- 1Qinghai-Tibetan Plateau Ethnic Medicinal Resources Protection and Utilization Key Laboratory of National Ethnic Affairs Commission of the People’s Republic of China, Southwest Minzu University, Chengdu, China
- 2Institute of Chinese Materia Medica Pharmacology and Toxicology, Sichuan Academy of Chinese Medicine Sciences, Chengdu, China
- 3Shimadzu China Co. LTD., Chengdu, China
- 4College of Resources and Environment, Aba Teachers College, Wenchuan, China
- 5Engineering Research Center for Pharmaceuticals and Equipments of Sichuan Province, School of pharmacy, Sichuan Industrial Institute of Antibiotics, Chengdu University, Chengdu, China
Background: Meconopsis quintuplinervia Regel (MQ) is a traditional Chinese medicine (TCM) used for clearing liver and lung heat in Tibetan medicine for over a thousand years. However, the lack of quality markers that correlate with pharmacological actions and absorption in vivo limits the safety and efficacy in its clinical application and on the drug market. Furthermore, a universal and rapid method for simultaneously determining quality markers in the MQ crude drug is still lacking.
Purpose: An absorption-based metabolite approach was used to discover and identify the quality markers of MQ. An efficient method based on polarity-switching ultra-performance liquid chromatography triple quadrupole mass spectrometry (UPLC-QQQ-MS/MS) was then established to determine the quality markers.
Methods: The absorbed compounds and metabolites were first characterized using UPLC plus Q-Exactive Orbitrap tandem mass spectrometry (UPLC-Q-Exactive Orbitrap-MS) after giving oral MQ extract to rats. Subsequently, the absorbed compounds and precursors of metabolites correlating with the hepatocyte protection activity were screened as quality markers. Finally, a polarity-switching UPLC-QQQ-MS/MS method was developed for the quantitative analysis of the MQ crude drug, enabling the detection of quality markers in both negative and positive ion modes in a single run. The MS2 characteristics of target compounds were investigated to select appropriate product ions.
Results: A total of 13 absorbed compounds and 30 metabolites were characterized. Among these, nine compounds, including five absorbed compounds and four precursors of metabolites intimately correlated with hepatocyte protection activity and absorption in vivo, were considered the quality markers of MQ in the current study. The quantification of quality markers was conducted using an Acquity UPLC HSS T3 (2.1 × 100 mm, 1.8 μm) column, the mobile phase consisting of acetonitrile and 0.1% formic acid solution (containing 10 mmol/L ammonium acetate). The validated UPLC-QQQ-MS/MS method was successfully applied to quantify the quality markers in the MQ crude drug.
Conclusion: We defined the quality markers and established a universal and rapid method for simultaneously determining the quality markers of MQ, which will be helpful for further investigation of the quality evaluation of MQ in clinical application and the drug market.
1 Introduction
Meconopsis quintuplinervia Regel (MQ) is a valuable botanical drug belonging to the Papaveraceae family, distributed only in the Qinghai–Tibetan plateau (Luo et al., 1984). It has been used as a traditional Chinese medicine (TCM) named Herba Meconopsis with a long clinical history dating back to the eighth century in the Tibetan book Yue Wang Yao Zhen and recorded in the Tibetan masterpieces The Four Medical Tantras and the Jing Zhu Materia Medica. It has traditionally been used for clearing liver and lung heat (Guo et al., 2016). Given its significant and reliable biological activities, MQ is widely used in more than 100 prescriptions of Tibetan medicine and 31 marketed prescription drugs, such as Ershiwuwei Luronghao Wan, Shiqiwei Hanshuishi Wan, and Shibawei Niuhuang San (Luo et al., 1984; Wang and Ma, 2009; Guo et al., 2016). Previous studies have shown that the pharmacological effect of MQ may be attributed to its chemical compounds such as flavonoids (Shang et al., 2002; Shang et al., 2006b), alkaloids (Shang et al., 2003; Wu et al., 2007), and phenolics (Shang et al., 2006a). The total flavonoid and phenolic fraction extracted from MQ exhibit an obvious hepatoprotective effect on experimental liver damage and hepatic fibrosis, which significantly decreases alanine aminotransferase (ALT), aspartate aminotransferase (AST), and malondialdehyde (MDA) levels, while increasing superoxide dismutase (SOD), catalase (CAT), and reduced glutathione (GSH) levels in carbon tetrachloride (CCl4)-induced liver damage mice. Additionally, these fractions reduce the levels of hydroxyproline and collagen in liver tissue, as well as liver fibrosis indicators such as hyaluronic acid, type III procollagen, type IV collagen, and laminin in rats with liver fibrosis (He et al., 2012; Wang et al., 2013a; Wang et al., 2013b). Total alkaloids extracted from MQ exhibit a strong anti-inflammatory effect on LPS-induced acute inflammation in mouse models. This is related to MQ’s ability to inhibit the expression of the pro-inflammatory cytokines tumor necrosis factor-α (TNF-α) and Interleukin-6 (IL-6) and the production of nitric oxide (NO) and to reduce inducible nitric oxide synthase (iNOS) activity (Yu et al., 2021). Kong et al. (2022) employed ultra-performance liquid chromatography plus Q-Exactive Orbitrap tandem mass spectrometry (UPLC-Q-Exactive Orbitrap/MS) and network analysis to analyze the active compounds acting against liver fibrosis. They found that MQ presumably exerted an anti-liver fibrosis effect through luteolin, isorhamnetin, quercetin, apigenin, kaempferide, amurine, 2-methylflavinantine, and allocryptopine on RAC-alpha serine/threonine-protein kinase (AKT1), proto-oncogene tyrosine-protein kinase Src (SRC), transcription factor Jun (JUN), epidermal growth factor receptor (EGFR), and other core targets, and regulated the phosphatidylinositol 3-kinases/protein kinase B (PI3K/AKT), forkhead box protein O (FoxO) and other signaling pathways (Kong et al., 2022).
Traditionally, MQ has primarily been obtained from wild sources, and several species of Meconopsis with blue flower were used as Herba Meconopsis in clinics and the medical industry. It is difficult to guarantee the clinical efficacy and safety of MQ. Furthermore, the “Pharmaceutical Standards of the Ministry of Health of the People’s Republic of China” for Herba Meconopsis uses only morphological and microscopic identification for the quality control of MQ (Chinese Pharmacopoeia Commission, 1995). However, these quality control methods do not reflect MQ’s clinical efficacy or pharmacological activity (Li Y. Z. et al., 2019; Zhang et al., 2019). With the discovery of new compounds and deeper research into the pharmacological activity of MQ, its quality control in clinical application and the market has become more important. It is thus necessary to discover scientific and reasonable quality markers for the quality control of MQ. “Quality markers” are several specific chemical compounds of botanical drugs and their products that are closely related to therapeutic effects and absorption in vivo and could be used for qualitative and quantitative analysis (Liu et al., 2016; Liu et al., 2018; Li Y. Z. et al., 2019). In recent years, some studies of quality markers have focused on characterizing the chemical compound–bioactivity association (Wei et al., 2021; Li Y. L. et al., 2022; Li et al., 2024) and prototype-based pharmacokinetic in vivo absorption (Li et al., 2018; Duan et al., 2020). These have been the general approach for the analysis of quality markers. However, some studies have shown that TCM metabolism is a crucial link between pharmacological activity in vivo and phytochemistry in vitro. In brief, the absorbed compounds and metabolites of TCM in vivo are the ultimate material basis for the expression of the efficacy of TCM (Xu et al., 2022). Some of the precursors of the metabolites, which are the effective forms of TCM, can be used as quality markers for its quality control (Zhang et al., 2022). This strategy based on metabolites, including absorption compounds and metabolites in vivo, we term “absorption-based metabolite strategy”. Unfortunately, few studies have focused on absorption-based metabolite strategy for quality evaluation research into TCM; it may be a powerful approach, and this is the first study of the quality markers of MQ.
Moreover, many methods have been developed to quantify one or several compounds as quality indicators in the MQ raw material. For example, Yue et al. (2010) determined four major flavonoids in hydrolyzed MQ samples by high-performance liquid chromatography (HPLC) coupled with diode array detection. Shi et al. (2011) established an HPLC method for determining one alkaloid in MQ raw material by HPLC. However, for the complex chemical compounds in MQ, the established quantification methods developed by HPLC were limited in their application for the quality control of multiple compounds in varying polarity with long running times and low sensitivity (Jiao et al., 2019). It is thus necessary to establish a universal and rapid method for the simultaneous determination of quality markers in MQ crude drug.
Ultra-performance liquid chromatography triple quadrupole mass spectrometry (UPLC-QQQ-MS/MS) is a widely utilized technology with high separation capability and sensitivity for quantification quality markers in TCM (Li X. Y. et al., 2022). The multiple reaction monitoring (MRM) mode of UPLC-QQQ-MS/MS is a powerful technology for screening specified molecular ion-to-product transitions to quantify quality markers in a specified condition which can effectively avoid interference peak (Jiao et al., 2019; Sun et al., 2023). More importantly, the polarity-switching function in UPLC-QQQ-MS/MS can simultaneously detect different polarity compounds in negative and positive ion modes in a single run (Shen et al., 2022). Therefore, UPLC-QQQ-MS/MS enables the simultaneous determination of different polarity quality markers of MQ simultaneously, sensitively, and rapidly.
In the current study, we explored MQ to discover, identify, and quantify the quality markers by an absorption-based metabolite strategy. First, we explored the metabolism of MQ extract in rats using UPLC-Q-Exactive Orbitrap/MS technology and elucidated the absorbed compounds and metabolites and the metabolic pathways of MQ compounds. Second, we screened the absorbed compounds and the precursors of metabolites that correlated with hepatocyte protection activity, and we validated by reported experimentation these absorbed compounds and the precursors of metabolites in vivo that were used as quality markers. Finally, an efficient method based on polarity-switching UPLC-QQQ-MS/MS technology for determining quality markers in MQ was established to determine the quality marker in MQ crude drug. To our knowledge, this is the first study to discover the quality markers of MQ based on the metabolism of absorbed compounds in vivo. Our research will be helpful for screening the quality markers of MQ and providing a useful perspective for discovering the quality markers of other TCMs.
2 Materials and methods
2.1 Chemical reagents
Quercetin (Lot: MUST-22042012), luteolin (Lot: MUST-22072315), apigenin (Lot: MUST-22111214), taxifolin (Lot: MUST-22101411), isorhamnetin (Lot: MUST-22082703), caffeic acid (Lot: MUST-22062118), chlorogenic acid (Lot: MUST-22111711), allocryptopine (Lot: MUST-22031114), and protopine (Lot: MUST-22062118) with purity ≥98% were purchased from Chengdu MUST Bio-technology Co., Ltd (Chengdu, China). Their structures are shown in Figure 1. HPLC-grade acetonitrile and formic acid were sourced both from Thermo Fisher (Waltham, MA, United States). Ultrapure water was purified at 18.2 MΩ using PERSEE GWB-1E ultrapure water system (Beijing, China). Other reagents were of analytical grade and were purchased locally.
2.2 Plant materials
Ten batches of MQ crude drug samples were collected or purchased from Qinghai, Gansu, and Sichuan, China. They were identified by Professor Yuan Liu and Dr. Yanfei Huang as M. quintuplinervia Regel (Supplementary Table S1). The voucher specimens of these samples were deposited at the Tibetan, Qiang, and Yi medicinal resources herbarium, Qinghai–Tibetan Plateau Ethnic Medicinal Resources Protection and Utilization Key Laboratory of the National Ethnic Affairs Commission of the People’s Republic of China, Southwest Minzu University.
MQ extract was prepared as per our previous study (Kong et al., 2022). MQ powders (1000 g) were mixed with 70% ethyl alcohol (v/v) (8 L) and extracted by heating reflux extraction for 1.5 h. This step was repeated three times. The extract was then filtered and dried in vacuum at 50°C and treated with D101 macroporous resins. Afterward, 30%, 50%, and 70% ethyl alcohol eluent was collected and mixed, and the extract was concentrated and freeze-dried into a powder.
2.3 Animals and metabolic study
Twelve male Sprague–Dawley rats (weighing 180–220 g) were obtained from the Experimental Animal Center of the Sichuan Academy of Chinese Medicine Sciences (animal production license number: SYXK (2018)-100, Chengdu, China). The rats were kept in an environmentally controlled animal room for 7 days, with water and food ad libitum twice daily. The rats were randomly divided into two groups—MQ and blank, with six rats per group—and were housed in metabolic cages for 3 days to acclimatize to the facilities prior to the experiments. MQ extract was suspended in 0.5% CMC-Na solution and orally administered to the MQ group at a daily dose of 50 mg/kg. The MQ extract administration lasted for 5 days, once a day at 9:00 a.m. The blank group was orally administered 0.5% CMC-Na solution at the same volume time. These animal experiments were conducted under the guidance of the Care and Use of Laboratory Animals published by the US National Institute of Health and were approved by the Biomedical Ethical Committee of Southwest Minzu University (approval no. SWU-202401160).
2.4 Biological sample collection and preparation
2.4.1 Urine and feces samples
Urine samples were collected after administration of MQ extract in the drug and blank groups at 0–24 h. The collection tubes for urine samples were pre-filled with a small volume of absolute ethanol as a preservative. After collection in 1 day, the samples were merged and evaporated to dryness under reduced pressure at 50°C using an electro thermostatic blast oven (DS-30H, Shanghai Dengsheng Instrument Manufacturing Co., Ltd., Shanghai, China). The dried samples of the different groups were then extracted with four-fold methanol (v/w) in an ultrasonic cleaner for 30 min to precipitate the endogenous substances. These samples were centrifuged at 5,000 rpm, and the supernatant was dried in vacuum at 40°C. Subsequently, each 1.0 g residue of the MQ and blank groups was reconstituted in 5.0 mL methanol and filtered through a 0.22-μm nylon filter before UPLC-Q-Exactive Orbitrap/MS analysis.
Feces samples from the drug group and the blank group were collected twice daily from 8:00 am and 8:00 pm and dried immediately at 50°C using an electro-thermostatic blast oven. Thence, pulverized feces samples (1.0 g) of each group were mixed with 5.0 mL methanol and extracted using an ultrasonic bath for 30 min three times. The extracted samples were centrifuged at 5,000 rpm for 15 min, and the supernatant of the two groups was collected and dried in a rotary evaporator at 40°C. Subsequently, 100 mg of the resulting residue for each group was dissolved in 3 mL methanol and filtered through a 0.22-μm nylon filter before UPLC-Q-Exactive Orbitrap/MS analysis.
2.4.2 Plasma samples
On day 5, after 0.5, 1.0, and 1.5 h of the last administration of MQ extract, blood samples of the two groups were collected into heparinized tubes through heart puncture while the rats were anesthetized by pentobarbital sodium (i.p. 70 mg/kg body weight). Two rats were sacrificed at one time point, and blood samples collected at the same time point from each of the two groups were combined into one sample. These blood samples were centrifuged at 5,000 rpm and 4°C for 10 min to obtain plasma. Next, 6 mL of the two groups of plasma samples (mixed from the three time points, 2 mL per time point) was supplemented with 24 mL methanol and ultrasonically extracted for 30 min to precipitate the protein. The extraction mixture of the drug and blank groups was then centrifuged at 5,000 rpm for 15 min. After that, the supernatant was condensed in a vacuum at 40°C, and the residue was dissolved in 300 μL methanol. Finally, the prepared samples were centrifuged at 15,000 rpm using a Sorvall ST 16R centrifuge (Thermo Fisher Scientific Inc., United States) for 10 min before UPLC-Q-Exactive Orbitrap/MS analysis.
2.4.3 Organ samples
After collecting blood samples from the two groups, the main organs, including the brain, heart, lung, liver, kidney, intestine, and spleen, were rapidly removed from the rats, flushed with pre-cooled normal saline, and frozen at −80°C before further processing. Each of the organs was homogenized in fourfold (volume/wet weight) pre-cooled normal saline. Eightfold methanol was then added to 6 mL homogenate, and extraction was performed in an ultrasonic bath for 30 min. The extraction mixtures of the two groups were centrifuged at 5,000 rpm for 15 min. Next, the supernatant was condensed in a vacuum at 40°C, and the residue was dissolved in 1 mL methanol and filtered through a 0.22-μm nylon filter before UPLC-Q-Exactive Orbitrap/MS analysis.
2.5 MQ sample preparation and standard solution
The primary stock solutions of 1,000 μg/mL quercetin, luteolin, apigenin, taxifolin, isorhamnetin, caffeic acid, chlorogenic acid, allocryptopine, and protopine were dissolved in 70% methanol. A series of working standard solutions were prepared by diluting the primary stock solution with 70% methanol. All solutions were stored at 4°C before analysis.
The dried MQ crude drugs were finely powdered in a grinder. One gram of the powder was accurately weighed and then extracted in 70% methanol (75 mL) with the help of heating reflux in 30 min. Afterward, the supernatants were filtered through a 0.22-μm membrane filter before analysis.
2.6 UPLC-Q-Exactive Orbitrap/MS analysis conditions
The UPLC-Q-Exactive Orbitrap/MS analyses were performed on a Thermo Scientific Vanquish Flex UPLC coupled with a Q-Exactive Orbitrap Mass analyzer (Thermo Fisher Scientific Inc., United States). The LC-HRMS data were processed by Xcalibur. Chromatography separation was performed on an ACQUITY HSS T3 column (100 × 2.1 mm, 1.8 μm) (Waters, United States) protected with an ACQUITY HSS T3 VanGuard pre-column (2.1 × 5 mm, 1.8 μm) (Waters, United States) at 30°C. The mobile phase consisted of 0.1% formic acid solution (A) and acetonitrile (B), and the gradient elution program was as follows: 5%–10% B at 0–10 min; 10%–20% B at 10–35 min; 20%–27% B at 35–40 min; 27%–33% B at 40–45 min; 33%–55% B at 45–50 min; 55%–90% B at 50–55 min. The flow rate was 0.2 mL/min, and the injection volume was 2 μL.
High-resolution mass spectra analysis conditions were as follows: the data were collected in electrospray ionization (ESI) mode with mass range of m/z 100–1000 Da of MS1 and m/z 50–1000 Da of MS2 in both positive and negative detection modes, with a mass resolution of 75,000. The capillary temperature was 320°C, interface voltage was 3.0 kV (−) and 3.5 kV (+), auxiliary gas heater temperature was 350°C, auxiliary gas flow rate was 3 L/min, and sheath gas flow rate was 12 L/min.
2.7 Metabolite characterization
Data analysis was conducted using Xcalibur software. The MQ metabolite extract in the rats was screened as per Li et al. (2017). The structural elucidation strategy of metabolites was conducted in accordance with Li et al. (2020), Zhang et al. (2022), and Su et al. (2023). First, the absorbed compounds were identified by comparison with the previously reported UPLC-Q-Exactive Orbitrap/MS analysis results of MQ (Kong et al., 2022). Second, the skeleton structure of the metabolites was identified by comparing the MS1 and MS2 data with references or elucidating the chemical structures from online databases, such as SciFinder, PubChem, and ChemSpider. Finally, the metabolic reaction type was confirmed by characteristic mass differences, such as 14.01 Da (CH2), 2.01 Da (H2), 176.03 Da (C6H8O6), and 79.95 Da (SO3), respectively denoting methylation, hydrogenation, glucuronidation, and sulfation metabolic reactions.
The absorbed compounds and metabolites were characterized through the analytical methods described above. Subsequently, the precursors of the MQ metabolites were obtained from the proposed metabolic pathways. Then, the quality markers were screened from the absorbed compounds and the precursors of metabolites were correlated with the hepatocyte protection activity, which was validated by reported experimental validation.
2.8 UPLC-QQQ-MS/MS analysis conditions
The UPLC-QQQ-MS/MS assay was performed on an LCMS 8050 triple quadrupole mass spectrometer coupled to the Nexera UPLC with data analysis software Labsolutions LC-MS (Shimadzu, Japan). The column used Acquity UPLC HSS T3 (2.1 × 100 mm, 1.8 μm, Waters) at a column temperature of 40°C. Mobile phase A was acetonitrile, and mobile phase B was 0.1% formic acid solution (containing 10 mmol/L ammonium acetate). The gradient elution procedure was: 5%–60% A at 0–6.5 min; 60%–95%A at 6.5–6.6 min; 95% A at 6.6–8 min; 95%–5%A at 8–8.01 min; 5% B at 8.01–10 min. The flow rate was 0.35 mL/min. Mass spectrometry data were obtained by MRM mode in both positive and negative modes with ESI source. The flow rate of the nebulizer was 3 L/min, drying gas flow rate was 10 L/min, interface temperature was set at 300°C, DL temperature was 250°C, and the heating block temperature was 300°C. The MRM analysis conditions of each target compound for simultaneous analysis are summarized in Table 1.
2.9 Method validation of the established UPLC-QQQ-MS/MS assay
In accordance with the guidelines for the analytical method verification of the Chinese Pharmacopoeia, 2020, Volume 4 (Pharmacopoeia Commission of PRC, 2020), various factors such as linear regression, precision, recovery, limit of detection (LOD), and limit of quantification (LOQ) were investigated. The calibration curve for each target compound was constructed by more than five different concentrations of mixed standard solutions. The sensitivity of the proposed method was evaluated by establishing LOD and LOQ, which were given by concentrations with signal-to-noise ratios (S/N) of 3:1 and 10:1, respectively. Recovery experiments were conducted through six samples of the same MQ sample and spiked authentic standards in MQ sample directly.
3 Results and discussion
3.1 Metabolite characterization of MQ extract in vivo
A total of 13 absorbed compounds (including seven alkaloids, three phenolic acids, two flavonoids, and one other constituent) and 30 metabolites were found in rat urine, feces, plasma, brain, heart, lung, liver, kidney, intestine, and spleen samples. The metabolites consisted of seven phase-I and 23 phase-II metabolites; the chemical structure of these metabolites included 13 alkaloids, 10 flavonoids, and 7 phenolic acids. According to the metabolic reactions, the 23 phase-II metabolites were divided into four groups: methylated metabolites (8), acetylated metabolites (2), sulfated metabolites (9), and glucuronidated metabolites (4). Detailed information of the identification of absorbed compounds, metabolites, and metabolic reactions and the MS data are summarized in Tables 2 and 3; the base peak chromatograms and the distribution of metabolites in main rat organs are shown in Supplementary Figures S1–S10 and Supplementary Tables S2, S3. The structural elucidation of a group of metabolites relevant to meconquintupline is presented below. M5 and M6 exhibited [M + H]+ at m/z 330.17, their molecular formula was predicted to be C19H23NO4, and the ring double bond (RDB) was 8. Compared with meconquintupline (MW: 318 Da, MF: C19H21NO4, RDB: 9), the MW of M5 and M6 were increased by 2 Da, and the RDB decreased by 1. Therefore, M5 and M6 were identified as dihydromeconoquintupline. M5 and M6 displayed the same fragment ions of m/z 271.10, m/z 255.10, m/z 241.08, m/z 195.08, and m/z 192.10 in MS2 spectra. Among these, m/z 192.10 (C11H14NO2) is a characteristic fragment ion produced by the B ring opening reaction for meconoquintupline (Kong et al., 2022). The loss of the whole bridge ring of M5 and M6 produces m/z 195.08. Furthermore, the dehydration of M5 was identified, which demonstrated that hydrogenation could occur in the carbonyl at the C-7 position, where the carbonyl was reduced to oxhydryl. In addition, hydrogenation in M6 may occur in the double bond of C-5 and C-6, given that the loss of carbonyl (C=O) occurred in M6 but not in M5. In particular, the retention time of M6 (tR: 18.2 min) was longer than that of M5 (tR: 14.0 min), which was consistent with a larger Clog P value meaning a longer tR in UPLC. In the present study, the Clog P values of M5 and M6 were 1.4 and 1.7, respectively, which demonstrated that M6 is more hydrophobic than M5. Therefore, M5 and M6 were identified as 7- dihydro meconoquintupline and dihydromeconoquintupline, respectively. The EICs and MS2 data and characteristic fragment ions are shown in Figure 2.
3.2 Potential metabolic pathways and precursors of metabolites
In the present study, the proposed precursors of the metabolites were identified by the parent chemical structures and proposed metabolic pathways of the metabolites. As an example, the proposed metabolic pathways of meconquintupline and caffeic acid are presented in Figure 3. The metabolites of 16 MQ compounds were compared with the relevant literature and analyzed to determine their precursors. Finally, we identified seven metabolites derived from oleracein E (M4, M20, M21, M22, M23, M24, and M25), six derived from caffeic acid (M8, M9, M10, M11, M12, and M13 five from meconquintupline (M5, M6, M7, M26, and M27), two from luteolin (M16 and M17), two from protopine (M29 and M30), and eight metabolites derived from eight MQ compounds—apigenin (M1), quercetin (M2), chlorogenic acid (M3), isorhamnetin (M14), kaempferide (M15), taxifolin (M18), isoquercetin (M19), and O-methylflavinantine (M28). Consequently, these absorbed compounds and the proposed metabolite precursors were selected as candidate quality markers.
3.3 Hepatocyte protection activity of screened candidate quality markers
In order to verify the reliability of the candidate quality markers screened from absorbed compounds and the precursors of metabolites, the references of experimental validation for hepatocyte protection activity were obtained and analyzed. Apigenin is a common natural flavonoid. Yang et al. (2018) have found that apigenin has the effect of improving hepatocellular carcinoma, one of the mechanisms being apigenin inhibiting cell proliferation and inducing autophagy by inhibiting the PI3K/AKT/mTOR pathway. The data obtained from Rashidi et al. (2023) revealed that isorhamnetin significantly decreases HSC-T6 activation in vitro and declines the expression of COLA1 and α-SMA and the protein level of phosphorylated AKT, demonstrating that isorhamnetin improves antifibrotic effect by suppressing the PI3K-AKT signaling pathway. Quercetin could ameliorate the lipid metabolism of nonalcoholic fatty liver disease (NAFLD) progression. The mechanism may be that quercetin treatment reduces gene expression, including AKT phosphorylation, inflammation, oxidative stress, and lipid metabolism, and regulates the PI3K/AKT pathway (Sandra et al., 2015). Luteolin also has similar activity. Ahmed et al. (2022) found that luteolin nanoparticles ameliorated NAFLD by regulating the PI3K/AKT/FoxO1 pathway. Taxifolin is an effective flavonoid for liver protection, possibly by inhibiting the activation of hepatic stellate cells and the production of the extracellular matrix (ECM) by regulating the PI3K/AKT/mTOR and TGF⁃β1/Smads pathways to play an anti-hepatic fibrosis role (Liu et al., 2021). Protopine can inhibit the viability of hepatocellular carcinoma cells and trigger apoptosis in a caspase-dependent manner. Additionally, it exerts an anti-hepatocellular carcinoma effect by inducing the accumulation of ROS in hepatocellular carcinoma cells, thereby inhibiting the PI3K/AKT signaling pathway (Nie et al., 2021). Studies have shown that caffeic acid can revert the imbalance of gut microbiota and ameliorate the inflammatory lipopolysaccharide-mediated responses in a NAFLD mouse model, inhibiting the dysregulation of the gene expression of lipid metabolism and improving NAFLD symptoms (Mu et al., 2021). Chlorogenic acid exhibited an effect of improving liver fibrosis in an NAFLD mice model, possibly by inhibiting HSC activation, which promotes mitochondrial biogenesis and reduces ECM production initiated by HMGB1 in hepatic vascular endothelial cells (Miao et al., 2022). Allocryptopine exhibits a significant hepatocyte protection effect, improvement of liver function, and anti-hepatic fibrosis effects in rats with CCl4-induced liver fibrosis. It can significantly reduce the expression of liver index, spleen index, AST, ALT, and collagen (CoI, CoIII) in liver tissue (Xiao et al., 2011). Therefore, nine candidate quality markers possess hepatoprotective effects and are absorbable, and these can be detected using reference standards. Consequently, we deduced that apigenin, isorhamnetin, quercetin, luteolin, taxifolin, protopine, allocryptopine, caffeic acid, and chlorogenic acid could be the quality markers of MQ.
The quality markers we have identified in MQ are common compounds, and no specific constituents unique to MQ have been used as quality markers. This poses certain challenges in distinguishing MQ from other botanical drugs of the genus Meconopsis during the quality control processes. However, our previous study on Blumea riparia and B. megacephala found that, although the chemical compounds were similar, their proportions could differ between the two botanical drugs (Su et al., 2023). Other studies have also observed this phenomenon (Kong et al., 2017; Yang et al., 2017). Therefore, we speculate that quality control based on multiple quality markers can achieve differentiation among different botanical drugs of the genus Meconopsis.
3.4 Method development and quantification of nine quality markers by UPLC-QQQ-MS/MS
3.4.1 Optimization of extraction and UPLC-QQQ-MS/MS conditions
To ensure the extraction efficiency of the compounds being investigated, we investigated as extraction methods different proportions of methanol solvent, material–liquid ratios, and extraction times of MQ samples. The results showed that 70% methanol as the extraction solvent, a material–liquid ratio of 1:75, and heating reflux extraction for 30 min were the best extraction conditions (Supplementary Tables S4–S7). For the chromatographic conditions, according to our previous study (Kong et al., 2022), an acetonitrile–0.1% formic acid solution mobile phase system was preferred for the peak separation of flavonoids and phenolic acids. However, several studies have demonstrated that ammonium acetate could improve the ionization and chromatographic peak shape for alkaloids (Wang et al., 2017). Therefore, acetonitrile-0.1% formic acid solution (containing 10 mmol ammonium acetate) was optimized as the mobile phase system.
To fully understand the MS characteristics and select appropriate product ions of the nine quality markers, the fragmentation behavior of these compounds was investigated. Among the nine compounds investigated, the chemical structure of apigenin, luteolin, quercetin, and isorhamnetin was that of flavones and flavonols, and they were analyzed in the negative mode. In the MS2 spectrogram after CID cracking, the products of Retro–Diels–Alder (RDA) cleavage were characteristic fragments, such as m/z 117 (1,3B−) from apigenin, m/z 133 (1,3B−) from luteolin, and m/z 151 (1,3A−) from quercetin and isorhamnetin. These fragments could be used as product ions in MRM analysis except for isorhamnetin, because the RDA product of isorhamnetin was not the dominant fragment ion. The dominant fragment ion of isorhamnetin was m/z 300 [M-H-CH3•]-•, which was the product from the loss of CH3•. Differently from these three compounds where C-2 and C-3 had a double bond, taxifolin is a flavanonol; for the saturated bond in C-2 and C-3, the dehydration product m/z 285 [M-H-H2O]- was the dominant fragment ion, which could be used as product ion in MRM analysis (Figure 4). Protopine and allocryptopine are isoquinoline alkaloids, and they were analyzed in the positive ion mode. The chemical structure of allocryptopine is similar to that of protopine, and the fragmentation pathway is also similar. Protopine and allocryptopine displayed abundant fragment ions of m/z 336, m/z 306, m/z 206, m/z 188, and m/z 149 in MS2 spectra. In particular, m/z 206 was a diagnostic fragment ion produced by RDA cleavage from the parent ion; the ion of m/z 188 was a dehydration product from m/z 206; m/z 188 was also the dominant fragment ion in MS2 spectra. Therefore, m/z 188 could be used as product ion in MRM analysis (Figure 4).
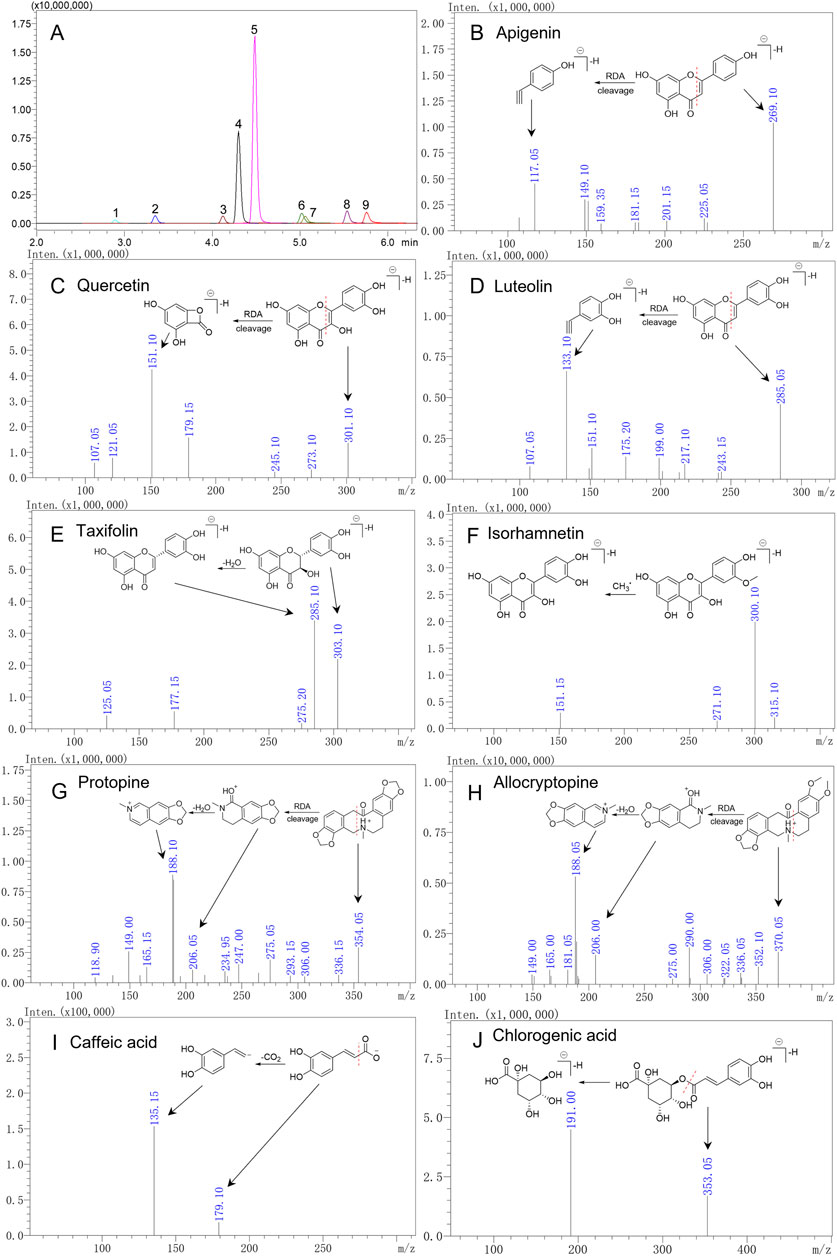
Figure 4. Extracted ion chromatogram of nine quality markers in the MRM mode (A) and secondary mass spectra of nine compounds (B–J). 1. Chlorogenic acid, 2. caffeic acid, 3. taxifolin, 4. protopine, 5. allocryptopine, 6. luteolin, 7. quercetin, 8. apigenin, and 9. isorhamnetin.
Yang W. Y. et al. (2016) and Shen et al. (2022) have sought to simultaneously determine the content of different polarity compounds in TCM. The highly specific MRM technique can accurately quantify the analytes of interest with MS or MS2 characteristics in the positive and negative ion modes. Hence, in the present study, polarity-switching used for MRM analysis was selected to analyze MQ samples in order to simultaneously determine flavonoids, phenolic acids, and alkaloids. In MRM analysis, it is crucial to determine the precursor ions of each compound and its product ions. Usually, the precursor ions were determined in Q1 MS mode, and then one to two product ions with higher abundance were selected according to the results of its product ion scanning. Finally, collision voltage (CE) was optimized to ensure the best MRM conditions. Under optimized conditions, nine quality markers were isolated and eluted in 10 min (Figure 4), with detailed parameters listed in Table 1.
3.4.2 Method validation of the developed UPLC-QQQ-MS/MS MRM assay
To evaluate the sensitivity and precision of the established UPLC-QQQ-MS/MS method, the linearity, LOD, LOQ, precision, stability, repeatability, and recovery of the nine target compounds were verified, with the results in Table 4 and Supplementary Tables S8–S10. The standard curves for all nine target compounds showed good linear fit in the range of 100–2000 ng/mL, and the correlation coefficients (r) were above 0.99. The LOD and LOQ values of the nine target compounds were 0.81–4.15 ng/mL and 2.47–12.59 ng/mL, respectively, indicating high sensitivity under the optimized chromatographic conditions. Precision was evaluated by the spiked standard at a concentration of 1000 ng/mL. The results showed that the RSD value of each compound varied 0.78–2.97%, indicating good precision. In addition, repeatability was tested for the MQ1 sample in sextuplicate; the concentrations of the nine target compounds were calculated, and the mean repeatability expressed as RSD was 2.49–5.68%. Accuracy was assessed by the MQ1 sample in sextuplicate with a known amount of standard with 100% level that was compared with the concentration of the nine target compounds in the MQ1 sample. Recovery was calculated by the following formula: recovery (%) = (amount detected − original amount)/100%. In the present study, the mean RSD of the recovery test in our developed analytical method was 85.71–103.78%. Finally, stability was examined under the MQ1 sample at 0, 2, 4, 6, 10, and 24 h in triplicate; the results showed that the RSD of the peak area of the nine target compounds was 1.37%–4.79%. These results indicated that the UPLC-QQQ-MS/MS method that we developed was accurate, precise, sensitive, and reliable enough to quantitatively determine simultaneously the nine quality markers in the MQ samples.
3.4.3 Quantification of quality markers in MQ by UPLC-QQQ-MS/MS
The UPLC-QQQ-MS/MS method developed was successfully applied to the quantitative analysis of quality markers belonging to flavonoids, alkaloids, and phenolics in MQ samples collected from different areas at different altitudes of Gansu, Qinghai, and Sichuan provinces in China. All compounds investigated were eluted within 10 min in the polarity-switching positive and negative ion modes of the ESI source. The content of the nine quality markers was detected at the average range of 1.02–137.29 μg/g; among them, luteolin and taxifolin had relatively large amounts in the MQ samples (Table 5). A comparison between the sample collected at the highest altitude (MQ1) and the batches collected at the lowest altitudes (MQ8) revealed a decreasing trend in alkaloid content with increasing altitude; for example, the protopine content in MQ1 was 5.83 μg/g but was 13.14 μg/g in MQ8. In contrast, the flavonoid content exhibited an increasing trend with higher altitudes; for example, the isorhamnetin content in MQ1 was 10.48 μg/g but was 6.22 μg/g in MQ8. Zhang et al. 2010) also found a similar trend in MQ, with the content of quercetin and luteolin significant increasing with rising altitude in the Qinghai Dalijia area. However, in the Lajishan area, there was a trend of first decrease and then increase. Pandey et al. (2018) also reported similar results in alkaloid and flavonoids. They found that the berberine content of Thalictrum foliolosum varied inversely with altitude, the flavonoid and phenolic content of T. foliolosum increased at higher altitudes, while the content of these compounds may still vary according to growth and season (Pandey et al., 2018).
4 Conclusion
In the present study, a total of 13 absorbed compounds (including seven alkaloids, three phenolic acids, two flavonoids, and one other compound) and 30 metabolites of MQ extract were found in rat urine, feces, plasma, brain, heart, lung, liver, kidney, intestine, and spleen samples. The nine absorbed compounds and the precursors of metabolites, luteolin, isorhamnetin, quercetin, apigenin, allocrytopine, taxifolin, protopine, caffeic acid, and chlorogenic acid were related to hepatocyte protection activity, which selected them as the quality markers.
The established UPLC-QQQ-MS/MS quantitative method presented reasonable linearity, precision, accuracy, repeatability, stability and recovery for the nine quality markers. These markers were all detected in MQ crude drug. Among them, luteolin and taxifolin had relatively large amounts in the MQ samples. There are variations in the quality of MQ samples sourced from different areas and altitudes, with the variations presenting a decreasing trend in alkaloids with increasing altitude and an increasing trend in flavonoids with increasing altitude.
This study used an absorption-based metabolite strategy to successfully explore, discover, and identify the quality markers of MQ, and a universal and rapid method based on the polarity-switching UPLC-QQQ-MS/MS technology was established for the simultaneous determination of quality markers in MQ. Our study provides a research strategy to optimize quality markers and more rigorous quality control of TCM.
Data availability statement
The original contributions presented in the study are included in the article/Supplementary Material, further inquiries can be directed to the corresponding author.
Ethics statement
The animal study was approved by the Biomedical Ethical Committee of Southwest Minzu University. The study was conducted in accordance with the local legislation and institutional requirements.
Author contributions
YT: formal analysis, investigation, methodology, and writing–original draft. YL: writing–original draft, conceptualization, and funding acquisition. YK: writing–original draft, formal analysis, and investigation. LL: formal analysis, investigation, and writing–review and editing. ZY: investigation, methodology, and writing–review and editing. WL: methodology and writing–review and editing. QL: methodology and writing–review and editing. CR: investigation and writing–review and editing. WS: investigation and writing–review and editing. YH: writing–review and editing, conceptualization, funding acquisition, project administration, and supervision.
Funding
The authors declare that financial support was received for the research, authorship, and/or publication of this article. This work was supported by the grants from the Natural Science Foundation of Sichuan Province (2022NSFSC1605), the Special Fund of Natural Science for the Central Universities (ZYN2023099), the Engineering Research Center for Pharmaceuticals and Equipment of Sichuan Province Fund (ERCPEF2201), and the First Batch of Scientific and Technological Innovation Team Projects for the Institute of the Qinghai–Tibet Plateau at Southwest Minzu University (2024CXTD04).
Conflict of interest
Author QL was employed by Shimadzu China Co. LTD.
The remaining authors declare that the research was conducted in the absence of any commercial or financial relationships that could be construed as a potential conflict of interest.
Publisher’s note
All claims expressed in this article are solely those of the authors and do not necessarily represent those of their affiliated organizations, or those of the publisher, the editors, and the reviewers. Any product that may be evaluated in this article, or claim that may be made by its manufacturer, is not guaranteed or endorsed by the publisher.
Supplementary material
The Supplementary Material for this article can be found online at: https://www.frontiersin.org/articles/10.3389/fphar.2024.1474768/full#supplementary-material
References
Ahmed, E. S., Mohamed, H. E., and Farrag, M. A. (2022). Luteolin loaded on zinc oxide nanoparticles ameliorates non-alcoholic fatty liver disease associated with insulin resistance in diabetic rats via regulation of PI3K/AKT/FoxO1 pathway. Int. J. Immunopathol. Pharmacol. 36, 03946320221137435. doi:10.1177/03946320221137435
Chinese Pharmacopoeia Commission (1995). Herba Meconopsis in: pharmaceutical standards of the Ministry of Health of the people’s Republic of China.
Duan, S., Niu, L., Yin, T., Li, L., Gao, S., Yuan, D., et al. (2020). A novel strategy for screening bioavailable quality markers of traditional Chinese medicine by integrating intestinal absorption and network pharmacology: application to Wu Ji Bai Feng Pill. Phytomedicine 76, 153226. doi:10.1016/j.phymed.2020.153226
Guo, Q., Bai, R. F., Zhao, B. S., Feng, X., Zhao, Y. F., Tu, P. F., et al. (2016). An ethnopharmacological, phytochemical and pharmacological review of the genus Meconopsis. Am. J. Chin. Med. 44, 439–462. doi:10.1142/S0192415X16500257
He, J. S., Huang, B., Ban, X. Q., Tian, J., Zhu, L., and Wang, Y. W. (2012). In vitro and in vivo antioxidant activity of the ethanolic extract from Meconopsis quintuplinervia. J. Ethnopharmacol. 141, 104–110. doi:10.1016/j.jep.2012.02.006
Huang, Y. J., Cheng, P., Zhang, Z. Y., Tian, S. J., Sun, Z. L., Zeng, J. G., et al. (2018). Biotransformation and tissue distribution of protopine and allocryptopine and effects of Plume Poppy total alkaloid on liver drug-metabolizing enzymes. Sci. Rep. 8, 537. doi:10.1038/s41598-017-18816-7
Jiao, Y., Chen, D. L., Fan, M. T., and Young Quek, S. (2019). UPLC-QqQ-MS/MS-based phenolic quantification and antioxidant activity assessment for thinned young kiwifruits. Food Chem. 281, 97–105. doi:10.1016/j.foodchem.2018.12.062
Kong, Y., Wang, Q. H., Shang, M. Y., Xiao, J. J., Meng, S. C., and Cai, S. Q. (2017). Effects of active constituents of Sinopodophylli Fructus on cell proliferation, cell cycle and mitochondrial membrane potential of human breast cancer cell. China Pharm. 28, 1368–1371.
Kong, Y. L., Zhang, J. G., Su, H. N., La, M. J., Lan, J. L., Yang, Z. M., et al. (2022). Chemical constituents and anti-liver fibrosis mechanism of Meconopsis quintuplinervia based on UPLC-Q-Exactive-MS/MS and network pharmacology. China J. Chin. Mat. Medica 44, 6097–6116. doi:10.19540/j.cnki.cjcmm.20220917.201
Li, H. F., Li, T., Yang, P., Wang, Y., Tang, X. J., Liu, L. J., et al. (2020). Global profiling and structural characterization of metabolites of ononin using HPLC-ESI-IT-TOF-MSn after oral administration to rats. J. Agric. Food Chem. 68, 15164–15175. doi:10.1021/acs.jafc.0c04247
Li, H. F., Xu, F., Yang, P., Liu, G. X., Shang, M. Y., Wang, X., et al. (2017). Systematic screening and characterization of prototype constituents and metabolites of total astragalosides using HPLC-ESI-IT-TOF-MSn after oral administration to rats. J. Pharm. Biomed. Anal. 142, 102–112. doi:10.1016/j.jpba.2017.05.009
Li, M. R., Si, D. D., Fu, Z. F., Sang, M. M., Zhang, Z., Liu, E. W., et al. (2019a). Enhanced identification of the in vivo metabolites of Ecliptae Herba in rat plasma by integrating untargeted data-dependent MS2 and predictive multiple reaction monitoring-information dependent acquisition-enhanced product ion scan. J. Chromatogr. B 1109, 99–111. doi:10.1016/j.jchromb.2019.02.001
Li, W. J., Cai, Y. F., Ouyang, Y., Li, X. Y., Shi, X. L., Cao, S. X., et al. (2024). Quality evaluation of Angelica Sinensis Radix dispensing granules by integrating microvascular activity and chemical analysis. J. Ethnopharmacol. 319, 117236. doi:10.1016/j.jep.2023.117236
Li, X. Y., Fu, Y. J., Fu, Y. F., Wei, W., Xu, C., Yuan, X. H., et al. (2022a). Simultaneous quantification of fourteen characteristic active compounds in Eucommia ulmoides Oliver and its tea product by ultra-high performance liquid chromatography coupled with triple quadrupole mass spectrometry (UPLC-QqQ-MS/MS). Food Chem. 389, 133106. doi:10.1016/j.foodchem.2022.133106
Li, Y. L., Ju, S. S., Lin, Z. J., Wu, H., Wang, Y., Jin, H. Y., et al. (2022b). Bioactive-chemical quality markers revealed: an integrated strategy for quality control of chicory. Front. Nutr. 9, 934176. doi:10.3389/fnut.2022.934176
Li, Y. Z., Xie, Y., He, Y. F., Hou, W. B., Liao, M. L., and Liu, C. X. (2019b). Quality markers of traditional Chinese medicine: concept, progress, and perspective. Engineering 5, 888–894. doi:10.1016/j.eng.2019.01.015
Li, Z. Q., Liu, J., Li, Y. Z., Du, X., Li, Y. F., Wang, R. H., et al. (2018). Identify super quality markers from prototype-based pharmacokinetic markers of Tangzhiqing tablet (TZQ) based on in vitro dissolution/permeation and in vivo absorption correlations. Phytomedicine 45, 59–67. doi:10.1016/j.phymed.2018.04.001
Liu, C. X., Chen, S. L., Xiao, X. H., Zhang, T. J., Hou, W. B., and Liao, M. L. (2016). A new concept on quality marker of Chinese materia medica: quality control for Chinese medicinal products. Chin. Tradit. Herb. Drugs 47, 1443–1457.
Liu, C. X., Guo, D. A., and Liu, L. (2018). Quality transitivity and traceability system of herbal medicine products based on quality markers. Phytomedicine 44, 247–257. doi:10.1016/j.phymed.2018.03.006
Liu, W. J., Li, W., Zhang, P. J., Gong, X. C., Tu, P., Tang, L., et al. (2022). Quality structural annotation for the metabolites of chlorogenic acid in rat. Food Chem. 379, 132134. doi:10.1016/j.foodchem.2022.132134
Liu, X. L., Liu, W. C., Ding, C. B., Zhao, Y. C., Chen, X., Ling, D., et al. (2021). Taxifolin, extracted from waste larix olgensis roots, attenuates CCl4-induced liver fibrosis by regulating the PI3K/AKT/mTOR and TGF-β1/Smads signaling pathways. Drug Des. devel. Ther. 15, 871–887. doi:10.2147/DDDT.S281369
Lu, L. L., Qian, D. W., Yang, J., Jiang, S., Guo, J. M., Shang, E. X., et al. (2013). Identification of isoquercitrin metabolites produced by human intestinal bacteria using UPLC-Q-TOF/MS. Biomed. Chromatogr. 27, 509–514. doi:10.1002/bmc.2820
Luo, D. S., Sun, A. L., and Xia, G. C. (1984). Tibetan drug in Qingzang plateau, a preliminary investigation of resources Meconopsis. Chin. Tradit. Herb. Drugs 15, 23–24.
Miao, H., Ouyang, H., Guo, Q., Wei, M. J., Lu, B., Kai, G. Y., et al. (2022). Chlorogenic acid alleviated liver fibrosis in methionine and choline deficient diet-induced nonalcoholic steatohepatitis in mice and its mechanism. J. Nutr. Biochem. 106, 109020. doi:10.1016/j.jnutbio.2022.109020
Mu, H. N., Zhou, Q., Yang, R. Y., Tang, W. Q., Li, H.-X., Wang, S. M., et al. (2021). Caffeic acid prevents non-alcoholic fatty liver disease induced by a high-fat diet through gut microbiota modulation in mice. Food Res. Int. 143, 110240. doi:10.1016/j.foodres.2021.110240
Ni, L. Q., Lu, H., Gao, M., and Fan, X. P. (2023). In vivo metabolites of isorhamnetin in mice after oral administration based on UPLC⁃Q/TOF⁃MS/MS. Mil. Med. Sci. 47, 364–370. doi:10.7644/j.issn.1674⁃9960.2023.05.008
Nie, C. H., Wang, B., Wang, B. Q., Lv, N., Yu, R., and Zhang, E. F. (2021). Protopine triggers apoptosis via the intrinsic pathway and regulation of ROS/PI3K/Akt signalling pathway in liver carcinoma. Cancer Cell Int. 21, 396. doi:10.1186/s12935-021-02105-5
Pandey, G., Khatoon, S., Pandey, M. M., and Rawat, A. K. S. (2018). Altitudinal variation of berberine, total phenolics and flavonoid content in Thalictrum foliolosum and their correlation with antimicrobial and antioxidant activities. J. Ayurveda Integr. Med. 9, 169–176. doi:10.1016/j.jaim.2017.02.010
Qin, X. L., Sun, H. Y., Yang, W., Li, Y. J., Zheng, L., Liu, T., et al. (2017). Analysis of metabolites of quercitrin in rat intestinal flora by using UPLC-ESI-Q-TOF-MS/MS. China J. Chin. Mat. Medica 42, 357–362. doi:10.19540/j.cnki.cjcmm.20161222.018
Rashidi, M., Matour, E., Beheshti Nasab, H., Cheraghzadeh, M., and Shakerian, E. (2023). Isorhamnetin exerts antifibrotic effects by attenuating platelet-derived growth factor-BB-induced HSC-T6 cells activation via suppressing PI3K-AKT signaling pathway. Iran. Biomed. J. 27, 199–204. doi:10.61186/ibj.3948
Sandra, P. V., Ángel, M. F., María, V. G. M., Susana, M. F., Anna, F., Marta, B., et al. (2015). Quercetin ameliorates dysregulation of lipid metabolism genes via the PI3K/AKT pathway in a diet-induced mouse model of nonalcoholic fatty liver disease. Mol. Nutr. Food Res. 59, 879–893. doi:10.1002/mnfr.201400913
Shang, X. Y., Li, C., Zhang, C. Z., Yang, Y. C., and Shi, J. G. (2006a). Non-alkaloid constituents from a Tibetan medicine Meconopsis quintuplinervia. China J. Chin. Mat. Medica 31, 468–471.
Shang, X. Y., Shi, J. G., Yang, Y. C., Liu, X., Li, C., and Zhang, C. Z. (2003). Alkaloids from a Tibetan medicine Meconopsis quintuplinervia Regel. Acta Pharm. Sin. 38, 276–278. doi:10.16438/j.0513-4870.2003.04.009
Shang, X. Y., Wang, Y. H., Li, C., Zhang, C. Z., Yang, Y. C., and Shi, J. G. (2006b). Acetylated flavonol diglucosides from Meconopsis quintuplinervia. Phytochemistry 67, 511–515. doi:10.1016/j.phytochem.2005.12.002
Shang, X. Y., Zhang, C. Z., Li, C., Yang, Y. C., and Shi, J. G. (2002). Studies on chemical constituents of Meconopsis quintuplinervia Regel. J. Chin. Med. Mat. 25, 250–252. doi:10.13863/j.issn1001-4454.2002.04.010
Shen, Q., Wang, H. X., Quan, B., Sun, X. H., Wu, G. H., Huang, D. R., et al. (2022). Rapid quantification of bioactive compounds in Salvia miltiorrhiza Bunge derived decoction pieces, dripping pill, injection, and tablets by polarity-switching UPLC-MS/MS. Front. Chem. 10, 964744. doi:10.3389/fchem.2022.964744
Shi, B. M., Yang, L. J., Gao, T., Ma, C., Li, Q., Nan, Y. F., et al. (2019). Pharmacokinetic profile and metabolite identification of bornyl caffeate and caffeic acid in rats by high performance liquid chromatography coupled with mass spectrometry. RSC Adv. 9, 4015–4027. doi:10.1039/c8ra07972b
Shi, X., Cheng, F., Guo, M., and Shao, J. (2011). Determination of O-methylflavinantine in a Tibetan medicine Meconopsis quintuplinervia by HPLC. China J. Chin. Mat. Medica 36, 3290–3292.
Su, H. N., Li, X. X., Li, Y., Kong, Y. L., Lan, J. L., Huang, Y. F., et al. (2023). Chemical profiling and rapid discrimination of Blumea riparia and Blumea megacephala by UPLC-Q-Exactive-MS/MS and HPLC. Chin. Herb. Med. 15, 317–328. doi:10.1016/j.chmed.2022.06.009
Sun, Z., Liu, X., Zuo, W., Fu, Q., Xu, T. T., Cui, L. Y., et al. (2023). Development of a robust UPLC-MS/MS method for the quantification of riluzole in human plasma and its application in pharmacokinetics. Front. Pharmacol. 14, 1227354. doi:10.3389/fphar.2023.1227354
Wang, H. Y., and Ma, W. Y. (2009). Fifty-six cases of chronic severe hepatitis treated by Tibetan Medicine Ershiwuwei Luronghao Wan. Chin. Community Dr. 11, 145.
Wang, J. L., Zheng, D. D., Wang, Y. Z., Zhang, C., and Sun, X. M. (2017). Pharmacokinetics study of Erhuang decoction extracts in rats by HPLC-MS/MS. J. Chromatogr. B-Anal. Technol. Biomed. Life Sci. 1059, 35–42. doi:10.1016/j.jchromb.2017.05.019
Wang, Z. W., Shao, J., Guo, M., Wang, R. Q., and Ren, Y. (2013a). Effect of total flavonoids and alkaloids from Meconopsis quintuplinervia on hepatic fibrosis in rat. Chin. Tradit. Pat. Med. 35, 1125–1128. doi:10.3969/j.issn.1001-1528.2013.06.004
Wang, Z. W., Wang, R. Q., Guo, M., Shao, J., and Ren, Y. (2013b). Study on liver protection of total flavones of Meconopsis quintuplinervia from Gansu province in mice. Chin. J. Exp. Tradit. Med. Formulae 19, 206–209.
Wei, X. Y., Guo, W. J., Chen, Z. Y., Qiu, Z. D., Guo, J., Cui, G. H., et al. (2021). Chemical-activity-based quality marker screening strategy for Viscum articulatum. Biomed. Chromatogr. 35, e5175. doi:10.1002/bmc.5175
Wu, H. F., Pan, L., Ding, L. S., and Zhang, X. F. (2007). Chemical constituents of a Tibetan medicine Meconopsis quintuplinervia Regel. Nat. Prod. Res. Dev. 19, 811–813. doi:10.16333/j.1001-6880.2007.05.022
Xiao, L., Zeng, J. G., Liu, L., Wang, Y. H., Zhong, M., He, X. H., et al. (2011). Effect of allocryptopine on antagonizing hepatic fibrosis. Chin. Tradit. Herb. Drugs 42, 1158–1163.
Xu, J. J., Xu, F., Wang, W., Zhang, Y. F., Hao, B.-Q., Shang, M. Y., et al. (2022). Elucidation of the mechanisms and effective substances of Paeoniae Radix Rubra against toxic heat and blood stasis syndrome with a stage-oriented strategy. Front. Pharmacol. 13, 842839. doi:10.3389/fphar.2022.842839
Yang, J. L., Pi, C. C., and Wang, G. H. (2018). Inhibition of PI3K/Akt/mTOR pathway by apigenin induces apoptosis and autophagy in hepatocellular carcinoma cells. Biomed. Pharmacother. 103, 699–707. doi:10.1016/j.biopha.2018.04.072
Yang, M. F., Li, H., Xu, X. R., Zhang, Q. Y., Wang, T. Y., Feng, L., et al. (2023). Comprehensive identification of metabolites and metabolic characteristics of luteolin and kaempferol in Simiao Yong′an Decoction in rats by UHPLC-LTQ-Orbitrap MS/MS. China J. Chin. Mat. Medica 48, 6191–6199. doi:10.19540/j.cnki.cjcmm.20230621.201
Yang, P., Xu, F., Li, H. F., Wang, Y., Li, F. C., Shang, M. Y., et al. (2016a). Detection of 191 taxifolin metabolites and their distribution in rats using HPLC-ESI-IT-TOF-MSn. Molecules 21, 1209. doi:10.3390/molecules21091209
Yang, W. Y., Han, Q. J., Guo, P. P., Wang, C. Y., and Zhang, Z. J. (2016b). Simultaneous detection of flavonoids, phenolic acids and alkaloids in Abri herba and Abri Mollis herba using liquid chromatography tandem mass spectrometry. Phytochem. Anal. 27, 50–56. doi:10.1002/pca.2598
Yang, Z. W., Xu, F., Liu, X., Cao, Y., Tang, Q., Chen, Q. Y., et al. (2017). An untargeted metabolomics approach to determine component differences and variation in their in vivo distribution between Kuqin and Ziqin, two commercial specifications of Scutellaria Radix. RSC Adv. 7, 54682–54695. doi:10.1039/C7RA10705F
Yu, R. X., Zhang, Y. P., Wu, N., Wang, X., Mei, L. J., and Yang, X. B. (2021). Study on anti-inflammatory effects of total alkaloids extracted from Meconopsis quintuplinervia Regel. Tradit. Chin. Drug Res. Clin. Pharmacol. 32, 461–466. doi:10.19378/j.issn.1003-9783.2021.04.002
Yue, P. P., Sun, J., Zhang, C. X., Ye, R. R., Lu, X. F., Zhou, Y. B., et al. (2010). HPLC-DAD separation and determination of major active constituents in an important Tibetan medicine Meconopsis quintuplinervia from different regions of Qinghai-Tibet Plateau. J. Med. Plants Res. 4, 1053–1058.
Zhang, C. X., Ye, R. R., Lu, X. F., Yue, P. P., Yang, S. B., Liu, W. H., et al. (2010). Variations on the contents of quercetin and luteolin of Meconopsis quintuplinervia Regel from different altitudes. Nat. Prod. Res. Dev. 22, 643–646.
Zhang, J., Lv, Y., Zhang, J., Shi, W. J., Guo, X. Y., Xu, J. J., et al. (2022). Metabolism of paeoniae radix rubra and its 14 constituents in mice. Front. Pharmacol. 13, 995641. doi:10.3389/fphar.2022.995641
Keywords: traditional Chinese medicine, Meconopsis quintuplinervia Regel, quality markers, metabolism, LC-MS, content determination
Citation: Tian Y, Liu Y, Kong Y, Li L, Yang Z, Li W, Li Q, Ren C, Sun W and Huang Y (2024) Discovery of quality markers of Meconopsis quintuplinervia based on an absorption-based metabolite approach and rapid quantification using polarity-switching UPLC-QQQ-MS/MS. Front. Pharmacol. 15:1474768. doi: 10.3389/fphar.2024.1474768
Received: 02 August 2024; Accepted: 11 November 2024;
Published: 06 December 2024.
Edited by:
Michael Heinrich, University College London, United KingdomReviewed by:
Xie-an Yu, Shenzhen Institute For Drug Control, ChinaMahmood Oppong, University of Ghana, Ghana
Copyright © 2024 Tian, Liu, Kong, Li, Yang, Li, Li, Ren, Sun and Huang. This is an open-access article distributed under the terms of the Creative Commons Attribution License (CC BY). The use, distribution or reproduction in other forums is permitted, provided the original author(s) and the copyright owner(s) are credited and that the original publication in this journal is cited, in accordance with accepted academic practice. No use, distribution or reproduction is permitted which does not comply with these terms.
*Correspondence: Yanfei Huang, aHVhbmd5ZkBiam11LmVkdS5jbg==
†These authors have contributed equally to this work