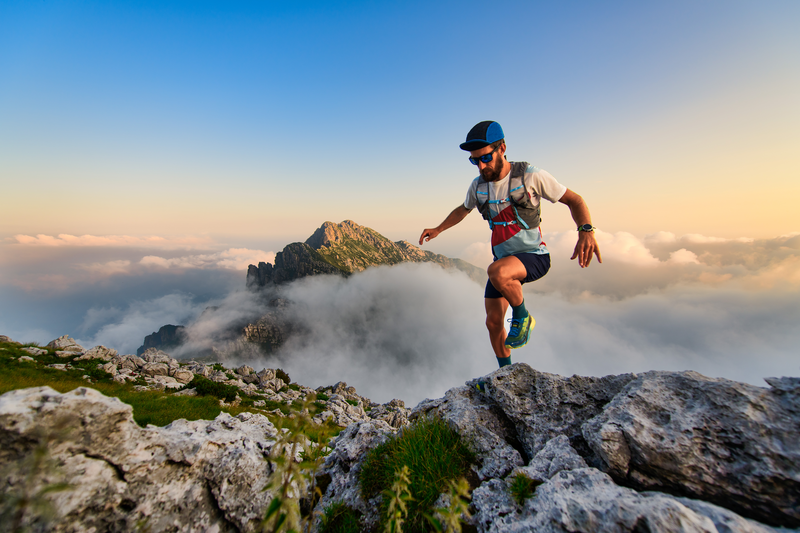
95% of researchers rate our articles as excellent or good
Learn more about the work of our research integrity team to safeguard the quality of each article we publish.
Find out more
REVIEW article
Front. Pharmacol. , 05 November 2024
Sec. Ethnopharmacology
Volume 15 - 2024 | https://doi.org/10.3389/fphar.2024.1475975
This article is part of the Research Topic Herbal Medicine for the Treatment of Chronic Metabolic Diseases, Volume II View all 17 articles
Heart failure (HF) is a clinical condition caused by abnormalities in the heart’s structure or function, primarily manifested as diminished ability of the heart to pump blood, which leads to compensatory activation of neurohormones and increased left ventricular filling pressure. HF is one of the fastest-growing cardiovascular diseases globally in terms of incidence and mortality, negatively impacting patients’ quality of life and imposing significant medical and economic burdens. Despite advancements in the treatment of HF, hospitalization and mortality remain rates high. In China, Chinese herbal medicine (CHM) has historically played a prominent role in addressing HF, with significant proven efficacy. MicroRNA (miRNA) exerts a pivotal regulatory influence on the maintenance of regular cardiac activity and the progression of HF. MiRNAs, a category of single-stranded RNA molecules, are characterized by their inability to code for proteins. They regulate gene expression by binding to the 3′-untranslated region (3′-UTR) of target mRNAs, thereby influencing the onset and progression of various diseases. Abnormal expression of specific miRNAs is closely associated with HF pathological processes, such as cardiomyocyte apoptosis, myocardial fibrosis, and cardiac hypertrophy. This abnormal expression can influence the pathological progression of HF through the regulation of miRNA expression. This article reviews the regulatory role of miRNAs in HF pathology discusses how CHM compounds and their active ingredients can ameliorate HF pathology through the regulation of miRNA expression. In conclusion, miRNAs represent promising therapeutic targets for HF, and CHM provides a novel strategy for treatment through the regulation of miRNA expression. Future studies must delve deeper into the precise mechanisms by which CHM modulates miRNAs and fully explore its potential for clinical application in HF treatment.
Heart failure (HF) is a clinical condition resulting from structural or functional impairments of the heart (Amgalan and Kitsis, 2019). Traditionally, HF is characterized by a decrease in the heart’s pumping and/or congestion ability, stemming from abnormal cardiac structure or function that results in insufficient cardiac output, leading to compensatory neurohormonal activation and increased left ventricular filling pressure (Savarese et al., 2023). HF frequently occurs in the terminal stages of various organic heart ailments and other diseases, making it one of the fastest-growing cardiovascular diseases globally in terms of incidence and mortality rates. Over the past few decades, despite considerable progress in the management of HF, hospitalization rates and mortality have remained stubbornly high, making HF a significant factor that severely impacts patients’ quality of life. Generally, the best treatment for HF is involves an individualized plan, which may include general treatment, drug therapy, surgical interventions, and other approaches aimed at alleviating physical discomfort. However, the prognosis for HF after treatment is still poor, and the recent hospitalization rate and related medical expenses of HF following treatment remains poor, and recent hospitalization rates and associated medical expenses have increased significantly (Iyngkaran et al., 2018; Ziaeian and Fonarow, 2016). In 2012, total expenditure on HF treatment in the United States amounted $30.7 billion. Forecasts indicate that by 2030, this amount is projected to escalate to an alarming $69.7 billion (Sinnenberg and Givertz, 2020). Furthermore, the absolute number of HF hospitalizations is also projected to increase dramatically over the next 25 years, potentially by as much as 50% (Al-Mohammad et al., 2010; Savarese and Lund, 2017). Therefore, it is imperative that we to comprehend the etiology of HF while concurrently innovating novel therapeutic approaches and more effective medications to combat this escalating health crisis.
Currently, the primary treatment for HF involves modern biomedicine; however, conventional treatment targets of modern biomedicine are relatively limited, lacking distinctive personalized treatment features and associated with numerous adverse drug reactions (Jia et al., 2020). Traditional Chinese Medicine (TCM), a unique medical system in China with a long history, is widely practiced in folklore and applied in the treatment and conditioning of various diseases. TCM not only demonstrates significant therapeutic effects but also exhibits fewer side effects (Fan et al., 2022) Furthermore, TCM, especially Chinese herbal medicine (CHM), holds unique advantages in regulating the body, preventing diseases, and promoting overall health. Consequently, TCM occupies a significant position within the modern medical system (Li et al., 2020). Recent studies have highlighted the positive effects of CHM active components, including tanshinone IIA (Tan IIA), Lycium barbarum polysaccharide (LBP), ginsenosides, Panax notoginseng saponins (PNS), and Total glucosides of paeony (TGP) on the cardiovascular system. These components exhibit anti-apoptotic, antioxidant, anti-inflammatory, and antifibrotic effects, providing substantial therapeutic benefits for HF (Hao et al., 2017). The multi-channel and multi-target advantages of TCM enable it to circumvent issues associated with modern biomedicine, such as adverse effects arising from genetic defects in therapeutic targets and diminished drug efficacy due to metabolic genetic variations (Wang et al., 2017). Therefore, CHM and its active ingredients exhibit considerable potential for application in the treatment of HF. Currently, a growing body of research is focused on the therapeutic effects of CHM on HF, aiming to elucidate its underlying mechanisms and explore its applications in modern medicine. Through systematic scientific research and clinical trials, CHM has the potential to become a significant supplement and a key direction for development in HF treatment.
MicroRNAs (miRNAs), characterized by their single-stranded RNA structure, comprise 19 to 25 nucleotides and are incapable of encoding proteins. By binding to the 3′-UTR, these molecules are known to suppress the post-transcriptional expression of mRNA (Liu et al., 2019). The process of miRNA biogenesis is initiated by the transcription of primary miRNA transcripts, known as pri-miRNAs. These pri-miRNAs are subsequently subjected to a series of modifications, transforming them into precursor miRNAs, known as pre-miRNAs. Following a cascade of events, the pre-miRNAs mature, resulting in functional miRNAs that integrate into the RNA-induced silencing complex (RISC) to execute their regulatory roles (Treiber et al., 2019) (Figure 1). MiRNAs are pivotal regulatory elements in post-transcriptional processes and have emerged as a novel category of gene expression modulators integral to both human health and the development of pathologies (Michlewski and Cáceres, 2019; Sucharov et al., 2017). Growing evidence suggests that miRNAs occupy a pivotal position in regulating various aspects of cardiac function and the progression of HF (Zhang R. et al., 2018). Therefore, enhancing the understanding of miRNAs’ roles could contribute to uncovering novel mechanisms, identifying biomarkers, and pinpointing potential targets for cardiovascular conditions.
Within cardiac tissue, miRNAs serve as regulators, impacting vital processes such as heart development, fibrosis progression, inflammatory responses, and tissue regeneration (Thum and Condorelli, 2015). The upregulation or downregulation of specific miRNAs can directly affect the function and structure of cardiomyocytes, thereby facilitating the progression of HF (Gargiulo et al., 2023). Currently, a multitude of research efforts are concentrating on the link between miRNAs and the application of CHM in treating HF. It is particularly noteworthy that CHM has demonstrated therapeutic effect on HF by regulating miRNA expression (Wu et al., 2023). These studies indicate that miRNAs have broad potential applications in the future practice of CHM of HF treatment and are expected to become key therapeutic intervention targets.
This article delves into the pivotal functions of miRNAs in processes such as myocardial hypertrophy, fibrosis, and apoptosis, as well as the specific mechanisms by which CHM regulates miRNAs. To this end, literature searches on HF were conducted using databases such as Web of Science, PubMed, ScienceDirect, Springer, Wiley, focusing on studies published between 2013 and 2023. The keywords “heart failure” and/or “miRNA” were employed, either individually or in combination with terms such as “CHM”, “fibrosis”, “autophagy”, “apoptosis”. The research findings from these searches are presented as follows.
The emergence and progression of HF are complex, involving mechanisms such as apoptosis, autophagy of cardiac myocytes, cardiac myocyte hypertrophy, cardiac fibrosis, and inflammation (Triposkiadis et al., 2022). However, miRNAs are involved in nearly all of these pathological processes associated with HF and play a crucial regulatory role (Figure 2).
Apoptosis of myocardial cells represents a crucial pathophysiological mechanism in HF and serves as a key driver in its progression (Xue et al., 2023). Apoptosis is a death process caused by a sequence of cellular death process resulting from a series of changes in response to environmental signals, altered environmental conditions, or compensatory injuries, playing a crucial role in the clearance of infected cells (Corsetti et al., 2019). Since mitochondria are the primary organelles responsible for reactive oxygen species and ATP within cardiomyocytes, disruptions in mitochondrial energy supply, cell death, and dysfunction play a critical role in the pathogenesis of HF (Chen et al., 2011). Increasingly, studies have demonstrated that miRNAs are involved in regulating mitochondrial morphology in cardiomyocytes, and in the process of endoplasmic reticulum stress-induced injury and apoptosis (Zhou et al., 2023). This section primarily discusses the impact of miRNA on mitochondrial functionality and the stress experienced by the endoplasmic reticulum in cardiomyocytes during HF, elucidating their protective role against apoptosis and potential therapeutic strategies (Table 1).
Wang et al. (2015d) discovered that miR-421 is involved in suppressing the translation of Pink1, leading to myocardial infarction, apoptosis, and mitochondrial fragmentation by decreasing Pink1 expression, a serine/threonine kinase that targets mitochondria and inhibits cardiomyocyte apoptosis (Siddall et al., 2013). Moreover, E2F1 is a key member of the E2F family of transcription factors, essential for cardiac function and primarily influencing cardiomyocyte metabolism (Dassanayaka et al., 2019). E2F1 can transcriptionally activate the expression of miR-421, thereby regulating mitochondrial fission and promoting apoptosis. Knockdown of E2F1 can suppress mitochondrial fission and apoptosis in myocardial cells. These findings establish the E2F1/miR-421/Pink1 signaling pathway as a critical regulatory factor for mitochondrial division and cell death in myocardial cells. Additional research indicates that miR-762 plays a crucial role in modulating mitochondrial function and inducing cardiomyocyte apoptosis via the core assembly subunit ND2 of mitochondrial complex I. As a novel contributor to mitochondrial dysfunction, miR-762 may also serve as an effective therapeutic target for HF (Yan et al., 2019). Wang et al. (2015c) found that miR-324–5p acts as a suppressor of translation for the mitochondrial fission regulator 1 (Mtfr1). They further elucidated that this miRNA mitigates mitochondrial fission, apoptosis, and myocardial infarction by decreasing expression levels of Mtfr1. However, NFAT4, a gene located on mouse chromosome 8, exerts a suppressive influence on miR-324–5p. This gene is pivotal in reducing voltage-gated potassium currents following myocardial infarction. Moreover, its regulatory role extends to influencing mitochondrial fission and the initiation of apoptosis. This study establishes the NFAT4/miR-324–5p/Mtfr1 pathway as involved in the modulation of mitochondrial division and programmed cell death in myocardial cells, offering a potential new therapeutic approach for heart disease. Similarly, another study found that miR-361 is involved in inhibiting the translation of prohibitin 1 (PHB1). MiR-361 triggers mitochondrial fission, apoptosis, and HF by reducing PHB1 levels. Knockdown of miR-361 increases PHB1 levels and reduces mitochondrial fragmentation and apoptosis both in vivo and in vitro, thereby protecting the heart from ischemic injury (Wang et al., 2015a). The research reveals a novel mechanism for regulating mitochondrial fragmentation and apoptosis involving miR-361 and PHB1. This finding may provide novel insights into therapeutic approaches for myocardial infarction and HF.
Additionally, some miRNAs are involved in regulating myocardial cell apoptosis through alternative pathways or signaling mechanisms. Zhang D. et al. (2023) discovered that increased levels of miR-144–3p mitigates HF and cardiomyocyte apoptosis induced by doxorubicin (Dox) in rats, acting through the SOCS2/PI3K/AKT signaling pathway. In Dox-treated cardiomyocytes, suppression of SOCS2 counteracted the downregulating effects of miR-144–3p. The upregulation of miR-144–3p reduced apoptosis and heart dysfunction caused by Dox by targeting SOCS2. This finding provides new insights into the role of miR-144–3p in HF. In another study, researchers observed a significant reduction in miR-125b levels in the cardiac muscle of mice afflicted by HF. Overexpression of miR-125b can inhibit myocardial cell apoptosis by targeting the Bcl-2 homologous antagonist/killer (BAK1), effectively alleviating cardiac dysfunction in HF mice. This suggests that targeting the miR-125b/BAK1 pathway may be a viable strategy for the detection or management of HF (Zhang et al., 2021). Yang et al. (2019) found that miR-19b-1, a key component of the miR-17/92 cluster, is essential for curbing cell death. miR-19b-1 targets the pro-apoptotic Bcl-2 family gene (Bcl2l11/BIM), reducing its mRNA and protein levels, thereby reversing ischemia-induced HF by inhibiting myocardial cell apoptosis.
Autophagy is a process in which impaired organelles and abnormal protein aggregates, among other large cellular components, are enclosed within lysosomes for subsequent degradation. This process is crucial for maintaining cell survival, differentiation, development, and homeostasis (Mizushima and Levine, 2010). Autophagy is intimately linked to the maintenance of cardiac equilibrium, both under healthy conditions and in pathological states such as myocardial hypertrophy, cardiac fibrosis, myocardial infarction, HF, and other structural heart diseases (Lampert and Gustafsson Å, 2018). Under stress stimuli, autophagy can function both as a protective mechanism that promote the survival of myocardial cells and as a maladaptive process that induces the death (Delbridge et al., 2017). Additionally, research indicates that miRNAs serve as pivotal regulators of the autophagy process, exerting significant influence over its modulation in cardiomyocytes through both transcriptional and post-transcriptional regulatory mechanisms. This section focuses on how autophagy and associated miRNAs participate in and influence the initiation and advancement progression of HF, offering a new perspective for exploring therapeutic strategies for HF.
Autophagy involves a suite of conserved Atg gene sequences that are present from yeast to humans. ATG7 is recognized as a significant biomarker for autophagy and also functions as an essential initiator of the autophagic process, playing key regulatory roles in cell death and survival (Pattison et al., 2011). In both in vivo and in vitro studies, Wang et al. (2015b) found that miR-188–3p specifically targets ATG7, thus inhibiting autophagy and apoptosis. However, research has demonstrated that an autophagy-promoting factor (APF) can directly interact with miR-188–3p, thereby dampening its activity and modulating autophagy and autophagic cell death through the miR-188–3p/ATG7 signaling pathway. This study reveals a novel miRNA-related model for regulating autophagy programs, with the potential for level modulation to be utilized as a diagnostic indicator and target for developing innovative treatments for HF. Su et al. (2015) discovered that in mice, overexpression of miR-221 specifically in the heart induced cardiac dysfunction and HF, attributed to the suppression of autophagy by miR-221. Specifically, p27, a cyclin-dependent kinase (CDK) inhibitor, is identified as a primary molecular target of miR-221 in cardiomyocytes. In cardiomyocytes, miR-221 orchestrates the p27/CDK2/mTOR signaling pathway, thereby influencing cardiac remodeling and autophagy in the heart. which is a classic pathway exerting negative regulation on autophagy (Zhang H. et al., 2018). miR-129–5p has been confirmed to suppress autophagy and apoptosis triggered by H2O2 in rat cardiomyocytes (H9C2) by lowering ATG14 levels and activating the PI3K/Akt/mTOR pathway (Zhang et al., 2020). Mi et al. (2023) found that the maternal expression of gene 3 (MEG3) mediates HF and excessive cardiac remodeling in mice induced by subcutaneous injections of isoproterenol (ISOP). The reduction of MEG3 levels notably inhibits the excessive apoptosis and autophagy in cardiomyocytes provoked by ISOP and H2O2. This effect is achieved by modulating the miRNA-129–5p/ATG14/Akt signaling pathway. Xue et al. (2023) found that the hepatic leukemia factor Hlf/FYVE and the protein containing coiled-coil domain 1 (FYCO1) are prospective targets for miR-103–3p. This miRNA exerts its effects on apoptosis and autophagy through repressing Hlf expression via binding to the 3′-UTR region of the Hlf/FYCO1 gene.
Myocardial fibrosis is associated with an unfavorable prognosis in heart-related conditions and is one of the most prevalent pathophysiological outcomes triggered by stimuli that can be either acute, such as myocardial infarction, or chronic, such as hypertension (Hinderer and Schenke-Layland, 2019; Xing et al., 2024). In the myocardium, cardiac fibrosis manifests as a scarring process, characterized by the proliferation and transformation of cardiac fibroblasts into myofibroblasts influenced by mechanical stimuli, pressure/volume overload, and circulating humoral factors. This leads to an overproduction of extracellular matrix (ECM), a reduction in the heart’s adaptability, and initiates structural transformation, culminating in HF (Gourdie et al., 2016; Travers et al., 2016). Furthermore, myocardial fibrosis correlates with both ventricular dysfunction and arrhythmias, serving as a negative predictor of outcomes for individuals with HF (Frangogiannis, 2019).
Recent findings indicate that the miR-29 family regulates cardiac metabolism across various cardiovascular tissues, including aortic tissues, myocardial tissues, vascular endothelial cells, and cardiomyocytes(Liu et al., 2021). The miR-29 family, specifically miR-29a and miR-29b, shows promise as potential predictive biomarkers for the progression of HF (Lakhani et al., 2018). In a canine model of CHF, the expression of miR-29b was reduced, accompanied by a marked increase in collagen I/III and fibrillin levels in extracted myocardial fibroblasts. Knocking down miR-29b in canine atrial fibroblasts using a lentivirus resulted in an increase in collagen expression. Conversely, elevated levels of miR-29b correlated with reduced collagen levels (Dawson et al., 2013). Activation of AMPK suppresses hepatocyte nuclear factor 4α (HNF-4α) transcription factor expression. This suppression leads to decrease TGF-β1 levels and increased miR-29 expression. These coordinated effects ultimately inhibit the progression of cardiac fibrosis and improve heart function in rats affected by this condition (Qi et al., 2017). Peli1, recognized as an E3 ubiquitin ligase, plays a broad role in signaling cascades mediated by tumor necrosis factor receptors (TNFRs) and Toll-like receptors (TLRs)/IL-1receptors (IL-1Rs) (Medvedev et al., 2015). Silencing Peli1 specifically in cardiomyocytes (CMs) can improve left ventricular fibrosis and cardiac dysfunction following myocardial infarction (Wu et al., 2014). Tang et al. (2023) discovered that Peli1 promotes myocardial fibrosis through cardiomyocyte-derived exosomes enriched with miR-494–3p in the HF model induced by pressure overload, offering a potential exosome miRNA-based treatment for cardiac fibrosis. In another exosome miRNA study. Wang Q. G. et al. (2021) identified a significant correlation between clinical markers of CHF and serum miR-320a levels. In contrast to exosomes derived from healthy individuals, exosomes extracted from patients with CHF exhibited a marked elevation in miR-320a expression. Subsequently, embryonic fibroblast-like cells (HEH2) were treated with miR-320a mimics and inhibitors. The investigation revealed that miR-320a from serum exosomes could enhance the growth of cardiac fibroblasts via the PIK3CA/Akt/mTOR signaling pathway in HEH2 cells. This finding suggests that miR-320a within serum exosomes may serve as a promising diagnostic indicator for CHF.
MiR-24–3p functions as a tumor suppressor and is involved in various cancer processes (He et al., 2020; Ye et al., 2016). Additionally, miR-24–3p reduces I/R-induced cardiomyocyte apoptosis and is associated with collagen synthesis in cardiac fibrosis (Yang et al., 2022). Prohibitin 2 (PHB2), an autophagy receptor located on the inner mitochondrial membrane, has been identified as a direct target of miR-24–3p. This miRNA mitigates cardiac fibrosis by suppressing autophagy in cardiac fibroblasts through the downregulation of PHB2 (Zhang et al., 2022b). Reduced levels of miR-135a correlate with the proliferation and invasiveness of cancer cells (Dang et al., 2014). This miRNA is also implicated in regulating the expression of the sodium-calcium exchanger, thereby influencing cardiac electrical activity (Duong et al., 2017). MiR-135a also demonstrate a potential link to cardiac fibrosis. In vitro experiments show that miR-135a expression is significantly reduced after ISOP treatment of neonatal rat cardiac fibroblasts. Furthermore, miR-135a mimics inhibit cardiac fibroblast proliferation and differentiation by suppressing transient receptor potential melastatin 7 (TRPM7) expression and its associated currents In vivo experiments indicate that, after ISOP-induced cardiac fibrosis in adult SD rats, TRPM7 expression is upregulated, while miR-135a expression is downregulated in cardiac tissue (Wu et al., 2018). miR-135a inhibits ISOP-induced cardiac fibrosis by regulating the TRPM7-mediated collagen production pathway, indicating its protective role in modulating cardiac fibrosis.
Pathological cardiac hypertrophy represents the heart’s adaptive mechanism in response to increased stress, which can arise from various cardiovascular afflictions, including valvular heart disease, hypertension, and myocardial infarction. Although initially beneficial in normalizing wall tension and maintaining cardiac output, persistent hypertrophy can lead to a decline in cardiac function, ultimately resulting in HF (Nakamura and Sadoshima, 2018; Shimizu and Minamino, 2016). Pathological cardiac hypertrophy is recognized as a precursor and risk factor for HF. Therefore, timely prevention of hypertrophy has the potential to slow the transition to HF and improve clinical outcomes. In recent years, significant progress has been made in understanding the molecular mechanisms underlying cardiac hypertrophy. Currently, miRNAs are recognized as a major class of epigenetic regulators that exert a vital influence on the progression of cardiac hypertrophy (Ding et al., 2021).
Elevated levels of miRNA-212 in cardiomyocytes have been shown to induce hypertrophy, while reducing miR-212 can partially reverse this cellular hypertrophy. Additionally, miR-212 targets the rat transcription factor 7-like protein 2 (TCF7L2) and suppresses its expression, potentially promoting cardiomyocyte hypertrophy via this pathway (Yuan and Yuan, 2022). Furthermore, miR-214 induces mitochondrial dysfunction by targeting Sirtuin 3 (SIRT3) and is involved in myocardial hypertrophy incited by angiotensin II (Ang II) in mice. This suggests miR-214 may serve as a viable target for developing interventions aimed at treating cardiac hypertrophy (Ding et al., 2021).
Recently, miR-27a-3p has gained recognition for its significant role in cardiovascular diseases. Research indicates that miR-27a-3p can alleviate damage to heart muscle cells caused by both hypoxia/reoxygenation and exposure to lipopolysaccharide (Lozano-Velasco et al., 2015; Zhang X. L. et al., 2019). Furthermore, it is intricately linked to the development of obesity, ventricular formation, and various cardiac functions (Sassoon et al., 2016). Cao et al. (2021) discovered that in the cardiomyocyte hypertrophy model stimulated by Ang II, both miR-27a-3p and hypertrophy-associated genes were markedly overexpressed. Inhibitors of miR-27a-3p were shown to mitigate both electrical remodeling and myocardial hypertrophy. Additionally, miR-27a-3p can directly target the 3′-UTR of the Hoxa10 gene to regulate its expression at the transcriptional level. Elevated levels of Hoxa10 have been shown to counteract the electrical remodeling effects and myocardial hypertrophy induced by Ang II in cardiac cells. Hoxa10 promotes the upregulation of the potassium channel protein Kv4.3, typically suppressed in hypertrophic cardiomyocytes. Therefore, the pathway involving miR-27a-3p, Hoxa10, and Kv4.3 represents a newly discovered regulatory mechanism crucial to myocardial cell hypertrophy triggered by Ang II, providing a novel target for the clinical strategies aimed at preventing and treating cardiac hypertrophy and HF.
Studies have shown that the NFATc3 (NFAT4)-dependent pathway of the activated T-cell nuclear factor (NFAT) subtype is related to the progression of an enlarged heart muscle (Chao et al., 2019). NFATc3 activates the expression of miR-153–3p, while Suppressing NFATc3 leads to a reduction in miR-153–3p levels and fosters both mitochondrial division and cardiomyocyte enlargement by impeding the translation of the mitochondrial outer membrane protein (Mfn1) (Wang et al., 2020). During Ang II-induced HF, heat shock factor 2 (HSF2) activates the signaling pathway of the insulin-like growth factor II receptor (IGF-IIR), inducing cardiac hypertrophy. In spontaneously hypertensive rats (SHR), HSF2 expression is predominantly regulated by miR-18 and significantly diminished upon the activation of the p53 protein in cardiac tissue. Meanwhile, the absence of miR-18 in cardiac tissue significantly hampers heart performance, primarily through mechanism involving IGF-IIR-induced cardiac enlargement. When miR-18 is overexpressed in cardiomyocytes, it can protect against cardiac hypertrophy and preserve cardiac function. This experimental evidence suggests that miR-18 may serve as a treatment target to regulate cardiac function and alleviate cardiomyopathy during hypertension-induced HF (Huang et al., 2017). Furthermore, a heart-related circular RNA (HRCR) shields the myocardium against pathological hypertrophy and HF by engaging with miR-223 while boosting the expression of the heart-specific anti-apoptotic protein (ARC). this finding reveals a new regulatory pathway involving ARC, miR-223, and HRCR (Wang et al., 2016).
Myocarditis presents histologically and clinically as diverse pathological immunological responses within cardiac tissue, although the etiology and pathogenesis remain unclear (Sagar et al., 2012). It is characterized by an absence of definitive diagnostic approaches and a lack of effective therapeutic options (Heymans et al., 2016). When certain factors—such as infectious pathogens, toxins, drugs, and autoimmune diseases—cause myocarditis, the inflammation may subside on its own or further evolve into dilated cardiomyopathy, potentially leading to acute HF (Caforio et al., 2015). Recent investigations have demonstrated that miRNAs play a role in both the causation and progression of myocarditis (Zhou et al., 2018). In recent years, advancements in molecular technology have allowed for a detailed analysis of the miRNA profile in myocarditis, revealing dysregulated miRNAs along with their corresponding mRNA and protein targets in both cardiac tissue samples (intracellular miRNA) and systemic fluids (known as circulating miRNA) (Fung et al., 2016). Dysregulated miRNAs in myocarditis exhibit phase-dependent changes closely related to cardiac function, arrhythmias, cardiomyocyte destruction, fibrosis, immune status, viral infection, and disease outcomes. Therefore, miRNAs represent potential targets for the diagnosis and treatment of myocarditis.
Heart macrophage infiltration is enhanced by cardiac extracellular vesicles (IR-EV), with miRNA-155–5p encapsulated within these IR-EVs potentially acting as an effector. IR-EVs transport miR-155–5p into macrophages, amplifying inflammatory responses by activating the JAK2/STAT1 signaling pathway. This interaction not only fosters localized inflammation in cardiac tissue but also triggers a systemic inflammatory response in distant organs. Therefore, Manipulating the IR-EVs-miR-155-5p-M1 signaling pathway through targeted offers potential for reducing inflammation and protecting the cardiovascular system(Ge et al., 2021). MiR-330–5p shows distinct expression patterns in both the brain and myocardial ischemia-reperfusion (I/R) injury, suggesting its potential as a biomarker for I/R-associated diseases(Wang L. et al., 2021). The inflammatory response triggered by I/R injury is mediated by the NOD-like receptor protein-3 (NLRP3) inflammasome signaling pathway. Evidence suggests miR-330–5p can mitigate myocardial I/R injury and inflammation by inhibiting the activation of this pathway (Zuo et al., 2021). MiR-532–3p plays a regulatory role in metabolic disorders like inflammation and obesity, and contributes to atherosclerotic thrombosis, which can lead to HF(Bauersachs et al., 2019; Huang et al., 2020). Long-chain non-coding RNA (lncRNA) metastasis-associated lung adenocarcinoma transcript 1 (MALAT1) competes with miR-532–3p, upregulating low-density lipoprotein receptor (LDLR) protein and worsening HF pathology. Suppression of MALAT1 can alleviate myocardial injury and inflammation in HF patients, while inhibiting miR-532–3p may reduce MALAT1’s protective effect on H9C2 cardiomyocyte injury. Additionally, miR-145–5p is a key miRNA involved in inflammation regulation, and taurine-upregulated gene 1 (TUG1) is a non-coding RNA linked to inflammation. MiR-145–5p reduces hypoxia-induced inflammatory in cardiac cells by directly regulating lncRNA TUG1 (Yuan et al., 2017). In CHF patients, miR-145–5p is downregulated, while TUG1 is significantly upregulated. Moreover, miR-145–5p and TUG1 closely linked to inflammatory markers such as TNF-α, IL-6, and CRP, indicating that the TUG1/miR-145–5p interaction is closely related to CHF inflammation and progression. This interaction may offer novel therapeutic targets for CHF treatment(Zhu et al., 2021).
Currently, therapeutic approaches based on miRNA have garnered significant attention in preclinical research. Numerous studies have shown that CHM compound formulas and their active ingredients can regulate the expression of miRNAs through various mechanisms (Li et al., 2021). For instance, commonly used CHM compound formulas, including Huiyangjiuji decoction (HYJJ), Fufang Zhenzhu Tiaozhi (FTZ), and Lingguizhugan decoction (LGZG) have been confirmed to regulate the expression of miRNAs associated with cardiovascular diseases (as shown in Table 2). Additionally, certain active ingredients of CHM, including Tan IIA, LBP and ginsenosides, have demonstrated potential therapeutic effects through the regulation of miRNA expression (as shown in Table 3). These CHMs and their active components enhance cardiovascular function through various pathways, demonstrating significant efficacy in treating HF. This affirmation not only underscores the therapeutic potential of CHM in addressing HF but also offers new perspectives and possibilities for miRNA-based therapeutic approaches.
The traditional Chinese medicinal formula HYJJ decoction has been shown to be effective in treating acute gastroenteritis and is also suitable for alleviating symptoms such as vomiting, diarrhea, and shock. In addressing cardiac conditions such as HF and myocardial infarction, HYJJ decoction has demonstrated therapeutic potential and can be utilized for managing various acute symptoms (Yu et al., 2021). Zhang H. et al. (2023) found that HYJJ decoction can rehabilitate cardiac performance in rats and prevent the programmed cell death of heart muscle cells in rats with CHF induced by Dox, thereby alleviating myocardial damage. Its mechanism of action may be related to the regulation of specific critical miRNAs or signaling pathways associated with CHF. In this study, differentially expressed (DE) lncRNAs were identified by comparing the expression levels of lncRNAs among the HYJJ-treated, model, and control groups. RNA sequencing and bioinformatics analyses revealed that, of the 548 DE lncRNAs, the expression levels of 511 miRNAs were significantly altered, with 90 DE miRNA genes identified in the HYJJ-treated rats. KEGG and Gene Ontology (GO) analyses indicated that the differentially expressed genes were primarily enriched in the adrenergic signaling pathway in cardiomyocytes, suggesting that HYJJ decoction may exert cardioprotective effects through this signaling pathway.
FTZ is well-known for its multi-target characteristics and is widely used in China to manage hyperlipidemia and metabolic syndrome (Hu et al., 2014). FTZ has demonstrated significant effects in improving cardiovascular diseases, particularly in cases of heart muscle damage in models of long-term ischemia (Song et al., 2021), acute myocardial ischemia-reperfusion (MIR) (Qiao and Xu, 2016), and HF caused by myocardial hypertrophy. In the study conducted Zhang et al. (2022a) to explore the underlying mechanism behind FTZ’s anti-hypertrophic properties, miRNAs associated with hypertrophy, such as miR-181a, miR-206, and miR-214, were identified. The findings suggested that FTZ reduced the expression of miR-214, concurrently leading to an upregulation of the intrinsic levels of SIRT3. FTZ has the potential to mitigate HF caused by pressure-induced cardiac hypertrophy by inactivating the miR-214/SIRT3 signaling pathway.
YiQiFuMai injection (YQFM), which is derived from the traditional prescription Sheng Mai San. Extensive clinical trials have demonstrated that the application of YQFM injection in the management of CHF is both effective and associated with minimal adverse effects (Li F. et al., 2019). For example, the active constituents of YQFM injection can mitigate myocardial damage caused by HF and hypoxia. The protective influence is achieved by suppressing the NF-κB signaling cascade and the production of cytokines (Zhang Y. et al., 2019). Furthermore, YQFM injection regulates the MAPK signaling pathway, thereby diminishing myocardial remodeling and HF triggered by coronary artery ligation (CAL) (Pang et al., 2017). However, limited literature exists regarding the heart-protecting benefits of YQFM injection through miRNA regulation. Zhao et al. (2018) found that YQFM injection regulates the expression of specific miRNAs. This includes the downregulation of miR-702–5p, miR-466c-5p, and miR-219a-2-3p, along with the upregulation of miR-542–3p, miR-381–3p, miR-216b-5p and miR-21–3p. This regulatory effect effectively improves the fractional shortening of the left ventricle (LVFS) and the left ventricular ejection fraction (LVEF), reduces the left ventricular size, and increase cardiac output, thereby providing protective benefits to the cardiac tissue.
LongShengZhi (LSZ) capsule is a carefully selected traditional Chinese medicine preparation, meticulously formulated from various herbal ingredients and animal medicinal materials. This complex formula endows LSZ capsule with significant therapeutic value in treating cardiovascular diseases, particularly in alleviating atherosclerosis (Ma et al., 2019), carrageenan-induced thrombosis (Li Q. et al., 2019), and doxorubicin-induced HF (Xu et al., 2020). Research has shown that LSZ capsule exerts a protective influence against cardiac hypertrophy and fibrosis induced by Ang II in rats, and this protective impact is associated with the expression levels of miR-150–5p. In the heart disease model induced by Ang II, the expression of miR-150–5p is diminished, whereas LSZ capsule is capable of preserving its expression levels. Overexpressing miR-150–5p in vivo alleviates cardiac fibrosis and hypertrophy caused by Ang II, and reverses the upregulation of biomarkers associated with heart disease (ANP, BNP, β-MHC). Additionally, the gene encoding matrix metalloproteinase 14 (MMP14) is regulated by miR-150–5p, establishing it as a molecular target. Its overexpression promotes remodeling of cardiac muscle and counteracts the protective effect of miR-150–5p on cardiac hypertrophy and fibrosis (Gu et al., 2022).
Qiliqiangxin (QLQX) capsule is a formula composed of 11 ancient Chinese medicinal herbs that have been extensively utilized in treating chronic HF and have demonstrated significant efficacy (Tang and Huang, 2013; Zou et al., 2012). Sun et al. (2020) revealed the mechanism of action of QLQX capsule, finding that QLQX capsule inhibits the TGF-β1/Smad3 signaling pathway by upregulating miR-345–3p and promotes the activation of the TGF-β3/Smad7 signaling pathway, thereby reducing myocardial remodeling and fibrosis. This discovery provides a new perspective on the use of QLQX capsule for treating cardiac fibrosis. Zhang et al. (2016) found that the elevated levels of miR-199a-5p significantly mitigate the anti-hypertrophic effect of QLQX capsule on cardiomyocytes, manifesting as increased cardiomyocyte volume, elevated hypertrophic markers, and enhanced protein synthesis. These results emphasize the critical role of miR-199a-5p in cardiac hypertrophy and suggests that the downregulation of miR-199a-5p may aid in treating cardiac hypertrophy, potentially contributing to the mechanism by which QLQX capsule exerts its cardioprotective effect. Among the most abundant miRNAs in healthy myocardial tissue, miR-133a emerges as a crucial contributor to a wide array of biological processes. These processes include those critical to heart function, such as growth, cell proliferation, and the development of distinct cell types (Puthanveetil and O'Hagan, 2022). Upregulation of miR-133a can inhibit apoptosis of cardiomyocytes triggered by hypoxic conditions in the myocardium. Research findings indicate that QLQX capsule can significantly increase the expression of miR-133a while simultaneously suppressing glucose-regulated protein 78 (GRP78) expression in the heart, and inhibiting overactivated endoplasmic reticulum stress (ERS), thereby reducing apoptosis of myocardial cells (Ji et al., 2024).
LGZG decoction is a time-honored herbal prescription derived from the ancient medical text Synopsis of Prescriptions for the Golden Chamber. It is effective in regulating Spleen Yang deficiency and resolving phlegm and dampness, and is commonly used to treat diseases caused by phlegm retention, including chronic congestive HF (Liu et al., 2013). According to the principles of TCM, phlegm retention and Spleen Yang deficiency are considered important etiologies of HF; thus, LGZG decoction has a long history of use in the management of cardiovascular diseases. Li X. et al. (2019) found that LGZG decoction positively impacts the remodeling of cardiac microstructure by suppressing miR-24 expression and enhancing Junctophilin-2 (JP-2) expression, thereby alleviating Doxorubicin-induced HF. These findings reveal the potential mechanism by which LGZG decoction improves HF, providing experimental support for its use a treatment for cardiovascular diseases.
Shenfu injection is a representative formula of traditional Chinese medicine used for replenishing Qi and warming Yang. This formula has been used in traditional practice for millennia and has demonstrated potential efficacy in the treatment of HF (Tao et al., 2023). The primary active ingredients of Panax ginseng C.A. Meyer [Araliaceae; Ginseng Radix] and Aconitum carmichaeli Debeaux [Ranunculaceae; Aconiti Carmichaeli Radix], with ginsenoside exerting a significant effect on regulating apoptosis (Peng et al., 2023). Yan et al. (2015) found that ginsenoside Rb1 can reverse the increase in miR-1, and the associated cell death caused by hypoxia and ischemia (H/I) in neonatal rat cardiomyocytes (NRCMs). Furthermore, Yan et al. (2018) discovered that Shenfu injection significantly impacts miRNAs related to cell apoptosis in HF rats (including miR-320, miR-21, miR-208, and miR-29), suggesting that Shenfu injection may balance the activation and inhibition of cell apoptosis by regulating these miRNAs, ultimately inhibiting cell apoptosis and improving cardiac function of HF rats. Mao et al. (2018) indicated that Shenfu injection can alleviate myocardial hypertrophy and reduce the mortality rate of HF and other cardiovascular conditions by modulating the concentration of miR-19a-3p, reducing the levels of myocyte enhancer factor 2A (MEF2A) mRNA and protein, and regulating proteins associated with the MEF2 signaling pathway.
Danshen, a well-known Chinese herb, contains active ingredients extracted from its dry roots and rhizomes that have shown substantial effects on addressing cardiovascular conditions. The active ingredients of Danshen include Tan IIA, tanshinone I, salvianolic acid, and dihydrotanshinone, with Tan IIA being the predominant component (Weng et al., 2015). Clinical applications indicate that Tan IIA can treat cardiovascular issues such as HF caused by cardiac hypertrophy. Its mechanism of action include inducing apoptosis of cardiomyocytes, inhibiting the hypertrophy, alleviating cardiac fibrosis and limiting oxidative stress, thereby improving cardiac function (Chan et al., 2011; Tan et al., 2011). Feng et al. (2012) revealed that Tan IIA can diminish myocardial apoptosis in HF rats by upregulating the level of miR-133. This discovery illustrates how Tan IIA protects the myocardium at the molecular level. Song et al. (2017) further found that Tan IIA can markedly reverse the downregulation of miR-133 under adverse conditions, inhibit the expression of Caspase-9 along with associated apoptotic mediators, and effectively reduce myocardial apoptosis by suppressing the Caspase-9 signaling pathway while promoting the synthesis of miR-133, aiding in the repair of myocardial injury. Additionally, Gu et al. (2016) found that Tan IIA can protect H9C2 heart cells from cell death induced by oxidative stress. The involvement of miR-133 and Akt pathways highlights the role of Tan IIA as a natural cardioprotective agent, showcasing its significant potential.
Lycium barbarum L., a prominent traditional Chinese herb and functional food, not only has a rich historical background in China but is also gaining popularity in North America and Europe. It is believed to nourish the liver, kidneys, and eyes while offering various health benefits (Yang et al., 2017). LBP are significant bioactive ingredients derived from lycium barbarum L., accounting for up to 40% of the dry weight of the fruit (Li et al., 2011). LBP have been scientifically proven possess various pharmacological and biological functions, including but not limited to antioxidant properties (Varoni et al., 2017), immune regulation (Bo et al., 2016), anti-tumor activity (Zhang et al., 2017), anti-aging effects(Yi et al., 2013), neuroprotection (Li et al., 2011), blood sugar and lipid reduction (Xiao et al., 2014), and promotion of male fertility (Qian and Yu, 2016). These functions demonstrate the broad application potential of LBP in preventing and treating various cardiovascular illnesses. Notably, LBP exhibit cardioprotective effects. Research has shown that LBP can reduce myocardial apoptosis and injury during I/R in rat hearts, thereby helping to prevent cardiovascular diseases. Zhang R. et al. (2018) found that LBP can restore cardiac function in a miR-1 transgenic mouse model resulting from the overexpression of miR-1. The underlying mechanism may involve LBP downregulating miR-1 expression, thereby reversing the inhibition of target proteins related to myocardial contractility induced by miR-1. This finding strongly suggests that LBP have protective effects on cardiac conduction function, providing a scientific basis for their use in treating heart conditions.
Ginseng (Panax ginseng C.A. Meyer), a well-known traditional Chinese herb, has been utilized since ancient times. Ginsenosides, the main active ingredient, possess a broad spectrum of pharmacological properties, including alleviation of neuroinflammation (Cai and Yang, 2016), inhibition of vascular smooth muscle cell proliferation (Gao et al., 2019), the reduction of oxidative stress and inflammation (Lee et al., 2020). Importantly, ginsenosides also exert numerous beneficial pharmacological impacts on the cardiovascular system, including angiogenic, anti-arrhythmic, and anti-ischemic effects (Cai and Yang, 2016). Research indicates that ginsenoside Re can diminish intrinsic rhythmicity and cardiac contractility, depending on dosage. This effect is attributed to its ability to enhance nitric oxide (NO) production from L-arginine and inhibit calcium (Ca2+) channel activity in endothelial cells of blood vessels (Kim et al., 2015). Additionally, ginsenosides exert heart-protective effects by regulating miRNAs. In the I/R rat model, miR-144–3p expression levels rose significantly. Excessive expression of miR-144–3p increased the ROS levels in H9C2 cells, making them sensitive to ferroptosis induced by Erastin, a ferroptosis activator. Ginsenoside Re can suppress miR-144–3p expression, leading to elevated levels of its target protein, cystine/glutamate antiporter SLC7A11, thereby inhibiting myocardial cell ferroptosis and alleviating cardiac damage caused by I/R (Ye et al., 2023). A study identified miR-489 as a key factor in myocardial cell fibrosis. It can directly target Myd88 and influence the processes of cardiac hypertrophy and fibrosis, which are regulated through the NF-κB signaling pathway. Ginsenoside Re can elevate miR-489 expression and suppress activation of the Myd88/NF-κB signaling pathway, thereby inhibiting myocardial fibrosis development (Sun et al., 2023). Another study found that ginsenoside Rb2 can reduce HF-induced myocardial remodeling, maintain cardiomyocyte morphology integrity, and restore cellular vitality (Peng et al., 2023). In this research, rats with HF and myocardial cells exposed to oxygen-glucose deprivation followed by reperfusion (OGD/r) exhibited a significant increase in miR-216a-5p expression. Ginsenoside Rb2 intervention could reduce miR-216a-5p activity and upregulate Beclin1, LC3B II/I, and Bcl2 expression in myocardial cells while downregulating Caspase-3, Bax, and p62 levels. Conversely, upregulation of miR-216a-5p enhances apoptosis and induce oxidative stress in cardiomyocytes. Concurrently, it suppressed autophagy, thereby neutralizing the beneficial effects of ginsenoside Rb2 on HF in both in vitro and in vivo settings.
Sanqi, also known as P. notoginseng, is an herbal medicine with a rich history usage. The primary bioactive components of P. notoginseng are its saponins, collectively referred to as PNS. This complex chemical composition is rich in various dammarane-type saponins (Xu et al., 2019). Extensive research has demonstrated the broad applications of PNS in both medical studies and clinical practice, including diabetes mellitus, atherosclerosis, malignancies, and cardiovascular disorders (Uzayisenga et al., 2014). The cardioprotective mechanism of PNS is linked to their ability to modulate gene expression via miRNAs. miR-29 functions as an anti-fibrotic agent that interacts with the coding sequence of TGF-β. This interaction effectively suppresses the TGF-β signaling cascade, thereby alleviating myocardial fibrosis. In mouse cardiac tissue induced by ISOP, a marked decrease in the expression levels of miR-29 is observed. Treatment with PNS enhance cardiac expression levels of miR-29, simultaneously reduces the activity of the TGF-β signaling pathway, and reverses the myocardial damage and fibrosis induced by ISOP (Liu et al., 2017).
Derived from the desiccated rhizomes of the Paeonia lactiflora Pall, TGP constitute a key component of this Chinese herb. This compound exhibits a range of pharmacological effects, including immunomodulatory capabilities, as well as antioxidant and anti-inflammatory properties, demonstrating significant potential in addressing various cardiovascular conditions (Wei et al., 2024). Evidence indicates that TGP significantly reduces inflammation in patients with stable CHF by suppressing proinflammatory cytokine production, thereby delaying disease progression (Lin et al., 2020). In cardiomyocytes, TGP mitigates apoptosis and oxidative stress triggered by I/R by suppressing the PI3K/Akt axis (Shen et al., 2018). Furthermore, TGP protects cardiomyocytes from damage by regulating miRNA. Evidence suggests that in H/R cardiomyocytes, upregulation of miR-181a-5p expression is observed. In addition to suppressing miR-181a-5p expression, TGP also regulates the adenosine cyclase 1 (ADCY1) gene by directly targeting this miRNA. When miR-181a-5p is overexpressed or an ADCY1 inhibitor is applied, the suppressive effect of TGP on H/R-triggered pyroptosis in cardiomyocytes is diminished or reversed (Yan and Huang, 2021).
HF represents a serious clinical condition defined by the heart’s diminished capacity to efficiently circulate blood, thereby failing to adequately meet the body’s needs. HF is typically triggered by various cardiovascular conditions, including hypertension, coronary heart disease (CHD), and different types of cardiomyopathies (Lehnart, 2013; Savarese et al., 2023). Treatment of HF typically involves the use of beta-adrenergic receptor blockers, diuretics, and angiotensin-converting enzyme inhibitors (ACEIs). These medications can alleviate symptoms, prevent disease progression, and improve both the quality of life and survival odds for patients (Al-Mohammad and Mant, 2011). However, prolonged use of such pharmaceutical agents may lead to severe consequences, such as hypotension, fluid depletion, and electrolyte imbalances, thereby negatively impacting the overall health of patients (Gupta et al., 2014; Yancy et al., 2013). Modern medical advances have significantly contributed to the reduction of both the incidence and mortality rates associated with HF (Bozkurt and Mann, 2013); however, the economic and social burden of HF continues to rise. Therefore, exploring the pathogenesis of HF and developing new effective drugs with fewer side effects will be crucial for its prevention and treatment. CHM is emerging as a novel approach to the prevention and treatment of diseases due to its multi-target mechanisms of action and fewer side effects (Wang et al., 2017). Clinical assessments and laboratory investigations have demonstrated that CHM and its derived compounds have the potential to serve as effective treatments for HF (Li and Xu, 2013).
Studies have demonstrated that CHM can regulate pathophysiological processes in HF through a complex array of mechanisms. This includes effects on relevant signaling pathways, non-coding microRNAs, and stem cells. Through these regulatory effects, CHM exerts multiple biological impacts, including inhibition of apoptosis, antioxidant effects, anti-cardiac myocyte hypertrophy, anti-fibrotic effects, and anti-inflammatory effects, thereby demonstrating its unique advantages in the treatment of HF (Hao et al., 2017). CHM typically contain a variety of bioactive components that can act on multiple molecular targets, thereby modulating pathological processes at various levels. The multi-target action characteristic of CHM enables intervention in the disease process from various angles when treating HF, potentially leading to more comprehensive therapeutic effects compared to traditional single-target drug therapies. Additionally, CHM typically has fewer side effects, which is particularly important for patients with chronic diseases requiring long-term treatment. Moreover, the efficacy of CHM has been shown to be significant in many cases, providing tangible clinical benefits to patients (Wu et al., 2022). For instance, the QLQX Capsule improved the prognosis of patients with HF in a double-blind clinical trial, with results published in the prestigious international journal Nature Medicine, highlighting the significant value and potential of Chinese medicine in HF treatment (Cheang et al., 2024). However, despite these positive findings, clinical trials of CHM for the treatment of HF remain relatively limited, and the quality of these studies varies. This situation may be attributed to the inherent complexity of CHM research, challenges in clinical trial design, and the lack of standardized assessments of CHM efficacy, among other factors. In light of this, there is an urgent need for broader, multi-institutional, high-caliber controlled clinical studies, along with practical research. Simultaneously, to provide empirical guidance for the clinical utilization of CHM, it is imperative to conduct a more in-depth exploration of the specific targets and associated signaling pathways of CHM and its diverse bioactive constituents. Furthermore, a multidisciplinary approach is required, combining traditional Chinese medical theory with contemporary biomedical techniques to design more rigorous and scientifically sound clinical trials, thereby promoting the use of CHM for the treatment of HF.
MiRNAs influence a multitude of biological processes by modulating gene expression, including the onset and progression of heart diseases. In recent years, a deeper understanding of miRNAs in cardiovascular diseases has led to a growing body of research focusing on the effects of CHM on miRNAs, opening novel avenues and methodologies for addressing HF (Wu et al., 2023). For example, certain compounds and active components of CHM, such as HYJJ decoction, YQFM Injection, QLQX, Tan IIA, and LBP, are reported to exert cardioprotective effects through the modulation of specific miRNAs. Although existing research has progressed in revealing the regulatory effects of miRNAs in CHM on cardiovascular diseases, providing initial insights into the mechanisms of action, overall knowledge of the complex interactions and specific mechanisms of these miRNA molecules under different disease conditions remains limited. Additionally, since CHM consists of a variety of complex ingredients, focusing solely on a single miRNA or molecular target may not fully reveal its therapeutic potential. Therefore, it is crucial to delve into the miRNA network and its complex molecular mechanisms underlying the action of CHM, and focusing on specific miRNAs as targets could emerge as a promising avenue for addressing HF.
In summary, miRNAs exert a pivotal regulatory influence on the onset and progression of HF, and CHM has demonstrated significant therapeutic potential by regulating miRNA expression. Future research should further investigate the specific mechanisms of interaction between CHM and miRNA to develop new treatment strategies based on miRNA regulation. This will not only enrich treatment methods for HF but also provide new perspectives for personalized therapy.
YW: Writing–review and editing, Writing–original draft. JL: Writing–review and editing, Investigation. ZC: Writing–review and editing, Supervision, Methodology. LS: Writing–review and editing. YM: Writing–review and editing, Investigation. JW: Writing–review and editing, Supervision, Funding acquisition.
The author(s) declare that financial support was received for the research, authorship, and/or publication of this article. This work was supported by the NATCM’s Project of High-level Construction of Key TCM Disciplines (grant number zyyzdxk-2023113), the Cultivation of Key Traditional Chinese Medicine Disciplines—Vertigo, designated by Gancai Society [2023] No. 70, and Jiangxi Province Key Laboratory of Traditional Chinese Medicine for Cardiovascular Diseases (grant number 2024SSY06301).
The authors declare that the research was conducted in the absence of any commercial or financial relationships that could be construed as a potential conflict of interest.
The reviewer DL declared a shared affiliation with the author ZC to the handling editor at the time of review.
All claims expressed in this article are solely those of the authors and do not necessarily represent those of their affiliated organizations, or those of the publisher, the editors and the reviewers. Any product that may be evaluated in this article, or claim that may be made by its manufacturer, is not guaranteed or endorsed by the publisher.
The Supplementary Material for this article can be found online at: https://www.frontiersin.org/articles/10.3389/fphar.2024.1475975/full#supplementary-material
Al-Mohammad, A., and Mant, J. (2011). The diagnosis and management of chronic heart failure: review following the publication of the NICE guidelines. Heart 97 (5), 411–416. doi:10.1136/hrt.2010.214999
Al-Mohammad, A., Mant, J., Laramee, P., and Swain, S.Chronic Heart Failure Guideline Development Group (2010). Diagnosis and management of adults with chronic heart failure: summary of updated NICE guidance. Bmj 341, c4130. doi:10.1136/bmj.c4130
Amgalan, D., and Kitsis, R. N. (2019). A mouse model for the most common form of heart failure. Nature 568 (7752), 324–325. doi:10.1038/d41586-019-00983-4
Bauersachs, R., Zeymer, U., Brière, J. B., Marre, C., Bowrin, K., and Huelsebeck, M. (2019). Burden of coronary artery disease and peripheral artery disease: a literature review. Cardiovasc. Ther. 2019, 8295054. doi:10.1155/2019/8295054
Bo, R., Zheng, S., Xing, J., Luo, L., Niu, Y., Huang, Y., et al. (2016). The immunological activity of Lycium barbarum polysaccharides liposome in vitro and adjuvanticity against PCV2 in vivo. Int. J. Biol. Macromol. 85, 294–301. doi:10.1016/j.ijbiomac.2015.12.089
Bozkurt, B., and Mann, D. L. (2013). The treatment of heart failure in the 21st century: is the glass half empty or half full? Methodist Debakey cardiovasc. J. 9 (1), 3–5. doi:10.14797/mdcj-9-1-3
Caforio, A. L., Marcolongo, R., Basso, C., and Iliceto, S. (2015). Clinical presentation and diagnosis of myocarditis. Heart 101 (16), 1332–1344. doi:10.1136/heartjnl-2014-306363
Cai, M., and Yang, E. J. (2016). Ginsenoside re attenuates neuroinflammation in a symptomatic ALS animal model. Am. J. Chin. Med. 44 (2), 401–413. doi:10.1142/s0192415x16500233
Cao, X., Zhang, Z., Wang, Y., Shan, W., Wang, R., Mao, S., et al. (2021). MiR-27a-3p/Hoxa10 Axis regulates angiotensin II-induced cardiomyocyte hypertrophy by targeting Kv4.3 expression. Front. Pharmacol. 12, 680349. doi:10.3389/fphar.2021.680349
Chan, P., Liu, J. C., Lin, L. J., Chen, P. Y., Cheng, T. H., Lin, J. G., et al. (2011). Tanshinone IIA inhibits angiotensin II-induced cell proliferation in rat cardiac fibroblasts. Am. J. Chin. Med. 39 (2), 381–394. doi:10.1142/s0192415x11008890
Chao, C. N., Lai, C. H., Badrealam, K. F., Lo, J. F., Shen, C. Y., Chen, C. H., et al. (2019). CHIP attenuates lipopolysaccharide-induced cardiac hypertrophy and apoptosis by promoting NFATc3 proteasomal degradation. J. Cell Physiol. 234 (11), 20128–20138. doi:10.1002/jcp.28614
Cheang, I., Yao, W., Zhou, Y., Zhu, X., Ni, G., Lu, X., et al. (2024). The traditional Chinese medicine Qiliqiangxin in heart failure with reduced ejection fraction: a randomized, double-blind, placebo-controlled trial. Nat. Med. 30 (8), 2295–2302. doi:10.1038/s41591-024-03169-2
Chen, Y., Liu, Y., and Dorn, G. W. (2011). Mitochondrial fusion is essential for organelle function and cardiac homeostasis. Circ. Res. 109 (12), 1327–1331. doi:10.1161/circresaha.111.258723
Corsetti, G., Chen-Scarabelli, C., Romano, C., Pasini, E., Dioguardi, F. S., Onorati, F., et al. (2019). Autophagy and oncosis/necroptosis are enhanced in cardiomyocytes from heart failure patients. Med. Sci. Monit. Basic Res. 25, 33–44. doi:10.12659/msmbr.913436
Dang, Z., Xu, W. H., Lu, P., Wu, N., Liu, J., Ruan, B., et al. (2014). MicroRNA-135a inhibits cell proliferation by targeting Bmi1 in pancreatic ductal adenocarcinoma. Int. J. Biol. Sci. 10 (7), 733–745. doi:10.7150/ijbs.8097
Dassanayaka, S., Brittian, K. R., Jurkovic, A., Higgins, L. A., Audam, T. N., Long, B. W., et al. (2019). E2f1 deletion attenuates infarct-induced ventricular remodeling without affecting O-GlcNAcylation. Basic Res. Cardiol. 114 (4), 28. doi:10.1007/s00395-019-0737-y
Dawson, K., Wakili, R., Ordög, B., Clauss, S., Chen, Y., Iwasaki, Y., et al. (2013). MicroRNA29: a mechanistic contributor and potential biomarker in atrial fibrillation. Circulation 127 (14), 1466–1475. doi:10.1161/circulationaha.112.001207
Delbridge, L. M. D., Mellor, K. M., Taylor, D. J., and Gottlieb, R. A. (2017). Myocardial stress and autophagy: mechanisms and potential therapies. Nat. Rev. Cardiol. 14 (7), 412–425. doi:10.1038/nrcardio.2017.35
Ding, Y. Q., Zhang, Y. H., Lu, J., Li, B., Yu, W. J., Yue, Z. B., et al. (2021). MicroRNA-214 contributes to Ang II-induced cardiac hypertrophy by targeting SIRT3 to provoke mitochondrial malfunction. Acta. Pharmacol. Sin. 42 (9), 1422–1436. doi:10.1038/s41401-020-00563-7
Duong, E., Xiao, J., Qi, X. Y., and Nattel, S. (2017). MicroRNA-135a regulates sodium-calcium exchanger gene expression and cardiac electrical activity. Heart rhythm 14 (5), 739–748. doi:10.1016/j.hrthm.2017.01.045
Fan, H., Xie, X., Kuang, X., Du, J., and Peng, F. (2022). MicroRNAs, key regulators in glioma progression as potential therapeutic targets for Chinese medicine. Am. J. Chin. Med. 50 (7), 1799–1825. doi:10.1142/s0192415x22500768
Feng, J., Li, S. S., and Liang, Q. S. (2012). Effects of Tanshinone II A on the myocardial apoptosis and the miR-133 levels in rats with heart failure. Zhongguo Zhong Xi Yi Jie He Za Zhi 32 (7), 930–933.
Frangogiannis, N. G. (2019). Cardiac fibrosis: cell biological mechanisms, molecular pathways and therapeutic opportunities. Mol. Asp. Med. 65, 70–99. doi:10.1016/j.mam.2018.07.001
Fung, G., Luo, H., Qiu, Y., Yang, D., and McManus, B. (2016). Myocarditis. Circ. Res. 118 (3), 496–514. doi:10.1161/circresaha.115.306573
Gao, Y., Zhu, P., Xu, S. F., Li, Y. Q., Deng, J., and Yang, D. L. (2019). Ginsenoside re inhibits PDGF-BB-induced VSMC proliferation via the eNOS/NO/cGMP pathway. Biomed. Pharmacother. 115, 108934. doi:10.1016/j.biopha.2019.108934
Gargiulo, P., Marzano, F., Salvatore, M., Basile, C., Buonocore, D., Parlati, A. L. M., et al. (2023). MicroRNAs: diagnostic, prognostic and therapeutic role in heart failure-a review. Esc. Heart Fail 10 (2), 753–761. doi:10.1002/ehf2.14153
Ge, X., Meng, Q., Wei, L., Liu, J., Li, M., Liang, X., et al. (2021). Myocardial ischemia-reperfusion induced cardiac extracellular vesicles harbour proinflammatory features and aggravate heart injury. J. Extracell. Vesicles 10 (4), e12072. doi:10.1002/jev2.12072
Gourdie, R. G., Dimmeler, S., and Kohl, P. (2016). Novel therapeutic strategies targeting fibroblasts and fibrosis in heart disease. Nat. Rev. Drug Discov. 15 (9), 620–638. doi:10.1038/nrd.2016.89
Gu, Y., Liang, Z., Wang, H., Jin, J., Zhang, S., Xue, S., et al. (2016). Tanshinone IIA protects H9c2 cells from oxidative stress-induced cell death via microRNA-133 upregulation and Akt activation. Exp. Ther. Med. 12 (2), 1147–1152. doi:10.3892/etm.2016.3400
Gu, Y., Zhang, S., Chen, X., Li, Y., and Liu, Y. (2022). LongShengZhi alleviated cardiac remodeling via upregulation microRNA-150-5p with matrix metalloproteinase 14 as the target. J. Ethnopharmacol. 291, 115156. doi:10.1016/j.jep.2022.115156
Gupta, A., Ghimire, G., and Hage, F. G. (2014). Guidelines in review: 2013 ACCF/AHA guideline for the management of heart failure. J. Nucl. Cardiol. 21 (2), 397–399. doi:10.1007/s12350-013-9832-x
Hao, P., Jiang, F., Cheng, J., Ma, L., Zhang, Y., and Zhao, Y. (2017). Traditional Chinese medicine for cardiovascular disease: evidence and potential mechanisms. J. Am. Coll. Cardiol. 69 (24), 2952–2966. doi:10.1016/j.jacc.2017.04.041
He, L., Ping, F., Fan, Z., Zhang, C., Deng, M., Cheng, B., et al. (2020). Salivary exosomal miR-24-3p serves as a potential detective biomarker for oral squamous cell carcinoma screening. Biomed. Pharmacother. 121, 109553. doi:10.1016/j.biopha.2019.109553
Heymans, S., Eriksson, U., Lehtonen, J., and Cooper, L. T. (2016). The quest for new approaches in myocarditis and inflammatory cardiomyopathy. J. Am. Coll. Cardiol. 68 (21), 2348–2364. doi:10.1016/j.jacc.2016.09.937
Hinderer, S., and Schenke-Layland, K. (2019). Cardiac fibrosis - a short review of causes and therapeutic strategies. Adv. Drug Deliv. Rev. 146, 77–82. doi:10.1016/j.addr.2019.05.011
Hu, X., Wang, M., Bei, W., Han, Z., and Guo, J. (2014). The Chinese herbal medicine FTZ attenuates insulin resistance via IRS1 and PI3K in vitro and in rats with metabolic syndrome. J. Transl. Med. 12, 47. doi:10.1186/1479-5876-12-47
Huang, C. Y., Pai, P. Y., Kuo, C. H., Ho, T. J., Lin, J. Y., Lin, D. Y., et al. (2017). p53-mediated miR-18 repression activates HSF2 for IGF-IIR-dependent myocyte hypertrophy in hypertension-induced heart failure. Cell Death Dis. 8 (8), e2990. doi:10.1038/cddis.2017.320
Huang, R., Cao, Y., Li, H., Hu, Z., Zhang, H., Zhang, L., et al. (2020). miR-532-3p-CSF2RA Axis as a key regulator of vulnerable atherosclerotic plaque formation. Can. J. Cardiol. 36 (11), 1782–1794. doi:10.1016/j.cjca.2019.12.018
Iyngkaran, P., Liew, D., Neil, C., Driscoll, A., Marwick, T. H., and Hare, D. L. (2018). Moving from heart failure guidelines to clinical practice: gaps contributing to readmissions in patients with multiple comorbidities and older age. Clin. Med. Insights Cardiol. 12, 1179546818809358. doi:10.1177/1179546818809358
Ji, X. D., Yang, D., Cui, X. Y., Lou, L. X., Nie, B., Zhao, J. L., et al. (2024). Mechanism of qili qiangxin capsule for heart failure based on miR133a-endoplasmic reticulum stress. Chin. J. Integr. Med. 30 (5), 398–407. doi:10.1007/s11655-024-3654-3
Jia, Q., Wang, L., Zhang, X., Ding, Y., Li, H., Yang, Y., et al. (2020). Prevention and treatment of chronic heart failure through traditional Chinese medicine: role of the gut microbiota. Pharmacol. Res. 151, 104552. doi:10.1016/j.phrs.2019.104552
Kim, Y. J., Zhang, D., and Yang, D. C. (2015). Biosynthesis and biotechnological production of ginsenosides. Biotechnol. Adv. 33 (6 Pt 1), 717–735. doi:10.1016/j.biotechadv.2015.03.001
Lakhani, H. V., Khanal, T., Gabi, A., Yousef, G., Alam, M. B., Sharma, D., et al. (2018). Developing a panel of biomarkers and miRNA in patients with myocardial infarction for early intervention strategies of heart failure in West Virginian population. PLoS One 13 (10), e0205329. doi:10.1371/journal.pone.0205329
Lampert, M. A., and Gustafsson Å, B. (2018). Balancing autophagy for a healthy heart. Curr. Opin. Physiol. 1, 21–26. doi:10.1016/j.cophys.2017.11.001
Lee, G. H., Lee, W. J., Hur, J., Kim, E., Lee, H. G., and Seo, H. G. (2020). Ginsenoside re mitigates 6-hydroxydopamine-induced oxidative stress through upregulation of GPX4. Molecules 25 (1), 188. doi:10.3390/molecules25010188
Lehnart, S. E. (2013). Understanding the physiology of heart failure through cellular and in vivo models-towards targeting of complex mechanisms. Exp. Physiol. 98 (3), 622–628. doi:10.1113/expphysiol.2012.068262
Li, F., Pang, L. Z., Zhang, L., Zhang, Y., Zhang, Y. Y., Yu, B. Y., et al. (2019a). YiQiFuMai powder injection ameliorates chronic heart failure through cross-talk between adipose tissue and cardiomyocytes via up-regulation of circulating adipokine omentin. Biomed. Pharmacother. 119, 109418. doi:10.1016/j.biopha.2019.109418
Li, Q., Chen, Y., Zhao, D., Yang, S., Zhang, S., Wei, Z., et al. (2019b). LongShengZhi Capsule reduces carrageenan-induced thrombosis by reducing activation of platelets and endothelial cells. Pharmacol. Res. 144, 167–180. doi:10.1016/j.phrs.2019.04.013
Li, S., and Xu, H. (2013). Integrative Western and Chinese medicine on coronary heart disease: where is the orientation? Evid. Based Complement. Altern. Med. 2013, 459264. doi:10.1155/2013/459264
Li, S. Y., Yang, D., Yeung, C. M., Yu, W. Y., Chang, R. C., So, K. F., et al. (2011). Lycium barbarum polysaccharides reduce neuronal damage, blood-retinal barrier disruption and oxidative stress in retinal ischemia/reperfusion injury. PLoS One 6 (1), e16380. doi:10.1371/journal.pone.0016380
Li, X., Li, L., Lei, W., Chua, H. Z., Li, Z., Huang, X., et al. (2021). Traditional Chinese medicine as a therapeutic option for cardiac fibrosis: pharmacology and mechanisms. Biomed. Pharmacother. 142, 111979. doi:10.1016/j.biopha.2021.111979
Li, X., Xu, G., Wei, S., Zhang, B., Yao, H., Chen, Y., et al. (2019c). Lingguizhugan decoction attenuates doxorubicin-induced heart failure in rats by improving TT-SR microstructural remodeling. BMC Complement. Altern. Med. 19 (1), 360. doi:10.1186/s12906-019-2771-6
Li, Y., Shen, Y., Yao, C. L., and Guo, D. A. (2020). Quality assessment of herbal medicines based on chemical fingerprints combined with chemometrics approach: a review. J. Pharm. Biomed. Anal. 185, 113215. doi:10.1016/j.jpba.2020.113215
Lin, S. S., Liu, C. X., Zhang, J. H., Wang, X. L., and Mao, J. Y. (2020). Efficacy and safety of oral Chinese patent medicine combined with conventional therapy for heart failure: an overview of systematic reviews. Evid. Based Complement. Altern. Med. 2020, 8620186. doi:10.1155/2020/8620186
Liu, L., Ning, B., Cui, J., Zhang, T., and Chen, Y. (2017). miR-29c is implicated in the cardioprotective activity of Panax notoginseng saponins against isoproterenol-induced myocardial fibrogenesis. J. Ethnopharmacol. 198, 1–4. doi:10.1016/j.jep.2016.12.036
Liu, M. N., Luo, G., Gao, W. J., Yang, S. J., and Zhou, H. (2021). miR-29 family: a potential therapeutic target for cardiovascular disease. Pharmacol. Res. 166, 105510. doi:10.1016/j.phrs.2021.105510
Liu, Q., Peng, F., and Chen, J. (2019). The role of exosomal MicroRNAs in the tumor microenvironment of breast cancer. Int. J. Mol. Sci. 20 (16), 3884. doi:10.3390/ijms20163884
Liu, T., Yang, L. L., Zou, L., Li, D. F., Wen, H. Z., Zheng, P. Y., et al. (2013). Chinese medicine formula lingguizhugan decoction improves Beta-oxidation and metabolism of Fatty Acid in high-fat-diet-induced rat model of Fatty liver disease. Evid. Based Complement. Altern. Med. 2013, 429738. doi:10.1155/2013/429738
Lozano-Velasco, E., Galiano-Torres, J., Jodar-Garcia, A., Aranega, A. E., and Franco, D. (2015). miR-27 and miR-125 distinctly regulate muscle-enriched transcription factors in cardiac and skeletal myocytes. Biomed. Res. Int. 2015, 391306. doi:10.1155/2015/391306
Ma, J., Zhao, D., Wang, X., Ma, C., Feng, K., Zhang, S., et al. (2019). LongShengZhi capsule reduces established atherosclerotic lesions in apoE-deficient mice by ameliorating hepatic lipid metabolism and inhibiting inflammation. J. Cardiovasc. Pharmacol. 73 (2), 105–117. doi:10.1097/fjc.0000000000000642
Mao, Z. J., Zhang, Q. L., Shang, J., Gao, T., Yuan, W. J., and Qin, L. P. (2018). Shenfu Injection attenuates rat myocardial hypertrophy by up-regulating miR-19a-3p expression. Sci. Rep. 8 (1), 4660. doi:10.1038/s41598-018-23137-4
Medvedev, A. E., Murphy, M., Zhou, H., and Li, X. (2015). E3 ubiquitin ligases Pellinos as regulators of pattern recognition receptor signaling and immune responses. Immunol. Rev. 266 (1), 109–122. doi:10.1111/imr.12298
Mi, S., Huang, F., Jiao, M., Qian, Z., Han, M., Miao, Z., et al. (2023). Inhibition of MEG3 ameliorates cardiomyocyte apoptosis and autophagy by regulating the expression of miRNA-129-5p in a mouse model of heart failure. Redox Rep. 28 (1), 2224607. doi:10.1080/13510002.2023.2224607
Michlewski, G., and Cáceres, J. F. (2019). Post-transcriptional control of miRNA biogenesis. Rna 25 (1), 1–16. doi:10.1261/rna.068692.118
Mizushima, N., and Levine, B. (2010). Autophagy in mammalian development and differentiation. Nat. Cell Biol. 12 (9), 823–830. doi:10.1038/ncb0910-823
Nakamura, M., and Sadoshima, J. (2018). Mechanisms of physiological and pathological cardiac hypertrophy. Nat. Rev. Cardiol. 15 (7), 387–407. doi:10.1038/s41569-018-0007-y
Pang, L. Z., Ju, A. C., Zheng, X. J., Li, F., Song, Y. F., Zhao, Y., et al. (2017). YiQiFuMai Powder Injection attenuates coronary artery ligation-induced myocardial remodeling and heart failure through modulating MAPKs signaling pathway. J. Ethnopharmacol. 202, 67–77. doi:10.1016/j.jep.2017.02.032
Pattison, J. S., Osinska, H., and Robbins, J. (2011). Atg7 induces basal autophagy and rescues autophagic deficiency in CryABR120G cardiomyocytes. Circ. Res. 109 (2), 151–160. doi:10.1161/circresaha.110.237339
Peng, Y., Liao, B., Zhou, Y., and Zeng, W. (2023). Ginsenoside Rb2 improves heart failure by down-regulating miR-216a-5p to promote autophagy and inhibit apoptosis and oxidative stress. J. Appl. Biomed. 21 (4), 180–192. doi:10.32725/jab.2023.024
Puthanveetil, P., and O'Hagan, K. P. (2022). miR-133a-A potential target for improving cardiac mitochondrial health and regeneration after injury. J. Cardiovasc. Pharmacol. 80 (2), 187–193. doi:10.1097/fjc.0000000000001279
Qi, H., Liu, Y., Li, S., Chen, Y., Li, L., Cao, Y., et al. (2017). Activation of AMPK attenuated cardiac fibrosis by inhibiting CDK2 via p21/p27 and miR-29 family pathways in rats. Mol. Ther. Nucleic. Acids. 8, 277–290. doi:10.1016/j.omtn.2017.07.004
Qian, L., and Yu, S. (2016). Protective effect of polysaccharides from Lycium barbarum on spermatogenesis of mice with impaired reproduction system induced by cyclophosphamide. Am. J. Reprod. Immunol. 76 (5), 383–385. doi:10.1111/aji.12558
Qiao, Z., and Xu, Y. (2016). Salvianolic acid b alleviating myocardium injury in ischemia reperfusion rats. Afr. J. Tradit. Complement. Altern. Med. 13 (4), 157–161. doi:10.21010/ajtcam.v13i4.20
Sagar, S., Liu, P. P., and Cooper, L. T. (2012). Myocarditis. Lancet 379 (9817), 738–747. doi:10.1016/s0140-6736(11)60648-x
Sassoon, D. J., Goodwill, A. G., Noblet, J. N., Conteh, A. M., Herring, B. P., McClintick, J. N., et al. (2016). Obesity alters molecular and functional cardiac responses to ischemia/reperfusion and glucagon-like peptide-1 receptor agonism. Basic Res. Cardiol. 111 (4), 43. doi:10.1007/s00395-016-0563-4
Savarese, G., Becher, P. M., Lund, L. H., Seferovic, P., Rosano, G. M. C., and Coats, A. J. S. (2023). Global burden of heart failure: a comprehensive and updated review of epidemiology. Cardiovasc. Res. 118 (17), 3272–3287. doi:10.1093/cvr/cvac013
Savarese, G., and Lund, L. H. (2017). Global public health burden of heart failure. Card. Fail. Rev. 3 (1), 7–11. doi:10.15420/cfr.2016:25:2
Shen, P., Chen, J., and Pan, M. (2018). The protective effects of total paeony glycoside on ischemia/reperfusion injury in H9C2 cells via inhibition of the PI3K/Akt signaling pathway. Mol. Med. Rep. 18 (3), 3332–3340. doi:10.3892/mmr.2018.9335
Shimizu, I., and Minamino, T. (2016). Physiological and pathological cardiac hypertrophy. J. Mol. Cell Cardiol. 97, 245–262. doi:10.1016/j.yjmcc.2016.06.001
Siddall, H. K., Yellon, D. M., Ong, S. B., Mukherjee, U. A., Burke, N., Hall, A. R., et al. (2013). Loss of PINK1 increases the heart's vulnerability to ischemia-reperfusion injury. PLoS One 8 (4), e62400. doi:10.1371/journal.pone.0062400
Sinnenberg, L., and Givertz, M. M. (2020). Acute heart failure. Trends cardiovasc. Med. 30 (2), 104–112. doi:10.1016/j.tcm.2019.03.007
Song, L., Zhang, D., Guo, C., Gu, Z., Wang, L., Yao, Y. S., et al. (2021). The traditional Chinese medicine formula Fufang-Zhenzhu-Tiaozhi protects myocardia from injury in diabetic minipigs with coronary heart disease. Biomed. Pharmacother. 137, 111343. doi:10.1016/j.biopha.2021.111343
Song, T., Yao, Y., Wang, T., Huang, H., and Xia, H. (2017). Tanshinone IIA ameliorates apoptosis of myocardiocytes by up-regulation of miR-133 and suppression of Caspase-9. Eur. J. Pharmacol. 815, 343–350. doi:10.1016/j.ejphar.2017.08.041
Su, M., Wang, J., Wang, C., Wang, X., Dong, W., Qiu, W., et al. (2015). MicroRNA-221 inhibits autophagy and promotes heart failure by modulating the p27/CDK2/mTOR axis. Cell Death Differ. 22 (6), 986–999. doi:10.1038/cdd.2014.187
Sucharov, C. C., Kao, D. P., Port, J. D., Karimpour-Fard, A., Quaife, R. A., Minobe, W., et al. (2017). Myocardial microRNAs associated with reverse remodeling in human heart failure. JCI Insight 2 (2), e89169. doi:10.1172/jci.insight.89169
Sun, J., Wang, R., Chao, T., Peng, J., Wang, C., and Chen, K. (2023). Ginsenoside re inhibits myocardial fibrosis by regulating miR-489/myd88/NF-κB pathway. J. Ginseng Res. 47 (2), 218–227. doi:10.1016/j.jgr.2021.11.009
Sun, X., Chen, G., Xie, Y., Jiang, D., Han, J., Chen, F., et al. (2020). Qiliqiangxin improves cardiac function and attenuates cardiac remodelling in doxorubicin-induced heart failure rats. Pharm. Biol. 58 (1), 417–426. doi:10.1080/13880209.2020.1761403
Tan, X., Li, J., Wang, X., Chen, N., Cai, B., Wang, G., et al. (2011). Tanshinone IIA protects against cardiac hypertrophy via inhibiting calcineurin/NFATc3 pathway. Int. J. Biol. Sci. 7 (3), 383–389. doi:10.7150/ijbs.7.383
Tang, C., Hou, Y. X., Shi, P. X., Zhu, C. H., Lu, X., Wang, X. L., et al. (2023). Cardiomyocyte-specific Peli1 contributes to the pressure overload-induced cardiac fibrosis through miR-494-3p-dependent exosomal communication. Faseb J. 37 (1), e22699. doi:10.1096/fj.202200597R
Tang, W. H. W., and Huang, Y. (2013). Cardiotonic modulation in heart failure: insights from traditional Chinese medicine. J. Am. Coll. Cardiol. 62 (12), 1073–1074. doi:10.1016/j.jacc.2013.05.028
Tao, L., Mo, Z., Li, Z., Li, S., Luo, Z., Li, D., et al. (2023). Efficacy and safety of Shenfu injection on acute heart failure: a systematic review and meta-analysis. Phytomedicine 110, 154641. doi:10.1016/j.phymed.2023.154641
Thum, T., and Condorelli, G. (2015). Long noncoding RNAs and microRNAs in cardiovascular pathophysiology. Circ. Res. 116 (4), 751–762. doi:10.1161/circresaha.116.303549
Travers, J. G., Kamal, F. A., Robbins, J., Yutzey, K. E., and Blaxall, B. C. (2016). Cardiac fibrosis: the fibroblast awakens. Circ. Res. 118 (6), 1021–1040. doi:10.1161/circresaha.115.306565
Treiber, T., Treiber, N., and Meister, G. (2019). Regulation of microRNA biogenesis and its crosstalk with other cellular pathways. Nat. Rev. Mol. Cell Biol. 20 (1), 5–20. doi:10.1038/s41580-018-0059-1
Triposkiadis, F., Xanthopoulos, A., Parissis, J., Butler, J., and Farmakis, D. (2022). Pathogenesis of chronic heart failure: cardiovascular aging, risk factors, comorbidities, and disease modifiers. Heart fail. Rev. 27 (1), 337–344. doi:10.1007/s10741-020-09987-z
Uzayisenga, R., Ayeka, P. A., and Wang, Y. (2014). Anti-diabetic potential of Panax notoginseng saponins (PNS): a review. Phytother. Res. 28 (4), 510–516. doi:10.1002/ptr.5026
Varoni, M. V., Pasciu, V., Gadau, S. D., Baralla, E., Serra, E., Palomba, D., et al. (2017). Possible antioxidant effect of Lycium barbarum polysaccharides on hepatic cadmium-induced oxidative stress in rats. Environ. Sci. Pollut. Res. Int. 24 (3), 2946–2955. doi:10.1007/s11356-016-8050-x
Wang, K., Liu, C. Y., Zhang, X. J., Feng, C., Zhou, L. Y., Zhao, Y., et al. (2015a). miR-361-regulated prohibitin inhibits mitochondrial fission and apoptosis and protects heart from ischemia injury. Cell Death Differ. 22 (6), 1058–1068. doi:10.1038/cdd.2014.200
Wang, K., Liu, C. Y., Zhou, L. Y., Wang, J. X., Wang, M., Zhao, B., et al. (2015b). APF lncRNA regulates autophagy and myocardial infarction by targeting miR-188-3p. Nat. Commun. 6, 6779. doi:10.1038/ncomms7779
Wang, K., Long, B., Liu, F., Wang, J. X., Liu, C. Y., Zhao, B., et al. (2016). A circular RNA protects the heart from pathological hypertrophy and heart failure by targeting miR-223. Eur. Heart J. 37 (33), 2602–2611. doi:10.1093/eurheartj/ehv713
Wang, K., Zhang, D. L., Long, B., An, T., Zhang, J., Zhou, L. Y., et al. (2015c). NFAT4-dependent miR-324-5p regulates mitochondrial morphology and cardiomyocyte cell death by targeting Mtfr1. Cell Death Dis. 6 (12), e2007. doi:10.1038/cddis.2015.348
Wang, K., Zhou, L. Y., Wang, J. X., Wang, Y., Sun, T., Zhao, B., et al. (2015d). E2F1-dependent miR-421 regulates mitochondrial fragmentation and myocardial infarction by targeting Pink1. Nat. Commun. 6, 7619. doi:10.1038/ncomms8619
Wang, L., Tan, Y., Zhu, Z., Chen, J., Sun, Q., Ai, Z., et al. (2021a). ATP2B1-AS1 promotes cerebral ischemia/reperfusion injury through regulating the miR-330-5p/TLR4-MyD88-NF-κB signaling pathway. Front. Cell Dev. Biol. 9, 720468. doi:10.3389/fcell.2021.720468
Wang, Q. G., Cheng, B. C., He, Y. Z., Li, L. J., Ling, Y., Luo, G., et al. (2021b). miR-320a in serum exosomes promotes myocardial fibroblast proliferation via regulating the PIK3CA/Akt/mTOR signaling pathway in HEH2 cells. Exp. Ther. Med. 22 (2), 873. doi:10.3892/etm.2021.10305
Wang, T., Zhai, M., Xu, S., Ponnusamy, M., Huang, Y., Liu, C. Y., et al. (2020). NFATc3-dependent expression of miR-153-3p promotes mitochondrial fragmentation in cardiac hypertrophy by impairing mitofusin-1 expression. Theranostics 10 (2), 553–566. doi:10.7150/thno.37181
Wang, Y., Wang, Q., Li, C., Lu, L., Zhang, Q., Zhu, R., et al. (2017). A review of Chinese herbal medicine for the treatment of chronic heart failure. Curr. Pharm. Des. 23 (34), 5115–5124. doi:10.2174/1381612823666170925163427
Wei, W., Li, C., Zhang, B., Huang, D., Li, Z., and Gao, J. (2024). Total glucosides of paeony ameliorate myocardial injury in chronic heart failure rats by suppressing PARP-1. J. Cardiovasc. Transl. Res. 17 (2), 388–402. doi:10.1007/s12265-023-10440-3
Weng, Y. S., Wang, H. F., Pai, P. Y., Jong, G. P., Lai, C. H., Chung, L. C., et al. (2015). Tanshinone IIA prevents Leu27IGF-II-induced cardiomyocyte hypertrophy mediated by estrogen receptor and subsequent Akt activation. Am. J. Chin. Med. 43 (8), 1567–1591. doi:10.1142/s0192415x15500895
Wu, C., Chen, F., Huang, S., Zhang, Z., Wan, J., Zhang, W., et al. (2023). Progress on the role of traditional Chinese medicine in therapeutic angiogenesis of heart failure. J. Ethnopharmacol. 301, 115770. doi:10.1016/j.jep.2022.115770
Wu, W., Hu, Y., Li, J., Zhu, W., Ha, T., Que, L., et al. (2014). Silencing of Pellino1 improves post-infarct cardiac dysfunction and attenuates left ventricular remodelling in mice. Cardiovasc. Res. 102 (1), 46–55. doi:10.1093/cvr/cvu007
Wu, Y., Liu, Y., Pan, Y., Lu, C., Xu, H., Wang, X., et al. (2018). MicroRNA-135a inhibits cardiac fibrosis induced by isoproterenol via TRPM7 channel. Biomed. Pharmacother. 104, 252–260. doi:10.1016/j.biopha.2018.04.157
Wu, Y., Wang, M., Xu, J., Wei, J., and Yang, H. (2022). Signature network-based survey of the effects of a traditional Chinese medicine on heart failure. J. Ethnopharmacol. 283, 114750. doi:10.1016/j.jep.2021.114750
Xiao, J., Xing, F., Huo, J., Fung, M. L., Liong, E. C., Ching, Y. P., et al. (2014). Lycium barbarum polysaccharides therapeutically improve hepatic functions in non-alcoholic steatohepatitis rats and cellular steatosis model. Sci. Rep. 4, 5587. doi:10.1038/srep05587
Xing, Z., Yang, C., Feng, Y., He, J., Peng, C., and Li, D. (2024). Understanding aconite's anti-fibrotic effects in cardiac fibrosis. Phytomedicine 122, 155112. doi:10.1016/j.phymed.2023.155112
Xu, C., Wang, W., Wang, B., Zhang, T., Cui, X., Pu, Y., et al. (2019). Analytical methods and biological activities of Panax notoginseng saponins: recent trends. J. Ethnopharmacol. 236, 443–465. doi:10.1016/j.jep.2019.02.035
Xu, S., Wang, Y., Yu, M., Wang, D., Liang, Y., Chen, Y., et al. (2020). LongShengZhi capsule inhibits doxorubicin-induced heart failure by anti-oxidative stress. Biomed. Pharmacother. 123, 109803. doi:10.1016/j.biopha.2019.109803
Xue, P., Liu, Y., Wang, H., Huang, J., and Luo, M. (2023). miRNA-103-3p-Hlf regulates apoptosis and autophagy by targeting hepatic leukaemia factor in heart failure. Esc. Heart Fail 10 (5), 3038–3045. doi:10.1002/ehf2.14493
Yan, K., An, T., Zhai, M., Huang, Y., Wang, Q., Wang, Y., et al. (2019). Mitochondrial miR-762 regulates apoptosis and myocardial infarction by impairing ND2. Cell Death Dis. 10 (7), 500. doi:10.1038/s41419-019-1734-7
Yan, X., and Huang, Y. (2021). Mechanism of total glucosides of paeony in hypoxia/reoxygenation-induced cardiomyocyte pyroptosis. J. Bioenerg. Biomembr. 53 (6), 643–653. doi:10.1007/s10863-021-09921-4
Yan, X., Wu, H., Ren, J., Liu, Y., Wang, S., Yang, J., et al. (2018). Shenfu Formula reduces cardiomyocyte apoptosis in heart failure rats by regulating microRNAs. J. Ethnopharmacol. 227, 105–112. doi:10.1016/j.jep.2018.05.006
Yan, X., Xue, J., Wu, H., Wang, S., Liu, Y., Zheng, S., et al. (2015). Ginsenoside-Rb1 protects hypoxic- and ischemic-damaged cardiomyocytes by regulating expression of miRNAs. Evid. Based Complement. Altern. Med. 2015, 171306. doi:10.1155/2015/171306
Yancy, C. W., Jessup, M., Bozkurt, B., Butler, J., Casey, D. E., Drazner, M. H., et al. (2013). 2013 ACCF/AHA guideline for the management of heart failure: a report of the American college of cardiology foundation/American heart association task force on practice guidelines. J. Am. Coll. Cardiol. 62 (16), e147–e239. doi:10.1016/j.jacc.2013.05.019
Yang, D., So, K. F., and Lo, A. C. (2017). Lycium barbarum polysaccharide extracts preserve retinal function and attenuate inner retinal neuronal damage in a mouse model of transient retinal ischaemia. Clin. Exp. Ophthalmol. 45 (7), 717–729. doi:10.1111/ceo.12950
Yang, W., Han, Y., Yang, C., Chen, Y., Zhao, W., Su, X., et al. (2019). MicroRNA-19b-1 reverses ischaemia-induced heart failure by inhibiting cardiomyocyte apoptosis and targeting Bcl2 l11/BIM. Heart Vessels 34 (7), 1221–1229. doi:10.1007/s00380-018-01336-3
Yang, Z., Duan, X., Wang, X., Li, D., Xu, Q., Xiang, S., et al. (2022). The effect of Q-switched 1064-nm dymium-doped yttrium aluminum garnet laser on the skin barrier and collagen synthesis through miR-24-3p. Lasers Med. Sci. 37 (1), 205–214. doi:10.1007/s10103-020-03214-9
Ye, J., Lyu, T. J., Li, L. Y., Liu, Y., Zhang, H., Wang, X., et al. (2023). Ginsenoside re attenuates myocardial ischemia/reperfusion induced ferroptosis via miR-144-3p/SLC7A11. Phytomedicine 113, 154681. doi:10.1016/j.phymed.2023.154681
Ye, S. B., Zhang, H., Cai, T. T., Liu, Y. N., Ni, J. J., He, J., et al. (2016). Exosomal miR-24-3p impedes T-cell function by targeting FGF11 and serves as a potential prognostic biomarker for nasopharyngeal carcinoma. J. Pathol. 240 (3), 329–340. doi:10.1002/path.4781
Yi, R., Liu, X. M., and Dong, Q. (2013). A study of Lycium barbarum polysaccharides (LBP) extraction technology and its anti-aging effect. Afr. J. Tradit. Complement. Altern. Med. 10 (4), 171–174. doi:10.4314/ajtcam.v10i4.27
Yu, W., Cheng, H., Zhu, B., and Yan, J. (2021). Network pharmacology-based validation of the efficacy of Huiyangjiuji decoction in the treatment of experimental colitis. Front. Pharmacol. 12, 666432. doi:10.3389/fphar.2021.666432
Yuan, J., and Yuan, G. (2022). miR-212 promotes cardiomyocyte hypertrophy through regulating transcription factor 7 like 2. Mediat. Inflamm. 2022, 5187218. doi:10.1155/2022/5187218
Yuan, M., Zhang, L., You, F., Zhou, J., Ma, Y., Yang, F., et al. (2017). MiR-145-5p regulates hypoxia-induced inflammatory response and apoptosis in cardiomyocytes by targeting CD40. Mol. Cell. Biochem. 431 (1-2), 123–131. doi:10.1007/s11010-017-2982-4
Zhang, B., Mao, S., Liu, X., Li, S., Zhou, H., Gu, Y., et al. (2021). MiR-125b inhibits cardiomyocyte apoptosis by targeting BAK1 in heart failure. Mol. Med. 27 (1), 72. doi:10.1186/s10020-021-00328-w
Zhang, D., Pan, A., Gu, J., Liao, R., Chen, X., and Xu, Z. (2023a). Upregulation of miR-144-3p alleviates Doxorubicin-induced heart failure and cardiomyocytes apoptosis via SOCS2/PI3K/AKT axis. Chem. Biol. Drug Des. 101 (1), 24–39. doi:10.1111/cbdd.14104
Zhang, H., Li, S., Zhou, Q., Sun, Q., Shen, S., Zhou, Y., et al. (2016). Qiliqiangxin attenuates phenylephrine-induced cardiac hypertrophy through downregulation of MiR-199a-5p. Cell Physiol. biochem. 38 (5), 1743–1751. doi:10.1159/000443113
Zhang, H., Zhang, L., Yin, K., Zhang, M., Hao, Y., Zhou, H., et al. (2023b). Analysis of function role and long noncoding RNA expression in chronic heart failure rats treated with hui Yang jiu ji decoction. J. Healthc. Eng. 2023, 7438567. doi:10.1155/2023/7438567
Zhang, H., Zhang, X., and Zhang, J. (2018a). MiR-129-5p inhibits autophagy and apoptosis of H9c2 cells induced by hydrogen peroxide via the PI3K/AKT/mTOR signaling pathway by targeting ATG14. Biochem. Biophys. Res. Commun. 506 (1), 272–277. doi:10.1016/j.bbrc.2018.10.085
Zhang, R., Xu, Y., Niu, H., Tao, T., Ban, T., Zheng, L., et al. (2018b). Lycium barbarum polysaccharides restore adverse structural remodelling and cardiac contractile dysfunction induced by overexpression of microRNA-1. J. Cell Mol. Med. 22 (10), 4830–4839. doi:10.1111/jcmm.13740
Zhang, X., Yang, K., Zhang, H., Dong, W., Peng, W., and Zhao, Y. (2020). Effect of typhaneoside on ventricular remodeling and regulation of PI3K/Akt/mTOR pathway. Herz 45 (Suppl. 1), 113–122. doi:10.1007/s00059-019-4819-2
Zhang, X. J., Yu, H. Y., Cai, Y. J., and Ke, M. (2017). Lycium barbarum polysaccharides inhibit proliferation and migration of bladder cancer cell lines BIU87 by suppressing Pi3K/AKT pathway. Oncotarget 8 (4), 5936–5942. doi:10.18632/oncotarget.13963
Zhang, X. L., An, B. F., and Zhang, G. C. (2019a). MiR-27 alleviates myocardial cell damage induced by hypoxia/reoxygenation via targeting TGFBR1 and inhibiting NF-κB pathway. Kaohsiung J. Med. Sci. 35 (10), 607–614. doi:10.1002/kjm2.12092
Zhang, Y., Sun, M., Wang, D., Hu, Y., Wang, R., Diao, H., et al. (2022a). FTZ protects against cardiac hypertrophy and oxidative injury via microRNA-214/SIRT3 signaling pathway. Biomed. Pharmacother. 148, 112696. doi:10.1016/j.biopha.2022.112696
Zhang, Y., Wang, Z., Lan, D., Zhao, J., Wang, L., Shao, X., et al. (2022b). MicroRNA-24-3p alleviates cardiac fibrosis by suppressing cardiac fibroblasts mitophagy via downregulating PHB2. Pharmacol. Res. 177, 106124. doi:10.1016/j.phrs.2022.106124
Zhang, Y., Zhang, L., Zhang, Y., Fan, X., Yang, W., Yu, B., et al. (2019b). YiQiFuMai powder injection attenuates coronary artery ligation-induced heart failure through improving mitochondrial function via regulating ROS generation and CaMKII signaling pathways. Front. Pharmacol. 10, 381. doi:10.3389/fphar.2019.00381
Zhao, P., Wang, Y., Zhang, L., Zhang, J., Liu, N., and Wang, H. (2021). Mechanism of long non-coding RNA metastasis-associated lung adenocarcinoma transcript 1 in lipid metabolism and inflammation in heart failure. Int. J. Mol. Med. 47 (3), 5. doi:10.3892/ijmm.2020.4838
Zhao, Y., Li, Y., Tong, L., Liang, X., Zhang, H., Li, L., et al. (2018). Analysis of microRNA expression profiles induced by yiqifumai injection in rats with chronic heart failure. Front. Physiol. 9, 48. doi:10.3389/fphys.2018.00048
Zhou, J., Tian, G., Quan, Y., Kong, Q., Huang, F., Li, J., et al. (2023). The long noncoding RNA THBS1-AS1 promotes cardiac fibroblast activation in cardiac fibrosis by regulating TGFBR1. JCI Insight 8 (6), e160745. doi:10.1172/jci.insight.160745
Zhou, S. S., Jin, J. P., Wang, J. Q., Zhang, Z. G., Freedman, J. H., Zheng, Y., et al. (2018). miRNAS in cardiovascular diseases: potential biomarkers, therapeutic targets and challenges. Acta. Pharmacol. Sin. 39 (7), 1073–1084. doi:10.1038/aps.2018.30
Zhu, Q., Li, S., Ji, K., Zhou, H., Luo, C., and Sui, Y. (2021). Differentially expressed TUG1 and miR-145-5p indicate different severity of chronic heart failure and predict 2-year survival prognosis. Exp. Ther. Med. 22 (6), 1362. doi:10.3892/etm.2021.10796
Ziaeian, B., and Fonarow, G. C. (2016). Epidemiology and aetiology of heart failure. Nat. Rev. Cardiol. 13 (6), 368–378. doi:10.1038/nrcardio.2016.25
Zou, Y., Lin, L., Ye, Y., Wei, J., Zhou, N., Liang, Y., et al. (2012). Qiliqiangxin inhibits the development of cardiac hypertrophy, remodeling, and dysfunction during 4 weeks of pressure overload in mice. J. Cardiovasc. Pharmacol. 59 (3), 268–280. doi:10.1097/FJC.0b013e31823f888f
Zuo, W., Tian, R., Chen, Q., Wang, L., Gu, Q., Zhao, H., et al. (2021). miR-330-5p inhibits NLRP3 inflammasome-mediated myocardial ischaemia-reperfusion injury by targeting TIM3. Cardiovasc. Drugs Ther. 35 (4), 691–705. doi:10.1007/s10557-020-07104-8
HF Heart Failure
CHM Chinese Herbal Medicine
miRNA MicroRNA
3′-UTR 3′-Untranslated Region
TCM Traditional Chinese Medicine
Tan IIA Tanshinone IIA
LBP Lycium Barbarum Polysaccharide
PNS Panax Notoginseng Saponins
TGP Total Glucosides of Paeony
Dox Doxorubicin
ATG Autophagy-related gene
APF Autophagy-promoting factor
mTOR Mechanistic Target of Rapamycin
CDK Cyclin-Dependent Kinase
ATP Adenosine Triphosphate
RISC RNA-Induced Silencing Complex
MEG3 Maternal Expression Gene 3
ECM Extracellular Matrix
AMPK AMP-activated Protein Kinase
HNF-4α Hepatocyte Nuclear Factor 4 Alpha
TGF-β1 Transforming Growth Factor Beta 1
Peli1 E3 Ubiquitin Ligase Peli1
TNFR Tumor Necrosis Factor Receptor
TLR Toll-like Receptor
IL-1R Interleukin-1 Receptor
CHF Congestive Heart Failure
HSF2 Heat Shock Factor 2
NFAT Nuclear Factor of Activated T-cells
TRPM7 Transient Receptor Potential Melastatin 7
lncRNA Long Non-coding RNA
LDLR Low-Density Lipoprotein Receptor
TUG1 Taurine-upregulated Gene 1
I/R Ischemia/Reperfusion
JAK2 Janus Kinase 2
STAT1 Signal Transducer and Activator of Transcription 1
HYJJ Huiyangjiuji Decoction
FTZ Fufang Zhenzhu Tiaozhi
LGZG Lingguizhugan Decoction
YQFM YiQiFuMai Injection
NF-κB Nuclear Factor kappa-light-chain-enhancer of activated B cells
MAPK Mitogen-Activated Protein Kinase
CAL Coronary Artery Ligation
DE Differentially Expressed
SIRT3 Sirtuin 3
Smad3 Sma- and Mad-Related Protein 3
LVEF Left Ventricular Ejection Fraction
LVFS Left Ventricular Fraction Shortening
MMP14 Matrix Metalloproteinase 14
GRP78 Glucose-Regulated Protein 78
JP-2 Junctophilin-2
H/I Hypoxia and Ischemia
MEF2A Myocyte Enhancer Factor 2A
NO Nitric Oxide
Ca2+ Calcium
ROS Reactive Oxygen Species
ADCY1 Adenylate Cyclase 1
PI3K Phosphoinositide 3-Kinase
Akt Protein Kinase B
ISOP Isoproterenol
H/R Hypoxia/Reperfusion
Keywords: Chinese herbal medicine, microRNA, heart failure, therapeutic strategies, cardiac hypertrophy, cardiac fibrosis, apoptosis
Citation: Wang Y, Lai J, Chen Z, Sun L, Ma Y and Wu J (2024) Exploring the therapeutic mechanisms of heart failure with Chinese herbal medicine: a focus on miRNA-mediated regulation. Front. Pharmacol. 15:1475975. doi: 10.3389/fphar.2024.1475975
Received: 04 August 2024; Accepted: 23 October 2024;
Published: 05 November 2024.
Edited by:
Yongsheng Chen, Jinan University, ChinaReviewed by:
Dan Li, Chengdu University of Traditional Chinese Medicine, ChinaCopyright © 2024 Wang, Lai, Chen, Sun, Ma and Wu. This is an open-access article distributed under the terms of the Creative Commons Attribution License (CC BY). The use, distribution or reproduction in other forums is permitted, provided the original author(s) and the copyright owner(s) are credited and that the original publication in this journal is cited, in accordance with accepted academic practice. No use, distribution or reproduction is permitted which does not comply with these terms.
*Correspondence: Jianguang Wu, d3VqaWFuZ3VhbmcwOTA2QDE2My5jb20=
Disclaimer: All claims expressed in this article are solely those of the authors and do not necessarily represent those of their affiliated organizations, or those of the publisher, the editors and the reviewers. Any product that may be evaluated in this article or claim that may be made by its manufacturer is not guaranteed or endorsed by the publisher.
Research integrity at Frontiers
Learn more about the work of our research integrity team to safeguard the quality of each article we publish.