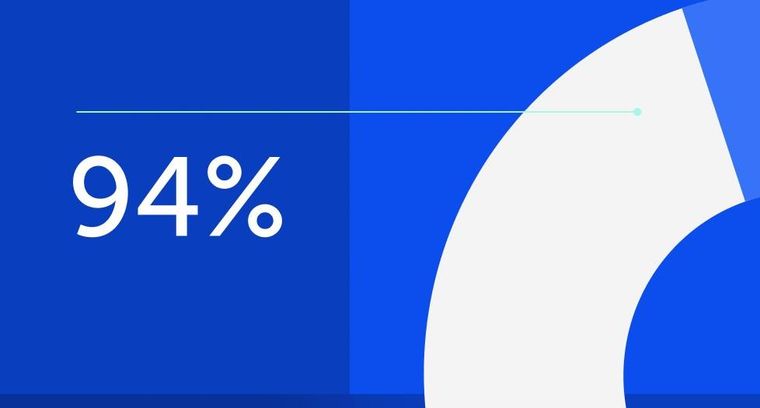
94% of researchers rate our articles as excellent or good
Learn more about the work of our research integrity team to safeguard the quality of each article we publish.
Find out more
ORIGINAL RESEARCH article
Front. Pharmacol., 07 January 2025
Sec. Inflammation Pharmacology
Volume 15 - 2024 | https://doi.org/10.3389/fphar.2024.1496874
Background: Oxyberberine (OBB) is a naturally occurring isoquinoline alkaloid that is believed to possess various health-promoting properties, including anti-fungus, hepatoprotection, anti-inflammation, and anti-intestinal mucositis effects. Despite several studies reporting the health benefits of OBB in treating ulcerative colitis (UC), its specific mechanism of action has yet to be fully elucidated.
Purpose: This investigation is designed to explore the potential protective efficacy of OBB and the latent mechanism using an in vitro model of UC-like inflammatory intestinal cells.
Methods: Caco-2 cells were pretreated with OBB and subsequently exposed to lipopolysaccharide (LPS). The transepithelial electrical resistance (TEER), paracellular permeability, and the distribution and expression of tight- and adherent junction proteins were determined to assess barrier integrity. The levels of proinflammatory cytokines, reactive oxygen species (ROS), Nrf2, and NF-κB signaling cascade were analyzed via ELISA, qRT-PCR, immunofluorescence, or Western blotting.
Results: OBB was found to mitigate the effects of LPS on Caco-2 cell monolayers, as evidenced by the improvement in TEER and the decrease in FITC-dextran flux. Moreover, OBB ameliorated the LPS-induced decrease in the distribution and expression of several tight junction markers, including ZO-1, occludin, and E-cadherin. In addition, OBB treatment effectively inhibited LPS-induced increases in ROS, apoptosis, and Keap1 and decreases in Nrf2 and HO-1. LPS-induced elevations in nuclear NF-κB p65 and p-IκBα were suppressed by OBB. In addition, ML385, an antagonist of Nrf2, abolished the protective role of OBB.
Conclusion: OBB has a pronounced beneficial effect on LPS-induced damage to enteral barrier function, and the regulation of the Nrf2/NF-κB pathway is an important mechanism responsible for the protection afforded by OBB.
Ulcerative colitis (UC), a main subtype of inflammatory bowel disease, is a chronic aspecific inflammation illness that primarily influences the distal colon and rectum (Feuerstein et al., 2019). At present, the occurrence and morbidity of UC are on the rise around the world, particularly in less-developed nations (Wei et al., 2021). Classical characteristics of UC involve bloody diarrhea, bellyache, urgent stools, and weight reduction. Clinically, patients suffering from UC often relapse and are susceptible to developing UC-associated cancer (Follin-Arbelet et al., 2023). To date, the therapeutic drugs of UC include aminosalicylic acids, thiopurines, immunosuppressors, glucocorticoids, and biologics (Li et al., 2022). However, the long-term use of these medicines has poor effectiveness, frequently results in serious adverse reactions, and imposes a weighty burden on society and families (Nasir et al., 2022; Tsujii et al., 2022).
A complicated interaction of inheritance, immunization, environment, and intestinal flora is considered to be the cause of UC (Lu et al., 2023; Ning et al., 2024). The exact etiology and pathogenic mechanism are still unclear. In recent years, numerous studies have proved that impaired gut barrier function is one of the primary traits of UC, and the impairment of enterocytes exerts an important effect on the pathogenesis of UC (Seidelin et al., 2021; Tian and Zhang, 2022). The gut epithelial barrier is a mechanical and physiological barrier that blocks the entry of antigens and pathogens into the bloodstream. It has been reported that disorders of the enteral barrier function could exacerbate inflammation and lead to the occurrence and development of UC (Han et al., 2022). However, no FDA-approved therapies target the epithelial barrier at present. Therefore, the search for efficient medicines that can reinforce the gut epithelial barrier is of enormous importance for the management of UC (Li C. L. et al., 2021; Zhang et al., 2023).
Lipopolysaccharide (LPS) exerts an important effect on enteric and full-body inflammation responses. Numerous studies have proved that LPS enhances tight junction (TJ) penetration and disrupts gut barrier functions (Han et al., 2023; Saxami et al., 2021). Moreover, continuous inflammatory stimulation leads to impaired gut TJ barrier function, which generates more pro-inflammation factors, creating a vicious spiral (Li M. H. et al., 2024). Thus, strategies that reduce inflammation reactions may be exciting therapeutic options for UC. A few in vitro experiments have proved that natural products such as berberine could alleviate LPS-elicited gut barrier impairment (Zhang et al., 2020). A ginger extract exhibited protection on the gut epithelium, with transepithelial electrical resistance (TEER) values rising compared to LPS-treated Caco-2 cells (Kim et al., 2017). Probiotic formulations prevented enteral barrier damage by modulating the expression of TJ proteins and inhibiting inflammatory responses (di Vito et al., 2022).
Berberine is a yellow coloring alkaloid acquired from the rhizome of Coptidis chinensis Franch. Over the years, berberine has attracted wide attention for its extensive pharmacological action comprising anti-tumor, anti-inflammation, anti-oxidation, and anti-bacterial activity (Khoshandam et al., 2022). A few studies showed that the application of berberine was impeded by its inferior bioavailability and rapid systemic elimination. Even with high dosages of berberine, the berberine concentrations are very low in serums and tissues (Thomas et al., 2021). Multiple studies have indicated that the metabolic products of berberine may contribute to its pharmacological actions in vivo (Cheng et al., 2022; Wang et al., 2017).
In our previous research, we first discovered that oxyberberine (OBB, C20H17NO5, Figure 1A), a gut microbiota-mediated oxidized product of berberine with a more active lactam ring, had a more favorable safety profile than berberine (Li et al., 2020). In vivo, OBB was an absorbable form of berberine administration, with an enteral absorption rate 5-fold higher than berberine (Feng et al., 2015). OBB is an isoquinoline alkaloid acquired from Coptidis chinensis Franch., Phellodendron chinense Schneid., and Argemone mexicana Linn. (Qian et al., 2015; Singh et al., 2016) that has a wide range of health-promoting properties. Importantly, OBB was shown to be more effective than berberine in various biological functions, including anti-inflammation (Li et al., 2019), anti-intestinal mucositis (Huang et al., 2022), anti-fungus (Singh et al., 2009), and anti-hypoglycemia (Dou et al., 2021). In previous studies, we first revealed that OBB alleviated dextran sulfate sodium (DSS) or 2, 4, 6-trinitrobenzenesulfonic acid (TNBS)-elicited murine UC via retarding inflammation, oxidative stress, and modulating the enteral microbiota (Li et al., 2020; Li et al., 2023). However, the specific mechanism is still not well illuminated.
Figure 1. Effects of OBB on Caco-2 cell viability and cytotoxicity. (A) Chemical composition of OBB. The Caco-2 viability was determined at increasing concentrations of OBB in the absence (B) or presence (C) of LPS (0.2 mg/mL) for 24 h. Cell viability was estimated using a CCK-8 assay. (D) Cytotoxicity was determined by LDH release. Results are expressed as mean ± SD (n = 6). ##P < 0.01 vs. Control; **P < 0.01 vs. LPS group; && P < 0.01 vs. OBB (40 μM) group.
To provide more profound evidence of the anti-UC effect and mechanism of OBB, our team sought to elucidate the likely role and molecular mechanism of OBB using an LPS-induced Caco-2 cell model. As far as we know, the present investigation is the foremost trial investigating the possible protection and underlying mechanisms of OBB in a UC cell model. The results indicated that OBB had a pronounced beneficial effect on LPS-mediated damage to gut barrier function and that regulation of the Nrf2/NF-κB pathway was a pivotal target responsible for the protection afforded by OBB. This research will provide further support for the potential of OBB as an innovative treatment for UC.
OBB was synthesized following the procedure described in our prior research (Li et al., 2020). ML385 was sourced from APExBIO. LPS (from Escherichia coli 055:B5) was obtained from Sigma-Aldrich (St. Louis, MO, United States). Human ELISA kits, including TNF-α, IL-1β, IL-2, and IL-6, were supplied by MLBIO (Shanghai, China), and IL-8 and IL-12 kits were supplied by Meimian (Jiangsu, China). The reactive oxygen species (ROS) detection kit was obtained from Meilunbio (Dalian, China). Lactate dehydrogenase (LDH) was obtained from Beyotime Biotechnology. The Annexin V-FITC/PI double staining apoptosis determination kit was acquired by BestBio (Shanghai, China). Primary antibodies for NF-κB p65, ZO-1, occludin, and E-cadherin were provided by Baijia Biosciences (Taizhou, China). Nrf2, Keap1, HO-1, IκBα, and p-IκBα were purchased from Affinity Biosciences (OH, United States). DyLight 488 AffiniPure Goat Anti-Rabbit IgG was obtained from Earthox. DAPI was provided by Solarbio (Beijing, China).
Human colon cancer cell line Caco-2 was purchased from iCell Bioscience Inc. (Shanghai, China). Caco-2 cells were cultivated in MEM media supplemented with 10% fetal bovine serum (FBS) and 1% penicillin-streptomycin antibiotics at 37°C in 5% CO2. The medium was replaced every 2 days. Cells were starved in serum-free media for 12 h prior to every test. Cells at passages 10–20 were used in the experiments.
The cellular viability of OBB was assessed via a CCK-8 experiment based on the manufacturer’s specifications. Caco-2 cells (1 × 104/well) were seated in a 96-well microplate and cultured in medium containing 10% FBS for 36 h. Then, the cells were washed twice with PBS and cultured in serum-free medium for 12 h. The cells were then subjected to distinct concentrations of OBB for 24 h or to OBB for 2 h and supplemented with LPS for a further 24 h. Subsequently, 10 μL CCK-8 solution was supplemented and further fostered for 2 h. Finally, the OD values were determined at 450 nm by a microplate reader.
LDH is a stable cytosolic enzyme present throughout the cell. It is promptly secreted into the supernatant of cell cultures when there is membrane damage, which is a critical characteristic of cells experiencing apoptosis, necrosis, and other types of cell injury (Kumar et al., 2018). The cytotoxic effects of OBB (10 μM, 20 μM, 40 μM) or OBB in combination with ML385 on Caco-2 cells were assessed via measurement of LDH leakage into the extracellular medium according to the manufacturer’s instructions.
TEER was inspected to estimate the barrier integrality of Caco-2 cells. Cells (1 × 104 cells/well) were cultured in the upper chamber of the transwell, complete medium was added to the lower chamber, and the plate was placed in a 37°C, 5% CO2 cell culture incubator. Cellular TEER values were detected at fixed times using a Millicell® ERS-2 cytoresistometer (Millipore, Merk, United States) until TEER values stabilized to indicate monolayer epithelial barrier formation. After 14–18 days, the cell resistance value was basically stable, and the cell monolayer barrier had formed. The transwell plates were gently washed twice with PBS, and the TEER values were measured at 0 h, 2 h, 6 h, 12 h, 24 h, and 48 h after adding the drugs.
The penetrability of the Caco-2 single-cell layer was determined through the flux of FITC-dextran (150 kDa, MCE, United States). FITC-dextran solution (1 mg/mL, dissolved in HBSS) was supplemented into the upper chamber of the transwell, and HBSS was added into the lower chamber. After incubating for 1 h, the lower chamber aliquots were gathered for the detection of fluorescence at 490 nm (excited wavelength) and 520 nm (emissive wavelength).
Caco-2 cells (1 × 104 cells/well) were seeded into 96-well plates and cultured in medium containing 10% FBS for 36 h. Then, the cells were washed twice with PBS and cultured in serum-free medium for 12 h. The cells were subsequently pre-treated with different concentrations of OBB (10 μM, 20 μM, and 40 μM) or OBB (40 μM) + ML385 (10 μM) for 2 h, and treatment was followed with LPS (0.2 mg/mL) for 24 h. The concentrations and exposure periods of the compounds used in the study are based on preliminary experiments. The supernatants were obtained, and the levels of inflammatory cytokines (TNF-α, IL-1β, IL-2, IL-6, IL-8, and IL-12) were assessed based on the directions of nitric oxide assay kit and ELISA kits, respectively.
ROS was assessed using a specific probe DCFH-DA, which can rapidly penetrate the cell membrane and be converted into DCFH within the cell. In the presence of ROS, DCFH was oxidized to form the fluorescent green substance DCF (Kim and Xue, 2020). Both a fluorescent microscope and spectrofluorometry were used to estimate ROS levels. Cells were dispersed in distinct concentrations of OBB or OBB combined ML385 for 2 h, followed by adding LPS for further incubation for 24 h. The cells were then washed with pre-cooling PBS and incubated with 10 μM DCFH-DA at 37°C for 20–30 min. Fluorescence intensity was examined at an excitation wavelength of 488 nm and an emission wavelength of 525 nm.
Cells (5 × 104/well) were cultured on a 24-well plate. After preparation, the cells were rinsed using PBS three times and fixed using 4% paraformaldehyde for 30 min. Triton-100 buffer (0.2%) was then applied to penetrate cells at room temperature for 15 min. After being washed with PBS three times, the cells were blocked using 2% BSA for 30 min. Subsequently, the primary antibodies, including ZO-1, occludin, and E-cadherin, were supplemented and coincubated with the cells at 4°C overnight. Then, the cells were coincubated with DyLight 488 AffiniPure Goat Anti-Rabbit IgG for 1 h under ambient temperature. After staining with DAPI solution under dark conditions for 10 min, a fluorescence microscope was employed to inspect and photograph the cells.
Cells were excited using 0.2 mg/mL LPS for 24 h with or without OBB and OBB combined with ML385 pretreatment for 2 h. Then, cells were assimilated, gathered, and rinsed using pre-cooling PBS. Flow cytometric analysis was conducted based on the instructions of the apoptotic kit.
Total RNA was acquired with TRIzol reagent following the manufacturer’s specification. cDNA was synthesized from total RNA. The conditions of RT-PCR were 95°C for 30 s, then 95°C for 15 s, and 60°C for 30 s, repeated 40 times. The reaction was carried out by a Real-Time PCR System (Bio-rad, CA, United States). The cycle threshold (Ct) value was counted and normalized to the abundance of a reference gene GAPDH. The specific primers are as follows: COX-2, forward, GGGTTGCTGGTGGTAGGAATG, reverse, CATAAAGCGTTTGCGGTACTCAT; iNOS, forward, GACTCACAGCCTTTGGACCTCA, reverse, GCTGGATGTCGGACTTTGTAGATT, GAPDH, forward, GGAAGCTTGTCATCAATGGAAATC, reverse, TGATGACCCTTTTGGCTCCC.
Total, cytoplasmic, and nuclear proteins were abstracted from Caco-2 cells using corresponding reagent kits. Protein levels were then detected by adopting a BCA protein assay kit (Beyotime). The protein sample was segregated using SDS-PAGE and shifted to a PVDF membrane. Following closure with 5% skim milk, the membrane was coincubated with proper primary antibodies overnight at 4°C and subsequently coincubated with secondary antibodies at ambient temperature for 2 h. Following three rinses with TBST, the blots were visualized through electrochemiluminescence plus reagent (Invitrogen). Image J software was used to quantify the band intensity.
The results are indicated by the mean ± standard deviation (SD). All statistics were determined using the IBM SPSS26.0 statistical program. All assays were performed at three or more times. One-way ANOVA followed by Tukey’s post hoc tests was adopted to compare the discrepancies between each group. The degree of significance was set at P < 0.05.
The possible cytotoxic impact of OBB (chemical structure shown in Figure 1A) was evaluated by the CCK-8 assay in Caco-2 cells. Figure 1B demonstrated that OBB alone did not display any cytotoxicity compared to the normal control up to 50 μM. In contrast, at a higher concentration of 100 μM, considerable cell toxicity was observed compared to the control group. Moreover, as shown in Figure 1C, 0.2 mg/mL LPS intervention for 24 h reduced the cellular activity compared to the control group (P < 0.01). However, OBB (10 μM, 20 μM, and 40 μM) elevated the cellular activity in a dosage-reliant mode compared to LPS alone (P < 0.05). Therefore, OBB at the concentrations of 10 μM, 20 μM, and 40 μM were employed in the below studies according to the cytotoxic result.
Nrf2 plays a role as a modulator in inhibiting oxidative damage and inflammation of colitis, and our previous research showed that OBB may reduce DSS-evoked colitis in mice via activating Nrf2. Therefore, ML385, a specific suppressor of Nrf2, was used in this study. LDH leakage was considered a marker of cellular toxicity. We investigated the possibility that the LPS-induced cytotoxic response to LDH leakage was abolished by OBB. As shown in Figure 1D, LPS (0.2 mg/mL) caused a marked cytotoxic influence on LDH leakage. However, pretreatment with OBB (10 μM, 20 μM, and 40 μM) reduced the cytotoxicity elicited by LPS, which was reversed by ML385.
TEER was employed to detect the single-layer integrity of Caco-2 cells. TEER levels were evaluated at 0 h, 2 h, 6 h, 12 h, 24 h, 36 h, and 48 h following LPS with or without OBB (10 μM, 20 μM, and 40 μM) and OBB combined with ML385 (10 μM) treatment. As shown in Figure 2A, LPS contributed to the marked decline of TEER values following 2 h interventions, which continued up to 48 h after treatment. In contrast, OBB elevated TEER values in a dose-dependent mode, which were then converted by ML385.
Figure 2. Effect of OBB on the intestinal barrier function in LPS-stimulated Caco-2 cells. (A) TEER values were measured at 0 h, 2 h, 6 h, 12 h, 24 h, and 48 h following LPS stimulation with or without OBB and OBB in combination with ML385 treatment. (B) The intestinal barrier permeability of Caco-2 cells was determined by employing the FITC-dextran flux assay. (C–F) The protein expressions of ZO-1, occludin, and E-cadherin in Caco-2 cells were inspected via Western blotting. β-actin was used as the internal control. Values in the figures are represented by mean ± SD (n = 3). ##P < 0.01 vs. Control; *P < 0.05, **P < 0.01 vs. LPS group; &P < 0.05, &&P < 0.01 vs. OBB (40 μM) group.
In addition, we determined the permeation of large solutes in Caco-2 cells by adopting the FITC-dextran flux test. Compared with the untreated group, the FITC-dextran levels in the basal chamber of the LPS group increased (P < 0.05). In contrast, OBB administration dose-dependently decreased the leakage of FITC-dextran caused by LPS treatment compared to the LPS group (Figure 2B), which was then attenuated by ML385. These findings indicated that OBB can attenuate LPS-mediated enteral epithelial barrier impairment in vitro.
Modifications in TJ protein expressions have been implicated in gut barrier disturbances triggered by inflammatory factors (Barbara et al., 2021). Therefore, we investigated the function of OBB on the expression of the TJ protein ZO-1, occludin, and E-cadherin in Caco-2 cells exposed to LPS. As illustrated in Figures 2C–F, the protein expression of cellular ZO-1, occludin, and E-cadherin was reduced by the treatment with LPS compared to the blank group (P < 0.05). The OBB application restored the levels of ZO-1, occludin, and E-cadherin in a dose-dependent mode (P < 0.05 vs. LPS), which was subsequently reversed by ML385.
Previous evidence has revealed that inflammation-induced gut barrier dysfunction was involved in the structural destruction and relocalization of TJ proteins (Cao et al., 2013). Therefore, we sought to determine whether OBB altered the morphological localization of TJ proteins in Caco-2 cells following exposure to LPS through immunofluorescent analysis. As shown in Figure 3, in the control group, the TJ proteins ZO-1, occludin, and E-cadherin were each localized to the intercellular TJ alongside the cell edge. Caco-2 cells treated with LPS exhibited a distinct reorganization of the TJ proteins ZO-1, occludin, and E-cadherin, resulting in irregular and discontinuous dispersion patterns. However, OBB therapy significantly mitigated the LPS-induced morphologic destruction of ZO-1, occludin, and E-cadherin in Caco-2 cells, and this protective effect was then mitigated by ML385. These results showed that OBB maintained the integrality of Caco-2 cells by restraining the decline and reconstitution of TJ proteins in LPS-evoked Caco-2 cells.
Figure 3. OBB suppresses the morphologic destruction of tight junctions evoked by LPS. Typical tight junction proteins ZO-1 (A), occludin (B), and E-cadherin (C) were visualized through immunofluorescent analysis and DAPI staining of nuclei in Caco-2 cells (scale bar 100 μm).
As displayed in Figure 4, compared to the untreated group, LPS disposal enhanced the levels of proinflammatory cytokines comprising TNF-α, IL-1β, IL-2, IL-6, IL-8, and IL-12, and upregulated the mRNA levels of iNOS and COX-2 (P < 0.05). OBB treatment could mitigate the inflammatory response compared to the LPS-evoked Caco-2 cells (P < 0.05). Furthermore, the improvement of OBB was subsequently reversed by ML385, which indicated that OBB could restrain the inflammatory reaction in LPS-evoked Caco-2 cells by activating Nrf2 signaling.
Figure 4. Influence of OBB toward LPS-elicited cellular inflammation. (A–F) The protein contents of TNF-α, IL-1β, IL-2, IL-6, IL-8, and IL-12 were detected via ELISA assay. (G, H) Relative mRNA expressions of iNOS and COX-2. The data are represented by mean ± SD (n = 3–6). #P < 0.05, ##P < 0.01 vs. Control; *P < 0.05, **P < 0.01 vs. LPS group; &P < 0.05, &&P < 0.01 vs. OBB (40 μM) group.
Fluorescence microscopy showed that the green fluorescence intensity for LPS-treated cells in Caco-2 cells was increased compared to the control group (Figures 5A, B). The spectrofluorometry study revealed that the intensity of ROS was increased in the LPS-treated Caco-2 cell (Figure 5C). By contrast, the pretreatment with OBB (10 μM, 20 μM, and 40 μM) inhibited the ROS intensity level in LPS-treated cells, which was reversed by ML385 treatment.
Figure 5. Inspection of cellular ROS via the DCFH-DA test. Influence of OBB on LPS-evoked ROS content was determined using a fluorescent microscope image (A), the average mean fluorescence intensity of ROS (B), and spectrophotometric fluorescence intensity (C). Scale bar represents 200 μm. The data are represented by mean ± SD (n = 3–6). ##P < 0.01 vs. Control; **P < 0.01 vs. LPS group; &&P < 0.01 vs. OBB (40 μM) group.
As displayed in Figure 6, LPS significantly elevated cellular apoptosis compared to the normal group (P < 0.01). The OBB remedy (10 μM, 20 μM, and 40 μM) reduced the apoptosis rate dose-dependently compared to LPS alone (P < 0.05). The anti-apoptosis role of OBB was reversed by ML385 treatment.
Figure 6. OBB restrains the cellular apoptosis of Caco-2 cells. (A) Following OBB intervention for 24 h, cellular apoptosis was inspected using the Annexin V FITC/PI method and flow cytometry. (B) Percentage of apoptosis cells. The apoptotic rate was determined by summing the percentages of early apoptosis and late apoptosis. Values are presented by mean ± SD (n = 3). ##P < 0.01 vs. Control; *P < 0.05, **P < 0.01 vs. LPS group.
The expressions of Nrf2, Keap1, HO-1, NF-κB p65 (nuclear), IκBα, and p-IκBα were inspected via Western blotting. As presented in Figure 7, the expressions of Keap1, nuclear p65, and p-IκBα were enhanced in LPS-elicited cells compared to the control group (P < 0.05). OBB (10 μM, 20 μM, and 40 μM) treatment lowered the levels of Keap1, nuclear p65, and p-IκBα/IκBα and elevated the expressions of Nrf2 and HO-1 in a dose-dependent manner (P < 0.05). However, the regulation of OBB on the above proteins was reversed by the Nrf2 inhibitor, ML385. The proofs indicated that OBB regulated the Nrf2/NF-κB signal paths to prevent LPS-elicited cellular damage in Caco-2 cells.
Figure 7. Impacts of OBB on Nrf2/NF-κB signal paths in LPS-elicited cellular damage in Caco-2 cells. The protein expressions of Nrf2, Keap1, HO-1, NF-κB p65 (nuclear), IκBα, and p-IκBα in Caco-2 cells were detected via Western blot. The data are represented by mean ± SD (n = 3). ##P < 0.01 vs. Control; *P < 0.05, **P < 0.01 vs. LPS group; &P < 0.05, &&P < 0.01 vs. OBB (40 μM) group.
Colitis is a chronic inflammatory gastrointestinal disease characterized by periods of remission and relapse. It affects the quality of life for many people in advanced nations worldwide (Cichewicz et al., 2023). The etiology of colitis is not yet fully comprehended, and present therapy is very unsatisfactory. It is highly ineffectual and also may cause severe adverse reactions among some patients (Panés et al., 2024; Zhang et al., 2024). Thus, novel interventions and therapeutical methods are required to fight inflammatory diseases.
Our preceding research has proved that OBB could ameliorate inflammatory reactions in DSS or TNBS-induced murine colitis. However, the role of OBB toward inflammatory reactions in IECs in damaged states is still unclear. The evidence of CCK-8 indicated that OBB reduced cellular activity at a 50 μM concentration compared to untreated cells (P < 0.05) after 24 h of culture. It indicated that OBB possessed no toxicity to Caco-2 cells after 24 h at concentrations less than 100 μM.
The required role of the enteric epithelial barrier is the intake of electrolytes and nutrients, but it also serves as a semipermeable barrier that exerts a crucial action in defending the body against microbiological pathogens and possibly toxic materials (Bergheim and Moreno-Navarrete, 2024; Yao et al., 2024). Dysfunction of the enteric epithelial barrier increases gut penetrability. It causes many kinds of serious enteric inflammatory diseases, including inflammatory bowel disease (IBD), necrotizing enterocolitis, irritable bowel syndrome (IBS), and metabolic syndrome (Barbara et al., 2021; Li H. L. et al., 2024). The appropriate function of the enteric epithelial barrier is kept by epithelial cells and intercellular TJs. TJs consist of a set of transmembranous proteins (occludins and claudins), peripheric membranous proteins (zonula occludens), and adjacent adherens junctions (E-cadherin), which interact with each other and constitute a selecting barrier through interplaying with adjacent cells (Peng et al., 2023). Former studies have proved that damaging TJs results in the increased permeability of the enteric epithelial barrier (Horowitz et al., 2023). Thus, TJ proteins are vital for maintaining the integrity of the enteric epithelial barrier.
We found that OBB ameliorated the enteric epithelial barrier dysfunction aroused by proinflammatory factors in vitro. Caco-2 single-cell layers treated with or without OBB for 2 h were subjected to 0.2 mg/mL LPS for a further 24 h. The results indicated that LPS injured epithelial barrier function and induced an abnormal inflammatory response, which is in agreement with the preceding investigation (Nathani et al., 2023; Zheng et al., 2022). The administration of OBB ameliorated the decline in TEER and the rise in intercellular penetrability in a dose-dependent manner compared to sole LPS intervention. These results suggested that OBB defends the barrier function of Caco-2 cells from LPS-evoked injury. In addition, the Western blot test showed that the protein expression of ZO-1, E-cadherin, and occludin was down-modulated in Caco-2 cells subjected to 0.2 mg/mL LPS. Administration of OBB up-modulated expression of the TJ proteins compared to sole LPS intervention. The immunofluorescent result of TJ proteins showed that OBB mitigated LPS-elicited changes in the distribution of TJ proteins. The results indicated that OBB exerts a main action in maintaining epithelial barrier function by mitigating LPS-elicited variations in the expression and distribution of TJ proteins.
Enteric inflammation was revealed to be related to enteric epithelial barrier damage. Many studies have proved that enteric epithelial inflammation could injure TJs by down-modulating the level of TJ proteins via inflammatory signal transduction (Zheng et al., 2023). NF-κB is a crucial transcriptional factor that adjusts inflammatory reactions by regulating the transcription of diversified cell genes and has been authenticated as a therapeutical target for the remedy of enteric inflammation and IBD (Amirshahrokhi and Imani, 2023; Tsopmejio et al., 2022). LPS exposure evokes IκBα phosphorylation, bringing about IκBα degradation, which leads to the release and translocation of NF-κB from the cytoplasm to the nucleus. Inside the nucleus, p65 upmodulates the transcription of proinflammatory mediators and cytokines such as COX-2, TNF-α, iNOS, and IL-6 (Ghasemi-Dehnoo et al., 2023; Kim et al., 2023). Studies have indicated that OBB suppressed the inflammatory response by restraining the NF-κB signal path. This study identified the NF-κB signaling pathway as a crucial mechanism underlying the regulation of the intestinal monolayer barrier by OBB. The results showed that LPS-evoked NF-κB excitation in Caco-2 cells was restrained by OBB. Furthermore, OBB reverted the up-modulation of LPS-elicited IL-6, iNOS, TNF-α, and COX-2 expression in a dose-dependent mode at the mRNA and protein levels.
Enteric epithelial oxidant stress, characterized by superfluous ROS generation, has been found to break the enteric epithelial barrier and induce mucosa disorders (Zeng et al., 2023). Additionally, elevated production of ROS can accelerate inflammatory reactions in the intestinal tract, leading to the pathogeny of IBD (Zhang et al., 2023). Nrf2 is regarded as the master cellular sensor of oxidant stress. Nrf2 is a basic-leucine zipper transcription factor that exists in the cytoplasm, binding to Keap1 in homeostatic circumstances (Ulasov et al., 2022). When cells are subjected to oxidant stress, Nrf2 dissociates from Keap1 and translocates into the nucleus, where it binds to the antioxidant-responsive element (ARE), bringing about the increased expression of antioxidative genes involving HO-1 and NQO-1 (Li J. C. et al., 2021). Earlier studies showed that the Nrf2/HO-1 path has assumed one of the upriver molecules considered targets for anti-NF-κB-evoked inflammation (Yan et al., 2021). Targeted activation of Nrf2 signaling can effectively regulate the intestinal mucosal barrier function (Dong et al., 2023). In the present research, OBB intervention restrained LPS-evoked down-modulation of Nrf2 and HO-1 and up-modulation of NF-κB. It is critical that the Nrf2 depressor ML385 abolished the protection of OBB on Caco-2 cells, partly counteracted OBB-elicited excitation of Nrf2 signaling, and suppressed the NF-κB signal path, indicating that OBB restrained the NF-κB-reliant inflammatory reaction via motivating the Nrf2/HO-1 path. Thus, the protection of OBB against LPS-elicited enteric epithelial barrier damage was partly modulated by excitation of the Nrf2 signal and suppression of the NF-κB signal path. By confirming the excitation of the antioxidant adaptive response and the restraint of inflammation, the Nrf2 and NF-κB signal paths increase the protection caused by OBB against LPS-evoked inflammation in the enteric epithelia.
In conclusion, the present study indicated that OBB plays a protective effect on LPS-evoked colitis by repressing oxidative damage, inflammatory reactions, and apoptosis and increasing intestinal TJ proteins in Caco-2 cells. Furthermore, Nrf2 was important in the development of colitis, and OBB was able to trigger Nrf2 signaling and inhibit the Nrf2-initiated NF-κB path to achieve its regulatory effect in human Caco-2 cells (Figure 8). Collectively, this evidence may help ensure the employment of OBB as a therapeutical method for inflammation-related disease control in patients with colitis.
Figure 8. Proposed mechanism of OBB amelioration of LPS-induced barrier function dysfunction, oxidative stress, and inflammatory reaction in Caco-2 cells.
The original contributions presented in the study are included in the article/Supplementary Material, further inquiries can be directed to the corresponding author.
Ethical approval was not required for the studies on humans in accordance with the local legislation and institutional requirements because only commercially available established cell lines were used. Ethical approval was not required for the studies on animals in accordance with the local legislation and institutional requirements because only commercially available established cell lines were used.
CL: conceptualization, funding acquisition, supervision, and writing–original draft. JW: data curation, investigation, methodology, and writing–original draft. HY: formal analysis, investigation, and writing–original draft. SL: investigation, validation, and writing–review and editing. QL: conceptualization, investigation, supervision, and writing–review and editing.
The author(s) declare that financial support was received for the research, authorship, and/or publication of this article. This study was supported by the National Natural Science Foundation of China (Nos 82003771, 82360809, 82160785, and 82304828), the future “Science and Technology Elite” project (No. ZYSE-2022-01), and the Science and Technology Foundation of Guizhou Province (No. QKHJC-ZK[2021]YB525).
The authors declare that the research was conducted in the absence of any commercial or financial relationships that could be construed as a potential conflict of interest.
All claims expressed in this article are solely those of the authors and do not necessarily represent those of their affiliated organizations, or those of the publisher, the editors and the reviewers. Any product that may be evaluated in this article, or claim that may be made by its manufacturer, is not guaranteed or endorsed by the publisher.
The Supplementary Material for this article can be found online at: https://www.frontiersin.org/articles/10.3389/fphar.2024.1496874/full#supplementary-material
Amirshahrokhi, K., and Imani, M. (2023). Levetiracetam attenuates experimental ulcerative colitis through promoting Nrf2/HO-1 antioxidant and inhibiting NF-κB, proinflammatory cytokines and iNOS/NO pathways. Int. Immunopharmacol. 119, 110165. doi:10.1016/j.intimp.2023.110165
Barbara, G., Barbaro, M. R., Fuschi, D., Palombo, M., Falangone, F., Cremon, C., et al. (2021). Inflammatory and microbiota-related regulation of the intestinal epithelial barrier. Front. Nutr. 8, 718356. doi:10.3389/fnut.2021.718356
Bergheim, I., and Moreno-Navarrete, J. M. (2024). The relevance of intestinal barrier dysfunction, antimicrobial proteins and bacterial endotoxin in metabolic dysfunction-associated steatotic liver disease. Eur. J. Clin. Invest. 54 (7), e14224. doi:10.1111/eci.14224
Cao, M., Wang, P., Sun, C. H., He, W., and Wang, F. J. (2013). Amelioration of IFN-γ and TNF-α-induced intestinal epithelial barrier dysfunction by berberine via suppression of MLCK-MLC phosphorylation signaling pathway. PLoS One 8 (5), e61944. doi:10.1371/journal.pone.0061944
Cheng, H., Liu, J., Tan, Y. Z., Feng, W. W., and Peng, C. (2022). Interactions between gut microbiota and berberine, a necessary procedure to understand the mechanisms of berberine. J. Pharm. Anal. 12 (4), 541–555. doi:10.1016/j.jpha.2021.10.003
Cichewicz, A., Tencer, T., Gupte-Singh, K., Egodage, S., Burnett, H., and Kumar, J. (2023). A systematic review of the economic and health-related quality of life impact of advanced therapies used to treat moderate-to-severe ulcerative colitis. Adv. Ther. 40 (5), 2116–2146. doi:10.1007/s12325-023-02488-z
di Vito, R., Conte, C., and Traina, G. (2022). A multi-strain probiotic formulation improves intestinal barrier function by the modulation of tight and adherent junction proteins. Cells 11 (16), 2617. doi:10.3390/cells11162617
Dong, X., Wang, Y., Zhu, X. X., Shen, L. Y., Chen, L., Niu, L. L., et al. (2023). Sodium butyrate protects against rotavirus-induced intestinal epithelial barrier damage by activating AMPK-Nrf2 signaling pathway in IPEC-J2 cells. Int. J. Biol. Macromol. 228, 186–196. doi:10.1016/j.ijbiomac.2022.12.219
Dou, Y. X., Huang, R. L., Li, Q. P., Liu, Y. H., Li, Y. C., Chen, H. B., et al. (2021). Oxyberberine, an absorbed metabolite of berberine, possess superior hypoglycemic effect via regulating the PI3K/Akt and Nrf2 signaling pathways. Biomed. Pharmacother. 137, 111312. doi:10.1016/j.biopha.2021.111312
Feng, R., Shou, J. W., Zhao, Z. X., He, C. Y., Ma, C., Huang, M., et al. (2015). Transforming berberine into its intestine-absorbable form by the gut microbiota. Sci. Rep. 5, 12155. doi:10.1038/srep12155
Feuerstein, J. D., Moss, A. C., and Farraye, F. A. (2019). Ulcerative colitis. Mayo Clin. Proc. 94 (7), 1357–1373. doi:10.1016/j.mayocp.2019.01.018
Follin-Arbelet, B., Smastuen, M. C., Hovde, O., Jelsness-Jorgensen, L. P., and Moum, B. (2023). Incidence of cancer in patients with ulcerative colitis 30 years after diagnosis (the IBSEN study). Scand. J. Gastroenterol. 58 (11), 1264–1270. doi:10.1080/00365521.2023.2223709
Ghasemi-Dehnoo, M., Amini-Khoei, H., Lorigooini, Z., AnjomShoa, M., and Rafieian-Kopaei, M. (2023). Ferulic acid ameliorates ulcerative colitis in a rat model via the inhibition of two LPS-TLR4-NF-κB and NF-κB-INOS-NO signaling pathways and thus alleviating the inflammatory, oxidative and apoptotic conditions in the colon tissue. Inflammopharmacology 31 (5), 2587–2597. doi:10.1007/s10787-023-01277-y
Han, F. F., Zhao, X., Li, X., Peng, L. Y., Liu, W. L., and Han, J. Z. (2022). Bovine lactoferricin ameliorates intestinal inflammation and mucosal barrier lesions in colitis through NF-κB/NLRP3 signaling pathways. J. Funct. Foods 93, 105090. doi:10.1016/j.jff.2022.105090
Han, H., You, Y., Cha, S., Kim, T. R., Sohn, M., and Park, J. (2023). Multi-species probiotic strain mixture enhances intestinal barrier function by regulating inflammation and tight junctions in lipopolysaccharides stimulated Caco-2 cells. Microorganisms 11 (3), 656. doi:10.3390/microorganisms11030656
Horowitz, A., Chanez-Paredes, S. D., Haest, X., and Turner, J. R. (2023). Paracellular permeability and tight junction regulation in gut health and disease. Nat. Rev. Gastroenterol. Hepatol. 20 (7), 417–432. doi:10.1038/s41575-023-00766-3
Huang, R. L., Ai, G. X., Zhong, L. J., Mai, L. T., Chen, J. N., Liu, Y. H., et al. (2022). Protective effects of oxyberberine in 5-fluorouracil-induced intestinal mucositis in the mice model. Evid-based Compl. Alt. Med. 2022, 1238358. doi:10.1155/2022/1238358
Khoshandam, A., Imenshahidi, M., and Hosseinzadeh, H. (2022). Pharmacokinetic of berberine, the main constituent of Berberis vulgaris L.: a comprehensive review. Phytother. Res. 36 (11), 4063–4079. doi:10.1002/ptr.7589
Kim, H., and Xue, X. (2020). Detection of total reactive oxygen species in adherent cells by 2′,7′-dichlorodihydrofluorescein diacetate staining. Jove J. Vis. Exp. 160, e60682. doi:10.3791/60682
Kim, M. G., Kim, S., Boo, K. H., Kim, J. H., and Kim, C. S. (2023). Anti-inflammatory effects of immature Citrus unshiu fruit extracts via suppression of NF-κB and MAPK signal pathways in LPS-induced RAW264.7 macrophage cells. Food Sci. Biotechnol. 33 (4), 903–911. doi:10.1007/s10068-023-01390-2
Kim, Y., Kim, D. M., and Kim, J. Y. (2017). Ginger extract suppresses inflammatory response and maintains barrier function in human colonic epithelial Caco-2 cells exposed to inflammatory mediators. J. Food Sci. 82 (5), 1264–1270. doi:10.1111/1750-3841.13695
Kumar, P., Nagarajan, A., and Uchil, P. D. (2018). Analysis of cell viability by the lactate dehydrogenase assay. Cold Spring Harb. Protoc. 2018 (6), pdb.prot095497–468. doi:10.1101/pdb.prot095497
Li, C. L., Ai, G. X., Wang, Y. F., Lu, Q., Luo, C. D., Tan, L. H., et al. (2020). Oxyberberine, a novel gut microbiota-mediated metabolite of berberine, possesses superior anti-colitis effect: impact on intestinal epithelial barrier, gut microbiota profile and TLR4-MyD88-NF-kappa B pathway. Pharmacol. Res. 152, 104603. doi:10.1016/j.phrs.2019.104603
Li, C. L., Dong, N., Wu, B. W., Mo, Z. M., Xie, J. H., and Lu, Q. (2021). Dihydroberberine, an isoquinoline alkaloid, exhibits protective effect against dextran sulfate sodium-induced ulcerative colitis in mice. Phytomedicine 90, 153631. doi:10.1016/j.phymed.2021.153631
Li, C. L., Liu, M. G., Deng, L., Luo, D. D., Ma, R. F., and Lu, Q. (2023). Oxyberberine ameliorates TNBS-induced colitis in rats through suppressing inflammation and oxidative stress via Keap1/Nrf2/NF-kappa B signaling pathways. Phytomedicine 116, 154899. doi:10.1016/j.phymed.2023.154899
Li, C. L., Tan, L. H., Wang, Y. F., Luo, C. D., Chen, H. B., Lu, Q., et al. (2019). Comparison of anti-inflammatory effects of berberine, and its natural oxidative and reduced derivatives from Rhizoma Coptidis in vitro and in vivo. Phytomedicine 52, 272–283. doi:10.1016/j.phymed.2018.09.228
Li, C. L., Wang, J. H., Ma, R. F., Li, L. H., Wu, W. F., Cai, D. K., et al. (2022). Natural-derived alkaloids exhibit great potential in the treatment of ulcerative colitis. Pharmacol. Res. 175, 105972. doi:10.1016/j.phrs.2021.105972
Li, H. L., Pu, X., Lin, Y. T., Yu, X. X., Li, J., Bo, L., et al. (2024). Sijunzi decoction alleviates inflammation and intestinal epithelial barrier damage and modulates the gut microbiota in ulcerative colitis mice. Front. Pharmacol. 15, 1360972. doi:10.3389/fphar.2024.1360972
Li, J. C., Lu, K. M., Sun, F. L., Tan, S. J., Zhang, X., Sheng, W., et al. (2021). Panaxydol attenuates ferroptosis against LPS-induced acute lung injury in mice by Keap1-Nrf2/HO-1 pathway. J. Transl. Med. 19 (1), 96. doi:10.1186/s12967-021-02745-1
Li, M. H., Zhao, D. Y., Guo, J. L., Pan, T. X., Niu, T. M., Jiang, Y. Q., et al. (2024). Bacillus halotolerans SW207 alleviates enterotoxigenic Escherichia coli-induced inflammatory responses in weaned piglets by modulating the intestinal epithelial barrier, the TLR4/MyD88/NF-κB pathway, and intestinal microbiota. Microbiol. Spectr. 12 (4), e0398823. doi:10.1128/spectrum.03988-23
Lu, Q., Xie, Y. H., Luo, J. B., Gong, Q. H., and Li, C. L. (2023). Natural flavones from edible and medicinal plants exhibit enormous potential to treat ulcerative colitis. Front. Pharmacol. 14, 1168990. doi:10.3389/fphar.2023.1168990
Nasir, S. A., Patel, R., Wojkiewicz, S., and Scatena, R. (2022). Infliximab-induced non-specific interstitial pneumonitis in a patient with ulcerative colitis (UC). Cureus J. Med. Sci. 14 (12), e33064. doi:10.7759/cureus.33064
Nathani, S., Das, N., Katiyar, P., Waghmode, B., Sircar, D., and Roy, P. (2023). Consumption of honey ameliorates lipopolysaccharide-induced intestinal barrier dysfunction via upregulation of tight junction proteins. Eur. J. Nutr. 62, 3033–3054. doi:10.1007/s00394-023-03203-y
Ning, H., Liu, J. M., Tan, J. Q., Yi, M. N., and Lin, X. Y. (2024). The role of the Notch signalling pathway in the pathogenesis of ulcerative colitis: from the perspective of intestinal mucosal barrier. Front. Med. 10, 1333531. doi:10.3389/fmed.2023.1333531
Panés, J., D'Haens, G. R., Sands, B. E., Ng, S. C., Lawendy, N., Kulisek, N., et al. (2024). Analysis of tofacitinib safety in ulcerative colitis from the completed global clinical developmental program up to 9.2 years of drug exposure. United Eur. Gastroenterol. J. 12, 793–801. doi:10.1002/ueg2.12584
Peng, J., Li, H., Olaolu, O. A., Ibrahim, S., Ibrahim, S., and Wang, S. Y. (2023). Natural products: a dependable source of therapeutic alternatives for inflammatory bowel disease through regulation of tight junctions. Molecules 28 (17), 6293. doi:10.3390/molecules28176293
Qian, X. C., Zhang, L., Tao, Y., Huang, P., Li, J. S., Chai, C., et al. (2015). Simultaneous determination of ten alkaloids of crude and wine-processed Rhizoma Coptidis aqueous extracts in rat plasma by UHPLC-ESI-MS/MS and its application to a comparative pharmacokinetic study. J. Pharm. Biomed. Anal. 105, 64–73. doi:10.1016/j.jpba.2014.11.049
Saxami, G., Kerezoudi, E. N., Mitsou, E. K., Koutrotsios, G., Zervakis, G. I., Pletsa, V., et al. (2021). Fermentation supernatants of Pleurotus eryngii mushroom ameliorate intestinal epithelial barrier dysfunction in lipopolysaccharide-induced Caco-2 cells via upregulation of tight junctions. Microorganisms 9 (10), 2071. doi:10.3390/microorganisms9102071
Seidelin, J. B., Bahl, M. I., Licht, T. R., Mead, B. E., Karp, J. M., Johansen, J. V., et al. (2021). Acute experimental barrier injury triggers ulcerative colitis-specific innate hyperresponsiveness and ulcerative colitis-type microbiome changes in humans. Cell. Mol. Gastroenterol. Hepatol. 12 (4), 1281–1296. doi:10.1016/j.jcmgh.2021.06.002
Singh, A., Singh, S., Singh, S., Singh, T., Singh, V., Pandey, V. B., et al. (2009). Fungal spore germination inhibition by alkaloids dehydrocorydalmine and oxyberberine. J. Plant Prot. Res. 49 (3), 287–289. doi:10.2478/v10045-009-0046-9
Singh, S., Verma, M., Malhotra, M., Prakash, S., and Singh, T. D. (2016). Cytotoxicity of alkaloids isolated from Argemone mexicana on SW480 human colon cancer cell line. Pharm. Biol. 54 (4), 740–745. doi:10.3109/13880209.2015.1073334
Thomas, A., Kamble, S., Deshkar, S., Kothapalli, L., and Chitlange, S. (2021). Bioavailability of berberine: challenges and solutions. Istanb. J. Pharm. 51 (1), 141–153. doi:10.26650/IstanbulJPharm.2020.0056
Tian, Y. G., and Zhang, D. K. (2022). Biological clock and inflammatory bowel disease review: from the standpoint of the intestinal barrier. Gastroenterol. Res. Pract. 2022, 2939921. doi:10.1155/2022/2939921
Tsopmejio, I. S. N., Ding, M., Wei, J. L., Zhao, C., Jiang, Y., Li, Y. T., et al. (2022). Auricularia polytricha and Flammulina velutipes ameliorate inflammation and modulate the gut microbiota via regulation of NF-κB and Keap1/Nrf2 signaling pathways on DSS-induced inflammatory bowel disease. Food Biosci. 47, 101426. doi:10.1016/j.fbio.2021.101426
Tsujii, Y., Nishida, T., Osugi, N., Fujii, Y., Sugimoto, A., Nakamatsu, D., et al. (2022). Classification and clinical features of adverse drug reactions in patients with ulcerative colitis treated with 5-aminosalicylate acid: a single-center, observational study. Scand. J. Gastroenterol. 57 (2), 190–196. doi:10.1080/00365521.2021.1998601
Ulasov, A. V., Rosenkranz, A. A., Georgiev, G. P., and Sobolev, A. S. (2022). Nrf2/Keap1/ARE signaling: towards specific regulation. Life Sci. 291, 120111. doi:10.1016/j.lfs.2021.120111
Wang, K., Feng, X. C., Chai, L. W., Cao, S. J., and Qiu, F. (2017). The metabolism of berberine and its contribution to the pharmacological effects. Drug Metab. Rev. 49 (2), 139–157. doi:10.1080/03602532.2017.1306544
Wei, S. C., Sollano, J., Hui, Y. T., Yu, W., Estrella, P. V. S., Llamado, L. J. Q., et al. (2021). Epidemiology, burden of disease, and unmet needs in the treatment of ulcerative colitis in Asia. Expert Rev. Gastroenterol. Hepatol. 15 (3), 275–289. doi:10.1080/17474124.2021.1840976
Yan, N., Xu, Z. P., Qu, C. H., and Zhang, J. J. (2021). Dimethyl fumarate improves cognitive deficits in chronic cerebral hypoperfusion rats by alleviating inflammation, oxidative stress, and ferroptosis via NRF2/ARE/NF-kappa B signal pathway. Int. Immunopharmacol. 98, 107844. doi:10.1016/j.intimp.2021.107844
Yao, Y. K., Shang, W. J., Bao, L. Y., Peng, Z. Y., and Wu, C. (2024). Epithelial-immune cell crosstalk for intestinal barrier homeostasis. Eur. J. Immunol. 54 (6), e2350631. doi:10.1002/eji.202350631
Zeng, N. Y., Wu, F., Lu, J. Q., Li, X., Lin, S. M., Zhou, L., et al. (2023). High-fat diet impairs gut barrier through intestinal microbiota-derived reactive oxygen species. Sci. China Life Sci. 67, 879–891. doi:10.1007/s11427-022-2283-4
Zhang, H., Mu, C. L., Gu, Y., Meng, F. Y., Qin, X. L., and Cao, H. L. (2024). Selection strategy of second-line biologic therapies in adult patients with ulcerative colitis following prior biologic treatment failure: systematic review and meta-analysis. Pharmacol. Res. 202, 107108. doi:10.1016/j.phrs.2024.107108
Zhang, Y., Lei, H. Y., Wang, P. C., Zhou, Q. Y., Yu, J., Leng, X., et al. (2023). Restoration of dysregulated intestinal barrier and inflammatory regulation through synergistically ameliorating hypoxia and scavenging reactive oxygen species using ceria nanozymes in ulcerative colitis. Biomater. Res. 27 (1), 75. doi:10.1186/s40824-023-00412-8
Zhang, Z., Li, X. Y., Li, C. L., Xiong, W., Zeng, R. R., Huang, J., et al. (2020). Effect of berberine on LPS-induced expression of NF-kappa B/MAPK signalling pathway and related inflammatory cytokines in porcine intestinal epithelial cells. Innate Immun. 26 (7), 627–634. doi:10.1177/1753425920930074
Zheng, J. S., Ahmad, A. A., Yang, Y. Y., Liang, Z. Y., Shen, W. X., Feng, M., et al. (2022). Lactobacillus rhamnosus CY12 enhances intestinal barrier function by regulating tight junction protein expression, oxidative stress, and inflammation response in lipopolysaccharide-induced Caco-2 cells. Int. J. Mol. Sci. 23 (19), 11162. doi:10.3390/ijms231911162
Zheng, S. M., Zhao, X., Huang, J. J., Tian, Q. M., Xu, S. Y., Bo, R. N., et al. (2023). Eugenol alleviates Salmonella Typhimurium-infected cecal injury by modulating cecal flora and tight junctions accompanied by suppressing inflammation. Microb. Pathog. 179, 106113. doi:10.1016/j.micpath.2023.106113
Keywords: ulcerative colitis, oxyberberine, intestinal barrier, inflammation, Nrf2, NF-κB
Citation: Li C, Wang J, Yang H, Luo S and Lu Q (2025) Oxyberberine alleviates lipopolysaccharide-induced intestinal barrier disruption and inflammation in human colonic Caco-2 cells in vitro. Front. Pharmacol. 15:1496874. doi: 10.3389/fphar.2024.1496874
Received: 15 September 2024; Accepted: 12 December 2024;
Published: 07 January 2025.
Edited by:
Alessandra Gambero, Pontifical Catholic University of Campinas, BrazilReviewed by:
Marta Szandruk-Bender, Wroclaw Medical University, PolandCopyright © 2025 Li, Wang, Yang, Luo and Lu. This is an open-access article distributed under the terms of the Creative Commons Attribution License (CC BY). The use, distribution or reproduction in other forums is permitted, provided the original author(s) and the copyright owner(s) are credited and that the original publication in this journal is cited, in accordance with accepted academic practice. No use, distribution or reproduction is permitted which does not comply with these terms.
*Correspondence: Qiang Lu, bHVxaWFuZ3ptdUAxNjMuY29t
Disclaimer: All claims expressed in this article are solely those of the authors and do not necessarily represent those of their affiliated organizations, or those of the publisher, the editors and the reviewers. Any product that may be evaluated in this article or claim that may be made by its manufacturer is not guaranteed or endorsed by the publisher.
Research integrity at Frontiers
Learn more about the work of our research integrity team to safeguard the quality of each article we publish.