- 1Ningxia Medical University, Ningxia of Traditional Chinese Medicine, Yinchuan, China
- 2Key Laboratory of Ningxia Minority Medicine Modernization, Ministry of Education, Ningxia Medical University, Yinchuan, China
Radix Codonopsis (Dangshen), derived from the dried root of plants in the Campanulaceae family, is a widely used Chinese herbal medicine. It is renowned for its pharmacological effects, including tonifying the middle qi, invigorating the spleen, benefiting the lungs, enhancing immunity, and nourishing the blood. Codonopsis extract is frequently incorporated into health products such as tablets and capsules, making it accessible for daily health maintenance. Additionally, it is commonly used in dietary applications like soups, teas, and porridges to nourish qi, enrich blood, and promote overall vitality. In recent years, increasing attention has been given to the anti-cancer potential of Radix Codonopsis. Studies have identified key active components such as luteolin, stigmasterol, polyacetylenes, lobetyolin, and glycitein, which exhibit anti-tumor properties through mechanisms like inhibiting cancer cell growth and proliferation, suppressing epithelial-mesenchymal transition (EMT), and inducing apoptosis. This review highlights the research progress on Radix Codonopsis, including its active constituents, anti-cancer mechanisms, and its role in the convergence of medicine and food in modern life. By doing so, it aims to provide valuable insights and references for future scientific studies and clinical applications of Radix Codonopsis.
1 Introduction
According to the latest cancer statistics of the International Agency for Research on Cancer (IARC), nearly 20 million new cancer cases were reported worldwide in 2022, and this number is expected to exceed 35 million by 2050 (Bray et al., 2024). These figures underscore that cancer remains a major global public health issue. Cancer cells have the ability to invade surrounding tissues and organs and can spread to other parts of the body through hematogenous or lymphatic metastasis. Additionally, many cancers do not present obvious symptoms in their early stages, making early detection difficult. As a result, they are often diagnosed at more advanced stages, complicating treatment efforts. Current cancer treatments primarily involve surgery and chemotherapy; however, chemotherapy is frequently associated with significant side effects that can substantially affect patients’ quality of life. Thus, there is a pressing need for more effective treatment options that minimize side effects and enhance the efficacy of chemotherapy.
Herbal medicine is a treasure trove of nature, and thousands of plants are used in medicine (Wang et al., 2016; Zeng et al., 2020; Gao et al., 2022), including many natural herbal extracts. These extracts are widely used in pharmaceutical research and drug development and have a variety of medical values (Jiang et al., 2018; Yi-wen et al., 2018; He et al., 2023). In addition, herbal remedies have great potential for the treatment of many diseases, including oncological diseases, and modern scientific research is gradually revealing their mechanisms of action, providing important support for the modernization and internationalization of traditional Chinese medicine (Li et al., 2022; Ruan et al., 2023). Radix Codonopsis, a commonly used tonic herb in traditional Chinese medicine (TCM), is recognized for its ability to invigorate the spleen and lungs, nourish blood, and generate body fluids. The root of Codonopsis pilosula is the primary medicinal part, and its chemical composition is complex, containing various biologically active substances, such as alkaloids, terpenoids, flavonoids, lignins, steroids, and sugars. These components confer a range of pharmacological effects, including neuroprotective properties, regulation of blood glucose and lipids, immune system modulation, digestive system support, improvement of blood circulation, and antibacterial and antiviral effects (Yang et al., 2024). In terms of anti-cancer potential, Radix Codonopsis has shown significant promise (Chen et al., 2018; Xin et al., 2012). Clinically, Radix Codonopsis is used to treat a variety of tumors, such as lung cancer, gastric cancer, liver cancer, pancreatic cancer, colorectal cancer, and so on (Zhao et al., 2024).
This paper provides a comprehensive review of the anticancer effects of Radix Codonopsis, aiming to offer insights for its clinical application in cancer treatment. An overview of the research flow is presented in Figure 1.
2 Network diagram of anti-tumor effect of Radix Codonopsis
Network Pharmacology is a multidisciplinary technology that integrates bioinformatics, network science, and pharmacology. It is a method for studying drugs and their mechanisms of action, especially in understanding the characteristics of multi-component, multi-target, and multi-pathway of TCM (Wang et al., 2021). By constructing and analyzing biological molecular networks, we can understand and predict the interactions between drugs and organisms (Noor et al., 2022; Cheng et al., 2024). In cancer research, it can identify key regulatory nodes through the analysis of cancer-related biomolecular networks, which may be potential therapeutic targets. This helps to understand the complex signaling pathways of cancer and provides a theoretical basis for the development of novel anti-tumor drugs targeting multiple targets.
2.1 Acquisition of ingredients and targets of Radix Codonopsis
The chemical composition information of Codonopsis pilosula was searched in TCMSP database (https://old.tcmsp-e.com/tcmsp.php (accessed on June 5, 2024)) with “Dangshen” as the key word. The active ingredients of Radix Codonopsis were screened according to the criteria of oral bioavailability (OB ≥ 30%) and drug class (DL ≥ 0.18), and the results were exported. Then use the RelateTargets column of TCMSP platform to collect relevant targets. For components that cannot successfully search for targets, the Canonical SMILE sequence of compounds can be queried through PubChem (https://pubchem.ncbi.nlm.nih.gov), and the target can be predicted using SwissTargetPrediction database (https://www.swisstargetprediction.ch). Finally, 21 active ingredients and 97 targets were obtained.
2.2 PPI network construction and bioinformatics analysis
The obtained target genes were imported into the STRING database (https://stringdb.org/(accessed on June 11, 2024)), and the Orgnism was set to “Homo sapiens” to obtain the PPI network graph, which was saved in the file format of “tsv” file format. The obtained results were imported into the network visualization tool Cytoscape 3.9.1 to show the network diagrams of the interactions between the 21 active ingredients and 97 corresponding genes. Pathway enrichment analysis of the 97 target genes was then performed using the DAVID database (https://david.ncifcrf.gov (accessed June 12, 2024)): by selecting “GENE SYMBOL” and “HUMAN” and submitting the data, we selected “Homo sapiens” and clicked “KEGG Pathways” to download the pathway files. Finally, the enrichment results were plotted on the online Microbiology website (https://www.bioinformatics.com.cn/login/). The results showed that the major active components of Codonopsis had significant pharmacological effects in cancer-related pathways, such as prostate cancer and bladder cancer, as shown in Figure 2. It indicates that Codonopsis pilosula has good application value in anticancer.
3 Compound attributes of Radix Codonopsis
Radix Codonopsis, a perennial herb from the Campanulaceae family, contains a diverse range of bioactive compounds, each contributing to its pharmacological effects. These compounds have found wide applications in various therapeutic areas. The basic information on the effective components of Radix Codonopsis is summarized in Table 1.
3.1 Flavonoids
Flavonoids are a large class of secondary metabolites commonly found in plants, distributed across various plant tissues. These compounds exhibit a wide range of biological and pharmacological activities, including antioxidant (Procházková et al., 2011), anti-inflammation (Serafini et al., 2010), anti-cancer (Dobrzynska et al., 2020), cardiovascular protection (Mladenka et al., 2010), anti-virus and anti-bacteria (Khater et al., 2019), and are used to treat and prevent various diseases. lavonoids are found in foods such as tea, coffee, soybeans, onions, and apples (Serafini et al., 2010). They belong to phenylpropanoid cycloheptene compounds with the basic structure of 2-phenylchromenone. This basic structure is composed of two benzene rings connected by a three-carbon chain to form a C6-C3-C6 skeleton (Preet et al., 2023). Given the structural diversity and complexity of flavonoids, they are a focal point of research in both phytochemistry and medicinal chemistry. Ongoing studies continue to explore their mechanisms of action in health and disease, as well as their future therapeutic potential.
3.2 Alkaloids
Alkaloids are nitrogen-containing organic compounds, widely distributed in the plant kingdom, known for their biological activity (Bhambhani et al., 2021). Plants such as the opium poppy, Catharanthus roseus, and Ephedra sinica are important alkaloid sources. Alkaloid research has expanded to encompass their biosynthetic pathways (Kishimoto et al., 2016), pharmacokinetics (Ziegler and Facchini, 2008), chemical structure, biological activity (Bhambhani et al., 2021) and potential applications in various fields. Alkaloids are used in clinical settings as pharmaceutical raw materials, such as morphine and its derivative, codeine, which serve as potent analgesics (Chida, 2011). Quinine, another alkaloid, is a primary treatment for malaria (Shanks, 2016). In addition, alkaloids like camptothecin and vincristine are used in cancer treatment (Patel et al., 2022). Vincristine is usually used to treat lymphoma and leukemia (Li et al., 2007). Camptothecin is commonly used in the treatment of ovarian, colorectal, and lung cancers (Al-Ghazzawi, 2019). Berberine, a notable isoquinoline alkaloid, is widely used for treating gastrointestinal diseases such as bacterial diarrhea and infections, while exhibiting antibacterial, anti-inflammatory, hypoglycemic, and anti-tumor properties (Song et al., 2020).
3.3 Steroids
Steroids are organic compounds with a four-ring structure, found in a variety of natural sources, including phytosterols, bile acids, cardiac glycosides, steroidal saponins, and steroidal alkaloids. Steroids are integral to various medical applications due to their unique molecular structure, which contains a parent nucleus known as “cyclopentane polyhydrophenanthrene” (Mensah-Nyagan et al., 2009). This structure confers a wide array of biological activities. In medicine, steroid compounds often mimic or regulate natural hormones in the human body, such as adrenal cortical hormones and sex hormones (Gupta et al., 2013). These compounds are used in hormone replacement therapies and contraceptives. For instance, androgens are used to treat menopausal symptoms, while estrogens and progesterones are key components of oral contraceptives (Frank and Schneider, 2013). Additionally, some steroidal compounds possess anti-tumor effects. For example, steroidal saponins can induce apoptosis, promote autophagy, inhibit tumor cell migration, and cause cell cycle arrest, thereby exhibiting anti-cancer properties (An et al., 2022). Steroids also play roles in anti-inflammatory processes, immune regulation, blood pressure control, and cardiovascular health (Racette et al., 2015; Giles et al., 2018; Li et al., 2021).
3.4 Terpenoids
Terpenoids are a vast class of natural organic compounds, either open-chain or cyclic, derived from isoprene units. As the largest group of plant secondary metabolites, they are central to various biological processes and widely used across industries such as medicine, food, and cosmetics (Bouvier et al., 2005; Nagegowda and Gupta, 2020). Terpenoids exhibit a broad spectrum of biological activities, including antibacterial, anti-inflammatory, anti-tumor, anti-malarial effects, coronary artery dilation, and immune system enhancement. Their specific biological effects are intricately linked to their unique chemical structures. For example, artemisinin, a well-known terpenoid lactone, is extensively used as an anti-malarial agent (O’Neill et al., 2010). Andrographolide, a diterpene derived from Andrographis paniculata, possesses potent antibacterial and anti-inflammatory properties (Zhang et al., 2021). Paclitaxel, another diterpenoid, is widely employed in the treatment of breast and ovarian cancers (Zhu and Chen, 2019). Tanshinone, a diterpene found in Salvia miltiorrhiza, provides cardiovascular protection (Li et al., 2020). Ginsenoside, a triterpenoid, is renowned for its immune-boosting effects (Choi and Kim, 2023). In addition, terpenoids exhibit neuroprotective activity, making them valuable in the treatment of neurodegenerative diseases (Manju and Bharadvaja, 2024). Given their extensive range of biological activities, terpenoids have become a critical focus in pharmaceutical research and development. As studies continue to explore their therapeutic potential, we can expect the emergence of novel drugs based on terpenoid compounds in the future.
4 Chemical structure formula of main anti-cancer components of Radix Codonopsis
Recent studies have demonstrated that Radix Codonopsis contains a variety of active ingredients with significant anticancer properties. The key anticancer components include luteolin, stigmasterol, lobetyolin, glycitein, taraxerol, and others. These compounds exert their anti-cancer effects through various mechanisms, such as inducing tumor cell apoptosis and inhibiting tumor cell proliferation. The chemical structures of these active ingredients are depicted in Figure 3.
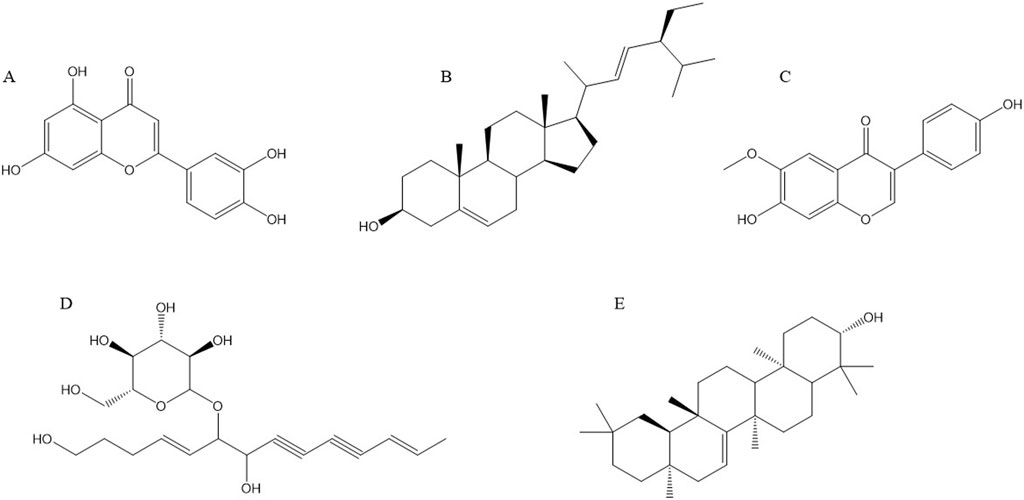
Figure 3. Structural formulae of representative compounds: (A) Luteolin, (B) Stigmasterol, (C) Glycitein, (D) Lobetyolin, (E) Taraxerol.
5 The anti-cancer effect of Radix Codonopsis in various systems
The anticancer effects of Radix Codonopsis’s active ingredients involve multiple systems, including the respiratory, digestive, and reproductive systems (see Figure 4). The mechanisms of action include direct induction of tumor cell apoptosis, inhibition of tumor cell proliferation, and anti-metastatic effects, as summarized in Table 2.
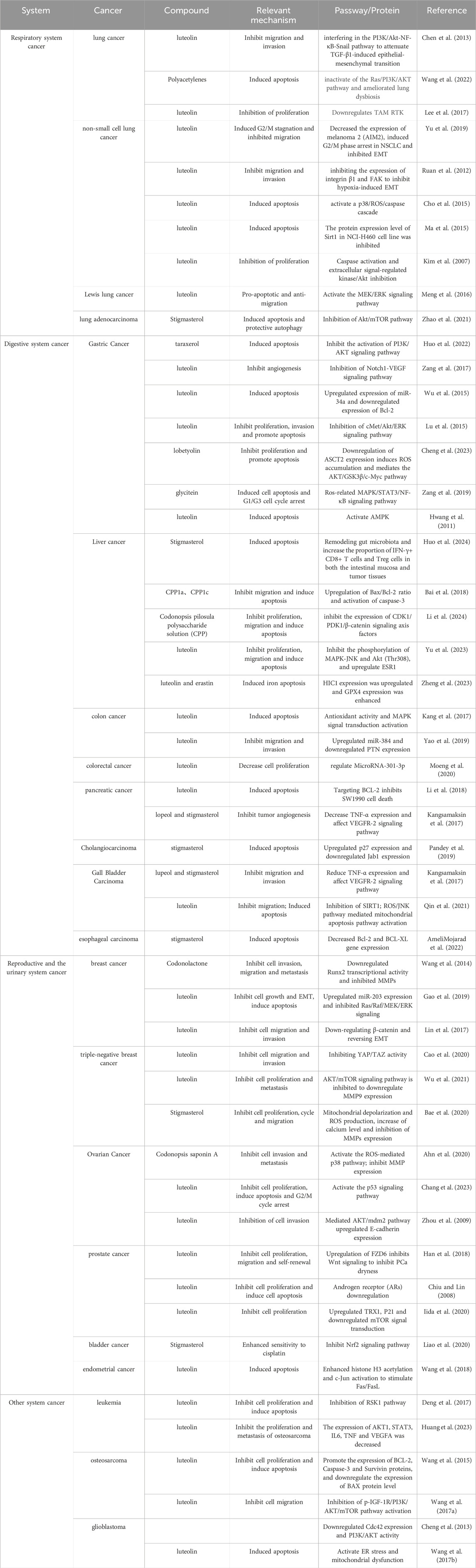
Table 2. Effect and mechanism of Codonopsis pilosula on respiratory system tumors, digestive system tumors, genitourinary system tumors and other system tumors.
5.1 Application in respiratory system cancer
Lung cancer, a malignant tumor originating from lung cells, is the leading cause of cancer-related death worldwide. It is mainly categorized into small-cell lung cancer and non-small-cell lung cancer, with the incidence being significantly higher in men than in women (Sung et al., 2021). The active components of Radix Codonopsis can influence lung cancer by inhibiting tumor cell proliferation and promoting apoptosis, as illustrated in Figure 5. Additionally, Radix Codonopsis has therapeutic effect for nasopharyngeal carcinoma.
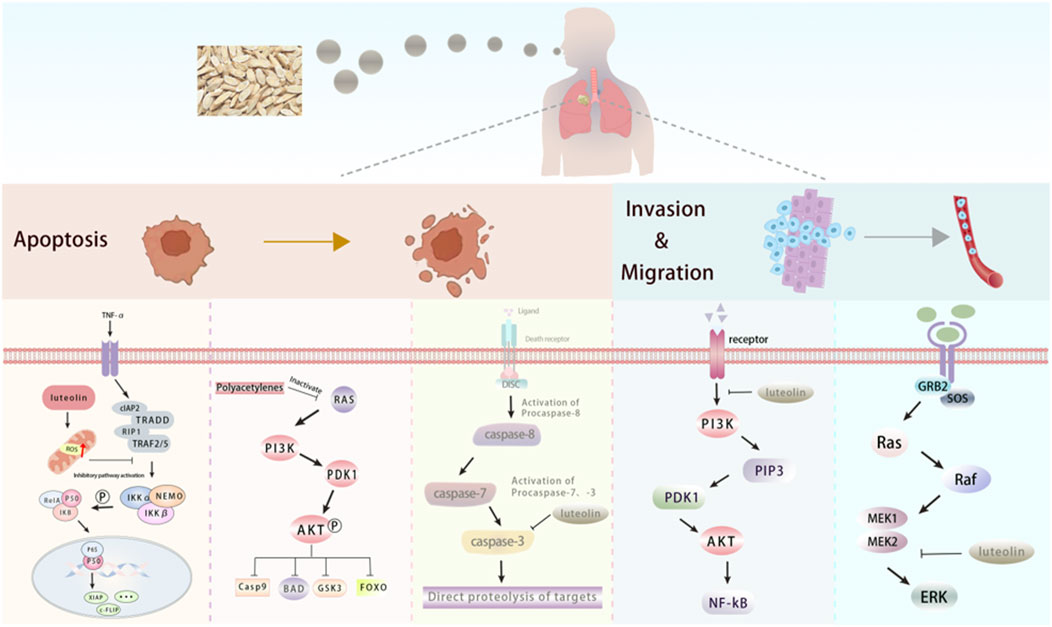
Figure 5. The active components of Radix Codonopsis promote lung cancer cell death and inhibitinvasion and metastasis. luteolin and Polyacetylenes can promote the apoptosis of lung cancercells by inhibiting PI3K/AKT, NF-kB and Caspase pathways. luteolin also attenuated EMT byinhibiting PI3K/AKT/NF-kB pathway and activating MEK/RK signaling pathway.
Polyacetylenes from Radix Codonopsis may induce apoptosis in lung adenocarcinoma cells by inhibiting the Ras/PI3K/AKT pathway, while also improving lung ecological imbalance by increasing microbial diversity and inhibiting common lung pathogens. At the same time, the study found that polyacetylenes did not affect the proliferation of human normal lung epithelial cells (Wang et al., 2022), indicating minimal side effects and strong clinical potential for lung cancer treatment. Luteolin, another bioactive compound in Radix Codonopsis, promotes apoptosis and inhibits migration of A549 lung adenocarcinoma cells by activating the MEK-ERK signaling pathway (Meng et al., 2016). Ma et al. (2015) studied the effect of luteolin on human large-cell lung cancer (NCI-H460) cells and found that luteolin could induce apoptosis of NCI-H460 cells, and its mechanism may be related to the downregulation of Sirt1 expression. The effect was further confirmed by lentivirus knockdown of Sirt1 protein level in NCI-H460 cells. Chen et al. (2013) observed the effect of luteolin on EMT of lung adenocarcinoma A549 cells. The results showed that luteolin inhibited the migration, invasion, and EMT ability of lung adenocarcinoma A549 cells. The mechanism may be related to the inhibition of the TGF-β1-induced EMT process in human lung cancer cells and further indicated that the PI3K/AKT/NF-kB/Snail/E-cadherin signaling pathway is closely related to TGF-β1-mediated EMT. It was found that luteolin significantly attenuated hypoxia-induced EMT in human non-small cell lung cancer cells. Further studies have found that luteolin inhibited the expression of integrin β1 and FAK, combined with the relationship between EMT and integrin β1 and FAK signal transduction, indicating that luteolin inhibited the EMT of human non-small cell lung cancer cells partly due to the inhibition of integrin β1 and FAK expression (Ruan et al., 2012). Yu et al. (2019) found that luteolin reduced the expression of AIM2 at both mRNA and protein levels in the study of the relationship between luteolin interfering with melanoma 2 (AIM2) and non-small cell lung cancer, thus inducing G2/M phase arrest and inhibiting EMT in non-small cell lung cancer (NSCLC). Further analysis revealed that luteolin’s anti-cancer effects in NSCLC are closely associated with AIM2 expression. Specifically, AIM2 knockdown diminished luteolin’s ability to exert its anti-tumor effects, while AIM2 overexpression reinforced luteolin’s therapeutic impact. These findings highlight the critical role of AIM2 in mediating luteolin’s efficacy against NSCLC. In addition, Lee et al. (2017) showed that luteolin can inhibit the proliferation of non-small cell lung cancer parental cells and drug-resistant cells, and the mechanism may be achieved by inhibiting the expression of TAM RTKs, rather than mediating the expression of IL-8. In Lewis lung cancer, luteolin induces cancer cell apoptosis by activating caspase 9 and 3-mediated mitochondrial apoptosis pathways. In addition, inhibition of ERK and Akt pathways is also one of the mechanisms of luteolin against Lewis lung cancer (Kim et al., 2007). Studies have shown that luteolin can be used as a radiosensitizer for non-small cell lung cancer. Luteolin combined with ionizing radiation (IR) can enhance the apoptosis of cancer cells both in vivo and in vitro. The mechanism is related to the activation of the p38/ROS/caspase pathway, which is of great help for the development of new treatment techniques for patients with non-small cell lung cance (Cho et al., 2015). In addition, studies have also revealed that luteolin can be used to treat nasopharyngeal carcinoma (Xiong et al., 2022). A study has also confirmed that luteolin can inhibit the cell cycle of human nasopharyngeal carcinoma (NPC) cells in a time and dose-dependent manner. The mechanism is related to the Akt/GSK-3β/Cyclin D1 pathway, which inhibits the proliferation of cancer cells by eliminating the effect of insulin on this pathway (Ong et al., 2010).
In summary, the active components of Radix Codonopsis, particularly luteolin, exhibit significant therapeutic effects against respiratory cancers. These compounds exert their pharmacological effects by inducing cancer cell apoptosis and inhibiting proliferation, migration, and invasion, highlighting the broad application prospects and clinical value of Radix Codonopsis.
5.2 Application in digestive system cancer
Globally, gastric cancer ranks as the fifth most common malignancy. In 2020, an estimated 1.1 million new cases were reported worldwide, resulting in approximately 800,000 deaths (Bray et al., 2024). These data highlight the seriousness of gastric cancer worldwide. The natural compounds extracted from Radix Codonopsis have a certain role in the treatment of gastric cancer. The active ingredient of Radix Codonopsis Stigmasterol belongs to sterol compounds and is a kind of plant sterol. Studies have shown that Stigmasterol can inhibit the proliferation of gastric cancer cells, and induce apoptosis and autophagy by blocking the AKT/mTOR signaling pathway, and autophagy has a protective effect on apoptosis. This study also showed that Stigmasterol could inhibit the growth of gastric cancer in vivo, suggesting that Stigmasterol may be a potential drug for the treatment of gastric cancer (Zhao et al., 2021). Taraxerol is another active ingredient of Radix Codonopsis, which is mainly found in plants, especially in Asteraceae plants such as dandelion. Huo et al. revealed the regulatory mechanism of taraxerol in gastric cancer through network pharmacology and verified the role of taraxerol in gastric cancer and its key signaling pathways through a series of in vitro experiments. Experiments have shown that taraxerol can inhibit the proliferation, migration, and invasion of gastric cancer cells, and induce cell cycle arrest and apoptosis. Its anti-gastric cancer effect is likely to be achieved by inhibiting the PI3K/AKT signaling pathway (Huo et al., 2022). However, this study was only verified at the cellular level, and further animal experiments are needed to prove its mechanism. Zang et al. (2017) showed that luteolin could reduce the migration and proliferation of human umbilical vein endothelial cells (HUVECs) in a dose-dependent manner, and significantly inhibit the tube formation and angiogenesis induced by gastric cancer cells. This inhibitory effect was achieved by reducing the secretion of VEGF and interfering with the interaction between gastric cancer cells and HUVECs, especially by down-regulating Notch1 expression. Overexpression of Notch1 partially reversed the effect of luteolin, confirming that luteolin inhibited gastric cancer angiogenesis and gastric cancer cell-derived tube formation through the Notch1-dependent pathway. Another study (Lu et al., 2015) showed that luteolin significantly inhibited tumor growth, reduced invasiveness, and promoted apoptosis in cMet-overexpressing gastric cancer models. The mechanism may be related to the inhibition of the cMet/Akt/ERK signaling pathway. Therefore, luteolin may be a potential drug for the treatment of cMet-overexpressing gastric cancer. Wu et al. (2015) found that luteolin affected the apoptosis of gastric cancer cells by regulating Bcl-2 protein. This experiment showed that the expression of miR-34a was downregulated in gastric cancer tissues, while luteolin could upregulate the expression of miR-34a and reduce the level of Bcl-2, which could be partially reversed by anti-miR-34a oligonucleotide. Therefore, the study revealed the important role of luteolin in inducing apoptosis of gastric cancer cells through the miR-34a pathway. In addition, lobetyolin extracted from Radix Codonopsis can inhibit the proliferation of gastric cancer cells and induce apoptosis. Its mechanism may be related to reducing glutamine uptake, down-regulating ASCT2 expression, inducing ROS accumulation, regulating AKT/GSK3β/c-Myc signaling pathway and reducing Nrf2 protein level, thus playing an anti-cancer role in gastric cancer (Cheng et al., 2023). In the study of Zang et al. (2019), it was shown that glycitein, an isoflavone compound contained in Radix Codonopsis, has a significant anti-tumor effect on human gastric cancer cells. It can play a role by increasing ROS production, reducing mitochondrial transmembrane potential, and inducing apoptosis and G0/G1 phase cell cycle arrest. The mechanism involves the activation of the MAPK signaling pathway and the inhibition of STAT3 and NF-κB signaling pathways, suggesting that glycitein may become a new targeted drug for the treatment of human gastric cancer. In summary, various active ingredients of Radix Codonopsis show significant potential and effects in anti-gastric cancer through different mechanisms of action.
Luteolin also has a good effect on anti-hepatocellular carcinoma. Through network pharmacology, 21 effective components and 98 potential target genes of Radix Codonopsis were screened, and 53 interacting genes were obtained by intersection with hepatocellular carcinoma (HCC) target genes. It was found that luteolin had an anti-hepatoma effect by affecting the ESR1 signaling pathway (Yu et al., 2023). In addition, Hwang et al.found that luteolin inhibited the growth of liver cancer cells and reduced tumor volume by activating AMPK, which may be related to the inhibition of NF-κB activity, indicating that AMPK is a potential target for cancer prevention (Hwang et al., 2011). A study (Huo et al., 2024) confirmed the inhibitory effect of Stigmasterol on tumor growth in HCC mice and found that it increased the proportion of IFN-γ + CD8 + T cells and Treg cells in intestinal mucosa and tumor tissues by affecting specific Lactobacillus species, resulting in increased apoptotic protein levels and tumor cell death, revealing the effect of Stigmasterol remodeling of intestinal flora on immune cells, which provides a theoretical basis for the clinical application of Stigmasterol in the treatment of hepatocellular carcinoma. Figure 6. In addition, the researchers determined the potential targets of Radix Codonopsis in hepatocellular carcinoma by screening the target proteins and key genes related to hepatocellular carcinoma. Through a series of experimental analyses, including cell experiments and animal models, it was found that Radix Codonopsis hair polysaccharide solution (CPP) could inhibit the proliferation, migration, and stem cell characteristics of liver cancer cells and induce apoptosis. The results show that Radix Codonopsis may inhibit the growth of hepatocellular carcinoma by regulating the CDK1/PDK1/β-catenin signaling axis, which provides a new insight into the role of Radix Codonopsis in the treatment of hepatocellular carcinoma (Li et al., 2024). Bai et al. studied two polysaccharides CPP1a and CPP1c isolated from Radix Codonopsis and confirmed that they were cytotoxic to HepG2 cells, inhibited cell migration, and induced apoptosis (Bai et al., 2018).
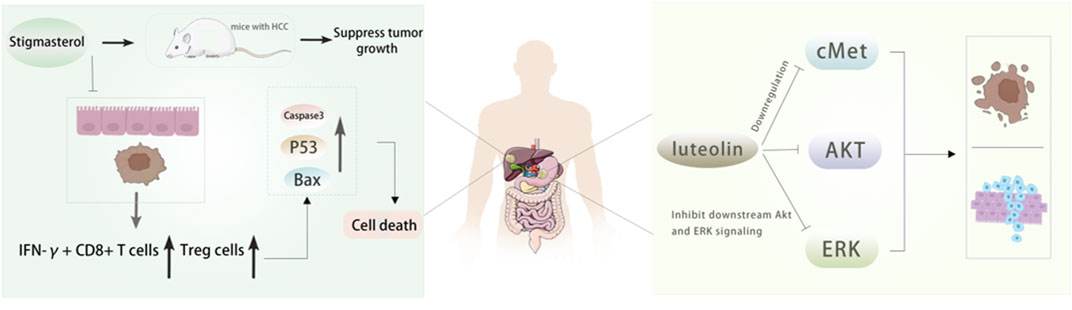
Figure 6. The active ingredients of Radix Codonopsis exert anticancer effects in gastric and livercancer. Stigmasterol was shown to inhibit tumor growth in mice. It was found that theincrease of IFN-y+ CD8+ T cells and Treg cells and the upregulation of Caspase3, Bax and P53 expression led to the death of tumor cells. luteolin can inhibit the proliferation, invasionand promote apoptosis of gastric cancer cells by inhibiting cMet/Akt/ERK signaling pathway.
In the study of gallbladder cancer and cholangiocarcinoma, some components of Radix Codonopsis also have a certain therapeutic effect on them. The effect of Stigmasterol on cell proliferation was evaluated by MTT and trypan blue test, and its regulation on the expression of Jab1 and p27 in human gallbladder cancer cells was detected by RT-PCR and Western blotting. Further analysis showed that Stigmasterol could induce apoptosis and activate the mitochondrial apoptosis signaling pathway. The results showed that Stigmasterol has the potential as a therapeutic agent for gallbladder cancer targeting Jab1 (Pandey et al., 2019). Lupeol and stigmasterol have anti-inflammatory and potential anti-cancer effects. Studies have found that they can inhibit endothelial cell viability and migration, reduce TNF-α expression, affect the VEGFR-2 signaling pathway, and destroy tumor angiogenesis in vivo experiments. Reduce cholangiocarcinoma (CCA) tumor growth (Kangsamaksin et al., 2017).
Luteolin, as a natural biological flavonoid, shows anti-cancer and apoptosis-inducing properties in HCT-15 colon cancer cells in vitro experiments. It can reduce cell viability, affect cell cycle and apoptosis-related protein expression, and may become a potential drug for the prevention or treatment of colon cancer. Yao et al. (2019) have shown that luteolin has an anti-cancer effect in colorectal cancer and can inhibit cell migration and invasion. The mechanism may be related to the upregulation of miR-384 and downregulation of pleiotropic protein (PTN) expression, suggesting that PTN may become a potential target for CRC treatment. It has also been found that luteolin can selectively reduce the viability of HT-29 colon cancer cells and induce their apoptosis, which involves the activation of the mitochondrial-mediated caspase pathway and the activation of antioxidant enzymes. Luteolin induces apoptosis by regulating the expression of Bax and Bcl-2, promoting the release of cytochrome c, increasing the activity of caspase-9 and caspase-3, and increasing the level of reduced glutathione and the expression of GSH synthase, which is mediated by mitogen-activated protein kinase signaling pathway (Kang et al., 2017). In addition, the resistance of colon cancer to the ferroptosis inducer erastin is a therapeutic problem. The study found that the combination of luteolin and erastin showed a synergistic inhibitory effect on colon cancer cells in vitro and in vivo, and enhanced the sensitivity of colon cancer cells to ferroptosis by reducing the expression of antioxidant enzyme GPX4 and up-regulating the expression of tumor suppressor HIC1 (Zheng et al., 2023).
In addition to the above-mentioned cancers, the effective anti-cancer components of Radix Codonopsis can also play a role in other cancers of the digestive system. For example, in oral cancer, the study of Tiioe et al.aimed to find approved drugs with selective toxicity to oral squamous cell carcinoma (OSCC). Through drug screening, it was found that luteolin, metixene hydrochloride, and nitazoxanide had high cytotoxicity to oral cancer cells, and the toxicity was lower than the existing standard drugs. Luteolin can affect the DNA repair pathway and may be used as a potent cytotoxic or adjuvant therapy for oral cancer. However, further in vivo studies are needed to verify the anti-cancer effect of luteolin (Tjioe et al., 2016). Paclitaxel as an anti-tumor drug has application limitations, but it may be effective in combination with other drugs. It was found that luteolin combined with low-dose paclitaxel showed synergistic anti-esophageal cancer effects in vitro and in vivo, and played a role by inhibiting SIRT1 and ROS/JNK pathways without obvious toxicity (Qin et al., 2021). Luteolin, as a natural small molecule inhibitor of BCL-2, shows pro-apoptotic activity in SW1990 pancreatic cancer cells promotes BAX release, and induces cancer cell death by directly binding to BCL-2. In vivo experiments, luteolin can also effectively inhibit tumor growth (Li et al., 2018). Moeng et al.’s study revealed that miR-301-3p plays a key role in regulating cell proliferation and enhancing the antitumor effect of tumor necrosis factor-related apoptosis-inducing ligand (TRAIL), and TRAIL can inhibit cell growth by inducing cell cycle arrest. Luteolin can inhibit the growth of human pancreatic cancer PANC-1 cells and increase their sensitivity to TRAIL by reducing the level of miR-301-3p (Moeng et al., 2020).
In summary, a variety of active ingredients in Radix Codonopsis, such as luteolin and Stigmasterol, can exert their corresponding anti-tumor effects on various cancers of the digestive system. Its application is expected to bring more benefits to patients with digestive system cancer.
5.3 Application in reproductive system and urinary system cancer
In recent years, the effective components of Radix Codonopsis have been studied, and it has been found that it also has potential curative effects on reproductive and urinary system cancers, such as prostate cancer and cervical cancer, which provides a direction for the in-depth study of Radix Codonopsis in the field of anti-cancer.
Breast cancer is a cancer that occurs in breast tissue, mainly affecting women, but it can also occur in men. Its incidence is rising worldwide. Therefore, it is necessary to carry out continuous research on it and constantly develop new therapeutic drugs and methods. A study evaluated the effect of Stigmastero on the expression of anti-apoptotic genes Bcl-2 and BCL-XL in human breast cancer MCF-7 cell line, as well as its effect on apoptosis, cell viability, and spontaneous breast tumor growth in vivo. The results showed that Stigmastero could significantly reduce the expression of these genes, induce apoptosis, reduce cell proliferation, and inhibit tumor growth (AmeliMojarad et al., 2022). Gao et al.showed that luteolin inhibited the growth and EMT of breast cancer cells. It exerts anti-tumor effects by reducing cell viability, accelerating apoptosis, regulating related protein expression, and inhibiting TGFβ1-induced EMT process. In addition, the anti-tumor effect of luteolin is related to the increase of miR-203 level and the inhibition of Ras/Raf/MEK/ERK signaling pathway, and miR-203 silencing will weaken its effect (Gao et al., 2019). Codonolactone (CLT) is a sesquiterpene lactone isolated from Radix Codonopsis. Wang et al. (2014) found that CLT can reduce the invasion and migration of breast cancer cells, and significantly inhibit the formation of lung metastases of breast cancer in vivo. Further studies have shown that this anti-metastasis effect may be related to CLT inhibition of MMP-9 and MMP-13 activity and expression, as well as downregulation of Runx2 transcriptional activity. Triple-negative breast cancer (TNBC) is associated with a high risk of early recurrence and metastasis, and EMT plays a key role in its development. Abnormal activation of YAP/TAZ promotes EMT. A study has shown that luteolin can inhibit YAP/TAZ activity and reverse EMT, and reduce TNBC cell migration and tumor growth, suggesting that luteolin may become a new drug for the treatment of TNBC (Cao et al., 2020). In addition, luteolin was found to have an anti-metastasis effect in TNBC, which could inhibit the migration and invasion of highly metastatic TNBC cells in a dose-dependent manner and reverse EMT. In vivo experiments, luteolin significantly reduced lung metastasis of breast cancer and inhibited the expression of EMT-related molecules and β-catenin. The results showed that luteolin effectively inhibited breast cancer metastasis by down-regulating β-catenin-mediated EMT reversal (Lin et al., 2017). Another study evaluated the therapeutic effect and mechanism of luteolin on androgen receptor-positive breast cancer cells. It was found that luteolin could significantly inhibit the proliferation and metastasis of these cells, and this effect was achieved by affecting the AKT/mTOR signaling pathway and reducing the expression of MMP9. The use of luteolin combined with AKT/mTOR inhibitors can enhance its inhibitory effect (Wu et al., 2021).
Ovarian cancer is one of the most lethal types of cancer in the female reproductive system. It often has no obvious symptoms in the early stage, so regular physical examination and improvement of prognosis are crucial. As a phytosterol, Stigmastero also has an anti-cancer effect on human ovarian cancer. Bae et al. (2020) found that Stigmastero can inhibit the growth of ovarian cancer cells, induce apoptosis, affect mitochondrial function and calcium levels, and inhibit cell migration and angiogenesis. Radix Codonopsis saponin A, the main triterpenoid saponin in the roots of Radix Codonopsis, can significantly inhibit the migration and invasion of human ovarian cancer cells and reduce the expression of matrix metalloproteinases (MMPs) -2 and -9. The mechanism may be related to the production of ROS and the activation of p38 MAP kinase (Ahn et al., 2020). There are also studies aimed at exploring the effect of luteolin as a new therapeutic drug targeting vaccine-associated kinase 1 (VRK1) on high-grade serous ovarian cancer (HGSOC). It was found that luteolin could reduce the proliferation of HGSOC cells, increase apoptosis and G2/M phase cell cycle arrest, and activate the p53 signaling pathway. In patient-derived xenograft models, oral or intraperitoneal injection of luteolin significantly inhibited tumor growth and showed a synergistic effect with cisplatin in combination therapy, especially for cisplatin-resistant cell lines. Therefore, luteolin is considered a promising candidate for HGSOC treatment (Chang et al., 2023). In addition, endometrial cancer is also one of the most common cancers in women. It is a malignant tumor originating from endometrial epithelial cells. However, the chemotherapy resistance of patients with endometrial cancer reduces their survival rate, and Nrf2 plays an important role in chemotherapy resistance. Chang et al.found that Nrf2 was highly expressed in endometrial cancer tissues, resulting in decreased sensitivity to cisplatin. As a new Nrf2 inhibitor, Stigmastero can improve the sensitivity of cancer cells to cisplatin and reduce the level of Nrf2 protein, thus playing a key role in overcoming chemotherapy resistance (Liao et al., 2020).
Prostate cancer is a very common malignant tumor in elderly men. In recent years, with the aging of China’s population and lifestyle changes, the incidence of prostate cancer in China is also gradually increasing. Luteolin was found to inhibit the invasion of prostate cancer cells by regulating the expression of E-cadherin. Studies have shown that luteolin affects E-cadherin through the AKT/MDM2 pathway, and can inhibit the lung metastasis of prostate cancer cells in nude mice in vivo (Zhou et al., 2009). In addition, Han et al. (2018) found that luteolin can reduce the proliferation, migration, self-renewal, and stem cell marker expression of prostate cancer cells in vitro. FZD6 is a tumor suppressor that can eliminate prostate cancer stemness. Further studies have shown that luteolin inhibits Wnt signaling by up-regulating FZD6 to inhibit prostate cancer stemness. Chiu and Lin’s study (Chiu and Lin, 2008) also revealed that luteolin can inhibit the proliferation of prostate cancer cells and induce apoptosis of prostate cancer LNCaP cells, while reducing prostate-specific antigen (PSA) level and Androgen receptor (AR) expression, leading to AR degradation by reducing the association between AR and heat shock protein 90, indicating that luteolin plays an anti-cancer role by targeting AR and may be used for the chemoprevention and treatment of prostate cancer.
In the study of Iida et al. (2020), it was found that luteolin may become a natural product therapeutic agent for the treatment of bladder cancer. Luteolin can inhibit the survival of human bladder cancer cell line T24 by regulating p21, TRX1, and mTOR signaling pathways, inducing cell cycle arrest, and reducing reactive oxygen species production. In animal models, luteolin significantly reduced the volume of bladder tumors and decreased the expression of phosphor (p) -S6 downstream of Ki67 and mTOR. This study also showed that luteolin metabolites were associated with inhibition of cell proliferation and mTOR signal transduction. Therefore, the effective components of Radix Codonopsis also have a good application in the treatment of reproductive and urinary system cancer.
5.4 Application in other system cancer
Modern pharmacological studies have shown that Radix Codonopsis not only has a positive effect on respiratory, digestive, reproductive, and urinary system cancers but also shows potential therapeutic effects on other types of tumors, such as leukemia, osteosarcoma, and other malignant tumor diseases.
Leukemia, also known as blood cancer, is a type of malignant tumor that affects blood and bone marrow. Improving its therapeutic effect and providing better treatment for patients is the goal pursued by researchers and medical workers. Wang et al.found that luteolin could significantly reduce the viability of human leukemia cells by inducing poly (ADP-ribose) polymerase (PARP) cleavage, nuclear fragmentation, and activating exogenous apoptotic pathways. This process is associated with upregulation of Fas/FasL expression, increased histone H3 acetylation, and activation of the c-Jun signaling pathway (Wang et al., 2018). In addition, the study found that RSK1 is overexpressed in bone marrow samples of untreated leukemia patients and is associated with shorter overall survival. Luteolin, as a novel RSK inhibitor, has been shown to inhibit growth, induce apoptosis, and reduce migration in leukemia cells. These effects of luteolin are related to the expression level of RSK1, and knockdown of RSK1 expression can enhance the effect of luteolin (Deng et al., 2017).
The incidence of osteosarcoma is high in adolescents and young adults, which has a significant impact on the physical and mental health and quality of life of patients. Therefore, it is necessary to study it to improve the therapeutic effect and the survival rate of patients. A study explored how luteolin induces apoptosis of MG-63 human osteosarcoma cells by inhibiting the expression of BCL-2, Caspase-3, and Survivin and promoting the expression of BAX, thereby inhibiting cell proliferation. The results showed that luteolin effectively inhibited the proliferation of osteosarcoma cells and induced apoptosis in a dose-dependent manner, suggesting that it may be used as a new drug for the treatment of osteosarcoma (Wang et al., 2015). Huang et al.found that luteolin can target multiple targets of osteosarcoma through network pharmacology analysis, especially key genes in the PI3K-AKT signaling pathway, such as AKT1, IL6, JUN, STAT3, TNF, and VEGFA. Experiments have confirmed that luteolin can inhibit the expression of these genes, reduce the viability of osteosarcoma cells, and inhibit tumor proliferation and metastasis in mouse models, indicating that luteolin may inhibit osteosarcoma by regulating related signaling pathways (Huang et al., 2023).
The study also found that luteolin can significantly inhibit the migration of human glioblastoma cells, which is related to the downregulation of MMP-2 and MMP-9 and the upregulation of TIMP-1 and TIMP-2, and affects epithelial-mesenchymal transition and p-IGF-1R/PI3K/AKT/mTOR signaling pathway. These results suggest that luteolin may be used for chemoprevention of glioblastoma (Wang et al., 2017a). In addition, studies have found that luteolin can inhibit the migration and invasion of glioblastoma cells at a non-toxic dose, and affect the level of Cdc42 protein and PI3K/AKT signaling pathway. These effects may be related to the promotion of Cdc42 protein degradation through the proteasome pathway (Cheng et al., 2013). Wang et al. (2017b) found that luteolin can induce apoptosis by increasing the level of intracellular reactive oxygen species, inducing endoplasmic reticulum stress response and mitochondrial dysfunction in human glioblastoma cell lines. The use of antioxidants can reverse this process. It indicates that luteolin may be an anticancer candidate for the treatment of glioblastoma.
6 Discussion and prospects
Cancer arises from dysregulated cell growth caused by genetic mutations. Its hallmark features—uncontrolled proliferation, evasion of apoptosis, induction of angiogenesis, and the capacity for invasion and metastasis—pose significant challenges for effective treatment. Consequently, developing safer and more effective anti-cancer therapies is of paramount importance. Recent studies have highlighted the potential of TCM and its bioactive components as anti-cancer agents, given their ability to exert synergistic, multi-component, multi-target, and multi-pathway regulatory effects. These components play roles in various tumor-related processes, including occurrence, progression, metastasis, and immune regulation. Radix Codonopsis, a TCM commonly recognized for both medicinal and nutritional value, has attracted attention for its broad range of pharmacological activities. In addition to its known benefits for cardiovascular and gynecological conditions, immune modulation, antioxidant and anti-aging effects, and fatigue resistance, Radix Codonopsis has shown promise in cancer treatment. However, no comprehensive review previously addressed its anticancer properties. Therefore, this paper reviews the research progress of the active ingredients of Radix Codonopsis in anti-tumor aspects, and summarizes in Table 2 that the active ingredients of Radix Codonopsis can play a role in various systems of cancer through multiple mechanisms of action. For example, some compounds can inhibit the proliferation, growth, and migration of cancer cells through the PI 3K/Akt signaling pathway, MEK/ERK signaling pathway, and p53 signaling pathway, and exert the therapeutic effect of Radix Codonopsis on various systems of cancer through multi-target and multi pathway approaches, in order to provide reference for future research and clinical applications.
Despite these promising findings, the mechanisms underlying the anticancer effects of Radix Codonopsis remain complex and warrant further investigation. Currently, the application of TCM in oncology often involves combining herbal therapies with conventional treatments to mitigate side effects and enhance therapeutic outcomes. While studies on luteolin and stigmasterol have advanced our understanding of Radix Codonopsis’s anticancer potential, other constituents—such as perlolyrine, frutinone A, and spinoside A—have been less explored. Further in-depth research is needed to fully elucidate their medicinal value, establish efficacy and safety profiles, and optimize their therapeutic use in cancer treatment.
Author contributions
C-YL: Writing–original draft, Writing–review and editing. ZL: Writing–review and editing. F-EC: Writing–review and editing. YN: Conceptualization, Methodology, Supervision, Writing–review and editing. W-QL: Funding acquisition, Supervision, Writing–review and editing.
Funding
The author(s) declare that financial support was received for the research, authorship, and/or publication of this article. This work was supported by grants from the National Natural Science Foundation of China (8226150600).
Conflict of interest
The authors declare that the research was conducted in the absence of any commercial or financial relationships that could be construed as a potential conflict of interest.
Generative AI statement
The authors declare that no Generative AI was used in the creation of this manuscript.
Publisher’s note
All claims expressed in this article are solely those of the authors and do not necessarily represent those of their affiliated organizations, or those of the publisher, the editors and the reviewers. Any product that may be evaluated in this article, or claim that may be made by its manufacturer, is not guaranteed or endorsed by the publisher.
References
Ahn, J.-H., Jang, D. S., and Choi, J.-H. (2020). Lancemaside A isolated from the root of codonopsis lanceolata inhibits ovarian cancer cell invasion via the reactive oxygen species (ROS)-Mediated p38 pathway. Am. J. Chin. Med. 48, 1021–1034. doi:10.1142/S0192415X20500494
Al-Ghazzawi, A. M. (2019). Anti-cancer activity of new benzyl isoquinoline alkaloid from Saudi plant Annona squamosa. BMC Chem. 13, 13. doi:10.1186/s13065-019-0536-4
AmeliMojarad, M., AmeliMojarad, M., and Pourmahdian, A. (2022). The inhibitory role of stigmasterol on tumor growth by inducing apoptosis in Balb/c mouse with spontaneous breast tumor (SMMT). BMC Pharmacol. Toxicol. 23, 42. doi:10.1186/s40360-022-00578-2
An, R., Zhang, W., and Huang, X. (2022). Developments in the antitumor activity, mechanisms of action, structural modifications, and structure-activity relationships of steroidal saponins. Mini Rev. Med. Chem. 22, 2188–2212. doi:10.2174/1389557522666220217113719
Bae, H., Song, G., and Lim, W. (2020). Stigmasterol causes ovarian cancer cell apoptosis by inducing endoplasmic reticulum and mitochondrial dysfunction. Pharmaceutics 12, 488. doi:10.3390/pharmaceutics12060488
Bai, R., Li, W., Li, Y., Ma, M., Wang, Y., Zhang, J., et al. (2018). Cytotoxicity of two water-soluble polysaccharides from Codonopsis pilosula Nannf. var. modesta (Nannf.) L.T.Shen against human hepatocellular carcinoma HepG2 cells and its mechanism. Int. J. Biol. Macromol. 120, 1544–1550. doi:10.1016/j.ijbiomac.2018.09.123
Bhambhani, S., Kondhare, K. R., and Giri, A. P. (2021). Diversity in chemical structures and biological properties of plant alkaloids. Molecules 26, 3374. doi:10.3390/molecules26113374
Bouvier, F., Rahier, A., and Camara, B. (2005). Biogenesis, molecular regulation and function of plant isoprenoids. Prog. Lipid Res. 44, 357–429. doi:10.1016/j.plipres.2005.09.003
Bray, F., Laversanne, M., Sung, H., Ferlay, J., Siegel, R. L., Soerjomataram, I., et al. (2024). Global cancer statistics 2022: GLOBOCAN estimates of incidence and mortality worldwide for 36 cancers in 185 countries. CA A Cancer J. Clin. 74, 229–263. doi:10.3322/caac.21834
Cao, D., Zhu, G.-Y., Lu, Y., Yang, A., Chen, D., Huang, H.-J., et al. (2020). Luteolin suppresses epithelial-mesenchymal transition and migration of triple-negative breast cancer cells by inhibiting YAP/TAZ activity. Biomed. Pharmacother. 129, 110462. doi:10.1016/j.biopha.2020.110462
Chang, X., Tamauchi, S., Yoshida, K., Yoshihara, M., Yokoi, A., Shimizu, Y., et al. (2023). Downregulating vaccinia-related kinase 1 by luteolin suppresses ovarian cancer cell proliferation by activating the p53 signaling pathway. Gynecol. Oncol. 173, 31–40. doi:10.1016/j.ygyno.2023.04.003
Chen, K.-C., Chen, C.-Y., Lin, C.-R., Yang, T.-Y., Chen, T.-H., Wu, L.-C., et al. (2013). Luteolin attenuates TGF-β1-induced epithelial-mesenchymal transition of lung cancer cells by interfering in the PI3K/Akt-NF-κB-Snail pathway. Life Sci. 93, 924–933. doi:10.1016/j.lfs.2013.10.004
Chen, M., Li, Y., Liu, Z., Qu, Y., Zhang, H., Li, D., et al. (2018). Exopolysaccharides from a Codonopsis pilosula endophyte activate macrophages and inhibit cancer cell proliferation and migration. Thorac. Cancer 9, 630–639. doi:10.1111/1759-7714.12630
Cheng, L., Zhai, H., Du, J., Zhang, G., and Shi, G. (2023). Lobetyolin inhibits cell proliferation and induces cell apoptosis by downregulating ASCT2 in gastric cancer. Cytotechnology 75, 435–448. doi:10.1007/s10616-023-00588-w
Cheng, M., Li, T., Hu, E., Yan, Q., Li, H., Wang, Y., et al. (2024). A novel strategy of integrating network pharmacology and transcriptome reveals antiapoptotic mechanisms of Buyang Huanwu Decoction in treating intracerebral hemorrhage. J. Ethnopharmacol. 319, 117123. doi:10.1016/j.jep.2023.117123
Cheng, W.-Y., Chiao, M.-T., Liang, Y.-J., Yang, Y.-C., Shen, C.-C., and Yang, C.-Y. (2013). Luteolin inhibits migration of human glioblastoma U-87 MG and T98G cells through downregulation of Cdc42 expression and PI3K/AKT activity. Mol. Biol. Rep. 40, 5315–5326. doi:10.1007/s11033-013-2632-1
Chida, N. (2011). Recent advances in the synthesis of morphine and related alkaloids. Top. Curr. Chem. 299, 1–28. doi:10.1007/128_2010_73
Chiu, F.-L., and Lin, J.-K. (2008). Downregulation of androgen receptor expression by luteolin causes inhibition of cell proliferation and induction of apoptosis in human prostate cancer cells and xenografts. Prostate 68, 61–71. doi:10.1002/pros.20690
Cho, H.-J., Ahn, K.-C., Choi, J. Y., Hwang, S.-G., Kim, W.-J., Um, H.-D., et al. (2015). Luteolin acts as a radiosensitizer in non-small cell lung cancer cells by enhancing apoptotic cell death through activation of a p38/ROS/caspase cascade. Int. J. Oncol. 46, 1149–1158. doi:10.3892/ijo.2015.2831
Choi, S., and Kim, T. (2023). Compound K - an immunomodulator of macrophages in inflammation. Life Sci. 323, 121700. doi:10.1016/j.lfs.2023.121700
Deng, L., Jiang, L., Lin, X., Tseng, K.-F., Lu, Z., and Wang, X. (2017). Luteolin, a novel p90 ribosomal S6 kinase inhibitor, suppresses proliferation and migration in leukemia cells. Oncol. Lett. 13, 1370–1378. doi:10.3892/ol.2017.5597
Dobrzynska, M., Napierala, M., and Florek, E. (2020). Flavonoid nanoparticles: a promising approach for cancer therapy. Biomolecules 10, 1268. doi:10.3390/biom10091268
Frank, E., and Schneider, G. (2013). Synthesis of sex hormone-derived modified steroids possessing antiproliferative activity. J. Steroid Biochem. Mol. Biol. 137, 301–315. doi:10.1016/j.jsbmb.2013.02.018
Gao, G., Ge, R., Li, Y., and Liu, S. (2019). Luteolin exhibits anti-breast cancer property through up-regulating miR-203. Artif. Cells Nanomed Biotechnol. 47, 3265–3271. doi:10.1080/21691401.2019.1646749
Gao, T.-H., Liao, W., Lin, L.-T., Zhu, Z.-P., Lu, M.-G., Fu, C.-M., et al. (2022). Curcumae rhizoma and its major constituents against hepatobiliary disease: pharmacotherapeutic properties and potential clinical applications. Phytomedicine 102, 154090. doi:10.1016/j.phymed.2022.154090
Giles, A. J., Hutchinson, M.-K. N. D., Sonnemann, H. M., Jung, J., Fecci, P. E., Ratnam, N. M., et al. (2018). Dexamethasone-induced immunosuppression: mechanisms and implications for immunotherapy. J. Immunother. Cancer 6, 51. doi:10.1186/s40425-018-0371-5
Gupta, A., Kumar, B. S., and Negi, A. S. (2013). Current status on development of steroids as anticancer agents. J. Steroid Biochem. Mol. Biol. 137, 242–270. doi:10.1016/j.jsbmb.2013.05.011
Han, K., Lang, T., Zhang, Z., Zhang, Y., Sun, Y., Shen, Z., et al. (2018). Luteolin attenuates Wnt signaling via upregulation of FZD6 to suppress prostate cancer stemness revealed by comparative proteomics. Sci. Rep. 8, 8537. doi:10.1038/s41598-018-26761-2
He, Q., Yang, J., Pan, Z., Zhang, G., Chen, B., Li, S., et al. (2023). Biochanin A protects against iron overload associated knee osteoarthritis via regulating iron levels and NRF2/System xc-/GPX4 axis. Biomed. and Pharmacother. 157, 113915. doi:10.1016/j.biopha.2022.113915
Huang, R., Xu, M., Guo, W., Cheng, M., Dong, R., Tu, J., et al. (2023). Network pharmacology and experimental verification-based strategy for exploring the mechanisms of luteolin in the treatment of osteosarcoma. Cancer Cell Int. 23, 213. doi:10.1186/s12935-023-03046-x
Huo, B., Song, Y., Tan, B., Li, J., Zhang, J., Zhang, F., et al. (2022). Research on the mechanisms of taraxerol for the treatment of gastric cancer effect based on network pharmacology. Int. J. Immunopathol. Pharmacol. 36, 20587384211063962. doi:10.1177/20587384211063962
Huo, R., Yang, W.-J., Liu, Y., Liu, T., Li, T., Wang, C.-Y., et al. (2024). Stigmasterol: remodeling gut microbiota and suppressing tumor growth through Treg and CD8+ T cells in hepatocellular carcinoma. Phytomedicine 129, 155225. doi:10.1016/j.phymed.2023.155225
Hwang, J.-T., Park, O. J., Lee, Y. K., Sung, M. J., Hur, H. J., Kim, M. S., et al. (2011). Anti-tumor effect of luteolin is accompanied by AMP-activated protein kinase and nuclear factor-κB modulation in HepG2 hepatocarcinoma cells. Int. J. Mol. Med. 28, 25–31. doi:10.3892/ijmm.2011.667
Iida, K., Naiki, T., Naiki-Ito, A., Suzuki, S., Kato, H., Nozaki, S., et al. (2020). Luteolin suppresses bladder cancer growth via regulation of mechanistic target of rapamycin pathway. Cancer Sci. 111, 1165–1179. doi:10.1111/cas.14334
Jiang, C.-H., Sun, T.-L., Xiang, D.-X., Wei, S.-S., and Li, W.-Q. (2018). Anticancer activity and mechanism of xanthohumol: a prenylated flavonoid from hops (humulus lupulus L.). Front. Pharmacol. 9, 530. doi:10.3389/fphar.2018.00530
Kang, K. A., Piao, M. J., Ryu, Y. S., Hyun, Y. J., Park, J. E., Shilnikova, K., et al. (2017). Luteolin induces apoptotic cell death via antioxidant activity in human colon cancer cells. Int. J. Oncol. 51, 1169–1178. doi:10.3892/ijo.2017.4091
Kangsamaksin, T., Chaithongyot, S., Wootthichairangsan, C., Hanchaina, R., Tangshewinsirikul, C., and Svasti, J. (2017). Lupeol and stigmasterol suppress tumor angiogenesis and inhibit cholangiocarcinoma growth in mice via downregulation of tumor necrosis factor-α. PLoS One 12, e0189628. doi:10.1371/journal.pone.0189628
Khater, M., Ravishankar, D., Greco, F., and Osborn, H. M. (2019). Metal complexes of flavonoids: their synthesis, characterization and enhanced antioxidant and anticancer activities. Future Med. Chem. 11, 2845–2867. doi:10.4155/fmc-2019-0237
Kim, J.-H., Lee, E.-O., Lee, H.-J., Ku, J.-S., Lee, M.-H., Yang, D.-C., et al. (2007). Caspase activation and extracellular signal-regulated kinase/Akt inhibition were involved in luteolin-induced apoptosis in Lewis lung carcinoma cells. Ann. N. Y. Acad. Sci. 1095, 598–611. doi:10.1196/annals.1397.102_2
Kishimoto, S., Sato, M., Tsunematsu, Y., and Watanabe, K. (2016). Evaluation of biosynthetic pathway and engineered biosynthesis of alkaloids. Molecules 21, 1078. doi:10.3390/molecules21081078
Lee, Y. J., Lim, T., Han, M. S., Lee, S.-H., Baek, S.-H., Nan, H.-Y., et al. (2017). Anticancer effect of luteolin is mediated by downregulation of TAM receptor tyrosine kinases, but not interleukin-8, in non-small cell lung cancer cells. Oncol. Rep. 37, 1219–1226. doi:10.3892/or.2016.5336
Li, A. F. Y., Wang, C.-L., Tai, H.-Y., Fu, Y.-J., Tsai, F.-T., Tsai, Y.-C., et al. (2021). Pandemic aspect of dexamethasone: molecular mechanisms and clinical application. J. Chin. Med. Assoc. 84, 245–247. doi:10.1097/JCMA.0000000000000485
Li, N., Yang, C., Xia, J., Wang, W., and Xiong, W. (2024). Molecular mechanisms of Codonopsis pilosula in inhibiting hepatocellular carcinoma growth and metastasis. Phytomedicine 128, 155338. doi:10.1016/j.phymed.2024.155338
Li, W., Shao, Y., Hu, L., Zhang, X., Chen, Y., Tong, L., et al. (2007). BM6, a new semi-synthetic vinca alkaloid, exhibits its potent in vivo anti-tumor activities via its high binding affinity for tubulin and improved pharmacokinetic profiles. Cancer Biol. Ther. 6, 787–794. doi:10.4161/cbt.6.5.4006
Li, Y., Liu, C., Luo, J., Zeng, Y., Meng, X., Wang, S., et al. (2022). Ershiwuwei Lvxue Pill alleviates rheumatoid arthritis by different pathways and produces changes in the gut microbiota. Phytomedicine 107, 154462. doi:10.1016/j.phymed.2022.154462
Li, Z., Zhang, Y., Chen, L., and Li, H. (2018). The dietary compound luteolin inhibits pancreatic cancer growth by targeting BCL-2. Food Funct. 9, 3018–3027. doi:10.1039/c8fo00033f
Li, Z., Zou, J., Cao, D., and Ma, X. (2020). Pharmacological basis of tanshinone and new insights into tanshinone as a multitarget natural product for multifaceted diseases. Biomed. Pharmacother. 130, 110599. doi:10.1016/j.biopha.2020.110599
Liao, H., Zhu, D., Bai, M., Chen, H., Yan, S., Yu, J., et al. (2020). Stigmasterol sensitizes endometrial cancer cells to chemotherapy by repressing Nrf2 signal pathway. Cancer Cell Int. 20, 480. doi:10.1186/s12935-020-01470-x
Lin, D., Kuang, G., Wan, J., Zhang, X., Li, H., Gong, X., et al. (2017). Luteolin suppresses the metastasis of triple-negative breast cancer by reversing epithelial-to-mesenchymal transition via downregulation of β-catenin expression. Oncol. Rep. 37, 895–902. doi:10.3892/or.2016.5311
Lu, J., Li, G., He, K., Jiang, W., Xu, C., Li, Z., et al. (2015). Luteolin exerts a marked antitumor effect in cMet-overexpressing patient-derived tumor xenograft models of gastric cancer. J. Transl. Med. 13, 42. doi:10.1186/s12967-015-0398-z
Ma, L., Peng, H., Li, K., Zhao, R., Li, L., Yu, Y., et al. (2015). Luteolin exerts an anticancer effect on NCI-H460 human non-small cell lung cancer cells through the induction of Sirt1-mediated apoptosis. Mol. Med. Rep. 12, 4196–4202. doi:10.3892/mmr.2015.3956
Manju, M., and Bharadvaja, N. (2024). Exploring the potential therapeutic approach using ginsenosides for the management of neurodegenerative disorders. Mol. Biotechnol. 66, 1520–1536. doi:10.1007/s12033-023-00783-2
Meng, G., Chai, K., Li, X., Zhu, Y., and Huang, W. (2016). Luteolin exerts pro-apoptotic effect and anti-migration effects on A549 lung adenocarcinoma cells through the activation of MEK/ERK signaling pathway. Chem. Biol. Interact. 257, 26–34. doi:10.1016/j.cbi.2016.07.028
Mensah-Nyagan, A. G., Meyer, L., Schaeffer, V., Kibaly, C., and Patte-Mensah, C. (2009). Evidence for a key role of steroids in the modulation of pain. Psychoneuroendocrinology 34 (Suppl. 1), S169–S177. doi:10.1016/j.psyneuen.2009.06.004
Mladenka, P., Zatloukalová, L., Filipský, T., and Hrdina, R. (2010). Cardiovascular effects of flavonoids are not caused only by direct antioxidant activity. Free Radic. Biol. Med. 49, 963–975. doi:10.1016/j.freeradbiomed.2010.06.010
Moeng, S., Son, S. W., Seo, H. A., Lee, J. S., Kim, C. K., Kuh, H. J., et al. (2020). Luteolin-regulated MicroRNA-301-3p targets caspase-8 and modulates TRAIL sensitivity in PANC-1 cells. Anticancer Res. 40, 723–731. doi:10.21873/anticanres.14003
Nagegowda, D. A., and Gupta, P. (2020). Advances in biosynthesis, regulation, and metabolic engineering of plant specialized terpenoids. Plant Sci. 294, 110457. doi:10.1016/j.plantsci.2020.110457
Noor, F., Tahir ul Qamar, M., Ashfaq, U. A., Albutti, A., Alwashmi, A. S. S., and Aljasir, M. A. (2022). Network pharmacology approach for medicinal plants: review and assessment. Pharm. (Basel) 15, 572. doi:10.3390/ph15050572
O’Neill, P. M., Barton, V. E., and Ward, S. A. (2010). The molecular mechanism of action of artemisinin--the debate continues. Molecules 15, 1705–1721. doi:10.3390/molecules15031705
Ong, C.-S., Zhou, J., Ong, C.-N., and Shen, H.-M. (2010). Luteolin induces G1 arrest in human nasopharyngeal carcinoma cells via the Akt-GSK-3β-Cyclin D1 pathway. Cancer Lett. 298, 167–175. doi:10.1016/j.canlet.2010.07.001
Pandey, P., Bajpai, P., Siddiqui, M. H., Sayyed, U., Tiwari, R., Shekh, R., et al. (2019). Elucidation of the chemopreventive role of stigmasterol against Jab1 in gall bladder carcinoma. Endocr. Metab. Immune Disord. Drug Targets 19, 826–837. doi:10.2174/1871530319666190206124120
Patel, A., Vanecha, R., Patel, J., Patel, D., Shah, U., and Bambharoliya, T. (2022). Development of natural bioactive alkaloids: anticancer perspective. Mini Rev. Med. Chem. 22, 200–212. doi:10.2174/1389557521666210712111331
Preet, G., Haj Hasan, A., Ramlagan, P., Fawdar, S., Boulle, F., and Jaspars, M. (2023). Anti-neurodegenerating activity: structure-activity relationship analysis of flavonoids. Molecules 28, 7188. doi:10.3390/molecules28207188
Procházková, D., Boušová, I., and Wilhelmová, N. (2011). Antioxidant and prooxidant properties of flavonoids. Fitoterapia 82, 513–523. doi:10.1016/j.fitote.2011.01.018
Qin, T., Zhao, J., Liu, X., Li, L., Zhang, X., Shi, X., et al. (2021). Luteolin combined with low-dose paclitaxel synergistically inhibits epithelial-mesenchymal transition and induces cell apoptosis on esophageal carcinoma in vitro and in vivo. Phytother. Res. 35, 6228–6240. doi:10.1002/ptr.7267
Racette, S. B., Lin, X., Ma, L., and Ostlund, R. E. (2015). Natural dietary phytosterols. J. AOAC Int. 98, 679–684. doi:10.5740/jaoacint.SGERacette
Ruan, J., Zhang, L., Yan, L., Liu, Y., Yue, Z., Chen, L., et al. (2012). Inhibition of hypoxia-induced epithelial mesenchymal transition by luteolin in non-small cell lung cancer cells. Mol. Med. Rep. 6, 232–238. doi:10.3892/mmr.2012.884
Ruan, Y., Yuan, P.-P., Li, P.-Y., Chen, Y., Fu, Y., Gao, L.-Y., et al. (2023). Tingli Dazao Xiefei Decoction ameliorates asthma in vivo and in vitro from lung to intestine by modifying NO-CO metabolic disorder mediated inflammation, immune imbalance, cellular barrier damage, oxidative stress and intestinal bacterial disorders. J. Ethnopharmacol. 313, 116503. doi:10.1016/j.jep.2023.116503
Serafini, M., Peluso, I., and Raguzzini, A. (2010). Flavonoids as anti-inflammatory agents. Proc. Nutr. Soc. 69, 273–278. doi:10.1017/S002966511000162X
Shanks, G. D. (2016). Historical review: problematic malaria prophylaxis with quinine. Am. J. Trop. Med. Hyg. 95, 269–272. doi:10.4269/ajtmh.16-0138
Song, D., Hao, J., and Fan, D. (2020). Biological properties and clinical applications of berberine. Front. Med. 14, 564–582. doi:10.1007/s11684-019-0724-6
Sung, H., Ferlay, J., Siegel, R. L., Laversanne, M., Soerjomataram, I., Jemal, A., et al. (2021). Global cancer statistics 2020: GLOBOCAN estimates of incidence and mortality worldwide for 36 cancers in 185 countries. CA Cancer J. Clin. 71, 209–249. doi:10.3322/caac.21660
Tjioe, K. C., Tostes Oliveira, D., and Gavard, J. (2016). Luteolin impacts on the DNA damage pathway in oral squamous cell carcinoma. Nutr. Cancer 68, 838–847. doi:10.1080/01635581.2016.1180411
Wang, M.-C., Wu, Y.-F., Yu, W.-Y., Yu, B., and Ying, H.-Z. (2022). Polyacetylenes from codonopsis lanceolata root induced apoptosis of human lung adenocarcinoma cells and improved lung dysbiosis. Biomed. Res. Int. 2022, 7713355. doi:10.1155/2022/7713355
Wang, Q., Wang, H., Jia, Y., Ding, H., Zhang, L., and Pan, H. (2017a). Luteolin reduces migration of human glioblastoma cell lines via inhibition of the p-IGF-1R/PI3K/AKT/mTOR signaling pathway. Oncol. Lett. 14, 3545–3551. doi:10.3892/ol.2017.6643
Wang, Q., Wang, H., Jia, Y., Pan, H., and Ding, H. (2017b). Luteolin induces apoptosis by ROS/ER stress and mitochondrial dysfunction in gliomablastoma. Cancer Chemother. Pharmacol. 79, 1031–1041. doi:10.1007/s00280-017-3299-4
Wang, S.-W., Chen, Y.-R., Chow, J.-M., Chien, M.-H., Yang, S.-F., Wen, Y.-C., et al. (2018). Stimulation of Fas/FasL-mediated apoptosis by luteolin through enhancement of histone H3 acetylation and c-Jun activation in HL-60 leukemia cells. Mol. Carcinog. 57, 866–877. doi:10.1002/mc.22807
Wang, T., Guo, R., Zhou, G., Zhou, X., Kou, Z., Sui, F., et al. (2016). Traditional uses, botany, phytochemistry, pharmacology and toxicology of Panax notoginseng (Burk.) F.H. Chen: a review. J. Ethnopharmacol. 188, 234–258. doi:10.1016/j.jep.2016.05.005
Wang, W., Chen, B., Zou, R., Tu, X., Tan, S., Lu, H., et al. (2014). Codonolactone, a sesquiterpene lactone isolated from Chloranthus henryi Hemsl, inhibits breast cancer cell invasion, migration and metastasis by downregulating the transcriptional activity of Runx2. Int. J. Oncol. 45, 1891–1900. doi:10.3892/ijo.2014.2643
Wang, X., Wang, Z.-Y., Zheng, J.-H., and Li, S. (2021). TCM network pharmacology: a new trend towards combining computational, experimental and clinical approaches. Chin. J. Nat. Med. 19, 1–11. doi:10.1016/S1875-5364(21)60001-8
Wang, Y., Kong, D., Wang, X., Dong, X., Tao, Y., and Gong, H. (2015). Molecular mechanisms of luteolin induced growth inhibition and apoptosis of human osteosarcoma cells. Iran. J. Pharm. Res. 14, 531–538.
Wu, H., Huang, M., Liu, Y., Shu, Y., and Liu, P. (2015). Luteolin induces apoptosis by up-regulating miR-34a in human gastric cancer cells. Technol. Cancer Res. Treat. 14, 747–755. doi:10.7785/tcrt.2012.500434
Wu, H.-T., Lin, J., Liu, Y.-E., Chen, H.-F., Hsu, K.-W., Lin, S.-H., et al. (2021). Luteolin suppresses androgen receptor-positive triple-negative breast cancer cell proliferation and metastasis by epigenetic regulation of MMP9 expression via the AKT/mTOR signaling pathway. Phytomedicine 81, 153437. doi:10.1016/j.phymed.2020.153437
Xin, T., Zhang, F., Jiang, Q., Chen, C., Huang, D., Li, Y., et al. (2012). The inhibitory effect of a polysaccharide from Codonopsis pilosula on tumor growth and metastasis in vitro. Int. J. Biol. Macromol. 51, 788–793. doi:10.1016/j.ijbiomac.2012.07.019
Xiong, Y., Zhong, W., Liu, J., Cheng, B., Fan, J., Zhou, F., et al. (2022). Luteolin isolated from polygonum cuspidatum is a potential compound against nasopharyngeal carcinoma. Biomed. Res. Int. 2022, 9740066. doi:10.1155/2022/9740066
Yang, Y., Yuan, L., Wang, K., Lu, D., Meng, F., Xu, D., et al. (2024). The role and mechanism of paeoniae Radix alba in tumor therapy. Molecules 29, 1424. doi:10.3390/molecules29071424
Yao, Y., Rao, C., Zheng, G., and Wang, S. (2019). Luteolin suppresses colorectal cancer cell metastasis via regulation of the miR-384/pleiotrophin axis. Oncol. Rep. 42, 131–141. doi:10.3892/or.2019.7136
Yi-wen, Z., Mei-hua, B., Xiao-ya, L., Yu, C., Jing, Y., and Hong-hao, Z. (2018). Effects of oridonin on hepatic cytochrome P450 expression and activities in PXR-humanized mice. Biol. Pharm. Bull. 41, 707–712. doi:10.1248/bpb.b17-00882
Yu, Q., Zhang, M., Ying, Q., Xie, X., Yue, S., Tong, B., et al. (2019). Decrease of AIM2 mediated by luteolin contributes to non-small cell lung cancer treatment. Cell Death Dis. 10, 218. doi:10.1038/s41419-019-1447-y
Yu, Y., Ding, S., Xu, X., Yan, D., Fan, Y., Ruan, B., et al. (2023). Integrating network pharmacology and bioinformatics to explore the effects of dangshen (codonopsis pilosula) against hepatocellular carcinoma: validation based on the active compound luteolin. Drug Des. Devel Ther. 17, 659–673. doi:10.2147/DDDT.S386941
Zang, M., Hu, L., Zhang, B., Zhu, Z., Li, J., Zhu, Z., et al. (2017). Luteolin suppresses angiogenesis and vasculogenic mimicry formation through inhibiting Notch1-VEGF signaling in gastric cancer. Biochem. Biophys. Res. Commun. 490, 913–919. doi:10.1016/j.bbrc.2017.06.140
Zang, Y.-Q., Feng, Y.-Y., Luo, Y.-H., Zhai, Y.-Q., Ju, X.-Y., Feng, Y.-C., et al. (2019). Glycitein induces reactive oxygen species-dependent apoptosis and G0/G1 cell cycle arrest through the MAPK/STAT3/NF-κB pathway in human gastric cancer cells. Drug Dev. Res. 80, 573–584. doi:10.1002/ddr.21534
Zeng, G., Wu, Z., Cao, W., Wang, Y., Deng, X., and Zhou, Y. (2020). Identification of anti-nociceptive constituents from the pollen of Typha angustifolia L. using effect-directed fractionation. Nat. Prod. Res. 34, 1041–1045. doi:10.1080/14786419.2018.1539979
Zhang, H., Li, S., Si, Y., and Xu, H. (2021). Andrographolide and its derivatives: current achievements and future perspectives. Eur. J. Med. Chem. 224, 113710. doi:10.1016/j.ejmech.2021.113710
Zhao, H., Zhang, X., Wang, M., Lin, Y., and Zhou, S. (2021). Stigmasterol simultaneously induces apoptosis and protective autophagy by inhibiting akt/mTOR pathway in gastric cancer cells. Front. Oncol. 11, 629008. doi:10.3389/fonc.2021.629008
Zhao, X., Wang, L., Suo, Q., Chen, H., Xie, Y., Zhang, H., et al. (2024). Research progress on the anti-tumor effects of dangshen(codonopsis Radix) and its active ingredients. Guid. J. Traditional Chin. Med. Pharm. 30, 121–125+155. doi:10.13862/j.cn43-1446/r.2024.03.024
Zheng, Y., Li, L., Chen, H., Zheng, Y., Tan, X., Zhang, G., et al. (2023). Luteolin exhibits synergistic therapeutic efficacy with erastin to induce ferroptosis in colon cancer cells through the HIC1-mediated inhibition of GPX4 expression. Free Radic. Biol. Med. 208, 530–544. doi:10.1016/j.freeradbiomed.2023.09.014
Zhou, Q., Yan, B., Hu, X., Li, X.-B., Zhang, J., and Fang, J. (2009). Luteolin inhibits invasion of prostate cancer PC3 cells through E-cadherin. Mol. Cancer Ther. 8, 1684–1691. doi:10.1158/1535-7163.MCT-09-0191
Zhu, L., and Chen, L. (2019). Progress in research on paclitaxel and tumor immunotherapy. Cell Mol. Biol. Lett. 24, 40. doi:10.1186/s11658-019-0164-y
Keywords: Radix Codonopsis, tumor, traditional Chinese medicine, mechanism, pharmacology
Citation: Liu C-Y, Li Z, Cheng F-E, Nan Y and Li W-Q (2025) Radix Codonopsis: a review of anticancer pharmacological activities. Front. Pharmacol. 15:1498707. doi: 10.3389/fphar.2024.1498707
Received: 11 October 2024; Accepted: 12 December 2024;
Published: 07 January 2025.
Edited by:
Javier Echeverria, University of Santiago, ChileReviewed by:
Afzal Basha Shaik, Jawaharlal Nehru Technological University, Kakinada, IndiaDaniel Gideon, St. Joseph’s University, India
Copyright © 2025 Liu, Li, Cheng, Nan and Li. This is an open-access article distributed under the terms of the Creative Commons Attribution License (CC BY). The use, distribution or reproduction in other forums is permitted, provided the original author(s) and the copyright owner(s) are credited and that the original publication in this journal is cited, in accordance with accepted academic practice. No use, distribution or reproduction is permitted which does not comply with these terms.
*Correspondence: Yi Nan, MjAwODAwMTFAbnhtdS5lZHUuY24=; Wei-Qiang Li, MjAwNjAwMTBAbnhtdS5lZHUuY24=