- 1Department of Biochemistry, Microbiology and Biotechnology, School of Pure and Applied Sciences, Kenyatta University, Nairobi, Kenya
- 2Department of Medical Biochemistry, School of Medicine, Mount Kenya University, Thika, Kenya
- 3Centre for Traditional Medicine and Drug Research, Kenya Medical Research Institute, Nairobi, Kenya
Background: Prostate cancer is a significant global health concern, particularly among ageing male populations, with a disproportionately higher burden in sub-Saharan Africa. Conventional treatments, though effective, are costly and cause devastating side effects which limit their clinical benefits. Hence, this study evaluated the in vitro antiprostate cancer properties and secondary metabolites of dichloromethane and ethyl acetate lead extracts of Vitex doniana to explore safer and efficacious natural alternatives based on ethnomedicinal claims.
Methods: Phytochemical profiling was conducted using gas chromatography-mass spectrometry (GC-MS) analysis to identify secondary metabolites in the extracts. The cytotoxic effects of the extracts were determined through the MTT assay using Vero CCL-81 cells and DU-145 cells. The expression profile of the selected genes (ar, bcl2, caspase-3, cdk1, and p53) in DU-145 cells treated with the study extracts was investigated using RT-qPCR.
Results: GC-MS analysis revealed 10 secondary metabolites in the dichloromethane extract and 27 secondary metabolites in the ethyl acetate extract of V. doniana leaves, with the majority being sesquiterpenes, diterpenoids, and phytosterols. The dichloromethane and ethyl acetate leaf extracts of V. doniana exhibited low cytotoxicity against normal mammalian epithelial cells (Vero CCL-81), with CC50 values of 1,238.85 μg/mL and 964.81 μg/mL, respectively. Besides, the ethyl acetate leaf extract of the studied plant demonstrated potent anti-prostate cancer activity against DU-145 cells, with an IC50 of 35.68 μg/mL and a high selectivity index (SI) of 27.04. Likewise, the dichloromethane leaf extract of this plant displayed cytotoxic effects (IC50: 287.01 μg/mL) and a selectivity index of 4.32. The reference drug (Doxorubicin) showed a higher toxicity against Vero CCL-81(IC50: 0.41 μg/mL) and DU-145 (IC50: 0.28 μg/mL) cells and a lower selectivity index of 1.46. The DU-145 cells treated with the studied plant extracts exhibited notable upregulation of ar and bcl2, and normalization of caspase 3, cdk1 and p53 expression.
Conclusion: The studied plant extracts possess in vitro anti-prostate cancer properties and could be promising candidates for further preclinical studies aimed at developing novel botanical-based therapies for the management of prostate cancer.
1 Introduction
Prostate cancer remains a significant public health challenge, particularly among aging male populations, and its global prevalence continues to rise despite advances in diagnostics and treatments (Menegoz et al., 2019). The burden of cancer is disproportionately high in low- and middle-income countries (LMICs), particularly in sub-Saharan Africa, such as Kenya (Ministry of Health MOH-Kenya, 2021; Sung et al., 2021). This disparity is attributed to a complex interplay of genetic, socioeconomic, and environmental factors (Menegoz et al., 2019). Epidemiological data shows prostate cancer is the leading malignancy among men in Kenya, accounting for approximately 8% (3,582) of new cancer diagnoses and 6.6% (2,029) of cancer-related deaths annually (Ministry of Health MOH-Kenya, 2021; Sung et al., 2021), and is exacerbated by weak healthcare infrastructure, the high cost of cancer treatment, and insufficient access to quality healthcare services (Price et al., 2012; Ocran Mattila et al., 2021). Moreover, despite the advancements in conventional medicine, the current therapeutic interventions for prostate cancer, such as chemotherapy and radiation are costly and often cause severe side effects, limiting their clinical benefits (Ahmed et al., 2018). These limitations underscore the need for alternative therapies that are safe and efficacious to mitigate the existing challenges.
The use of botanical preparations as a complementary or alternative approach to cancer treatment has existed in various cultures since antiquity (Kareru et al., 2007; James et al., 2018; Aumeeruddy and Mahomoodally, 2021). However, despite its widespread application, the integration of these botanical therapies into conventional oncology still faces considerable challenges (Gakuya et al., 2020). For instance, there is a paucity of empirical data to validate the safety and therapeutic potential of traditionally utilized botanical products (Gakuya et al., 2020; George, 2011). Furthermore, many botanical preparations contain metabolites that remain unidentified, heightening the risk of toxicity and adverse interactions with conventional cancer therapies (Nasri and Shirzad, 2013; Wanjiru et al., 2022). This highlights the urgent need for scientific investigations to assess the safety, efficacy, and pharmacological actions of these botanical drugs. Such research is crucial for addressing existing knowledge gaps and facilitating the development of evidence-based strategies to harness the therapeutic potential of botanical drugs in cancer care.
Vitex doniana (Sweet), is native Kenyan tree belonging to the Verbenaceae family. It is locally known as “Muhuru” (Kikuyu), “Muekelwet” (Kipsigis), “Mfundu” (Swahili), and “Jwelu” (Luo) (Patrick et al., 2005). Its leaves are used traditionally by various communities to treat microbial infections, inflammatory diseases, and allergies, and cancer (Jean et al., 2019; Das et al., 2022). Previous studies have identified a wide range of secondary metabolites, including alkaloids, terpenes, saponins, flavonoids, as well as essential vitamins (A, B1, C), and minerals such as potassium, iron, magnesium, calcium, and zinc (Das et al., 2022; Ifeanacho et al., 2019; Dah-Nouvlessounon et al., 2023). These metabolites likely contribute to the plant’s medicinal properties. Scientific research has shown that Vitex doniana exhibits antimicrobial, antioxidant, anti-inflammatory, and hepatoprotective activities (Jean et al., 2019; Das et al., 2022; Kamal et al., 2022). However, despite its widespread usage among Kenyan communities to treat prostate cancer, there is a paucity of empirical information to validate its safety and efficacy.
Therefore, this study aimed to investigate the antioxidative stress, anti-prostate cancer, and phytochemical profile of V. doniana to appraise its pharmacological potential. These findings will pave the way for future studies aimed at integrating botanical drugs into clinical applications, offering an integrative approach to prostate cancer management with fewer adverse effects than conventional treatments.
2 Materials and methods
2.1 Plant material
Mature fresh leaves of V. doniana were harvested sparingly from its natural habitat in Mbeere North Sub-County, Embu County, Kenya, according to a procedure outlined by Carter et al. (Carter et al., 2007). This plant was selected based on its ethnomedicinal use by the local community for managing prostate cancer. It was first Identified locally as “Muburu,” by an acknowledged traditional herbalist and then authenticated macroscopically and microscopically by a competent taxonomist (Mr. Kennedy Matheka) at the Department of Botany, of the National Museums of Kenya (NMK/BOT/CTX1/4), where duplicate specimens were preserved for future reference. The collected leaves were transported in woven sisal bags to our research laboratory, at Department of Biochemistry, Microbiology, and Biotechnology, Kenyatta University, where they were air-dried for 2 weeks in a well-ventilated room away from direct sunlight. The dried leaves were ground into a powder using an electric mill, carefully packed in labelled khaki envelopes, and stored on a clean dry shelf until required for extraction.
2.2 Extraction
A modified cold maceration procedure described previously (Harborne, 1998) and adapted by Moriasi et al. (Moriasi et al., 2021) was used in this study. In brief, two 250-g portions of the plant material were separately soaked in 1,000 mL of analytical grade ethyl acetate and dichloromethane, stirred and shaken intermittently for 48 h. After that, the mixtures were decanted and filtered through Whatman No. 1 filter paper. The ethyl acetate and dichloromethane filtrates were concentrated in vacuo at 50°C, and 30°C, respectively, using a rotary evaporator. The percentage yields were calculated according to the method of Truong et al. (Truong et al., 2019), as shown in (Equation 1) and the extracts were stored in brown glass vials awaiting experimentation.
2.3 GC-MS analysis
For this study, analysis of the secondary metabolites in the two plant extracts was performed using a Shimadzu QP 2010-SE GC-MS system with an auto sampler connection following a previously established protocol (Ahmad et al., 2023). Analytical-grade dichloromethane and ethyl acetate were sourced from Sigma-Aldrich. The dichloromethane and ethyl acetate leaf extracts of V. doniana were dissolved in their respective solvents to obtain a concentration of 1 mg/mL. The prepared extract solutions were filtered through 0.45 µm PTFE syringe filters after which they were injected in split mode at a 10:1 into the GC-MS system. Ultrapure Helium (He) was used as the carrier gas at a linear velocity of 35 cm/s. A BPX5 nonpolar column (30 m × 0.25 mm ID; 0.25 μm film thickness) was used for separation. The GC temperature was programmed as follows: 60°C; 10°C/min to 200°C (hold time1min); 10 °C/min to 280°C (10 min). The total runtime was 33 min. Injection temperature was set to 250°C, and interface temperature was set at 250°C. The EI ion source was set at 200°C, working in electron impact (EI) mode at 70 eV. Mass analysis was done in Scan mode, with a scan range of m/z values of 35–550 a.m.u.
2.4 Determination of in vitro cytotoxicity and anti-prostate cancer effects
2.4.1 Preparation of plant extracts
Each extract was precisely weighed (100 mg), dissolved in 10 mL of dimethyl sulfoxide, and vortexed to obtain a working stock solution. From this solution, 100 μL was diluted with phosphate-buffered saline to a final volume of 1,000 μL, resulting in a concentration of 1,000 μg/mL. Then, three-fold serial dilution was performed producing seven concentrations: 1.37 μg/mL, 4.12 μg/mL, 12.35 μg/mL, 37.04 μg/mL, 111.11 μg/mL, 333.33 μg/mL, and 1,000.00 μg/mL. Similarly, the reference drug, Doxorubicin, was serially diluted to produce working concentrations of 0.04 μg/mL, 0.12 μg/mL, 0.37 μg/mL, 1.11 μg/mL, 3.33 μg/mL, 10 μg/mL, and 30 μg/mL.
2.4.2 Cell culture and maintenance
The experimental American Type Culture Collection (ATCC) cell lines were retrieved from the tissue culture laboratory hosted at the Centre for Virus Research (CVR) of the Kenya Medical Research Institute (KEMRI), cultured, and maintained according to previously established protocols (Markossian et al., 2021). Vero CCL-81 cells were grown in Eagle’s Minimum Essential Medium (EMEM) enriched with 10% foetal bovine serum (FBS), 2 mM L-glutamine, and an antibiotic-antimycotic mixture (100 units/mL penicillin and 0.1 mg/mL streptomycin), under sterile conditions. Prostate cancer (DU-145) cells were maintained in high glucose Dulbecco’s Modified Eagle’s Medium (DMEM), supplemented with 10% FBS, 2 mM L-glutamine, and 1% antibiotic-antimycotic mixture. All cell lines were incubated in a humidified (65%) chamber with 5% CO2 at 37°C for 48 h. Cell growth was monitored three times a week, and upon reaching ≥90% confluence, they were trypsinised, passaged, and resuspended in fresh media for subsequent assays.
2.4.3 The 3-(4,5-dimethylthiazol-2-yl)-2,5-diphenyltetrazolium bromide (MTT) assay
The cytotoxicity of plant extracts on Vero CCL-81 cells was assessed in vitro using a modified MTT assay method (Markossian et al., 2021). Briefly, 100 µL of medium was transferred into 96-well plates, where cells were seeded at a density of 2 × 104 cells per well and allowed to attach overnight under specified conditions (Section 2.4.2). Following cell attachment, varying concentrations (0–1,000 μg/mL) of the plant extracts, dissolved in 0.1% dimethyl sulfoxide (DMSO), and the reference drug (doxorubicin) were added to the wells in triplicate, and the plates were incubated for 48 h as described in Section 2.4.2. Subsequently, 10 µL of the MTT reagent (5 mg/mL) was added to each well, and after a 4-h incubation, the supernatant was aspirated and replaced with 100 µL of 0.1% DMSO. The experiment was performed in four replicates. The absorbance of the formazan crystals was measured at 570 nm and used to calculate the percentage cytotoxicity and inhibition of cancer cell proliferation as shown in (Equation 2).
2.4.4 Determination of the extracts’ cytotoxic and antiproliferative efficacy
The median cytotoxic concentrations (CC50) for Vero CCL-81 cells and median inhibitory concentrations (IC50) for DU-145 cells were interpolated from a linear regression plot of percentage cytotoxicity/inhibition versus concentration, to appraise the extracts’ safety and efficacy. Additionally, the selectivity indices (SI) for DU-145 cells were calculated using the formula provided in Equation 3.
2.4.5 Determination of the expression profiles of cancer-associated genes in the DU-145 treated with the selected plant extracts
The expression profile of key genes associated with cancer initiation and progression were analysed using quantitative real-time polymerase chain reaction (RT-qPCR). The DU-145 cells (1 × 106) were seeded in 96-well plates and treated with the plant extracts at concentrations corresponding to their IC50 values and incubated for 48 h at conditions described in Section 2.4.2. Total RNA was extracted using a commercial total RNA Miniprep Kit (Solis BioDyne), and its concentration and purity were assessed using a Nanodrop spectrophotometer (ThermoFisher Scientific). The RNA (2 µg) was reverse transcribed into complementary DNA (cDNA) using a cDNA synthesis kit (Solis BioDyne). Afterward, RT-qPCR of the cDNA was added SYBR Green dye and the target genes (Bcl2, AR, CDK1, p53, caspase 3) were amplified using specific primers (Table 1) using a QuantStudio™ 5 System (ThermoFisher Scientific). Gene expression levels were analysed using the comparative threshold (CT) method and normalized with gapdh and actb. The fold changes were calculated using the relative quantification (2−ΔΔCt) approach (Rao et al., 2013), and expressed as fold changes (fold changes).
2.5 Data management, statistical analysis, and reporting
Quantitative data, from in vitro cytotoxicity and antiproliferative assays were organised using Microsoft Excel (Office 365) before being analysed using GraphPad Prism version 10.2. Descriptive statistics were presented as mean ± standard deviation
3 Results
3.1 Phytochemical compounds identified in the dichloromethane and ethyl acetate leaf extract of Vitex doniana
GC-MS analysis of the dichloromethane and ethyl acetate leaf extracts from V. doniana revealed a diverse array of metabolites, as detailed in Table 2. The dichloromethane extract contained ten distinct metabolites, predominantly diterpenoids, steroids, phytosterols, and fatty acid esters. γ-Sitosterol was the most prevalent metabolite, accounting for 41.75% of the extract, while Stigmasta-3,5-dien-7-one and Stigmast-4-en-3-one accounted for 21.19% and 10.91%, respectively (Table 2). Conversely, Neophytadiene and Phytol acetate were present in small amounts, at 1.18% and 0.51%, respectively (Table 2).
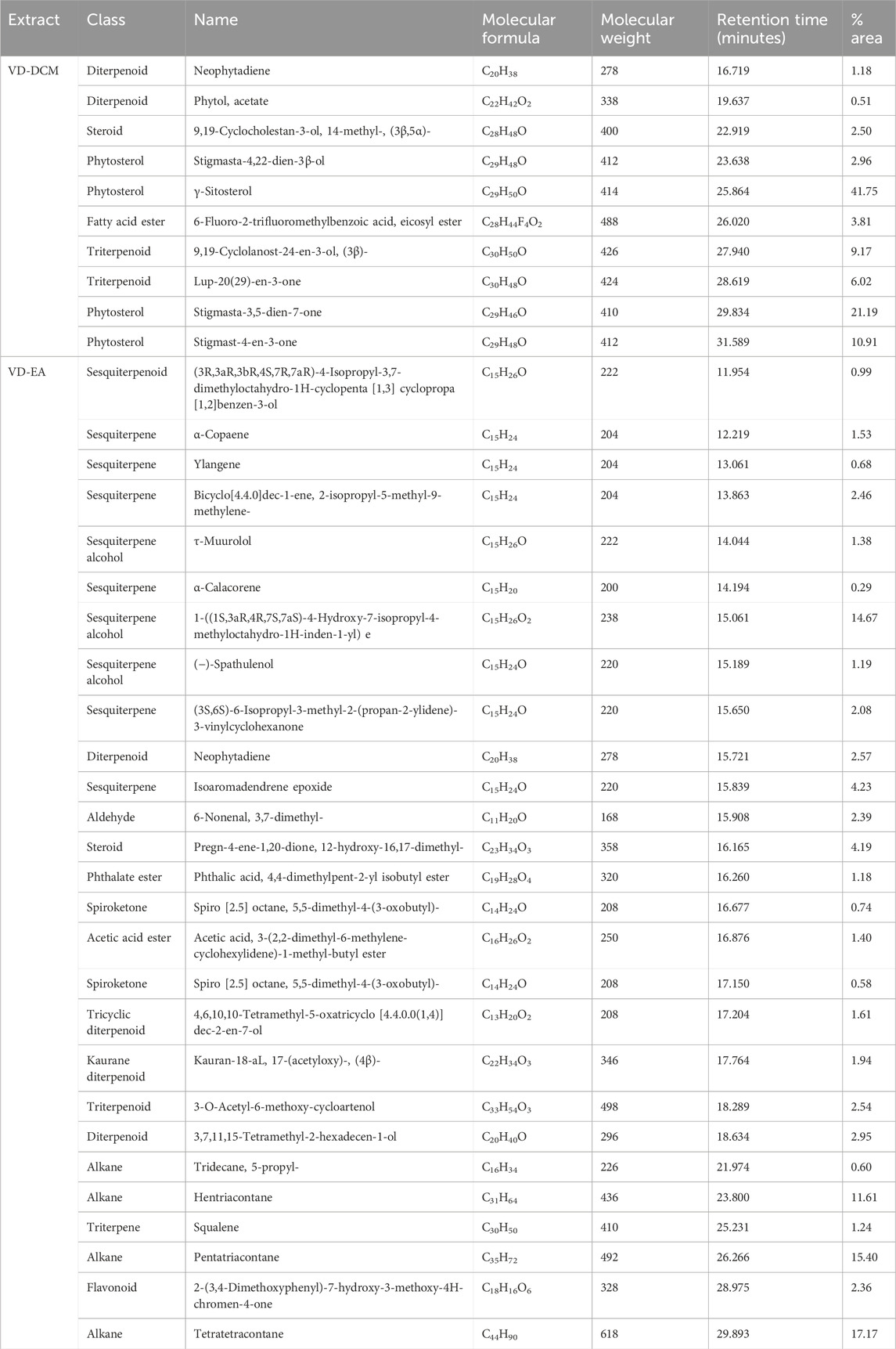
Table 2. Secondary metabolites identified in the Dichloromethane (VD-DCM) and Ethyl Acetate (VD-EA) leaf Extracts of Vitex doniana.
In the ethyl acetate extract, 27 secondary metabolites were identified, including sesquiterpenoids, sesquiterpenes, diterpenoids, and flavonoids (Table 2). Tetratetracontane was the most abundant (17.17%) of the total peak area, followed by pentatriacontane (15.40%), and the sesquiterpene alcohol 1-((1S,3aR,4R,7S,7aS)-4-Hydroxy-7-isopropyl-4-methyloctahydro-1H-inden-1-yl) (14.67%) in the ethyl acetate leaf extract. α-Calacorene (0.29%), Spiro [2.5] octane (0.58%) and Ylangene (0.68%) were the least abundant in the ethyl acetate leaf extract of the study plant (Table 2). Notably, the ethyl acetate extract was rich in alkanes, such as Hentriacontane and Pentatriacontane, as well as various sesquiterpene alcohols and sesquiterpenes (Table 2).
3.2 In vitro cytotoxicity of the leaf extracts of Vitex doniana
3.2.1 Cytotoxicity of the study extracts against Vero CCL-81 cells
Generally, the results showed significant concentration-dependent increases in percentage cytotoxicity in Vero CCL-81 cells treated with the ethyl acetate and dichloromethane leaf extracts of V. doniana (P< 0.001; Figure 1). Comparatively, the ethyl acetate leaf extract of V. doniana exhibited significantly higher percentage cytotoxicity than the dichloromethane extract at a concentration of 1.37 μg/mL (P< 0.001; Figure 1). No significant difference was observed between the percentage cytotoxicity observed in cells treated with the ethyl acetate and dichloromethane extracts at concentrations of 4.12 μg/mL and 12.35 μg/mL (P> 0.001; Figure 1). However, at concentrations of 37.04 μg/mL, 111.11 μg/mL, 333.33 μg/mL, and 1,000 μg/mL, the dichloromethane leaf extract of V. doniana exhibited significantly higher cytotoxicity than the ethyl acetate extract (P< 0.001; Figure 1). Notably, Doxorubicin imparted significant cytotoxicity (P< 0.001) to Vero CCL-81 cells and assumed a concentration-dependent pattern, with a very low CC50 (0.41 μg/mL) as shown in Figure 1. Moreover, the dichloromethane leaf extract of V. doniana had a higher CC50 (1,238.85 μg/mL) compared to the ethyl acetate extract (CC50 = 964.81 μg/mL) (Figure 1).
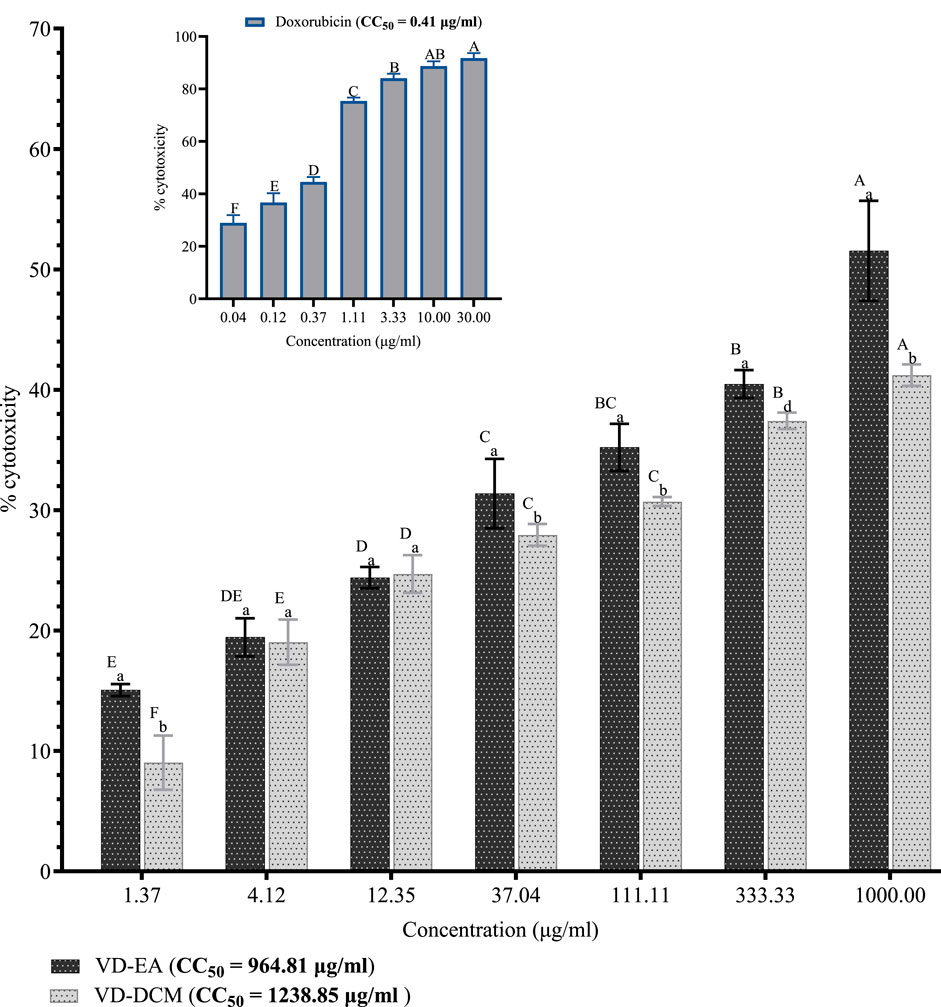
Figure 1. Cytotoxic effects of V. doniana leaf extracts against Vero CCL-81 cells. The results are presented as for four replicate experiments. Bars with different superscript uppercase letters across concentrations are significantly different (One-Way ANOVA with Tukey’s post hoc test), and those with different subscript lowercase letters within the same concentration are significantly different (P< 0.001; unpaired student t-test); VD-DCM: Dichloromethane leaf extract of V. doniana; VD-EA: Ethyl acetate leaf extract of V. doniana; CC50: Median cytotoxic concentration.
3.2.2 Effects of the leaf extracts of Vitex doniana on prostate cancer cells (DU-145)
This study also investigated the cytotoxic effects of the ethyl acetate and dichloromethane leaf extracts of V. doniana on prostate cancer cells (DU-145). The results showed that the percentage inhibitions of DU-145 cell growth by the ethyl acetate leaf extracts of V. doniana increased significantly with increasing concentration (P< 0.001; Figure 2). Notably, the ethyl acetate extract of this plant exerted significantly higher inhibitions of DU-145 cells’ growth than the dichloromethane extract at all the studied concentrations (P< 0.001; Figure 2). Moreover, this study revealed that the IC50 value of the ethyl acetate leaf extract of V. doniana was lower than that of the dichloromethane extract against DU-145 cells, and both were higher than that of doxorubicin (Figure 2).
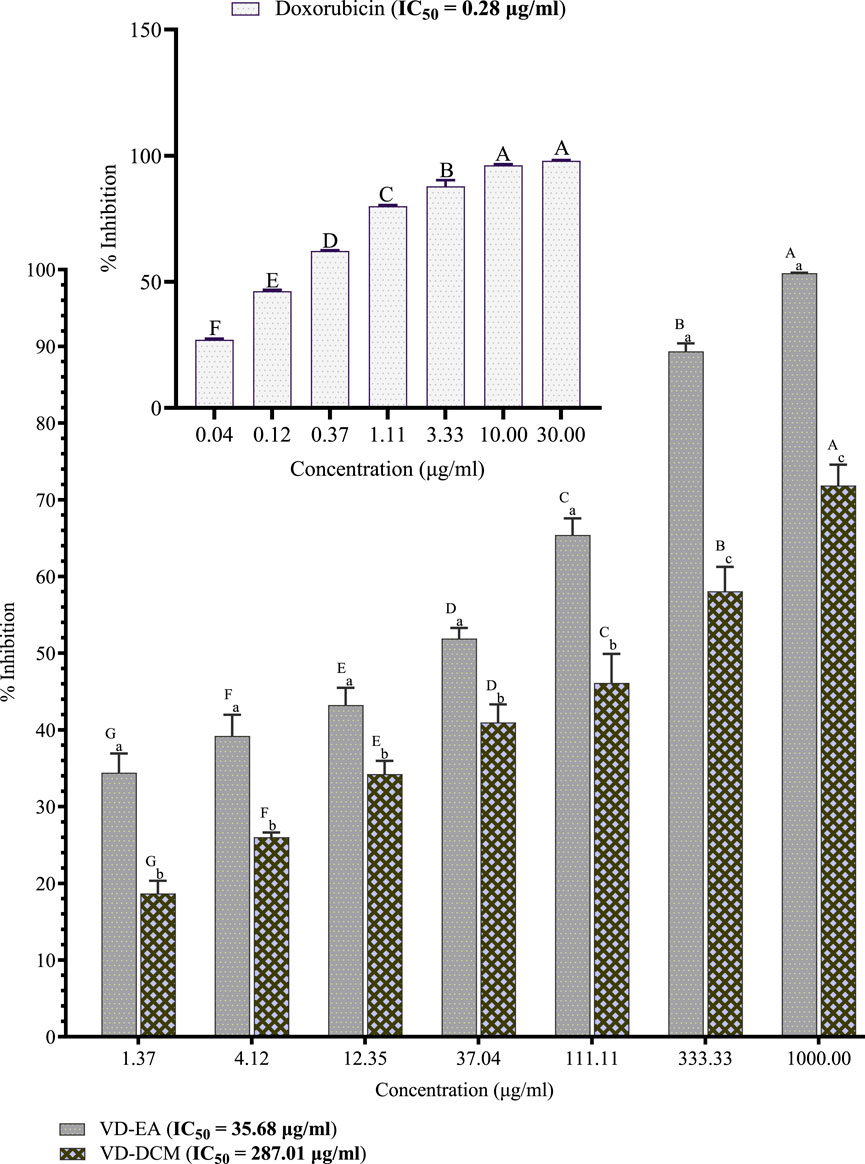
Figure 2. Anti-prostate cancer effects of Vitex doniana leaf extracts against DU-145 cells. The results are presented as for four replicate experiments. Bars with different superscript uppercase letters across concentrations are significantly different (One-Way ANOVA with Tukey’s post hoc test), and those with different subscript lowercase letters within the same concentration are significantly different (P< 0.001; unpaired student t-test); VD-DCM: Dichloromethane leaf extract of V. doniana; VD-EA: Ethyl acetate leaf extract of V. doniana; IC50: Median cytotoxic concentration.
3.2.3 Selectivity indices
The selectivity indices (SI) of each studied plant extracts were computed to determine their ability to exert cytotoxic effects on cancer cells selectively while sparing normal cells. The results showed that the ethyl acetate leaf extract of V. doniana was relatively higher than that of the dichloromethane extract (Table 3).
3.3 Expression levels of selected genes in DU-145 cells treated with the plant extracts
The expression profile of the selected genes in DU-145 cells treated with the plant extracts was determined in this study. The RT-qPCR output is shown in Figure 3 and the results are summarised in Table 4. The results showed that the expression of the ar gene in DU-145 cells treated with the ethyl acetate leaf extract of V. doniana was significantly higher than in similar cells treated with the dichloromethane extract of the same plant (P< 0.001; Table 4). It was also observed that the expression of the bcl2 gene in DU-145 cells treated with the dichloromethane leaf extract of V. doniana was significantly higher than in cells treated with the ethyl acetate extract and the control (P< 0.001; Table 4).

Figure 3. RT-qPCR amplification plot of the selected genes in DU-145 cells treated with the dichloromethane and ethyl acetate leaf extracts of V. doniana.
The results showed a significantly higher expression of the caspase-3 gene in DU-145 cells treated with the dichloromethane leaf extract of V. doniana (P< 0.001); however, these expression levels were significantly lower than that observed in the control cells (P< 0.001; Table 4). Besides, the expression levels of cdk-1 and p53 genes in DU-145 cells treated with the ethyl acetate leaf extract of V. doniana were significantly higher than those observed in cells treated with the dichloromethane extract (P< 0.001); however, these expression levels were significantly lower than that observed in control cells (P< 0.001; Table 4).
4 Discussion
Despite significant advancements in modern medicine, anticancer therapy continues to face considerable hurdles, including prohibitive costs, limited availability, resistance to chemotherapy, and the severe side effects, often resulting in detrimental long-term consequences (Zugazagoitia et al., 2016). These challenges are particularly pronounced in less-developed regions, such as Sub-Saharan Africa, where inadequate healthcare infrastructure exacerbates the cancer burden (Sung et al., 2021). Considering these issues, there is an urgent need for alternative approaches that focus on identifying, optimising, and developing efficacious, safe, and cost-effective treatments, particularly those derived from botanical sources. Accordingly, this study investigated the cytotoxicity and anti-prostate cancer potential of the studied extracts as potential sources of safe and efficacious chemotherapeutic lead molecules for drug development.
This study employed Gas Chromatography-Mass Spectrometry (GC-MS) to investigate the secondary metabolite composition of the studied V. doniana leaf extracts. The dichloromethane extract contained ten metabolites, with γ-Sitosterol emerging as the most abundant. γ-Sitosterol possesses considerable anti-inflammatory, hypolipidemic, and anticancer properties, which it exerts by inducing apoptosis and inhibiting cancer cell proliferation (Ahamed et al., 2022). The presence of other bioactive secondary metabolites such as stigmasta-3,5-dien-7-one and Stigmast-4-en-3-one, known for their antioxidant and anti-inflammatory capabilities, further supports the medicinal potential of the plant for developing anticancer agents (Bakrim et al., 2022). Furthermore, lup-20(29)-en-3-one detected in the dichloromethane leaf extract of V. doniana has been reported to exhibit anti-cancer activity towards different types of cancer cells lines, including breast cancer (MCF7), colon carcinoma (HCT116), human lung adenocarcinoma (A549), and prostate cancer (PC3) by promoting apoptosis, modulating the cell cycle regulatory proteins (Yan et al., 2018). Besides, lup-20(29)-en-3-one can prevent tumour angiogenesis and metastasis through its effects on the vascular endothelial growth factor (VEGF) and epithelial-mesenchymal transition (EMT) signalling pathways (Tsepaeva et al., 2017; Liu et al., 2017). Undoubtedly, the antiprostate cancer effects exhibited by this extract in the present study were attributed to these secondary metabolites, whose mechanism of action is believed to be similar to those reported previously (Ahamed et al., 2022; Bakrim et al., 2022; Yan et al., 2018).
The ethyl acetate leaf extract of V. doniana revealed 27 secondary metabolites, including sesquiterpenoids, diterpenoids, aldehydes, phthalate esters, and flavonoids. Research has shown that sesquiterpene alcohols could possibly inhibit the growth and spread of cancer cells by aiming at and inhibiting important tumour-promoting signals in the cell cycle and apoptotic cascade that participate in tumour advancement (do Nascimento et al., 2018). This study identified notable sesquiterpene alcohol, especially 1-((1S,3aR,4R,7S,7aS)-4-Hydroxy-7-isopropyl-4-methyloctahydro-1H-inden-1-yl), e τ-muurolol, and (−)-spathulenol in the ethyl acetate leaf extract of V. doniana, recognised for their anti-inflammatory and anticancer properties (do Nascimento et al., 2018). These metabolites potentially exert their anticancer effects by disrupting critical signalling pathways, including cell cycle regulation and apoptosis, associated with prostate cancer tumour development and progression (Madriwala et al., 2022). The broad spectrum of bioactivities exhibited by the secondary metabolites of the ethyl acetate leaf extract of V. doniana, especially the terpenoids, sesquiterpenes, and diterpenoids, highlight the potential to contribute to cancer treatment (Mandour et al., 2023).
The contrasting profiles of metabolites observed between the dichloromethane and ethyl acetate extracts can be attributed to the varying polarities of the solvents, which influence the types and quantities of metabolites extracted (Truong et al., 2019). These differences suggest that optimising extraction parameters, such as solvent polarity and method efficiency, could enhance the quality and quantity of secondary metabolites isolated from V. doniana and other medicinal plants (Truong et al., 2019). However, translating the promising anticancer potential of these secondary metabolites into clinical applications remains a significant challenge due to issues with standardisation, bioavailability, and regulatory hurdles (Arora et al., 2019). Nonetheless, with continued research and advances in extraction and analytical techniques, the pharmacological promise of V. doniana could pave the way for the discovery and development of novel anticancer therapies.
The in vitro cytotoxicity of the studied plant extracts was examined on both normal mammalian cells (Vero CCL-81) and cancerous cell line (DU-145) using the well-established MTT assay technique (Markossian et al., 2021). This method is renowned for its high sensitivity, reliability, and reproducibility, making it a widely used approach in assessing cell viability for drug toxicity and anticancer efficacy studies (Ghasemi et al., 2021). The assay leverages the activity of mitochondrial NAD(P)H-dependent cellular oxidoreductases, especially succinate dehydrogenase, which reduces MTT dye into a water-insoluble formazan complex. This formazan’s absorbance is measured at 570 nm after dissolution in dimethyl sulphoxide, providing a quantitative assessment of cellular metabolic activity, thereby allowing for the discernment of cytotoxic or cytostatic effects with high accuracy (Ghasemi et al., 2021).
In this study, the cytotoxicity of the plant extracts exhibited a dose-dependent effect, with higher concentrations correlating to increased toxicity towards Vero CCL-81 cells and a marked inhibition of proliferation of DU-145 cells. These findings align with previous research demonstrating a similar concentration-dependent increase in cytotoxicity and growth inhibition, attributed to the presence of specific secondary metabolites, such as γ-sitosterol, stigmasta-3,5-dien-7-one, and stigmast-4-en-3-one (Muruthi et al., 2023). The cytotoxic and antiproliferative actions of these secondary metabolites are thought to occur through mechanisms such as inhibiting cellular efflux at high concentrations (Moyo and Mukanganyama, 2015) and modulating receptor-mediated intracellular signalling pathways, including suppression of endoplasmic reticulum signalling (Almanza et al., 2019). Consistent with previous studies, the dichloromethane and ethyl acetate leaf extracts of V. doniana were found to be non-toxic to normal cells, further substantiating their anti-prostate cancer potential. Notably, the presence of crucial sesquiterpenes, such as τ-muurolol and (−)-spathulenol in the ethyl acetate extract and diterpenes like Neophytadiene, triterpenoids like lup-20(29)-en-3-one as well as phytosterols like Stigmasta-3,5-dien-7-one and stigmast-4-en-3-one in the dichloromethane extract target specific pathways in prostatic cancer, such as averting oxidative stress, reversing inflammation, triggering DNA repair mechanisms, among others, thereby halting its initiation and development (da Silva et al., 2019).
Cancer development in somatic cells is driven by a dynamic and multifaceted accumulation of genetic mutations rather than a singular defect (Somarelli et al., 2020). These mutations trigger uncontrolled cellular proliferation, leading to tumour growth, morphological aberrations, and malignancy due to the disruption of essential genes that regulate the cell cycle, proto-oncogenes, and tumour-suppressor genes (Roszkowska et al., 2022). This deregulation of cellular checkpoints promotes unchecked mutation accumulation, characterized by autonomous growth signalling, resistance to growth inhibitors, evasion of apoptosis, limitless replication potential, angiogenesis, tissue invasion, and metastasis (Ramachandran and Dörk, 2021). The present study aimed to evaluate the expression profiles of key cancer-related genes to assess the efficacy of selected plant extracts, considering their high selectivity indices against DU-145 cells and to elucidate their probable molecular mechanisms of action.
In prostate cancer (PCa) and other cancers linked to sex hormones, the androgen receptor (AR) plays a pivotal role in tumour progression (Eisermann and Fraizer, 2017). Upon ligand binding, AR translocates to the nucleus, dimerises, and binds to androgen response elements (ARE) on target genes, initiating transcriptional activation by recruiting transcriptional machinery (Fujita and Nonomura, 2019). Pharmacological strategies to inhibit AR function often focus on disrupting ligand binding or interactions with coregulatory proteins to impede disease progression (Shah et al., 2020). Nevertheless, resistance to AR-targeting therapies remains a significant challenge in PCa management, often driven by reactivation of the AR axis via amplification, mutations, or the expression of AR variants (ARVs) that maintain constitutive activity independent of androgens, as observed in castration-resistant prostate cancer (Messner et al., 2020). Notably, treatment of DU-145 cells with the dichloromethane and ethyl acetate leaf extracts from V. doniana demonstrated limited influence on AR signalling, suggesting that the antiproliferative effects of these extracts may be mediated through an intricate modulation of other genes’ expression profiles.
Research indicates that bcl-2 plays a critical role in inhibiting apoptosis by preventing cytochrome c release and impeding the activation of caspases, which are essential for cell death (Warren et al., 2019). This overexpression of bcl-2 is a hallmark of various cancers, promoting their progression by initiating angiogenesis and enhancing cell survival (Pisani et al., 2020). Consequently, targeting the bcl-2 gene and its protein product through chemotherapeutic agents has emerged as a pivotal cancer treatment strategy (Qian et al., 2022). Plant extracts that inhibit bcl-2 gene overexpression hold significant potential as anticancer agents or lead compounds in drug development, given their regulatory influence on cell growth, proliferation, cell cycle, DNA repair, and tumour development (Zhang et al., 2021). In this study, DU-145 cells treated with the dichloromethane leaf extract of V. doniana exhibited significantly higher bcl-2 expression than those treated with the ethyl acetate extract of this plant or control cells, underscoring the apoptotic potential of these extracts. These results align with previous research that has demonstrated reduced bcl-2 expression in cancer cells treated with plant extracts, reinforcing their potential to induce apoptosis (Haselager et al., 2020).
Caspase-3 plays a multifaceted role in tumour cell death mechanisms, exhibiting distinct effects compared to other caspases (Kostova et al., 2021). The intrinsic apoptotic pathway is initiated by mitochondrial damage, resulting in the release of cytochrome c, which forms an apoptosome with Apaf-1 and procaspase-9, thereby activating caspase-9 (Silva et al., 2022). This activation subsequently cleaves and activates pro-Caspase-3/7, leading to cell death via cleavage of endogenous substrates (Boice and Bouchier-Hayes, 2020). In contrast, the extrinsic pathway is triggered by tumour necrosis factor-α (TNF-α) binding to death receptors on the cell surface, leading to the activation of caspase-8, which directly cleaves pro-Caspase-3, inducing apoptosis (Kostova et al., 2021). In this study, a normal expression of the Caspase-3 gene was observed in prostate cancer cells (DU-145) treated with dichloromethane and ethyl acetate leaf extracts of V. doniana, suggesting the presence of secondary metabolites that modulate Caspase-3 expression and activity, to execute apoptosis (Pisani et al., 2020). However, research indicate that Caspase-3 overexpression may correlate with reduced survival rates in cancer patients and may promote tumour regrowth, chemotherapy resistance, and other detrimental effects. Thus, Caspase-3 appears to exhibit a paradoxical role in tumour development and progression, necessitating further research to elucidate the complex dynamics between proCaspase-3 and Caspase-3 in cancer (Eskandari and Eaves, 2022).
Cyclin-dependent kinases (CDKs), a family of serine/threonine kinases, play essential roles in controlling both cell division and transcription in response to external and internal signals, with their activation dependent on complex formation with cyclins (Łukasik et al., 2021). The cdk1’s centrality to the G2/M and G1/S phase transitions renders it indispensable for cell cycle progression, with its dysregulation frequently driving oncogenesis (Sofi et al., 2022). Interestingly, analysis of The Cancer Genome Atlas (TCGA) data highlights cdk1’s overexpression in malignancies (Chandrashekar et al., 2022). Notably, treatment with dichloromethane and ethyl acetate extracts of V. doniana normalised cdk1 expression in DU-145 cancer cells, suggesting these extracts may restore cdk1’s function and mitigate prostate cancer progression (Wang et al., 2023).
Acting as a transcription factor, p53 responds to various cellular stress signals—such as oncogene activation, DNA damage, and replication stress—by undergoing post-translational modifications that influence specific gene transcription, dictating cellular outcomes (Voskarides and Giannopoulou, 2023). Recent research has demonstrated p53’s role in pathways involving autophagy, cell metabolism, ferroptosis, and reactive oxygen species production (Hernández Borrero and El-Deiry, 2021). Its mutation is evinced in advanced prostate cancer, where germline and somatic mutations in DNA damage repair genes are prevalent (Roszkowska et al., 2022). In this study, we investigated the expression of the p53 gene in prostate cancer (DU-145) cells treated with plant extracts. Notably, the relatively normal p53 expression observed in cells treated with dichloromethane and ethyl acetate extracts from V. doniana suggests that their secondary metabolites such as phytosterols, triterpenes, sesquiterpenes, and flavonoids probably modulated its expression to drive apoptosis and ultimately avert cancer development and progression (Tsepaeva et al., 2017; Liu et al., 2017; Mandour et al., 2023).
Therefore, considering the longstanding usage of this plant in traditional medicine to treat prostate cancer among other conditions, this study, for the first time, provides a partial validation of its medicinal potential. Besides, the findings reported herein lay a basis for further empirical studies aimed at establishing its in vivo safety and efficacy, potentially leading to the discovery of botanical-based antiprostate cancer drugs.
5 Conclusions and recommendations
Based on the study findings, the dichloromethane and ethyl acetate leaf extracts of V. doniana contain bioactive secondary metabolites with antioxidant, anti-inflammatory, and anticancer properties, positioning them as potential sources of lead compounds for managing oxidative stress-related diseases, especially prostate cancer. These extracts exhibit selective cytotoxicity against prostate cancer cells (DU-145) while sparing normal mammalian cells (Vero CCL-81), underscoring their antiprostate cancer potential. Moreover, these extracts modulated the expression of key genes involved in cancer initiation and progression, providing an insight into their probable molecular mechanism of action. Future research should focus on isolating and characterising these secondary metabolites, assessing their in vivo efficacy and safety, and clarifying their precise mechanisms of action. Additionally, investigations into other extracts of V. doniana and their combinations, as well as translational studies to facilitate clinical application, are recommended.
Data availability statement
All the data and materials used in this study are included in the article. Additional information may be provided by the corresponding author(s) upon reasonable request.
Ethics statement
The present study and all its protocols were approved by the Kenyatta University Ethics Review Committee (reference number: PKU/2652/11787) and the National Commission for Science, Technology, and Innovation (reference number: NACOSTI/P/23/25162).
Author contributions
GM: Writing–review and editing, Writing–original draft, Visualization, Validation, Software, Resources, Project administration, Methodology, Investigation, Funding acquisition, Formal Analysis, Data curation, Conceptualization. MN: Writing–review and editing, Validation, Supervision, Resources, Project administration, Conceptualization. PM: Writing–review and editing, Validation, Supervision, Resources, Project administration, Methodology. GO: Writing–review and editing, Visualization, Validation, Supervision, Software, Resources, Project administration, Methodology.
Funding
The author(s) declare that financial support was received for the research, authorship, and/or publication of this article. This study was partly supported by the German Academic Exchange (DAAD) scholarship for PhD Study granted to Gervason Moriasi under the In-Country/In-Region Scholarship Programme (Ref: 91843013).
Acknowledgments
We are grateful for the technical assistance provided by the laboratory technologists, particularly Daniel Mwaniki (Department of Biochemistry, Microbiology, and Biotechnology, Kenyatta University), Elias Mandela (Department of Biological Sciences, Mount Kenya University), and Sally Kamau and Gilbert Kipkorir (Centre for Traditional Medicine and Drug Research-Kenya Medical Research Institute).
Conflict of interest
The authors declare that the research was conducted in the absence of any commercial or financial relationships that could be construed as a potential conflict of interest.
Publisher’s note
All claims expressed in this article are solely those of the authors and do not necessarily represent those of their affiliated organizations, or those of the publisher, the editors and the reviewers. Any product that may be evaluated in this article, or claim that may be made by its manufacturer, is not guaranteed or endorsed by the publisher.
References
Ahamed, J. I., K, F., Priya, A. V., PremaKumari, J., Steiny, R. P., Kamalarajan, P., et al. (2022). Computational and biological efficacy of stigmasterol against HeLa cells and Vero cells-first time isolated from the ethanolic extract of Annonamuricata Linn leaves. J. Mol. Struct. 1252, 132186. doi:10.1016/j.molstruc.2021.132186
Ahmad, R., Alqathama, A., Alam, M. M., Riaz, M., Abdalla, A. N., Aldholmi, M., et al. (2023). Biological quality and phytochemical profiling of olive fruits using gas chromatography–mass spectrometry (GCMS) analysis. Chem. Biol. Technol. Agric. 10 (1), 45. doi:10.1186/s40538-023-00413-8
Ahmed, M., Khalid, A., Penech, F., and Mesaik, M. A. (2018) Anti prostate cancer, antioxidant activity and cytotoxicity of some Sudanese medicinal plants. Contemporary Chemistry Contemp Chem. 1 (1), 1–5.
Almanza, A., Carlesso, A., Chintha, C., Creedican, S., Doultsinos, D., Leuzzi, B., et al. (2019). Endoplasmic reticulum stress signalling – from basic mechanisms to clinical applications. FEBS J. 286, 241–278. doi:10.1111/febs.14608
Arora, S., and Suvartan, R. (2019). “Phytochemicals: benefits, concerns and challenges,” in Advancement in functional food ingredients. Editors H. Kumar, and N. Kumar (Delhi, India: Jaya Publishing House), 205–227.
Aumeeruddy, M. Z., and Mahomoodally, M. F. (2021). Global documentation of traditionally used medicinal plants in cancer management: a systematic review. South Afr. J. Bot. 138, 424–494. doi:10.1016/j.sajb.2021.01.006
Bakrim, S., Benkhaira, N., Bourais, I., Benali, T., Lee, L. H., El Omari, N., et al. Dietary phenolic compounds as anticancer natural drugs: recent update on molecular mechanisms and clinical trials. Health Benefits Pharmacol. Prop. Stigmasterol.11, 3323, doi:10.3390/foods112133232022
Boice, A., and Bouchier-Hayes, L. (2020). Targeting apoptotic caspases in cancer. Biochim. Biophys. Acta. Mol. Cell Res., 1867, 118688. doi:10.1016/j.bbamcr.2020.118688
Carter, R., Bryson, C. T., and Darbyshire, S. J. (2007). Preparation and use of voucher specimens for documenting research in weed science. Weed Technol. 21 (4), 1101–1108. doi:10.1614/wt-07-007.1
Chandrashekar, D. S., Karthikeyan, S. K., Korla, P. K., Patel, H., Shovon, A. R., Athar, M., et al. (2022). UALCAN: an update to the integrated cancer data analysis platform. Neoplasia (United States) 25, 18–27. doi:10.1016/j.neo.2022.01.001
Dah-Nouvlessounon, D., Esse Agossou, A., Hoteyi, I. M. S., Koda, D., N’tcha, C., Nounagnon, M., et al. (2023). Phytochemical analysis, antioxidant and antimicrobial properties of vitex doniana sweet leaves and fruits extracts. Article Asian J. Biol. Sci. 13 (1), 14–24. doi:10.5923/j.ajb.20231301.03
Das, N., Salgueiro, A. C. F., Choudhury, D. R., Mandal, S. K., Logesh, R., Hassan, M. M., et al. (2022). Traditional uses, phytochemistry, and pharmacology of genus Vitex (Lamiaceae). Phytotherapy Res. 36 (2), 571–671. doi:10.1002/ptr.7330
da Silva, P. V. B., Ramiro, M. M., Iriguchi, E. K. K., Corrêa, W. A., Lowe, J., Cardoso, C. A. L., et al. (2019). Antidiabetic, cytotoxic and antioxidant activities of oil extracted from Acrocomia aculeata pulp. Nat. Prod. Res. 33 (16), 2413–2416. doi:10.1080/14786419.2018.1446006
do Nascimento, K. F., Moreira, F. M. F., Alencar Santos, J., Kassuya, C. A. L., Croda, J. H. R., Cardoso, C. A. L., et al. (2018). Antioxidant, anti-inflammatory, antiproliferative and antimycobacterial activities of the essential oil of Psidium guineense Sw. and spathulenol. J. Ethnopharmacol. 210, 351–358. doi:10.1016/j.jep.2017.08.030
Eisermann, K., and Fraizer, G. (2017) “The androgen receptor and VEGF: mechanisms of androgen-regulated angiogenesis in prostate cancer,”Cancers (Basel)., 9 32. doi:10.3390/cancers9040032
Eskandari, E., and Eaves, C. J. (2022). Paradoxical roles of caspase-3 in regulating cell survival, proliferation, and tumorigenesis. J. Cell Biol., 221, e202201159. doi:10.1083/jcb.202201159
Fujita, K., and Nonomura, N. (2019). Role of androgen receptor in prostate cancer: a review. World J. Men?s Health. Korean Soc. Sex. Med. Androl. 37, 288–295. doi:10.5534/wjmh.180040
Gakuya, D. W., Okumu, M. O., Kiama, S. G., Mbaria, J. M., Gathumbi, P. K., Mathiu, P. M., et al. (2020). Traditional medicine in Kenya: past and current status, challenges, and the way forward. Sci. Afr. 8, e00360. doi:10.1016/j.sciaf.2020.e00360
George, P. (2011). Concerns regarding the safety and toxicity of medicinal plants - an overview. J. Appl. Pharm. Sci. 1 (6), 40–44.
Ghasemi, M., Turnbull, T., Sebastian, S., and Kempson, I. (2021). The mtt assay: utility, limitations, pitfalls, and interpretation in bulk and single-cell analysis. Int. J. Mol. Sci. 22 (23), 12827. doi:10.3390/ijms222312827
Harborne, J. B. (1998). Phytochemical methods. A guide to modern techniques of plant analysis. 5th Edition. London: Chapman and Hall Ltd, 21–72.
Haselager, M. V., Kielbassa, K., Ter Burg, J., Bax, D. J. C., Fernandes, S. M., Borst, J., et al. (2020). Changes in Bcl-2 members after ibrutinib or venetoclax uncover functional hierarchy in determining resistance to venetoclax in CLL. Available at: http://ashpublications.org/blood/article-pdf/136/25/2918/1794551/bloodbld2019004326.pdf.136 2918–2926. doi:10.1182/blood.2019004326
Hernández Borrero, L. J., and El-Deiry, W. S. (2021). Tumor suppressor p53: biology, signaling pathways, and therapeutic targeting. Biochim. Biophys. Acta. Rev. Cancer, 1876, 188556. doi:10.1016/j.bbcan.2021.188556
Ifeanacho, M. O., Ogunwa, S. C., and Amadi, P. U. (2019). Phytochemical composition of vitex doniana. Anal. Chem. Lett. 9 (6), 863–875. doi:10.1080/22297928.2020.1722221
James, P. B., Wardle, J., Steel, A., and Adams, J. (2018). Traditional, complementary and alternative medicine use in Sub-Saharan Africa: a systematic review. BMJ Glob. Health 3 (5), e000895. doi:10.1136/bmjgh-2018-000895
Jean, B. M., Nag-Tiero, M. R., Hermann, O. Y., and Germaine, N. O. (2019). Traditional uses, phytochemistry and pharmacology review of 2 Vitex: diversifolia and doniana. Int. J. Res. Dev. Pharm. and Life Sci. 8 (2), 29–36. doi:10.21276/ijrdpl.2278-0238.2019.8(2).29-36
Kamal, N., Mio Asni, N. S., Rozlan, I. N. A., Mohd Azmi, M. A. H., Mazlan, N. W., Mediani, A., et al. (2022). Traditional medicinal uses, phytochemistry, biological properties, and health applications of vitex sp. Plants (Basel). 11, 1944, doi:10.3390/plants11151944
Kareru, P. G., Kenji, G. M., Gachanja, A. N., Keriko, J. M., and Mungai, G. (2007). Traditional medicines among the Embu and Mbeere peoples of Kenya. Afr. J. Trad. CAM 4 (1), 75–86. doi:10.4314/ajtcam.v4i1.31193
Kostova, I., Mandal, R., Becker, S., and Strebhardt, K. (2021). The role of caspase-8 in the tumor microenvironment of ovarian cancer. Cancer Metastasis Rev. 40, 303–318. doi:10.1007/s10555-020-09935-1
Liu, X. Q., Zou, Q. P., Huang, J. J., Yook, C. S., Whang, W. K., Lee, H. K., et al. (2017). Inhibitory effects of 3α-hydroxy-lup-20(29)-en-23, 28-dioic acid on lipopolysaccharide-induced TNF-α, IL-1β, and the high mobility group box 1 release in macrophages. Biosci. Biotechnol. Biochem. 81 (7), 1305–1313. doi:10.1080/09168451.2017.1301803
Łukasik, P., Załuski, M., and Gutowska, I. (2021). Cyclin-dependent kinases (Cdk) and their role in diseases development–review. Int. J. Mol. Sci. 22, 2935–3033. doi:10.3390/ijms22062935
Madriwala, B., Suma, B. V., and Jays, J. (2022). Molecular docking study of hentriacontane for anticancer and antitubercular activity. Int. J. Chem. Res. 1, 1–4. doi:10.22159/ijcr.2022v6i4.208
Mandour, Y. M., Refaat, E., and Hassanein, H. D. (2023). Anticancer activity, phytochemical investigation and molecular docking insights of Citrullus colocynthis (L.) fruits. Sci. Rep. 13 (1), 20038. doi:10.1038/s41598-023-46867-6
Markossian, S., Grossman, A., Brimacombe Michelle Arkin, K., Auld, D., Austin Jonathan Baell, C. P., Chung, T. D., et al. (2021). Assay guidance manual. Available at: https://ncats.nih.gov/expertise/preclinical/agm, 351–358.
Menegoz, F., Lutz, J. M., Mousseau, M., Orfeuvre, H., and Schaerer, R. (2019). Descriptive epidemiology of prostate cancer. World J. Oncol. 10 (2), 1–6. doi:10.1159/000423644
Messner, E. A., Steele, T. M., Tsamouri, M. M., Hejazi, N., Gao, A. C., Mudryj, M., et al. (2020). The androgen receptor in prostate cancer: effect of structure, ligands and spliced variants on therapy. Biomedicines 8, 422–519. doi:10.3390/biomedicines8100422
Ministry of Health (MOH)-Kenya (2021). National strategic plan for the prevention and control of non-communicable diseases (NCDs) 2021/22-2025/26. Available at: www.health.go.ke.
Moriasi, G. A., Ireri, A. M., Nelson, E. M., and Ngugi, M. P. (2021). In vivo anti-inflammatory, anti-nociceptive, and in vitro antioxidant efficacy, and acute oral toxicity effects of the aqueous and methanolic stem bark extracts of Lonchocarpus eriocalyx (Harms.). Heliyon 7 (5), e07145. doi:10.1016/j.heliyon.2021.e07145
Moyo, B., and Mukanganyama, S. (2015). Antiproliferative activity of T. welwitschii extract on Jurkat T cells in vitro. Biomed. Res. Int. 2015, 817624. doi:10.1155/2015/817624
Muruthi, C. W., Ngugi, M. P., Runo, S. M., and Mwitari, P. G. (2023). In vitro antiproliferative effects and phytochemical characterization of carissa edulis ((Forssk) vahl) and pappea capensis (eckyl and zeyh) extracts. J. Evid. Based Integr. Med. 28, 2515690X231187711. doi:10.1177/2515690X231187711
Nasri, H., and Shirzad, H. (2013). Toxicity and safety of medicinal plants. J. HerbMed Pharmacol. 2 (2), 21–22.
Ocran Mattila, P., Ahmad, R., Hasan, S. S., and Babar, Z. U. D. (2021) “Availability, affordability, access, and pricing of anti-cancer medicines in low- and middle-income countries: a systematic review of literature,”Front. Public Health 9 628744. doi:10.3389/fpubh.2021.628744
Patrick, M., and Tengnas, B. (2005). “Useful trees and shrubs for kenya,” in World Agroforestry Centre-Easte and Central Africa Regional Programme (lCRAF -ECA). Editors S. T. Anna K Lindqvist 35th Edition. World Agroforestry Centre.
Pisani, C., Ramella, M., Boldorini, R., Loi, G., Billia, M., Boccafoschi, F., et al. (2020). Apoptotic and predictive factors by Bax, Caspases 3/9, Bcl-2, p53 and Ki-67 in prostate cancer after 12 Gy single-dose. Sci. Rep. 10 (1), 7050. doi:10.1038/s41598-020-64062-9
Price, A. J., Ndom, P., Atenguena, E., Mambou Nouemssi, J. P., and Ryder, R. W. (2012). Cancer care challenges in developing countries. Cancer 118 (14), 3627–3635. doi:10.1002/cncr.26681
Qian, S., Wei, Z., Yang, W., Huang, J., Yang, Y., and Wang, J. (2022). The role of BCL-2 family proteins in regulating apoptosis and cancer therapy. Front. Oncol., 12, 985363. doi:10.3389/fonc.2022.985363
Ramachandran, D., and Dörk, T. (2021). Genomic risk factors for cervical cancer. Cancers 13, 5137. doi:10.3390/cancers13205137
Rao, X., Huang, X., Zhou, Z., and Lin, X. (2013). An improvement of the 2ˆ (-delta delta CT) method for quantitative real-time polymerase chain reaction data analysis. Biostat. Bioinforma. Biomath. 3 (3), 71–85.
Roszkowska, K. A., Piecuch, A., Sady, M., Gajewski, Z., and Flis, S. (2022). Gain of function (GOF) mutant p53 in cancer-current therapeutic approaches. Int. J. Mol. Sci. 23. doi:10.3390/ijms
Shah, K., Gagliano, T., Garland, L., O’Hanlon, T., Bortolotti, D., Gentili, V., et al. (2020). Androgen receptor signaling regulates the transcriptome of prostate cancer cells by modulating global alternative splicing. Oncogene 39 (39), 6172–6189. doi:10.1038/s41388-020-01429-2
Silva, F. F. V. e., Padín-Iruegas, M. E., Caponio, V. C. A., Lorenzo-Pouso, A. I., Saavedra-Nieves, P., Chamorro-Petronacci, C. M., et al. (2022). Caspase 3 and cleaved caspase 3 expression in tumorogenesis and its correlations with prognosis in head and neck cancer: a systematic review and meta-analysis. Int. J. Mol. Sci. 23, 11937. doi:10.3390/ijms231911937
Sofi, S., Mehraj, U., Qayoom, H., Aisha, S., Almilaibary, A., Alkhanani, M., et al. (2022). Targeting cyclin-dependent kinase 1 (CDK1) in cancer: molecular docking and dynamic simulations of potential CDK1 inhibitors. Med. Oncol. 39 (9), 133. doi:10.1007/s12032-022-01748-2
Somarelli, J. A., Gardner, H., Cannataro, V. L., Gunady, E. F., Boddy, A. M., Johnson, N. A., et al. (2020). Molecular biology and evolution of cancer: from discovery to action. Mol. Biol. Evol. 37, 320–326. doi:10.1093/molbev/msz242
Sung, H., Ferlay, J., Siegel, R. L., Laversanne, M., Soerjomataram, I., Jemal, A., et al. (2021). Global cancer statistics 2020: GLOBOCAN estimates of incidence and mortality worldwide for 36 cancers in 185 countries. CA Cancer J. Clin. 71 (3), 209–249. doi:10.3322/caac.21660
Truong, D. H., Nguyen, D. H., Ta, N. T. A., Bui, A. V., Do, T. H., and Nguyen, H. C. (2019). Evaluation of the use of different solvents for phytochemical constituents, antioxidants, and in vitro anti-inflammatory activities of severinia buxifolia. J. Food Qual. 2019, 1–9. doi:10.1155/2019/8178294Available at: https://www.hindawi.com/journals/jfq/2019/8178294/.
Tsepaeva, O. V., Nemtarev, A. V., Abdullin, T. I., Grigor’Eva, L. R., Kuznetsova, E. V., Akhmadishina, R. A., et al. (2017). Design, synthesis, and cancer cell growth inhibitory activity of triphenylphosphonium derivatives of the triterpenoid betulin. J. Nat. Prod. 80 (8), 2232–2239. doi:10.1021/acs.jnatprod.7b00105
Voskarides, K., and Giannopoulou, N. (2023). The role of TP53 in adaptation and evolution. Cells, 12, 512. doi:10.3390/cells12030512
Wang, Q., Bode, A. M., and Zhang, T. (2023). Targeting CDK1 in cancer: mechanisms and implications. NPJ Precis. Oncol. 7 (1), 58. Available from: https://www.nature.com/articles/s41698-023-00407-7.
Wanjiru, J. N., Maitho, T. E., Mbaria, J. M., Moriasi, G. A., Schum., K., and Lin, W. C. (2022). Subacute toxicity effects of the aqueous shoot extract of Yushania alpina (K. Schum.) W.C.lin in sprague dawley rats: an appraisal of its safety in ethnomedicinal usage. J. Toxicol. 2022, 6283066. doi:10.1155/2022/6283066
Warren, C. F. A., Wong-Brown, M. W., and Bowden, N. A.BCL-2 family isoforms in apoptosis and cancer. 10, 177, doi:10.1038/s41419-019-1407-6Cell Death Dis. 2019.BCL-2 family isoforms in apoptosis and cancer
Yan, X., Yang, L., Feng, G., Yu, Z., Xiao, M., Cai, W., et al. (2018). Lup-20(29)-en-3β,28-di-yl-nitrooxy acetate affects MCF-7 proliferation through the crosstalk between apoptosis and autophagy in mitochondria. Cell Death Dis. 9 (2), 241. doi:10.1038/s41419-017-0255-5
Zhang, L., Lu, Z., Zhao, X., Lu, X., and Xiong, Y. (2021). Targeting ion channels for the treatment of lung cancer. Biochim. Biophys. Acta. Rev. Cancer, 1876, 188629. doi:10.1016/j.bbcan.2021.188629
Keywords: ethnomedicine, MTT assay, RT-qPCR, Vero-CCL-81, DU-145, natural pruducts, gene expression profile
Citation: Moriasi G, Ngugi M, Mwitari P and Omwenga G (2024) In vitro anti-prostate cancer efficacy and phytochemical composition of the dichloromethane and ethyl acetate leaf extracts of Vitex doniana (sweet). Front. Pharmacol. 15:1483856. doi: 10.3389/fphar.2024.1483856
Received: 20 August 2024; Accepted: 29 October 2024;
Published: 14 November 2024.
Edited by:
Arman Zargaran, Tehran University of Medical Sciences, IranReviewed by:
Giuseppe Antonio Malfa, University of Catania, ItalyKiran George, University of Oklahoma Health Sciences Center, United States
Copyright © 2024 Moriasi, Ngugi, Mwitari and Omwenga. This is an open-access article distributed under the terms of the Creative Commons Attribution License (CC BY). The use, distribution or reproduction in other forums is permitted, provided the original author(s) and the copyright owner(s) are credited and that the original publication in this journal is cited, in accordance with accepted academic practice. No use, distribution or reproduction is permitted which does not comply with these terms.
*Correspondence: Gervason Moriasi, gmoriasi1@outlook.com
†ORCID ID: Gervason Moriasi, orcid.org/0000-0001-5604-9987