin. Pharm. J. 58 (7), 584–591. doi:10.11669/cpj.2023.07.005
Guoguo, Z., Bingjie, S., Tianyan, Z., Shaoxiu, J. I., Jingwei, L. I., Yanni, D., et al. (2024). Efficacy of Ganshuang granules on non-alcoholic fatty liver and underlying mechanism: a network pharmacology and experimental verification. J. Tradit. Chin. Med. 44 (1), 122–130. doi:10.19852/j.cnki.jtcm.20231215.001
Han, R., Qiu, H., Zhong, J., Zheng, N., Li, B., Hong, Y., et al. (2021). Si Miao Formula attenuates non-alcoholic fatty liver disease by modulating hepatic lipid metabolism and gut microbiota. Phytomedicine Stuttg. 85, 153544. doi:10.1016/j.phymed.2021.153544
He, D., Wang, H., Xu, L., Wang, X., Peng, K., Wang, L., et al. (2016). Saikosaponin-a attenuates oxidized LDL uptake and prompts cholesterol efflux in THP-1 cells. J. Cardiovasc Pharmacol. 67 (6), 510–518. doi:10.1097/fjc.0000000000000373
Heeren, J., and Scheja, L. (2021). Metabolic-associated fatty liver disease and lipoprotein metabolism. Mol. Metab. 50, 101238. doi:10.1016/j.molmet.2021.101238
Higuchi, N., Kato, M., Shundo, Y., Tajiri, H., Tanaka, M., Yamashita, N., et al. (2008). Liver X receptor in cooperation with SREBP-1c is a major lipid synthesis regulator in nonalcoholic fatty liver disease. Hepatol. Res. 38 (11), 1122–1129. doi:10.1111/j.1872-034X.2008.00382.x
Ho, C., Gao, Y., Zheng, D., Liu, Y., Shan, S., Fang, B., et al. (2019). Alisol A attenuates high-fat-diet-induced obesity and metabolic disorders via the AMPK/ACC/SREBP-1c pathway. J. Cell Mol. Med. 23 (8), 5108–5118. doi:10.1111/jcmm.14380
Hong, M., Li, S., Wang, N., Tan, H. Y., Cheung, F., and Feng, Y. (2017). A biomedical investigation of the hepatoprotective effect of radix salviae miltiorrhizae and network pharmacology-based prediction of the active compounds and molecular targets. Int. J. Mol. Sci. 18 (3), 620. doi:10.3390/ijms18030620
Hong, W., Li, S., Cai, Y., Zhang, T., Yang, Q., He, B., et al. (2020). The target MicroRNAs and potential underlying mechanisms of yiqi-bushen-tiaozhi recipe against-non-alcoholic steatohepatitis. Front. Pharmacol. 11, 529553. doi:10.3389/fphar.2020.529553
Hotamisligil, G. S. (2010). Endoplasmic reticulum stress and the inflammatory basis of metabolic disease. Cell 140 (6), 900–917. doi:10.1016/j.cell.2010.02.034
Iizuka, K., Bruick, R. K., Liang, G., Horton, J. D., and Uyeda, K. (2004). Deficiency of carbohydrate response element-binding protein (ChREBP) reduces lipogenesis as well as glycolysis. Proc. Natl. Acad. Sci. U. S. A. 101 (19), 7281–7286. doi:10.1073/pnas.0401516101
Iizuka, K., and Horikawa, Y. (2008). ChREBP: a glucose-activated transcription factor involved in the development of metabolic syndrome. Endocr. J. 55 (4), 617–624. doi:10.1507/endocrj.k07e-110
Ipsen, D. H., Lykkesfeldt, J., and Tveden-Nyborg, P. (2018). Molecular mechanisms of hepatic lipid accumulation in non-alcoholic fatty liver disease. Cell Mol. Life Sci. 75 (18), 3313–3327. doi:10.1007/s00018-018-2860-6
Jacome-Sosa, M. M., and Parks, E. J. (2014). Fatty acid sources and their fluxes as they contribute to plasma triglyceride concentrations and fatty liver in humans. Curr. Opin. Lipidol. 25 (3), 213–220. doi:10.1097/mol.0000000000000080
James, O. F., and Day, C. P. (1998). Non-alcoholic steatohepatitis (NASH): a disease of emerging identity and importance. J. Hepatol. 29 (3), 495–501. doi:10.1016/s0168-8278(98)80073-1
Jia, L., Song, N., Yang, G., Ma, Y., Li, X., Lu, R., et al. (2016). Effects of Tanshinone IIA on the modulation of miR-33a and the SREBP-2/Pcsk9 signaling pathway in hyperlipidemic rats. Mol. Med. Rep. 13 (6), 4627–4635. doi:10.3892/mmr.2016.5133
Jia, W., Wang, K., Zhang, S., Lu, W., Du, A., Li, J., et al. (2022). Integrating network pharmacology and in vivo experimental validation to reveal the alleviation of mailuoning oral liquid on non-alcoholic fatty liver disease. J. Artic. Phytomedicine 104, 154326. doi:10.1016/j.phymed.2022.154326
Jiang, H., Mao, T., Liu, Y., Tan, X., Sun, Z., Cheng, Y., et al. (2022). Protective effects and mechanisms of yinchen linggui zhugan decoction in HFD-induced nonalcoholic fatty liver disease rats based on network pharmacology and experimental verification. Front. Pharmacol. 13, 908128. doi:10.3389/fphar.2022.908128
Jiang, W. N., Li, D., Jiang, T., Guo, J., Chen, Y. F., Wang, J., et al. (2018). Protective effects of Chaihu shugan san on nonalcoholic fatty liver disease in rats with insulin resistance. Chin. J. Integr. Med. 24 (2), 125–132. doi:10.1007/s11655-016-2252-4
Karkucinska-Wieckowska, A., Simoes, I. C. M., Kalinowski, P., Lebiedzinska-Arciszewska, M., Zieniewicz, K., Milkiewicz, P., et al. (2022). Mitochondria, oxidative stress and nonalcoholic fatty liver disease: a complex relationship. Eur. J. Clin. Invest 52 (3), e13622. doi:10.1111/eci.13622
Kaufman, R. J. (1999). Stress signaling from the lumen of the endoplasmic reticulum: coordination of gene transcriptional and translational controls. Genes Dev. 13 (10), 1211–1233. doi:10.1101/gad.13.10.1211
Khan, R. S., Bril, F., Cusi, K., and Newsome, P. N. (2019). Modulation of insulin resistance in nonalcoholic fatty liver disease. Hepatology 70 (2), 711–724. doi:10.1002/hep.30429
Kim, I., Xu, W., and Reed, J. C. (2008). Cell death and endoplasmic reticulum stress: disease relevance and therapeutic opportunities. Nat. Rev. Drug Discov. 7 (12), 1013–1030. doi:10.1038/nrd2755
Kim, M. S., Krawczyk, S. A., Doridot, L., Fowler, A. J., Wang, J. X., Trauger, S. A., et al. (2016). ChREBP regulates fructose-induced glucose production independently of insulin signaling. J. Clin. Invest 126 (11), 4372–4386. doi:10.1172/jci81993
Kirakosyan, A., Seymour, E., Kaufman, P. B., Warber, S., Bolling, S., and Chang, S. C. (2003). Antioxidant capacity of polyphenolic extracts from leaves of Crataegus laevigata and Crataegus monogyna (Hawthorn) subjected to drought and cold stress. J. Agric. Food Chem. 51 (14), 3973–3976. doi:10.1021/jf030096r
Kohjima, M., Enjoji, M., Higuchi, N., Kato, M., Kotoh, K., Yoshimoto, T., et al. (2007). Re-evaluation of fatty acid metabolism-related gene expression in nonalcoholic fatty liver disease. Int. J. Mol. Med. 20 (3), 351–358. doi:10.3892/ijmm.20.3.351
Kumashiro, N., Erion, D. M., Zhang, D., Kahn, M., Beddow, S. A., Chu, X., et al. (2011). Cellular mechanism of insulin resistance in nonalcoholic fatty liver disease. Proc. Natl. Acad. Sci. U. S. A. 108 (39), 16381–16385. doi:10.1073/pnas.1113359108
Lan, T., Yu, Y., Zhang, J., Li, H., Weng, Q., Jiang, S., et al. (2021). Cordycepin ameliorates nonalcoholic steatohepatitis by activation of the AMP-activated protein kinase signaling pathway. Hepatology 74 (2), 686–703. doi:10.1002/hep.31749
Lan Q., Q., Ren, Z., Chen, Y., Cui, G., Choi, I. C., Ung, C., et al. (2021). Hepatoprotective effect of Qushihuayu formula on non-alcoholic steatohepatitis induced by MCD diet in rat. Chin. Med. 16 (1), 27. doi:10.1186/s13020-021-00434-1
Law, B. Y., Mo, J. F., and Wong, V. K. (2014). Autophagic effects of Chaihu (dried roots of Bupleurum chinense DC or Bupleurum scorzoneraefolium WILD). Chin. Med. 9, 21. doi:10.1186/1749-8546-9-21
Lei, S., Zhao, S., Huang, X., Feng, Y., Li, Z., Chen, L., et al. (2022). Chaihu Shugan powder alleviates liver inflammation and hepatic steatosis in NAFLD mice: a network pharmacology study and in vivo experimental validation. Front. Pharmacol. 13, 967623. doi:10.3389/fphar.2022.967623
Leng, J., Huang, F., Hai, Y., Tian, H., Liu, W., Fang, Y., et al. (2020). Amelioration of non-alcoholic steatohepatitis by Qushi Huayu decoction is associated with inhibition of the intestinal mitogen-activated protein kinase pathway. Phytomedicine 66, 153135. doi:10.1016/j.phymed.2019.153135
Li, C., Chen, Y., Yuan, X., He, L., Li, X., Huang, S., et al. (2020). Vitexin ameliorates chronic stress plub high fat diet-induced nonalcoholic fatty liver disease by inhibiting inflammation. Eur. J. Pharmacol. 882, 173264. doi:10.1016/j.ejphar.2020.173264
Li, C., Yu, S., Li, X., Cao, Y., Li, M., Ji, G., et al. (2022). Medicinal formula huazhi-rougan attenuates non-alcoholic steatohepatitis through enhancing fecal bile acid excretion in mice. Front. Pharmacol. 13, 833414. doi:10.3389/fphar.2022.833414
Li, Q., and Qu, H. (2012). Study on the hypoglycemic activities and metabolism of alcohol extract of Alismatis Rhizoma. Fitoterapia 83 (6), 1046–1053. doi:10.1016/j.fitote.2012.05.009
Li, S., Qian, Q., Ying, N., Lai, J., Feng, L., Zheng, S., et al. (2020). Activation of the AMPK-SIRT1 pathway contributes to protective effects of Salvianolic acid A against lipotoxicity in hepatocytes and NAFLD in mice. Front. Pharmacol. 11, 560905. doi:10.3389/fphar.2020.560905
Li, W., Cao, T., Luo, C., Cai, J., Zhou, X., Xiao, X., et al. (2020). Crosstalk between ER stress, NLRP3 inflammasome, and inflammation. Appl. Microbiol. Biotechnol. 104 (14), 6129–6140. doi:10.1007/s00253-020-10614-y
Li, X., Li, X., Huang, N., Liu, R., and Sun, R. (2018). A comprehensive review and perspectives on pharmacology and toxicology of saikosaponins. Phytomedicine 50, 73–87. doi:10.1016/j.phymed.2018.09.174
Lin, X. P., and Yu, L. H. (2021). Therapeutic effect analysis of rhubaric acid on non-alcoholic fatty liver disease in mice. Chin. J. Clin. Med. 28 (01), 106–110. doi:10.12025/j.issn.1008-6358.2021.20200155
Liu, W., Shang, J., Deng, Y., Han, X., Chen, Y., Wang, S., et al. (2022). Network pharmacology analysis on mechanism of Jian Pi Qing Gan Yin decoction ameliorating high fat diet-induced non-alcoholic fatty liver disease and validated in vivo. J. Ethnopharmacol. 295, 115382. doi:10.1016/j.jep.2022.115382
Liu, Y., Tan, Y., Huang, J., Wu, C., Fan, X., Stalin, A., et al. (2022). Revealing the mechanism of huazhi rougan granule in the treatment of nonalcoholic fatty liver through intestinal flora based on 16S rRNA, metagenomic sequencing and network pharmacology. Front. Pharmacol. 13, 875700. doi:10.3389/fphar.2022.875700
Lu, H. F., Lai, Y. H., Huang, H. C., Lee, I. J., Lin, L. C., Liu, H. K., et al. (2020). Ginseng-plus-Bai-Hu-Tang ameliorates diet-induced obesity, hepatic steatosis, and insulin resistance in mice. J. Ginseng Res. 44 (2), 238–246. doi:10.1016/j.jgr.2018.10.005
Luo, D., Yang, L., Pang, H., Zhao, Y., Li, K., Rong, X., et al. (2022). Tianhuang formula reduces the oxidative stress response of NAFLD by regulating the gut microbiome in mice. Front. Microbiol. 13, 984019. doi:10.3389/fmicb.2022.984019
Luo, H., Wu, H., Wang, L., Xiao, S., Lu, Y., Liu, C., et al. (2021). Hepatoprotective effects of Cassiae Semen on mice with non-alcoholic fatty liver disease based on gut microbiota. Commun. Biol. 4 (1), 1357. doi:10.1038/s42003-021-02883-8
Machado, M. V., and Cortez-Pinto, H. (2014). Non-alcoholic fatty liver disease: what the clinician needs to know. World J. Gastroenterol. 20 (36), 12956–12980. doi:10.3748/wjg.v20.i36.12956
Mansouri, A., Gattolliat, C. H., and Asselah, T. (2018). Mitochondrial dysfunction and signaling in chronic liver diseases. Gastroenterology 155 (3), 629–647. doi:10.1053/j.gastro.2018.06.083
Masarone, M., Rosato, V., Dallio, M., Gravina, A. G., Aglitti, A., Loguercio, C., et al. (2018). Role of oxidative stress in pathophysiology of nonalcoholic fatty liver disease. Oxid. Med. Cell Longev. 2018, 9547613. doi:10.1155/2018/9547613
Meng, Q., Duan, X. P., Wang, C. Y., Liu, Z. H., Sun, P. Y., Huo, X. K., et al. (2017). Alisol B 23-acetate protects against non-alcoholic steatohepatitis in mice via farnesoid X receptor activation. Acta Pharmacol. Sin. 38 (1), 69–79. doi:10.1038/aps.2016.119
Meng, Y., Liu, Y., Fang, N., and Guo, Y. (2019). Hepatoprotective effects of Cassia semen ethanol extract on non-alcoholic fatty liver disease in experimental rat. Pharm. Biol. 57 (1), 98–104. doi:10.1080/13880209.2019.1568509
Muzurović, E., Mikhailidis, D. P., and Mantzoros, C. (2021). Non-alcoholic fatty liver disease, insulin resistance, metabolic syndrome and their association with vascular risk. Metabolism 119, 154770. doi:10.1016/j.metabol.2021.154770
Nassir, F. (2022). NAFLD: mechanisms, treatments, and biomarkers. Biomolecules 12 (6), 824. doi:10.3390/biom12060824
Neuschwander-Tetri, B. A. (2017). Non-alcoholic fatty liver disease. BMC Med. 15 (1), 45. doi:10.1186/s12916-017-0806-8
Neuschwander-Tetri, B. A., Loomba, R., Sanyal, A. J., Lavine, J. E., Van Natta, M. L., Abdelmalek, M. F., et al. (2015). Farnesoid X nuclear receptor ligand obeticholic acid for non-cirrhotic, non-alcoholic steatohepatitis (FLINT): a multicentre, randomised, placebo-controlled trial. Lancet 385 (9972), 956–965. doi:10.1016/s0140-6736(14)61933-4
Newsome, P. N., Buchholtz, K., Cusi, K., Linder, M., Okanoue, T., Ratziu, V., et al. (2021). A placebo-controlled trial of subcutaneous semaglutide in nonalcoholic steatohepatitis. N. Engl. J. Med. 384 (12), 1113–1124. doi:10.1056/NEJMoa2028395
Nguyen, P., Leray, V., Diez, M., Serisier, S., Le Bloc'h, J., Siliart, B., et al. (2008). Liver lipid metabolism. J. Anim. Physiol. Anim. Nutr. Berl. 92 (3), 272–283. doi:10.1111/j.1439-0396.2007.00752.x
Ni, Y., Wang, X., Wu, Q., Yao, Y., Xu, Y., Li, Y., et al. (2023). Qushi Huayu decoction ameliorates non-alcoholic fatty liver disease in rats by modulating gut microbiota and serum lipids. Front. Endocrinol. (Lausanne) 14, 1272214. doi:10.3389/fendo.2023.1272214
Pan, M., Deng, Y., Qiu, Y., Pi, D., Zheng, C., Liang, Z., et al. (2024). Shenling Baizhu powder alleviates non-alcoholic fatty liver disease by modulating autophagy and energy metabolism in high-fat diet-induced rats. Phytomedicine 130, 155712. doi:10.1016/j.phymed.2024.155712
Pan, M. X., Zheng, C. Y., Deng, Y. J., Tang, K. R., Nie, H., Xie, J. Q., et al. (2021). Hepatic protective effects of Shenling Baizhu powder, a herbal compound, against inflammatory damage via TLR4/NLRP3 signalling pathway in rats with nonalcoholic fatty liver disease. J. Integr. Med. 19 (5), 428–438. doi:10.1016/j.joim.2021.07.004
Park, S. W., Zhou, Y., Lee, J., Lu, A., Sun, C., Chung, J., et al. (2010). The regulatory subunits of PI3K, p85alpha and p85beta, interact with XBP-1 and increase its nuclear translocation. Nat. Med. 16 (4), 429–437. doi:10.1038/nm.2099
Pessayre, D., Mansouri, A., and Fromenty, B. (2002). Nonalcoholic steatosis and steatohepatitis. V. Mitochondrial dysfunction in steatohepatitis. Am. J. Physiol. Gastrointest. Liver Physiol. 282 (2), G193–G199. doi:10.1152/ajpgi.00426.2001
Peverill, W., Powell, L. W., and Skoien, R. (2014). Evolving concepts in the pathogenesis of NASH: beyond steatosis and inflammation. Int. J. Mol. Sci. 15 (5), 8591–8638. doi:10.3390/ijms15058591
Polyzos, S. A., Kountouras, J., Mantzoros, C. S., Polymerou, V., and Katsinelos, P. (2017). Effects of combined low-dose spironolactone plus vitamin E vs vitamin E monotherapy on insulin resistance, non-invasive indices of steatosis and fibrosis, and adipokine levels in non-alcoholic fatty liver disease: a randomized controlled trial. Diabetes Obes. Metab. 19 (12), 1805–1809. doi:10.1111/dom.12989
Porras, D., Nistal, E., Martínez-Flórez, S., Pisonero-Vaquero, S., Olcoz, J. L., Jover, R., et al. (2017). Protective effect of quercetin on high-fat diet-induced non-alcoholic fatty liver disease in mice is mediated by modulating intestinal microbiota imbalance and related gut-liver axis activation. Free Radic. Biol. Med. 102, 188–202. doi:10.1016/j.freeradbiomed.2016.11.037
Postic, C., and Girard, J. (2008). Contribution of de novo fatty acid synthesis to hepatic steatosis and insulin resistance: lessons from genetically engineered mice. J. Clin. Invest 118 (3), 829–838. doi:10.1172/jci34275
Puri, P., Mirshahi, F., Cheung, O., Natarajan, R., Maher, J. W., Kellum, J. M., et al. (2008). Activation and dysregulation of the unfolded protein response in nonalcoholic fatty liver disease. Gastroenterology 134 (2), 568–576. doi:10.1053/j.gastro.2007.10.039
Qian, F., Ouyang, B., Cai, Z., Zhu, D., Yu, S., Zhao, J., et al. (2024). Compound Shouwu Jiangzhi Granule regulates triacylglyceride synthesis to alleviate hepatic lipid accumulation. Phytomedicine 129, 155691. doi:10.1016/j.phymed.2024.155691
Qin, B. C., Zhang, T., Yuan, J. F., Lu, X. L., Xu, J. L., Shi, J. P., et al. (2013). Effect of rhubaric acid on prevention and treatment of nonalcoholic fatty liver disease induced by high fat diet in rats. Chin. Arch. Tradit. Chin. Med. 31 (03), 545–547+709. doi:10.13193/j.archtcm.2013.03.99.cenbch.054
Qiu, M., Xiao, F., Wang, T., Piao, S., Zhao, W., Shao, S., et al. (2020). Protective effect of Hedansanqi Tiaozhi Tang against non-alcoholic fatty liver disease in vitro and in vivo through activating Nrf2/HO-1 antioxidant signaling pathway. Phytomedicine 67, 153140. doi:10.1016/j.phymed.2019.153140
Ruan, X., Zhang, X., Liu, L., and Zhang, J. (2024). Mechanism of Xiaoyao San in treating non-alcoholic fatty liver disease with liver depression and spleen deficiency: based on bioinformatics, metabolomics and in vivo experiments. J. Biomol. Struct. Dyn. 42 (10), 5128–5146. doi:10.1080/07391102.2023.2231544
Sanders, F. W., and Griffin, J. L. (2016). De novo lipogenesis in the liver in health and disease: more than just a shunting yard for glucose. Biol. Rev. Camb Philos. Soc. 91 (2), 452–468. doi:10.1111/brv.12178
Sanyal, A. J., Chalasani, N., Kowdley, K. V., McCullough, A., Diehl, A. M., Bass, N. M., et al. (2010). Pioglitazone, vitamin E, or placebo for nonalcoholic steatohepatitis. N. Engl. J. Med. 362 (18), 1675–1685. doi:10.1056/NEJMoa0907929
Sanyal, A. J., Mofrad, P. S., Contos, M. J., Sargeant, C., Luketic, V. A., Sterling, R. K., et al. (2004). A pilot study of vitamin E versus vitamin E and pioglitazone for the treatment of nonalcoholic steatohepatitis. Clin. Gastroenterol. Hepatol. 2 (12), 1107–1115. doi:10.1016/s1542-3565(04)00457-4
Schreuder, T. C., Verwer, B. J., van Nieuwkerk, C. M., and Mulder, C. J. (2008). Nonalcoholic fatty liver disease: an overview of current insights in pathogenesis, diagnosis and treatment. World J. Gastroenterol. 14 (16), 2474–2486. doi:10.3748/wjg.14.2474
Schrieber, S. J., Hawke, R. L., Wen, Z., Smith, P. C., Reddy, K. R., Wahed, A. S., et al. (2011). Differences in the disposition of silymarin between patients with nonalcoholic fatty liver disease and chronic hepatitis C. Drug Metab. Dispos. 39 (12), 2182–2190. doi:10.1124/dmd.111.040212
Schweiger, M., Schreiber, R., Haemmerle, G., Lass, A., Fledelius, C., Jacobsen, P., et al. (2006). Adipose triglyceride lipase and hormone-sensitive lipase are the major enzymes in adipose tissue triacylglycerol catabolism. J. Biol. Chem. 281 (52), 40236–40241. doi:10.1074/jbc.M608048200
Seino, Y., Fukushima, M., and Yabe, D. (2010). GIP and GLP-1, the two incretin hormones: similarities and differences. J. Diabetes Investig. 1 (1-2), 8–23. doi:10.1111/j.2040-1124.2010.00022.x
Serviddio, G., Bellanti, F., and Vendemiale, G. (2013). Free radical biology for medicine: learning from nonalcoholic fatty liver disease. Free Radic. Biol. Med. 65, 952–968. doi:10.1016/j.freeradbiomed.2013.08.174
Shang, Z., Gao, Y., Xue, Y., Zhang, C., Qiu, J., Qian, Y., et al. (2024). Shenge Formula attenuates high-fat diet-induced obesity and fatty liver via inhibiting ACOX1. Phytomedicine 123, 155183. doi:10.1016/j.phymed.2023.155183
Shao, G., Liu, Y., Lu, L., Zhang, G., Zhou, W., Wu, T., et al. (2022). The pathogenesis of HCC driven by NASH and the preventive and therapeutic effects of natural products. Front. Pharmacol. 13, 944088. doi:10.3389/fphar.2022.944088
Shen, S., Wang, K., Zhi, Y., Shen, W., and Huang, L. (2020). Gypenosides improves nonalcoholic fatty liver disease induced by high-fat diet induced through regulating LPS/TLR4 signaling pathway. Cell Cycle 19 (22), 3042–3053. doi:10.1080/15384101.2020.1829800
Sheng, X., Wang, M., Lu, M., Xi, B., Sheng, H., and Zang, Y. Q. (2011). Rhein ameliorates fatty liver disease through negative energy balance, hepatic lipogenic regulation, and immunomodulation in diet-induced obese mice. Am. J. Physiol. Endocrinol. Metab. 300 (5), E886–E893. doi:10.1152/ajpendo.00332.2010
Shi, H., Qiao, F., Huang, K., Lu, W., Zhang, X., Ke, Z., et al. (2022). Exploring therapeutic mechanisms of San-Huang-Tang in nonalcoholic fatty liver disease through network pharmacology and experimental validation. J. Ethnopharmacol. 296, 115477. doi:10.1016/j.jep.2022.115477
Shi, M. J., Dong, B. S., Yang, W. N., Su, S. B., and Zhang, H. (2019). Preventive and therapeutic role of Tanshinone ⅡA in hepatology. Biomed. Pharmacother. 112, 108676. doi:10.1016/j.biopha.2019.108676
Shi, T., Wu, L., Ma, W., Ju, L., Bai, M., Chen, X., et al. (2020). Nonalcoholic fatty liver disease: pathogenesis and treatment in traditional Chinese medicine and western medicine. Evid. Based Complement. Altern. Med. 2020, 8749564. doi:10.1155/2020/8749564
Song, D., Yin, L., Wang, C., and Wen, X. (2020). Zhenqing recipe attenuates non-alcoholic fatty liver disease by regulating the SIK1/CRTC2 signaling in experimental diabetic rats. BMC Complement. Med. Ther. 20 (1), 27. doi:10.1186/s12906-019-2811-2
Stefan, N., Kantartzis, K., and Häring, H. U. (2008). Causes and metabolic consequences of Fatty liver. Endocr. Rev. 29 (7), 939–960. doi:10.1210/er.2008-0009
Stofan, M., and Guo, G. L. (2020). Bile acids and FXR: novel targets for liver diseases. Front. Med. (Lausanne) 7, 544. doi:10.3389/fmed.2020.00544
Tadić, V. M., Dobrić, S., Marković, G. M., Dordević, S. M., Arsić, I. A., Menković, N. R., et al. (2008). Anti-inflammatory, gastroprotective, free-radical-scavenging, and antimicrobial activities of hawthorn berries ethanol extract. J. Agric. Food Chem. 56 (17), 7700–7709. doi:10.1021/jf801668c
Tan, Y., Huang, Z., Liu, Y., Li, X., Stalin, A., Fan, X., et al. (2023). Integrated serum pharmacochemistry, 16S rRNA sequencing and metabolomics to reveal the material basis and mechanism of Yinzhihuang granule against non-alcoholic fatty liver disease. J. Ethnopharmacol. 310, 116418. doi:10.1016/j.jep.2023.116418
Tang, N., Ji, L., Shi, X., Xiong, Y., Xiong, X., Zhao, H., et al. (2024). Effects of ganjianglingzhu decoction on lean non-alcoholic fatty liver disease in mice based on untargeted metabolomics. Pharm. (Basel) 17 (4), 502. doi:10.3390/ph17040502
Teng, M. L., Ng, C. H., Huang, D. Q., Chan, K. E., Tan, D. J., Lim, W. H., et al. (2023). Global incidence and prevalence of nonalcoholic fatty liver disease. Clin. Mol. Hepatol. 29 (Suppl. l), S32–s42. doi:10.3350/cmh.2022.0365
Ter Horst, K. W., Gilijamse, P. W., Versteeg, R. I., Ackermans, M. T., Nederveen, A. J., la Fleur, S. E., et al. (2017). Hepatic diacylglycerol-associated protein kinase cε translocation links hepatic steatosis to hepatic insulin resistance in humans. Cell Rep. 19 (10), 1997–2004. doi:10.1016/j.celrep.2017.05.035
Tian, H., Fang, Y., Liu, W., Wang, J., Zhao, J., Tang, H., et al. (2023). Inhibition on XBP1s-driven lipogenesis by Qushi Huayu Decoction contributes to amelioration of hepatic steatosis induced by fructose. J. Ethnopharmacol. 301, 115806. doi:10.1016/j.jep.2022.115806
Tilg, H., and Moschen, A. R. (2010). Evolution of inflammation in nonalcoholic fatty liver disease: the multiple parallel hits hypothesis. Hepatology 52 (5), 1836–1846. doi:10.1002/hep.24001
van Stee, M. F., de Graaf, A. A., and Groen, A. K. (2018). Actions of metformin and statins on lipid and glucose metabolism and possible benefit of combination therapy. Cardiovasc Diabetol. 17 (1), 94. doi:10.1186/s12933-018-0738-4
Wang, H., Xu, Z., Wang, Q., and Shu, S. (2022). Danshao Shugan Granule therapy for non-alcoholic fatty liver disease. Lipids Health Dis. 21 (1), 76. doi:10.1186/s12944-022-01689-9
Wang, M., Xie, T., Chang, Z., Wang, L., Xie, X., Kou, Y., et al. (2015). A new type of liquid silymarin proliposome containing bile salts: its preparation and improved hepatoprotective effects. PLoS One 10 (12), e0143625. doi:10.1371/journal.pone.0143625
Wang, Y. C., Kong, W. Z., Jin, Q. M., Chen, J., and Dong, L. (2015). Effects of salvianolic acid B on liver mitochondria of rats with nonalcoholic steatohepatitis. World J. Gastroenterol. 21 (35), 10104–10112. doi:10.3748/wjg.v21.i35.10104
Winnay, J. N., Boucher, J., Mori, M. A., Ueki, K., and Kahn, C. R. (2010). A regulatory subunit of phosphoinositide 3-kinase increases the nuclear accumulation of X-box-binding protein-1 to modulate the unfolded protein response. Nat. Med. 16 (4), 438–445. doi:10.1038/nm.2121
Wu, C., Jing, M., Yang, L., Jin, L., Ding, Y., Lu, J., et al. (2018). Alisol A 24-acetate ameliorates nonalcoholic steatohepatitis by inhibiting oxidative stress and stimulating autophagy through the AMPK/mTOR pathway. Chem. Biol. Interact. 291, 111–119. doi:10.1016/j.cbi.2018.06.005
Wu, Y. L., Wu, J. X., Shen, T. T., Chai, H. S., Chen, H. F., and Zhang, Q. (2022). Quzhi formula alleviates nonalcoholic steatohepatitis by impairing hepatocyte lipid accumulation and inflammation via bip/eIF2α signaling. J. Clin. Transl. Hepatol. 10 (6), 1050–1058. doi:10.14218/JCTH.2021.00458
MEIm, X. D., Cao, Y. F., Che, Y. Y., Li, J., Shang, Z. P., Zhao, W. J., et al. (2019). Danshen: a phytochemical and pharmacological overview. Chin. J. Nat. Med. 17 (1), 59–80. doi:10.1016/s1875-5364(19)30010-x
Xiaoling, L., Fengxia, S., Zimeng, S., Yingxue, Z., Jie, L., and Qiuxiang, Z. (2022). Effect of Dangfei Liganning capsule on liver X receptor α/steroid regulatory element binding protein-1/fatty acid synthase signal pathway in rats with metabolic-associated fatty liver disease. J. Traditional Chin. Med. 42 (06), 940–947. doi:10.19852/j.cnki.jtcm.2022.06.007
Xu, J., Jin, Y., Song, C., Chen, G., Li, Q., Yuan, H., et al. (2023). Comparative analysis of the synergetic effects of Diwuyanggan prescription on high fat diet-induced non-alcoholic fatty liver disease using untargeted metabolomics. Heliyon 9 (11), e22151. doi:10.1016/j.heliyon.2023.e22151
Xu, L., and Cui, H. (2023). Yinchenhao Tang alleviates high fat diet induced NAFLD by increasing NR1H4 and APOA1 expression. J. Tradit. Complement. Med. 13 (4), 325–336. doi:10.1016/j.jtcme.2023.02.010
Yan, B. F., Wang, Y., Wang, W. B., Ding, X. J., Wei, B., Liu, S. J., et al. (2023). Huangqin decoction mitigates hepatic inflammation in high-fat diet-challenged rats by inhibiting TLR4/NF-κB/NLRP3 pathway. J. Ethnopharmacol. 303, 115999. doi:10.1016/j.jep.2022.115999
Yang, G. L., Jia, L. Q., Wu, J., Ma, Y. X., Cao, H. M., Song, N., et al. (2017). Effect of tanshinone IIA on oxidative stress and apoptosis in a rat model of fatty liver. Exp. Ther. Med. 14 (5), 4639–4646. doi:10.3892/etm.2017.5162
Yang, H., Wei, D., Zhang, Y., and Jian, W. (2024). Huangqin-huanglian decoction protects liver against non-alcoholic fatty liver disease in high fat-diet mice. Endocr. Metab. Immune Disord. Drug Targets 24 (6), 691–708. doi:10.2174/0118715303257018230927182802
Yang, H., Yang, T., Heng, C., Zhou, Y., Jiang, Z., Qian, X., et al. (2019). Quercetin improves nonalcoholic fatty liver by ameliorating inflammation, oxidative stress, and lipid metabolism in db/db mice. Phytother. Res. 33 (12), 3140–3152. doi:10.1002/ptr.6486
Yang, J. M., Sun, Y., Wang, M., Zhang, X. L., Zhang, S. J., Gao, Y. S., et al. (2019). Regulatory effect of a Chinese herbal medicine formula on non-alcoholic fatty liver disease. World J. Gastroenterol. 25 (34), 5105–5119. doi:10.3748/wjg.v25.i34.5105
Yin, J., Luo, Y., Deng, H., Qin, S., Tang, W., Zeng, L., et al. (2014). Hugan Qingzhi medication ameliorates hepatic steatosis by activating AMPK and PPARα pathways in L02 cells and HepG2 cells. J. Ethnopharmacol. 154 (1), 229–239. doi:10.1016/j.jep.2014.04.011
Yoo, J. H., Liu, Y., and Kim, H. S. (2016). Hawthorn fruit extract elevates expression of Nrf2/HO-1 and improves lipid profiles in ovariectomized rats. Nutrients 8 (5), 283. doi:10.3390/nu8050283
You, L., Wang, T., Li, W., Zhang, J., Zheng, C., Zheng, Y., et al. (2024). Xiaozhi formula attenuates non-alcoholic fatty liver disease by regulating lipid metabolism via activation of AMPK and PPAR pathways. J. Ethnopharmacol. 329, 118165. doi:10.1016/j.jep.2024.118165
Younossi, Z. M., Koenig, A. B., Abdelatif, D., Fazel, Y., Henry, L., and Wymer, M. (2016). Global epidemiology of nonalcoholic fatty liver disease-Meta-analytic assessment of prevalence, incidence, and outcomes. Hepatology 64 (1), 73–84. doi:10.1002/hep.28431
Younossi, Z. M., Wong, G., Anstee, Q. M., and Henry, L. (2023). The global burden of liver disease. Clin. Gastroenterol. Hepatol. 21 (8), 1978–1991. doi:10.1016/j.cgh.2023.04.015
Yu, X., Hao, M., Liu, Y., Ma, X., Lin, W., Xu, Q., et al. (2019). Liraglutide ameliorates non-alcoholic steatohepatitis by inhibiting NLRP3 inflammasome and pyroptosis activation via mitophagy. Eur. J. Pharmacol. 864, 172715. doi:10.1016/j.ejphar.2019.172715
Zeng, L., Lu, M., Mori, K., Luo, S., Lee, A. S., Zhu, Y., et al. (2004). ATF6 modulates SREBP2-mediated lipogenesis. Embo J. 23 (4), 950–958. doi:10.1038/sj.emboj.7600106
Zeng, W., Shan, W., Gao, L., Gao, D., Hu, Y., Wang, G., et al. (2015). Inhibition of HMGB1 release via salvianolic acid B-mediated SIRT1 up-regulation protects rats against non-alcoholic fatty liver disease. Sci. Rep. 5, 16013. doi:10.1038/srep16013
Zhang, B., Ni, M., Li, X., Liu, Q., Hu, Y., and Zhao, Y. (2021). QSHY granules promote white adipose tissue browning and correct BCAAs metabolic disorder in NAFLD mice. Diabetes Metab. Syndr. Obes. 14, 4241–4251. doi:10.2147/DMSO.S332659
Zhang, J., Du, H., Shen, M., Zhao, Z., and Ye, X. (2020). Kangtaizhi granule alleviated nonalcoholic fatty liver disease in high-fat diet-fed rats and HepG2 cells via AMPK/mTOR signaling pathway. J. Immunol. Res. 2020, 3413186. doi:10.1155/2020/3413186
Zhang, J., Zhao, Y., Xu, C., Hong, Y., Lu, H., Wu, J., et al. (2014). Association between serum free fatty acid levels and nonalcoholic fatty liver disease: a cross-sectional study. Sci. Rep. 4, 5832. doi:10.1038/srep05832
Zhang, L., Wang, X., Cueto, R., Effi, C., Zhang, Y., Tan, H., et al. (2019). Biochemical basis and metabolic interplay of redox regulation. Redox Biol. 26, 101284. doi:10.1016/j.redox.2019.101284
Zhang, L. L., Xu, W., Xu, Y. L., Chen, X., Huang, M., and Lu, J. J. (2017). Therapeutic potential of Rhizoma Alismatis: a review on ethnomedicinal application, phytochemistry, pharmacology, and toxicology. Ann. N. Y. Acad. Sci. 1401 (1), 90–101. doi:10.1111/nyas.13381
Zhang, M., Yuan, Y., Wang, Q., Li, X., Men, J., and Lin, M. (2018). The Chinese medicine Chai Hu Li Zhong Tang protects against non-alcoholic fatty liver disease by activating AMPKα. Biosci. Rep. 38 (6). doi:10.1042/BSR20180644
Zhang, P., Cao, J., Liang, X., Su, Z., Zhang, B., Wang, Z., et al. (2024). Lian-Mei-Yin formula alleviates diet-induced hepatic steatosis by suppressing Yap1/FOXM1 pathway-dependent lipid synthesis. Acta Biochim. Biophys. Sin. (Shanghai) 56 (4), 621–633. doi:10.3724/abbs.2024025
Zhang, S. S., and Li, J. X. (2017). Expert consensus on TCM diagnosis and treatment of nonalcoholic fatty liver disease (2017). J. Clin. Hepatobiliary Dis. 33 (12), 2270–2274. doi:10.3969/j.issn.1001-5256.2017.12.002
Zhang, Z., Chang, Q., Zhu, M., Huang, Y., Ho, W. K., and Chen, Z. (2001). Characterization of antioxidants present in hawthorn fruits. J. Nutr. Biochem. 12 (3), 144–152. doi:10.1016/s0955-2863(00)00137-6
Zhang, Z., Huang, Y., Fan, Y., and Liu, M. (2018). Effect of Xuezhikang on hepatitis and oxidative stress in rats with nonalcoholic fatty liver disease. Clin. J. Med. Officers 46 (6), 605–609. doi:10.16680/j.1671-3826.2018.06.01
Zhao, W., Yu, Y., Liu, L., and Wang, Y. (2014). Experimental study of Kezhi capsule on PPAR-γand IR expression in non-alcoholic steatohepatitis rats. Chin. J. Integr. Traditional West. Med. Dig. 22 (9), 501–505. doi:10.3969/j.issn.1671-038X.2014.09.04
Zhao, Z., Deng, Z. T., Huang, S., Ning, M., Feng, Y., Shen, Y., et al. (2022). Alisol B alleviates hepatocyte lipid accumulation and lipotoxicity via regulating rarα-pparγ-CD36 cascade and attenuates non-alcoholic steatohepatitis in mice. Nutrients 14 (12), 2411. doi:10.3390/nu14122411
Zhao, Z. Y., Liu, D., Cao, W. J., Sun, M., Song, M. S., Wang, W., et al. (2018). Association between IgG N-glycans and nonalcoholic fatty liver disease in han Chinese. Biomed. Environ. Sci. 31 (6), 454–458. doi:10.3967/bes2018.059
Keywords: non-alcoholic fatty liver disease (NAFLD), Chinese herb medicine (CHM), insulin resistance, lipid metabolism disorder, mitochondrial dysfunction, oxidative stress, endoplasmic reticulum stress
Citation: Liu Y, Fan Y, Liu J, Liu X, Li X and Hu J (2024) Application and mechanism of Chinese herb medicine in the treatment of non-alcoholic fatty liver disease. Front. Pharmacol. 15:1499602. doi: 10.3389/fphar.2024.1499602
Received: 21 September 2024; Accepted: 30 October 2024;
Published: 13 November 2024.
Edited by:
Qinge Ma, Jiangxi University of Traditional Chinese Medicine, ChinaReviewed by:
Li Hui, Guangzhou University of Chinese Medicine, ChinaCopyright © 2024 Liu, Fan, Liu, Liu, Li and Hu. This is an open-access article distributed under the terms of the Creative Commons Attribution License (CC BY). The use, distribution or reproduction in other forums is permitted, provided the original author(s) and the copyright owner(s) are credited and that the original publication in this journal is cited, in accordance with accepted academic practice. No use, distribution or reproduction is permitted which does not comply with these terms.
*Correspondence: Jingqing Hu, Z2NwMzA2QDEyNi5jb20=
†These authors have contributed equally to this work and share first authorship
Disclaimer: All claims expressed in this article are solely those of the authors and do not necessarily represent those of their affiliated organizations, or those of the publisher, the editors and the reviewers. Any product that may be evaluated in this article or claim that may be made by its manufacturer is not guaranteed or endorsed by the publisher.
Research integrity at Frontiers
Learn more about the work of our research integrity team to safeguard the quality of each article we publish.