- 1Chemical Research Division, BCSIR Dhaka Laboratories, Bangladesh Council of Scientific and Industrial Research (BCSIR), Dhaka, Bangladesh
- 2Department of Pharmaceutical Chemistry, Faculty of Pharmacy, University of Dhaka, Dhaka, Bangladesh
- 3Department of Pharmacy, Faculty of Science and Engineering, International Islamic University Chittagong, Chittagong, Bangladesh
Background: Colocasia affinis Schott and Colocasia gigantea Hook.f. are two commonly found vegetable species of the genus Colocasia, found mainly in the Asian region.
Objectives: The objective of this study was to isolate bioactive phytochemicals from C. affinis and C. gigantea and elucidate their structure employing the NMR technique followed by bioactivity evaluation.
Methodology: Column chromatography was utilized to isolate phytochemicals, followed by NMR analysis for characterization. An in vivo analgesic test was performed through an acetic acid-induced writhing test, an anti-inflammatory test was performed through a formalin-induced licking test, and an antidiarrheal test was performed through a castor oil-induced diarrhea model. The in vitro antimicrobial test was executed through the disc diffusion method. Computer-aided simulation was also implemented to validate the wet laboratory results.
Results: Six compounds from C. affinis and C. gigantea were isolated and characterized from the dichloromethane (DCM)-soluble fractions of the methanolic extracts of these two species. Three of the compounds were from C. gigantea and proposed as penduletin (C1), a mixture of α-amyrin (C2a), β-amyrin (C2b), and monoglyceride of stearic acid (C3), while the remaining three compounds were from C. affinis and proposed as penduletin (C4) (which was also isolated from C. gigantea), 7,8-(3″,3″-dimethyl-pyrano)-4′-hydroxy flavonol (C5), and lastly a mixture of 7,8-(3″,3″-dimethyl-pyrano)-4′-hydroxy flavonol (C5) and 4′,7,8-trihydroxy flavonol (C6). These compounds showed promising zones of inhibition against Gram-positive and Gram-negative bacteria and fungi. In the antidiarrheal test, C5 demonstrated the highest reduction in castor oil-induced diarrhea (44.44%) at a dose of 20 mg/kg compared to loperamide’s 77.78% reduction. However, the analgesic assessment showed a noteworthy inhibition of acetic acid-induced writhing by C1/C4 and C2 (56.52%) at a dose of 20 mg/kg compared to the 76.09% by diclofenac sodium. In comparison, C2 showed pronounced anti-inflammatory action by 68.15% and 52.06% reduction, respectively, in the early and later phases compared to the ibuprofen’s outcomes of 73.54% and 74.68%. Plausible targets such as dihydrofolate reductase (DHFR) for antimicrobial, kappa opioid receptor (KOR) for antidiarrheal, and cyclooxygenase 2 (COX-2) for anti-inflammatory and analgesic activities showed a noteworthy binding affinity with isolated compounds, and ADME/T studies displayed these phytochemicals’ drug-likeness profiles.
Conclusion: To the best of our knowledge, this is the first report on compound isolation from these plants, which demands further extensive research for more absolute findings.
Introduction
Since time immemorial, mankind has used plant extracts from different medicinal plants to cure many diseases and thus relieve physical agony, playing an essential role in the world’s healthcare (Yadav et al., 2006). A wide array of phytoconstituents, called secondary metabolites, which do not appear to contribute directly to plant growth and reproduction, are responsible for the pharmacological and therapeutic effects. Thus, purification, isolation, and bioactive assessment of such secondary metabolites from medicinal and vegetative plants are widely practiced (Duraipandiyan et al., 2006).
Araceae plants (family Arum ) are commonly known as “aroids” and are distributed all over the world, and are abundant in tropical and sub-tropical regions (Ara and Hassan, 2019). Colocasia is one of the 27 genera of the Araceae family available in Bangladesh. It is also native to southeastern Asia and the Indian subcontinent (Wagner et al., 1990; Ara and Hassan, 2019). In Bangladesh, this genus of flowering plants is known to contain the following nine species: C. affinis Schott, C. esculenta (L.) Schott, C. fallax Schott, C. gigantea (Blume) Hook. f., C. heterochroma H. Li et Z.X. and Wei, C. lihengiae C.L. Long et K.M. Liu, C. mannii Hook. f., C. oresbia A. Hay, and C. virosa Kunth (Ara and Hassan, 2012). Colocasia leaves have demonstrated antidiabetic, antihypertensive, immunoprotective, neuroprotective, and anticarcinogenic activities. Detailed assessment of the phytochemical compounds present in various extracts of the leaves has shown the presence of active chemical compounds like anthraquinones, apigenin, catechins, cinnamic acid derivatives, vitexin, and isovitexin, which are possibly responsible for the exhibited biological properties (Gupta et al., 2019). Phytochemical extraction and structural elucidation of Colocasia leaves also yield notable bioactive chemical compounds, such as isoorientin, orientin, isoschaftoside, Lut-6-C-Hex-8-C-Pent, vicenin, alpha-amyrin, beta-amyrin, monoglycerol stearic acid, penduletin anthraquinones, apigenin, catechins, cinnamic acid derivatives, vitexin, and isovitexin (Gupta et al., 2019).
Pathogenic bacteria are one of the leading causes of morbidity and mortality in humans, driving pharmaceutical companies to develop numerous new antibacterial agents to address infections, which have become a global concern. Clinical microbiologists are particularly interested in plant-derived antimicrobials for two primary reasons: the potential of phytochemicals to serve as effective antimicrobial agents prescribed by healthcare professionals, and the need to increase awareness of the risks associated with the misuse of conventional antibiotics. Additionally, diarrhea is a common condition marked by frequent bouts of watery bowel movements and abdominal pain. It is a major problem in developing countries, often leading to malnutrition and even death (Workneh et al., 2024). Plant extracts have shown promise in treating diarrhea by helping the body reabsorb water, preventing electrolyte loss, and slowing gut movements (Agbor et al., 2004; Alam et al., 2020). Furthermore, inflammation manifests through a multitude of pathways and different mediators, leading to a spectrum of adverse effects like necrosis, degeneration, and various forms of exudation (Medzhitov, 2008). To alleviate pain and inflammation, analgesic medications such as opioids (like morphine and fentanyl), NSAIDs, and emerging treatments such as gabapentin, carbamazepine, and ketamine are commonly employed. Glucocorticoids exert their effects by binding to receptors, resulting in enhanced transcription of anti-inflammatory proteins (such as IL-1 antagonists) and inhibition of activated transcription factors (e.g., NF-κB) (Barnes, 1998). NSAIDs also inhibit cyclooxygenase enzymes (COX-1 and COX-2), which are responsible for synthesizing various inflammatory mediators. Despite the array of NSAIDs available, they pose significant side effects, including gastrointestinal ulceration, liver toxicity, and kidney disease, particularly with prolonged usage (Sostres et al., 2010). More specifically, COX-2 selective inhibitors run the risk of harming the heart, while non-selective inhibitors tend to wreak havoc on the gut and kidneys (Emon et al., 2021a). Hence, exploring novel phytochemicals holds promise for the development of more effective alternatives (Liu, 2007).
In addition to in vitro and in vivo studies, in today’s scientific realms, computational biology plays a pivotal role, in facilitating the generation and validation of vast amounts of data used by today’s molecular and experimental biologists. Presently, this paradigm enables the meticulous exploration and verification of drug design endeavors for nascent molecules. The adoption of computer-aided drug discovery (CADD) techniques alongside molecular docking stands as an efficacious and expedient in silico approach (Parmar et al., 2023; Parmar et al., 2020). Prudent molecular docking methodologies must adeptly discern the positioning of the native ligand within the three-dimensional confines of the binding site of the protein structure while also taking into account their intricate physicochemical interactions (Parmar et al., 2022; Guedes et al., 2014).
The present work was carried out for the isolation and identification of bioactive secondary metabolites from the species C. gigantea and C. affinis, along with in vitro, in vivo, and in silico investigation of the bioactivities of the identified compounds. Isolation and identification were carried out by chromatographic separation, followed by 1H-NMR analysis. Then, the identified compounds were employed for the assessment of their antibacterial antidiarrheal and analgesic potentials. Furthermore, the potential of the identified compounds for these activities was also assessed by molecular docking. An ADME/T study was done for their pharmacokinetic properties.
Materials and methods
Plant collection
Whole plants of C. gigantea and C. affinis were collected from Bandarban and Moulovibazar, Bangladesh, in May 2019 (Figure 1). The plants were identified by the experts of Bangladesh National Herbarium, Mirpur, Dhaka, and voucher specimens (DACB; Accession numbers 57,065 and 57,066, respectively) were deposited for these collections.
Extraction of plant material
After a proper wash, whole plants were sun-dried for several days, followed by grinding to a coarse powder using a high-capacity grinding machine. Two clean 5-L round bottom flasks were each filled with 800 g of powdered plant material soaked in 2.4 L of methanol. The containers were kept for 30 days with daily shaking and stirring. The total mixture obtained from the two containers was then filtered through a fresh cotton plug and finally through a Whatman No.1 filter paper. The volume of two filtrates was reduced by using a Buchi Rotavapor at low temperature and reduced pressure. The total weight of the crude extracts of C. gigantea and C. affinis was found to be 60.82 gm and 71.25 gm, respectively.
Drugs and chemicals
Analytical-grade medicines and substances were employed in this investigation. Methanol and Tween-80 were bought from Merck (Darmstadt, Germany). Diclofenac sodium, loperamide, ibuprofen, azithromycin, amoxicillin, ciprofloxacin, and fluconazole were purchased from Square Pharmaceuticals Ltd., Bangladesh.
Test microorganisms
For the antimicrobial assay, Gram-positive bacteria (Sarcina lutea, Bacillus megaterium, Staphylococcus aureus, Bacillus cereus, and Bacillus subtilis), Gram-negative bacteria (Pseudomonas aeruginosa, Salmonella typhi, Salmonella paratyphi, Escherichia coli, Shigella dysenteriae), and fungal strains (Aspergillus niger, Saccharomyces cerevisiae, and Candida albicans) were utilized, provided by the University of Dhaka, Bangladesh.
Experimental animal models
To conduct the in vivo experiment, 4–5 week-old Swiss albino mice of both sexes were acquired from the Animal Resource Branch of the International Centre for Diarrheal Diseases and Research, Bangladesh (ICDDR,B). The mice were kept in standard polypropylene cages with a 12-h light-dark cycle. Other optimal conditions, including controlled room temperature of 24°C ± 2°C and relative humidity of 60%–70%, and feeding with formulated rodent food and water (ad libitum), were also maintained. During the experiments, all the guidelines regarding the use and care of laboratory animals, ethical rules, and regulations were implemented while designing the research and experiments. An intraperitoneal anesthetic overdose of ketamine HCl (100 mg/kg) and xylazine (7.5 mg/kg) was administered to the mouse models at the end of the experiment, followed by euthanasia. All experiments were conducted following the guidelines for the care and use of laboratory animals, which were approved by the institutional ethics committee (Zimmermann, 1983). The Animal Ethics Number for the experimental animal models of this work is 2023-01-04/SUB/A-ERC/002, indicating approval by the Animal Ethics Committee, State University of Bangladesh. This ethical certificate was issued by Prof. Dr. Mohammed Ibrahim, Chairman, Animal Ethics Committee, State University of Bangladesh (Date: 04/01/2023).
General experimental procedures for compound isolation
Solvent–solvent partitioning was done by using the protocol designed by Kupchan and modified by VanWagenen et al. (1993) to avail four fractions: n-hexane, dichloromethane, ethyl acetate, and aqueous fraction. Gel permeation chromatography (GPC/SEC) was performed on Sephadex (LH-20) (Sigma-Aldrich) along with PTLC and TLC conducted on a silica gel 60 F254 on aluminum sheets with a thickness of 0.25 mm (Merck, Germany), which were observed under a UV lamp (UVGL-58, United States) at 254 nm and 365 nm. Visualization of the developed plates was done after spraying the vanillin-sulfuric acid mixture, followed by heating for 5 min at 100°C. Thus, pure compounds were isolated by the PTLC method, and the purities of the compounds were analyzed by the subsequent spot TLC method (Figure 2). The isolated compounds were then subjected to 1H-NMR. The 1H-NMR techniques were performed on a Bruker VNMRS 500 and Bruker Ascend 400 instrument using CDCl3 as a solvent, and the chemical shifts were documented in the δ ppm scale, keeping TMS as a reference (Ashrafi et al., 2022).
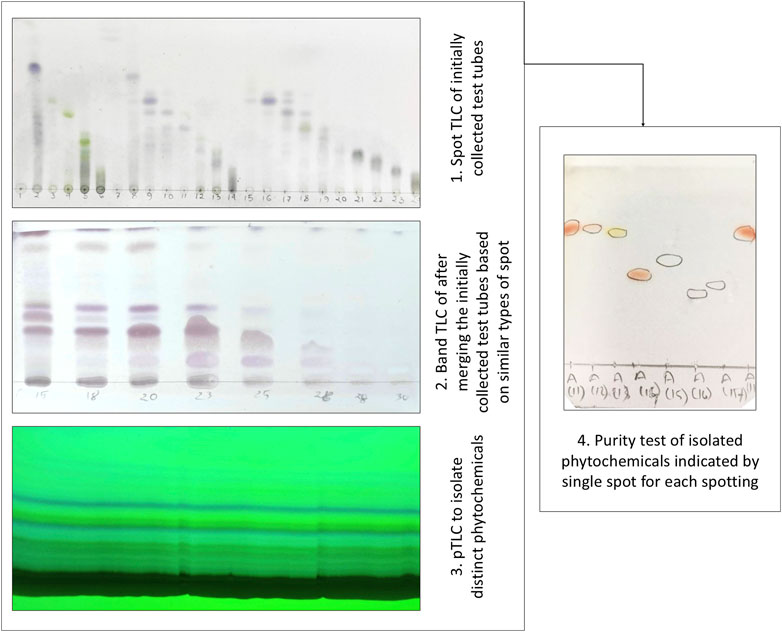
Figure 2. Isolation and purity testing of phytochemicals employing thin layer chromatographic (TLC) techniques.
Experimental design
In vitro tests
Antibacterial assay: disk diffusion test
The disk diffusion technique was used to evaluate the antibacterial properties of various fractionates of the crude extract (Huys et al., 2002). In this conventional approach, test samples were evenly distributed on nutrient agar medium that had been pre-inoculated with test bacteria using sterilized and desiccated filter paper discs (6 mm). Three commercially available antibiotic discs (azithromycin, amoxicillin, and ciprofloxacin) were considered positive standard blank discs, while fluconazole was used against fungal strains. The plates were kept for approximately 24 h upside down at a low temperature (4°C) to enable maximum diffusion of the test samples into the medium. After being flipped over, the plates were kept in an incubator set at 37°C for a whole day. Samples with antibacterial potential were deeply diluted in the medium and exhibited growth inhibition.
In vivo tests
Antidiarrheal bioassay: castor oil-induced diarrhea test
To assess the antidiarrheal potential of the isolated and identified compounds from C. gigantea and C. affinis, they were tested on castor oil-induced diarrheal mice. In this study, the mice were divided into twelve groups: control, positive control, and ten test groups, each containing three mice. The control group was administered with a dose of 10 mL/kg of 1% Tween 80 in a water vehicle orally. The positive control or standard group was administered with a dose of 5 mg/kg of loperamide orally (Rudra et al., 2020). Simultaneously, the two test groups were administered orally with the test compounds C1, C2, C3, and C5, and the mixture of C5 and C6 each at doses of 10 mg/kg and 20 mg/kg, respectively. One hour following the administration of the test samples, 1 mL of highly pure analytical-grade castor oil was fed to each mouse to induce diarrhea. After that, each mouse was individually placed on the box floor lined with transparent paper, and during an observation period of 5 h, the number of diarrheal feces excreted by each animal was recorded.
Throughout the treatment, each mouse was placed in an individual cage, and the floor lining was changed every hour. To evaluate the antidiarrheal activity of the test compounds, the observations of the test groups were compared against those of the control group. During the observational period of 4 h, the number of fecal stool spots was documented for each mouse. The percent inhibition of diarrhea was calculated by the following equation.
Analgesic bioassay: acetic acid-induced writhing test
The peripheral analgesic activity of the isolated and identified compounds from C. gigantea and C. affinis was investigated in the acetic acid-induced writhing test in mice. In this study protocol, mice were divided into twelve groups: control, positive control, and ten test groups, each containing three mice. The mice in the negative control group were administered 0.1 mL of acetic acid intraperitoneally. The positive control group received standard diclofenac sodium at an oral dose of 5 mg/kg b.w. (Ahmad et al., 2010).
The two test groups were administered orally with the test compounds C1, C2, C3, C5, and the mixture of C5 and C6 each at a dose of 10 mg/kg and 20 mg/kg (b.w.; p.o.), respectively. Following the administration of the test samples, the count of writhing movements was recorded 5 min after the injection of acetic acid and documented over a period of 25 min. The percentage of writhing inhibition was subsequently calculated using the following formula:
Anti-inflammatory bioassay: formalin-induced paw-licking test
Four groups of mice, each consisting of five mice, with weights ranging from 20 g to 25 g, were subjected to the formalin-induced licking test following the protocol of Emon et al. (2021b), Alam et al. (2021a), and Sultana et al. (2022). Subcutaneous injections of 20 μL of 1% formalin solution in 0.9% saline were administered to the dorsal side of each mouse’s hind paw. The mice were transferred to a transparent observation space immediately following the injection. Then the authors measured the duration of licking time of the injected paw in rodents. Doses of 10 mg/kg and 20 mg/kg of the test compounds C1–C5 and a combination of C5 and C6 extracted from C. affinis and C. gigantea were administered orally to the groups participating in the experiment. As a standard control, a separate group was administered ibuprofen (10 mg/kg, intraperitoneally, i.p.). Additionally, a control group was administered normal saline (0.1 mL/10 g) as a baseline to compare the analgesic effects of the test extracts.
In silico tests
Molecular docking
Software
In the in silico studies, the docking scores of the identified six compounds from C. affinis and C. gigantea were evaluated against the three selected biologically active target enzyme/receptor macromolecules. Various software programs, including Discovery Studio 4.5, Swiss-PDB viewer, PyRx, and PyMOL 2.3, were employed to assess the molecular interactions comprehensively (Jiko et al., 2024; Shahriar et al., 2024).
Ligand preparation
The structures of compounds 1–4, along with the standard drug molecules, were downloaded from the PubChem database (https://pubchem.ncbi.nlm.nih.gov/), and the structures of compounds 5 and 6 were drawn using ChemDraw Ultra 12.0 software. The structures of the identified compounds and standard drugs are presented in Figure 3. The ligands were downloaded in 3D SDF format and serially loaded into Discovery Studio 4.5. To improve the docking accuracy, the semiempirical technique was used to optimize all the phytoconstituents (Bikadi and Hazai, 2009).
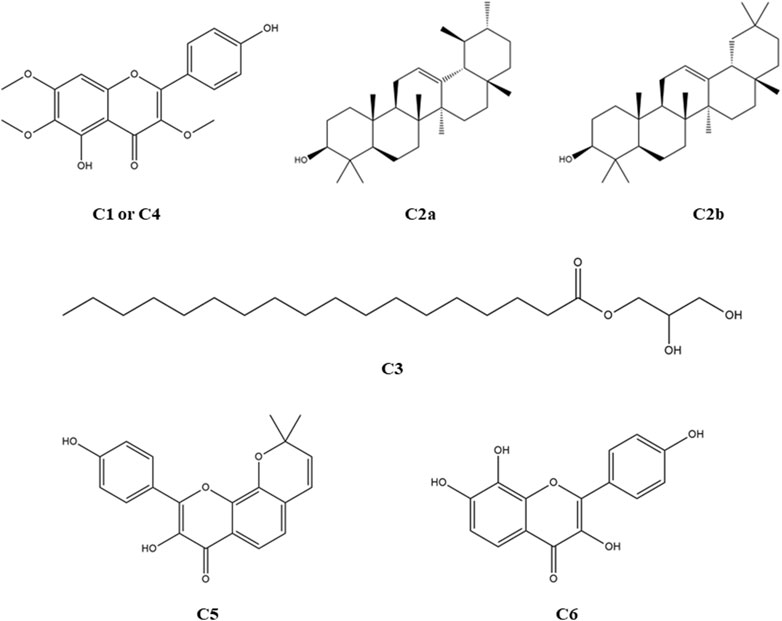
Figure 3. Structures of the identified phytocompounds from C. affinis, C. gigantea, and the standard drug molecules considered for the respective bioactivities.
Target protein preparation
The target macromolecules were obtained as 3D crystal structures from the RCBS Protein Data Bank (https://www.rcsb.org/structure) in the PDB format. For antibacterial, antidiarrheal, and peripheral analgesic activity assessment, dihydrofolate reductase (DHFR) enzyme [PDB ID: 4M6J] (Khatun et al., 2021), kappa opioid receptor (KOR) [PDB ID: 6VI4] (Alam et al., 2021b), and cyclooxygenase-2 (COX-2) enzyme [PDB ID: 1CX2] (Muhammad et al., 2015) were employed. After that, all water molecules and heteroatoms were removed from the proteins using Discovery Studio 2021, and Swiss-PDB Viewer’s energy minimization tool was used to optimize biomolecules by arranging nonpolar hydrogen atoms.
Ligand–protein interaction
To gain insight into how molecules interact at the molecular level, the potential binding patterns and binding affinities were predicted through a computer-aided ligand–protein interaction diagram. The software PyRx was employed for the molecular drug–protein binding procedure. A careful selection of particular amino acids, along with their corresponding IDs, was undertaken from the scientific literature for each enzyme/receptor individually, aiming for precise target docking (Khatun et al., 2021; Alam et al., 2021b; Muhammad et al., 2015). Subsequently, the protein was prepared by loading and formatting as the necessary macromolecule, guaranteeing specific ligand binding to the intended targets.
To enhance the docking process with the chosen macromolecules, ligand SD files were brought in and transformed into pdbqt format using the Open Babel tool within the PyRx software. Grid mapping identified the active amino sites within specific grid boxes, following the predetermined center and dimensional axes outlined in Table 1. During this stage, basic supportive default functions remained unchanged (Muhammad et al., 2015). The last step entailed interpreting the results and employing BIOVIA Discovery Studio version 4.5 to forecast the optimal 2D and 3D models.
ADME/T study
For the evaluation of pharmacokinetic parameters like absorption, distribution, metabolism, excretion, and toxicity (ADME/T), pkCSM (http://structure.bioc.cam.ac.uk/Pkcsm) was employed (Pires et al., 2015). Concurrently, the Swiss ADME (http://www.swissadme.ch), which predicts drug-likeness based on Lipinski’s rules and pharmacokinetic parameters (Daina et al., 2017; Shompa et al., 2024), was used to determine the drug-likeness and bioavailability score of selected compounds.
Statistical analysis
The statistical analysis was done through the presentation of mean values accompanied by the standard error of the mean (SEM). These values were meticulously compared to those of the control group and discerned to be statistically significant (***p < 0.001, **p < 0.01, and *p < 0.05), following a rigorous one-way analysis of variance (ANOVA) supplemented by Dunnett’s test. All statistical manipulations were conducted utilizing GraphPad Prism Version 5.2 (San Diego, CA).
Results
Identification of compounds
A total of six compound structures, shown in Figure 3, were isolated and identified from the Colocasia gigantea and Colocasia affinis.
Penduletin (C1 or C4): Pale yellow crystals, 1H NMR (500 MHz, CDCl3): δH 6.59 (1H, s, H-8), 7.90 (1H, d, J = 9.0 Hz, H-2′), 7.04 (1H, d, J = 9.0 Hz, H-3′), 7.04 (1H, d, J = 9.0 Hz, H-5′), 7.90 (1H, d, J = 9.0 Hz, H-6′), 3.90 (3H, s, 3-OCH3), 4.02 (3H, s, 6-OCH3), 4.04 (3H, s, 7-OCH3), 12.79 (1H, s, 5-OH), 6.37 (1H, s, 4′-OH). The corresponding 1H-NMR spectrum is depicted in Figure 4.
A mixture of α-amyrin and β-amyrin (C2) α-Amyrin (C2a): White powder, 1H NMR (400 MHz, CDCl3): δH 5.28 (1H, t, H-12), 3.24 (1H, dd, J = 4.4, 4.8 Hz, H-3), 1.16 (3H, s, H-27), 1.11 (6H, s, H-26), 1.01 (6H,s, H-28), 0.98 (6H, s, H-25), 0.93 (3H, d, J = 3.2 Hz, H-30), 0.82 (3H, d, J = 3.2 Hz, H-29), 0.80 (s, 6H, H-23), 0.79 (s, 6H, H-24). The corresponding 1H-NMR spectrum is depicted in Figure 5.
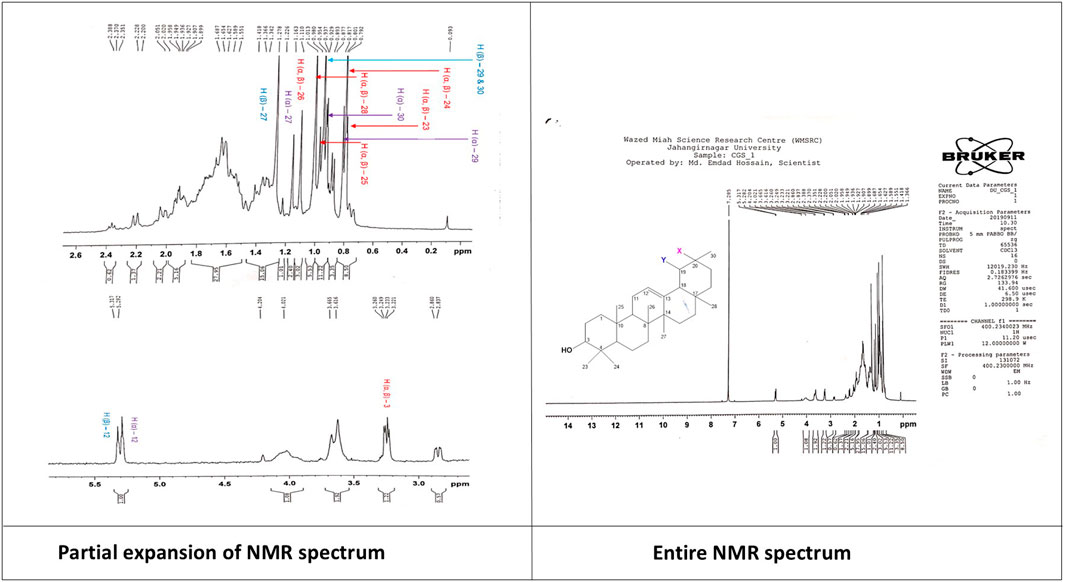
Figure 5. 1H-NMR spectrum of the compound mixture of α-amyrin and β-amyrin isolated from C. gigantea.
β-Amyrin (C2b): White powder, 1H NMR (400 MHz, CDCl3): δH 5.31 (t, 1H, H-12), 3.24 (dd, 2H, J = 4.4, 4.8 Hz, H-3), 1.28 (s, 3H, H-27), 1.11 (s, 6H, H-26), 1.01 (s, 6H, H-28), 0.98 (s, 6H, H-25), 0.95 (s, 6H, H-29,30) 0.80 (s, 6H, H-23), 0.79 (s, 6H, H-24). The corresponding 1H-NMR spectrum is depicted in Figure 5.
Monoglyceride of stearic acid (C3): Colorless crystals, 1H NMR (400 MHz, CDCl3); Glycerol backbone: -CH2-O-CO: 4.195 (2H, dd, J = 13.6, 5.6 Hz), -CH: 3.957 (1H, m), -CH2: 3.732 (2H, dd, J = 11.6, 4.0 Hz), 3.617 (1H, dd, J = 11.6, 5.6 Hz). Stearic acid part: -CH2-CO-O: 2.37 (t, 2H), -CH2: 1.64 (d, 2H), 4-CH2: 1.31 (d, 8H), 10-CH2: 1.25 (s, 20H), -CH3: 0.90 (t, 3H). The corresponding 1H-NMR spectrum is depicted in Figure 6.
7,8 -(3′′,3′′-dimethyl-pyrano)-4′-hydroxy flavonol (C5): White powder, 1H NMR (500 MHz, CDCl3): δH 7.46 (1H, d, J = 8.5 Hz, H-5), 6.86 (1H, d, J = 8.5 Hz, H-6), 7.68 (1H, d, J = 8.5 Hz, H-2′), 7.68 (1H, d, J = 8.5 Hz, H-6′), 6.96 (2H, d, J = 12.5 Hz, H-1″), 6.84 (1H, d, J = 9.0 Hz, H-3′), 6.84 (1H, d, J = 9.0 Hz, H-5′), 5.87 (1H, d, J = 12.5 Hz, H-2″), 1.55 (6H, s, H-4″, H-5″). The corresponding 1H-NMR spectrum is depicted in Figure 7.
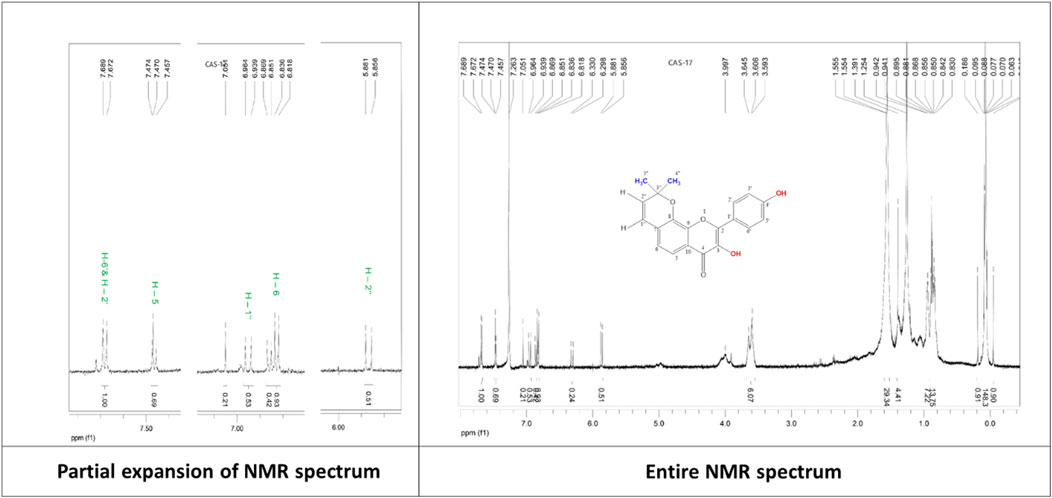
Figure 7. 1H-NMR spectrum of the compound 7,8-(3″,3″-dimethyl-pyrano)-4′-hydroxy flavonol isolated from C. affinis.
Mixture of 7,8-(3′′,3′′-dimethyl-pyrano)-4′-hydroxy flavonol (C5) and 4′,7,8-trihydroxy flavonol (C6): White powder, 1H NMR (500 MHz, CDCl3): δH 6.81 (1H, s, H-3), 7.46 (1H, d, J =9.0 Hz, H-5), 7.30 (1H, d, J = 9.0 Hz, H-6), 8.00 (1H, d, J = 8.5 Hz, H-2′), 6.88 (1H, d, J = 8.5 Hz, H-3′), 6.88 (1H, d, J = 8.5 Hz, H-5′), 8.00 (1H, d, J = 8.5 Hz, H-6′). The corresponding 1H-NMR spectrum is depicted in Figure 8.
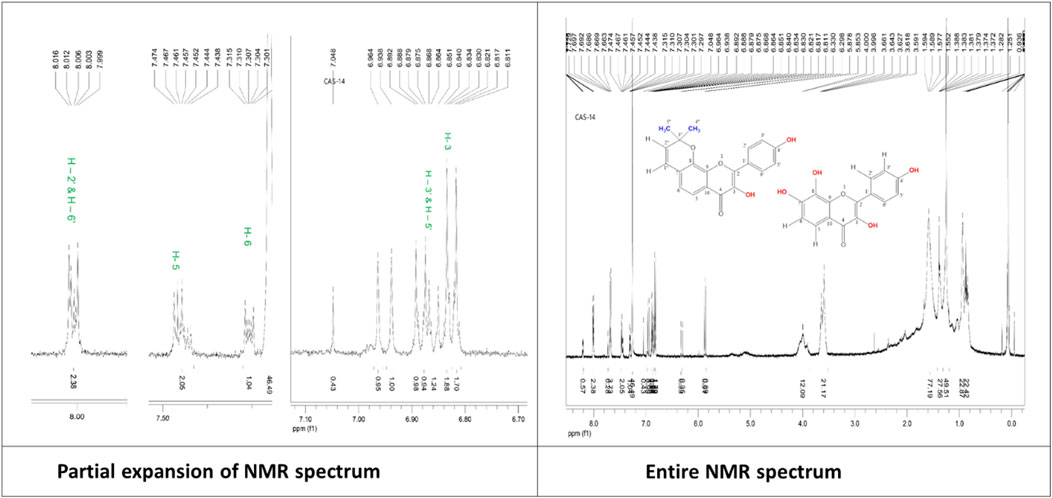
Figure 8. 1H-NMR spectrum of the compound mixture of 7,8-(3″,3″-dimethyl-pyrano)-4′-hydroxy flavonol and 4',7,8-trihydroxy flavonol isolated from C. affinis.
In vitro testing
Effect of the identified test compounds on the disc diffusion assay
The antibacterial activity of all the partitions was tested against five strains of gram-positive and gram-negative bacteria and three strains of fungi. As a reference standard, azithromycin, amoxicillin, ciprofloxacin, and fluconazole were taken to test the respective antimicrobial activity. The zone of inhibition (ZOI) of the test samples ranged from 6 mm to 20 mm and is summarized in Table 2. C1/C4, C2, C3, and C5 showed considerable antibacterial activity, whereas C1/C4 and C3 exhibited promising antifungal attributes. As per ZOI, the fractionated extracts exerted notable antimicrobial activities against B. cereus, B. megaterium, B. subtilis, S. aureus, S. lutea, E. coli, P. aeruginosa, and S. dysenteriae and relatively lower ZOI against S. paratyphi, S. typhi, A. niger, C. albicans, and S. cerevisiae. In addition, all the standard drugs exhibited the expected pronounced ZOIs against all the tested strains, ranging from 27 mm to 45 mm.
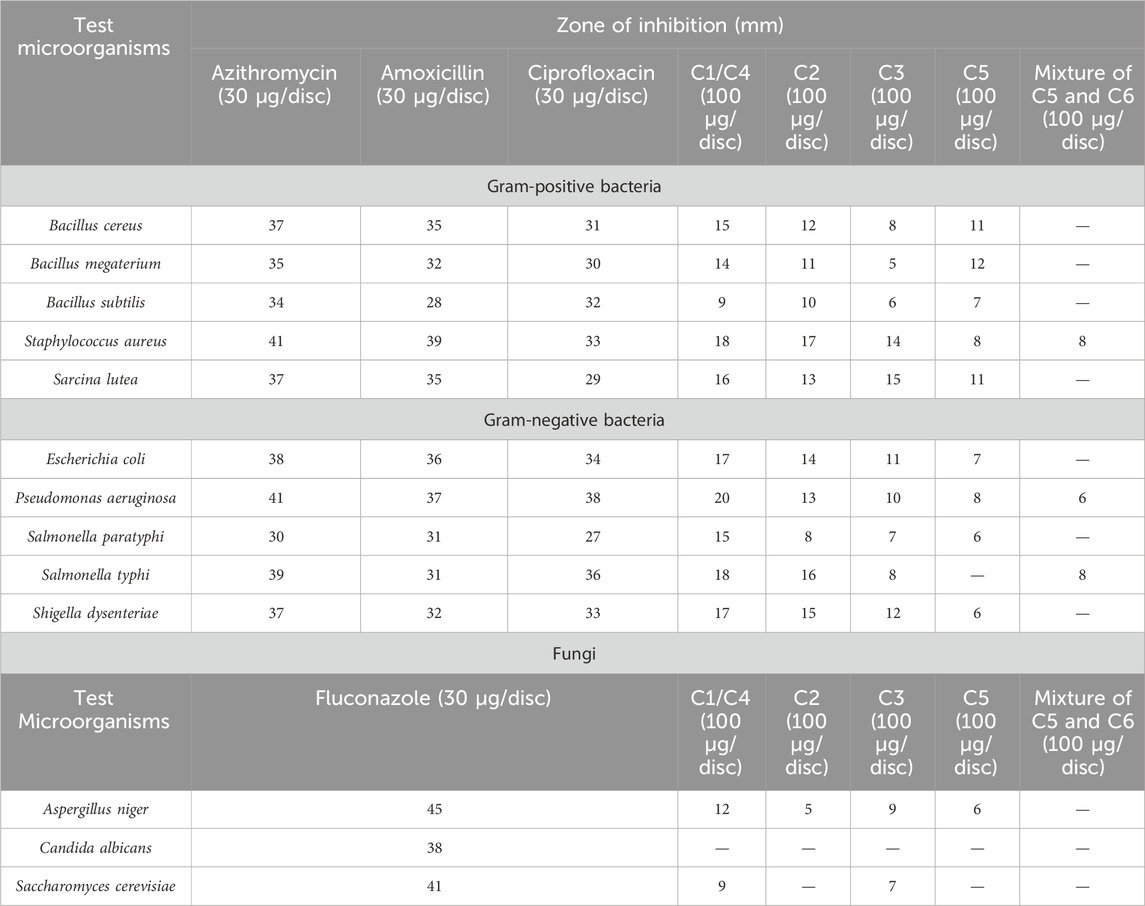
Table 2. Antibacterial activity of the isolated compounds from C. gigantea and C. affinis against Gram-positive and Gram-negative bacteria.
In vivo study
Effect of the identified test compounds on castor oil-induced diarrhea
Compounds C2, C5, and the mixture of C5 and C6, each at doses of 10 mg/kg and 20 mg/kg, and compound C1 at a dose of 20 mg/kg exhibited significant (p < 0.05, p < 0.01) reduction in the number of feces (Table 3). In terms of wet feces number, C2, C5, and the mixture of C5 and C6 demonstrated percentages of diarrhea inhibition of 29.63%, 37.04%, and 25.93%, respectively, at the 10 mg/kg dose, while at the 20 mg/kg dose, C1, C2, C5, and the mixture of C5 and C6 exhibited reductions of 33.33%, 40.74%, 44.44%, and 37.04%, respectively. The value of the standard loperamide was 77.78%.
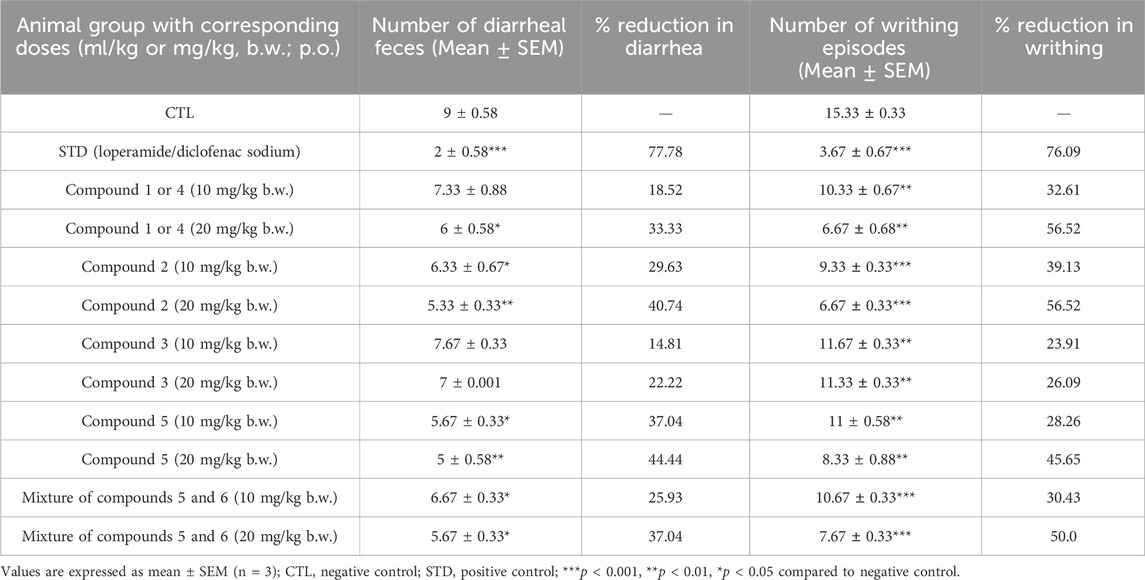
Table 3. Antidiarrheal and analgesic activities of isolated compounds from C. gigantea and C. affinis, respectively, on castor oil-induced diarrhea and acetic acid-induced writhing test in mice.
Effect of the identified test compounds on acetic acid-induced writhing in the mice model
All the test compounds C1, C2, C3, C5, and the mixture of C5 and C6 each at 10 and 20 mg/kg doses exhibited significant (p < 0.01, p < 0.001) analgesia with a considerable percentage reduction of acetic acid-induced writhing compared to the standard diclofenac sodium (Table 3). Among them, C1 and C2 exhibited the highest percentage reduction of writhing with 56.52% at the 20 mg/kg dose when compared to the standard of 76.09%.
Effect of the identified test compounds on formalin-induced licking in the mice model
The administration of test compounds C1/C4, C2, C3, and C5, and the combination of C5 and C6 at doses of 10 mg/kg and 20 mg/kg resulted in significant analgesic effects, as evidenced by a marked reduction in formalin-induced paw licking behavior. The observed anti-inflammatory effect was statistically significant (p < 0.01, p < 0.001) (Table 4), demonstrating a marked reduction in formalin-induced writhing relative to the control group receiving ibuprofen. C5 and C2 demonstrated the highest anti-inflammatory efficacy among the compounds evaluated, resulting in a 64.50% and 68.15% reduction in licking during the early phase and a 66.66% and 52.06% reduction during the later phase, respectively, at a dosage of 20 mg/kg. On the other hand, the standard drug ibuprofen led to a decrease of 73.54% and 74.68% in early and late phase subsequently. The findings indicate that compounds C5 and C2 demonstrate substantial potential for development as anti-inflammatory agents, primarily due to their marked reduction in pain responses in the examined mice.
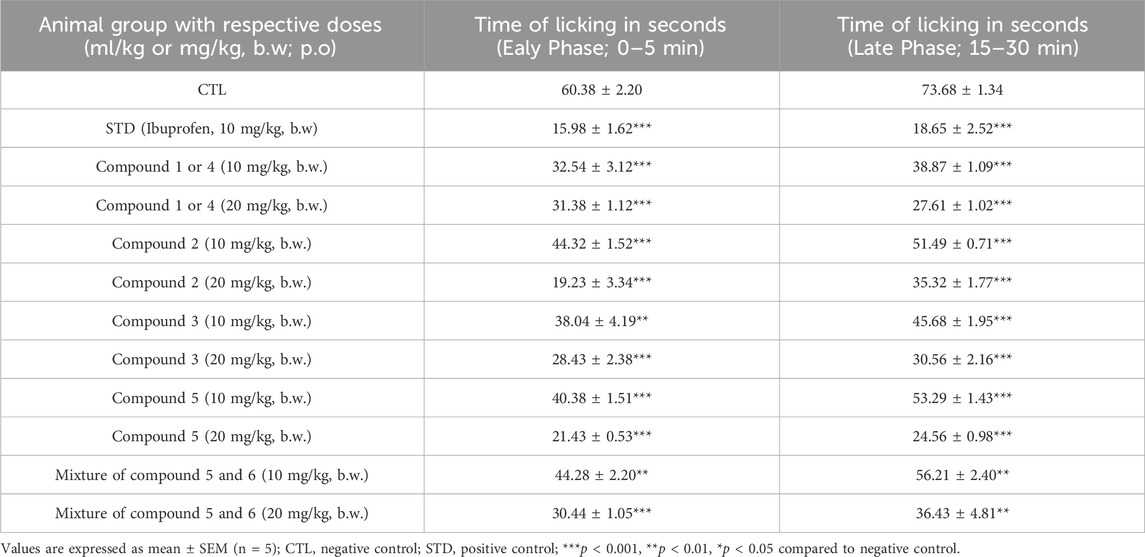
Table 4. Anti-inflammatory effect of the isolated compounds from C. gigantea and C. affinis on the formalin-induced mouse model.
In silico study
Molecular docking
The docking scores of the identified compounds from the methanolic extracts of C. affinis and C. gigantea to selected targets are depicted in Table 5. For the enzyme DHFR, C5 showed the highest docking score of −9.1 kcal/mol, followed by C2a, C2b, and C1/C4 with promising docking scores of −8.8 kcal/mol, −8.8 kcal/mol, and −7.9 kcal/mol, respectively, when compared to the standard drug ciprofloxacin with a docking score of −8.1 kcal/mol. The 3D and 2D graphical representations of the molecular interactions of these compounds and the standard drug ciprofloxacin with the DHFR enzyme are depicted in Figures 9, 12, respectively. In the case of the KOR receptor, the standard drug loperamide showed a docking score of −9.1 kcal/mol, while the test compound C2b showed the highest docking score of −10.5 kcal/mol. The other compounds also showed significant scores of −9.7 kcal/mol (C5), −9.4 kcal/mol (C2a), −8 kcal/mol (C6), and −7.7 kcal/mol (C1/C4). The 3D and 2D graphical representations of the molecular interactions of these compounds and the standard loperamide with the KOR receptor are depicted in Figures 10, 12. Additionally, with the COX-2 enzyme, the compound C2b showed a promising docking score of −8.7 kcal/mol, along with other notable docking scores: −7.3 kcal/mol (C6), −7.2 kcal/mol (C5), and −7 kcal/mol (C1 and C3). The docking score of the standard drug diclofenac sodium to the COX-2 enzyme was −7.8 kcal/mol. The 3D and 2D graphical representations of the molecular interactions of these compounds and the standard diclofenac sodium with COX-2 enzyme are depicted in Figures 11, 12. The corresponding binding interactions and the binding sites of highly active compounds against the targets, including DHFR, KOR, and COX-2, are presented in Table 6.
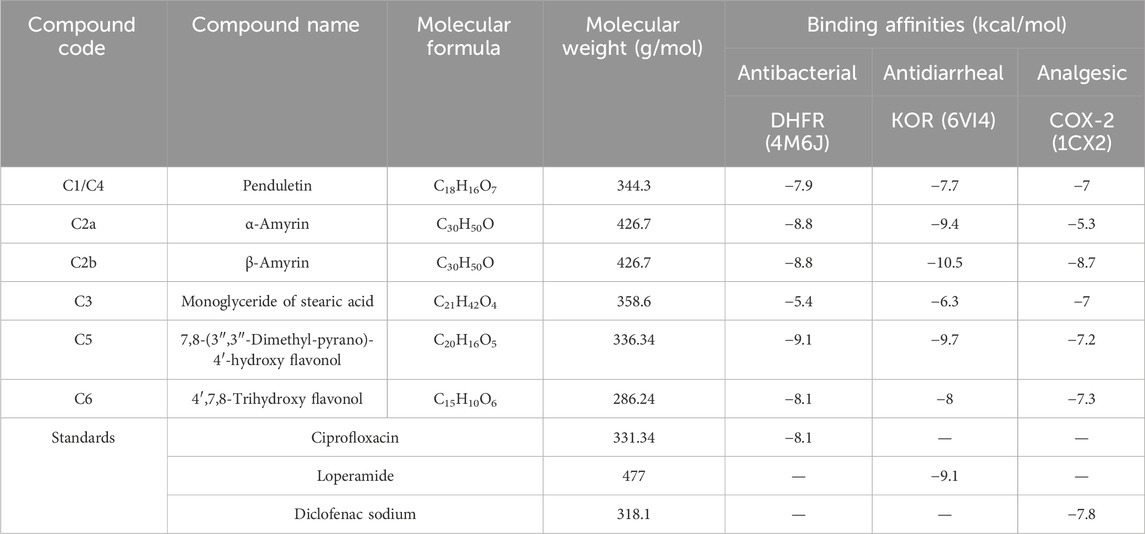
Table 5. Binding affinities of the identified compounds from C. gigantea and C. affinis and standards against three macromolecules, namely, DHFR, KOR, and COX-2, representing antibacterial, antidiarrheal, and peripheral analgesic activities, respectively.
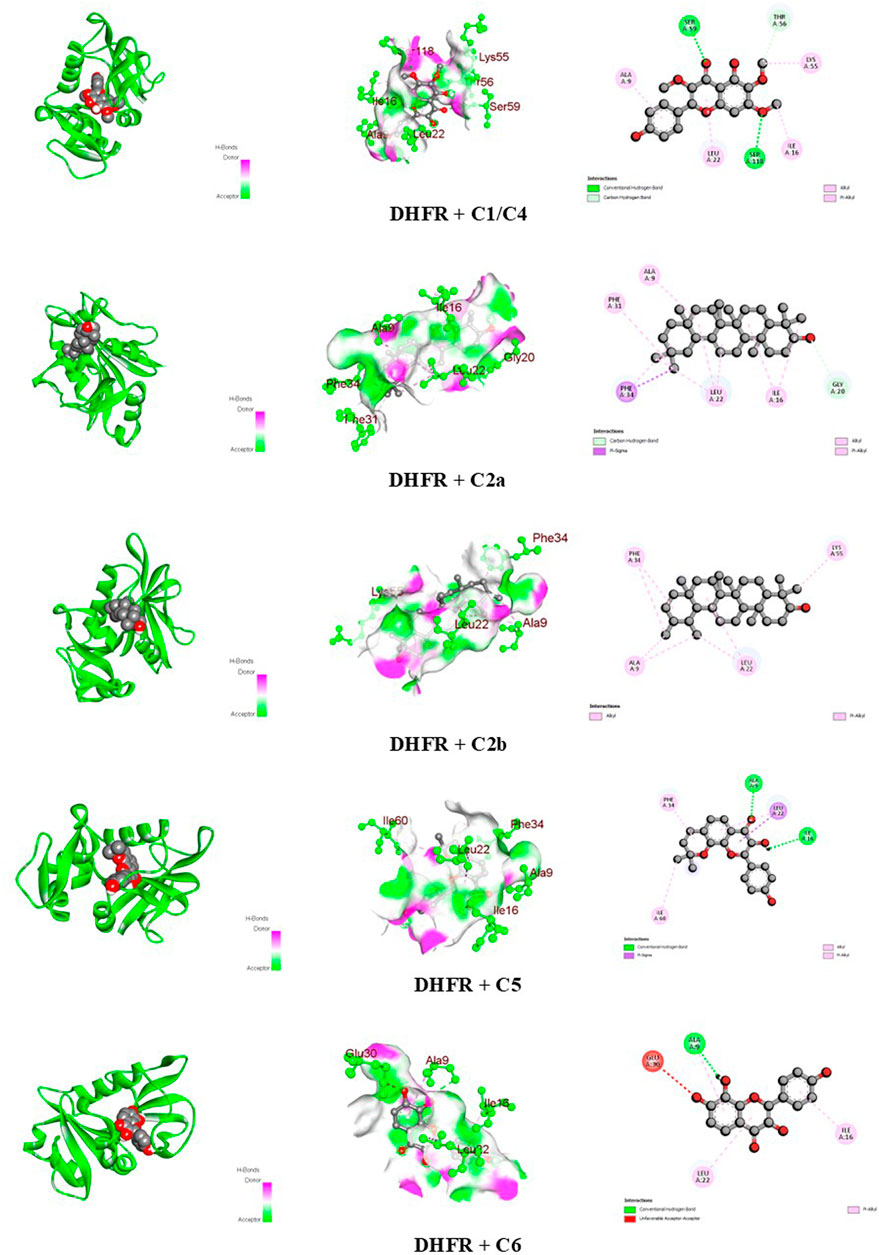
Figure 9. Graphical representation of the molecular interactions of the isolated phytocompounds with the DHFR enzyme with 3D and 2D visualization.
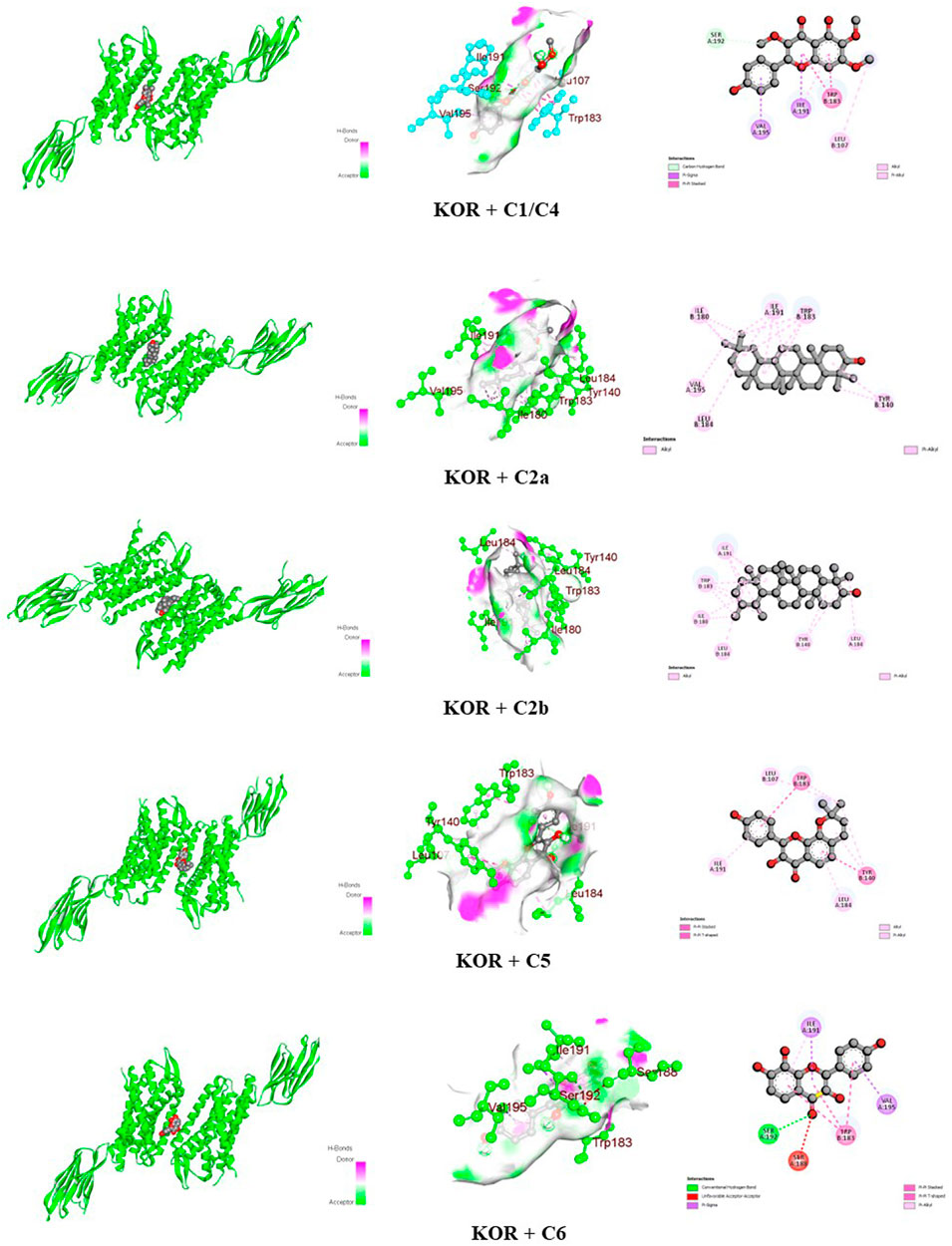
Figure 10. Graphical 3D and 2D visualization of the molecular interactions of the isolated phytocompounds with the KOR receptor.
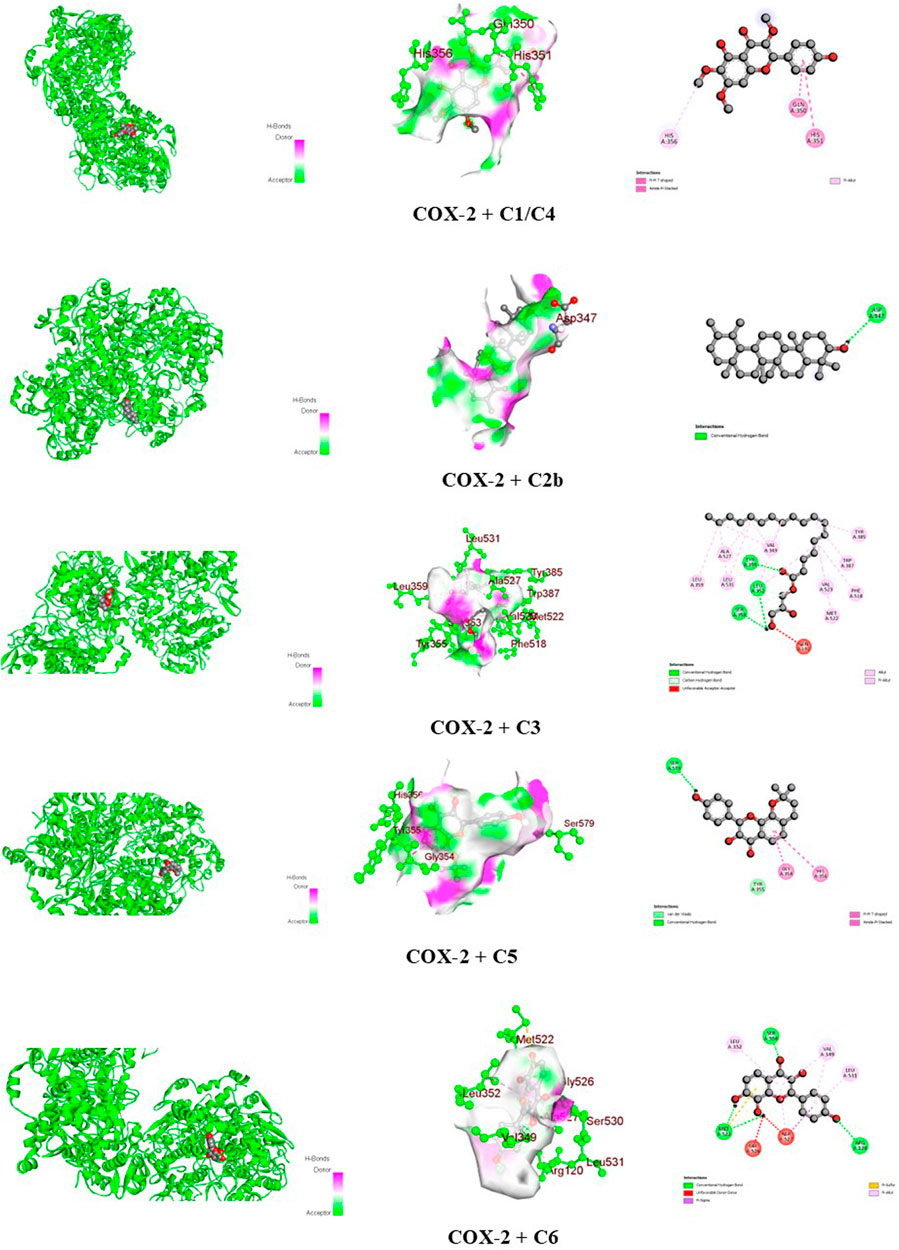
Figure 11. Graphical 3D and 2D visualization of the molecular interactions of the isolated phytocompounds with the COX-2 enzyme.
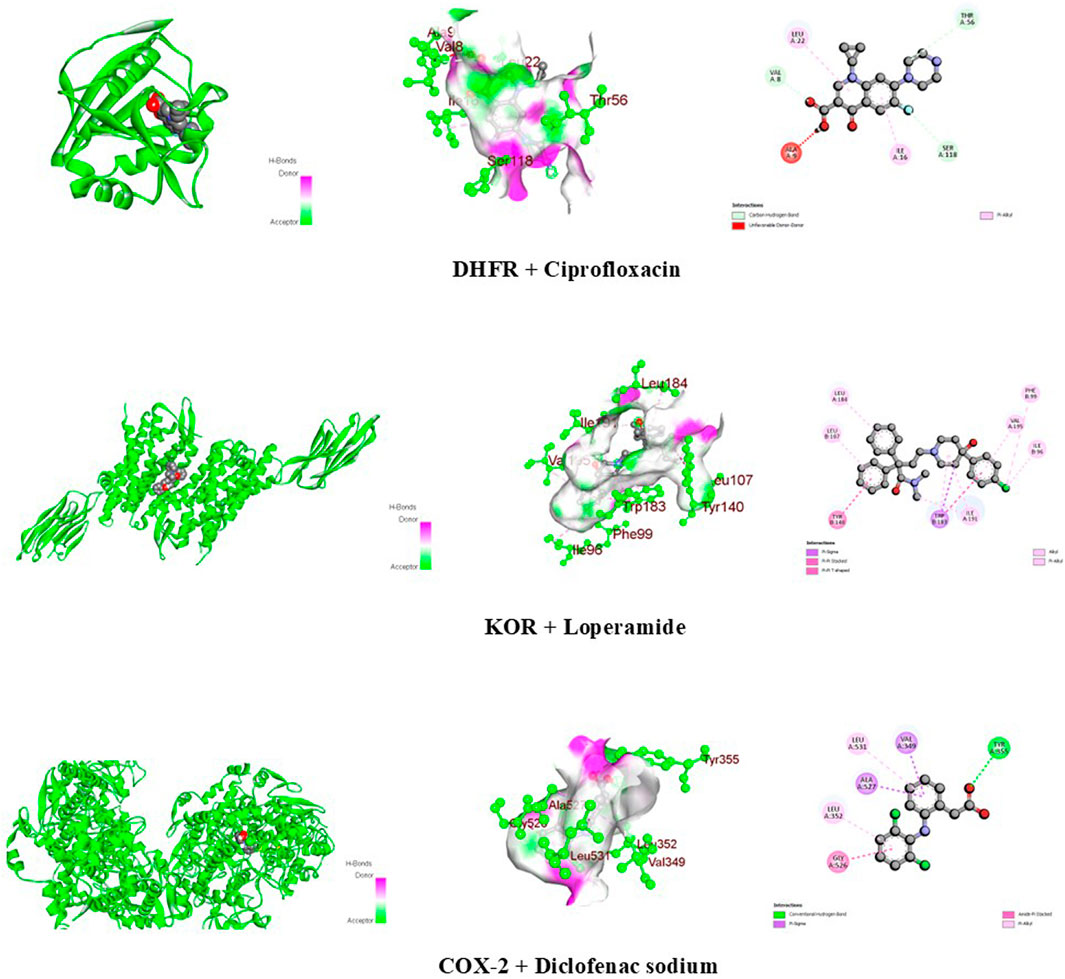
Figure 12. Graphical 3D and 2D visualization of the molecular interactions of the standard drug molecules with their respective enzymes.
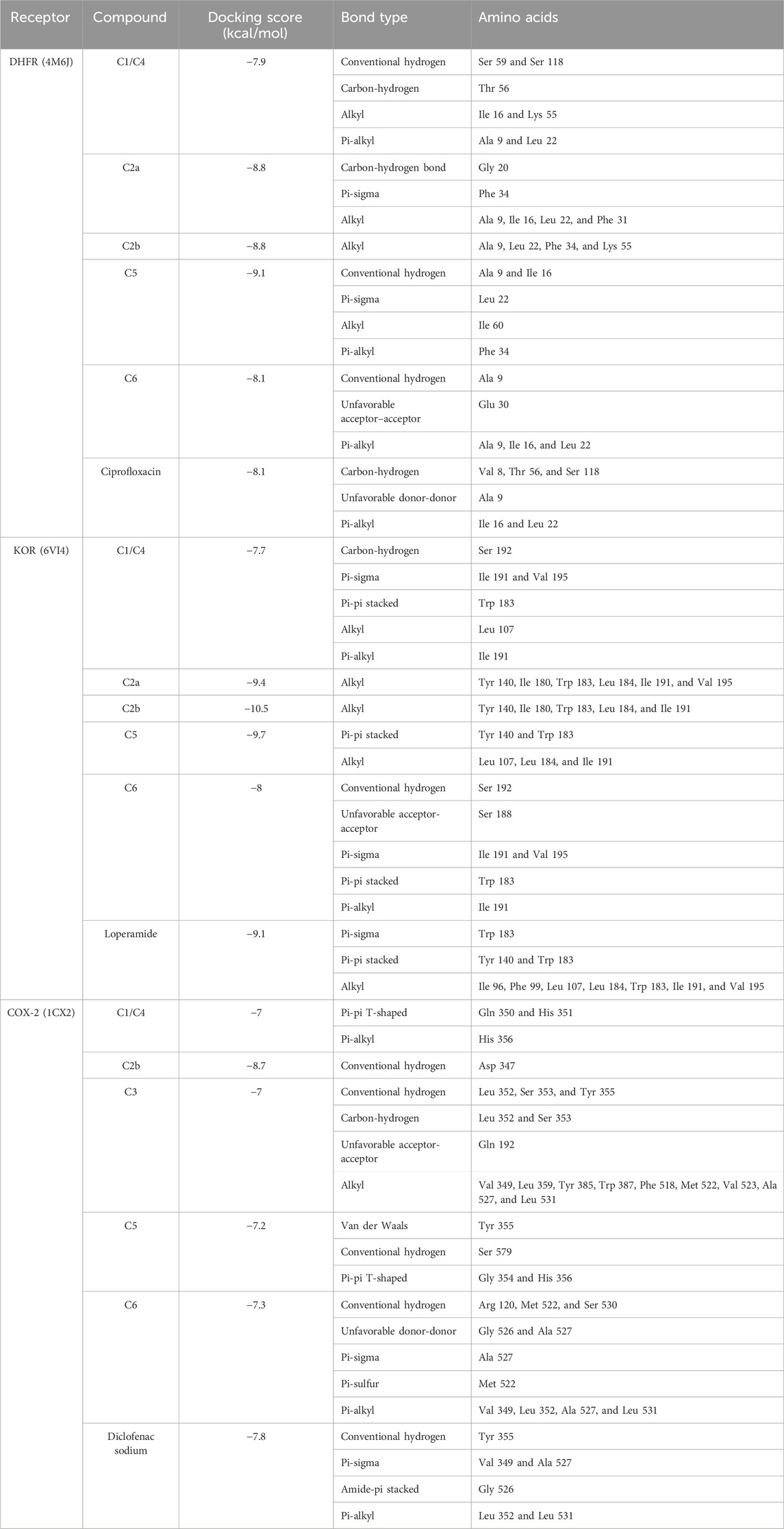
Table 6. Bonds and binding sites of the identified best-binding compounds from C. gigantea and C. affinis against different targets, including DHFR, KOR, and COX-2.
ADME/T study
The in silico study also analyzed the ADME/T parameters of the identified compounds. The bioavailability score and Lipinski’s rule of five data were also considered for the assessment of drug-likeness of the compounds, which are demonstrated in Table 7.
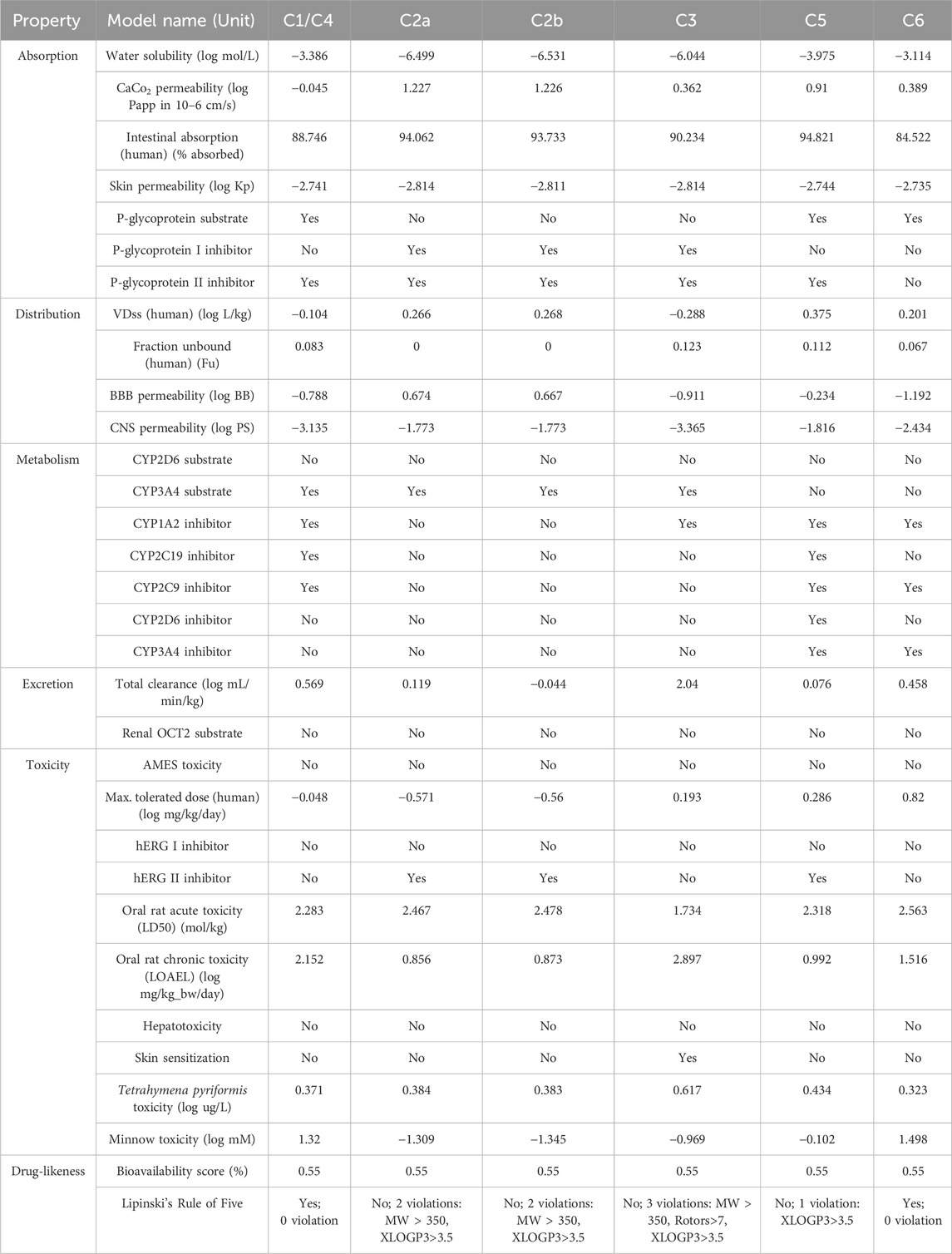
Table 7. ADME/T study of the identified best-binding compounds from C. gigantea and C. affinis against DHFR, KOR, and COX-2 macromolecules.
Discussion
This study revealed the first-time report on compound isolation ascertained by structure elucidation employing NMR technology from two Colocasia species, namely, C. gigantea and C. affinis. Previous reports on these plants only focused on phytochemical profiling through HPLC-DAD and GC-MS (Zilani et al., 2021; Alam et al., 2024). Thus, phytochemical isolation and the elucidation of their structures are the novelty of this current research. These revealed three bioactive flavonoids along with two triterpenoids and one monoglyceride in total, which sheds light on the polyphenol-rich candidacy of these plants, which may result in promising lead compounds for future drug discovery and development. Dichloromethane fractions of both plants were used in phytochemical screening and isolation of compounds. A total of four compounds were elucidated from the dichloromethane soluble fraction of C. gigantea as a result of consecutive chromatographic separation and purification. The compounds isolated from C. gigantea are penduletin (1), a mixture of α-amyrin (2a) and β-amyrin (2b), and a monoglyceride of stearic acid (3). Another three compounds were isolated from the dichloromethane-soluble fraction of C. affinis following the same technique: penduletin (4) (which was also isolated from C. gigantea), 7,8 -(3″,3″-dimethyl-pyrano)-4′-hydroxy flavonol (5), and a mixture of 7,8 -(3″,3″-dimethyl-pyrano)-4′-hydroxy flavonol (5) and 4′,7,8-trihydroxy flavonol (6).
The 1HNMR spectrum (500 MHz, CDCl3) of compounds 1 and 4 (Figure 4) in CDCl3 indicated the presence of three methoxy groups at δH 4.04 (3H, s), δH 4.02 (3H, s), and δH 3.90 (3H, s). The two pairs of ortho-coupled (J = 9.0 Hz) doublets at δH 7.04 and δH 7.90 showed that ring B is monosubstituted at C-4’. The peak at δH 12.79 belongs to 5-OH. There is a one-proton singlet at δH 6.59. There are three possible positions for this singlet: C-3, C-6, and C-8. Another proton singlet at δH 6.37 could be assigned to the -OH group at C-4’. These 1H NMR data are in close agreement with the published value of penduletin (Wang et al., 1989; Makhmoor and Choudhary, 2010), where the singlet at δH 6.59 is at the C-8 position, and the three methoxy groups are at the C-3, C-6, and C-7 positions. Thus, the structure of compounds 1 and 4 can be concluded as penduletin.
The 1H NMR spectrum (400 MHz, CDCl3) of compound 2 (Figure 5) showed two triplets at δH 5.32 ppm and δH 5.28 ppm characteristic of the olefinic proton (H-12) of α- and β-amyrin, respectively. In addition, eight singlets and two doublets of nCH3 protons were identified in the range δH 1.28-0.79 ppm. The singlet at δH 0.89 (3H) and δH 0.88 ppm (3H) indicated the presence of CH3-28. This means that the analyzed component was not an acid with the carboxyl group at C-17. The peaks of the methyl groups of α-amyrin were identified at δH 1.16 ppm (singlet; CH3-27), δH 0.93 ppm (doublet-doublet; J = 3.2 Hz; CH3-30), and δH 0.82 ppm (singlet; CH3-29). The protons of the CH3-27, CH3-29, and CH3-30 groups of β-amyrin had peaks at δH 1.28 ppm (singlet; 3H; CH3-27) and δH 0.95 ppm (singlet; 6H; CH3-29 and 30). The other signals were identical for both amyrins: δH 1.11 (6H, s; CH3-26), δH 1.01 (6H, s; CH3-28), δH 0.98 (6H, s; CH3-25), δH 0.82 (6H, s; CH3-23), and δH 0.80 (6H, s; CH3-24). The above data identified compound 2 as a mixture of α-amyrin (2a) and β-amyrin (2b) with a ratio of 4:3 based on the 1H NMR peak heights. This identity was further confirmed by a direct comparison of its 1H NMR spectrum with that recorded for a mixture of α-amyrin and β-amyrin in CDCl3 (400 MHz, CDCl3) (Migas et al., 2005).
The structure of compound 3 can be proposed as a monoglyceride of saturated fatty acid. The 1H NMR spectrum (400 MHz, CDCl3) showed signals at δH 4.195 (2H, dd, J = 13.6, 5.6 Hz), δH 3.957 (1H, m), and δH 3.732 (2H, dd, J = 11.6, 4.0 Hz). This group of signals can be attributed to the protons of the (-CH2-CH-CH2-) backbone (glycerol structure). The upfield peak at δH 0.903 with three proton intensities corresponds to one –CH3 proton. Two additional distinct groups of upfield signals at δH 2.374 (2H, t, J = 7.6 Hz), δH 1.64 (2H, m), δH 1.313 (8H, m), and δH 1.25 (20H, m) could correspond to 16 (sixteen) methylene groups. The presence of an additional oxymethine proton in the carbon chain can be justified by the signal at δH 3.617 (1H, dd, J = 11.6, 5.6 Hz). Comparing all the spectral data on the structure of compound 3 with the previously documented study (Kavadia et al., 2017) supports identifying this compound as a monoglyceride of stearic acid with the structure drawn in Figure 6.
The 1H NMR spectrum (CDCl3, 500 MHz) of compound 5 (Figure 7) showed two aromatic systems, including one 1,4-disubstituted benzene ring with the doublet at δH 7.68 (2H, d, J = 8.5 Hz), one tetra-substituted benzene ring with the 1H signals at δH 6.86 (1H, d, J = 8.5 Hz), δH 7.46 (1H, d, J = 8.5 Hz), and a 3″,3″-dimethyl-pyrano group. The presence of 3″, 3″-dimethyl-pyrano group can be proved by two tertiary methyl signals at δH 1.55 (6H, s) and two olefinic proton signals at δH 5.87 (1H, d, J = 12.5 Hz) and δH 6.96 (1H, d, J = 12.5 Hz). All these chemical shift values are in close agreement with the published values of citrusinol except for the presence of an extra aromatic proton signal that could be assigned to H-5. Another change in the chemical shift of the H-6 proton at δH 6.84 differs largely in values, which is at δH 6.21 for citrusinol (Qing-Hui et al., 2017). This high value of the chemical shift can be justified only if the position of the oxygen atom of the pyran ring is at C-8 rather than C-7. Thus, the structure of compound 5 can be proposed as 7, 8-(3″,3″-dimethyl-pyrano)-4′-hydroxy flavonol.
The 1H NMR spectrum (CDCl3, 500 MHz) of compound 6 shows signals that exactly match those of compound 5 (7,8-(3″,3″-dimethyl-pyrano)-4′-hydroxy flavonol). But the presence of additional peaks suggest the presence of another compound as a mixture. The additional signals include two para-coupled doublets with J = 8.5 Hz at δH 8.00 and δH 6.88, each integrating for two protons, which were assigned to the coupled H-2′ and H-6′, and H-3′ and H-5′, respectively. A typical ortho-coupled signal for protons is found at C-5 and C-6 at δH 7.46 (1H,d, J = 9.0 Hz) and δH 7.30 (1H,d, J = 9.0 Hz). All these additional proton signal values are in close agreement with published values (Ponce et al., 2009) of the structure (Figure 8) and suggested as 4′,7,8-trihydroxy flavonol. Thus, the structure of compound 6 appeared as a mixture of 4′,7,8-trihydroxy flavonol with 7,8-(3″,3″-dimethyl-pyrano)-4′-hydroxy flavonol (compound 5) in a ratio of 1:2, according to the peak heights in the 1H NMR spectrum.
Diarrhea, characterized by disrupted intestinal movements and fluid accumulation coupled with increased peristalsis, occurs due to disruptions in the electrolyte permeability of the intestinal membrane (Bristy et al., 2020). Infectious diarrhea primarily arises from the invasion and spread of pathogens, particularly Salmonella and Shigella (Panda et al., 2012), against which traditional remedies have proven effective (Koné et al., 2004). The study of phytochemicals extracted from medicinal plants showcased promising antidiarrheal effects attributed to their various phytoconstituents, notably flavonoids, which are known for their antidiarrheal properties (Otshudi et al., 2000). Flavonoids are thought to achieve this by lessening motility in both the small and large intestine and suppressing bowel contractions (Capasso et al., 1991; Meli et al., 1990). All of the identified phytochemicals exhibited more pronounced antidiarrheal activity at higher doses (20 mg/kg b.w.) than at lower doses (10 mg/kg b.w.) by reducing the average weight of total and wet feces, as well as decreasing the total number of feces at a dose of 20 mg/kg, akin to the efficacy of loperamide, the standard drug used to treat diarrhea in this investigation, despite not displaying quite a dose-dependent response. However, unlike flavonoids and amyrin, the ester did not exert noteworthy antidiarrheal activity in mouse models.
The human body orchestrates a myriad of chemical reactions through various catabolic and anabolic processes, yielding a plethora of substances. These reactions, which can induce pain, inflammation, and oxidative stress, are often triggered by inflammatory mediators and reactive oxygen and nitrogen species (RONS) (Wilhelm et al., 2016). Inflammatory cascades, spurred by triggers such as microbial invasion or tissue distress, prompt the mobilization of defense cells like leukocytes to the affected site, a phenomenon orchestrated by receptors such as toll-like receptors (TLRs) and NOD-like receptors (NLRs) (Abdel Motaal and Abdel Maguid, 2005). Furthermore, an array of inflammatory mediators such as eicosanoids, cytokines, chemokines, and vasoactive amines, displays the inflammatory milieu (Sun et al., 2014). Flavonoids have demonstrated the capacity to curtail the abundance of inflammatory cells and the synthesis of MMP-9 (matrix metalloproteinase) and other inflammatory mediators (Li et al., 2012). Moreover, several flavonoids have demonstrated anti-inflammatory prowess by inhibiting the expression of the COX-2 gene (Chen et al., 2000). In the present study, the isolated flavonoids exhibited significant inhibition of writhing and licking compared to the standard. Hence, it can be conjectured that the secondary metabolites, notably flavonoids, may exert an analgesic effect by quelling the inflammatory cascade. Interestingly, other compounds, especially a mixture of α- and β- amyrin, also showed noteworthy activity. Previous studies also support the candidacy of the analgesic activity of amyrin through a prospective association of prostaglandins and TNF-alpha inhibition (Aragao et al., 2008). A monoester extracted from the plant showed relatively mild analgesic activity compared to other compounds, which invalidates its strong candidacy as a lead compound for further drug development exploration.
DHFR, an enzyme present in all living organisms, plays a vital role in cellular metabolic pathways. It facilitates the conversion of dihydrofolate to tetrahydrofolate using reduced nicotinamide adenine dinucleotide phosphate (NADPH) as a cofactor. Tetrahydrofolate is further utilized in the synthesis of thymidylate, purines, and amino acids through biosynthetic processes (Canh Pham and Truong, 2022). Thus, binding to DHFR and inhibition of this enzyme leads to the disruption of nucleic acid synthesis, ultimately causing cell death (Kalogris et al., 2014). Although ciprofloxacin primarily targets GyrA and ParC, docking ciprofloxacin with DHFR would be an exploratory computational exercise to see if there are any meaningful interactions between the drug and the enzyme (Dhillon et al., 2013). In the in silico study, ciprofloxacin showed a docking score of −8.1 kcal/mol with the DHFR enzyme, including carbon-hydrogen bond, pi-alkyl, and unfavorable donor-donor interactions with the respective amino acids (Table 6; Figure 12). Compounds C5, C2a, and C2b surpassed this value and gave docking scores of −9.1 kcal/mol, −8.8 kcal/mol, and −8.8 kcal/mol, respectively (Table 5). Among all the compounds, C5 showed the highest binding affinity with interactions of pi-sigma, alkyl, pi-alkyl, and conventional hydrogen bonds (Table 6; Figure 9). The binding interactions for the other compounds are briefly depicted in Table 6. These binding interactions in molecular docking are important as they determine the strength and stability of the ligand binding to the target protein, which directly influences the efficacy of a potential drug or inhibitor (Morris and Lim-Wilby, 2008).
µ, κ, and δ are the opioid receptors that modulate the activity of the enteric nervous system and the release of neurotransmitters, thereby influencing both stimulatory and inhibitory motor pathways. This sequence of events influences gastrointestinal motility and stool consistency by slowing colonic transit, reducing the sensitivity of enteric nerves, and altering the secretion and transportation of fluids (Pannemans and Corsetti, 2018). Loperamide is an opioid agonist and serves as a potential antidiarrheal agent (Kopsky et al., 2019). In computational docking studies, for antidiarrheal activity, the standard loperamide drug molecule showed a binding affinity of −9.1 kcal/mol with the KOR receptor, including pi-sigma, pi-pi stacked, and alkyl bond interactions (Tables 5, 6; Figure 12). Compounds C2b, C5, and C2a had higher docking scores of −10.5 kcal/mol, −9.7 kcal/mol, and −9.4 kcal/mol, respectively, compared to the standard drug (Table 5). The compound β-amyrin (C2b) exhibited the highest affinity among all the compounds, including alkyl bond interactions with the amino acids Tyr 140, Ile 180, Trp 183, Leu 184, and Ile 191 (Table 5; Figure 10). Considering these binding interactions is for in predicting the binding affinity and stability of a ligand–protein complex, which ultimately affects the design of more effective drugs. Effective docking must consider these interactions to identify strong binders with good pharmacological potential (Morris and Lim-Wilby, 2008).
The identified compounds were docked against the COX-2 enzyme to investigate the peripheral analgesic properties. The prostaglandins synthesized from arachidonic acids by the COX-2 enzyme are responsible for the sensation of pain and inflammation (Lee et al., 2005). Therefore, COX-2 inhibitors are considered to work in chronic pain relief and post-surgical analgesia by reducing the production of prostaglandins responsible for inflammation, pain, and fever, along with the minimization of gastrointestinal side effects (Camu et al., 2003). Diclofenac sodium is a well-established COX-2 inhibitor, and it interferes with the production of prostaglandin G2, which is the precursor to other prostaglandins (Kaur and Sanyal, 2011). In computational docking modeling, this standard drug showed a binding affinity of −7.8 kcal/mol against the COX-2 enzyme, including pi-sigma, amide-pi stacked, pi-alkyl, and conventional hydrogen bond interactions (Tables 5, 6; Figure 12). The compound β-amyrin (C2b) showed a higher docking score of −8.7 kcal/mol than the standard and demonstrated a strong conventional hydrogen bond interaction with the amino acid Asp 347 (Tables 5, 6; Figure 11). Compounds C5 and C6 also exhibited notable binding affinities of −7.2 kcal/mol and −7.3 kcal/mol, respectively. These interactions are crucial for specific binding, as they enhance the affinity and selectivity of the ligand toward the target. The interactions improve docking scores, lead to more stable complexes, and play a key role in fine-tuning the fit of the ligand within the binding pocket, ensuring proper spatial alignment (Morris and Lim-Wilby, 2008).
The ADME/T study of the identified compounds also exhibited promising results, which are listed in Table 7. According to Lipinski, if the following requirements, such as molecular weight <500 amu, hydrogen bond acceptor sites <10, hydrogen bond donor sites <5, and lipophilicity value LogP ≤5 are met, then that particular substance is likely to be orally active (Zhang and Wilkinson, 2007). Compounds penduletin (C1/C4) and 4′,7,8-trihydroxy flavonol (C6) did not violate the five Lipinski principles. Additionally, 7,8-(3″,3″-dimethyl-pyrano)-4′-hydroxy flavonol (C5) violated only one of Lipinski’s rules. However, the compounds α- and β-amyrin (C2a and C2b) each had two violations, and the monoglyceride of stearic acid (C3) compound had three violations. The results suggest that the compounds C1/C4 and C5 conform to Lipinski standards, signifying their oral safety and potential as promising candidates for medicinal purposes.
pkCSM is a novel approach to predicting pharmacokinetic and toxicological consequences that use graph-based signatures to represent the chemistry and topology of small compounds (Pires et al., 2015). Table 7 shows that all the test compounds possessed negative water solubility values (log mol/L), suggesting that they are lipophilic, which promotes effective absorption (Kuentz and Arnold, 2009). The bioavailability scores of all compounds were found to be 0.55%. None of the identified test compounds exhibited any hepatotoxicity. Moreover, none of the compounds was found to inhibit hERG I, and except for C2a, C2b, and C5, none of them inhibited hERG II, indicating that these compounds may not be cardiotoxic (Muster et al., 2008).
Finally, from all the hypotheses of the in silico study, the identified compounds that gave promising docking scores with the respective receptors, adhered to Lipinski’s rule, and conformed satisfactorily with pharmacokinetic and toxicological assumptions should be considered for their drug candidacy and further investigation. Compared with existing therapeutic agents, the identified compounds exhibited remarkable effects in bioassays as well as remarkable binding affinities with the corresponding biomolecules.
Conclusion
Vegetables are a source of nutrition and disease management and can be crucial as nutritional strategies for integrative healthcare. The discovery of promising pharmacological activities and bioactive phytochemicals in locally consumed vegetables can add a new dimension to therapeutics, pharmacological research, and development. The present study represents the first-time report on compound isolation from C. gigantea and C. affinis, employing NMR technique. The outcome reveals three bioactive flavonoids, two triterpenoids, and a monoglyceride, underscoring these species’ polyphenol-rich attributes. Upon biological investigations, these compounds showed prospective antimicrobial, analgesic, and antidiarrheal potentials, justifying the tribal use of these taro vegetables. Further extensive scientific research on the isolated compounds in dosage form on a clinical trial basis is highly recommended to ascertain their safety and efficacy profile and mode of action to ease the process of future drug discovery and development.
Data availability statement
The original contributions presented in the study are included in the article/supplementary material; further inquiries can be directed to the corresponding author.
Ethics statement
The Animal Ethics Number for the experimental animal models of this work is 2023-01-04/SUB/A-ERC/002, which indicates approval by the Animal Ethics Committee, State University of Bangladesh. The study was conducted in accordance with the local legislation and institutional requirements.
Author contributions
SA: conceptualization, data curation, formal analysis, investigation, methodology, writing–original draft, and writing–review and editing. FR: conceptualization, data curation, investigation, methodology, software, writing–original draft, and writing–review and editing. NE: Investigation, software, writing–original draft, and writing–review and editing. AC: supervision, writing–original draft, and writing–review and editing. CH: data curation, supervision, writing–original draft, and writing–review and editing. MH: funding acquisition, supervision, writing–original draft, and writing–review and editing.
Funding
The author(s) declare that financial support was received for the research, authorship, and/or publication of this article. This work received a government fund from the Ministry of Science and Technology, Bangladesh, Reference No: 39.00.0000.012.02.009.23.159 (Date: 9 September 2023), Section: Biology, Medical Science and Nutrition Science Group, SL: 54, GO: 108.
Conflict of interest
The authors declare that the research was conducted in the absence of any commercial or financial relationships that could be construed as a potential conflict of interest.
Publisher’s note
All claims expressed in this article are solely those of the authors and do not necessarily represent those of their affiliated organizations, or those of the publisher, the editors, and the reviewers. Any product that may be evaluated in this article, or claim that may be made by its manufacturer, is not guaranteed or endorsed by the publisher.
References
Abdel Motaal, N. A. R., and Abdel Maguid, A. (2005). Effect of fractionated and single doses gamma irradiation on certain mammalian organs. Egypt. J. Hosp. Med. 19, 111–122. doi:10.21608/ejhm.2005.18115
Agbor, G. A., Léopold, T., and Jeanne, N. Y. (2004). The antidiarrhoeal activity of Alchornea cordifolia leaf extract. Phyther. Res. An Int. J. Devoted Pharmacol. Toxicol. Eval. Nat. Prod. Deriv. 18, 873–876. doi:10.1002/ptr.1446
Ahmad, N. S., Waheed, A., Farman, M., and Qayyum, A. (2010). Analgesic and anti-inflammatory effects of Pistacia integerrima extracts in mice. J. Ethnopharmacol. 129, 250–253. doi:10.1016/j.jep.2010.03.017
Alam, M. M., Emon, N. U., Alam, S., Rudra, S., Akhter, N., Mamun, M. M. R., et al. (2021a). Assessment of pharmacological activities of Lygodium microphyllum Cav. leaves in the management of pain, inflammation, pyrexia, diarrhea, and helminths: in vivo, in vitro and in silico approaches. Biomed. Pharmacother. 139, 111644. doi:10.1016/j.biopha.2021.111644
Alam, S., Emon, N. U., Shahriar, S., Richi, F. T., Haque, M. R., Islam, M. N., et al. (2020). Pharmacological and computer-aided studies provide new insights into Millettia peguensis Ali (Fabaceae). Saudi Pharm. J. 28, 1777–1790. doi:10.1016/j.jsps.2020.11.004
Alam, S., Richi, F. T., Hasnat, H., Ahmed, F., Emon, N. U., Uddin, M. J., et al. (2024). Chemico-pharmacological evaluations of the dwarf elephant ear (Colocasia affinis Schott) plant metabolites and extracts: health benefits from vegetable source. Front. Pharmacol. 15, 1428341. doi:10.3389/fphar.2024.1428341
Alam, S., Sarker, M. M. R., Afrin, S., Richi, F. T., Zhao, C., Zhou, J.-R., et al. (2021b). Traditional herbal medicines, bioactive metabolites, and plant products against COVID-19: update on clinical trials and mechanism of actions. Front. Pharmacol. 12, 671498. doi:10.3389/fphar.2021.671498
Ara, H., and Hassan, A. (2019). Four new varieties of the family araceae from Bangladesh on the family Araceae were made. Hetterscheid Boyce. doi:10.3329/bjpt.v26i1.41913
Ara, H., and Hassan, M. A. (2012). Five new records of aroids for Bangladesh. Bangladesh J. Plant Taxon. 19, 17–23. doi:10.3329/bjpt.v19i1.10937
Aragao, G. F., Pinheiro, M. C. C., Bandeira, P. N., Lemos, T. L. G., and Viana, G. S. de B. (2008). Analgesic and anti-inflammatory activities of the isomeric mixture of alpha-and beta-amyrin from Protium heptaphyllum (Aubl.) march. J. Herb. Pharmacother. 7, 31–47. doi:10.1300/j157v07n02_03
Ashrafi, S., Alam, S., Islam, A., Emon, N. U., Islam, Q. S., and Ahsan, M. (2022). Chemico-biological profiling of blumea lacera (Burm.f.) DC. (Family: asteraceae) provides new insights as a potential source of antioxidant, cytotoxic, antimicrobial, and antidiarrheal agents. Evidence-based Complement. Altern. Med. 2022, 2293415. doi:10.1155/2022/2293415
Barnes, P. J. (1998). Anti-inflammatory actions of glucocorticoids: molecular mechanisms. Clin. Sci. 94, 557–572. doi:10.1042/cs0940557
Bikadi, Z., and Hazai, E. (2009). Application of the PM6 semi-empirical method to modeling proteins enhances docking accuracy of AutoDock. J. Cheminform. 1, 15. doi:10.1186/1758-2946-1-15
Bristy, T. A., Barua, N., Montakim Tareq, A., Sakib, S. A., Etu, S. T., Chowdhury, K. H., et al. (2020). Deciphering the pharmacological properties of methanol extract of psychotria calocarpa leaves by in vivo, in vitro and in silico approaches. Pharmaceuticals 13, 183. doi:10.3390/ph13080183
Camu, F., Shi, L., and Vanlersberghe, C. (2003). The role of COX-2 inhibitors in pain modulation. Drugs 63, 1–7. doi:10.2165/00003495-200363001-00002
Canh Pham, E., and Truong, T. N. (2022). Design, microwave-assisted synthesis, antimicrobial and anticancer evaluation, and in silico studies of some 2-naphthamide derivatives as DHFR and VEGFR-2 inhibitors. ACS Omega 7, 33614–33628. doi:10.1021/acsomega.2c05206
Capasso, A., Pinto, A., Sorrentino, R., and Capasso, F. (1991). Inhibitory effects of quercetin and other flavonoids on electrically-induced contractions of Guinea pig isolated ileum. J. Ethnopharmacol. 34, 279–281. doi:10.1016/0378-8741(91)90048-i
Chen, Y.-C., Yang, L.-L., and Lee, T. J. F. (2000). Oroxylin A inhibition of lipopolysaccharide-induced iNOS and COX-2 gene expression via suppression of nuclear factor-kappaB activation. Biochem. Pharmacol. 59, 1445–1457. doi:10.1016/s0006-2952(00)00255-0
Daina, A., Michielin, O., and Zoete, V. (2017). SwissADME: a free web tool to evaluate pharmacokinetics, drug-likeness and medicinal chemistry friendliness of small molecules. Sci. Rep. 7, 42717. doi:10.1038/srep42717
Dhillon, S. K., Jayaraman, , Sakharkar, P. K., Lim, D., Siddiqui, , and Sakharkar, M. K. (2013). Novel phytochemical& ndash;antibiotic conjugates as multitarget inhibitors of Pseudomononas aeruginosa GyrB/ParE and DHFR. Drug Des. devel. Ther. 449. doi:10.2147/DDDT.S43964
Duraipandiyan, V., Ayyanar, M., and Ignacimuthu, S. (2006). Antimicrobial activity of some ethnomedicinal plants used by Paliyar tribe from Tamil Nadu, India. BMC Complement. Altern. Med. 6, 35–37. doi:10.1186/1472-6882-6-35
Emon, N. U., Alam, S., Rudra, S., Haidar, I. K.Al, Farhad, M., Rana, M. E. H., et al. (2021a). Antipyretic activity of Caesalpinia digyna (Rottl.) leaves extract along with phytoconstituent's binding affinity to COX-1, COX-2, and mPGES-1 receptors: in vivo and in silico approaches. Saudi J. Biol. Sci. 28, 5302–5309. doi:10.1016/j.sjbs.2021.05.050
Emon, N. U., Rudra, S., Alam, S., Haidar, I. K.Al, Paul, S., Richi, F. T., et al. (2021b). Chemical, biological and protein-receptor binding profiling of Bauhinia scandens L. stems provide new insights into the management of pain, inflammation, pyrexia and thrombosis. Biomed. Pharmacother. 143, 112185. doi:10.1016/j.biopha.2021.112185
Guedes, I. A., de Magalhães, C. S., and Dardenne, L. E. (2014). Receptor–ligand molecular docking. Biophys. Rev. 6, 75–87. doi:10.1007/s12551-013-0130-2
Gupta, K., Kumar, A., Tomer, V., Kumar, V., and Saini, M. (2019). Potential of Colocasia leaves in human nutrition: review on nutritional and phytochemical properties. J. Food Biochem. 43, e12878. doi:10.1111/jfbc.12878
Huys, G., D’haene, K., and Swings, J. (2002). Influence of the culture medium on antibiotic susceptibility testing of food-associated lactic acid bacteria with the agar overlay disc diffusion method. Lett. Appl. Microbiol. 34, 402–406. doi:10.1046/j.1472-765x.2002.01109.x
Jiko, P. A., Mohammad, M., Richi, F. T., Islam, M. A., Alam, S., Taher, M. A., et al. (2024). Anti-inflammatory, analgesic and anti-oxidant effects of shirakiopsis indica (willd). Fruit extract: a mangrove species in the field of inflammation research. J. Inflamm. Res. 17, 5821–5854. doi:10.2147/JIR.S470835
Kalogris, C., Garulli, C., Pietrella, L., Gambini, V., Pucciarelli, S., Lucci, C., et al. (2014). Sanguinarine suppresses basal-like breast cancer growth through dihydrofolate reductase inhibition. Biochem. Pharmacol. 90, 226–234. doi:10.1016/j.bcp.2014.05.014
Kaur, J., and Sanyal, S. N. (2011). Diclofenac, a selective COX-2 inhibitor, inhibits DMH-induced colon tumorigenesis through suppression of MCP-1, MIP-1α and VEGF. Mol. Carcinog. 50, 707–718. doi:10.1002/mc.20736
Kavadia, M. R., Yadav, M. G., Odaneth, A. A., and Lali, A. M. (2017). Production of glycerol monostearate by immobilized Candida antarctica B lipase in organic media. J. Appl. Biotechnol. Bioeng. 2, 1–7. doi:10.15406/jabb.2017.02.00031
Khatun, M. C. S., Muhit, M. A., Hossain, M. J., Al-Mansur, M. A., and Rahman, S. M. A. (2021). Isolation of phytochemical constituents from Stevia rebaudiana (Bert.) and evaluation of their anticancer, antimicrobial and antioxidant properties via in vitro and in silico approaches. Heliyon 7, e08475. doi:10.1016/j.heliyon.2021.e08475
Koné, W. M., Atindehou, K. K., Terreaux, C., Hostettmann, K., Traore, D., and Dosso, M. (2004). Traditional medicine in North Côte-d’Ivoire: screening of 50 medicinal plants for antibacterial activity. J. Ethnopharmacol. 93, 43–49. doi:10.1016/j.jep.2004.03.006
Kopsky, D., Bhaskar, A., Zonneveldt, H., and Keppel Hesselink, J. (2019). Topical loperamide for the treatment of localized neuropathic pain: a case report and literature review. J. Pain Res. 12, 1189–1192. doi:10.2147/JPR.S196927
Kuentz, M. T., and Arnold, Y. (2009). Influence of molecular properties on oral bioavailability of lipophilic drugs – mapping of bulkiness and different measures of polarity. Pharm. Dev. Technol. 14, 312–320. doi:10.1080/10837450802626296
Lee, Y., Rodriguez, C., and Dionne, R. (2005). The role of COX-2 in acute pain and the use of selective COX-2 inhibitors for acute pain relief. Curr. Pharm. Des. 11, 1737–1755. doi:10.2174/1381612053764896
Li, L., Bao, H., Wu, J., Duan, X., Liu, B., Sun, J., et al. (2012). Baicalin is anti-inflammatory in cigarette smoke-induced inflammatory models in vivo and in vitro: a possible role for HDAC2 activity. Int. Immunopharmacol. 13, 15–22. doi:10.1016/j.intimp.2012.03.001
Liu, R. H. (2007). Whole grain phytochemicals and health. J. Cereal Sci. 46, 207–219. doi:10.1016/j.jcs.2007.06.010
Makhmoor, T., and Choudhary, M. I. (2010). Radical scavenging potential of compounds isolated from Vitex agnus-castus. Turk. J. Chem. 34, 119–126. doi:10.3906/kim-0805-46
Medzhitov, R. (2008). Origin and physiological roles of inflammation. Nature 454, 428–435. doi:10.1038/nature07201
Meli, R., Autore, G., Di Carlo, G., and Capasso, F. (1990). Inhibitory action of quercetin on intestinal transit in mice. Phyther. Res. 4, 201–202. doi:10.1002/ptr.2650040509
Migas, P., Cisowski, W., and Dembinska-Migas, W. (2005). Isoprene derivatives from the leaves and callus cultures of Vaccinium corymbosum var. bluecrop. Acta Pol. Pharm. 62, 45–51.
Morris, G. M., and Lim-Wilby, M. (2008). Molecular docking. Methods Mol. Biol. 443, 365–382. doi:10.1007/978-1-59745-177-2_19
Muhammad, N., Lal Shrestha, R., Adhikari, A., Wadood, A., Khan, H., Khan, A. Z., et al. (2015). First evidence of the analgesic activity of govaniadine, an alkaloid isolated from Corydalis govaniana Wall. Nat. Prod. Res. 29, 430–437. doi:10.1080/14786419.2014.951933
Muster, W., Breidenbach, A., Fischer, H., Kirchner, S., Müller, L., and Pähler, A. (2008). Computational toxicology in drug development. Drug Discov. Today 13, 303–310. doi:10.1016/j.drudis.2007.12.007
Otshudi, A. L., Vercruysse, A., and Foriers, A. (2000). Contribution to the ethnobotanical, phytochemical and pharmacological studies of traditionally used medicinal plants in the treatment of dysentery and diarrhoea in Lomela area, Democratic Republic of Congo (DRC). J. Ethnopharmacol. 71, 411–423. doi:10.1016/s0378-8741(00)00167-7
Panda, S. K., Niranjan Patra, N. P., Gunanidhi Sahoo, G. S., Bastia, A. K., and Dutta, S. K. (2012). Anti-diarrheal activities of medicinal plants of similipal biosphere reserve, odisha, India.
Pannemans, J., and Corsetti, M. (2018). Opioid receptors in the GI tract: targets for treatment of both diarrhea and constipation in functional bowel disorders? Curr. Opin. Pharmacol. 43, 53–58. doi:10.1016/j.coph.2018.08.008
Parmar, G., Chudasama, J. M., Shah, A., and Patel, A. (2023). in Silico pharmacology and drug repurposing approaches, 253–281. doi:10.1007/978-981-99-1316-9_11
Parmar, G., Shah, A., Shah, S., and Seth, A. K. (2022). Identification of bioactive phytoconstituents from the plant euphorbia hirta as potential inhibitor of sars-cov-2: an in-silico approach. Biointerface Res. Appl. Chem. 12, 1385–1396. doi:10.33263/BRIAC122.13851396
Parmar, G. R., Shah, A. P., Sailor, G. U., and Seth, A. K. (2020). In silico discovery of novel phytoconstituents of amyris pinnata as a mitotic spindle kinase inhibitor. Curr. Drug Res. Rev. Former. Curr. Drug Abus. Rev. 12, 175–182. doi:10.2174/2589977512666200220122211
Pires, D. E. V., Blundell, T. L., and Ascher, D. B. (2015). pkCSM: predicting small-molecule pharmacokinetic and toxicity properties using graph-based signatures. J. Med. Chem. 58, 4066–4072. doi:10.1021/acs.jmedchem.5b00104
Ponce, M. A., Bompadre, M. J., Scervino, J. M., Ocampo, J. A., Chaneton, E. J., and Godeas, A. M. (2009). Flavonoids, benzoic acids and cinnamic acids isolated from shoots and roots of Italian rye grass (Lolium multiflorum Lam.) with and without endophyte association and arbuscular mycorrhizal fungus. Biochem. Syst. Ecol. 37, 245–253. doi:10.1016/j.bse.2009.03.010
Qing-Hui, W., Shuai, G. U. O., Xue-Yan, Y., Zhang, Y.-F., Shang, M.-Y., Shang, Y.-H., et al. (2017). Flavonoids isolated from Sinopodophylli Fructus and their bioactivities against human breast cancer cells. Chin. J. Nat. Med. 15, 225–233. doi:10.1016/S1875-5364(17)30039-0
Rudra, S., Sawon, M., Emon, N., Alam, S., Tareq, S., Islam, M., et al. (2020). Biological investigations of the methanol extract of Tetrastigma leucostaphylum (Dennst.) Alston ex Mabb. (Vitaceae): in vivo and in vitro approach. J. Adv. Biotechnol. Exp. Ther. 3, 216. doi:10.5455/jabet.2020.d127
Shahriar, S., Shermin, S. A., Hasnat, H., Hossain, F., Han, A., Geng, P., et al. (2024). Chemico-pharmacological evaluation of the methanolic leaf extract of Catharanthus ovalis: GC–MS/MS, in vivo, in vitro, and in silico approaches. Front. Pharmacol. 15, 1347069. doi:10.3389/fphar.2024.1347069
Shompa, S. A., Hasnat, H., Riti, S. J., Islam, M. M., Nur, F., Alam, S., et al. (2024). Phyto-pharmacological evaluation and characterization of the methanolic extract of the Baccaurea motleyana Müll. Arg. seed: promising insights into its therapeutic uses. Front. Pharmacol. 15, 1359815–1359826. doi:10.3389/fphar.2024.1359815
Sostres, C., Gargallo, C. J., Arroyo, M. T., and Lanas, A. (2010). Adverse effects of non-steroidal anti-inflammatory drugs (NSAIDs, aspirin and coxibs) on upper gastrointestinal tract. Best. Pract. Res. Clin. Gastroenterol. 24, 121–132. doi:10.1016/j.bpg.2009.11.005
Sultana, N., Chung, H. J., Emon, N. U., Alam, S., Taki, M. T. I., Rudra, S., et al. (2022). Biological functions of Dillenia pentagyna roxb. Against pain, inflammation, fever, diarrhea, and thrombosis: evidenced from in vitro, in vivo, and molecular docking study. Front. Nutr. 9, 911274–911313. doi:10.3389/fnut.2022.911274
Sun, X., Sit, A., and Feinberg, M. W. (2014). Role of miR-181 family in regulating vascular inflammation and immunity. Trends cardiovasc. Med. 24, 105–112. doi:10.1016/j.tcm.2013.09.002
VanWagenen, B. C., Larsen, R., Cardellina, J. H., Randazzo, D., Lidert, Z. C., and Swithenbank, C. (1993). Ulosantoin, a potent insecticide from the sponge Ulosa ruetzleri. J. Org. Chem. 58, 335–337. doi:10.1021/jo00054a013
Wagner, W. L., Herbst, D. R., and Sohmer, S. H. (1990). in Manual of the flowering plants of hawai’i (Hawaii, United States: University of Hawaii Press).
Wang, Y., Hamburger, M., Gueho, J., and Hostettmann, K. (1989). Antimicrobial flavonoids from Psiadia trinervia and their methylated and acetylated derivatives. Phytochemistry 28, 2323–2327. doi:10.1016/s0031-9422(00)97976-7
Wilhelm, J., Vytášek, R., Uhlík, J., and Vajner, L. (2016). Oxidative stress in the developing rat brain due to production of reactive oxygen and nitrogen species. Oxid. Med. Cell. Longev. 2016, 5057610. doi:10.1155/2016/5057610
Workneh, B. S., Mekonen, E. G., Ali, M. S., Gonete, A. T., Techane, M. A., Wassie, M., et al. (2024). Recommended homemade fluid utilization for the treatment of diarrhea and associated factors among children under five in sub-Saharan African countries: a multilevel analysis of the recent demographic and health survey. BMC Pediatr. 24, 322. doi:10.1186/s12887-024-04810-2
Yadav, J. P., Kumar, S., and Siwach, P. (2006). Folk medicine used in gynecological and other related problems by rural population of Haryana.
Zhang, M.-Q., and Wilkinson, B. (2007). Drug discovery beyond the ‘rule-of-five. Curr. Opin. Biotechnol. 18, 478–488. doi:10.1016/j.copbio.2007.10.005
Zilani, M. N. H., Islam, M. A., Biswas, P., Anisuzzman, M., Hossain, H., Shilpi, J. A., et al. (2021). Metabolite profiling, anti-inflammatory, analgesic potentials of edible herb Colocasia gigantea and molecular docking study against COX-II enzyme. J. Ethnopharmacol. 281, 114577. doi:10.1016/j.jep.2021.114577
Keywords: Colocasia gigantea, Colocasia affinis, vegetable, NMR, antibacterial, antidiarrheal, analgesic, anti-inflammatory
Citation: Alam S, Richi FT, Emon NU, Chowdhury AA, Hasan CM and Haque MR (2024) First-time report on compound isolation from two Colocasia species: vegetable-derived bioactive metabolites and their medicinal potential. Front. Pharmacol. 15:1474706. doi: 10.3389/fphar.2024.1474706
Received: 02 August 2024; Accepted: 28 October 2024;
Published: 04 December 2024.
Edited by:
Alessandra Durazzo, Council for Agricultural Research and Economics, ItalyReviewed by:
Laiba Arshad, Forman Christian College, PakistanSudip Mandal, Dr. B. C. Roy College of Pharmacy and Allied Health Sciences, India
Ghanshyam Ratilal Parmar, Sumandeep Vidyapeeth University, India
Abhijit Das, Noakhali Science and Technology University, Bangladesh
Titilayo Omolara Johnson, University of Jos, Nigeria
A. H. M. Khurshid Alam, University of Rajshahi, Bangladesh
Copyright © 2024 Alam, Richi, Emon, Chowdhury, Hasan and Haque. This is an open-access article distributed under the terms of the Creative Commons Attribution License (CC BY). The use, distribution or reproduction in other forums is permitted, provided the original author(s) and the copyright owner(s) are credited and that the original publication in this journal is cited, in accordance with accepted academic practice. No use, distribution or reproduction is permitted which does not comply with these terms.
*Correspondence: Mohammad Rashedul Haque, aGFxdWVtckBkdS5hYy5iZA==
†ORCID: Safaet Alam, orcid.org/0000-0002-1831-2278; Fahmida Tasnim Richi, orcid.org/0000-0002-7271-4219; Nazim Uddin Emon, orcid.org/0000-0001-7567-4796; Abu Asad Chowdhury, orcid.org/0000-0003-0952-8250; Choudhury Mahmood Hasan, orcid.org/0000-0001-7026-4368; Mohammad Rashedul Haque, orcid.org/0000-0001-5980-3877