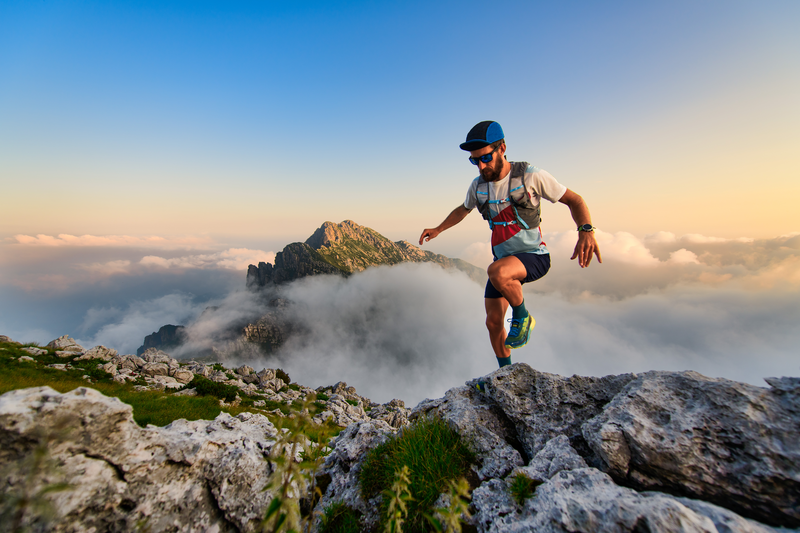
94% of researchers rate our articles as excellent or good
Learn more about the work of our research integrity team to safeguard the quality of each article we publish.
Find out more
ORIGINAL RESEARCH article
Front. Pharmacol. , 14 November 2024
Sec. Pharmacogenetics and Pharmacogenomics
Volume 15 - 2024 | https://doi.org/10.3389/fphar.2024.1480657
This article is part of the Research Topic Pharmacogenomics for Improving Drug Safety and Efficacy in Cancer View all 8 articles
Background: Methotrexate (MTX) is the primary drug used in the treatment of pediatric acute lymphoblastic leukemia (ALL). However, some patients exhibit delayed clearance of high-dose (HD) MTX, which induces severe nephrotoxicity, mucositis, hepatotoxicity, and neurotoxicity. We sought to identify relevant variants associated with delayed clearance of HD-MTX in pediatric patients with ALL.
Methods: Whole-exome sequencing of germline DNA was performed in 51 Korean pediatric patients with ALL. A total of 341 HD-MTX infusion data points from 51 patients were analyzed. MTX levels and laboratory measurements reflecting toxicity outcomes were obtained. Correlations between peak serum MTX levels at 24 h and toxicity outcomes were assessed. Analyses were performed to identify variants affecting delayed MTX clearance.
Results: The 24 h MTX level strongly correlated with the subsequent creatinine (Cr) level. Moreover, rs2229866 in contactin 2 (CNTN2), rs200687372 in myotubularin Related Protein 9 (MTMR9), rs777260512 in polymerase iota (POLI), rs16954698 in polycystic kidney disease 1-like 2 (PKD1L2), rs117765468 in NSE1 Homolog, SMC5-SMC6 Complex Component (NSMCE1), and rs1800956 in endoglin (ENG) were identified as candidate variants associated with delayed MTX clearance. In particular, ENG rs1800956 was significantly associated with delayed MTX clearance in all analyses and PKD1L2 rs16954698 was replicated in an external dataset (phs000637.v1.p1) from the Database of Genotypes and Phenotypes (dbGaP).
Conclusion: This is the first whole-exome sequencing-based analysis of delayed MTX clearance in pediatric patients with ALL. ENG rs1800956 and PKD1L2 rs16954698 were found to be potentially influential variants associated with delayed MTX clearance. These findings provide insights into HD-MTX-induced nephrotoxicity and may contribute to reducing adverse reactions through treatment modification.
HD-MTX plays an important role in the treatment of childhood and adult cancers, such as ALL, lymphoma, and osteosarcoma (Treon and Chabner, 1996). It has been used in consolidation therapy for ALL to overcome resistance and prevent central nervous system (CNS) relapse (Larsen et al., 2016). However, the delayed clearance of HD-MTX induces multiple side effects, including nephrotoxicity, mucositis, hepatotoxicity, and neurotoxicity. To prevent toxicity, proper hydration with alkalinization and leucovorin rescue are administered, while nonsteroidal anti-inflammatory drugs, penicillin, and aspirin-containing medications should be avoided in combination with MTX. Studies emphasized the need to monitor serum MTX levels, perform liver and renal function tests, and decide whether to increase leucovorin or use glucarpidase (carboxypeptidase G2) at the right time (Ramsey et al., 2018). In South Korea, to reduce HD-MTX toxicity, the infusion time was shortened from 24 h to 4 h compared with the COG AALL0232 protocol (Larsen et al., 2016). Despite appropriate efforts, severe renal toxicity occurs in some patients, requiring dialysis and sometimes leading to chronic renal disease. Therefore, many studies have aimed to elucidate the interindividual variability in the pharmacokinetics explained by pharmacogenomics of MTX (Song et al., 2021; Taylor et al., 2021). However, most previous studies were conducted based on candidate gene panel analyses with a few genes or mutations related to the folate pathway or transporter genes. Very few studies have conducted whole-genome or whole exome sequencing (WES).
Racial and ethnic disparities were observed in adverse drug events, and studies involving Asians were fewer compared to other races, such as white people, black people, and Hispanics (Baehr et al., 2015). Despite the growing need to consider racial factors when determining MTX toxicities or responses (Torres et al., 2022; Xu et al., 2022; Liu et al., 2024), no genomic research has been performed on the pharmacokinetics of MTX in childhood ALL in South Korea. In this study, we aimed to investigate the genomic variants related to delayed clearance of HD-MTX using WES in pediatric patients with ALL.
Clinical data and DNA were collected from 51 Korean pediatric patients with ALL treated with HD-MTX at three hospitals: Seoul National University Hospital (SNUH), Asan Medical Center (AMC), and Samsung Seoul Medical Center (SMC) between August 2012 and July 2019. HD-MTX was administered to patients with T-cell ALL, slow early responders in high-risk B-cell ALL, testis involvement, CNS-3 status, or >100,000/μL white blood cells at diagnosis. HD-MTX was included in two interim maintenance (IM) courses (four HD-MTX sessions every 2 weeks per the IM course) of the treatment protocol. Intrathecal methotrexate was administered every 4 weeks, and 6-mercaptopurine 25 mg/m2 was administered daily. Accordingly, patients were administered a maximum of eight doses of HD-MTX (5 g/m2/dose) as a 4-h infusion according to the treatment protocol. The clinical data of 341 HD-MTX infusions were collected from 51 patients.
To monitor MTX-induced toxicity, serum MTX levels and laboratory results as toxicity markers were collected until the level <0.1 μmol/L was confirmed twice in each MTX administration. Methotrexate level was measured by the homogenous enzyme immunoassay (ARK Diagnostics, Fremont, CA, USA), and limit of detection and quantitation was 0.02 and 0.04 μmol/L, respectively.The toxicity markers consisted of serial serum levels of Cr, blood urea nitrogen (BUN), alanine aminotransferase (ALT), aspartate aminotransferase (AST), and total bilirubin (TB). Cr, BUN, ALT, AST, and TB prefixed with “Baseline” were measured before HD-MTX administration (Table 1; Supplementary Table S1). Toxicity grade was defined according to the Common Terminology Criteria for Adverse Events (CTCAE) version 4.0 of the National Cancer Institute, and the estimated glomerular filtration rate (eGFR) was calculated using the Cr-based bedside Schwartz equation (Schwartz and Work, 2009).
This study was approved by the institutional review boards of SNUH, AMC, and SMC (IRB no. H-1702-109-833). Written informed consent was obtained from all patients and from the parents and/or legal guardians of patients aged <18 years.
Germline DNA was extracted from the blood (n = 37), hair (n = 6), and bone marrow (n = 8). WES was performed, and data were obtained using a bioinformatics pipeline previously described (Park et al., 2019). Allele frequencies, consequences, and amino acid changes were annotated by in silico prediction using the Ensembl Variant Effects Predictor (McLaren et al., 2016). To assess the impact of variants, Sorts Intolerant From Tolerant (SIFT) (Kumar et al., 2009), Polymorphism Phenotyping v2 (PolyPhen-2) (Adzhubei et al., 2013), and Combined Annotation-Dependent Depletion (CADD) scores were utilized. Based on the authors’ recommendations and the literature (Castellana and Mazza, 2013; CADD, 2014), variants with a SIFT score of <0.05, a PolyPhen-2 score of >0.85, and a CADD score of >15 were classified as deleterious, probably damaging, and deleteriousness, respectively.
Tri-allelic variants, sex-chromosomal variants, and variants not reported in both the 1000 Genomes Project (1KGP) and the Genome Aggregation Database (gnomAD) were excluded from the statistical analysis (Genomes Project et al., 2015; Karczewski et al., 2020). From the initial list of variants, only nonsynonymous variants were included in the analysis. To remove false-positive variants, the reads of significant variants obtained from the statistical analysis were manually reviewed.
Pearson’s correlation coefficient was used to assess the correlation between serum MTX levels and toxicity markers. The 24-h concentration of MTX was used as the peak level to estimate the correlation with toxicities, except for one patient without 24-h MTX level. For each infusion, the ratio of the baseline Cr to the post-infusion Cr values was defined as the fold change in Cr (fold Crs). An infusion with the maximum value among the fold Crs in one patient was defined as an infusion with the maximum-fold Cr (max fold Cr) in that patient. The infusion with the max fold Cr was selected as the patient’s representative infusion for statistical analysis (Figure 1).
Figure 1. Flowchart of the analyses. From 51 Korean pediatric patients with ALL treated with HD-MTX, the infusion with the maximum fold change in creatinine was selected as the representative infusion for the statistical analyses.
To determine the difference in serum MTX levels by variants over time, a longitudinal analysis was performed using a linear mixed-effect model (LMM), assuming a dominant genetic model. Age, sex, and body mass index (BMI) were included as covariates in the LMM analysis. Genetic variants that satisfied the Bonferroni-corrected p-value <0.01 for variants over time were considered significant.
Under the assumption of a dominant genetic model, multiple linear regression (MLR), adjusted for age, sex, and BMI, was used to evaluate the relationship between the 24-h MTX level and genetic variants. The threshold for the statistical significance of the variants was a Bonferroni-adjusted p-value of <0.01.
Six variants were derived by defining candidate variants as the intersection of the variants obtained in the continuous outcome and longitudinal analyses. Additional analysis was performed to identify significant associations (nominal p < 0.05) between candidate variants and delayed MTX clearance as a binary outcome (case–control) using Fisher’s exact test (FET). The case group was defined as serum MTX level (μmol/L) at 24 h ≥ 15, 48 h ≥ 1.5, 72 h ≥ 0.15, or 168 h ≥ 0.1, otherwise considered the controls (Santucci et al., 2010). Moreover, the toxicities were compared according to the genotypes of the variant with statistical significance in analyses.
To validate the six candidate variants, an external dataset (phs000637.v1.p1) was utilized as a replication cohort from the Database of Genotypes and Phenotypes (dbGaP) (Mailman et al., 2007). This replication cohort consisted of 1,267 patients with B-precursor ALL and ≤21 years of age. The patients’ ancestry groups were White (n = 798; 63.0%), Hispanic (n = 266; 21.0%), Black (n = 57; 4.5%), Asian (n = 22, 1.7%), and other (n = 124; 9.8%). Patients received HD-MTX as part of protocol-directed therapy for ALL and corresponding serum MTX clearance, which adjusted for age, sex, race, and treatment arm (residual from a linear regression model), was included in the dataset. Imputation was performed for each ancestral group using Michigan Imputation Server (Das et al., 2016) and 1KGP Phase 3 was applied as a reference panel. The analysis of variance (ANOVA) and t-test were used to test the association between candidate variants and MTX clearance.
The Wilcoxon rank-sum test, t-test, MLR and LMM were applied to analyze numerical variables, and FET was used for categorical variables. All statistical analyses were performed using R software version 3.6.1.
The patient characteristics are summarized in Table 1. The clinical data of 341 HD-MTX infusions were collected from 51 patients. This study included 28 male (55%) and 23 female (45%). The mean age in total infusions was 9.8 (1.4–17.3) years. Patients received a median of eight HD-MTX (range, 1–8) infusions. Significant differences were found in MTX level at 24, 48, 72, 96, 120, and 144 h, max Cr, min eGFR, delayed MTX clearance percentage, and CTCAE acute kidney injury (AKI) percentage between the total and 51 infusions showing max fold Cr. The baseline eGFR level was normal in all patients, although a statistically significant difference was found between the total and 51 infusions with max fold Cr. Delayed clearance occurred in 80/341 (23.5%) infusions, and 18 patients experienced delayed MTX clearance during the first HD-MTX infusion (Table 1; Supplementary Table S2).
In 51 infusions, the mean MTX concentrations at 24, 48, 72, 96, 120, 144, and 168 h of infusion were 6.36, 1.9, 0.77, 0.59, 0.54, 0.73, and 0.35 mmol/L, respectively. Grade 2–4 AKI developed in 13 (25.5%) of the 51 infusions. One patient (2.0%) experienced grade 4 toxicity and required dialysis. No grade 5 toxicity occurred. Delayed clearance occurred in 25 (49%) of the 51 infusions, showing a maximum-fold increase in Cr.
A similar trend was observed in 341 and 51 infusions with the max fold Cr among all toxicity markers (Table 2; Supplementary Table S3). In both total and infusions showing max fold Cr, the 24-h MTX level showed a statistically significant positive correlation (p-value<0.01) with the Cr level in nearly all time points. In particular, high correlation coefficients (>0.5) were observed between Cr levels and the MTX level, except for 168 h of infusions showing a max fold increase in Cr. By contrast, other toxicity markers such as ALT, AST, and TB rarely showed significant correlations with the 24-h MTX level.
Twelve variants derived from the longitudinal analysis were statistically significant and six variants showed significant associations with the 24-h MTX level (Bonferroni-corrected p-value<0.01) (Supplementary Table S4). The intersection of the variants obtained from these two analyses was defined as a candidate variant. The trajectories of MTX levels and candidate variants are shown in Figure 2; Supplementary Figure S1.
Figure 2. Longitudinal trajectories of serum MTX levels. MTX level changes over time for 51 Korean patients are shown for ENG rs1800956 (A) and PKD1L2 rs16954698 (B). Patients carrying the variants exhibited a slower decline in serum MTX levels.
To test the binary outcome (case–control), additional analyses of these candidate variants were performed using the FET (Table 3). The case group was defined as patients with delayed MTX clearance. The rs1800956 in ENG showed statistical significance in the binary analysis [relative risk: 2.53 (95% CI: 175, 3.66), nominal p-value = 0.0017]. Only rs1800956 in ENG showed significance in all three statistical tests.
No significant differences were found in baseline clinical indicators such as sex, age, and baseline toxicity markers according to ENG rs1800956 and PKD1L2 rs16954698 (Table 4; Supplementary Table S5). All eight patients with ENG rs1800956 exhibited delayed MTX clearance. When reviewing the 341 infusions data, five of the eight patients with ENG rs1800956 experienced delayed clearance at the very first HD-MTX infusion (median, 1, range 1–4). In one patient, HD-MTX infusion was permanently discontinued after the first HD-MTX infusion. In six out of seven patients, delayed clearance also occurred in infusions other than the representative infusions (Supplementary Table S2). ENG rs1800956 was significantly associated with Cr and eGFR after HD-MTX injection but was not associated with liver function indicators such as ALT, AST, and TB. Heterozygotes of this variant had higher mean Cr (wild type, 0.60; heterozygote, 0.94; p-value = 0.0267) and lower mean eGFR (wild type, 105; heterozygote, 75; p-value = 0.0475) values than wild types after HD-MTX infusion. In addition, the ratio of AKI severity showed an increasing trend in heterozygotes compared with wild types. Patients with grade ≥1 AKI appeared at a higher rate in heterozygotes (87.5%) than in the wild types (46.5%), although this difference was not statistically significant (p-value = 0.0528).
Of all three patients with PKD1L2 rs16954698, two had delayed MTX clearance with first infusion and one with the third infusion. Compared to the wild-types of this variant, heterozygotes showed statistically significantly worse status in all renal function indices such as Cr, eGFR, BUN, and grade ≥2 AKI after HD-MTX administration (Supplementary Table S5).
Five out of the six candidate variants were genotyped or imputed in the replication cohort (Supplementary Table S6). Among them, only PKD1L2 rs16954698 and CNTN2 rs2229866 possessed both wild-types and heterozygotes or homozygotes, enabling a comparison of MTX clearance with the variant. Other variants were rare and had the highest frequency in East Asians in the 1KGP used as an imputation reference panel, but only 1.7% of patients in the replication cohort were Asians, so the genotypes of the variants were only wild-types or no variant was found.
MTX clearance was reduced depending on the genotypes of PKD1L2 rs16954698 (Figure 3A), and the carriers of the variant has significantly lower MTX clearance than the wild-types (p-value = 0.034) (Figure 3B). The mean MTX clearance for carriers was 137.57 ± 30.88 (mean ± SD), and for wild-types, it was 143.22 ± 33.82. These results showed similar trends to those observed in the analyses of 51 Korean patients, where patients with the variant had high MTX levels in the blood (Figure 2B; Supplementary Table S4; Supplementary Table S5). On the other hand, in the case of Contactin 2 (CNTN2) rs2229866, there was no significant difference in MTX clearance between the groups (wild-types, 142.88 ± 32.48; carriers, 142.21 ± 34.10) (Supplementary Figure S2). Additionally, this trend was opposite to results in the analyses of 51 Korean patients (Supplementary Figure S1A; Supplementary Table S4).
Figure 3. MTX clearance for PKD1L2 rs16954698 in replication cohort. MTX clearance tends to decrease depending on the A allele of rs16954698 (A). Patients carrying the variant showed a statistically significant reduction in clearance (B). WT, wild-types; HET, heterozygotes; HOM, homozygotes.
We identified variants that potentially contribute to delayed HD-MTX clearance from serum measurements after HD-MTX infusion in pediatric patients with ALL. Delayed clearance of HD-MTX increases the risk of severe nephrotoxicity and consequently induces severe life-threatening complications in some cases (Howard et al., 2016). Our data also showed a strong correlation between the 24-h MTX level and the subsequent Cr levels. The incidence of delayed clearance was significantly higher in infusions showing max fold Cr than in total infusions (49% vs 23.5%; p = 0.0002). These findings suggest that the peak levels and delayed MTX clearance are associated with subsequent nephrotoxicity.
In this study, 23.5% of the total infusions (80/341 infusions) corresponded to delayed clearance of HD-MTX. AKI occurred in 12.9% of the infusions (44/341 infusions). Delayed MTX clearance has been described in several studies. In a study of pediatric and adult patients with osteosarcoma in China, the frequency of delayed clearance was reported to be 6.19% and 0.86% when delayed and severely delayed clearances were defined as concentration >5 μmol/L at 24 h and concentration >20 μmol/L at 24 h, respectively (Zhang et al., 2016). Another study reported that 22% of patients with lymphoma or patients with leukemia aged 16–84 years experienced delayed clearance of HD-MTX based on the definition of MTX concentration ≥1 μmol/L 48 h or ≥0.1 μmol/L 72 h after HD-MTX (Narita et al., 2017). The frequency of delayed clearance in our study was higher than that reported in other studies, partially because of the broad criteria used.
MTX-induced renal toxicity is caused by the precipitation of MTX and its metabolites in the renal tubules or by the direct toxic effects of MTX on the renal tubules (Garneau et al., 2015). In a meta-analysis of the toxicity of HD-MTX in osteosarcoma, the incidence of grade ≥ II MTX renal toxicity was reported to be 1.5% (range, 0.0%–12.4%) (Widemann et al., 2004). Amitai et al. reported that 13% of patients with hematological malignancies experienced AKI after HD-MTX (median dose, 1941; range, 743–5442 mg/m2) (Amitai et al., 2020). Another retrospective study reported that 38.6% of adult patients with hematological malignancies experience renal toxicity (Wiczer et al., 2016).
In particular, renal disorders can reduce renal function and hinder drug clearance (Garza et al., 2022). Renal clearance of drugs depends on the GFR, renal blood flow (RBF), and tubular function (Richard et al., 2020). MTX toxicity may occur unpredictably even without an underlying patient’s condition or external factors, and genetic polymorphisms, such as transporter-related polymorphisms, may be involved. Therefore, the genetic variants associated with delayed clearance and AKI after HD-MTX infusion must be identified.
In this study, ENG rs1800956 and PKD1L2 rs16954698 were identified as promising biomarkers for predicting delayed MTX clearance. These two variants satisfied at least two of the following criteria: deleterious (SIFT), probably damaging (PolyPhen-2), and deleteriousness (CADD) (Supplementary Table S7). Notably, all criteria were met for ENG rs1800956. Since SIFT, PolyPhen-2, and CADD are prediction tools that evaluate scores based on the protein’s structure, sequence conservation, and functional information (Eilbeck et al., 2017), these two variants could have both structural and functional impacts on the proteins they encode.
Endoglin (ENG) is a transmembrane glycoprotein expressed on endothelial cells that functions as a co-receptor for several ligands of the transforming growth factor beta (TGF-β) family (Rossi et al., 2019). The kidney contains various types of endothelial cells. Renal endothelial function plays a role in maintaining to RBF (Basile and Yoder, 2014) and common types of chronic kidney disease (CKD) are characterized by renal endothelial dysfunction (Jourde-Chiche et al., 2019). Specifically, the glomerular endothelium is highly fenestrated, covered by a rich glycocalyx, and plays a key role in the filtration properties of the glomerular barrier and the maintenance of podocyte structure. Capillary glomerular renal endothelial cells show enriched expression of genes associated with the TGF-β–BMP signaling pathway, including ENG, which is involved in glomerular capillary formation. Overexpression of TGF-β induces proteinuria and glomerulosclerosis (Dumas et al., 2021).
Previous studies have shown that TGF-β plays diverse roles in renal fibrosis and inflammation, and an infusion of TGF-β2 resulted in a gradual decline in renal medullary blood (Spurgeon et al., 2005; Gu et al., 2020). Although the most important mechanism of MTX-induced AKI is intratubular crystal precipitation, decreased RBF or urine flow can also affect drug elimination and AKI (Pentel and Benowitz, 1984; Perazella and Luciano, 2015; Garza et al., 2022). Cyclosporine (CSA), an immunosuppressant used in organ and bone marrow transplants as well as inflammatory conditions, stimulates renal and systemic productions of TGF-β, and experimental and clinical studies have suggested that TGF-β overexpression may be an important factor in the development of CSA nephrotoxicity (Burdmann et al., 2003).
Moreover, ENG protein is highly expressed in kidney (HPA, 2015), and its vascular expression is elevated in animal models of renal injury, potentially contributing to disease severity by promoting endothelial cell activation and inflammation (Bus et al., 2018). ENG is upregulated in various CKD, and interstitial ENG expression is correlated with eGFR (Gerrits et al., 2022). In addition, ENG was downregulated by MTX in monocyte-derived human macrophages (Rios et al., 2023). These imply the involvement of ENG in MTX elimination and nephrotoxicity. In our study, ENG rs1800956 also showed negative (−0.09) and positive (17.79) coefficients in association with MTX levels and 24 h MTX levels, respectively (Supplementary Table S4). This suggests that heterozygotes for this variant have a slower decline in blood MTX concentration. The allele frequency of ENG rs1800956 was higher in Koreans and East Asians than in Europeans with gnomAD (Korea 9.0%, East Asia 10.9%, and Europe 0.1%). The high allele frequency in Koreans might have contributed to the identification of variants as significant in our study.
The polycystic kidney disease 1-like 2 (PKD1L2) gene encodes a polycystin-1-like subfamily, and the protein has ion channel segments that are highly conserved among polycystin-2 family members, suggesting that they possess cation channel functions (Li et al., 2003; Yuasa et al., 2004). According to the Gene Ontology (GO) for PKD1L2, the GO classes included calcium ion (Ca2+) transmembrane transport and calcium channel activity (AmiGO, 2017). Ca2+ is a critical signaling molecule in kidney development, all kidney cellular functions, and emergence of kidney diseases (Cao et al., 2006). The activation of Ca2+ channels regulates renal function by controlling the contraction of the renal vasculature, and Ca2+ channel blockers significantly increase the RBF and GFR significantly (Cao et al., 2006). Besides, PKD1L2 was associated with resistance and sensitivity to MTX in the breast cancer and gastric cancer (Udagawa et al., 2020). These studies have indicated that the functions of PKD1L2 are not only relevant to RBF and drug elimination, but also to be related to MTX.
The polymerase iota (POLI, Polι), polymerases eta (Polη), kappa (Polκ), and Rev1 belong to the Y-family of polymerases (McIntyre, 2020). The activities of Polι and other noncanonical human polymerases also appear responsible for the toxicity of nucleoside analogs in human immunodeficiency virus and hepatitis B virus (McIntyre, 2020). In addition, a deficiency of Polι in human cell lines was found to result in an increased sensitivity to oxidative damage, and this evidence suggests a role for pol ι in the cellular response to DNA damage induced by oxidative stress (Parsons et al., 2013). Based on these findings, POLI may be involved in adverse drug reactions as a drug-related gene.
The hypoxic microenvironment, which downregulates calcium transporter proteins and inhibits apoptotic cell signaling, is considered a contributing factor that promotes DNA instability in prostate cancer cells by upregulating NSE1 Homolog, SMC5-SMC6 Complex Component (NSMCE1)-related E3 ubiquitin ligases and inhibiting efficient DNA repair (Luo et al., 2020). NSMCE1 is upregulated in hypoxic environments; however, evidence linking this to drug-induced nephrotoxicity is lacking.
Human myotubularin-related proteins (MTMRs) six and nine belong to the myotubularin family (Clague and Lorenzo, 2005). MTMR6 inhibits the activity of calcium-activated potassium channels (type KCa3.1) and is localized in the plasma membrane (Srivastava et al., 2005; Choudhury et al., 2006; Srivastava et al., 2006). A high MTMR6 expression level has been observed in B-cell lymphoid leukemia cells with increased resistance to irradiation-induced apoptosis, implicating MTMR6 as a regulator of apoptosis (Vallat et al., 2003). According to a previous study (Zou et al., 2009), MTMR9 influences MTMR6 function at multiple levels, such as by increasing its stability, co-localization, and co-expression with MTMR6. Furthermore, the co-expression of MTMR6 and MTMR9 decreased etoposide-induced apoptosis (Zou et al., 2009). MTMR6 and MTMR9 may influence the response to chemotherapeutic agents. However, the frequency of some candidate variants was very rare, so replication analysis could not be performed. Therefore, additional analysis is needed on large scale data for these.
ABCG2 rs2231142, MTHFD1 rs2236225, MTHFR rs1801133, SLCO1B1 rs2306283 and rs4149056, and SLC19A1 rs1051266, which were found to be significant in previous studies, were not statistically significant in our study (Cheng et al., 2021; Schulte et al., 2021; Taylor et al., 2021). Other variants in ABCB1, SLCO1B1, and ABCC2 did not appear to be associated with delayed MTX clearance in our analysis because they were located in introns, were synonymous variants, or were very rare in East Asians.
If MTX toxicity occurs, an immediate increase in leucovorin dose may be helpful (Ackland and Schilsky, 1987). In severe cases, MTX may be removed through hemodialysis. Recombinant bacterial enzyme glucarpidase has been approved by the US Food and Drug Administration for use in patients with delayed MTX clearance. Glucarpidase converts MTX into its inactive metabolites and provides a nonrenal pathway for MTX elimination. Recently, a clinical decision support tool for predicting delayed MTX clearance was introduced (Taylor et al., 2020). However, delayed clearance was not predicted when MTX was administered. Furthermore, glucarpidase are difficult to use in many countries. Pharmacogenetic findings can help predict MTX nephrotoxicity in advance and allow the use of glucarpidase or other alternative chemotherapeutic agents instead of MTX in patients with variants related to delayed clearance.
This study has some limitations. First, because the variants were relatively frequent in Asians, some were not detected in replication cohorts with few Asians and could not be validated. Therefore, further analysis is required with large-scale datasets involving diverse ethnic backgrounds. Second, future research should be conducted using multi-omics data, such as transcriptomic and epigenomic data, to achieve an integrated understanding. Third, experimental work is needed to how the variants or genes affect MTX levels and toxicities. Due to the low variant frequencies and small sample size in our cohort, identifying interactions between candidate variants was challenging. It is also possible that MTX clearance may be delayed by a related gene variant that is fully linked to the ENG rs1800956. Further studies with larger cohorts could help to more clearly elucidate the combined effects of multiple variants, but our study offers a meaningful contribution to understanding individual genetic variability in this context.
To the best of our knowledge, this is the first study to identify the variants associated with MTX nephrotoxicity in Korean pediatric patients with ALL. In conclusion, ENG rs1800956 and PKD1L2 rs16954698 were the most significantly associated with delayed MTX clearance, and other genetic variants were also found. These findings will help us understand HD-MTX-induced nephrotoxicity and reduce adverse reactions caused by treatment modification.
The original contributions presented in the study are publicly available. This data can be found here: dbGaP repository, accession number phs000637.v1.p1.
The studies involving humans were approved by this study was approved by the institutional review boards of Seoul National University Hospital (IRB no. H-1702-109-833). The studies were conducted in accordance with the local legislation and institutional requirements. Written informed consent for participation in this study was provided by the participant’s legal guardians/next of kin.
JC: Conceptualization, Formal Analysis, Investigation, Methodology, Validation, Visualization, Writing–original draft, Writing–review and editing. HoK: Conceptualization, Formal Analysis, Investigation, Methodology, Validation, Visualization, Writing–original draft, Writing–review and editing. HyK: Conceptualization, Investigation, Writing–review and editing. KH: Investigation, Writing–review and editing. YM: Investigation, Writing–review and editing. K-NK: Investigation, Writing–review and editing. SY: Investigation, Writing–review and editing. KY: Conceptualization, Investigation, Writing–review and editing. SS: Investigation, Writing–review and editing. HI: Conceptualization, Funding acquisition, Investigation, Supervision, Writing–review and editing. JK: Conceptualization, Funding acquisition, Investigation, Project administration, Supervision, Writing–review and editing. HJK: Conceptualization, Funding acquisition, Investigation, Project administration, Supervision, Writing–review and editing.
The author(s) declare that financial support was received for the research, authorship, and/or publication of this article. This study was supported by a grant (16183MFDS541) from the Ministry of Food and Drug Safety in 2019.
The authors declare that the research was conducted in the absence of any commercial or financial relationships that could be construed as a potential conflict of interest.
All claims expressed in this article are solely those of the authors and do not necessarily represent those of their affiliated organizations, or those of the publisher, the editors and the reviewers. Any product that may be evaluated in this article, or claim that may be made by its manufacturer, is not guaranteed or endorsed by the publisher.
The Supplementary Material for this article can be found online at: https://www.frontiersin.org/articles/10.3389/fphar.2024.1480657/full#supplementary-material
Ackland, S. P., and Schilsky, R. L. (1987). High-dose methotrexate: a critical reappraisal. J. Clin. Oncol. 5 (12), 2017–2031. doi:10.1200/JCO.1987.5.12.2017
Adzhubei, I., Jordan, D. M., and Sunyaev, S. R. (2013). Predicting functional effect of human missense mutations using PolyPhen-2. Curr. Protoc. Hum. Genet. Chapter 7, Unit7.20. doi:10.1002/0471142905.hg0720s76
AmiGO (2017). Available at: https://amigo.geneontology.org/amigo/search/annotation?q=PKD1L2.[Accessed July 9 2024].
Amitai, I., Rozovski, U., El-Saleh, R., Shimony, S., Shepshelovich, D., Rozen-Zvi, B., et al. (2020). Risk factors for high-dose methotrexate associated acute kidney injury in patients with hematological malignancies. Hematol. Oncol. 38 (4), 584–588. doi:10.1002/hon.2759
Baehr, A., Pena, J. C., and Hu, D. J. (2015). Racial and ethnic disparities in adverse drug events: a systematic review of the literature. J. Racial Ethn. Health Disparities 2 (4), 527–536. doi:10.1007/s40615-015-0101-3
Basile, D. P., and Yoder, M. C. (2014). Renal endothelial dysfunction in acute kidney ischemia reperfusion injury. Cardiovasc Hematol. Disord. Drug Targets 14 (1), 3–14. doi:10.2174/1871529x1401140724093505
Burdmann, E. A., Andoh, T. F., Yu, L., and Bennett, W. M. (2003). Cyclosporine nephrotoxicity. Semin. Nephrol. 23 (5), 465–476. doi:10.1016/s0270-9295(03)00090-1
Bus, P., Gerrits, T., Heemskerk, S. A. C., Zandbergen, M., Wolterbeek, R., Bruijn, J. A., et al. (2018). Endoglin mediates vascular endothelial growth factor-A-induced endothelial cell activation by regulating akt signaling. Am. J. Pathol. 188 (12), 2924–2935. doi:10.1016/j.ajpath.2018.08.005
CADD (2014). Available at: https://cadd.bihealth.org/info.[Accessed September 28 2024].
Cao, C., Goo, J. H., Lee-Kwon, W., and Pallone, T. L. (2006). Vasa recta pericytes express a strong inward rectifier K+ conductance. Am. J. Physiol. Regul. Integr. Comp. Physiol. 290 (6), R1601–R1607. doi:10.1152/ajpregu.00877.2005
Castellana, S., and Mazza, T. (2013). Congruency in the prediction of pathogenic missense mutations: state-of-the-art web-based tools. Brief. Bioinform 14 (4), 448–459. doi:10.1093/bib/bbt013
Cheng, Y., Chen, M. H., Zhuang, Q., Lin, B. J., Chen, Y. Y., Yang, L., et al. (2021). Genetic factors involved in delayed methotrexate elimination in children with acute lymphoblastic leukemia. Pediatr. Blood Cancer 68 (5), e28858. doi:10.1002/pbc.28858
Choudhury, P., Srivastava, S., Li, Z., Ko, K., Albaqumi, M., Narayan, K., et al. (2006). Specificity of the myotubularin family of phosphatidylinositol-3-phosphatase is determined by the PH/GRAM domain. J. Biol. Chem. 281 (42), 31762–31769. doi:10.1074/jbc.M606344200
Clague, M. J., and Lorenzo, O. (2005). The myotubularin family of lipid phosphatases. Traffic 6 (12), 1063–1069. doi:10.1111/j.1600-0854.2005.00338.x
Das, S., Forer, L., Schonherr, S., Sidore, C., Locke, A. E., Kwong, A., et al. (2016). Next-generation genotype imputation service and methods. Nat. Genet. 48 (10), 1284–1287. doi:10.1038/ng.3656
Dumas, S. J., Meta, E., Borri, M., Luo, Y., Li, X., Rabelink, T. J., et al. (2021). Phenotypic diversity and metabolic specialization of renal endothelial cells. Nat. Rev. Nephrol. 17 (7), 441–464. doi:10.1038/s41581-021-00411-9
Eilbeck, K., Quinlan, A., and Yandell, M. (2017). Settling the score: variant prioritization and Mendelian disease. Nat. Rev. Genet. 18 (10), 599–612. doi:10.1038/nrg.2017.52
Garneau, A. P., Riopel, J., and Isenring, P. (2015). Acute methotrexate-induced crystal nephropathy. N. Engl. J. Med. 373 (27), 2691–2693. doi:10.1056/NEJMc1507547
Genomes Project, C., Auton, A., Brooks, L. D., Durbin, R. M., Garrison, E. P., Kang, H. M., et al. (2015). A global reference for human genetic variation. Nature 526 (7571), 68–74. doi:10.1038/nature15393
Gerrits, T., Brouwer, I. J., Dijkstra, K. L., Wolterbeek, R., Bruijn, J. A., Scharpfenecker, M., et al. (2022). Endoglin is an important mediator in the final common pathway of chronic kidney disease to end-stage renal disease. Int. J. Mol. Sci. 24 (1), 646. doi:10.3390/ijms24010646
Gu, Y. Y., Liu, X. S., Huang, X. R., Yu, X. Q., and Lan, H. Y. (2020). Diverse role of TGF-β in kidney disease. Front. Cell Dev. Biol. 8, 123. doi:10.3389/fcell.2020.00123
Howard, S. C., McCormick, J., Pui, C. H., Buddington, R. K., and Harvey, R. D. (2016). Preventing and managing toxicities of high-dose methotrexate. Oncologist 21 (12), 1471–1482. doi:10.1634/theoncologist.2015-0164
HPA (2015). Available at: https://www.proteinatlas.org/ENSG00000106991-ENG/tissue.[Accessed September 28 2024].
Jourde-Chiche, N., Fakhouri, F., Dou, L., Bellien, J., Burtey, S., Frimat, M., et al. (2019). Endothelium structure and function in kidney health and disease. Nat. Rev. Nephrol. 15 (2), 87–108. doi:10.1038/s41581-018-0098-z
Karczewski, K. J., Francioli, L. C., Tiao, G., Cummings, B. B., Alfoldi, J., Wang, Q., et al. (2020). The mutational constraint spectrum quantified from variation in 141,456 humans. Nature 581 (7809), 434–443. doi:10.1038/s41586-020-2308-7
Kumar, P., Henikoff, S., and Ng, P. C. (2009). Predicting the effects of coding non-synonymous variants on protein function using the SIFT algorithm. Nat. Protoc. 4 (7), 1073–1081. doi:10.1038/nprot.2009.86
Larsen, E. C., Devidas, M., Chen, S., Salzer, W. L., Raetz, E. A., Loh, M. L., et al. (2016). Dexamethasone and high-dose methotrexate improve outcome for children and young adults with high-risk B-acute lymphoblastic leukemia: a report from children's oncology group study AALL0232. J. Clin. Oncol. 34 (20), 2380–2388. doi:10.1200/JCO.2015.62.4544
Li, A., Tian, X., Sung, S. W., and Somlo, S. (2003). Identification of two novel polycystic kidney disease-1-like genes in human and mouse genomes. Genomics 81 (6), 596–608. doi:10.1016/s0888-7543(03)00048-x
Liu, B., Liu, G., Liu, B., Guo, Y., Peng, N., and Li, T. (2024). Correlation between gene polymorphism and adverse reactions of high-dose methotrexate in osteosarcoma patients: a systematic review and meta-analysis. World J. Surg. Oncol. 22 (1), 19. doi:10.1186/s12957-023-03287-0
Luo, A., Gong, Y., Kim, H., and Chen, Y. (2020). Proteome dynamics analysis identifies functional roles of SDE2 and hypoxia in DNA damage response in prostate cancer cells. Nar. Cancer 2 (2), zcaa010. doi:10.1093/narcan/zcaa010
Mailman, M. D., Feolo, M., Jin, Y., Kimura, M., Tryka, K., Bagoutdinov, R., et al. (2007). The NCBI dbGaP database of genotypes and phenotypes. Nat. Genet. 39 (10), 1181–1186. doi:10.1038/ng1007-1181
McIntyre, J. (2020). Polymerase iota - an odd sibling among Y family polymerases. DNA Repair (Amst) 86, 102753. doi:10.1016/j.dnarep.2019.102753
McLaren, W., Gil, L., Hunt, S. E., Riat, H. S., Ritchie, G. R., Thormann, A., et al. (2016). The Ensembl variant effect predictor. Genome Biol. 17 (1), 122. doi:10.1186/s13059-016-0974-4
Narita, K., Kobayashi, H., Abe, Y., Takeuchi, M., and Matsue, K. (2017). Evaluation of risk factors and survival effect of delayed excretion of high-dose methotrexate in patients with leukemia and lymphoma. Blood 130.
Park, Y., Kim, H., Choi, J. Y., Yun, S., Min, B. J., Seo, M. E., et al. (2019). Star allele-based haplotyping versus gene-wise variant burden scoring for predicting 6-mercaptopurine intolerance in pediatric acute lymphoblastic leukemia patients. Front. Pharmacol. 10, 654. doi:10.3389/fphar.2019.00654
Parsons, J. L., Nicolay, N. H., and Sharma, R. A. (2013). Biological and therapeutic relevance of nonreplicative DNA polymerases to cancer. Antioxid. Redox Signal 18 (8), 851–873. doi:10.1089/ars.2011.4203
Pentel, P., and Benowitz, N. (1984). Pharmacokinetic and pharmacodynamic considerations in drug therapy of cardiac emergencies. Clin. Pharmacokinet. 9 (4), 273–308. doi:10.2165/00003088-198409040-00001
Perazella, M. A., and Luciano, R. L. (2015). Review of select causes of drug-induced AKI. Expert Rev. Clin. Pharmacol. 8 (4), 367–371. doi:10.1586/17512433.2015.1045489
Ramsey, L. B., Balis, F. M., O'Brien, M. M., Schmiegelow, K., Pauley, J. L., Bleyer, A., et al. (2018). Consensus guideline for use of glucarpidase in patients with high-dose methotrexate induced acute kidney injury and delayed methotrexate clearance. Oncologist 23 (1), 52–61. doi:10.1634/theoncologist.2017-0243
Richard, J., Martin, A. A. F., and Walsh, M. C. (2020). Fanaroff and martin's neonatal-perinatal medicine. Elsevier.
Rios, I., Lopez-Navarro, B., Torres-Torresano, M., Soler Palacios, B., Simon-Fuentes, M., Dominguez-Soto, A., et al. (2023). GSK3β inhibition prevents macrophage reprogramming by high-dose methotrexate. J. Innate Immun. 15 (1), 283–296. doi:10.1159/000526622
Rossi, E., Bernabeu, C., and Smadja, D. M. (2019). Endoglin as an adhesion molecule in mature and progenitor endothelial cells: a function beyond TGF-β. Front. Med. (Lausanne) 6, 10. doi:10.3389/fmed.2019.00010
Santucci, R., Leveque, D., Lescoute, A., Kemmel, V., and Herbrecht, R. (2010). Delayed elimination of methotrexate associated with co-administration of proton pump inhibitors. Anticancer Res. 30 (9), 3807–3810.
Schulte, R. R., Choi, L., Utreja, N., Van Driest, S. L., Stein, C. M., and Ho, R. H. (2021). Effect of SLCO1B1 polymorphisms on high-dose methotrexate clearance in children and young adults with leukemia and lymphoblastic lymphoma. Clin. Transl. Sci. 14 (1), 343–353. doi:10.1111/cts.12879
Schwartz, G. J., and Work, D. F. (2009). Measurement and estimation of GFR in children and adolescents. Clin. J. Am. Soc. Nephrol. 4 (11), 1832–1843. doi:10.2215/CJN.01640309
Song, Z., Hu, Y., Liu, S., Jiang, D., Yi, Z., Benjamin, M. M., et al. (2021). The role of genetic polymorphisms in high-dose methotrexate toxicity and response in hematological malignancies: a systematic review and meta-analysis. Front. Pharmacol. 12, 757464. doi:10.3389/fphar.2021.757464
Spurgeon, K. R., Donohoe, D. L., and Basile, D. P. (2005). Transforming growth factor-beta in acute renal failure: receptor expression, effects on proliferation, cellularity, and vascularization after recovery from injury. Am. J. Physiol. Ren. Physiol. 288 (3), F568–F577. doi:10.1152/ajprenal.00330.2004
Srivastava, S., Ko, K., Choudhury, P., Li, Z., Johnson, A. K., Nadkarni, V., et al. (2006). Phosphatidylinositol-3 phosphatase myotubularin-related protein 6 negatively regulates CD4 T cells. Mol. Cell Biol. 26 (15), 5595–5602. doi:10.1128/MCB.00352-06
Srivastava, S., Li, Z., Lin, L., Liu, G., Ko, K., Coetzee, W. A., et al. (2005). The phosphatidylinositol 3-phosphate phosphatase myotubularin-related protein 6 (MTMR6) is a negative regulator of the Ca2+-activated K+ channel KCa3.1. Mol. Cell Biol. 25 (9), 3630–3638. doi:10.1128/MCB.25.9.3630-3638.2005
Taylor, Z. L., Mizuno, T., Punt, N. C., Baskaran, B., Navarro Sainz, A., Shuman, W., et al. (2020). MTXPK.org: a clinical decision support tool evaluating high-dose methotrexate pharmacokinetics to inform post-infusion care and use of glucarpidase. Clin. Pharmacol. Ther. 108 (3), 635–643. doi:10.1002/cpt.1957
Taylor, Z. L., Vang, J., Lopez-Lopez, E., Oosterom, N., Mikkelsen, T., and Ramsey, L. B. (2021). Systematic review of pharmacogenetic factors that influence high-dose methotrexate pharmacokinetics in pediatric malignancies. Cancers (Basel) 13 (11), 2837. doi:10.3390/cancers13112837
Torres, R. P., Santos, F. P., and Branco, J. C. (2022). Methotrexate: implications of pharmacogenetics in the treatment of patients with rheumatoid arthritis. ARP Rheumatol. 1 (3), 225–229.
Treon, S. P., and Chabner, B. A. (1996). Concepts in use of high-dose methotrexate therapy. Clin. Chem. 42 (8 Pt 2), 1322–1329. doi:10.1093/clinchem/42.8.1322
Udagawa, C., Sasaki, Y., Tanizawa, Y., Suemizu, H., Ohnishi, Y., Nakamura, Y., et al. (2020). Whole-exome sequencing of 79 xenografts as a potential approach for the identification of genetic variants associated with sensitivity to cytotoxic anticancer drugs. PLoS One 15 (9), e0239614. doi:10.1371/journal.pone.0239614
Vallat, L., Magdelenat, H., Merle-Beral, H., Masdehors, P., Potocki de Montalk, G., Davi, F., et al. (2003). The resistance of B-CLL cells to DNA damage-induced apoptosis defined by DNA microarrays. Blood 101 (11), 4598–4606. doi:10.1182/blood-2002-06-1743
Wiczer, T., Dotson, E., Tuten, A., Phillips, G., and Maddocks, K. (2016). Evaluation of incidence and risk factors for high-dose methotrexate-induced nephrotoxicity. J. Oncol. Pharm. Pract. 22 (3), 430–436. doi:10.1177/1078155215594417
Widemann, B. C., Balis, F. M., Kempf-Bielack, B., Bielack, S., Pratt, C. B., Ferrari, S., et al. (2004). High-dose methotrexate-induced nephrotoxicity in patients with osteosarcoma. Cancer 100 (10), 2222–2232. doi:10.1002/cncr.20255
Xu, M., Wu, S., Wang, Y., Zhao, Y., Wang, X., Wei, C., et al. (2022). Association between high-dose methotrexate-induced toxicity and polymorphisms within methotrexate pathway genes in acute lymphoblastic leukemia. Front. Pharmacol. 13, 1003812. doi:10.3389/fphar.2022.1003812
Yuasa, T., Takakura, A., Denker, B. M., Venugopal, B., and Zhou, J. (2004). Polycystin-1L2 is a novel G-protein-binding protein. Genomics 84 (1), 126–138. doi:10.1016/j.ygeno.2004.02.008
Zhang, W., Zhang, Q., Zheng, T. T., Zhen, J. C., and Niu, X. H. (2016). Delayed high-dose methotrexate excretion and influencing factors in osteosarcoma patients. Chin. Med. J. Engl. 129 (21), 2530–2534. doi:10.4103/0366-6999.192781
Keywords: methotrexate, children, acute lymphoblastic leukemia, pediatric, pharmacogenomics, adverse reactions, delayed clearance
Citation: Choi JY, Kwon H, Kim H, Hong KT, Ma Y, Koh K-N, Yun S, Yoo KH, Song SH, Im HJ, Kim JH and Kang HJ (2024) Novel genomic variants influencing methotrexate delayed clearance in pediatric patients with acute lymphoblastic leukemia. Front. Pharmacol. 15:1480657. doi: 10.3389/fphar.2024.1480657
Received: 14 August 2024; Accepted: 24 October 2024;
Published: 14 November 2024.
Edited by:
Zhi-Yao He, Sichuan University, ChinaCopyright © 2024 Choi, Kwon, Kim, Hong, Ma, Koh, Yun, Yoo, Song, Im, Kim and Kang. This is an open-access article distributed under the terms of the Creative Commons Attribution License (CC BY). The use, distribution or reproduction in other forums is permitted, provided the original author(s) and the copyright owner(s) are credited and that the original publication in this journal is cited, in accordance with accepted academic practice. No use, distribution or reproduction is permitted which does not comply with these terms.
*Correspondence: Ho Joon Im, aG9qaW1AYW1jLnNlb3VsLmty; Ju Han Kim, anVoYW5Ac251LmFjLmty; Hyoung Jin Kang, a2FuZ2hqQHNudS5hYy5rcg==
†These authors have contributed equally to this work.
Disclaimer: All claims expressed in this article are solely those of the authors and do not necessarily represent those of their affiliated organizations, or those of the publisher, the editors and the reviewers. Any product that may be evaluated in this article or claim that may be made by its manufacturer is not guaranteed or endorsed by the publisher.
Research integrity at Frontiers
Learn more about the work of our research integrity team to safeguard the quality of each article we publish.