- 1Pharmaceutical Sciences Research Center, Department of Pharmacy, Children’s Hospital of Nanjing Medical University, Nanjing, China
- 2Department of Children Healthcare, Children’s Hospital of Nanjing Medical University, Nanjing, China
Non-stimulant atomoxetine is recognized in various current clinical guidelines as an important alternative to stimulants for the pharmacological treatment of attention deficit/hyperactivity disorder (ADHD) in children. While its efficacy and tolerability for core symptoms are established, there is considerable inter-individual variability in response and exposure, highlighting the need for personalized dosing. In this review, we evaluated existing studies and summarized comprehensive evidence supporting the clinical implementation of therapeutic drug monitoring (TDM) and personalized dosing of atomoxetine, organized around a series of logically structured questions. Although there are notable gaps in achieving personalized dosing across multiple critical elements, the available evidence is helpful to endorse personalized dose adjustments based on TDM and CYP2D6 genotyping “whenever possible.” We advocate for ongoing improvement and enhancement in clinical practice. Future advancements will rely on a deeper understanding of ADHD, facilitating more precise diagnoses and personalized treatment strategies.
1 Introduction
Attention deficit-hyperactivity disorder (ADHD) is identified by symptoms of hyperactivity and impulsivity, inattention, or a combination of these, which exceed the expected development level and interfere with daily functioning (Posner et al., 2020; Fu et al., 2021). It is one of the most frequently diagnosed neurodevelopmental disorder in children, with up to 70% of cases showing symptoms that persist into adulthood. Approximately 5% of children and adolescents, as well as 2.5% of adults worldwide, are affected by ADHD, and the overall prevalence of the disorder has remained consistent over the past 3 decades (Posner et al., 2020; Faraone et al., 2015; Faraone et al., 2024). However, there has been a significant rise in new ADHD diagnoses and reported prevalence during the COVID-19 pandemic, particularly in countries like Finland (Auro et al., 2024) and the United States (Danielson et al., 2024; QuickStats, 2024), alongside a global increase in ADHD symptoms (Rogers and MacLean, 2023). This trend suggests that more individuals may now be eligible for treatment with atomoxetine in the aftermath of pandemic.
Treatment for individuals with ADHD may include pharmacological, non-pharmacological, or a combination of both approaches (Table 1). The available medications consist of stimulants, such as methylphenidate and amphetamines, as well as non-stimulants, including atomoxetine, extended-release clonidine, and guanfacine (Cortese, 2020). Currently, the process of selecting the most suitable medication for each patient is largely based on a trial-and-error, as our understanding of the neurobiology underlying ADHD is still inadequate to guide medication choices (Cortese, 2020).
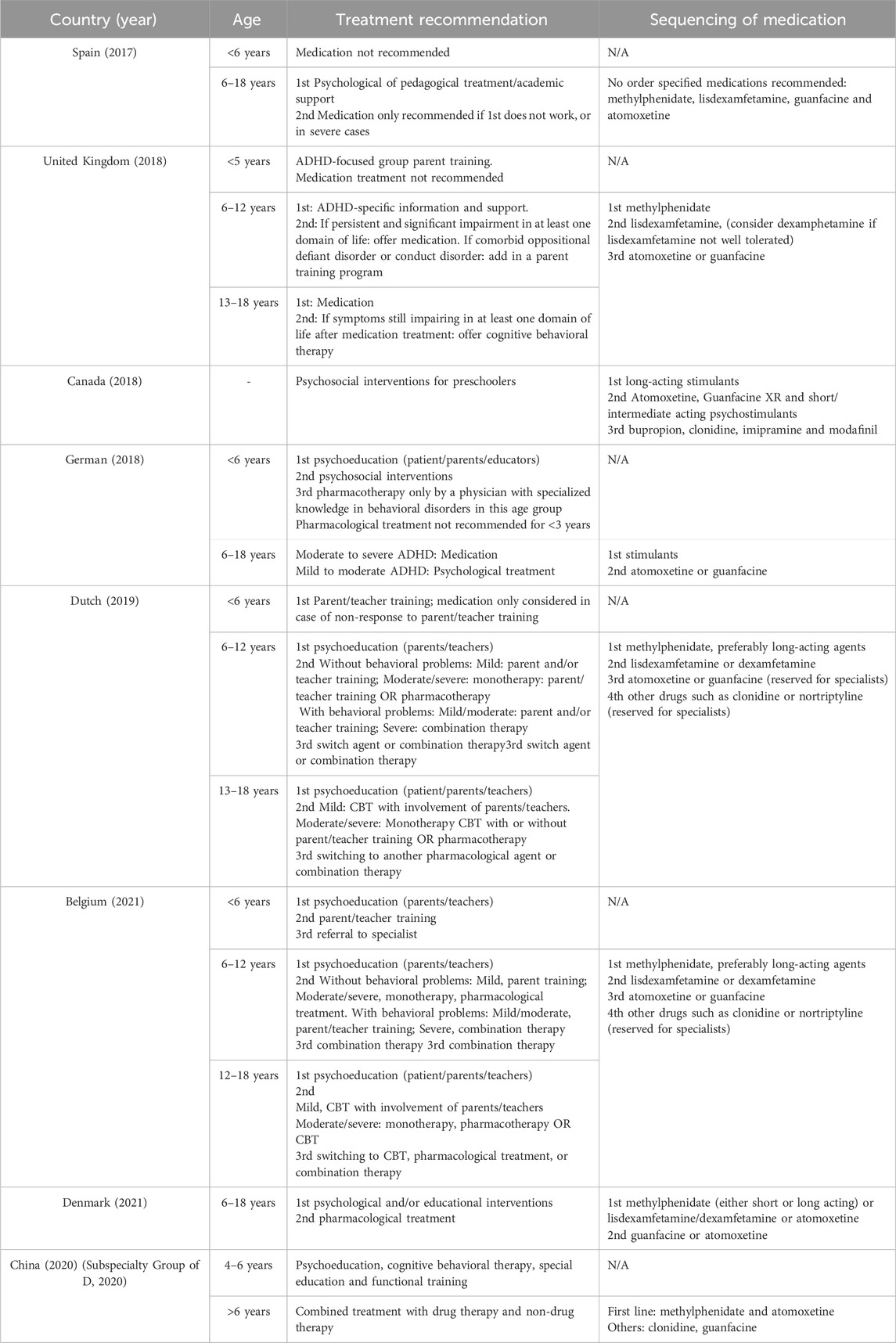
Table 1. Treatment ladders and sequencing of medications (Coghill et al., 2023; Van Vyve et al., 2024).
In clinical settings, the American Academy of Pediatrics recommends stimulants, atomoxetine, extended-release guanfacine, and extended-release clonidine for children aged 6–11 years, listed in order of the strength of evidence. Similarly, the National Institute for Health and Care Excellence (NICE) advises starting medication for children aged 5 and older and young people with methylphenidate, lisdexamfetamine (or dexamphetamine if lisdexamfetamine causes unacceptable side effects), atomoxetine or guanfacine, ranked by preference. According to the ADHD German Guidelines, second-line pharmacotherapy for children aged 6 and older and young people with mild-to-moderate ADHD should include stimulants, atomoxetine or guanfacine, also in descending order of preference, following psychoeducation. For cases of moderate-to-severe ADHD, however, stimulants, atomoxetine or guanfacine should be prioritized as the first-line medication after psychoeducation (Cortese, 2020; Coghill et al., 2023). Despite these guidelines, a lack of high-quality, long-term evidence is evident in clinical guidance (Kazda et al., 2024). A one-dose-fits-all medication approach may not be appropriate for individuals with ADHD, and clinicians often face the significant challenge of tailoring pharmacological formulations and doses to align with each patient’s biological characteristics and social needs.
Approved by the US Food and Drug Administration (FDA) in 2002 as the first non-stimulant medication for the treatment of ADHD in children over 6 years and adults, atomoxetine functions as a selective inhibitor of the presynaptic noradrenaline transporter, thereby extending the activity of noradrenaline in the synaptic cleft (Figure 1) (Garland and Kirkpatrick, 2004). According to European and North American ADHD guidelines, atomoxetine is typically used as a second or third-line treatment (Coghill et al., 2023). However, in countries like China and Japan, it is regarded as a first-line option, equivalent to stimulants (Fu et al., 2023). It is also important to recognize that some patients discontinue treatment prematurely due to inadequate titration, lack of clinical monitoring, or insufficient ongoing evaluations (Vertessen et al., 2024). Consequently, regardless of its classification as a first, second, or third-line medication, if we consider the choice of atomoxetine for ADHD as an integrative, evidence-based decision for specific patients, the critical question becomes how to personalize the dosage regimen, given the individual differences in treatment response.
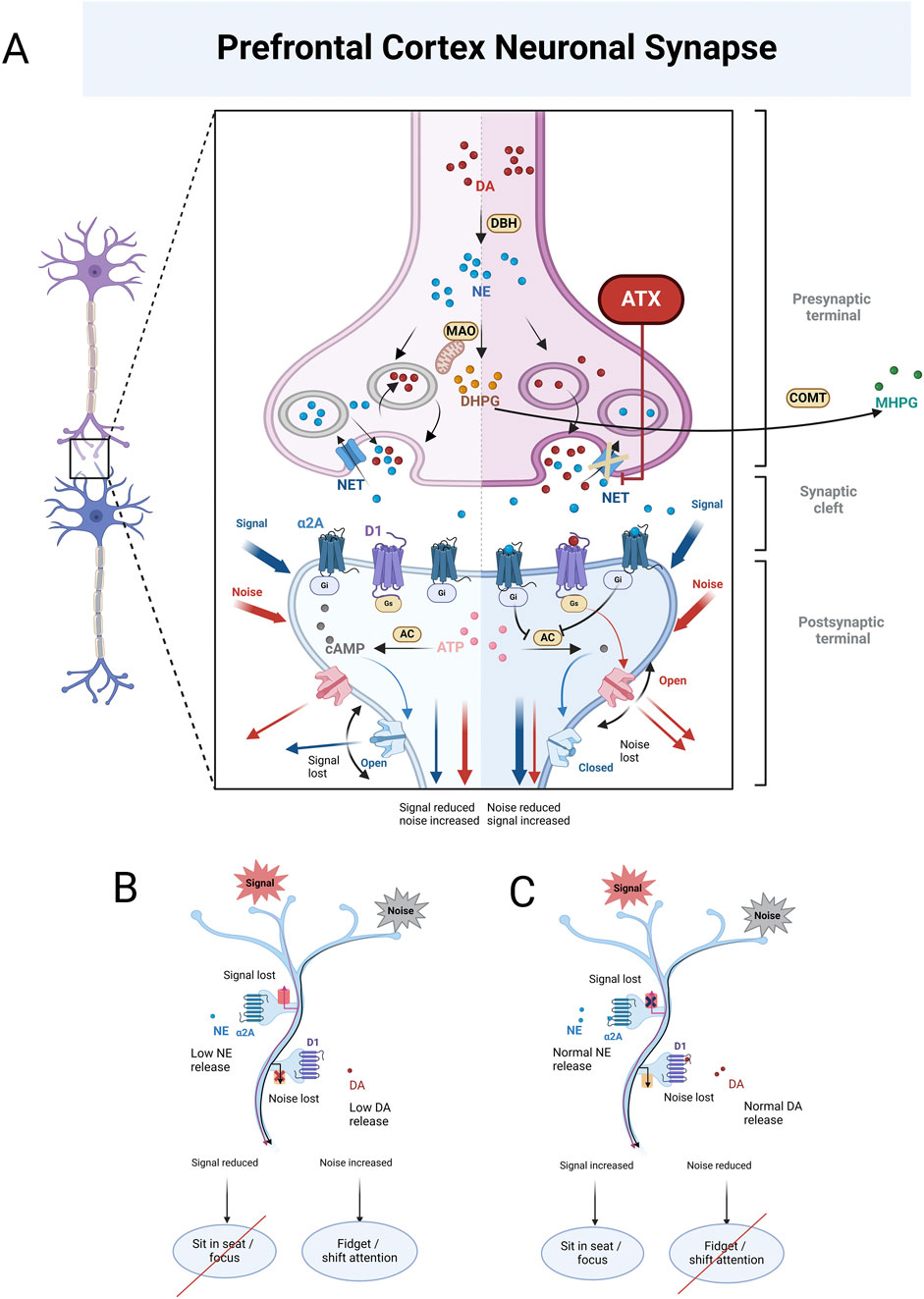
Figure 1. (A) illustrates the enzymatic conversion of dopamine (DA) into norepinephrine (NE) mediated by dopamine β-Hydroxylase (DBH). Once formed, NE is metabolized on the mitochondrial membrane by monoamine oxidase (MAO), generating 3,4-dihydroxy phenylethylene glycol (DHPG). DHPG is then further metabolized extracellularly by catechol-O-methyltransferase (COMT), resulting in the production of 3-methoxy-4-hydroxyphenylglycol (MHPG). At low to moderate concentrations, NE binds to α2A receptors, which activates G proteins associated with these receptors. These activated G proteins inhibit adenylyl cyclase (AC), the enzyme responsible for converting adenosine triphosphate (ATP) into cyclic adenosine monophosphate (cAMP). This reduction in cAMP levels leads to the closure of hyperpolarization-activated cyclic nucleotide-gated (HCN) channels, which are responsible for signal leakage, thereby enhancing the surviving signal. In contrast, at low to moderate DA concentrations, DA binds to D1 receptors, reducing interference noise in the brain. The left panel of (A) illustrates the state of neurons in the prefrontal cortex (PFC) of individuals with ADHD. In this context, both NE and DA levels in the synaptic cleft are low, impairing their ability to perform their respective functions. This deficiency leads to reduced signals and increased noise within the brain. Conversely, the right panel of (A) depicts individuals with ADHD after receiving atomoxetine (ATX) treatment. ATX selectively inhibits NET, preventing the reuptake of NE from the synaptic cleft back into the presynaptic terminal. This inhibition results in higher synaptic concentrations of NE and, subsequently, lower intra-neuronal NE levels. The decrease in intra-neuronal DHPG levels contributes to an increase in NE concentrations in the synaptic cleft. Importantly, the PFC has low levels of the dopamine transporter (DAT), responsible for DA reuptake. As a result, DA in this brain region is predominantly inactivated by NET inhibition, leading to elevated levels of both DA and NE in the PFC. This enables NE to effectively bind to α2A receptors, and DA to D1 receptors, allowing them to fulfill their functions as previously described. (B) illustrates the PFC condition in individuals with ADHD, showing that lower levels of NE and DA result in the ineffective receptor binding, which ultimately impairs their functions. (C) illustrates the PFC condition in individuals with ADHD when NE and DA levels are normal. In this scenario, NE binds to α2A receptors, and DA binds to D1 receptors, enabling NE to effectively enhance signaling while DA successfully reduces noise.
Despite the existence of evidence-based guidelines, a notable gap remains between these guidelines and their practical application in clinical settings, leading to uncertainty regarding the optimal utilization of the ADHD medication (Table 2). While less common, there are guidelines that offer specific recommendations for tailoring dosage regimen of atomoxetine (Hiemke et al., 2018; Brown et al., 2019). These guidelines suggest reference ranges for blood drug concentrations aligned with the timing of blood sampling and dosing schedules, which can help enhance clinical efficacy and manage adverse reactions. However, the low level of supporting evidence (Hiemke et al., 2018) and broad reference ranges present further challenges to implementing these guidelines. Thankfully, these recommendations have attracted considerable attention and have prompted significant advancements in this field.
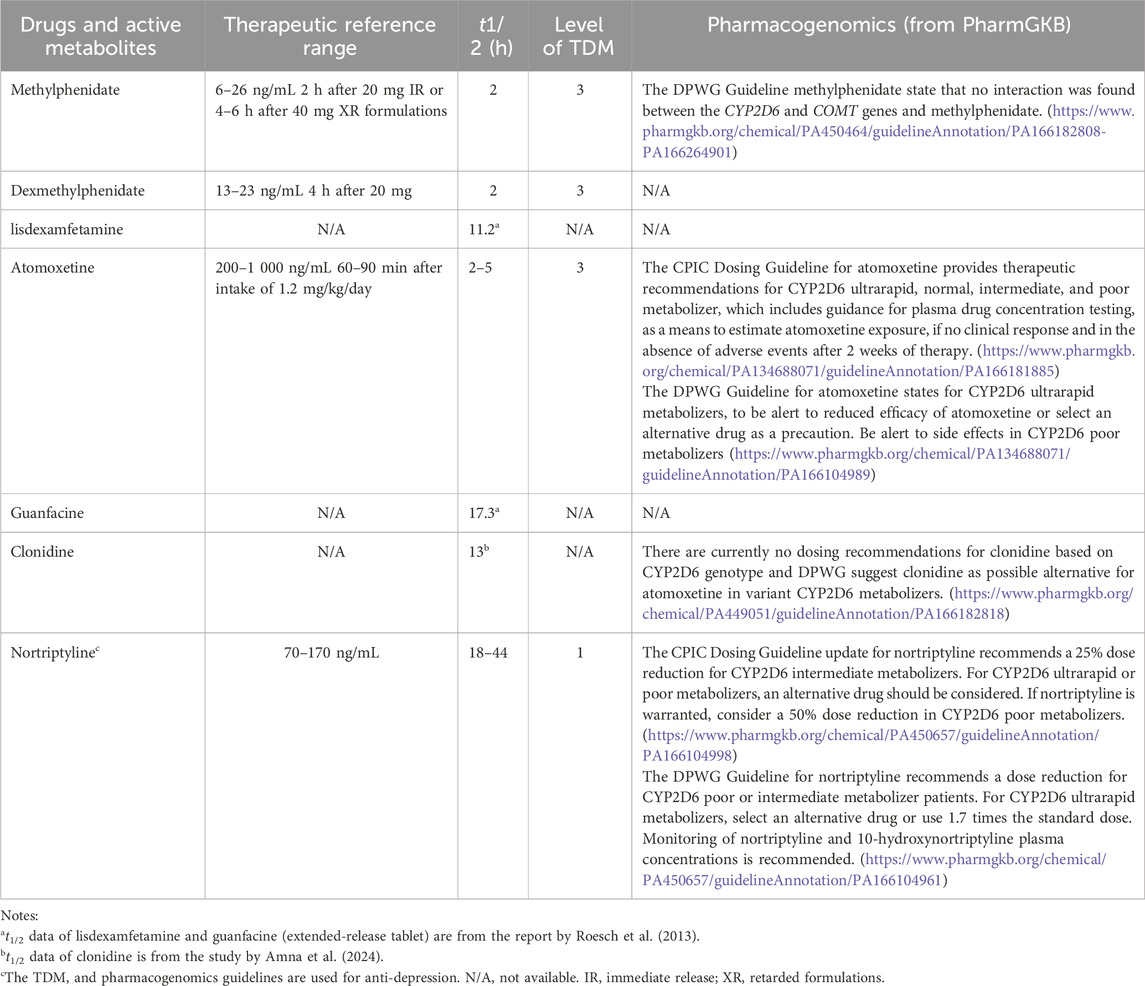
Table 2. Recommended therapeutic reference ranges, elimination half-life (t1/2) ranges, levels of recommendation to use TDM from AGNP and pharmacogenomics guidelines from PharmGKB.
In this review, we focus on the personalized dosing of atomoxetine. We will apply the framework established by Beumer et al. (2019) to systematically evaluate the available published studies, compiling extensive evidence for the clinical implementation of therapeutic drug monitoring (TDM) and personalized dosing of atomoxetine through a series of logically structured questions.
2 Body weight (BW)-based dosing strategy: one dose fits all?
Currently, the dosing of atomoxetine is primarily based on the BWs of children (Farhat et al., 2022). For those weighing up to 70 kg, the recommended initial total daily dose is approximately 0.5 mg/kg, which can be increased after a minimum of 3 days to reach a target dose of approximately 1.2 mg/kg. This can be administered as a single daily dose in the morning (q.m.) or divided into doses taken in the morning and late afternoon/early evening doses (b.i.d.) (Farhat et al., 2022). It's important to highlight that doses exceeding 1.2 mg/kg/day have not shown additional benefit (Brown et al., 2016). Indeed, for children and adolescents, the maximum total daily dose should not exceed 1.4 mg/kg or 100 mg, whichever is less.
Additionally, for those taking strong CYP2D6 inhibitors (such as paroxetine, fluoxetine, and quinidine) (Ring et al., 2002), or identified as CYP2D6 poor metabolizers (PMs), atomoxetine should also be started at 0.5 mg/kg/day. The dose may be increased to the typical target of 1.2 mg/kg/day only if there is no improvement after 4 weeks and the initial dose is well tolerated.
For children and adolescents weighing over 70 kg, the recommended initial total daily dose of atomoxetine is 40 mg, consistent with adult dosing guidelines. This dosage may be increased after a minimum of 3 days to reach a target total daily dose of about 80 mg. The medication can be administered either as a single daily dose in the morning or divided into doses taken in the morning and late afternoon/early evening. Following an additional 2–4 weeks, the dose may be further raised to a maximum of 100 mg for those who have not achieved an optimal response. It's worth noting that there is no evidence indicating that higher doses provide increased effectiveness.
In cases where strong CYP2D6 inhibitors are administered for children and adolescents over 70 kg, atomoxetine should also be started at 40 mg/day. The dosage can then be increased to the typical target dose of 80 mg/day only if symptoms do not improve after 4 weeks and the initial dose is well tolerated.
For ADHD patients with hepatic insufficiency (HI), dosage adjustments are necessary (Chalon et al., 2003). For those classified with moderate HI (Child-Pugh Class B), both the initial and target doses should be reduced to 50% of the standard dose. In cases of severe HI (Child-Pugh Class C), both the initial dose and target doses should be cut to 25% of the normal dosage.
However, administering the recommended starting dose of 0.5 mg/kg to children results in a 30-fold range in exposure, as indicated by dose-corrected AUC0-∞19, without considering the CYP2D6 genotype or predicted phenotype. Simulated steady-state exposure profiles at the maximum recommended dose suggest that most children are unlikely to achieve adequate levels of atomoxetine exposure (Brown et al., 2016).
Given that a relatively small percentage of the population are CYP2D6 PMs [e.g., around 7% in Caucasians (de Leon, 2015)], some experts argue that the currently approved clinical dosing may serve as a compromise for the majority of non-PMs. This is because the dosages are slightly lower to accommodate the potential tolerability or adverse reactions in PMs. However, this can lead to insufficient exposure among non-PMs, potentially affecting drug efficacy (Brown et al., 2016). Clearly, simply dose tailoring based solely on BW does not fulfill the need for personalized medication.
In our clinical practice, recent plasma atomoxetine monitoring revealed some intriguing trends: some children achieved higher levels of exposure at very low doses, while others had high doses but low systemic exposure. Meanwhile, some pediatric patients tolerated atomoxetine poorly with low exposure, while others managed well at high drug concentrations. In another scenario, some children on low doses experienced low exposures and tolerated the drug well, but demonstrated poor clinical efficacy. It is puzzling that the decision was made to not tailor the dosing, but instead to choose alternative medications (Fu et al., 2023).
3 Framework for assessing evidence backing personalized dosing of atomoxetine
Personalized prescribing for atomoxetine, like that for other medications, involves tailoring doses based on pharmacokinetic and pharmacodynamic mechanism that impact its safety and effectiveness. We utilize the Arbeitsgemeinschaft für Neuropsychopharmakologie und Pharmakopsychiatrie (AGNP) consensus guidelines to create a framework for evaluating the evidence supporting TDM of atomoxetine (Hiemke et al., 2018). This guideline offers recommendations regarding the timing of blood sampling after administration of atomoxetine, reference concentration ranges, and laboratory alert values; however, the evidence level provided is classified as Grade 3. In 2019, the Clinical Pharmacogenetic Implementation Consortium (CPIC) guidelines (Brown et al., 2019) estimated the activity score (AS) of CYP2D6 based on genotyping results, subsequently defining the phenotype of patients with these alleles. The guidelines made recommendations for the timing of blood sampling and the expected drug concentration range after atomoxetine use, with corresponding dose adjustments if the target concentration was not achieved.
We are particularly interested in exploring how these guidelines have contributed to advancing the practical implementation of personalized medication with atomoxetine and what new advancements have emerged in this field (Fu et al., 2023). To better organize information from previous reports, we modified the questions originally posed by Beumer et al. (2019) to focus on the clinical pharmacology of the medication, emphasizing key aspects relevant to TDM evaluation and personalized dosing.
4 Pharmacokinetics
4.1 Is there significant inter-individual variability in plasma concentrations using the current BW-based dosing regimen?
As shown in Table 3, the inter-individual variability in the plasma clearance of atomoxetine, corrected by oral bioavailability (CL/F), ranges between 14%–62% CV in children. This variability is closely associated with the CYP2D6 phenotype. Significant differences in plasma atomoxetine concentrations among children exist, depending on the route of administration (Guo et al., 2024). Most recently, Guo et al. (2024) identified sex, BW, and CYP2D6 phenotype were the primary factors influencing individual exposure to atomoxetine, with the phenotype exerting the most significant impact. Although the impact of CYP2D6 phenotype on pharmacokinetics of atomoxetine in children has been observed, studies in this area remains quite limited.
4.2 Is there limited intra-individual variability in plasma concentrations?
Currently, reports on the intra-individual differences in pharmacokinetic parameters, such as plasma atomoxetine concentrations and total CL, are very limited. Recently, Cheng et al. (2023) utilized a population pharmacokinetic (PPK) modeling approach to estimate the residual unexplained variability (i.e., intra-individual variability) in plasma concentrations of atomoxetine and its major metabolite, 4-OH-atomoxetine, in children and adolescents, yielding 21.3% and 29.6% CV, respectively. In addition, early studies have indicated that, food intake reduces its peak concentration and delays the time to peak concentration, although it does not affect the absorption of atomoxetine (Sauer et al., 2005). This factor should also be considered as contributing to both intra-individual and inter-individual variability.
4.3 Do drug-drug interactions (DDIs) impact the pharmacokinetic parameters of atomoxetine?
When ADHD occurs alongside other conditions such as anxiety or depression, there may be a need for using atomoxetine in combination with other medications; however, this is more common in adults (Todor et al., 2017; Todor et al., 2016; Belle et al., 2002; Sauer et al., 2004; Kratochvil et al., 2005). Research has evaluated the impact of bupropion, fluvoxamine, paroxetine, desipramine, and fluoxetine on the pharmacokinetics of atomoxetine, as a victim drug (Table 3). Studies involving fluvoxamine (Todor et al., 2017) and desipramine (Sauer et al., 2004) indicated that interactions, if present, were slight; however, fluoxetine increased atomoxetine peak concentration by 3.4 fold (Kratochvil et al., 2005). Of note, bupropion exhibited significant inhibitory effects on atomoxetine’s metabolism in patients classified as CYP2D6 EMs, while the effects were minimal in CYP2D6 PMs (Todor et al., 2016). Interestingly, a Canadian guideline classifies bupropion as a third-line treatment for ADHD (Schoretsanitis et al., 2019). In addition, a case report has noted an improved response to atomoxetine in a patient, likely classified as a CYP2D6 EM, following the addition of paroxetine (Paulzen et al., 2016), indicating that these inhibitors can be utilized to enhance the response to atomoxetine in individuals identified as CYP2D6 EMs.
Conversely, a recent study involving children and adolescent with ADHD revealed that the use of concomitant medications is quite rare, particularly regarding herbal medicines (Guo et al., 2024). Nevertheless, instances in adults where the concurrent use of medications as potential inhibitors of CYP2D6 (Table 4) significantly alters the systemic exposure to atomoxetine warrant careful consideration by pediatricians. If similar combinations of medications become necessary for children and adolescents, it may be important to adjust doses to account for changes in exposure due to DDIs (Sauer et al., 2004) in order to ensure efficacy and minimize adverse reactions (Fu et al., 2023).
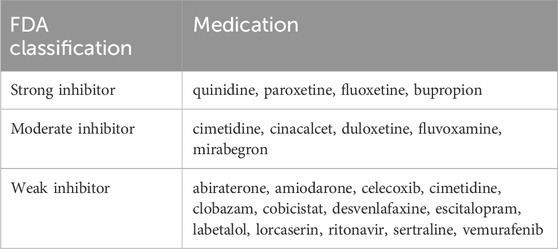
Table 4. FDA examples of clinical inhibitors for CYP2D6 (Cicali et al., 2020).
It is crucial to highlight that whether CYP2D6 inhibitors have a practical effect is closely linked to the patient’s CYP2D6 metabolic phenotype. They do not work in CYP2D6 PMs but can enhance systemic exposure to atomoxetine in CYP2D6 non-PMs. Therefore, gathering genotype and phenotype information about the patient’s CYP2D6 status becomes necessary.
5 Pharmacodynamics (PD)
5.1 Is there a narrow therapeutic window?
As of now, the therapeutic window for atomoxetine in treating ADHD in children has not been clearly established. The recommended concentration range of 200–1,000 ng/mL, as outlined in guidelines (Hiemke et al., 2018; Brown et al., 2019), primarily focuses on identifying the lowest concentration necessary to achieve efficacy. However, the link between plasma levels of atomoxetine and its clinical effectiveness remains unclear, complicating the relationship between tolerability and concentration even further (Guo et al., 2024). Consequently, it is more plausible that we will first define a minimum concentration required for therapeutic effect, while finding a corresponding concentration that ensures tolerability is challenging, as tolerability does not always have a straightforward relationship with drug concentration.
5.2 Are there easy and clinically relevant biomarkers to predict response and/or toxicity at a given dose?
5.2.1 CYP2D6
Atomoxetine is primarily cleared from the body through oxidative metabolism, with the majority of its oxidative metabolites being excreted in the urine. This metabolic process is predominantly facilitated by CYP2D6, making the polymorphism of CYP2D6 significantly relevant to the pharmacokinetics of atomoxetine (Sauer et al., 2005). CYP2D6 affects both the efficacy and tolerability of atomoxetine by influencing its pharmacokinetic processes in the body (Guo et al., 2024), positioning it as a crucial biomarker to monitor and investigate at this time (Nijenhuis et al., 2023; Brown, 2022; Michelson et al., 2007).
To date, over 160 star alleles of CYP2D6 have been cataloged in the PharmGKB and CPIC databases. Each star allele can exhibit varying levels of activity, which may clinically manifest as normal function, increased function, decreased function, or no function at all. It is important to highlight that the functionality of over half of the alleles remains uncertain or unknown, leading to ambiguity or complexity regarding the ability of individuals with these alleles to metabolize atomoxetine (Table 5).
While there are over 14,700 possible combinations of CYP2D6 diplotype (CYP2D6 Diplotype-Phenotype Table, https://www.pharmgkb.org/page/cyp2d6RefMaterials; last accessed, 2024/4/4), they can be generally categorized into the following phenotypes based on AS: ultrarapid metabolizer (UM), normal metabolizer (NM; formerly extensive metabolizers, EM (Nofziger et al., 2020)), intermediate metabolizer (IM), and PM. The prevalence of these phenotypes varied significantly across biogeographical groups, with the majority of populations classified as NM and IM, whereas UM and PM phenotypes are less frequently observed (Table 6).
In Oceania, the frequency of UMs is nearly 20%, indicating that patients with enhanced function alleles may experience very low systemic exposure levels from the same dose of atomoxetine, which could lead to poor efficacy. Conversely, the frequency of UMs in East Asia is below 1%, making the likelihood of this scenario one-twentieth that of Oceania. Furthermore, PMs have the lowest distribution frequencies in both Oceania and East Asia, at 0.31% and 0.79% respectively. This suggests that the risk of excessive atomoxetine exposure due to non-functional metabolizing enzymes is relatively low, implying a potentially reduced likelihood of poor tolerability in these populations compared to others (Table 6).
For example, the oral clearance in CYP2D6 PMs was only 6.0% of that observed in the EM2 group, potentially resulting in higher exposure to atomoxetine. In PMs, the half-life (t1/2) was 2.9 times longer than t in the IMs, and 5.4 to 5.9 times longer than in both EM1 and EM2 groups, with the AUC 0-∞ showing a variability of 29.6 times across the study cohort (Brown et al., 2016). Similarly, at comparable doses for children and adolescents with ADHD, the mean peak atomoxetine concentrations in CYP2D6 PMs were approximately 5 times higher than those in EMs (Michelson et al., 2007).
In a study examining the correlation between pharmacogenetics and treatment response, 589 participants—30 CYP2D6 PMs and 559 CYP2D6 EMs—completed a treatment period lasting 6–8 weeks, during which their responses were evaluated. The average improvements, assessed using the ADHDRS IV Parent Interview, were 14.1 points for EMs and 20.9 points for PMs. The response rates, defined as a 25% decrease from baseline in ADHDRS-IV-Parent: Inv total score at study endpoint, were 59.4% for EMs and 80% for PMs, respectively (Michelson et al., 2007).
In a group of 100 children, Ter Laak et al. (2010) identified 10 candidates for CYP2D6 genotyping due to delayed response or poor tolerability. Among these, 8 children were found to be CYP2D6 PMs; 4 experienced improved therapeutic effects after dose reduction, while the remaining 4 discontinued treatment due to initial adverse reactions. As a result, the authors suggested that pre-emptive genotyping for CYP2D6 could enhance the efficacy of atomoxetine and help manage its adverse effects. Additionally, cases with rs1135840 (Chatterjee et al., 2023) (4180 G>C, decreased function) “CC” showed improvement after atomoxetine treatment. However, some other studies indicated that routine genotyping might not be necessary, as researchers managed to dose atomoxetine effectively, achieving similar efficacy and safety levels in both EMs (n = 1,239) and PMs (n = 87) without prior knowledge of their metabolizer status (Trzepacz et al., 2008). Nevertheless, the clinical characteristics of PMs prompted healthcare providers to consider reducing dosages for these individuals, even without information about their metabolic status.
Selecting the appropriate clinical CYP2D6 genotyping alleles is crucial for standardizing gene testing across clinical labs. Recently, several organizations, including the Association for Molecular Pathology, College of American Pathologists, Dutch Pharmacogenetics Working Group of the Royal Dutch Pharmacists Association, and the European Society for Pharmacogenomics and Personalized Therapy, released a Joint consensus recommendation regarding the selection of these alleles (Pratt et al., 2021). This guidance outlines a foundational set of variant alleles (Tier 1) and an expanded set (Tier 2) to aid clinical labs in developing CYP2D6 testing assays. Briefly, the Tier 1 recommended CYP2D6 variant star alleles include *2 through *6, *9, *10, *17, *29, and *41, along with the determination of gene duplication or multiplication status. The Tier 2 recommended CYP2D6 variant alleles consist of *7, *8, *12, *14, *15, *21, *31, *40, *42, *49, *56, and *59, as well as hybrid genes that contain segments of both CYP2D6 and CYP2D7.
The next consideration is how to achieve rapid and cost-effective genotyping. In clinical labs, various methods (Table 7) for detecting CYP2D6 haplotypes are already being used, including techniques capable of identifying hybrid arrangements and quantify copy number variants (CNVs). Long-range polymerase chain reaction (PCR) or extra-long-range PCR methods are designed to amplify the entire CYP2D6 gene, allowing for the detection of multiple copies or whole-gene deletions. While these methods are robust and reliable, they can be time-consuming and may not be suitable for the rapid screening of a wide range of alleles (Taylor et al., 2020). In addition, long range-PCR followed by Sanger sequence is considered the gold-standard for definitive CYP2D6 genotype determination when CNVs are present; however, this approach is labor-intensive and involves complex procedures (Atiq et al., 2023).
Pyrosequencing is an cost-effective high-throughput sequencing method compared to traditional Sanger sequencing, although it presents challenges in interpretation and requires additional instrumentation and workflows to for implementation (Siqueira et al., 2012). The Taqman assay offers an alternative method, using bioluminescent tagged probes that provide high specificity and accurate quantification, along with short experimental duration, albeit with challenges in probe design (Mbavha et al., 2023). Additionally, there are specialized commercial products, such as the AmpliChip CYP450 assay and GenoChip CYP2D6 macroarray, which are effective tools for CYP2D6 genotypes (Heller et al., 2006; Bank et al., 2015). These methods provide an efficient and rapid means of advancing the application of pharmacogenetics in clinical settings.
Recent advances in next-generation sequencing (NGS) have led to the development of several algorithms for inferring CYP2D6 haplotype from NGS data, including Stargazer, Aldy, Constellation, Cypiripi and Cyrius (Lee et al., 2019; Rosenbaum, 2020; Numanagic et al., 2018; Twist et al., 2016; Chen et al., 2021). These approaches provide a valuable way for predicting an individual’s metabolism, making the use existing data more cost-effective and widely accessible.
However, there are various reasons why genotyping for CYP2D6 may not be feasible (Brown et al., 2021; Chenoweth et al., 2020). In such cases, alternative methods for sequencing the CYP2D6 gene become particularly important. For example, Shimizu et al. revealed that utilizing AUC values for average daily urinary excretion could be an effective way to estimate the CYP2D6 phenotype in pediatric patients (Shimizu et al., 2023). Additionally, the relatively narrow ranges of 4-hydroxyatomoxetine and N-desmethyl-atomoxetine concentration ratios in spot urine samples from children could serve as a simple, semi-quantitative indicator of CYP2D6 IMs (Shimizu et al., 2023).
By obtaining the AS of CYP2D6 through methods other than genotyping, it becomes possible to predict the pharmacokinetic parameters of atomoxetine. For example, physiologically based pharmacokinetic (PBPK) models have been successfully used to describe and predict the AS-dependent metabolism of CYP2D6 substrates like atomoxetine based on plasma concentration-time profiles. In the absence of CYP2D6 genotype data, plasma atomoxetine concentrations have been successfully predicted using generally known AS values (Rudesheim et al., 2022). Furthermore, Cheng et al. (2023) developed an comprehensive PPK model to describe the pharmacokinetic profiles of atomoxetine and its metabolites in both plasma and urine, incorporating the effects of CYP2D6’ ASs and BW on model parameters, which is anticipated to aid in future optimization of atomoxetine dosing.
5.2.2 Other potential biomarkers
Based on the mechanism of action of atomoxetine (Figure 1), some studies have explored the potential of other substances as neurodevelopmental biomarkers. Examples include 3,4-dihydroxy phenylethylene glycol (DHPG) (Kielbasa and Lobo, 2015; Kielbasa et al., 2015; Bieck et al., 2016; Montoya et al., 2011), dopamine β-hydroxylase (DBH) (Fang et al., 2015), norepinephrine transporter (NET) (Chatterjee et al., 2023; Gul et al., 2022; Yang et al., 2013), and Brain-derived neurotrophic factor (BDNF) (Demirci et al., 2022; Ramos-Quiroga et al., 2014). In addition, various plasma and urinary metabolites from children with ADHD have also been identified, which may serve as potential markers for further study (Wang et al., 2021; Tian et al., 2022).
6 Exposure-response (PK-PD)
6.1 Is there an accepted and clinically relevant metric for systemic exposure to atomoxetine?
Atomoxetine is taken orally, and main pharmacokinetic parameters identified in the literature include plasma peak concentration (Cmax), AUC, and CL/F (Table 3), as along with plasma concentrations measured at specific intervals after administration (e.g., 12 h). Current clinical evidence strongly associates Cmax with the efficacy of atomoxetine, which is why it is recommended as a primary monitoring parameter in guidelines (Hiemke et al., 2018; Brown et al., 2019). Although establishing a connection between Cmax and adverse reactions can be more difficult, some findings in the literature address this relationship as well (Guo et al., 2024).
6.2 Is there evidence for the relationship between plasma atomoxetine concentration and clinical activity?
A systematic review and dose-response meta-analysis found that the effectiveness of atomoxetine increased up to a dosage of 1.4 mg/kg, after which it plateaued (Terao et al., 2024). There is considerable interest in determining if specific plasma concentrations of atomoxetine can predict the level of clinical response. In an early investigation, Michelson and co-researchers applied a nonlinear model to analyze peak concentrations and the relative change from baseline in the ADHDRS-IV-Parent: Inv total score. This model indicated that the maximum expected improvement compared to baseline would be −23.5, aligning with a plasma atomoxetine concentration of 400 ng/mL (Michelson et al., 2007). However, Hazell et al. revealed that while certain patients may benefit from higher plasma atomoxetine levels (>800 ng/mL), mere exposure to these levels dose not reliably predict the therapeutic outcomes in children with ADHD, suggesting that other factors also influence the response to atomoxetine (Hazell et al., 2009).
In a recent non-randomized prospective interventional study, Sugimoto et al. (2021) found that children with ADHD aged 6–12 years (n = 43) were more likely to respond to respond to atomoxetine treatment when its steady-state plasma concentration exceeded 64.60 ng/mL. Similarly, Guo et al. (2024) identified a lower threshold of 268 ng/mL as a potential therapeutic reference range for pediatric patients receiving q.m. atomoxetine, suggesting that effectiveness increases when this level is surpassed. Conversely, Ruppert et al. (2022) found that neither a concentration-effect relationship nor a dose-effect relationship was observed.
6.3 Is there evidence for the relationship between plasma atomoxetine concentration and tolerability?
In general, CYP2D6 PMs are more likely to experience side effects from atomoxetine than non-PMs, likely due to their higher exposure to the drug (Brown et al., 2019; Michelson et al., 2007). However, it is still uncertain whether drug exposure metrics like Cmax or AUC have a significant influence on tolerability, as there are only a few studies investigating the relationship between plasma atomoxetine concentrations and clinical outcomes. One early clinical trial found no correlation between plasma atomoxetine concentrations and its tolerability (Hazell et al., 2009). Similarly, a TDM study involving children and adolescents with ADHD did not reveal any clear relationship between serum concentrations and side effects (Ruppert et al., 2022). In contrast, a recent study by Guo et al. (2024) did identify a correlation between certain adverse reactions and plasma atomoxetine concentration. Specifically, in CYP2D6 IMs receiving once-daily dosing or EMs receiving twice-daily dosing, a significant difference was observed in the occurrence of gastrointestinal (e.g., 510 vs. 386 ng/mL, p = 0.0411) and neurological adverse reactions, even at plasma atomoxetine concentrations where no adverse reactions were reported.
7 Evaluation of TDM
TDM involves measuring and interpreting drug concentrations in biological fluids such as plasma and serum to tailor drug dosages or schedules, maximizing therapeutic benefits while minimizing toxicity for individual patients. Since 2000, the AGNP TDM guidelines have offered valuable direction for adjusting dosages of various psychiatric medications (Hiemke et al., 2018; Baumann et al., 2004; Ulrich et al., 2007; Schafer et al., 2016; Hiemke, 2016). The AGNP TDM guidelines in neuropsychopharmacology, established in 2011, along with the 2019 CPIC guidelines (Brown et al., 2019), both recommend TDM for atomoxetine. These guidelines may have played a crucial role in promoting the appropriate use of atomoxetine for patients with ADHD.
7.1 Is there evidence that TDM improves effectiveness in patients receiving atomoxetine?
As of now, no studies have directly compared the therapeutic effects of atomoxetine before and after the implementation of TDM. However, clinicians at our hospital believe that TDM has significantly enhanced their ability to select medications and adjust dosages. With TDM support, they can make more timely clinical decisions, such as switching from atomoxetine to alternative medications or tailoring the dosage. This approach has allowed for a more efficient determination of the optimal dosage for pediatric patients using atomoxetine. Notably, some children with ADHD have experienced effective control with relatively lower doses, an outcome that was less common prior to TDM implementation. We are currently gathering such real-world clinical data and planning to design clinical trials to systematically evaluate the benefits of implementing TDM for atomoxetine.
7.2 Is there evidence that TDM reduces tolerability in patients receiving atomoxetine?
Toxicity from overdose is thought to arise from elevated synaptic NE levels, which can induce an excessive noradrenergic-mediated sympathomimetic syndrome, which typically presents as tachycardia and hypertension. In cases of atomoxetine overdose, clinical manifestations are generally mild. Common symptoms include drowsiness (particularly in children), agitation, hyperactivity, gastrointestinal disturbances, tremors, hyperreflexia, tachycardia, hypertension, and seizures. Fortunately, these symptoms typically resolve quickly, with complete recovery usually occurring within 24 h post-overdose (Spiller et al., 2013). However, some patients may need to discontinue atomoxetine due to inability to tolerate several common adverse reactions reported in clinical trials, including nausea, vomiting, fatigue, decreased appetite, abdominal pain, and somnolence (Mechler et al., 2022).
Similarly, there has been no public report to date examining whether the tolerability of atomoxetine improves before and after the implementation of TDM. Additionally, the correlation between tolerability and concentration has also not been established. However, it is indeed more likely for PMs to experience adverse reactions compared to non-PMs (Brown et al., 2019). From this perspective, implementing TDM is expected to improve tolerability. For example, TDM could help identify children on low doses of atomoxetine who have low exposure, resulting in poor tolerance and inadequate efficacy. In such cases, a timely medication switch may be appropriate, potentially avoiding the need for further dose escalation to achieve efficacy. Conversely, for children with high exposure who show good efficacy and good tolerance, a dose reduction can be considered to alleviate the body’s burden of atomoxetine. This area warrants exploration in clinical trial and represents a significant clinical issue that should be prioritized.
8 Clinical implementation
8.1 Are reliable assays available?
Various bioanalytical assays have been established to measure atomoxetine in human plasma, serum, urine, or hair, using techniques such as (high-performance) liquid chromatography combined with detection methods such as UV detector (Patel et al., 2007; Guo et al., 2007; Teichert et al., 2020), fluorescence detector (Stegmann et al., 2016; Zhu et al., 2007), or by (tandem) mass spectrometry (Mullen et al., 2005; Papaseit et al., 2012; Papaseit et al., 2013; Sim et al., 2017; Choi et al., 2012; Marchei et al., 2012; Xia et al., 2021; Skaalvik et al., 2021). Most of these methods are lab-developed and may have limited general applicability. Recently, new strategies have emerged recently. For example, Abu-Hassan developed a Nano-level assay based on molecular-size-based resonance Rayleigh scattering to detect atomoxetine in both its prescribed dosage form and plasma samples. This environmentally friendly fluorometric technique shows considerable promise for application due to its significant advantages, such as intelligent selectivity, exceptional sensitivity, minimal solvent consumption, widespread availability in laboratories, rapid analysis times, and ease of use (Abu-Hassan, 2023). Importantly, the choice of method is less critical than ensuring accurate determination of atomoxetine concentration in biological samples; researchers can select an assay based on its accessibility.
8.2 Is the proper sampling timing and handling established?
When performing TDM for atomoxetine, several factors need to be considered regarding blood collection methods. First, the genetic polymorphism of CYP2D6 and metabolic phenotypes (UM, NM, IM, and PM) of CYP2D6 significantly influence the drug’s metabolism, leading to significant differences in its t1/2. Notably, the t1/2 of atomoxetine in PMs was 4-fold higher than that of EMs (Sauer et al., 2005; Michelson et al., 2007; Byeon et al., 2015). In clinical practice, Cmax is primarily used as a parameter to assess the correlation between systemic exposure to atomoxetine, its effectiveness, and adverse reactions. Consequently, patients with different CYP2D6 phenotypes may experience varying peak times even under the same dosing regimen. Given the pharmacokinetic variations linked to CYP2D6 phenotypes that affect Cmax and t1/2, the CPIC guideline recommends that prescribers consider measuring peak concentrations at specific time intervals: 1) 1–2 h post-dose in known CYP2D6 UMs, NMs, and IMs with high activity (AS 1.0 without the CYP2D6*10 allele); 2) 2–4 h post-dose in CYP2D6 IMs with low activity (AS 0.5) and individuals with an AS of 1 who carry the CYP2D6*10 allele; and 3) 4 h post-dose for PMs (Brown et al., 2019).
Second, the dosing regimen also affects the timing blood sample collection. For q.m. and b.i.d. regimens, it's generally straightforward to collect blood samples 1–4 h after drug administration. However, for children who take medication once at night (q.n.; not many, but seen (Mechler et al., 2022)), determining the interval (e.g., 12 h) (Guo et al., 2024; Sugimoto et al., 2021) for blood sample collection can be a challenging issue. This situation may necessitate prior communication with the physician regarding the timing of the previous night’s medication and the blood collection time the following day.
Third, if genetic and phenotypic information is not available, or even if it is, a concentration obtained from a single time-point sample may not accurately represent the Cmax. The CPIC guideline also suggest collecting blood sample within a specific time window after dosing (Brown et al., 2019). Therefore, it may be necessary to consider sampling at steady state, despite studies that investigate the relationship between steady-state trough concentrations and clinical response (Sugimoto et al., 2021).
8.3 Is there a recommended therapeutic exposure range based on the clinical evidence?
As of now, two guidelines provide recommendations for the therapeutic reference range of atomoxetine. According to the AGNP TDM Expert Group consensus guidelines, peak plasma concentrations between 200 and 1,000 ng/mL, measured 60–90 min after a dose of 1.2 mg/kg/day, are commonly regarded as the therapeutic reference range, but this has only been studied in adults (Hiemke et al., 2018). The latest CPIC guideline also establishes a therapeutic reference range for peak plasma concentration at 200 and 1,000 ng/mL, noting that adequate responses can be achieved when the plasma concentrations exceed 400 ng/mL (Brown et al., 2019).
Interestingly, a recent retrospective study by Guo et al. involving children with ADHD, recommended a minimum Cmax of 268 ng/mL associated with achieving a favorable therapeutic effect for patients receiving q.m. dosing of atomoxetine (Guo et al., 2024). Additionally, a naturalistic study in children and adolescents with ADHD proposed a therapeutic reference range of 100–400 ng/mL (Sugimoto et al., 2021). Researchers also recommend the minimum steady-state trough concentration of 64.6 mg/mL necessary for a good control of ADHD symptoms (Sugimoto et al., 2021).
8.4 Is there a dose-adaptation strategy?
In 2019, the CPIC released guidelines proposing the use of plasma concentration in conjunction with an individual’s CYP2D6 genotype to assist clinicians in dose selection and titration. For patients classified as CYP2D6 UMs and NMs, if the peak concentration is <200 ng/mL and there is no clinical response, it is advisable to increase the dose proportionately to achieve approximately 400 ng/mL. For CYP2D6 PMs, IMs, and NMs with an AS of 1 who carry the CYP2D6*10 allele and taking a standard starting dose, the recommendation is to consider a proportional dose adjustment to reach about 400 ng/mL if there is an inadequate response without side effects (Brown et al., 2019).
In recent years, there have been extensive efforts to create personalized dosing strategies for atomoxetine using PBPK (Shimizu et al., 2023; Rudesheim et al., 2022; Dinh et al., 2016; Kim et al., 2018; Notsu et al., 2020; Alsmadi et al., 2022) and PPK (Cheng et al., 2023) models. Of note, recent PPK simulations revealed that the majority of individuals with a CYP2D6 AS of 1–3 may not achieve a steady-state Cmax of 400 ng/mL with a 0.5 mg/kg once daily (q.d.) dosage, whereas most individuals with a CYP2D6 AS<1 could reach this concentration. This suggests that individuals with CYP2D6 AS of 1–3 may require a higher dose of atomoxetine compared to those with scores <1. To achieve a steady-state atomoxetine Cmax comparable to that of individuals with an AS of 0 following a 0.5 mg/kg q.d. dose of atomoxetine, individuals with CYP2D6 AS 1-3 would require an approximately 1.2 mg/kg q.d. dose (Cheng et al., 2023). These findings largely align with the dosing recommendations outlined in the above noted CPIC guideline (Brown et al., 2019).
9 Cost effectiveness analysis of TDM and genotyping testing
9.1 Is there a cost effectiveness analysis of TDM testing for atomoxetine?
Cost-effectiveness analysis in healthcare, particularly for TDM, is still developing. Initially, TDM was only shown to be cost-effective for aminoglycosides (Touw et al., 2005). However, recent evidence indicates that TDM interventions can also be cost-effective in the application of antibody drugs (Martelli et al., 2017) and anti-cancer medications (Vithanachchi et al., 2021). While there is some rationale supporting the TDM of atomoxetine, comprehensive cost-effectiveness analyses have not yet been conducted. Consequently, the emphasis should extend beyond just cost-effectiveness to encompass how these interventions can be implemented in a clinically beneficial and economically sustainable way.
9.2 Is there a cost effectiveness analysis of CYP2D6 genotyping testing for atomoxetine?
When integrating pharmacogenomics (PGx) into clinical practice, cost is also a crucial consideration for both healthcare systems and patients (Morris et al., 2022). Despite a substantial decrease in of PGx testing costs over the past decade, it continues to pose a significant barrier to widespread implementation in children’s hospitals (Brown et al., 2021). For certain medications, such as clopidogrel and warfarin, there is considerable cost data available that provide strong support for the use of PGx testing (Dong et al., 2020; Zhu et al., 2021). Additionally, cost-effectiveness analyses of CYP2D6 genotyping have primarily focused on antidepressant medications (Groessl et al., 2018; Maciel et al., 2018), with no relevant studies on atomoxetine thus far.
9.3 Is there a cost effectiveness analysis of combined TDM and CYP2D6 genotyping testing for atomoxetine?
For certain medications, integrating TDM with pharmacogenomics proves to be an effective approach to optimize treatment, emphasizing the importance of assessing the cost-effectiveness of both methods. One study found that a combined strategy of NUDT15/TPMT genotype screening prior to initiating azathioprine treatment, along with on-going TDM for management, was more cost-effective than alternatives that involved either genotyping NUDT15 or TPMT alone or conducting genotyping without TDM in patients with inflammatory bowel disease (Zeng et al., 2021). However, comprehensive cost evaluations for atomoxetine are still lacking.
10 Perspectives and conclusions
Following the generic framework proposed by Beumer et al. (Beumer et al., 2019), we conducted a comprehensive literature review, evaluation, and summary to create a table (BOX 1) that prominently presents the critical questions of interest along with the evidence gathered to date. More importantly, we identified gaps in existing knowledge related to the goal of personalized dosing and identified areas for future research.
A fundamental aspect of achieving precision medicine is to distinguish a given patient from others with similar clinical presentations by combining genetic, biomarker, phenotypic, or psychosocial characteristics (Jameson and Longo, 2015). In this review article, we focus on personalized dosing of atomoxetine in children with ADHD, aiming to provide strategies for adjusting doses specifically for children who have been accurately diagnosed and are considered appropriate candidates for atomoxetine therapy. Our goal is to maximize therapeutic benefits while minimizing adverse reactions. In essence, we seek to determine the “right dose” for the “right person”. However, we still face numerous challenges.
10.1 Challenge 1: there is no established association between exposure and clinical response
The first challenge in implementing personalized dose adjustment lies in the unclear relationship between atomoxetine exposure levels and both its efficacy and adverse reactions. ADHD is a complex and heterogeneous disorder (Posner et al., 2020; LaBianca et al., 2024), highlighting the need to evaluate medication responses in relation to the pharmacokinetics and duration of action of the selected formulation. Optimal symptom management and functional improvement occur when blood levels of the medication are adequately maintained for the periods of greatest need and for the specific tasks at hand (Faraone et al., 2024). Current evidence generally supports identifying the lowest concentration or concentration range that ensures optimal efficacy; however, data regarding the maximum tolerable concentration levels still relatively sparse. Additionally, there is also scarce data on the relationship between exposure levels to atomoxetine and its adverse reactions. Consequently, there is no clear therapeutic window defined for atomoxetine. Without this defined window, there is no established “target value” for dose selection, complicating the process of making personalized dose adjustments. Also, the lack of a defined therapeutic window has compromised the role of PPK/PBPK models in predicting personalized doses, posing a significant challenge for future efforts in this area. In response to these challenges, machine learning-based predictive models have emerged as a promising strategy (Faraone et al., 2021; Faraone et al., 2022).
10.2 Challenge 2: there are no recognized predictors for atomoxetine therapy response
The second challenge involves the lack of predictors for treatment response to atomoxetine. By integrating biomarkers and clinical predictors of both response and adverse effects, clinicians could potentially tailor treatment for individual patients. However, there are currently no available clinical or biological predictors of response for ADHD (Buitelaar et al., 2022). At this time, the genotypes and phenotypes of CYP2D6 may serve as the most “reliable” predictor. As the primary metabolic enzyme for atomoxetine, CYP2D6’s metabolic activity directly influences the drug’s pharmacokinetic behavior, thereby linking exposure levels to both efficacy and adverse reactions. In other words, variations in CYP2D6 activity fundamentally “determine” the differences in both the efficacy and adverse reactions of atomoxetine. However, the predictive power of CYP2D6 is limited, mainly due to the lack of a well-established exposure-response relationship, as previously noted. Additionally, the inherent uncertainties in predicting drug response based solely on genetic markers, given the potential for false negatives or positives in CYP2D6 genotyping, also pose challenges in clinical practice as well. Investigating ways to standardize the translation of genotyping data into actionable, evidence-based prescribing decisions is an important endeavor. Nonetheless, personalized dosing strategies that utilize CYP2D6 stratification remain crucial and significantly continue to the rational use of atomoxetine (Brown et al., 2019; Guo et al., 2024).
10.3 Challenge 3: quantitative assessment indicators for evaluating ADHD
The third challenge comes from how to objectively and accurately assess the clinical efficacy of atomoxetine (Wong et al., 2019; Raman et al., 2018). To tackle this issue, we require more objective and quantifiable indicators that can accurately depict changes in symptoms and reflect treatment outcomes. Currently, it is recognized that the diagnostic rate for female ADHD patients is lower than that for males (Martin, 2024). If this discrepancy continues to affect efficacy assessments, it could hinder the effective implementation of personalized dosing strategies.
10.4 Challenge 4: socio-political barriers to TDM and genotyping implementation
As early as 2015, it was clearly understood that achieving precision medicine would necessitate overcoming major challenges across various domains, including technological and socio-political aspects. TDM and pharmacogenomics, as key elements of precision medicine, face few technical hurdles; however, socio-political factors such as public support, affordability, and education pose even more obstacles (Kohane, 2015). Indeed, numerous challenges will persist in clinical practice, limiting the widespread implementation of precision medicine in clinical settings (Chenoweth et al., 2020).
While TDM and genotyping technologies do not inherently pose obstacles to implementing personalized medicine, their widespread use in clinical settings is not encouraging, particularly in children’s hospitals (Brown et al., 2021; Chenoweth et al., 2020; Just et al., 2019; Duarte et al., 2021), remains limited. Few institutions have the capability to conduct both TDM and genetic testing simultaneously, hindering individualized dose adjustments. For example, a nationwide survey by Jacob et al. found that only four centers implemented TDM for atomoxetine alongside CYP2D6 genotyping (Brown et al., 2021). Similarly, our recent survey on the clinical implementation of PGx testing revealed that only four children’s hospital conducted CYP2D6 genotyping (Wu et al., 2024). Additionally, the cost associated with implementing genotyping and TDM in clinical practice raises significant concern for healthcare systems and patients, making cost evaluations critical (Morris et al., 2022). Furthermore, effective implementation also requires collaboration among genotyping and TDM labs, bioinformatics/IT for result analysis and communication, and clinicians for integrating patient care. Notably, only one center offered clinical decision support for atomoxetine and CYP2D6 interaction within its electronic prescribing system (Brown et al., 2021). Therefore, building multidisciplinary teams around personalized dosing is crucial (Barker et al., 2022); where such teams are in place, they can significantly enhance the effectiveness of personalized dosing strategies.
10.5 Challenge 5: comprehensive understanding of ADHD
Ultimately, a comprehensive understanding of the ADHD itself may present the greatest challenge. Our unwavering goal is to pursue personalized atomoxetine treatment, which depends on a deeper understanding of the condition’s heterogeneity through extensive studies into its etiology, pathophysiology, and clinical manifestations. Integrating multi-omics studies can facilitate the discovery and validation of biomarkers that could serve as potential clinical predictors of response (Hubers et al., 2024; Hagenbeek et al., 2023).
Additionally, it is essential to address medication adherence. Once adherence issues are resolved (Brikell et al., 2024), prioritizing the establishment of a precise match between patients and optimal atomoxetine treatment will become a focus for future research.
Overall, while this study extensively references various guidelines and theoretical frameworks, it lacks specific examples of clinical outcomes derived from these approaches. The key challenge is to effectively translate these theoretical concepts into practical, real-world clinical applications. Nonetheless, clinicians can gain valuable insights from the existing evidence, particularly in identifying the limitations of current guidelines and implementing personalized treatment across different clinical settings.
Author contributions
H-LG: Formal Analysis, Funding acquisition, Investigation, Methodology, Resources, Writing–original draft, Writing–review and editing. JH: Investigation, Methodology, Writing–original draft, Writing–review and editing. JW: Data curation, Formal Analysis, Methodology, Writing–review and editing. LF: Data curation, Formal Analysis, Writing–review and editing. YL: Formal Analysis, Investigation, Methodology, Writing–review and editing. D-DW: Data curation, Formal Analysis, Methodology, Writing–review and editing. Q-QL: Data curation, Formal Analysis, Methodology, Writing–review and editing. FC: Data curation, Formal Analysis, Funding acquisition, Investigation, Methodology, Writing–original draft, Writing–review and editing.
Funding
The author(s) declare that financial support was received for the research, authorship, and/or publication of this article. This research was supported by the Specially Appointed Medical Expert Project of Jiangsu Commission of Health (2019), the Talent Project established by Chinese Pharmaceutical Association Hospital Pharmacy department (NO. CPA-Z05-ZC-2022-003), and by a grant from Jiangsu Research Hospital Association for Precision Medication (JY202208). This study was also supported by the Scientific Research Foundation for Top Young Scholars at the Children’s Hospital of Nanjing Medical University (2020).
Conflict of interest
The authors declare that the research was conducted in the absence of any commercial or financial relationships that could be construed as a potential conflict of interest.
Publisher’s note
All claims expressed in this article are solely those of the authors and do not necessarily represent those of their affiliated organizations, or those of the publisher, the editors and the reviewers. Any product that may be evaluated in this article, or claim that may be made by its manufacturer, is not guaranteed or endorsed by the publisher.
References
Abu-Hassan, A. A. (2023). Nano-level assay of attention-deficit/hyperactivity disorder medicament, atomoxetine by molecular-size-based resonance Rayleigh scattering strategy. Employment in content uniformity, dosage form, and plasma analysis. BMC Chem. 17 (1), 175. doi:10.1186/s13065-023-01094-y
Alsmadi, M. M., Al Eitan, L. N., Idkaidek, N. M., and Alzoubi, K. H. (2022). The development of a PBPK model for atomoxetine using levels in plasma, saliva and brain extracellular fluid in patients with normal and deteriorated kidney function. CNS Neurol. Disord. Drug Targets 21 (8), 704–716. doi:10.2174/1871527320666210621102437
Amna, S., Ohlenschlaeger, T., Saedder, E. A., Sigaard, J. V., and Bergmann, T. K. (2024). Review of clinical pharmacokinetics and pharmacodynamics of clonidine as an adjunct to opioids in palliative care. Basic Clin. Pharmacol. Toxicol. 134 (4), 485–497. doi:10.1111/bcpt.13979
Atiq, M. A., Peterson, S. E., Langman, L. J., Baudhuin, L. M., Black, J. L., and Moyer, A. M. (2023). Determination of the duplicated CYP2D6 allele using real-time PCR signal: an alternative approach. J. Pers. Med. 13 (6), 883. doi:10.3390/jpm13060883
Auro, K., Holopainen, I., Perola, M., Havulinna, A. S., and Raevuori, A. (2024). Attention-deficit/hyperactivity disorder diagnoses in Finland during the COVID-19 pandemic. JAMA Netw. Open 7 (6), e2418204. doi:10.1001/jamanetworkopen.2024.18204
Bank, P. C., Swen, J. J., Guchelaar, H. J., and van der Straaten, T. (2015). GenoChip CYP2D6 macroarray as a method to genotype for CYP2D6 variants: results of a validation study in a Caucasian population. Pharmacogenomics 16 (7), 681–687. doi:10.2217/pgs.15.30
Barker, C. I. S., Groeneweg, G., Maitland-van der Zee, A. H., Rieder, M. J., Hawcutt, D. B., Hubbard, T. J., et al. (2022). Pharmacogenomic testing in paediatrics: clinical implementation strategies. Br. J. Clin. Pharmacol. 88 (10), 4297–4310. doi:10.1111/bcp.15181
Baumann, P., Hiemke, C., Ulrich, S., Eckermann, G., Gaertner, I., Gerlach, M., et al. (2004). The AGNP-TDM expert group consensus guidelines: therapeutic drug monitoring in psychiatry. Pharmacopsychiatry 37 (6), 243–265. doi:10.1055/s-2004-832687
Belle, D. J., Ernest, C. S., Sauer, J. M., Smith, B. P., Thomasson, H. R., and Witcher, J. W. (2002). Effect of potent CYP2D6 inhibition by paroxetine on atomoxetine pharmacokinetics. J. Clin. Pharmacol. 42 (11), 1219–1227. doi:10.1177/009127002762491307
Beumer, J. H., Chu, E., Allegra, C., Tanigawara, Y., Milano, G., Diasio, R., et al. (2019). Therapeutic drug monitoring in oncology: international association of therapeutic drug monitoring and clinical toxicology recommendations for 5-fluorouracil therapy. Clin. Pharmacol. Ther. 105 (3), 598–613. doi:10.1002/cpt.1124
Bieck, P. R., Leibowitz, M., Lachno, D. R., Ledent, E., Padich, R., and Jhee, S. (2016). Dihydroxyphenylglycol as a biomarker of norepinephrine transporter inhibition by atomoxetine: human model to assess central and peripheral effects of dosing. J. Clin. Psychopharmacol. 36 (6), 675–683. doi:10.1097/JCP.0000000000000611
Brikell, I., Yao, H., Li, L., Astrup, A., Gao, L., Gillies, M. B., et al. (2024). ADHD medication discontinuation and persistence across the lifespan: a retrospective observational study using population-based databases. Lancet Psychiatry 11 (1), 16–26. doi:10.1016/S2215-0366(23)00332-2
Brown, J. T. (2022). The pharmacogenetic impact on the pharmacokinetics of ADHD medications. Methods Mol. Biol. 2547, 427–436. doi:10.1007/978-1-0716-2573-6_15
Brown, J. T., Abdel-Rahman, S. M., van Haandel, L., Gaedigk, A., Lin, Y. S., and Leeder, J. S. (2016). Single dose, CYP2D6 genotype-stratified pharmacokinetic study of atomoxetine in children with ADHD. Clin. Pharmacol. Ther. 99 (6), 642–650. doi:10.1002/cpt.319
Brown, J. T., Bishop, J. R., Sangkuhl, K., Nurmi, E. L., Mueller, D. J., Dinh, J. C., et al. (2019). Clinical pharmacogenetics implementation Consortium guideline for cytochrome P450 (CYP)2D6 genotype and atomoxetine therapy. Clin. Pharmacol. Ther. 106 (1), 94–102. doi:10.1002/cpt.1409
Brown, J. T., Ramsey, L. B., Van Driest, S. L., Aka, I., and Colace, S. I. (2021). Characterizing pharmacogenetic testing among children's hospitals. Clin. Transl. Sci. 14 (2), 692–701. doi:10.1111/cts.12931
Buitelaar, J., Bolte, S., Brandeis, D., Caye, A., Christmann, N., Cortese, S., et al. (2022). Toward precision medicine in ADHD. Front. Behav. Neurosci. 16, 900981. doi:10.3389/fnbeh.2022.900981
Byeon, J. Y., Kim, Y. H., Na, H. S., Jang, J. H., Kim, S. H., Lee, Y. J., et al. (2015). Effects of the CYP2D6*10 allele on the pharmacokinetics of atomoxetine and its metabolites. Arch. Pharm. Res. 38 (11), 2083–2091. doi:10.1007/s12272-015-0646-z
Chalon, S. A., Desager, J. P., Desante, K. A., Frye, R. F., Witcher, J., Long, A. J., et al. (2003). Effect of hepatic impairment on the pharmacokinetics of atomoxetine and its metabolites. Clin. Pharmacol. Ther. 73 (3), 178–191. doi:10.1067/mcp.2003.25
Chatterjee, M., Saha, S., Maitra, S., Ray, A., Sinha, S., and Mukhopadhyay, K. (2023). Post-treatment symptomatic improvement of the eastern Indian ADHD probands is influenced by CYP2D6 genetic variations. Drug Metab. Pers. Ther. 38 (1), 45–56. doi:10.1515/dmpt-2022-0120
Chen, X., Shen, F., Gonzaludo, N., Malhotra, A., Rogert, C., Taft, R. J., et al. (2021). Cyrius: accurate CYP2D6 genotyping using whole-genome sequencing data. Pharmacogenomics J. 21 (2), 251–261. doi:10.1038/s41397-020-00205-5
Cheng, S., Al-Kofahi, M., Leeder, J. S., and Brown, J. T. (2023). Population pharmacokinetic analysis of atomoxetine and its metabolites in children and adolescents with attention-deficit/hyperactivity disorder. Clin. Pharmacol. Ther. 115, 1033–1043. doi:10.1002/cpt.3155
Chenoweth, M. J., Giacomini, K. M., Pirmohamed, M., Hill, S. L., van Schaik, R. H. N., Schwab, M., et al. (2020). Global pharmacogenomics within precision medicine: challenges and opportunities. Clin. Pharmacol. Ther. 107 (1), 57–61. doi:10.1002/cpt.1664
Choi, C. I., Bae, J. W., Lee, H. I., Jang, C. G., Sohn, U. D., and Lee, S. Y. (2012). Determination of atomoxetine metabolites in human plasma by liquid chromatography/tandem mass spectrometry and its application to a pharmacokinetic study. J. Chromatogr. B Anal. Technol. Biomed. Life Sci. 885-886, 103–108. doi:10.1016/j.jchromb.2011.12.023
Choi, C. I., Bae, J. W., Lee, Y. J., Lee, H. I., Jang, C. G., and Lee, S. Y. (2014). Effects of CYP2C19 genetic polymorphisms on atomoxetine pharmacokinetics. J. Clin. Psychopharmacol. 34 (1), 139–142. doi:10.1097/JCP.0b013e3182a608a2
Cicali, E. J., Smith, D. M., Duong, B. Q., Kovar, L. G., Cavallari, L. H., and Johnson, J. A. (2020). A scoping review of the evidence behind cytochrome P450 2D6 isoenzyme inhibitor classifications. Clin. Pharmacol. Ther. 108 (1), 116–125. doi:10.1002/cpt.1768
Coghill, D., Banaschewski, T., Cortese, S., Asherson, P., Brandeis, D., Buitelaar, J., et al. (2023). The management of ADHD in children and adolescents: bringing evidence to the clinic: perspective from the European ADHD Guidelines Group (EAGG). Eur. Child. Adolesc. Psychiatry 32 (8), 1337–1361. doi:10.1007/s00787-021-01871-x
Cortese, S. (2020). Pharmacologic treatment of attention deficit-hyperactivity disorder. N. Engl. J. Med. 383 (11), 1050–1056. doi:10.1056/NEJMra1917069
Cui, Y. M., Teng, C. H., Pan, A. X., Yuen, E., Yeo, K. P., Zhou, Y., et al. (2007). Atomoxetine pharmacokinetics in healthy Chinese subjects and effect of the CYP2D6*10 allele. Br. J. Clin. Pharmacol. 64 (4), 445–449. doi:10.1111/j.1365-2125.2007.02912.x
Danielson, M. L., Claussen, A. H., Bitsko, R. H., Katz, S. M., Newsome, K., Blumberg, S. J., et al. (2024). ADHD prevalence among U.S. Children and adolescents in 2022: diagnosis, severity, Co-occurring disorders, and treatment. J. Clin. Child. Adolesc. Psychol. 53 (3), 343–360. doi:10.1080/15374416.2024.2335625
de Leon, J. (2015). Translating pharmacogenetics to clinical practice: do cytochrome P450 2D6 ultrarapid metabolizers need higher atomoxetine doses? J. Am. Acad. Child. Adolesc. Psychiatry 54 (7), 532–534. doi:10.1016/j.jaac.2015.04.003
Demirci, E., Sener, E. F., Gul, M. K., Onal, M. G., and Dal, F. (2022). A view of response and resistance to atomoxetine treatment in children with ADHD: effects of CYP2C19 polymorphisms and BDNF levels. Eur. J. Clin. Pharmacol. 78 (7), 1095–1104. doi:10.1007/s00228-022-03321-2
Dinh, J. C., Pearce, R. E., Van Haandel, L., Gaedigk, A., and Leeder, J. S. (2016). Characterization of atomoxetine biotransformation and implications for development of PBPK models for dose individualization in children. Drug Metab. Dispos. 44 (7), 1070–1079. doi:10.1124/dmd.116.069518
Dong, O. M., Wheeler, S. B., Cruden, G., Lee, C. R., Voora, D., Dusetzina, S. B., et al. (2020). Cost-effectiveness of multigene pharmacogenetic testing in patients with acute coronary syndrome after percutaneous coronary intervention. Value Health 23 (1), 61–73. doi:10.1016/j.jval.2019.08.002
Duarte, J. D., Dalton, R., Elchynski, A. L., Smith, D. M., Cicali, E. J., Lee, J. C., et al. (2021). Multisite investigation of strategies for the clinical implementation of pre-emptive pharmacogenetic testing. Genet. Med. 23 (12), 2335–2341. doi:10.1038/s41436-021-01269-9
Fang, Y., Ji, N., Cao, Q., Su, Y., Chen, M., Wang, Y., et al. (2015). Variants of dopamine beta hydroxylase gene moderate atomoxetine response in children with attention-deficit/hyperactivity disorder. J. Child. Adolesc. Psychopharmacol. 25 (8), 625–632. doi:10.1089/cap.2014.0178
Faraone, S. V., Asherson, P., Banaschewski, T., Biederman, J., Buitelaar, J. K., Ramos-Quiroga, J. A., et al. (2015). Attention-deficit/hyperactivity disorder. Nat. Rev. Dis. Prim. 1, 15020. doi:10.1038/nrdp.2015.20
Faraone, S. V., Bellgrove, M. A., Brikell, I., Cortese, S., Hartman, C. A., Hollis, C., et al. (2024). Attention-deficit/hyperactivity disorder. Nat. Rev. Dis. Prim. 10 (1), 11. doi:10.1038/s41572-024-00495-0
Faraone, S. V., Gomeni, R., Hull, J. T., Busse, G. D., Melyan, Z., O'Neal, W., et al. (2021). Early response to SPN-812 (viloxazine extended-release) can predict efficacy outcome in pediatric subjects with ADHD: a machine learning post-hoc analysis of four randomized clinical trials. Psychiatry Res. 296, 113664. doi:10.1016/j.psychres.2020.113664
Faraone, S. V., Gomeni, R., Hull, J. T., Chaturvedi, S. A., Busse, G. D., Melyan, Z., et al. (2022). Predicting efficacy of viloxazine extended-release treatment in adults with ADHD using an early change in ADHD symptoms: machine learning post hoc analysis of a phase 3 clinical trial. Psychiatry Res. 318, 114922. doi:10.1016/j.psychres.2022.114922
Farhat, L. C., Flores, J. M., Behling, E., Avila-Quintero, V. J., Lombroso, A., Cortese, S., et al. (2022). The effects of stimulant dose and dosing strategy on treatment outcomes in attention-deficit/hyperactivity disorder in children and adolescents: a meta-analysis. Mol. Psychiatry 27 (3), 1562–1572. doi:10.1038/s41380-021-01391-9
Fu, D., Guo, H. L., Hu, Y. H., Fang, W. R., Liu, Q. Q., Xu, J., et al. (2023). Personalizing atomoxetine dosing in children with ADHD: what can we learn from current supporting evidence. Eur. J. Clin. Pharmacol. 79 (3), 349–370. doi:10.1007/s00228-022-03449-1
Fu, D., Wu, D. D., Guo, H. L., Hu, Y. H., Xia, Y., Ji, X., et al. (2021). The mechanism, clinical efficacy, safety, and dosage regimen of atomoxetine for ADHD therapy in children: a narrative review. Front. Psychiatry 12, 780921. doi:10.3389/fpsyt.2021.780921
Garland, M., and Kirkpatrick, P. (2004). Atomoxetine hydrochloride. Nat. Rev. Drug Discov. 3 (5), 385–386. doi:10.1038/nrd1387
Groessl, E. J., Tally, S. R., Hillery, N., Maciel, A., and Garces, J. A. (2018). Cost-effectiveness of a pharmacogenetic test to guide treatment for major depressive disorder. J. Manag. Care Spec. Pharm. 24 (8), 726–734. doi:10.18553/jmcp.2018.24.8.726
Gul, M. K., Sener, E. F., Onal, M. G., and Demirci, E. (2022). Role of the norepinephrine transporter polymorphisms in atomoxetine treatment: from response to side effects in children with ADHD. J. Psychopharmacol. 36 (6), 715–722. doi:10.1177/02698811211015245
Guo, H. L., Wu, D. D., Fu, D., Li, Y., Wang, J., Zhang, Y. Y., et al. (2024). Individualized atomoxetine response and tolerability in children with ADHD receiving different dosage regimens: the need for CYP2D6 genotyping and therapeutic drug monitoring to dance together. Transl. Psychiatry 14 (1), 151. doi:10.1038/s41398-024-02859-2
Guo, W., Li, W., Guo, G., Zhang, J., Zhou, B., Zhai, Y., et al. (2007). Determination of atomoxetine in human plasma by a high performance liquid chromatographic method with ultraviolet detection using liquid-liquid extraction. J. Chromatogr. B Anal. Technol. Biomed. Life Sci. 854 (1-2), 128–134. doi:10.1016/j.jchromb.2007.04.007
Hagenbeek, F. A., van Dongen, J., Pool, R., Roetman, P. J., Harms, A. C., Hottenga, J. J., et al. (2023). Integrative multi-omics analysis of childhood aggressive behavior. Behav. Genet. 53 (2), 101–117. doi:10.1007/s10519-022-10126-7
Hazell, P., Becker, K., Nikkanen, E. A., Trzepacz, P. T., Tanaka, Y., Tabas, L., et al. (2009). Relationship between atomoxetine plasma concentration, treatment response and tolerability in attention-deficit/hyperactivity disorder and comorbid oppositional defiant disorder. Atten. Defic. Hyperact. Disord. 1 (2), 201–210. doi:10.1007/s12402-009-0012-4
Heller, T., Kirchheiner, J., Armstrong, V. W., Luthe, H., Tzvetkov, M., Brockmoller, J., et al. (2006). AmpliChip CYP450 GeneChip: a new gene chip that allows rapid and accurate CYP2D6 genotyping. Ther. Drug Monit. 28 (5), 673–677. doi:10.1097/01.ftd.0000246764.67129.2a
Hiemke, C. (2016). Consensus guideline based therapeutic drug monitoring (TDM) in psychiatry and neurology. Curr. Drug Deliv. 13 (3), 353–361. doi:10.2174/1567201812666151029100909
Hiemke, C., Bergemann, N., Clement, H. W., Conca, A., Deckert, J., Domschke, K., et al. (2018). Consensus guidelines for therapeutic drug monitoring in neuropsychopharmacology: update 2017. Pharmacopsychiatry 51 (1-02), e1–e62. doi:10.1055/s-0037-1600991
Hubers, N., Hagenbeek, F. A., Pool, R., Dejean, S., Harms, A. C., Roetman, P. J., et al. (2024). Integrative multi-omics analysis of genomic, epigenomic, and metabolomics data leads to new insights for Attention-Deficit/Hyperactivity Disorder. Am. J. Med. Genet. B Neuropsychiatr. Genet. 195 (2), e32955. doi:10.1002/ajmg.b.32955
Jameson, J. L., and Longo, D. L. (2015). Precision medicine--personalized, problematic, and promising. N. Engl. J. Med. 372 (23), 2229–2234. doi:10.1056/NEJMsb1503104
Just, K. S., Turner, R. M., Dolzan, V., Cecchin, E., Swen, J. J., Gurwitz, D., et al. (2019). Educating the next generation of pharmacogenomics experts: global educational needs and concepts. Clin. Pharmacol. Ther. 106 (2), 313–316. doi:10.1002/cpt.1471
Kazda, L., Bell, K., Thomas, R., Hardiman, L., Heath, I., and Barratt, A. (2024). Attention deficit/hyperactivity disorder (ADHD) in children: more focus on care and support, less on diagnosis. BMJ 384, e073448. doi:10.1136/bmj-2022-073448
Kielbasa, W., and Lobo, E. (2015). Pharmacodynamics of norepinephrine reuptake inhibition: modeling the peripheral and central effects of atomoxetine, duloxetine, and edivoxetine on the biomarker 3,4-dihydroxyphenylglycol in humans. J. Clin. Pharmacol. 55 (12), 1422–1431. doi:10.1002/jcph.551
Kielbasa, W., Pan, A., and Pereira, A. (2015). A pharmacokinetic/pharmacodynamic investigation: assessment of edivoxetine and atomoxetine on systemic and central 3,4-dihydroxyphenylglycol, a biochemical marker for norepinephrine transporter inhibition. Eur. Neuropsychopharmacol. 25 (3), 377–385. doi:10.1016/j.euroneuro.2014.12.009
Kim, S. H., Byeon, J. Y., Kim, Y. H., Lee, C. M., Lee, Y. J., Jang, C. G., et al. (2018). Physiologically based pharmacokinetic modelling of atomoxetine with regard to CYP2D6 genotypes. Sci. Rep. 8 (1), 12405. doi:10.1038/s41598-018-30841-8
Kohane, I. S. (2015). Health care policy. ten things we have to do to achieve precision medicine. Science 349 (6243), 37–38. doi:10.1126/science.aab1328
Kratochvil, C. J., Newcorn, J. H., Arnold, L. E., Duesenberg, D., Emslie, G. J., Quintana, H., et al. (2005). Atomoxetine alone or combined with fluoxetine for treating ADHD with comorbid depressive or anxiety symptoms. J. Am. Acad. Child. Adolesc. Psychiatry 44 (9), 915–924. doi:10.1097/01.chi.0000169012.81536.38
LaBianca, S., Brikell, I., Helenius, D., Loughnan, R., Mefford, J., Palmer, C. E., et al. (2024). Polygenic profiles define aspects of clinical heterogeneity in attention deficit hyperactivity disorder. Nat. Genet. 56 (2), 234–244. doi:10.1038/s41588-023-01593-7
Lee, S. B., Wheeler, M. M., Patterson, K., McGee, S., Dalton, R., Woodahl, E. L., et al. (2019). Stargazer: a software tool for calling star alleles from next-generation sequencing data using CYP2D6 as a model. Genet. Med. 21 (2), 361–372. doi:10.1038/s41436-018-0054-0
Maciel, A., Cullors, A., Lukowiak, A. A., and Garces, J. (2018). Estimating cost savings of pharmacogenetic testing for depression in real-world clinical settings. Neuropsychiatr. Dis. Treat. 14, 225–230. doi:10.2147/NDT.S145046
Marchei, E., Papaseit, E., Garcia-Algar, O. Q., Farre, M., Pacifici, R., and Pichini, S. (2012). Determination of atomoxetine and its metabolites in conventional and non-conventional biological matrices by liquid chromatography-tandem mass spectrometry. J. Pharm. Biomed. Anal. 60, 26–31. doi:10.1016/j.jpba.2011.11.009
Martelli, L., Olivera, P., Roblin, X., Attar, A., and Peyrin-Biroulet, L. (2017). Cost-effectiveness of drug monitoring of anti-TNF therapy in inflammatory bowel disease and rheumatoid arthritis: a systematic review. J. Gastroenterol. 52 (1), 19–25. doi:10.1007/s00535-016-1266-1
Martin, J. (2024). Why are females less likely to be diagnosed with ADHD in childhood than males? Lancet Psychiatry 11 (4), 303–310. doi:10.1016/S2215-0366(24)00010-5
Matsui, A., Azuma, J., Witcher, J. W., Long, A. J., Sauer, J. M., Smith, B. P., et al. (2012). Pharmacokinetics, safety, and tolerability of atomoxetine and effect of CYP2D6*10/*10 genotype in healthy Japanese men. J. Clin. Pharmacol. 52 (3), 388–403. doi:10.1177/0091270011398657
Mbavha, B. T., Thelingwani, R. S., Chikwambi, Z., Nyakabau, A. M., Masimirembwa, C., the Consortium for, G., et al. (2023). Pharmacogenetics and pharmacokinetics of tamoxifen in a Zimbabwean breast cancer cohort. Br. J. Clin. Pharmacol. 89 (10), 3209–3216. doi:10.1111/bcp.15827
Mechler, K., Banaschewski, T., Hohmann, S., and Hage, A. (2022). Evidence-based pharmacological treatment options for ADHD in children and adolescents. Pharmacol. Ther. 230, 107940. doi:10.1016/j.pharmthera.2021.107940
Michelson, D., Read, H. A., Ruff, D. D., Witcher, J., Zhang, S., and McCracken, J. (2007). CYP2D6 and clinical response to atomoxetine in children and adolescents with ADHD. J. Am. Acad. Child. Adolesc. Psychiatry 46 (2), 242–251. doi:10.1097/01.chi.0000246056.83791.b6
Montoya, A., Escobar, R., Garcia-Polavieja, M. J., Lachno, D. R., Alda, J. A., Artigas, J., et al. (2011). Changes of urine dihydroxyphenylglycol to norepinephrine ratio in children with attention-deficit hyperactivity disorder (ADHD) treated with atomoxetine. J. Child. Neurol. 26 (1), 31–36. doi:10.1177/0883073810371981
Moric-Janiszewska, E., Smolik, S., Szydlowski, L., and Kapral, M. (2023). Associations between selected ADRB1 and CYP2D6 gene polymorphisms in children with ventricular and supraventricular arrhythmias. Med. Kaunas. 59 (12), 2057. doi:10.3390/medicina59122057
Morris, S. A., Alsaidi, A. T., Verbyla, A., Cruz, A., Macfarlane, C., Bauer, J., et al. (2022). Cost effectiveness of pharmacogenetic testing for drugs with clinical pharmacogenetics implementation Consortium (CPIC) guidelines: a systematic review. Clin. Pharmacol. Ther. 112 (6), 1318–1328. doi:10.1002/cpt.2754
Mullen, J. H., Shugert, R. L., Ponsler, G. D., Li, Q., Sundaram, B., Coales, H. L., et al. (2005). Simultaneous quantification of atomoxetine as well as its primary oxidative and O-glucuronide metabolites in human plasma and urine using liquid chromatography tandem mass spectrometry (LC/MS/MS). J. Pharm. Biomed. Anal. 38 (4), 720–733. doi:10.1016/j.jpba.2005.02.007
Nijenhuis, M., Soree, B., Jama, W. O. M., de Boer-Veger, N. J., Buunk, A. M., Guchelaar, H. J., et al. (2023). Dutch pharmacogenetics working group (DPWG) guideline for the gene-drug interaction of CYP2D6 and COMT with atomoxetine and methylphenidate. Eur. J. Hum. Genet. 31 (12), 1364–1370. doi:10.1038/s41431-022-01262-z
Nofziger, C., Turner, A. J., Sangkuhl, K., Whirl-Carrillo, M., Agundez, J. A. G., Black, J. L., et al. (2020). PharmVar GeneFocus: CYP2D6. Clin. Pharmacol. Ther. 107 (1), 154–170. doi:10.1002/cpt.1643
Notsu, Y., Shimizu, M., Sasaki, T., Nakano, A., Ota, M., Yoshida, S., et al. (2020). Simple pharmacokinetic models accounting for drug monitoring results of atomoxetine and its 4-hydroxylated metabolites in Japanese pediatric patients genotyped for cytochrome P450 2D6. Drug Metab. Pharmacokinet. 35 (2), 191–200. doi:10.1016/j.dmpk.2019.08.005
Numanagic, I., Malikic, S., Ford, M., Qin, X., Toji, L., Radovich, M., et al. (2018). Allelic decomposition and exact genotyping of highly polymorphic and structurally variant genes. Nat. Commun. 9 (1), 828. doi:10.1038/s41467-018-03273-1
Numanagic, I., Malikic, S., Pratt, V. M., Skaar, T. C., Flockhart, D. A., and Sahinalp, S. C. (2015). Cypiripi: exact genotyping of CYP2D6 using high-throughput sequencing data. Bioinformatics 31 (12), i27–i34. doi:10.1093/bioinformatics/btv232
Papaseit, E., Marchei, E., Farre, M., Garcia-Algar, O., Pacifici, R., and Pichini, S. (2013). Concentrations of atomoxetine and its metabolites in plasma and oral fluid from paediatric patients with attention deficit/hyperactivity disorder. Drug Test. Anal. 5 (6), 446–452. doi:10.1002/dta.1370
Papaseit, E., Marchei, E., Mortali, C., Aznar, G., Garcia-Algar, O., Farre, M., et al. (2012). Development and validation of a liquid chromatography-tandem mass spectrometry assay for hair analysis of atomoxetine and its metabolites: application in clinical practice. Forensic Sci. Int. 218 (1-3), 62–67. doi:10.1016/j.forsciint.2011.10.012
Patel, C., Patel, M., Rani, S., Nivsarkar, M., and Padh, H. (2007). A new high performance liquid chromatographic method for quantification of atomoxetine in human plasma and its application for pharmacokinetic study. J. Chromatogr. B Anal. Technol. Biomed. Life Sci. 850 (1-2), 356–360. doi:10.1016/j.jchromb.2006.12.011
Paulzen, M., Haen, E., Stegmann, B., Hiemke, C., Grunder, G., Lammertz, S. E., et al. (2016). Body mass index (BMI) but not body weight is associated with changes in the metabolism of risperidone; A pharmacokinetics-based hypothesis. Psychoneuroendocrinology 73, 9–15. doi:10.1016/j.psyneuen.2016.07.009
Posner, J., Polanczyk, G. V., and Sonuga-Barke, E. (2020). Attention-deficit hyperactivity disorder. Lancet 395 (10222), 450–462. doi:10.1016/S0140-6736(19)33004-1
Pratt, V. M., Cavallari, L. H., Del Tredici, A. L., Gaedigk, A., Hachad, H., Ji, Y., et al. (2021). Recommendations for clinical CYP2D6 genotyping allele selection: a Joint consensus recommendation of the association for molecular Pathology, College of American Pathologists, Dutch pharmacogenetics working group of the royal Dutch Pharmacists association, and the European society for pharmacogenomics and personalized therapy. J. Mol. Diagn 23 (9), 1047–1064. doi:10.1016/j.jmoldx.2021.05.013
QuickStats (2024). Percentage* of children and adolescents aged 5-17 Years who had ever received a diagnosis of attention-deficit/hyperactivity disorder, dagger by urbanization level(section sign) and age group - national health Interview survey, United States, 2020-2022(paragraph sign). MMWR Morb. Mortal. Wkly. Rep. 73 (5), 116. doi:10.15585/mmwr.mm7305a6
Raman, S. R., Man, K. K. C., Bahmanyar, S., Berard, A., Bilder, S., Boukhris, T., et al. (2018). Trends in attention-deficit hyperactivity disorder medication use: a retrospective observational study using population-based databases. Lancet Psychiatry 5 (10), 824–835. doi:10.1016/S2215-0366(18)30293-1
Ramos-Quiroga, J. A., Corominas-Roso, M., Palomar, G., Gomez-Barros, N., Ribases, M., Sanchez-Mora, C., et al. (2014). Changes in the serum levels of brain-derived neurotrophic factor in adults with attention deficit hyperactivity disorder after treatment with atomoxetine. Psychopharmacol. Berl. 231 (7), 1389–1395. doi:10.1007/s00213-013-3343-y
Ring, B. J., Gillespie, J. S., Eckstein, J. A., and Wrighton, S. A. (2002). Identification of the human cytochromes P450 responsible for atomoxetine metabolism. Drug Metab. Dispos. 30 (3), 319–323. doi:10.1124/dmd.30.3.319
Roesch, B., Corcoran, M. E., Fetterolf, J., Haffey, M., Martin, P., Preston, P., et al. (2013). Pharmacokinetics of coadministered guanfacine extended release and lisdexamfetamine dimesylate. Drugs R. D. 13 (2), 119–128. doi:10.1007/s40268-013-0014-8
Rogers, M. A., and MacLean, J. (2023). ADHD symptoms increased during the covid-19 pandemic: a meta-analysis. J. Atten. Disord. 27 (8), 800–811. doi:10.1177/10870547231158750
Rosenbaum, P. (2020). Visual Function Classification System for children with cerebral palsy: development of a new tool. Dev. Med. Child. Neurol. 62 (1), 14. doi:10.1111/dmcn.14279
Rudesheim, S., Selzer, D., Murdter, T., Igel, S., Kerb, R., Schwab, M., et al. (2022). Physiologically based pharmacokinetic modeling to describe the CYP2D6 activity score-dependent metabolism of paroxetine, atomoxetine and risperidone. Pharmaceutics 14 (8), 1734. doi:10.3390/pharmaceutics14081734
Ruppert, K., Geffert, C., Clement, H. W., Bachmann, C., Haberhausen, M., Schulz, E., et al. (2022). Therapeutic drug monitoring of atomoxetine in children and adolescents with attention-deficit/hyperactivity disorder: a naturalistic study. J. Neural Transm. (Vienna) 129 (7), 945–959. doi:10.1007/s00702-022-02483-8
Sauer, J. M., Long, A. J., Ring, B., Gillespie, J. S., Sanburn, N. P., DeSante, K. A., et al. (2004). Atomoxetine hydrochloride: clinical drug-drug interaction prediction and outcome. J. Pharmacol. Exp. Ther. 308 (2), 410–418. doi:10.1124/jpet.103.058727
Sauer, J. M., Ponsler, G. D., Mattiuz, E. L., Long, A. J., Witcher, J. W., Thomasson, H. R., et al. (2003). Disposition and metabolic fate of atomoxetine hydrochloride: the role of CYP2D6 in human disposition and metabolism. Drug Metab. Dispos. 31 (1), 98–107. doi:10.1124/dmd.31.1.98
Sauer, J. M., Ring, B. J., and Witcher, J. W. (2005). Clinical pharmacokinetics of atomoxetine. Clin. Pharmacokinet. 44 (6), 571–590. doi:10.2165/00003088-200544060-00002
Schafer, A., Hiemke, C., and Baumann, P. (2016). Consensus guideline for therapeutic drug monitoring in psychiatry (2004): bibliometric analysis of citations for the period 2004-2011. Nord. J. Psychiatry 70 (3), 202–207. doi:10.3109/08039488.2015.1080296
Schoretsanitis, G., de Leon, J., Eap, C. B., Kane, J. M., and Paulzen, M. (2019). Clinically significant drug-drug interactions with agents for attention-deficit/hyperactivity disorder. CNS Drugs 33 (12), 1201–1222. doi:10.1007/s40263-019-00683-7
Shang, D. W., Guo, W., Zhou, F. C., Wang, X. P., Li, A. N., Zhang, L., et al. (2013). Relative bioequivalence evaluation of two oral atomoxetine hydrochloride capsules: a single dose, randomized, open-label, 2-period crossover study in healthy Chinese volunteers under fasting conditions. Drug Res. (Stuttg) 63 (11), 564–567. doi:10.1055/s-0033-1349070
Shimizu, M., Uehara, S., Ohyama, K., Nishimura, H., Tanaka, Y., Saito, Y., et al. (2023). NiP450 2D6</b>. Drug Metab. Dispos. 52, 35–43. doi:10.1124/dmd.123.001481
Sim, J., Kim, E., Yang, W., Woo, S., and In, S. (2017). An LC-MS/MS method for the simultaneous determination of 15 antipsychotics and two metabolites in hair and its application to rat hair. Forensic Sci. Int. 274, 91–98. doi:10.1016/j.forsciint.2017.01.001
Siqueira, J. F., Fouad, A. F., and Rocas, I. N. (2012). Pyrosequencing as a tool for better understanding of human microbiomes. J. Oral Microbiol. 4, 10743. doi:10.3402/jom.v4i0.10743
Skaalvik, T. G., Oiestad, E. L., Trones, R., Pedersen-Bjergaard, S., and Hegstad, S. (2021). Determination of psychoactive drugs in serum using conductive vial electromembrane extraction combined with UHPLC-MS/MS. J. Chromatogr. B Anal. Technol. Biomed. Life Sci. 1183, 122926. doi:10.1016/j.jchromb.2021.122926
Spiller, H. A., Hays, H. L., and Aleguas, A. (2013). Overdose of drugs for attention-deficit hyperactivity disorder: clinical presentation, mechanisms of toxicity, and management. CNS Drugs 27 (7), 531–543. doi:10.1007/s40263-013-0084-8
Stegmann, B., Dorfelt, A., and Haen, E. (2016). Quantification of methylphenidate, dexamphetamine, and atomoxetine in human serum and oral fluid by HPLC with fluorescence detection. Ther. Drug Monit. 38 (1), 98–107. doi:10.1097/FTD.0000000000000245
Subspecialty Group of D (2020). Consensus on pediatric clinical practice of early identification, standardized diagnosis and treatment of attention deficit hyperactivity disorder. Zhonghua Er Ke Za Zhi 58 (3), 188–193. doi:10.3760/cma.j.issn.0578-1310.2020.03.006
Sugimoto, A., Suzuki, Y., Orime, N., Hayashi, T., Yoshinaga, K., Egawa, J., et al. (2021). The lowest effective plasma concentration of atomoxetine in pediatric patients with attention deficit/hyperactivity disorder: a non-randomized prospective interventional study. Med. Baltim. 100 (27), e26552. doi:10.1097/MD.0000000000026552
Taylor, C., Crosby, I., Yip, V., Maguire, P., Pirmohamed, M., and Turner, R. M. (2020). A review of the important role of CYP2D6 in pharmacogenomics. Genes. (Basel) 11 (11), 1295. doi:10.3390/genes11111295
Teichert, J., Rowe, J. B., Ersche, K. D., Skandali, N., Sacher, J., Aigner, A., et al. (2020). Determination of atomoxetine or escitalopram in human plasma by HPLC: applications in neuroscience research studies. Int. J. Clin. Pharmacol. Ther. 58 (8), 426–438. doi:10.5414/CP203705
Terao, I., Kodama, W., and Tsuda, H. (2024). The dose-response relationship of atomoxetine for the treatment of children with ADHD: a systematic review and dose-response meta-analysis of double-blind randomized placebo-controlled trials. J. Atten. Disord. 28 (4), 431–438. doi:10.1177/10870547231214988
ter Laak, M. A., Temmink, A. H., Koeken, A., van 't Veer, N. E., van Hattum, P. R., and Cobbaert, C. M. (2010). Recognition of impaired atomoxetine metabolism because of low CYP2D6 activity. Pediatr. Neurol. 43 (3), 159–162. doi:10.1016/j.pediatrneurol.2010.04.004
Tian, X., Liu, X., Wang, Y., Liu, Y., Ma, J., Sun, H., et al. (2022). Urinary metabolomic study in a healthy children population and metabolic biomarker discovery of attention-deficit/hyperactivity disorder (ADHD). Front. Psychiatry 13, 819498. doi:10.3389/fpsyt.2022.819498
Todor, I., Popa, A., Neag, M., Muntean, D., Bocsan, C., Buzoianu, A., et al. (2016). Evaluation of a potential metabolism-mediated drug-drug interaction between atomoxetine and bupropion in healthy volunteers. J. Pharm. Pharm. Sci. 19 (2), 198–207. doi:10.18433/J3H03R
Todor, I., Popa, A., Neag, M., Muntean, D., Bocsan, C., Buzoianu, A., et al. (2017). Evaluation of the potential pharmacokinetic interaction between atomoxetine and fluvoxamine in healthy volunteers. Pharmacology 99 (1-2), 84–88. doi:10.1159/000452223
Touw, D. J., Neef, C., Thomson, A. H., and Vinks, A. A. (2005). Cost-effectiveness of therapeutic drug monitoring committee of the international association for therapeutic drug M, clinical T. Cost-Effectiveness of therapeutic drug monitoring: a systematic review. Ther. Drug Monit. 27 (1), 10–17. doi:10.1097/00007691-200502000-00004
Trzepacz, P. T., Williams, D. W., Feldman, P. D., Wrishko, R. E., Witcher, J. W., and Buitelaar, J. K. (2008). CYP2D6 metabolizer status and atomoxetine dosing in children and adolescents with ADHD. Eur. Neuropsychopharmacol. 18 (2), 79–86. doi:10.1016/j.euroneuro.2007.06.002
Twist, G. P., Gaedigk, A., Miller, N. A., Farrow, E. G., Willig, L. K., Dinwiddie, D. L., et al. (2016). Constellation: a tool for rapid, automated phenotype assignment of a highly polymorphic pharmacogene, CYP2D6, from whole-genome sequences. NPJ Genom Med. 1, 15007. doi:10.1038/npjgenmed.2015.7
Ulrich, S., Hiemke, C., Laux, G., Muller-Oerlinghausen, B., Havemann-Reinecke, U., Riederer, P., et al. (2007). Value and actuality of the prescription information for therapeutic drug monitoring of psychopharmaceuticals: a comparison with the medico-scientific evidence. Pharmacopsychiatry 40 (3), 121–127. doi:10.1055/s-2007-977712
Van Vyve, L., Dierckx, B., Lim, C. G., Danckaerts, M., Koch, B. C. P., Hage, A., et al. (2024). Pharmacotherapy for ADHD in children and adolescents: a summary and overview of different European guidelines. Eur. J. Pediatr. 183 (3), 1047–1056. doi:10.1007/s00431-023-05370-w
Vertessen, K., Luman, M., and Oosterlaan, J. (2024). ADHD medication adherence: the importance of adequate prescribing. Lancet Psychiatry 11 (1), 2–3. doi:10.1016/S2215-0366(23)00393-0
Vithanachchi, D. T., Maujean, A., Downes, M. J., and Scuffham, P. (2021). A comprehensive review of economic evaluations of therapeutic drug monitoring interventions for cancer treatments. Br. J. Clin. Pharmacol. 87 (2), 271–283. doi:10.1111/bcp.14494
Wang, L. J., Chou, W. J., Tsai, C. S., Lee, M. J., Lee, S. Y., Hsu, C. W., et al. (2021). Novel plasma metabolite markers of attention-deficit/hyperactivity disorder identified using high-performance chemical isotope labelling-based liquid chromatography-mass spectrometry. World J. Biol. Psychiatry 22 (2), 139–148. doi:10.1080/15622975.2020.1762930
Witcher, J. W., Long, A., Smith, B., Sauer, J. M., Heilgenstein, J., Wilens, T., et al. (2003). Atomoxetine pharmacokinetics in children and adolescents with attention deficit hyperactivity disorder. J. Child. Adolesc. Psychopharmacol. 13 (1), 53–63. doi:10.1089/104454603321666199
Wong, I. C. K., Banaschewski, T., Buitelaar, J., Cortese, S., Dopfner, M., Simonoff, E., et al. (2019). Emerging challenges in pharmacotherapy research on attention-deficit hyperactivity disorder-outcome measures beyond symptom control and clinical trials. Lancet Psychiatry 6 (6), 528–537. doi:10.1016/s2215-0366(19)30096-3
Wu, W. W., Guo, H. L., Li, Y., Hu, Y. H., He, H., Xu, J., et al. (2024). A national-wide survey on clinical implementation of PGx testing into precision therapeutics for Chinese children: a long way before standard clinical practice. BMC Health Serv. Res. 24 (1), 1089. doi:10.1186/s12913-024-11535-6
Xia, Y., Guo, H. L., Hu, Y. H., Long, J. Y., Chen, J., Chen, F., et al. (2021). Determination of atomoxetine levels in human plasma using LC-MS/MS and clinical application to Chinese children with ADHD based on CPIC guidelines. Anal. Methods 13 (21), 2434–2441. doi:10.1039/d1ay00521a
Yang, L., Qian, Q., Liu, L., Li, H., Faraone, S. V., and Wang, Y. (2013). Adrenergic neurotransmitter system transporter and receptor genes associated with atomoxetine response in attention-deficit hyperactivity disorder children. J. Neural Transm. (Vienna) 120 (7), 1127–1133. doi:10.1007/s00702-012-0955-z
Zeng, D., Huang, X., Lin, S., Lin, R., Weng, X., and Huang, P. (2021). Cost-effectiveness analysis of genotype screening and therapeutic drug monitoring in patients with inflammatory bowel disease treated with azathioprine therapy: a Chinese healthcare perspective using real-world data. Ann. Transl. Med. 9 (14), 1138. doi:10.21037/atm-21-1980
Zhu, H. J., Wang, J. S., Donovan, J. L., DeVane, C. L., Gibson, B. B., and Markowitz, J. S. (2007). Sensitive quantification of atomoxetine in human plasma by HPLC with fluorescence detection using 4-(4,5-diphenyl-1H-imidazole-2-yl) benzoyl chloride derivatization. J. Chromatogr. B Anal. Technol. Biomed. Life Sci. 846 (1-2), 351–354. doi:10.1016/j.jchromb.2006.08.019
Zhu, Y., Moriarty, J. P., Swanson, K. M., Takahashi, P. Y., Bielinski, S. J., Weinshilboum, R., et al. (2021). A model-based cost-effectiveness analysis of pharmacogenomic panel testing in cardiovascular disease management: preemptive, reactive, or none? Genet. Med. 23 (3), 461–470. doi:10.1038/s41436-020-00995-w
Keywords: atomoxetine, attention deficit/hyperactivity disorder (ADHD), children, therapeutic drug monitoring (TDM), CYP2D6, inter-individual variability, precision pharmacotherapy, biomarkers
Citation: Guo H-L, Huang J, Wang J, Fan L, Li Y, Wu D-D, Liu Q-Q and Chen F (2024) Precision pharmacotherapy of atomoxetine in children with ADHD: how to ensure the right dose for the right person?. Front. Pharmacol. 15:1484512. doi: 10.3389/fphar.2024.1484512
Received: 22 August 2024; Accepted: 11 October 2024;
Published: 29 October 2024.
Edited by:
Wei Zhao, Shandong University, ChinaReviewed by:
Catherine M. T. Sherwin, University of Western Australia, AustraliaKristen Ward, University of Michigan, United States
Copyright © 2024 Guo, Huang, Wang, Fan, Li, Wu, Liu and Chen. This is an open-access article distributed under the terms of the Creative Commons Attribution License (CC BY). The use, distribution or reproduction in other forums is permitted, provided the original author(s) and the copyright owner(s) are credited and that the original publication in this journal is cited, in accordance with accepted academic practice. No use, distribution or reproduction is permitted which does not comply with these terms.
*Correspondence: Feng Chen, cy.chen508@gmail.com
†These authors have contributed equally to this work