- 1Institute of Urology, The Third Affiliated Hospital of Shenzhen University, Shenzhen University, Shenzhen, China
- 2Institute of Pain, The Affiliated Hospital of Southwest Jiaotong University, The Chengdu Third People’s Hospital, Chengdu, China
Oridonin (ORI), an ent-kaurane diterpenoid derived from Rabdosia rubescens (Hemsl.) H.Hara, serves as the primary bioactive component of this plant. It demonstrates a broad spectrum of therapeutic activities, including moderate to potent anticancer properties, alongside anti-inflammatory, antibacterial, antifibrotic, immunomodulatory, and neuromodulatory effects, thus influencing diverse biological processes. However, its clinical potential is significantly constrained by poor aqueous solubility and limited bioavailability. In alignment with the approach of developing drug candidates from natural compounds, various strategies, such as structural modification and nanocarrier systems, have been employed to address these challenges. This review provides an overview of ORI-based nano-delivery systems, emphasizing their potential to improve the clinical applicability of oridonin in oncology. Although some progress has been made in advancing ORI nano-delivery research, it remains insufficient for clinical implementation, necessitating further investigation.
1 Introduction
Cancer remains a critical global health concern and continues to rank as the second leading cause of death in the United States (Siegel et al., 2024). In 2020, approximately 19.3 million new cancer cases were reported globally, along with 10 million deaths attributed to cancer (Sung et al., 2021). Conventional treatment modalities, including surgery, chemotherapy, and radiotherapy, remain the cornerstone of cancer management but are often accompanied by severe toxic side effects and the emergence of drug resistance (Bell, 2010). Medicinal plants have long been recognized as a vital source for the discovery of novel anticancer agents (Luo et al., 2019). Notably, over half of the new drugs approved between 1982 and 2002 were either directly or indirectly derived from natural sources. Historically, more than 60% of natural compounds and traditional Chinese herbal medicines have been utilized for their anticancer properties (Tran et al., 2017). Oridonin (ORI), an ent-kaurane diterpenoid extracted from Rabdosia rubescens (Hemsl.) H.Hara, is the principal active compound of this herb, known as Donglingcao in traditional East Asian medicine (Tan et al., 2011). This plant exhibits a range of pharmacological effects, including anti-tumor (Liu et al., 2012; Liu et al., 2021; Li et al., 2023a), anti-inflammatory (Ku and Lin, 2013), anti-microbial (Chen et al., 2023a; Wang et al., 2024), anti-aging (An et al., 2022; Yasuda et al., 2022), anti-fibrotic (Gao et al., 2022), and central nervous system modulatory activities (Liu and Du, 2020; Li et al., 2023b). ORI has garnered increasing attention due to its potent ability to induce apoptosis, inhibit protein synthesis (Li et al., 2016), and exert anti-angiogenic and anti-metastatic effects in both in vitro and in vivo tumor models (Abdullah et al., 2021; Tian et al., 2017).
Extensive research has demonstrated ORI’s ability to suppress the proliferation of various cancer types, such as breast (Wang et al., 2013; Wu et al., 2016; Yao et al., 2020), gastric (Gao et al., 2023), colorectal (Zhou et al., 2023), thyroid (Liu et al., 2022), and ovarian cancers (Wang and Zhu, 2019). Nevertheless, ORI’s clinical utility is limited by its poor water solubility, low bioavailability, and associated toxicities, including pharmacological and cardiotoxic effects observed in preclinical studies (Li et al., 2021; Xu et al., 2024). Consequently, developing strategies to mitigate these side effects is essential for advancing ORI as a viable anticancer agent (Zhang et al., 2020). Nanoparticles (NPs), as drug carriers, are capable of improving the distribution of drugs in the body, increasing their bioavailability, and enabling targeted delivery to tumor tissues due to their small size and modifiable surfaces. In recent years, nanotechnology has been employed to develop various diagnostic and therapeutic strategies for cancer. Several relatively safe and low-toxicity nanotechnology strategies have demonstrated clinical development potential, including photodynamic therapy (PDT) (Hao et al., 2022; Gunaydin et al., 2021), photothermal therapy (PTT) (Zhao et al., 2021; Qin et al., 2023), and immunotherapy (Barnestein et al., 2022; Li et al., 2022). The focus of drug delivery systems based on nanocarriers lies in enhancing the permeability and retention of drugs or functionalizing the carrier surfaces for targeted delivery (Lv et al., 2024). These systems offer numerous advantages, such as minimizing off-target toxicity, shielding cytotoxic drugs from degradation, extending drug half-life, increasing drug payload and solubility, and reducing renal clearance, making them highly promising for cancer treatment (Zhang et al., 2023a). This review explores various ORI delivery platforms and fabrication techniques, including polymeric nanoparticles, micelles, liposomes, microemulsions, protein NPs, metallic and non-metallic NPs, and cocrystal formations. The review also evaluates the potential of ORI-based drug delivery systems in enhancing bioavailability and specificity, reducing toxicity, and highlights future research directions based on current progress in the field.
2 ORI properties
2.1 Plant origin and distribution
Isodon rubescens has been utilized in traditional Chinese medicine (TCM) for over a millennium, first documented in the Shen Nong Ben Cao Jing. In the Compendium of Materia Medica, it is categorized as a superior herb, traditionally prescribed for symptoms such as cough, wheezing, phlegm, and chest tightness. The Supplement to Materia Medica also notes its efficacy in treating persistent cough, hemoptysis, and chest pain. In the early 1970s, during a survey of traditional medicine resources in China, researchers discovered that residents of Linzhou City, Henan Province—an area with a high incidence of esophageal cancer—were using a solution derived from Isodon rubescens, which alleviated symptoms of esophageal cancer and pharyngitis. This discovery spurred interest in its anticancer properties, leading to the isolation and identification of its medicinal components, including the extraction of its primary active ingredient, ORI. Isodon rubescens grows predominantly in the Taihang Mountains of Hunan Province, China, flourishing on sun-exposed slopes at altitudes ranging from 100 to 2,800 m (Chen et al., 2022).
Isodon rubescens is classified into four varieties based on the types and concentrations of its primary chemical constituents, ent-kauranoid diterpenoids. The first variety, found in the Taihang Mountains in Jiyuan, Henan Province, mainly contains 7,20-epoxide ent-kauranoid diterpenoid derivatives. The second variety, including Lu Rabdosia and Gui Rabdosia from Henan Province, predominantly features 6,7-fractured-ent-kaurane diterpenoids and ORI. The third variety consists of Rabdosia rubescensin and Lushan Rubescensin, while the fourth, Xin Rabdosia, primarily contains unoxidized ent-kaurane diterpenoids at the C-20 position. Notably, ORI and ORI B are found exclusively in the Jiyuan variety from Henan Province. ORI’s main chemical structure is the diterpenoid compound, specifically the ent-kaurene tetracyclic diterpenoid represented by its molecular formula C20H28O6 (Sun et al., 2006; Xie et al., 2011) (Figure 1).
2.2 Molecular characteristics and anticancer effects of ORI
Various ORI derivatives have shown enhanced efficacy and improved pharmacokinetic properties (Zhang et al., 2020; Sobral et al., 2023). The α-methylene cyclopentanone on ORI’s D-ring plays a critical role in its anticancer function, which can be diminished by ring cleavage or methylene saturation (Sun et al., 1995). Furthermore, the hydrogen bond between the 6-hydroxy and 15-carbonyl groups increases the electrophilicity of C-17, thereby strengthening its interaction with electrophilic enzymes in tumor cells. For example, cytotoxicity and water solubility have been improved by adding hydrophilic side chains to the 1-O- and 14-O-hydroxyl groups (Xu et al., 2016). Modifying the A-ring of ORI A, such as by introducing new functional groups, has been shown to enhance apoptosis induction (Ding et al., 2013). The D-ring enone of dong quinone is also essential for its anticancer effects, and derivatives with substituted benzene moieties at the C-17 position have demonstrated improved apoptotic induction and G2 phase arrest (Shen et al., 2018) (Figure 1).
Despite the well-documented anticancer potential of ORI, its clinical application is hindered by rapid blood concentration decline following intravenous administration, poor water solubility, and oral bioavailability of less than 5% (Xu et al., 2006). Advances in nanotechnology have led to the development of multifunctional nanoplatforms designed to improve ORI’s bioavailability, holding promise for clinical use (Ali et al., 2024). However, while ORI derivatives have been widely investigated in cancer therapy, a comprehensive review focusing on the anticancer advantages and mechanisms of ORI-based nanoparticle drug delivery systems (DDS) is still lacking.
2.3 Anticancer mechanisms of ORI
Decades of research have revealed that ORI exerts anticancer effects through multiple mechanisms, including inducing tumor cell apoptosis and autophagy, inhibiting proliferation, preventing angiogenesis and metastasis, and enhancing radiosensitivity (Liu et al., 2012; Li et al., 2011a). Recently, ORI has gained recognition as a promising anticancer agent due to its multifaceted activity across various malignancies via distinct pathways (Figure 2).
2.3.1 Proliferation inhibition
Cdc2, a key regulator of mitosis in fission yeast, functions as a protein kinase activated through cyclin binding, thereby driving cell cycle progression (Suski et al., 2021). Cyclin B-CDK1, critical for controlling events associated with cell cycle regulation, predominantly governs mitotic progression and cell division, leading to G2/M phase arrest (Bury et al., 2021). Furthermore, ataxia telangiectasia mutated enhances G2/M arrest in A549 cells (Zheng et al., 2017). Recent studies suggest ORI induces G2/M arrest through these pathways (Qi et al., 2012). In addition, ORI inhibits HepG2 proliferation in a time- and dose-dependent manner, arresting the cell cycle at the S phase (Li et al., 2020a).
2.3.2 Autophagy promotion
Autophagy, a vital metabolic process, involves the degradation and recycling of cellular components through lysosomal delivery. Research shows that low doses of active ORI enhance the phagocytosis of apoptotic cells by macrophage-like U937 cells and induce autophagy in these cells (Zang et al., 2012). ORI also promotes macrophage phagocytosis of apoptotic bodies via the autophagy-lysosomal pathway, mediated by toll-like receptor (TLR)4 signaling, enhancing cell migration (Zang et al., 2019). Additionally, ORI triggers apoptosis through the extrinsic apoptotic pathway involving Fas and FasL signaling cascades (Zang et al., 2013).
2.3.3 Apoptosis induction
ORI induces apoptosis or necrosis of tumor cells in a dose-dependent manner by modulating several pathways linked to apoptosis, including the mitogen-activated protein kinase (MAPK) family and phosphoinositide 3-kinase (PI3K)/AKT pathways. In breast cancer cells, ORI enhances apoptosis by promoting autophagy. Furthermore, it increases the radiosensitivity of lung cancer cells by upregulating Bax and downregulating Bcl-2, facilitating radiation-induced cell death by accelerating DNA damage in non-small-cell lung cancer (Li et al., 2018a).
2.3.4 Metastasis inhibition
ORI also inhibits cancer invasion and metastasis by suppressing the expression of VEGF family members such as ascular endothelial growth factor (VEGF)-A, VEGFR-2, and VEGFR-3 (Jiang et al., 2020). It impedes metastasis through mechanisms such as the inhibition of the TGF-β/SMAD signaling pathway, blockade of the EGF/epidermal growth factor receptor (EGFR)/extracellular signal-regulated kinase (ERK) signaling cascade (Bu et al., 2019; Che et al., 2021), reduction of mechanistic target of rapamycin (mTOR) signaling pathway phosphorylation, downregulation of lncRNA AFAP1-AS1, and enhancement of p53 expression (Ye et al., 2012; Li et al., 2007). These actions collectively underscore ORI’s potential as an effective therapeutic agent against cancer invasion and metastasis.
2.3.5 Metabolism regulation
The aerobic glycolysis characteristic of tumors, known as the Warburg effect, is closely linked to the overexpression of hypoxia-inducible factor 1 alpha (HIF-1α), a pivotal regulator of tumor energy metabolism (Chelakkot et al., 2023). Research has shown that ORI suppresses glycolytic flux by reducing HIF-1α expression and concurrently lowering vascular endothelial factor levels, thereby inhibiting angiogenesis in breast cancer cells such as MDA-MB-231 and 4T1 (Liu et al., 2024a). This ultimately results in the inhibition of breast cancer cell proliferation. Additionally, studies indicate that ORI upregulates miR-214 expression in H9c2 rat cardiomyocytes, affecting the PI3K/AKT/mTOR signaling pathway, which subsequently downregulates HIF-1α expression and inhibits glycolytic activity. This process induces apoptosis and autophagy in H9c2 cells under hypoxic conditions.
2.3.6 Mechanism of action of ORI in different cancers
Previous research has extensively explored ORI’s effects across various cancer cell lines, including colorectal, breast, lung, gastric, and esophageal cancers, by investigating its mechanisms and pathways of action. The inhibition mechanisms of ORI across different cancers are detailed in Table 1.
3 Current status of bibliometric analysis of DDS for ORI
To assess the current landscape of drug delivery research on Rabdosia rubescens meglumine, a comprehensive analysis of keywords and study distribution was conducted. An extensive review of publications related to Rabdosia rubescens in cancer treatment from 2004 to 2024 was performed using search engines such as Web of Science and PubMed. Figure 3A reveals a general upward trend in research publications, with peaks in 2016, 2018, and 2020, followed by a notable decline in 2023 and a slight recovery in 2024.
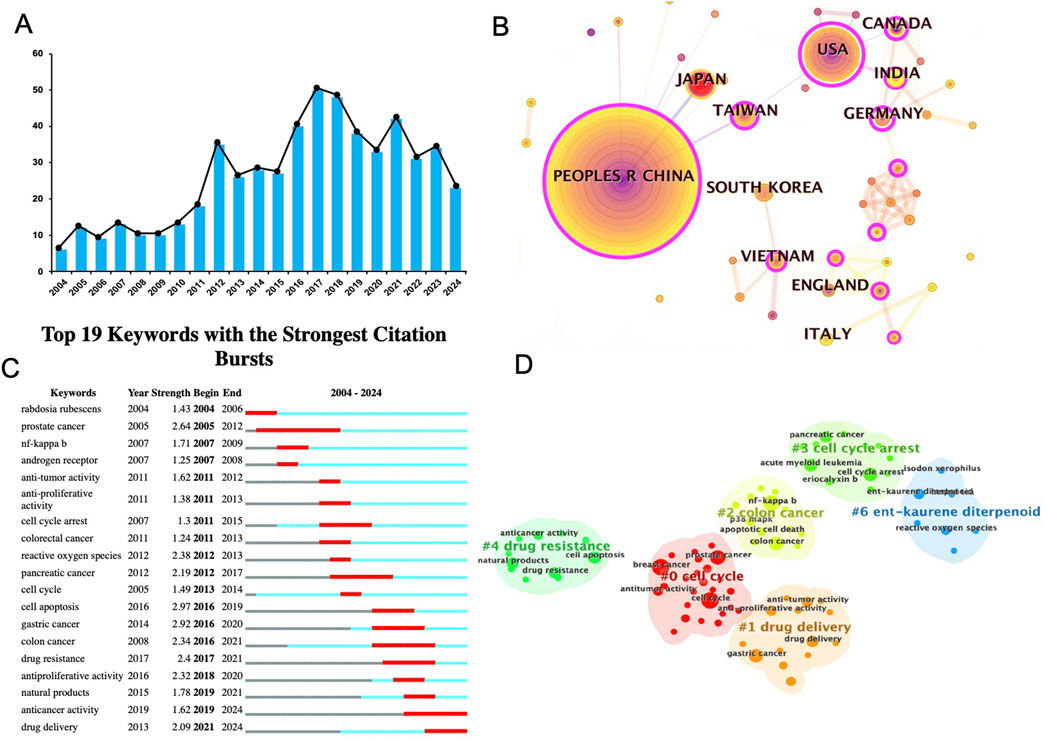
Figure 3. Current status of bibliometric analysis of DDS for ORI. (A) Annual publication trends (2004–2024); (B) Global research distribution; (C) Top keywords with the strongest citation bursts (2004–2024); (D) Keyword clusters in Rabdosia rubescens research.
Additionally, a global research distribution map (Figure 3B) was developed to summarize the countries contributing to studies on Rabdosia rubescens meglumine for cancer therapy. China emerged as the leading contributor, followed by the United States, Japan, and Germany. Other notable contributors included South Korea, India, Canada, and several European nations, such as the United Kingdom and Italy. This distribution reflects the global interest in leveraging Rabdosia rubescens to advance cancer treatment, particularly in drug delivery systems.
Figure 3C highlights the top 19 keywords linked to Rabdosia rubescens research that experienced significant citation bursts, signaling periods of heightened focus on these topics. Keywords such as “drug resistance,” “drug delivery,” and “antiproliferative activity” showed particularly strong bursts, reflecting critical areas of study across various years and emphasizing the clinical significance and potential of ongoing ORI-DDS research.
A brief overview of ORI’s anticancer mechanisms was also conducted, alongside an extraction of keyword clusters related to R. rubescens research, as depicted in Figure 3D. This keyword co-occurrence map reveals major clusters focused on key topics such as Cell Cycle (#0), Drug Delivery (#1), Colon Cancer (#2), Cell Cycle Blockade (#3), Drug Resistance (#4), and Ent-Kaurene Diterpenoid (#6). These clusters illustrate key research areas, including mechanisms of action (e.g., cell cycle arrest), therapeutic applications (e.g., drug delivery), and specific cancer types (e.g., colon cancer).
The “Drug Delivery” panel is particularly relevant to this review, as it emphasizes the various technologies developed to optimize cynarin delivery. Innovations such as nanodelivery systems, liposomes, and other advanced DDS have been designed to enhance the therapeutic efficacy of Rabdosia rubescens while minimizing potential side effects.
Overall, this analysis provides a thorough examination of R. rubescens research in cancer therapeutics, focusing particularly on drug delivery systems. By capturing trends, highlighting key contributors, and identifying significant research topics, the analysis underscores the importance of innovative drug delivery approaches in improving the efficacy of Rabdosia rubescens for cancer treatment.
4 Nano drug delivery system with ORI as a functional molecule
Studies indicate that ORI shows significant potential as an anticancer agent. However, its clinical application is hindered by poor water solubility and low bioavailability, which stands at only 5%. Although ORI is generally regarded as safe, recent research has revealed a certain level of cytotoxicity to liver tissue, possibly linked to the inhibition of embryonic development. Additionally, exposure to oridonin (ORI) at concentrations of 25 μM (μM) or higher for 48 h notably elevates cytosolic Ca2+ concentration, promotes ceramide formation, and induces erythrocyte death through suicidal pathways (Jilani et al., 2011).
To address these challenges, the development of advanced drug delivery systems is essential to improve ORI’s solubility, enhance bioavailability, and reduce toxicity (Table 2; Figure 4). Several novel delivery platforms, including liposomes, microemulsions, micelles, protein-based nanocarriers, metal-organic frameworks (MOFs), and nanosuspensions, have been investigated to boost bioavailability, prolong systemic circulation, and mitigate the adverse effects of ORI (Lu et al., 2016).
4.1 Liposomes
The clinical advancement of ORI in cancer therapy remains restricted due to its low bioavailability and rapid plasma clearance. Liposomes, a well-established nanocarrier, present significant advantages such as high biocompatibility, low toxicity, and improved drug bioavailability (Wang et al., 2021). They can be engineered for tumor-specific drug delivery, enabling precise targeting of active compounds to tumor cells (Li et al., 2020b). Structurally, liposomes are spherical vesicles comprising one or more phospholipid bilayers, capable of encapsulating both amphiphilic and lipophilic molecules within their matrix (Shah et al., 2020).
ORI has shown the capacity to disrupt reactive oxygen species (ROS) through covalent interactions with glutathione. Aleimide-liposomes, noted for their efficiency in ROS-targeting delivery systems, have enhanced ORI’s solubility and pharmacokinetic profile, amplifying its ROS-disruptive capabilities. Additionally, ORI-loaded liposomes targeting acute myeloid leukemia (AML) facilitated intracellular glutathione (GSH) depletion, increasing the concentration and specificity of ORI within AML cells (Figure 5) (Liu et al., 2024b). Compared to control groups, ORI liposomes significantly extended the survival of tumor-bearing mice, exhibiting stronger cytotoxic effects and higher safety margins.
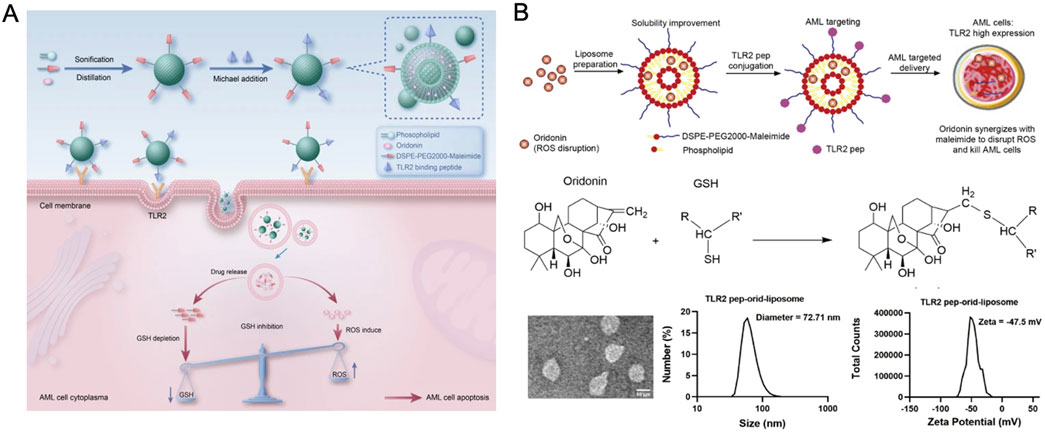
Figure 5. (A) Schematic of TLR2 pep-ORI-liposome preparation. (B) Material characterization of TLR2 pep-ORI-liposome. Reproduced with permission from Ref (Liu et al., 2024b). Copyright 2024, Springer Nature.
Studies have further demonstrated that ethanol-optimized ORI liposomes enter cells via endocytosis, leading to enhanced cellular uptake and accumulation in targeted cells. The strong affinity of liposomes for cell membranes allows ORI encapsulated within liposomes to penetrate cells more efficiently, thereby enhancing its antitumor effects (Wang et al., 2022). Zheng et al. developed a nanostructured lipid carrier (NLC) loaded with ORI, which exhibited higher area under the curve (AUC) values and prolonged bloodstream retention compared to ORI solution, underscoring the potential of NLCs to mitigate ORI’s rapid plasma clearance (Zheng et al., 2012a).
Wang et al. (2009) utilized polyethylene glycol-distearyl phosphatidylethanolamine (PEG2000-DSPE) as a surface coating to formulate stealth liposomes loaded with ORI, which demonstrated significant efficacy in inhibiting solid tumor growth. These liposomes extended the circulation time of ORI in mice, decreased its accumulation in the reticuloendothelial system, and enhanced its anticancer activity. Additionally, ORI-loaded liposome microbubbles targeting the folate receptor exhibited a higher binding affinity for HepG-2 cells, leading to significantly increased anticancer potency (Wang et al., 2017).
Wang et al. (2019) further developed an innovative drug delivery system by covalently linking ORI-containing liposomal microbubbles (LUMO) with folic acid-coupled heme-loaded multi-walled carbon nanotubes (FMTP-LUMO). This dual-targeting platform improved the therapeutic effect in liver cancer, particularly when combined with chemo-sonodynamic therapy, where the tumor inhibition rate surpassed 90%, significantly higher than that of FMTP (42.8%) and LUMO (32.5%) alone (Wang et al., 2019).
Recent studies suggest that anisodamine-calcium lipid phosphate NPs (AS-LCPS) hold promise as an effective carrier for ORI, showing excellent stability and sustained release properties in lung cancer cells. In vivo experiments revealed that surface modifications on AS-ORI LCPs enhanced cellular endocytosis, leading to increased intracellular drug accumulation (Shen and Ma, 2022).
Traditional liposomes often lack tumor-specific targeting and sustained-release capabilities, which can limit their ability to significantly improve bioavailability. To address this, researchers have developed various modified liposome formulations. Ethanol and polyethylene glycol-optimized liposomes, for instance, have shown improved tumor cell affinity and enhanced anticancer activity for ORI (Ren et al., 2021). Moreover, complex liposomes targeting tumor cells have been designed to further increase bioavailability, enhance anticancer efficacy, reduce off-target effects, and induce persistent immune memory while curbing metastasis (Lin et al., 2023; Xin et al., 2023a). Combining liposome carriers with sonodynamic and thermal therapies has also yielded promising anticancer results (Xin et al., 2023b).
4.2 Micelle
Micelles, synthesized from amphiphilic surfactants in aqueous environments, possess the remarkable ability to self-assemble into nanoscale structures, a key property that makes them ideal drug delivery vehicles (Ghosh and Biswas, 2021). When surfactant concentrations surpass the critical micelle concentration, these molecules organize into nanostructures known as micelles, which are effective carriers for hydrophobic drugs with low water solubility, such as ORI (Del Regno et al., 2021; Guerrero-Hernández et al., 2022).
Research indicates that oral drug delivery can improve patient compliance compared to injectable methods. Among various oral preparation techniques, hybrid micelle systems have emerged as one of the most effective for enhancing drug delivery. Due to their core-shell structure, these systems significantly increase the solubility of hydrophobic drugs (Chen et al., 2015). For instance, a nanohybrid micellar system, ORN-M, consisting of Soluplus® (SOL) and Pluronic P105, was developed for the oral delivery of ORI. ORN-M exhibited a long-acting, sustained-release profile in vitro, showing stronger inhibition of tumor cell growth. Its anticancer efficacy was further enhanced by improved in vivo solubility, permeability, and greater resistance to drug efflux (Ke et al., 2017) (Figure 6).
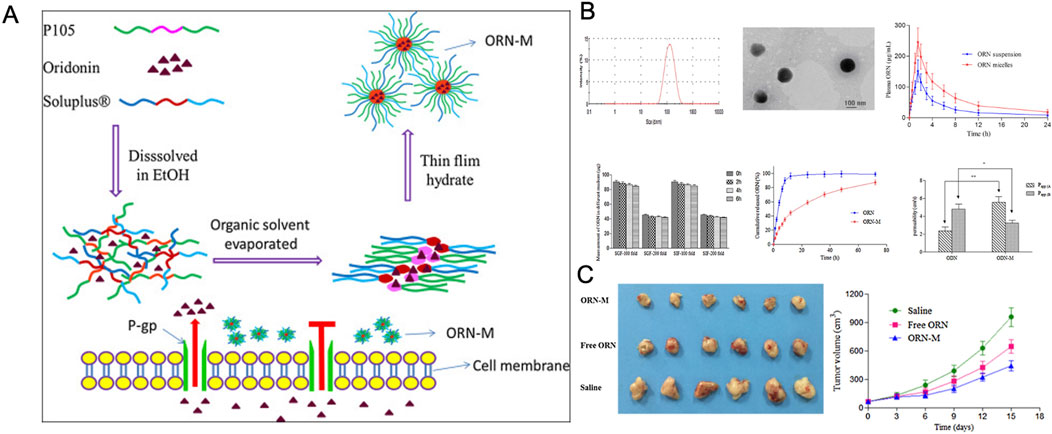
Figure 6. (A) Schematic illustration of ORN-loaded mixed micelles. (B) Characterization of ORN-suspension and ORN-M materials. (C) Tumor inhibition effects after treatment in each group. Reproduced with permission from Ref (Ke et al., 2017). Copyright 2016, Elsevier.
In another study, monomethoxy poly (ethylene glycol)-poly (epsilon-caprolactone) (MPEG-PCL) was employed as a drug carrier to enhance ORI’s solubility. ORI-loaded MPEG-PCL micelles, prepared using the thin-film hydration method, successfully encapsulated ORI while preserving its anticancer properties. These micelles released ORI in a sustained manner in vitro, prolonging its therapeutic action (Xue et al., 2012).
Targeting hepatocellular carcinoma (HCC), Fang et al. designed a hybrid micelle system modified by the peptide Ala-Pro-Asp-Thr-Lys-Thr-Gln (APDTKTQ), aimed at the receptor for advanced glycation end products (RAGE), which is overexpressed in HCC. The ORI-loaded micelles showed improved cellular uptake and induced more apoptosis compared to free ORI micelles, with a uniform spherical shape and no aggregation (Fang et al., 2016).
Xu et al. (2021) synthesized redox-sensitive ORI polymer prodrug formulations, which self-assembled into micelles (P-ss-ORI) via covalent linkage of ORI to polyethylene glycol block polylysine with a disulfide linker. These micelles exhibited small size and high drug-loading efficiency. In gastric cancer treatment, under conditions of high intracellular GSH and low pH, P-ss-ORI demonstrated prolonged ORI retention, tumor tissue accumulation, efficient endocytosis by cancer cells, and rapid, complete drug release (Xu et al., 2021).
Another innovative formulation involved coupling D-α-tocopherol polyethylene glycol succinate (TPGS) with poly (lactic-co-glycolic acid) (PLGA) via a disulfide linker (TPGS-S-S-PLGA) to form ORI micelles. In HCC cells, this design increased cellular uptake and apoptosis induction (Fang et al., 2016). These studies collectively highlight the potential of micelles and their modified versions to enhance anticancer activity, in vivo solubility, permeability, and resistance to drug efflux.
4.3 Metal-organic framework (MOF)
MOFs represent an emerging class of hybrid porous materials, composed of metal ions or clusters connected by organic linkers (Giliopoulos et al., 2020), which offer significant potential as drug delivery carriers due to their highly tunable structure, pore size, versatile functionality, and improved biocompatibility (Wu and Yang, 2017).
Among the MOF family, MOF-5 (also known as IRMOF-1) is one of the most well-studied examples, featuring a three-dimensional framework made from terephthalic acid and Zn4O metal clusters (Karimzadeh et al., 2019). MOF-5 is particularly noted for its open skeleton structure, controlled pore size and surface area, and high thermal stability, making it an attractive candidate for medical applications (Javanbakht et al., 2020). Chen et al. utilized direct addition synthesis to produce MOF-5 and solvent adsorption techniques to load ORI (ORI@MOF-5). The ORI@MOF-5 system demonstrated sustained release properties under various pH conditions, along with good biocompatibility and biodegradability. This sustained-release functionality helps to minimize drug toxicity and side effects, making it a promising candidate for anticancer therapy (Chen et al., 2019). Additionally, efforts have been made to modify MOFs with substituents to improve ORI delivery by personalizing DDS and tailoring the physicochemical properties of MOFs to meet specific drug-targeting needs (Cai et al., 2020) (Figure 7).
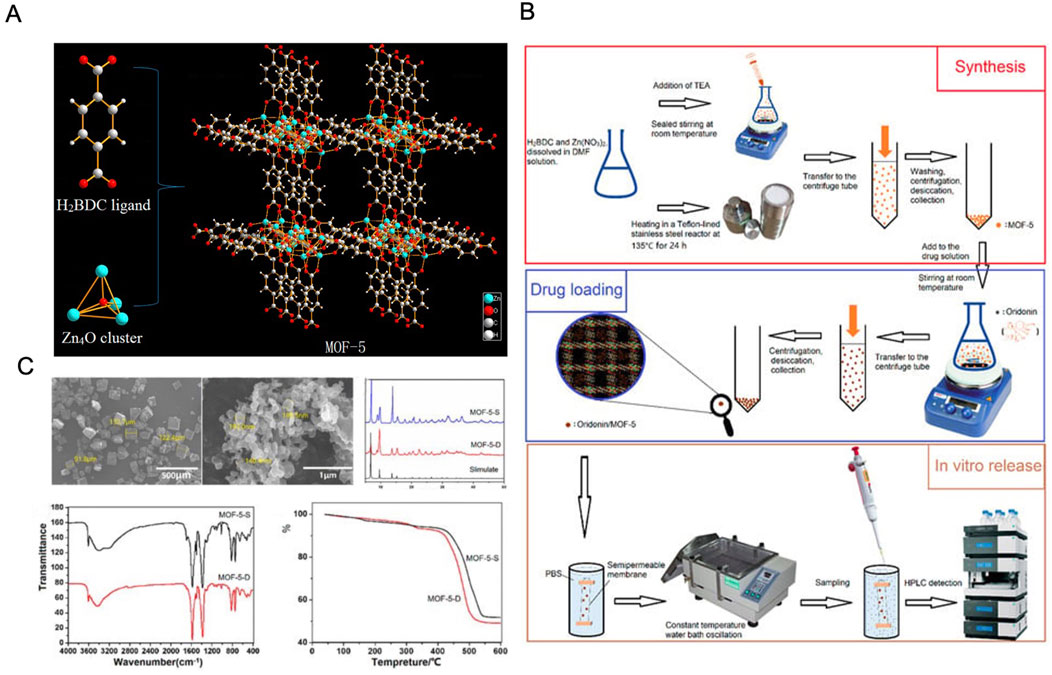
Figure 7. (A) Schematic illustration of the MOF-5 construction. (B) Schematic diagram of the MOF-5 preparation process. (C) Characterization of the MOF-5. Reproduced with permission from Ref (Chen et al., 2019). Copyright 2019, MDPI.
Sustained-release formulations remain a key focus in cancer drug research. Iron carboxylate MOFs, composed of iron ions and terephthalic acid, exhibit non-toxicity and biocompatibility. Leng et al. (2018) synthesized a flexible porous MOF as a carrier for ORI, confirming its high drug-loading capacity, favorable biocompatibility, and excellent sustained-release characteristics. These findings underscore the potential of MOF-based delivery systems to enhance drug loading efficiency and biocompatibility, making them promising candidates for the development of sustained-release agents to bolster the anticancer activity and therapeutic efficacy of ORI (Cai et al., 2022; Shen et al., 2024).
4.4 Microemulsions
Melf-microemulsifying drug delivery systems (SMEDDS) are isotropic mixtures composed of oil, surfactants, co-surfactants, and drug compounds (Dokania and Joshi, 2015). These systems enhance the solubility of poorly water-soluble drugs, thereby improving their absorption through oral administration (Kovačević et al., 2022).
Zhang et al. (2008) developed an ORI-based oral microemulsion using Maisine 35-1 and Labrafac CC, which, compared to a suspension, significantly enhanced the drug’s bioavailability, indicating its potential for oral use. The performance of SMEDDS is influenced by the ratio of its components and the drug concentration. To address this, Zhang et al. optimized the formulation by identifying the ideal ratio of microemulsifiers, leading to improved intestinal absorption and rapid drug release characteristics. Additionally, SMEDDS, as a lipid-based delivery system, facilitates partial absorption through the lymphatic pathway, which may bypass first-pass hepatic metabolism and further enhance bioavailability (Figure 8).
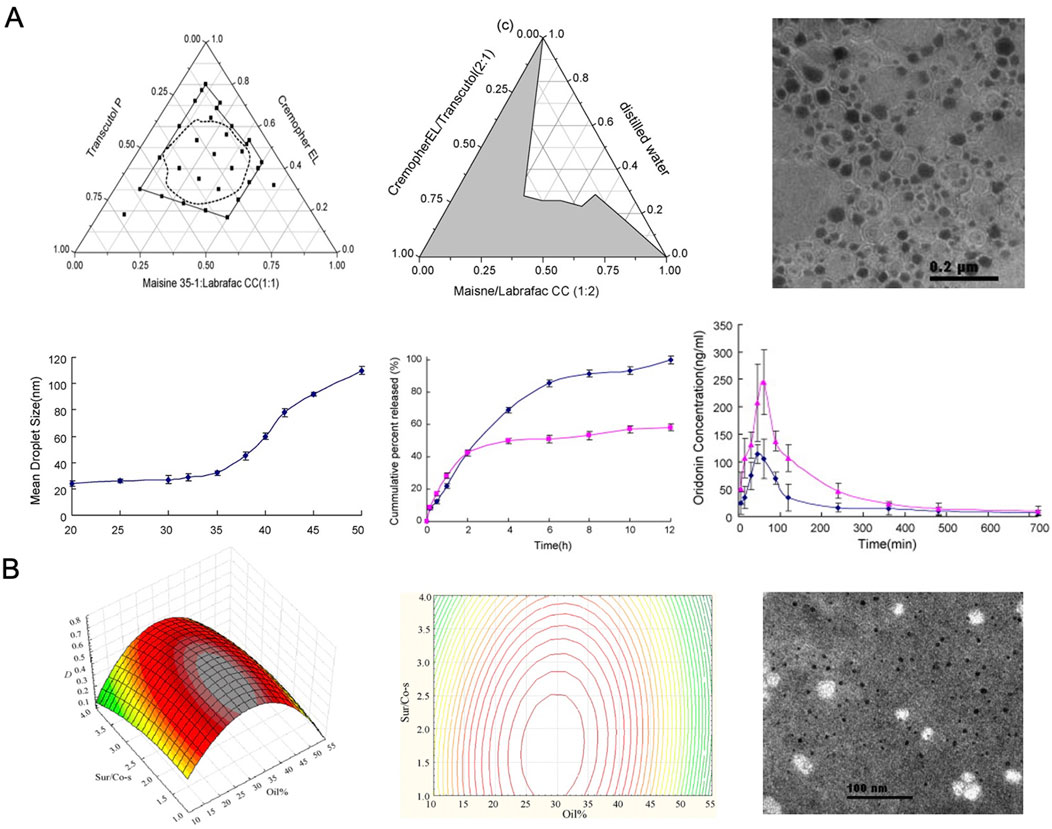
Figure 8. (A) Spherical microemulsion droplets. Reproduced with permission from Ref (Zhang et al., 2008). Copyright 2008, Elsevier. (B) Optimized SMEDDS formulation. Reproduced with permission from Ref (Liu et al., 2009). Copyright 2009, Elsevier.
4.5 Nano suspension
Nano suspensions represent a carrier-free colloidal drug delivery system, consisting primarily of pure drug NPs smaller than 100 nm, stabilized with minimal surfactant (Kovalchuk et al., 2019). These systems offer versatility in administration, supporting oral, intravenous, and parenteral delivery routes (Gao et al., 2008). One example is the hydroxypropyl-β-cyclodextrin (HP-β-CD) inclusion complex of ORI, which was used to create nanosuspension solutions for oral administration. The inclusion of HP-β-CD significantly enhanced intestinal solubility, effective permeability, and bioavailability (Zhang et al., 2016).
Both in vitro and in vivo studies have demonstrated the superior antitumor effects of ORI formulated as a nanosuspension compared to its free drug form. In vitro, the nanosuspension displayed greater cytotoxicity and a higher rate of apoptosis induction. In vivo studies in mice showed that ORI nanosuspensions were better tolerated, offering enhanced anti-tumor efficacy with reduced toxicity (Lou et al., 2009; Feng et al., 2011). Specifically, ORI nanosuspension exhibited stronger cytotoxicity against human pancreatic cancer PANC-1 cells (Qi et al., 2012), and its formulation significantly boosted the anti-tumor activity in human breast cancer MCF-7 cells, increasing both cell cycle arrest and apoptosis (Feng et al., 2011) (Figure 9).
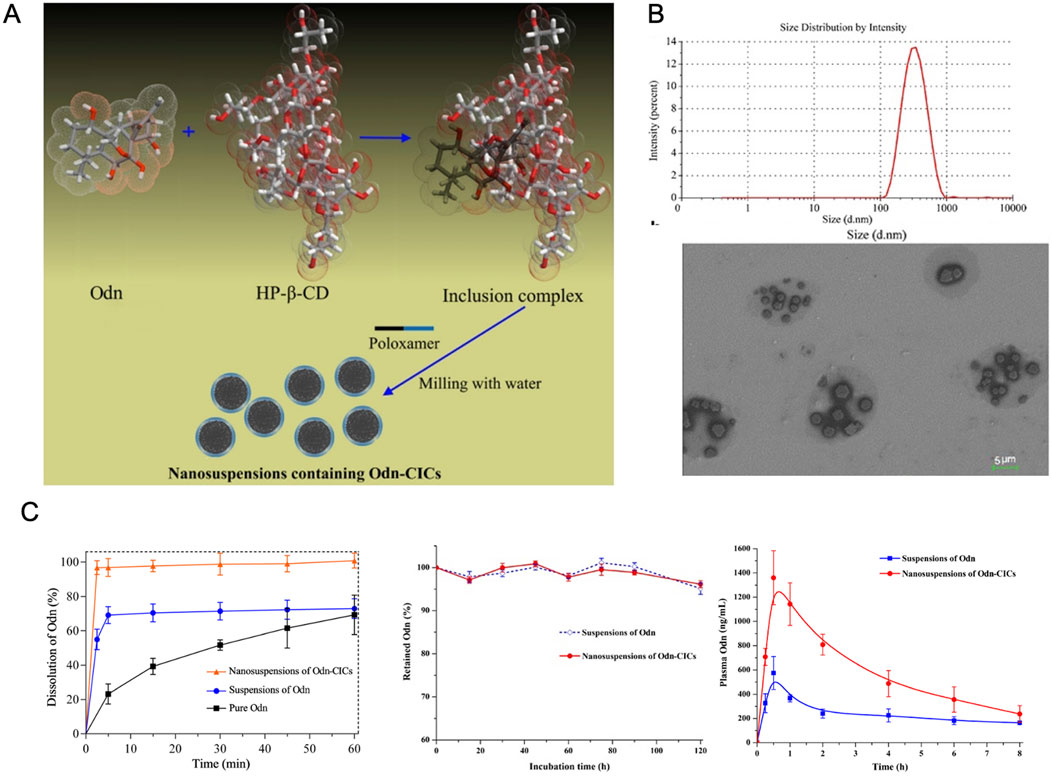
Figure 9. (A) Illustrations of the inclusion and preparation processes of ORI/HP-β-CD. (B) Characterization of HP-β-CD. (C) Pharmacokinetics of different drug delivery systems. Reproduced with permission from Ref (Zhang et al., 2016). Copyright 2010, Taylor & Francis.
Further in vitro studies on human prostate cancer PC-3 cells revealed that ORI nanosuspensions provided more pronounced inhibition of cell proliferation, significantly enhancing growth inhibition and apoptosis induction (Zhang et al., 2010). Lou et al. (2011) confirmed that ORI nanosuspension also demonstrated superior antitumor activity in H22 tumor-bearing mice while reducing toxicity. Additionally, it has been shown that particle size significantly affects the pharmacokinetics and tissue distribution of ORI nanosuspensions following intravenous administration. Optimizing particle size and developing high-bioavailability oral formulations could make ORI nanosuspensions a more viable candidate for clinical use (Gao et al., 2007).
4.6 Protein-based nanocarriers
Among functional nanomaterials, proteins are considered highly effective drug carriers due to their excellent adsorption properties, non-toxicity, non-immunogenicity, and favorable in vivo stability. Bovine serum albumin (BSA) NPs, in particular, have emerged as a promising drug delivery platform (Wang and Zhang, 2018; He et al., 2023). These macromolecular proteins protect active drug substances from hydrolytic degradation, reduce cardiotoxicity, enhance water solubility and biocompatibility, prolong blood circulation time, and minimize toxic side effects (Teran-Saavedra et al., 2019; Wang and Zhang, 2018). A BSA-based ORI-loaded galactosylated BSA nanoparticle system (ORI-GB-NP) has been developed for the liver-targeted delivery of ORI (Li et al., 2013). Parenteral delivery experiments revealed that ORI-GB-NP increased plasma drug levels, extended circulation time, and enhanced liver delivery while reducing toxicity in the heart, lungs, and kidneys (Li et al., 2014).
Additionally, wheat germ lectin-modified lipid-polymer hybrid NPs (WGA-LPNs) possess receptor-mediated endocytosis and bioadhesive properties, promoting cellular uptake after oral administration (Liu et al., 2011). ORI-loaded WGA-LPNs exhibited significantly greater extracellular uptake and intestinal diffusion than the control group, leading to a 1.96-fold increase in oral bioavailability and pronounced induction of cell apoptosis (Figure 10) (Liu et al., 2017). NPs, due to their unique physical and chemical properties, inherently possess high surface free energy, which makes them unstable. However, stability can be achieved by binding NPs to biomolecules such as proteins, which helps reduce free surface energy. Albumin-based NPs have demonstrated the ability to enhance plasma drug concentration, improve solubility, achieve predictable biodistribution, ensure biocompatibility, and effectively bind to a range of therapeutic agents (Mariam et al., 2016).
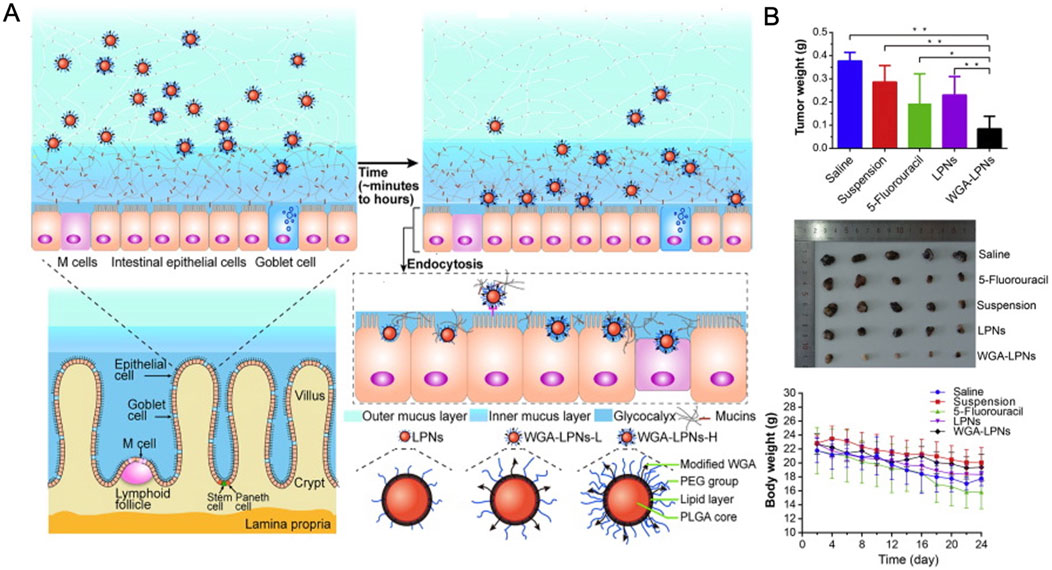
Figure 10. (A) Preparation and mechanism of WGA-LPNs. (B) Tumor inhibition effects after treatment in each group. Reproduced with permission from Ref (Liu et al., 2017). Copyright 2017, Elsevier.
4.7 Polymer NPs
Polymeric NPs offer chemical diversity and the potential for long-term and targeted drug delivery in vivo (Ferreira Soares et al., 2020). Sun et al. (2024) developed a liver-targeted ORI delivery system using Angelica sinensis polysaccharide (ASP) as the carrier and formulated ORI-loaded ASP-deoxycholic acid (DOCA) NPs (ORI/ASP-DOCA NPs). This system achieved high drug loading and utilized asialoglycoprotein receptor-mediated targeting in H22 tumor-bearing mice, resulting in significant anticancer effects while minimizing systemic toxicity (Sun et al., 2024).
In another study, ORI-loaded random poly (D, L-lactic acid) NPs (ORI-PLA-NPs) were prepared via a modified spontaneous emulsification solvent diffusion method. The pharmacokinetics, tissue distribution, and anticancer activity of these NPs were evaluated in mice with H22-derived tumors. The results showed that ORI-PLA-RGD-NPs exhibited superior tumor targeting and anti-tumor efficacy compared to ORI-PLA-NPs or ORI solution, with a higher accumulation of ORI in tumor tissues (Xu et al., 2012) (Figure 11).
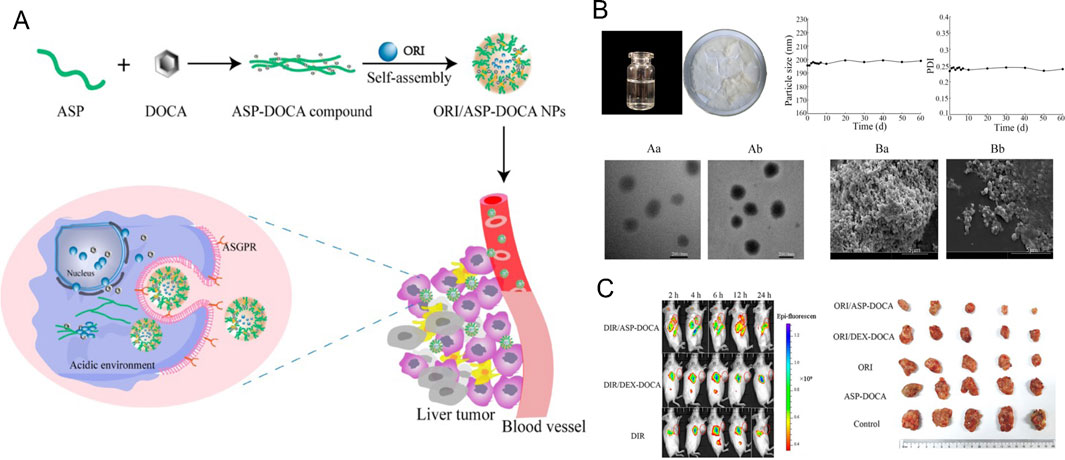
Figure 11. (A) Schematic design of ORI/ASP-DOCA NPs. (B) Characterization of ORI/ASP-DOCA NPs. (C) Tumor inhibition effects after treatment in each group. Reproduced with permission from Ref (Zhang et al., 2016). Copyright 2024, MDPI.
Zheng et al. (2012b) also developed galactose-chitosan-coated ORI-loaded NPs (ORI-GC-NP) for targeted cancer therapy. These NPs exhibited pH-dependent release behavior in acidic environments, enhancing ORI release specifically within the acidic tumor microenvironment. ORI-GC-NP demonstrated strong liver-targeting capabilities and prolonged plasma retention, making it a promising strategy for liver cancer treatment (Zheng et al., 2012b). Various polymers have been explored for developing ORI delivery systems, with some exhibiting excellent biocompatibility and tumor-targeting properties, while others have shown enhanced tumor-specific release, improved solubility, and extended circulation time. Polymeric NPs are proving to be an especially promising carrier for ORI.
5 Advantages of nano-delivery systems
5.1 Pharmacokinetic properties
The study of drug pharmacokinetics, encompassing absorption, distribution, metabolism, and excretion, plays a critical role in minimizing adverse reactions and determining appropriate dosages. It also aids in the design of dosage regimens and optimization of clinical efficacy (Glassman and Muzykantov, 2019).
Pharmacokinetic analysis has become a key component of both preclinical and clinical drug development (Miller et al., 2019). For example, in studies assessing ORI in rat plasma after intragastric administration, the liver was identified as the primary site of metabolism. Verapamil, known to inhibit P-glycoprotein (P-gp) activity, has been shown to significantly affect the pharmacokinetic profile of ORI, leading to increased peak plasma concentration and bioavailability by enhancing its absorption (Liu et al., 2019).
A notable case is the ORI/2-hydroxypropyl-β-cyclodextrin inclusion complex nanosuspension, which substantially improved relative bioavailability, highlighting the potential of nanosuspensions prepared from inclusion complexes for oral anticancer drugs. In another study, Li et al. (2011b) developed a solid dispersion using gas antisolvent technology, where ethanol served as the solvent, CO2 as the antisolvent, and polyvinylpyrrolidone K17 as the carrier matrix. This solid dispersion formulation resulted in a 26.4-fold increase in ORI bioavailability (Li et al., 2011b).
Lin et al. (2023) developed and validated a highly sensitive HPLC method with UV detection for quantifying ORI in rat plasma. ORI liposome administration led to a significant extension of the elimination half-life and an increase in the AUC, along with a reduction in the clearance rate (Lin et al., 2023). Nanocarriers play a vital role in improving drug bioavailability and prolonging half-life. Understanding the alterations in pharmacokinetics and conducting thorough pharmacokinetic studies are crucial for elucidating the biological functions and mechanisms of these carriers in vivo. Optimizing pharmacokinetic properties is essential for the successful application of nanomedicine in cancer therapy.
5.2 Enhanced tumor inhibition
The type and proportion of ligands on the surface of NPs, along with the particle size of the delivery system, can significantly influence the circulation, cellular uptake, and drug delivery profile of NPs. These factors play a key role in promoting cellular endocytosis and modulating the rate of drug delivery, which can impact the penetration and uptake of ORI within the body (Danaei et al., 2018).
A reduction in ORI’s bioavailability may limit its anticancer efficacy in vivo, as its poor solubility presents a major challenge in clinical applications (Fan et al., 2018). Therefore, enhancing ORI bioavailability, particularly for oral DDS, is critical, given the barriers posed by the gastrointestinal tract (Parodi et al., 2021). Studies have shown that PEG-modified nanocarriers can improve drug permeability, thus enhancing bioavailability and boosting the anticancer potency of drugs like ORI (Ren et al., 2021; Fang et al., 2016; Chai et al., 2019).
Particle size is another important factor, affecting both intravenous administration and oral absorption of ORI. While reducing particle size can improve tissue permeability, it may also compromise stability, necessitating careful selection of an appropriate particle size to optimize the delivery effect of each DDS (Danaei et al., 2018).
Long-circulating drug carriers are particularly advantageous in DDS, as prolonged circulation can increase drug accumulation at the target site, while overly rapid clearance may prevent drugs from achieving their full therapeutic potential (Ou et al., 2018). For instance, nanostructured lipid carriers loaded with ORI have demonstrated extended circulation times (Zheng et al., 2012a), and stealth liposomes have been shown to significantly lengthen blood circulation times in pharmacokinetic studies (Wang et al., 2009) (Li et al., 2014). Achieving the right balance between circulation time and stability is therefore essential for maximizing the therapeutic efficacy of ORI in cancer treatment.
5.3 Reduce systemic toxicity
A significant challenge in oncology is managing the adverse drug reactions (ADRs) associated with anticancer agents, which can severely impact patient safety and quality of life (Mhandire and Goey, 2022). Reducing drug toxicity while improving safety are critical factors for the successful development of anticancer therapies. The use of protein-based nanocarriers has proven effective in decreasing toxicity and the incidence of adverse effects linked to pharmaceutical agents. Protein modifications, in particular, can help mitigate ORI’s toxicity to organs such as the heart, lungs, and kidneys (Spada et al., 2021; Haddadzadegan et al., 2022). In vitro studies of ORI release have revealed a biphasic drug release profile, with an initial burst followed by sustained release.
Active targeting, which employs ligand-modified carriers to deliver drugs directly to specific targets, holds promise for enhancing drug accumulation at the site of action while reducing systemic toxicity, offering a substantial improvement over passive targeting (Tang et al., 2021; Bandyopadhyay et al., 2023). For instance, folate receptor (FR)-targeting ORI-loaded liposome microbubbles (F-LMB-ORI) demonstrated a higher binding affinity to HepG-2 cells, significantly improving drug efficacy (Wang et al., 2017).
In summary, the ORI delivery systems reviewed in this paper enhance anticancer activity by prolonging circulation time, improving tumor targeting, enhancing solubility and bioavailability, optimizing particle size, and reducing toxicity.
6 Market application and clinical trials
ORI has shown notable clinical applicability and the potential for further therapeutic development. Several ORI-based formulations have already received approval and are available for treating various inflammatory conditions, such as tonsillitis, pharyngitis, laryngitis, and stomatitis (Wu et al., 2024). For example, ORI tablets and ORI drop pills are recommended as adjuvant cancer therapies (Table 3). Hu et al. developed a series of enmein-type diterpenoid amino acid ester derivatives, including L-alanine-(14-ORI) trifluoroacetate, one of which (Compound 19) exhibited potent cytotoxicity against both HCC Bel-7402 and chronic myelogenous leukemia K562 cells, while demonstrating some selectivity (Hu et al., 2019). Hengrui Medicine Co., a Chinese pharmaceutical company, conducted the first human clinical trial (CTR20150246) of HAO472, an L-alanine-(14-ORI) ester trifluoroacetate, as a potential treatment for acute myelogenous leukemia (Hu et al., 2020). Additionally, Rabdosia rubescens Drops are currently being evaluated in a phase IV clinical study (ChiCTR1800015210) for their efficacy and safety in preventing and treating radiological oral mucositis in patients with nasopharyngeal carcinoma, as registered in the China Clinical Trials Registry (Table 4).
At present, most ORI-based drug formulations available on the market consist of conventional tablets, drops, and capsules, while no ORI-based nanomedicines have been marketed to date. This suggests that ORI nanodrug development is still largely in the animal testing phase, indicating that widespread clinical application remains some distance away. Furthermore, most marketed drugs and clinical trials are based in China, the origin of traditional Chinese medicine. Expanding ORI’s therapeutic indications and promoting its global usage are critical areas for future research.
7 Conclusion and future considerations
The ideal DDS maintains drug concentrations within the therapeutic range or targets delivery specifically to affected organs and tissues while minimizing exposure to other regions, thereby reducing systemic toxicity. Despite ORI’s well-documented anticancer efficacy (Tarantino et al., 2023), its clinical use is limited by poor solubility and bioavailability (Unsoy and Gunduz, 2018). Overall, the continuous development of ORI-based DDSs provides promising avenues for cancer therapy, though certain limitations remain, accompanied by specific drawbacks and challenges. Some nanocarriers exhibit incomplete biodegradability, which may lead to liver or kidney toxicity, while the precise targets of some carriers remain unclear, and research on the accurate tissue and organ distribution of ORI is still limited (Ding et al., 2016).
Specifically, ORI liposomal DDSs may degrade in vivo due to lipid oxidation or hydrolysis, leading to premature drug release and thus compromising therapeutic efficacy. Their stability issues also pose challenges for storage and transportation (Liu et al., 2024b). ORI micellar DDSs, often composed of polymers and surfactants, are not sufficiently stable under physiological conditions. In particular, micellar particles may disassemble in the bloodstream, resulting in premature drug release and reduced targeting efficiency. Several MOF DDSs have been employed in previous studies on ORI nanotechnology, but these have primarily served as frameworks for ORI delivery to enhance its stability, with the MOFs themselves did not exhibit significant antitumor effects (Chen et al., 2019). ORI suspensions may aggregate during storage or transport, leading to increased particle size, which can affect the uniformity and stability of drug distribution. Proteins used to deliver ORI, as natural biomolecules, are susceptible to enzymatic degradation, thermal denaturation, or alterations in pH within the body, potentially causing rapid DDS degradation and premature drug release, thereby diminishing therapeutic efficacy (Teran-Saavedra et al., 2019). While the tumor microenvironment is generally acidic, not all tumor sites maintain a consistently low pH. Even if certain ORI DDSs show favorable release profiles in vitro under acidic conditions, it cannot be guaranteed that they will function effectively in all tumors, especially in those with high heterogeneity (Shen et al., 2024).Although targeted ORI DDSs demonstrate high selectivity, in the complex tumor microenvironment, targeting molecules may sometimes fail to bind precisely to the target receptors, particularly in tumors with heterogeneous expression of these molecules (Sun et al., 2024).
While several ORI-based drugs have been introduced to the market, their range of indications is relatively narrow, focusing primarily on common inflammatory diseases and serving as adjunctive therapies for cancer (Liu et al., 2020; Yan et al., 2020). Current formulations, such as tablets and capsules, are conventional and lack innovative delivery methods. This limited variety in formulation design not only constrains the broader clinical applicability of ORI but may also impact its bioavailability and patient compliance. The absence of diverse and advanced DDSs restricts ORI’s therapeutic potential, particularly when addressing complex diseases or conditions involving multiple complications. Moreover, ORI-based nano-drugs are still in the experimental stage and have not yet been commercialized, preventing the full clinical translation of their potential therapeutic benefits (Hu et al., 2020). Although laboratory studies indicate that ORI nano-drugs exhibit excellent efficacy and targeting capabilities, the lack of large-scale clinical trials leaves their safety and effectiveness unproven.
Future research on ORI should emphasize several critical aspects to advance its role in drug development. Although nano-drug formulations offer significant potential, addressing toxicity and safety remains paramount. The use of biocompatible delivery materials, such as polymer NPs and liposomes, is recommended to mitigate potential side effects. Current studies largely focus on ORI, yet Rabdosia rubescens contains multiple active constituents. A broader investigation into these components and their synergistic effects is warranted (Liu et al., 2020; Yan et al., 2020). Combining ORI with other extracts to develop composite nano-drug systems could further enhance therapeutic efficacy and expand its clinical applications. Additionally, beyond cancer treatment, the potential of ORI in managing cancer-related symptoms like pain, nausea, and fatigue should be explored. Conducting clinical studies to investigate ORI’s efficacy in alleviating these symptoms could expand its systemic utility and increase its market potential.
With the rapid advancements in nanotechnology, researchers should explore and develop more sophisticated and rational novel nanodrug delivery systems, such as targeted delivery technologies that promote the accumulation of ORI nano-drugs in specific tissues. This would improve therapeutic outcomes. Furthermore, smart drug delivery systems capable of precise, controlled drug release may unlock new possibilities for ORI’s clinical use. Certain noble metals (Au NPs, Ag NPs, and Pt NPs) (Qin et al., 2022), and non-noble metals (Cu NPs and Mg NPs) exhibit strong antitumor effects (Zhao et al., 2022a), functioning both as drug carriers and tumor inhibitors (Chen et al., 2024b). Their combination with ORI DDS may lead to better efficacy and present the possibility of combination with photothermal therapy. Multi-strategy combination therapy is an inevitable direction for future clinical practice (Chen et al., 2021; Zhang et al., 2024), and the existing sonodynamic DDS and chemodynamic DDS are far from sufficient to meet clinical needs. There is a necessity to further improve carrier design and adopt rational research strategies, aiming to develop more DDS based on PTT (Zhao et al., 2022b), electrodynamic therapy (Qiao et al., 2022), radiodynamic therapy (Matsuyama et al., 2022), magnetic hyperthermia (Vangijzegem et al., 2023), immunotherapy (Lu et al., 2024), and gene nanotherapy (Liu et al., 2024c). Given that ORI research spans multiple disciplines, including pharmacology, materials science, and clinical medicine, interdisciplinary collaboration is essential for advancing ORI-based drug development. Collaborations with biomedical engineers to design innovative nano-carriers and partnerships with clinical researchers for multi-center trials could accelerate progress in this area.
While ORI faces certain challenges in drug development, its therapeutic potential remains significant. Addressing toxicity, safety, active component analysis, expanding indications, and fostering technological innovation will likely solidify ORI’s role in modern medicine. As research progresses and technology evolves, ORI-based nano-drugs could offer substantial benefits to a broader range of patients, contributing to the modernization of traditional Chinese medicine.
Author contributions
YS: Visualization, Writing–original draft, Conceptualization, Formal Analysis, Methodology. LL: Writing–review and editing. CL: Writing–original draft. DD: Formal Analysis, Writing–original draft. YL: Writing–original draft. MH: Writing–original draft. YW: Writing–original draft. KL: Writing–original draft. HW: Writing–original draft. QC: Writing–original draft. GH: Writing–review and editing.
Funding
The author(s) declare financial support was received for the research, authorship, and/or publication of this article. This study was supported by the the Shenzhen Key Medical Discipline (No. SZXK021, China), the Sanming Project of Medicine in Shenzhen (No. SASM202201024, China) and 2023 College Students Innovation and Entrepreneurship Training Project of SUMC(S202310560147).
Acknowledgments
We thank Bullet Edits Limited for the linguistic editing and proofreading of the manuscript.
Conflict of interest
The authors declare that the research was conducted in the absence of any commercial or financial relationships that could be construed as a potential conflict of interest.
Publisher’s note
All claims expressed in this article are solely those of the authors and do not necessarily represent those of their affiliated organizations, or those of the publisher, the editors and the reviewers. Any product that may be evaluated in this article, or claim that may be made by its manufacturer, is not guaranteed or endorsed by the publisher.
References
Abdullah, N. A., Md Hashim, N. F., Ammar, A., and Muhamad Zakuan, N. (2021). An insight into the anti-angiogenic and anti-metastatic effects of oridonin: current knowledge and future potential. Mol. Basel, Switz. 26 (4), 775. doi:10.3390/molecules26040775
Ali, M. A., Khan, N., Ali, A., Akram, H., Zafar, N., Imran, K., et al. (2024). Oridonin from Rabdosia rubescens: an emerging potential in cancer therapy - a comprehensive review. Food Sci. & Nutr. 12 (5), 3046–3067. doi:10.1002/fsn3.3986
An, Y., Zhu, J., Wang, X., Sun, X., Luo, C., Zhang, Y., et al. (2022). Oridonin delays aging through the AKT signaling pathway. Front. Pharmacol. 13, 888247. doi:10.3389/fphar.2022.888247
Bandyopadhyay, A., Das, T., Nandy, S., Sahib, S., Preetam, S., Gopalakrishnan, A. V., et al. (2023). Ligand-based active targeting strategies for cancer theranostics. Naunyn-Schmiedeberg's archives Pharmacol. 396 (12), 3417–3441. doi:10.1007/s00210-023-02612-4
Barnestein, R., Galland, L., Kalfeist, L., Ghiringhelli, F., Ladoire, S., and Limagne, E. (2022). Immunosuppressive tumor microenvironment modulation by chemotherapies and targeted therapies to enhance immunotherapy effectiveness. Oncoimmunology 11 (1), 2120676. doi:10.1080/2162402X.2022.2120676
Bell, R. M. (2010). A review of complementary and alternative medicine practices among cancer survivors. Clin. J. Oncol. Nurs. 14 (3), 365–370. doi:10.1188/10.CJON.365-370
Bi, E., Liu, D., Li, Y., Mao, X., Wang, A., and Wang, J. (2018). Oridonin induces growth inhibition and apoptosis in human gastric carcinoma cells by enhancement of p53 expression and function. Braz. J. Med. Biol. Res. = Revista brasileira de pesquisas medicas e Biol. 51 (12), e7599. doi:10.1590/1414-431X20187599
Bu, H. Q., Liu, D. L., Wei, W. T., Chen, L., Huang, H., Li, Y., et al. (2014). Oridonin induces apoptosis in SW1990 pancreatic cancer cells via p53-and caspase-dependent induction of p38 MAPK. Oncol. Rep. 31 (2), 975–982. doi:10.3892/or.2013.2888
Bu, H. Q., Shen, F., and Cui, J. (2019). The inhibitory effect of oridonin on colon cancer was mediated by deactivation of TGF-β1/Smads-PAI-1 signaling pathway in vitro and vivo. OncoTargets Ther. 12, 7467–7476. doi:10.2147/OTT.S220401
Bury, M., Le Calvé, B., Ferbeyre, G., Blank, V., and Lessard, F. (2021). New insights into CDK regulators: novel opportunities for cancer therapy. Trends cell Biol. 31 (5), 331–344. doi:10.1016/j.tcb.2021.01.010
Cai, M., Fu, T., Zhu, R., Hu, P., Kong, J., Liao, S., et al. (2024). An iron-based metal-organic framework nanoplatform for enhanced ferroptosis and oridonin delivery as a comprehensive antitumor strategy. Acta Pharm. Sin. B 14 (9), 4073–4086. doi:10.1016/j.apsb.2024.05.015
Cai, M., Liang, W., Wang, K., Yin, D., Fu, T., Zhu, R., et al. (2022). Aperture modulation of isoreticular metal organic frameworks for targeted antitumor drug delivery. ACS Appl. Mater. & interfaces 14 (32), 36366–36378. doi:10.1021/acsami.2c07450
Cai, M., Qin, L., You, L., Yao, Y., Wu, H., Zhang, Z., et al. (2020). Functionalization of MOF-5 with mono-substituents: effects on drug delivery behavior. RSC Adv. 10 (60), 36862–36872. doi:10.1039/d0ra06106a
Chai, D., Hao, B., Hu, R., Zhang, F., Yan, J., Sun, Y., et al. (2019). Delivery of oridonin and methotrexate via PEGylated graphene oxide. ACS Appl. Mater. & interfaces 11 (26), 22915–22924. doi:10.1021/acsami.9b03983
Che, X., Zhan, J., Zhao, F., Zhong, Z., Chen, M., Han, R., et al. (2021). Oridonin promotes apoptosis and restrains the viability and migration of bladder cancer by impeding TRPM7 expression via the ERK and AKT signaling pathways. BioMed Res. Int. 2021, 4340950. doi:10.1155/2021/4340950
Chelakkot, C., Chelakkot, V. S., Shin, Y., and Song, K. (2023). Modulating glycolysis to improve cancer therapy. Int. J. Mol. Sci. 24 (3), 2606. doi:10.3390/ijms24032606
Chen, F., Liao, J., Wu, P., Cheng, L., Ma, Y., Zhang, L., et al. (2023b). Oridonin inhibits the occurrence and development of colorectal cancer by reversing the Warburg effect via reducing PKM2 dimer formation and preventing its entry into the nucleus. Eur. J. Pharmacol. 954, 175856. doi:10.1016/j.ejphar.2023.175856
Chen, G., Luo, J., Cai, M., Qin, L., Wang, Y., Gao, L., et al. (2019). Investigation of metal-organic framework-5 (MOF-5) as an antitumor drug oridonin sustained release carrier. Mol. Basel, Switz. 24 (18), 3369. doi:10.3390/molecules24183369
Chen, G., Yang, Z., Wen, D., Li, P., Xiong, Q., and Wu, C. (2023a). Oridonin inhibits Mycobacterium marinum infection-induced oxidative stress in vitro and in vivo. Pathog. Basel, Switz. 12 (6), 799. doi:10.3390/pathogens12060799
Chen, Q., Fang, C., Xia, F., Wang, Q., Li, F., and Ling, D. (2024b). Metal nanoparticles for cancer therapy: precision targeting of DNA damage. Acta Pharm. Sin. B 14 (3), 1132–1149. doi:10.1016/j.apsb.2023.08.031
Chen, T., Chu, Q., Li, M., Han, G., and Li, X. (2021). Fe(3)O(4)@Pt nanoparticles to enable combinational electrodynamic/chemodynamic therapy. J. nanobiotechnology 19 (1), 206. doi:10.1186/s12951-021-00957-7
Chen, X., Dai, X., Liu, Y., He, X., and Gong, G. (2022). Isodon rubescens (hemls.) hara.: a comprehensive review on traditional uses, phytochemistry, and pharmacological activities. Front. Pharmacol. 13, 766581. doi:10.3389/fphar.2022.766581
Chen, X., Mao, H., Peng, F., Fan, J., and Yang, F. (2024a). Novel co-delivery of oridonin and docetaxel nanoliposome for an enhanced antitumor effect on esophageal cancer. J. gene Med. 26 (8), e3725. doi:10.1002/jgm.3725
Chen, Y., Zhang, W., Huang, Y., Gao, F., Sha, X., and Fang, X. (2015). Pluronic-based functional polymeric mixed micelles for co-delivery of doxorubicin and paclitaxel to multidrug resistant tumor. Int. J. Pharm. 488 (1-2), 44–58. doi:10.1016/j.ijpharm.2015.04.048
Danaei, M., Dehghankhold, M., Ataei, S., Hasanzadeh Davarani, F., Javanmard, R., Dokhani, A., et al. (2018). Impact of particle size and polydispersity index on the clinical applications of lipidic nanocarrier systems. Pharmaceutics 10 (2), 57. doi:10.3390/pharmaceutics10020057
Del Regno, A., Warren, P. B., Bray, D. J., and Anderson, R. L. (2021). Critical micelle concentrations in surfactant mixtures and blends by simulation. J. Phys. Chem. B 125 (22), 5983–5990. doi:10.1021/acs.jpcb.1c00893
Ding, C., Zhang, Y., Chen, H., Yang, Z., Wild, C., Ye, N., et al. (2013). Oridonin ring A-based diverse constructions of enone functionality: identification of novel dienone analogues effective for highly aggressive breast cancer by inducing apoptosis. J. Med. Chem. 56 (21), 8814–8825. doi:10.1021/jm401248x
Ding, Y., Ding, C., Ye, N., Liu, Z., Wold, E. A., Chen, H., et al. (2016). Discovery and development of natural product oridonin-inspired anticancer agents. Eur. J. Med. Chem. 122, 102–117. doi:10.1016/j.ejmech.2016.06.015
Dokania, S., and Joshi, A. K. (2015). Self-microemulsifying drug delivery system (SMEDDS)--challenges and road ahead. Drug Deliv. 22 (6), 675–690. doi:10.3109/10717544.2014.896058
Fan, M., Geng, S., Liu, Y., Wang, J., Wang, Y., Zhong, J., et al. (2018). Nanocrystal technology as a strategy to improve drug bioavailability and antitumor efficacy for the cancer treatment. Curr. Pharm. Des. 24 (21), 2416–2424. doi:10.2174/1381612824666180515154109
Fang, X. B., Xu, Y. Q., Chan, H. F., Wang, C. M., Zheng, Q., Xiao, F., et al. (2016). A redox-sensitive and RAGE-targeting nanocarrier for hepatocellular carcinoma therapy. Mol. Pharm. 13 (11), 3613–3625. doi:10.1021/acs.molpharmaceut.6b00116
Feng, F. F., Zhang, D. R., Tian, K. L., Lou, H. Y., Qi, X. L., Wang, Y. C., et al. (2011). Growth inhibition and induction of apoptosis in MCF-7 breast cancer cells by oridonin nanosuspension. Drug Deliv. 18 (4), 265–271. doi:10.3109/10717544.2010.536271
Feng, N., Wu, P., Li, Q., Mei, Y., Shi, S., Yu, J., et al. (2008). Oridonin-loaded poly(epsilon-caprolactone)-poly(ethylene oxide)-poly(epsilon-caprolactone) copolymer nanoparticles: preparation, characterization, and antitumor activity on mice with transplanted hepatoma. J. drug Target. 16 (6), 479–485. doi:10.1080/10611860802200938
Ferreira Soares, D. C., Domingues, S. C., Viana, D. B., and Tebaldi, M. L. (2020). Polymer-hybrid nanoparticles: current advances in biomedical applications. Biomed. & Pharmacother. = Biomedecine & Pharmacother. 131, 110695. doi:10.1016/j.biopha.2020.110695
Gao, C., Zhang, L., Tang, Z., Fang, Z., Ye, X., and Yu, W. (2021). Preparation, characterization, and anti-colon cancer activity of oridonin-loaded long-circulating liposomes. Pharm. Dev. Technol. 26 (10), 1073–1078. doi:10.1080/10837450.2021.1982966
Gao, F. H., Hu, X. H., Li, W., Liu, H., Zhang, Y. J., Guo, Z. Y., et al. (2010). Oridonin induces apoptosis and senescence in colorectal cancer cells by increasing histone hyperacetylation and regulation of p16, p21, p27 and c-myc. BMC cancer 10 (4), 610. doi:10.1186/1471-2407-10-610
Gao, F. H., Liu, F., Wei, W., Liu, L. B., Xu, M. H., Guo, Z. Y., et al. (2012). Oridonin induces apoptosis and senescence by increasing hydrogen peroxide and glutathione depletion in colorectal cancer cells. Int. J. Mol. Med. 29 (4), 649–655. doi:10.3892/ijmm.2012.895
Gao, J., Li, C., Wang, X., Sun, X., Zhang, R., Chen, C., et al. (2022). Oridonin attenuates lung inflammation and fibrosis in silicosis via covalent targeting iNOS. Biomed. & Pharmacother. = Biomedecine & Pharmacother. 153, 113532. doi:10.1016/j.biopha.2022.113532
Gao, L., Zhang, D., Chen, M., Duan, C., Dai, W., Jia, L., et al. (2008). Studies on pharmacokinetics and tissue distribution of oridonin nanosuspensions. Int. J. Pharm. 355 (1-2), 321–327. doi:10.1016/j.ijpharm.2007.12.016
Gao, L., Zhang, D., Chen, M., Zheng, T., and Wang, S. (2007). Preparation and characterization of an oridonin nanosuspension for solubility and dissolution velocity enhancement. Drug Dev. industrial Pharm. 33 (12), 1332–1339. doi:10.1080/03639040701741810
Gao, S., Tan, H., and Li, D. (2023). Oridonin suppresses gastric cancer SGC-7901 cell proliferation by targeting the TNF-alpha/androgen receptor/TGF-beta signalling pathway axis. J. Cell. Mol. Med. 27 (18), 2661–2674. doi:10.1111/jcmm.17841
Gao, S., Tan, H., Zhu, N., Gao, H., Lv, C., Gang, J., et al. (2016). Oridonin induces apoptosis through the mitochondrial pathway in human gastric cancer SGC-7901 cells. Int. J. Oncol. 48 (6), 2453–2460. doi:10.3892/ijo.2016.3479
Ghosh, B., and Biswas, S. (2021). Polymeric micelles in cancer therapy: state of the art. J. Control. release official J. Control. Release Soc. 332, 127–147. doi:10.1016/j.jconrel.2021.02.016
Giliopoulos, D., Zamboulis, A., Giannakoudakis, D., Bikiaris, D., and Triantafyllidis, K. (2020). Polymer/metal organic framework (MOF) nanocomposites for biomedical applications. Mol. Basel, Switz. 25 (1), 185. doi:10.3390/molecules25010185
Glassman, P. M., and Muzykantov, V. R. (2019). Pharmacokinetic and pharmacodynamic properties of drug delivery systems. J. Pharmacol. Exp. Ther. 370 (3), 570–580. doi:10.1124/jpet.119.257113
Guerrero-Hernández, L., Meléndez-Ortiz, H. I., Cortez-Mazatan, G. Y., Vaillant-Sánchez, S., and Peralta-Rodríguez, R. D. (2022). Gemini and bicephalous surfactants: a review on their synthesis, micelle formation, and uses. Int. J. Mol. Sci. 23 (3), 1798. doi:10.3390/ijms23031798
Gunaydin, G., Gedik, M. E., and Ayan, S. (2021). Photodynamic therapy for the treatment and diagnosis of cancer-A review of the current clinical status. Front. Chem. 9, 686303. doi:10.3389/fchem.2021.686303
Haddadzadegan, S., Dorkoosh, F., and Bernkop-Schnürch, A. (2022). Oral delivery of therapeutic peptides and proteins: technology landscape of lipid-based nanocarriers. Adv. drug Deliv. Rev. 182, 114097. doi:10.1016/j.addr.2021.114097
Hao, Y., Chung, C. K., Yu, Z., Huis In 't Veld, R. V., Ossendorp, F. A., Ten Dijke, P., et al. (2022). Combinatorial therapeutic approaches with nanomaterial-based photodynamic cancer therapy. Pharmaceutics 14 (1), 120. doi:10.3390/pharmaceutics14010120
He, Z., Chen, K., An, Y., He, J., Zhang, X., Tang, L., et al. (2023). BSA modification of bacterial surface: a promising anti-cancer therapeutic strategy. BMC Microbiol. 23 (1), 105. doi:10.1186/s12866-023-02830-z
Hu, X., Bai, Z., Qiao, J., Li, H., Xu, S., Wang, X., et al. (2019). Effective enmein-type mimics of clinical candidate HAO472: design, synthesis and biological evaluation. Eur. J. Med. Chem. 171, 169–179. doi:10.1016/j.ejmech.2019.03.046
Hu, X., Wang, Y., Gao, X., Xu, S., Zang, L., Xiao, Y., et al. (2020). Recent progress of oridonin and its derivatives for the treatment of acute myelogenous leukemia. Mini Rev. Med. Chem. 20 (6), 483–497. doi:10.2174/1389557519666191029121809
Huang, H., Weng, H., Dong, B., Zhao, P., Zhou, H., and Qu, L. (2017). Oridonin triggers chaperon-mediated proteasomal degradation of BCR-ABL in leukemia. Sci. Rep. 7, 41525. doi:10.1038/srep41525
Hwang, T. L., and Chang, C. H. (2023). Oridonin enhances cytotoxic activity of natural killer cells against lung cancer. Int. Immunopharmacol. 122, 110669. doi:10.1016/j.intimp.2023.110669
Javanbakht, S., Hemmati, A., Namazi, H., and Heydari, A. (2020). Carboxymethylcellulose-coated 5-fluorouracil@MOF-5 nano-hybrid as a bio-nanocomposite carrier for the anticancer oral delivery. Int. J. Biol. Macromol. 155, 876–882. doi:10.1016/j.ijbiomac.2019.12.007
Jiang, J. H., Pi, J., and Cai, J. Y. (2020). Oridonin exhibits anti-angiogenic activity in human umbilical vein endothelial cells by inhibiting VEGF-induced VEGFR-2 signaling pathway. Pathology, Res. Pract. 216 (8), 153031. doi:10.1016/j.prp.2020.153031
Jiang, J. H., Pi, J., Jin, H., and Cai, J. Y. (2019). Oridonin-induced mitochondria-dependent apoptosis in esophageal cancer cells by inhibiting PI3K/AKT/mTOR and Ras/Raf pathways. J. Cell. Biochem. 120 (3), 3736–3746. doi:10.1002/jcb.27654
Jilani, K., Qadri, S. M., Zelenak, C., and Lang, F. (2011). Stimulation of suicidal erythrocyte death by oridonin. Archives Biochem. biophysics 511 (1-2), 14–20. doi:10.1016/j.abb.2011.05.001
Karimzadeh, Z., Javanbakht, S., and Namazi, H. (2019). Carboxymethylcellulose/MOF-5/Graphene oxide bio-nanocomposite as antibacterial drug nanocarrier agent. BioImpacts BI 9 (1), 5–13. doi:10.15171/bi.2019.02
Ke, Z., Zhang, Z., Wu, H., Jia, X., and Wang, Y. (2017). Optimization and evaluation of Oridonin-loaded Soluplus®-Pluronic P105 mixed micelles for oral administration. Int. J. Pharm. 518 (1-2), 193–202. doi:10.1016/j.ijpharm.2016.12.047
Kovačević, M., German Ilić, I., Bolko Seljak, K., and Zvonar Pobirk, A. (2022). High-shear wet granulation of SMEDDS based on mesoporous carriers for improved carvedilol solubility. Pharmaceutics 14 (10), 2077. doi:10.3390/pharmaceutics14102077
Kovalchuk, N. M., Johnson, D., Sobolev, V., Hilal, N., and Starov, V. (2019). Interactions between nanoparticles in nanosuspension. Adv. colloid interface Sci. 272, 102020. doi:10.1016/j.cis.2019.102020
Ku, C. M., and Lin, J. Y. (2013). Anti-inflammatory effects of 27 selected terpenoid compounds tested through modulating Th1/Th2 cytokine secretion profiles using murine primary splenocytes. Food Chem. 141 (2), 1104–1113. doi:10.1016/j.foodchem.2013.04.044
Leng, X., Dong, X., Wang, W., Sai, N., Yang, C., You, L., et al. (2018). Biocompatible Fe-based micropore metal-organic frameworks as sustained-release anticancer drug carriers. Mol. Basel, Switz. 23 (10), 2490. doi:10.3390/molecules23102490
Li, C., Wang, Q., Shen, S., Wei, X., and Li, G. (2018b). Oridonin inhibits VEGF-A-associated angiogenesis and epithelial-mesenchymal transition of breast cancer in vitro and in vivo. Oncol. Lett. 16 (2), 2289–2298. doi:10.3892/ol.2018.8943
Li, C., Zhang, D., Guo, H., Hao, L., Zheng, D., Liu, G., et al. (2013). Preparation and characterization of galactosylated bovine serum albumin nanoparticles for liver-targeted delivery of oridonin. Int. J. Pharm. 448 (1), 79–86. doi:10.1016/j.ijpharm.2013.03.019
Li, C., Zhang, D., Guo, Y., Guo, H., Li, T., Hao, L., et al. (2014). Galactosylated bovine serum albumin nanoparticles for parenteral delivery of oridonin: tissue distribution and pharmacokinetic studies. J. Microencapsul. 31 (6), 573–578. doi:10.3109/02652048.2014.898705
Li, C. Y., Wang, E. Q., Cheng, Y., and Bao, J. k. (2011a). Oridonin: an active diterpenoid targeting cell cycle arrest, apoptotic and autophagic pathways for cancer therapeutics. Int. J. Biochem. & cell Biol. 43 (5), 701–704. doi:10.1016/j.biocel.2011.01.020
Li, D., Han, T., Liao, J., Hu, X., Xu, S., Tian, K., et al. (2016). Oridonin, a promising ent-kaurane diterpenoid lead compound. Int. J. Mol. Sci. 17 (9), 1395. doi:10.3390/ijms17091395
Li, D., Wu, L. J., Tashiro, S., Onodera, S., and Ikejima, T. (2007). Oridonin-induced A431 cell apoptosis partially through blockage of the Ras/Raf/ERK signal pathway. J. Pharmacol. Sci. 103 (1), 56–66. doi:10.1254/jphs.fpj06016x
Li, G. Q., Gao, S. X., Wang, F. H., Kang, L., Tang, Z. Y., and Ma, X. D. (2023a). Anticancer mechanisms on pyroptosis induced by Oridonin: new potential targeted therapeutic strategies. Biomed. & Pharmacother. = Biomedecine & Pharmacother. 165, 115019. doi:10.1016/j.biopha.2023.115019
Li, H., Mu, J., Sun, J., Xu, S., Liu, W., Xu, F., et al. (2020a). Hydrogen sulfide releasing oridonin derivatives induce apoptosis through extrinsic and intrinsic pathways. Eur. J. Med. Chem. 187, 111978. doi:10.1016/j.ejmech.2019.111978
Li, L., Song, J. J., Zhang, M. X., Zhang, H. W., Zhu, H. Y., Guo, W., et al. (2023b). Oridonin ameliorates caspase-9-mediated brain neuronal apoptosis in mouse with ischemic stroke by inhibiting RIPK3-mediated mitophagy. Acta Pharmacol. Sin. 44 (4), 726–740. doi:10.1038/s41401-022-00995-3
Li, Q., Shi, Z., Zhang, F., Zeng, W., Zhu, D., and Mei, L. (2022). Symphony of nanomaterials and immunotherapy based on the cancer-immunity cycle. Acta Pharm. Sin. B 12 (1), 107–134. doi:10.1016/j.apsb.2021.05.031
Li, S., Liu, Y., Liu, T., Zhao, L., Zhao, J., and Feng, N. (2011b). Development and in-vivo assessment of the bioavailability of oridonin solid dispersions by the gas anti-solvent technique. Int. J. Pharm. 411 (1-2), 172–177. doi:10.1016/j.ijpharm.2011.04.006
Li, S., Shi, D., Zhang, L., Yang, F., and Cheng, G. (2018a). Oridonin enhances the radiosensitivity of lung cancer cells by upregulating Bax and downregulating Bcl-2. Exp. Ther. Med. 16 (6), 4859–4864. doi:10.3892/etm.2018.6803
Li, X., Zhang, C. T., Ma, W., Xie, X., and Huang, Q. (2021). Oridonin: a review of its pharmacology, pharmacokinetics and toxicity. Front. Pharmacol. 12, 645824. doi:10.3389/fphar.2021.645824
Li, Y., Cong, H., Wang, S., Yu, B., and Shen, Y. (2020b). Liposomes modified with bio-substances for cancer treatment. Biomaterials Sci. 8 (23), 6442–6468. doi:10.1039/d0bm01531h
Liao, M., Dong, Q., Chen, R., Xu, L., Jiang, Y., Guo, Z., et al. (2021). Oridonin inhibits DNMT3A R882 mutation-driven clonal hematopoiesis and leukemia by inducing apoptosis and necroptosis. Cell death Discov. 7 (1), 297. doi:10.1038/s41420-021-00697-5
Lin, F. E., Zhang, X. Y., Zhang, Y. P., and Wang, J. (2023). Preparation, characterization, and pharmacokinetics of oridonin-loaded liposomes. Biomed. Chromatogr. BMC 37 (5), e5603. doi:10.1002/bmc.5603
Liu, D., Qin, H., Yang, B., Du, B., and Yun, X. (2020). Oridonin ameliorates carbon tetrachloride-induced liver fibrosis in mice through inhibition of the NLRP3 inflammasome. Drug Dev. Res. 81 (4), 526–533. doi:10.1002/ddr.21649
Liu, J., Bai, Y., Li, Y., Li, X., and Luo, K. (2024c). Reprogramming the immunosuppressive tumor microenvironment through nanomedicine: an immunometabolism perspective. EBioMedicine 107, 105301. doi:10.1016/j.ebiom.2024.105301
Liu, J., Zhang, N., Li, N., Fan, X., and Li, Y. (2019). Influence of verapamil on the pharmacokinetics of oridonin in rats. Pharm. Biol. 57 (1), 787–791. doi:10.1080/13880209.2019.1688844
Liu, P., and Du, J. (2020). Oridonin is an antidepressant molecule working through the PPAR-γ/AMPA receptor signaling pathway. Biochem. Pharmacol. 180, 114136. doi:10.1016/j.bcp.2020.114136
Liu, S., Wang, X., Sun, X., Wei, B., Jiang, Z., Ouyang, Y., et al. (2024a). Oridonin inhibits bladder cancer survival and immune escape by covalently targeting HK1. Phytomedicine Int. J. phytotherapy Phytopharm. 126, 155426. doi:10.1016/j.phymed.2024.155426
Liu, W., Wang, X., Wang, L., Mei, Y., Yun, Y., Yao, X., et al. (2022). Oridonin represses epithelial-mesenchymal transition and angiogenesis of thyroid cancer via downregulating JAK2/STAT3 signaling. Int. J. Med. Sci. 19 (6), 965–974. doi:10.7150/ijms.70733
Liu, X., Xu, J., Zhou, J., and Shen, Q. (2021). Oridonin and its derivatives for cancer treatment and overcoming therapeutic resistance. Genes & Dis. 8 (4), 448–462. doi:10.1016/j.gendis.2020.06.010
Liu, Y., Liu, J., Liang, J., Zhang, M., Li, Z., Wang, Z., et al. (2017). Mucosal transfer of wheat germ agglutinin modified lipid-polymer hybrid nanoparticles for oral delivery of oridonin. Nanomedicine Nanotechnol. Biol. Med. 13 (7), 2219–2229. doi:10.1016/j.nano.2017.05.003
Liu, Y., Wang, P., Sun, C., Zhao, J., Du, Y., Shi, F., et al. (2011). Bioadhesion and enhanced bioavailability by wheat germ agglutinin-grafted lipid nanoparticles for oral delivery of poorly water-soluble drug bufalin. Int. J. Pharm. 419 (1-2), 260–265. doi:10.1016/j.ijpharm.2011.07.019
Liu, Y., Wang, X., Feng, H., Li, X., Yang, R., Zhang, M., et al. (2024b). Glutathione-depleting liposome adjuvant for augmenting the efficacy of a glutathione covalent inhibitor oridonin for acute myeloid leukemia therapy. J. nanobiotechnology 22 (1), 299. doi:10.1186/s12951-024-02574-6
Liu, Y., Zhang, P., Feng, N., Zhang, X., Wu, S., and Zhao, J. (2009). Optimization and in situ intestinal absorption of self-microemulsifying drug delivery system of oridonin. Int. J. Pharm. 365 (1-2), 136–142. doi:10.1016/j.ijpharm.2008.08.009
Liu, Z., Ouyang, L., Peng, H., and Zhang, W. Z. (2012). Oridonin: targeting programmed cell death pathways as an anti-tumour agent. Cell Prolif. 45 (6), 499–507. doi:10.1111/j.1365-2184.2012.00849.x
Lou, H., Gao, L., Wei, X., Zhang, Z., Zheng, D., Zhang, D., et al. (2011). Oridonin nanosuspension enhances anti-tumor efficacy in SMMC-7721 cells and H22 tumor bearing mice. Colloids surfaces B, Biointerfaces 87 (2), 319–325. doi:10.1016/j.colsurfb.2011.05.037
Lou, H., Zhang, X., Gao, L., Feng, F., Wang, J., Wei, X., et al. (2009). In vitro and in vivo antitumor activity of oridonin nanosuspension. Int. J. Pharm. 379 (1), 181–186. doi:10.1016/j.ijpharm.2009.06.022
Lu, Q., Kou, D., Lou, S., Ashrafizadeh, M., Aref, A. R., Canadas, I., et al. (2024). Nanoparticles in tumor microenvironment remodeling and cancer immunotherapy. J. Hematol. & Oncol. 17 (1), 16. doi:10.1186/s13045-024-01535-8
Lu, Y., Li, Y., and Wu, W. (2016). Injected nanocrystals for targeted drug delivery. Acta Pharm. Sin. B 6 (2), 106–113. doi:10.1016/j.apsb.2015.11.005
Luo, H., Vong, C. T., Chen, H., Gao, Y., Lyu, P., Qiu, L., et al. (2019). Naturally occurring anti-cancer compounds: shining from Chinese herbal medicine. Chin. Med. 14, 48. doi:10.1186/s13020-019-0270-9
Lv, Y., Li, W., Liao, W., Jiang, H., Liu, Y., Cao, J., et al. (2024). Nano-drug delivery systems based on natural products. Int. J. nanomedicine 19, 541–569. doi:10.2147/IJN.S443692
Mariam, J., Sivakami, S., and Dongre, P. M. (2016). Albumin corona on nanoparticles - a strategic approach in drug delivery. Drug Deliv. 23 (8), 2668–2676. doi:10.3109/10717544.2015.1048488
Matsuyama, Y., Nakamura, T., Yoshida, K., Hagi, T., Iino, T., Asanuma, K., et al. (2022). Radiodynamic therapy with acridine orange local administration as a new treatment option for primary and secondary bone tumours. Bone & Jt. Res. 11 (10), 715–722. doi:10.1302/2046-3758.1110.BJR-2022-0105.R2
Mhandire, D. Z., and Goey, A. K. L. (2022). The value of pharmacogenetics to reduce drug-related toxicity in cancer patients. Mol. diagnosis & Ther. 26 (2), 137–151. doi:10.1007/s40291-021-00575-x
Miller, N. A., Reddy, M. B., Heikkinen, A. T., Lukacova, V., and Parrott, N. (2019). Physiologically based pharmacokinetic modelling for first-in-human predictions: an updated model building strategy illustrated with challenging industry case studies. Clin. Pharmacokinet. 58 (6), 727–746. doi:10.1007/s40262-019-00741-9
Ou, H., Cheng, T., Zhang, Y., Liu, J., Ding, Y., Zhen, J., et al. (2018). Surface-adaptive zwitterionic nanoparticles for prolonged blood circulation time and enhanced cellular uptake in tumor cells. Acta biomater. 65, 339–348. doi:10.1016/j.actbio.2017.10.034
Parodi, A., Buzaeva, P., Nigovora, D., Baldin, A., Kostyushev, D., Chulanov, V., et al. (2021). Nanomedicine for increasing the oral bioavailability of cancer treatments. J. nanobiotechnology 19 (1), 354. doi:10.1186/s12951-021-01100-2
Qi, X., Zhang, D., Xu, X., Feng, F., Ren, G., Chu, Q., et al. (2012). Oridonin nanosuspension was more effective than free oridonin on G2/M cell cycle arrest and apoptosis in the human pancreatic cancer PANC-1 cell line. Int. J. nanomedicine 7, 1793–1804. doi:10.2147/IJN.S29483
Qiao, Z., Zhang, K., Liu, J., Cheng, D., Yu, B., Zhao, N., et al. (2022). Biomimetic electrodynamic nanoparticles comprising ginger-derived extracellular vesicles for synergistic anti-infective therapy. Nat. Commun. 13 (1), 7164. doi:10.1038/s41467-022-34883-5
Qin, J., Wang, X., Fan, G., Lv, Y., and Ma, J. (2023). Recent advances in nanodrug delivery system for tumor combination treatment based on photothermal therapy. Adv. Ther. 6 (3), 2200218. doi:10.1002/adtp.202200218
Qin, J., Zhang, J., Fan, G., Wang, X., Zhang, Y., Wang, L., et al. (2022). Cold atmospheric plasma activates selective photothermal therapy of cancer. Molecules 27 (18), 5941. doi:10.3390/molecules27185941
Ren, L., Li, J., Liu, L., Wu, W., Zhao, D., Zhang, K., et al. (2021). Resolving hepatic fibrosis via suppressing oxidative stress and an inflammatory response using a novel hyaluronic acid modified nanocomplex. Biomaterials Sci. 9 (24), 8259–8269. doi:10.1039/d1bm01499d
Shah, S., Dhawan, V., Holm, R., Nagarsenker, M. S., and Perrie, Y. (2020). Liposomes: advancements and innovation in the manufacturing process. Adv. drug Deliv. Rev. 154-155, 102–122. doi:10.1016/j.addr.2020.07.002
Shen, J., Gao, F., Pan, Q., Zong, Z., and Liang, L. (2024). Synthesis and application of a pH-responsive functional metal-organic framework: in vitro investigation for delivery of oridonin in cancer therapy. Vitro Investigation Deliv. Oridonin Cancer Ther. 29 (11), 2643. doi:10.3390/molecules29112643
Shen, Q. K., Chen, Z. A., Zhang, H. J., Li, J. L., Liu, C. F., Gong, G. H., et al. (2018). Design and synthesis of novel oridonin analogues as potent anticancer agents. J. enzyme inhibition Med. Chem. 33 (1), 324–333. doi:10.1080/14756366.2017.1419219
Shen, Y., and Ma, H. (2022). Oridonin-loaded lipid-coated calcium phosphate nanoparticles: preparation, characterization, and application in A549 lung cancer. Pharm. Dev. Technol. 27 (5), 598–605. doi:10.1080/10837450.2022.2090958
Siegel, R. L., Giaquinto, A. N., and Jemal, A. (2024). Cancer statistics, 2024. CA a cancer J. Clin. 74 (1), 12–49. doi:10.3322/caac.21820
Sobral, P. J. M., Vicente, A. T. S., and Salvador, J. A. R. (2023). Recent advances in oridonin derivatives with anticancer activity. Front. Chem. 11 (9), 1066280. doi:10.3389/fchem.2023.1066280
Spada, A., Emami, J., Tuszynski, J. A., and Lavasanifar, A. (2021). The uniqueness of albumin as a carrier in nanodrug delivery. Mol. Pharm. 18 (5), 1862–1894. doi:10.1021/acs.molpharmaceut.1c00046
Sun, B., Wang, G., Liu, H., Liu, P., Twal, W. O., Cheung, H., et al. (2018). Oridonin inhibits aberrant AKT activation in breast cancer. Oncotarget 9 (35), 23878–23889. doi:10.18632/oncotarget.24378
Sun, H., Nai, J., Deng, B., Zheng, Z., Chen, X., Zhang, C., et al. (2024). Angelica sinensis polysaccharide-based nanoparticles for liver-targeted delivery of oridonin. Mol. Basel, Switz. 29 (3), 731. doi:10.3390/molecules29030731
Sun, H. D., Huang, S. X., and Han, Q. B. (2006). Diterpenoids from Isodon species and their biological activities. Nat. Product. Rep. 23 (5), 673–698. doi:10.1039/b604174d
Sun, H. D., Lin, Z. W., Niu, F. D., Shen, P. Q., Pan, L. T., Lin, L. Z., et al. (1995). Diterpenoids from Isodon eriocalyx var. laxiflora. Phytochemistry 38 (6), 1451–1455. doi:10.1016/0031-9422(94)00815-b
Sun, K. W., Ma, Y. Y., Guan, T. P., Xia, Y. J., Shao, C. M., Chen, L. G., et al. (2012). Oridonin induces apoptosis in gastric cancer through Apaf-1, cytochrome c and caspase-3 signaling pathway. World J. gastroenterology 18 (48), 7166–7174. doi:10.3748/wjg.v18.i48.7166
Sung, H., Ferlay, J., Siegel, R. L., Laversanne, M., Soerjomataram, I., Jemal, A., et al. (2021). Global cancer statistics 2020: GLOBOCAN estimates of incidence and mortality worldwide for 36 cancers in 185 countries. CA a cancer J. Clin. 71 (3), 209–249. doi:10.3322/caac.21660
Suski, J. M., Braun, M., Strmiska, V., and Sicinski, P. (2021). Targeting cell-cycle machinery in cancer. Cancer cell 39 (6), 759–778. doi:10.1016/j.ccell.2021.03.010
Tan, W., Lu, J., Huang, M., Li, Y., Chen, M., Wu, G., et al. (2011). Anti-cancer natural products isolated from Chinese medicinal herbs. Chin. Med. 6 (1), 27. doi:10.1186/1749-8546-6-27
Tang, T., Huang, X., Zhang, G., Hong, Z., Bai, X., and Liang, T. (2021). Advantages of targeting the tumor immune microenvironment over blocking immune checkpoint in cancer immunotherapy. Signal Transduct. Target. Ther. 6 (1), 72. doi:10.1038/s41392-020-00449-4
Tarantino, P., Ricciuti, B., Pradhan, S. M., and Tolaney, S. M. (2023). Optimizing the safety of antibody-drug conjugates for patients with solid tumours. Nat. Rev. Clin. Oncol. 20 (8), 558–576. doi:10.1038/s41571-023-00783-w
Teran-Saavedra, N. G., Sarabia-Sainz, J. A., Silva-Campa, E., Burgara-Estrella, A. J., Guzmán-Partida, A. M., Ramos-Clamont Montfort, G., et al. (2019). Lactosylated albumin nanoparticles: potential drug nanovehicles with selective targeting toward an in vitro model of hepatocellular carcinoma. Mol. Basel, Switz. 24 (7), 1382. doi:10.3390/molecules24071382
Tian, L., Xie, K., Sheng, D., Wan, X., and Zhu, G. (2017). Antiangiogenic effects of oridonin. BMC complementary Altern. Med. 17 (1), 192. doi:10.1186/s12906-017-1706-3
Tran, S., DeGiovanni, P. J., Piel, B., and Rai, P. (2017). Cancer nanomedicine: a review of recent success in drug delivery. Clin. Transl. Med. 6 (1), 44. doi:10.1186/s40169-017-0175-0
Unsoy, G., and Gunduz, U. (2018). Smart drug delivery systems in cancer therapy. Curr. drug targets 19 (3), 202–212. doi:10.2174/1389450117666160401124624
Vangijzegem, T., Lecomte, V., Ternad, I., Van Leuven, L., Muller, R. N., Stanicki, D., et al. (2023). Superparamagnetic iron oxide nanoparticles (SPION): from fundamentals to state-of-the-art innovative applications for cancer therapy. Pharmaceutics 15 (1), 236. doi:10.3390/pharmaceutics15010236
Wang, C., Li, W., and Hu, B. (2017). The anti-tumor effect of folate-targeted liposome microbubbles loaded with oridonin as ultrasound-triggered tumor-targeted therapeutic carrier system. J. drug Target. 25 (1), 83–91. doi:10.1080/1061186X.2016.1200588
Wang, C., Wei, Y., Yu, L., and Zhang, L. (2009). The effect of stealth liposomes on pharmacokinetics, tissue distribution and anti-tumor activity of oridonin. PDA J. Pharm. Sci. Technol. 63 (5), 409–416.
Wang, C. J., Wang, H. Z., and Li, W. (2019). A novel conjunction of folate-targeted carbon nanotubes containing protohemin and oridonin-liposome loaded microbubbles for cancer chemo-sonodynamic therapy. J. drug Target. 27 (10), 1076–1083. doi:10.1080/1061186X.2019.1591422
Wang, J., Gong, J., and Wei, Z. (2021). Strategies for liposome drug delivery systems to improve tumor treatment efficacy. AAPS PharmSciTech 23 (1), 27. doi:10.1208/s12249-021-02179-4
Wang, J., and Zhang, B. (2018). Bovine serum albumin as a versatile platform for cancer imaging and therapy. Curr. Med. Chem. 25 (25), 2938–2953. doi:10.2174/0929867324666170314143335
Wang, L., Li, H., Chen, J., Wang, Y., Gu, Y., and Jiu, M. (2024). Antibacterial mechanisms and antivirulence activities of oridonin against pathogenic aeromonas hydrophila AS 1.1801. Microorganisms 12 (2), 415. doi:10.3390/microorganisms12020415
Wang, S., Zhong, Z., Wan, J., Tan, W., Wu, G., Chen, M., et al. (2013). Oridonin induces apoptosis, inhibits migration and invasion on highly-metastatic human breast cancer cells. Am. J. Chin. Med. 41 (1), 177–196. doi:10.1142/S0192415X13500134
Wang, Y., Wang, M., Lin, F., Zhang, X., Zhao, Y., Guo, C., et al. (2022). Preparation, characterization, and evaluation of liposomes containing oridonin from Rabdosia rubescens. Mol. Basel, Switz. 27 (3), 860. doi:10.3390/molecules27030860
Wang, Y., and Zhu, Z. (2019). Oridonin inhibits metastasis of human ovarian cancer cells by suppressing the mTOR pathway. Archives Med. Sci. AMS 15 (4), 1017–1027. doi:10.5114/aoms.2018.77068
Wu, J., Ding, Y., Chen, C. H., Zhou, Z., Ding, C., Chen, H., et al. (2016). A new oridonin analog suppresses triple-negative breast cancer cells and tumor growth via the induction of death receptor 5. Cancer Lett. 380 (2), 393–402. doi:10.1016/j.canlet.2016.06.024
Wu, M. X., and Yang, Y. W. (2017). Metal-organic framework (MOF)-Based drug/cargo delivery and cancer therapy. Adv. Mater. Deerf. Beach, Fla 29 (23), 214–223. doi:10.1002/adma.201606134
Wu, Q., Gao, X., Lin, Y., Wu, C., Zhang, J., Chen, M., et al. (2024). Integrating epigenetics, proteomics, and metabolomics to reveal the involvement of wnt/β-catenin signaling pathway in oridonin-induced reproductive toxicity. Toxics 12 (5), 339. doi:10.3390/toxics12050339
Xia, S., Zhang, X., Li, C., and Guan, H. (2017). Oridonin inhibits breast cancer growth and metastasis through blocking the Notch signaling. Saudi Pharm. J. 25 (4), 638–643. SPJ : the official publication of the Saudi Pharmaceutical Society. doi:10.1016/j.jsps.2017.04.037
Xie, R. J., Yan, F. L., Hai, G. F., Hou, R. J., Ding, M. M., and Bai, Y. X. (2011). Two new diterpenoids and other constituents from Isodon rubescens. Fitoterapia 82 (4), 726–730. doi:10.1016/j.fitote.2011.03.003
Xin, X., Li, J., Wu, W., Zhao, P., Yang, Y., Zhu, Y., et al. (2023a). ROS-scavenging nanomedicine for “multiple crosstalk” modulation in non-alcoholic fatty liver disease. Biomaterials Sci. 11 (10), 3709–3725. doi:10.1039/d2bm02161g
Xin, X., Zhou, Y., Li, J., Zhang, K., Qin, C., and Yin, L. (2023b). CXCL10-coronated thermosensitive “stealth” liposomes for sequential chemoimmunotherapy in melanoma. Nanomedicine Nanotechnol. Biol. Med. 48, 102634. doi:10.1016/j.nano.2022.102634
Xu, J., Zhao, J. H., Liu, Y., Feng, N. P., and Zhang, Y. T. (2012). RGD-modified poly(D,L-lactic acid) nanoparticles enhance tumor targeting of oridonin. Int. J. nanomedicine 7, 211–219. doi:10.2147/IJN.S27581
Xu, L., Bi, Y., Xu, Y., Zhang, Z., Xu, W., Zhang, S., et al. (2020). Oridonin inhibits the migration and epithelial-to-mesenchymal transition of small cell lung cancer cells by suppressing FAK-ERK1/2 signalling pathway. J. Cell. Mol. Med. 24 (8), 4480–4493. doi:10.1111/jcmm.15106
Xu, L., Jiang, Y., Bi, Y., Zheng, S., Wu, Y., Wu, Y., et al. (2024). Suppression of PERK/eIF2α/CHOP pathway enhances oridonin-induced apoptosis by inhibiting autophagy in Small-Cell lung cancer cells. Biomed. & Pharmacother. = Biomedecine & Pharmacother. 175, 116684. doi:10.1016/j.biopha.2024.116684
Xu, L., Zhu, L., Zheng, K., Liu, J., Tian, P., Hu, D., et al. (2021). The design and synthesis of redox-responsive oridonin polymeric prodrug micelle formulation for effective gastric cancer therapy. J. Mater. Chem. B 9 (13), 3068–3078. doi:10.1039/d1tb00127b
Xu, W., Sun, J., Zhang, T. T., Ma, B., Cui, S. m., Chen, D. w., et al. (2006). Pharmacokinetic behaviors and oral bioavailability of oridonin in rat plasma. Acta Pharmacol. Sin. 27 (12), 1642–1646. doi:10.1111/j.1745-7254.2006.00440.x
Xu, Z. Z., Fu, W. B., Jin, Z., Guo, P., Wang, W. F., and Li, J. M. (2016). Reactive oxygen species mediate oridonin-induced apoptosis through DNA damage response and activation of JNK pathway in diffuse large B cell lymphoma. Leukemia & lymphoma 57 (4), 888–898. doi:10.3109/10428194.2015.1061127
Xue, B., Wang, Y., Tang, X., Xie, P., Wang, Y., Luo, F., et al. (2012). Biodegradable self-assembled MPEG-PCL micelles for hydrophobic oridonin delivery in vitro. J. Biomed. Nanotechnol. 8 (1), 80–89. doi:10.1166/jbn.2012.1358
Yan, Y., Tan, R. Z., Liu, P., Li, J. C., Zhong, X., Liao, Y., et al. (2020). Oridonin alleviates IRI-induced kidney injury by inhibiting inflammatory response of macrophages via AKT-related pathways. Med. Sci. Monit. Int. Med. J. Exp. Clin. Res. 26, e921114. doi:10.12659/MSM.921114
Yao, H., Xie, S., Ma, X., Liu, J., Wu, H., Lin, A., et al. (2020). Identification of a potent oridonin analogue for treatment of triple-negative breast cancer. J. Med. Chem. 63 (15), 8157–8178. doi:10.1021/acs.jmedchem.0c00408
Yao, Z., Xie, F., Li, M., Liang, Z., Xu, W., Yang, J., et al. (2017). Oridonin induces autophagy via inhibition of glucose metabolism in p53-mutated colorectal cancer cells. Cell death & Dis. 8 (2), e2633. doi:10.1038/cddis.2017.35
Yasuda, S., Horinaka, M., Iizumi, Y., Goi, W., Sukeno, M., and Sakai, T. (2022). Oridonin inhibits SASP by blocking p38 and NF-κB pathways in senescent cells. Biochem. biophysical Res. Commun. 590, 55–62. doi:10.1016/j.bbrc.2021.12.098
Ye, Y. C., Wang, H. J., Xu, L., Liu, W. w., Liu, B. b., Tashiro, S. I., et al. (2012). Oridonin induces apoptosis and autophagy in murine fibrosarcoma L929 cells partly via NO-ERK-p53 positive-feedback loop signaling pathway. Acta Pharmacol. Sin. 33 (8), 1055–1061. doi:10.1038/aps.2012.53
Zang, L., He, H., Xu, Q., Yu, Y., Zheng, N., Liu, W., et al. (2013). Reactive oxygen species H2O2 and •OH, but not O2•(-) promote oridonin-induced phagocytosis of apoptotic cells by human histocytic lymphoma U937 cells. Int. Immunopharmacol. 15 (2), 414–423. doi:10.1016/j.intimp.2013.01.004
Zang, L., Wang, J., Ren, Y., Liu, W., Yu, Y., Zhao, S., et al. (2019). Activated toll-like receptor 4 is involved in oridonin-induced phagocytosis via promotion of migration and autophagy-lysosome pathway in RAW264.7 macrophages. Int. Immunopharmacol. 66, 99–108. doi:10.1016/j.intimp.2018.11.014
Zang, L., Xu, Q., Ye, Y., Li, X., Liu, Y., Tashiro, S. I., et al. (2012). Autophagy enhanced phagocytosis of apoptotic cells by oridonin-treated human histocytic lymphoma U937 cells. Archives Biochem. biophysics 518 (1), 31–41. doi:10.1016/j.abb.2011.11.019
Zhang, B., Huang, Y., and Huang, Y. (2024). Advances in nanodynamic therapy for cancer treatment. Nanomater. Basel, Switz. 14 (7), 648. doi:10.3390/nano14070648
Zhang, P., Liu, Y., Feng, N., and Xu, J. (2008). Preparation and evaluation of self-microemulsifying drug delivery system of oridonin. Int. J. Pharm. 355 (1-2), 269–276. doi:10.1016/j.ijpharm.2007.12.026
Zhang, W., Shi, L., Zhou, W., Liu, X., Xi, Y., Wang, X., et al. (2023b). Oridonin impedes breast cancer growth by blocking cells in S phase and inhibiting the PI3K/AKT/mTOR signaling pathway. Heliyon 9 (7), e18046. doi:10.1016/j.heliyon.2023.e18046
Zhang, X., Zhang, T., Lan, Y., Wu, B., and Shi, Z. (2016). Nanosuspensions containing oridonin/HP-β-Cyclodextrin inclusion complexes for oral bioavailability enhancement via improved dissolution and permeability. AAPS PharmSciTech 17 (2), 400–408. doi:10.1208/s12249-015-0363-4
Zhang, Y., Wang, S., Dai, M., Nai, J., Zhu, L., and Sheng, H. (2020). Solubility and bioavailability enhancement of oridonin: a review. Mol. Basel, Switz. 25 (2), 332. doi:10.3390/molecules25020332
Zhang, Y., Wu, Y., Du, H., Zhi, L., Xiao, B., Yang, W., et al. (2023a). Nano-drug delivery systems in oral cancer therapy: recent developments and prospective. Pharmaceutics 16 (1), 7. doi:10.3390/pharmaceutics16010007
Zhang, Z., Zhang, X., Xue, W., Yangyang, Y., Xu, D., Zhao, Y., et al. (2010). Effects of oridonin nanosuspension on cell proliferation and apoptosis of human prostatic carcinoma PC-3 cell line. Int. J. nanomedicine 5, 735–742. doi:10.2147/IJN.S13537
Zhao, L., Jiang, M., Xu, Z., Sun, F., Wu, X., Zhang, M., et al. (2022b). Selective thermotherapy of tumor by self-regulating photothermal conversion system. J. colloid interface Sci. 605, 752–765. doi:10.1016/j.jcis.2021.07.134
Zhao, L., Wang, X., Lou, H., Jiang, M., Wu, X., Qin, J., et al. (2022a). Buffet-style Cu(II) for enhance disulfiram-based cancer therapy. J. colloid interface Sci. 624, 734–746. doi:10.1016/j.jcis.2022.06.009
Zhao, L., Zhang, X., Wang, X., Guan, X., Zhang, W., and Ma, J. (2021). Recent advances in selective photothermal therapy of tumor. J. nanobiotechnology 19 (1), 335. doi:10.1186/s12951-021-01080-3
Zheng, D., Dai, W., Zhang, D., Duan, C., Jia, L., Liu, Y., et al. (2012a). In vivo studies on the oridonin-loaded nanostructured lipid carriers. Drug Deliv. 19 (6), 286–291. doi:10.3109/10717544.2012.704096
Zheng, D., Duan, C., Zhang, D., Jia, L., Liu, G., Liu, Y., et al. (2012b). Galactosylated chitosan nanoparticles for hepatocyte-targeted delivery of oridonin. Int. J. Pharm. 436 (1-2), 379–386. doi:10.1016/j.ijpharm.2012.06.039
Zheng, M., Zhu, Z., Zhao, Y., Yao, D., Wu, M., and Sun, G. (2017). Oridonin promotes G2/M arrest in A549 cells by facilitating ATM activation. Mol. Med. Rep. 15 (1), 375–379. doi:10.3892/mmr.2016.6008
Keywords: oridonin, drug delivery system, nanoparticle, antitumor, carrier
Citation: Su Y, Liu L, Lin C, Deng D, Li Y, Huang M, Wang Y, Ling K, Wang H, Chen Q and Huang G (2024) Enhancing cancer therapy: advanced nanovehicle delivery systems for oridonin. Front. Pharmacol. 15:1476739. doi: 10.3389/fphar.2024.1476739
Received: 06 August 2024; Accepted: 18 November 2024;
Published: 03 December 2024.
Edited by:
Olivier Feron, Université catholique de Louvain, BelgiumReviewed by:
Ravi Prakash Shukla, Icahn School of Medicine at Mount Sinai, United StatesJinlong Ma, Shandong Second Medical University, China
Copyright © 2024 Su, Liu, Lin, Deng, Li, Huang, Wang, Ling, Wang, Chen and Huang. This is an open-access article distributed under the terms of the Creative Commons Attribution License (CC BY). The use, distribution or reproduction in other forums is permitted, provided the original author(s) and the copyright owner(s) are credited and that the original publication in this journal is cited, in accordance with accepted academic practice. No use, distribution or reproduction is permitted which does not comply with these terms.
*Correspondence: Lisha Liu, bGlzYWxpdUBjZHV0Y20uZWR1LmNu; Guixiao Huang, aHVhbmdneDcxQDE2My5jb20=
†These authors have contributed equally to this work