- 1Student Research Committee, Arak University of Medical Sciences, Arak, Iran
- 2Department of Genetics, Faculty of Advanced Science and Technology, Tehran Medical Sciences, Islamic Azad University, Tehran, Iran
- 3Department of Microbiology, School of Medicine, Hamadan University of Medical Sciences, Hamadan, Iran
- 4Infectious Diseases Research Center (IDRC), Arak University of Medical Sciences, Arak, Iran
Staphylococcus aureus is an important pathogen due to its ability to form strong biofilms and antibiotic resistance. Biofilms play an important role in bacterial survival against the host immune system and antibiotics. Natural compounds (NCs) have diverse bioactive properties with a low probability of resistance, making them promising candidates for biofilm control. NC such as curcumin, cinnamaldehyde, carvacrol, eugenol, thymol, citral, linalool, 1,8-cineole, pinene, cymene, terpineol, quercetin, and limonene have been widely utilized for the inhibition and destruction of S. aureus biofilms. NCs influence biofilm formation through several procedures. Some of the antibiofilm mechanisms of NCs are direct bactericidal effect, disrupting the quorum sensing system, preventing bacteria from aggregation and attachment to surfaces, reducing the microbial surface components recognizing adhesive matrix molecules (MSCRAMMs), interfering with sortase A enzyme, and altering the expression of biofilm-associated genes such as icaADBC, agr, and sarA. Furthermore, these compounds affect extracellular polymeric substances (EPS) and their components, such as polysaccharide intercellular adhesin (PIA) and eDNA. However, some disadvantages, such as low water solubility and bioavailability, limit their clinical usage. Therefore, scientists have considered using nanotechnology and drug platforms to improve NC’s efficacy. Some NC, such as thymol and curcumin, can also enhance photodynamic therapy against S. aurous biofilm community. This article evaluates the anti-biofilm potential of NC, their mechanisms of action against S. aureus biofilms, and various aspects of their application.
Introduction
Staphylococcus aureus is a bacterium that forms biofilms widely linked to infections acquired in community and hospital settings (Mastoor et al., 2022). The bacterium’s capacity to build biofilms restricts the effectiveness of antimicrobial drugs, heightening the infection’s severity and potentially exacerbating the disease’s consequences (e.g., cystic fibrosis), presenting a significant clinical obstacle (Ramasamy et al., 2017b).
The ability of S. aureus to attach firmly to both natural and abiotic surfaces is attributed to the presence of proteins that facilitate adhesion to host tissues and surfaces. As a result, it produces biofilms that are both mechanically and chemically resilient (Ramasamy et al., 2017a). A key characteristic of this bacterium is its high concentration of microbial adhesion molecules, referred to as Microbial Surface Component Recognizing Adhesive Matrix Molecules (MSCRAMMs). Intracellular adhesion (IcaA), clumping factors A and B (ClfA and ClfB), collagen-binding adhesion (cna), fibronectin-binding proteins (fnb), and other similar proteins are types of adhesion proteins (Simpson et al., 2004). Notably, while several factors affect the formation of biofilms in S. aureus, polysaccharide intercellular adhesins (PIA) expressed by the ica operon have the main impact (Mastoor et al., 2022).
A biofilm is a complex network of closely packed, membrane-like structures created by bacteria that attach to a surface and release a matrix of polysaccharides, fibrin, lipid proteins, and other substances (Xu et al., 2022). Intricate aggregation of extracellular polymers on the biofilm surface results in a complex and organized overall structure that successfully safeguards the stability of the biofilm on the carrier surface. Full eradication of biofilm using conventional methods is often challenging (Srinivasan et al., 2008). Bacterial biofilms enable survival in hostile conditions and frequently exhibit resistance to drugs and human defenses, therefore playing a role in developing persistent illnesses (Kim Y. et al., 2022). Specifically, avoiding the development of harmful biofilms on food and surfaces, especially those of medical equipment, is immensely significant. Multiple processes contribute to the antimicrobial resistance of biofilms, including decreased antibiotic penetration, varying growth rates of bacterial cells, nutritional gradients within the biofilm, and the existence of latent variations (persister phenomena) that are highly resistant to antibiotics. The presence of antibiotics triggers additional mechanisms contributing to the antimicrobial resistance of biofilm. These mechanisms include the production of unique antibiotic-resistance genes specific to biofilm and mutational processes (Kot et al., 2019).
In addition to being resistant to β-lactam antibiotics, methicillin-resistant S. aureus (MRSA) strains frequently exhibit resistance to other widely used antibiotic groups, including aminoglycosides, fluoroquinolones, macrolides, tetracycline, and chloramphenicol (Kot et al., 2020). The constrained therapeutic alternatives for MRSA infections lead to elevated mortality rates and escalated budgetary burdens. Consequently, novel approaches, such as nanoparticles (NPs), bacteriophages, enzymes, and natural compounds, have garnered more interest. Natural compounds, such as botanical extracts, oils, and their derived chemicals, have demonstrated efficacy against various microorganisms and have been employed to fight against diseases and infections (Mastoor et al., 2022; Kargaran et al., 2024). A diverse range of secondary metabolites, primarily phenols or their oxygen-substituted derivatives, created by several medicinal plants exhibit a broad spectrum of antibacterial properties (Nostro et al., 2015).
Recent studies have shown that certain natural chemicals, including curcumin, cinnamaldehyde, eugenol, carvacrol, and thymol, not only prevent the production of biofilms but also remove fully developed biofilm formations (Doke et al., 2014; Rangel et al., 2018). Moreover, the concurrent administration of antibacterial medications and various natural compounds can serve as a highly efficient approach to addressing prevalent bacterial infections owing to its heightened potency and efficacy, diminished drug toxicity, optimized dosages, and decreased probability of acquiring resistance strains (Ushimaru et al., 2012). Therefore, this study focuses on the interactions between natural compounds and biofilm communities of S. aureus, as well as different pharmacological platforms utilized to enhance the effectiveness of natural compounds against this bacterial biofilm community.
Carvacrol
Carvacrol, scientifically also referred to as 2-methyl-5-(1-methyl ethyl)-phenol, is a monoterpene phenol found in the essential oils of several Lamiaceae species such as Thymus, Origanum, Thymbra, Satureja, and Coridothymus. It has been determined that Origanum vulgare contains the greatest quantity of carvacrol (Baser, 2008; Aprotosoaie et al., 2019). This compound is categorized as Generally Recognized as Safe (GRAS) by the U.S. Food and Drug Administration (FDA), and it is used as a flavoring agent in sweets, beverages, and chewing gum (Center for Food and Applied, 2006; Burdock, 2009). The broad-spectrum antibacterial activity and biofilm inhabitation capacity of carvacrol have been extensively investigated (Dorman and Deans, 2000; Inouye et al., 2001; Burt, 2004; Nostro et al., 2015). In this regard, recently published studies have demonstrated the anti-biofilm effect of carvacrol against S. aureus (Nostro et al., 2007; Burt et al., 2014; García-Salinas et al., 2018; Peng et al., 2018; Mouwakeh et al., 2019; Kostoglou et al., 2020; Walczak et al., 2021; Li et al., 2022b). For example, in one study, 4–8 μg/mL of carvacrol inhibited S. aureus biofilm formation (Peng et al., 2023).
Carvacrol interacts with the lipid bilayer of the bacterial cytoplasmic membrane, leading to a disruption of its integrity, collapse of the proton motive force, extrusion of cellular material, and a reduction in energy metabolism that affects genetic material synthesis (Ben Arfa et al., 2006; García-Salinas et al., 2018; Martínez et al., 2021). Increased membrane damage may hinder the early bacterial attachment phase and disrupt the normal formation of biofilms (Nostro et al., 2012a; Nostro et al., 2012b; Kerekes et al., 2013). In addition, the polar groups present in carvacrol minimize the contact angle values of the material, resulting in a reduction in surface hydrophobicity. This phenomenon may subsequently impact the early stage of bacterial adhesion and undermine biofilms’ typical formation. An alternative hypothesis is that the existence of these molecules on the surface decreased the available space for bacterial invasion (Nostro et al., 2012b). For example, a study found that adding carvacrol and curcumin improved the properties of Poly (Butylene Succinate)-based films. The films displayed significant antibiofilm activity and reduced biofilm formation by 8.22%–87.91%. Due to these properties, the authors suggested that these films can be used in food packaging, medical and pharmaceutical products, and related applications (Łopusiewicz et al., 2021).
The biofilm-reducing potency of carvacrol is not necessarily correlated with its biocidal properties. Experimental evidence has demonstrated that carvacrol can impede biofilms’ development without diminishing cell survival. Actually, carvacrol may involve something opposite to the immediate eradication of bacteria (Kachur and Suntres, 2020). It was hypothesized that carvacrol affects the gene coding for quorum sensing (QS). An essential set of regulatory genes involved in biofilm development include sarA, agrA, and agrB. AgrA and AgrB are the primary regulatory molecules of the QS system. Inhibiting their signaling impacts the maturation phase of the biofilm (Burt et al., 2014; Peng et al., 2023). The accessory gene regulator (agr) regulates the QS mechanism and the pathways involved in synthesizing the exopolysaccharide matrix. At sub-inhibitory concentration, carvacrol produced inhibitory effects on the expression of sarA and agrA (Figure1) (Valliammai et al., 2020b; Li et al., 2023; Martínez et al., 2023; Peng et al., 2023). By regulating agrA, carvacrol disrupts QS signaling and subsequently influences biofilm matrix synthesis (Martínez et al., 2023). As a global regulator of biofilm formation, staphylococcal accessory regulator A (SarA) upregulates ica operon expression and promotes biofilm development by binding to the ica promoter (Tormo María et al., 2005). The icaADBC operon encodes PIA, also known as poly-N-acetylglucosamine (PNAG), which is a significant component of the biofilm matrix in S. aureus (Jefferson et al., 2004). PIA/PNAG primary role is to facilitate intercellular aggregation, enhance bacterial attachment to the carrier surface, and enable immune evasion, therefore becoming the determining element in the adhesive aggregation stage of the biofilm (Peng et al., 2023).
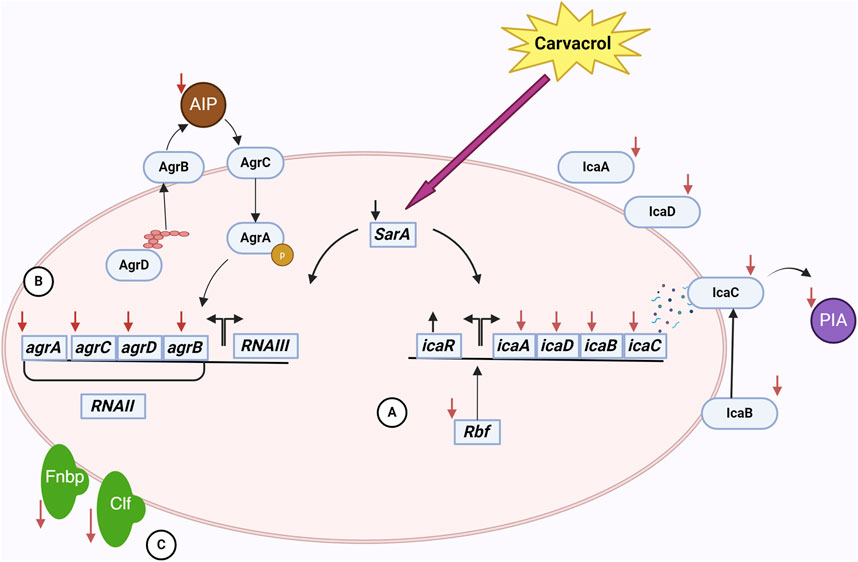
Figure 1. The interactions of carvacrol and S. aureus cell in a biofilm community. Carvacrol decreases the expression of the sarA gene. This gene affects the ica operon and the agr system. (A) By reducing the expression of icaADBC operon and increasing the expression of icaR following the use of carvacrol, the expression of proteins affecting the production, processing, and release of PIA/PNAG, an essential component of EPS, occurs. Also, reducing the expression of rbf by carvacrol has a negative effect on the ica operon. As a result, EPS and, subsequently, biofilm formation is affected. (B) Decreased expression of agr system genes following carvacrol treatment affects the function of the quorum sensing system. In this system, the production, processing, and release of AIP are done by the proteins of this system, and as a result of these changes, the production of AIP decreases. As a result, the communication of cells with each other is disturbed, adversely affecting biofilm formation. (C) Also, using carvacrol decreases the expression of fnbA and fnbB genes. It disrupts the function of ClfB, which results in a decrease in the attachment of bacteria to tissues and disruption in the early stages of biofilm formation.
In addition to PIA/PNAG, SarA also controls adhesion proteins FnbA and FnbB (Fibronectin-binding proteins A and B), which are essential for the attachment of bacterial cells (Brahma et al., 2019; Kot et al., 2019; Li et al., 2019; Valliammai et al., 2020b). As a consequence of the downregulation of SarA, the levels of icaA, icaD, fnbA, and fnbB were likewise reduced by the administration of carvacrol (Selvaraj et al., 2020; Alfaiz, 2021; Uc-Cachón et al., 2024). Moreover, carvacrol interacts with SarA through anionic bonding, subsequently affecting biofilm matrix synthesis (Selvaraj et al., 2020). Carvacrol also binds to clumping factor B (ClfB) with high affinity (Alfaiz, 2021). ClfB is an S. aureus protein that plays a crucial role in biofilm formation by adhering to host tissues through binding to ligands such as fibrinogen, cytokeratin 10, and other proteins. It can also act to mediate bacterial aggregation and thus enhance the ability of the bacteria to form structured biofilm. Therefore, carvacrol disrupts biofilm formation in this manner (Wertheim et al., 2008; Abraham and Jefferson, 2012).
Additionally, carvacrol negatively regulates the expression of the rbf gene (Martínez et al., 2023). The rbf gene enhances biofilm formation by stimulating the expression of the icaADBC operon, which subsequently leads to increased production of PIA/PNAG (Cue et al., 2009; Cue et al., 2012). Accordingly, by downregulating the rbf gene, carvacrol affects biofilm formation. An interesting result of the upregulation of icaR by carvacrol is that this gene encodes a transcriptional repressor that decreases the expression of the icaADBC operon, resulting in the suppression of PIA synthesis (Peng et al., 2023). By inhibiting the expression of icaA, icaB, icaD, icaC, sarA, fnbA, fnbB, rbf, and agrA, and upregulate icaR, carvacrol diminishes PIA/PNAG production, impedes bacterial adhesion, affects bacterial morphology, disrupts QS, and ultimately destabilizes the biofilm (Peng et al., 2023).
In the end, it is noteworthy to mention that cell death and decreased bacterial density leads to the reduction in the expression of QS activation of genes (Gonçalves et al., 2012; Espina et al., 2015; Gobin et al., 2022). Due to the lower initial bacterial counts, the concentration of autoinducers (small molecules secreted by bacteria) decreased. For activation of the QS response, the concentration of autoinducers exceeded a requisite threshold (Karatan and Watnick, 2009). Carvacrol significantly reduced the Autoinducer-2 (AI-2) of S. aureus biofilms. This inhibition of AI-2 activity helps reduce biofilm formation and bacterial virulence in S. aureus (Li et al., 2020).
The stability of biofilms is attributed to the presence of a matrix composed of extracellular polymeric substances (EPS) generated by bacteria. EPS are the primary constituents of bacterial biofilms and consist of polysaccharides, proteins, and nucleic acids (Krogsgård Nielsen et al., 2017; Nagaraj et al., 2017). EPS reduction may impact the biofilm’s structure and trigger bacterial susceptibility to external stimuli (Selvaraj et al., 2021). Carvacrol has been found to interfere with the synthesis of EPS, making the bacterial community more exposed to environmental threats (Li et al., 2020; Selvaraj et al., 2020). PIA/PNAG is one of the important components of EPS, and as previously mentioned, carvacrol can inhibit PIA/PNAG synthesis (Peng et al., 2023). Another component of EPS is extracellular DNA (eDNA); carvacrol can also reduce the production of eDNA (Li et al., 2023). Additionally, carvacrol inhibits the formation of biofilms by effects on membrane lipids, therefore preventing the buildup of proteins and stopping the microcolony stage (Knowles et al., 2005; Nostro et al., 2007; Miladi et al., 2016; Kasthuri et al., 2022). Furthermore, carvacrol reduces the synthesis of S. aureus slime (Sethupathy et al., 2017; Kannappan et al., 2019; Selvaraj et al., 2020). It is important to note that slime synthesis is crucial in biofilm formation (Daniela et al., 2014). Due to its relatively hydrophilic nature, carvacrol exhibits the ability to penetrate through biofilms, which alters their physical stability and destroys the enclosed bacteria (Ben Arfa et al., 2006; Nostro et al., 2012a; Suntres et al., 2015; Li et al., 2020). These results prove that carvacrol can disrupt the biofilm matrix and strengthen the bacterial removal process (Kasthuri et al., 2022).
Factors such as instability, volatility, and low water solubility might reduce the antibacterial effectiveness of essential oils and their components. Additionally, the direct use of carvacrol still faces restrictions (Hyldgaard et al., 2012; Scaffaro et al., 2018). To this end, novel approaches have been devised to generate active systems capable of enhancing the stability and extending the biological efficacy of carvacrol (Nostro et al., 2015; Scaffaro et al., 2018; Ayres Cacciatore et al., 2020; Cui et al., 2024). A practical approach to address this problem is the utilization of liposomes. Liposomes are sphere-shaped colloidal entities with phospholipid bilayer membranes and an interior aqueous compartment. They can encapsulate and regulate vital oil components’ release, enhancing stability and facilitating their biological effects (Desai et al., 2012; Cui et al., 2016a; Cui et al., 2016b). In a study, carvacrol and its isomer, thymol, were encapsulated in liposomes and examined against S. aureus and Salmonella enterica (Engel et al., 2017). The data obtained indicate a reduced release rate of encapsulated thymol/carvacrol. Short-term therapies with free carvacrol and thymol may be more effective in managing bacterial populations, particularly against S. aureus. However, due to their long-lasting antibacterial effects, encapsulated antimicrobials should be considered for disinfecting surfaces and equipment and using them as food preservatives (Pan et al., 2014; Cui et al., 2016a; Engel et al., 2017). In another study, carvacrol was incorporated into electrospun membranes of poly (lactic acid) (PLA) (Scaffaro et al., 2018). The progressive liberation of carvacrol from PLA membranes demonstrated substantial antibacterial efficacy over 144 h, reducing biofilm formation by 92%–96% and 88%–95% for S. aureus and Candida albicans in single and mixed cultures. Furthermore, a significant reduction in the number of cells, biomass, metabolic activity, and vitality of biofilms formed after 24 and 48 h was shown (Scaffaro et al., 2018). Therefore, as mentioned, the findings of recently published studies highlighted the potential of nanobiotechnology, specifically electrospun nanofibrous membranes, as a viable delivery system for carvacrol. This technology offers an ecological alternative in developing novel antibiofilm strategies and shows promise as an agent for controlling infections associated with S. aureus biofilms. Briefly, carvacrol disrupts biofilm formation through different mechanisms, including interference with QS, membrane disruption, inhibition of bacterial adhesion, matrix penetration, inhibition of EPS production, and gene expression changes. Therefore, these manifold effects make carvacrol a potent agent against S. aureus biofilms.
Curcumin
Curcumin is an orange-yellow pigment found in the rhizome of Curcuma longa (Borra et al., 2014). Curcumin exhibits a wide range of therapeutic effects, including antimicrobial, and antiseptic activities (Prakash et al., 2011; Kunnumakkara et al., 2017; Wang H. et al., 2019). Curcumin has been shown in recent research to effectively suppress the development of biofilms, particularly in Gram-positive bacteria (Moshe et al., 2011; Batista de Andrade Neto et al., 2021; Alqahtani et al., 2024). An in vitro study demonstrated that a 100 μg/mL concentration of curcumin successfully inhibits the development of S. aureus biofilm (Moshe et al., 2011). Noteworthy, curcumin has the potential to disrupt the structural integrity of the bacterial cell membrane before the initial stages of biofilm development, which include the attachment of cells to a surface, the assembly of cells to form micro colonies, and the maturation of the biofilm into a cohesive structure (Tan et al., 2019; Pamukçu et al., 2022). Additionally, curcumin can interfere with the planktonic cells and further inhibit biofilm reformation (Tan et al., 2019).
The previously published research findings indicated that the curcumin concentration needed to suppress biofilm formation was far lower than the dosage needed to suppress S. aureus growth. Accordingly, the authors proposed that the inhibitory effect of curcumin on biofilm formation is attributed to its ability to impede the process of biofilm formation itself rather than its bactericidal properties (Moshe et al., 2011). Therefore, curcumin has shown good potential by targeting bacterial adhesion and preventing biofilm formation. One of the possible mechanisms for this phenomenon is an interaction of curcumin with enzymes necessary for bacterial attachment to the host cells. For example, sortase A, an enzyme essential for the ability to attachment to host tissues, is one of these enzymes. By inhibiting sortase A, cells cannot bind to cell-matrix proteins, such as fibronectin, thus curcumin disrupting the process of adhesion (Park et al., 2005; Loo et al., 2016). Apart from targeting sortase A, the fnbA gene and clumping factor A (clfA) were downregulated by curcumin (Khaleghian et al., 2023). FnbA and ClfA facilitate bacterial aggregation and adherence to host tissues and surfaces by binding to fibronectin and fibrinogen. This interaction is essential for the initial stages of biofilm development (Lebeaux et al., 2013; Murai et al., 2016). Also, curcumin significantly decreases elastin-binding protein (ebp) expression, which plays a role in the binding of S. aureus to the host elastin protein and promotes bacterial attachment and invasion (Targhi et al., 2021). Consequently, curcumin interferes with the attachment of bacteria during biofilm formation.
Besides anti-adhesion activity, curcumin prevented biofilm formation by interfering with EPS synthesis. Effective interaction of curcumin with the biofilm-forming proteins of S. aureus results in reduced microbial biomass and generation of EPS, which are crucial for biofilm structure (Akhtar and Khan, 2021; Akhtar et al., 2021; Gao et al., 2023; Sharma et al., 2023). One of those that curcumin interacts with to affect biofilm formation is N-acetylglucosaminyl transferase (IcaD), a protein that produces PIA (Khaleghian et al., 2023). Additionally, a recently published study reported that curcumin downregulated the expression of the icaADBC operon genes (icaA, icaB, icaC, icaD) (Khaleghian et al., 2023). As mentioned earlier, the icaADBC operon encodes proteins and enzymes responsible for PIA synthesis. Therefore, by inhibiting this operon, curcumin interrupts the synthesis of PIA, the attachment of bacteria to each other and surfaces, and thus affects the formation and preservation of biofilm (Vuong et al., 2004). This change makes the biofilm more susceptible to mechanical removal and the action of antimicrobial agents.
Additionally, curcumin can reduce the expression of some genes associated with QS and enhance the proliferation of biofilms (Khaleghian et al., 2023; Sharma et al., 2023). An essential function of the agr system, which consists of AgrB, AgrC, AgrA, and AgrD, is to control virulence factors and biofilm development in S. aureus (Bezar et al., 2019). AgrB and AgrD are responsible for producing and processing autoinducible peptide (AIP) (Zhang et al., 2002; Zhang and Ji, 2004). AgrC is a histidine kinase receptor located in the bacterial cell membrane and detects the presence of AIP in the environment (Lina et al., 1998). When AgrC is activated, it phosphorylates AgrA. AgrA then upregulates or downregulates various target genes (Novick et al., 1995). Recent studies showed that curcumin downregulated genes responsible for QS, such as agrA, agrB, and agrC (Khaleghian et al., 2023; Sharma et al., 2023). Studies have shown that the suppression of agr system is important for developing biofilms, whereas the activation of the agr system is crucial for separating biofilms (Boles and Horswill, 2008; Dastgheyb et al., 2015).
Recently published studies have employed the combination of photodynamic therapy (PDT) and curcumin for S. aureus biofilm elimination (Table 1). In these studies, curcumin was used as a photosensitizer (PS) and produced reactive oxygen species (ROS) such as superoxide radicals and singlet oxygen molecules (1O2) (Akhtar et al., 2021; Sharma et al., 2023). ROS oxidizes the biomolecules of microorganisms, resulting in biological damage and decreasing microbial growth, metabolic activities, microbial biomass, and bacterial adhesion ability, and considerable changes in the carbohydrate and protein composition of the extracellular matrix of S. aureus (Ribeiro et al., 2022; Sharma et al., 2023). Bacterial cells include ample scavengers, including catalase, peroxidase, and superoxide dismutase, to counteract the bactericidal effects caused by free radicals. However, these scavengers cannot counteract the singlet oxygen molecule, resulting in extensive cell damage when exposed to 1O2 (Kim et al., 2001; Akhtar et al., 2021). This oxidative stress can damage bacterial cells and inhibit their ability to form and sustain biofilms (Sharma et al., 2023). In addition, the cost of curcumin compared to other photosensitizers is low (Araújo et al., 2014).
Considerable attempts have been undertaken to enhance the administration of curcumin (Alemi et al., 2018). For example, curcumin loaded on chitosan nanoparticles (CSNP) was used to improve the therapeutic performance of curcumin by increasing its bioavailability (Ma et al., 2020). CSNPs have attracted significant attention as a therapeutic carrier because of their biodegradability, biocompatibility, and freedom from toxicity (Das et al., 2010; Li et al., 2018). A positively charged CSNP can transport curcumin into biofilms and induce its release within the biofilm, affecting the cells therein. However, the inhibitory effect of CSNP-Cur on the biofilm development of S. aureus bacteria was somewhat weaker than that of free curcumin. The sustained release of curcumin from CSNP-Cur led to a reduced concentration and diminished antibiofilm action (Ma et al., 2020). However, the diffusion of free curcumin into a preformed biofilm is hindered by the EPS of the biofilm, thereby diminishing the antibiofilm effects of curcumin. Conversely, CSNP-Cur demonstrated superior antibiofilm efficacy compared to free curcumin (Ma et al., 2020). In another study, a niosome was used to encapsulate curcumin to solve the low solubility and stability issue. Niosomal curcumin exhibited a 2-4-fold reduction in multi-drug resistant (MDR) S. aureus biofilm relative to free curcumin (Khaleghian et al., 2023).
Additionally, in another study, curcumin was encapsulated in liposomes. In addition to promoting the uptake of this compound in bacterial cells, liposomes provide regulated release of medications. Encapsulating curcumin in liposomes halved its minimum inhibitory concentration (MIC) for S. aureus compared to the free form, and antibiofilm activity was observed at lower concentrations (Bhatia et al., 2021). Also, combining curcumin with metal ions can enhance its properties. Curcumin-based metallodrugs increase stabilization and improve curcumin’s bioavailability and solubility (Wanninger et al., 2015). Curcumin conjugating to RuII–polypyridyl complexes [Ru (bpy)2 (cur)] (PF6) showed promising results. Its MIC against S. aureus was 1 μg/mL and reduced the biofilm by 48% at 10 × MIC compared to the untreated (Srivastava et al., 2019). The aforementioned findings demonstrate that different drug delivery systems can be employed to augment the effectiveness of curcumin in suppressing biofilm formation. Nevertheless, the available data in this field are currently somewhat restricted, and it is imperative to conduct more comprehensive studies before the clinical application of curcumin-based drug delivery systems.
Curcumin has several ways to disrupt S. aureus biofilms, including inhibiting sortase A activity, interfering with attachment, changing bacterial surface properties, interacting with biofilm matrix, and inducing oxidative stress. Together, these factors diminish the ability of S. aureus to form or protect its biofilm communities, making them more sensitive to host defenses and traditional antimicrobial treatments.
Cinnamaldehyde
Cinnamaldehyde is a bioactive compound derived from cinnamon bark, known for its diverse spectrum of effects, including anticancer, antifungal, and antibacterial properties. It has been classified as GRAS by the Flavoring Extract Manufacturers’ Association and has been authorized by the FDA for use in food (Nostro et al., 2012b; Xu et al., 2022). In recent years, scientists have shown interest in utilizing cinnamon and its derivative components, particularly cinnamaldehyde, to suppress S. aureus biofilms, in addition to its antibacterial properties (Jia et al., 2011; Nostro et al., 2012b; Zodrow et al., 2012; Budri et al., 2015; Nostro et al., 2015; Campana et al., 2017; García-Salinas et al., 2018; Kot et al., 2018; Mishra et al., 2021; Wang et al., 2021; Kim Y. et al., 2022; Mastoor et al., 2022).
Cinnamaldehyde blocks ATPase and cell-wall biosynthesis and alters membrane structure and integrity to suppress bacteria, yeasts, and filamentous molds (Deng et al., 2018). The results of the Xu et al. study demonstrated that cinnamaldehyde induced the destruction of the cell wall of S. aureus and altered the permeability of the cell membrane, leading to the release of potassium ions, alkaline phosphatase, protein, and multiple other compounds (Xu et al., 2022). The results of this study indicated a continual increase in the extracellular potassium ion content in the bacterial solution treated with 1 × MIC of cinnamaldehyde, demonstrating the detrimental effects of this compound on the bacteria (Xu et al., 2022). In line with these findings, another study proposed that the mechanism by which cinnamaldehyde acts may be associated with cell death and/or the deactivation of bacterial virulence factors, regardless of showing high affinity or not to the non-native penicillin-binding protein (PBP2a) responsible for S. aureus (Fernandez-Soto et al., 2023).
In addition to the abovementioned research, several studies have investigated the molecular interactions between cinnamaldehyde and S. aureus biofilms. An investigation carried out by Mastoor et al. revealed that the application of α-methyl-trans-cinnamaldehyde and α-bromo-trans-cinnamaldehyde led to a notable reduction in the expression of icaA, clfA, and fnbA genes in the isolates that were treated. Given the crucial function of icaA in biofilm development in S. aureus, reducing its gene expression in the treated group could perhaps elucidate the mechanism by which cinnamaldehyde acts against biofilms (Knobloch et al., 2002). In addition, the adhesin proteins ClfA and FnbA, along with other MSCRAMMs, facilitate the early adherence of bacteria to surfaces and are present in all isolates of biofilm-forming S. aureus. Hence, the reduction in its expression offers a valuable understanding of the specific mechanism by which the chemical inhibits the development of biofilms (Mastoor et al., 2022). Furthermore, the metabolic activity of S. aureus in biofilm was considerably reduced when trans-cinnamaldehyde was present at 1/2 minimum biofilm inhibition concentration (MBIC). Both the weakly and highly adherent strains exhibited reduced expression levels of the genes encoding laminin-binding protein (eno), elastin-binding protein (ebps), and fibrinogen-binding protein (fib) in the presence of trans-cinnamaldehyde at 1/2 MBIC compared to the untreated biofilm. The expression level of icaA and icaD, which are involved in the manufacture of polysaccharide intercellular adhesion, was more than half lower in the poorly adhering strain with the presence of trans-cinnamaldehyde compared to biofilm without trans-cinnamaldehyde. The findings suggested that trans-cinnamaldehyde effectively inhibits the attachment of MRSA to key components of the extracellular matrix, including elastin and laminin. This inhibition thus hinders the spread of staphylococcal cells and the onset of colonization in host tissue. Thus, the authors postulated that trans-cinnamaldehyde shows potential as an anti-biofilm therapeutic for the treatment of MRSA biofilm-associated infection (Kot et al., 2019).
Finally, the results of the recently published study demonstrated that combining cinnamaldehyde and β-lactam antibiotics can synergistically enhance the activity and sensitivity of clinical MRSA isolates to β-lactam treatment while preventing MRSA biofilm formation. Mechanistic investigations revealed that the potentiating impact of cinnamaldehyde on β-lactams was primarily due to the suppression of mecA expression via the targeting of the staphylococcal accessory regulator sarA. Cinnamaldehyde alone or in combination with β-lactams reduced the sarA expression and enhanced the SarA protein’s phosphorylation. This process, in turn, hindered the binding of sarA to the mecA promoter element and suppressed the expression of virulence genes, including those responsible for biofilm formation, α-hemolysin, and adhesin. Impediment of sarA–mecA interaction disrupted PBP2a production, reducing MRSA resistance to β-lactams. Moreover, cinnamaldehyde completely reinstated the anti-MRSA effects of β-lactam antibiotics in live experimental models of bacteremia and biofilm infections in mice. The authors asserted that cinnamaldehyde functions as a β-lactam adjuvant and can be used as an alternate treatment to address multidrug-resistant MRSA infections (Li J. et al., 2024).
Various drug delivery platforms could be useful in improving cinnamaldehyde efficacy. Ramasamy et al. proposed that nanodispersions containing cinnamaldehyde (CNMA) may have exerted their effects by numerous mechanisms, including the inhibition of QS, attachment to cell walls facilitated by the lipophilic character of CNMA, interaction with cytoplasmic contents, release of CNMA, or induction of protein precipitation. Crucially, the activity of cinnamaldehyde attached to the surface of gold nanoparticles (CNMA-GNPs) was significantly higher than that of free CNMA. This finding provides evidence that nanodispersions enhance contact with biofilms. The authors also asserted that the small dimensions of CNMA-GNPs could enable them to penetrate the protective layers of EPS and effectively eliminate bacteria. Moreover, the low pH in biofilm environments can break down nanodispersions and facilitate the persistent release of CNMA (Ramasamy et al., 2017a; Ramasamy et al., 2017b).
Recently published studies reported antibacterial and antibiofilm activity for cinnamaldehyde against S. aureus. However, the exact interaction of cinnamaldehyde and this bacterium’s biofilm community is not yet elucidated. Hence, more confirmatory studies are needed in this field, and the usage of nanotechnology to improve the clinical usage of cinnamaldehyde should be considered in future studies.
Thymol
Thymol, also known as 2-isopropyl-5-methylphenol, is a monoterpene phenol that is widely distributed in several plant species, including Ocimum gratissimum, Thymus vulgaris, Thymus ciliates, Carum copticum, Thymus zygis, and Satureja intermedia (Nagoor Meeran et al., 2017). Thymol is categorized as GRAS by the FDA for use in foods for human consumption or as food additives (Jo et al., 2022). Studies have demonstrated the good antibacterial activity of thymol against various strains of bacteria, including S. aureus (Aksoy et al., 2020; Nunes et al., 2021). Furthermore, this compound showed antibiofilm activity against this bacterium in several studies (Nostro et al., 2007; Kifer et al., 2016; Peng et al., 2018; Aksoy et al., 2020; Kostoglou et al., 2020; Jo et al., 2022). For example, in one study, 0.33–0.59 mg/mL of thymol inhibited 90% of S. aureus biofilm formation (Kifer et al., 2016).
In a discussion on the antibiofilm activity of thymol, the primary effect is related to its impact on bacterial cell death. Thymol may induce membrane potential depolarization in S. aureus, impairing membrane integrity and cellular demise. Consequently, thymol induces an elevation in NADP + levels and a reduction in cytoplasmic NADPH and ATP. Such observation suggests the potential leakage of intracellular constituents and the disturbance of the physiological equilibrium between NADP+ and NADPH. Furthermore, thymol caused a substantial rise in the levels of lipid oxidation throughout the cell membrane (Gómez-Sequeda et al., 2020; Li et al., 2022a). Biofilms treated with thymol showed decreased bacteria and viable cells (Yuan et al., 2020; Jo et al., 2022; Uc-Cachón et al., 2024). In addition, inhibition of bacterial growth and proliferation is achieved by thymol by modification of membrane permeability, which disrupts both protein synthesis and binary fission (Yuan et al., 2020; Walczak et al., 2021). Therefore, with bacterial cell death, the number of cells required to form a biofilm decreases, and thus, the early stages of biofilm formation are disturbed.
As mentioned in the previous part, biofilm formation is initiated by the adhesion of planktonic microorganisms to surfaces and is regarded as a critical phase in the development of biofilms. Thymol significantly reduces the adhesion of S. aureus and thus suppresses the first stage of biofilm formation (Valliammai et al., 2020a; Jo et al., 2022). Additionally, thymol decreased the expression of fnbA and fnbB genes, which reduces the adhesion of S. aureus to the host tissue (Schröder et al., 2006; Valliammai et al., 2020a).
Biofilms are attached to surfaces by non-specific hydrophobic bonds. These bonds play an important role in the stability and adhesion of biofilms (Rouws et al., 2010; Ali Mirani et al., 2018). Any disruption in these hydrophobic bonds affects the ability of bacteria to attach to surfaces (Wojnicz et al., 2012). In this regard, thymol, as the main compound of Plectranthus amboinicus, affected the hydrophobicity of the surface of S. aureus, and the surface of bacterial cells became hydrophilic. These changes can affect the adhesion and aggregation of bacteria (Sawant et al., 2022). Therefore, thymol showed anti-adhesion properties that can be used in medical equipment (Bertuola et al., 2018; Valliammai et al., 2021). For example, to control the corrosion of AZ31 Mg alloy as a biodegradable implant and prevent bacterial adhesion, a polymer layer was developed through thymol electro polymerization (TOH). The bacterial adhesion on polyTOH-AZ31 was more than 30-fold smaller than the bare AZ31 alloy. Moreover, PolyTOH-AZ31 increased the effectiveness of antibiotics and inhibited planktonic growth at half of the MIC of the antibiotic (Bertuola et al., 2018).
Thymol can decrease the synthesis of PIA/PNAG as the main components of the EPS matrix in S. aureus biofilms (Valliammai et al., 2020a; Yuan et al., 2020; Jo et al., 2022; Uc-Cachón et al., 2024). A recent study indicated that bacteria without PIA/PNAG can initially attach to biomaterials but cannot develop a biofilm at later stages due to a significant decrease in cell-to-cell adhesion (Yuan et al., 2020). Thymol decreased the expression of sarA in S. aureus and inhibited the expression of other sarA-regulated genes, such as icaA and icaD (Valliammai et al., 2020a; Yuan et al., 2020; Valliammai et al., 2021; Kim B. C. et al., 2022). Notably, these genes significantly affected biofilm formation, and by reducing their expression, the synthesis of PIA, and consequently the formation of biofilm, was affected. In addition, thymol inhibited the release of eDNA, which plays key roles in bacterial adhesion, aggregation, microcolony formation, and biofilm architecture (Yuan et al., 2020). Moreover, thymol downregulated the cidA gene in S. aureus (Yuan et al., 2020). The holin-like protein (CidA) has been shown to positively increase the release of eDNA during biofilm development (Rice et al., 2007). Besides, thymol, due to its relative hydrophilic nature conferred by the free hydroxyl group, can permeate the polysaccharide matrix of the biofilm and may disrupt it due to its potent inherent antibacterial attributes (Nostro et al., 2007; Miladi et al., 2017; Kostoglou et al., 2020).
Like other natural compounds, the strong antimicrobial effect of thymol is practically limited by its high volatility, insolubility in water, and weak oxidative stability (Amiri et al., 2024). These factors restrict its usage in various practical applications. Therefore, scientists considered the use of new approaches. For instance, thymol loading in chitosan silver nanoparticles (T-C@AgNPs) showed excellent antibacterial activity with MIC = 100 μg/mL against MRSA. Moreover, T-C@AgNPs effectively reduced the attachment of bacteria and downregulated the transcription of the Coa, Eap, and SpA exoprotein genes. The decrease in the mentioned genes indicated a slow binding and a reduction in the coagulation mechanism (Manukumar et al., 2017). Another study has developed thymol-loaded chitosan nanogels (Ty-CsNG) against Gram-negative and Gram-positive MDR bacteria, including S. aureus. Ty-CsNG reduced the MIC by 4–6 times compared to free thymol. Moreover, antibiofilm activity and negligible cytotoxicity were observed (Piri-Gharaghie et al., 2022). Utilizing these methods leads to improved pharmacokinetic outcomes for thymol and expands the range of its applications in medicine. Noteworthy, other studies that used drug-platform to improve natural compounds efficacy against S. aureus biofilm are presented in Table 2.
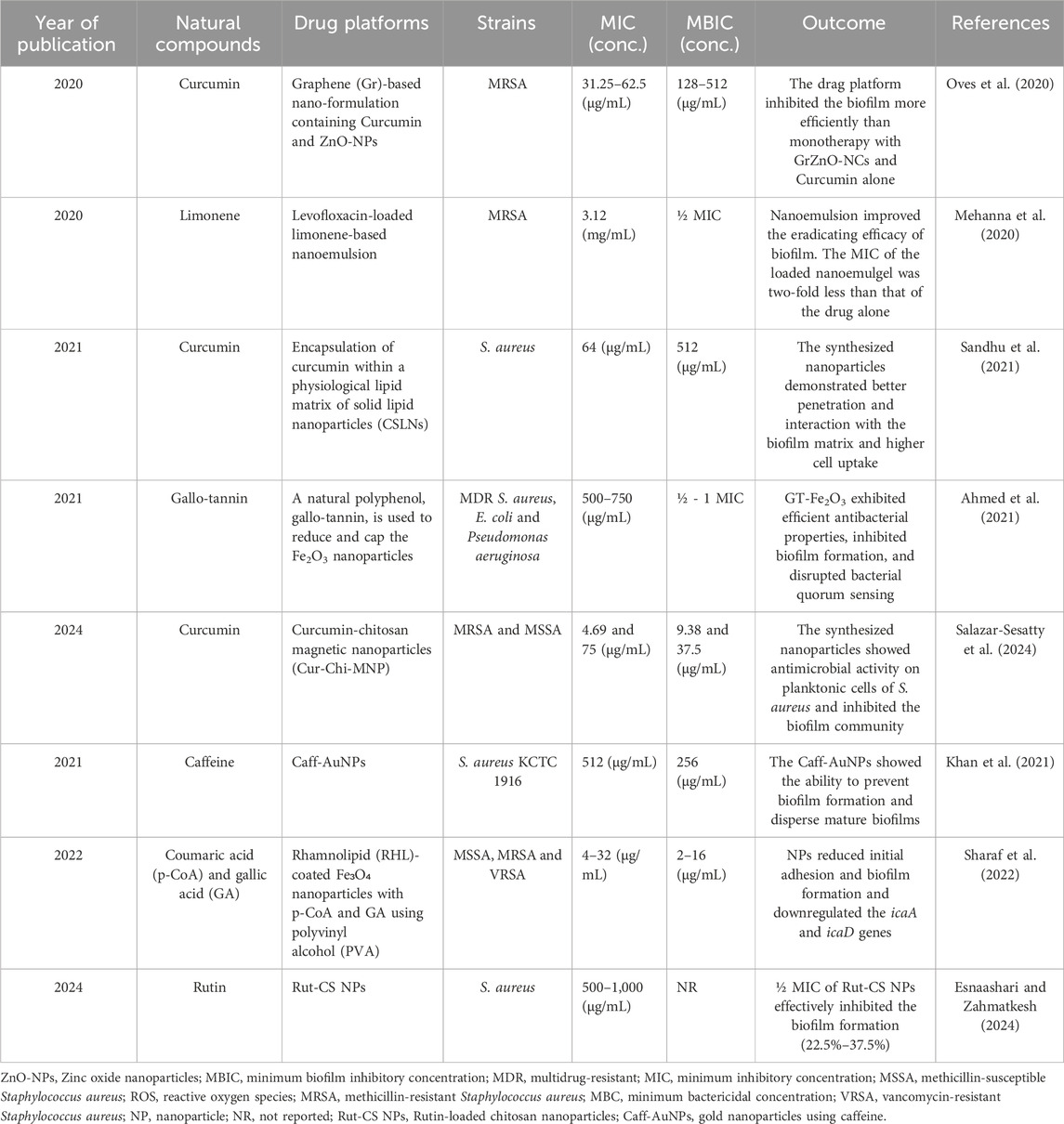
Table 2. Studies utilizing nanoparticles as a delivery platform for various natural compounds to inhibit and eradicate Staphylococcus aureus biofilm.
In the end, thymol can also be used in PDT (Wang Z. et al., 2019; Lu et al., 2021). Thymol acts as a “pro-photosensitizer” and is oxidized to thymoquinone (TQ) and thymohydroquinone (THQ) only in bacteria by blue light. The resultant TQ and THQ act as photosensitizers, enhancing ROS production exponentially and rapidly killing pathogens (Lu et al., 2021). ROS indiscriminately damages cellular components, including lipids, proteins, plasma membranes, and nucleic acids. The 1 × MIC thymol combined with 75 J/cm2 or 100 J/cm2 blue light could completely remove the viable biofilms of MRSA (Lu et al., 2021). In conventional PDT methods, the photosensitizer enters both bacterial and mammalian cells, generating ROS in both cell types, and posing safety and efficacy challenges. In contrast, thymol as a pro-photosensitizer is only converted to an active photosensitizer in bacteria, and thus, it has higher safety and therapeutic properties. It has the potential for application in topical therapy and biofilm-related treatments, preventing subsequent bacterial invasion or dissemination without causing any adverse effects on the host cells (Lu et al., 2021).
Ultimately, how thymol can suppress the formation of S. aureus biofilm includes bacterial death before biofilm formation, inhibiting bacterial movement and attachment, interfering with the structure of the biofilm matrix, and generating reactive oxygen species in photodynamic treatments. However, some drawbacks limit the clinical usage of this natural compound. To this end, scientists should consider using thymol-based drug platforms more when managing bacterial biofilm.
Eugenol
Eugenol, 4-allyl-2-methoxyphenol, is an odorous oily liquid extracted from specific essential oils, particularly clove and cinnamon, colorless to pale yellow. It has been a flavoring agent in food and cosmetic formulations (Zhang et al., 2018). Empirical investigations have demonstrated that eugenol possesses several potentially advantageous biological characteristics, such as antibacterial, antioxidant, and anti-inflammatory effects (Gill and Holley, 2004; Mohammed and Al-Bayati, 2009; Yadav et al., 2013). Additionally, several studies have demonstrated the eugenol potential for inhibiting and eradicating S. aureus biofilm (García-Salinas et al., 2018; Kostoglou et al., 2020). For instance, in one study, a 240–320 μg/mL concentration of eugenol eradicates 50% of S. aureus biofilm (Miladi et al., 2017).
Eugenol can decrease biofilm cell density by killing or inhibiting bacterial growth. When the density of biofilm cells decreases, aggregation and cell-to-cell connections also decrease so that the loosely arranged cells easily separate from each other (Yadav et al., 2015). Since eugenol is a lipophilic molecule, it can disturb the organization of several strata of polysaccharides, fatty acids, and phospholipids, therefore modifying the fluidity and permeability of the cell membrane and finally resulting in cell lysis (Yadav et al., 2015; Wijesinghe et al., 2021). This cell membrane destruction by eugenol has led to the cells’ rough and shrunken appearance, and bacterial cells lose their normal morphology (Yadav et al., 2015). Additionally, it interferes with the intracellular interactions that are crucial for the development of structured biofilms and the establishment of bacterial colonies. The perturbation of these structures can lead to the separation of cells within the biofilm, enabling their facile removal by washing (Yadav et al., 2015). The findings suggested that eugenol’s antibiofilm effect may be attributed to the suppression of cell-to-cell interactions and subsequent cell lysis.
Notably, eugenol exhibited antibiofilm effectiveness against S. aureus strains, particularly during the first stages of biofilm development (Kim and Chin, 2023). Biofilm disposal is most effective during the attachment phase of planktonic bacterial cells, which lasts from 0 to 5 h. During this period, at subinhibitory doses, eugenol demonstrated a substantial inhibitory effect on the adhesion ability of S. aureus (Apolónio et al., 2014; Kim and Chin, 2023). In addition, in the presence of eugenol, a reduction in the expression of the sarA gene was detected (Dunman et al., 2001; El-Far et al., 2021). As previously stated, this gene influences several virulence genes of S. aureus and the production of fibronectin, fibrinogen-binding proteins, and toxins. Consequently, it decreases cell adherence to tissues (Dunman et al., 2001; El-Far et al., 2021).
Moreover, the gene expression of clfA and fnbA, which mediate the initial attachment of bacteria to surfaces, is downregulated by eugenol (Mastoor et al., 2022). Additionally, another study reported that the Cna gene’s expression decreases in eugenol’s presence (Mastoor et al., 2022). The collagen-binding protein, Collagen Adhesin (Cna), allows S. aureus to adhere to collagen, a key component of the extracellular matrix in host tissues (Patti et al., 1994; Montanaro et al., 1999). All these events lead to a decrease in cell adhesion for biofilm formation. The death of plankton cells and the reduction of cell attachment for biofilm formation negatively affect the next stages of biofilm formation, including biomass production and communication between cells through QS. This property of eugenol can be used to create antimicrobial coatings and polymer films that inhibit the formation of bacterial biofilms on medical and industrial devices (Nostro et al., 2013; Holban et al., 2014; Venkateswaran et al., 2016). For example, one project involved the development of a chitosan-based antimicrobial coating, including embedded mesoporous silica nanoparticles (MSNs) to encapsulate and transport eugenol. The objective was to prevent the formation of biofilms on medical devices. The controlled release of eugenol from the MSNs and coatings occurred sequentially, starting with a low release, then reaching a peak, then decreasing, and finally reaching a plateau. In contrast to coatings lacking eugenol, which had minimal antibacterial properties and still permitted biofilm development after 24 h, the coating containing eugenol not only decreased biofilm formation but also effectively eliminated most of the bacteria attached (Nguyen et al., 2024).
In another study, researchers prepared nanofibers of polyvinylidene difluoride (PVDF) enriched with thymol and eugenol. These nanofibers demonstrated antifouling activity, suppressing biofilm formation by Escherichia coli and S. aureus, with no aggregation of bacterial cells observed. As a result, this method may address the disadvantage of the short lifespan of nanofibers as a filtration membrane due to clogging by bacteria in water treatment (Bartošová et al., 2022).
As mentioned, eugenol possesses both hydrophilic and hydrophobic properties, facilitating its diffusion in the biofilm matrix (Miladi et al., 2017; Kostoglou et al., 2020). As a result of this diffusion, eugenol can exert its effects on mature biofilms; for instance, the biomass of established biofilms was significantly decreased by the eugenol treatment (Melo et al., 2019; Wijesinghe et al., 2021; Kim and Chin, 2023). Furthermore, the eugenol-treated biofilms substantially reduced the population of live bacteria (Yadav et al., 2015; Melo et al., 2019; Kim and Chin, 2023). In the presence of eugenol, the components of EPS, namely, carbohydrates, protein, and nucleic acids, were significantly decreased (Ni et al., 2022). Also, in one study, DNA/RNA fragments, tryptophan, lipid, carotenoid, and amide of S. aureus biofilm in the presence of eugenol were significantly reduced (Kim and Chin, 2023). Furthermore, following eugenol treatment, a significant downregulation occurs in the gene expression of sarA, icaA, and icaD (Yadav et al., 2015; El-Far et al., 2021; Mastoor et al., 2022). As previously mentioned, these genes are involved in synthesizing PIA/PNAG, which is the main component of the EPS matrix in S. aureus biofilm. As a result of these alters, the integrity of the biofilm and its protective capacity and stability are reduced, and the biofilm becomes more sensitive to other external agents and antimicrobial substances.
In summary, eugenol influences the initial stages of biofilm formation by decreasing the number of viable cells before biofilm development and inhibiting their attachment to surfaces (Figure 2). Also, even after biofilm formation, eugenol can disrupt it. Like other natural compounds, eugenol has some disadvantages, such as low water solubility, poor physicochemical properties, chemical instability, and low bioavailability. These issues can be addressed by combining eugenol with nanoparticles or other drug platforms.
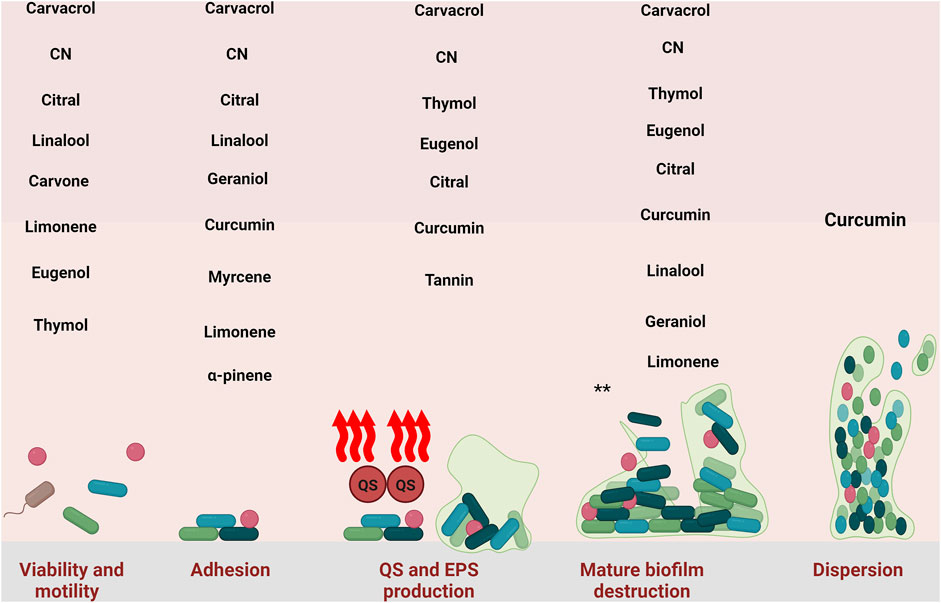
Figure 2. Inhibitory effect of natural compounds against different stage of S. aureus biofilm. CN: cinnamaldehyde. *: all of the natural compounds with detrimental effect against mature biofilm are: Carvacrol-thymol- Cinnamaldehyde, Eugenol, Curcumin, Citral, Linalool, Geraniol, Myrcene, Limonene, Myrtenyl Acetate, 1,8-Cineole, α-Pinene, Terpinolene, Linalyl acetate, α-Terpineol, Terpinen-4- ol, Tannin, and Ellagic acid.
Quercetin
Quercetin (3,5,7,3ʹ,4ʹ-pentahydroxy flavone) is classified under the flavonol subclass of flavonoids. Quercetin is prevalent in vegetables and fruits, including medicinal herbs like Hypericum perforatum, also called Ginkgo (Dengler et al., 2015). Studies have shown the antibacterial and antibiofilm properties of plant extracts containing this compound against S. aureus (Sharma et al., 2018; Radojević et al., 2023; de Oliveira et al., 2024). In a study, the MIC value of quercetin against S. aureus was found to be 256 μg/mL, and the MBIC value of this compound was determined to be 128 μg/mL (Wu et al., 2023).
Quercetin effectively compromised bacterial cell membranes and walls, resulting in deformation, cytoplasmic leakage, and cellular cavitation while not impacting division and proliferation (Kang et al., 2022; Nguyen and Bhattacharya, 2022). Additionally, this compound demonstrated an inhibitory effect on nucleic acid synthesis and the production of virulence factors in bacterial cells, resulting in a significant antibacterial action (Wang et al., 2018). In the presence of quercetin, both the biofilm thickness and the bacterial count within the biofilm diminished, resulting in a sheet-like dispersion of tiny clusters, with the biofilm manifesting as a single-layer cell aggregation (Lee et al., 2024; Liu et al., 2024).
Studies have shown that quercetin affects cell adhesion for biofilm formation (Kang et al., 2022; D'Arcangelo et al., 2024). Molecular docking and kinetic simulation showed that quercetin could bind ClfB (Kang et al., 2022). In addition, the expression of fnbA and fnbB altered and significantly downregulated when treated with quercetin (Wu et al., 2023). Quercetin significantly reduced the expression levels of srtA, which encodes sortase A enzyme, and the expression of sigB (sigma factor B) (Lee et al., 2013; Li Y. et al., 2024). σB is a product of the sigB operon and serves as the primary regulator of S. aureus response to environmental stress. This factor is crucial in developing bacterial drug resistance, the regulatory expression of virulence-associated genes, and biofilm formation (Peng et al., 2022). σB facilitates the synthesis of many cell surface proteins associated with the initial adherence of biofilms, including FnbA and ClfA. σB enhances the transcription of fnbA during early growth and markedly increases the transcription of clfA in late growth (Entenza et al., 2005). Therefore, by preventing the expression and function of proteins related to cell adhesion in S. aureus, one of the key stages of biofilm formation is affected and disrupted by quercetin.
Quercetin significantly decreased EPS synthesis and secretion (Li Y. et al., 2024; Liu et al., 2024). Additionally, the secretion of eDNA was significantly inhibited with increasing quercetin concentrations (Liu et al., 2024). Further study of the polysaccharide and protein percentages in EPS revealed that quercetin exerted a more pronounced influence on protein secretion (Li Y. et al., 2024).
The transcription levels of extracellular metalloproteinase Aur (aureolysin) and extracellular nuclease Nuc (nuclease) were markedly elevated following quercetin therapy (Liu et al., 2024). Extracellular proteases are crucial in the protein-dependent process. The augmented release of extracellular proteases restricts biofilm development, with metalloproteinase Aur exhibiting the most significant inhibitory effect (Loughran et al., 2014). The concentration of eDNA in the biofilm is modulated by Nuc, which can destroy eDNA and diminish biofilm formation (Kiedrowski et al., 2011). Also, it was reported that the transcription of aur and nuc is negatively regulated by SarA, while the expression of sarA is reduced by quercetin (Liu et al., 2024). Besides, as mentioned earlier, the expression of σB is reduced by quercetin, and on the other hand, σB affects the expression of sarA (Bischoff et al., 2001).
In addition to EPS, surface proteins, and eDNA, functional amyloids are one of the components of S. aureus biofilm matrix (Schwartz et al., 2012; Karygianni et al., 2020). Biofilm-associated protein (Bap) is a surface-associated protein that assumes an amyloid-like structure under specific environmental circumstances (Di Martino, 2016; Taglialegna et al., 2016). The protein may manifest as amyloid-like clumps on the bacterial surface, facilitating the formation of a robust biofilm structure. These persistent aggregates enable bacteria to cling to diverse surfaces and enhance their resistance to environmental conditions, including antibiotic exposure (Taglialegna et al., 2016). Quercetin inhibits S. aureus biofilm development by affecting the production of Bap amyloid-like aggregates without altering Bap expression (Matilla-Cuenca et al., 2020).
Quercetin acts as a quorum-quenching inhibitor. It obstructs bacterial communication by inhibiting the interaction between QS signaling molecules and their receptors, consequently diminishing bacterial motility, proliferation, and metabolic activity (Li Y. et al., 2024). For S. aureus, quercetin significantly reduced the expression levels of QS genes (agrA) (Lee et al., 2013; Wu et al., 2023; Li Y. et al., 2024). Additionally, as discussed earlier, the expression of sarA diminishes due to quercetin, and SarA can affect agr expression. Quercetin functioned as an exogenous inhibitor, suppressing interbacterial communication by modulating the expression of the AGR receptor protein gene in S. aureus, thereby managing the expression of downstream genes associated with biofilm formation, bacterial growth and metabolism and effectively diminishing biofilm secretion (Li Y. et al., 2024).
Simply inhibiting QS is insufficient to avert biofilm development. Quercetin may be utilized alongside antibiotics or other antibacterial agents to enhance their antibiofilm effectiveness (Vipin et al., 2020). For example, a study created bi-functional nanoparticles by co-assembling quercetin and copper ions. Copper eradicated bacteria by compromising the cell membrane’s integrity, whereas quercetin interfered with QS processes important for biofilm formation by downregulating the expression of specific genes, effectively inhibiting biofilm development (Cheng et al., 2024).
Like other flavonoids, quercetin exhibits prevalent issues associated with natural bioactive compounds, including inadequate water solubility and diminished bioavailability (Sun et al., 2015). Therefore, various types of drug delivery methods have been studied to overcome this problem, such as hydrogels, nano-micelles, nanoliposomes, and nanoparticles (Akhlaghi and Najafpour-Darzi, 2023; Nain et al., 2023; Yang et al., 2025). For instance, a study concentrated on synthesizing quercetin-encapsulated chitosan sodium alginate nanoparticles (Q-CSNPs). Q-CSNPs employed against E. coli and S. aureus. The findings indicated that quercetin nanoparticles may suppress or eliminate the bacterial biofilm, regardless of whether treatment occurred before or following biofilm formation. Furthermore, Q-CSNPs demonstrated significant antioxidant ability and notably affected planarians’ oxidative stress (Sun et al., 2024). In another study, hyaluronic acid-modified azithromycin/quercetin micelles (HA-AZI/Qe-M) were produced using thin film hydration. HA-AZI/Qe-M exhibited remarkable antibacterial efficacy in vitro and showed the capacity to penetrate deeply into the MRSA biofilm, effectively inhibiting and eradicating it. Moreover, following treatment with HA-AZI/Qe-M, the bacterial count in the thigh muscle tissue of mice was dramatically diminished (Zhang et al., 2024). In the end, the poly (ε-caprolactone)-monomethoxyl poly (ethylene glycol) (PCL-mPEG) micelles, loaded with quercetin and rifampicin (QRMs), were synthesized. The results indicated that the small-sized QRMs may infiltrate the inside of MRSA biofilm to disperse and eliminate it. Subsequently, antibiotics are discharged and concentrated within the acidic biofilm milieu. QRMs may eradicate germs by enhancing bacterial membrane permeability and modifying membrane potential and fluidity. Furthermore, QRMs enhanced drugs’ intracellular and cytoplasmic transport efficiency to epithelial cells (Chen et al., 2022).
In short, quercetin exerts its inhibitory effect on S. aureus biofilm by inhibiting bacterial growth, disrupting cell adhesion, reducing the biofilm matrix, altering the expression of genes involved in biofilm formation, and preventing the proper function of QS. Additionally, to enhance its efficiency and reduce its limitations, it can be combined with other drugs and incorporated into drug delivery platforms.
In the end, it is noteworthy that other natural compounds that showed inhibitory effects against S. aureus biofilm are presented in Table 3.

Table 3. Numerous investigations have utilized diverse natural compounds to hinder and break down the biofilm formed by S. aureus.
Conclusion
Using natural compounds as an anti-biofilm treatment for S. aureus demonstrated significant potential for developing new therapeutic approaches. These compounds affect various stages of biofilm formation, including the QS system, biofilm matrix, the attachment of S. aureus cells to surfaces and tissues, and the viability of bacteria. Furthermore, these compounds exhibit lower toxicity than traditional antibacterial agents, and because they have multiple purposes, there is less chance of resistance to them occurring. While natural compounds have shown significant potential in laboratory tests, more research is needed to determine their effectiveness in vivo. We should not forget that in nature and several other habitats (e.g., the food industry and healthcare), biofilms may be composed of different types of microorganisms that interact with each other in relatively complex ways. Exposure to multispecies biofilms requires investigation. As mentioned, natural compounds have disadvantages such as low bioavailability, insolubility in water, and rapid metabolism and degradation; hence, further studies are needed to optimize their delivery methods. For example, delivery systems based on nanoparticles can improve their penetration into biofilms and increase their stability in complex environments. In addition, their combination with antibiotics and other natural agents can lead to synergistic effects and increase their ability to disrupt the biofilm. Finally, natural compounds–based -photodynamic therapy should also be considered by scientists as a promising approach for managing the biofilm community of S. aureus. Therefore, natural compounds are an effective and low-risk option promising to manage S. aureus biofilm-related issues.
Author contributions
MK: Investigation, Software, Writing–original draft. MN: Investigation, Methodology, Writing–original draft. ZC: Investigation, Software, Writing–original draft, Writing–review and editing. AS: Conceptualization, Methodology, Writing–original draft, Writing–review and editing.
Funding
The author(s) declare that no financial support was received for the research, authorship, and/or publication of this article.
Acknowledgments
We greatly appreciate the input from the BioRender team (BioRender.com) for their collaboration with us in figure design.
Conflict of interest
The authors declare that the research was conducted in the absence of any commercial or financial relationships that could be construed as a potential conflict of interest.
Publisher’s note
All claims expressed in this article are solely those of the authors and do not necessarily represent those of their affiliated organizations, or those of the publisher, the editors and the reviewers. Any product that may be evaluated in this article, or claim that may be made by its manufacturer, is not guaranteed or endorsed by the publisher.
Abbreviations
MSCRAMMs, Microbial Surface Component Recognizing Adhesive Matrix Molecules; MRSA, methicillin-resistant S. aureus; PIA, polysaccharide intercellular adhesins; NPs, nanoparticles; QS; quorum sensing; EPS, extracellular polymeric substances; eDNA, extracellular DNA; PLA, poly (lactic acid); PS, photosensitizer; ROS, reactive oxygen species; NPs, Nanoparticles; MIC, minimum inhibitory concentration.
References
Abd El-Hamid, M. I., El-Tarabili, R. M., Bahnass, M. M., Alshahrani, M. A., Saif, A., Alwutayd, K. M., et al. (2023). Partnering essential oils with antibiotics: proven therapies against bovine Staphylococcus aureus mastitis. Front. Cell Infect. Microbiol. 13, 1265027. doi:10.3389/fcimb.2023.1265027
Abdel Samad, R., El Darra, N., Al Khatib, A., Chacra, H. A., Jammoul, A., and Raafat, K. (2023). Novel dual-function GC/MS aided ultrasound-assisted hydrodistillation for the valorization of Citrus sinensis by-products: phytochemical analysis and anti-bacterial activities. Sci. Rep. 13 (1), 12547. doi:10.1038/s41598-023-38130-9
Abo-Elghiet, F., Rushdi, A., Ibrahim, M. H., Mahmoud, S. H., Rabeh, M. A., Alshehri, S. A., et al. (2023). Chemical profile, antibacterial, antibiofilm, and antiviral activities of pulicaria crispa most potent fraction: an in vitro and in silico study. Molecules 28 (10), 4184. doi:10.3390/molecules28104184
Abraham, N. M., and Jefferson, K. K. (2012). Staphylococcus aureus clumping factor B mediates biofilm formation in the absence of calcium. Microbiol. Read. 158 (Pt 6), 1504–1512. doi:10.1099/mic.0.057018-0
Ahmed, B., Syed, A., Ali, K., Elgorban, A. M., Khan, A., Lee, J., et al. (2021). Synthesis of gallotannin capped iron oxide nanoparticles and their broad spectrum biological applications. RSC Adv. 11 (17), 9880–9893. doi:10.1039/d1ra00220a
Aiemsaard, J., Aiumlamai, S., Aromdee, C., Taweechaisupapong, S., and Khunkitti, W. (2011). The effect of lemongrass oil and its major components on clinical isolate mastitis pathogens and their mechanisms of action on Staphylococcus aureus DMST 4745. Res. Vet. Sci. 91 (3), e31–e37. doi:10.1016/j.rvsc.2011.01.012
Akhlaghi, N., and Najafpour-Darzi, G. (2023). Thermosensitive injectable dual drug-loaded chitosan-based hybrid hydrogel for treatment of orthopedic implant infections. Carbohydr. Polym. 320, 121138. doi:10.1016/j.carbpol.2023.121138
Akhtar, F., and Khan, A. U. (2021). Antimicrobial photodynamic therapy (aPDT) against vancomycin resistant Staphylococcus aureus (VRSA) biofilm disruption: a putative role of phagocytosis in infection control. Photodiagnosis Photodyn. Ther. 36, 102552. doi:10.1016/j.pdpdt.2021.102552
Akhtar, F., Khan, A. U., Misba, L., Akhtar, K., and Ali, A. (2021). Antimicrobial and antibiofilm photodynamic therapy against vancomycin resistant Staphylococcus aureus (VRSA) induced infection in vitro and in vivo. Eur. J. Pharm. Biopharm. 160, 65–76. doi:10.1016/j.ejpb.2021.01.012
Aksoy, C. S., Avci, F. G., Ugurel, O. M., Atas, B., Sayar, N. A., and Sariyar Akbulut, B. (2020). Potentiating the activity of berberine for Staphylococcus aureus in a combinatorial treatment with thymol. Microb. Pathog. 149, 104542. doi:10.1016/j.micpath.2020.104542
Alarjani, K. M., and Skalicky, M. (2021). Antimicrobial resistance profile of Staphylococcus aureus and its in-vitro potential inhibition efficiency. J. Infect. Public Health 14 (12), 1796–1801. doi:10.1016/j.jiph.2021.10.018
Albutti, A., Gul, M. S., Siddiqui, M. F., Maqbool, F., Adnan, F., Ullah, I., et al. (2021). Combating biofilm by targeting its formation and dispersal using gallic acid against single and multispecies bacteria causing dental plaque. Pathogens 10 (11), 1486. doi:10.3390/pathogens10111486
Alemi, A., Zavar Reza, J., Haghiralsadat, F., Zarei Jaliani, H., Haghi Karamallah, M., Hosseini, S. A., et al. (2018). Paclitaxel and curcumin coadministration in novel cationic PEGylated niosomal formulations exhibit enhanced synergistic antitumor efficacy. J. Nanobiotechnology 16 (1), 28. doi:10.1186/s12951-018-0351-4
Alfaiz, F. A. (2021). Molecular studies of immunological enzyme clumping factor B for the inhibition of Staphylococcus aureus with essential oils of Nigella sativa. J. Mol. Recognit. 34 (12), e2941. doi:10.1002/jmr.2941
Ali Mirani, Z., Fatima, A., Urooj, S., Aziz, M., Khan, M., and Abbas, T. (2018). Relationship of cell surface hydrophobicity with biofilm formation and growth rate: a study on Pseudomonas aeruginosa, Staphylococcus aureus, and Escherichia coli. J Iran. J. Basic Med. Sci. 21 (7), 760–769. doi:10.22038/ijbms.2018.28525.6917
Alqahtani, M., Almukainzi, M., Alghoribi, M. F., and El-Mahdy, A. M. (2024). Antivirulence effects of trans-resveratrol and curcumin on methicillin-resistant Staphylococcus aureus (MRSA) from Saudi arabia. Life (Basel) 14 (4), 491. doi:10.3390/life14040491
Alves, F., Gomes Guimarães, G., Mayumi Inada, N., Pratavieira, S., Salvador Bagnato, V., and Kurachi, C. (2021). Strategies to improve the antimicrobial efficacy of photodynamic, sonodynamic, and sonophotodynamic therapies. Lasers Surg. Med. 53 (8), 1113–1121. doi:10.1002/lsm.23383
Alves, F., Pratavieira, S., Inada, N. M., Barrera Patiño, C. P., and Kurachi, C. (2023). Effects on colonization factors and mechanisms involved in antimicrobial sonophotodynamic inactivation mediated by curcumin. Pharmaceutics 15 (10), 2407. doi:10.3390/pharmaceutics15102407
Amiri, S., Sepahvand, S., Radi, M., and Abedi, E. (2024). A comparative study between the performance of thymol-nanoemulsion and thymol-loaded nanostructured lipid carriers on the textural, microbial, and sensory characteristics of sausage. Curr. Res. Food Sci. 8, 100704. doi:10.1016/j.crfs.2024.100704
Apinundecha, C., Teethaisong, Y., Suknasang, S., Ayamuang, I. O., and Eumkeb, G. (2023). Synergistic interaction between boesenbergia rotunda (L.) mansf. Essential oil and cloxacillin on methicillin-resistant Staphylococcus aureus (MRSA) inhibition. Evid. Based Complement. Altern. Med. 2023, 3453273. doi:10.1155/2023/3453273
Apolónio, J., Faleiro, M. L., Miguel, M. G., and Neto, L. (2014). No induction of antimicrobial resistance in Staphylococcus aureus and Listeria monocytogenes during continuous exposure to eugenol and citral. Fems Microbiol. Lett. 354 (2), 92–101. doi:10.1111/1574-6968.12440
Aprotosoaie, A. C., Luca, V. S., Trifan, A., and Miron, A. (2019). “Chapter 7 - antigenotoxic potential of some dietary non-phenolic phytochemicals,” in Studies in natural products chemistry. Editor R. Atta Ur (Elsevier), 223–297.
Araújo, N. C., Fontana, C. R., Bagnato, V. S., and Gerbi, M. E. (2014). Photodynamic antimicrobial therapy of curcumin in biofilms and carious dentine. Lasers Med. Sci. 29 (2), 629–635. doi:10.1007/s10103-013-1369-3
Araújo, T. S. D., Rodrigues, P. L. F., Santos, M. S., De Oliveira, J. M., Rosa, L. P., Bagnato, V. S., et al. (2018). Reduced methicillin-resistant Staphylococcus aureus biofilm formation in bone cavities by photodynamic therapy. Photodiagnosis Photodyn. Ther. 21, 219–223. doi:10.1016/j.pdpdt.2017.12.011
Ayres Cacciatore, F., Dalmás, M., Maders, C., Ataíde Isaía, H., Brandelli, A., and Da Silva Malheiros, P. (2020). Carvacrol encapsulation into nanostructures: characterization and antimicrobial activity against foodborne pathogens adhered to stainless steel. Food Res. Int. 133, 109143. doi:10.1016/j.foodres.2020.109143
Bakkiyaraj, D., Nandhini, J. R., Malathy, B., and Pandian, S. K. (2013). The anti-biofilm potential of pomegranate (Punica granatum L.) extract against human bacterial and fungal pathogens. Biofouling 29 (8), 929–937. doi:10.1080/08927014.2013.820825
Bartošová, L., Sedlaříková, J., Peer, P., Janalíková, M., and Pleva, P. (2022). Antibacterial and antifouling efficiency of essential oils-loaded electrospun polyvinylidene difluoride membranes. Int. J. Mol. Sci. 24 (1), 423. doi:10.3390/ijms24010423
Baser, K. H. (2008). Biological and pharmacological activities of carvacrol and carvacrol bearing essential oils. Curr. Pharm. Des. 14 (29), 3106–3119. doi:10.2174/138161208786404227
Batista De Andrade Neto, J., Pessoa De Farias Cabral, V., Brito Nogueira, L. F., Rocha Da Silva, C., Gurgel Do Amaral Valente Sá, L., Ramos Da Silva, A., et al. (2021). Anti-MRSA activity of curcumin in planktonic cells and biofilms and determination of possible action mechanisms. Microb. Pathog. 155, 104892. doi:10.1016/j.micpath.2021.104892
Ben Arfa, A., Combes, S., Preziosi-Belloy, L., Gontard, N., and Chalier, P. (2006). Antimicrobial activity of carvacrol related to its chemical structure. Lett. Appl. Microbiol. 43 (2), 149–154. doi:10.1111/j.1472-765X.2006.01938.x
Bertuola, M., Miñán, A., Grillo, C. A., Cortizo, M. C., and Fernández Lorenzo De Mele, M. A. (2018). Corrosion protection of AZ31 alloy and constrained bacterial adhesion mediated by a polymeric coating obtained from a phytocompound. Colloids Surf. B Biointerfaces 172, 187–196. doi:10.1016/j.colsurfb.2018.08.025
Bezar, I. F., Mashruwala, A. A., Boyd, J. M., and Stock, A. M. (2019). Drug-like fragments inhibit agr-mediated virulence expression in Staphylococcus aureus. Sci. Rep. 9 (1), 6786. doi:10.1038/s41598-019-42853-z
Bhatia, E., Sharma, S., Jadhav, K., and Banerjee, R. (2021). Combinatorial liposomes of berberine and curcumin inhibit biofilm formation and intracellular methicillin resistant Staphylococcus aureus infections and associated inflammation. J. Mater Chem. B 9 (3), 864–875. doi:10.1039/d0tb02036b
Bischoff, M., Entenza, J. M., and Giachino, P. (2001). Influence of a functional sigB operon on the global regulators sar and agr in Staphylococcus aureus. J. Bacteriol. 183 (17), 5171–5179. doi:10.1128/jb.183.17.5171-5179.2001
Boles, B. R., and Horswill, A. R. (2008). Agr-mediated dispersal of Staphylococcus aureus biofilms. Plos Pathog. 4 (4), e1000052. doi:10.1371/journal.ppat.1000052
Borra, S. K., Mahendra, J., Gurumurthy, P., Iqbal, S. S., and Mahendra, L. (2014). Effect of curcumin against oxidation of biomolecules by hydroxyl radicals. J. Clin. Diagn Res. 8 (10), Cc01–05. doi:10.7860/jcdr/2014/8517.4967
Boutoub, O., El-Guendouz, S., Matos, I., El Ghadraoui, L., Costa, M. C., Carlier, J. D., et al. (2024). Chemical characterization and biological properties assessment of Euphorbia resinifera and Euphorbia officinarum Moroccan propolis. Antibiot. (Basel) 13 (3), 230. doi:10.3390/antibiotics13030230
Brahma, U., Sharma, P., Murthy, S., Sharma, S., Chakraborty, S., Appalaraju, S. N., et al. (2019). Decreased expression of femXAB genes and fnbp mediated biofilm pathways in OS-MRSA clinical isolates. Sci. Rep. 9 (1), 16028. doi:10.1038/s41598-019-52557-z
Budri, P. E., Silva, N. C., Bonsaglia, E. C., Fernandes Júnior, A., Araújo Júnior, J. P., Doyama, J. T., et al. (2015). Effect of essential oils of Syzygium aromaticum and Cinnamomum zeylanicum and their major components on biofilm production in Staphylococcus aureus strains isolated from milk of cows with mastitis. J. Dairy Sci. 98 (9), 5899–5904. doi:10.3168/jds.2015-9442
Budzyńska, A., Wieckowska-Szakiel, M., Sadowska, B., Kalemba, D., and Rózalska, B. (2011). Antibiofilm activity of selected plant essential oils and their major components. Pol. J. Microbiol. 60 (1), 35–41. doi:10.33073/pjm-2011-005
Burt, S. (2004). Essential oils: their antibacterial properties and potential applications in foods--a review. Int. J. Food Microbiol. 94 (3), 223–253. doi:10.1016/j.ijfoodmicro.2004.03.022
Burt, S. A., Ojo-Fakunle, V. T., Woertman, J., and Veldhuizen, E. J. (2014). The natural antimicrobial carvacrol inhibits quorum sensing in Chromobacterium violaceum and reduces bacterial biofilm formation at sub-lethal concentrations. Plos One 9 (4), e93414. doi:10.1371/journal.pone.0093414
Campana, R., Casettari, L., Fagioli, L., Cespi, M., Bonacucina, G., and Baffone, W. (2017). Activity of essential oil-based microemulsions against Staphylococcus aureus biofilms developed on stainless steel surface in different culture media and growth conditions. Int. J. Food Microbiol. 241, 132–140. doi:10.1016/j.ijfoodmicro.2016.10.021
Caputo, L., Capozzolo, F., Amato, G., De Feo, V., Fratianni, F., Vivenzio, G., et al. (2022). Chemical composition, antibiofilm, cytotoxic, and anti-acetylcholinesterase activities of Myrtus communis L. leaves essential oil. BMC Complement. Med. Ther. 22 (1), 142. doi:10.1186/s12906-022-03583-4
Caputo, L., Smeriglio, A., Trombetta, D., Cornara, L., Trevena, G., Valussi, M., et al. (2020). Chemical composition and biological activities of the essential oils of Leptospermum petersonii and Eucalyptus gunnii. Front. Microbiol. 11, 409. doi:10.3389/fmicb.2020.00409
Center for Food, S., and Applied, N. (2006). EAFUS: a food additive database. Washington DC: US Food and Drug Administration, Center for Food and Applied Nutrition.
Chen, Y., Zhao, Q., Han, J., Lan, X., Che, J., Chen, M., et al. (2022). Dual drug loaded pH-sensitive micelles for efficient bacterial infection treatment. Pharm. Res. 39 (6), 1165–1180. doi:10.1007/s11095-022-03182-5
Cheng, J., Zhang, H., Lu, K., Zou, Y., Jia, D., Yang, H., et al. (2024). Bi-functional quercetin/copper nanoparticles integrating bactericidal and anti-quorum sensing properties for preventing the formation of biofilms. Biomater. Sci. 12 (7), 1788–1800. doi:10.1039/d4bm00034j
Chipenzi, T., Baloyi, G., Mudondo, T., Sithole, S., Fru Chi, G., and Mukanganyama, S. (2020). An evaluation of the antibacterial properties of tormentic acid congener and extracts from callistemon viminalis on selected ESKAPE pathogens and effects on biofilm formation. Adv. Pharmacol. Pharm. Sci. 2020, 8848606. doi:10.1155/2020/8848606
Cue, D., Lei, M. G., Luong, T. T., Kuechenmeister, L., Dunman, P. M., O'donnell, S., et al. (2009). Rbf promotes biofilm formation by Staphylococcus aureus via repression of icaR, a negative regulator of icaADBC. J. Bacteriol. 191 (20), 6363–6373. doi:10.1128/jb.00913-09
Cue, D. R., Lei, M. G., and Lee, C. (2012). Genetic regulation of the intercellular adhesion locus in staphylococci. Front. Cell Infect. Microbiol. 2, 38. doi:10.3389/fcimb.2012.00038
Cui, H., Li, W., Li, C., Vittayapadung, S., and Lin, L. (2016a). Liposome containing cinnamon oil with antibacterial activity against methicillin-resistant Staphylococcus aureus biofilm. Biofouling 32 (2), 215–225. doi:10.1080/08927014.2015.1134516
Cui, H., Zhou, H., and Lin, L. (2016b). The specific antibacterial effect of the Salvia oil nanoliposomes against Staphylococcus aureus biofilms on milk container. Food control. 61, 92–98. doi:10.1016/j.foodcont.2015.09.034
Cui, Z., Chen, Y., Song, S., Wang, J., Wei, Y., Wu, X., et al. (2024). A carrier-free, injectable, and self-assembling hydrogel based on carvacrol and glycyrrhizin exhibits high antibacterial activity and enhances healing of MRSA-infected wounds. Colloids Surf. B Biointerfaces 241, 114068. doi:10.1016/j.colsurfb.2024.114068
Daniela, E., Alejandra, C., Pedro, R., Claudia, M., Lucía, A., Carlos, T., et al. (2014). Antibacterial activity of mulinum spinosum extracts against slime-producing Staphylococcus aureus and methicillin-resistant Staphylococcus aureus isolated from nasal carriers. ScientificWorldJournal 2014 (1), 342143. doi:10.1155/2014/342143
D'arcangelo, S., Di Fermo, P., Diban, F., Ferrone, V., D'ercole, S., Di Giulio, M., et al. (2024). Staphylococcus aureus/Staphylococcus epidermidis from skin microbiota are balanced by Pomegranate peel extract: an eco-sustainable approach. Plos One 19 (8), e0308211. doi:10.1371/journal.pone.0308211
Darmasiwi, S., Aramsirirujiwet, Y., and Kimkong, I. (2022). Antibiofilm activity and bioactive phenolic compounds of ethanol extract from the Hericium erinaceus basidiome. J. Adv. Pharm. Technol. Res. 13 (2), 111–116. doi:10.4103/japtr.japtr_1_22
Das, R. K., Kasoju, N., and Bora, U. (2010). Encapsulation of curcumin in alginate-chitosan-pluronic composite nanoparticles for delivery to cancer cells. Nanomedicine 6 (1), 153–160. doi:10.1016/j.nano.2009.05.009
Das, S., Paul, P., Chatterjee, S., Chakraborty, P., Sarker, R. K., Das, A., et al. (2021). Piperine exhibits promising antibiofilm activity against Staphylococcus aureus by accumulating reactive oxygen species (ROS). Arch. Microbiol. 204 (1), 59. doi:10.1007/s00203-021-02642-7
Dastgheyb, S. S., Villaruz, A. E., Le, K. Y., Tan, V. Y., Duong, A. C., Chatterjee, S. S., et al. (2015). Role of phenol-soluble modulins in formation of Staphylococcus aureus biofilms in synovial fluid. Infect. Immun. 83 (7), 2966–2975. doi:10.1128/iai.00394-15
Deng, J., Wang, G., Li, J., Zhao, Y., and Wang, X. (2018). Effects of cinnamaldehyde on the cell wall of A. fumigatus and its application in treating mice with invasive pulmonary aspergillosis. Evid. Based Complement. Altern. Med. 2018, 5823209. doi:10.1155/2018/5823209
Dengler, V., Foulston, L., Defrancesco Alicia, S., and Losick, R. (2015). An electrostatic net model for the role of extracellular DNA in biofilm formation by Staphylococcus aureus. J. Bacteriol. 197 (24), 3779–3787. doi:10.1128/jb.00726-15
De Oliveira, L. D., Ribeiro, A. L. M., Dias, S. O., Da Cruz, G. M., De Menezes, R. T., De Carvalho, L. S., et al. (2024). Phytochemical composition and antimicrobial and antibiofilm effect of myrciaria cauliflora hydroethanolic extract against Staphylococcus aureus and acinetobacter baumannii. Methods Protoc. 7 (4), 60. doi:10.3390/mps7040060
Desai, M. A., Soni, K. A., Nannapaneni, R., Schilling, M. W., and Silva, J. L. (2012). Reduction of Listeria monocytogenes biofilms on stainless steel and polystyrene surfaces by essential oils. J. Food Prot. 75 (7), 1332–1337. doi:10.4315/0362-028x.Jfp-11-517
Di Martino, P. (2016). Bap: a new type of functional amyloid. Trends Microbiol. 24 (9), 682–684. doi:10.1016/j.tim.2016.07.004
Doke, S. K., Raut, J. S., Dhawale, S., and Karuppayil, S. M. (2014). Sensitization of Candida albicans biofilms to fluconazole by terpenoids of plant origin. J. Gen. Appl. Microbiol. 60 (5), 163–168. doi:10.2323/jgam.60.163
Dong, Y., Yang, C., Zhong, W., Shu, Y., Zhang, Y., and Yang, D. (2022). Antibacterial effect and mechanism of anthocyanin from Lycium ruthenicum Murr. Front. Microbiol. 13, 974602. doi:10.3389/fmicb.2022.974602
Dorman, H. J., and Deans, S. G. (2000). Antimicrobial agents from plants: antibacterial activity of plant volatile oils. J. Appl. Microbiol. 88 (2), 308–316. doi:10.1046/j.1365-2672.2000.00969.x
Do Vale, J. P. C., Vasconcelos, M. A., Arruda, F. V. S., Firmino, N. C. S., Pereira, A. L., Andrade, A. L., et al. (2021). Evaluation of antimicrobial and antioxidant potential of essential oil from Croton piauhiensis müll. Arg. Curr. Microbiol. 78 (5), 1926–1938. doi:10.1007/s00284-021-02449-1
Dunman, P. M., Murphy, E., Haney, S., Palacios, D., Tucker-Kellogg, G., Wu, S., et al. (2001). Transcription profiling-based identification ofStaphylococcus aureus genes regulated by the agrand/or sarA loci. J. Bacteriol. 183 (24), 7341–7353. doi:10.1128/jb.183.24.7341-7353.2001
Dürig, A., Kouskoumvekaki, I., Vejborg, R. M., and Klemm, P. (2010). Chemoinformatics-assisted development of new anti-biofilm compounds. Appl. Microbiol. Biotechnol. 87 (1), 309–317. doi:10.1007/s00253-010-2471-0
El-Far, A., Samir, S., El-Gebaly, E., Taha, N. Y., Fahmy, E. M., Diab, T. M., et al. (2021). Assessment of eugenol inhibitory effect on biofilm formation and biofilm gene expression in methicillin resistant Staphylococcus aureus clinical isolates in Egypt. Infect. Genet. Evol. 89, 104722. doi:10.1016/j.meegid.2021.104722
Elghali, F., Ibrahim, I., Guesmi, M., Frikha, F., and Mnif, S. (2024). Unveiling the impact of selected essential oils on MRSA strain ATCC 33591: antibacterial efficiency, biofilm disruption, and staphyloxanthin inhibition. Braz J. Microbiol. 55 (3), 2057–2069. doi:10.1007/s42770-024-01374-2
Engel, J. B., Heckler, C., Tondo, E. C., Daroit, D. J., and Da Silva Malheiros, P. (2017). Antimicrobial activity of free and liposome-encapsulated thymol and carvacrol against Salmonella and Staphylococcus aureus adhered to stainless steel. Int. J. Food Microbiol. 252, 18–23. doi:10.1016/j.ijfoodmicro.2017.04.003
Entenza, J. M., Moreillon, P., Senn, M. M., Kormanec, J., Dunman, P. M., Berger-Bächi, B., et al. (2005). Role of sigmaB in the expression of Staphylococcus aureus cell wall adhesins ClfA and FnbA and contribution to infectivity in a rat model of experimental endocarditis. Infect. Immun. 73 (2), 990–998. doi:10.1128/iai.73.2.990-998.2005
Esnaashari, F., and Zahmatkesh, H. (2024). Antivirulence activities of Rutin-loaded chitosan nanoparticles against pathogenic Staphylococcus aureus. BMC Microbiol. 24 (1), 328. doi:10.1186/s12866-024-03446-7
Espina, L., Pagán, R., López, D., and García-Gonzalo, D. (2015). Individual constituents from essential oils inhibit biofilm mass production by multi-drug resistant Staphylococcus aureus. Molecules 20 (6), 11357–11372. doi:10.3390/molecules200611357
Federman, C., Ma, C., and Biswas, D. (2016). Major components of orange oil inhibit Staphylococcus aureus growth and biofilm formation, and alter its virulence factors. J. Med. Microbiol. 65 (7), 688–695. doi:10.1099/jmm.0.000286
Fernandez-Soto, P., Celi, D., Tejera, E., Alvarez-Suarez, J. M., and Machado, A. (2023). Cinnamomum sp. and Pelargonium odoratissimum as the main contributors to the antibacterial activity of the medicinal drink horchata: a study based on the antibacterial and chemical analysis of 21 plants. Molecules 28 (2), 693. doi:10.3390/molecules28020693
Gambino, E., Maione, A., Guida, M., Albarano, L., Carraturo, F., Galdiero, E., et al. (2022). Evaluation of the pathogenic-mixed biofilm formation of Pseudomonas aeruginosa/Staphylococcus aureus and treatment with limonene on three different materials by a dynamic model. Int. J. Environ. Res. Public Health 19 (6), 3741. doi:10.3390/ijerph19063741
Gao, K., Zhang, B., and Zhao, F. (2023). Antibiofilm effect of curcumin against Staphylococcus aureus surface wound biofilm-associated infection: in vitro and in silico. Appl. Biochem. Biotechnol. 195 (9), 5329–5337. doi:10.1007/s12010-022-03844-5
Gao, S., Liu, G., Li, J., Chen, J., Li, L., Li, Z., et al. (2020). Antimicrobial activity of lemongrass essential oil (cymbopogon flexuosus) and its active component citral against dual-species biofilms of Staphylococcus aureus and Candida species. Front. Cell Infect. Microbiol. 10, 603858. doi:10.3389/fcimb.2020.603858
García-Salinas, S., Elizondo-Castillo, H., Arruebo, M., Mendoza, G., and Irusta, S. (2018). Evaluation of the antimicrobial activity and cytotoxicity of different components of natural origin present in essential oils. Molecules 23 (6), 1399. doi:10.3390/molecules23061399
Gill, A. O., and Holley, R. A. (2004). Mechanisms of bactericidal action of cinnamaldehyde against Listeria monocytogenes and of eugenol against L. monocytogenes and Lactobacillus sakei. Appl. Environ. Microbiol. 70 (10), 5750–5755. doi:10.1128/aem.70.10.5750-5755.2004
Gobin, M., Proust, R., Lack, S., Duciel, L., Des Courtils, C., Pauthe, E., et al. (2022). A combination of the natural molecules gallic acid and carvacrol eradicates P. aeruginosa and S. aureus mature biofilms. Int. J. Mol. Sci. 23 (13), 7118. doi:10.3390/ijms23137118
Gómez-Sequeda, N., Cáceres, M., Stashenko, E. E., Hidalgo, W., and Ortiz, C. (2020). Antimicrobial and antibiofilm activities of essential oils against Escherichia coli O157:H7 and methicillin-resistant Staphylococcus aureus (MRSA). Antibiot. (Basel) 9 (11), 730. doi:10.3390/antibiotics9110730
Gonçalves, T. B., Braga, M. A., De Oliveira, F. F., Santiago, G. M., Carvalho, C. B., Brito E Cabral, P., et al. (2012). Effect of subinihibitory and inhibitory concentrations of Plectranthus amboinicus (Lour.) Spreng essential oil on Klebsiella pneumoniae. Phytomedicine 19 (11), 962–968. doi:10.1016/j.phymed.2012.05.013
Holban, A. M., Grumezescu, V., Grumezescu, A. M., Vasile, B., Truşcă, R., Cristescu, R., et al. (2014). Antimicrobial nanospheres thin coatings prepared by advanced pulsed laser technique. Beilstein J. Nanotechnol. 5, 872–880. doi:10.3762/bjnano.5.99
Hyldgaard, M., Mygind, T., and Meyer, R. L. (2012). Essential oils in food preservation: mode of action, synergies, and interactions with food matrix components. Front. Microbiol. 3, 12. doi:10.3389/fmicb.2012.00012
Inouye, S., Takizawa, T., and Yamaguchi, H. (2001). Antibacterial activity of essential oils and their major constituents against respiratory tract pathogens by gaseous contact. J. Antimicrob. Chemother. 47 (5), 565–573. doi:10.1093/jac/47.5.565
Jefferson, K. K., Pier, D. B., Goldmann, D. A., and Pier, G. B. (2004). The teicoplanin-associated locus regulator (TcaR) and the intercellular adhesin locus regulator (IcaR) are transcriptional inhibitors of the ica locus in Staphylococcus aureus. J. Bacteriol. 186 (8), 2449–2456. doi:10.1128/jb.186.8.2449-2456.2004
Jia, P., Xue, Y. J., Duan, X. J., and Shao, S. H. (2011). Effect of cinnamaldehyde on biofilm formation and sarA expression by methicillin-resistant Staphylococcus aureus. Lett. Appl. Microbiol. 53 (4), 409–416. doi:10.1111/j.1472-765X.2011.03122.x
Jo, E. R., Oh, J., and Cho, S. I. (2022). Inhibitory effect of thymol on tympanostomy tube biofilms of methicillin-resistant Staphylococcus aureus and ciprofloxacin-resistant Pseudomonas aeruginosa. Microorganisms 10 (9), 1867. doi:10.3390/microorganisms10091867
Kachur, K., and Suntres, Z. (2020). The antibacterial properties of phenolic isomers, carvacrol and thymol. Crit. Rev. Food Sci. Nutr. 60 (18), 3042–3053. doi:10.1080/10408398.2019.1675585
Kang, X., Ma, Q., Wang, G., Li, N., Mao, Y., Wang, X., et al. (2022). Potential mechanisms of quercetin influence the ClfB protein during biofilm formation of Staphylococcus aureus. Front. Pharmacol. 13, 825489. doi:10.3389/fphar.2022.825489
Kannappan, A., Balasubramaniam, B., Ranjitha, R., Srinivasan, R., Packiavathy, I. a.S. V., Balamurugan, K., et al. (2019). In vitro and in vivo biofilm inhibitory efficacy of geraniol-cefotaxime combination against Staphylococcus spp. Food Chem. Toxicol. 125, 322–332. doi:10.1016/j.fct.2019.01.008
Karatan, E., and Watnick, P. (2009). Signals, regulatory networks, and materials that build and break bacterial biofilms. Microbiol. Mol. Biol. Rev. 73 (2), 310–347. doi:10.1128/mmbr.00041-08
Kargaran, M., Moradabadi, A. R., Arjomandzadegan, M., Hosseini, H., Habibi, G., Tayeboon, M., et al. (2024). Effects of the aqueous extract of aloe vera on the morphological and physiological properties of E. coli. Iran. Red Crescent Med. J. (IRCMJ) 19 (2), 1–6. doi:10.5812/ircmj.23896
Karygianni, L., Ren, Z., Koo, H., and Thurnheer, T. (2020). Biofilm matrixome: extracellular components in structured microbial communities. Trends Microbiol. 28 (8), 668–681. doi:10.1016/j.tim.2020.03.016
Kasthuri, T., Swetha, T. K., Bhaskar, J. P., and Pandian, S. K. (2022). Rapid-killing efficacy substantiates the antiseptic property of the synergistic combination of carvacrol and nerol against nosocomial pathogens. Arch. Microbiol. 204 (9), 590. doi:10.1007/s00203-022-03197-x
Kerekes, E. B., Deák, É., Takó, M., Tserennadmid, R., Petkovits, T., Vágvölgyi, C., et al. (2013). Anti-biofilm forming and anti-quorum sensing activity of selected essential oils and their main components on food-related micro-organisms. J. Appl. Microbiol. 115 (4), 933–942. doi:10.1111/jam.12289
Kerekes, E. B., Vidács, A., Takó, M., Petkovits, T., Vágvölgyi, C., Horváth, G., et al. (2019). Anti-biofilm effect of selected essential oils and main components on mono- and polymicrobic bacterial cultures. Microorganisms 7 (9), 345. doi:10.3390/microorganisms7090345
Khaleghian, M., Sahrayi, H., Hafezi, Y., Mirshafeeyan, M., Moghaddam, Z. S., Farasati Far, B., et al. (2023). In silico design and mechanistic study of niosome-encapsulated curcumin against multidrug-resistant Staphylococcus aureus biofilms. Front. Microbiol. 14, 1277533. doi:10.3389/fmicb.2023.1277533
Khan, F., Park, S. K., Bamunuarachchi, N. I., Oh, D., and Kim, Y. M. (2021). Caffeine-loaded gold nanoparticles: antibiofilm and anti-persister activities against pathogenic bacteria. Appl. Microbiol. Biotechnol. 105 (9), 3717–3731. doi:10.1007/s00253-021-11300-3
Kiedrowski, M. R., Kavanaugh, J. S., Malone, C. L., Mootz, J. M., Voyich, J. M., Smeltzer, M. S., et al. (2011). Nuclease modulates biofilm formation in community-associated methicillin-resistant Staphylococcus aureus. PLOS ONE 6 (11), e26714. doi:10.1371/journal.pone.0026714
Kifer, D., Mužinić, V., and Klarić, M. (2016). Antimicrobial potency of single and combined mupirocin and monoterpenes, thymol, menthol and 1,8-cineole against Staphylococcus aureus planktonic and biofilm growth. J. Antibiot. (Tokyo) 69 (9), 689–696. doi:10.1038/ja.2016.10
Kim, B. C., Kim, H., Lee, H. S., Kim, S. H., Cho, D. H., Jung, H. J., et al. (2022). 4-Chloro-2-Isopropyl-5-Methylphenol exhibits antimicrobial and adjuvant activity against methicillin-resistant Staphylococcus aureus. J. Microbiol. Biotechnol. 32 (6), 730–739. doi:10.4014/jmb.2203.03054
Kim, E. S., Kang, S. Y., Kim, Y. H., Lee, Y. E., Choi, N. Y., You, Y. O., et al. (2015). Chamaecyparis obtusa essential oil inhibits methicillin-resistant Staphylococcus aureus biofilm formation and expression of virulence factors. J. Med. Food 18 (7), 810–817. doi:10.1089/jmf.2014.3309
Kim, J., and Chin, Y. W. (2023). Antimicrobial agent against methicillin-resistant Staphylococcus aureus biofilm monitored using Raman spectroscopy. Pharmaceutics 15 (7), 1937. doi:10.3390/pharmaceutics15071937
Kim, J. H., Kim, Y. H., Park, B. I., Choi, N. Y., and Kim, K. J. (2023). Pinus koraiensis essential oil attenuates the pathogenicity of superbacteria by suppressing virulence gene expression. Molecules 29 (1), 37. doi:10.3390/molecules29010037
Kim, S. Y., Kwon, O. J., and Park, J.-W. (2001). Inactivation of catalase and superoxide dismutase by singlet oxygen derived from photoactivated dye. Biochimie 83 (5), 437–444. doi:10.1016/S0300-9084(01)01258-5
Kim, Y., Kim, S., Cho, K. H., Lee, J. H., and Lee, J. (2022). Antibiofilm activities of cinnamaldehyde analogs against uropathogenic Escherichia coli and Staphylococcus aureus. Int. J. Mol. Sci. 23 (13), 7225. doi:10.3390/ijms23137225
Knidel, C., Pereira, M. F., Barcelos, D. H. F., Gomes, D. C. O., Guimarães, M. C. C., and Schuenck, R. P. (2021). Epigallocatechin gallate has antibacterial and antibiofilm activity in methicillin resistant and susceptible Staphylococcus aureus of different lineages in non-cytotoxic concentrations. Nat. Prod. Res. 35 (22), 4643–4647. doi:10.1080/14786419.2019.1698575
Knobloch, J. K., Horstkotte, M. A., Rohde, H., and Mack, D. (2002). Evaluation of different detection methods of biofilm formation in Staphylococcus aureus. Med. Microbiol. Immunol. 191 (2), 101–106. doi:10.1007/s00430-002-0124-3
Knowles, J. R., Roller, S., Murray, D. B., and Naidu, A. S. (2005). Antimicrobial action of carvacrol at different stages of dual-species biofilm development by Staphylococcus aureus and Salmonella enterica serovar Typhimurium. Appl. Environ. Microbiol. 71 (2), 797–803. doi:10.1128/aem.71.2.797-803.2005
Kostoglou, D., Protopappas, I., and Giaouris, E. (2020). Common plant-derived terpenoids present increased anti-biofilm potential against Staphylococcus bacteria compared to a quaternary ammonium biocide. Foods 9 (6), 697. doi:10.3390/foods9060697
Kot, B., Sytykiewicz, H., Sprawka, I., and Witeska, M. (2019). Effect of trans-cinnamaldehyde on methicillin-resistant Staphylococcus aureus biofilm formation: metabolic activity assessment and analysis of the biofilm-associated genes expression. Int. J. Mol. Sci. 21 (1), 102. doi:10.3390/ijms21010102
Kot, B., Wierzchowska, K., Grużewska, A., and Lohinau, D. (2018). The effects of selected phytochemicals on biofilm formed by five methicillin-resistant Staphylococcus aureus. Nat. Prod. Res. 32 (11), 1299–1302. doi:10.1080/14786419.2017.1340282
Kot, B., Wierzchowska, K., Piechota, M., and Grużewska, A. (2020). Antimicrobial resistance patterns in methicillin-resistant Staphylococcus aureus from patients hospitalized during 2015-2017 in hospitals in Poland. Med. Princ. Pract. 29 (1), 61–68. doi:10.1159/000501788
Krogsgård Nielsen, C., Kjems, J., Mygind, T., Snabe, T., Schwarz, K., Serfert, Y., et al. (2017). Antimicrobial effect of emulsion-encapsulated isoeugenol against biofilms of food pathogens and spoilage bacteria. Int. J. Food Microbiol. 242, 7–12. doi:10.1016/j.ijfoodmicro.2016.11.002
Kunnumakkara, A. B., Bordoloi, D., Padmavathi, G., Monisha, J., Roy, N. K., Prasad, S., et al. (2017). Curcumin, the golden nutraceutical: multitargeting for multiple chronic diseases. diseases 174 (11), 1325–1348. doi:10.1111/bph.13621
Laplante, K. L., Sarkisian, S. A., Woodmansee, S., Rowley, D. C., and Seeram, N. P. (2012). Effects of cranberry extracts on growth and biofilm production of Escherichia coli and Staphylococcus species. Phytother. Res. 26 (9), 1371–1374. doi:10.1002/ptr.4592
Lebeaux, D., Chauhan, A., Rendueles, O., and Beloin, C. (2013). From in vitro to in vivo models of bacterial biofilm-related infections. Pathogens 2 (2), 288–356. doi:10.3390/pathogens2020288
Lee, J. H., Kim, Y. G., Choi, J. S., Jeong, Y. T., Hwang, B. S., and Lee, J. (2024). Antibiofilm and antihemolytic activities of actinostemma lobatum extract rich in quercetin against Staphylococcus aureus. Pharmaceutics 16 (8), 1075. doi:10.3390/pharmaceutics16081075
Lee, J. H., Park, J. H., Cho, H. S., Joo, S. W., Cho, M. H., and Lee, J. (2013). Anti-biofilm activities of quercetin and tannic acid against Staphylococcus aureus. Biofouling 29 (5), 491–499. doi:10.1080/08927014.2013.788692
Li, J., Jiang, F., Chi, Z., Han, D., Yu, L., and Liu, C. (2018). Development of Enteromorpha prolifera polysaccharide-based nanoparticles for delivery of curcumin to cancer cells. Int. J. Biol. Macromol. 112, 413–421. doi:10.1016/j.ijbiomac.2018.02.002
Li, J., Lu, T., Chu, Y., Zhang, Y., Zhang, J., Fu, W., et al. (2024). Cinnamaldehyde targets SarA to enhance β-lactam antibiotic activity against methicillin-resistant Staphylococcus aureus. mLife 3 (2), 291–306. doi:10.1002/mlf2.12121
Li, J. G., Chen, X. F., Lu, T. Y., Zhang, J., Dai, S. H., Sun, J., et al. (2023). Increased activity of β-lactam antibiotics in combination with carvacrol against MRSA bacteremia and catheter-associated biofilm infections. ACS Infect. Dis. 9 (12), 2482–2493. doi:10.1021/acsinfecdis.3c00338
Li, Q., Huang, K. X., Pan, S., Su, C., Bi, J., and Lu, X. (2022a). Thymol disrupts cell homeostasis and inhibits the growth of Staphylococcus aureus. Contrast Media Mol. Imaging 2022, 8743096. doi:10.1155/2022/8743096
Li, Q., Yu, S., Han, J., Wu, J., You, L., Shi, X., et al. (2022b). Synergistic antibacterial activity and mechanism of action of nisin/carvacrol combination against Staphylococcus aureus and their application in the infecting pasteurized milk. Food Chem. 380, 132009. doi:10.1016/j.foodchem.2021.132009
Li, X., Huang, T., Xu, K., Li, C., and Li, Y. (2019). Molecular characteristics and virulence gene profiles of Staphylococcus aureus isolates in Hainan, China. BMC Infect. Dis. 19 (1), 873. doi:10.1186/s12879-019-4547-5
Li, Y., Dai, J., Ma, Y., Yao, Y., Yu, D., Shen, J., et al. (2024). The mitigation potential of synergistic quorum quenching and antibacterial properties for biofilm proliferation and membrane biofouling. Water Res. 255, 121462. doi:10.1016/j.watres.2024.121462
Li, Y., Huang, T.-Y., Ye, C., Chen, L., Liang, Y., Wang, K., et al. (2020). Formation and control of the viable but non-culturable state of foodborne pathogen Escherichia coli O157:H7. Front. Microbiol. 11, 1202. doi:10.3389/fmicb.2020.01202
Lim, A. C., Tang, S. G. H., Zin, N. M., Maisarah, A. M., Ariffin, I. A., Ker, P. J., et al. (2022). Chemical composition, antioxidant, antibacterial, and antibiofilm activities of backhousia citriodora essential oil. Molecules 27 (15), 4895. doi:10.3390/molecules27154895
Lina, G., Jarraud, S., Ji, G., Greenland, T., Pedraza, A., Etienne, J., et al. (1998). Transmembrane topology and histidine protein kinase activity of AgrC, the agr signal receptor in Staphylococcus aureus. Mol. Microbiol. 28 (3), 655–662. doi:10.1046/j.1365-2958.1998.00830.x
Liu, C., Cheng, F., Aisa, H. A., and Maiwulanjiang, M. (2023). Comprehensive study of components and antimicrobial properties of essential oil extracted from Carum carvi L. Seeds. Antibiot. (Basel) 12 (3), 591. doi:10.3390/antibiotics12030591
Liu, P., Kang, X., Chen, X., Luo, X., Li, C., and Wang, G. (2024). Quercetin targets SarA of methicillin-resistant Staphylococcus aureus to mitigate biofilm formation. Microbiol. Spectr. 12 (1), e0272223. doi:10.1128/spectrum.02722-23
Liu, T., Wang, J., Gong, X., Wu, X., Liu, L., and Chi, F. (2020). Rosemary and tea tree essential oils exert antibiofilm activities in vitro against Staphylococcus aureus and Escherichia coli. J. Food Prot. 83 (7), 1261–1267. doi:10.4315/0362-028x.Jfp-19-337
Loo, C. Y., Rohanizadeh, R., Young, P. M., Traini, D., Cavaliere, R., Whitchurch, C. B., et al. (2016). Combination of silver nanoparticles and curcumin nanoparticles for enhanced anti-biofilm activities. J. Agric. Food Chem. 64 (12), 2513–2522. doi:10.1021/acs.jafc.5b04559
Łopusiewicz, Ł., Macieja, S., Bartkowiak, A., and El Fray, M. (2021). Antimicrobial, antibiofilm, and antioxidant activity of functional poly(butylene succinate) films modified with curcumin and carvacrol. Mater. (Basel) 14 (24), 7882. doi:10.3390/ma14247882
Loughran, A. J., Atwood, D. N., Anthony, A. C., Harik, N. S., Spencer, H. J., Beenken, K. E., et al. (2014). Impact of individual extracellular proteases on Staphylococcus aureus biofilm formation in diverse clinical isolates and their isogenic sarA mutants. MicrobiologyOpen 3 (6), 897–909. doi:10.1002/mbo3.214
Lu, M., Li, Y., and Wu, M. X. (2021). Bacteria-specific pro-photosensitizer kills multidrug-resistant Staphylococcus aureus and Pseudomonas aeruginosa. Commun. Biol. 4 (1), 408. doi:10.1038/s42003-021-01956-y
Ma, S., Moser, D., Han, F., Leonhard, M., Schneider-Stickler, B., and Tan, Y. (2020). Preparation and antibiofilm studies of curcumin loaded chitosan nanoparticles against polymicrobial biofilms of Candida albicans and Staphylococcus aureus. Carbohydr. Polym. 241, 116254. doi:10.1016/j.carbpol.2020.116254
Manukumar, H. M., Chandrasekhar, B., Rakesh, K. P., Ananda, A. P., Nandhini, M., Lalitha, P., et al. (2017). Novel T-C@AgNPs mediated biocidal mechanism against biofilm associated methicillin-resistant Staphylococcus aureus (Bap-MRSA) 090, cytotoxicity and its molecular docking studies. Medchemcomm 8 (12), 2181–2194. doi:10.1039/c7md00486a
Martínez, A., Manrique-Moreno, M., Klaiss-Luna, M. C., Stashenko, E., Zafra, G., and Ortiz, C. (2021). Effect of essential oils on growth inhibition, biofilm formation and membrane integrity of Escherichia coli and Staphylococcus aureus. Antibiot. (Basel) 10 (12), 1474. doi:10.3390/antibiotics10121474
Martínez, A., Stashenko, E. E., Sáez, R. T., Zafra, G., and Ortiz, C. (2023). Effect of essential oil from lippia origanoides on the transcriptional expression of genes related to quorum sensing, biofilm formation, and virulence of Escherichia coli and Staphylococcus aureus. Antibiotics 12, 845. doi:10.3390/antibiotics12050845
Mastoor, S., Nazim, F., Rizwan-Ul-Hasan, S., Ahmed, K., Khan, S., Ali, S. N., et al. (2022). Analysis of the antimicrobial and anti-biofilm activity of natural compounds and their analogues against Staphylococcus aureus isolates. Molecules 27 (20), 6874. doi:10.3390/molecules27206874
Matilla-Cuenca, L., Gil, C., Cuesta, S., Rapún-Araiz, B., Žiemytė, M., Mira, A., et al. (2020). Antibiofilm activity of flavonoids on staphylococcal biofilms through targeting BAP amyloids. Sci. Rep. 10 (1), 18968. doi:10.1038/s41598-020-75929-2
Mawang, C. I., Lim, Y. Y., Ong, K. S., Muhamad, A., and Lee, S. M. (2017). Identification of α-tocopherol as a bioactive component of Dicranopteris linearis with disrupting property against preformed biofilm of Staphylococcus aureus. J. Appl. Microbiol. 123 (5), 1148–1159. doi:10.1111/jam.13578
Mehanna, M. M., Mneimneh, A. T., and Abed El Jalil, K. (2020). Levofloxacin-loaded naturally occurring monoterpene-based nanoemulgel: a feasible efficient system to circumvent MRSA ocular infections. Drug Dev. Ind. Pharm. 46 (11), 1787–1799. doi:10.1080/03639045.2020.1821048
Melo, R. S., Albuquerque Azevedo Á, M., Gomes Pereira, A. M., Rocha, R. R., Bastos Cavalcante, R. M., Carneiro Matos, M. N., et al. (2019). Chemical composition and antimicrobial effectiveness of Ocimum gratissimum L. Essential oil against multidrug-resistant isolates of Staphylococcus aureus and Escherichia coli. Molecules 24 (21), 3864. doi:10.3390/molecules24213864
Merghni, A., Marzouki, H., Hentati, H., Aouni, M., and Mastouri, M. (2015). Antibacterial and antibiofilm activities of Laurus nobilis L. essential oil against Staphylococcus aureus strains associated with oral infections. Pathol. Biol. Paris. 64, 29–34. doi:10.1016/j.patbio.2015.10.003
Merghni, A., Noumi, E., Hadded, O., Dridi, N., Panwar, H., Ceylan, O., et al. (2018). Assessment of the antibiofilm and antiquorum sensing activities of Eucalyptus globulus essential oil and its main component 1,8-cineole against methicillin-resistant Staphylococcus aureus strains. Microb. Pathog. 118, 74–80. doi:10.1016/j.micpath.2018.03.006
Miladi, H., Zmantar, T., Chaabouni, Y., Fedhila, K., Bakhrouf, A., Mahdouani, K., et al. (2016). Antibacterial and efflux pump inhibitors of thymol and carvacrol against food-borne pathogens. Microb. Pathog. 99, 95–100. doi:10.1016/j.micpath.2016.08.008
Miladi, H., Zmantar, T., Kouidhi, B., Al Qurashi, Y. M. A., Bakhrouf, A., Chaabouni, Y., et al. (2017). Synergistic effect of eugenol, carvacrol, thymol, p-cymene and γ-terpinene on inhibition of drug resistance and biofilm formation of oral bacteria. Microb. Pathog. 112, 156–163. doi:10.1016/j.micpath.2017.09.057
Mirzahosseinipour, M., Khorsandi, K., Hosseinzadeh, R., Ghazaeian, M., and Shahidi, F. K. (2020). Antimicrobial photodynamic and wound healing activity of curcumin encapsulated in silica nanoparticles. Photodiagnosis Photodyn. Ther. 29, 101639. doi:10.1016/j.pdpdt.2019.101639
Mishra, P., Gupta, P., and Pruthi, V. (2021). Cinnamaldehyde incorporated gellan/PVA electrospun nanofibers for eradicating Candida biofilm. Mater Sci. Eng. C Mater Biol. Appl. 119, 111450. doi:10.1016/j.msec.2020.111450
Mohammadi Pelarti, S., Karimi Zarehshuran, L., Babaeekhou, L., and Ghane, M. (2021). Antibacterial, anti-biofilm and anti-quorum sensing activities of Artemisia dracunculus essential oil (EO): a study against Salmonella enterica serovar Typhimurium and Staphylococcus aureus. Arch. Microbiol. 203 (4), 1529–1537. doi:10.1007/s00203-020-02138-w
Mohammed, M. J., and Al-Bayati, F. A. (2009). Isolation and identification of antibacterial compounds from Thymus kotschyanus aerial parts and Dianthus caryophyllus flower buds. Phytomedicine 16 (6-7), 632–637. doi:10.1016/j.phymed.2008.12.026
Montanaro, L., Arciola, C. R., Baldassarri, L., and Borsetti, E. (1999). Presence and expression of collagen adhesin gene (cna) and slime production in Staphylococcus aureus strains from orthopaedic prosthesis infections. Biomaterials 20 (20), 1945–1949. doi:10.1016/s0142-9612(99)00099-x
Morán, A., Gutiérrez, S., Martínez-Blanco, H., Ferrero, M. A., Monteagudo-Mera, A., and Rodríguez-Aparicio, L. B. (2014). Non-toxic plant metabolites regulate Staphylococcus viability and biofilm formation: a natural therapeutic strategy useful in the treatment and prevention of skin infections. Biofouling 30 (10), 1175–1182. doi:10.1080/08927014.2014.976207
Moshe, M., Lellouche, J., and Banin, E. (2011). “Curcumin: a natural antibiofilm agent,” in Science and technology against microbial pathogens (World Scientific), 89–93.
Mouwakeh, A., Kincses, A., Nové, M., Mosolygó, T., Mohácsi-Farkas, C., Kiskó, G., et al. (2019). Nigella sativa essential oil and its bioactive compounds as resistance modifiers against Staphylococcus aureus. Phytother. Res. 33 (4), 1010–1018. doi:10.1002/ptr.6294
Murai, M., Moriyama, H., Hata, E., Takeuchi, F., and Amemura-Maekawa, J. (2016). Variation and association of fibronectin-binding protein genes fnbA and fnbB in Staphylococcus aureus Japanese isolates. Microbiol. Immunol. 60 (5), 312–325. doi:10.1111/1348-0421.12377
Nagaraj, V., Skillman, L., Li, D., Foreman, A., Xie, Z., and Ho, G. (2017). Characterisation of extracellular polysaccharides from bacteria isolated from a full-scale desalination plant. Desalination 418, 9–18. doi:10.1016/j.desal.2017.05.021
Nagoor Meeran, M. F., Javed, H., Al Taee, H., Azimullah, S., and Ojha, S. K. (2017). Pharmacological properties and molecular mechanisms of thymol: prospects for its therapeutic potential and pharmaceutical development. Front. Pharmacol. 8, 380. doi:10.3389/fphar.2017.00380
Nain, A., Tseng, Y. T., Gupta, A., Lin, Y. F., Arumugam, S., Huang, Y. F., et al. (2023). NIR-activated quercetin-based nanogels embedded with CuS nanoclusters for the treatment of drug-resistant biofilms and accelerated chronic wound healing. Nanoscale Horiz. 8 (12), 1652–1664. doi:10.1039/d3nh00275f
Nguyen, Q. M., Hutchison, P., Palombo, E., Yu, A., and Kingshott, P. (2024). Antibiofilm activity of eugenol-loaded chitosan coatings against common medical-device-contaminating bacteria. ACS Appl. Bio Mater 7 (2), 918–935. doi:10.1021/acsabm.3c00949
Nguyen, T. L. A., and Bhattacharya, D. (2022). Antimicrobial activity of quercetin: an approach to its mechanistic principle. Molecules 27 (8), 2494. doi:10.3390/molecules27082494
Ni, K., Cai, D., Lu, J., and Tian, J. (2022). Eugenol-Mediated inhibition of biofilm formed by S. aureus: a potent organism for pediatric digestive system diseases. Appl. Biochem. Biotechnol. 194 (3), 1340–1358. doi:10.1007/s12010-021-03682-x
Nostro, A., Cellini, L., Zimbalatti, V., Blanco, A. R., Marino, A., Pizzimenti, F., et al. (2012a). Enhanced activity of carvacrol against biofilm of Staphylococcus aureus and Staphylococcus epidermidis in an acidic environment. Apmis 120 (12), 967–973. doi:10.1111/j.1600-0463.2012.02928.x
Nostro, A., Roccaro, A. S., Bisignano, G., Marino, A., Cannatelli, M. A., Pizzimenti, F. C., et al. (2007). Effects of oregano, carvacrol and thymol on Staphylococcus aureus and Staphylococcus epidermidis biofilms. J. Med. Microbiol. 56 (Pt 4), 519–523. doi:10.1099/jmm.0.46804-0
Nostro, A., Scaffaro, R., Botta, L., Filocamo, A., Marino, A., and Bisignano, G. (2015). Effect of temperature on the release of carvacrol and cinnamaldehyde incorporated into polymeric systems to control growth and biofilms of Escherichia coli and Staphylococcus aureus. Biofouling 31 (8), 639–649. doi:10.1080/08927014.2015.1079703
Nostro, A., Scaffaro, R., D'arrigo, M., Botta, L., Filocamo, A., Marino, A., et al. (2012b). Study on carvacrol and cinnamaldehyde polymeric films: mechanical properties, release kinetics and antibacterial and antibiofilm activities. Appl. Microbiol. Biotechnol. 96 (4), 1029–1038. doi:10.1007/s00253-012-4091-3
Nostro, A., Scaffaro, R., D'arrigo, M., Botta, L., Filocamo, A., Marino, A., et al. (2013). Development and characterization of essential oil component-based polymer films: a potential approach to reduce bacterial biofilm. Appl. Microbiol. Biotechnol. 97 (21), 9515–9523. doi:10.1007/s00253-013-5196-z
Novick, R. P., Projan, S. J., Kornblum, J., Ross, H. F., Ji, G., Kreiswirth, B., et al. (1995). The agr P2 operon: an autocatalytic sensory transduction system in Staphylococcus aureus. Mol. Gen. Genet. 248 (4), 446–458. doi:10.1007/bf02191645
Nunes, D. O. S., Vinturelle, R., Martins, F. J., Dos Santos, T. F., Valverde, A. L., Ribeiro, C. M. R., et al. (2021). Biotechnological potential of eugenol and thymol derivatives against Staphylococcus aureus from bovine mastitis. Curr. Microbiol. 78 (5), 1846–1855. doi:10.1007/s00284-021-02344-9
Oliveira, H. B. M., Selis, N. D. N., Sampaio, B. A., Júnior, M. N. S., De Carvalho, S. P., De Almeida, J. B., et al. (2021). Citral modulates virulence factors in methicillin-resistant Staphylococcus aureus. Sci. Rep. 11 (1), 16482. doi:10.1038/s41598-021-95971-y
Oura, Y., Shimamura, Y., Kan, T., and Masuda, S. (2024). Effect of polyphenols on inflammation induced by membrane vesicles from Staphylococcus aureus. Cells 13 (5), 387. doi:10.3390/cells13050387
Oves, M., Rauf, M. A., Ansari, M. O., Aslam Parwaz Khan, A., H, A. Q., Alajmi, M. F., et al. (2020). Graphene decorated Zinc oxide and curcumin to disinfect the methicillin-resistant Staphylococcus aureus. Nanomater. (Basel) 10 (5), 1004. doi:10.3390/nano10051004
Pamukçu, A., Erdoğan, N., and Şen Karaman, D. (2022). Polyethylenimine-grafted mesoporous silica nanocarriers markedly enhance the bactericidal effect of curcumin against Staphylococcus aureus biofilm. J. Biomed. Mater Res. B Appl. Biomater. 110 (11), 2506–2520. doi:10.1002/jbm.b.35108
Pan, K., Chen, H., Davidson, P. M., and Zhong, Q. (2014). Thymol nanoencapsulated by sodium caseinate: physical and antilisterial properties. J. Agric. Food Chem. 62 (7), 1649–1657. doi:10.1021/jf4055402
Park, B. S., Kim, J. G., Kim, M. R., Lee, S. E., Takeoka, G. R., Oh, K. B., et al. (2005). Curcuma longa L. constituents inhibit sortase A and Staphylococcus aureus cell adhesion to fibronectin. J. Agric. Food Chem. 53 (23), 9005–9009. doi:10.1021/jf051765z
Patti, J. M., Bremell, T., Krajewska-Pietrasik, D., Abdelnour, A., Tarkowski, A., Rydén, C., et al. (1994). The Staphylococcus aureus collagen adhesin is a virulence determinant in experimental septic arthritis. Infect. Immun. 62 (1), 152–161. doi:10.1128/iai.62.1.152-161.1994
Peng, L., Xiong, Y., Wang, M., Han, M., Cai, W., and Li, Z. (2018). Chemical composition of essential oil in mosla chinensis maxim cv. Jiangxiangru and its inhibitory effect on Staphylococcus aureus biofilm formation. Open Life Sci. 13, 1–10. doi:10.1515/biol-2018-0001
Peng, Q., Tang, X., Dong, W., Sun, N., and Yuan, W. (2022). A review of biofilm formation of Staphylococcus aureus and its regulation mechanism. Antibiot. (Basel) 12 (1), 12. doi:10.3390/antibiotics12010012
Peng, Q., Tang, X., Dong, W., Zhi, Z., Zhong, T., Lin, S., et al. (2023). Carvacrol inhibits bacterial polysaccharide intracellular adhesin synthesis and biofilm formation of mucoid Staphylococcus aureus: an in vitro and in vivo study. RSC Adv. 13 (41), 28743–28752. doi:10.1039/d3ra02711b
Piasecki, B., Balázs, V. L., Kieltyka-Dadasiewicz, A., Szabó, P., Kocsis, B., Horváth, G., et al. (2023). Microbiological studies on the influence of essential oils from several Origanum species on respiratory pathogens. Molecules 28 (7), 3044. doi:10.3390/molecules28073044
Piri-Gharaghie, T., Beiranvand, S., Riahi, A., Shirin, N. J., Badmasti, F., Mirzaie, A., et al. (2022). Fabrication and characterization of thymol-loaded chitosan nanogels: improved antibacterial and anti-biofilm activities with negligible cytotoxicity. Chem. Biodivers. 19 (3), e202100426. doi:10.1002/cbdv.202100426
Porfírio, E. M., Melo, H. M., Pereira, A. M. G., Cavalcante, T. T. A., Gomes, G. A., De Carvalho, M. G., et al. (2017). In vitro antibacterial and antibiofilm activity of lippia alba essential oil, citral, and carvone against Staphylococcus aureus. ScientificWorldJournal 2017, 4962707. doi:10.1155/2017/4962707
Prakash, P., Misra, A., Surin, W. R., Jain, M., Bhatta, R. S., Pal, R., et al. (2011). Anti-platelet effects of Curcuma oil in experimental models of myocardial ischemia-reperfusion and thrombosis. Thromb. Res. 127 (2), 111–118. doi:10.1016/j.thromres.2010.11.007
Qian, W., Liu, M., Fu, Y., Zhang, J., Liu, W., Li, J., et al. (2020). Antimicrobial mechanism of luteolin against Staphylococcus aureus and Listeria monocytogenes and its antibiofilm properties. Microb. Pathog. 142, 104056. doi:10.1016/j.micpath.2020.104056
Qin, J., Yu, L., Peng, F., Ye, X., Li, G., Sun, C., et al. (2023). Tannin extracted from Penthorum chinense Pursh, a potential drug with antimicrobial and antibiofilm effects against methicillin-sensitive Staphylococcus aureus and methicillin-resistant Staphylococcus aureus. Front. Microbiol. 14, 1134207. doi:10.3389/fmicb.2023.1134207
Qin, N., Tan, X., Jiao, Y., Liu, L., Zhao, W., Yang, S., et al. (2014). RNA-Seq-based transcriptome analysis of methicillin-resistant Staphylococcus aureus biofilm inhibition by ursolic acid and resveratrol. Sci. Rep. 4, 5467. doi:10.1038/srep05467
Radojević, I. D., Grujović, M., Milošević-Djordjević, O., Vukajlović, J. T., Marković, A., Grujičić, D., et al. (2023). Putative application of Najas marina L. extracts as a source of bioactive compounds and their antioxidant, antimicrobial, antibiofilm, and genotoxic properties. Lett. Appl. Microbiol. 76 (5), ovad055. doi:10.1093/lambio/ovad055
Ramasamy, M., Lee, J. H., and Lee, J. (2017a). Development of gold nanoparticles coated with silica containing the antibiofilm drug cinnamaldehyde and their effects on pathogenic bacteria. Int. J. Nanomedicine 12, 2813–2828. doi:10.2147/ijn.s132784
Ramasamy, M., Lee, J. H., and Lee, J. (2017b). Direct one-pot synthesis of cinnamaldehyde immobilized on gold nanoparticles and their antibiofilm properties. Colloids Surf. B Biointerfaces 160, 639–648. doi:10.1016/j.colsurfb.2017.10.018
Rangel, M. L., De Aquino, S. G., De Lima, J. M., Castellano, L. R., and De Castro, R. D. (2018). In vitro effect of cinnamomum zeylanicum blume essential oil on Candida spp. involved in oral infections. Evid. Based Complement. Altern. Med. 2018, 4045013. doi:10.1155/2018/4045013
Ribeiro, I. P., Pinto, J. G., Souza, B. M. N., Miñán, A. G., and Ferreira-Strixino, J. (2022). Antimicrobial photodynamic therapy with curcumin on methicillin-resistant Staphylococcus aureus biofilm. Photodiagnosis Photodyn. Ther. 37, 102729. doi:10.1016/j.pdpdt.2022.102729
Rice, K. C., Mann, E. E., Endres, J. L., Weiss, E. C., Cassat, J. E., Smeltzer, M. S., et al. (2007). The cidA murein hydrolase regulator contributes to DNA release and biofilm development in Staphylococcus aureus. Proc. Natl. Acad. Sci. U. S. A. 104 (19), 8113–8118. doi:10.1073/pnas.0610226104
Rouws, L. F., Meneses, C. H., Guedes, H. V., Vidal, M. S., Baldani, J. I., and Schwab, S. (2010). Monitoring the colonization of sugarcane and rice plants by the endophytic diazotrophic bacterium Gluconacetobacter diazotrophicus marked with gfp and gusA reporter genes. Lett. Appl. Microbiol. 51 (3), 325–330. doi:10.1111/j.1472-765X.2010.02899.x
Salazar-Sesatty, H. A., Montoya-Hinojosa, E. I., Villarreal-Salazar, V., Alvizo-Baez, C. A., Camacho-Ortiz, A., Terrazas-Armendariz, L. D., et al. (2024). Biofilm eradication and inhibition of methicillin-resistant Staphylococcus clinical isolates by curcumin-chitosan magnetic nanoparticles. Jpn. J. Infect. Dis. 77, 260–268. doi:10.7883/yoken.JJID.2024.034
Salem, S. S., Elsayed, H. E., Shabana, S., Khazaal, M. T., and Moharram, F. A. (2023). Phytochemical profile and antimicrobial activity of essential oils from two Syzygium species against selected oral pathogens. BMC Complement. Med. Ther. 23 (1), 448. doi:10.1186/s12906-023-04277-1
Sandhu, S. K., Kumar, S., Raut, J., Singh, M., Kaur, S., Sharma, G., et al. (2021). Systematic development and characterization of novel, high drug-loaded, photostable, curcumin solid lipid nanoparticle hydrogel for wound healing. Antioxidants (Basel) 10 (5), 725. doi:10.3390/antiox10050725
Sang, H., Jin, H., Song, P., Xu, W., and Wang, F. (2024). Gallic acid exerts antibiofilm activity by inhibiting methicillin-resistant Staphylococcus aureus adhesion. Sci. Rep. 14 (1), 17220. doi:10.1038/s41598-024-68279-w
Sawant, S., Baldwin, T. C., Khan, H., and Rahman, A. (2022). Evaluation of the effect of leaf development in Plectranthus amboinicus L. On antimicrobial activity and virulence factors of Pseudomonas aeruginosa PAO1 and Staphylococcus aureus NCTC8325. Curr. Microbiol. 80 (1), 24. doi:10.1007/s00284-022-03126-7
Scaffaro, R., Lopresti, F., D'arrigo, M., Marino, A., and Nostro, A. (2018). Efficacy of poly(lactic acid)/carvacrol electrospun membranes against Staphylococcus aureus and Candida albicans in single and mixed cultures. Appl. Microbiol. Biotechnol. 102 (9), 4171–4181. doi:10.1007/s00253-018-8879-7
Schröder, A., Schröder, B., Roppenser, B., Linder, S., Sinha, B., Fässler, R., et al. (2006). Staphylococcus aureus fibronectin binding protein-A induces motile attachment sites and complex actin remodeling in living endothelial cells. Mol. Biol. Cell 17 (12), 5198–5210. doi:10.1091/mbc.e06-05-0463
Schwartz, K., Syed, A. K., Stephenson, R. E., Rickard, A. H., and Boles, B. R. (2012). Functional amyloids composed of phenol soluble modulins stabilize Staphylococcus aureus biofilms. PLoS Pathog. 8 (6), e1002744. doi:10.1371/journal.ppat.1002744
Selvaraj, A., Valliammai, A., Muthuramalingam, P., Priya, A., Suba, M., Ramesh, M., et al. (2020). Carvacrol targets SarA and CrtM of methicillin-resistant Staphylococcus aureus to mitigate biofilm formation and staphyloxanthin synthesis: an in vitro and in vivo approach. ACS Omega 5 (48), 31100–31114. doi:10.1128/mSystems.00986-20
Selvaraj, A., Valliammai, A., Premika, M., Priya, A., Bhaskar, J. P., Krishnan, V., et al. (2021). Sapindus mukorossi Gaertn. and its bioactive metabolite oleic acid impedes methicillin-resistant Staphylococcus aureus biofilm formation by down regulating adhesion genes expression. Microbiol. Res. 242, 126601. doi:10.1016/j.micres.2020.126601
Sethupathy, S., Vigneshwari, L., Valliammai, A., Balamurugan, K., and Pandian, S. K. (2017). l-Ascorbyl 2,6-dipalmitate inhibits biofilm formation and virulence in methicillin-resistant Staphylococcus aureus and prevents triacylglyceride accumulation in Caenorhabditis elegans. RSC Adv. 7 (38), 23392–23406. doi:10.1039/C7RA02934A
Sharaf, M., Sewid, A. H., Hamouda, H. I., Elharrif, M. G., El-Demerdash, A. S., Alharthi, A., et al. (2022). Rhamnolipid-Coated iron oxide nanoparticles as a novel multitarget candidate against major foodborne E. coli serotypes and methicillin-resistant S. aureus. Microbiol. Spectr. 10 (4), e0025022. doi:10.1128/spectrum.00250-22
Sharifi, A., Mohammadzadeh, A., Zahraei Salehi, T., and Mahmoodi, P. (2018). Antibacterial, antibiofilm and antiquorum sensing effects of Thymus daenensis and Satureja hortensis essential oils against Staphylococcus aureus isolates. J. Appl. Microbiol. 124 (2), 379–388. doi:10.1111/jam.13639
Sharma, K., Mahato, N., and Lee, Y. R. (2018). Systematic study on active compounds as antibacterial and antibiofilm agent in aging onions. J. Food Drug Anal. 26 (2), 518–528. doi:10.1016/j.jfda.2017.06.009
Sharma, K., Pandey, S., Sekar, H., Alan, T., and Gundabala, V. (2023). Microfluidics based generation of curcumin loaded microfibrous mat against Staphylococcus aureus biofilm by photodynamic therapy. ACS Appl. Bio Mater 6 (3), 1092–1104. doi:10.1021/acsabm.2c00971
Sheikhy, M., Karbasizade, V., Ghanadian, M., and Fazeli, H. (2024). Evaluation of chlorogenic acid and carnosol for anti-efflux pump and anti-biofilm activities against extensively drug-resistant strains of Staphylococcus aureus and Pseudomonas aeruginosa. Microbiol. Spectr. 12 (9), e0393423. doi:10.1128/spectrum.03934-23
Shen, F., Ge, C., and Yuan, P. (2020). Metabolomics study reveals inhibition and metabolic dysregulation in Staphylococcus aureus planktonic cells and biofilms induced by carnosol. Front. Microbiol. 11, 538572. doi:10.3389/fmicb.2020.538572
Simpson, K. H., Bowden, M. G., Peacock, S. J., Arya, M., Höök, M., and Anvari, B. (2004). Adherence of Staphylococcus aureus fibronectin binding protein A mutants: an investigation using optical tweezers. Biomol. Eng. 21 (3-5), 105–111. doi:10.1016/j.bioeng.2004.08.001
Srinivasan, S., Harrington, G. W., Xagoraraki, I., and Goel, R. (2008). Factors affecting bulk to total bacteria ratio in drinking water distribution systems. Water Res. 42 (13), 3393–3404. doi:10.1016/j.watres.2008.04.025
Srivastava, P., Shukla, M., Kaul, G., Chopra, S., and Patra, A. K. (2019). Rationally designed curcumin based ruthenium(ii) antimicrobials effective against drug-resistant Staphylococcus aureus. Dalton Trans. 48 (31), 11822–11828. doi:10.1039/c9dt01650c
Sun, D., Nuan, L., Zhang, W., Yang, E., Mou, Z., Zhao, Z., et al. (2015). Quercetin-loaded PLGA nanoparticles: a highly effective antibacterial agent in vitro and anti-infection application in vivo. J. Nanoparticle Res. 18, 3. doi:10.1007/s11051-015-3310-0
Sun, N., Jiang, X., Meng, Q., Jiang, H., Yuan, Z., and Zhang, J. (2024). Preparation of nanoparticles loaded with quercetin and effects on bacterial biofilm and LPS-induced oxidative stress in dugesia japonica. Appl. Biochem. Biotechnol. 196 (1), 32–49. doi:10.1007/s12010-023-04543-5
Sun, Y., Sun, F., Feng, W., Wang, Q., Liu, F., Xia, P., et al. (2022). Luteolin inhibits the biofilm formation and cytotoxicity of methicillin-resistant Staphylococcus aureus via decreasing bacterial toxin synthesis. Evid. Based Complement. Altern. Med. 2022, 4476339. doi:10.1155/2022/4476339
Suntres, Z. E., Coccimiglio, J., and Alipour, M. (2015). The bioactivity and toxicological actions of carvacrol. Crit. Rev. Food Sci. Nutr. 55 (3), 304–318. doi:10.1080/10408398.2011.653458
Taglialegna, A., Navarro, S., Ventura, S., Garnett, J. A., Matthews, S., Penades, J. R., et al. (2016). Staphylococcal Bap proteins build amyloid scaffold biofilm matrices in response to environmental signals. PLoS Pathog. 12 (6), e1005711. doi:10.1371/journal.ppat.1005711
Tan, Y., Leonhard, M., Moser, D., Ma, S., and Schneider-Stickler, B. (2019). Antibiofilm efficacy of curcumin in combination with 2-aminobenzimidazole against single- and mixed-species biofilms of Candida albicans and Staphylococcus aureus. Colloids Surf. B Biointerfaces 174, 28–34. doi:10.1016/j.colsurfb.2018.10.079
Targhi, A. A., Moammeri, A., Jamshidifar, E., Abbaspour, K., Sadeghi, S., Lamakani, L., et al. (2021). Synergistic effect of curcumin-Cu and curcumin-Ag nanoparticle loaded niosome: enhanced antibacterial and anti-biofilm activities. Bioorg Chem. 115, 105116. doi:10.1016/j.bioorg.2021.105116
Teixeira, C. G. S., Sanitá, P. V., Ribeiro, A. P. D., Dias, L. M., Jorge, J. H., and Pavarina, A. C. (2020). Antimicrobial photodynamic therapy effectiveness against susceptible and methicillin-resistant Staphylococcus aureus biofilms. Photodiagnosis Photodyn. Ther. 30, 101760. doi:10.1016/j.pdpdt.2020.101760
Tormo María, Á., Martí, M., Valle, J., Manna Adhar, C., Cheung Ambrose, L., Lasa, I., et al. (2005). SarA is an essential positive regulator of Staphylococcus epidermidis biofilm development. J. Bacteriol. 187 (7), 2348–2356. doi:10.1128/jb.187.7.2348-2356.2005
Trigo-Gutierrez, J. K., Calori, I. R., De Oliveira Bárbara, G., Pavarina, A. C., Gonçalves, R. S., Caetano, W., et al. (2023). Photo-responsive polymeric micelles for the light-triggered release of curcumin targeting antimicrobial activity. Front. Microbiol. 14, 1132781. doi:10.3389/fmicb.2023.1132781
Uc-Cachón, A. H., Calvo-Irabien, L. M., Dzul-Beh, A. J., Dzib-Baak, H. E., Grijalva-Arango, R., and Molina-Salinas, G. M. (2024). Potential anti-infectious activity of essential oil chemotypes of lippia origanoides kunth on antibiotic-resistant Staphylococcus aureus strains. Plants (Basel) 13 (9), 1172. doi:10.3390/plants13091172
Ushimaru, P. I., Barbosa, L. N., Fernandes, A. A., Di Stasi, L. C., and Fernandes, A. (2012). In vitro antibacterial activity of medicinal plant extracts against Escherichia coli strains from human clinical specimens and interactions with antimicrobial drugs. Nat. Prod. Res. 26 (16), 1553–1557. doi:10.1080/14786419.2011.568943
Valliammai, A., Selvaraj, A., Mathumitha, P., Aravindraja, C., and Pandian, S. K. (2021). Polymeric antibiofilm coating comprising synergistic combination of citral and thymol prevents methicillin-resistant Staphylococcus aureus biofilm formation on titanium. Mater Sci. Eng. C Mater Biol. Appl. 121, 111863. doi:10.1016/j.msec.2021.111863
Valliammai, A., Selvaraj, A., Yuvashree, U., Aravindraja, C., and Karutha Pandian, S. (2020a). sarA-dependent antibiofilm activity of thymol enhances the antibacterial efficacy of rifampicin against Staphylococcus aureus. Front. Microbiol. 11, 1744. doi:10.3389/fmicb.2020.01744
Valliammai, A., Sethupathy, S., Ananthi, S., Priya, A., Selvaraj, A., Nivetha, V., et al. (2020b). Proteomic profiling unveils citral modulating expression of IsaA, CodY and SaeS to inhibit biofilm and virulence in methicillin-resistant Staphylococcus aureus. Int. J. Biol. Macromol. 158, 208–221. doi:10.1016/j.ijbiomac.2020.04.231
Venkateswaran, S., Henrique Dos Santos, O. D., Scholefield, E., Lilienkampf, A., Gwynne, P. J., Swann, D. G., et al. (2016). Fortified interpenetrating polymers - bacteria resistant coatings for medical devices. J. Mater Chem. B 4 (32), 5405–5411. doi:10.1039/c6tb01110a
Vipin, C., Saptami, K., Fida, F., Mujeeburahiman, M., Rao, S. S., Arun, A. B., et al. (2020). Potential synergistic activity of quercetin with antibiotics against multidrug-resistant clinical strains of Pseudomonas aeruginosa. PLoS One 15 (11), e0241304. doi:10.1371/journal.pone.0241304
Vitanza, L., Maccelli, A., Marazzato, M., Scazzocchio, F., Comanducci, A., Fornarini, S., et al. (2019). Satureja Montana L. essential oil and its antimicrobial activity alone or in combination with gentamicin. Microb. Pathog. 126, 323–331. doi:10.1016/j.micpath.2018.11.025
Von Salm, J. L., Witowski, C. G., Fleeman, R. M., Mcclintock, J. B., Amsler, C. D., Shaw, L. N., et al. (2016). Darwinolide, a new diterpene scaffold that inhibits methicillin-resistant Staphylococcus aureus biofilm from the antarctic sponge dendrilla membranosa. Org. Lett. 18 (11), 2596–2599. doi:10.1021/acs.orglett.6b00979
Vuong, C., Kocianova, S., Voyich, J. M., Yao, Y., Fischer, E. R., Deleo, F. R., et al. (2004). A crucial role for exopolysaccharide modification in bacterial biofilm formation, immune evasion, and virulence. J. Biol. Chem. 279 (52), 54881–54886. doi:10.1074/jbc.M411374200
Walczak, M., Michalska-Sionkowska, M., Olkiewicz, D., Tarnawska, P., and Warżyńska, O. (2021). Potential of carvacrol and thymol in reducing biofilm formation on technical surfaces. Molecules 26 (9), 2723. doi:10.3390/molecules26092723
Wang, H., Gong, X., Guo, X., Liu, C., Fan, Y.-Y., Zhang, J., et al. (2019). Characterization, release, and antioxidant activity of curcumin-loaded sodium alginate/ZnO hydrogel beads. Int. J. Biol. Macromol. 121, 1118–1125. doi:10.1016/j.ijbiomac.2018.10.121
Wang, S., Kang, O. H., and Kwon, D. Y. (2021). Trans-cinnamaldehyde exhibits synergy with conventional antibiotic against methicillin-resistant Staphylococcus aureus. Int. J. Mol. Sci. 22 (5), 2752. doi:10.3390/ijms22052752
Wang, S., Yao, J., Zhou, B., Yang, J., Chaudry, M. T., Wang, M., et al. (2018). Bacteriostatic effect of quercetin as an antibiotic alternative in vivo and its antibacterial mechanism in vitro. J. Food Prot. 81 (1), 68–78. doi:10.4315/0362-028X.JFP-17-214
Wang, W., Bao, X., Bové, M., Rigole, P., Meng, X., Su, J., et al. (2022). Antibiofilm activities of borneol-citral-loaded pickering emulsions against Pseudomonas aeruginosa and Staphylococcus aureus in physiologically relevant chronic infection models. Microbiol. Spectr. 10 (5), e0169622. doi:10.1128/spectrum.01696-22
Wang, Z., Bai, H., Lu, C., Hou, C., Qiu, Y., Zhang, P., et al. (2019). Light controllable chitosan micelles with ROS generation and essential oil release for the treatment of bacterial biofilm. Carbohydr. Polym. 205, 533–539. doi:10.1016/j.carbpol.2018.10.095
Wanninger, S., Lorenz, V., Subhan, A., and Edelmann, F. T. (2015). Metal complexes of curcumin – synthetic strategies, structures and medicinal applications. Chem. Soc. Rev. 44 (15), 4986–5002. doi:10.1039/C5CS00088B
Wertheim, H. F. L., Walsh, E., Choudhurry, R., Melles, D. C., Boelens, H. a.M., Miajlovic, H., et al. (2008). Key role for clumping factor B in Staphylococcus aureus nasal colonization of humans. PLOS Med. 5 (1), e17. doi:10.1371/journal.pmed.0050017
Wijesinghe, G. K., Feiria, S. B., Maia, F. C., Oliveira, T. R., Joia, F., Barbosa, J. P., et al. (2021). In-vitro antibacterial and antibiofilm activity of cinnamomum verum leaf oil against Pseudomonas aeruginosa, Staphylococcus aureus and Klebsiella pneumoniae. Acad Bras Cienc 93 (1), e20201507. doi:10.1590/0001-3765202120201507
Wojnicz, D., Kucharska, A. Z., Sokół-Łętowska, A., Kicia, M., and Tichaczek-Goska, D. (2012). Medicinal plants extracts affect virulence factors expression and biofilm formation by the uropathogenic Escherichia coli. Urol. Res. 40 (6), 683–697. doi:10.1007/s00240-012-0499-6
Wu, X., Ma, G. L., Chen, H. W., Zhao, Z. Y., Zhu, Z. P., Xiong, J., et al. (2023). Antibacterial and antibiofilm efficacy of the preferred fractions and compounds from Euphorbia humifusa (herba euphorbiae humifusae) against Staphylococcus aureus. J. Ethnopharmacol. 306, 116177. doi:10.1016/j.jep.2023.116177
Xu, J., Lin, Q., Sheng, M., Ding, T., Li, B., Gao, Y., et al. (2022). Antibiofilm effect of cinnamaldehyde-chitosan nanoparticles against the biofilm of Staphylococcus aureus. Antibiot. (Basel) 11 (10), 1403. doi:10.3390/antibiotics11101403
Yadav, M. K., Chae, S. W., Im, G. J., Chung, J. W., and Song, J. J. (2015). Eugenol: a phyto-compound effective against methicillin-resistant and methicillin-sensitive Staphylococcus aureus clinical strain biofilms. PLoS One 10 (3), e0119564. doi:10.1371/journal.pone.0119564
Yadav, M. K., Park, S.-W., Chae, S.-W., Song, J.-J., and Kim, H. C. (2013). Antimicrobial activities of Eugenia caryophyllata extract and its major chemical constituent eugenol against Streptococcus pneumoniae. APMIS 121 (12), 1198–1206. doi:10.1111/apm.12067
Yang, N., Wu, T., Li, M., Hu, X., Ma, R., Jiang, W., et al. (2025). Silver-quercetin-loaded honeycomb-like Ti-based interface combats infection-triggered excessive inflammation via specific bactericidal and macrophage reprogramming. Bioact. Mater 43, 48–66. doi:10.1016/j.bioactmat.2024.09.012
Ye, Y., Yang, Q., Fang, F., and Li, Y. (2015). The camelliagenin from defatted seeds of Camellia oleifera as antibiotic substitute to treat chicken against infection of Escherichia coli and Staphylococcus aureus. BMC Vet. Res. 11, 214. doi:10.1186/s12917-015-0529-z
Yuan, Q., Feng, W., Wang, Y., Wang, Q., Mou, N., Xiong, L., et al. (2022). Luteolin attenuates the pathogenesis of Staphylococcus aureus by interfering with the agr system. Microb. Pathog. 165, 105496. doi:10.1016/j.micpath.2022.105496
Yuan, Z., Dai, Y., Ouyang, P., Rehman, T., Hussain, S., Zhang, T., et al. (2020). Thymol inhibits biofilm formation, eliminates pre-existing biofilms, and enhances clearance of methicillin-resistant Staphylococcus aureus (MRSA) in a mouse peritoneal implant infection model. Microorganisms 8 (1), 99. doi:10.3390/microorganisms8010099
Yuyama, K. T., Rohde, M., Molinari, G., Stadler, M., and Abraham, W. R. (2020). Unsaturated fatty acids control biofilm formation of Staphylococcus aureus and other gram-positive bacteria. Antibiot. (Basel) 9 (11), 788. doi:10.3390/antibiotics9110788
Zhang, H., Zhou, W., Zhang, W., Yang, A., Liu, Y., Jiang, Y., et al. (2014). Inhibitory effects of citral, cinnamaldehyde, and tea polyphenols on mixed biofilm formation by foodborne Staphylococcus aureus and Salmonella enteritidis. J. Food Prot. 77 (6), 927–933. doi:10.4315/0362-028x.Jfp-13-497
Zhang, L., Gray, L., Novick, R. P., and Ji, G. (2002). Transmembrane topology of AgrB, the protein involved in the post-translational modification of AgrD in Staphylococcus aureus. J. Biol. Chem. 277 (38), 34736–34742. doi:10.1074/jbc.M205367200
Zhang, L., and Ji, G. (2004). Identification of a staphylococcal AgrB segment(s) responsible for group-specific processing of AgrD by gene swapping. J. Bacteriol. 186 (20), 6706–6713. doi:10.1128/jb.186.20.6706-6713.2004
Zhang, X., Guo, Y., Guo, L., Jiang, H., and Ji, Q. (2018). In vitro evaluation of antioxidant and antimicrobial activities of melaleuca alternifolia essential oil. Essent. Oil 2018 (1), 2396109. doi:10.1155/2018/2396109
Zhang, Z., Chen, M., Wang, J., Liu, M., Guo, R., Zhang, L., et al. (2024). Hyaluronic acid-modified micelles of azithromycin and quercetin against infections caused by methicillin-resistant Staphylococcus aureus. Int. J. Nanomedicine 19, 9637–9658. doi:10.2147/ijn.S476471
Zhao, N., Cai, R., Zhang, Y., Wang, X., and Zhou, N. (2022). A pH-gated functionalized hollow mesoporous silica delivery system for photodynamic sterilization in Staphylococcus aureus biofilm. Mater. (Basel) 15 (8), 2815. doi:10.3390/ma15082815
Keywords: S. aureus, biofilm, natural compounds, curcumin, cinnamaldehyde, carvacrol, eugenol, thymol
Citation: Kashi M, Noei M, Chegini Z and Shariati A (2024) Natural compounds in the fight against Staphylococcus aureus biofilms: a review of antibiofilm strategies. Front. Pharmacol. 15:1491363. doi: 10.3389/fphar.2024.1491363
Received: 04 September 2024; Accepted: 08 November 2024;
Published: 20 November 2024.
Edited by:
Alejandro Madrid, Universidad de Playa Ancha, ChileReviewed by:
Gabriela Cristina Fernandez, National Scientific and Technical Research Council (CONICET), ArgentinaJaroslaw Widelski, Medical University of Lublin, Poland
Copyright © 2024 Kashi, Noei, Chegini and Shariati. This is an open-access article distributed under the terms of the Creative Commons Attribution License (CC BY). The use, distribution or reproduction in other forums is permitted, provided the original author(s) and the copyright owner(s) are credited and that the original publication in this journal is cited, in accordance with accepted academic practice. No use, distribution or reproduction is permitted which does not comply with these terms.
*Correspondence: Aref Shariati, YXJlZnNoYXJpYXRpMDExMUBnbWFpbC5jb20=; Zahra Chegini, cGFyaXNhLmNoZWdpbmk3MkBnbWFpbC5jb20=