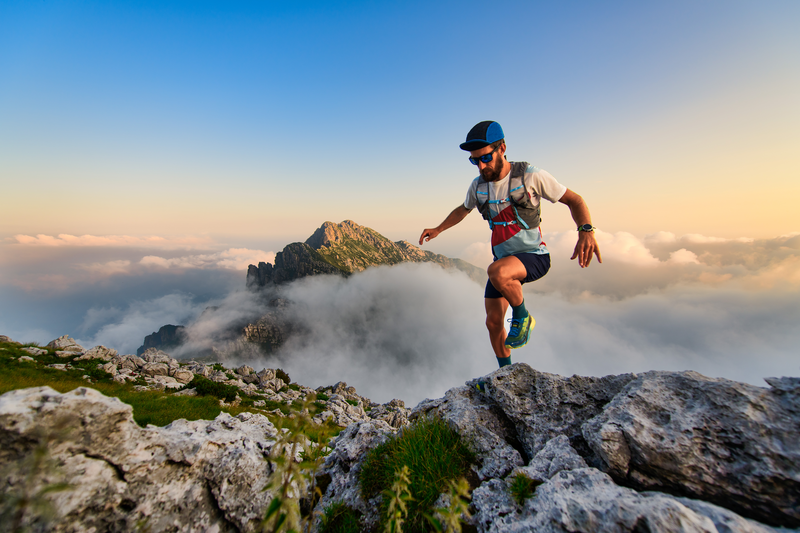
94% of researchers rate our articles as excellent or good
Learn more about the work of our research integrity team to safeguard the quality of each article we publish.
Find out more
POLICY AND PRACTICE REVIEWS article
Front. Pharmacol. , 03 February 2025
Sec. Ethnopharmacology
Volume 15 - 2024 | https://doi.org/10.3389/fphar.2024.1496792
Climate change and human activities severely impact the viability of plants and ecosystems, threatening the environment, biodiversity, and the sustainable development of plant-based products. Biotic and abiotic (ecosystem) determinants affect species distribution and long-term survival, which in turn influence the quality of plants used as herbal medicines and other high-value products. In recent decades, diverse anthropogenic impacts have significantly affected these quality aspects. Climate change, excessive plant exploitation, habitat loss, species vulnerability, and other factors have adversely affected the growth, reproduction, and adaptation of species populations, as well as the quality and volume of primary plant materials supplied to pharmaceutical markets. Despite these growing challenges, there is limited knowledge of potential strategies to prevent or mitigate these impacts, particularly for vulnerable species collected from the wild or harvested from traditional production systems. Hence, effective strategies for preserving and increasing plant populations are urgently needed. In this study, we propose a new framework including the main sustainability factors to better understand and address the vulnerability of a species, hence mitigate the impact of climate change. We assess the applicability of our proposed framework via seven case studies of vulnerable species (i.e., Aquilaria malaccensis Lam., Boswellia sacra Flück., Crocus sativus L., Panax quinquefolius L., Pilocarpus microphyllus Stapf ex Wardlew., Rhodiola rosea L., and Warburgia salutaris (G.Bertol.) Chiov.) from main biogeographic realms, all widely used as medicinal plants. These species present various challenges related to the sustainability of their use, impacting their current and future status locally and globally. Their economic importance, combined with rising demands and specific risks of overexploitation, are also key factors considered here. The suggested framework for the sustainability of medicinal and other high-value plant-based products in the phytopharmaceutical industry emphasises strategies that promote conservation and sustainable resource use. It can also be adapted for other vulnerable species requiring urgent attention.
Plants are a rich source of unique primary and secondary metabolites, many of which serve as medicines. They play a critical role as key ingredients in pharmaceuticals, functional/health foods, cosmetics, fragrances, agrochemicals, flavours, colouring agents, spices, biopesticides, and general food additives. There has been a dramatic growth (Howes et al., 2020) in demand for such ingredients not only in established economies but also in fast-emerging markets, for example, the People’s Republic of China, Middle Eastern countries, India, Brazil, México, and South Africa (Rezaie et al., 2012; Carvalho et al., 2018; Qu et al., 2014; Liu et al., 2023). Despite the strict quality control standards for medicinal plants in pharmacopoeias (Heinrich, 2015), there is no systematic framework for ensuring sustainable practices in sourcing current and future medicinal and other high-value plant-based products, nor to address the critical research and development needs in the context of climate change.
Dramatic changes to the global climate (Young et al., 2015), which have accelerated enormously over the last few decades, strongly impact the supply and sourcing of wild plant populations and cultivated resources, especially the established and traditional production systems (Applequist et al., 2020; Schindler and Hilborn, 2015; Chen et al., 2016). The dangerously unsustainable rates of anthropogenic damage–to the atmosphere, topsoil, forests, freshwater, ocean resources, and biodiversity–were central concerns of the first “World Scientists’ Warning to Humanity” (1992) (Ripple et al., 2017). Despite this, there remains a lack of broad societal understanding of our responsibilities and insufficient scientific methods and tools to evaluate how the production and trade of medicinal/health food plants (MHFPs) contribute to climate change and how climate change impacts their sustainable sourcing. This includes effects on supply, costs, quality, and consumer acceptance of MHFPs. This disruption may lead to reduced production of herbal medicines or increased risk of adulteration. Furthermore, the impacts of climate on plant resources and their implications for pharmaceutical supply chains have not been systematically documented. The medicinal plant sector needs to assess its environmental footprint, especially regarding how production and sourcing may contribute to or mitigate climate change (Howes et al., 2020; Pacifici et al., 2015). Unlike other areas, such as animal protection, where research and actions have been prioritised (Advani, 2023; Patni et al., 2022), the environmental impacts of MHFP sourcing and production require a more dedicated focus.
Previous studies, including surveys, frameworks, and systematic analyses of published data, have mainly focused on the problem of climate change and plants (Young et al., 2015; Eckardt et al., 2023; Parmesan and Hanley, 2015; Abbass et al., 2022; Ali et al., 2024), tracking population changes among populations of vulnerable species in this context (Pacifici et al., 2015; Pearce-Higgins et al., 2022; Yesuf et al., 2021) and exploring regionally restricted conservation strategy (Chen et al., 2016; Feigin et al., 2023; Wang et al., 2024a; McLaughlin et al., 2022) to save plants and biodiversity (Howes et al., 2020; Advani, 2023; Wang et al., 2024a; Mori et al., 2024). However, no comprehensive approach enables researchers to determine the critical stages of a species’ status or vulnerability in the context of pharmaceutical/health food needs, including the harvesting of high-quality and pharmacognostically well-defined primary material. The framework for developing the necessary advancements based on evidence-based scientific approaches to achieve sustainability and the conservation of specific species is poorly developed. Contrary to high-value, large volume food crops, medicinal plants are typically high-value, low-volume products (Atanasov et al., 2015; Booker et al., 2012). Sustainably managing MHFP is essential for both biodiversity conservation and ecosystem resilience. Ecosystem services (Reid et al., 2005; Hawken et al., 2021), including climate regulation and pollination are strengthened by diverse plant ecosystems.
The concept of ecosystem services focuses on the benefits derived from nature, allowing an evaluation of different benefits beyond strictly economic or environmental trade-offs alone. This approach requires a comprehensive understanding of ecological functions, sustainable practices, and socio-economic dynamics. Adapting research strategies to focus on ecosystem services is essential, as this approach integrates ecological, economic, and social factors, supporting sustainable development and the long-term availability of plant-based resources. By aligning research strategies with sustainability goals, we can help protect biodiversity, support human health, and strengthen resilience in the face of climate change. Here, we focus on utilitarian aspects, but ecosystem services also include the cultural importance of these species and the environments from which they are extracted (Milcu et al., 2013; Daniel et al., 2012).
In this study, we propose a framework to redefine research priorities in the research and development of MHFP. Our proposed conceptual approach addresses the research and development needs linking specific actions for specific species and suggests main environmental factors that influence species abundance, spanning biotic and anthropogenic determinants (Figure 1). For instance, increased species vulnerability–represented by colour changes in the central circle–arises under the influence of biological and anthropogenic determinants. These broader determinants can be understood based on significant shifts in one or more of the four specific determinants of a species’ sustainability: “habitat/habitat loss”; “reproductive success”; “climate change”; and “bio-economy (uses)”, which we propose as key sustainability indicators for addressing a species vulnerability in the context of climate change (Figure 1). We define three levels of concern (with a simple colour coding) to assess the degree of a species’ vulnerability (Table 1), which, of course, represents a continuum of risks.
Figure 1. The conceptual framework for addressing the degree of a vulnerability of a species based on selected sustainability determinants in the context of climate change, incorporating a spectrum from biotic to anthropogenic determinants.
Table 1. Degree of vulnerability of a species (key sustainability indicators) in the context of climate change defined using three levels of concern (colour coding). All levels are estimates based on complex datasets and they provide a qualitative orientation.
While the proposed framework will not directly influence climate change or policy in the short term, it provides the necessary scientific foundation and strategic direction to drive future advancements in these areas. By outlining core research priorities, the framework aims to support impactful changes that align with long-term goals for environmental sustainability, research and policy development Centered around the Sustainable Development Goals (SDGs). The focus here is on species that are of major economic importance in the respective regions and which are core elements within the regional environments (i.e., they can be conserved using locally suitable strategies). While the focus here is on the vulnerability of individual species, any change to a species abundance clearly impacts both the wider ecosystem in a region and the communities using these resources (both positively and negatively).
While risks are often associated with the overexploitation of resources such as specific species or a habitat, developing a research strategy requires a clear distinction between biological (natural) and anthropogenic determinants. These biological determinants include biotic and abiotic elements related to a species’ habitat, such as the extent of its distribution and its reproductive capacity. Key considerations involve the physical environment and the specific biological conditions for a species’ survival and successful reproduction. Abiotic determinants are not considered here in detail, since, while important, they can generally not be influenced. Anthropogenic determinants include changes in production systems related to climate, climate change, and the demand for specific species as ingredients. These anthropogenic (or human) determinants are closely interconnected with biotic (and abiotic) determinants that modulate the ecosystem, each having the potential to impact the other (Figure 1). Understanding these interactions is crucial for creating a comprehensive research strategy that safeguards ecological balance and promotes the sustainable use of plant resources.
The first key biotic (and abiotic) determinant is habitat, encompassing the natural environment where species usually live. In this context. It also includes environments created through human activities like agricultural production systems and secondary forests. Understanding both the global abundance of materia prima and the impact of human-induced habitat changes is essential for assessing climate change effects on resource utilisation. Habitat shifts–driven by urbanisation, agriculture, or climate change–can directly influence the availability and sustainability of plant-based resources. The second core biotic determinant is a species’ vulnerability, which is closely tied to reproductive ability (weediness) and adaptability to changing conditions as well as to its habitat. Medicinal plant species such as Hypericum perforatum L. (St. John’s wort) demonstrate high reproductive success (Koperdáková et al., 2004; Barcaccia et al., 2006), making reproductive ability a vital criterion for assessing ecological risks and resilience. While this reproductive strength can offer a buffer against environmental threats, it also underscores the need to balance the risks and benefits associated with its use and cultivation.
Two anthropogenic determinants are crucial: one is a species response to climate change. In other words, the impact of increasing average temperatures, changes to the rainfall pattern, and lower minimum temperatures. The second key biotic determinant, influenced by climate and impacting plants directly, is insect activity, which significantly affects plant populations. For example, orchid species such as Cephalanthera rubra (L.) Rich. and Cephalanthera longifolia (L.) Fritsch (Taura and Gudžinskas, 2024) fruited abundantly in the second half of the 20th century. However, their recent population decline is believed to be linked to climate change. One possible explanation is a desynchronisation between flowering times and pollinator activity (Pyke et al., 2016). Detailed studies of flowering phenology and pollinator dynamics across the species’ range are essential to validate this hypothesis.
Lastly, the bioeconomy, or the level of resource use (including overuse), is a crucial aspect to consider. This covers all elements related to production per se and how resources are utilised within both global and regional economic frameworks. Two major drivers stand out: first, the direct destruction of habitats due to urban expansion and other land-use changes, which reduce the availability of natural resources. Second, the recent surge in demand for certain “boom” products over the last 3 decades, fueled by internet-based commodification, such as roseroot (Rhodiola rosea L.), elder (Sambucus nigra L.), and maca (Lepidium meyenii Walp.). Understanding which production systems and value chains (Booker and Heinrich, 2016) can minimise the negative impact of a species’ use within the global bioeconomy is crucial. This includes addressing challenges associated with botanical drugs like root/rhizome and bark, which are particularly vulnerable to overharvesting, and commercialisation is vital for maintaining both ecological balance and economic viability.
To evaluate the applicability of the proposed framework, we selected seven case studies of vulnerable species: Aquilaria malaccensis Lam., Boswellia sacra Flück., Crocus sativus L., Panax quinquefolius L., Pilocarpus microphyllus Stapf ex Wardlew., Rhodiola rosea L. and Warburgia salutaris (G.Bertol.) Chiov.) (Figure 2). These species present unique, and example challenges related to their use, affecting their current and future status at both local and global scales. The main selection criteria included here are their presence across different biogeographic realms, economic importance, widespread use, rising demand, and specific risks of overexploitation (Figure 2).
Figure 2. Overview of the seven selected case studies. The selection criteria for these species were: 1) The different challenges related to their use that impact on their current and future status at both local and global levels; 2) The different biogeographic realms; 3) The economic importance of the species, wide use, combined with increasing demands and particular risks of overexploitation (UN Environmental Programme, 2024; Convention on International Trade in Endangered Species CITES, 2024; CITES, 2024; IUCN, 2021).
The seven case studies are categorised using the three levels of concerns shown in Table 1, with individual Tables provided for each case study in their respective sections. Through this framework, we evaluate the available knowledge on each species in relation to the four main sustainability determinants in the context of climate change and their implications for redefining research and development strategies (Figure 2; Tables 2–8).
Table 2. Aquilaria malaccensis Lam. as a case study for vulnerable plant species in SE Asia/Oceania; for the three levels of concern, see Table 1.
Table 3. Boswellia sacra Flück as a case study for vulnerable species in the Middle East; for the three levels of concern, see Table 1.
Table 4. Crocus sativus L. as a case study for vulnerable species in Europe/Middle East; for the three levels of concern, see Table 1.
Table 5. Panax quinquefolius L. as a case study of vulnerable species in North America; for the three levels of concern, see Table 1.
Table 6. Pilocarpus microphyllus Stapf ex Wardlew. as a case study for vulnerable species in South America; for the three levels of concern, see Table 1.
Table 7. Rhodiola rosea L. as a case study for vulnerable species in Asia, Europe and the entire holarctic; for the three levels of concern, see Table 1.
Table 8. Warburgia salutaris (G.Bertol.) Chiov., as a case study for vulnerable species in southern Africa; for the three levels of concern, see Table 1.
A. malaccensis is widely distributed in Bangladesh, Bhutan, India, Indonesia, Malaysia, Myanmar, Nepal, Philippines, Singapore, and Thailand (Oldfield et al., 1998). It is now listed as critically endangered by the IUCN in all those countries (IUCN, 2024). A. malaccensis produces a highly valuable fragrant resin that impregnates the heartwood (agarwood) in response to fungal and other microbial infections (Chhipa and Kaushik, 2017).
The wood has been traded globally for centuries and is deeply rooted in some religions’ spiritual culture. Beyond its cultural and religious uses, it is also traditionally used for health problems, such as skin problems, thyroid problems, and joint pain (Grosvenor et al., 1995). The versatile uses of agarwood have led to a global increase in demand, endangering the natural populations of A. malaccensis. Overharvesting and unsustainable harvesting techniques are the main problems causing the decline of wild populations. In the past, collectors harvested agarwood from the forest by cutting dozens of Aquilaria trees because they could not accurately identify which trees contained resin and were ready to be harvested (Irnayuli et al., 2011).
Regarding production, Indonesia, Malaysia, and Thailand are the largest exporting countries in South Asia. From 2000 to 2023, these countries exported 2.448,5, 2.158,5, and 1.827,6 metric tons of agarwood chips, respectively. In the case of Indonesia and Malaysia, practically all material is collected from the wild (99,6%). Thailand and Malaysia are the biggest exporters of essential oil products, with around 4,2 and 1.5 metric tons from 2000 to 2023. On the other hand, around 57% of Thailand’s oil production comes from plantation agarwood of three species, whereas only 19% is produced in Malaysia (Thompson et al., 2022).
It is estimated that more than 60 million trees have been planted (IUCN, 2024) and resin induction techniques have been developed to meet high market demand and maintain the sustainability of wild plant habitats (Thompson et al., 2022). In contrast, the trees are found in low densities (up to 2/ha) in the wild, and only a small percentage of all non-cultivated trees–between 1% and 10% – produce agarwood with valuable resin (Thompson et al., 2022). Artificial methods for inducing infection have been developed to produce the resin using highly virulent fungi like Fusarium spp. This method has successfully increased the relative share in exports of products from cultivation compared to wild-harvested products (Thompson et al., 2022). However, the increase in the ratio does not necessarily prevent a decline in wild populations. Illegal harvesting still occurs in almost all producing countries because the agarwood harvested from wild Aquilaria is generally considered higher quality than cultivated Aquilaria (Kanazawa, 2017; Tamuli et al., 2005).
Another strategy to address overexploitation is logging restrictions and prohibitions for the species. Wild Aquilaria logging is illegal in several countries, with only Indonesia and Malaysia still allowing it under quotas and controls. China, Malaysia, Nepal, and Indonesia use assisted natural regeneration to rebuild wild populations. Only oil and live plants are subject to export licenses in India, while raw materials are unavailable (Thompson et al., 2022).
Although the survival and habitat of A. malaccensis seem to be impacted by climate change, it depends mainly on annual average rainfall, soil pH, and yearly average temperature. Changes in temperature, rainfall, and precipitation associated with climate change will affect the survival of this species. Tropical plants are predicted to face the most significant risk from climate warming as they experience temperatures closer to their upper germination limits (Sentinella et al., 2020). This is supported by evidence that recent droughts in parts of Southeast Asia (e.g., Thailand and Myanmar) have resulted in high agarwood tree mortality in some plantations (Thompson et al., 2022). Moreover, pests and diseases also threaten the life of this species. In several cultivation sites in India, most plantations face high mortality rates due to dry soil, moist weather, and high temperatures (Bhattacharjee et al., 2024). Furthermore, sap-suckers, leaf defoliators, and wood borers (insects from the orders Coleoptera and Lepidoptera), and several diseases are known to damage leaves, stems, and roots, especially in young plants (Samsuddin et al., 2019). Overharvesting and unsustainable harvesting are the main problems with this species’ availability in nature. Changes in temperature and rainfall correlated with climate change can also change the distribution and population, accelerating the decline of the wild population (Table 2).
B. sacra trees and other species produce an aromatic resin known as frankincense, widely used in religious rituals, perfumes, and as an ingredient in traditional medicines. Frankincense has a known medical and religious history dating back nearly 5,000 years (DeCarlo et al., 2023). This resin has been used to treat wounds, skin infections, inflammatory diseases, dementia, and other ailments, in medicine systems including Ayurvedic and TCM. Its widespread use over thousands of years makes it one of the oldest globally traded commodities (DeCarlo et al., 2020; Khalifa et al., 2023).
B. sacra is native to the arid regions of Oman, Yemen, Ethiopia, Somalia, and Kenya (Hamdiah et al., 2022; Eshete et al., 2012; DeCarlo et al., 2023). Harsh climatic conditions, including high temperatures and low rainfall, are the characteristics of these areas. Climate change poses an additional threat to this species, as rising temperatures and changes in precipitation patterns may affect its growth and resin production (Mwijuke, 2024; DeCarlo et al., 2020; Eshete et al., 2012; Gonzalez, 2020). Climate models predict increased temperatures and decreased precipitation in the growing regions of B. sacra and Boswellia papyrifera (Rajpoot et al., 2020; Gidey et al., 2020). This may lead to habitat loss and reduced species viability. Other factors influencing the decline of tree populations include fires, animal grasing, changes in land use, insect attacks, and improper harvesting (DeCarlo et al., 2020). In Ethiopia, the world’s largest exporter of frankincense, the Boswellia tree is predicted to become almost extinct within the next 3 decades (Johnson et al., 2022; DeCarlo et al., 2020).
Trade in frankincense has increased significantly in recent decades due to high demand in global markets. However, this increased demand has led to overexploitation, threatening the sustainability of the species (Gonzalez, 2020). In impoverished regions of Somali, cutting down and collecting is a primary and crucial source of income for the deprived populated areas (DeCarlo et al., 2020). The problem is aggravated by the fact that Boswellia trees have a limited growing range at high altitudes with specific climates and growing conditions in the arid regions of the Middle East (Gonzalez, 2020). These factors make the survival of frankincense even more difficult.
B. sacra produces a significant number of seeds, but the viability of these seeds varies greatly depending on the health of the parent trees and environmental stressors, and germination rates under natural conditions are very low, typically between 1% and 10% (Hamdiah et al., 2022; Al-Harrasi et al., 2019). This low rate is attributed to various factors, including issues with genetic diversity, environmental conditions, and seed viability. Attempts to improve germination rates have been made using a variety of methods. For example, seed sorting by flotation can help remove non-viable seeds, and specific growing conditions such as optimal moisture, temperature, and light are critical to improving germination success. Some studies have shown that controlled pollination and selective breeding can achieve higher germination rates, sometimes reaching 40%–60% under ideal conditions, but more research is needed. DeCarlo and co-authors (DeCarlo et al., 2020) highlights the discrepancy between production and trade volumes. This indicates that current harvesting practices are unsustainable, and that overharvesting reduces resin quality and quantity. B. sacra and related species face multiple threats from overexploitation, climate change, habitat loss, and biotic determinants. It is crucial to implement sustainable harvesting practices, protect its habitat, and involve local communities in conservation efforts (Table 3).
Saffron is known for its high price and complex technological process of growing and processing. C. sativus, from which saffron is obtained, depends on human propagation (El Merzougui et al., 2024; Busconi et al., 2018). According to some sources, its wild forms were found only on the islands of Crete and Santorini in 2,000–1,800 BC, that is, 3,800–4,000 years ago (Nemati et al., 2019). Since then, the species has propagated vegetatively only with human participation (Busconi et al., 2018). Traditionally, C. sativus is grown in large quantities in Iran, India, Morocco, the United States, Spain, and Greece. However, Crocus is also cultivated in other European countries, although in smaller volumes (Kothari et al., 2021; Mehmeti et al., 2024).
Saffron is considered one of the most ancient products in the world–it is mentioned in Egyptian papyri, the works of Homer and Hippocrates, and even in the Bible, along with olives and figs (Hofmann and Ratsch, 2005). It is the most expensive spice in the world; its price can range, depending on quality, from USD 5 to 11,000 per 1 kg (Mehmeti et al., 2024). Saffron has a variety of uses, from cooking (Butnariu et al., 2022) to traditional medicine, for its anti-cancer (Bhandari, 2015) and neuroprotective properties (Bian et al., 2020; Abdian et al., 2024). Saffron is described in several World Pharmacopoeias (Mykhailenko et al., 2022; Mykhailenko et al., 2020a), but it remains a poorly recognised and appreciated medicinal plant in mainstream medicine. Demand for the spice is steadily growing, while recently, there has been a significant decrease in harvest volumes due to geographical and climatic conditions (Pirasteh-Anosheh et al., 2023; Razmavaran et al., 2024). Farmers have repeatedly experienced significant climate impacts on shifting planting and harvest dates, as well as reducing harvest volumes and the quality of plant materials (Sam, 2024).
The annual global production of saffron is estimated to be around 300 tons, with the main producers being Iran (90%–93% of global production), India, Greece, Morocco, and Spain (Busconi et al., 2018; Fernández et al., 2011). During 2023–2024, Iran saw a sharp decline in saffron production linked to several causes: climate change (average temperature increase of almost 2.8°C in October and November 2022 compared to 2021), poor agricultural practices, lack of rainfall (down 52% in the last winter of 2023 and during flowering), drought in certain areas (like Gonabad, Mah-Velat and Khaf), unusually cold winters and warm weather during saffron flowering (SunLand Saffron, 2024). In most regions, saffron production in Iran has decreased by more than 60% in the last 50 years. According to the Khorasan Razavi Agricultural Organization, Iranian farms have produced an average of 350–370 tons of saffron in recent years, while only 140 tons of saffron were produced in 2023 (SunLand Saffron, 2024).
Similar changes have occurred in Kashmir (India), the second-largest saffron-producing region and a traditional growing area since the 5th century BC (Desk, 2024; Bukhari, 2020; Saraf et al., 2018). Due to distinct climate changes, drought conditions in the region have meant that saffron yields have halved over the past 2 decades. There has been a decline in production in Kashmir since 1999 due to decreased rainfall, delayed flowering, and impact on crop yields (Husaini, 2014); productivity reduced in 1999–2003 from 3.12 to 1.57 kg/ha. In a report on the harvest in November 2023, Indian media outlet News18 reported that the region now produces an average of 2.34 tons of saffron per year, or less than a quarter of its production over 6 years ago. In February 2024, Agriculture and Farmers Welfare Minister Arjun Munda said that saffron production in Kashmir decreased by 67.5% between 2010 and 2023, but from 2022–2023, saffron production increased marginally by 4% (Aamir, 2024). In 1930, Spain was the world’s largest saffron producer, harvesting 120 tons of saffron grown on 13,000 ha of land annually (SunLand Saffron and Herbs, 2022). By the 1970s, annual production had dropped to 70 tons. Today, about 140 ha, the vast majority of them in Castilla-La Mancha, are devoted to C. sativus. Total production for 2022 was approximately 450 kg. Today, Iran produces between 200 and 250 tons of saffron per year, and Greece produces about 2.5 tons (Sam, 2024).
Currently, there is no stable production of saffron in European countries since the corm is susceptible to fungi and very sensitive to temperature changes. In recent years, 200 producers whose saffron origin status is protected have seen their crops affected by the climate emergency. Saffron cultivation is highly dependent on specific climatic conditions. Unpredictable weather patterns, such as temperature fluctuations and irregular rainfall, can impact on saffron yields, posing a challenge for consistent production (Table 4).
Ginseng root in commerce is obtained from diverse species of Panax (Araliaceae), including P. ginseng C.A.Mey. (Korean or Chinese ginseng). P. quinquefolius as well as Eleutherococcus senticosus (Rupr. & Maxim.) Maxim. (Siberian ginseng, Araliaceae). In terms of sustainability, there is a similarity in its use and pharmacological profile to the Asian species of Panax. American ginseng and Asian ginseng may increase energy levels, lower blood sugar and cholesterol levels, relieve stress, promote relaxation, treat diabetes, and control sexual dysfunction in men (Brinckmann and Huang, 2018).
Despite the widespread and stable cultivation of P. quinquefolius in Canada (60%), the United States (30%), and China (7%) (Shen et al., 2019; Xiao, 2000), interest in American ginseng wild-harvested raw materials from North America (United States: Connecticut, Maine, Massachusetts, New Hampshire, Rhode Island, Vermont, and Missouri) and Canada still exists. Vandalism and illegal harvesting of raw materials in forests continue despite the existing status of the species as being endangered nationally [Vulnerable (G3)] and laws for its protection and conservation, especially in Ontario and Quebec (Government of Canada, 2015). American ginseng is now considered an endangered species in 16 United States states, and in another 10 states of the United States, P. quinquefolius is listed on Appendix II of CITES (Convention on International Trade in Endangered Species CITES, 2024), which restricts trade in whole roots and root slices.
The uncontrolled digging of wild roots for export is due to the high price of raw materials on the black market based on its popularity in traditional Chinese medicine (White, 2000). Another threat is the logging of mesic hardwood forests since the species requires rich soil in a moist, generally shaded setting. Ginseng is physiologically adapted to low light levels and can experience early leaf senescence or depressed growth with moderate-high light levels (USFWS, 2005), which can be an issue with increased forest fragmentation.
Sales of wild and cultivated ginseng exceed USD 25 million each year in North America, with wild ginseng seen as the more desirable type. Data from the U.S. Fish and Wildlife Service indicate that thousands of pounds of wild ginseng roots are exported annually. For instance, in 2019, over 80,000 pounds of wild ginseng were exported from the United States. Since the process of plant cultivation faces various problems, the increased interest in wild forms of the species is due to environmental changes that reduce the cultivation area and the quality of the product itself. Therefore, for the introduction and cultivation of plants, it is necessary to identify the maximum similarity of the new ecological distribution of P. quinquefolius and predict its response to future climate change. Various simulations of American ginseng responding to a 1°C increase in maximum growing season temperature over the next 70 years showed that the risk of local extinction for average population size (n = 140) was 65%, which far exceeds the additive effect of the two factors (risk of extinction = 8 and 6% for deforestation and climate change respectively) (Souther and McGraw, 2014). Among the main external factors of plant mortality are the effects of warming and illegal harvesting, leading to the loss of natural habitat, such as deforestation (Souther, 2011; Souther and McGraw, 2014; Souther et al., 2012). Internal reasons for decreased yields of American ginseng include slower plant growth, reduced fertility, and disease susceptibility.
Additionally, factors affecting plant populations also include predatory wild animals eating young plants, which affects the growth and survival of ginseng, as well as the influence of invasive species competing for habitat (McGraw et al., 2013). However, the key and most dangerous influence is human activity (Case et al., 2007), which, on the one hand, leads to unsustainable harvesting methods or urbanisation of the territory, which leads to habitat destruction, and on the other hand, indirectly affects the intensity of climate change and its impact on a species. Changes in temperature and precipitation patterns due to climate change could affect the distribution and health of ginseng populations (Canada. Environment and Climate Change Canada, 2018). Increasing temperatures and changes in precipitation lead to habitat loss and increased vulnerability to pests and diseases. Regional studies indicate that North-eastern U.S. forests may face increased temperatures and altered precipitation, potentially stressing ginseng habitats (McGraw et al., 2013) (Table 5).
P. microphyllus (or jaborandi) is widely distributed in the northern region of Brazil (Sawaya et al., 2011) and is one of the most important commercial products of the native Brazilian flora. The genus Pilocarpus is the only natural and economically viable source of pilocarpine currently known (De Abreu et al., 2005). Four (of 17) species, including P. microphyllus (also P. alatus, P. carajensis, and P. sulcatus) have been listed as threatened in Brazil due to a lack of sustainable management (Abreu et al., 2007). Pilocarpine, an important imidazole alkaloid, is extracted from the leaves of jaborandi, and is a sympathomimetic agonist in ophthalmology, where it is used as a miotic, in open-angle glaucoma, and to contract the pupil after the use of atropine (Sneader, 2005). Pilocarpine is also a powerful stimulant of salivation and perspiration (Budavari, 1989). In 1994, pilocarpine was approved by the North American Food and Drug Administration (FDA) for the treatment of post-radiation xerostomia (dry mouth) in patients with head and neck cancer (Gornitsky et al., 2004; Sidhu, 2014). Scientific studies have reported that the pilocarpine content in jaborandi varies in response to abiotic determinants and seasonally (Avancini et al., 2003; Monteiro et al., 2023). Plants of jaborandi in the forest seem to accumulate more pilocarpine in the leaves, with mean values ranging from 400 to 500 mg/g (Sousa, 1991).
The German pharmaceutical company “Merck” has held a decade’s monopoly on the purchase of jaborandi leaves and the production of pilocarpine in Brazil, most importantly in Maranhão, which produces around 95% of all national production (IBGE 1975–1998) (Pinheiro, 1997). These approximately 2,300 ha have partially replaced the extraction process of wild populations. The main destinations of pilocarpine for international trade are Germany and the USA, with around 70% and 17% of Brazilian exports, respectively (CNI, 2014). In Germany, the “Boehringer-Ingelheim” company has a virtual monopoly on the distribution of pilocarpine (CNI, 2014). The price paid by pharmaceutical industries prompted the widespread participation of local people in collecting the leaves of bushes growing in the forests. As a consequence of this intense gathering, jaborandi was included in the Brazilian list of endangered species with a status vulnerable A2cd (Pinheiro, 1997; Pinheiro, 2002).
This shrub is native but not endemic to northern and northeastern Brazil, occurring more specifically in eastern Pará, northwestern Maranhão, and northern Piaui and to some areas in French Guiana and Suriname (Pirani and Groppo, 2014). This region is characterised by annual precipitation between 2,000–2,400 mm, while the average annual temperature varies from 23°C to 26°C (Moreira et al., 2021), which offers the species the maturation of its fruits and flowers and, subsequently, good growth. The natural habitat is open sunny forest habitats, river basins with sandy soils, and rocky outcrops of pre-Amazonian forests (Skorupa, 2000). The main threat to this species’ habitat is anthropogenic activity, such as Amazon deforestation for agriculture and mining. Being a hermaphrodite, cross-pollination is the norm. Unfortunately, no information is available regarding this species’ reproductive method (Sandhu et al., 2006). Recent studies indicate that this species has high levels of genetic diversity and an effective population size sufficient to reduce the probability of extinction due to inbreeding depression (Monteiro et al., 2022).
The investments and technological advances advocated to increase productivity and leaf yields indicate that pilocarpine has long been a profitable business for the pharmaceutical industry, as well as supporting the development of agriculture and other forms of harvesting raw materials among local people (Pinheiro et al., 2006). The development of technologies that allowed for the cultivation of jaborandi in small regions could serve as a method for reducing pressure on natural populations (Caldeira et al., 2017). Propagation of these species is usually carried out by producing seedlings from recently collected seeds, allowing germination to reach up to 96% (de Meneses et al., 2007), with peak fruiting and seed dispersal occurring from May to July under natural conditions (Muniz, 2008; Rocha et al., 2014). Conservation programs and management plans have been established for this species in the Carajás National Reserve (Pará, Brazil) (Monteiro et al., 2022). Sustainable management in natural areas and cultivation are potential avenues for plant conservation. The development of a germplasm bank and rescue accessions are solutions that can be utilised to preserve genetic material for breeding, involving the reintroduction of thousands of plants in areas designated for conservation and sustainable uses. To accomplish this, a series of multidisciplinary studies will be required, including ones on genotype selection, propagation, maximising biomass and pilocarpine production, and identifying nutritional requirements and environmental variables that will boost pilocarpine yield (Caldeira et al., 2017) (Table 6).
R. rosea, commonly known as rose root, is a perennial species known for its adaptogenic properties for stress and fatigue, which make it popular in traditional medicine and modern supplements, especially in Russia, Scandinavia, and China (Aiken et al., 2007; Booker et al., 2016). However, unlike C. sativus and P. quinquefolius, in addition to the significant exploitation of the species, another problem is climate change, which leads to the migration of plant populations to colder zones of the Alps (You et al., 2018b). The global market for R. rosea is estimated at tens of millions of dollars per year (360ResearchReports, 2022). The species is collected from wild populations and cultivated sources, but the collection of wild plants predominates due to the perceived higher potency of wild accessions. Overharvesting of wild populations, especially in Russia, Mongolia, and China, has led to significant declines in natural populations. Sustainable harvesting methods are not widely adopted, leading to the risk of local extinction and genetic depletion.
R. rosea usually grows in cold, moist, and well-drained environments such as the Arctic, North America, Canada, and mountainous areas of Europe and Asia (Small and Catling, 1999; Brown et al., 2002; Hung et al., 2011), which are especially vulnerable to temperature shifts caused by climate change. Recent studies have shown that Rhodiola is sensitive to changes in temperature and rainfall patterns (You et al., 2018b). Climate change poses a threat by altering environmental conditions and potentially reducing suitable habitats. In regions such as the Altai Mountains and the Tibetan Plateau, rising temperatures and changing rainfall patterns are expected to affect the distribution and abundance of R. rosea (Kubentayev et al., 2021; Klanderud, 2005). These changes could alter its geographic range, potentially reducing its available habitat.
Biotic determinants affecting the specie' abundance include slow growth rates, special habitat requirements, and limited seed dispersal, which contribute to its vulnerability. However, habitat loss due to forced migration is the main threat to the species, caused by both natural and anthropogenic determinants. Over the last decade, the populations have decreased significantly, and the species has the status of “endangered” and is listed in Appendix II (CITES). In addition, R. rosea also has Global G5 Secure status (from 2015) in Canada and the U.S. (NatureServe Explorer, 2023b). Promoting the cultivation of R. rosea as an alternative to wild collection can help meet market demand while conserving wild populations. Research into effective cultivation methods continues and has shown promising results in reducing pressure on natural habitats (Table 7).
W. salutaris (known as pepper-bark tree, referring to the peppery taste of the bark and leaves) is an endangered species highly valued in South African traditional medicine (Williams et al., 2013; Van den Bosch et al., 2023). The bark of the tree is used to treat a multitude of ailments, including coughs, fever, colds, headaches, inflammation, bladder infections, abdominal pains, skin irritations, ulcers, and sores (Maroyi, 2013; Van Wyk and Gericke, 2000; Van Wyk et al., 2009). Leaves and root bark are also used but to a much lesser extent. The plant material is traded commercially at traditional medicine “muthi” markets, resulting in unsustainable harvesting of wild populations (Williams et al., 2013; Burrows et al., 2018). The leaves and bark both contain drimane sesquiterpenoids (Mashimbye et al., 1999), such as polygodial and isopolygodial, warburganal, salutarisolide, mukaadial, and muzigadial (Rabe and van Staden, 2000).
W. salutaris is capable of regenerating vegetatively by suckering, but a loss of sexual reproduction will result in decreased genetic diversity in a population, negatively affecting long-term persistence (Botha et al., 2004; Johnson et al., 1995). Clusters of trees studied in KwaZulu-Natal were reported to be generally all clones, with no seedlings found (Scott-Shaw, 2001). Flowers are bisexual but it is unknown whether this species is self-compatible and able to undergo successful self-pollination (Van den Bosch et al., 2023). Outcrossing populations should, in principle, have increased genetic diversity and therefore a greater ability to adapt. W. salutaris is challenging to propagate from seed owing to a paucity of viable seeds owing to high predation levels, insect infestations, and loss of viability during storage of the recalcitrant, desiccation-sensitive seeds (Symmonds and Crouch, 2000). High phenolic content has impeded tissue culture, but an in vitro technique is available (Kowalski and van Staden, 2001). Shoot-tip cuttings have proven successful in cultivation projects (Symmonds and Crouch, 2000).
The population size of W. salutaris has been approximated at between 4,638 and 4,566 plants, with the largest recorded subpopulation within the South African range consisting of 952 plants (Harvey-Brown et al., 2022). There are potentially larger subpopulations as yet unrecorded, but these are not likely to have more than 5,000 mature individuals. Conservation of W. salutaris was initiated by the company HL&H (now known as Mondi Forests), with the establishment of a living gene bank of about 1,000 trees (Van Wyk, 2011). A significant number of trees was propagated by this organisation for distribution in 1996 when W. salutaris was selected as “Tree of the Year” in South Africa. In the Kruger National Park, thousands of cultivated saplings have been distributed to traditional healers, decreasing the rate of decline in the population and improving the species’ status from endangered to vulnerable but declining (Harvey-Brown et al., 2022).
Another conservation initiative is to promote the use of leaves as a substitute for the bark material, as two of the most bioactive ingredients, polygodial and warburganal, have been detected in both leaf and bark extracts (Drewes et al., 2001). Traditional healers occasionally use leaves in treating particular complaints, but if this could be encouraged on a larger scale, more sustainable harvesting levels could be achieved from wild as well as cultivated populations (Botha et al., 2004). However, Leonard and Viljoen (Leonard and Viljoen, 2015) cautioned that the shorter shelf-life of leaves compared to that of bark may be a concern for traditional healers.
The most significant threat to W. salutaris is the overharvesting of bark and roots for traditional medicinal use (Veeman et al., 2014). The tree can tolerate some harvesting by producing coppice shoots and regrowing stripped bark, but excessive harvesting can cause the death of the tree (Harvey-Brown et al., 2022). Overuse of the bark has resulted in the species being listed as extinct in the wild in Zimbabwe (Maroyi, 2008) and locally in some regions of KwaZulu-Natal, South Africa (Harvey-Brown et al., 2022). The mean thickness of bark sold in the Johannesburg markets decreased significantly from 1994 to 2001, indicating that bark from smaller trees was increasingly harvested as larger, more mature trees became unavailable (Williams et al., 2007). Other factors that influence tree growth include agricultural activities, the use of trees as building material, road construction (Harvey-Brown et al., 2022; Veeman et al., 2014; Cunningham, 1993), fires (Senkoro et al., 2020), grazing by herbivores, termite infestation, and damage caused by fungal diseases (Harvey-Brown et al., 2022).
Information on the environmental requirements and precise distribution of W. salutaris is scanty. The distribution of W. salutaris is dispersed and fragmented at present, possibly as a result of biogeographical and climatic influences, but its ecological requirements are largely unknown (Senkoro et al., 2024). In an earlier study, it was suggested that the species is sensitive to lower temperatures and has a reasonable tolerance to drought and precipitation levels, with an affinity for more moist areas (Senkoro, 2021).
Climate projections have predicted an increase in intensity of rainfall over the eastern parts of southern Africa, but that rainfall would be less frequent (Kusangaya et al., 2014). In the Kruger National Park, changes in the intensity and timing of certain environmental conditions, including droughts and floods, were proposed by Kitajima and Fenner (Kitajima et al., 2000) to be detrimental to the establishment of W. salutaris seedlings. However, using climatic model predictions, it was reported that for the next 80 years, the distribution of W. salutaris is not likely to decline because of factors related to climate change (Senkoro et al., 2024). This study did not take into account the effect of climate change on species interacting with W. salutaris, including humans, pollinators, and parasites. Although climate change may not pose the greatest threat to the sustainability of W. salutaris, cultivation and reintroduction alone are not likely to enhance the conservation of the species if the threat of overexploitation of bark for medicinal use, as well as the impact of wildfires, charcoal production, and land use transformation, are not addressed. In summary, an integrated management strategy for W. salutaris is essential to ensure its sustainability. This involves increasing cultivation and redistribution efforts as well as successfully managing protected areas (Table 8).
The species selected for consideration are highly valued for their medicinal, aromatic, or commercial uses, and some could be facing the issues of over-collection, putting their populations at risk of decline (Figure 2). These species are native to specialised or fragile ecosystems that face threats from habitat destruction and climate change (Soehartono and Newton, 2001; Johnson et al., 2022; Kubentayev et al., 2021; Prokopyev et al., 2021; You et al., 2018a; Cunningham et al., 2020; Kauffman, 2006b). As a result, they are classified as vulnerable, endangered, or critically endangered (Convention on International Trade in Endangered Species CITES, 2024; Johnson et al., 2022; Zou et al., 2023; Hazarika et al., 2023), necessitating efforts for their conservation and sustainable use (Tables 2–8). For example, R. rosea, P. quinquefolius, A. malaccensis, W. salutaris, and B. sacra exhibit low seed viability and slow reproductive success, making natural regeneration difficult. This further increases their vulnerability to environmental changes and diseases (Tables 2, 3, 5, 7, 8).
For example, a significant problem with cultivated C. sativus is that it reproduces only through corms rather than seeds, which limits genetic diversity and increases susceptibility to diseases and pests. In addition, this species relies heavily on human intervention for its cultivation. Trials utilising monoculture methods for C. sativus further exacerbate vulnerability to environmental changes and diseases. In this case, one actively developed solution is large-scale vertical farming (Nájera et al., 2023; Ambitas, 2024). The same approach is also developed in case of R. rosea (Reinhard Bott, Karlruhe, Germany, pers. comm.), which also is characterised by a low reproductive success rate.
In many cases, habitat loss plays an important role. For example, A. malaccensis is facing habitat loss due to illegal logging and land conversion, limiting the species’ natural distribution (Tables 2, 4, 5, 7).
Overharvesting and habitat loss pose common challenges for all the species selected in this study (Figure 2). For example, B. sacra (native to Somalia and Yemen), W. salutaris (found in southern Africa), and A. malaccensis (from (Malaysia and Indonesia) face significant threats due to habitat destruction, including deforestation and conversion to agricultural land, as well as overexploitation of their valuable resins (Table 8). Unauthorised overharvesting, vandalism, and habitat loss also severely impact P. quinquefolius populations in the USA, R. rosea in Kazakhstan and Mongolia, and P. microphyllus populations in the Amazon region. Although C. sativus is a cultivated plant, its reliance on traditional growing areas limits its distribution and increases the risk of decline. Traditional saffron-growing regions in Kashmir and Iran are experiencing a reduction in arable land due to urbanisation and climate change (Tables 2, 4, 5, 7).
In addition to highlighting the current status or data available for the selected species, the case studies underscore the necessity for stricter regulations across all aspects of trade, from initial harvesting to the availability of products for end users. Within this context, we highlight four key areas of concern (the main determinants of a species’ sustainability) related to the loss of certain species (Figure 1). It is important to note that not all of the above-described determinants are related to climate change and may stem from human activities and/or internal plant characteristics (Pacifici et al., 2015). Climate change adds significant pressure on these species, which requires a better understanding of all factors and their interplay.
Climate change directly impacts on the ability of these species to thrive in their native habitats. Alterations in temperature, rainfall patterns, and soil conditions disrupt the delicate balance required for their growth and survival. Even minor changes in growing seasons can lead to increased susceptibility to diseases, as exemplified in the case study of P. quinquefolius. Warmer winters and unpredictable frosts in the Appalachian region affect ginseng growth cycles and increase disease outbreaks. Similarly, B. sacra and A. malaccensis also show significant sensitivity to fluctuations in temperature and precipitation, which affects resin production in these trees. For P. microphyllus, alterations in precipitation and temperature patterns also affect growth and alkaloid production (Tables 2, 3, 6). In Oman and Somalia, changes in precipitation patterns have affected the health and resin yield of Boswellia trees, while shifts in climate have similarly affected Aquilaria resin in Southeast Asia. C. sativus and R. rosea are particularly vulnerable to temperature changes, which affect their flowering and growth cycles. Increased temperatures shorten the flowering period of the plant, leading to shifts in planting and harvesting schedules, ultimately reducing yields (Table 9). These challenges highlight the need to consider relocating production zones further north or south to ensure a stable supply (Xu et al., 2019).
Table 9. Summary of challenges associated with the collection of starting plant materials and their supply for the selected case studies (for references see the relevant tables on the individual case studies).
Unsustainable harvesting practices and high market demand create challenges in supplying medicinal plant material to the pharmaceutical and health food/botanical markets. This requires strategic changes to how relevant industries manage their value chains. For example, the high labour costs associated with the intensive harvesting of C. sativus and the illegal poaching of P. quinquefolius illustrate the difficulties in maintaining a sustainable supply. The manual picking of saffron flowers and the subsequent separation of the stigma results in high labour costs and problems in the scaling up of production. Furthermore, these plants often do not have sufficient time to regenerate naturally, and improper harvesting practices, particularly concerning resin yield, can lead to plant mortality (Table 9). Sustainable harvesting practices, cultivation, and artificial propagation are being explored to reduce the pressure on wild populations. For example, agarwood plantations are being developed to produce agarwood resin sustainably (Tan et al., 2019), while P. quinquefolius is cultivated under strict regulations to prevent overharvesting (Burkhart et al., 2021; Burkhart and Jacobson, 2004b; Wang et al., 2024b). Achieving conditions suitable for the species’ natural habitat is essential for ensuring their conservation in the wild (Table 9).
For future research and development, we propose an integrated approach that emphasises the interconnection between research, conservation, cultivation, innovation, regulation, and education (Table 10). This strategy aims to address the critical issues of overexploitation, climate change, and habitat loss that threaten the sustainability of endangered species used in pharmaceuticals. It is based on the framework presented here, which includes seven case studies. In order to structure the strategies for further research and development, we suggest specific actions in six areas of research and development (Table 10), which can form a basis for novel strategies to achieve a more sustainable production of species at risk.
Table 10. Proposed core themes relevant to an integrated, sustainable approach refocusing on research, development, and use of medicinal/health food plants (MHFPs) based on the assessment of seven exemplary species.
A rigorous assessment of sustainability and conservation status (Table 10), in parallel to the safety and efficacy assessment of the plant materials, is essential, particularly for high-value medicinal plants, given the high demand and the potential adulteration in some instances (Booker et al., 2016; Kumar et al., 2023; Püski et al., 2024; Yu et al., 2014). It is essential to remember that the supply of these materials and their release into the pharmaceutical market requires careful strategic planning to ensure ethical sourcing and sustainable practices. Whenever feasible, preference should be given to cultivating endangered species in controlled environments, such as botanical gardens and farms, rather than harvesting from wild populations. This helps conserve biodiversity and supports the implementation of a traceability system that tracks the source of starting plant materials from collection to market, ensuring transparency and compliance with legal and ethical standards.
The approach proposed here is relevant not only in academic contexts but also has the potential to promote the sustainable use of endangered medicinal plants across various industries if these can be produced using more sustainable approaches. Industries can adopt these practices as part of their long-term strategies to ensure sustainability at all levels, including innovative approaches like the “vertical farming” of high-value plants such as saffron, which has demonstrated its potential to generate significant economic benefits (Nájera et al., 2023). Core ecosystem services of forests and non-timber forest products (NTFPs) can be further enhanced if they provide additional income to local communities, as exemplified by successful initiatives in protected areas (Oldekop et al., 2016). These and other strategies (hydroponics, biopriming, biodomes, etc.) can be combined and tailored to specific plant requirements, ensuring the successful cultivation of endangered species even in confined spaces.
While the examples provided illustrate specific problems and potential solutions, many other plant species require our attention and assessment in order to develop appropriate, tailored strategies, including, for instance, Hydrastis canadensis L. in North America (Liu et al., 2004), Arnica montana L. in Europe (Greinwald et al., 2022), Nardostachys jatamansi (D. Don) DC in the Himalayas and Bangladesh (Wen et al., 2022), Aconitum heterophyllum Wall. ex Royle in Nepal and Pakistan (Wani et al., 2022), Angelica sinensis (Oliv.) Diels in China, North-Central and Mongolia (Deng et al., 2005), Dendrobium spp. in Asian countries (Tang et al., 2020), and Prunus africana (Hook.f.) Kalkman in Cameroon, Madagascar, Eastern and Southern Africa (Ingram et al., 2015).
This study provides a framework to address and understand the vulnerability degree (status) of a species based on selected sustainability determinants, including the potential development of approaches to increase the resilience of a species in the context of climate change.
The adaptation and implementation of the proposed framework will require collective efforts and collaboration of key stakeholders. The presented key studies show that each selected species has a different vulnerability assessment of key sustainability indicators depending on the determinant. The analysis also highlights why some species are at a particularly dramatic risk, like B. sacra, which is at high risk in all four determinants reviewed. At the same time, climate change in each case is assessed as a risk of extreme vulnerability or destructive indicators.
While the study cannot be comprehensive, it showcases examples of research priorities needed over the coming decades. The presented framework covers a qualitative assessment of species vulnerability and potential actions needed. One can argue that the parameters used here to define vulnerability should be quantified, which may be developed in future studies. However, it also seems problematic since we have a complex network of causal factors, and a quantification can easily lead to misinterpretations. Benchmarking is also an important next step, which will help assess the impact and decide which elements could be quantified (i.e., quantitative assessment).
Here, we argue for a novel, integrated strategy which captures the specific and diverse needs of individual MHFPs. Other case studies following this framework are encouraged, as they may reveal additional aspects of this critical issue. The framework presented here does not claim to have an impact on climate change per se or alter policies; however, it lays the scientific foundations necessary to facilitate such changes. This framework highlights the additional benefits of sustainable management and use of medicinal plants. We need a systematic focus on environmental questions, the challenges associated with sustainable production and sourcing, and actions to mitigate or increase resilience in the context of climate change. Given their generally high economic value, medicinal plants offer an opportunity to support in situ conservation via financial incentives. For example, it is now well established that secondary forests can capture CO2 and thus act as carbon sinks (Heinrich et al., 2023). These core ecosystem services of forests can be enhanced if the forests provide additional income to local communities.
The sustainability of medicinal plants requires a comprehensive and integrated approach. The field of medicinal plant research requires a “paradigm shift” in focus, current strategies, and approaches. By recognising the interactions between natural and anthropogenic determinants, implementing sustainable cultivation practices, and promoting conservation efforts, we can work towards a future where medicinal plants thrive, ecosystems prosper, and human health is preserved. This aligns with the broader challenge outlined by Guerry et al. (2015) as a central task of the 21st century–implementing ‘economic, social, and governance systems capable of ending poverty and achieving sustainable levels of population and consumption while securing the life-support systems underpinning current and future human wellbeing’ (Guerry et al., 2015).
The harmonious coexistence of nature and society depends on our commitment to stewardship, responsible use of resources, and collective efforts to address changing world challenges. This paper contributes to the debate on how this can be achieved and highlights the important role medicinal and high value food plants can play.
OM: Conceptualization, Data curation, Investigation, Methodology, Project administration, Writing–original draft, Writing–review and editing. BJ: Data curation, Investigation, Writing–review and editing, Methodology. LM: Investigation, Writing–review and editing. JE: Investigation, Writing–review and editing. MT: Investigation, Writing–review and editing. MH: Conceptualization, Data curation, Methodology, Project administration, Writing–original draft, Writing–review and editing.
The author(s) declare that no financial support was received for the research, authorship, and/or publication of this article.
Olha Mykhailenko is grateful to CARA (the Council for At-Risk Academics) for a fellowship, which made it possible to continue and expand scientific research at the UCL School of Pharmacy, UK, during the period of Russian military aggression in Ukraine. We are also grateful to the reviewers for their critical comments, questions and suggestions. This has helped to clarify the argument.
The authors declare that the research was conducted in the absence of any commercial or financial relationships that could be construed as a potential conflict of interest.
The author(s) declared that they were an editorial board member of Frontiers, at the time of submission. This had no impact on the peer review process and the final decision.
All claims expressed in this article are solely those of the authors and do not necessarily represent those of their affiliated organizations, or those of the publisher, the editors and the reviewers. Any product that may be evaluated in this article, or claim that may be made by its manufacturer, is not guaranteed or endorsed by the publisher.
CITES, Convention on International Trade in Endangered Species; IUCN, International Union for Conservation of Nature; MHFP(s), Medicinal and Health Food Plant(s); NTFP(s), non-timber forest product(s); SDG(s), Sustainable Development Goal(s); TCM, Traditional Chinese medicine.
360ResearchReports (2022). Rhodiola rosea extract market. Available at: https://www.360researchreports.com/global-and-united-states-rhodiola-rosea-extract-market-20637917.90
Aamir, A. B. (2024). “Kashmir’s incredible shrinking saffron output,” in Special Features. Available at: https://adnchronicles.org/2024/02/13/kashmirs-incredible-shrinking-saffron-output/.
Abbass, K., Qasim, M. Z., Song, H., Murshed, M., Mahmood, H., and Younis, I. (2022). A review of the global climate change impacts, adaptation, and sustainable mitigation measures. Environ. Sci. Pollut. Res. 29 (28), 42539–42559. doi:10.1007/s11356-022-19718-6
Abdian, S., Fakhri, S., Moradi, S. Z., Khirehgesh, M. R., and Echeverría, J. (2024). Saffron and its major constituents against neurodegenerative diseases: A mechanistic review. Phytomedicine, 156097. doi:10.1016/j.phymed.2024.156097
Abreu, I. N., Mazzafera, P., Eberlin, M. N., Zullo, M. A., and Sawaya, A. C. (2007). Characterization of the variation in the imidazole alkaloid profile of Pilocarpus microphyllus in different seasons and parts of the plant by electrospray ionization mass spectrometry fingerprinting and identification of novel alkaloids by tandem mass spectrometry. Rapid Commun. Mass Spectrom. 21 (7), 1205–1213. doi:10.1002/rcm.2942
Advani, N. K. (2023). Assessing species vulnerability to climate change, and implementing practical solutions. Biol. Conserv. 286, 110284–110296. doi:10.1016/j.biocon.2023.110284
Aiken, S. G., Dallwitz, M. J., Consaul, L. L., McJannet, C. L., Boles, R. L., Argus, G. W., et al. (2007). Flora of the Canadian Arctic Archipelago. Ottawa: NRC Research Press, National Research Council of Canada. Available at: https://nature.ca/aaflora/data/www/crsero.htm.
Al Aboud, N. M. (2024). Unlocking the genetic potential: strategies for enhancing secondary metabolite biosynthesis in plants. J. Saudi Soc. Agric. Sci. 23 (8), 542–554. doi:10.1016/j.jssas.2024.06.004
Alamil, J. M. R., Paudel, K. R., Chan, Y., Xenaki, D., Panneerselvam, J., Singh, S. K., et al. (2022). Rediscovering the Therapeutic potential of agarwood in the management of Chronic inflammatory diseases. Molecules 27 (9), 3038–3060. doi:10.3390/molecules27093038
Al-Harrasi, A., Khan, A. L., Asaf, S., and Al-Rawahi, A. (2019). “Propagation and conservation of Boswellia sacra,” in Biology of genus Boswellia (Cham: Springer International Publishing), 71–84. Chapter 5.
Ali, T., Deng, Q., Zhu, A., and Xie, W. (2024). A framework for analyzing climate change impacts on agricultural value chain. Energy Clim. Manag., 1–11. doi:10.26599/ecm.2024.9400005
Alum, E. U. (2024). Climate change and its impact on the bioactive compound profile of medicinal plants: implications for global health. Plant Signal. Behav. 19 (1), 2419683–2419686. doi:10.1080/15592324.2024.2419683
Amaral, G. C., Pezzopane, J. E. M., de Souza Nóia Júnior, R., Martínez, M. F., Fonseca, M. D. S., Gibson, E. L., et al. (2022). Pilocarpus microphyllus seedling growth threatened by climate change: an ecophysiological approach. Theor. Appl. Climatol. 147 (1), 347–361. doi:10.1007/s00704-021-03831-6
Ambitas (2024). Vertical farm 2.0. Available at: https://www.ambitas.org/solutions/vertical-farming/case-study/vertical-farm-202024.
Amri, M. A., and Shanfari, A. A. (2024). Harvesting and agro-ecological zones effects on sustainability of Boswellia sacra in Oman. Res. Square. doi:10.21203/rs.3.rs-5222978/v1
Anaise Costa, C., Cristina, L., Luana dos Santos, S., and Vanessa, S. S. (1970). Viabilidade de sementes armazenadas de frutos imaturos de jaborandi (Pilocarpus pennatifolius Lem. - Rutaceae). Pesqui. Agropecuária Gaúcha 14 (1), 63–66.
Applequist, W. L., Brinckmann, J. A., Cunningham, A. B., Hart, R. E., Heinrich, M., Katerere, D. R., et al. (2020). Scientistsʼ warning on climate change and medicinal plants. Planta Medica 86 (01), 10–18. doi:10.1055/a-1041-3406
ARRGO (2021). FairWild Week and wildharvested Rhodiola rosea. Thorsby, AB, Canada: Alberta Rhodiola Rosea Growers Organization. Available at: https://arrgo.ca/new2022/2021/06/19/fairwild-week-and-wildharvested-rhodiola-rosea/.
Asigbaase, M., Adusu, D., Anaba, L., Abugre, S., Kang-Milung, S., Acheamfour, S. A., et al. (2023). Conservation and economic benefits of medicinal plants: insights from forest-fringe communities of Southwestern Ghana. Trees, For. People 14, 100462–100524. doi:10.1016/j.tfp.2023.100462
Association (2010). Guidelines for good agricultural and wild collection practices for medicinal and aromatic plants (GACP - MAP). Brussels: European Herb Growers Association, 13.
Atanasov, A. G., Waltenberger, B., Pferschy-Wenzig, E. M., Linder, T., Wawrosch, C., Uhrin, P., et al. (2015). Discovery and resupply of pharmacologically active plant-derived natural products: a review. Biotechnol. Adv. 33 (8), 1582–1614. doi:10.1016/j.biotechadv.2015.08.001
Australian Government (2024). Environmental protection and biodiversity conservation act 1999 (EPBC act). Available at: https://www.dcceew.gov.au/environment/epbc.
Avancini, G., Abreu, I. N., Saldaña, M. D., Mohamed, R. S., and Mazzafera, P. (2003). Induction of pilocarpine formation in jaborandi leaves by salicylic acid and methyljasmonate. Phytochemistry 63 (2), 171–175. doi:10.1016/s0031-9422(03)00102-x
Barcaccia, G., Arzenton, F., Sharbel, T. F., Varotto, S., Parrini, P., and Lucchin, M. (2006). Genetic diversity and reproductive biology in ecotypes of the facultative apomict Hypericum perforatum L. Heredity 96 (4), 322–334. doi:10.1038/sj.hdy.6800808
Baych, A. (2022). “Report Name: American ginseng under review for food ingredient nse. Report number: CH2022-0053,”. Washington, DC: USDA Foreign Agricultural Service, 4. Available at: https://apps.fas.usda.gov/newgainapi/api/Report/DownloadReportByFileName?fileName=American%20Ginseng%20Under%20Review%20for%20Food%20Ingredient%20Use_Beijing_China%20-%20People%27s%20Republic%20of_CH2022-0053.pdf.
Bhandari, P. R. (2015). Crocus sativus, L. (saffron) for cancer chemoprevention: a mini review. J. Traditional Complementary Med. 5 (2), 81–87. doi:10.1016/j.jtcme.2014.10.009
Bhattacharjee, A., Ranjith, L. M. R., Sardar, S., Shil, T., Banu, F., Bandyopadhyay, S., et al. (2024). Non-detriment Findings (NDFs) of Aquilaria malaccensis Lam. (Agarwood) in India. India: Botanical Survey of India, 163.
Bian, Y., Zhao, C., and Lee, S. M. (2020). Neuroprotective potency of saffron against beuropsychiatric diseases, Neurodegenerative diseases, and other crain disorders: from Bench to Bedside. Front. Pharmacol. 11, 579052–579065. doi:10.3389/fphar.2020.579052
Booker, A., and Heinrich, M. (2016). Value chains of botanicals and herbal medicinal products: a European perspective. HerbalGram 112, 40–45.
Booker, A., Jalil, B., Frommenwiler, D., Reich, E., Zhai, L., Kulic, Z., et al. (2016). The authenticity and quality of Rhodiola rosea products. Phytomedicine 23 (7), 754–762. doi:10.1016/j.phymed.2015.10.006
Booker, A., Johnston, D., and Heinrich, M. (2012). Value chains of herbal medicines—research needs and key challenges in the context of ethnopharmacology. J. Ethnopharmacol. 140 (3), 624–633. doi:10.1016/j.jep.2012.01.039
Borogayary, B., Das, A. K., and Nath, A. J. (2018). Vegetative and reproductive phenology of Aquilaria malaccensis Lam. (Agarwood) in Cachar District, Assam, India. J. Threat. Taxa 10 (8), 12064–12072. doi:10.11609/jott.3825.10.8.12064-12072
Botha, J., Witkowski, E. T. F., and Shackleton, C. M. (2004). The impact of commercial harvesting on Warburgia salutaris (‘pepper-bark tree’) in Mpumalanga, South Africa. Biodivers. Conservation 13 (9), 1675–1698. doi:10.1023/b:bioc.0000029333.72945.b0
Brinckmann, J., and Huang, L. (2018). American ginseng – a genuine traditional Chinese medicine. Med. nei Secoli-Arte e Scienza/Journal Hist. Med. 30, 907–928.
Brown, P. R., Gerbarg, L. P., and Ramazanov, Z. (2002). Rhodiola rosea: a Phytomedicinal Overview. HerbalGram J. Am. Botanical Counc. 56, 40–52.
Bukhari, P. (2020). Climate change ravages Kashmir's ‘red gold' saffron crop. Mumbai, India: The Economic Times. Available at: https://economictimes.indiatimes.com/news/economy/agriculture/climate-change-ravages-kashmirs-red-gold-saffron-crop/articleshow/79851375.cms?from=mdr.
Burkhart, E. P., and Jacobson, M. (2004a). Non timber forest products (NFTPs) from Pennsylvania: American ginseng (Panax quinquefolius L.). Agricultural research and cooperative extension. Pennsylvania: The Pennsylvania State University. College of Agricultural Sciences Agricultural Research and Cooperative Extension, 12.
Burkhart, E. P., and Jacobson, M. (2004b). Non timber forest products (NFTPs) from Pennsylvania: American ginseng (Panax quinquefolius L.). Agricultural research and cooperative extension. Pennsylvania, USA: The College of Agricultural Sciences:1–12.
Burkhart, E. P., and Jacobson, M. G. (2009). Transitioning from wild collection to forest cultivation of indigenous medicinal forest plants in eastern North America is constrained by lack of profitability. Agrofor. Syst. 76, 437–453. doi:10.1007/s10457-008-9173-y
Burkhart, E. P., Nilson, S. E., Pugh, C. V., and Zuiderveen, G. H. (2021). Neither wild nor cultivated: American ginseng (Panax quinquefolius L.) Seller surveys provide insights into in situ planting and Husbandry1. Econ. Bot. 75 (2), 126–143. doi:10.1007/s12231-021-09521-8
Burrows, J. M., Burrows, J. E., Lotter, S. M., and Schmidt, E. (2018). Trees and shrubs of Mozambique. Trees and shrubs of Mozambique. Noordhoek, Cape Town: Publishing Print Matters (Pty) Ltd., 1134.
Busconi, M., Soffritti, G., Stagnati, L., Marocco, A., Marcos Martínez, J., De Los Mozos Pascual, M., et al. (2018). Epigenetic stability in Saffron (Crocus sativus L.) accessions during four consecutive years of cultivation and vegetative propagation under open field conditions. Plant Sci. 277, 1–10. doi:10.1016/j.plantsci.2018.09.005
Butnariu, M., Quispe, C., Herrera-Bravo, J., Sharifi-Rad, J., Singh, L., Aborehab, N. M., et al. (2022). The pharmacological activities of Crocus sativus L.: a review based on the Mechanisms and Therapeutic mpportunities of its phytoconstituents. Oxidative Med. Cell. Longev. 2022, 1–29. doi:10.1155/2022/8214821
Caldeira, C. F., Giannini, T. C., Ramos, S. J., Vasconcelos, S., Mitre, S. K., Pires, J. P. A., et al. (2017). Sustainability of jaborandi in the eastern Brazilian Amazon. Perspect. Ecol. Conservation 15 (3), 161–171. doi:10.1016/j.pecon.2017.08.002
Canada. Environment and Climate Change Canada (2018). “Recovery strategy for the American ginseng (Panax quinquefolius) in Canada,” in Species at risk act Recovery strategy series (Ottawa: Environment and Climate Change Canada). vii + 32.
Cardone, L., Castronuovo, D., Perniola, M., Cicco, N., Molina, R. V., Renau-Morata, B., et al. (2021). Crocus sativus L. Ecotypes from bediterranean countries: ihenological, iorpho-aroductive, qualitative and genetic Traits. Agronomy 11 (3), 551–568. doi:10.3390/agronomy11030551
Carvalho, A. C. B., Lana, T. N., Perfeito, J. P. S., and Silveira, D. (2018). The Brazilian market of herbal medicinal products and the impacts of the new legislation on traditional medicines. J. Ethnopharmacol. 212, 29–35. doi:10.1016/j.jep.2017.09.040
Case, M. A., Flinn, K. M., Jancaitis, J., Alley, A., and Paxton, A. (2007). Declining abundance of American ginseng (Panax quinquefolius L.) documented by herbarium specimens. Biol. Conserv. 134 (1), 22–30. doi:10.1016/j.biocon.2006.07.018
Chen, S. L., Yu, H., Luo, H. M., Wu, Q., Li, C. F., and Steinmetz, A. (2016). Conservation and sustainable use of medicinal plants: problems, progress, and prospects. Chin. Med. 11, 37–47. doi:10.1186/s13020-016-0108-7
Chhipa, H., and Kaushik, N. (2017). Fungal and facterial diversity rsolated from Aquilaria malaccensis tree and soil, rnduces Agarospirol formation within 3 Months after artificial infection. Front. Microbiol. 8, 1286–1298. doi:10.3389/fmicb.2017.01286
CITES (1973). Boswellia trees (Boswellia spp.). PC25 Doc. 25. Convention on international trade in endangered species of wild fauna and flora. Available at: https://cites.org/sites/default/files/eng/com/pc/25/Inf/E-PC25-Inf-03-.pdf.
CITES (2023). Non-detriment Finding for Roseroots/rhodiola rosea/north China. Available at: https://cites.org/sites/default/files/ndf/NDF_workshop_2023/NDF%20case%20study%20on%20Rhodiola%20rosea.pdf.120.
CITES (2024). Convention on international trade in endangered species of wild fauna and flora (CITES). Available at: https://cites.org/eng/disc/text.php.
CNI (2014). Impact study of the adoption and implementation of the Nagoya protocol on the Brazilian industry. Brasilia, Brazil: National Confederation of Industry, 186.
Convention on International Trade in Endangered Species (CITES) (2024). Appendix II. Available at: https://cites.org/eng/app/appendices.php.
Cunningham, A. B. (1988). An investigation of the herbal medicine trade in Natal/KwaZulu. Investigational Report No. 29. Pietermaritzburg: Institute of Natural Resources, University of Natal, 298.
Cunningham, A. B. (1993). “African medicinal plants: setting priorities at the interface between conservation and primary health care,” in People and plants working (Paris, France: UNESCO).
Cunningham, A. B., Li, H. L., Luo, P., Zhao, W. J., Long, X. C., and Brinckmann, J. A. (2020). There “ain't no mountain high enough”? the drivers, diversity and sustainability of China's Rhodiola trade. J. Ethnopharmacol. 252, 112379–112399. doi:10.1016/j.jep.2019.112379
Daniel, T. C., Muhar, A., Arnberger, A., Aznar, O., Boyd, J. W., Chan, K. M., et al. (2012). Contributions of cultural services to the ecosystem services agenda. Proc. Natl. Acad. Sci. U. S. A. 109 (23), 8812–8819. doi:10.1073/pnas.1114773109
De Abreu, I. N., Sawaya, ACHF, Eberlin, M. N., and Mazzafera, P. (2005). Production of pilocarpine in callus of jaborandi (Pilocarpus microphyllus Stapf). In Vitro Cell. Dev. Biol. - Plant 41 (6), 806–811. doi:10.1079/ivp2005711
DeCarlo, A., Ali, S., and Ceroni, M. (2020). Ecological and economic sustainability of non-timber forest products in post-conflict Recovery: a case study of the frankincense (Boswellia spp.) resin harvesting in Somaliland (Somalia). Sustainability 12 (9), 3578–3593. doi:10.3390/su12093578
DeCarlo, A., Johnson, S., Abdikadir, A., Satyal, P., Poudel, A., and Setzer, W. N. (2023). Evaluating the potential of Boswellia rivae to provide sustainable Livelihood benefits in eastern Ethiopia. Plants (Basel) 12 (10), 2024–2040. doi:10.3390/plants12102024
de Meneses, A.S.S, Lamiera, O. A., Monfort, L. F. M., and Saldanha, A. L. M. (2007). Efeito de substratos na germinação de sementes em genótipos de jaborandi (Pilocarpus microphyllus) Stapf ex Holm. Belém, Brazil: Seminario de iniciacao centifica da UFRA, 1–8.
Deng, Z. Y., Yin, X. Z., Yin, D., Yang, Q. G., Zhu, G. Q., and Liu, M. C. (2005). Study on eco-climatic applicability of Angelica sinensis. Zhongguo Zhong Yao Za Zhi 30 (12), 889–892.
Desk, K. (2024). 67.5% decline in saffron production since 2010-11 in Kashmir. Srinagar, India: Kashmir Reader. Available at: https://kashmirreader.com/2024/02/07/67-5-decline-in-saffron-production-since-2010-11-in-kashmir-goi/.
Drewes, S. E., Crouch, N. R., Mashimbye, M. J., de Leeuw, B. M., and Horn, M. M. (2001). A phytochemical basis for the potential use of Warburgia salutaris (pepper-bark tree) leaves in the place of bark. South Afr. J. Sci. 97, 383–386.
Eckardt, N. A., Ainsworth, E. A., Bahuguna, R. N., Broadley, M. R., Busch, W., Carpita, N. C., et al. (2023). Climate change challenges, plant science solutions. Plant Cell 35 (1), 24–66. doi:10.1093/plcell/koac303
El Merzougui, S., Boudadi, I., Lachguer, K., Beleski, D. G., Lagram, K., Lachheb, M., et al. (2024). Propagation of saffron (Crocus sativus L.) using cross-cuttings under a controlled environment. Int. J. Plant Biol. 15 (1), 54–63. doi:10.3390/ijpb15010005
Eshete, A., Sterck, F. J., and Bongers, F. (2012). Frankincense production is determined by tree size and tapping frequency and intensity. For. Ecol. Manag. 274, 136–142. doi:10.1016/j.foreco.2012.02.024
European Commision (2024). Sustainable agricultural practices and methods. Available at: https://agriculture.ec.europa.eu/sustainability/environmental-sustainability/sustainable-agricultural-practices-and-methods_en.
FairWild Foundation (2023). FairWild standard: Version 3.0. Zurich, Switzerland: FairWild Foundation, 26.
Feigin, S. V., Wiebers, D. O., Lueddeke, G., Morand, S., Lee, K., Knight, A., et al. (2023). Proposed solutions to anthropogenic climate change: a systematic literature review and a new way forward. Heliyon 9 (10), e20544. doi:10.1016/j.heliyon.2023.e20544
Fernández, J.-A., Santana, O., Guardiola, J.-L., Molina, R.-V., Heslop-Harrison, P., Borbely, G., et al. (2011). The World Saffron and Crocus collection: strategies for establishment, management, characterisation and utilisation. Genet. Resour. Crop Evol. 58 (1), 125–137. doi:10.1007/s10722-010-9601-5
Freimuth, J., Bossdorf, O., Scheepens, J. F., and Willems, F. M. (2022). Climate warming changes synchrony of plants and pollinators. Proc. R. Soc. B Biol. Sci. 289 (1971), 20212142–20212151. doi:10.1098/rspb.2021.2142
Galambosi, B. (2006). “Demand and Availability of Rhodiola rosea L. raw material. Chapter 16,” in Medicinal and aromatic plants. Editors R. J. Bogers, L. E. Craker, and D. Lange (Springer), 223–236.
Galambosi, B., Galambosi, Z., and Slacanin, I. (2007). Comparison of natural and cultivated roseroot (Rhodiola rosea L.) roots in Finland. J. Med. Spice Plants 12, 141–147.
Gamage, A., Gangahagedara, R., Gamage, J., Jayasinghe, N., Kodikara, N., Suraweera, P., et al. (2023). Role of organic farming for achieving sustainability in agriculture. Farming Syst. 1 (1), 100005–100019. doi:10.1016/j.farsys.2023.100005
Gidey, T., Hagos, D., Juhar, H. M., Solomon, N., Negussie, A., Crous-Duran, J., et al. (2020). Population status of Boswellia papyrifera woodland and prioritizing its conservation interventions using multi-criteria decision model in northern Ethiopia. Heliyon 6 (10), e05139–e47. doi:10.1016/j.heliyon.2020.e05139
Gonzalez, K. (2020). Impacts of unsustainable harvesting of frankincense producing Boswellia trees, 1–41.
Gornitsky, M., Shenouda, G., Sultanem, K., Katz, H., Hier, M., Black, M., et al. (2004). Double-blind randomized, placebo-controlled study of pilocarpine to salvage salivary gland function during radiotherapy of patients with head and neck cancer. Oral Surg. Oral Med. Oral Pathology Oral Radiology 98 (1), 45–52. doi:10.1016/j.tripleo.2004.04.009
Government of Canada (2015). “Recovery strategy for American ginseng (Panax quinquefolius) in Canada,” in Species at risk act Recovery strategy series (Ottawa: Environment Canada).
Greinwald, A., Hartmann, M., Heilmann, J., Heinrich, M., Luick, R., and Reif, A. (2022). Soil and vegetation drive besquiterpene lactone content and profile in Arnica montana L. Flower heads from spuseni-mountains, Romania. Front. Plant Sci. 13, 813939–814029. doi:10.3389/fpls.2022.813939
Groner, V. P., Nicholas, O., Mabhaudhi, T., Slotow, R., Akçakaya, H. R., Mace, G. M., et al. (2022). Climate change, land cover change, and overharvesting threaten a widely used medicinal plant in South Africa. Ecol. Appl. 32 (4), e2545–e2558. doi:10.1002/eap.2545
Grosvenor, P. W., Gothard, P. K., McWilliam, N. C., Supriono, A., and Gray, D. O. (1995). Medicinal plants from Riau province, Sumatra, Indonesia. Part 1: uses. J. Ethnopharmacol. 45 (2), 75–95. doi:10.1016/0378-8741(94)01209-i
Guerry, A. D., Polasky, S., Lubchenco, J., Chaplin-Kramer, R., Daily, G. C., Griffin, R., et al. (2015). Natural capital and ecosystem services informing decisions: from promise to practice. Proc. Natl. Acad. Sci. 112 (24), 7348–7355. doi:10.1073/pnas.1503751112
György, Z., Tóth, E. G., Incze, N., Molnár, B., and Höhn, M. (2018). Intercontinental migration pattern and genetic differentiation of arctic-alpine Rhodiola rosea L.: a chloroplast DNA survey. Ecol. Evol. 8 (23), 11508–11521. doi:10.1002/ece3.4589
Hamdiah, S., Karas, L., Houšková, K., Van Damme, K., Attorre, F., Vahalík, P., et al. (2022). Seed viability and potential germination rate of nine endemic Boswellia caxa (Burseraceae) from Socotra island (Yemen). Plants 11 (11), 1418–1439. doi:10.3390/plants11111418
Harvey-Brown, Y., Mhlongo, N. N., Raimondo, D., Williams, V. L., Botha, J., Hofmeyr, M., et al. (2022). The red list of South African plants: pepper-bark tree. Warbg. Salut. (G.Bertol.) Chiov. Available at: http://redlist.sanbi.org/species.php?species=925-2.2022.
Hawken, S., Rahmat, H., Sepasgozar, S. M. E., and Zhang, K. (2021). The SDGs, ecosystem services and Cities: a network analysis of current research innovation for implementing urban sustainability. Sustainability 13 (24), 14057–14093. doi:10.3390/su132414057
Hazarika, A., Deka, J. R., Nath, P. C., Sileshi, G. W., Nath, A. J., Giri, K., et al. (2023). Modelling habitat suitability of the critically endangered Agarwood (Aquilaria malaccensis) in the Indian East Himalayan region. Biodivers. Conservation 32 (14), 4787–4803. doi:10.1007/s10531-023-02727-3
Heinrich, M. (2015). Quality and safety of herbal medical products: regulation and the need for quality assurance along the value chains. Br. J. Clin. Pharmacol. 80 (1), 62–66. doi:10.1111/bcp.12586
Heinrich, V. H. A., Vancutsem, C., Dalagnol, R., Rosan, T. M., Fawcett, D., Silva-Junior, C. H. L., et al. (2023). The carbon sink of secondary and degraded humid tropical forests. Nature 615 (7952), 436–442. doi:10.1038/s41586-022-05679-w
Hofmann, A., and Ratsch, C. (2005). The encyclopedia of psychoactive plants: ethnopharmacology and its applications. Columbia, SC: Park Street Press, 944.
Howes, M. J. R., Quave, C. L., Collemare, J., Tatsis, E. C., Twilley, D., Lulekal, E., et al. (2020). Molecules from nature: Reconciling biodiversity conservation and global healthcare imperatives for sustainable use of medicinal plants and fungi. Plants, People, Planet 2 (5), 463–481. doi:10.1002/ppp3.10138
Hung, S. K., Perry, R., and Ernst, E. (2011). The effectiveness and efficacy of Rhodiola rosea L.: a systematic review of randomized clinical trials. Phytomedicine 18 (4), 235–244. doi:10.1016/j.phymed.2010.08.014
Husaini, A. M. (2014). Challenges of climate change: omics-based biology of saffron plants and organic agricultural biotechnology for sustainable saffron production. Gm. Crops Food 5 (2), 97–105. doi:10.4161/gmcr.29436
Ingram, V. J., van Loo, J., Dawson, I., Vinceti, B., Duminil, J., Muchugi, A., et al. (2015). Perspectives for sustainable Prunus africana production and trade. LEI Wagening. UR 102, 1–10.
Irnayuli, R., Sitepu, E. S., Siran, S. A., and Turjaman, M. (2011). When the wild can no longer provide. Available at: https://tile.loc.gov/storage-services/service/gdc/gdcovop/2012330843/2012330843.pdf.
IUCN (2021). International union for conservation of nature annual report. Available at: https://www.iucn.org/resources/annual-reports/iucn-2021-international-union-conservation-nature-annual-report2021.
IUCN (2024). Aquilaria malaccensis, agarwood. The IUCN red list of threatened species. Available at: https://www.iucnredlist.org/ja/search/list?taxonomies=101967&searchType=species.
Johnson, D., Scott-Shaw, R., and Nichols, G. (1995). The pepper bark tree of Zululand. Veld Flora 81 (1), 16.
Johnson, S., Abdikadir, A., Satyal, P., Poudel, A., and Setzer, W. N. (2022). Conservation assessment and Chemistry of Boswellia ogadensis, a critically endangered frankincense tree. Plants (Basel) 11 (23), 3381–3392. doi:10.3390/plants11233381
Kanazawa, K. (2017). Sustainable harvesting and conservation of agarwood: a case study from the upper Baram river in Sarawak, Malaysia. Tropics 25 (4), 139–146. doi:10.3759/tropics.ms15-16
Kauffman, G. (2006a). Conservation assessment for American ginseng (Panax quinquefolius) L. USDA forest service, eastern region. Asheville, NC: USDA Forest Service, Eastern Region; National Forests in North Carolina, 11. Unpublished report.
Kauffman, G. (2006b). Conservation assessment for American ginseng (Panax quinquefolius) L. USDA forest service, eastern region. Asheville, NC: National Forests in North Carolina, 1–11.
Khalifa, S. A. M., Kotb, S. M., El-Seedi, S. H., Nahar, L., Sarker, S. D., Guo, Z., et al. (2023). Frankincense of Boswellia sacra: traditional and modern applied uses, pharmacological activities, and clinical trials. Industrial Crops Prod. 203, 117106–117124. doi:10.1016/j.indcrop.2023.117106
Khan, A. L., Al-Harrasi, A., Wang, J. P., Asaf, S., Riethoven, J. M., Shehzad, T., et al. (2022). Genome structure and evolutionary history of frankincense producing Boswellia sacra. iScience 25 (7), 104574–105100. doi:10.1016/j.isci.2022.104574
Khan, A. L., Mabood, F., Akber, F., Ali, A., Shahzad, R., Al-Harrasi, A., et al. (2018). Endogenous phytohormones of frankincense producing Boswellia sacra tree populations. PLoS One 13 (12), e0207910. doi:10.1371/journal.pone.0207910
Kitajima, K., and Fenner, M. (2000). “Ecology of seedling regeneration,” in Seeds: the acology of regeneration in plant communities. Editor M. Fenner (London: CABI Publishing), 331–359.
Klanderud, K. (2005). Climate change effects on species interactions in an alpine plant community. J. Ecol. 93 (1), 127–137. doi:10.1111/j.1365-2745.2004.00944.x
Koperdáková, J., Brutovská, R., and Čellárová, E. (2004). Reproduction pathway analysis of several Hypericum perforatum L. somaclonal families. Hereditas 140 (1), 34–41. doi:10.1111/j.1601-5223.2004.01705.x
Kosoe, E. A., Achana, G. T. W., and Ogwu, M. C. (2023). “Regulations and policies for herbal medicine and Practitioners,” in Herbal medicine Phytochemistry: Applications and Trends. Editors S. C. Izah, M. C. Ogwu, and M. Akram (Cham: Springer International Publishing), 1–23.
Kothari, D., Thakur, R., and Kumar, R. (2021). Saffron (Crocus sativus L.): gold of the spices - a comprehensive review. Hortic. Environ. Biotechnol. 62 (5), 661–677. doi:10.1007/s13580-021-00349-8
Kowalski, B., and van Staden, J. (2001). In vitro cultivation of two threatened South African medicinal trees – Ocotea bullata and Warburgia salutaris. Plant Growth Regul. 34, 223–228. doi:10.1023/a:1013362615531
Krystal, G. (2020). Impacts of unsustainable harvesting of frankincense producing Boswellia trees. Orego: Oregon State University: Master of Natural Resources. Available at: https://ir.library.oregonstate.edu/concern/graduate_projects/4j03d620f.
Kubentayev, S. A., Zhumagul, M. Z., Kurmanbayeva, M. S., Alibekov, D. T., Kotukhov, J. A., Sitpayeva, G. T., et al. (2021). Current state of populations of Rhodiola rosea L. (Crassulaceae) in east Kazakhstan. Bot. Stud. 62 (1), 19–39. doi:10.1186/s40529-021-00327-4
Kumar, P., Tripathi, S., Rout, P. K., Khare, S. K., and Naik, S. (2023). Quality control analysis of high-value agarwood oil by thermogravimetric analysis (TGA). Flavour Fragr. J. 38 (1), 27–36. doi:10.1002/ffj.3721
Kusangaya, S., Warburton, M. L., Archer van Garderen, E., and Jewitt, G. P. W. (2014). Impacts of climate change on water resources in southern Africa: a review. Phys. Chem. Earth 67-69, 47–54. doi:10.1016/j.pce.2013.09.014
Lee, H. (2023). Ex situ conservation efforts for plant diversity protection with A focus on seeds. Open Agric. J. 17 (1), e187433152307250-e187433152307258. doi:10.2174/18743315-v17-230822-2023-15
Leonard, C. M., and Viljoen, A. M. (2015). Warburgia: a comprehensive review of the botany, traditional uses and phytochemistry. J. Ethnopharmacol. 165, 260–285. doi:10.1016/j.jep.2015.02.021
Liu, C.-Z., Murch, S. J., Jain, J. C., and Saxena, P. K. (2004). Goldenseal (Hydrastis canadensis L.): in vitro regeneration for germplasm conservation and elimination of heavy metal contamination. Vitro Cell. Dev. Biol. - Plant 40 (1), 75–79. doi:10.1079/ivp2003499
Liu, H., Burkhart, E. P., Chen, V. Y. J., and Wei, X. (2021). Promotion of in situ forest farmed American ginseng (Panax quinquefolius L.) as a sustainable bse strategy: Opportunities and challenges. Front. Ecol. Evol. 9, 652103–652121. doi:10.3389/fevo.2021.652103
Liu, Z., Wang, Z., Xu, M., Ma, J., Sun, Y., and Huang, Y. (2023). The priority areas and possible pathways for health cooperation in BRICS countries. Glob. Health Res. Policy 8 (1), 36–44. doi:10.1186/s41256-023-00318-x
Mander, M. (1998). Marketing of medicinal plants in South Africa: a case study in KwaZulu–Natal. Rome, Italy: Food and Agriculture Organization of the United Nations, 151.
Maroyi, A. (2008). Ethnobotanical study of two threatened medicinal plants in Zimbabwe. Int. J. Biodivers. Sci. Manag. 4 (3), 148–153. doi:10.3843/biodiv.4.3:2
Maroyi, A. (2013). Warburgia salutaris (Bertol.f.) Chiov.: a multi-use ethnomedicinal plant species. J. Med. Plants Res. 7, 53–60.
Mashimbye, M. J., Maumela, M. C., and Drewes, S. E. (1999). A drimane sesquiterpenoid lactone from Warburgia salutaris. Phytochemistry 51 (3), 435–438. doi:10.1016/s0031-9422(98)00753-5
McGraw, J. B., Lubbers, A. E., Van der Voort, M., Mooney, E. H., Furedi, M. A., Souther, S., et al. (2013). Ecology and conservation of ginseng (Panax quinquefolius) in a changing world. Ann. N. Y. Acad. Sci. 1286, 62–91. doi:10.1111/nyas.12032
McLaughlin, B. C., Skikne, S. A., Beller, E., Blakey, R. V., Olliff-Yang, R. L., Morueta-Holme, N., et al. (2022). Conservation strategies for the climate crisis: an update on three decades of biodiversity management recommendations from science. Biol. Conserv. 268, 109497–110109. doi:10.1016/j.biocon.2022.109497
Mehmeti, A., Candido, V., Canaj, K., Castronuovo, D., Perniola, M., D’Antonio, P., et al. (2024). Energy, environmental, and economic sustainability of saffron cultivation: insights from the first European (Italian) case study. Sustainability 16 (3), 1179–1200. doi:10.3390/su16031179
Milcu, A. I., Hanspach, J., Abson, D., and Fischer, J. (2013). Cultural ecosystem services A literature review and prospects for future research. Ecol. Soc. 18 (3), 44–78. doi:10.5751/es-05790-180344
Ministry of Environment and Forestry B (2024). Non detriment aindings (NDF) of Aquilaria malaccencis from Indonesia. Available at: https://cites.org/sites/default/files/ndf_material/240711%20NDF_Aquilaria%20malaccesis.pdf.
Mir, A. B., and Aasifa, A. (2023). The production and problems of saffron industry in Jammu and Kashmir - a brief analysis. Int. J. Humanit. Soc. Sci. Manag. (IJHSSM) 3, 574–579.
Molina, R. V., Valero, M., Navarro, Y., Guardiola, J. L., and García-Luis, A. (2005). Temperature effects on flower formation in saffron (Crocus sativus L.). Sci. Hortic. 103 (3), 361–379. doi:10.1016/j.scienta.2004.06.005
Monteiro, W. P., Dalapicolla, J., Carvalho, C. S., Costa Veiga, J., Vasconcelos, S., Ramos, S. J., et al. (2022). Genetic diversity and structure of an endangered medicinal plant species (Pilocarpus microphyllus) in eastern Amazon: implications for conservation. Conserv. Genet. 23 (4), 745–758. doi:10.1007/s10592-022-01454-6
Monteiro, W. P., de Souza, E. B., Miranda, L. S., Anjos, L. J. S., and Caldeira, C. F. (2023). Potential distribution of Pilocarpus microphyllus in the Amazonia/Cerrado Biomes under aear-future climate change Scenarios. Plants (Basel) 12 (11), 2106–2120. doi:10.3390/plants12112106
Moreira, P.RKVP, Lameira, O. A., Campelo, M. F., and Ramires, A. C. S. (2021). Estudo fenológico do germoplasma de Pilocarpus microphyllus Stapf Ex Wardleworth correlacionado com elementos climáticos. Research. Soc. Dev. 10, e7710514626-e7710514638.
Mori, A. S., Gonzalez, A., Seidl, R., Reich, P. B., Dee, L., Ohashi, H., et al. (2024). Urgent climate action is needed to ensure effectiveness of protected areas for biodiversity benefits. One Earth 7 (10), 1874–1885. doi:10.1016/j.oneear.2024.08.003
Muniz, F. H. (2008). Padrões de floração e frutificação de árvores da Amazônia Maranhense. Acta Amaz. 38 (4), 617–626. doi:10.1590/s0044-59672008000400004
Mwijuke, G. (2024). Climate change threatens frankincense-bearing Boswellia trees. Guardian. Available at: https://www.theguardian.com/environment/2024/sep/16/frankincense-trees-extinction-resin-trade-africa-arabia-western-wellness-industry-fragrance.
Mykhailenko, O., Desenko, V., Ivanauskas, L., and Georgiyants, V. (2020a). Standard operating procedure of Ukrainian saffron cultivation According with good agricultural and collection practices to assure quality and traceability. Industrial Crops Prod. 151, 112376–112387. doi:10.1016/j.indcrop.2020.112376
Mykhailenko, O., Gudžinskas, Z., Kovalyov, V., Desenko, V., Ivanauskas, L., Bezruk, I., et al. (2020b). Effect of ecological factors on the accumulation of phenolic compounds in Iris species from Latvia, Lithuania and Ukraine. Phytochem. Anal. 31 (5), 545–563. doi:10.1002/pca.2918
Mykhailenko, O., Saidov, N. B., Ivanauskas, L., and Georgiyants, V. (2022). Model implementation of the legal regulation on medicinal plant cultivation for pharmaceutical purposes. Case study of Crocus sativus cultivation in Ukraine. Botanica 28, 27–38. doi:10.35513/botlit.2022.1.4
Nájera, C., Gallegos-Cedillo, V. M., Ros, M., and Pascual, J. A. (2023). Role of spectrum-light on productivity, and plant quality over vertical farming systems: Bibliometric analysis. Hortic. (Basel) 9 (1), 63–86. doi:10.3390/horticulturae9010063
NatureServe Explorer (2023a). Panax quinquefolius L. Available at: https://explorer.natureserve.org/Taxon/ELEMENT_GLOBAL.2.130734/Panax_quinquefolius.
NatureServe Explorer (2023b). Rhodiola rosea. Available at: https://explorer.natureserve.org/Taxon/ELEMENT_GLOBAL.2.155970/Rhodiola_rosea.
Ncube, B., Finnie, J. F., and Van Staden, J. (2012). Quality from the field: the impact of environmental factors as quality determinants in medicinal plants. South Afr. J. Bot. 82, 11–20. doi:10.1016/j.sajb.2012.05.009
Neils, A. L., Brisco-McCann, E. I., Harlan, B. R., and Hausbeck, M. K. (2021). Management strategies for Alternaria leaf blight on American ginseng. Crop Prot. 139, 105302–105309. doi:10.1016/j.cropro.2020.105302
Nemati, Z., Harpke, D., Gemicioglu, A., Kerndorff, H., and Blattner, F. R. (2019). Saffron (Crocus sativus) is an autotriploid that evolved in Attica (Greece) from wild Crocus cartwrightianus. Mol. Phylogenetics Evol. 136, 14–20. doi:10.1016/j.ympev.2019.03.022
Obahiagbon, E. G., and Ogwu, M. C. (2023a). “Consumer Perception and demand for sustainable herbal medicine products and market,” in Herbal medicine thytochemistry: Applications and Trends. Editors S. C. Izah, M. C. Ogwu, and M. Akram (Cham: Springer International Publishing), 1–34.
Obahiagbon, E. G., and Ogwu, M. C. (2023b). “Sustainable supply chain management in the herbal medicine industry,” in Herbal medicine Phytochemistry: Applications and Trends. Editors S. C. Izah, M. C. Ogwu, and M. Akram (Cham: Springer International Publishing), 1–29.
Oldekop, J. A., Holmes, G., Harris, W. E., and Evans, K. L. (2016). A global assessment of the social and conservation outcomes of protected areas. Conserv. Biol. 30 (1), 133–141. doi:10.1111/cobi.12568
Oldfield, S., Lusty, C., and MacKinven, A. (1998). The world list of threatened trees. Cambridge, UK: World Conversation Press.
Pacifici, M., Foden, W. B., Visconti, P., Watson, J. E. M., Butchart, S. H. M., Kovacs, K. M., et al. (2015). Assessing species vulnerability to climate change. Nat. Clim. Change 5 (3), 215–224. doi:10.1038/nclimate2448
Pant, P., Pandey, S., and Dall'Acqua, S. (2021). The influence of environmental conditions on secondary metabolites in medicinal plants: a literature review. Chem. Biodivers. 18 (11), e2100345–e2100349. doi:10.1002/cbdv.202100345
Parmesan, C., and Hanley, M. E. (2015). Plants and climate change: complexities and surprises. Ann. Bot. 116 (6), 849–864. doi:10.1093/aob/mcv169
Patni, B., Bhattacharyya, M., kumari, A., and purohit, V. K. (2022). Alarming influence of climate change and compromising quality of medicinal plants. Plant Physiol. Rep. 27 (1), 1–10. doi:10.1007/s40502-021-00616-x
Pearce-Higgins, J. W., Antão, L. H., Bates, R. E., Bowgen, K. M., Bradshaw, C. D., Duffield, S. J., et al. (2022). A framework for climate change adaptation indicators for the natural environment. Ecol. Indic. 136, 108690–109100. doi:10.1016/j.ecolind.2022.108690
Philpott, M., Pence, V. C., and Coffey, E. E. D. (2022). Building capacity in the conservation of exceptional plant species. Appl. Plant Sci. 10, e11498–e11501. doi:10.1002/aps3.11498
Pinheiro, C. U. (1997). Jaborandi (Pilocarpus sp., Rutaceae): a wild species. Econ. Bot. 51, 49–58. doi:10.1007/bf02910403
Pinheiro, C. U. B. (2002). Extrativismo, cultivo e privatização do jaborandi (Pilocarpus microphyllus Stapf ex Holm.; Rutaceae) no Maranhão, Brasil. Acta Bot. Bras. 16 (2), 141–150. doi:10.1590/s0102-33062002000200002
Pinheiro, C. U. B. (2006). “Human impacts on Amazonia. 13. Extractivism, domestication, and Privatization of a native plant resource: the case of jaborandi (Pilocarpus microphyllus Stapf ex colmes) in Maranhão, Brazil,” in The role of traditional ecological knowledge in conservation and development. Editors D. A. Posey, and M. J. Balick (Columbia University Press), 210–221.
Pirani, J. R., and Groppo, M. (2014). Rutaceae in Lista de Espécies da Flora do Brasil. Jard. Botânico do Rio J., 1–5. Available at: http://floradobrasil.jbrj.gov.br/jabot/floradobrasil/FB21.
Pirasteh-Anosheh, H., Babaie-Zarch, M. J., Nasrabadi, M., Parnian, A., Alavi-Siney, S. M., Beyrami, H., et al. (2023). Climate and management factors influence saffron yield in different environments. Agrosystems, Geosciences Environ. 6 (3), 18–31. doi:10.1002/agg2.20418
Prokopyev, A. S., Yamburov, M. S., Chernova, O. D., Kataeva, T. N., Prokopyeva, E. S., Machkinis, E. Y., et al. (2021). Ecological and morphological features of Rhodiola rosea L. in natural populations in the Altai Mountains. Acta Biol. Sib. 7, 529–544. doi:10.3897/abs.7.e78936
Püski, P., Körmöczi, T., Berkecz, R., Barta, A., Bajtel, Á., and Kiss, T. (2024). Rapid detection of adulteration in Boswellia extracts with hitric acid by UPLC-HRMS and 1H NMR. J. Diet. Suppl. 21 (4), 462–477. doi:10.1080/19390211.2023.2299886
Pyke, G. H., Thomson, J. D., Inouye, D. W., and Miller, T. J. (2016). Effects of climate change on phenologies and distributions of bumble bees and the plants they visit. Ecosphere 7, e01267–e86. doi:10.1002/ecs2.1267
Qu, L., Zou, W., Zhou, Z., Zhang, T., Greef, J., and Wang, M. (2014). Non-European traditional herbal medicines in Europe: a community herbal monograph perspective. J. Ethnopharmacol. 156, 107–114. doi:10.1016/j.jep.2014.08.021
Rabe, T., and van Staden, J. (2000). Isolation of an antibacterial sesquiterpenoid from Warburgia salutaris. J. Ethnopharmacoly 73 (1-2), 171–174. doi:10.1016/s0378-8741(00)00293-2
Rajpoot, R., Adhikari, D., Verma, S., Saikia, P., Kumar, A., Grant, K. R., et al. (2020). Climate models predict a divergent future for the medicinal tree Boswellia serrata Roxb. in India. Glob. Ecol. Conservation 23, e01040–e01050. doi:10.1016/j.gecco.2020.e01040
Razmavaran, M. H., Sepaskhah, A. R., and Ahmadi, S. H. (2024). Water footprint and production of rain-fed saffron under different planting methods with ridge plastic mulch and pre-flowering irrigation in a semi-arid region. Agric. Water Manag. 291, 108632–108646. doi:10.1016/j.agwat.2023.108632
Reid, W. V., Mooney, H. A., Cropper, A., Capistrano, D., Carpenter, S. R., Chopra, K., et al. (2005). Ecosystems and human well-being - Synthesis: A report of the Millennium ecosystem assessment. Washington D.C.: Island Press, 137.
Rezaie, R., McGahan, A. M., Frew, S. E., Daar, A. S., and Singer, P. A. (2012). Emergence of biopharmaceutical innovators in China, India, Brazil, and South Africa as global competitors and collaborators. Health Res. Policy Syst. 10, 18–13. doi:10.1186/1478-4505-10-18
Ripple, W. J., Wolf, C., Newsome, T. M., Galetti, M., Alamgir, M., Crist, E., et al. (2017). World Scientists’ warning to Humanity: a second notice. BioScience 67 (12), 1026–1028. doi:10.1093/biosci/bix125
Rocha, J. A., Vasconcelos, S., Da Silva, F. M. M., Jurkiewicz Melo, A., Souza Silva, M. F., De Miranda, J. A. L., et al. (2014). ISSR primer selection for genetic nariability analyses with jaborandi (Pilocarpus microphyllus Stapf ex Wardlew., Rutaceae). For. Res. Open Access 3 (4), 1000126–1000131. doi:10.4172/2168-9776.1000126
Sabá, R. T., Lameira, O. A., Luz, J. M. Q., Gomes, APDR, and Innecco, R. (2002). Micropropagação do jaborandi. Hortic. Bras. 20 (1), 106–109. doi:10.1590/s0102-05362002000100021
Sam, J. (2024). Climate crisis and neglect threaten Spain’s saffron crop. Available at: https://www.theguardian.com/world/2023/jan/22/climate-crisis-and-neglect-threaten-spains-saffron-crop.
Samsuddin, A. S., Lee, S. Y., Ong, S. P., and Mohamed, R. (2019). Damaging insect pests and diseases and their threats to agarwood tree plantations. Sains Malays. 48 (3), 497–507. doi:10.17576/jsm-2019-4803-02
Sandhu, S. S., Abreu, I. N., Colombo, C. A., and Mazzafera, P. (2006). Pilocarpine content and molecular diversity in jaborandi. Sci. Agric. 63 (5), 478–482. doi:10.1590/s0103-90162006000500010
Saraf, S. A., Wani, M. H., Wani Sa, , Rauf, S. A., Baba, S. H., Shaheen, F. A., et al. (2018). Economics of saffron cultivation in Kashmir. Acta Hortic. 1200, 165–176. doi:10.17660/actahortic.2018.1200.27
Sawaya, ACHF, Vaz, B. G., Eberlin, M. N., and Mazzafera, P. (2011). Screening species of Pilocarpus (Rutaceae) as sources of pilocarpine and other imidazole alkaloids. Genet. Resour. Crop Evol. 58 (3), 471–480. doi:10.1007/s10722-011-9660-2
Schindler, D. E., and Hilborn, R. (2015). Sustainability. Prediction, precaution, and policy under global change. Science 347 (6225), 953–954. doi:10.1126/science.1261824
Schmidt, J. P., Cruse-Sanders, J., Chamberlain, J. L., Ferreira, S., and Young, J. A. (2019). Explaining harvests of wild-harvested herbaceous plants: American ginseng as a case study. Biol. Conserv. 231, 139–149. doi:10.1016/j.biocon.2019.01.006
Scott-Shaw, C. R. (2001). Rare and threatened plants of KwaZulu-Natal and Neighbouring regions. Plant Syst. Evol. 227 (3/4), 245–248.
Selwal, N., Rahayu, F., Herwati, A., Latifah, E., Suhara, C., Suastika, I. B. K., et al. (2023). Enhancing secondary metabolite production in plants: exploring traditional and modern strategies. J. Agric. Food Res. 14, 100702. doi:10.1016/j.jafr.2023.100702
Senkoro, A. M. (2021). “Ethnobotany and conservation biology of Warburgia salutaris (G.Bertol.) Chiov., a threatened medicinal plant in southern Mozambique,”. PhD Thesis (Makhanda, South Africa: Rhodes University), 234.
Senkoro, A. M., Munt, D. D., Shackleton, C. M., Ribeiro-Barros, A. I., and Voeks, R. A. (2024). The case of a threatened medicinal tree with optimistic prospects under climate change. Glob. Ecol. Conservation 54, e03126. doi:10.1016/j.gecco.2024.e03126
Senkoro, A. M., Shackleton, C. M., Voeks, R. A., and Ribeiro, A. I. (2019). Uses, knowledge, and management of the threatened pepper-bark tree (Warburgia salutaris) in southern Mozambique. Econ. Bot. 73, 304–324. doi:10.1007/s12231-019-09468-x
Senkoro, A. M., Talhinhas, P., Simões, F., Batista-Santos, P., Shackleton, C. M., Voeks, R. A., et al. (2020). The genetic legacy of fragmentation and overexploitation in the threatened medicinal African pepper-bark tree, Warburgia salutaris. Sci. Rep. 10 (1), 19725–19738. doi:10.1038/s41598-020-76654-6
Sentinella, A. T., Warton, D. I., Sherwin, W. B., Offord, C. A., Moles, A. T., and Wang, Z. (2020). Tropical plants do not have narrower temperature tolerances, but are more at risk from warming because they are close to their upper thermal limits. Glob. Ecol. Biogeogr. 29 (8), 1387–1398. doi:10.1111/geb.13117
Shen, L., Li, X.-W., Meng, X.-X., Wu, J., Tang, H., Huang, L.-F., et al. (2019). Prediction of the globally ecological suitability of Panax quinquefolius by the geographic information system for global medicinal plants (GMPGIS). Chin. J. Nat. Med. 17 (7), 481–489. doi:10.1016/S1875-5364(19)30069-X
Skorupa, L. A. (2000). Espécies de Pilocarpus Vahl (Rutaceae) da Amazônia brasileira. Acta Amaz. 30 (1), 59–70. doi:10.1590/1809-43922000301070
Small, E., and Catling, P. (1999). “Rhodiola rosea (roseroot),” in Canadian medicinal crops (Ottawa, Ontario, Canada: NRC Research Press), 134–139.
Sneader, W. (2005). Drug Discovery. A history. Chapter 9: Alkaloids. Chichester, UK: John Wiley and Sons Ltd., 88–105.
Soehartono, T., and Newton, A. C. (2001). Conservation and sustainable use of tropical trees in the genus Aquilaria II.: the impact of gaharu harvesting in Indonesia. Biol. Conserv. 97 (1), 29–41. doi:10.1016/s0006-3207(00)00089-6
Sousa, M. (1991). Constituintes químicos ativos de plantas medicinais brasileiras. Fortaleza, Brazil: Edições UFC Fortaleza, 416.
Souther, S. (2011). “Demographic response of American ginseng (Panax quinquefolius L.) to climate change,”. Graduate Theses, Dissertations, and Problem Reports (Morgantown, West Virginia: West Virginia University), 219.
Souther, S., Lechowicz, M. J., and McGraw, J. B. (2012). Experimental test for adaptive differentiation of ginseng populations reveals complex response to temperature. Ann. Bot. 110 (4), 829–837. doi:10.1093/aob/mcs155
Souther, S., and McGraw, J. B. (2014). Synergistic effects of climate change and harvest on extinction risk of American ginseng. Ecol. Appl. 24 (6), 1463–1477. doi:10.1890/13-0653.1
SunLand Saffron (2024). Why saffron production rate decreased in 2023-2024? Available at: https://sunlandsaffron.com/why-saffron-production-rate-decreased-in-2023-2024/.
SunLand Saffron and Herbs (2022). Learn more about Spanish saffron. Available at: https://sunlandsaffron.com/learn-more-about-spanish-saffron/.
Sutomo, I. R., and Kurniawati, F. (2021). Habitat suitability model of Agarwood in a changing climate. IOP Conf. Ser. Earth Environ. Sci. 724 (1), 012022. doi:10.1088/1755-1315/724/1/012022
Symmonds, R., and Crouch, N. (2000). Propagating the pepper-bark tree (Warburgia salutaris): the Silverglen experience. PlantLife 22, 24–26.
Tamuli, P., Boruah, P., Nath, S. C., and Leclercq, P. (2005). Essential oil of eaglewood tree: a product of pathogenesis. J. Essent. Oil Res. 17 (6), 601–604. doi:10.1080/10412905.2005.9699008
Tan, C. S., Isa, N. M., Ismail, I., and Zainal, Z. (2019). Agarwood induction: current developments and future Perspectives. Front. Plant Sci. 10, 122–135. doi:10.3389/fpls.2019.00122
Tang, X., Yuan, Y., and Zhang, J. (2020). How climate change will alter the distribution of suitable Dendrobium habitats. Front. Ecol. Evol. 8, 536339–536356. doi:10.3389/fevo.2020.536339
Taura, L., and Gudžinskas, Z. (2024). What factors determine the natural fruit Set of Cephalanthera longifolia and Cephalanthera rubra? Diversity 16 (6), 333–357. doi:10.3390/d16060333
Terletskaya, N. V., Turzhanova, A. S., Khapilina, O. N., Zhumagul, M. Z., Meduntseva, N. D., Kudrina, N. O., et al. (2023). Genetic diversity in natural populations of Rhodiola species of different adaptation strategies. Genes (Basel) 14 (4), 794–808. doi:10.3390/genes14040794
The World Health Organization (WHO) (1993). Guidelines on the conservation of medicinal plants. Gland, Switzerland: WWF – World Wide Fund for Nature. IUCN -The World Conservation Union; WWF-World Wide Fund for Nature: The International Union for Conservation of Nature and Natural Resources (IUCN), Gland, Switzerland, in partnership with The World Health Organization (WHO). Geneva, Switzerland.
Thompson, I., Lim, T., and Turjaman, M. (2022). Expensive, Exploited, and Endangered. A review of the agarwood-producing genera Aquilaria and Gyrinops. CITES considerations, trade patterns, conservation, and management ITTO Technical. Available at: https://cites.org/sites/default/files/documents/E-CoP19-Inf-12.pdf.
Thulin, M., Decarlo, A., and Johnson, S. P. (2019). Boswellia occulta (Burseraceae), a new species of frankincense tree from Somalia (Somaliland). Phytotaxa 394, 219–226. doi:10.11646/phytotaxa.394.3.3
UN Environmental Programme (2024). Landmark UN report: the world’s migratory species of animals are in decline, and the global extinction risk is increasing. Available at: https://www.unep.org/news-and-stories/press-release/landmark-un-report-worlds-migratory-species-animals-are-decline.
USDA. China (2022). American Ginseng approved for use as a food ingredient under Guangdong pilot program. Washington, DC: USDA Foreign Agricultural Service. Available at: https://fas.usda.gov/data/china-american-ginseng-approved-use-food-ingredient-under-guangdong-pilot-program.
USFWS (2005). Convention permit applications for wild American ginseng harvested in 2005. Washington, D.C.: U.S. Fish and Wildlife Service USFWS. Available at: https://www.fws.gov/international-affairs/permits/ginseng.20240
Van den Bosch, K., Witkowski, E. T. F., Thompson, D. I., and Cron, G. V. (2023). Reproductive ecology offers some answers to the pepperbark tree persistence puzzle in the Kruger National Park, South Africa. Glob. Ecol. Conservation 41, e02330. doi:10.1016/j.gecco.2022.e02330
Van der Voort, M. E. (2005). An ecological study of Panax quinquefolius in central Appalachia: seedling growth, harvest impacts and geographic variation in demography. Morgantown, West Virginia: West Virginia University, 167.
Van Wyk, B. E. (2011). The potential of South African plants in the development of new medicinal products. South Afr. J. Bot. 77 (4), 812–829. doi:10.1016/j.sajb.2011.08.011
Van Wyk, B.-E., and Gericke, N. (2000). People's plants: a Guide to useful plants of southern Africa. Pretoria: Briza Publications, 351.
Van Wyk, B.-E., Van Oudtshoorn, B., and Gericke, N. (2009). Medicinal plants of South Africa. Revised and expanded edition. Pretoria: Briza Publications, 336.
Veeman, T. S., Cunningham, A. B., and Kozanayo, W. (2014). “The economics of production of rare medicinal species introduced in southwestern Zimbabwe: Warburgia salutaris,” in Bark Use, management and commerce in Africa. Editor A. B. Cunningham (New York: The New York Botanical Garden Press), 179–188.
Wang, C., Peng, D., Liu, Y., Wu, Y., Guo, P., and Wei, J. (2021). Agarwood Alcohol extract protects against Gastric ulcer by Inhibiting Oxidation and inflammation. Evid. Based Complementary Altern. Med. 2021, 9944685–9944696. doi:10.1155/2021/9944685
Wang, Z., Wang, T., Hu, J., Jiao, H., Jin, Y., Sun, J., et al. (2024b). Comparisons of wild and cultivated American ginseng (Panax quinquefolius L.) genomes provide insights into changes in root growth and metabolism during domestication. Plant Biotechnolology J. 22 (7), 1963–1965. doi:10.1111/pbi.14316
Wang, Z., Wang, T., Zhang, X., Wang, J., Yang, Y., Sun, Y., et al. (2024a). Biodiversity conservation in the context of climate change: facing challenges and management strategies. Sci. Total Environ. 937, 173377–173391. doi:10.1016/j.scitotenv.2024.173377
Wani, T. A., Kaloo, Z. A., and Dangroo, N. A. (2022). Aconitum heterophyllum Wall. ex Royle: a critically endangered medicinal herb with rich potential for use in medicine. J. Integr. Med. 20 (2), 104–113. doi:10.1016/j.joim.2021.12.004
Wen, J., Zhou, L., Liu, L., and He, Y. (2022). Analysis of the impact of climate change on the distribution and active compound content of the plateau medicinal plant Nardostachys jatamansi (D. Don) DC. Industrial Crops Prod. 187, 115438–115447. doi:10.1016/j.indcrop.2022.115438
White, D. (2000). Raiding Mother Earth's treasure chest. Lady-Slipper Newsl. Ky. Native Plant Soc. 15 (2-3-Summer), 4.
Williams, V. L., Victor, J. E., and Crouch, N. R. (2013). Red Listed medicinal plants of South Africa: status, trends, and assessment challenges. South Afr. J. Bot. 86, 23–35. doi:10.1016/j.sajb.2013.01.006
Williams, V. L., Witkowski, E. T. F., and Balkwill, K. (2007). Relationship between bark thickness and diameter at breast height for six tree species used medicinally in South Africa. South Afr. J. Bot. 73 (3), 449–465. doi:10.1016/j.sajb.2007.04.001
Xiao, M. (2000). The Canadian ginseng industry: preparing for the 21st century. Ottawa, Canada: The Canadian Ginseng Industry, 7. Agrifood Canada -The Canadian Ginseng Industry - Preparing for the 21st Century - 1999.h11/8/01.
Xu, Y., Huang, J., Lu, X., Ding, Y., and Zang, R. (2019). Priorities and conservation gaps across three biodiversity dimensions of rare and endangered plant species in China. Biol. Conserv. 229, 30–37. doi:10.1016/j.biocon.2018.11.010
Yang, M., Sun, L., Yu, Y., Zhang, H., Malik, I., Wistuba, M., et al. (2023). Predicting the potential geographical distribution of Rhodiola L. In China under climate change Scenarios. Plants (Basel) 12 (21), 3735–3749. doi:10.3390/plants12213735
Yesuf, G. U., Brown, K. A., Walford, N. S., Rakotoarisoa, S. E., and Rufino, M. C. (2021). Predicting range shifts for critically endangered plants: is habitat connectivity irrelevant or necessary? Biol. Conserv. 256, 109033–109046. doi:10.1016/j.biocon.2021.109033
You, J., Qin, X., Ranjitkar, S., Lougheed, S. C., Wang, M., Zhou, W., et al. (2018a). Response to climate change of montane herbaceous plants in the genus Rhodiola predicted by ecological niche modelling. Sci. Rep. 8 (1), 5879–5891. doi:10.1038/s41598-018-24360-9
You, J., Qin, X., Ranjitkar, S., Lougheed, S. C., Wang, M., Zhou, W., et al. (2018b). Response to climate change of montane herbaceous plants in the genus Rhodiola predicted by ecological niche modelling. Sci. Rep. 8 (1), 5879–5891. doi:10.1038/s41598-018-24360-9
Young, B. E., Dubois, N. S., and Rowland, E. L. (2015). Using the climate change vulnerability index to inform adaptation planning: lessons, innovations, and next steps. Wildl. Soc. Bull. 39 (1), 174–181. doi:10.1002/wsb.478
Yu, C., Wang, C. Z., Zhou, C. J., Wang, B., Han, L., Zhang, C. F., et al. (2014). Adulteration and cultivation region identification of American ginseng using HPLC coupled with multivariate analysis. J. Pharm. Biomed. Analysis 99, 8–15. doi:10.1016/j.jpba.2014.06.031
Zhang, J., Lu, J., Zhu, Y., Huang, Q., Qin, L., and Zhu, B. (2022). Rhizosphere microorganisms of Crocus sativus as antagonists against pathogenic Fusarium oxysporum. Front. Plant Sci. 13, 1045147–1045160. doi:10.3389/fpls.2022.1045147
Zou, H., Chen, B., Zhang, B., Zhou, X., Zhang, X., Wang, J., et al. (2023). Conservation planning for the endemic and endangered medicinal plants under the climate change and human disturbance: a case study of Gentiana manshurica in China. Front. Plant Sci. 14, 1184556–1184576. doi:10.3389/fpls.2023.1184556
Keywords: endangered medicinal plants, ecosystem factors, climate change, sustainable practices, conservation strategies, traditional medicine, ethnopharmacology, key sustainability indicators
Citation: Mykhailenko O, Jalil B, McGaw LJ, Echeverría J, Takubessi M and Heinrich M (2025) Climate change and the sustainable use of medicinal plants: a call for “new” research strategies. Front. Pharmacol. 15:1496792. doi: 10.3389/fphar.2024.1496792
Received: 15 September 2024; Accepted: 24 December 2024;
Published: 03 February 2025.
Edited by:
John Thor Arnason, University of Ottawa, CanadaReviewed by:
Michael Rapinski, UMR7206 Eco Anthropologie et Ethnobiologie (EAE), FranceCopyright © 2025 Mykhailenko, Jalil, McGaw, Echeverría, Takubessi and Heinrich. This is an open-access article distributed under the terms of the Creative Commons Attribution License (CC BY). The use, distribution or reproduction in other forums is permitted, provided the original author(s) and the copyright owner(s) are credited and that the original publication in this journal is cited, in accordance with accepted academic practice. No use, distribution or reproduction is permitted which does not comply with these terms.
*Correspondence: Olha Mykhailenko, by5teWtoYWlsZW5rb0B1Y2wuYWMudWs= Michael Heinrich, bS5oZWlucmljaEB1Y2wuYWMudWs=
Disclaimer: All claims expressed in this article are solely those of the authors and do not necessarily represent those of their affiliated organizations, or those of the publisher, the editors and the reviewers. Any product that may be evaluated in this article or claim that may be made by its manufacturer is not guaranteed or endorsed by the publisher.
Research integrity at Frontiers
Learn more about the work of our research integrity team to safeguard the quality of each article we publish.