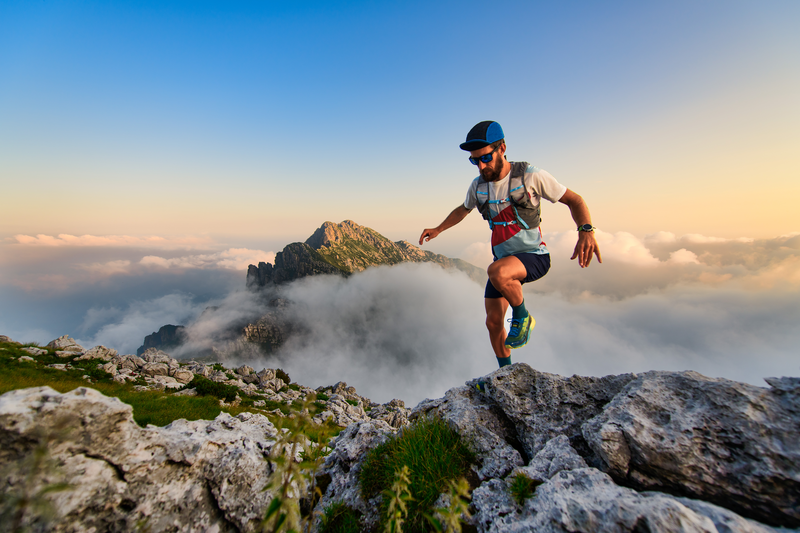
95% of researchers rate our articles as excellent or good
Learn more about the work of our research integrity team to safeguard the quality of each article we publish.
Find out more
REVIEW article
Front. Pharmacol. , 06 January 2025
Sec. Pharmacology of Anti-Cancer Drugs
Volume 15 - 2024 | https://doi.org/10.3389/fphar.2024.1481587
This article is part of the Research Topic Regulated cell death (RCD) in cancer: signaling pathways activated by natural products and their nano-formulations View all 6 articles
The natural world is a vast reservoir of exceptionally varied and inventive chemical compositions. Natural products are used as initial compounds to create combinatorial libraries by targeted modifications and then by analyzing their structure-activity connections. This stage is regarded as a crucial milestone in drug discovery and development. Bergenin, a naturally occurring secondary metabolite, has been extracted from several plant components. It is a constituent found in herbal and Ayurvedic preparations. It demonstrates antiviral, antifungal, antitussive, antiplasmodial, anti-inflammatory, antihepatotoxic, antiarrhythmic, antitumor, antiulcerogenic, antidiabetic, and wound healing activities. Bergenin efficiently inhibited the proliferation of human cancer cells by stimulating the production of intracellular reactive oxygen species (ROS), causing DNA damage and leading to cell cycle arrest in the G1/G2 phases by blocking cell signaling pathways. More comprehensive reviews are needed on the anticancer properties of bergenin. Therefore, our review aimed to update the multifaceted benefits of bergenin to the future scientists and researchers, which can be leveraged to formulate safer and novel cancer therapies, while also establishing a robust framework for future investigations into bergenin in cancer treatment. More preclinical and clinical investigations are needed to validate the candidature of bergenin as a potent anticancer agent.
Bergenin is a bioactive compound found in plants belonging to the genus Bergenia. Studies have shown that bergenin possesses several biological actions, such as anti-inflammatory and immunomodulatory characteristics. Bergenin offers a new and innovative option as an antioxidant, with potential uses in multiple sectors such as pharmaceuticals, cosmetics, and food production (Koul et al., 2020). Bergenin is a compound that consists of trihydroxybenzoic acid and is a derivative of 4-O-methyl gallic acid. It functions as a metabolite. This compound is a hydrolysable phenolic glycoside that is synthesized as colorless crystals. It has limited solubility in water and readily degrades in essential solutions and its stability relies on the circumstances in which it is stored (Salimo et al., 2023). Tschitschibabin et al. (1929) were the first to propose the structure of bergenin (Tschitschibabin et al., 1929). Initially, a chemical structure consisting of merely two rings and a single aliphatic chain was suggested (Ye et al., 2004). Subsequently, in 1950, Shimokoriyama made revisions to the structure and put out a proposal consisting of three rings, each composed of six members. Bergenin possesses five hydroxyl groups deemed to have potential activity (Figure 1). Takahashi et al. synthesized a range of Bergenin derivatives with significantly improved antioxidant activity by chemically catalyzing the esterification of hydroxyl groups using various fatty acids (Takahashi et al., 2003).
It is extracted from many plants, including Bergenia crassifolia (B. crassifolia), Corylopsis spicata (C. spicata), Caesalpinia digyna (C. digyna), Mallotus japonicus (M. japonicas), Sacoglottis gabonensis (S. gabonensis), and among others (Salimo et al., 2023) (Table 1). Several researchers have examined various elements of this plant-derived molecule, but no comprehensive review provides specific information on the medicinal potential of bergenin (Bajracharya, 2015). This review represents the initial effort to provide information on the efficacy of this remarkable herb in combating various types of human carcinomas. However, this method is constrained by the insufficient and inconsistent levels of bergenin found in these medicinal plants. Furthermore, the chemical synthesis of bergenin encounters numerous obstacles, particularly regarding the selective placement of C-sugar and O-methyl groups in certain regions or with specific stereochemistry.
The synthesis of bergenin is intricately linked to the synthesis of gallic acid, which arises from the fusion of erythrose-4-phosphate with phosphoenolpyruvate, resulting in the production of 3-dehydroquinic acid. The dehydration of the latter compound resulted in the production of 3-dehydroshikimic acid, which gets further transformed into gallic acid by oxidation and enolization processes (Patel et al., 2012). Additional potential pathways included the breakdown of the side chain of hydroxycinnamic acids or the combination of an acetyl-CoA group with three malonyl-CoA units. Previous experiments have yielded conflicting results about the C-glycosylation phase. Isotopically labeled benzoic acid and hydroxycinnamic acids have indicated that C-glycosylation is more likely to occur in C6-C3 compounds rather than in C6-C1 derivatives like benzoic acid. Alternatively, when D-[U-14C] glucose is combined with labeled gallic acid, it demonstrates that the C-glycosylation process primarily occurs in the C6-C1 derivative (Taneyama and Yoshida, 1979). The glycosidic component undergoes condensation with the carboxylic acid of the gallic acid section, producing the lactone portion seen in the bergenin structure. Recent investigations indicate that the C-glycosylation process precedes the O-methylation of the phenolic hydroxyl of the gallic acid-derived part by O-methyltransferases (Yan et al., 2024) (Figure 2).
Figure 2. Pictorial representation of the Sequential pathways involved in the synthesis of Bergenin from phosphoenolpyruvic acid and erythrose-4-phosphate (related to gallic acid biosynthetic pathway) (Patel et al., 2012).
Various bergenin derivatives have been extracted from plants, although not all exhibited significant biological activities (Nazir et al., 2011). The primary compounds obtained from bergenin that have this particular potential are demethylated analogues or those that are esterified with phenolic acids such as gallic acid. Notable natural derivatives of bergenin include “riverbergenin” (Ali et al., 2011). Norbergenin was extracted from the leaves of A. japonica and separated from the trunk of R. hypocrateriformis. 11-O-galloylbergenin was isolated from the rhizome of A. gigantifolia, 11-O-veratroylbergenin was extracted from the rhizome of A. gigantifolia, whereas 4-O-galloylnorbergenin was obtained from the stem bark of M. japonicus. 8-O-methylnorbegenin is derived from all parts of S. stolonifera, 11-O-acetylbergenin is obtained from the aerial parts of F. virosa, and 11-O-vanilloylbergenin is extracted from the roots of A. crenata. Three compounds, namely, 4-O-syringoylnorbergenin, 11-O-p-hydroxybenzolynorbergenin, and 4-O-(3′-O-methylgalloyl) norbergenin were isolated from the stem bark of D. sanza-minika (Costa et al., 2021). Furthermore, certain semisynthetic derivatives have been specifically developed to enhance their pharmacological capabilities, necessitating the proposal of various alterations.
Pharmacokinetic studies are essential for determining the effectiveness of natural medications and can also reveal the active ingredients. The pharmacokinetic investigation conducted in the past 10 years has confirmed the intracorporal mechanisms of bergenin (Table 2).
Following data was obtained using smile (OC [C@H]1O [C@@H]2 [C@@H]([C@H]([C@@H]1O)O)OC(=O)c1c2c(O)c (c (c1)O)OC) (From PUBCHEM database) in the SWISS ADME server (http://www.swissadme.ch/index.php) to analyses the pharmacokinetics, drug likeness, and physicochemical properties needed to validate its candidature for drug designing. In Table 2, several parameters including molecular weight, H bond donor and acceptor is mentioned to validate the drug likeness potential of bergenin. Furthermore, scientists also carried out a pharmacokinetic investigation of bergenin when delivered orally to rats at 22.5 mg/kg as a single dose. The findings suggested that bergenin underwent rapid degradation in the digestive system, including a first-pass metabolism, and exhibited limited absorption from the gastrointestinal tract in rats. The absorption parameter of any substance can be evaluated using Caco-2 permeability, P-glycoprotein inhibitors, P-glycoprotein substrates, and human intestinal absorption (HIA). Prior to entering systemic circulation, an oral medication must traverse intestinal cell membranes through passive diffusion, carrier-mediated absorption, or active transport mechanisms. The human colon adenocarcinoma cell lines (Caco-2) serve as a viable model for the human intestinal epithelium and are frequently employed to assess in vivo drug permeability owing to their morphological and functional resemblances. Consequently, Caco-2 cell permeability has emerged as a significant criterion for a viable candidate therapeutic molecule. The anticipated Caco-2 permeability of bergenin is −6.191 log cm/s, and it is measured at < −5.15 log cm/s, indicating that bergenin exhibits poor Caco-2 permeability. The P-glycoprotein inhibitor is a membrane protein that belongs to the ATP-binding cassette transporter superfamily. Bergenin is a highly effective inhibitor of P-glycoprotein. The modulation of P-glycoprotein-mediated transport has considerable pharmacokinetic consequences for P-glycoprotein substrates, which may be utilized for particular therapeutic benefits or lead to contraindications. Bergenin possesses the highest likelihood of being employed as a P-glycoprotein substrate. The absorption of an oral medicine in the human intestine is a fundamental requirement for its perceived efficacy. HIA serves as a potential alternative measure for oral bioavailability, and it is evident that bergenin exhibits inadequate human intestine absorption (less than 30%). A key route of drug absorption and distribution is plasma protein binding (PPB), hence, the affinity of a medication for plasma proteins significantly affects its pharmacodynamic properties. PPB can directly affect oral bioavailability as the free concentration of the drug is compromised when it binds to serum proteins during this process. Bergeninn is stated to possess a proper PPB of 33.498% (predicted value <90%) and has a low therapeutic index. Pharmaceuticals that exert effects on the central nervous system must traverse the blood-brain barrier to access their molecular targets. Conversely, for medications targeting peripheral sites, minimal or no blood-brain barrier penetration may be necessary to prevent central nervous system side effects. Bergenin possesses a modest blood-brain barrier (BBB) permeability, hence restricting its efficacy for central nervous system (CNS) illnesses. Clearance is a crucial pharmacokinetic parameter that, along with the volume of distribution and half-life, determines the dosage frequency of a drug. The clearance value of the bergenin compound is reported to be 8.395 mL/min/kg, indicating a high clearance rate. The half-life of a medication is a composite notion that encompasses clearance and volume of distribution; thus, obtaining accurate estimates of these two qualities is probably more pertinent. The Bergenin compound has a half-life of 0.927.
Following oral ingestion in humans, bergenin was rapidly but partially absorbed, exhibiting a brief half-life and limited bioavailability. According to a study conducted by Jiangsu New College Med, the maximum concentration (Cmax) was observed in plasma 1–4 h after intramuscular dose in dogs. In urine, the Cmax was observed 2–7 h after administration (Yu et al., 2019). Singh et al. determined that the limited ability of bergenin to be absorbed by the body may be due to its susceptibility to breakdown in the digestive system (Singh et al., 2024). The in vivo origin of bergenin was established by the mechanisms occurring in the human body during normal or pathological conditions. The bioavailability of bergenin was determined by administering this bioactive compound at doses of 50 mg/kg and 5 mg/kg, using the oral and intravenous routes, respectively. Following oral administration of bergenin at a dose of 12 mg/kg, the whole amount is excreted in the bile within 24 h. The majority of bergenin, approximately 97.67%, is eliminated during the first 12 h. Within 24 h, approximately 8.97% of the medication is recovered in the bile. Within the first 24 h, 95.69% of the urine included the drug, and only 22.34% of the drug was retrieved within that same period. A 100% recovery of bergenin and its glucuronide metabolite was identified, with 52.51% recovered overall. Specifically, 32.20% was found in urine within 48 h and 20.31% in bile within 24 h (Madaan et al., 2022). The effectiveness of pharmacokinetic tests was determined by measuring the concentration of bergenin in the plasma of rats following intravenous administration at dosages of 30.0, 15.0, and 7.5 mg/kg, using water as the solvent. The doses administered are not correlated with the half-lives for elimination and distribution. The plasma concentration-time graph evaluated a biphasic event characterized by rapid distribution and a prolonged elimination phase. The kinetics of this event were found to follow first-order kinetics. The Vc (central volume of distribution) was 0.67 L/kg, but the Vp (peripheral distribution) was 11.35 L/kg. The presence of bergenin in rats was found to be widely distributed. The T1/2β of bergenin in rats was 4.13 h, indicating a moderate elimination rate (Mehta et al., 2022).
Plants produce bergenin and other natural compounds to adapt to abiotic and biotic challenges. Natural products are a compelling alternative to synthetic medicines because of their proven safety record and ability to target cancer-related characteristics such as inflammation and angiogenesis (Valakatte et al., 2024). These compounds also serve to attract animals and provide protection against ultraviolet radiation. Bergenin has garnered growing interest in recent years because of its occurrence in food and medicinal plants, such as the Amazonian herb “uchi” (Endopleura uchi) (Dhalwal et al., 2008). The fruit of this plant serves both as a food and a medicinal product. Additionally, the oil extracted from its seeds can be used in cooking and treating sinusitis in children and constipation in adults. The seeds of this plant are utilized in the production of handicrafts, for smoking foods, and as amulets (Okoro et al., 2021). Research on uchi fruit pulp has shown that it contains diverse nutrients, including fatty acids, fiber, steroids, mineral salts, and vitamins C and E. Furthermore, research indicates that bergenin possesses several qualities, such as antimalarial, antidiabetic, antioxidant, antiviral, and anti-inflammatory activity (Zhang et al., 2013). The impact of bergenin on oxidative stress and apoptosis were assessed in H9c2 cells (embryonic rat cardiomyocytes), produced by oxygen-glucose deprivation as a model of acute myocardial infarction. Bergenin exhibited a dose-dependent reduction in the impact of oxygen-glucose deprivation by decreasing ROS and MDA production while simultaneously boosting the amount of superoxide dismutase (SOD). Western blot and flow cytometry and analysis demonstrated that bergenin reduced cell death generated by oxygen-glucose deprivation by activating the SIRT1/FOXO3a/MnSOD pathway (Zhang and Chai, 2022). Idiopathic pulmonary fibrosis (IPF) can significantly impair lung function, resulting in life-threatening outcomes, and there is currently a shortage of targeted treatment medications.
Bergenin is an isocoumarin molecule with several biological properties, notably antioxidant action. Bergenin mitigated the development of lung fibrosis caused by bleomycin by alleviating oxidative stress, decreasing the accumulation of the extracellular matrix (ECM), and suppressing the generation of myofibroblasts. Additionally, bergenin stimulated phosphorylation and expression of p62 and activate Nrf2. It was shown that Nrf2 is necessary for the upregulation of p62 induced by bergenin, and the reduction of p62 through knockdown resulted in a decrease in bergenin-induced Nrf2 activity. Significantly, the antioxidant activity of bergenin and its ability to prevent TGF-β-induced ECM deposition and myofibroblast differentiation gets nullified by knocking down Nrf2 or p62. Consequently, p62 and Nrf2 established a regulatory loop for treating pulmonary fibrosis with bergenin as a critical target (Zeng et al., 2022). Bergenin has shown good anticancer potential against numerous carcinomas, including cervical cancer, colorectal cancer, bladder cancer, Oral squamous cell cancer (OSCC), non-small cell lung cancer (NSCLC), osteosarcoma, and prostate cancer (Table 3).
Bergenin exhibited a dose-dependent effect on the viability of HeLa cervical cancer cells, causing a reduction in cell viability. However, the therapeutic impact of bergenin on normal cervical cells was observed to be relatively diminished. Moreover, the primary mechanism by which bergenin exhibits its anticancer properties is the induction of apoptosis in HeLa cervical cancer cells. Significantly, bergenin also increased the expression of Bax and reduced the expression of Bcl-2. The impact of bergenin on the distribution of cell cycle phases in HeLa cells was also examined, revealing that bergenin can trigger G0/G1 cell cycle arrest. In addition, bergenin can hinder the movement of HeLa cancer cells and the process of phosphorylation of STAT3. Bergenin shows potential as a viable option for treating cervical cancer (Shi et al., 2019). The water-based extract of E. agallocha effectively reduced the growth of SiHa cervical cancer cells by triggering autophagy and apoptosis. The simultaneous activation of mitophagy and cell growth arrest at the G2/M phase accompanied this process. These findings suggest that Bergenin, the primary compound in the plant extract, is responsible for its anti-cancer properties (Sultana et al., 2022). Mcl-1, a protein associated with myeloid leukemia 1, is commonly expressed at high levels in several types of cancer and has shown potential as a target for pharmacological therapy. Additional research data reveals a new understanding of how bergenin inhibits the growth of blood vessels in cervical carcinoma cells by affecting various proteins involved in blood vessel formation, such as Galectin-3 and MMP-9. These findings support the potential use of bergenin as a treatment for cervical cancer.
Bergenia ciliata (haw.) Sternb is a widely recognized medicinal plant that has long been utilized for its therapeutic effects in treating various conditions, including diabetes, microbiological infections, and kidney stones. This is due to its notable anti-inflammatory and anti-tussive characteristics. B. ciliata possesses strong anti-cancer characteristics due to its capacity to regulate the unfolded protein response (UPR) and ROS pathways. The methanolic extract of B. ciliata (BcME) was tested on MDA-MB-231 and C6-Glioma cell lines. The plant extract specifically inhibited the cytoprotective UPR by blocking the IRE1-Xbp1 and ATF6 pathways while simultaneously boosting the PERK-ATF4-CHOP pathway, which is known to shift the UPR towards apoptosis. In addition, B. ciliata substantially impacted ROS formation in cancerous cells and hindered the activity of antioxidant enzymes superoxide dismutase and catalase. The findings strongly indicate that BcME cooperates with the UPR and ROS pathways to enhance apoptosis and eradicate cancer cells. Therefore, it can potentially be a valuable source of bioactive compounds targeting cancer cells (Qadri et al., 2023). Bergenin efficiently inhibited oxidative stress and apoptosis in ovarian granulosa cells (OGCs) by regulating the Nrf2 signaling pathway. In conclusion, this study reveals that bergenin can effectively control oxidative stress by activating the Nrf2 pathway, decreasing apoptosis of OGCs, and alleviating ovarian damage in POF mice induced by triptolide. However, the authors could not confirm the role of Nrf2-mediated oxidative stress in the protective effect of bergenin against premature ovarian failure (POF) in our animal trials. To overcome this constraint, future studies can establish a method to deactivate Nrf2 in OGCs, allowing the required validation selectively (Zhu et al., 2024).
It is specifically a C-glycoside of 4-O-methylgallic acid. Reverse docking calculations were utilized to identify potential biological targets of bergenin. The reverse docking analysis indicated that bergenin can target galectin-3. Gelectin-3 is an enzyme that has significant involvement in various cellular processes such as cell-to-cell adhesion, interactions between cells and the extracellular matrix, activation of macrophages, formation of new blood vessels, metastasis, and programmed cell death (apoptosis). Because of its crucial function in cancer, Gelectin-3 is a widely studied target for developing drugs that help combat cancer. The interaction between bergenin and galectin-3 was examined using traditional forward docking calculations. The stability of the galectin-3: bergenin complex was investigated using classical molecular dynamics (MD) simulations. Computations of docking and MD analysis revealed that bergenin can firmly attach to the carbohydrate recognition domain (CRD) of galectin-3. The binding of bergenin to galectin-3 also involves a few robust hydrogen bonds.
Furthermore, a credible π-stacking interaction exists between the aromatic component of bergenin and the His158 residue located at the binding site. A 50-nanosecond molecular dynamics simulation was conducted for the bergenin: galectin-3 complex in a water box with periodic boundary conditions. The MD analysis demonstrated excellent stability of bergenin: galectin-3 complex and validated the accuracy of docking results. These findings supported the hypothesis that bergenin have an inhibitory impact on galectin-3. This study provided fresh insights into the anticancer properties of bergenin and highlighted its potential as a promising candidate for developing more powerful galectin-3 inhibitors. The study offered scientific evidence that supports using plants containing bergenin in cancer treatments within Eastern traditional medicine (Jayakody et al., 2018).
The polyphenol-rich fraction of Bergenia ligulata (PFBL) is derived from a plant commonly utilized in Indian folk and traditional medicine due to its anti-inflammatory and antineoplastic effects. The LCMS study revealed that PFBL is composed of approximately fifteen distinct compounds. PFBL promoted apoptotic cell death in both androgen-dependent LNCaP cells androgen-refractory PC3 and DU145 cells. However, it had no impact on NKE and WI38 cells. Subsequent examination uncovered that PFBL performed its function via increasing ROS through the improved catalytic activity of Monoamine oxidase A (MAO-A). The differential inactivation of the NRF2-antioxidant response pathway by PFBL led to the death of PC3 cells compared to NKE cells. This process involved the activity of GSK-3β, which was assisted by the inhibition of AKT. PFBL effectively decreased the size of PC3-tumor xenografts in NOD-SCID mice both on its own and in combination with Paclitaxel, resulting in a synergistic effect. The tumor tissues in mice treated with PFBL exhibited an increase in the same cell death method found in isolated PC3 cells. This mechanism involved an elevation in the catalytic activity of MAO-A, the creation of ROS, and the activation of the β-TrCP-GSK-3β axis, which leads to the degradation of NRF2. The safety of PFBL in healthy mice was confirmed through tests of blood counts, liver function, and splenocyte sensitivity (Ghosh et al., 2021).
11-O-Galloyl bergenin (OGAL) was extracted from the leaves of Corylopsis coreanas and tested on human osteosarcoma cells. In this study, we discovered that OGAL effectively suppressed the growth of MG63 cells and triggered programmed cell death, as indicated by the presence of cleaved-PARP, cleaved-caspase 3, TUNEL-positive cells, and Annexin V-positive cells. An increase in p53 and p21 levels, BAX expression, and a decrease in Bcl-2 and CDK2 characterized OGAL-induced apoptosis. Furthermore, OGAL triggered autophagy by deactivating AKT, increasing the expression of LC3II, and promoting the production of autophagosomes in MG63 cells. OGAL-induced autophagy was observed to be associated with elevated p38 phosphorylation, but the activities of JNK and ERK1/2 remained unaltered when investigating the MAPK signaling pathway. In addition, experiments on wound healing and Boyden chamber assays demonstrated that OGAL inhibited the migration and invasion of MG63 cells. The study showed that OGAL exhibits anticancer effects by enhancing apoptosis and autophagy through upregulation of p53, AKT, and p38 signaling. These findings suggested that OGAL could be a promising therapeutic approach for treating osteosarcoma (Park et al., 2021).
Inflammation is a defensive biological response to infection and has adverse effects. Despite the numerous pharmacological qualities of bergenin, a derivative of 4-O-methyl gallic acid, there is currently no study that has shown the effectiveness of bergenin in reducing inflammatory mediator levels, including prostaglandin E2 (PGE2) and histamine. Prior administration of bergenin significantly decreased edema caused by carrageenan, compound 48/80, histamine, and PGE2. Furthermore, the safety of bergenin administration is reassuring. The administration of bergenin (2,000 mg/kg) did not result in any observable indicators of toxicity in the screening of vital signs, animal weight, water and food consumption, the weight of significant organs, histological examination, and hematological and biochemical parameters. These findings demonstrate that the bergenin derived from P. dubium Taub possesses anti-inflammatory properties against various inflammation models. It effectively decreases neutrophil migration and mitigates the deleterious effects of lipid peroxidation and oxidative stress (Oliveira et al., 2019). Oxytosis refers to an imbalance between the production and expression of reactive oxygen species and the capacity of a biological system to repair the associated damage. Bergenin provided cellular protection against glutamate-induced toxicity at a concentration of 10 μM, and its effectiveness increased in a dose-dependent manner until reaching maximum protection at 30 μM. As shown by a cell viability experiment, prior administration of bergenin significantly inhibited the increase of glutamate-induced reactive oxygen species. It protected HT22 (murine neuronal cell) cells from glutamate-induced cell death. Protection is diminished by bergenin due to the restoration of glutathione levels. In addition to increasing glutathione activity, the antioxidant’s capacity to eliminate ROS also triggers the activation of the Peroxisome Proliferator-Activated Receptor, peroxisome proliferator-activated receptor gamm (PPARγ), which regulates many proinflammatory cytokines (Shukry et al., 2019).
Hepatic ischemia-reperfusion (IR) hinders the advancement of liver transplantation technology. Bergenin suppressed the release of ROS, decreased the expression of inflammatory markers, and hindered apoptosis and autophagy. In the Bergenin pre-treatment groups, the expression of PPAR-γ-related genes was enhanced. In contrast, the phosphorylation of P38 MAPK, NF-κB p65, and JAK2/STAT1-related proteins was reduced depending on the dosage. Bergenin provided hepatic protection in this model of hepatic ischemia-reperfusion injury by reducing ROS, modulating the release of inflammatory molecules, and regulating the expression of genes linked to apoptosis and autophagy through the PPAR-γ pathway (Xiang et al., 2020). Bergenin’s phenolic hydroxyl groups were shielded using benzyl bromide to form benzyl-protected bergenin. The dibenzyl bergenin compound was subjected to acid treatment in the presence of EDC·HCl and DMAP in CH2Cl2. Subsequently, the resulting product was hydrogenated using Pd/C catalysts, resulting in bergenin ester derivatives. Multiple bergenin derivatives, with triple substitutions at positions 3a, 4a, 5a, 6a, and 7a, and double substitutions at positions 8b and 9b, showed more significant cytotoxicity than bergenin. The study demonstrated that the cytotoxic activity of bergenin esters is significantly influenced by the size of substituents and the lipophilicity of the compounds (De-Biao et al., 2014).
Bergenin is a compound derived from gallic acid known for its antioxidant and hepatoprotective properties. Sodium selenite caused damage to HepG2 cells and in vivo models by disrupting the equilibrium between oxidants and antioxidants. Bergenin had a defensive impact against oxidative stress induced by sodium selenite in HepG2 cells. In addition, bergenin mitigated the liver damage caused by sodium selenite by reducing alkaline phosphatase, plasma aspartate aminotransferase, and alanine aminotransferase. It also produced ROS, decreasing lipid peroxidation in both plasma and liver tissues. This resulted in a reduction in nuclear pyknosis and necrotic areas in the liver. Bergenin also normalized the levels and activities of liver antioxidant enzymes (superoxide dismutase, catalase, and glutathione peroxidase) and restored the balance of hepatic glutathione. Ultimately, bergenin restored the expression of cytochrome P450 2E1 mRNA to its normal levels. Overall, bergenin effectively mitigated liver damage caused by reduced sodium selenite by restoring the equilibrium between oxidants and antioxidants and decreasing lipid peroxidation through various mechanisms (Sriset et al., 2021a).
The hepatoprotective effects of bergenin were investigated against oxidative stress induced by ethanol and tert-butyl hydroperoxide (TBHP) in HepG2 cells. HepG2 cells were simultaneously exposed to ethanol or TBHP (100 µM) and either bergenin or gallic acid for 24 h. Both ethanol and TBHP caused damage to HepG2 cells, resulting in the loss of cell nuclei as observed through cell morphology. Exposure of HepG2 cells to either ethanol or TBHP resulted in a decrease in SOD and catalase (CAT) activities and a depletion of total glutathione (GSH) content in comparison to the control. Bergenin and gallic acid strengthened the physical structure of cells, increased cell survival, reduced lactate dehydrogenase (LDH) toxicity, restored aspartate aminotransferase (AST), alanine transaminase (ALT), and malondialdehyde (MDA) levels, stimulated SOD and CAT activities, and improved the overall GSH content of HepG2 cells treated with ethanol and TBHP. Bergenin demonstrated hepatoprotective action by restoring the oxidant-antioxidant system, making it a promising candidate for hepatoprotective treatment (Sriset et al., 2021b). Fourteen bergenin/cinnamic acid hybrids were synthesized, described, and assessed for their antitumor activity in laboratory settings and living organisms. The researchers synthesized novel bergenin and cinnamic acid hybrids and assessed their antitumor effectiveness through in vitro and in vivo experiments. Chemical 5c demonstrated significant efficacy in stopping the growth of HepG2 cells in the G2/M phase and triggering apoptosis through the mitochondria. This chemical is a promising inhibitor of Akt/Bcl-2 and should be further investigated in preclinical research (Liang et al., 2017).
The administration of Bergenin resulted in a substantial reduction in cell viability and an increase in G1 phase arrest in bladder cancer cells. This was accompanied by a decrease in the expression of Ki67, cyclin D1, and cyclin B1. Bergenin caused apoptosis in bladder cancer cells, as demonstrated by elevated levels of Bax and cleaved caspase-3 proteins and reduced levels of Bcl-2 in cells treated with bergenin. The mechanism investigations demonstrated that bergenin administration effectively stimulated the PPARγ/PTEN/AKT signal pathway, as evidenced by the upregulation of nuclear PPARγ and phosphatase and tensin homolog (PTEN) expression and the downregulation of AKT expression. Furthermore, the treatment of a PPARγ inhibitor reversed the effects of bergenin on the activity of bladder cancer cells, namely, in terms of proliferation, apoptosis, invasion, and migration. The study showed that bergenin can hinder the advancement of bladder cancer by activating the PPARγ/PTEN/AKT signaling pathway. This suggests that bergenin can be used as a therapeutic medication for treating bladder cancer (Liu et al., 2021).
The dried leaves of Bergenia purpurascens were used to produce ethanolic extracts. These extracts were then analyzed, discovering and identifying a new aromatic glycoside called 1-O-β-D-glucopyranosyl-2-methoxy-3-hydroxyl-phenylethene.Additionally, 19 additional compounds (2–20) that were already recognized were also found in the extracts. Compound 1’s structure was elucidated through a comprehensive examination utilizing various analytical techniques (D and 2D NMR). Chemical 1 was assessed for its in vitro growth inhibitory efficacies against selected human cancer cells. Compound 1 exhibited the highest potency level, as indicated by its IC50 values of 14.36 ± 1.04 μM against T24 cells. Subsequent bioactivity research revealed that compound 1 triggered apoptosis in T24 cells (urinary bladder cancer) by modifying anti- and pro-apoptotic proteins, resulting in mitochondrial malfunction and the activation of caspase-3, ultimately leading to cell demise. This study showed that compound 1 can be utilized as a candidate for antitumor chemotherapy (Zhang et al., 2018).
A novel bergenin derivative, 4-O-p-hydroxybenzoylbergenin (compound 1), was extracted from whole plants of Ardisia maclurei Merr., in addition to three previously identified compounds: wogonin (compound 2), wogonoside (compound 3), and α-spinasterol (compound 4). Compound 1 demonstrated inhibitory effects on A549 cells, with an IC50 value of 20 μmol/mL, as reported by Gu et al., in 2016. Bergenin efficiently inhibited the proliferation of colorectal adenocarcinoma cells via stimulating ROS within the cells, causing DNA damage and cell growth arrest during the G1 phase. This action is achieved by blocking the PI3K/AKT/mTOR pathway (Gao et al., 2017). Survivin is highly expressed in various types of human cancers and is associated with an adverse prognosis. Bergenin inhibited the growth and development of cell colonies in A549, H1299, and H460 cells and decreased the amount of survivin protein in a manner that depends on the dosage. The mechanistic study demonstrated that bergenin hindered the phosphorylation process of survivin by controlling the Akt/Wee1/CDK1 signaling pathway. As a result, it strengthened the connection between survivin and E3 ligase Fbxl7. Furthermore, bergenin amplified the activation of cleaved-PARP and caspase 3, hence facilitated the process of apoptosis in NSCLC cells. In the in vivo investigation, it was observed that the tumor volume, weight, and expression of Ki67 and survivin were significantly reduced in the group treated with bergenin. These data demonstrated that bergenin exhibits a notable antitumor effect and is promising as a treatment drug for NSCLC (Li et al., 2022). Hexokinase 2 (HK2), the primary enzyme that controls the speed of glycolysis, is closely linked to the growth and progression of cancer. There is a pressing demand for therapeutic medications that specifically target HK2. Bergenin demonstrated a suppressive impact on the development of cancer cells, decelerated the metabolic process of glycolysis, and induced intrinsic apoptosis in OSCC via lowering the expression of HK2. Bergenin effectively restored the efficacy of radiation therapy in the radio resistant OSCC cells. A comprehensive analysis revealed that bergenin augmented PTEN protein level by promoting the association between PTEN and ubiquitin-specific protease 13 (USP13), thereby boosting the stability of PTEN. Consequently, this pathway ultimately impeded the phosphorylation of protein kinase B (AKT) and inhibited the production of hexokinase 2 (HK2). Bergenin has been identified as a novel therapeutic medication that can efficiently suppress glycolysis, hence overcoming radio resistance in OSCC (Li et al., 2023a).
Chemoresistance significantly contributes to treatment failure and tumor recurrence in persons diagnosed with NSCLC. Survivin, a protein associated with cancer, is often found at high levels in human tumors and is associated with a poor prognosis. Survivin overexpression is essential for maintaining many malignant traits of NSCLC cells. Bergenin suppressed the AKT/Wee1/CDK1 signaling pathway, resulting in a reduction in the phosphorylation of survivin. Consequently, this led to an enhanced association between survivin and E3 ligase Fbxl7, facilitating the ubiquitination process and subsequent survivin degradation. Furthermore, bergenin augmented the resilience of NSCLC cells that had been rendered susceptible to pemetrexed treatment once more. Bergenin exerts a potent anticancer effect on NSCLC by selectively targeting survivin. Therefore, it has excellent potential as a therapeutic medication for treating NSCLC (Li et al., 2023b).
HCT116 cancer cells were exposed to different doses of bergenin during 24 and 48 h. Bergenin exerted a pronounced inhibitory effect on the viability of HCT116 cells. In addition, bergenin caused cells to gather in the G1 phase and led to DNA damage in HCT116 cells. Additionally, it resulted in a significant buildup of intracellular ROS, which is known to cause DNA strand breakage in HCT116 cells. Remarkably, bergenin suppressed the PI3K/AKT/mTOR pathway. In conclusion, Bergenin efficiently inhibited the growth of colorectal adenocarcinoma by causing the production of ROS inside cells, damaging DNA, and resulting in G1 growth phase arrest via blocking the PI3K/AKT/mTOR pathway (Gao et al., 2017). Bergenin exhibited a dose-dependent effect on colorectal cancer (CRC) cells by drastically decreasing their viability and colony formation and promoting apoptosis. Bergenin triggered programmed cell death in colorectal cancer cells using FBW7-mediated Mcl-1 ubiquitination. In vivo experiments demonstrated that bergenin effectively decreased the weight and size of tumors in mice while not causing any harm to essential organs. In summary, bergenin suppressed the growth of CRC cells by promoting the degradation of Mcl-1. This indicates that interfering with the process of Mcl-1 ubiquitination could serve as a viable approach for anticancer therapy, as proposed by Gan et al., in 2023. Altogether, this review provides a fresh insights into the anticancer properties of bergenin and highlighted its potential as a promising candidate for using plants containing bergenin in cancer treatments. Figure 3 describes the mechanism of action associated with the anticancer potential of bergenin against several types of human cancers.
Figure 3. Deregulated cell signaling pathways targeted by Bergenin. This figure hypothesizes the mechanism of action associated with the anticancer potential of bergenin against several types of human cancers via targeting several deregulated components of cell signaling pathways including (A) ERK1/2 (Park et al., 2021), (B) JAK/STAT (Shi et al., 2019), (C) STAT3/Akt (Shi et al., 2019; Liu et al., 2021), (D) NF-kB (Xiang et al., 2020) and associated with the progression of human cancers. Bergenin has been reported to modulate the oncogene and tumor suppressor gene expression to inhibit the carcinogenesis. Modulation of these dysregulated components leads to several mechanisms that inhibit the growth of cancer cells such as apoptosis induction, growth arrest at G0/S/M phases, angiogenesis and glycolysis inhibition. PTEN, Phosphatase and TENsin homolog; AKT, AKT serine-threonine protein kinase family; mTOR, mammalian target of rapamycin; MAPK, mitogen-activated protein kinase; STAT-3, Signal transducer and activator of transcription 3.
The burgeoning field of biological nanoparticle synthesis is gaining traction due to its myriad pharmaceutical and therapeutic applications. Bergenin has demonstrated significant efficacy against a wide range of chronic ailments. However, bergenin ’s pharmacological significance and therapeutic effectiveness are restricted due to its inadequate oral absorption, low solubility in water, and limited capacity to pass across membranes. To overcome these obstacles in the pharmaceutical field, it is necessary to devise novel formulation procedures. Lately, the scientific community has been primarily concerned with developing nano-based carrier systems. The innovative approaches encompass phospholipid complex, herbal gel, extended-release core tablets, phospholipid complex solid dispersion, prodrug, polyherbal ointment, nanoparticles, and poly lactic acid polymers. Given the constraints, scientists have developed multiple drug delivery systems to improve its effectiveness in treatment. Despite the extensive utilization of bergenin, its limited oral bioavailability remains a barrier to its continued employment. Bergenin, classified as a class IV chemical, exhibits insufficient solubility and permeability to achieve total absorption (Bajracharya, G. B., 2015). Various strategies have been employed to enhance the bioavailability of the substance, including structural modifications, prodrugs, and the development of new dosage forms such as β-cyclodextrin inclusion complexes and phospholipid complexes (Gao et al., 2018; Stella, V. J., & Rajewski, 2020).
Earlier studies have presented that reduced particle size of bergenin employing nanosuspensions can promote its solubility (Rao et al., 2018). Applying BgAgNps (Bergenia ligulata silver nanoparticles) resulted in significant cytotoxic effects on MCF-7 cells, decreasing cell viability, migratory capacity, and the loss of typical morphological features. BgAgNps demonstrated a notable increase in programmed cell death in MCF-7 breast cancer cells, potentially triggering ROS production through oxidative stress and causing a decline in mitochondrial membrane potential (MMP). In addition, investigations based on molecular mechanisms have shown that BgAgNps significantly increased the levels of p53 and its downstream targets, such as Bax and cleaved caspase 3, in MCF-7 cells. It is worth mentioning that BgAgNps did not have a significant cytotoxic impact on p53-deficient cancer cells, specifically MDA-MB-231 and SW-620 (Faheem et al., 2022).
A nanostructured lipid carrier (NLC) containing bergenin was formulated to enhance the effectiveness of oral therapy. The results of the solid-state characterization demonstrated that bergenin was fully enclosed within the NLC matrix. The improved bergenin NLC demonstrated a considerably longer release duration than pure bergenin. The Korsmeyer-Peppas model was determined to be the most suitable kinetic model. Additionally, it was shown that the formulation had a 3.2 times higher rate of movement in the intestines than pure bergenin, with improved penetration across the membrane studied. The relative bioavailability findings of bergenin NLC show a markedly superior bioavailability compared to pure bergenin. The improved formulation achieved the most significant reduction in swelling when compared to pure bergenin and the conventional medication indomethacin. The formulation had a prolonged effect, lasting for 12 h. These results suggested that including bergenin in the NLC matrix might enhance the effectiveness of treatments (Zafar et al., 2024).
In a separate study, Guan et al. investigated the mechanism by which the bergenin–phospholipid combination enhances the oral absorption of bergenin. Diverse models utilizing the everted rat gut sac (ex vivo) and Caco-2 cells (in vitro) were utilized. The results from the ex vivo model of everted rat gut sac indicated that the small intestine is more favorable for the absorption of this bioactive substance compared to the colon. Moreover, the incorporation of chitosan facilitated the opening of tight junctions in intestinal epithelial cells, hence significantly improved the transport of bergenin via the paracellular pathway (Gao et al., 2018). The field of synthesizing metal and semiconductor nanoparticles is growing as researchers explore their possible applications in advancing new technologies. This study presents a new method for synthesizing zinc oxide nanoparticles using the rhizome extract of the plant B. ciliata. The study conducted by Dulta et al. (2021) demonstrated that ZnO nanoparticles had a notable and specific toxicity towards the HeLa and HT-29 cell lines, which are associated with human cervical and colon cancer, respectively. Additional examination revealed that bergenin is a powerful anti-angiogenic substance, as it decreased the levels of essential angiogenic proteins, such as Galectin 3 and MMP-9, in cervical cancer cells at the cellular level, as demonstrated by a western blotting assay. These data collectively offer a new understanding of how Bergenin inhibited the growth of blood vessels in cervical carcinoma cells. This is achieved by affecting the activity of various proteins involved in blood vessel formation, such as Galectin-3 and MMP-9. These findings supported the potential use of Bergenin as a treatment for cervical cancer (Singh et al., 2024). The efficacy of this phytochemical is involved in various mechanisms, including inhibiting lipid peroxidation, scavenging free radicals, initiating apoptosis and arresting growth at G0/G1 phase, inhibiting STAT3 proteins phosphorylation, inducing the production of TNF-α, NO, IFN-γ, IL-17, IL-12, and inhibiting the α-glucosidase enzyme.
Plant-derived secondary metabolites have garnered significant attention as bioactive compounds over the years. This review aimed to outline the anticancer effects of bergenin on several cancer types by focusing on apoptosis, angiogenesis, and the suppression of dysregulated cell signaling pathways, including ERK1/2, MAP kinase, and SAPK/JNK. The anti-cancer action of bergenin has also been documented through the modulation of many angiogenic proteins, including STAT-3 and MMP-9. Furthermore, nanoformulations of bergenin have inhibited its depletion in healthy tissues. However, future research is also required to explore the synergistic effects of bergenin with additional natural compounds or established chemotherapeutic agents. Consequently, researchers must concentrate not only on the efficacy of bergenin, which is of significant interest, but also on drug delivery systems capable of addressing pharmacokinetic challenges, as well as investigating derivatives with enhanced biological activity and bioavailability.
PP: Conceptualization, Formal Analysis, Methodology, Visualization, Writing–original draft, Writing–review and editing. SL: Formal Analysis, Investigation, Methodology, Visualization, Writing–original draft, Writing–review and editing. DM: Conceptualization, Project administration, Visualization, Writing–original draft, Writing–review and editing. HK: Formal Analysis, Investigation, Methodology, Validation, Visualization, Writing–review and editing. BK: Formal Analysis, Investigation, Supervision, Visualization, Writing–review and editing. SK: Data curation, Formal Analysis, Investigation, Validation, Visualization, Writing–review and editing. JC: Formal Analysis, Methodology, Validation, Visualization, Writing–review and editing. SM: Investigation, Methodology, Validation, Visualization, Writing–review and editing. SP: Formal Analysis, Investigation, Methodology, Visualization, Writing–review and editing. SB: Data curation, Formal Analysis, Validation, Visualization, Writing–review and editing. Sanjay Kumar: Data curation, Supervision, Validation, Visualization, Writing–review and editing. FK: Investigation, Supervision, Validation, Visualization, Writing–original draft, Writing–review and editing. BK: Data curation, Investigation, Validation, Visualization, Writing–original draft, Writing–review and editing.
The author(s) declare that financial support was received for the research, authorship, and/or publication of this article. This research was supported by Basic Science Research Program through the National Research Foundation of Korea (NRF) funded by the Ministry of Education (NRF-2020R1I1A2066868), the National Research Foundation of Korea (NRF) grant funded by the Korea government (MSIT) (No. 2020R1A5A2019413), Basic Science Research Program through the National Research Foundation of Korea (NRF) grant funded by the Korea government (MSIT) (RS-2024-00350362), and the National Research Foundation of Korea. grant numbers: 2021R1C1C2014229.
The authors declare that they have no known competing financial interests or personal relationships that could have appeared to influence the work reported in this paper.
The author(s) declared that they were an editorial board member of Frontiers, at the time of submission. This had no impact on the peer review process and the final decision.
All claims expressed in this article are solely those of the authors and do not necessarily represent those of their affiliated organizations, or those of the publisher, the editors and the reviewers. Any product that may be evaluated in this article, or claim that may be made by its manufacturer, is not guaranteed or endorsed by the publisher.
Ali, I., Hussain, H., Ahmad, V. U., Qaiser, M., Amyn, A., Mohammad, F. V., et al. (2011). Two new antioxidant bergenin derivatives from the stem of Rivea hypocrateriformis. Fitoterapia 82 (4), 722–725. doi:10.1016/j.fitote.2011.03.002
Alkadi, K. A., Adam, A., Taha, M., Hasan, M. H., and Shah, S. A. (2013). Antiplatelet aggregation activity of 5-hydroxyflavone, 2’-hydroxyflavanone, paeonol and bergenin isolated from stem bark of Garcinia malaccensis in human whole blood. Orient J. Chem. 29 (3), 871–875. doi:10.13005/ojc/290304
Bajracharya, G. B. (2015). Diversity, pharmacology and synthesis of bergenin and its derivatives: potential materials for therapeutic usages. Fitoterapia 101, 133–152. doi:10.1016/j.fitote.2015.01.001
Borges, J. C., Ripardo Filho, H. D. S., Guilhon, G. M. S. P., Carvalho, J. C., Santos, L. S., and Sousa, P. J. (2011). Antinociceptive activity of acetylbergenin in mice. Lat. Am. J. Pharm. 30 (7), 1303–1308.
Costa, R. A., da Silva, J. N., Oliveira, V. G., Anselmo, L. M., Araujo, M. M., Oliveira, K. M., et al. (2021). New insights into structural, electronic, reactivity, spectroscopic and pharmacological properties of Bergenin: Experimental, DFT calculations, MD and docking simulations. J. Mol. Liq. 330, 115625. doi:10.1016/j.molliq.2021.115625
De-Biao, Y. A. N., Zhang, D. P., Ming, L. I., Wen-Yuan, L. I. U., Feng, F. E. N. G., Bin, D. I., et al. (2014). Synthesis and cytotoxic activity of 3, 4, 11-trihydroxyl modified derivatives of bergenin. Chin. J. Nat. Med. 12 (12), 929–936. doi:10.1016/S1875-5364(14)60136-9
Deng, J., Xiao, X., Tong, X., and Li, G. (2010). Preparation of bergenin from Ardisia crenata sims and Rodgersia sambucifolia Hemsl based on microwave-assisted extraction/high-speed counter-current chromatography. Sep. Purif. Technol. 74 (2), 155–159. doi:10.1016/j.seppur.2010.05.018
Dhalwal, K., Shinde, V. M., Biradar, Y. S., and Mahadik, K. R. (2008). Simultaneous quantification of bergenin, catechin, and gallic acid from Bergenia ciliata and Bergenia ligulata by using thin-layer chromatography. J. food Compos. analysis 21 (6), 496–500. doi:10.1016/j.jfca.2008.02.008
Dulta, K., Koşarsoy Ağçeli, G., Chauhan, P., Jasrotia, R., and Chauhan, P. K. (2021). A novel approach of synthesis zinc oxide nanoparticles by bergenia ciliata rhizome extract: antibacterial and anticancer potential. J. Inorg. Organomet. Polym. Mater. 31, 180–190. doi:10.1007/s10904-020-01684-6
Faheem, M. M., Bhagat, M., Sharma, P., and Anand, R. (2022). Induction of p53 mediated mitochondrial apoptosis and cell cycle arrest in human breast cancer cells by plant mediated synthesis of silver nanoparticles from Bergenia ligulata (Whole plant). Int. J. Pharm. 619, 121710. doi:10.1016/j.ijpharm.2022.121710
Gan, Y., Li, X., Han, S., Zhou, L., and Li, W. (2023). Targeting Mcl-1 degradation by Bergenin inhibits tumorigenesis of colorectal cancer cells. Pharmaceuticals 16 (2), 241. doi:10.3390/ph16020241
Gao, H., Wei, Y., Xi, L., Sun, Y., and Zhang, T. (2018). Evaluation of intestinal absorption and bioavailability of a bergenin–phospholipid complex solid dispersion in rats. AAPS PharmSciTech 19, 1720–1729. doi:10.1208/s12249-018-0984-5
Gao, X., Wang, Y., Zhang, J., Lin, L., Yao, Q., and Xiang, G. (2017). Bergenin suppresses the growth of colorectal cancer cells by inhibiting PI3K/AKT/mTOR signaling pathway. Trop. J. Pharm. Res. 16 (10), 2307–2313. doi:10.4314/tjpr.v16i10.1
Ghosh, S., Dutta, N., Banerjee, P., Gajbhiye, R. L., Sareng, H. R., Kapse, P., et al. (2021). Induction of monoamine oxidase A-mediated oxidative stress and impairment of NRF2-antioxidant defence response by polyphenol-rich fraction of Bergenia ligulata sensitizes prostate cancer cells in vitro and in vivo. Free Radic. Biol. Med. 172, 136–151. doi:10.1016/j.freeradbiomed.2021.05.037
Gu, Y., Li, P., Lei, F., and Huang, Z. (2016). A new bergenin derivative from Ardisia maclurei. Chem. Nat. Compd. 52, 224–226. doi:10.1007/s10600-016-1600-0
Jayakody, R. S., Wijewardhane, P., and Herath, C. (2018). Bergenin: a computationally proven promising scaffold for novel galectin-3 inhibitors. J. Mol. Model. 24, 302. doi:10.1007/s00894-018-3831-4
Kashima, Y., and Miyazawa, M. (2012). Synthesis and biological evaluation of bergenin analogues as mushroom tyrosinase inhibitors. Archives pharmacal Res. 35, 1533–1541. doi:10.1007/s12272-012-0903-3
Koul, B., Kumar, A., Yadav, D., and Jin, J. O. (2020). Bergenia genus: traditional uses, phytochemistry and pharmacology. Molecules 25 (23), 5555. doi:10.3390/molecules25235555
Li, X., Gan, Y., Han, S., Liao, J., Zhou, L., and Li, W. (2022). Bergenin exerts antitumor effects by promoting survivin degradation.
Li, X., Liang, Q., Zhou, L., Deng, G., Xiao, Y., Gan, Y., et al. (2023a). Survivin degradation by bergenin overcomes pemetrexed resistance. Cell. Oncol. 46 (6), 1837–1853. doi:10.1007/s13402-023-00850-5
Li, X., Xie, L., Zhou, L., Gan, Y., Han, S., Zhou, Y., et al. (2023b). Bergenin inhibits tumor growth and overcomes radioresistance by targeting aerobic glycolysis. Am. J. Chin. Med. 51 (07), 1905–1925. doi:10.1142/S0192415X23500842
Li, Y. F., Hu, L. H., Lou, F. C., Li, J., and Shen, Q. (2005). PTP1B inhibitors from Ardisia japonica. J. Asian Nat. Prod. Res. 7 (1), 13–18. doi:10.1080/10286020310001596033
Liang, C., Pei, S., Ju, W., Jia, M., Tian, D., Tang, Y., et al. (2017). Synthesis and in vitro and in vivo antitumour activity study of 11-hydroxyl esterified bergenin/cinnamic acid hybrids. Eur. J. Med. Chem. 133, 319–328. doi:10.1016/j.ejmech.2017.03.053
Liu, J., Zhang, Y., Yu, C., Zhang, P., Gu, S., Wang, G., et al. (2021). Bergenin inhibits bladder cancer progression via activating the PPARγ/PTEN/Akt signal pathway. Drug Dev. Res. 82 (2), 278–286. doi:10.1002/ddr.21751
Madaan, R., Singla, R. K., Kumar, S., Dubey, A. K., Kumar, D., Sharma, P., et al. (2022). Bergenin-a biologically active scaffold: Nanotechnological perspectives. Curr. Top. Med. Chem. 22 (2), 132–149. doi:10.2174/1568026621666211015092654
Mehta, S., Kadian, V., Dalal, S., Dalal, P., Kumar, S., Garg, M., et al. (2022). A fresh look on bergenin: vision of its novel drug delivery systems and pharmacological activities. Future Pharmacol. 2 (1), 64–91. doi:10.3390/futurepharmacol2010006
Mukherjee, H., Ojha, D., Bharitkar, Y. P., Ghosh, S., Mondal, S., Kaity, S., et al. (2013). Evaluation of the wound healing activity of Shorea robusta, an Indian ethnomedicine, and its isolated constituent (s) in topical formulation. J. Ethnopharmacol. 149 (1), 335–343. doi:10.1016/j.jep.2013.06.045
Musgrave, O. C., and Skoyles, D. (1974). Ebenaceae extractives. Part IV. Diosindigo A, a blue pigment from several Diospyros species. J. Chem. Soc. Perkin Trans. 1, 1128–1131. doi:10.1039/p19740001128
Nazir, N., Koul, S., Qurishi, M. A., Najar, M. H., and Zargar, M. I. (2011). Evaluation of antioxidant and antimicrobial activities of Bergenin and its derivatives obtained by chemoenzymatic synthesis. Eur. J. Med. Chem. 46 (6), 2415–2420. doi:10.1016/j.ejmech.2011.03.025
Okoro, N. O., Odiba, A. S., Osadebe, P. O., Omeje, E. O., Liao, G., Fang, W., et al. (2021). Bioactive phytochemicals with anti-aging and lifespan extending potentials in Caenorhabditis elegans. Molecules 26 (23), 7323. doi:10.3390/molecules26237323
Oliveira, G. A., Araujo, A. K., Pacheco, G., Oliveira, A. P., Carvalho, J. L., Chaves, L. S., et al. (2019). Anti-inflammatory properties of bergenin in mice. J. Appl. Pharm. Sci. 9 (7), 069–077. doi:10.7324/JAPS.2019.90709
Park, K. R., Kwon, Y. J., Cho, M., Kwon, I. K., Hong, J. T., and Yun, H. M. (2021). 11-O-Galloyl bergenin from Corylopsis coreanas leaves induces autophagy and apoptosis in human osteosarcoma. Am. J. Chin. Med. 49 (08), 2017–2031. doi:10.1142/S0192415X21500956
Patel, D. K., Patel, K., Kumar, R., Gadewar, M., and Tahilyani, V. (2012). Pharmacological and analytical aspects of bergenin: a concise report. Asian Pac. J. Trop. Dis. 2 (2), 163–167. doi:10.1016/s2222-1808(12)60037-1
Qadri, O., Hilal, N., and Fazili, K. M. (2023). Unveiling the anti-cancer potential of bergenia ciliata (haw.) Sternb: a mechanistic study on UPR modulation and ROS generation. J. Biol. Act. Prod. Nat. 13 (2), 129–144. doi:10.1080/22311866.2023.2220312
Raj, M. K., Duraipandiyan, V., Agustin, P., and Ignacimuthu, S. (2012). Antimicrobial activity of bergenin isolated from Peltophorum pterocarpum DC. flowers. Asian Pac. J. Trop. Biomed. 2 (2), S901–S904. doi:10.1016/s2221-1691(12)60333-5
Rao, K., Roome, T., Aziz, S., Razzak, A., Abbas, G., Imran, M., et al. (2018). Bergenin loaded gum xanthan stabilized silver nanoparticles suppress synovial inflammation through modulation of the immune response and oxidative stress in adjuvant induced arthritic rats. J. Mater. Chem. B 6 (27), 4486–4501. doi:10.1039/c8tb00672e
Roselli, M., Lentini, G., and Habtemariam, S. (2012). Phytochemical, antioxidant and anti-α-glucosidase activity evaluations of Bergenia cordifolia. Phytotherapy Res. 26 (6), 908–914. doi:10.1002/ptr.3655
Roy, S. K., Agrahari, U. C., Gautam, R., Srivastava, A., and Jachak, S. M. (2012). Isointricatinol, a new antioxidant homoisoflavonoid from the roots of Caesalpinia digyna Rottler. Nat. Prod. Res. 26 (8), 690–695. doi:10.1080/14786419.2010.548813
Salimo, Z. M., Yakubu, M. N., da Silva, E. L., de Almeida, A. C., Chaves, Y. O., Costa, E. V., et al. (2023). Chemistry and pharmacology of bergenin or its derivatives: a promising molecule. Biomolecules 13 (3), 403. doi:10.3390/biom13030403
Sanogo, R., Vassallo, A., Malafronte, N., Imparato, S., Russo, A., and Piaz, F. D. (2009). New phenolic glycosides from Securinega virosa and their antioxidant activity. Nat. Product. Commun. 4 (12), 1645–1650. doi:10.1177/1934578x0900401207
Shi, X., Xu, M., Luo, K., Huang, W., Yu, H., and Zhou, T. (2019). Anticancer activity of bergenin against cervical cancer cells involves apoptosis, cell cycle arrest, inhibition of cell migration and the STAT3 signalling pathway. Exp. Ther. Med. 17 (5), 3525–3529. doi:10.3892/etm.2019.7380
Shukry, M., Hafez, A., Ghazy, E. W., Farrag, F., and Abumandour, M. (2019). Bergenin Ameliorates glutamate-induced death of Hippocampal neuronal cells through modulation of the antioxidant activity and reducing the redox oxygen species creation. Alexandria J. Veterinary Sci. 62 (1), 79. doi:10.5455/ajvs.55719
Singh, M., Chauhan, R., Malhotra, L., Gupta, A., Dagar, G., Das, D., et al. (2024). Abstract 1830: bergenin inhibits growth of human cervical cancer cells by decreasing galectin-3 and MMP-9 expression. Cancer Res. 84 (6_Suppl. ment), 1830. doi:10.1158/1538-7445.am2024-1830
Sriset, Y., Chatuphonprasert, W., and Jarukamjorn, K. (2021a). Bergenin attenuates sodium selenite-induced hepatotoxicity via improvement of hepatic oxidant-antioxidant balance in HepG2 cells and ICR mice. J. Biol. Act. Prod. Nat. 11 (2), 97–115. doi:10.1080/22311866.2021.1908162
Sriset, Y., Chatuphonprasert, W., and Jarukamjorn, K. (2021b). Hepatoprotective activity of bergenin against xenobiotics-induced oxidative stress in human hepatoma (HepG2) cells. CMUJ. Nat. Sci. 20 (1), e2021011. doi:10.12982/CMUJNS.2021.011
Stella, V. J., and Rajewski, R. A. (2020). Sulfobutylether-β-cyclodextrin. Int. J. Pharm. 583, 119396. doi:10.1016/j.ijpharm.2020.119396
Sultana, T., Mitra, A. K., and Das, S. (2022). Evaluation of anti-cancer potential of Excoecaria agallocha (L.) leaf extract on human cervical cancer (SiHa) cell line and assessing the underlying mechanism of action. Future J. Pharm. Sci. 8 (1), 3. doi:10.1186/s43094-021-00389-y
Takahashi, H., Kosaka, M., Watanabe, Y., Nakade, K., and Fukuyama, Y. (2003). Synthesis and neuroprotective activity of bergenin derivatives with antioxidant activity. Bioorg. and Med. Chem. 11 (8), 1781–1788. doi:10.1016/s0968-0896(02)00666-1
Taneyama, M., and Yoshida, S. (1979). Studies on C-glycosides in higher plants: II. Incorporation of 14 C-glucose into bergenin and arbutin in Saxifraga stolonifera. botanical magazine= Shokubutsu-gaku-zasshi 92, 69–73. doi:10.1007/bf02488302
Tschitschibabin, A. E., Kirssanow, A. W., Korolew, A. J., and Woroschzow, N. N. (1929). Über Nichtgerbende Substanzen Des Extraktes Aus Dem Wurzelstock Des Badans Saxifraga crassifolia. I. Bergenin. Justus Liebigs Ann. Chem. 469 (1), 93–127. doi:10.1002/jlac.19294690109
Valakatte, M. R., Viswanath, V., Aithal, M., Karunakar, P., and Narayanappa, S. K. (2024). In-vitro and in-silico evaluation of anticancer potential of Oxalis corniculata. Adv. Traditional Med. 24, 1229–1239. doi:10.1007/s13596-024-00777-8
Xiang, S., Chen, K., Xu, L., Wang, T., and Guo, C. (2020). Bergenin exerts hepatoprotective effects by inhibiting the release of inflammatory factors, apoptosis and autophagy via the PPAR-γ pathway. Drug Des. Dev. Ther. 14, 129–143. doi:10.2147/DDDT.S229063
Yan, R., Xie, B., Xie, K., Liu, Q., Sui, S., Wang, S., et al. (2024). Unravelling and reconstructing the biosynthetic pathway of bergenin. Nat. Commun. 15 (1), 3539. doi:10.1038/s41467-024-47502-2
Ye, Y. P., Sun, H. X., and Pan, Y. J. (2004). Bergenin monohydrate from the rhizomae of Astilbe chinensis. Acta Crystallogr. Sect. C. Cryst. Struct. Commun. 60 (6), o397–o398. doi:10.1107/S0108270104008364
Yu, Z., Luo, X., Guo, F., Zhang, Z., and Peng, L. (2019). Determination of flunixin in swine plasma, urine and feces by UPLC-MS/MS and its application in the real samples. Curr. Pharm. Anal. 15 (1), 51–60. doi:10.2174/1573412913666170918163625
Zafar, A., Yasir, M., Panda, D. S., and Singh, L. (2024). Bergenin nano-lipid carrier to improve the oral delivery: development, optimization, in vitro and in vivo evaluation. J. Drug Deliv. Sci. Technol. 96, 105655. doi:10.1016/j.jddst.2024.105655
Zhang, J., Nishimoto, Y., Tokuda, H., Suzuki, N., Yasukawa, K., Kitdamrongtham, W., et al. (2013). Cancer chemopreventive effect of bergenin from Peltophorum pterocarpum wood. Chem. and Biodivers. 10 (10), 1866–1875. doi:10.1002/cbdv.201300182
Zhang, S. S., Liao, Z. X., Huang, R. Z., Gong, C. C., Ji, L. J., and Sun, H. F. (2018). A new aromatic glycoside and its anti-proliferative activities from the leaves of Bergenia purpurascens. Nat. Prod. Res. 32 (6), 668–675. doi:10.1080/14786419.2017.1338278
Zhang, W., and Chai, R. (2022). Bergenin alleviates oxidative stress and apoptosis of cardiomyocytes induced by oxygen-glucose deprivation via activating SIRT1/FOXO3a/MnSOD pathway. Curr. Top. Nutraceutical Res. 20 (3), 447–453. doi:10.37290/ctnr2641-452x.20:447-453
Zhang, Y. H., Fang, L. H., Lee, M. K., and Ku, B. S. (2003). In vitro inhibitory effects of bergenin and norbergenin on bovine adrenal tyrosine hydroxylase. Phytotherapy Res. Int. J. Devoted Pharmacol. Toxicol. Eval. Nat. Prod. Deriv. 17 (8), 967–969. doi:10.1002/ptr.1292
Zhao, J., Zeng, L. H., Li, X., Dong, X. P., Yan, Y. M., and Cheng, Y. X. (2011). Brachystemols A–C, three new furan derivatives from Brachystemma calycinum. J. Asian Nat. Prod. Res. 13 (10), 915–919. doi:10.1080/10286020.2011.600250
Zeng, Q., Zhou, T., Zhao, F., Xiong, D., He, B., Hua, Q., et al. (2022). p62-Nrf2 regulatory loop mediates the anti-pulmonary fibrosis effect of Bergenin. Antioxidants 11, 307. doi:10.3390/antiox11020307
Zhu, Y., Yao, L., Guo, Y., Zhang, J., Xia, Y., Wei, Z., et al. (2024). Bergenin attenuates triptolide-caused premature ovarian failure in mice based on the antioxidant activity. Reprod. Toxicol. 126, 108608. doi:10.1016/j.reprotox.2024.108608
ROS Reactive oxygen species
ECM Extracellular matrix
HIA Human intestinal absorption
CaCo-2 Human colon adenocarcinoma cell line
PPB Plasma protein binding
BBB Blood-brain barrier
CNS Central nervous system
IPF Idiopathic pulmonary fibrosis
OSCC Oral squamous cell cancer
NSCLC Non-small cell lung cancer
UPR Unfolded protein response
PPARγ Peroxisome proliferator-activated receptor gamma
MD Molecular dynamics
CRD Carbohydrate recognition domain
POF Premature ovarian failure
OGCs Ovarian granulosa cells
PFBL Polyphenol-rich fraction of Bergenia ligulata
MAO-A Monoamine oxidase A
OGAL O-Galloyl bergenin
PGE2 Prostaglandin E2
IR Ischemia-reperfusion
TBHP Tert-butyl hydroperoxide
HepG2 Human hepatoma cells
SOD Superoxide dismutase
CAT Catalase
GSH Glutathione
LDH Lactate dehydrogenase
AST Aspartate aminotransferase
ALT Alanine transaminase
MDA Malondialdehyde
PTEN Phosphatase and tensin homolog is a phosphatase in humans
USP13 Ubiquitin-specific protease 13
AKT Protein kinase B
HK2 Hexokinase 2
CRC Colorectal cancer
Keywords: bergenin, anticancer, pharmacokinetic, cancer, therapeutics
Citation: Pandey P, Lakhanpal S, Mahmood D, Kang HN, Kim B, Kang S, Choi J, Moon S, Pandey S, Ballal S, Kumar S, Khan F and Kim B (2025) Bergenin, a bioactive flavonoid: advancements in the prospects of anticancer mechanism, pharmacokinetics and nanoformulations. Front. Pharmacol. 15:1481587. doi: 10.3389/fphar.2024.1481587
Received: 16 August 2024; Accepted: 16 December 2024;
Published: 06 January 2025.
Edited by:
Siddhartha Kumar Mishra, University of Lucknow, IndiaReviewed by:
Tomasz M. Karpiński, Poznan University of Medical Sciences, PolandCopyright © 2025 Pandey, Lakhanpal, Mahmood, Kang, Kim, Kang, Choi, Moon, Pandey, Ballal, Kumar, Khan and Kim. This is an open-access article distributed under the terms of the Creative Commons Attribution License (CC BY). The use, distribution or reproduction in other forums is permitted, provided the original author(s) and the copyright owner(s) are credited and that the original publication in this journal is cited, in accordance with accepted academic practice. No use, distribution or reproduction is permitted which does not comply with these terms.
*Correspondence: Fahad Khan, ZmFoYWRpbnRlZ3JhbGlhbkBnbWFpbC5jb20=; Bonglee Kim, Ym9uZ2xlZWtpbUBraHUuYWMua3I=
Disclaimer: All claims expressed in this article are solely those of the authors and do not necessarily represent those of their affiliated organizations, or those of the publisher, the editors and the reviewers. Any product that may be evaluated in this article or claim that may be made by its manufacturer is not guaranteed or endorsed by the publisher.
Research integrity at Frontiers
Learn more about the work of our research integrity team to safeguard the quality of each article we publish.