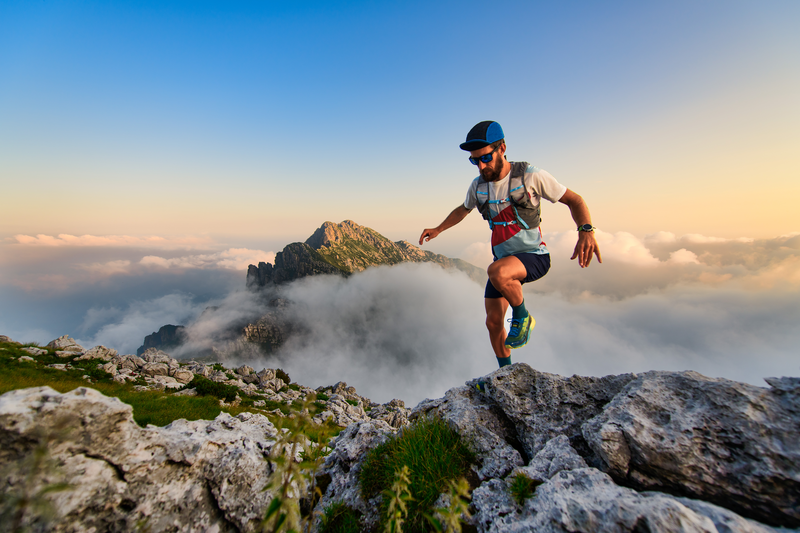
95% of researchers rate our articles as excellent or good
Learn more about the work of our research integrity team to safeguard the quality of each article we publish.
Find out more
ORIGINAL RESEARCH article
Front. Pharmacol. , 10 December 2024
Sec. Neuropharmacology
Volume 15 - 2024 | https://doi.org/10.3389/fphar.2024.1489888
Objective: Resistance to antiseizure medications (ASMs) is a major challenge in the treatment of patients with epilepsy. Despite numerous newly marketed ASMs, the proportion of drug-resistant people with epilepsy has not significantly decreased over the years. Therefore, novel and innovative seizure models for preclinical drug screening are highly desirable. Here, we explore the efficacy of a broad spectrum of ASMs in suppressing seizure activity in two established Drosophila melanogaster bang-sensitive mutants. These mutants respond with seizures to mechanical stimulation, providing a promising platform for screening novel ASMs.
Methods: Seven frequently used ASMs (brivaracetam, cenobamate, lacosamide, lamotrigine, levetiracetam, phenytoin, and valproate) were administered to the bang-sensitive mutants easily shocked2F (eas2F) and paralyticbss1 (parabss1). After 48 h of treatment, the flies were vortexed to induce mechanical stimulation. The seizure probability (i.e., ratio of seizing and non-seizing flies) as well as the seizure duration were analyzed.
Results: In case of eas2F mutants, treatment with the sodium channel blockers phenytoin and lamotrigine resulted in a robust reduction of seizure probability, whereas flies treated with lacosamide showed a decrease in seizure duration. Treatment with valproate resulted in both a reduction in seizure probability and in seizure duration. In contrast, levetiracetam, brivaracetam and cenobamate had no effect on the bang-sensitive phenotype of eas2F flies. In case of parabss1 flies, none of the tested medications significantly reduced seizure activity, supporting its role as a model of intractable epilepsy.
Significance: Our results show that particularly sodium channel blockers as well as valproate are effective in suppressing seizure activity in the bang-sensitive mutant eas2F. These findings demonstrate the usability of Drosophila for screening drugs with antiseizure properties. Due to fewer ethical concerns, the short life cycle, and low maintenance costs, Drosophila might provide an attractive and innovative high-throughput model for the discovery of novel antiseizure compounds.
Epilepsy is one of the most common chronic neurological disorders that affects more than 45 million people worldwide (Beghi et al., 2019). The main objective of epilepsy treatment is to achieve complete seizure control. However, about one-third of patients remain refractory to currently available antiseizure medications (ASMs) and experience ongoing seizures (Kwan and Brodie, 2000). Drug-resistant epilepsy is associated with an increased risk of psychosocial dysfunction, injuries, restricted quality of life, and premature death (Loscher et al., 2020). Thus, there is an unmet clinical need to develop more effective therapeutics, particularly with novel mechanisms of action.
The identification of potential novel therapeutics requires preclinical seizure models. A broad range of different animal models has been introduced over the years, such as the maximal electroshock seizure (MES) and the subcutaneous pentylenetetrazole (PTZ) seizure tests in rodents (Loscher, 2011; Loscher, 2017). However, despite the introduction of numerous new ASMs, the rate of drug-resistant people with epilepsy has not significantly declined (Chen et al., 2018). One possible explanation could be that current models might only identify substances with similar characteristics and therefore have no efficacy in case of drug-resistant epilepsies (Loscher, 2011). In addition, it must be considered that rodent models have some further limitations. For instance, the throughput of candidate compounds in drug screenings is considerably limited due to regulatory restrictions (e.g., by Institutional Animal Care and Use Committees), high costs as well as laborious experimental procedures. Additional model organisms enabling high-throughput screenings in a more cost-effective manner are highly desirable. Recent research has therefore focused on the development of alternative seizure models, including non-mammalian model organisms, such as roundworms, zebrafish, and fruit flies (Baraban, 2007; Cunliffe et al., 2015; Johan Arief et al., 2018; Rosch et al., 2019; Kasture et al., 2022; Fischer et al., 2023; Miguel Sanz et al., 2023; Shah et al., 2024).
The fruit fly Drosophila melanogaster has served as a model organism in biomedical research for more than a century (Bellen and Yamamoto, 2015) and has greatly advanced the understanding of many fundamental biological processes, such as genetics, inheritance, and development (Jennings, 2011; Yamaguchi and Yoshida, 2018). Among features that make Drosophila an attractive model organism are: fewer ethical restrictions (in line with the 3R principles - Replacement, Reduction, Refinement), a short generation time, the ease of maintenance, the cost-effectiveness, and the availability of a broad spectrum of sophisticated genetic tools (Jennings, 2011). The genome of the fly has been fully sequenced and encodes around 13,600 genes distributed across four pairs of chromosomes (Adams et al., 2000). Approximately 75% of all human disease-associated genes have a corresponding gene in Drosophila, making it a suitable model to study human disorders (Reiter et al., 2001). Despite obvious anatomical differences between fruit flies and humans, fundamental cellular and molecular processes show a high degree of similarity between these two species. The adult fly exhibits structures that are functionally analogous to the mammalian brain, heart, lung, kidney, gut, and reproductive system (Pandey and Nichols, 2011). The fly brain consists of some 100,000 neurons capable of mediating a wide range of complex behaviors, such as courtship, navigation, sleep, learning and memory (Pandey and Nichols, 2011). In addition, flies respond to various drugs that act within the central nervous system in a similar way to mammals (Pandey and Nichols, 2011), making it a suitable tool for drug screening (Desai et al., 2006; Chang et al., 2008).
The fruit fly has been used as a model to study epilepsy since the discovery of bang-sensitive mutants (for review see Fischer et al., 2023). These mutant flies characteristically respond to a mechanical shock, termed “bang”, with stereotypic seizure activity. This complex behavioral response can be divided into different phases, including an initial seizure, followed by temporary paralysis, and a recovery seizure (Song and Tanouye, 2008; Parker et al., 2011a; Fischer et al., 2023). A well-established example of a bang-sensitive mutant is easily shocked2F (eas2F), carrying a variant in the gene encoding ethanolamine kinase, an enzyme involved in the synthesis of phosphatidylethanolamine. Presumably, mutations in this gene alter the phospholipid composition of membranes, which then leads to disturbances in neuronal excitability (Pavlidis et al., 1994). Paralyticbss1 (parabss1), another bang-sensitive fly line, harbors a gain-of-function mutation in the voltage-gated sodium channel gene para (Parker et al., 2011b), which is orthologous to the human SCN1A to SCN5A and SCN7A to SCN11A genes (Takai et al., 2020). This mutant is characterized by the most severe phenotype of all bang-sensitive mutants. Furthermore, it has been proposed as a model for intractable epilepsy because its phenotype was the most difficult to suppress by ASMs and seizure-suppressor mutations in previous analyses (Kroll et al., 2015).
In this study, we screened the capacity of seven frequently used ASMs to suppress seizure activity in two established Drosophila bang-sensitive mutants, namely eas2F and parabss1. We show that several sodium channel blockers as well as valproate can suppress seizure activity in the eas2F mutant, whereas none of the tested drugs significantly influences the bang-sensitive phenotype of parabss1 flies.
All flies were kept in plastic vials containing standard cornmeal food at 25°C. The eas2F flies were kindly donated by Richard Baines (University of Manchester, United Kingdom). The parabss1 flies were a gift from Deepti Trivedi (National Centre for Biological Sciences, India). The Canton-S flies (#64349) were obtained from the Bloomington Drosophila Stock Center (IN, United States). The following drugs were used: brivaracetam (Tocris Bioscience), cenobamate (Alsachim), lacosamide (Sigma-Aldrich), lamotrigine (Selleck Chemicals), levetiracetam (Sigma-Aldrich), phenytoin (Sigma-Aldrich) and valproate (Sigma-Aldrich). Levetiracetam, brivaracetam and valproate were dissolved in water, whereas lacosamide, lamotrigine, phenytoin and cenobamate were dissolved in ethanol. In case of the eas2F mutant, three different drug concentrations were used, i.e., 0.03, 0.3 and 3 mM. These concentrations were selected based on a recommendation for pilot drug screenings in Drosophila (Pandey and Nichols, 2011). In case of parabss1, we only used the highest concentration (3 mM). The method of drug preparation was adapted from Polet et al. (2024). 100 μL of the drug solution were equally distributed by pipet on top of the food and left to impregnate overnight. The concentrations noted in the graphs refer to the concentration of the solution added on top of the food (e.g., 100 μL of a 3 mM solution). Flies were kept on ASM containing food for 48 h. This period was chosen based on previous studies (e.g., Kasture et al., 2022). Solvent only treated flies served as control.
Adult male flies were collected using CO2 1–3 days after eclosion and transferred to a food vial prepared with the respective drug (max. 10 flies per vial). After 48 h, the flies were transferred to an empty vial (max. 5 flies per vial) and allowed to recover from anesthesia for 1–2 h. For seizure induction, the vials were vortexed using a Vortex-Genie 2 (Scientific Industries) at maximum speed for 10 s as described previously (Kuebler and Tanouye, 2000; Mituzaite et al., 2021). The seizure activity was recorded using a video camera. The ratio of seizing and non-seizing flies (termed seizure probability) as well as the seizure duration were measured. The seizure duration was defined as the time from the end of the vortex stimulation until the fly regained posture and mobility.
To test whether the rate of seizing flies differed between the testing conditions, we performed a Fisher’s exact test. For seizure duration, statistical outliers were excluded using the ROUTE method, and normal distribution was confirmed by Shapiro-Wilk test. The seizure duration was analyzed by a one-way ANOVA followed by Tukey’s post hoc t-tests. Statistical significance was set at p < 0.05 with *p < 0.05, **p < 0.01, ***p < 0.001. All error bars shown represent the standard deviation (SD).
To validate the utility of Drosophila as a screening tool for antiseizure compounds, we fed two established bang-sensitive mutants with a broad spectrum of frequently used ASMs for 48 h. The tested drugs comprised (i) sodium channel blockers (phenytoin, lacosamide, lamotrigine), (ii) synaptic vesicle glycoprotein 2 A (SV2A) modulators (levetiracetam, brivaracetam) and (iii) drugs with multiple mechanisms of action (valproate, cenobamate). Drug consumption was verified using food coloring (Supplementary Figure S1). For the initial screening, we chose the eas2F mutant because it features a relatively short seizure duration compared to other bang-sensitive mutants and is thus a more suitable model for large-scale screens. The seizure activity was induced by vortex stimulation (Kuebler and Tanouye, 2000; Mituzaite et al., 2021) and the seizure probability and seizure duration were measured.
To analyze possible confounding effects of the solvent on the seizure activity, we compared the seizure activity of eas2F flies treated with either water, ethanol, or dimethyl sulfoxide (DMSO) alone. Treatment with DMSO lead to a significant decrease in seizure probability and seizure duration. In contrast, no differences were observed between flies treated with water or ethanol (Figures 1A, B). Therefore, we used ethanol as a solvent for hydrophobic agents instead of DMSO in our screening experiments.
Figure 1. Seizure activity of eas2F flies treated with different solvents (A) Percentage of seizing eas2F flies after 10 s of vortex stimulation. Before testing, the flies were fed with 100 μL of water, ethanol or DMSO for 48 h. The data for flies treated with water or ethanol were derived from experiments shown in Figure 2. For statistical analysis, we performed a Fisher’s exact test (***p < 0.001, compared to water and ethanol). N indicates the total number of flies tested per condition. (B) Seizure duration in seconds of the same eas2F flies. The data was analyzed by a one-way ANOVA followed by Tukey’s post hoc t-tests (**p < 0.01, ***p < 0.001). Error bars indicate SD.
In our screening paradigm, we tested three different concentrations (0.03, 0.3, and 3 mM) of each ASM, based on a recommendation for pilot drugs screenings in Drosophila (Pandey and Nichols, 2011). Treatment with the sodium channel blockers phenytoin and lamotrigine resulted in a dose-dependent reduction of seizure probability, whereas no significant effect was observed for the sodium channel blocker lacosamide (Figure 2). In addition to phenytoin and lamotrigine, a robust decrease in seizure probability was observed in flies treated with valproate (Figure 2). Interestingly, this effect was only significant for 0.03 and 0.3 mM but not for 3 mM. In contrast, no effects on the seizure probability were observed for the SV2A modulators levetiracetam and brivaracetam as well as for cenobamate (Figure 2). Next, we investigated the effects of the same ASMs on the seizure duration of eas2F flies. Here, flies treated with lacosamide showed a significantly shorter seizure duration than the control flies, but only at the highest concentration (3 mM) (Figure 3). In addition, flies treated with valproate also showed a significantly reduced seizure duration, whereas no changes were observed for phenytoin, lacosamide, levetiracetam, brivaracetam, and cenobamate (Figure 3).
Figure 2. Seizure probability of eas2F flies treated with seven different ASMs. Percentage of seizing flies after 10 s of vortex stimulation. Before testing, the flies were treated with three different concentrations (0.03, 0.3, 3 mM) of seven different ASMs (phenytoin, lacosamide, lamotrigine, cenobamate, valproate, levetiracetam, brivaracetam) for 48 h. For statistical analysis, we performed a Fisher’s exact test (*p < 0.05, **p < 0.01, ***p < 0.001, compared to 0 mM). N indicates the total number of tested flies per condition.
Figure 3. Seizure duration of eas2F flies treated with seven different ASMs. Seizure duration in seconds of flies after 10 s of vortex stimulation. Before testing, the flies were treated with three different concentrations (0.03, 0.3, 3 mM) of seven different ASMs (phenytoin, lacosamide, lamotrigine, cenobamate, valproate, levetiracetam, brivaracetam) for 48 h. For statistical analysis, we performed a one-way ANOVA followed by Tukey’s post hoc t-tests (*p < 0.05, ***p < 0.001, compared to 0 mM). Error bars indicate SD.
To investigate whether the observed effects on the seizure activity are specific for the eas2F flies, we additionally tested the same ASMs in parabss1, another bang-sensitive mutant and model of intractable epilepsy. Here, we only tested the highest concentration (3 mM) of each ASM. None of our ASMs showed a significant effect on the seizure probability of these flies (Figure 4A). However, we noted a tendency for some sodium channel inhibitors (lamotrigine and cenobamate) to reduce the rate of seizing flies, which seems not surprising as parabss1 is known to carry a gain-of-function mutation in the voltage-gated sodium channel. In addition, none of our ASMs had a significant effect on the seizure duration of parabss1 flies (Figure 4B). Importantly, these results confirm parabss1 as a model of refractory epilepsy.
Figure 4. Seizure activity of parabss1 flies treated with seven different ASMs. (A) Percentage of seizing flies after 10 s of vortex stimulation. Before testing, the flies were treated with 3 mM of seven different ASMs (phenytoin, lacosamide, lamotrigine, cenobamate, valproate, levetiracetam, brivaracetam) for 48 h. For statistical analysis, we performed a Fisher’s exact test (no significance, compared to control). N indicates the total number of tested flies per condition. (B) Seizure duration in seconds of the same flies described above. For statistical analysis, we performed a one-way ANOVA followed by Tukey’s post hoc t-tests (no significance, compared to control). Error bars indicate SD.
To demonstrate the utility of the fruit fly Drosophila as a screening tool for novel antiseizure compounds, we tested the capacity of seven frequently used ASMs to suppress seizure activity in two established bang-sensitive mutants, eas2F and parabss1. To avoid possible confounding effects caused by the solvents in which the ASMs are dissolved, we also investigated the effects of water, ethanol, and DMSO on the seizure activity of the eas2F mutant. Surprisingly, we found that treatment with DMSO alone caused a strong decrease in seizure probability and seizure duration, whereas no significant differences were found between flies treated with water or ethanol. The exact mechanisms of how DMSO suppressed seizure activity in this model are not clear. However, DMSO is known to block activation of sodium channels (Larsen et al., 1996) and to decrease GABA-, NMDA- and AMPA-induced ion currents (Nakahiro et al., 1992; Lu and Mattson, 2001). These effects might alter the balance between inhibition and excitation and thus affect neuronal excitability. Our findings are also consistent with a recent study demonstrating a short-lasting decrease in epileptiform activity in a mouse model of chronic temporal lobe epilepsy after injection of 100% DMSO (Widmann et al., 2023). Therefore, the use of DMSO as a solvent should be evaluated cautiously when studying seizure activity in Drosophila bang-sensitive mutants. A potential antiseizure effect of DMSO should be monitored closely by control experiments.
In case of eas2F flies, we found that treatment with the sodium channel blockers phenytoin and lamotrigine caused a robust reduction in seizure probability, whereas treatment with lacosamide resulted in a decrease in seizure duration. Interestingly, cenobamate, which is also classified as a sodium channel blocker, had no significant effect on the seizure activity of eas2F flies. These divergent effects among sodium channel blockers might be explained by subtle differences in their mechanism of action. For instance, phenytoin and lamotrigine exert their antiseizure effects predominantly by enhancing fast inactivation of sodium channels, whereas the sodium channel blocker lacosamide primarily increases slow inactivation (Rogawski et al., 2015). In contrast, cenobamate has a pronounced effect on persistent rather than transient sodium currents. In addition, it is also an allosteric modulator of GABAA receptors (Roberti et al., 2021). Apart from phenytoin, lamotrigine and lacosamide, also valproate showed a significant effect on the seizure activity of eas2F flies. Notably, valproate caused both a reduction in seizure probability and in seizure duration. This pronounced effect might be due to the combination of different mechanisms by which valproate exerts its antiseizure effects, including inhibition of voltage-gated sodium and T-type calcium channels as well as by increasing the concentration of GABA (Broicher et al., 2007; Loscher and Klein, 2021). Of note, the effect on seizure probability was not significant at the highest concentration, which might be explained by toxic effects that have been reported at higher doses (Polet et al., 2024). No significant effects on the seizure activity were found for the SV2A modulators levetiracetam and brivaracetam. There are several possible reasons for this observation. For instance, the binding to the Drosophila orthologue of SV2A, CG3168, might be insufficient or the Drosophila orthologue is functionally not amply identical to the human SV2A. Interestingly, levetiracetam was also devoid of any antiseizure activity in two well-established screening models for ASMs - the MES and PTZ seizure tests - highlighting its unique profile among current ASMs (Klitgaard et al., 1998). In addition to eas2F, we also tested the same ASMs in another bang-sensitive mutant, i.e., parabss1. This mutant carries a gain-of-function mutation in the Drosophila voltage-gated sodium channel (Parker et al., 2011b) and is characterized by the most severe phenotype of all bang-sensitive mutants. Moreover, its phenotype is the most difficult to suppress by ASMs or seizure suppressor mutations. Hence, it has been proposed as a model of intractable epilepsy (Kroll et al., 2015). Concordantly, none of our tested drugs had a significant effect on the seizure probability or seizure duration of this mutant, supporting its role as a model of refractory epilepsy. However, it should be noted that we only tested the highest concentration of each ASM in the parabss1 mutant. Hence, we cannot rule out a potential effect at lower doses, e.g., of valproate as seen in the eas2F mutant. Importantly, variability in drug response is also commonly observed in people with epilepsy, a phenomenon attributed to genetic differences, the heterogeneous nature of epilepsy syndromes, and individual variations in drug metabolism (Heavin et al., 2019; Wolking et al., 2020a; Wolking et al., 2020b; Wolking et al., 2021). As demonstrated in this study, the use of Drosophila mutants effectively models this variability. Investigating why certain drugs are not effective in one mutant but succeed in another could reveal new targets for ASMs or novel mechanisms of drug resistance, which could be pivotal for overcoming treatment-resistant forms of epilepsy in humans.
Drosophila offers several advantages as a model for drug screening, including fewer ethical concerns, low maintenance costs, and high progeny numbers for robust statistical analysis. Furthermore, screening novel drug candidates in an in vivo model, such as Drosophila, enables the identification of high-quality hits that already exhibit key features such as oral availability, metabolic stability, and low toxicity (Pandey and Nichols, 2011). These characteristics cannot yet be adequately mimicked in cell culture experiments or biochemical assays (Pandey and Nichols, 2011). Another advantage of Drosophila is the ease of generating transgenic flies expressing human disease-associated variants (Link and Bellen, 2020), offering a promising approach to test personalized therapy strategies. Indeed, there are already several studies exploring disease-specific therapies in Drosophila. For instance, a recent study investigated the effectiveness of several ASMs in a Drosophila model of KCNT1-epilepsy (Hussain et al., 2024). The authors found that cannabidiol decreased the seizure phenotype of flies expressing the human KCNT1 gene in GABAergic neurons with three different patient mutations. Furthermore, they showed that vigabatrin significantly reduced the seizure activity in two of the tested mutants, but increased seizure activity in the third one. Another study explored the efficacy of several ASMs in a Drosophila model of North Sea progressive myoclonus epilepsy (Polet et al., 2024). The authors showed that treatment with sodium barbital, clonazepam, ganaxolone and ethosuximide resulted in a significant reduction of heat-induced seizures in flies with glial knockdown of Membrin, the orthologue of human GOSR2. Another study found that the serotonin precursor 5-hydroxytryptophan (5-HTP) suppressed heat-induced seizures in a Drosophila model of Dravet Syndrome but increased seizure-activity in a model of genetic epilepsy with febrile seizures plus (GEFS+) (Schutte et al., 2014). Importantly, these studies further support Drosophila as a model for drug screening.
However, some limitations should be considered when using whole animals in large-scale screens. For instance, the actual physiological concentrations of candidate compounds within the model organism can hardly be predicted. Therefore, it is hard to interpret whether negative findings result from poor absorption, distribution issues, or an actual absence of biological activity (Giacomotto and Segalat, 2010). At least in our case, food coloring was used to verify consumption of the drugs. However, it is noteworthy that it is still hardly possible to determine the exact amount of the ingested drug. Hence, we cannot rule out subtle differences in drug intake. Furthermore, the duration of treatment that is required to achieve a positive effect is difficult to estimate. Thus, we cannot rule out that a longer treatment would have resulted in a stronger antiseizure effect in our experiments. However, the main purpose of using small animals in large-scale screens is to streamline the pool of candidate compounds to those of higher quality, which ultimately promises both time and cost efficiency (Giacomotto and Segalat, 2010).
In the present study, we screened the capacity of a broad range of ASMs to suppress seizures in two established Drosophila bang-sensitive mutants. We found a distinct, ASM-dependent pattern of seizure response for sodium channel blockers and valproate in eas2F flies and broad drug-resistance in parabss1 flies. Our results imply that the fruit fly might be a suitable model for large-scale screening of anti-seizure agents, promising a higher pace and throughput, and bearing the potential to advance the discovery of novel antiseizure compounds. Finally, we want to emphasize that the fruit fly is not meant to replace rodent and other animal models in drug discovery but complement current drug discovery pipelines as a high-throughput instrument.
The raw data supporting the conclusions of this article will be made available by the authors, without undue reservation.
FF: Conceptualization, Formal Analysis, Investigation, Methodology, Visualization, Writing–original draft, Writing–review and editing. RK: Methodology, Writing–review and editing. HK: Conceptualization, Methodology, Resources, Supervision, Writing–review and editing. AV: Conceptualization, Resources, Supervision, Writing–review and editing, Formal Analysis, Methodology. YW: Conceptualization, Resources, Supervision, Writing–review and editing, Funding acquisition. SW: Conceptualization, Formal Analysis, Funding acquisition, Supervision, Writing–review and editing.
The author(s) declare that financial support was received for the research, authorship, and/or publication of this article. This work was supported by the German Research Foundation (WO 2385/2-1 to SW), the DFG/FNR INTER Research Unit FOR2715 (WE 4896/4-1 and WE 4896/4-2 to YW), and the Federal Ministry for Education and Research (Treat-ION, 01GM2210B to YW).
SW has received travel support and speaker honoraria from Angelini Pharma. YW is member of an advisory board of Angelini Pharma.
The remaining authors declare that the research was conducted in the absence of any commercial or financial relationships that could be construed as a potential conflict of interest.
All claims expressed in this article are solely those of the authors and do not necessarily represent those of their affiliated organizations, or those of the publisher, the editors and the reviewers. Any product that may be evaluated in this article, or claim that may be made by its manufacturer, is not guaranteed or endorsed by the publisher.
The Supplementary Material for this article can be found online at: https://www.frontiersin.org/articles/10.3389/fphar.2024.1489888/full#supplementary-material
SUPPLEMENTARY FIGURE S1 | Verification of drug intake. The following drugs were diluted with food coloring (brilliant blue FCF; E 133) to a final concentration of 3 mM: brivaracetam (BRV), cenobamate (CBM), lacosamide (LCM), lamotrigine (LTG), levetiracetam (LEV), phenytoin (PHT), valproate (VPA). 100 µL of the drug/food coloring solution were equally distributed by pipet on top of the food and left to impregnate overnight. Adult male flies (Canton-S) were collected using CO2 1–3 days after eclosion and transferred to a food vial prepared with the respective drug/food coloring solution (10 flies per vial). After 48 h, the abdomen of each fly was visually inspected under a microscope. All flies exhibited a blue colored abdomen, confirming ingestion of each ASM. Untreated flies (without food coloring) served as control.
Adams, M. D., Celniker, S. E., Holt, R. A., Evans, C. A., Gocayne, J. D., Amanatides, P. G., et al. (2000). The genome sequence of Drosophila melanogaster. Science 287 (5461), 2185–2195. doi:10.1126/science.287.5461.2185
Baraban, S. C. (2007). Emerging epilepsy models: insights from mice, flies, worms and fish. Curr. Opin. Neurol. 20 (2), 164–168. doi:10.1097/WCO.0b013e328042bae0
Beghi, E., Giussani, G., Nichols, E., Abd-Allah, F., Abdela, J., Abdelalim, A., et al. (2019). Global, regional, and national burden of epilepsy, 1990–2016: a systematic analysis for the Global Burden of Disease Study 2016. Lancet Neurology 18 (4), 357–375. doi:10.1016/s1474-4422(18)30454-x
Bellen, H. J., and Yamamoto, S. (2015). Morgan's legacy: fruit flies and the functional annotation of conserved genes. Cell 163 (1), 12–14. doi:10.1016/j.cell.2015.09.009
Broicher, T., Seidenbecher, T., Meuth, P., Munsch, T., Meuth, S. G., Kanyshkova, T., et al. (2007). T-current related effects of antiepileptic drugs and a Ca2+ channel antagonist on thalamic relay and local circuit interneurons in a rat model of absence epilepsy. Neuropharmacology 53 (3), 431–446. doi:10.1016/j.neuropharm.2007.05.030
Chang, S., Bray, S. M., Li, Z., Zarnescu, D. C., He, C., Jin, P., et al. (2008). Identification of small molecules rescuing fragile X syndrome phenotypes in Drosophila. Nat. Chem. Biol. 4 (4), 256–263. doi:10.1038/nchembio.78
Chen, Z., Brodie, M. J., Liew, D., and Kwan, P. (2018). Treatment outcomes in patients with newly diagnosed epilepsy treated with established and new antiepileptic drugs: a 30-year longitudinal cohort study. JAMA Neurol. 75 (3), 279–286. doi:10.1001/jamaneurol.2017.3949
Cunliffe, V. T., Baines, R. A., Giachello, C. N., Lin, W. H., Morgan, A., Reuber, M., et al. (2015). Epilepsy research methods update: understanding the causes of epileptic seizures and identifying new treatments using non-mammalian model organisms. Seizure 24, 44–51. doi:10.1016/j.seizure.2014.09.018
Desai, U. A., Pallos, J., Ma, A. A., Stockwell, B. R., Thompson, L. M., Marsh, J. L., et al. (2006). Biologically active molecules that reduce polyglutamine aggregation and toxicity. Hum. Mol. Genet. 15 (13), 2114–2124. doi:10.1093/hmg/ddl135
Fischer, F. P., Karge, R. A., Weber, Y. G., Koch, H., Wolking, S., and Voigt, A. (2023). Drosophila melanogaster as a versatile model organism to study genetic epilepsies: an overview. Front. Mol. Neurosci. 16, 1116000. doi:10.3389/fnmol.2023.1116000
Giacomotto, J., and Segalat, L. (2010). High-throughput screening and small animal models, where are we? Br. J. Pharmacol. 160 (2), 204–216. doi:10.1111/j.1476-5381.2010.00725.x
Heavin, S. B., McCormack, M., Wolking, S., Slattery, L., Walley, N., Avbersek, A., et al. (2019). Genomic and clinical predictors of lacosamide response in refractory epilepsies. Epilepsia Open 4 (4), 563–571. doi:10.1002/epi4.12360
Hussain, R., Lim, C. X., Shaukat, Z., Islam, A., Caseley, E. A., Lippiat, J. D., et al. (2024). Drosophila expressing mutant human KCNT1 transgenes make an effective tool for targeted drug screening in a whole animal model of KCNT1-epilepsy. Sci. Rep. 14 (1), 3357. doi:10.1038/s41598-024-53588-x
Jennings, B. H. (2011). Drosophila – a versatile model in biology and medicine. Mater. Today 14 (5), 190–195. doi:10.1016/s1369-7021(11)70113-4
Johan Arief, M. F., Choo, B. K. M., Yap, J. L., Kumari, Y., and Shaikh, M. F. (2018). A systematic review on non-mammalian models in epilepsy research. Front. Pharmacol. 9, 655. doi:10.3389/fphar.2018.00655
Kasture, A. S., Fischer, F. P., Kunert, L., Burger, M. L., Burgstaller, A. C., El-Kasaby, A., et al. (2022). Drosophila melanogaster as a model for unraveling unique molecular features of epilepsy elicited by human GABA transporter 1 variants. Front. Neurosci. 16, 1074427. doi:10.3389/fnins.2022.1074427
Klitgaard, H., Matagne, A., Gobert, J., and Wulfert, E. (1998). Evidence for a unique profile of levetiracetam in rodent models of seizures and epilepsy. Eur. J. Pharmacol. 353 (2-3), 191–206. doi:10.1016/s0014-2999(98)00410-5
Kroll, J. R., Saras, A., and Tanouye, M. A. (2015). Drosophila sodium channel mutations: contributions to seizure-susceptibility. Exp. Neurol. 274 (Pt A), 80–87. doi:10.1016/j.expneurol.2015.06.018
Kuebler, D., and Tanouye, M. A. (2000). Modifications of seizure susceptibility in Drosophila. J. Neurophysiol. 83 (2), 998–1009. doi:10.1152/jn.2000.83.2.998
Kwan, P., and Brodie, M. J. (2000). Early identification of refractory epilepsy. N. Engl. J. Med. 342 (5), 314–319. doi:10.1056/NEJM200002033420503
Larsen, J., Gasser, K., and Hahin, R. (1996). An analysis of dimethylsulfoxide-induced action potential block: a comparative study of DMSO and other aliphatic water soluble solutes. Toxicol. Appl. Pharmacol. 140 (2), 296–314. doi:10.1006/taap.1996.0225
Link, N., and Bellen, H. J. (2020). Using Drosophila to drive the diagnosis and understand the mechanisms of rare human diseases. Development 147 (21), dev191411. doi:10.1242/dev.191411
Loscher, W. (2011). Critical review of current animal models of seizures and epilepsy used in the discovery and development of new antiepileptic drugs. Seizure 20 (5), 359–368. doi:10.1016/j.seizure.2011.01.003
Loscher, W. (2017). Animal models of seizures and epilepsy: past, present, and future role for the discovery of antiseizure drugs. Neurochem. Res. 42 (7), 1873–1888. doi:10.1007/s11064-017-2222-z
Loscher, W., and Klein, P. (2021). The pharmacology and clinical efficacy of antiseizure medications: from bromide salts to cenobamate and beyond. CNS Drugs 35 (9), 935–963. doi:10.1007/s40263-021-00827-8
Loscher, W., Potschka, H., Sisodiya, S. M., and Vezzani, A. (2020). Drug resistance in epilepsy: clinical impact, potential mechanisms, and new innovative treatment options. Pharmacol. Rev. 72 (3), 606–638. doi:10.1124/pr.120.019539
Lu, C., and Mattson, M. P. (2001). Dimethyl sulfoxide suppresses NMDA- and AMPA-induced ion currents and calcium influx and protects against excitotoxic death in hippocampal neurons. Exp. Neurol. 170 (1), 180–185. doi:10.1006/exnr.2001.7686
Miguel Sanz, C., Martinez Navarro, M., Caballero Diaz, D., Sanchez-Elexpuru, G., and Di Donato, V. (2023). Toward the use of novel alternative methods in epilepsy modeling and drug discovery. Front. Neurol. 14, 1213969. doi:10.3389/fneur.2023.1213969
Mituzaite, J., Petersen, R., Claridge-Chang, A., and Baines, R. A. (2021). Characterization of seizure induction methods in Drosophila. eNeuro 8 (4), ENEURO.0079–21.2021. doi:10.1523/ENEURO.0079-21.2021
Nakahiro, M., Arakawa, O., Narahashi, T., Ukai, S., Kato, Y., Nishinuma, K., et al. (1992). Dimethyl sulfoxide (DMSO) blocks GABA-induced current in rat dorsal root ganglion neurons. Neurosci. Lett. 138 (1), 5–8. doi:10.1016/0304-3940(92)90459-k
Pandey, U. B., and Nichols, C. D. (2011). Human disease models in Drosophila melanogaster and the role of the fly in therapeutic drug discovery. Pharmacol. Rev. 63 (2), 411–436. doi:10.1124/pr.110.003293
Parker, L., Howlett, I. C., Rusan, Z. M., and Tanouye, M. A. (2011a). Seizure and epilepsy: studies of seizure disorders in Drosophila. Int. Rev. Neurobiol. 99, 1–21. doi:10.1016/B978-0-12-387003-2.00001-X
Parker, L., Padilla, M., Du, Y., Dong, K., and Tanouye, M. A. (2011b). Drosophila as a model for epilepsy: bss is a gain-of-function mutation in the para sodium channel gene that leads to seizures. Genetics 187 (2), 523–534. doi:10.1534/genetics.110.123299
Pavlidis, P., Ramaswami, M., and Tanouye, M. A. (1994). The Drosophila easily shocked gene: a mutation in a phospholipid synthetic pathway causes seizure, neuronal failure, and paralysis. Cell 79 (1), 23–33. doi:10.1016/0092-8674(94)90397-2
Polet, S. S., de Koning, T. J., Lambrechts, R. A., Tijssen, M. A. J., Sibon, O. C. M., and Gorter, J. A. (2024). Conventional and novel anti-seizure medications reveal a particular role for GABA(A) in a North Sea progressive myoclonus Epilepsy Drosophila model. Epilepsy Res. 203, 107380. doi:10.1016/j.eplepsyres.2024.107380
Reiter, L. T., Potocki, L., Chien, S., Gribskov, M., and Bier, E. (2001). A systematic analysis of human disease-associated gene sequences in Drosophila melanogaster. Genome Res. 11 (6), 1114–1125. doi:10.1101/gr.169101
Roberti, R., De Caro, C., Iannone, L. F., Zaccara, G., Lattanzi, S., and Russo, E. (2021). Pharmacology of cenobamate: mechanism of action, pharmacokinetics, drug-drug interactions and tolerability. CNS Drugs 35 (6), 609–618. doi:10.1007/s40263-021-00819-8
Rogawski, M. A., Tofighy, A., White, H. S., Matagne, A., and Wolff, C. (2015). Current understanding of the mechanism of action of the antiepileptic drug lacosamide. Epilepsy Res. 110, 189–205. doi:10.1016/j.eplepsyres.2014.11.021
Rosch, R., Burrows, D. R. W., Jones, L. B., Peters, C. H., Ruben, P., and Samarut, E. (2019). Functional genomics of epilepsy and associated neurodevelopmental disorders using simple animal models: from genes, molecules to brain networks. Front. Cell Neurosci. 13, 556. doi:10.3389/fncel.2019.00556
Schutte, R. J., Schutte, S. S., Algara, J., Barragan, E. V., Gilligan, J., Staber, C., et al. (2014). Knock-in model of Dravet syndrome reveals a constitutive and conditional reduction in sodium current. J. Neurophysiol. 112 (4), 903–912. doi:10.1152/jn.00135.2014
Shah, N., Kasture, A. S., Fischer, F. P., Sitte, H. H., Hummel, T., and Sucic, S. (2024). A transporter’s doom or destiny: SLC6A1 in health and disease, novel molecular targets and emerging therapeutic prospects. Front. Mol. Neurosci. 17, 1466694. doi:10.3389/fnmol.2024.1466694
Song, J., and Tanouye, M. A. (2008). From bench to drug: human seizure modeling using Drosophila. Prog. Neurobiol. 84 (2), 182–191. doi:10.1016/j.pneurobio.2007.10.006
Takai, A., Yamaguchi, M., Yoshida, H., and Chiyonobu, T. (2020). Investigating developmental and epileptic encephalopathy using Drosophila melanogaster. Int. J. Mol. Sci. 21 (17), 6442. doi:10.3390/ijms21176442
Widmann, M., Lieb, A., Mutti, A., and Schwarzer, C. (2023). Dimethyl sulfoxide's impact on epileptiform activity in a mouse model of chronic temporal lobe epilepsy. Epilepsy Res. 197, 107235. doi:10.1016/j.eplepsyres.2023.107235
Wolking, S., Campbell, C., Stapleton, C., McCormack, M., Delanty, N., Depondt, C., et al. (2021). Role of common genetic variants for drug-resistance to specific anti-seizure medications. Front. Pharmacol. 12, 688386. doi:10.3389/fphar.2021.688386
Wolking, S., Moreau, C., Nies, A. T., Schaeffeler, E., McCormack, M., Auce, P., et al. (2020a). Testing association of rare genetic variants with resistance to three common antiseizure medications. Epilepsia 61 (4), 657–666. doi:10.1111/epi.16467
Wolking, S., Schulz, H., Nies, A. T., McCormack, M., Schaeffeler, E., Auce, P., et al. (2020b). Pharmacoresponse in genetic generalized epilepsy: a genome-wide association study. Pharmacogenomics 21 (5), 325–335. doi:10.2217/pgs-2019-0179
Keywords: animal model, antiseizure medications, Drosophila melanogaster, drug screening, epilepsy, seizure model
Citation: Fischer FP, Karge RA, Koch H, Voigt A, Weber YG and Wolking S (2024) The fruit fly Drosophila melanogaster as a screening model for antiseizure medications. Front. Pharmacol. 15:1489888. doi: 10.3389/fphar.2024.1489888
Received: 02 September 2024; Accepted: 18 November 2024;
Published: 10 December 2024.
Edited by:
Michael J. Marino, Research Laboratories Merck, United StatesReviewed by:
Scott Barbee, University of Denver, United StatesCopyright © 2024 Fischer, Karge, Koch, Voigt, Weber and Wolking. This is an open-access article distributed under the terms of the Creative Commons Attribution License (CC BY). The use, distribution or reproduction in other forums is permitted, provided the original author(s) and the copyright owner(s) are credited and that the original publication in this journal is cited, in accordance with accepted academic practice. No use, distribution or reproduction is permitted which does not comply with these terms.
*Correspondence: Florian P. Fischer, ZmxmaXNjaGVyQHVrYWFjaGVuLmRl
Disclaimer: All claims expressed in this article are solely those of the authors and do not necessarily represent those of their affiliated organizations, or those of the publisher, the editors and the reviewers. Any product that may be evaluated in this article or claim that may be made by its manufacturer is not guaranteed or endorsed by the publisher.
Research integrity at Frontiers
Learn more about the work of our research integrity team to safeguard the quality of each article we publish.