- 1School of Pharmacy and Department of Hepatology, The Affiliated Hospital of Hangzhou Normal University, Hangzhou Normal University, Hangzhou, China
- 2Key Laboratory of Elemene Class Anti-Cancer Chinese Medicines, Engineering Laboratory of Development and Application of Traditional Chinese Medicines, Collaborative Innovation Center of Traditional Chinese Medicines of Zhejiang Province, Hangzhou Normal University, Hangzhou, Zhejiang, China
Cancer is a serious public health problem in humans, and prevention and control strategies are still necessary. Therefore, the development of new therapeutic drugs is urgently needed. Targeting programmed cell death, particularly via the induction of cancer cell apoptosis, is one of the cancer treatment approaches employed. Recently, an increasing number of studies have shown that compounds from natural plants can target programmed cell death and kill cancer cells, laying the groundwork for use in future anticancer treatments. In this review, we focus on the latest research progress on the role and mechanism of natural plant active ingredients in different forms of programmed cell death, such as apoptosis, autophagy, necroptosis, ferroptosis, and pyroptosis, to provide a strong theoretical basis for the clinical development of antitumor drugs.
1 Introduction
Cancers are the leading cause of death worldwide, with an estimated 20 million new cases and 9.7 million deaths in 2022, among which the most common types are breast, lung, colorectal, and liver cancers (Bray et al., 2024). At present, the mainstream approach to cancer treatment is surgery, supplemented by drug therapy (Aquina et al., 2021). However, surgery may induce the spread of cancer cells and cause ischemia or reperfusion injury, acute and chronic pain, and large wounds (Siddiqi and Johnston, 2023; Chen et al., 2019; Jacobs et al., 2020). Moreover, among the currently approved cytostatic drugs, many have relatively low specificity for tumors and are highly toxic (Schirrmacher, 2019). Therefore, the development of new treatments to improve the prognosis of cancer patients is urgently needed.
Natural plant products have always been an important source of new drugs. In ancient times, people used herbs and formulations as medicines to treat various diseases. In 1806, Friedrich Wilhelm Sertürner separated morphine from poppy, marking the beginning of the search for active ingredients from natural plants in modern times (Klockgether-Radke, 2002). Since then, many natural compounds have been isolated from plants, such as artemisinin, quinine and atropine, and these products and their structural analogues have historically made significant contributions to disease treatment (Zhang L. et al., 2020). Recently, natural products from plants, such as curcumin for treating pancreatic cancer and paclitaxel for treating lung cancer, breast cancer and other cancers, have played increasingly important roles in the treatment of malignant tumors. Furthermore, these products can be used as sensitizers to enhance the anticancer effects of chemotherapy drugs and radiotherapy and to reduce cancer resistance (Lin et al., 2020). Traditional Chinese medicines such as Radix Astragali, Baizhu, and Poria have been found to be effective in treating lung cancer when combined with EGFR inhibitors (Sui et al., 2020a). In general, the active ingredients in plants play important roles in cancer treatment. It has been reported that 20% of the active ingredients in plants, including terpenoids, flavonoids, and steroids, have significant potential as drugs (Yu et al., 2022). Interestingly, on the basis of molecular expression profiles and genomic analysis, the applications of natural plant products, especially traditional Chinese medicines, are being continuously optimized and modernized, and an increasing number of active ingredients are being discovered and utilized for cancer treatment (Hui et al., 2024).
Programmed cell death (PCD) is a cell death process regulated by a series of molecular programs. PCD currently includes apoptosis, autophagy, necroptosis, pyroptosis, ferroptosis, and cuproptosis (Mishra et al., 2018). Caspase and Bcl family proteins are central regulatory molecules in the apoptosis pathway and are closely associated with proapoptotic signals (Peng et al., 2022). ULK1, Beclin-1, LC3, and p62 are key regulatory factors in autophagy, and studies have shown that autophagy is modulated by the PI3K/Akt/mTOR, MAPK/mTOR and AMPK/mTOR pathways (Yoshida, 2017). Ferroptosis, a newly discovered PCD pathway, is closely associated with glutathione peroxidase (GPX family) and the cystine/glutamate transporter (SLC7A11). In pyroptosis, gasdermin (GSDM) family members are key mediators that are regulated by caspase-related proteins and ultimately cause cell death (Tong et al., 2022).
The use of natural plant compounds to target key signaling pathways or genes involved in PCD provides a new approach for cancer treatment. Active ingredients in plants, such as saponins, quinones, alkaloids and flavonoids, can target apoptosis or necroptosis pathways in cancer cells (Yang et al., 2023; Gali-Muhtasib et al., 2015). Terpenoids and flavonoids promote apoptosis and have been applied in the systemic treatment of patients with colon cancer, breast cancer, and liver cancer (Hui et al., 2024).
This review summarizes the roles and mechanisms of major categories of natural plant active ingredients in different PCD processes and provides a strong theoretical basis for clinical cancer management (Figure 1). The types and sources of natural plant products, mechanisms involved in PCD, and cancer types described in this review are presented in Table 1, and the chemical structural formulas of the related natural plant products are presented in Table 2.
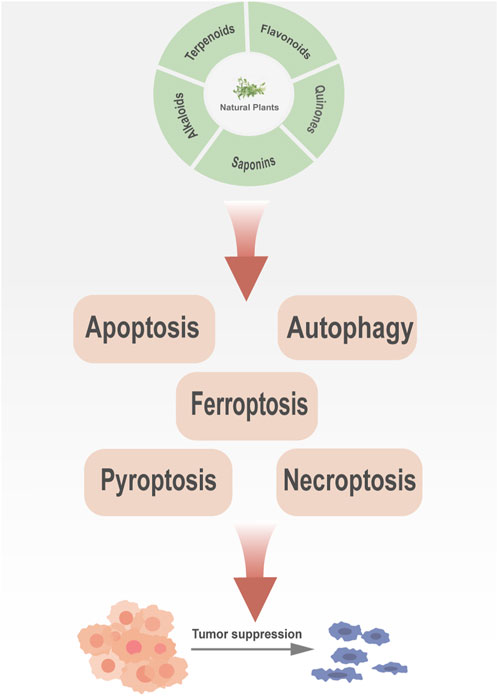
Figure 1. Natural plant products can inhibit cancer development via targeting different programmed cell death pathways.

Table 2. Structural formula of natural plant active ingredients that target programmed cell death in cancer cell.
2 Modulation of PCD in cancer via natural plant compounds
2.1 Apoptosis
Apoptosis was the first form of PCD to be identified; it is a strictly controlled mode of cell death characterized by cell shrinkage, nuclear condensation, and DNA fragmentation (Mishra et al., 2018). The natural plant compounds that target tumor cell apoptosis are summarized in Figure 2.
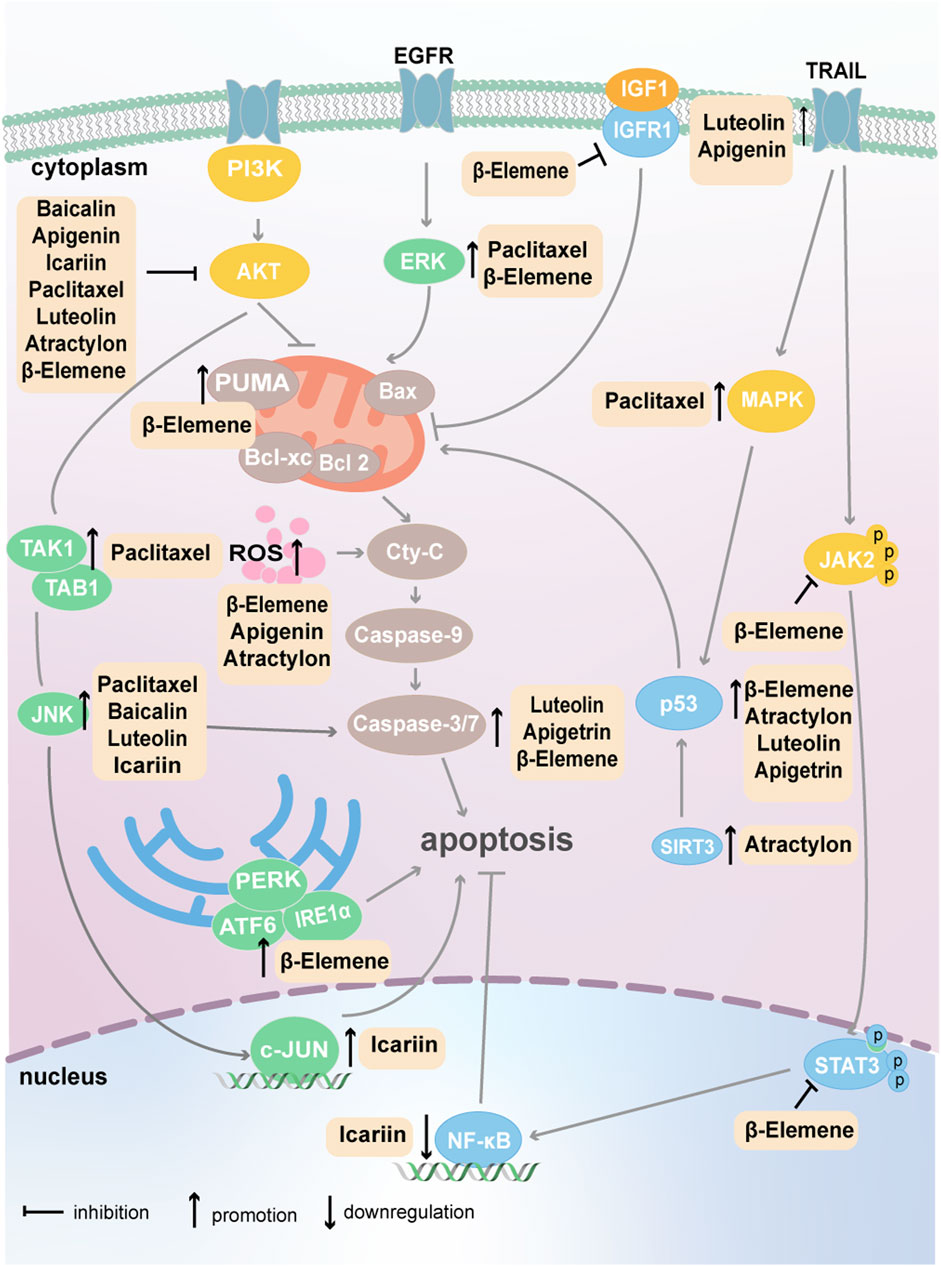
Figure 2. Plant natural compounds induce the apoptosis of cancer cells via mitochondrial and endoplasmic reticulum pathways.
2.1.1 Terpenoids
Terpenoids are a large class of organic compounds widely distributed in plants, insects, microorganisms and marine organisms. Some natural terpenoids have attracted increasing attention from researchers because of their strong anticancer and anti-inflammatory activities.
2.1.1.1 β-Elemene
β-Elemene comes from the root of Curcuma wenyujin and is now widely used to treat lung cancer, liver cancer, respiratory and digestive tract tumors, and malignant pleural effusion (Liu et al., 2020a). Trending with the latest innovative concept of the “molecular compatibility theory”, the research and development of β-Elemene dosage forms has become more diverse (Jiang et al., 2024). In addition to widely used β-Elemene emulsions, PEGylated β-Elemene liposomes exhibit enhanced antitumor effects (Zhai et al., 2020). Moreover, structural modification products of β-Elemene, nitric oxide derivatives (Zhu et al., 2023; Bai et al., 2022), hydroxyl carboximates (Gao et al., 2022), and macrocycles (Qi et al., 2022) all have significant antitumor effects.
Among the antitumor mechanisms of β-Elemene, the promotion of cancer cell apoptosis is one of the main mechanisms and involves the regulation of multiple signaling pathways and proteins, such as MAPK, PI3K/AKT, AMPK, STAT3 and p53 (Bai et al., 2021). β-Elemene can trigger apoptosis by activating the intrinsic pathway [mediated by a reduction in the mitochondrial membrane potential and release of cytochrome c from mitochondria or excessive endoplasmic reticulum (ER) stress] or extrinsic pathway (mediated by death receptors) and ultimately upregulate caspase-3 expression to initiate apoptosis (Liu et al., 2020b).
The combination of β-Elemene with arsenene nanodots acts on the mitochondrial apoptosis pathway, drives the production of excess reactive oxygen species (ROS), results in cytochrome c release, and induces cancer cell apoptosis (Liu et al., 2020b; Liu et al., 2021). β-Elemene alone also affects the generation of ROS in cancer; it can reduce the GSH content in lung cancer cells and inhibit glutathione synthesis, leading to the massive production of ROS (Song et al., 2023). In addition to effects on the intrinsic mitochondrial pathway, the effect of β-Elemene on cell apoptosis can also occur through the ER-mediated apoptosis pathway. β-Elemene can upregulate the expression of the unfolded protein response-related proteins PERK, IRE1α and ATF6, increase ER stress, and ultimately promote non-small cell lung cancer (NSCLC) cell apoptosis (Liu et al., 2017).
Additionally, β-Elemene can activate the AMPK pathway and inhibit the MAPK/ERK and AKT/mTOR pathways, resulting in the apoptosis of lung cancer cells (Wang J. et al., 2021). In bladder cancer and colorectal cancer (CRC), β-Elemene can induce apoptosis by generating a large amount of ROS and activating the AMPK pathway (Wang et al., 2022; Liu et al., 2020c). Yang et al. discovered that high concentrations of β-Elemene significantly stimulate the expression of p53 and ultimately cause glioma cells to undergo apoptosis (Yang D. et al., 2021). Intriguingly, another study showed that β-Elemene significantly promotes apoptosis in prostate cancer cells, an effect that may be associated with the p53 pathway (Dong et al., 2024). In ovarian cancer, β-Elemene significantly suppresses STAT3 phosphorylation, thereby promoting cell apoptosis (Xiaomeng et al., 2020). Cai et al. revealed that β-Elemene inhibits Janus-activated kinase 2 (JAK2) and Src phosphorylation and inactivated the downstream STAT3 pathway, leading to the massive production of ROS and the apoptosis of glioma cells (Cai SZ. et al., 2021).
In other mechanistic studies, β-Elemene was shown to suppress the growth of triple-negative breast cancer (TNBC) cells by reducing the expression of insulin-like growth factor 1 (IGF1), insulin-like growth factor 1 receptor (IGF1R) and Bcl-2; promoting the expression of cleaved caspase-3; and thus initiating apoptosis (Xie et al., 2022). In Burkitt’s lymphoma, β-Elemene stimulates the expression of p53 upregulated modulator of apoptosis (PUMA), a proapoptotic gene, which subsequently increases the expression of proteins such as Bax and Bak to induce cell apoptosis (Hu and Gao, 2018).
2.1.1.2 Atractylon
Atractylon is derived from the perennial herb Atractylodes macrocephala of the Asteraceae family. It has antitumor, antibacterial, anti-inflammatory, antiaging, antioxidant, and neuroprotective activities (Cheng et al., 2016).
Atractylon suppresses the PI3K/AKT/mTOR signaling pathway and downregulates the expression of PI3K, AKT, mTOR and Bcl-2 to induce the apoptosis of CRC cells (Mao et al., 2022). In glioma, atractylon can promote apoptosis by increasing the expression of sirtuin 3 and downstream p53 and Bcl-2 (Sun et al., 2022). Although molecular docking revealed that atractylon can bind to sirtuin 3, the detailed mechanism by which atractylon controls the expression of sirtuin 3 remains unclear. Cheng et al. reported that the apoptosis of liver cancer cells increases after treatment with atractylon, which was shown to increase ROS levels and Bax and cleaved caspase-3 expression and inhibit Bcl-2 expression (Cheng et al., 2019).
2.1.1.3 Paclitaxel
Paclitaxel is derived from the yew tree. Owing to its highly efficient biological activity, unique mechanism of action, and broad antitumor spectrum, paclitaxel has been widely used in clinical cancer treatment (Zhao et al., 2022). The main mechanism of action of paclitaxel is binding to tubulin, especially β-tubulin, which inhibits microtubule depolymerization and blocks spindle formation and cell division. Paclitaxel blocks the mitotic process, causing tumor cells to stay in G2 and M phases, which triggers apoptotic signals and ultimately leads to the death of tumor cells (Hardin et al., 2017). The regulatory mechanism by which paclitaxel induces cancer cell apoptosis has also been well studied, and paclitaxel-induced apoptosis is known to be related to the PI3K/AKT, AMPK, mTOR and JNK pathways. For example, in breast cancer, paclitaxel inhibits the PI3K/AKT signaling pathway, upregulates cleaved caspase-3 and Bax expression, and downregulates Bcl-2 expression, and the combination of the AKT inhibitor Capivasertib with paclitaxel significantly prolongs the progression-free survival and overall survival of breast cancer patients (Li et al., 2020). Furthermore, studies have shown that paclitaxel can increase the protein levels of transforming growth factor-beta-activated kinase 1 (TAK1) and TAK1-binding protein 1 (TAB1), which are modulators of the JNK pathway, and increase the phosphorylation of JNK and the expression of cleaved caspase-7 and PARP, ultimately leading to the apoptosis of thyroid cancer cells (Yu-Wei et al., 2020). In prostate cancer, HIF-1α expression has been shown to significantly increase after paclitaxel treatment, stimulating the activation of JNK/caspase-3 signaling and promoting cell apoptosis (Zhang Y. et al., 2022).
The mTOR signaling pathway is involved in many cancers, and paclitaxel may have dual effects on this pathway. Paclitaxel can promote mucin20 expression and subsequently activate the mTOR pathway, thereby inducing the apoptosis of esophageal cancer cells (Li M. et al., 2023). However, the relationship between mucin20 and the mTOR pathway needs to be elucidated. Nevertheless, in breast cancer and lung cancer cells, paclitaxel activates AMPK, inhibits mTOR signaling and increases apoptosis (Rocha et al., 2011). This discrepancy in results means that how paclitaxel modulates the mTOR pathway needs to be discussed case by case according to different cancer types.
2.1.2 Flavonoids
2.1.2.1 Baicalin
Baicalin is a flavonoid compound extracted from the root of Scutellaria baicalensis Georgi. In addition to its antibacterial, diuretic, and anti-inflammatory effects, baicalin is currently also widely used to treat various cancers (Wen Y. et al., 2023). Baicalin induces the apoptosis of cholangiocarcinoma cells by activating the AMPK pathway and inhibiting the mTOR pathway (Jia et al., 2022a). Similarly, a study on lung cancer showed that baicalin can inactivate the AKT/mTOR pathway and promote apoptosis (Sui et al., 2020b). In pancreatic cancer, baicalin triggers cell apoptosis by targeting the JNK pathway (Huang et al., 2019).
2.1.2.2 Luteolin
Luteolin is a natural flavonoid compound found in many plants. Its anti-cancer mechanisms involve reversing the epithelial-mesenchymal transition (EMT), blocking the cell cycle, increasing ROS and regulating tumor cell apoptosis by controlling multiple cell signaling pathways (Imran et al., 2019). In tamoxifen-resistant breast cancer, luteolin promotes cell apoptosis by suppressing the PI3K/AKT/mTOR pathway. Further mechanistic research has revealed that luteolin stimulates the expression of mixed-lineage leukemia 3 (MLL3) and that MLL3 epigenetically inhibits the expression of Ras family genes, which are activators of PI3K (Wu HT. et al., 2020). Moreover, luteolin can function as a sensitizer in liver cancer when combined with sorafenib or tumor necrosis factor-related apoptosis-inducing ligand (TRAIL), a cytokine that initiates cancer cell apoptosis, synergistically causing cell apoptosis by activating the JNK signaling pathway (Feng et al., 2018; Nazim and Park, 2019a). In addition to affecting signaling pathways, luteolin significantly increases p53 phosphorylation and colon cancer cell apoptosis (Yoo et al., 2022).
In addition, another unique anti-cancer mechanism of luteolin is inhibiting the Wnt/β-catenin signaling pathway (Pandurangan et al., 2013). Luteolin has been shown to reverse the EMT by down-regulating β-catenin in TNBC, and to attenuate chemoresistance in osteosarcoma by inhibiting the β-catenin signaling pathway (Qin et al., 2022; Lin et al., 2017). Recently, Yang et al.‘s molecular docking analysis revealed that luteolin could form a stable hydrogen bond with β-catenin, leading to the loss of its α-helix structure and inducing its conformational change, ultimately inhibiting Wnt signaling and promoting the apoptosis of cholangiocarcinoma (CCA) cells (Yang et al., 2024).
2.1.2.3 Icariin
Epimedium is a perennial herb in the Berberidaceae family, and icariin is its main active ingredient. Icariin is a natural flavonoid monomer with various functions in the biological processes of cancer (Zhang X. et al., 2023). Song et al. reported that icariin suppresses the protein level of the NF-κB p65 subunit in the nucleus; stimulates the expression of sirtuin 6, which deacetylates histone H3 lysine 9 around the promoters of NF-κB target genes; and impairs NF-κB signaling to induce the apoptosis of TNBC cells (Song et al., 2020). Additionally, in breast cancer, icariin reduces the protein expression of JNK and the phosphorylation levels of JNK and c-JUN, ultimately inducing cell apoptosis. Molecular docking has shown that icariin can bind c-Jun through hydrogen bonds, with a binding affinity of −11 kcal mol−1 (Gao et al., 2023).
In addition to icariin, other active ingredients in epimedium, such as baohuoside-1, also target apoptosis to exert anticancer effects. Baohuoside-1 inactivates the mTOR signaling pathway in hepatocellular carcinoma (HCC) in a dose-dependent manner, reduces Bcl-2 expression, and enhances cleaved caspase3 and Bax expression (Guo et al., 2020).
2.1.2.4 Apigenin
Apigenin is a flavonoid compound that has significant preventative effects against HCC, CRC, breast cancer, gastric cancer and prostate cancer. In HCC, apigenin activates apoptosis through different mechanisms. First, it inhibits the classic signaling pathway of apoptosis, the PI3K/AKT pathway, resulting in the significant downregulation of Bcl-2 expression and significant upregulation of Bax and Bak expression (Yang et al., 2018). Second, apigenin stimulates the expression of death receptor 5 (DR5), which interacts with TRAIL and triggers canonical apoptotic signaling (Kang et al., 2018). Moreover, apigenin can enhance the anti-HCC effect of sorafenib by inducing apoptosis, but the detailed mechanism has yet to be been reported (Şirin et al., 2020).
Apigenin can decrease 5-fluorouracil (5-FU) resistance in CRC cells by targeting apoptosis; mechanistically, it promotes the expression of p53 and the production of ROS (Yang C. et al., 2021). Moreover, Hamadou et al. reported that apigenin and its derivatives induce the apoptosis of CRC cells through p53 (Hamadou et al., 2021).
2.2 Necroptosis
Necroptosis is a regulated form of necrosis, a process of self-destruction that is activated by preventing apoptosis. Necroptosis differs from apoptosis and other forms of PCD because it is independent of caspase activity and is primarily mediated by receptor interacting serine/threonine protein kinase 1 (RIPK1), RIPK3, and mixed lineage kinase domain-like (MLKL) (Gong et al., 2019).
2.2.1 Flavonoids
2.2.1.1 Apigenin, acacetin and fisetin
Research on the role of natural flavonoids in necroptosis is limited and has focused mainly on the RIPK family and MLKL. Apigenin significantly inhibits the viability of malignant mesothelioma cells by activating RIPK3 and MLKL, increasing ROS, and thereby promoting the occurrence of necroptosis (Lee YJ. et al., 2020). Similarly, in breast cancer, acacetin can promote the expression of RIPK1 and RIPK3 and the production of ROS, resulting in cell necroptosis (Kandhari et al., 2023). Liu et al. reported that fisetin increases the expression of Z-DNA-binding protein 1, a key stimulator of cell necroptosis, activates the RIPK3/MLKL pathway and induces necroptosis in ovarian cancer (Liu et al., 2022; Lomphithak et al., 2023).
2.2.1.2 Quercetin, kaempferol, isobavachalcone and gambogic acid
Quercetin and kaempferol, which are natural flavonoids, when used in combination with Smac mimetics, induce the proteasomal degradation of cellular inhibitor of apoptosis proteins one and 2 (cIAP1/2) and synergistically kill cholangiocarcinoma cells through RIPK1/RIPK3/MLKL-mediated necroptosis (Lomphithak et al., 2023). The treatment of TNBC cells with isobavachalcone downregulates AKT and phosphorylated AKT (p-AKT) expression and upregulates RIPK3, p-RIPK3 and MLKL expression, subsequently inducing necroptosis (Wu CZ. et al., 2022). Gambogic acid derived from Garcinia hanburyi trees promotes gastric cancer cell death through necroptosis; regarding the mechanism of action, gambogic acid increases the p-RIPK1/RIPK1, p-RIPK3/RIPK3, and p-MLKL/MLKL ratios; enhances necrosome complex formation; activates the downstream effector proteins phosphoglycerate mutase family member 5 (PGAM5) and Drp-1 (Chen et al., 2024).
2.2.2 Quinones
2.2.2.1 Shikonin
Owing to their “dominant skeletons” in medicinal chemistry, the advantages of quinones in cancer treatment are gradually becoming apparent. Shikonin is derived from the Chinese herb Lithospermum erythrorhizon. Studies have shown that shikonin induces necroptosis in pancreatic cancer cells. Importantly, the mechanism of action might be cell-type specific. Shikonin functions mainly through RIPK1 in the PANC-1 cell line and has no obvious effect on RIPK3; however, in AsPC-1 cells, it targets RIPK3 to induce cell necroptosis (Chen et al., 2017), and in nasopharyngeal carcinoma, shikonin upregulates both RIPK1 and RIPK3 expression and MLKL expression to promote necroptosis (Liu et al., 2019).
2.2.2.2 Emodin
Emodin is an anthraquinone compound, and studies of glioma have shown that it increases the protein levels of TNF-α, RIPK1, RIPK3 and MLKL in vivo and in vitro, resulting in cell necroptosis (Zhou et al., 2020). In prostate cancer, emodin treatment causes necroptosis-mediated cell death by stimulating the expression of HSP90 and PGAM5, a central modulator of mitochondrial fission, leading to the massive generation of ROS and necroptosis (Zhou et al., 2023).
2.2.3 Terpenoids
2.2.3.1 Oridonin and paclitaxel
Oridonin is a diterpenoid derived from Rabdosia rubescens, and in renal cancer, it can upregulate the expression of RIPK1 and RIPK3, promoting necroptosis (Zheng et al., 2018).
c-Src phosphorylates caspase 8 at tyrosine 380 in lung adenocarcinoma cells, and paclitaxel triggers necroptosis through p-caspase 8. After paclitaxel treatment, p-caspase 8 interacts with RIPK1 and RIPK3, which reinforces RIPK1 and RIPK3 binding, resulting in the activation of the RIPK1/RIPK3/MLKL pathway (Diao et al., 2016).
2.2.3.2 IDOAMP
The Rosin derivative IDOAMP is a diterpenoid. In prostate cancer, IDOAMP inhibits the binding of Aurora A to RIPK1 and RIPK3, and these interactions block necrosome activation. Moreover, IDOAMP stimulates the binding of RIPK1 to RIPK3 or MLKL and the phosphorylation of RIPK1, RIPK3 and MLKL, thereby inducing necroptosis in cells (Xu H. et al., 2022).
2.2.4 Other natural compounds
2.2.4.1 Progenin III, gallic acid, tanshinol a and matrine
Many other natural medicines from plants have clinical application value because they play roles in the mechanism of necroptosis. For example, the natural medicine progenin III derived from Raphia vinifera P. Beauv induces necroptosis in leukemia cells; however, the specific mechanism has not yet been elucidated (Mbaveng et al., 2020). In cervical cancer, gallic acid causes cancer cell death through the necroptosis pathway (Tang and Cheung, 2019). Interestingly, tanshinol A induces necroptosis via MLKL but not through RIPK1 or RIPK3 in lung cancer; it causes the massive production of ROS, increases the phosphorylation level of MLKL and promotes MLKL transfer to the plasma membrane to execute necroptosis (Liu X. et al., 2020). Matrine, an alkaloid derived from Sophora flavescens, upregulates RIPK3 expression and promotes MLKL translocation from the cytoplasm to the cell membrane, ultimately inducing necroptosis in cholangiocarcinoma (Xu et al., 2017).
2.3 Pyroptosis
Pyroptosis, also known as inflammatory PCD, is characterized by the formation of plasma membrane pores by gasdermin family proteins. Some studies have shown that pyroptosis is regulated by multiple signaling pathways and noncoding RNAs and can control cancer cell proliferation, invasion and metastasis (Fang et al., 2020).
2.3.1 Flavonoids
2.3.1.1 Baicalin and nobiletin
In diffuse large B-cell lymphoma cells, baicalin dose-dependently regulates the production of ROS and increases the expression of NACHT, LRR and PYD domains-containing protein 3 (NLRP3), gasdermin-D N terminal (GSDMD-N), and GSDME-N, leading to pyroptosis (Lu et al., 2023). A study by Liu et al. revealed that baicalin significantly promotes pyroptosis in gastric cancer through the generation of ROS and the upregulation of NLRP3 and GSDMD-N expression; further research revealed that baicalin also increases the protein level of NF-κB and activates this pathway to trigger pyroptosis (Liu J. et al., 2024). Nobiletin is a flavonoid isolated from citrus fruits, and the treatment of ovarian cancer cells with nobiletin significantly reduces the mitochondrial membrane potential and induces ROS production, promoting GSDMD/GSDME-mediated pyroptosis (Zhang et al., 2020b).
2.3.1.2 Chalcones, kaempferol and luteolin
Chalcones belong to the flavonoid family and are commonly found in edible and medicinal plants. After structural modification, chalcone derivatives significantly inhibit lung cancer cell proliferation and growth through the ROS-mediated pyroptosis pathway (Zhu et al., 2018). In gastric cancer, kaempferol, like baicalin, activates the NF-κB pathway, thereby upregulating the expression of caspase-1, GSDMD, and IL-18 (Qi et al., 2024). Luteolin significantly promotes the expression of caspase 1, GSDMD and IL-1β in CRC cells, thereby inducing pyroptosis (Chen Y. et al., 2022).
2.3.1.3 Quercetin and isobavachalcone
As previously mentioned, studies have shown that in cancer treatment, quercetin triggers cell apoptosis; in addition, recent reports have indicated that quercetin affects pyroptosis. For example, quercetin induces pyroptosis in gastric cancer cells by increasing the expression of pyroptosis markers such as GSDMD, GSDME, and NLRP3 in a concentration-dependent manner (Rong et al., 2023). In contrast, isobavachalcone prevents glioblastoma development by inhibiting pyroptosis and triggering apoptosis. Mechanistically, molecular docking and surface plasmon resonance assays revealed that isobavachalcone directly binds estrogen receptor α (ERα), an NLRP3 transcription factor, thus inhibiting NLRP3 transcription and the expression of caspase 1, GSDMD and IL-1β (Wu Y. et al., 2022).
2.3.1.4 Alpinum isoflavone, icariin and galangin
Alpinum isoflavone, a flavonoid isolated from derriseriocarpa, inhibits HCC cell proliferation and metastasis by inducing pyroptosis through NLRP3 inflammasome activation (Zhang Y. et al., 2020). circCACNA2D1 expression is downregulated in gastric cancer; it sponges miR-223-3p, and miR-223-3p targets NLRP3. Icariin modulates this signaling axis and induces pyroptosis (Zhang F. et al., 2022). In glioblastoma, galangin suppresses cell proliferation by inducing pyroptosis through the upregulation of GSDME expression, with no effect on GSDMD expression (Kong et al., 2019).
2.3.2 Alkaloids
2.3.2.1 Camptothecin, matrine, chaetoglobosin E, ajmalicine and sophorin A
Alkaloids are a class of nitrogen-containing alkaline organic compounds that exist mainly in plants. Among these compounds, research has confirmed that camptothecin and matrine have antitumor effects but that their mechanisms of action are different. Chaetoglobosin E activates pyroptosis by targeting polo-like kinase one in esophageal squamous cell carcinoma, potentially suppressing GSDME activation (Chen JH. et al., 2022). Ajmalicine is a small-molecule alkaloid, and Sun et al. reported that it promotes pyroptosis in the H22 liver cancer cell line, mainly by increasing ROS production and upregulating the expression of caspase 3 and GSDME-N (Sun et al., 2024). Sophorin A, a novel matrine derivative isolated from Sophora japonica, promotes NSCLC cell death by inducing pyroptosis through NLRP3/caspase-1/GSDMD signaling pathway activation (Luo et al., 2023).
2.3.3 Terpenoids
2.3.3.1 Tanshinone I and tanshinone IIA
Tanshinones, a class of compounds extracted from the roots of Danshen, include tanshinone I, tanshinone IIA, and cryptotanshinone. In gastric cancer, tanshinone I suppresses cisplatin-resistant cell growth by activating the NF-κB/caspase-3/8/GSDME signaling pathway and stimulating pyroptosis (Wang G. et al., 2024). Treatment with tanshinone IIA decreases the expression of miR-125b, which targets the transcription factor foxp3 and suppresses caspase-1 and GSDMD-N expression, ultimately increasing pyroptosis and inhibiting the proliferation of nasopharyngeal carcinoma cells; however, the relationship between foxp3 and caspase-1/GSDMD is unclear (Wang Y. et al., 2021). In cervical cancer, tanshinone IIA stimulates the expression of miR-145, GSDMD and IL-1β to cause HeLa cell pyroptosis, but how miR-145 modulates pyroptosis-related gene expression needs to be clarified (Tong et al., 2020).
2.3.3.2 Triptolide
Triptolide is extracted from the plant Tripterygium wilfordii, and studies have shown that it can reduce the protein level of mitochondrial hexokinase II, which blocks the translocation of BAD and BAX proteins to mitochondria and the activation of caspase 3, thus preventing the cleavage of GSDME and pyroptosis to eliminate head and neck cancer cells (Cai J. et al., 2021). On this basis, Wang et al. modified the dosage form of triptolide and applied it to melanoma; triptolide nanoparticles more efficiently enhanced caspase-3-mediated GSDME cleavage and induced cell pyroptosis (Wang et al., 2023).
2.3.3.3 Mallotucin D, Paclitaxel and Cucurbitacin B.
Mallotucin D is a diterpenoid compound from Croton crassifolius that increases the expression of NLRP3, the GSDMD-N/GADMD ratio and mature IL-1β/pro-IL-1β ratio, thus inducing pyroptosis in HCC (Dai et al., 2022). Paclitaxel has been widely used in cancer treatment; it induces caspase 3 activation and GSDME cleavage in a time-dependent manner in lung cancer (Zhang et al., 2019). Another natural triterpenoid, cucurbitacin B, induces NSCLC cell pyroptosis by promoting the activation of the NLRP3 inflammasome. Further investigations revealed that cucurbitacin B directly binds to Toll-like receptor 4 (TLR4) and stabilizes it, while TLR4 activates the NLRP3 inflammasome. Moreover, cucurbitacin B promotes mitochondrial ROS generation and thus triggers GSDMD-dependent cell pyroptosis (Yuan et al., 2021).
2.4 Ferroptosis
Ferroptosis is a newly discovered type of PCD, first proposed by Dixon et al., in 2012; its mechanism is related mainly to iron metabolism, which is mediated by transferrin; the amino acid antioxidant system, which is mediated by SLC7A11; and lipid peroxides, which are mediated by GPX4 (Zhang C. et al., 2022). The active ingredients in natural plants that target tumor cell ferroptosis are shown in Figure 3.
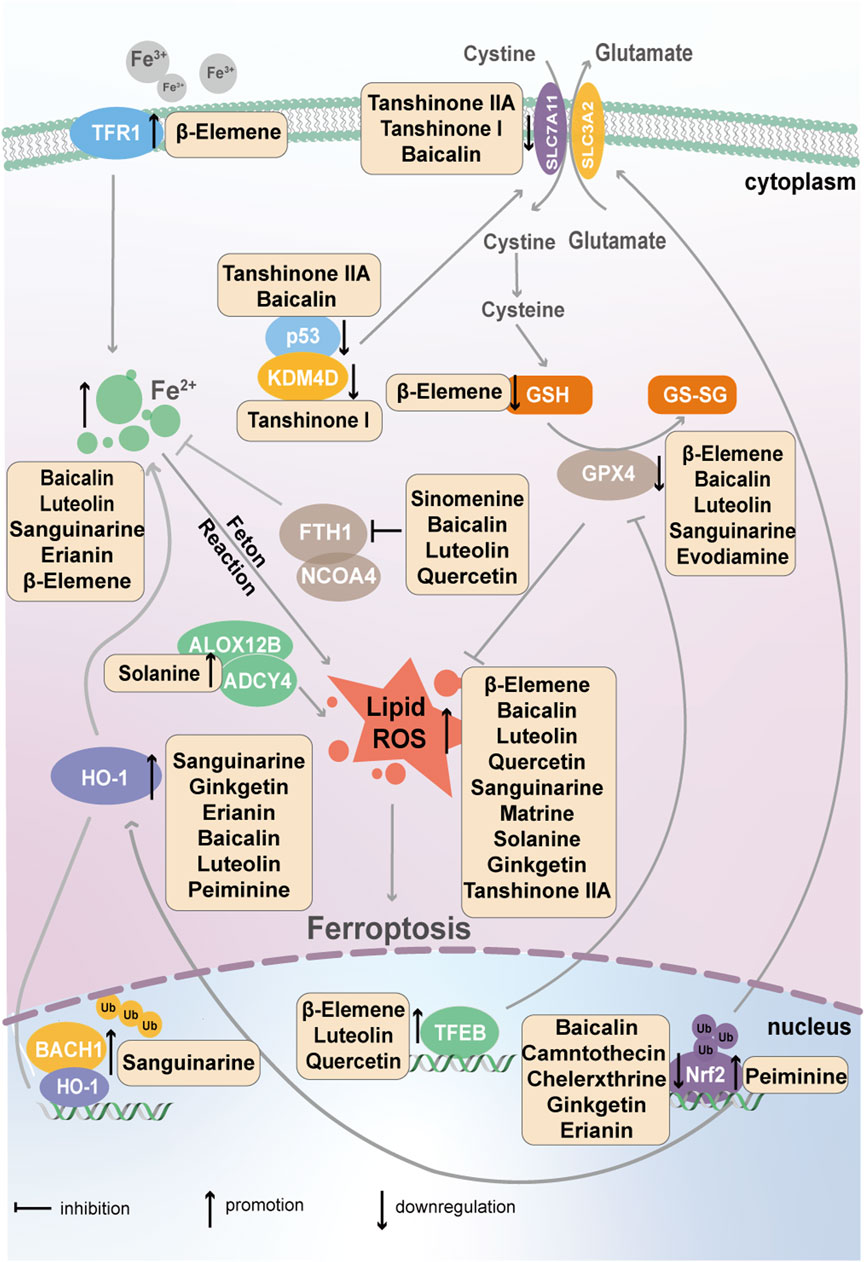
Figure 3. Natural active ingredients from plants, such as flavonoids, terpenoids and alkaloids, target the ferroptosis of cancer cells via transferrin, SLC7A11 and GPX4.
2.4.1 Terpenoids
2.4.1.1 β-Elemene
Studies have shown that β-Elemene can directly bind to transcription factor EB (TFEB), a master modulator of lysosome activation, and stimulate its activation and nuclear translocation, which in turn leads to GPX4 lysosomal degradation in NSCLC. As a key player in ferroptosis, GPX4 reduction promotes ferroptosis in cells, accompanied by the generation of massive amounts of ROS (Zhao et al., 2023). Moreover, β-Elemene enhances the sensitivity of many targeted drugs by triggering ferroptosis. Xu et al. showed that the combination of β-Elemene and erlotinib induces ferroptosis in EGFR-mutant NSCLC cells by promoting the expression of the lncRNA H19; however, the regulatory mechanism between lncRNA H19 and ferroptosis is unclear (Xu et al., 2023). In CRC, β-Elemene combined with cetuximab induces iron-dependent ROS accumulation, GSH depletion, the upregulation of transferrin expression, and lipid peroxidation, thereby resulting in ferroptosis (Chen et al., 2020a).
2.4.1.2 Tanshinones I and tanshinone IIA
In gastric cancer, molecular docking revealed lysine-specific demethylase 4D (KDM4D) as the target of tanshinone I; this interaction reduces the protein level of KDM4D, which directly binds to p53 and suppresses its transcriptional activation of the downstream gene SLC7A11, ultimately leading to ferroptosis (Xia et al., 2023). Additionally, in gastric cancer, tanshinone IIA significantly activates lipid peroxidation and downregulates the expression of SLC7A11 by regulating p53 (Guan et al., 2020), leading to ferroptosis and decreased cell stemness (Ni et al., 2022).
In breast cancer, tanshinone IIA induces ferroptosis by reducing the SUMOylation of SLC7A11 and increasing its ubiquitin-mediated degradation. Further studies revealed that tanshinone IIA inhibits the expression of lysine-specific demethylase 1A (KDM1A), which epigenetically activates protein inhibitor of activated STAT4 (PIAS4) transcription, decreases the direct interaction between PIAS4 and SLC7A11 and inhibits SLC7A11 SUMOylation (Luo et al., 2024).
2.4.2 Flavonoids
2.4.2.1 Baicalin
Many investigations have revealed that baicalin can facilitate the anticancer effects of chemotherapeutic drugs through ferroptosis. For example, baicalin increases the sensitivity of gastric cancer cells to 5-fluorouracil through ROS-mediated ferroptosis (Yuan et al., 2023) and the sensitivity of gastric cancer cells to oxaliplatin by inducing ferroptosis via the p53/SLC7A11 pathway (Shao et al., 2024). In bladder cancer and oral squamous cell carcinoma, baicalin targets the key protein of ferroptosis, ferritin heavy chain 1 (FTH1), inhibits its expression and induces ferroptosis (Wen et al., 2024; Kong et al., 2021). Nrf2 is a transcription factor that stimulates the expression of many cytoprotective genes, such as heme oxygenase-1 (HO-1), to control the cellular oxidative stress response; however, HO-1 has dual roles in ferroptosis. In osteosarcoma cells, baicalin binds Nrf2 and promotes its ubiquitin-mediated degradation, leading to the downregulation of SLC7A11 and GPX4 expression and triggering ferroptosis (Wen RJ. et al., 2023).
2.4.2.2 Luteolin
As a commonly used natural medicine, luteolin has been studied in the field of ferroptosis. In clear cell renal cell carcinoma, luteolin may promote the accumulation of unstable iron and heme degradation by directly binding HO-1 and stabilizing it, leading to the Fenton reaction and lipid peroxidation, thereby inducing ferroptosis (Han et al., 2022). Luteolin can also increase ferroptosis by regulating TFEB, increasing TFEB expression and nuclear translocation and subsequent FTH1 lysosomal degradation in prostate cancer (Fu et al., 2024). In colon cancer, luteolin combined with erastin targets the tumor suppressor hypermethylated in cancer 1 (HIC1) gene, which inhibits GPX4 expression, thereby inducing ferroptosis; however, HIC1 regulation underlying ferroptosis remains unclear (Zheng et al., 2023).
2.4.2.3 Quercetin
Like luteolin, quercetin activates TFEB to cause FTH1 lysosomal degradation, thereby inducing ferroptosis in HCC, CRC and breast cancer cells. In addition, the generation of ROS caused by quercetin synergistically leads to lipid peroxidation and promotes ferroptosis (Wang ZX. et al., 2021; An and Hu, 2022).
2.4.3 Alkaloids
2.4.3.1 Sanguinarine
Research on natural alkaloid medicines and ferroptosis has increased annually. Sanguinarine, a classic representative of alkaloid drugs, has been widely used in cancer treatment. Ferroptosis can be induced by ROS production, a widely studied phenomenon. ROS, especially H2O2, reduce SLC7A11 and GPX4 expression in cervical cancer and prostate cancer (Liu S. et al., 2024; Alakkal et al., 2022); additionally, ROS stimulate BTB domain and CNC homolog 1 (BACH1) degradation by decreasing the expression of ubiquitin carboxyl-terminal hydrolase 47 (USP47), which can deubiquitinate BACH1. BACH1 binds to the HO-1 enhancer and suppresses its transcription; therefore, sanguinarine upregulates HO-1 expression, causing intracellular iron overload and ultimately inducing ferroptosis (Liu S. et al., 2024). In addition, sanguinarine facilitates the ubiquitin-mediated degradation of GPX4 through the E3 ligase STIP1 homology and U box-containing protein 1 (STUB1) in NSCLC cells and triggers ferroptosis (Xu R. et al., 2022).
2.4.3.2 Camptothecin, chelerythrine, peiminine and matrine
Some studies have reported that camptothecin, peiminine, and chelerythrine target Nrf2 and promote ferroptosis. Camptothecin and chelerythrine have been shown to reduce Nrf2 expression in HCC and ovarian cancer, respectively (Zhou et al., 2024; Elkateb et al., 2023). In contrast, peiminine increases Nrf2 and downstream HO-1 expression in breast cancer (Yi et al., 2024). Intriguingly, matrine triggers ferroptosis in cervical cancer via piezo1 but has no effect on SLC7A11 or the transferrin receptor (TFR). The activated piezo1 channel enhances the influx of Ca2+, resulting in ROS generation and ferroptosis (Jin et al., 2024).
2.4.3.3 Solanine, sinomenine and evodiamine
Solanine promotes the expression of arachidonate 12-lipoxygenase, 12R-type (ALOX12B) by enhancing its interaction with ADCY4, which stabilizes ALOX12B, leading to lipid peroxidation and ferroptosis in CRC (Ma et al., 2024). Sinomenine is an active alkaloid from Sinomenium acutum, and its derivative facilitates interactions between nuclear receptor coactivator 4 (NCOA4) and FTH1, accelerating Fe2+ release and ultimately promoting ferroptosis in colon cancer cells (Zhu et al., 2024). Yu et al. revealed that evodiamine can induce ferroptosis in prostate cancer through the upregulation of GPX4 expression (Yu et al., 2023).
2.4.4 Other natural compounds
Lou et al. reported that ginkgetin enhances the anticancer effect of cisplatin and augments the unstable iron pool and lipid peroxidation, two hallmarks of ferroptosis, via Nrf2/HO-1 signaling inactivation in NSCLC (Lou et al., 2021). Erianin, a natural dibenzyl compound extracted from Dendrobium chrysotoxum (Dong et al., 2020), has also been found to downregulate Nrf2 and HO-1 expression to induce ferroptosis in bladder cancer (Xiang Y. et al., 2021). In lung cancer, erianin targets calmodulin and promotes its expression, and elevated calmodulin level facilitate Ca2+ and Fe2+ uptake, causing ferroptosis (Chen et al., 2020b).
2.5 Autophagy
Autophagy is a process in which cells, under the fine regulation of a series of genes, degrade their own damaged organelles and macromolecules in lysosomes to achieve metabolic needs and the renewal of certain organelles (Kim and Lee, 2014). The natural plant compounds that target tumor cell autophagy are shown in Figure 4.
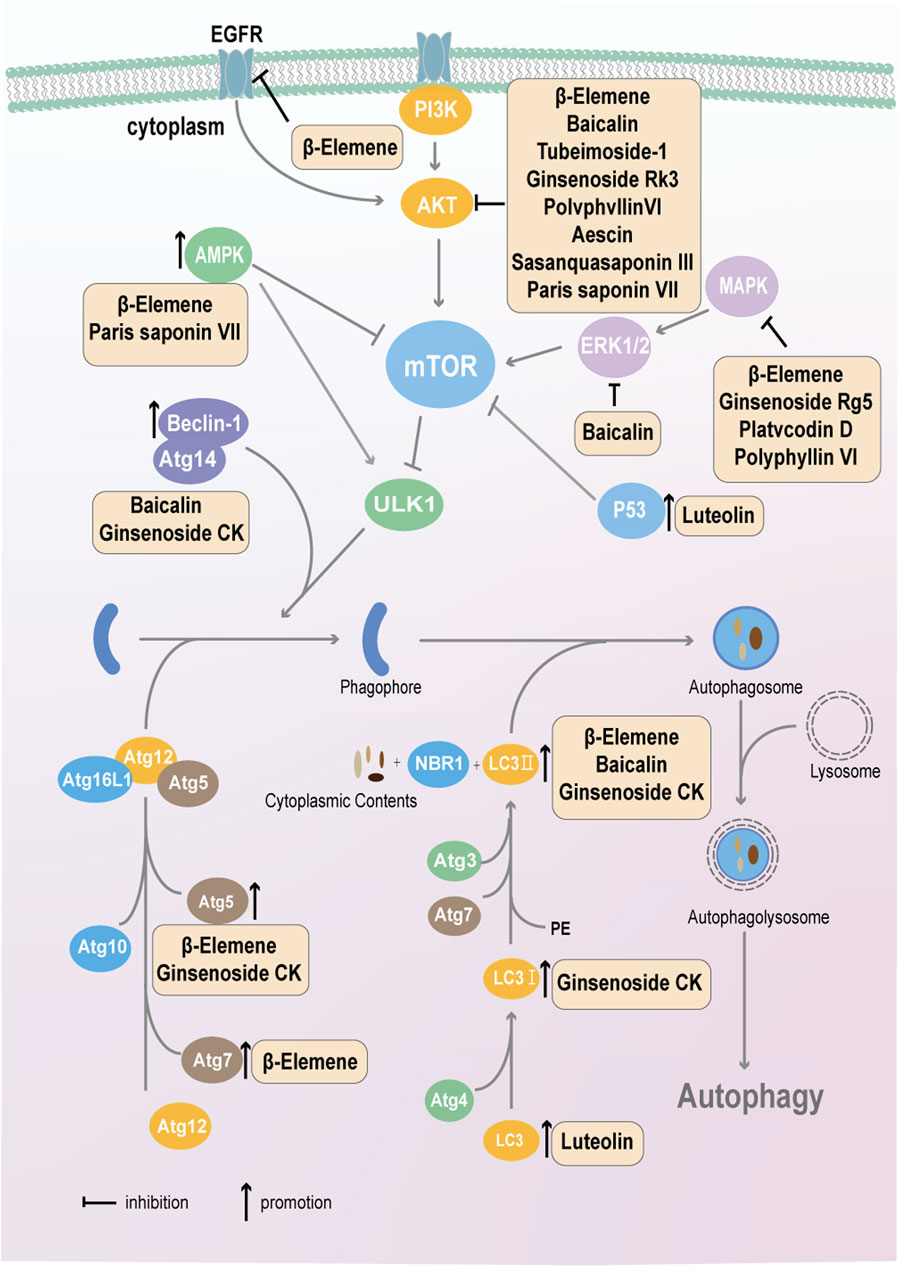
Figure 4. Natural active components from plants, such as flavonoids, terpenoids and saponins, target autophagy in cancer cells via the mTOR-ULK1-autophagosome pathway.
2.5.1 Terpenoids
2.5.1.1 β-Elemene
β-Elemene plays a role in promoting autophagy in cancer cells; it significantly promotes the conversion of LC3Ⅰ to LC3Ⅱ and then the formation of autophagosomes to promote autophagy in breast cancer and Ewing sarcoma (Guan et al., 2014; Zhang T. et al., 2023). In addition to directly targeting biomarkers of autophagy, β-Elemene also acts on upstream pathways, such as the PI3K/AKT/mTOR, MAPK/ERK, AMPK, and EGFR/AKT pathways, to control autophagy. In NSCLC, gastric cancer and renal cell carcinoma, β-Elemene significantly suppresses the activation of the PI3K/AKT/mTOR pathway, and in renal cell carcinoma, it also inhibits the MAPK/ERK pathway, thereby promoting the autophagy of cancer cells (Liu et al., 2012a; Zhan et al., 2012a; Liu et al., 2011). Furthermore, β-Elemene stimulates AMPK, thereby reducing the p-mTOR/mTOR ratio and ultimately causing autophagy in CRC cells (Wang et al., 2022).
A β-Elemene isopropanolamine derivative reduces the expression of 6-phosphofructo-2-kinase/fructose-2,6-bisphosphatase 4 (PFKFB4), a key enzyme in glycolysis, thus inhibiting glycolysis and triggering autophagy in melanoma cells (Jalal et al., 2022). β-Elemene sensitizes cells to chemotherapy drugs by targeting autophagy. For example, in NSCLC, β-Elemene enhances the effect of gefitinib by inducing autophagy; mechanistically, it decreases the protein level of the m6A methyltransferase METTL3 and the m6A methylation level in resistant cells, downregulates the expression of ATG5 and ATG7, which are critical components of the autophagy pathway, and ultimately inhibits autophagy (Liu et al., 2020e). β-Elemene also reverses resistance to gefitinib in GBM through the inactivation of the EGFR/AKT signaling pathway and activation of autophagy and enhances the anticancer effect of 5-fluorouracil in CRC by triggering autophagy; however, the detailed mechanism remains unknown (Mu et al., 2016; Zhang et al., 2020d).
2.5.2 Flavonoids
2.5.2.1 Baicalin
Baicalin might induce autophagy in osteosarcoma cells by directly binding to PI3Kγ and inhibiting the PI3K/AKT/mTOR, ERK1/2 and β-catenin signaling pathways (Pang et al., 2022a). Similarly, in bladder cancer, autophagy is achieved by inhibiting AKT expression and activity (Lin et al., 2013a). Additionally, baicalin can trigger autophagy in HCC cells and upregulate CD147 expression, which increases Beclin one expression through the activation of the PI3K/AKT pathway (Zhang et al., 2012a; Gou et al., 2009a). However, in nasopharyngeal carcinoma, baicalin downregulates LC3Ⅱ and Beclin 1 expression and inhibits autophagy, thereby reversing radioresistance (Wang et al., 2020).
2.5.2.2 Luteolin
The role of luteolin in cancer autophagy may be controversial; even in one GBM type, luteolin has been reported to have dual functions in autophagy. Chakrabarti et al. reported that luteolin inhibits the expression of protein kinase C alpha type (PKCα) and autophagy (Chakrabarti and Ray, 2016); however, Lee et al. reported that it triggers autophagy and promotes cell survival but induces apoptosis to kill cells (Lee HS. et al., 2021). In liver cancer, luteolin stimulates autophagic flux, and one study reported that luteolin enhances cell viability (Lee and Kwon, 2019), whereas another study reported that it leads to TRAIL-induced apoptosis and cell death, possibly due to the use of distinct cell lines (Nazim and Park, 2019a).
There are also reports that luteolin promotes autophagy via p53; however, activated autophagy has no effect on the apoptosis or growth of colon cancer cells (Yoo et al., 2022). In TNBC, RNA sequencing and molecular docking demonstrated that luteolin targets serum and glucocorticoid-induced protein kinase 1 (SGK1) and inhibits its expression. The downregulation of SGK1 expression leads to decreased phosphorylation and the increased stability of the transcription factor forkhead box O 3a (FOXO3a), which activates the expression of bcl2/adenovirus e1b-interacting protein 3 (BNIP3), an inducer of autophagy through interactions with LC3 (Wu et al., 2023). Moreover, luteolin may increase cisplatin sensitivity by inhibiting autophagy in ovarian cancer (Liu et al., 2018). In summary, the regulatory effect of luteolin on cancer autophagy needs to be viewed objectively on the basis of different types of cancer and cell lines.
2.5.3 Saponins
2.5.3.1 Tubeimoside-1, ginsenoside Rk3, polyphyllin VI, aescin, sasanquasaponin ΙΙΙ, ginsenoside Rg5 and platycodin D
Saponins exert their anticancer effects mainly by suppressing cell proliferation and migration, inducing autophagy and promoting cancer cell apoptosis. In terms of mechanism, many reports have shown that saponin natural products target the upstream signaling pathway of autophagy. Tubeimoside-1 in breast cancer, ginsenoside Rk3 in HCC, polyphyllin VI in NSCLC, aescin in lung cancer, and sasanquasaponin ΙΙΙ in melanoma mainly target the PI3K/AKT/mTOR signaling pathway to induce cell autophagy; autophagy activated by tubeimoside-1 protects cells from death (Jiang et al., 2019), whereas the other four compounds result in cell death (Qu et al., 2023; Singh et al., 2023; Liang et al., 2019; Teng et al., 2019). The MAPK pathway also plays a vital role in cell autophagy. Research has revealed that ginsenoside Rg5 in gastric cancer, platycodin D in colon cancer, and polyphyllin VI in NSCLC promote cell autophagy and inhibit cell proliferation by inactivating the MAPK signaling pathway (Teng et al., 2019; Liu and Fan, 2019; Han et al., 2024).
2.5.3.2 Paris saponin VII and ginsenoside CK
Paris saponin VII can activate the AMPK pathway, a signaling pathway that is opposite to AKT and MAPK, thereby inhibiting the mTOR signaling pathway and causing autophagy and cell death in NSCLC (Xiang YC. et al., 2021). In addition, Xiang et al. reported that Paris saponin VII directly interacts with the MST2-MOB1-LATS1 complex, increasing their interaction, thereby promoting the MOB1-mediated phosphorylation of LATS1 and LATS1-mediated phosphorylation and degradation of YAP, and that the inhibition of YAP ultimately activates the Hippo pathway and autophagy in breast cancer cells (Xiang et al., 2022). Ginsenoside CK, a saponin derivative, can trigger cell autophagy by downregulating the expression of SQSTM1/p62, a negative modulator of autophagy, and promoting the expression of Beclin-1, ATG5 and LC3-II (Yin et al., 2021).
2.6 The interrelationships between different PCD pathways in cancer cells
In recent years, it has been known that various PCD pathways can crosstalk with each other through regulatory signaling pathways and molecules. For instance, many studies have shown that ferroptosis plays an important role in the communications between different types of PCD. Iron overload is one of the causes of ferroptosis, it leads to the opening of the mitochondrial permeability transition pore, enhanced RIPK1 phosphorylation and ultimately necroptosis (Zhou et al., 2021). Ferroptosis and pyroptosis are antagonistic to each other, their interplay is regulated by cellular localization of 3-hydroxy-3-methylglutaryl-coenzyme A reductase (HMGCR) (Wang H. et al., 2024). Some natural plant compounds can simultaneously target multiple PCD pathways and lead to cancer cell death.
2.6.1 Terpenoids
2.6.1.1 β-Elemene
As mentioned above, β-Elemene can significantly inhibit PI3K/AKT/mTOR, MAPK pathways to induce apoptosis and autophagy, and combination of it with autophagy inhibitors can significantly enhance its anti-tumor effect (Liu et al., 2012b; Zhan et al., 2012b). Moreover, β-Elemene could reduce GSH synthesis and increase ROS to promote cancer cell apoptosis and ferroptosis (Chen et al., 2020a).
2.6.1.2 Paclitaxel
Paclitaxel can increase PARP expression (Yu-Wei et al., 2020), and PARP is involved in TNF-induced necroptosis, therefore, upregulated PARP plays a pivotal role in promoting cancer cell apoptosis and necroptosis (Khing et al., 2019). Furthermore, the application of paclitaxel activates caspase-3/7 in multiple types of cancer cells, thereby inducing apoptosis and activating GSDME to cause pyroptosis (Zhang Y. et al., 2022).
2.6.2 Flavonoids
2.6.2.1 Luteolin
Luteolin simultaneously modulates cancer cell apoptosis and autophagy via two different mechanisms. First, it regulated p53 pathway and triggered apoptosis and autophagy of colon cancer cell (Yoo et al., 2022). Second, it activates JNK pathway and upregulates death receptor 5 to inducing apoptosis and autophagy (Nazim and Park, 2019b; Wu B. et al., 2020).
2.6.2.2 Baicalin
Similar to β-Elemene, baicalin also triggers cancer cell apoptosis and autophagy by inhibiting the PI3K/AKT/mTOR pathway (Sui et al., 2020c; Pang et al., 2022b; Lin et al., 2013b; Zhang et al., 2012b; Gou et al., 2009b), but baicalin could also induce cell apoptosis via activating the AMPK pathway (Jia et al., 2022b).
2.6.2.3 Apigenin
Apigenin treatment can lead to lots of ROS and induce apoptosis and necroptosis of cancer cells (Yang C. et al., 2021; Lee YJ. et al., 2020). In addition, it inhibits the PI3K/AKT/mTOR pathway to promote apoptosis and autophagy of HCC cells (Yang et al., 2018).
3 Conclusion
Cancer is a malignant disease caused by uncontrolled cell proliferation, and its pathogenic mechanisms are relatively complex and primarily involve the activation of oncogenes and the inactivation of tumor suppressor genes. In recent decades, the number of cancer cases and deaths has markedly increased annually; however, although the clinical management of cancer is constantly improving, the survival time and quality of life of cancer patients are still poor. Traditional Chinese medicines or a variety of monomeric herbal extracts, to a certain extent, strongly inhibit the occurrence and development of some cancers (Zou et al., 2024). Therefore, the use of active ingredients from plants to treat cancer has gradually attracted the interest of many researchers. These compounds are diverse and include terpenoids, alkaloids, flavonoids, etc. Using modern pharmaceutical technology to modify the formulation of these compounds enhances their anticancer effects; therefore, in addition to most herbal monomers, this review also introduces a few formulations containing monomers and their derivatives.
The antitumor mechanisms of these active ingredients are varied, and among these mechanisms, PCD is one of the most common and plays a pivotal role in the development of cancer. To date, there are many new research achievements on PCD in tumors. For instance, some groups have investigated the immunogenicity of zeolitic imidazolate framework-8 (ZIF-8) nanoparticles in tumor therapy and found that ZIF-8 nanoparticles can induce pyroptosis through the caspase-1/GSDMD pathway, leading to cancer cell necrosis, reprograming of the tumor microenvironment and activation of antitumor immunity (Ding et al., 2023). This research provides innovative ideas for the biomedical application of ZIF-8, and the combination of ZIF-8 with active plant ingredients that target cancer cell pyroptosis may show a synergistic effect. Similarly, studies have investigated the role of ferroptosis in tumor immunity. Drugs can induce ferroptosis in malignant cells but also simultaneously trigger ferroptosis in immune cells, which may compromise anti-tumor immunity. Interestingly, Li et al. found that the compound N6F11 could selectively induce GPX4 degradation and ferroptosis in pancreatic cancer cells and initiate CD8 T-cell anti-tumor immunity but had no impairment on immune cells. Mechanistically, N6F11 interacts with the E3 ubiquitin ligase TRIM25 and promotes the K48-linked ubiquitination and proteasomal degradation of GPX4 (Li J. et al., 2023). Immunotherapy is a popular treatment for cancer at present. It mainly includes immune checkpoint inhibitor (ICI) therapy, adoptive cell therapies (TILs, TCR-T and CAR-T) and tumor vaccines. However, the response rate of immunotherapy is still relatively low. To address this issue, the combination of immunotherapy with other drugs, for example, the combination of the commonly used PD-1/PD-L1 inhibitors and mature natural plant products, including luteolin, baicalin and β-Elemene (Lee J. et al., 2021), is a promising research direction.
Active ingredients from plants that target different forms of PCD can significantly suppress cancer cell viability or enhance the anticancer effect of chemotherapeutics and radiotherapy, ultimately inhibiting the occurrence and development of cancer, indicating that they have great potential as antitumor drugs or can be combined with other management strategies to synergistically increase their effectiveness. In summary, cell apoptosis, autophagy and ferroptosis are the main forms of PCD targeted by plant natural compounds, whereas other forms of PCD, such as necroptosis and pyroptosis, have been less studied. Specifically, these drugs directly regulate key components in different PCD pathways or modulate their upstream signaling pathways to control PCD. All of these findings provide a reference for the pharmacological study of newly discovered plant-derived antitumor drugs.
Recently, RNA sequencing and molecular docking have accelerated the mechanistic investigation of natural antitumor drugs (Zhang J. et al., 2022); however, the specific mechanisms of many compounds are still unknown. Metabolomics is widely used in basic tumor research. Tumor metabolism and metabolites, such as lactate and lactylation, are hot topics in tumor research. Thus, metabolomics can be applied to the study of antitumor drugs, which may help us find targets and deepen and broaden our research into the mechanisms of antitumor drugs. For example, it is worth studying and exploring whether drugs can alter lactate metabolism, regulate the lactylation of key molecules in the PCD pathway, and subsequently cause cell death. In recent years, studies have shown that mitochondrial dysfunction can cause TCA cycle failure, leakage of the electronic respiratory chain and the production of ROS, which ultimately leads to abnormal signal transduction and cancer. Therefore, mitochondria have become a new target for the treatment of cancer, and many plant compounds have been developed to target mitochondria. For example, matrine has been shown to activate mitochondrial fission through the Mst1-JNK pathway, leading to mitochondrial dysfunction, oxidative stress and cell apoptosis, thereby exerting anti-liver cancer effects (Cao et al., 2019). Apomorphine can induce mitochondrial depolarization, calcium overload and energy deprivation to trigger the apoptosis of human choriocarcinoma cells (Lee JY. et al., 2020). Furthermore, another study revealed that apomorphine could significantly inhibit lipid peroxides and ferroptosis (Miyauchi et al., 2024). However, this research was conducted in fibroblasts, and whether apomorphine has the same effect in cancer needs to be validated.
In the development of natural plant products, due to their large numbers of targets, their transformation into clinical drugs are limited to a certain extent. Moreover, the yields of some extracted plant compounds are very low, making it difficult to meet market demands. Although some natural products have therapeutic activities, the high incidence of their toxic side effects limit their use. Furthermore, many compounds are potential candidate drugs for cancer treatment by regulating PCD, most of them are currently based on experiments in cells and mice, and there is a lack of clinical research evaluating the clinical effects of such compounds. Since natural active ingredients from plants can be effective potential drugs for clinical cancer treatment, in the future, more such compounds should be identified to expand the candidate drug pool. Furthermore, the modification and biosynthesis of these compounds or their formulations to achieve the requirements of clinical trials is also needed, and more attention should be given to these compounds in clinical trials so that they can be used to treat cancer patients and improve their clinical efficacy.
Author contributions
QL: Conceptualization, Formal Analysis, Funding acquisition, Writing–original draft, Writing–review and editing. YT: Formal Analysis, Writing–original draft, Writing–review and editing. JC: Funding acquisition, Supervision, Writing–original draft, Writing–review and editing. TX: Funding acquisition, Supervision, Writing–original draft, Writing–review and editing.
Funding
The author(s) declare that financial support was received for the research, authorship, and/or publication of this article. This research was supported by the “Pioneer” and “Leading Goose” R&D Program of Zhejiang (2022C03004), the Zhejiang Provincial Natural Science Foundation of China (LY21H160044); the National Natural Science Foundation of China (81902507); and the National Administration of Traditional Chinese Medicine-Zhejiang Province Co-construction Project (GZY- ZJ-KJ-24045).
Conflict of interest
The authors declare that the research was conducted in the absence of any commercial or financial relationships that could be construed as a potential conflict of interest.
The author(s) declared that they were an editorial board member of Frontiers, at the time of submission. This had no impact on the peer review process and the final decision.
Publisher’s note
All claims expressed in this article are solely those of the authors and do not necessarily represent those of their affiliated organizations, or those of the publisher, the editors and the reviewers. Any product that may be evaluated in this article, or claim that may be made by its manufacturer, is not guaranteed or endorsed by the publisher.
References
Alakkal, A., Thayyullathil, F., Pallichankandy, S., Subburayan, K., Cheratta, A. R., and Galadari, S. (2022). Sanguinarine induces H(2)O(2)-dependent apoptosis and ferroptosis in human cervical cancer. Biomedicines 10 (8), 1795. doi:10.3390/biomedicines10081795
An, S., and Hu, M. (2022). Quercetin promotes TFEB nuclear translocation and activates lysosomal degradation of ferritin to induce ferroptosis in breast cancer cells. Comput. Intell. Neurosci. 2022, 5299218. doi:10.1155/2022/5299218
Aquina, C. T., Ejaz, A., Tsung, A., Pawlik, T. M., and Cloyd, J. M. (2021). National trends in the use of neoadjuvant therapy before cancer surgery in the US from 2004 to 2016. JAMA Netw. Open 4 (3), e211031. doi:10.1001/jamanetworkopen.2021.1031
Bai, R., Zhu, J., Bai, Z., Mao, Q., Zhang, Y., Hui, Z., et al. (2022). Second generation β-Elemene nitric oxide derivatives with reasonable linkers: potential hybrids against malignant brain glioma. J. Enzyme Inhib. Med. Chem. 37 (1), 379–385. doi:10.1080/14756366.2021.2016734
Bai, Z., Yao, C., Zhu, J., Xie, Y., Ye, X. Y., Bai, R., et al. (2021). Anti-tumor drug discovery based on natural product β-elemene: anti-tumor mechanisms and structural modification. Molecules 26 (6), 1499. doi:10.3390/molecules26061499
Bray, F., Laversanne, M., Sung, H., Ferlay, J., Siegel, R. L., Soerjomataram, I., et al. (2024). Global cancer statistics 2022: GLOBOCAN estimates of incidence and mortality worldwide for 36 cancers in 185 countries. CA Cancer J. Clin. 74 (3), 229–263. doi:10.3322/caac.21834
Cai, J., Yi, M., Tan, Y., Li, X., Li, G., Zeng, Z., et al. (2021b). Natural product triptolide induces GSDME-mediated pyroptosis in head and neck cancer through suppressing mitochondrial hexokinase-ΙΙ. J. Exp. Clin. Cancer Res. 40 (1), 190. doi:10.1186/s13046-021-01995-7
Cai, S. Z., Xiong, Q. W., Zhao, L. N., Ji, Y. T., Luo, Z. X., and Ma, Z. R. (2021a). β-Elemene triggers ROS-dependent apoptosis in glioblastoma cells through suppressing STAT3 signaling pathway. Pathol. Oncol. Res. 27, 594299. doi:10.3389/pore.2021.594299
Cao, J., Wei, R., and Yao, S. (2019). Matrine has pro-apoptotic effects on liver cancer by triggering mitochondrial fission and activating Mst1-JNK signalling pathways. J. Physiol. Sci. 69 (2), 185–198. doi:10.1007/s12576-018-0634-4
Chakrabarti, M., and Ray, S. K. (2016). Anti-tumor activities of luteolin and silibinin in glioblastoma cells: overexpression of miR-7-1-3p augmented luteolin and silibinin to inhibit autophagy and induce apoptosis in glioblastoma in vivo. Apoptosis 21 (3), 312–328. doi:10.1007/s10495-015-1198-x
Chen, C., Xiao, W., Huang, L., Yu, G., Ni, J., Yang, L., et al. (2017). Shikonin induces apoptosis and necroptosis in pancreatic cancer via regulating the expression of RIP1/RIP3 and synergizes the activity of gemcitabine. Am. J. Transl. Res. 9 (12), 5507–5517.
Chen, J., Zhao, L., Xu, M. F., Huang, D., Sun, X. L., Zhang, Y. X., et al. (2024). Novel isobavachalcone derivatives induce apoptosis and necroptosis in human non-small cell lung cancer H1975 cells. J. Enzyme Inhib. Med. Chem. 39 (1), 2292006. doi:10.1080/14756366.2023.2292006
Chen, J. H., Guo, Q. F., Liu, Q. G., He, B. X., Song, W. P., Yin, Z. H., et al. (2022b). Chaetoglobosin E inhibits tumor growth and promotes the anti-tumor efficacy of cytotoxic drugs in esophageal squamous cell carcinoma by targeting PLK1. Ann. Transl. Med. 10 (22), 1236. doi:10.21037/atm-22-5320
Chen, P., Li, X., Zhang, R., Liu, S., Xiang, Y., Zhang, M., et al. (2020a). Combinative treatment of β-elemene and cetuximab is sensitive to KRAS mutant colorectal cancer cells by inducing ferroptosis and inhibiting epithelial-mesenchymal transformation. Theranostics 10 (11), 5107–5119. doi:10.7150/thno.44705
Chen, P., Wu, Q., Feng, J., Yan, L., Sun, Y., Liu, S., et al. (2020b). Erianin, a novel dibenzyl compound in Dendrobium extract, inhibits lung cancer cell growth and migration via calcium/calmodulin-dependent ferroptosis. Signal Transduct. Target Ther. 5 (1), 51. doi:10.1038/s41392-020-0149-3
Chen, Y., Ma, S., Pi, D., Wu, Y., Zuo, Q., Li, C., et al. (2022a). Luteolin induces pyroptosis in HT-29 cells by activating the Caspase1/Gasdermin D signalling pathway. Front. Pharmacol. 13, 952587. doi:10.3389/fphar.2022.952587
Chen, Z., Zhang, P., Xu, Y., Yan, J., Liu, Z., Lau, W. B., et al. (2019). Surgical stress and cancer progression: the twisted tango. Mol. Cancer 18 (1), 132. doi:10.1186/s12943-019-1058-3
Cheng, Y., Chen, T., Yang, X., Xue, J., and Chen, J. (2019). Atractylon induces apoptosis and suppresses metastasis in hepatic cancer cells and inhibits growth in vivo. Cancer Manag. Res. 11, 5883–5894. doi:10.2147/CMAR.S194795
Cheng, Y., Mai, J. Y., Hou, T. L., Ping, J., and Chen, J. J. (2016). Antiviral activities of atractylon from atractylodis rhizoma. Mol. Med. Rep. 14 (4), 3704–3710. doi:10.3892/mmr.2016.5713
Dai, X., Sun, F., Deng, K., Lin, G., Yin, W., Chen, H., et al. (2022). Mallotucin D, a clerodane diterpenoid from Croton crassifolius, suppresses HepG2 cell growth via inducing autophagic cell death and pyroptosis. Int. J. Mol. Sci. 23 (22), 14217. doi:10.3390/ijms232214217
Diao, Y., Ma, X., Min, W., Lin, S., Kang, H., Dai, Z., et al. (2016). Dasatinib promotes paclitaxel-induced necroptosis in lung adenocarcinoma with phosphorylated caspase-8 by c-Src. Cancer Lett. 379 (1), 12–23. doi:10.1016/j.canlet.2016.05.003
Ding, B., Chen, H., Tan, J., Meng, Q., Zheng, P., Ma, P., et al. (2023). ZIF-8 nanoparticles evoke pyroptosis for high-efficiency cancer immunotherapy. Angew. Chem. Int. Ed. Engl. 62 (10), e202215307. doi:10.1002/anie.202215307
Dong, H., Wang, M., Chang, C., Sun, M., Yang, F., Li, L., et al. (2020). Erianin inhibits the oncogenic properties of hepatocellular carcinoma via inducing DNA damage and aberrant mitosis. Biochem. Pharmacol. 182, 114266. doi:10.1016/j.bcp.2020.114266
Dong, X. M., Chen, L., Wu, P., Cheng, L. H., Wang, Y., Yang, Y., et al. (2024). Targeted metabolomics reveals PFKFB3 as a key target for elemene-mediated inhibition of glycolysis in prostate cancer cells. Phytomedicine 123, 155185. doi:10.1016/j.phymed.2023.155185
Elkateb, A. S., Nofal, S., Ali, S. A., and Atya, H. B. (2023). Camptothecin sensitizes hepatocellular carcinoma cells to sorafenib- induced ferroptosis via suppression of Nrf2. Inflammation 46 (4), 1493–1511. doi:10.1007/s10753-023-01823-4
Fang, Y., Tian, S., Pan, Y., Li, W., Wang, Q., Tang, Y., et al. (2020). Pyroptosis: a new frontier in cancer. Biomed. Pharmacother. 121, 109595. doi:10.1016/j.biopha.2019.109595
Feng, X. Q., Rong, L. W., Wang, R. X., Zheng, X. L., Zhang, L., Zhang, L., et al. (2018). Luteolin and sorafenib combination kills human hepatocellular carcinoma cells through apoptosis potentiation and JNK activation. Oncol. Lett. 16 (1), 648–653. doi:10.3892/ol.2018.8640
Fu, W., Xu, L., Chen, Y., Zhang, Z., Chen, S., Li, Q., et al. (2024). Luteolin induces ferroptosis in prostate cancer cells by promoting TFEB nuclear translocation and increasing ferritinophagy. Prostate 84 (3), 223–236. doi:10.1002/pros.24642
Gali-Muhtasib, H., Hmadi, R., Kareh, M., Tohme, R., and Darwiche, N. (2015). Cell death mechanisms of plant-derived anticancer drugs: beyond apoptosis. Apoptosis 20 (12), 1531–1562. doi:10.1007/s10495-015-1169-2
Gao, S., Zhang, X., Liu, J., Ji, F., Zhang, Z., Meng, Q., et al. (2023). Icariin induces triple-negative breast cancer cell apoptosis and suppresses invasion by inhibiting the JNK/c-Jun signaling pathway. Drug Des. Devel Ther. 17, 821–836. doi:10.2147/DDDT.S398887
Gao, Y., Mao, N. D., Che, H., Xu, L., Bai, R., Wang, L. W., et al. (2022). Novel hydroxyl carboximates derived from β-Elemene: design, synthesis and anti-tumour activities evaluation. J. Enzyme Inhib. Med. Chem. 37 (1), 2403–2416. doi:10.1080/14756366.2022.2117314
Gong, Y., Fan, Z., Luo, G., Yang, C., Huang, Q., Fan, K., et al. (2019). The role of necroptosis in cancer biology and therapy. Mol. Cancer 18 (1), 100. doi:10.1186/s12943-019-1029-8
Gou, X., Ru, Q., Zhang, H., Chen, Y., Li, L., Yang, H., et al. (2009a). HAb18G/CD147 inhibits starvation-induced autophagy in human hepatoma cell SMMC7721 with an involvement of Beclin 1 down-regulation. Cancer Sci. 100 (5), 837–843. doi:10.1111/j.1349-7006.2009.01113.x
Gou, X., Ru, Q., Zhang, H., Chen, Y., Li, L., Yang, H., et al. (2009b). HAb18G/CD147 inhibits starvation-induced autophagy in human hepatoma cell SMMC7721 with an involvement of Beclin 1 down-regulation. Cancer Sci. 100 (5), 837–843. doi:10.1111/j.1349-7006.2009.01113.x
Guan, C., Liu, W., Yue, Y., Jin, H., Wang, X., and Wang, X. J. (2014). Inhibitory effect of β-Elemene on human breast cancer cells. Int J Clin Exp Pathol. Int. J. Clin. Exp. Pathol. 7 (7), 3948–3956.
Guan, Z., Chen, J., Li, X., and Dong, N. (2020). Tanshinone IIA induces ferroptosis in gastric cancer cells through p53-mediated SLC7A11 down-regulation. Biosci. Rep. 40 (8). doi:10.1042/BSR20201807
Guo, Y., Zhu, H., Weng, M., Chen, B., Wang, C., and Sun, L. (2020). Baohuoside-1 targeting mTOR inducing apoptsis to inhibit hepatocellular carcinoma proliferation, invasion and migration. Biomed. Pharmacother. 128, 110366. doi:10.1016/j.biopha.2020.110366
Hamadou, M. H., Kerkatou, M., Gatto, P., Pancher, M., Bisio, A., Inga, A., et al. (2021). Apigenin rich-Limonium duriusculum (de Girard) Kuntze promotes apoptosis in HCT116 cancer cells. Nat. Prod. Res. 35 (17), 2910–2914. doi:10.1080/14786419.2019.1672070
Han, S., Lin, F., Qi, Y., Liu, C., Zhou, L., Xia, Y., et al. (2022). HO-1 contributes to luteolin-triggered ferroptosis in clear cell renal cell carcinoma via increasing the labile iron pool and promoting lipid peroxidation. Oxid. Med. Cell Longev. 2022, 1–26. doi:10.1155/2022/3846217
Han, S. H., Lee, J. H., Woo, J. S., Jung, G. H., Jung, S. H., Han, E. J., et al. (2024). Platycodin D induces apoptosis via regulating MAPK pathway and promotes autophagy in colon cancer cell. Biomed. Pharmacother. 172, 116216. doi:10.1016/j.biopha.2024.116216
Hardin, C., Shum, E., Singh, A. P., Perez-Soler, R., and Cheng, H. (2017). Emerging treatment using tubulin inhibitors in advanced non-small cell lung cancer. Expert Opin. Pharmacother. 18 (7), 701–716. doi:10.1080/14656566.2017.1316374
Hu, T., and Gao, Y. (2018). β-elemene against Burkitt's lymphoma via activation of PUMA mediated apoptotic pathway. Biomed. Pharmacother. 106, 1557–1562. doi:10.1016/j.biopha.2018.07.124
Huang, Q., Zhang, J., Peng, J., Zhang, Y., Wang, L., Wu, J., et al. (2019). Effect of baicalin on proliferation and apoptosis in pancreatic cancer cells. Am. J. Transl. Res. 11 (9), 5645–5654.
Hui, Z., Wen, H., Zhu, J., Deng, H., Jiang, X., Ye, X. Y., et al. (2024). Discovery of plant-derived anti-tumor natural products: potential leads for anti-tumor drug discovery. Bioorg Chem. 142, 106957. doi:10.1016/j.bioorg.2023.106957
Imran, M., Rauf, A., Abu-Izneid, T., Nadeem, M., Shariati, M. A., Khan, I. A., et al. (2019). Luteolin, a flavonoid, as an anticancer agent: a review. Biomed. Pharmacother. 112, 108612. doi:10.1016/j.biopha.2019.108612
Jacobs, A., Lemoine, A., Joshi, G. P., Van de Velde, M., and Bonnet, F.PROSPECT Working Group collaborators# (2020). PROSPECT guideline for oncological breast surgery: a systematic review and procedure-specific postoperative pain management recommendations. Anaesthesia 75 (5), 664–673. doi:10.1111/anae.14964
Jalal, S., Zhang, T., Deng, J., Wang, J., Xu, T., Zhang, T., et al. (2022). β-Elemene isopropanolamine derivative LXX-8250 induces apoptosis through impairing autophagic flux via PFKFB4 repression in melanoma cells. Front. Pharmacol. 13, 900973. doi:10.3389/fphar.2022.900973
Jia, M., Yang, F., Xu, Y., Xu, Q., Zeng, Y., Dai, R., et al. (2022a). Baicalin induced apoptosis of human cholangiocarcinoma cell through activating AMPK/mTORC1/p70S6K signaling pathway. Bull. Exp. Biol. Med. 173 (3), 366–370. doi:10.1007/s10517-022-05550-y
Jia, M., Yang, F., Xu, Y., Xu, Q., Zeng, Y., Dai, R., et al. (2022b). Baicalin induced apoptosis of human cholangiocarcinoma cell through activating AMPK/mTORC1/p70S6K signaling pathway. Bull. Exp. Biol. Med. 173 (3), 366–370. doi:10.1007/s10517-022-05550-y
Jiang, S. L., Guan, Y. D., Chen, X. S., Ge, P., Wang, X. L., Lao, Y. Z., et al. (2019). Tubeimoside-1, a triterpenoid saponin, induces cytoprotective autophagy in human breast cancer cells in vitro via Akt-mediated pathway. Acta Pharmacol. Sin. 40 (7), 919–928. doi:10.1038/s41401-018-0165-9
Jiang, X. Y., Shi, L. P., Zhu, J. L., Bai, R. R., and Xie, T. (2024). Elemene antitumor drugs development based on “molecular compatibility theory” and clinical application: a retrospective and prospective outlook. Chin. J. Integr. Med. 30 (1), 62–74. doi:10.1007/s11655-023-3714-0
Jin, J., Fan, Z., Long, Y., Li, Y., He, Q., Yang, Y., et al. (2024). Matrine induces ferroptosis in cervical cancer through activation of piezo1 channel. Phytomedicine 122, 155165. doi:10.1016/j.phymed.2023.155165
Kandhari, K., Mishra, J. P. N., Agarwal, R., and Singh, R. P. (2023). Acacetin induces sustained ERK1/2 activation and RIP1-dependent necroptotic death in breast cancer cells. Toxicol. Appl. Pharmacol. 462, 116409. doi:10.1016/j.taap.2023.116409
Kang, C. H., Molagoda, I. M. N., Choi, Y. H., Park, C., Moon, D. O., and Kim, G. Y. (2018). Apigenin promotes TRAIL-mediated apoptosis regardless of ROS generation. Food Chem. Toxicol. 111, 623–630. doi:10.1016/j.fct.2017.12.018
Khing, T. M., Po, W. W., and Sohn, U. D. (2019). Fluoxetine enhances anti-tumor activity of paclitaxel in gastric adenocarcinoma cells by triggering apoptosis and necroptosis. Anticancer Res. 39 (11), 6155–6163. doi:10.21873/anticanres.13823
Kim, K. H., and Lee, M. S. (2014). Autophagy--a key player in cellular and body metabolism. Nat. Rev. Endocrinol. 10 (6), 322–337. doi:10.1038/nrendo.2014.35
Klockgether-Radke, A. P. (2002). (F. W. Sertürner and the discovery of morphine. 200 years of pain therapy with opioids). Anasthesiol Intensivmed. Notfallmed Schmerzther 37 (5), 244–249. doi:10.1055/s-2002-30132
Kong, N., Chen, X., Feng, J., Duan, T., Liu, S., Sun, X., et al. (2021). Baicalin induces ferroptosis in bladder cancer cells by downregulating FTH1. Acta Pharm. Sin. B 11 (12), 4045–4054. doi:10.1016/j.apsb.2021.03.036
Kong, Y., Feng, Z., Chen, A., Qi, Q., Han, M., Wang, S., et al. (2019). The natural flavonoid galangin elicits apoptosis, pyroptosis, and autophagy in glioblastoma. Front. Oncol. 9, 942. doi:10.3389/fonc.2019.00942
Lee, H. S., Park, B. S., Kang, H. M., Kim, J. H., Shin, S. H., and Kim, I. R. (2021a). Role of luteolin-induced apoptosis and autophagy in human glioblastoma cell lines. Med. Kaunas. 57 (9), 879. doi:10.3390/medicina57090879
Lee, J., Han, Y., Wang, W., Jo, H., Kim, H., Kim, S., et al. (2021b). Phytochemicals in cancer immune checkpoint inhibitor therapy. Biomolecules 11 (8), 1107. doi:10.3390/biom11081107
Lee, J. Y., Ham, J., Lim, W., and Song, G. (2020b). Apomorphine induces mitochondrial-dysfunction-dependent apoptosis in choriocarcinoma. Reproduction 160 (3), 367–377. doi:10.1530/REP-20-0230
Lee, Y., and Kwon, Y. H. (2019). Regulation of apoptosis and autophagy by luteolin in human hepatocellular cancer Hep3B cells. Biochem. Biophys. Res. Commun. 517 (4), 617–622. doi:10.1016/j.bbrc.2019.07.073
Lee, Y. J., Park, K. S., Nam, H. S., Cho, M. K., and Lee, S. H. (2020a). Apigenin causes necroptosis by inducing ROS accumulation, mitochondrial dysfunction, and ATP depletion in malignant mesothelioma cells. Korean J. Physiol. Pharmacol. 24 (6), 493–502. doi:10.4196/kjpp.2020.24.6.493
Li, G., Xu, D., Sun, J., Zhao, S., and Zheng, D. (2020). Paclitaxel inhibits proliferation and invasion and promotes apoptosis of breast cancer cells by blocking activation of the PI3K/AKT signaling pathway. Adv. Clin. Exp. Med. 29 (11), 1337–1345. doi:10.17219/acem/127681
Li, J., Liu, J., Zhou, Z., Wu, R., Chen, X., Yu, C., et al. (2023b). Tumor-specific GPX4 degradation enhances ferroptosis-initiated antitumor immune response in mouse models of pancreatic cancer. Sci. Transl. Med. 15 (720), eadg3049. doi:10.1126/scitranslmed.adg3049
Li, M., Feng, Z., Han, R., Hu, B., Zhang, R., and Wang, H. (2023a). Paclitaxel promotes mTOR signaling-mediated apoptosis in esophageal cancer cells by targeting MUC20. Thorac. Cancer 14 (31), 3089–3096. doi:10.1111/1759-7714.15091
Liang, Q. P., Xu, T. Q., Liu, B. L., Lei, X. P., Hambrook, J. R., Zhang, D. M., et al. (2019). Sasanquasaponin ΙΙΙ from Schima crenata Korth induces autophagy through Akt/mTOR/p70S6K pathway and promotes apoptosis in human melanoma A375 cells. Phytomedicine 58, 152769. doi:10.1016/j.phymed.2018.11.029
Lin, C., Tsai, S. C., Tseng, M. T., Peng, S. F., Kuo, S. C., Lin, M. W., et al. (2013a). AKT serine/threonine protein kinase modulates baicalin-triggered autophagy in human bladder cancer T24 cells. Int. J. Oncol. 42 (3), 993–1000. doi:10.3892/ijo.2013.1791
Lin, C., Tsai, S. C., Tseng, M. T., Peng, S. F., Kuo, S. C., Lin, M. W., et al. (2013b). AKT serine/threonine protein kinase modulates baicalin-triggered autophagy in human bladder cancer T24 cells. Int. J. Oncol. 42 (3), 993–1000. doi:10.3892/ijo.2013.1791
Lin, D., Kuang, G., Wan, J., Zhang, X., Li, H., Gong, X., et al. (2017). Luteolin suppresses the metastasis of triple-negative breast cancer by reversing epithelial-to-mesenchymal transition via downregulation of β-catenin expression. Oncol. Rep. 37 (2), 895–902. doi:10.3892/or.2016.5311
Lin, S. R., Chang, C. H., Hsu, C. F., Tsai, M. J., Cheng, H., Leong, M. K., et al. (2020). Natural compounds as potential adjuvants to cancer therapy: preclinical evidence. Br. J. Pharmacol. 177 (6), 1409–1423. doi:10.1111/bph.14816
Liu, C., Sun, S., Feng, Q., Wu, G., Wu, Y., Kong, N., et al. (2021). Arsenene nanodots with selective killing effects and their low-dose combination with ß-elemene for cancer therapy. Adv. Mater. 33 (37), e2102054. doi:10.1002/adma.202102054
Liu, J., Hu, X. J., Jin, B., Qu, X. J., Hou, K. Z., and Liu, Y. P. (2012a). β-Elemene induces apoptosis as well as protective autophagy in human non-small-cell lung cancer A549 cells. J. Pharm. Pharmacol. 64 (1), 146–153. doi:10.1111/j.2042-7158.2011.01371.x
Liu, J., Hu, X. J., Jin, B., Qu, X. J., Hou, K. Z., and Liu, Y. P. (2012b). β-Elemene induces apoptosis as well as protective autophagy in human non-small-cell lung cancer A549 cells. J. Pharm. Pharmacol. 64 (1), 146–153. doi:10.1111/j.2042-7158.2011.01371.x
Liu, J., Qi, X., Gu, P., Wang, L., Song, S., and Shu, P. (2024a). Baicalin induces gastric cancer cell pyroptosis through the NF-κB-NLRP3 signaling Axis. J. Cancer 15 (2), 494–507. doi:10.7150/jca.89986
Liu, J., Zhang, Y., Qu, J., Xu, L., Hou, K., Zhang, J., et al. (2011). β-Elemene-induced autophagy protects human gastric cancer cells from undergoing apoptosis. BMC Cancer 11, 183. doi:10.1186/1471-2407-11-183
Liu, Q., Zhu, D., Hao, B., Zhang, Z., and Tian, Y. (2018). Luteolin promotes the sensitivity of cisplatin in ovarian cancer by decreasing PRPA1-medicated autophagy. Cell Mol. Biol. (Noisy-le-grand) 64 (6), 17–22. doi:10.14715/cmb/2018.64.6.4
Liu, S., Li, Q., Chen, K., Zhang, Q., Li, G., Zhuo, L., et al. (2020b). The emerging molecular mechanism of m(6)A modulators in tumorigenesis and cancer progression. Biomed. Pharmacother. 127, 110098. doi:10.1016/j.biopha.2020.110098
Liu, S., Li, Q., Li, G., Zhang, Q., Zhuo, L., Han, X., et al. (2020e). The mechanism of m6A methyltransferase METTL3-mediated autophagy in reversing gefitinib resistance in NSCLC cells by β-elemene. Cell Death Dis. 11 (11), 969. doi:10.1038/s41419-020-03148-8
Liu, S., Tao, Y., Wu, S., Lin, J., Fu, S., Lu, J., et al. (2024b). Sanguinarine chloride induces ferroptosis by regulating ROS/BACH1/HMOX1 signaling pathway in prostate cancer. Chin. Med. 19 (1), 7. doi:10.1186/s13020-024-00881-6
Liu, T., Sun, X., and Cao, Z. (2019). Shikonin-induced necroptosis in nasopharyngeal carcinoma cells via ROS overproduction and upregulation of RIPK1/RIPK3/MLKL expression. Onco Targets Ther. 12, 2605–2614. doi:10.2147/OTT.S200740
Liu, X., Zhang, Y., Gao, H., Hou, Y., Lu, J. J., Feng, Y., et al. (2020d). Induction of an MLKL mediated non-canonical necroptosis through reactive oxygen species by tanshinol A in lung cancer cells. Biochem. Pharmacol. 171, 113684. doi:10.1016/j.bcp.2019.113684
Liu, Y., Cao, H., Zhao, Y., Shan, L., and Lan, S. (2022). Fisetin-induced cell death in human ovarian cancer cell lines via zbp1-mediated necroptosis. J. Ovarian Res. 15 (1), 57. doi:10.1186/s13048-022-00984-4
Liu, Y., Chen, L., Zhang, R., Chen, B., Xiang, Y., Zhang, M., et al. (2020a). Efficacy and safety of elemene combined with chemotherapy in advanced gastric cancer: a Meta-analysis. Med. Baltim. 99 (11), e19481. doi:10.1097/MD.0000000000019481
Liu, Y., Chen, L., Zhang, R., Chen, B., Xiang, Y., Zhang, M., et al. (2020c). Efficacy and safety of elemene combined with chemotherapy in advanced gastric cancer: a Meta-analysis. Med. Baltim. 99 (11), e19481. doi:10.1097/md.0000000000019481
Liu, Y., and Fan, D. (2019). Ginsenoside Rg5 induces G2/M phase arrest, apoptosis and autophagy via regulating ROS-mediated MAPK pathways against human gastric cancer. Biochem. Pharmacol. 168, 285–304. doi:10.1016/j.bcp.2019.07.008
Liu, Y., Jiang, Z. Y., Zhou, Y. L., Qiu, H. H., Wang, G., Luo, Y., et al. (2017). β-elemene regulates endoplasmic reticulum stress to induce the apoptosis of NSCLC cells through PERK/IRE1α/ATF6 pathway. Biomed. Pharmacother. 93, 490–497. doi:10.1016/j.biopha.2017.06.073
Lomphithak, T., Jaikla, P., Sae-Fung, A., Sonkaew, S., and Jitkaew, S. (2023). Natural flavonoids quercetin and kaempferol targeting G2/M cell cycle-related genes and synergize with smac mimetic LCL-161 to induce necroptosis in cholangiocarcinoma cells. Nutrients 15 (14), 3090. doi:10.3390/nu15143090
Lou, J. S., Zhao, L. P., Huang, Z. H., Chen, X. Y., Xu, J. T., Tai, W. C., et al. (2021). Ginkgetin derived from Ginkgo biloba leaves enhances the therapeutic effect of cisplatin via ferroptosis-mediated disruption of the Nrf2/HO-1 axis in EGFR wild-type non-small-cell lung cancer. Phytomedicine 80, 153370. doi:10.1016/j.phymed.2020.153370
Lu, M., He, C. L., Wu, Z. T., Lyu, Y., Duan, X. H., Wang, B. X., et al. (2023). Effect of baicalin on pyroptosis of diffuse large B-cell lymphoma cell lines DB and its mechanism. Zhongguo Shi Yan Xue Ye Xue Za Zhi 31 (6), 1706–1713. doi:10.19746/j.cnki.issn.1009-2137.2023.06.016
Luo, D., Dai, X., Tian, H., Fan, C., Xie, H., Chen, N., et al. (2023). Sophflarine A, a novel matrine-derived alkaloid from Sophora flavescens with therapeutic potential for non-small cell lung cancer through ROS-mediated pyroptosis and autophagy. Phytomedicine 116, 154909. doi:10.1016/j.phymed.2023.154909
Luo, N., Zhang, K., Li, X., Hu, Y., and Guo, L. (2024). Tanshinone IIA destabilizes SLC7A11 by regulating PIAS4-mediated SUMOylation of SLC7A11 through KDM1A, and promotes ferroptosis in breast cancer. J. Adv. Res. doi:10.1016/j.jare.2024.04.009
Ma, X., Li, Y., Liang, D., Jiang, F., Zhang, L., Song, W., et al. (2024). Solanine induces ferroptosis in colorectal cancer cells through ALOX12B/ADCY4 molecular axis. J. Pharm. Pharmacol. 76 (3), 224–235. doi:10.1093/jpp/rgad122
Mao, J., Wang, X., Yu, M., and Sun, C. (2022). Effects of atractylon on proliferation and apoptosis of intestinal cancer cells through PI3K/AKT/mTOR signaling pathway. Cell Mol. Biol. (Noisy-le-grand) 68 (5), 153–160. doi:10.14715/cmb/2022.68.5.21
Mbaveng, A. T., Chi, G. F., Nguenang, G. S., Abdelfatah, S., Tchangna Sop, R. V., Ngadjui, B. T., et al. (2020). Cytotoxicity of a naturally occuring spirostanol saponin, progenin III, towards a broad range of cancer cell lines by induction of apoptosis, autophagy and necroptosis. Chem. Biol. Interact. 326, 109141. doi:10.1016/j.cbi.2020.109141
Mishra, A. P., Salehi, B., Sharifi-Rad, M., Pezzani, R., Kobarfard, F., Sharifi-Rad, J., et al. (2018). Programmed cell death, from a cancer perspective: an overview. Mol. Diagn Ther. 22 (3), 281–295. doi:10.1007/s40291-018-0329-9
Miyauchi, A., Watanabe, C., Yamada, N., Jimbo, E. F., Kobayashi, M., Ohishi, N., et al. (2024). Apomorphine is a potent inhibitor of ferroptosis independent of dopaminergic receptors. Sci. Rep. 14 (1), 4820. doi:10.1038/s41598-024-55293-1
Mu, L., Wang, T., Chen, Y., Tang, X., Yuan, Y., and Zhao, Y. (2016). β-Elemene enhances the efficacy of gefitinib on glioblastoma multiforme cells through the inhibition of the EGFR signaling pathway. Int. J. Oncol. 49 (4), 1427–1436. doi:10.3892/ijo.2016.3626
Nazim, U. M., and Park, S. Y. (2019a). Luteolin sensitizes human liver cancer cells to TRAIL-induced apoptosis via autophagy and JNK-mediated death receptor 5 upregulation. Int. J. Oncol. 54 (2), 665–672. doi:10.3892/ijo.2018.4633
Nazim, U. M., and Park, S. Y. (2019b). Luteolin sensitizes human liver cancer cells to TRAIL-induced apoptosis via autophagy and JNK-mediated death receptor 5 upregulation. Int. J. Oncol. 54 (2), 665–672. doi:10.3892/ijo.2018.4633
Ni, H., Ruan, G., Sun, C., Yang, X., Miao, Z., Li, J., et al. (2022). Tanshinone IIA inhibits gastric cancer cell stemness through inducing ferroptosis. Environ. Toxicol. 37 (2), 192–200. doi:10.1002/tox.23388
Pandurangan, A. K., Dharmalingam, P., Sadagopan, S. K., Ramar, M., Munusamy, A., and Ganapasam, S. (2013). Luteolin induces growth arrest in colon cancer cells through involvement of Wnt/β-catenin/GSK-3β signaling. J. Environ. Pathol. Toxicol. Oncol. 32 (2), 131–139. doi:10.1615/jenvironpatholtoxicoloncol.2013007522
Pang, H., Wu, T., Peng, Z., Tan, Q., Peng, X., Zhan, Z., et al. (2022a). Baicalin induces apoptosis and autophagy in human osteosarcoma cells by increasing ROS to inhibit PI3K/Akt/mTOR, ERK1/2 and β-catenin signaling pathways. J. Bone Oncol. 33, 100415. doi:10.1016/j.jbo.2022.100415
Pang, H., Wu, T., Peng, Z., Tan, Q., Peng, X., Zhan, Z., et al. (2022b). Baicalin induces apoptosis and autophagy in human osteosarcoma cells by increasing ROS to inhibit PI3K/Akt/mTOR, ERK1/2 and β-catenin signaling pathways. J. Bone Oncol. 33, 100415. doi:10.1016/j.jbo.2022.100415
Peng, F., Liao, M., Qin, R., Zhu, S., Peng, C., Fu, L., et al. (2022). Regulated cell death (RCD) in cancer: key pathways and targeted therapies. Signal Transduct. Target Ther. 7 (1), 286. doi:10.1038/s41392-022-01110-y
Qi, X., Jiang, S., Hui, Z., Gao, Y., Ye, Y., Lirussi, F., et al. (2022). Design, synthesis and antitumor efficacy evaluation of a series of novel β-Elemene-based macrocycles. Bioorg Med. Chem. 74, 117049. doi:10.1016/j.bmc.2022.117049
Qi, X., Liu, J., Wang, L., Gu, P., Song, S., and Shu, P. (2024). Kaempferol-induced mitochondrial damage promotes NF-κB-NLRP3-caspase-1 signaling axis-mediated pyroptosis in gastric cancer cells. Heliyon 10 (7), e28672. doi:10.1016/j.heliyon.2024.e28672
Qin, T., Zhu, W., Kan, X., Li, L., and Wu, D. (2022). Luteolin attenuates the chemoresistance of osteosarcoma through inhibiting the PTN/β-catenin/MDR1 signaling axis by upregulating miR-384. J. Bone Oncol. 34, 100429. doi:10.1016/j.jbo.2022.100429
Qu, L., Liu, Y., Deng, J., Ma, X., and Fan, D. (2023). Ginsenoside Rk3 is a novel PI3K/AKT-targeting therapeutics agent that regulates autophagy and apoptosis in hepatocellular carcinoma. J. Pharm. Anal. 13 (5), 463–482. doi:10.1016/j.jpha.2023.03.006
Rocha, G. Z., Dias, M. M., Ropelle, E. R., Osório-Costa, F., Rossato, F. A., Vercesi, A. E., et al. (2011). Metformin amplifies chemotherapy-induced AMPK activation and antitumoral growth. Clin. Cancer Res. 17 (12), 3993–4005. doi:10.1158/1078-0432.CCR-10-2243
Rong, Y., Liu, S. H., Tang, M. Z., and Yang, X. J. (2023). Quercetin inhibits the proliferative effect of gastric cancer cells by activating the pyroptosis pathway. Asian J. Surg. 46 (11), 5286–5288. doi:10.1016/j.asjsur.2023.07.051
Schirrmacher, V. (2019). From chemotherapy to biological therapy: a review of novel concepts to reduce the side effects of systemic cancer treatment (Review). Int. J. Oncol. 54 (2), 407–419. doi:10.3892/ijo.2018.4661
Shao, L., Zhu, L., Su, R., Yang, C., Gao, X., Xu, Y., et al. (2024). Baicalin enhances the chemotherapy sensitivity of oxaliplatin-resistant gastric cancer cells by activating p53-mediated ferroptosis. Sci. Rep. 14 (1), 10745. doi:10.1038/s41598-024-60920-y
Siddiqi, A., and Johnston, F. M. (2023). The perioperative and operative management of esophageal and gastric cancer. Surg. Oncol. Clin. N. Am. 32 (1), 65–81. doi:10.1016/j.soc.2022.07.006
Singh, J., Hussain, Y., Meena, A., Luqman, S., and Sinha, R. A. (2023). Molecular regulation of autophagy and suppression of protein kinases by aescin, a triterpenoid saponin impedes lung cancer progression. Int J Biol Macromol. Int. J. Biol. Macromol. 252, 126328. doi:10.1016/j.ijbiomac.2023.126328
Şirin, N., Elmas, L., Seçme, M., and Dodurga, Y. (2020). Investigation of possible effects of apigenin, sorafenib and combined applications on apoptosis and cell cycle in hepatocellular cancer cells. Gene 737, 144428. doi:10.1016/j.gene.2020.144428
Song, G. Q., Wu, P., Dong, X. M., Cheng, L. H., Lu, H. Q., Lin, Y. Y., et al. (2023). Elemene induces cell apoptosis via inhibiting glutathione synthesis in lung adenocarcinoma. J. Ethnopharmacol. 311, 116409. doi:10.1016/j.jep.2023.116409
Song, L., Chen, X., Mi, L., Liu, C., Zhu, S., Yang, T., et al. (2020). Icariin-induced inhibition of SIRT6/NF-κB triggers redox mediated apoptosis and enhances anti-tumor immunity in triple-negative breast cancer. Cancer Sci. 111 (11), 4242–4256. doi:10.1111/cas.14648
Sui, X., Han, X., Chen, P., Wu, Q., Feng, J., Duan, T., et al. (2020b). Baicalin induces apoptosis and suppresses the cell cycle progression of lung cancer cells through downregulating akt/mTOR signaling pathway. Front. Mol. Biosci. 7, 602282. doi:10.3389/fmolb.2020.602282
Sui, X., Han, X., Chen, P., Wu, Q., Feng, J., Duan, T., et al. (2020c). Baicalin induces apoptosis and suppresses the cell cycle progression of lung cancer cells through downregulating akt/mTOR signaling pathway. Front. Mol. Biosci. 7, 602282. doi:10.3389/fmolb.2020.602282
Sui, X., Zhang, M., Han, X., Zhang, R., Chen, L., Liu, Y., et al. (2020a). Combination of traditional Chinese medicine and epidermal growth factor receptor tyrosine kinase inhibitors in the treatment of non-small cell lung cancer: a systematic review and meta-analysis. Med. Baltim. 99 (32), e20683. doi:10.1097/MD.0000000000020683
Sun, S., Shi, J., Wang, X., Huang, C., Huang, Y., Xu, J., et al. (2022). Atractylon inhibits the tumorigenesis of glioblastoma through SIRT3 signaling. Am. J. Cancer Res. 12 (5), 2310–2322.
Sun, Z., Ma, C., and Zhan, X. (2024). Ajmalicine induces the pyroptosis of hepatoma cells to exert the antitumor effect. J. Biochem. Mol. Toxicol. 38 (1), e23614. doi:10.1002/jbt.23614
Tang, H. M., and Cheung, P. C. K. (2019). Gallic acid triggers iron-dependent cell death with apoptotic, ferroptotic, and necroptotic features. Toxins (Basel). 11 (9), 492. doi:10.3390/toxins11090492
Teng, J. F., Qin, D. L., Mei, Q. B., Qiu, W. Q., Pan, R., Xiong, R., et al. (2019). Polyphyllin VI, a saponin from Trillium tschonoskii Maxim. induces apoptotic and autophagic cell death via the ROS triggered mTOR signaling pathway in non-small cell lung cancer. Pharmacol. Res. 147, 104396. doi:10.1016/j.phrs.2019.104396
Tong, W., Guo, J., and Yang, C. (2020). Tanshinone II A enhances pyroptosis and represses cell proliferation of HeLa cells by regulating miR-145/GSDMD signaling pathway. Biosci. Rep. 40 (4). doi:10.1042/BSR20200259
Tong, X., Tang, R., Xiao, M., Xu, J., Wang, W., Zhang, B., et al. (2022). Targeting cell death pathways for cancer therapy: recent developments in necroptosis, pyroptosis, ferroptosis, and cuproptosis research. J. Hematol. Oncol. 15 (1), 174. doi:10.1186/s13045-022-01392-3
Wang, C., Yang, Y., Sun, L., Wang, J., Jiang, Z., Li, Y., et al. (2020). Baicalin reverses radioresistance in nasopharyngeal carcinoma by downregulating autophagy. Cancer Cell Int. 20, 35. doi:10.1186/s12935-020-1107-4
Wang, G., Li, Y., Guo, Z., He, Q., Liu, Z., and Deng, B. (2024a). Tanshinone I stimulates pyroptosis of cisplatin-resistant gastric cancer cells by activating the NF-κB/Caspase-3(8)/GSDME signaling pathway. DNA Cell Biol. 43 (4), 185–196. doi:10.1089/dna.2023.0293
Wang, G. Y., Zhang, L., Geng, Y. D., Wang, B., Feng, X. J., Chen, Z. L., et al. (2022). β-Elemene induces apoptosis and autophagy in colorectal cancer cells through regulating the ROS/AMPK/mTOR pathway. Chin. J. Nat. Med. 20 (1), 9–21. doi:10.1016/s1875-5364(21)60118-8
Wang, H., Shu, L., Lv, C., Liu, N., Long, Y., Peng, X., et al. (2024b). BRCC36 deubiquitinates HMGCR to regulate the interplay between ferroptosis and pyroptosis. Adv. Sci. (Weinh) 11 (11), e2304263. doi:10.1002/advs.202304263
Wang, J., Xu, C., Chen, Y., Shao, L., Li, T., Fan, X., et al. (2021a). β-Elemene enhances the antitumor activity of erlotinib by inducing apoptosis through AMPK and MAPK pathways in TKI-resistant H1975 lung cancer cells. J. Cancer 12 (8), 2285–2294. doi:10.7150/jca.53382
Wang, S., Guo, Q., Xu, R., Lin, P., Deng, G., and Xia, X. (2023). Combination of ferroptosis and pyroptosis dual induction by triptolide nano-MOFs for immunotherapy of Melanoma. J. Nanobiotechnology 21 (1), 383. doi:10.1186/s12951-023-02146-0
Wang, Y., Jin, W., and Wang, J. (2021b). Tanshinone IIA regulates microRNA-125b/foxp3/caspase-1 signaling and inhibits cell viability of nasopharyngeal carcinoma. Mol. Med. Rep. 23 (5), 371. doi:10.3892/mmr.2021.12010
Wang, Z. X., Ma, J., Li, X. Y., Wu, Y., Shi, H., Chen, Y., et al. (2021c). Quercetin induces p53-independent cancer cell death through lysosome activation by the transcription factor EB and Reactive Oxygen Species-dependent ferroptosis. Br. J. Pharmacol. 178 (5), 1133–1148. doi:10.1111/bph.15350
Wen, R. J., Dong, X., Zhuang, H. W., Pang, F. X., Ding, S. C., Li, N., et al. (2023b). Baicalin induces ferroptosis in osteosarcomas through a novel Nrf2/xCT/GPX4 regulatory axis. Phytomedicine 116, 154881. doi:10.1016/j.phymed.2023.154881
Wen, Y., Wang, Y., Zhao, C., Zhao, B., and Wang, J. (2023a). The pharmacological efficacy of baicalin in inflammatory diseases. Int. J. Mol. Sci. 24 (11), 9317. doi:10.3390/ijms24119317
Wen, Z., Zhang, Y., Gao, B., and Chen, X. (2024). Baicalin induces ferroptosis in oral squamous cell carcinoma by suppressing the activity of FTH1. J. Gene Med. 26 (2), e3669. doi:10.1002/jgm.3669
Wu, B., Xiong, J., Zhou, Y., Wu, Y., Song, Y., Wang, N., et al. (2020b). Luteolin enhances TRAIL sensitivity in non-small cell lung cancer cells through increasing DR5 expression and Drp1-mediated mitochondrial fission. Arch. Biochem. Biophys. 692, 108539. doi:10.1016/j.abb.2020.108539
Wu, C. Z., Gao, M. J., Chen, J., Sun, X. L., Zhang, K. Y., Dai, Y. Q., et al. (2022a). Isobavachalcone induces multiple cell death in human triple-negative breast cancer MDA-MB-231 cells. Molecules 27 (20), 6787. doi:10.3390/molecules27206787
Wu, H. T., Liu, Y. E., Hsu, K. W., Wang, Y. F., Chan, Y. C., Chen, Y., et al. (2020a). MLL3 induced by luteolin causes apoptosis in tamoxifen-resistant breast cancer cells through H3K4 monomethylation and suppression of the PI3K/AKT/mTOR pathway. Am. J. Chin. Med. 48 (5), 1221–1241. doi:10.1142/S0192415X20500603
Wu, L., Lin, Y., Gao, S., Wang, Y., Pan, H., Wang, Z., et al. (2023). Luteolin inhibits triple-negative breast cancer by inducing apoptosis and autophagy through SGK1-FOXO3a-BNIP3 signaling. Front. Pharmacol. 14, 1200843. doi:10.3389/fphar.2023.1200843
Wu, Y., Chang, J., Ge, J., Xu, K., Zhou, Q., Zhang, X., et al. (2022b). Isobavachalcone's alleviation of pyroptosis contributes to enhanced apoptosis in glioblastoma: possible involvement of NLRP3. Mol. Neurobiol. 59 (11), 6934–6955. doi:10.1007/s12035-022-03010-2
Xia, M., Wu, Y., Zhu, H., and Duan, W. (2023). Tanshinone I induces ferroptosis in gastric cancer cells via the KDM4D/p53 pathway. Hum. Exp. Toxicol. 42, 9603271231216963. doi:10.1177/09603271231216963
Xiang, Y., Chen, X., Wang, W., Zhai, L., Sun, X., Feng, J., et al. (2021a). Natural product erianin inhibits bladder cancer cell growth by inducing ferroptosis via NRF2 inactivation. Front. Pharmacol. 12, 775506. doi:10.3389/fphar.2021.775506
Xiang, Y. C., Peng, P., Liu, X. W., Jin, X., Shen, J., Zhang, T., et al. (2022). Paris saponin VII, a Hippo pathway activator, induces autophagy and exhibits therapeutic potential against human breast cancer cells. Acta Pharmacol. Sin. 43 (6), 1568–1580. doi:10.1038/s41401-021-00755-9
Xiang, Y. C., Shen, J., Si, Y., Liu, X. W., Zhang, L., Wen, J., et al. (2021b). Paris saponin VII, a direct activator of AMPK, induces autophagy and exhibits therapeutic potential in non-small-cell lung cancer. Chin. J. Nat. Med. 19 (3), 195–204. doi:10.1016/S1875-5364(21)60021-3
Xiaomeng, F., Lei, L., Jinghong, A., Juan, J., Qi, Y., and Dandan, Y. (2020). Treatment with β-elemene combined with paclitaxel inhibits growth, migration, and invasion and induces apoptosis of ovarian cancer cells by activation of STAT-NF-κB pathway. Braz J. Med. Biol. Res. 53 (6), e8885. doi:10.1590/1414-431x20208885
Xie, L., Zhang, J., Yan, H., Cai, Y., and Xu, L. (2022). β-Elemene induced apoptosis and senescence of triple-negative breast cancer cells through IGF1/IGF1R pathway. Tissue Cell 79, 101914. doi:10.1016/j.tice.2022.101914
Xu, B., Xu, M., Tian, Y., Yu, Q., Zhao, Y., Chen, X., et al. (2017). Matrine induces RIP3-dependent necroptosis in cholangiocarcinoma cells. Cell Death Discov. 3, 16096. doi:10.1038/cddiscovery.2016.96
Xu, C., Jiang, Z. B., Shao, L., Zhao, Z. M., Fan, X. X., Sui, X., et al. (2023). β-Elemene enhances erlotinib sensitivity through induction of ferroptosis by upregulating lncRNA H19 in EGFR-mutant non-small cell lung cancer. Pharmacol. Res. 191, 106739. doi:10.1016/j.phrs.2023.106739
Xu, H., Zeng, X., Wei, X., Xue, Z., Chen, N., Zhu, W., et al. (2022a). Rosin derivative IDOAMP inhibits prostate cancer growth via activating RIPK1/RIPK3/MLKL signaling pathway. Oxid. Med. Cell Longev. 2022, 1–11. doi:10.1155/2022/9325973
Xu, R., Wu, J., Luo, Y., Wang, Y., Tian, J., Teng, W., et al. (2022b). Sanguinarine represses the growth and metastasis of non-small cell lung cancer by facilitating ferroptosis. Curr. Pharm. Des. 28 (9), 760–768. doi:10.2174/1381612828666220217124542
Yang, C., Song, J., Hwang, S., Choi, J., Song, G., and Lim, W. (2021b). Apigenin enhances apoptosis induction by 5-fluorouracil through regulation of thymidylate synthase in colorectal cancer cells. Redox Biol. 47, 102144. doi:10.1016/j.redox.2021.102144
Yang, D., Xu, X., Wang, X., Feng, W., Shen, X., Zhang, J., et al. (2021a). β-Elemene promotes the senescence of glioma cells through regulating YAP-CDK6 signaling. Am. J. Cancer Res. 11 (2), 370–388.
Yang, H., Zhao, Y., Song, W., and Fan, G. (2024). The inhibition of β-catenin activity by luteolin isolated from Paulownia flowers leads to growth arrest and apoptosis in cholangiocarcinoma. Int. J. Biol. Macromol. 254 (Pt 1), 127627. doi:10.1016/j.ijbiomac.2023.127627
Yang, J., Pi, C., and Wang, G. (2018). Inhibition of PI3K/Akt/mTOR pathway by apigenin induces apoptosis and autophagy in hepatocellular carcinoma cells. Biomed. Pharmacother. 103, 699–707. doi:10.1016/j.biopha.2018.04.072
Yang, Y., Chen, Y., Wu, J. H., Ren, Y., Liu, B., Zhang, Y., et al. (2023). Targeting regulated cell death with plant natural compounds for cancer therapy: a revisited review of apoptosis, autophagy-dependent cell death, and necroptosis. Phytother. Res. 37 (4), 1488–1525. doi:10.1002/ptr.7738
Yi, N., Wang, L., Jiang, Z., Xu, G., Li, L., Zhang, Y., et al. (2024). Peiminine triggers ferroptosis to inhibit breast cancer growth through triggering Nrf2 signaling. Tissue Cell 87, 102323. doi:10.1016/j.tice.2024.102323
Yin, Q., Chen, H., Ma, R. H., Zhang, Y. Y., Liu, M. M., Thakur, K., et al. (2021). Ginsenoside CK induces apoptosis of human cervical cancer HeLa cells by regulating autophagy and endoplasmic reticulum stress. Food Funct. 12 (12), 5301–5316. doi:10.1039/d1fo00348h
Yoo, H. S., Won, S. B., and Kwon, Y. H. (2022). Luteolin induces apoptosis and autophagy in HCT116 colon cancer cells via p53-dependent pathway. Nutr. Cancer 74 (2), 677–686. doi:10.1080/01635581.2021.1903947
Yoshida, G. J. (2017). Therapeutic strategies of drug repositioning targeting autophagy to induce cancer cell death: from pathophysiology to treatment. J. Hematol. Oncol. 10 (1), 67. doi:10.1186/s13045-017-0436-9
Yu, S. X., Liang, Z. M., Wu, Q. B., Shou, L., Huang, X. X., Zhu, Q. R., et al. (2022). A novel diagnostic and therapeutic strategy for cancer patients by integrating Chinese medicine syndrome differentiation and precision medicine. Chin. J. Integr. Med. 28 (10), 867–871. doi:10.1007/s11655-022-3671-z
Yu, Y., Huang, X., Liang, C., and Zhang, P. (2023). Evodiamine impairs HIF1A histone lactylation to inhibit Sema3A-mediated angiogenesis and PD-L1 by inducing ferroptosis in prostate cancer. Eur. J. Pharmacol. 957, 176007. doi:10.1016/j.ejphar.2023.176007
Yuan, J., Khan, S. U., Yan, J., Lu, J., Yang, C., and Tong, Q. (2023). Baicalin enhances the efficacy of 5-Fluorouracil in gastric cancer by promoting ROS-mediated ferroptosis. Biomed. Pharmacother. 164, 114986. doi:10.1016/j.biopha.2023.114986
Yuan, R., Zhao, W., Wang, Q. Q., He, J., Han, S., Gao, H., et al. (2021). Cucurbitacin B inhibits non-small cell lung cancer in vivo and in vitro by triggering TLR4/NLRP3/GSDMD-dependent pyroptosis. Pharmacol. Res. 170, 105748. doi:10.1016/j.phrs.2021.105748
Yu-Wei, D., Li, Z. S., Xiong, S. M., Huang, G., Luo, Y. F., Huo, T. Y., et al. (2020). Paclitaxel induces apoptosis through the TAK1-JNK activation pathway. FEBS Open Bio 10 (8), 1655–1667. doi:10.1002/2211-5463.12917
Zhai, B., Wu, Q., Wang, W., Zhang, M., Han, X., Li, Q., et al. (2020). Preparation, characterization, pharmacokinetics and anticancer effects of PEGylated β-elemene liposomes. Cancer Biol. Med. 17 (1), 60–75. doi:10.20892/j.issn.2095-3941.2019.0156
Zhan, Y. H., Liu, J., Qu, X. J., Hou, K. Z., Wang, K. F., Liu, Y. P., et al. (2012a). β-Elemene induces apoptosis in human renal-cell carcinoma 786-0 cells through inhibition of MAPK/ERK and PI3K/Akt/mTOR signalling pathways. Asian Pac J. Cancer Prev. 13 (6), 2739–2744. doi:10.7314/apjcp.2012.13.6.2739
Zhan, Y. H., Liu, J., Qu, X. J., Hou, K. Z., Wang, K. F., Liu, Y. P., et al. (2012b). β-Elemene induces apoptosis in human renal-cell carcinoma 786-0 cells through inhibition of MAPK/ERK and PI3K/Akt/mTOR signalling pathways. Asian Pac J. Cancer Prev. 13 (6), 2739–2744. doi:10.7314/apjcp.2012.13.6.2739
Zhang, C., Liu, X., Jin, S., Chen, Y., and Guo, R. (2022c). Ferroptosis in cancer therapy: a novel approach to reversing drug resistance. Mol. Cancer 21 (1), 47. doi:10.1186/s12943-022-01530-y
Zhang, C. C., Li, C. G., Wang, Y. F., Xu, L. H., He, X. H., Zeng, Q. Z., et al. (2019). Chemotherapeutic paclitaxel and cisplatin differentially induce pyroptosis in A549 lung cancer cells via caspase-3/GSDME activation. Apoptosis 24 (3-4), 312–325. doi:10.1007/s10495-019-01515-1
Zhang, F., Yin, Y., Xu, W., Song, Y., Zhou, Z., Sun, X., et al. (2022b). Icariin inhibits gastric cancer cell growth by regulating the hsa_circ_0003159/miR-223-3p/NLRP3 signaling axis. Hum. Exp. Toxicol. 41, 9603271221097363. doi:10.1177/09603271221097363
Zhang, J., Wang, R., Qin, Y., and Feng, C. (2022d). Defining the potential targets for biological activity of isoegomaketone based on network pharmacology and molecular docking methods. Life (Basel). 12 (12), 2115. doi:10.3390/life12122115
Zhang, L., Song, J., Kong, L., Yuan, T., Li, W., Zhang, W., et al. (2020a). The strategies and techniques of drug discovery from natural products. Pharmacol. Ther. 216, 107686. doi:10.1016/j.pharmthera.2020.107686
Zhang, R., Chen, J., Mao, L., Guo, Y., Hao, Y., Deng, Y., et al. (2020b). Nobiletin triggers reactive oxygen species-mediated pyroptosis through regulating autophagy in ovarian cancer cells. J. Agric. Food Chem. 68 (5), 1326–1336. doi:10.1021/acs.jafc.9b07908
Zhang, R., Pan, T., Xiang, Y., Zhang, M., Feng, J., Liu, S., et al. (2020d). β-Elemene reverses the resistance of p53-deficient colorectal cancer cells to 5-fluorouracil by inducing pro-death autophagy and cyclin D3-dependent cycle arrest. Front. Bioeng. Biotechnol. 8, 378. doi:10.3389/fbioe.2020.00378
Zhang, T., Zhang, T., Gao, C., Jalal, S., Yuan, R., Teng, H., et al. (2023b). Antitumor effects of β-elemene through inducing autophagy-mediated apoptosis in ewing sarcoma family tumor cells. DNA Cell Biol. 42 (9), 532–540. doi:10.1089/dna.2023.0084
Zhang, X., Tang, B., Wen, S., Wang, Y., Pan, C., Qu, L., et al. (2023a). Advancements in the biotransformation and biosynthesis of the primary active flavonoids derived from epimedium. Molecules 28 (20), 7173. doi:10.3390/molecules28207173
Zhang, X., Tang, X., Liu, H., Li, L., Hou, Q., and Gao, J. (2012a). Autophagy induced by baicalin involves downregulation of CD147 in SMMC-7721 cells in vitro. Oncol. Rep. 27 (4), 1128–1134. doi:10.3892/or.2011.1599
Zhang, X., Tang, X., Liu, H., Li, L., Hou, Q., and Gao, J. (2012b). Autophagy induced by baicalin involves downregulation of CD147 in SMMC-7721 cells in vitro. Oncol. Rep. 27 (4), 1128–1134. doi:10.3892/or.2011.1599
Zhang, Y., Tang, Y., Tang, X., Wang, Y., Zhang, Z., Yang, H., et al. (2022a). Nerve regeneration effect of a composite bioactive carboxymethyl chitosan-based nerve conduit with a radial texture. Molecules 27 (21), 9039. doi:10.3390/molecules27249039
Zhang, Y., Yang, H., Sun, M., He, T., Liu, Y., Yang, X., et al. (2020c). Alpinumisoflavone suppresses hepatocellular carcinoma cell growth and metastasis via NLRP3 inflammasome-mediated pyroptosis. Pharmacol. Rep. 72 (5), 1370–1382. doi:10.1007/s43440-020-00064-8
Zhao, L. P., Wang, H. J., Hu, D., Hu, J. H., Guan, Z. R., Yu, L. H., et al. (2023). β-Elemene induced ferroptosis via TFEB-mediated GPX4 degradation in EGFR wide-type non-small cell lung cancer. J. Adv. Res. doi:10.1016/j.jare.2023.08.018
Zhao, S., Tang, Y., Wang, R., and Najafi, M. (2022). Mechanisms of cancer cell death induction by paclitaxel: an updated review. Apoptosis 27 (9-10), 647–667. doi:10.1007/s10495-022-01750-z
Zheng, W., Zhou, C. Y., Zhu, X. Q., Wang, X. J., Li, Z. Y., Chen, X. C., et al. (2018). Oridonin enhances the cytotoxicity of 5-FU in renal carcinoma cells by inducting necroptotic death. Biomed. Pharmacother. 106, 175–182. doi:10.1016/j.biopha.2018.06.111
Zheng, Y., Li, L., Chen, H., Zheng, Y., Tan, X., Zhang, G., et al. (2023). Luteolin exhibits synergistic therapeutic efficacy with erastin to induce ferroptosis in colon cancer cells through the HIC1-mediated inhibition of GPX4 expression. Free Radic. Biol. Med. 208, 530–544. doi:10.1016/j.freeradbiomed.2023.09.014
Zhou, J., Li, G., Han, G., Feng, S., Liu, Y., Chen, J., et al. (2020). Emodin induced necroptosis in the glioma cell line U251 via the TNF-α/RIP1/RIP3 pathway. Invest New Drugs 38 (1), 50–59. doi:10.1007/s10637-019-00764-w
Zhou, J., Wang, Y., Fu, Y., Lin, Z., Lin, H., Lv, G., et al. (2024). Chelerythrine induces apoptosis and ferroptosis through Nrf2 in ovarian cancer cells. Cell Mol. Biol. (Noisy-le-grand) 70 (3), 174–181. doi:10.14715/cmb/2024.70.3.26
Zhou, X., Yeasmin Khusbu, F., Xie, Y., and Yang, P. (2023). Emodin-induced necroptosis in prostate cancer cells via the mitochondrial fission HSP90/MLKL/PGAM pathway. Chem. Biodivers. 20 (6), e202201130. doi:10.1002/cbdv.202201130
Zhou, Y., Liao, J., Mei, Z., Liu, X., and Ge, J. (2021). Insight into crosstalk between ferroptosis and necroptosis: novel therapeutics in ischemic stroke. Oxid. Med. Cell Longev. 2021, 9991001. doi:10.1155/2021/9991001
Zhu, J., Jiang, X., Luo, X., Zhao, R., Li, J., Cai, H., et al. (2023). Combination of chemotherapy and gaseous signaling molecular therapy: novel β-Elemene nitric oxide donor derivatives against leukemia. Drug Dev. Res. 84 (4), 718–735. doi:10.1002/ddr.22051
Zhu, L., Chen, C., Cai, Y., Li, Y., Gong, L., Zhu, T., et al. (2024). Identification of a ferritinophagy inducer via sinomenine modification for the treatment of colorectal cancer. Eur. J. Med. Chem. 268, 116250. doi:10.1016/j.ejmech.2024.116250
Zhu, M., Wang, J., Xie, J., Chen, L., Wei, X., Jiang, X., et al. (2018). Design, synthesis, and evaluation of chalcone analogues incorporate α,β-Unsaturated ketone functionality as anti-lung cancer agents via evoking ROS to induce pyroptosis. Eur. J. Med. Chem. 157, 1395–1405. doi:10.1016/j.ejmech.2018.08.072
Keywords: cancer therapy, plant natural compound, programmed cell death, pharmacology, signaling pathways
Citation: Li Q, Tong Y, Chen J and Xie T (2024) Targeting programmed cell death via active ingredients from natural plants: a promising approach to cancer therapy. Front. Pharmacol. 15:1491802. doi: 10.3389/fphar.2024.1491802
Received: 05 September 2024; Accepted: 09 October 2024;
Published: 01 November 2024.
Edited by:
Xuelin Zhou, Capital Medical University, ChinaReviewed by:
Zechen Wang, Recode Therapeutics, United StatesXiaodong Zou, Memorial Sloan Kettering Cancer Center, United States
Ruo Wang, Shanghai Jiao Tong University, China
Yingjie Guo, Stanford University, United States
Dan Shan, Temple University, United States
Jiangshu Liu, St. Jude Children’s Research Hospital, United States
Copyright © 2024 Li, Tong, Chen and Xie. This is an open-access article distributed under the terms of the Creative Commons Attribution License (CC BY). The use, distribution or reproduction in other forums is permitted, provided the original author(s) and the copyright owner(s) are credited and that the original publication in this journal is cited, in accordance with accepted academic practice. No use, distribution or reproduction is permitted which does not comply with these terms.
*Correspondence: Jianxiang Chen, Y2hlbmp4QGh6bnUuZWR1LmNu; Tian Xie, dGlhbnhpZUBoem51LmVkdS5jbg==