- 1College of Pharmacy and Meishan Hospital, Chengdu University of Traditional Chinese Medicine, Chengdu, China
- 2School of Ethmic Medicine, Chengdu University of Taditional Chinese Medicine, Chengdu, Sichuan, China
- 3Research Institute of Integrated TCM and Western Medicine, Chengdu University of Traditional Chinese Medicine, Chengdu, China
Terminalia chebula Retz. (T. Chebula, ཨ་རུ་ར།) is highly utilized in ethnic medicine. Its medicinal value is gradually being recognized and shows great potential in the improvement of mild cognitive impairment (MCI) disorders. Tibetan medicine theory classifies this type of disease as one of the “Jie Xie Syndrome (བརྗེད་བྱེད།).” The role of T. Chebula in such diseases has been increasingly studied. This work aimed to elucidate the research progress of T. Chebula in alleviating MCI. The review offers a critical update on the current understanding of the effect of T. Chebula on MCI and highlights new opportunities for exploring its therapeutic potential. This review discusses the role of T. Chebula in alleviating MCI and provides a comprehensive overview of the traditional medicinal uses, chemical composition, toxicology, and quality control aspects of T. Chebula. This review covers 171 chemical constituents and 11 active constituents targeting MCI, such as flavonoids, which can alleviate MCI, primarily through its antioxidative, anti-inflammatory, and neuroprotective properties. T. Chebula shows potential as a natural medicine for the treatment and prevention of MCI. As an important part of ethnomedicinal resources, this work offers valuable insights for future research on T. Chebula-containing ethnomedicines. Research on traditional drug treatments, optimized treatment standards, improved societal knowledge about MCI, and development of an early detection system is essential to the diagnosis and treatment of MCI. These efforts will provide better treatment resources for patients with MCI.
Highlights
• Terminalia chebula has antioxidant, anti-inflammatory, repairing synaptic plasticity damage and neuroprotective properties for the treatment and prevention of mild cognitive impairment (MCI).
• Terminalia chebula is able to improve MCI.
• This review will be helpful for the succession and development of Tibetan medicine.
1 Introduction
Population aging is a significant aspect of social development and reflects the progress of human civilization. It has become a foundational national condition in China (Zhabokritsky et al., 2023). With the growing elderly population, cognitive disorders, such as dementia, have become a leading cause of death among seniors and present considerable health, medical, and socio-economic challenges (Bode et al., 2023; Ellen et al., 2017). Mild cognitive impairment (MCI), an intermediate stage between normal aging and dementia, involves a progressive decline in memory and cognitive function without meeting dementia criteria and poses a high risk for the progression of Alzheimer’s disease if left untreated (Petersen, 2011). Moreover, residing in high-altitude, low-oxygen environments, such as Tibet, can induce cognitive dysfunction and structural brain changes, especially above 4,000 m (Liu et al., 2023).
Tibetan medicine, an ancient tradition, has evolved into a distinct theoretical system over millennia (Dakpa, 2014). Understanding MCI through ethnomedical theories could complement modern clinical treatments. In Tibetan medicine, MCI is classified as “amnesia,” categorized under “Jie Xie Syndrome,” which is described in classic texts, such as Four Medical Tantras and the Blue Beryl and attributed to disturbances in “Long,” including a weak heart, excessive worry, and anxiety. Tibetan medicine addresses age-related diseases by preventing aging and tonifying Yang to regulate “Long” disorders and restore the body’s balance (Zhuoma and Renqing, 2020). The complexity of the etiology of MCI often complicates Western medical treatments, making them variable in efficacy and prone to adverse effects. Incorporating ethnomedical theories could aid in identifying effective ethnomedicines and potentially enhance MCI drug development (Yangmao, 2017; Campbell et al., 2018; Kessler et al., 2014; Lapeyre-Mestre, 2016; Matsunaga et al., 2019; Wang et al., 2022; Zhao et al., 2022).
Terminalia chebula Retz. (T. Chebula, ཨ་རུ་ར།), a prominent Tibetan medicinal herb, is renowned for its diverse therapeutic properties, including dispelling wind (qi), promoting blood circulation, and relieving toxicity. It is used for various conditions, from wind–heat rash to chronic diarrhea and epilepsy (Liu et al., 2018; Yang et al., 2017). Modern pharmacological studies have highlighted its antibacterial, antioxidant, hypoglycemic, antiviral, anti-inflammatory, and antitumor properties, thereby supporting its therapeutic potential, particularly in antioxidant and antidiabetic activities (Zhang et al., 2016).
While extensive previous research has detailed the pharmacological activities of Terminalia chebula (T. Chebula), there are not enough studies on its role in alleviating mild cognitive impairment. This review systematically elucidates the role of T. Chebula in mitigating MCI and provides a comprehensive overview of its traditional medicinal uses, chemical composition, toxicology, and quality control aspects. We emphasize pharmacological mechanisms and future research trends regarding T. Chebula’s potential as a natural medicine for the treatment and prevention of MCI. A thorough literature search was conducted across multiple databases, including CNKI, PubMed, and Web of Science, from January 1990 to March 2024, focusing on the chemical components and pharmacological activities of T. Chebula. The review highlights T. Chebula’s promise in alleviating MCI symptoms, offering essential insights and directions for the future development and application of T. Chebula in MCI treatment.
2 Traditional uses
Traditional Tibetan medicine, T. chebula, refers to the dried fruit of a plant in the Combretaceae family. It is primarily grown in Malaysia, India, and Myanmar and is also found in various regions of China, including Yunnan, Tibet, Guangdong, and Guangxi (Ekambaram et al., 2020) (Figure 1). It has a few common names such as dark myrobalan, ink tree, or chebulic myrobalan (English), haritaki (Sanskrit and Bengali), Harad (Hindi), Harada (Marathi and Gujrati), Karkchettu (Telgu), and Kadukkaya (Tamil). It is notable as “haritaki” in Tibet. The synonyms of T. Chebula inciuding T. parviflora Thwaites, T. reticulate Roth, T. tomentella Kurz, T. aruta Buch. -Ham., ex G. Don, T. zeylannica Van Heurck and Muell. Arg (Bulbul et al., 2022). In Tibetan medicine, T. Chebula is typically combined with other herbs to treat various diseases. T. Chebula is extensively cited in ethnomedicine texts and is described in “Du Mu Ben Cao” as having thick leaves, with the fruit differing in traits and morphology; as such, it is classified into eight, seven, or five types. In Tibet, the dried fruit of T. Chebula is categorized into five kinds due to distinctive characteristics and is commonly used medicinally. Therapeutic and practical uses can vary depending on the processing method. Modern preparation methods include removing the seed, utilizing the pulp, and stir frying with either soil or bran (Rao and Nammi, 2006). T. Chebula is used to treat asthma, bronchitis, hepatitis, dyspepsia, eye diseases, hoarseness, and promote hair growth. The flesh of the plant has been used for the treatment of diarrhea, leprosy, and edema. It improves appetite, reduces cholesterol and blood pressure, strengthen the immune system, prevent aging, and enhance resistance against infections (Gupta et al., 2020). In clinical use, the therapeutic effects of specific preparations can be customized to treat various diseases and optimized by combining them according to distinct symptoms of cold and heat. Traditionally, T. Chebula is typically ingested as pills, powders, or decoctions (Table 1). In the Four Medical Tantras, T. Chebula is specifically described for its use in treating “Jie Xie Syndrome” (Feng et al., 2019).
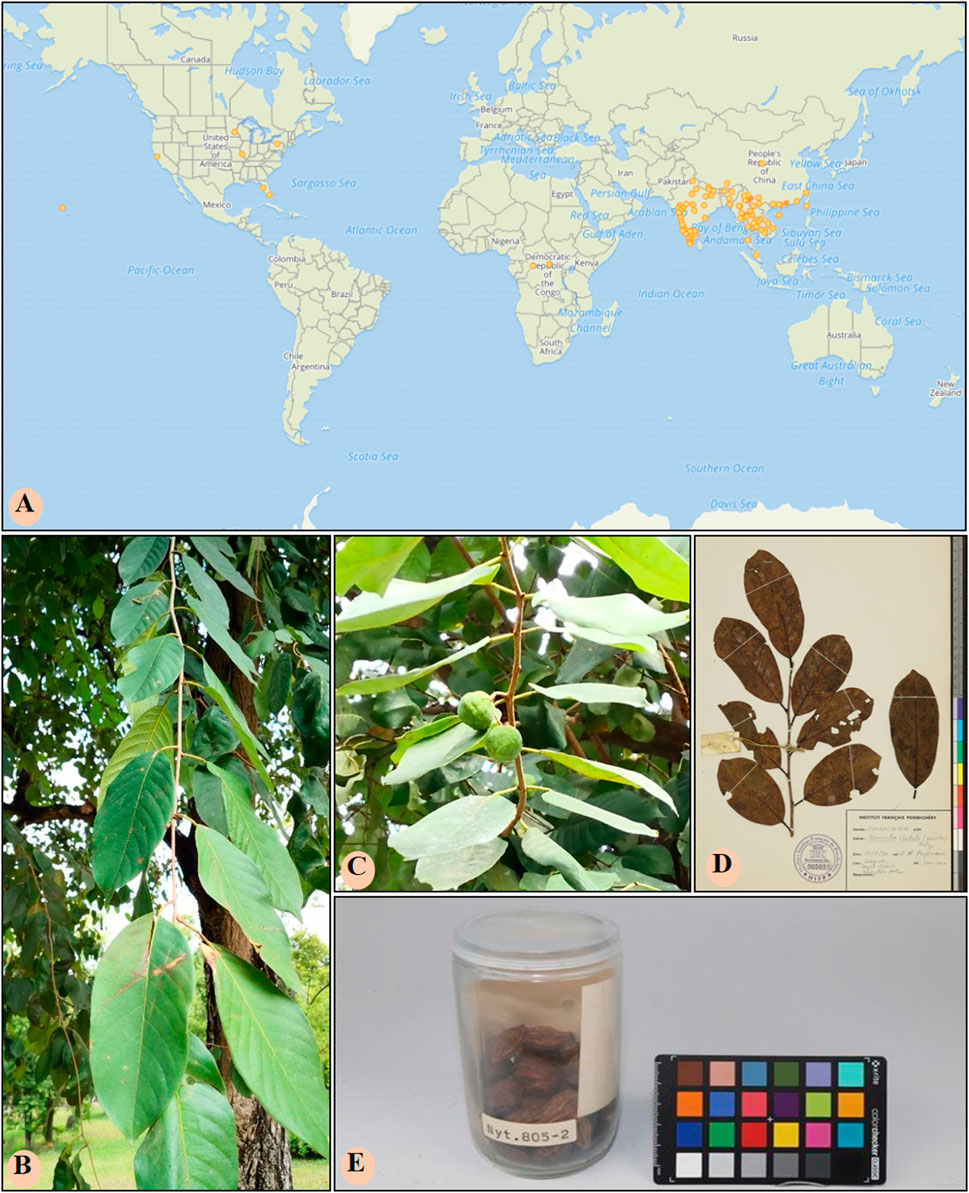
Figure 1. The geographical distribution and botanical morphology of T. chebula. (A) The geographical distribution of T. chebula in the world. (B) The leaf of T. chebula. (C) The fruit of T. chebula. (D) Specimen diagram of T. chebula leaves. (E) Specimen diagram of T. chebula fruit. The above pictures were all accessed through GBIF.org (https://www.gbif.org/) on 2023-05-22.
3 Phytochemistry
T. Chebula contains a rich and diverse array of chemical constituents, which primarily include phenolic acids, tannins, triterpenoids, flavonoids, and volatile oils. A total of 171 compounds have been isolated from T. Chebula and comprise 83 tannins, 16 phenolic acids, 6 flavonoids, 29 triterpenoids, 17 volatile compounds, and 20 other compounds. This review focuses on the chemical constituents of T. Chebula and their potential role in improving the symptoms of MCI (Table 2).
3.1 Tannin-based
Tannins are polyphenolic compounds classified into hydrolyzable and condensed types, with gallic acid and glucose as their structural units. T. Chebula contains a substantial amount of tannin compounds, comprising 23.6%–37.36% of its composition. A total of 83 tannin compounds have been extracted and isolated from T. Chebula (Reddy et al., 1994; Li et al., 2019). Seven polyphenolic compounds were isolated from T. Chebula (Ding et al., 2001). Ellagic acid 59, terchebin 60, chebulinic acid 61, corilagin 29, and punicalagin 39 were isolated from T. Chebula (Lin et al., 1990). Chebulagic acid 69 and terchebulin 70 were identified from the ethyl acetate extract of T. Chebula (Lee et al., 2007). The components currently isolated from T. chebula are glucogallin 71, casuarinin 72, chebulanin 11, chebumeinin B 73, punicalagin A 75, and punicalagin B 77 (Jeong et al., 2019; Yao et al., 2023; Zhao et al., 2022). Some of these key active ingredients play an important role in improving MCI (Figures 2–4).
3.2 Phenolic acid
T. Chebula contains a large amount of phenolic acids. These compounds contain a large number of active sites in their structure and have a variety of biopharmacological activities, such as anti-inflammatory, antiviral and immunomodulatory effects, etc. Phenolic compounds are also excellent antioxidants and their phenolic hydroxyl groups have obvious scavenging effects on free radicals such as peroxyl radicals (OOH) and hydroxyl radicals (OH), which can cause structural and functional damage to the membranes of biological tissues due to peroxidation (Chang and Lin, 2012). T. Chebula contains trihydroxybenzoic acid and dihydroxybenzoic acid (Walia et al., 2011). A total of 16 phenolic acids have been compiled (84–99). Ethyl gallate 84, shikimic acid 85, methyl shikimate 87, trans-cinnamic acid 88, and 2,4-Dihydroxybenzoic acid 91 were isolated from T. Chebula (Zhi et al., 2020). Quinic acid 99 was also identified. Furthermore, 11-methyl chebulate 95, 13-methyl chebulate 96, 11,12-dimethylchebulate 97, and brervifolincaboxylic acid 98 were isolated from T. Chebula (Pfundstein et al., 2010). Other compounds were also isolated from T. Chebula (Lu et al., 1991) (Figure 5).
3.3 Triterpenoid
T. Chebula mainly contains pentacyclic triterpenes and their glycoside components. Triterpene glycosides typically feature hydroxyl groups at C-2 and C-3, angular methyl groups at C-8, C-10, and C-14, and double bonds at C-12 and C-13. Triterpenoids exhibit a range of biological activities, including hypoglycemic, anti-tumor, antioxidant, hepatoprotective, antibacterial, renal, and immune system-regulating effects. These compounds demonstrate neuroprotective properties through various molecular mechanisms, encompassing the modulation of neuroinflammation, oxidative stress, and autophagy. Furthermore, they may mitigate neurological disorders by improving mitochondrial function and inhibiting endoplasmic reticulum stress (Liu et al., 2012). A total of 29 triterpenoids (100–128) have been identified. Triterpenoids isolated from T. Chebula fruits include arjunic acid 109, arjugenin 112, chebupentol 103, maslinic acid 116, 2α-hydroxyursolic acid 115, and daucosterol 118 (Manosroi et al., 2010) (Figures 6, 7).
3.4 Flavonoid
The majority of flavonoids are derivatives containing hydroxyl groups and often have methoxy or other substituents on the parent nucleus. Seven major flavonoid compounds (129–134) have been identified, including quercetin (129), quercetin-3-O-rhamnoside (130), and rutin (131). Components 130, 131, and 132 were isolated from the fruits of T. chebula (Tang et al., 2012). Several in vitro studies have demonstrated the neuroprotective effects of compound 129 and its potential to enhance cognitive performance clinically (Khan et al., 2019) (Figure 8).
3.5 Volatile
Volatile components primarily consist of fatty acids. T. Chebula also contains volatile components. A total of 16 volatile components have been identified (135–151), such as tetradecanoic acid 135, palmitic acid 136, linoleic acid 137, oleic acid 138, and octadecadienoic acid 139 (Jokar et al., 2016). Gas–mass spectrometry was employed to analyze the volatile components of T. Chebula (Lin et al., 1996) (Figure 9).
3.6 Other components
The 19 other compounds (152–171) found in T. Chebula include amino acid compounds such as L-aspartic acid 152, L-glutamic acid 153, L-arginine 154, L-lysine 155, and proline 156; sugar compounds such as fructose 157, glucose 158, sucrose 159, β-arabinose 160, and rhamnose 161; aliphatic compounds such as mannitol 162, tricarboxylic acid 163, soft fatty acids 164, and carotene 165 (Rai and Joshi, 2009; Nigam et al., 2020) (Figure 10).
4 Pharmacological activity of T. Chebula to improve MCI
Various components of T. Chebula possess multiple pharmacological activities, mainly including anti-inflammatory, antioxidant, neuroprotective, cognitive enhancement, antimicrobial, anti-tumor, and angiogenesis effects (Bulbul et al., 2022) (Figure 11). The pharmacological effects aimed at improving MCI symptoms are particularly notable (Tables 3, 4).
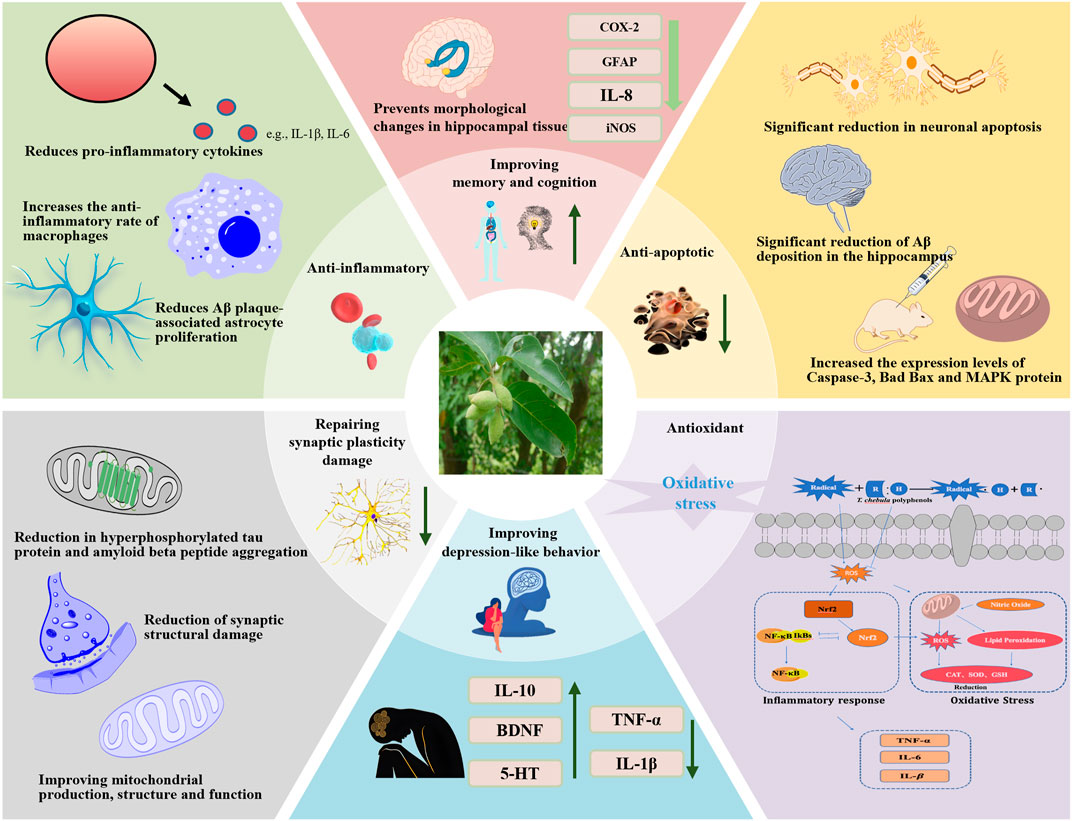
Figure 11. Molecular mechanism of MCI alleviation by T. chebula. Abbreviations: IL-6, interleukin-6; IL-1β, interleukin-1β; TNF-α, tumor necrosis factor-α; ROS, reactive oxygen species; Nrf2, nuclear factor erythroid 2-related factor 2; CAT, catalase; SOD, superoxide dismutase; GSH, Glutathione; NF-κB, nuclear transcription factor-κB; MAPK, mitogen-activated protein kinase.
4.1 Improvement of depression-like behavior
MCI and depression are closely related, and depression and anxiety symptoms are considered to be important risk factors for the progression of MCI to dementia (Dean et al., 2014). About 35%–85% of patients with MCI have neuropsychiatric symptoms in addition to cognitive impairment (Gallagher et al., 2017). Neuropsychiatric symptoms include depression, apathy, anxiety, and irritability, with depression and anxiety symptoms as the most common. Patients with MCI are more likely to be depressed than those with normal cognitive function, with depressive symptoms occurring in 26.3% and 18.0%, respectively (Dooley et al., 2020; Hidaka et al., 2012). Patients with MCI combined with a depressive mood have severe behavioral symptoms and verbally provocative behavior (Van der Mussele et al., 2013). Therefore, the assessment of depressive and anxiety symptoms and early intervention in patients with MCI are of great importance to prevent the deterioration of MCI and prevent dementia (Wiesli et al., 2017). The antidepressant-like and anxiolytic-like effects of an ethanolic extract of T. Chebula fruit were studied, and test results revealed significant improvements in mice (Mani et al., 2021). Additionally, the acute anxiolytic activity of an aqueous extract of T. Chebula was evaluated; the findings showed that the extract reduced fatigue, increased exploratory behavior, and relieved anxiety, thereby performing comparably with the standard anxiolytic drug valium (Chandrashekar et al., 2013). Furthermore, the antidepressant activity and mechanistic effects of the aqueous extract of T. Chebula were investigated; the results demonstrated that higher doses of the extract exhibited antidepressant activity. This outcome is likely mediated through the monoaminergic pathway (Dattatray et al., 2014). T. Chebula contains ellagic acid 59, a rich source of polyphenolic di-lactones with significant anti-inflammatory and neuroimmunomodulatory activities. The antidepressant effect of ellagic acid was evaluated using a chronic unpredictable mild stress model in mice. Ellagic acid improved neuroendocrine and inflammatory responses in the model mice and exerted a notable antidepressant effect. Additionally, ellagic acid can improve anxiety, possibly by inhibiting toll-like receptor 4 and activating NF-E2-related factor, to reduce anxiety (Huang, 2020; Wang et al., 2020b). The anxiolytic effects of T. Chebula tannin extract was investigated using a heroin-induced anxiety model in mice. The outcomes demonstrated that the tannin extract acts as a natural product for neurodegenerative illnesses and exhibits substantial anxiolytic activity (Chandrasekhar et al., 2018).
4.2 Improvement of memory and cognitive level
Memory impairment and cognitive deficits are the primary clinical manifestations of MCI, with memory impairment as the core problem (Peritogiannis et al., 2022). Patients with MCI and memory impairment have a 38% risk of developing Alzheimer’s disease (Irish et al., 2011). Currently, trials indicate that memory training, a feasible non-pharmacological intervention, can improve cognitive function in patients with MCI and result in positive performance changes in older adults who have cognitive impairment (Olchik et al., 2013). Corilagin 29, extracted from T. Chebula, significantly improved sleep deprivation-induced memory impairment, which was associated with the inhibition of NADPH oxidase 2 and the activation of NF-E2-related factor (Wang et al., 2020a). Polyphenolic compounds, such as quercetin 129 and rutin 131, demonstrated potential protective effects on inhibitory avoidance memory deficits in zebrafish, suggesting their use in preventing and treating neurodegenerative diseases (Richetti et al., 2011). Rutin prevents morphological changes in hippocampal tissue by lowering the expression of cyclooxygenase-2, glial fibrillary acidic protein, interleukin-8, and inducible nitric oxide synthase, thereby attenuating STZ-induced inflammatory responses, as demonstrated in a rat model of cognitive impairment (Javed et al., 2012). Studies have confirmed that rutin can prevent cognitive problems. A passive avoidance learning memory test assessed learning and memory in normal and diabetic rats after 30 days of rutin administration and revealed enhanced cognitive performance in both groups (Hasanein et al., 2020). Additionally, rutin exhibits multi-targeted therapeutic potential for cognitive deficits associated with chronic cerebral hypoperfusion (Qu et al., 2014). Insulin deficiency in the brain, or “diabetes in the brain”, potentially caused by a high-cholesterol diet, is associated with cognitive dysfunction and neurodegenerative diseases. Rutin may reverse high cholesterol-induced inflammatory changes, apoptosis activation, and cognitive deficits (Tomycz and Friedlander, 2011; Sun et al., 2021). Furthermore, ellagic acid 59 can hinder scopolamine and diazepam-induced cognitive impairment, indicating its potential as a memory restorer in dementia treatment and in improving cognitive behavior (Jha et al., 2018; Mansouri et al., 2016).
4.3 Antioxidant effect
T. Chebula and its chemical constituents can improve the pathogenesis of various neurodegenerative diseases. Tannins, a unique class of phytochemicals in T. Chebula, exhibit a broad range of potential health benefits, particularly due to their antioxidant capacity (Hussain et al., 2018a; Kennedy and Wightman, 2011). This antioxidant property is valuable because it reduces oxidative damage, a major marker of almost all diseases (Hussain et al., 2018b). T. Chebula is rich in plant polyphenols, with a total polyphenol content reaching 13.27%. These polyphenols contain phenolic hydroxyl groups that can be dehydrogenated and oxidized during lipid peroxidation in cell membranes. This reaction provides electrons to reactive oxygen species (ROS), thereby effectively terminating the chain reaction of free radicals and protecting cell structures from damages (Gupta et al., 2020). Polyphenols in T. Chebula can effectively scavenge -OH radicals and significantly inhibit lecithin lipid peroxidation damage, with antioxidant effects comparable with those of rutin 131, which are known for its strong antioxidant properties. The tannin (polyphenolic) antioxidant content of Asian ellagic acid in T. Chebula is up to 32%, which is closely related to the high antioxidant properties of T. Chebula (Chang and Lin, 2012). In a model of sodium oxalate-induced renal oxidative imbalance in female rats, T. Chebula extract (TCE) significantly increased the catalase (CAT), total reduced glutathione (GSH), and superoxide dismutase (SOD) activity, while reducing lipid peroxidation (LPO) induced by sodium oxalate (Saha and Verma, 2015). Increased inflammatory mediators can impair cognitive function and predispose individuals to neurological disorders. Pro-inflammatory cytokines and ROS production lead to oxidative stress, making antioxidants a potential therapeutic approach for these disorders. Ellagic acid (EA) stands out among antioxidants; it modulates immune response by significantly reducing glial cell expression, attenuating phosphorylated Tau and oxidative damage, improving the antioxidant system, and preventing increased acetylcholinesterase activity (Dornelles et al., 2020). The antioxidant effects of EA are due to its free radical scavenging properties and enhancement of endogenous antioxidants, such as GSH, SOD, catalase, glutathione reductase, and glutathione peroxidase (Javed et al., 2021). Sleep disorders (SD) can cause neurobehavioral deterioration, including cognitive impairment and memory deficits. Pilot studies found that EA reduced damages by activating the Nrf2/HO-1 pathway and inhibiting the TLR4-induced inflammatory response. Nrf2 regulates the antioxidant system and can be activated in response to oxidative stress (Butterfield et al., 2023). EA exerts antioxidant effects by activating Nrf2, protecting against mitochondrial dysfunction in rats through upregulation of Nrf2/HO-1, and inhibition of NF-κB signaling pathways (Wang et al., 2020b). In summary, the active ingredients of T. Chebula, primarily tannins and phenolic acids, exert their antioxidant effects by scavenging free radicals, affecting oxidative enzyme activity, and combating oxidative stress.
4.4 Anti-inflammatory effect
T. Chebula contains phenolic acid components, such as gallic acid 16, which has significant anti-inflammatory activity. Gallic acid reduces neuroinflammation by decreasing Aβ plaque-associated microgliosis and astrocytosis, decreasing cytokine levels in microglia, and protecting neurons from Aβ-induced neurotoxicity by inhibiting NF-κB acetyltransferase. Tannic acid, a hydrolysable glycosidic polyphenol polymer of gallic acid, also exhibits anti-inflammatory effects, primarily by reducing brain lipid peroxidation (MDA) and pro-inflammatory cytokines (IL-1β, IL-6, and TNF-α) to improve memory and cognitive impairment (Zhang et al., 2017; Caruso et al., 2022; Mirshekar et al., 2018). T. Chebula is also rich in flavonoids, which inhibit neuroinflammation by directly reacting with and removing neurotoxic substances and pro-inflammatory factors produced in the brain as a result of aging (Calderaro et al., 2022; Spencer, 2010). Corilagin 29, a member of the tannin family, has significant anti-inflammatory effects. Its intrinsic anti-inflammatory mechanism involves significantly reducing the production of pro-inflammatory cytokines and mediating TNF-α, IL-1β, IL-6, NO (iNOS), and COX-2 nuclear translocation by blocking NF-κB at the protein and gene levels (Zhao et al., 2008). These findings were further verified, showing that corilagin reduces NO production and the mortality of microglia stimulated by lipopolysaccharide (LPS), scavenges diphenyl-1-propenylhydrazyl (DPPH) radicals by 96%, and decreases malondialdehyde (MDA) levels, thereby inhibiting inflammatory and oxidative processes (Gaire et al., 2013). Studies have also evaluated the anti-inflammatory properties of TCE on LPS-induced inflammation in microglia. TCE significantly reduced LPS-induced inflammation, increased the anti-inflammatory rate in mouse macrophages, and influenced inflammation and anti-inflammatory mediators (Rahimi et al., 2018). TCE has been proven effective in healing neuroinflammatory disorders, indicating its potential as an anti-inflammatory agent in treating central nervous system inflammatory diseases.
4.5 Repairing synaptic plasticity damage
Plastic synapses may be crucial and unique biological neural networks associated with learning and memory and maintain stable adaptive properties in response to external environmental changes (Brown and Piscopo, 2013; Yang et al., 2018). Increasing evidence indicates that phytochemicals have multiple biochemical functions (Skaper et al., 2017). For instance, quercetin 129, found in T. Chebula, significantly reduces memory impairment in rats. Quercetin improves mitochondrial production, structure, and function in rat hippocampal neurons under low-pressure and low-oxygen conditions, significantly reducing synaptic structural damage. It also regulates the expression of fusion and fission-related proteins in mitochondria and promotes the expression of brain-derived neurotrophic factor (Liu, 2016). Quercetin can repair lead-induced synaptic plasticity damage in the hippocampus. It reduces synaptic plasticity damage in the DG region of rats exposed to chronic lead, suggesting that quercetin could be a potential treatment for lead-induced cognitive impairment (Hu, 2008). The colorimetric method of tetramethylazolium salt (MTT) and DAPI staining indicated that quercetin increased the survival rate of PC12 cells in the glutamate-treated group in a concentration- and time-dependent manner. Quercetin may protect against glutamate-induced neurological damage by inhibiting outward potassium currents in hippocampal pyramidal neurons, indicating its reparative effects on nerves (Zhao et al., 2010). Additionally, ellagic acid 59, in T. Chebula, alleviates synaptic plasticity impairment in rats with acute kidney injury (AKI). Treatment with ellagic acid improved brain electrophysiology, spatial learning, and memory indices in AKI rats (Sarkaki et al., 2022).
4.6 Anti-apoptotic effect
Apoptosis of nerve cells significantly impacts neurodegenerative diseases. Flavonoids in T. Chebula exhibit various neuroprotective effects and improve cognitive dysfunction by interacting with key proteins and lipid kinase signaling cascades, thereby inhibiting apoptosis triggered by neurotoxic species and promoting neuronal survival (Vauzour et al., 2008). This cytoprotective ability is likely related to their anti-apoptotic properties. Studies using H2O2-treated PC12 cells created a model of apoptosis, characterized by cell death not accompanied by LDH release (Dajas et al., 2003). The ethanolic extract of T. Chebula fruit effectively inhibited COX-1, COX-2, and 5-LOX; mechanistic studies showed that T. Chebula acts as a dual inhibitor of COX-2 and 5-LOX and can inhibit apoptosis (Reddy et al., 2009). T. Chebula also contains ellagic acid 59, which plays an anti-apoptotic role in neurodegenerative diseases. Pilot studies demonstrated that ellagic acid significantly improved spatial learning and memory deficits and reduced neuronal apoptosis and Aβ deposition in the hippocampus (Zhong et al., 2018). Ellagic acid also inhibits tau hyperphosphorylation and reduces glycogen synthase activity, and these effects are partially mediated by the AKT/GSK3β signaling pathway. Rutin 131, another flavonoid in T. Chebula, was investigated for its effect on isoflurane-induced apoptosis. It significantly decreased isoflurane-induced apoptosis, as measured by TUNEL assays, and increased the expression levels of caspase-3, Bad, Bax, and MAPK proteins. Rutin provided neuroprotection against isoflurane-induced neuronal apoptosis and improved learning and memory in rats by effectively regulating MAPK protein expression levels (Li et al., 2017).
5 Safety evaluation
T. Chebula is widely used in conventional and modern medicine, and related safety studies are crucial for determining safe doses for further clinical trials. Various studies have evaluated the in vitro and in vivo toxicity of methanolic extracts of T. Chebula fruit (TCF) and skin (TAB) using cytotoxicity, hemolytic activity, mutagenicity, and genotoxicity tests (Suganthy et al., 2018). Acute and subacute toxicity studies indicated that oral administration of TCF and TAB is relatively non-toxic. Specifically, aqueous, ethanol, and ethyl acetate extracts of TCF showed no cytotoxicity to sheep erythrocytes. Acute oral toxicity tests in rats demonstrated that aqueous extracts from dried TCF had no acute or chronic toxicity in either female or male rats (Sireeratawong et al., 2013). Additionally, the mutagenicity of the T. Chebula ethyl acetate (EtOAc) soluble fraction was assessed in vitro, and a continuous 14-day oral administration experiment revealed no adverse effects on rats at a dose of 2000 mg/kg (Kim et al., 2012). Furthermore, T. Chebula had genotoxic effects in the VITOTOX test and the Ames test (Bag et al., 2013).
6 Quality control
The quality control level of T. Chebula needs improvement. The 2020 edition of the Chinese Pharmacopoeia lacks requirements for content determination, leading to gaps in quality control for T. Chebula herbs. In this regard, a high-performance liquid chromatography (HPLC) method was established to determine chebulagic acid and chebulinic acid in T. Chebula; it enhanced the content determination guidelines in the 2020 Chinese Pharmacopoeia and facilitated improved quality control of T. Chebula herbs (Wang et al., 2024). Quality testing standards typically focus on a few key components in T. Chebula. The total tannin content of T. Chebula was determined using HPLC-DAD analysis, and active site profiles were constructed. This method allows for accurate identification and quality control of various types of T. Chebula available in the market (He et al., 2017). Near-infrared (NIR) diffuse reflectance spectroscopy, which is widely used for rapid detection, was combined with partial least square regression (PLS) to establish a quantitative model for gallic acid determination in T. Chebula. The results showed no significant difference between the predicted and conventional methods, indicating the feasibility of this technique for T. Chebula quality determination (Luo et al., 2016). Additionally, an HPLC method was developed for the simultaneous determination of several key constituents in chebulanic herbs: gallic acid 16, punicalagin 39, punicalagin B 77, methyl gallate 17, corilagin 29, pentagalloylglucose 82, and ellagic acid 59. The concentrations were 0.186–3.720 μg for gallic acid, 0.046–0.552 μg for punicalagin A, 0.029–0.192 μg for punicalagin B, 0.182–2.730 μg for methyl gallate, 0.069–1.388 μg for corilagin, 0.324–6.480 μg for pentagalloylglucose, and 0.167–1.672 μg for ellagic acid, and all of which showed good linearity (Qi, 2018).
7 Conclusion and future perspectives
MCI poses significant hazards, including cognitive decline, psychological health issues, decreased ability to perform daily activities, reduced social engagement, risk of progression to dementia, and burden on families (De Leon et al., 1997). MCI is a high-risk stage for Alzheimer’s disease, with some patients with MCI potentially progressing to dementia within a few years, leading to severe cognitive and functional impairments (Forrester et al., 2016; Kohler et al., 2016). Tibetan medicine offers advantages in treating MCI, such as holistic regulation, individualized treatment, high safety, symptom improvement, and slowing disease progression. By comprehensively applying traditional Tibetan therapeutic methods, effective interventions can be provided to patients with MCI to improve their quality of life and delay disease progression. MCI falls under the category of “Jie Xie Syndrome” in Tibetan medicine, which believes that regulating the imbalance of “Long” can treat “Jie Xie Syndrome.”
This article primarily reviews the active components and pharmacological effects of T. Chebula in treating MCI. We identified 171 compounds from T. Chebula, including 83 tannins, 16 phenolic acids, 6 flavonoids, 29 triterpenoids, 17 volatile oils, and 20 other compounds. Among these, 11 compounds significantly improve MCI symptoms. The pharmacological activities of T. Chebula that help alleviate MCI symptoms mainly include antioxidant, hypoglycemic, antiviral, and anti-inflammatory effects. However, current research on the quality standards of T. Chebula is insufficient, and the specific chemical components of T. Chebula need further exploration. Scholars need to conduct advanced research into the extraction and isolation of the chemical constituents of T. Chebula.
Neurodegenerative diseases involve oxidative stress, inflammation, abnormal protein accumulation, and mitochondrial dysfunction, thereby disrupting physiological processes and contributing to various neurological disorders, including depression. T. Chebula shows promise in alleviating depression and anxiety symptoms in MCI. Its active components can potentially reverse inflammation and apoptosis induced by high cholesterol and improve cognitive function and memory in patients with MCI. The neuroprotective effects of T. Chebula originate from its potent anti-inflammatory and antioxidant properties. It shields neurons from oxidative stress and neurotoxicity, reduces neuronal inflammation, and supports synaptic plasticity while enhancing cerebral blood flow. Moreover, T. Chebula appears beneficial for sleep, a critical issue in MCI, although additional research is needed to fully understand its mechanisms in improving sleep quality. In summary, T. Chebula exhibits diverse pharmacological effects including antioxidant, anti-inflammatory, synaptic plasticity repair, anti-apoptotic, and enhancement of depression-like behavior, memory, and cognitive function. Toxicological studies, encompassing cytotoxicity, hemolytic activity, mutagenicity, and genotoxicity tests, indicate the relative safety of T. Chebula in in vitro and in vivo settings. However, further refinement of the specific mechanisms underlying these pharmacological actions is necessary, as well as research into optimal dosage control. Experimental studies using various animal models and cell lines support the potential of T. Chebula in alleviating symptoms of MCI and achieving specific therapeutic goals. However, the majority of these studies are confined to cellular and animal research, with limited clinical trials conducted. This gap impedes the validation of the efficacy of T. Chebula in humans and poses challenges for the development of new treatments based on T. Chebula.
Despite the progress in the study of MCI, treating MCI still faces multiple challenges and issues. First, MCI diagnostic results are inconsistent due to the lack of uniform early diagnostic criteria and the difficulty of screening (Roberts and Knopman, 2013). Its mild symptoms are not easily recognized, which may cause patients to miss the optimal intervention period. Second, the effectiveness and application of treatment methods are limited. Although various methods, such as drug therapy, cognitive training, and lifestyle interventions, exist, they have its limitations (Eshkoor et al., 2015). Drug treatments, such as the use of cholinesterase inhibitors and NMDA receptor antagonists, may show some effects but generally have limited efficacy and may come with side effects. The development of multi-component, multi-target traditional Tibetan medicines with therapeutic advantages, such as Tibetan medicine T. Chebula, is lacking. Non-drug treatments, such as cognitive training, exercise, and dietary adjustments, have potential but their effects vary from person to person and lack large-scale, long-term validation. Third, the lack of individualized treatment is a significant issue. The causes, symptoms, and progression rates of patients with MCI vary, but current treatments often use a uniform approach and lack tailored treatments for individual differences. Fourth, treatment adherence and long-term management issues deserve attention. Patients with MCI may lack the motivation and adherence to persist with long-term treatments and interventions, thereby affecting the treatment outcomes (Portet et al., 2006). Long-term management is challenging because MCI is a gradual, progressive condition that requires continuous monitoring and management. Fifth, the inadequacy of psychological and social support systems affects overall treatment outcomes. Patients with MCI and their families often face psychological stress and anxiety, but psychological support and counseling services are relatively insufficient. Additionally, social support systems and resources for patients with MCI are limited, thereby affecting their quality of life and social participation (Jongsiriyanyong and Limpawattana, 2018). Finally, research and development of related drugs face numerous challenges. A deeper understanding of the pathogenesis of MCI is still lacking, which limits the development of new treatments. MCI involves multiple pathological mechanisms, including neuroinflammation, oxidative stress, and metabolic abnormalities. Single-action mechanism drugs often struggle to effectively treat these complexities. Furthermore, clinical trials require long-term follow-up and large sample sizes due to the mild and slow-progressing nature of MCI symptoms, thereby increasing the difficulty and cost of research (Hendrix, 2012). In summary, treating MCI involves numerous issues, such as difficulty in early diagnosis, limited treatment effectiveness, lack of individualized treatment, challenges in adherence and long-term management, inadequate psychological and social support, and research and development challenges.
To address the numerous challenges in treating MCI, future research should focus on multiple aspects to advance therapeutic methods. First, developing and promoting standardized diagnostic tools is essential to ensure diagnostic accuracy and consistency. Initiatives include increasing public and medical personnel awareness of early MCI symptoms and the importance of early detection through regular screening. Second, efforts should be intensified in developing new drugs, especially those targeting the pathological mechanisms of MCI. Exploring the combination of pharmacological and non-pharmacological interventions, such as cognitive training, exercise, and dietary adjustments, to form comprehensive treatment plans can enhance efficacy (Poptsi et al., 2022). Additionally, research and development should be strengthened for traditional medicines with multi-component and multi-target actions, such as Tibetan medicine T. Chebula (Klimova et al., 2019). Third, the importance of personalized treatment must be emphasized. Treatment plans should be tailored to the specific conditions of each patient, including the choice of medication and lifestyle interventions. Research on biomarkers should be strengthened to develop predictive tools that help identify high-risk patients and guide personalized treatment. Fourth, psychological counseling and support services should be enhanced to provide necessary emotional support and counseling for patients with MCI and their families. Establishing and improving social support networks can offer various forms of assistance and resources to patients with MCI, thereby improving their quality of life and social participation. Finally, promoting interdisciplinary collaboration is crucial for in-depth research into the pathological mechanisms of MCI. Establishing large-scale MCI databases that integrate genomic, proteomic, and metabolomic data can help uncover potential pathological mechanisms and biomarkers. Utilizing artificial intelligence and machine learning techniques to analyze complex data can reveal new research directions and therapeutic targets. Innovative clinical trial designs, such as adaptive and crossover designs, should be employed to increase trial efficiency and provide effective treatment options for MCI. In summary, addressing the challenges of MCI treatment requires a multifaceted approach, including the development of standardized diagnostic tools, new drug development, personalized treatment plans, enhanced psychological and social support, and interdisciplinary research to uncover new therapeutic targets and improve treatment efficacy.
As future research progresses, Tibetan medicine T. Chebula shows immense promise in treating MCI and offers potential benefits to patients by improving the overall human quality of life.
Author contributions
HG: Conceptualization, Data curation, Writing–original draft, Writing–review and editing. HL: Resources, Supervision, Writing–review and editing. NF: Data curation, Formal Analysis, Writing–review and editing. JS: Project administration, Validation, Visualization, Writing–review and editing. RL: Methodology, Project administration, Writing–review and editing. WW: Investigation, Methodology, Writing–review and editing. YZ: Resources, Visualization, Writing–review and editing.
Funding
The author(s) declare that financial support was received for the research, authorship, and/or publication of this article. This work was funded by the National Key R&D Program of China (2023YFC3504400), and the National Natural Science Foundation of China (82204761 and 82130113).
Conflict of interest
The authors declare that the research was conducted in the absence of any commercial or financial relationships that could be construed as a potential conflict of interest.
Publisher’s note
All claims expressed in this article are solely those of the authors and do not necessarily represent those of their affiliated organizations, or those of the publisher, the editors and the reviewers. Any product that may be evaluated in this article, or claim that may be made by its manufacturer, is not guaranteed or endorsed by the publisher.
References
Ajala, O. S., Jukov, A., and Ma, C. M. (2014). Hepatitis C virus inhibitory hydrolysable tannins from the fruits of Terminalia chebula. Fitoterapia 99, 117–123. doi:10.1016/j.fitote.2014.09.014
An, Y. Y., Ju, C. G., Zhang, Q., Hao, J., Chen, J. M., and Wang, W. (2022). Content changes of chemical constituents and comparison of the anti-ulcerative colitis effect of processed products of Terminalia chebula at different temperatures. Chin. Pharm., 332–337. doi:10.6039/j.issn.1001-0408.2022.03.13
Asif, M., Ahmed, M. W., Khair, S., Murugeswaran, R., Meena, R., Alam, M., et al. (2019). Pharmacognostical studies of Halela Siyah (Terminalia chebula Retz.): an important Unani medicinal plant. Res. J Pharmacogn. Phytochemistry 11, 205–211. doi:10.5958/0975-4385.2019.00035.9
Bag, A., Bhattacharyya, S. K., and Chattopadhyay, R. R. (2013). The development of Terminalia chebula Retz. (Combretaceae) in clinical research. Asian Pac J. Trop. Biomed. 244-252, 244–252. doi:10.1016/S2221-1691(13)60059-3
Barthakur, N. N., and Arnold, N. P. (1991). Nutritive value of the chebulic myrobalan (Terminalia chebula Retz.) and its potential as a food source. Food Chem. 40, 213–219. doi:10.1016/0308-8146(91)90105-W
Bode, M., Sulzer, P., Schulte, C., Becker, S., Brockmann, K., Elben, S., et al. (2023). Multidomain cognitive training increases physical activity in people with Parkinson’s disease with mild cognitive impairment. Park. Relat. D. 113, 105330. doi:10.1016/j.parkreldis.2023.105330
Brown, E. R., and Piscopo, S. (2013). Synaptic plasticity in cephalopods; more than just learning and memory? Invertebr. Neurosci. 13, 35–44. doi:10.1007/s10158-013-0150-4
Bulbul, M. R. H., Chowdhury, M. N. U., Naima, T. A., Sami, S. A., Imtiaj, M. S., Huda, N., et al. (2022). A comprehensive review on the diverse pharmacological perspectives of Terminalia chebula Retz. Heliyon 8, e10220. doi:10.1016/j.heliyon.2022.e10220
Butterfield, D. A., Boyd-Kimball, D., and Reed, T. T. (2023). Cellular stress response (hormesis) in response to bioactive nutraceuticals with relevance to alzheimer disease. Antioxid. Redox Sign, 643–669. doi:10.1089/ars.2022.0214
Cai, X., Xie, B., and Du, H. (2008). Advances in research on chemical constituents and pharmacological action of Terminalia chebula retz. Prog. Pharm. Sci., 212–215.
Calderaro, A., Patane, G. T., Tellone, E., Barreca, D., Ficarra, S., Misiti, F., et al. (2022). The neuroprotective potentiality of flavonoids on alzheimer’s disease. Int. J. Mol. Sci. 14835, 14835. doi:10.3390/ijms232314835
Campbell, N. L., Lane, K. A., Gao, S., Boustani, M. A., and Unverzagt, F. (2018). Anticholinergics influence transition from normal cognition to mild cognitive impairment in older adults in primary care. Pharmacotherapy 38, 511–519. doi:10.1002/phar.2106
Caruso, G., Godos, J., Privitera, A., Lanza, G., Castellano, S., Chillemi, A., et al. (2022). Phenolic acids and prevention of cognitive decline: polyphenols with a neuroprotective role in cognitive disorders and alzheimer’s disease. Nutrients 819, 819. doi:10.3390/nu14040819
Chandrasekhar, Y., Kumar, G. P., Navya, K., Ramya, E. M., and Anilakumar, K. R. (2018). Tannins from Terminalia chebula fruits attenuates GABA antagonist-induced anxiety-like behaviour via modulation of neurotransmitters. J. Pharm. Pharmacol. 70, 1662–1674. doi:10.1111/jphp.13007
Chandrashekar, R., Manohar, V. R., and Rao, S. N. (2013). Acute anxiolytic activity of aqueous extract of Terminalia chebula fruit pulp in rats. I J Res. Ayurveda Pharm 4, 112–115. doi:10.7897/2277-4343.04138
Chang, C. L., and Lin, C. S. (2012). Phytochemical composition, antioxidant activity, and neuroprotective effect of Terminalia chebula retzius extracts. Evid. Based Complement. Altern. Med. 2012, 125247. doi:10.1155/2012/125247
Conrad, J., Vogler, B., Klaiber, I., Roos, G., Walter, U., and Kraus, W. (1998). Two triterpene esters from Terminalia macroptera bark. Phytochemistry 48, 647–650. doi:10.1016/S0031-9422(98)00154-X
Dajas, F., Rivera, F., Blasina, F., Arredondo, F., Echeverry, C., Lafon, L., et al. (2003). Cell culture protection and in vivo neuroprotective capacity of flavonoids. Neurotox. Res. 5, 425–432. doi:10.1007/BF03033172
Dakpa, T. (2014). Unique aspect of Tibetan medicine. Acupunct. Electrother. Res. 39, 27–43. doi:10.3727/036012914x13966138791145
Dattatray, B. P., Padmaja, A. M., and Nirmala, N. R. (2014). Antidepressant activity of aqueous extracts of fruits of Terminalia chebula and phyllanthus emblica in behavioural models of depression: involvement of monoaminergic system. Int. J. Pharm. Sci., 615–620.
Dean, K., Oulhaj, A., Zamboni, G., DeJager, C. A., and Wilcock, G. K. (2014). Role of depression in predicting time to conversion to mild cognitive impairment. Am. J. Geriatr. Psychiatry 22, 727–734. doi:10.1016/j.jagp.2012.12.025
De Leon, M. J., George, A. E., Golomb, J., Tarshish, C., Convit, A., Kluger, A., et al. (1997). Frequency of hippocampal formation atrophy in normal aging and Alzheimer’s disease. Neurobiol. Aging 18, 1–11. doi:10.1016/S0197-4580(96)00213-8
Ding, G., Liu, Y., Song, M., Zou, D., and Sheng, L. (2001). Polyphenols from Terminalia chebula. J. China Pharma Univ., 193–196. doi:10.3321/j.issn:1000-5048.2001.03.008
Dooley, J., Bailey, C., Xanthopoulou, P., Bass, N., and McCabe, R. (2020). Communication and understanding of mild cognitive impairment diagnoses. Int. J. Geriatr. Psychiatry 35, 662–670. doi:10.1002/gps.5284
Dornelles, G. L., de Oliveira, J. S., de Almeida, E. J. R., Mello, C. B. E., Br, E. R., da Silva, C. B., et al. (2020). Ellagic acid inhibits neuroinflammation and cognitive impairment induced by lipopolysaccharides. Neurochem. Res. 45, 2456–2473. doi:10.1007/s11064-020-03105-z
Ekambaram, S. P., Perumal, S. S., Erusappan, T., and Srinivasan, A. (2020). Hydrolysable tannin-rich fraction from Terminalia chebula Retz. fruits ameliorates collagen-induced arthritis in BALB/c mice. Inflammopharmacology 28, 275–287. doi:10.1007/s10787-019-00629-x
Ellen, M. E., Panisset, U., de Carvalho, I. A., Goodwin, J., and Beard, J. (2017). A Knowledge Translation framework on ageing and health. Health Policy 121, 282–291. doi:10.1016/j.healthpol.2016.12.009
Eshkoor, S. A., Hamid, T. A., Mun, C. Y., and Ng, C. K. (2015). Mild cognitive impairment and its management in older people. Clin. Interv. Aging 10, 687–693. doi:10.2147/CIA.S73922
Feng, X., Lu, X., Kan, Z., and Zhong, G. (2019). Quality standard study on Tibetan medicine wuwei jinse pill. Chin. J. Mod. Appl. Pharm. 1992-1997. doi:10.13748/j.cnki.issn1007-7693.2019.16.002
Forrester, S. N., Gallo, J. J., Smith, G. S., and Leoutsakos, J. M. (2016). Patterns of neuropsychiatric symptoms in mild cognitive impairment and risk of dementia. Am. J. Geriatr. Psychiatry 24, 117–125. doi:10.1016/j.jagp.2015.05.007
Gaire, B. P., Jamarkattel-Pandit, N., Lee, D., Song, J., Kim, J. Y., Park, J., et al. (2013). Terminalia chebula extract protects OGD-R induced PC12 cell death and inhibits lps induced microglia activation. Molecules 18, 3529–3542. doi:10.3390/molecules18033529
Gallagher, D., Fischer, C. E., and Iaboni, A. (2017). Neuropsychiatric symptoms in mild cognitive impairment. Can. J. Psychiatry 62, 161–169. doi:10.1177/0706743716648296
Gupta, A., Kumar, R., Bhattacharyya, P., Bishayee, A., and Pandey, A. K. (2020). Terminalia bellirica (Gaertn.) roxb. (Bahera) in health and disease: a systematic and comprehensive review. Phytomedicine 77, 153278. doi:10.1016/j.phymed.2020.153278
Hasanein, P., Emamjomeh, A., Chenarani, N., and Bohlooli, M. (2020). Beneficial effects of rutin in diabetes-induced deficits in acquisition learning, retention memory and pain perception in rats. Nutr. Neurosci. 23, 563–574. doi:10.1080/1028415X.2018.1533269
He, M., Deng, X., Gao, Q., and Gao, P. (2017). Screening of antibacterial active components and a new method for total tannin content determination from Terminalia. Genomics Appl. Biol., 5167–5172. doi:10.13417/j.gab.036.005167
Hendrix, S. B. (2012). Measuring clinical progression in MCI and pre-MCI populations: enrichment and optimizing clinical outcomes over time. Alzheimers Res. Ther. 24, 24. doi:10.1186/alzrt127
Hidaka, S., Ikejima, C., Kodama, C., Nose, M., Yamashita, F., Sasaki, M., et al. (2012). Prevalence of depression and depressive symptoms among older Japanese people: comorbidity of mild cognitive impairment and depression. Int. J. Geriatr. Psych. 27, 271–279. doi:10.1002/gps.2715
Hu, P. (2008). Relieving effect of quercetin on lead-induced impairment of synaptic plasticity in rat hippocampus and the inhibitory effect of Natratoxin neurotoxin on A-type K+ currents in rat dorsal root ganglion neurons. University of Science and Technology of China.
Huang, X. (2020). The effect and mechanism of Ellagic Acid on the improvement of depression-like behavior in chronic stress model mice. Hainan Univ. doi:10.27073/d.cnki.ghadu.2020.001278
Hussain, G., Rasul, A., Anwar, H., Aziz, N., Razzaq, A., Wei, W., et al. (2018a). Role of plant derived alkaloids and their mechanism in neurodegenerative disorders. Int. J. Biol. Sci. 14, 341–357. doi:10.7150/ijbs.23247
Hussain, G., Zhang, L., Rasul, A., Anwar, H., Sohail, M. U., Razzaq, A., et al. (2018b). Role of plant-derived flavonoids and their mechanism in attenuation of alzheimer’s and Parkinson’s diseases: an update of recent data. Molecules 814, 814. doi:10.3390/molecules23040814
Irish, M., Lawlor, B. A., Coen, R. F., and O’Mara, S. M. (2011). Everyday episodic memory in amnestic mild cognitive impairment: a preliminary investigation. Bmc Neurosci. 12, 80–13. doi:10.1186/1471-2202-12-80
Javaid, N., Shah, M. A., Rasul, A., Chauhdary, Z., Saleem, U., Khan, H., et al. (2021). Neuroprotective effects of ellagic acid in alzheimer’s disease: focus on underlying molecular mechanisms of therapeutic potential. Curr. Pharm. Des. 27, 3591–3601. doi:10.2174/1381612826666201112144006
Javed, H., Khan, M. M., Ahmad, A., Vaibhav, K., Ahmad, M. E., Khan, A., et al. (2012). Rutin prevents cognitive impairments by ameliorating oxidative stress and neuroinflammation in rat model of sporadic dementia of Alzheimer type. Neuroscience 210, 340–352. doi:10.1016/j.neuroscience.2012.02.046
Jeong, H. K., Lee, D., Kim, H. P., and Baek, S. H. (2019). Structure analysis and antioxidant activities of an amylopectin-type polysaccharide isolated from dried fruits of Terminalia chebula. Carbohydr. Polym. 211, 100–108. doi:10.1016/j.carbpol.2019.01.097
Jha, A. B., Panchal, S. S., and Shah, A. (2018). Ellagic acid: insights into its neuroprotective and cognitive enhancement effects in sporadic Alzheimer’s disease. Pharmacol. Biochem. Be 175, 33–46. doi:10.1016/j.pbb.2018.08.007
Jokar, A., Masoomi, F., Sadeghpour, O., Nassiri-Toosi, M., and Hamedi, S. (2016). Potential therapeutic applications for Terminalia chebula in Iranian traditional medicine. J. Tradit. Chin. Med. 36, 250–254. doi:10.1016/S0254-6272(16)30035-8
Jongsiriyanyong, S., and Limpawattana, P. (2018). Mild cognitive impairment in clinical practice: a review article. Am. J. Alzheimers Dis. Other Demen 33, 500–507. doi:10.1177/1533317518791401
Juang, L. J., Sheu, S. J., and Lin, T. C. (2004). Determination of hydrolyzable tannins in the fruit of Terminalia chebula Retz. by high-performance liquid chromatography and capillary electrophoresis. J. Sep. Sci. 27, 718–724. doi:10.1002/jssc.200401741
Kennedy, D. O., and Wightman, E. L. (2011). Herbal extracts and phytochemicals: plant secondary metabolites and the enhancement of human brain function. Adv. Nutr. 2, 32–50. doi:10.3945/an.110.000117
Kessler, E. M., Sudhof, J. K., and Frolich, L. (2014). Dementia worry in memory clinic patients not diagnosed with organic mental disorder. Int. Psychogeriatr. 26, 1049–1051. doi:10.1017/S1041610214000349
Khan, H., Ullah, H., Aschner, M., Cheang, W. S., and Akkol, E. K. (2019). Neuroprotective effects of quercetin in alzheimer’s disease. Biomolecules 59, 59. doi:10.3390/biom10010059
Kim, H. J., Song, H. K., Park, S. H., Jang, S., Park, K. S., Song, K. H., et al. (2022). Terminalia chebula Retz. extract ameliorates the symptoms of atopic dermatitis by regulating anti-inflammatory factors in vivo and suppressing STAT1/3 and NF-ĸB signaling in vitro. Phytomedicine 104, 154318. doi:10.1016/j.phymed.2022.154318
Kim, J. H., Koo, Y. C., Hong, C. O., Yang, S. Y., Jun, W., and Lee, K. W. (2012). Mutagenicity and oral toxicity studies of Terminalia chebula. Phytother. Res. 26, 39–47. doi:10.1002/ptr.3504
Kim, M. S., Lee, D. Y., Sung, S. H., and Jeon, W. K. (2018). Anti-cholinesterase activities of hydrolysable tannins and polyhydroxytriterpenoid derivatives from retz. Fruit. Rec. Nat. Prod., 285–290. doi:10.25135/rnp.29.17.07.130
Klimova, B., Kuca, K., Valis, M., and Hort, J. (2019). Traditional Chinese medicine as an effective complementary non-pharmacological approach to mild cognitive impairment: a call for collaboration. J. Alzheimers Dis. 68, 1185–1192. doi:10.3233/JAD-181281
Kohler, C. A., Magalhaes, T. F., Oliveira, J. M., Alves, G. S., Knochel, C., Oertel-Knochel, V., et al. (2016). Neuropsychiatric disturbances in mild cognitive impairment (MCI): a systematic review of population-based studies. Curr. Alzheimer Res. 13, 1066–1082. doi:10.2174/1567205013666160502123129
Lapeyre-Mestre, M. (2016). A review of adverse outcomes associated with psychoactive drug use in nursing home residents with dementia. Drugs Aging 33, 865–888. doi:10.1007/s40266-016-0414-x
Lee, D. Y., Kim, H. W., Yang, H., and Sung, S. H. (2017). Hydrolyzable tannins from the fruits of Terminalia chebula Retz and their α-glucosidase inhibitory activities. Phytochemistry 137, 109–116. doi:10.1016/j.phytochem.2017.02.006
Lee, H. S., Jung, S. H., Yun, B. S., and Lee, K. W. (2007). Isolation of chebulic acid from Terminalia chebula Retz. and its antioxidant effect in isolated rat hepatocytes. Arch. Toxicol. 81, 211–218. doi:10.1007/s00204-006-0139-4
Li, H. S., Liu, Y. J., Yang, H. L., and Liu, Y. G. (2022). Research progress on chemical constituents, pharmacological mechanism, quality control and processing of Terminalia chebula Retz. Nat. Prod. Res. Dev., 2130–2141. doi:10.16333/j.1001-6880.2022.12.017
Li, K., Han, X., Li, R., Xu, Z., Pan, T., Liu, J., et al. (2019). Composition, antivirulence activity, and active property distribution of the fruit of Terminalia chebula retz. J. Food Sci. 84, 1721–1729. doi:10.1111/1750-3841.14655
Li, W., Li, D. Y., Zhao, S. M., Zheng, Z. J., Hu, J., Li, Z. Z., et al. (2017). Rutin attenuates isoflurane-induced neuroapoptosis via modulating JNK and p38 MAPK pathways in the hippocampi of neonatal rats. Exp. Ther. Med. 13, 2056–2064. doi:10.3892/etm.2017.4173
Lian, H., Huang, Q. B., and Zhao, Y. Q. (2008). Progress in the study of the chemical composition and biological activity of the Chinese medicine Terminalia Chebula Retz. Asia-Pacific Tradit. Med., 46–48. doi:10.3321/j.issn:0253-2670.2007.12.051
Lin, L., Xu, H., Liu, J., and Wang, X. (1996). Study on the volatile constituents of Terminalia chebula retz. J. Chin. Med. Mat., 462–463. doi:10.13863/j.issn1001-4454.1996.09.019
Lin, T. C., Nonaka, G. I., Nishioka, I., and Ho, F. c. (1990). Tannins and related compounds. CII.: structures of terchebulin, an ellagitannin having a novel tetraphenylcarboxylic acid (terchebulic acid) moiety, and biogenetically related tannins from Terminalia chebula RETZ. Chem. Pharm. Bull. 38, 3004–3008. doi:10.1248/cpb.38.3004
Liu, F., Qin, H. F., and Liu, S. Q. (2012). Progress in the study of chemical composition and pharmacological activity of Terminalia Chebula Retz. China Pharm., 670–672. doi:10.6039/j.issn.1001-0408.2012.07.35
Liu, H., Zhao, C. Y., Zhang, W., Zhang, Y., and Nie, J. (2018). Study on medication laws of Tibetan medicine in treatment of plateau disease based on data mining technology. Zhongguo Zhong Yao Za Zhi 43, 1726–1731. doi:10.19540/j.cnki.cjcmm.20180112.001
Liu, J. H., Qi, Y. H., and Yang, G. C. (2023). Structure analysis of brain volume in patients with mild cognitive impairment at high altitude. Chin. J. Magn. Reson Imaging, 15–18+32. doi:10.12015/issn.1674-8034.2023.12.003
Liu, P. (2016). The protection of several polyphenolson on memory of rats under hypobaric hypoxia and the underying molecular mechanism. Army Medical University.
Liu, Y. Z., Yu, B. W., Ding, G., et al. (1998). Terminalic acid, a new tannin from the fruit of Terminalia chebula. Chin. Chem. Lett. 827-828. CNKI:SUN:FXKB.0.1998-09-013.
Lu, P., Liu, X., Li, X., and Wen, J. (1991). Studies on the chemical constituents of fruits of Terminalia chebula Retz. Acta Univ. Tradit. Medicalis Sin. Pharmacol. Shanghai, 233–235.
Lu, P. P., Liu, X. K., Li, X. C., and Zhang, D. C. (1992). Studies on the triterpene composition of Terminalia chebula retz. J. Integr. Plant Biol., 126–132.
Luo, Z., Wu, Z., Zhong, T., Liu, T., Liu, C., and Huang, S. (2016). Deveolopment and reliability analysis of near infrared spectroscopy (NIR) models to forecast the content of gallic acid in Terminalia chebula. J. Kashi Univ., 32–34+37. doi:10.13933/j.cnki.2096-2134.2016.06.009
Mani, V., Sajid, S., Rabbani, S. I., Alqasir, A. S., Alharbi, H. A., and Alshumaym, A. (2021). Anxiolytic-like and antidepressant-like effects of ethanol extract of Terminalia chebula in mice. J. Tradit. Compl Med. 11, 493–502. doi:10.1016/j.jtcme.2021.04.003
Manosroi, A., Jantrawut, P., Akazawa, H., Akihisa, T., and Manosroi, J. (2010). Biological activities of phenolic compounds isolated from galls of Terminalia chebula Retz. (Combretaceae). Nat. Prod. Res. 24, 1915–1926. doi:10.1080/14786419.2010.488631
Mansouri, M. T., Farbood, Y., Naghizadeh, B., Shabani, S., Mirshekar, M. A., and Sarkaki, A. (2016). Beneficial effects of ellagic acid against animal models of scopolamine- and diazepam-induced cognitive impairments. Pharm. Biol. 54, 1947–1953. doi:10.3109/13880209.2015.1137601
Matsunaga, S., Fujishiro, H., and Takechi, H. (2019). Efficacy and safety of cholinesterase inhibitors for mild cognitive impairment:A systematic review and meta-analysis. J. Alzheimers Dis. 71, 513–523. doi:10.3233/JAD-190546
Mirshekar, M. A., Sarkaki, A., Farbood, Y., Naseri, M. K. G., Badavi, M., Mansouri, M. T., et al. (2018). Neuroprotective effects of gallic acid in a rat model of traumatic brain injury: behavioral, electrophysiological and molecular studies. Iran. J. Basic Med. Sci. 21, 1056–1063. doi:10.22038/IJBMS.2018.29639.7165
Nigam, M., Mishra, A. P., Adhikari-Devkota, A., Dirar, A. I., Hassan, M. M., Adhikari, A., et al. (2020). Fruits of Terminalia chebula Retz.: a review on traditional uses, bioactive chemical constituents and pharmacological activities. Phytother. Res. 34, 2518–2533. doi:10.1002/ptr.6702
Olchik, M. R., Farina, J., Steibel, N., Teixeira, A. R., and Yassuda, M. S. (2013). Memory training (MT) in mild cognitive impairment (MCI) generates change in cognitive performance. Arch. Gerontol. Geriatr. 56, 442–447. doi:10.1016/j.archger.2012.11.007
Peritogiannis, V., Roganaki, A., Siarava, E., and Samakouri, M. (2022). Mild cognitive impairment in rural areas: research advances and implications for clinical practice and healthcare policy. Healthcare-Basel 1340, 1340. doi:10.3390/healthcare10071340
Petersen, R. C. (2011). Clinical practice. Mild cognitive impairment. N. Engl. J. Med. 364, 2227–2234. doi:10.1056/NEJMcp0910237
Pfundstein, B., El Desouky, S. K., Hull, W. E., Haubner, R., Erben, G., and Owen, R. W. (2010). Polyphenolic compounds in the fruits of Egyptian medicinal plants (Terminalia bellerica, Terminalia chebula and Terminalia horrida): characterization, quantitation and determination of antioxidant capacities. Phytochemistry 71, 1132–1148. doi:10.1016/j.phytochem.2010.03.018
Poptsi, E., Tsatali, M., Agogiatou, C., Bakoglidou, E., Batsila, G., Dellaporta, D., et al. (2022). Longitudinal cognitive and physical training effectiveness in MCI, based on the experience of the alzheimer’s hellas day care Centre. J. Geriatr. Psychiatry Neurol. 35, 512–526. doi:10.1177/08919887211016057
Portet, F., Ousset, P. J., Visser, P. J., Frisoni, G. B., Nobili, F., Scheltens, P., et al. (2006). Mild cognitive impairment (MCI) in medical practice: a critical review of the concept and new diagnostic procedure. Report of the MCI Working Group of the European Consortium on Alzheimer’s Disease. J. Neurol. Neurosurg. Psychiatry 77 (6), 714–718. doi:10.1136/jnnp.2005.085332
Qi, Q. (2018). Study on the extraction and purification process of chebulin tannin parts and quality control method. Beijing Univ. Chin. Med. doi:10.26973/d.cnki.gbjzu.2018.000254
Qu, J., Zhou, Q., Du, Y., Zhang, W., Bai, M., Zhang, Z., et al. (2014). Rutin protects against cognitive deficits and brain damage in rats with chronic cerebral hypoperfusion. Br. J. Pharmacol. 171, 3702–3715. doi:10.1111/bph.12725
Rahimi, V. B., Askari, V. R., Shirazinia, R., Soheili-Far, S., Askari, N., Rahmanian-Devin, P., et al. (2018). Protective effects of hydro-ethanolic extract of Terminalia chebula on primary microglia cells and their polarization (M (1)/M (2) balance). Mult. Scler. Relat. Disord. 25, 5–13. doi:10.1016/j.msard.2018.07.015
Rai, A. K., and Joshi, R. (2009). Evaluation of antimicrobial properties of fruit extracts of Terminalia chebula against dental caries pathogens. Jundishapur J. Microb., 105–111.
Rao, N. K., and Nammi, S. (2006). Antidiabetic and renoprotective effects of the chloroform extract of Terminalia chebula Retz. seeds in streptozotocin-induced diabetic rats. BMC Complement. Altern. Med. 17, 17. doi:10.1186/1472-6882-6-17
Reddy, B. M., Rao, N. K., Ramesh, M., Rao, A. V. N. A., Lin, L. J., Lin, L. Z., et al. (1994). Chemical investigation of the fruits of Terminalia chebula. Pharm. Bio 32, 352–356. doi:10.3109/13880209409083014
Reddy, D. B., Reddy, T. C., Jyotsna, G., Sharan, S., Priya, N., Lakshmipathi, V., et al. (2009). Chebulagic acid, a COX-LOX dual inhibitor isolated from the fruits of Terminalia chebula Retz., induces apoptosis in COLO-205 cell line. J. Ethnopharmacol. 124, 506–512. doi:10.1016/j.jep.2009.05.022
Richetti, S. K., Blank, M., Capiotti, K. M., Piato, A. L., Bogo, M. R., Vianna, M. R., et al. (2011). Quercetin and rutin prevent scopolamine-induced memory impairment in zebrafish. Behav. Brain Res. 217, 10–15. doi:10.1016/j.bbr.2010.09.027
Roberts, R., and Knopman, D. S. (2013). Classification and epidemiology of MCI. Clin. Geriatr. Med. 29, 753–772. doi:10.1016/j.cger.2013.07.003
Saha, S., and Verma, R. J. (2015). In vitro and in silico study of antioxidant effect of Bergenia ciliata and Terminalia chebula against sodium oxalate induced oxidative stress. Toxicol. Environ. Health Sci. 7, 50–57. doi:10.1007/s13530-015-0220-6
Sarkaki, A., Hoseinynejad, S., Khombi Shooshtari, M., and Rashno, M. (2022). Synaptic plasticity and cognitive impairment consequences to acute kidney injury: protective role of ellagic acid. Iran. J. Basic Med. Sci. 25, 621–628. doi:10.22038/IJBMS.2022.62015.13729
Singh, A., Bajpai, V., Kumar, S., Sharma, K. R., and Kumara, B. (2016). Profiling of gallic and ellagic acid derivatives in different plant parts of Terminalia arjuna by HPLC-ESI-QTOF-MS/MS. Nat. Product. Commun. 11 (2), 239–244. doi:10.1177/1934578x1601100227
Sireeratawong, S., Jaijoy, K., Panunto, W., Nanna, U., Lertprasertsuke, N., and Soonthornchareonnon, N. (2013). Acute and chronic toxicity studies of the water extract from dried fruits of Terminalia bellerica (Gaertn.) Roxb. In Spargue-Dawley rats. Afr. J. Tradit. Complement. Altern. Med. 10, 223–231. doi:10.4314/ajtcam.v10i2.6
Skaper, S. D., Facci, L., Zusso, M., and Giusti, P. (2017). Synaptic plasticity, dementia and alzheimer disease. CNS and neurol disord drug targets 3, 220–233. doi:10.2174/1871527316666170113120853
Sornwatana, T., Bangphoomi, K., Roytrakul, S., Wetprasit, N., Choowongkomon, K., and Ratanapo, S. (2015). Chebulin: Terminalia chebula Retz. fruit-derived peptide with angiotensin-I-converting enzyme inhibitory activity. Biotechnol. Appl. Bioc 62, 746–753. doi:10.1002/bab.1321
Spencer, J. P. (2010). Beyond antioxidants: the cellular and molecular interactions of flavonoids and how these underpin their actions on the brain. Proc. Nutr. Soc. 69, 244–260. doi:10.1017/S0029665110000054
Suganthy, N., Muniasamy, S., and Archunan, G. (2018). Safety assessment of methanolic extract of Terminalia chebula fruit, Terminalia arjuna bark and its bioactive constituent 7-methyl gallic acid: in vitro and in vivo studies. Regul. Toxicol. Pharmacol. 92, 347–357. doi:10.1016/j.yrtph.2017.12.019
Sun, X. Y., Li, L. J., Dong, Q. X., Zhu, J., Huang, Y. R., Hou, S. J., et al. (2021). Rutin prevents tau pathology and neuroinflammation in a mouse model of Alzheimer’s disease. J. Neuroinflammation 131. doi:10.1186/s12974-021-02182-3
Tang, Y., Gao, C., Xing, M., Li, Y., Zhu, L., Wang, D., et al. (2012). Quercetin prevents ethanol-induced dyslipidemia and mitochondrial oxidative damage. Food Chem. Toxicol. 50, 1194–1200. doi:10.1016/j.fct.2012.02.008
Tomycz, N. D., and Friedlander, R. M. (2011). Battling brain diabetes: antioxidants may reduce cognitive deficits induced by cholesterol. Neurosurgery 68, N15–N17. doi:10.1227/01.neu.0000398207.79197.5d
Van der Mussele, S., Bekelaar, K., Le Bastard, N., Vermeiren, Y., Saerens, J., Somers, N., et al. (2013). Prevalence and associated behavioral symptoms of depression in mild cognitive impairment and dementia due to Alzheimer’s disease. Int. J. Geriatr. Psych. 28, 947–958. doi:10.1002/gps.3909
Vauzour, D., Vafeiadou, K., Rodriguez-Mateos, A., Rendeiro, C., and Spencer, J. P. (2008). The neuroprotective potential of flavonoids: a multiplicity of effects. Genes Nutr. 3, 115–126. doi:10.1007/s12263-008-0091-4
Walia, H., Kumar, S., and Arora, S. (2011). Comparative analysis of antioxidant and phenolic content of chloroform extract/fraction of Terminalia chebula. J. Basic Clin. Pharm. 2, 143–149.
Wang, J. Y. (2018). Isolation and identification of the chemical constituents of Terminalia Chebula fruits and comparison of the effects of two fractionation methods. Inner Mongolia University.
Wang, W., Ali, Z., Li, X. C., Shen, Y., and Khan, I. A. (2010). Triterpenoids from two Terminalia species. Planta Med. 76, 1751–1754. doi:10.1055/s-0030-1249809
Wang, W., Wang, S., Liu, Y., Wang, X., Nie, J., Meng, X., et al. (2022). Ellagic acid: a dietary-derived phenolic compound for drug discovery in mild cognitive impairment. Front. Aging Neurosci. 925855, 925855. doi:10.3389/fnagi.2022.925855
Wang, W., Yang, L., Liu, T., Ma, Y., Huang, S., He, M., et al. (2020a). Corilagin ameliorates sleep deprivation-induced memory impairments by inhibiting NOX2 and activating Nrf2. Brain Res. Bull. 160, 141–149. doi:10.1016/j.brainresbull.2020.03.010
Wang, W., Yang, L., Liu, T., Wang, J., Wen, A., and Ding, Y. (2020b). Ellagic acid protects mice against sleep deprivation-induced memory impairment and anxiety by inhibiting TLR4 and activating Nrf2. Aging (Albany NY) 12, 10457–10472. doi:10.18632/aging.103270
Wang, W., Zhang, Q., Yang, W., Hao, J., Chen, J., An, Y., et al. (2024). Determination of chebulagic acid and chebulinic acid in Terminalia Chebula Retz.by high performance liquid chromatography. Chem Anal Meterage, 22–26+64. doi:10.3969/j.issn.1008-6145.2024.01.005
Wiesli, D., Meyer, A., Fuhr, P., and Gschwandtner, U. (2017). Influence of mild cognitive impairment, depression, and anxiety on the quality of life of patients with Parkinson disease. Dement. Geriatr. Cogn. Dis. Extra 7, 297–308. doi:10.1159/000478849
Yang, J. R., Sun, F. Y., Li, Z. H., Du, G. H., and Qing, H. L. (2008). Chemical constituents from Terminalia chebula retz. Nat. Prod. Res. Dev., 450–451. doi:10.16333/j.1001-6880.2008.03.028
Yang, W. J., Zeweng, Y. Z., Zhang, Y., Geng, Z. J., and Nie, J. (2017). Analysis on composition principles of Tibetan medicine containing Terminalia chebula by data mining. Zhongguo Zhong Yao Za Zhi 42, 1207–1212. doi:10.19540/j.cnki.cjcmm.20170121.019
Yang, X. Y., and Tang, R. P. (2012). Studies on chemical compoents of chebulinic. Journal of Xichang University (Natural Science Edition, 65–66.
Yang, Y. (2016). Progress in the study of chemical composition, biological activity and analytical methods of Terminalia Chebula Retz. Xizang Sci. Technol., 34–39. doi:10.3969/j.issn.1004-3403.2016.09.011
Yang, Y., Lu, J., and Zuo, Y. (2018). Changes of synaptic structures associated with learning, memory and diseases. Brain Sci. Adv. 4, 99–117. doi:10.26599/BSA.2018.2018.9050012
Yangmao, C. (2017). Study on Tibetan medical external therapies for geriatric and aging disorders. Qinghai University.
Yao, G., Miao, X., Wu, M., Lv, Z., Bai, Y., Chang, Y., et al. (2023). Pharmacokinetics of active compounds of a Terminalia chebula Retz. Ethanolic extract after oral administration rats using UPLC-MS/MS. Front. Pharmacol. 14, 1067089. doi:10.3389/fphar.2023.1067089
Zhabokritsky, A., Clarke, R., Rosenes, R., Smith, G., Loutfy, M., Andany, N., et al. (2023). Correlates of healthy aging in geriatric HIV (CHANGE HIV)-CTN 314. Viruses 517, 517. doi:10.3390/v15020517
Zhang, H. L., Chen, K., Pei, Y. H., and Hua, H. M. (2001). Research on the chemical constituents of Terminalia chebula Retz. J Shenyang Pharmaceutical University, 417–418.
Zhang, X. J., He, L. J., Lu, Q., and Li, D. Y. (2016). Pharmacological activity of Terminalia chebula. Zhongguo Zhong Yao Za Zhi 41, 619–623. doi:10.4268/cjcmm20160412
Zhang, Y., Liu, X., Gao, S., Qian, K., Liu, Q., and Yin, X. (2017). Research on the neuro-protective compounds in Terminalia chebula retz extracts in-vivo by UPLC–Q-TOF-MS. Acta Chromatogr. 30, 169–174. doi:10.1556/1326.2017.00147
Zhang, Y. Y., Zeng, H. T., Yuan, Y. J., and Li, X. M. (2018). Progress in the study of the chemical composition and pharmacological activity of the Tibetan medicine Terminalia Chebula Retz. China Pharm. 2002–2006. doi:10.6039/j.issn.1001-0408.2018.14.29
Zhao, H. H., Li, Y. J., Guo, Z. H., and Chen, J. (2022). Screening of acetylcholinesterase inhibitory and antioxidant active compounds from Terminalia chebula fruits by spectrum-effect relationship and liquid chromatography-mass spectrometry analysis. J. Sep. Sci. 45, 3412–3421. doi:10.1002/jssc.202200295
Zhao, J., Yao, Y., Zhang, T., and Yang, Z. (2010). Quercetin protects against glutamate-induced injury of PC12 cell and modulates potassium channels in hippocampal CA1 pyramidal neurons. Acta Biophys. Sin. 799–804. doi:10.1016/S1876-3804(11)60004-9
Zhao, L., Zhang, S. L., Tao, J. Y., Pang, R., Jin, F., Guo, Y. J., et al. (2008). Preliminary exploration on anti-inflammatory mechanism of Corilagin (beta-1-O-galloyl-3,6-(R)-hexahydroxydiphenoyl-D-glucose) in vitro. Int. Immunopharmacol. 8, 1059–1064. doi:10.1016/j.intimp.2008.03.003
Zhi, M., Liu, K., Han, S., Xu, J., Li, W., Li, F., et al. (2020). Influence of different dosage forms on pharmacokinetics of 6 alkaloids in raw aconiti kusnezoffii radix (caowu) and chebulae fructus- (hezi-) processed caowu by UPLC-MS/MS. Biomed. Res. Int. 2020, 1942849. doi:10.1155/2020/1942849
Zhong, L., Liu, H., Zhang, W., Liu, X., Jiang, B., Fei, H., et al. (2018). Ellagic acid ameliorates learning and memory impairment in APP/PS1 transgenic mice via inhibition of β-amyloid production and tau hyperphosphorylation. Exp. Ther. Med. 16, 4951–4958. doi:10.3892/etm.2018.6860
Zhou, K., J, P., Liang, W. Y., Liang, L. J., et al. (2020). Analysis on chemical constituents from Terminalia chebula retz. And Terminalia bellerica (gaertn.) roxb. By UPLC-Q-exactive quadrupole-orbitrap mass spectrometry. J. Chin. Mass Spectrom. Soc. 254–267. doi:10.7538/zpxb.2019.0070
Zhuoma, X., and Renqing, D. (2020). An overview of Tibetan medicine’s understanding of Alzheimer's disease. J Med and Pharm Chin Mino. 71–73. doi:10.16041/j.cnki.cn15-1175.2020.05.029
Glossary
T. Chebula Terminalia chebula Retz.
TCM traditional Chinese medicine
MCI mild cognitive impairment
TCE T. Chebula extract
TCF T. Chebula fruit
EtOAc ethyl acetate
NPS neuropsychiatric symptoms
MAO-A monoamine oxidase alpha
FST forced swim test
TST tail suspension test
EA Ellagic acid
CUMS chronic unpredictable mild stress model
EPM elevated plus maze
OFT open field test
LDT light/dark box test
VCT Vogel’s conflict test
MT memory training
COX-2 cyclooxygenase-2
GFAP glial fibrillary acidic protein
IL-8 interleukin-8
iNOS inducible nitric oxide synthase
SOD superoxide dismutase
LPO lipid peroxide
ROS reactive oxygen species
BDNF brain-derived neurotrophic factor
MCAO middle cerebral artery occlusion
NO nitric oxide
PC12 pheochromocytoma cell
SD sleep disorders
DPPH diphenyl-1-propenylhydrazyl
LPS lipopolysaccharide
Keywords: Terminalia chebula, MCI, Tibetan medicine, safety evaluation, phytochemistry, quality control, traditional medicinal uses
Citation: Gao H, Lu H, Fang N, Su J, Li R, Wang W and Zhang Y (2024) The potential of Terminalia chebula in alleviating mild cognitive impairment: a review. Front. Pharmacol. 15:1484040. doi: 10.3389/fphar.2024.1484040
Received: 21 August 2024; Accepted: 07 October 2024;
Published: 18 October 2024.
Edited by:
Adolfo Andrade-Cetto, National Autonomous University of Mexico, MexicoCopyright © 2024 Gao, Lu, Fang, Su, Li, Wang and Zhang. This is an open-access article distributed under the terms of the Creative Commons Attribution License (CC BY). The use, distribution or reproduction in other forums is permitted, provided the original author(s) and the copyright owner(s) are credited and that the original publication in this journal is cited, in accordance with accepted academic practice. No use, distribution or reproduction is permitted which does not comply with these terms.
*Correspondence: Wenjun Wang, d2VuanVud2FuZzAxOEAxNjMuY29t; Yi Zhang, emhhbmd5aUBjZHV0Y20uZWR1LmNu; Rui Li, cnVpbGVlQGNkdXRjbS5lZHUuY24=
†These authors have contributed equally to this work and share first authorship