- 1Department of Stomatology, The First Affiliated Hospital of Hainan Medical University, Haikou, China
- 2School of Stomatology, Hainan Medical University, Haikou, China
- 3Integrated Department, Hainan Stomatological Hospital, Haikou, China
The main bioactive components of agarwood, derived from Aquilaria sinensis, include sesquiterpenes, 2-(2-phenethyl) chromone derivatives, aromatic compounds, and fatty acids, which typically exert anti-inflammatory, antioxidant, immune-modulating, hypoglycemic, and antitumor pharmacological effects in the form of essential oils. Agarwood tree leaves, rich in flavonoids, 2-(2-phenethyl) chromone compounds, and flavonoid compounds, also exhibit significant anti-inflammatory, antioxidant, and immune-modulating effects. These properties are particularly relevant to the treatment of periodontitis, given that inflammatory responses, oxidative stress, and immune dysregulation are key pathological mechanisms of the disease, highlighting the substantial potential of agarwood and agarwood tree leaves in this therapeutic area. However, the low solubility and poor bioavailability of essential oils present challenges that necessitate the development of improved active formulations. In this review, we will introduce the bioactive components, extraction methods, pharmacological actions, and clinical applications of agarwood and agarwood tree leaves, analyzing its prospects for the treatment of periodontitis.
1 Introduction
Periodontitis is an inflammatory disease of the periodontal tissues with plaque as the initiating factor and the host immune response as the promoting factor, which can cause clinical symptoms such as bleeding gums, periodontal pocket formation, alveolar bone resorption, loose and shifting teeth and even tooth loss. The 1999 Classification of Periodontal Diseases divides periodontal disease into gingivitis and periodontitis. The 2017 International Classification Symposium on Periodontal and Peri-implant Diseases addresses the revision of the 1999 Classification of Periodontal Diseases by simplifying the classification of periodontitis and adding staging and grading of periodontitis, which will help to make the diagnosis of periodontitis more precise and provide individualized diagnostic and therapeutic strategies for patients with periodontitis. In the new 2018 classification of periodontitis, the clinical definition is a microbial-associated, host-mediated inflammatory response to loss of periodontal attachment. The clinical diagnostic criteria are the presence of adjacent clinical attachment loss (CAL) on two or more teeth that are not adjacent to each other or the presence of buccal or lingual CAL ≥3 mm with periodontal pockets ≥3 mm on the buccal or lingual side of 2 or more teeth. The diagnosis of periodontitis can be confirmed by fulfilling one of these criteria (Tonetti and Sanz, 2019). Nowadays, periodontitis has been classified as a major dental disease by the World Health Organization (WHO) and is a public health problem that affects the oral and systemic health of the global population. Studies have shown that the global prevalence of chronic periodontitis is approximately 11.2%, making it the sixth most prevalent disease in the world, with approximately 743 million people worldwide suffering from chronic periodontitis (Marcenes et al., 2013). The prevalence of periodontitis increases with age, with the highest incidence in the 30–40-year-old age group, and with the aging of the global population, the prevalence of periodontitis is not encouraging (Kassebaum et al., 2014). The results of China’s fourth national oral epidemiology survey in 2017 showed that approximately 90% of adults suffer from varying degrees of periodontitis, and the prevalence of severe periodontitis in middle-aged and elderly patients increases significantly with higher age (Jiao et al., 2021). Due to the long-term inflammatory state of periodontal tissues, irreversible resorption of alveolar bone, resulting in tooth loosening and displacement, is the main cause of tooth loss in adults, resulting in a lower quality of life for patients and an increased financial burden of oral health maintenance.
Periodontitis can not only destroy periodontal tissues, but its dominant pathogenic bacteria, bacterial products and inflammatory mediators of periodontal tissues could reach the systemic organs with the help of blood circulation. A large body of evidence demonstrates that periodontitis is closely related to systemic diseases such as diabetes mellitus, cardiovascular disease, chronic obstructive pulmonary disease, chronic nephritis, and other systemic diseases (Chapple et al., 2013) and that the specific mechanisms may be related to metastatic infections, inflammatory responses, inflammatory mediators, and adaptive immune responses (Sanz et al., 2020). Periodontitis, the sixth most common complication of diabetes mellitus, microinflammation of periodontal tissues can affect glycemic control (Linden et al., 2013). Besides, a hyperglycemic environment, advanced glycosylation end-products, and hyperlipidemia can also exacerbate the inflammatory response of periodontal tissues (Sharma et al., 2016). There was a correlation between the severity of periodontitis and atherosclerotic plaque thickness, and the presence of Porphyromonas gingivalis was detected within the atherosclerotic plaque (Van Dyke and van Winkelhoff, 2013). Serum concentrations of C-reactive protein and low-density lipoprotein were elevated in patients with periodontitis, and expression levels of cardiovascular risk markers decreased after periodontal non-surgical treatment (Saini et al., 2011).
Nowadays, the treatment of periodontitis is mainly based on mechanical treatment, such as basic periodontal treatment and periodontal surgical treatment, supplemented by chemical medication, such as minocycline hydrochloride, metronidazole, chlorhexidine, doxycycline (Cai et al., 2024). However, the long-term use of antimicrobial drugs for periodontitis treatment is prone to adverse reactions such as drug resistance phenomenon and dysbiosis. In contrast, natural drug treatment has the advantage of fewer adverse reactions, so the search for natural drug treatment for periodontitis has turned out to be a new research direction.
In inflammatory treatment, phytotherapy can exert anti-inflammatory effects by modulating inflammatory mechanisms and releasing inflammatory mediators (Chang et al., 2013). Agarwood refers to the resin component formed after the Aquilaria or Gyrinops tree of the Thymelaeaceae family has been injured by the outside world, whose effective components are mainly composed of sesquiterpenes, 2-(2-phenylethyl) chromones, and aromatic components, with a wide range of pharmacological effects, such as anti-inflammatory, antioxidant, anti-bacterial, anti-tumor, and other effects (Muluke et al., 2016). Advantages of agarwood oil also exist in aromatherapy for mental disorders such as depression, anxiety, and insomnia (Zheng et al., 2018). In traditional Chinese medicine, agarwood is also used to alleviate symptoms such as chest and abdominal distension, gastric cold vomiting and dyspnea. In this article, we review the existing literature on the effective ingredients, extraction methods, pharmacological effects, and clinical applications of agarwood, summarizing the latest research in agarwood.
2 Major chemical composition of agarwood
Agarwood is commonly found in tropical forested areas, mainly in Southeast Asia, Southern China, and Indonesia. Globally, there are about 13 species of trees that can produce agarwood after external damage. These species include A. baillonii, A. beccariana, A. crassna, A. filaria, A. hirta, A. khasiana, A. malaccensis, A. microcarpa, A. rostrata, A. rugosa, A. sinensis, A. subintegra and A. yunnanensis (Lee and Mohamed, 2016). Agarwood owns a wide range of pharmacological effects, whose chemical composition are mainly made up of sesquiterpenes, 2-(2-phenylethyl) chromones, and aromatics (Pavlic et al., 2021).
2.1. Sesquiterpenoid
Sesquiterpenoids, as one of the active ingredients of agarwood, are formed by three isoprene units, and its content in agarwood extracts is high, accounting for 52% of the chemical composition of agarwood (Chen et al., 2012). It can be regarded as a criterion for grading the quality of agarwood. More than 210 sesquiterpenes have been extracted from agarwood, which can be categorized into eight main types (Smiley et al., 2015). Sesquiterpenes from agarwood exhibit a wide range of pharmacological effects, including anti-inflammatory, anti-tumor, antibacterial activities, inhibition of glycosidase, and regulation of the central nervous system (Wang Y. et al., 2022; Chen X. et al., 2022; Yang et al., 2019). A study by Xie demonstrated that sesquiterpenes from agarwood have significant anti-inflammatory activity, with an IC50 value for NO in lipopolysaccharide-induced RAW264.7 cells ranging from 5.46 to 14.07 μM (using aminoguanidine as a positive control with an IC50 of 20.33 ± 1.08 μM) (Xie et al., 2021). Huang’s research showed that sesquiterpenes from agarwood are effective against tumors and malaria (Huang X. L. et al., 2023). Additionally, the 2-(2-phenylethyl) chromone-sesterterpene hybrid compounds found in agarwood have protective effects on gastric mucosal cells and may be used to treat renal fibrosis by inhibiting the phosphorylation of Smad 3 (Huang et al., 2022; Zhang et al., 2023).
2.2 2-(2-Phenylethyl)chromone
2-(2-Phenylethyl) chromone is synthesized through the substitution of the phenylethyl moiety at the C-2 position of the chromogenic ketone, which accounts for 41% of the content in agarwood (Lee and Mohamed, 2016). The high cost of obtaining agarwood samples and chromone standards presents a significant challenge in the identification of agarwood chromone derivatives. To address this, Du has developed an enhanced data integration and filtering strategy that provides a detailed characterization and summary of the characteristic fragments and cleavage rules for 2-(2-phenylethyl) chromone monomers and their dimers, which introduces a novel research approach for the identification of agarwood chromone monomers and dimers (Li W. et al., 2021). As a principal bioactive constituent of agarwood, 2-(2-Phenylethyl)chromone exhibits a wide range of therapeutic properties, including anti-inflammatory, anti-tumor, neuroprotective functions, and the inhibition of acetylcholinesterase and glucosidases (Chen et al., 2012). The 2-(2-phenylethyl)chromone derivatives extracted from agarwood exhibit significant inhibitory effects on α-glucosidase, with IC50 values ranging from 7.8 ± 0.3 to 137.7 ± 3.0 μM (Acarbose, 743.4 ± 3.3 μM; Genistein, 8.3 ± 0.1 μM) (Wang Y. et al., 2022). Sarmah utilized computational simulation methods such as molecular docking, absorption, distribution, metabolism, excretion, toxicity, and molecular dynamics to discover that 2-(2-phenylethyl) chromone from agarwood can efficiently inhibit cyclooxygenase (COX) and lipoxygenase (LOX), with a higher affinity compared to ibuprofen, which suggests its potential as an effective inhibitor for the treatment of inflammatory diseases (Yang et al., 2019). Agarwood possesses unique advantages in the treatment of cardiovascular diseases. Derivatives of 2-(2-phenylethyl) chromone have been shown to mitigate the expression of CD36 in macrophages mediated by endoplasmic reticulum stress, thereby inhibiting the formation of foam cells and exerting a therapeutic effect on atherosclerosis (Chen L. et al., 2022). This mechanism of action represents a promising approach in the management of cardiovascular conditions, where the regulation of cellular processes and inflammation plays a pivotal role. The ability of these chromone derivatives to modulate key pathways involved in atherosclerotic plaque development highlights their potential as novel therapeutic agents. In addition, research by Ma has demonstrated that agarwood extracts rich in 2-(2-phenylethyl)chromone can ameliorate taurocholic acid-induced apoptosis in gastric epithelial cells by modulating endoplasmic reticulum stress, thereby exhibiting therapeutic efficacy against bile reflux gastritis (Xie et al., 2021). This finding underscores the potential of 2-(2-phenylethyl) chromone-enriched agarwood extracts in treating gastrointestinal conditions, particularly those characterized by cellular damage and inflammatory responses.
2.3 Low-weight aromatic compounds (LACs)
Agarwood has a long-standing history in aromatherapy (Huang X. L. et al., 2023), where its unique fragrance emitted upon heating is known for its beneficial effects on sleep disorders. The smoke from burning agarwood contains a significant amount of LACs, which, when inhaled, can aid in sleep induction and improvement (Huang et al., 2022). Benzyl acetone is a key component responsible for the sedative effects (Zhang et al., 2023). Research by Castro has indicated that while LACs from agarwood exhibit sedative potential when inhaled individually, the combined use of these compounds results in a diminished sedative effect (Castro and Ito, 2021). This reduction in efficacy may be attributed to antagonistic actions due to the structural similarities among the LACs (Table 1).
3 Induction methods of agarwood
Due to its unique aromatic odor and valuable medicinal value, agarwood is widely demanded in the world, which leads to its high price (Naziz et al., 2019). Agarwood is composed of resin secreted by trees after the outside world has stimulated them, leading to the scarcity of wild agarwood, coupled with its high price, exacerbating the uncontrolled logging of wild agarwood, making the survival of agarwood trees even more serious (Azren et al., 2018). Thus, it is particularly important to find ways to produce agarwood sustainably.
The formation pathways of agarwood are primarily divided into natural occurrence and artificial induction. In the natural process, agarwood is often formed when a tree is struck by lightning, bitten by animals, or invaded by microorganisms, leading to the formation of wounds that trigger the tree’s defense response and subsequent resin secretion. The advantage of this method is that it can produce high-quality agarwood without the need for human intervention and at a low cost. However, the disadvantages are also apparent: the long time required for agarwood formation, low yield, inconsistent quality, and the increased risk of indiscriminate harvesting of wild trees (Azren et al., 2018).
3.1 Traditional induction methods
As mentioned above, the natural formation of agarwood has several drawbacks, making the technology of artificial induction of agarwood a key solution to address the issues mentioned above, with the aim of increasing agarwood production to meet market demand. With the advancement of technology, the quality of artificially induced agarwood has significantly improved. Research by Ma has shown that the chemical composition of artificially induced agarwood is similar to that of wild agarwood, and its antioxidant properties, as well as its ability to inhibit glucosidase and acetylcholinesterase, are comparable to those of wild agarwood (Ma et al., 2023).
The artificial induction of agarwood formation is primarily categorized into traditional methods, biological induction, and chemical induction (Yan et al., 2019). Traditional methods involve creating wounds on the tree through manual drilling, hole-making, burning, or chopping to simulate the natural conditions under which a tree would be injured, thereby inducing resin secretion and the formation of agarwood. The advantage of these methods is that they do not require guidance from specialized technical personnel. However, the downside is that agarwood is formed only locally at the site of injury, leading to inconsistent yields and average quality (Azren et al., 2018). Xu’s research on the dynamic response of agarwood to mechanical damage has revealed the molecular mechanisms behind agarwood formation, providing a new reference for the quality assessment of agarwood (Xu et al., 2023).
3.2 Biological induction methods
Artificial biological induction involves drilling into the tree and implanting biological inducers to simulate the natural infection of trees. However, not all microorganisms are capable of inducing healthy agarwood trees to form agarwood. Commonly used fungal species include Fusarium, Lasiodiplodia, Penicillium, and Aspergillus (Chen et al., 2017; Sangareswari Nagajothi et al., 2016). Phaeoacremonium rubrigenum can promote the accumulation of sesquiterpene compounds in agarwood by inducing plant host phosphorylation (Liu et al., 2022). Huang has demonstrated that agarwood induced artificially using fungal inducers prepared from wild agarwood is similar in quality to wild agarwood (Huang M. et al., 2023). Wu has developed the Biologically Agarwood-Inducing Technique (Agar-Bit), where biological inducers are injected into the heartwood part of the agarwood tree (Wu and Yu, 2023). This method can induce agarwood formation within 6 months while maintaining the health and vitality of the tree, offering a new approach to whole-tree agarwood induction. The advantages of biological induction include low cost and ease of operation. However, the downside is the potential inconsistency in agarwood quality due to variations in microbial populations at the inoculation sites (Naziz et al., 2019).
3.3 Chemical induction methods
When it was discovered that certain signaling molecules could trigger the defense response of agarwood trees, promoting the secretion of resin components in healthy agarwood to resist external stimuli, chemical induction became a new research hotspot (Wu et al., 2024). Chemical induction can solve the problem of poor repeatability in the quality of agarwood formed through biological induction, and it appears to be the most ideal and reliable alternative to natural agarwood formation.
Chemical induction can solve the problem of poor reproducibility of agarwood formation quality in biological induction and seems to be the most ideal and reliable alternative to natural agarwood formation. Chemical induction refers to the direct application of chemical agents such as ferrous chloride, hydrogen peroxide, and methyl jasmonate to trees to induce agarwood formation. For example, hydrogen peroxide promotes programmed cell death in agarwood and the accumulation of salicylic acid and sesquiterpene compounds (Lv et al., 2019; Liu et al., 2015). Methyl jasmonate promotes the formation of sesquiterpene compounds in agarwood (Xu et al., 2016; Sun P. W. et al., 2020). Currently, several techniques for chemical induction of agarwood have been developed, such as the Cultivated Agarwood Kit (CA-kit), Biological Agarwood Induction Technology (Agar-bit), and Whole-Tree Agarwood Induction Technology (Agar-Wit) (Tan et al., 2019). The advantages of chemical induction include convenient operation, stable and reliable agarwood quality, and the ability to induce the entire tree. However, the downside is that it can easily cause tree rot, high concentrations of chemical agents can cause the death of the tree, and the potential environmental hazards of chemical agents must still be considered (Azren et al., 2018; Faizal et al., 2021). Therefore, before the large-scale promotion of the chemical induction method, extensive experiments are still needed to verify its safety and feasibility repeatedly (Table 2).
4 Methods of agarwood essential oil extraction
The pharmacological effects of agarwood are extensive, and its active ingredients often exist in the form of essential oil. However, since the extraction process of agarwood essential oil is not the primary focus of this article, a brief introduction to the relevant extraction methods will be provided below. Traditional methods of agarwood essential oil extraction include steam distillation and hydro-distillation, both of which are based on the principle that water and essential oil are incompatible with each other to achieve the purpose of separation and purification of essential oil. However, they have been gradually replaced by new extraction methods due to the shortcomings of long time-consuming, low production capacity and high energy consumption (Alamil et al., 2022). In contrast, supercritical fluid extraction and subcritical water extraction are recognized for their high efficiency, stable quality, and the richness of active components they yield (Ibrahim et al., 2011). Research by Tian has demonstrated that agarwood oil extracted using supercritical extraction exhibits superior antioxidant activity and antimicrobial effects compared to that obtained through steam distillation (Tian et al., 2019). At a concentration of 10 g/L, the agarwood essential oil extracted by supercritical fluid extraction achieved a scavenging rate of 96.2%, while steam distillation only reached a scavenging rate of 67.7%. Regarding the DPPH radical scavenging ability, the essential oil of agarwood extracted by supercritical fluid extraction showed an increase in the scavenging rate from 33.69% to 92.15% at concentrations ranging from 1 to 10 g/L. In contrast, the essential oil extracted by steam distillation reached a maximum scavenging rate of only 40.98% at concentrations between 8 and 14 g/L. Samadi conducted a comparison between hydro-distillation and subcritical water extraction methods for agarwood essential oil extraction and found that subcritical water extraction can produce agarwood essential oil of higher quality with a greater abundance of active components (Samadi et al., 2020). Analysis with GC-MS revealed that HD, involving a 1-week soaking period followed by 16 h of distillation, yielded an essential oil from which 43 chemical constituents could be identified. In contrast, the essential oil extracted using the SCWE method, which took only 13 min, contained 50 identifiable chemical constituents. The proportion of bioactive components such as sesquiterpenes and chromone compounds was higher in the essential oil obtained by SCWE than in HD. Additionally, the essential oil extracted via the SCWE method contained a greater variety of bioactive compounds, including guaiacol, syringol, furfural, vanillin, and phenylacetaldehyde.
5 Pharmacological effects of agarwood
Due to its richness in sesquiterpenes, 2-(2-phenylethyl) chromones, aromatic compounds and its potent anti-inflammatory, antioxidant, antibacterial, antitumor, sedative and analgesic pharmacological effects, agarwood is commonly used to treat cardiovascular, nervous system, digestive, and respiratory diseases. The following sections will introduce its pharmacological effects (Figures 1).
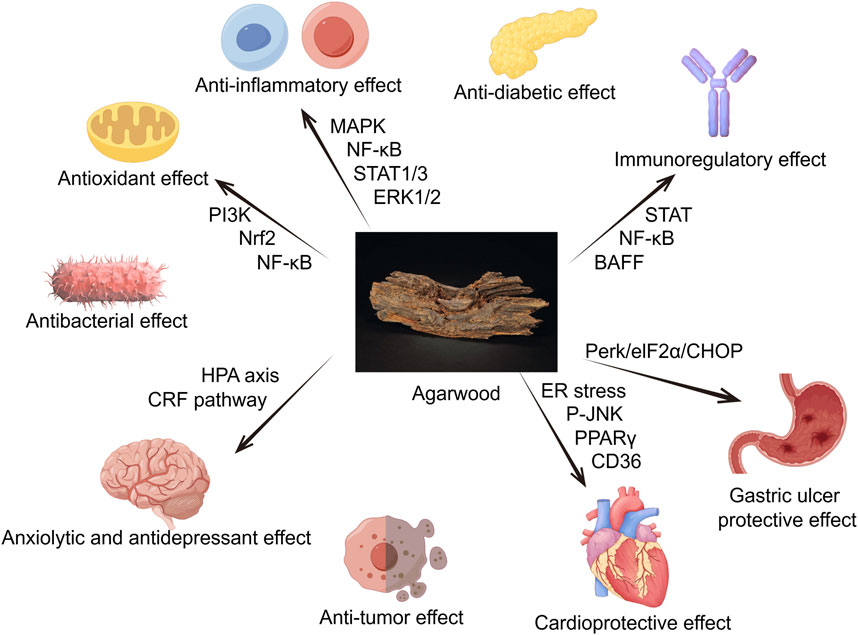
Figure 1. Agarwood has a positive effect on multiple organs and physiological activities throughout the body, such as anti-inflammatory, antioxidant, antibacterial, anxiolytic and antidepressant, anti-tumor, anti-diabetic, acetylcholinesterase inhibition and other effects (By Figdraw).
5.1 Anti-inflammatory effect
Inflammatory response refers to maintaining homeostasis by eliminating pathogenic microorganisms through intrinsic and adaptive immune responses when pathogenic microorganisms stimulate the organism. There has been a significant amount of research on the anti-inflammatory effects of agarwood essential oil against inflammatory diseases, and the anti-inflammatory effects of agarwood are attributed to its ability to modulate the production of inflammatory mediators and reduce oxidative stress, which are critical factors in the pathogenesis of various inflammatory diseases (Alamil et al., 2022). As previously mentioned, sesquiterpenes and 2-(2-phenylethyl) chromone compounds exhibit potent anti-inflammatory activities. Numerous vitro studies have demonstrated that sesquiterpene compounds isolated from agarwood can effectively inhibit the release of nitric oxide by macrophages induced by lipopolysaccharide (Xie et al., 2021; Zhao et al., 2016; Hu et al., 2024). However, the specific mechanisms by which sesquiterpene compounds exert their anti-inflammatory effects remain to be elucidated. In contrast, 2-(2-phenylethyl) chromone compounds have been shown to suppress the release of nitric oxide in RAW 264.7 cells during inflammation by inhibiting the activation of NF-κB without cytotoxicity (Wang S. L. et al., 2018; Yu et al., 2020; Yang et al., 2023). Additionally, 2-(2-phenylethyl)chromone compounds can inhibit the release of inflammatory mediators such as NO, TNF-α, IL-6, IL-1β, and PGE2 by suppressing the STAT and MAPK signaling pathways (Zhu et al., 2016a). Gao administered agarwood essential oil via gavage, effectively suppressing ear edema induced by dimethyl benzene and foot edema caused by carrageenan (Gao et al., 2019). This anti-inflammatory effect may be associated with the inhibition of p-STAT3 protein expression. In addition, agarwood extracts have been shown to provide protective effects against gastric ulcers and intestinal mucositis by suppressing inflammatory responses and alleviating anxiety symptoms (Wang C. et al., 2021; Zheng et al., 2019; Wang S. et al., 2018). Inflammatory responses are closely related to conditions such as cancer and immune reactions, and agarwood has demonstrated inhibitory effects on various inflammatory diseases, which could potentially validate the broad pharmacological activities of agarwood from a different perspective (Table 3).
5.2 Antioxidant effect
Chronic respiratory diseases such as chronic obstructive pulmonary disease and asthma often exhibit pathological characteristics of oxidative stress and inflammatory responses. Agarwood essential oil, rich in functionally active components such as sesquiterpenes and 2-(2-phenylethyl) chromones, can be a potential source of antioxidant active materials. Chen’s research indicates that agarwood essential oil’s DPPH and ABTS radical scavenging abilities are positively correlated with concentration (Chen L. et al., 2022). At a 5 mg/mL concentration, agarwood essential oil obtained by supercritical extraction exhibited a DPPH radical scavenging rate of over 50%. At a concentration of 2 mg/mL, the ABTS radical scavenging rate ranged from 53.03% to 83.26%. Ma’s research shows that the DPPH and ABTS radical scavenging capabilities of Induced agarwood (IA) and wild agarwood (WA) are close (Ma et al., 2023). At a concentration of 0.8 mg/mL, the DPPH radical scavenging rates for IA and WA were 91.26% and 91.59%, respectively. At 0.2 mg/mL, the ABTS radical scavenging rates for IA and WA were 91.03% and 94.80%, respectively.
Due to the poor solubility and low bioavailability of agarwood essential oil, these factors have become barriers to its clinical application and promotion. To address this issue, Rubis utilized ultrasonication to prepare agarwood essential oil into a nanoemulsion, investigating its potential antioxidant and anti-inflammatory effects on airway basal epithelial cells under cigarette smoke extract stimulation. The experimental results showed that the agarwood nanoemulsion could inhibit oxidative stress by inducing the expression of antioxidant genes and exert protective effects by suppressing inflammatory responses, demonstrating the therapeutic potential of agarwood for chronic obstructive pulmonary disease (De Rubis et al., 2023). In further research, Malik prepared agarwood oil nanoemulsions and explored its antioxidant and anti-inflammatory capabilities on lipopolysaccharide-induced RAW264.7 macrophages. The results demonstrated that the agarwood oil nanoemulsions significantly reduced the production of reactive oxygen species (ROS) and the expression of the antioxidant gene heme oxygenase-1 (HO-1), revealing a strong antioxidant potential (Malik et al., 2023). In addition to the studies above, Wang prepared an animal model of alcoholic fatty liver disease and found that agarwood extract could effectively reduce the expression levels of alanine aminotransferase (ALT), aspartate aminotransferase (AST), triglyceride (TG), and cholesterol (CHO), lower blood lipid levels, and improve liver function through its antioxidant effects, alleviating the damage to the liver caused by a high-fat diet and alcohol consumption (Wang et al., 2023). Through in vivo studies on the effects of agarwood extracts on myocardial ischemia, Wang discovered that agarwood extracts could upregulate and activate the Nrf2-ARE pathway, exhibiting significant antioxidant effects, thereby playing a protective role against myocardial infarction (Wang et al., 2020) (Table 4).
5.3 Antibacterial effect
The antibacterial activity of agarwood is beneficial in combating bacterial and fungal infections. An increasing body of research in vitro has demonstrated that agarwood essential oil exhibits significant inhibitory effects against Staphylococcus aureus and Bacillus subtilis (Chen et al., 2011). Dahham isolated β-caryophyllene from agarwood essential oil and investigated its antimicrobial and antioxidant properties. In vitro, experimental results indicated that β-caryophyllene present in agarwood has notable antibacterial effects against Bacillus subtilis, Staphylococcus aureus, Escherichia coli, Klebsiella pneumoniae, and Pseudomonas aeruginosa. Moreover, its antifungal activity against Trichoderma reesei is superior to that of kanamycin (Dahham et al., 2015). Hu compared the antimicrobial activity of smoke produced by burning both Qi-Nan agarwood and ordinary agarwood (induced by the knife-cutting method), revealing that both demonstrated potent antimicrobial activity against Escherichia coli, Pseudomonas aeruginosa, and Staphylococcus aureus, with Qi-Nan agarwood showing greater efficacy than ordinary agarwood (Hu et al., 2023). Nurminah’s research suggested that agarwood distillate water extracts also possess inhibitory effects on Streptococcus mutans in vitro (Nurminah et al., 2021) (Table 5).
5.4 Anxiolytic and antidepressant effect
Aromatherapy has long been utilized to relax the mind and body and relieve stress. Agarwood essential oil, known for its unique aromatic scent and practical components, is commonly used in the treatment of insomnia, anxiety, and depression, offering distinct advantages in the treatment of psychiatric disorders. Agarwood emits a distinctive fragrance when heated, and inhaling the smoke generated can help with sleep induction and improve sleep disorders (Wang C. et al., 2022). Agarwood essential oil is also calming by regulating the expression of GABAA receptors and enhancing receptor function (Wang et al., 2017). Through experiments such as the light-dark box test and the tail suspension test, Wang found that agarwood essential oil may exert anxiolytic and antidepressant effects by inhibiting the release of corticotropin-releasing factor and the activation of the hypothalamic-pituitary-adrenal (HPA) axis, with effects similar to those of diazepam (2.5 mg/kg) (Wang S. et al., 2018). Li, through preparing a mixed essential volatile oil of Aquilaria sinensis (Lour.) Gilg and Aucklandia costus Falc, arrived at conclusions consistent with those of Wang’s research (Li H. et al., 2021). Using network pharmacology combined with solid-phase microextraction/gas chromatography-time of flight mass spectrometry, Pang discovered that sesquiterpenes in agarwood can exert anxiolytic effects by acting on multiple targets and signaling pathways (Pang et al., 2024). Han, through the injection of scopolamine to induce a model of learning and memory impairments, demonstrated significant alleviation of symptoms after inhalation of agarwood smoke (Han et al., 2021) (Table 6).
5.5 Anti-tumor effect
Agarwood’s anti-tumor effects are of interest in oncology, as it may inhibit tumor growth and induce apoptosis in cancer cells. Studies have indicated that oral administration of agarwood essential oil at doses of 100 mg/kg and 500 mg/kg for 28 consecutive days had no significant impact on the vital signs of mice. However, it exhibited inhibitory effects on the growth of colon cancer cells (Dahham et al., 2016). As its active constituents, the sesquiterpenes and chromone compounds in agarwood have also been extensively studied for their cytotoxicity. A new sesquiterpene compound was isolated from Aquilaria sinensis, which exhibited strong sensitivity to breast cancer cells. The relevant mechanism may be promoting the expression of reactive oxygen species (ROS) in cells, which promotes cancer cell apoptosis. This compound could potentially become a promising anticancer agent (Chen L. et al., 2022). Utilizing LC-MS-guided fractionation, Chen obtained five new derivatives of 2-(2-phenylethyl) chromone, which significantly inhibited the growth of five types of human cancer cells (IC50 13.40–28.96 μM), with cisplatin serving as a positive control (IC50 3.08–11.29 μM) (Chen et al., 2023). Similar findings were observed in cytotoxicity studies of 2-(2-phenylethyl) chromone derivatives isolated from agarwood (Xia et al., 2019). β-Caryophyllene isolated from the essential oil of Aquilaria crassna effectively inhibited the proliferation, migration, invasion, and spheroid formation of colorectal cancer cells (Dahham et al., 2015) (Table 7).
5.6 Anti-diabetic effect
As a key enzyme in glucose metabolism, α-glucosidase has the dual role of hydrolysis and transglycosidation in the catalytic reaction of sugar. Inhibition of α-glucosidase can effectively improve the efficiency of glucose absorption in the intestine, ameliorate postprandial blood glucose levels, and exert effects in delaying the progression of diabetes. The active component of agarwood, 2-(2-phenylethyl)chromone derivatives, possesses significant inhibitory effects on α-glucosidase (IC50 7.8 ± 0.3–137.7 ± 3.0 μM), which is more potent than acarbose (IC50 743.4 ± 3.3 μM) (Mi et al., 2021). Sesquiterpenes in agarwood also exhibit inhibitory effects on α-glucosidase, with effects superior to acarbose (Mi et al., 2019; Yang et al., 2019). Furthermore, phenethyl chromone compounds from agarwood may improve diabetes by modulating adiponectin secretion (Ahn et al., 2019) (Table 8).
5.7 Acetylcholinesterase inhibitory effect
Acetylcholinesterase (AChE) is responsible for the breakdown of acetylcholine at synaptic junctions, terminating the excitatory action of the neurotransmitter on the postsynaptic membrane. The deficiency of acetylcholine is a primary cause of Alzheimer’s disease, and the inhibition of AChE can slow the progression of the condition. The compound 5,6,7,8-Tetrahydro-2-(2-phenylethyl)chromones, isolated from agarwood, has been found to exhibit potent inhibitory effects against AChE, with an inhibition rate of up to 47.9% (with tacrine as a positive control, showing an inhibition rate of 66.7%) (Liao et al., 2017). Epoxy-5,6,7,8-tetrahydro-2-(2-phenylethyl) chromones, isolated from agarwood by Li, also demonstrated significant inhibitory activity, with an AChE inhibition rate of 47.9% ± 0.3% at a concentration of 50 μg/mL (Li et al., 2019). In contrast, 2-(2-phenylethyl) chromone derivatives isolated by Chen, Yang, and others from agarwood exhibited weaker inhibitory activity, with inhibition rates ranging from 12.0% to 15.7% (Yang et al., 2017; Yang et al., 2014) (Table 9).
5.8 Other pharmacological effects
In addition to the aforementioned pharmacological effects, agarwood possesses various other pharmacological properties, including immune modulation, neuroprotection, and gastrointestinal protection.
The agarwood epoxy compound 2-(2-phenylethyl) chromone derivative (GYF-21) can inhibit both innate and adaptive immune responses by suppressing the STAT1/3 and NF-κB signaling pathways, making it a potential candidate for the treatment of multiple sclerosis (Guo et al., 2017). GYF-21 can also inhibit B cells’ activation, proliferation, and differentiation functions by inhibiting the BAFF signaling pathway, offering potential therapeutic effects for systemic lupus erythematosus (Guo et al., 2019). Furthermore, the sesquiterpene derivative from agarwood (HHX-5) can regulate immune responses by inhibiting macrophage activation, CD4+ T cell differentiation, and B cell activation by suppressing the STAT pathway (Zhu et al., 2016b). Also, the hybrid of agarwood 2-(2-phenylethyl)chromone-sesquiterpene can protect gastric mucosal cells by downregulating endoplasmic reticulum stress induced by taurocholic acid (Zhang et al., 2023). The dimer of sesquiterpenes from agarwood wood also exhibits antimalarial effects (IC50 21.67 ± 1.25 nM), with artemisinin serving as a positive control (IC50 559.4 ± 66.55 nM) (Huang X. L. et al., 2023). Agarwood also has therapeutic effects in treating cardiovascular diseases, where 2-(2-phenylethyl) chromone derivatives can mitigate atherosclerosis by reducing endoplasmic reticulum stress-mediated macrophage CD36 expression and inhibiting foam cell formation (Ma et al., 2024).
6 Methods of agarwood tree leaves extraction
Agarwood tree leaves have a wide range of pharmacological effects. However, the extraction process of agarwood tree leaves is not the focus of this article, so only a brief introduction to the relevant extraction processes is provided below. Wang extracted the essential oil from agarwood tree leaves using steam distillation, supercritical CO2 extraction, and enzymatic treatment combined with supercritical extraction. The essential oil yields from these three processes were 0.065%, 0.142%, and 0.425%, respectively (Wang et al., 2014). Li used the leaves of “Qi-Nan” agarwood as raw material and ethanol as the solvent, employing an ultrasonic-assisted method to extract flavonoids. The study found that a material-liquid ratio of 1:50, an ethanol concentration of 60%, and an ultrasonic extraction time of 30 min were the ideal conditions for extracting flavonoids. At this time, the content of flavonoids in the extract was 6.68%. The flavonoids had strong antioxidant capacity, with DPPH and ABTS radical scavenging IC50 values of 12.64 μg/mL and 66.58 μg/mL, respectively (Li et al., 2024). Zhang conducted comparative experiments on the anti-inflammatory activity of agarwood tree leaves extracts using different solvents (water, diethyl ether, and normal butanol). It was found that the normal butanol extract had the most potent anti-inflammatory activity. After resin filtration and separation of the normal butanol extract, HPLC detection revealed the highest content of mangiferin, suggesting that mangiferin could serve as a quality control standard for agarwood tree leaf extracts (Zhang, 2014). Using an ultrasonic extraction method, Liu explored the optimal extraction process for mangiferin from agarwood tree leaves. The results indicated that an extraction time of 7 min, microwave ultrasonic power of 408 W, and a material-liquid ratio of 1:44 maximized the extraction rate of mangiferin, which could reach up to 3.229% (Liu M. et al., 2020) (Table 10).
7 Pharmacological effects of agarwood tree leaves
Agarwood is renowned for its diverse pharmacological activities, and agarwood tree leaves, rich in bioactive compounds such as 2-(2-phenylethyl)chromones, flavonoids, phenolic acids, flavones, and terpenoids, have demonstrated significant anti-inflammatory, antioxidant, immunomodulatory, antibacterial, and antidiabetic effects (Tay et al., 2014; Adam et al., 2017). Studies have shown that agarwood leaf extracts can markedly alleviate ear edema induced by dimethyl benzene, paw edema caused by carrageenan, and leukocyte migration triggered by CMC-Na, exhibiting pronounced anti-inflammatory and analgesic properties (Zhou et al., 2008). Furthermore, agarwood leaf extracts have been found to reduce NO production in macrophages stimulated by LPS, with flavonoid compounds likely being the primary active constituents (Yang et al., 2012; Qi et al., 2009). Research by Pang et al. (2023) has further revealed that agarwood leaf extracts can effectively modulate the immune response mediated by macrophages by regulating the expression levels of TNF-α, IL-6, COX2, and IL-10, as well as by decreasing the expression of iNOS. Regarding antibacterial activity, Hendra’s research has discovered that agarwood tree leaves possess inhibitory effects against Staphylococcus aureus and Escherichia coli, with mature leaves showing superior antibacterial effects compared to young leaves (Hendra et al., 2016a). The mechanism of action may be related to the disruption of bacterial cell wall synthesis and the inhibition of biofilm formation (Kamonwannasit et al., 2013). Agarwood leaf extracts also exhibit α-glucosidase inhibitory activity, thereby reducing blood glucose levels, akin to the effects of agarwood itself (Yang et al., 2024; Feng et al., 2011). Extracts from Aquilaria crassna leaves can also counteract the neurotoxicity and aging effects of hyperglycemia, with stigmasterol and β-sitosterol being potential active components (Pattarachotanant et al., 2022). Besides, the active component Clionasterol in Aquilaria crassna leaves has been shown to protect neuronal cells and Caenorhabditis elegans from toxic damage (Pattarachotanant et al., 2023). Regarding the safety of agarwood leaf extracts, studies have indicated that no significant toxic reactions or deaths were observed in mice administered a dose of 2000 mg/kg for 14 consecutive days, and no abnormalities were found in pathological examinations (Kamonwannasit et al., 2013). Nevertheless, further research is warranted to confirm the safety profile of agarwood leaf extracts (Table 11; Figures 2).
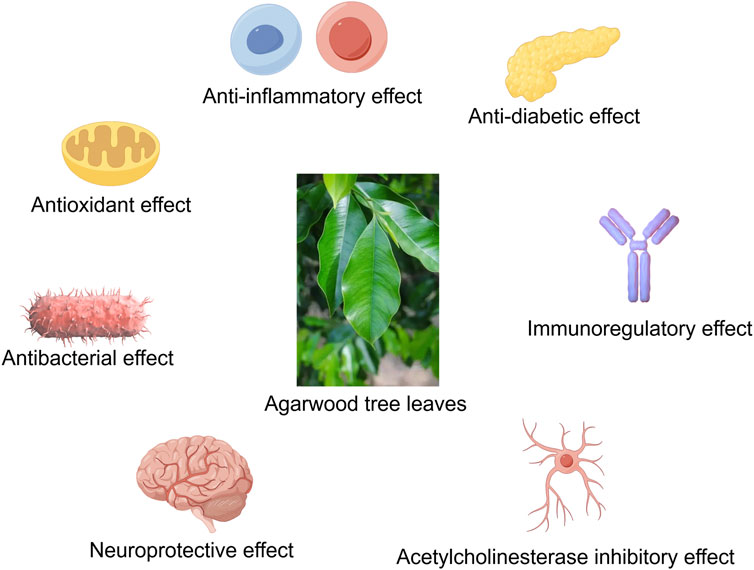
Figure 2. The agarwood tree leaves have anti-inflammatory, antioxidant, antibacterial, neuroprotective, anti-diabetic, immune regulation, acetylcholinesterase inhibition and other effects (By Figdraw).
8 Research on the correlation between agarwood and periodontitis
Although dental plaque is the initiating factor of periodontitis, the host’s immune response is crucial in destroying periodontal tissues. Macrophages, CD4+ T cells, and B cells mediate both Innate and adaptive immune responses, and signaling pathways such as receptor activator of NF-κB ligand (RANKL) and STAT 1, along with inflammatory factors like TNF-α, IL-1β, IL-6, PGE2, play significant roles in the progression of periodontal inflammation. Chronic inflammation can lead to irreversible tissue damage. As mentioned above, agarwood possesses a wide range of pharmacological effects, including potent anti-inflammatory, antioxidant, and immunomodulatory properties. Inflammation, oxidative stress, and immune imbalance are the primary pathological responses in periodontitis, suggesting that agarwood holds great potential in treating periodontal disease. Concurrently, our preliminary experimental research has found that agarwood extracts can effectively reduce attachment loss in rats with periodontitis and improve the biological parameters of alveolar bone Figures 3.
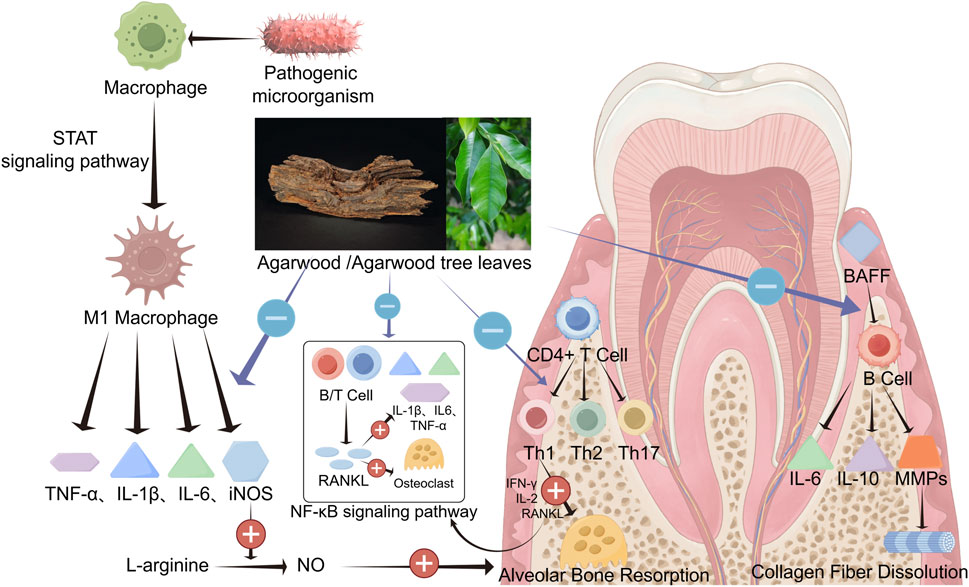
Figure 3. Agarwood and agarwood tree leaves own a strong role and broad prospects in the treatment of periodontitis. The various active substances in agarwood can inhibit or downregulate inflammation levels in periodontal tissue, reduce immune cell differentiation and activation, prevent periodontal tissue destruction, and inhibit the progression of periodontal inflammation by suppressing signaling pathways such as NF-κB and STAT (By Figdraw).
8.1 Immune cells
Macrophages play a crucial role in maintaining homeostasis and defense in periodontal tissues. However, their excessive aggregation and activation can damage periodontal tissue. Under the stimulation of pathogenic microorganisms and inflammatory cells, macrophages can polarize into M1 type macrophages through the STAT 1 signaling pathway (Han et al., 2020; Wang W. et al., 2021), and secrete inflammatory factors such as PGE2, TNF-α, IL-1β, IL-6, and iNOS. PGE2 is the most potent cytokine in promoting periodontal bone destruction, which can mediate destructive processes of alveolar bone and cementum by downregulating the mineralization ability of osteoblasts and promoting osteoclast formation (Oka et al., 2007). TNF-α, IL-1β, and IL-6 primarily stimulate the chemotaxis and migration of inflammatory cells to periodontal tissues, enhance the expression of neutrophil adhesion factors on the vascular surface, and augment the synthesis of other pro-inflammatory cytokines. They also exert destructive effects on bone and connective tissues by promoting the expression of MMPs and RANKL (Cekici et al., 2014). iNOS can catalyze NO production from L-arginine until the substrate is depleted (Fretwurst et al., 2020). Low NO concentrations within the periodontal tissue are beneficial for exerting anti-inflammatory effects. In contrast, high NO concentrations can exacerbate inflammatory responses caused by bacteria and endotoxins, accelerating the destruction of alveolar bone (Sun S. et al., 2020). Zhu investigated the anti-inflammatory activity of agarwood 2-(2-phenylethyl)chromone derivative (GYF-17) against LPS-induced RAW264.7 cells and its potential mechanisms, and the results showed that GYF-17 could reduce the expression of iNOS induced by LPS and downregulate the release of NO. In addition, GYF-17 significantly inhibited the expression and secretion of vital pro-inflammatory factors such as IL-1β, IL-6, PGE2, and TNF-α (Zhu et al., 2016a). Wang’s research indicates that agarwood extract can effectively reduce the expression levels of inflammatory cytokines such as TNF-α and IL-6 (Wang et al., 2023).
In states of inflammation, CD4+ T cells in the periodontal tissue can proliferate and differentiate into various subtypes of Th cells, such as Th1, Th2, and Th17. Th1 cells, characterized by their role in cell-mediated immunity, can lead to alveolar bone resorption and promote the progression of periodontal inflammation by releasing IFN-γ, IL-2, and RANKL. Th17 cells produce vital cytokines such as IL-17, which plays a positive role in bone remodeling and homeostasis (Yu et al., 2007). However, excessive IL-17 production can exacerbate periodontal tissue destruction by upregulating the expression of chemokine ligands (CXCL1, CXCL2, and CXCL5), IL-1β, MMPs, granulocyte colony-stimulating factors (G-CSF) and granulocyte-macrophage colony-stimulating factors (GM-CSF) (Veldhoen, 2017). The upregulation of G-CSF and GM-CSF increases tissue permeability, mediating chemotaxis and activation in periodontal tissues, thereby exacerbating damage through phagocytosis and cellular immunity.
In periodontitis patients, the proportion of B cells in the lesion tissue correlates positively with the severity of periodontitis (Reinhardt et al., 1988). Under the stimulation of pathogenic microorganisms, B cells perform antigen presentation to trigger immune responses in CD4+ T cells and differentiate themselves into plasma cells that secrete immunoglobulins to neutralize antigens. B cells can also produce inflammatory cytokines such as TGF-β, TNF-α, IL-10, and IL-6 to modulate inflammatory responses and generate matrix metalloproteinases (MMPs), which are crucial in physiological and pathological processes. Collagenases like MMP-1 and MMP-8 can dissolve types I, II, and III collagen fibers, leading to collagen dissolution and periodontal connective tissue destruction (Zou et al., 2022; Nędzi-Góra et al., 2021; Luchian et al., 2022). B cell activating factor (BAFF) plays a critical regulatory role, enhancing B cell survival, proliferation, and antigen presentation by binding to receptors, including BAFFR, and promoting plasma cell differentiation. As mentioned earlier, agarwood possesses significant immunomodulatory functions, and its active components could potentially serve as a source of immunosuppressive agents. The sesquiterpene derivative from agarwood (HHX-5) can effectively inhibit the activation, proliferation, and differentiation of CD4+ and CD8+ T cells, thereby suppressing cell-mediated immune responses (Zhu et al., 2016b). Furthermore, the 2-(2-phenylethyl) chromone derivative from agarwood (GYF-21) can inhibit B cells’ activation, proliferation, and differentiation by blocking the signaling pathway activated by BAFF (Guo et al., 2019).
8.2 Signaling pathways
8.2.1 NF-κB signaling pathway
The NF-κB signaling pathway has long been recognized as an essential and common pro-inflammatory signaling pathway. Within this pathway, RANKL is a crucial activator. Under its stimulation, NF-κB-induced mitogen-activated protein kinase is activated, phosphorylating protein kinase NIK and its downstream IKKα, which leads to the phosphorylation and degradation of NF-κB p100, forming dimers such as RelB/p52. These components enter the nucleus to regulate the transcription of target genes, causing cells to produce corresponding inflammatory and stress responses (Sun, 2011). RANKL is also a major regulatory factor for the differentiation and function of osteoclasts, with its primary function being the activation of osteoclasts and the upregulation of the expression of pro-inflammatory cytokines TNF-α, IL-6, and IL-1β. Periodontal tissues, including the gingiva, alveolar bone, cementum, and periodontal ligament, all of which can express RANKL (Usui et al., 2021). Under inflammatory conditions, cells of the periodontal tissues, B lymphocytes, and activated T cells can release large amounts of RANKL and pro-inflammatory factors. The aforementioned inflammatory pathways and mechanisms promote the development of inflammation within the periodontal tissues, upregulate the production of osteoclasts, and subsequently cause the resorption of alveolar bone (Fischer and Haffner-Luntzer, 2022). Inhibition of RANKL release would be beneficial for the protection of periodontal tissues. Studies have indicated that pretreatment with ethanol extracts of agarwood can suppress the activation of RANKL and reduce the expression of TNF-α, IL-6, and IL-1β (Wang C. et al., 2021). GYF-21 has been shown to exert significant anti-inflammatory effects by inhibiting the NF-κB signaling pathway (Guo et al., 2019), which suggests that agarwood may inhibit the progression of periodontal inflammation by suppressing the RANKL pathway.
8.2.2 STAT signaling pathway
In inflammatory cytokine and signaling molecule production, the STAT is a primary regulatory component. STAT 1, as a crucial regulatory factor in inflammatory responses, is involved in processes that inhibit cell proliferation and differentiation. Under inflammatory stimuli, the expression level of STAT 1 in periodontal ligament cells significantly increases compared to the control group. High levels of STAT 1 have a marked inhibitory effect on the osteogenic differentiation capability of periodontal ligament cells, disrupting the osteogenic-osteoclastic balance and leading to the destruction and resorption of supportive tissues such as alveolar bone (Lin et al., 2023; Kim et al., 2003). Additionally, the osteogenic gene Runt-related transcription factor 2 (Runx2) can promote osteoblast differentiation. Meanwhile, STAT 1, acting as a negative regulator of Runx2, can inhibit Runx2 from entering the nucleus, thereby preventing the expression of osteogenic differentiation genes (Kim et al., 2003). Beyond its negative impact on osteogenesis, STAT1 also promotes the polarization of macrophages to the M1 phenotype. LPS-induced activation of Toll-like receptor 4 (TLR4) via the JAK2/STAT1 pathway is critical for M1 polarization. LPS induces interferon-β, which facilitates the formation of STAT1-STAT2 heterodimers, leading to the assembly of the IFN-stimulated gene factor 3 complex and regulation of M1-associated gene expression (Li et al., 2018). The presence of numerous M1 macrophages in periodontal tissues can result in inflammatory responses characterized by cytotoxicity and tissue damage.
Apart from STAT1, STAT3 also plays a significant role in the progression of periodontitis. STAT3, an integral component of the JAK/STAT signaling pathway, is highly phosphorylated in inflammatory tissues and crucial for regulating the expression of cytokines, chemokines, and other mediators that induce and sustain an inflammatory environment (Garama et al., 2015). Research by Song indicates that the knockdown of STAT-3 suppresses IL-1β-induced expression of MMP-1 and MMP-3 (Song et al., 2021). STAT3 also acts as a sensor for various metabolic stressors, including ROS. Phosphorylated STAT3 translocates from the cytoplasm to the nucleus, where it functions as a transcription factor, inducing the expression of downstream oxidative stress and inflammatory factors, thereby causing further tissue damage (Lu et al., 2019). Studies suggest that inhibiting this signaling can control the expression of inflammatory factors and ROS (Li et al., 2020).
In summary, STAT proteins in periodontal tissues can exert destructive effects by inhibiting periodontal osteogenesis, promoting macrophage polarization, pro-inflammatory actions, and oxidative stress. Research has indicated that HHX-5 can significantly inhibit the activation of the STAT 1 signaling pathway in macrophages and CD4+ T cells under inflammatory stimuli (Zhu et al., 2016b), and GYF-17 can suppress the progression of inflammation by inhibiting the STAT 1/3 and ERK 1/2 pathways, suggesting that agarwood may act through the STAT 1 pathway to inhibit periodontitis (Zhu et al., 2016a).
9 Research progress of agarwood essential oils nanoemulsions
Plant essential oils are a common form of plant extracts, primarily composed of hydrocarbons such as monoterpenes and sesquiterpenes, as well as aromatic compounds like alcohols, aldehydes, and ketones (Tongnuanchan and Benjakul, 2014). Numerous studies have demonstrated the broad pharmacological effects of plant essential oils, including antioxidant, anti-inflammatory, and analgesic properties (Silva et al., 2015). However, after ingestion, plant essential oils rapidly undergo glucuronidation and are metabolized and eliminated by the urinary or respiratory systems. Their short half-life limits the promotion of their clinical applications (Kohlert et al., 2000).
As an advanced drug delivery system, nanoemulsion has been proven to enhance solubility or bioavailability effectively. The tiny droplets in nanoemulsion, typically with diameters ranging from 10 to 100 nm, can increase the total surface area, thereby improving the absorption efficiency of drugs (Singh et al., 2017). By encapsulating drugs in oily or aqueous materials according to the properties and intended use of the drug, their stability can be enhanced, preventing premature or rapid degradation in the body, thus achieving the goal of increasing drug bioavailability (Deshmukh et al., 2021). Research by Liang et al. has shown that cinnamon essential oil nanoemulsions remain undigested in the mouth, with the digestion process mainly occurring in the stomach and small intestine, demonstrating the potential of nanoemulsion to effectively enhance drug bioavailability (Liang et al., 2022).
As previously mentioned, agarwood possesses a broad range of pharmacological effects, including potent anti-inflammatory, antioxidant, antitumor, antimicrobial, and hypoglycemic properties. However, the bioactive components of agarwood are primarily present in the form of essential oils, which results in poor solubility and low bioavailability, limiting its clinical applications. Advanced drug delivery systems can effectively address this issue, and among them, nanoemulsions have emerged as a preferred solution due to their simple preparation process, strong stability, and good biocompatibility (Jamir et al., 2024; Shetta et al., 2024).
Currently, no research compares Agarwood nanoemulsion’s bioavailability and stability with traditional Agarwood essential oils. Research on agarwood nanoemulsions has primarily focused on respiratory system diseases, with no reports found related to the oral field. Malik’s research revealed that agarwood nanoemulsions effectively inhibited the expression of inflammatory factors TNF-α, IL-1β, IL-6, and iNOS while also reducing the secretion of ROS and NO levels, demonstrating anti-inflammatory solid and antioxidant effects (Malik et al., 2023). Alamil found that agarwood essential oil nanoemulsions could downregulate the expression of IL-6 and IL-8 in bronchial epithelial cells stimulated by inflammatory mediators (Alamil et al., 2023). Rubis’s research indicated that agarwood essential oil nanoemulsions possess anti-inflammatory and antioxidant properties, capable of inducing the expression of antioxidant genes such as GCLC and GSTP1 and downregulating the expression of pro-inflammatory factors like IL-8, IL-1α, IL-1β, as well as upregulating the expression of anti-inflammatory mediators IFN-γ, IL-10, IL-18BP, and GH, suggesting their significant potential in treating chronic obstructive pulmonary disease (COPD) (De Rubis et al., 2023). In summary, it is suggested that Agarwood nanoemulsion also possesses significant anti-inflammatory and antioxidant effects in the oral field. Moreover, because nanoemulsion technology can mprove drug stability and bioavailability, Agarwood nanoemulsion may be more effective than traditional Agarwood essential oils, indicating a broad application prospect.
10 Conclusion and prospects
Agarwood has long captivated people due to its rich bioactive components, extensive pharmacological effects, and unique aromatic scent. However, the high demand for agarwood, its long production time, low yield, and inconsistent quality have led to a persistent shortage and high prices. Currently, induction methods for agarwood formation are categorized into natural formation and traditional induction, biological induction, and chemical induction. Among these, chemical induction stands out as the most ideal and reliable alternative to natural agarwood formation because it can stably induce the formation of high-quality agarwood and effectively addresses the issue of poor repeatability in the quality of artificially induced agarwood. However, due to the limited research conducted thus far, extensive experimentation is still required to verify the safety and feasibility of chemical induction before it can be widely adopted. Regarding the distillation of agarwood essential oil, supercritical fluid extraction (SFE) and subcritical water extraction (SCWE) are currently preferred methods. These techniques offer several advantages, including high extraction efficiency, stable oil quality, and a rich content of active components. As research in this field continues to advance, it is expected that more efficient and environmentally friendly methods for agarwood production and essential oil extraction will be developed, further expanding the applications and availability of this precious resource.
Agarwood is rich in sesquiterpenes, 2-(2-phenylethyl) chromone derivatives, aromatic compounds, and fatty acids, which endow it with a broad spectrum of pharmacological effects, such as anti-inflammatory, antioxidant, immunomodulatory, hypoglycemic, and antitumor properties. Since inflammatory responses, oxidative stress, and immune dysregulation are the primary pathological mechanisms of periodontitis, agarwood essential oil suggests significant potential in treating periodontal disease. However, despite being abundant in bioactive components of agarwood, essential oils suffer from drawbacks such as low solubility and poor bioavailability, which limit their clinical applications. Therefore, improving the active form is crucial. Nano-encapsulated drug delivery systems are an effective measure to address these issues. Existing researchs have demonstrated that agarwood nanoemulsion possesses potent anti-inflammatory and antioxidant effects. Perhaps using agarwood nanoemulsions to treat periodontitis is a feasible strategy. However, more in vivo and in vitro experiments are needed to substantiate the feasibility of the experiments, drug dosage, and potential toxicity issues.
The research focus on agarwood is predominantly centered on the pharmacological activities of its resin components and their applications in clinical medicine. In comparison, research on the leaves of agarwood trees has been relatively scarce. However, existing research findings indicate that agarwood tree leaves are rich in pharmacologically active constituents. They hold potential therapeutic value and present unique advantages in resource utilization and medicinal research due to their lower economic cost and relatively more straightforward acquisition. Therefore, agarwood tree leaves have the potential to emerge as a new hotspot in future research. The findings in this area are expected to bring innovative breakthroughs to the pharmaceutical and health industries.
Author contributions
CX: Conceptualization, Writing–original draft. J-ZD: Conceptualization, Writing–original draft. B-SL: Project administration, Writing–original draft. P-YY: Visualization, Writing–original draft. Y-SZ: Writing–original draft, Visualization. X-YD: Visualization, Writing–original draft. C-EL: Project administration, Writing–original draft. XZ: Funding acquisition, Writing–review and editing.
Funding
The author(s) declare that financial support was received for the research, authorship, and/or publication of this article. The paper has received funding support from the Hainan Province Science and Technology Special Fund (ZDYF2021SHFZ113), Health Science Research Project of Hainan Province (22A200040), Hainan Medical University Graduate Student Innovation Project (Qhys2023-494) and Student Innovation and Entrepreneurship Training Program of Hainan Medical University (S202411810022).
Acknowledgments
The authors would like to thank the reviewers and authors of all references.
Conflict of interest
The authors declare that the research was conducted in the absence of any commercial or financial relationships that could be construed as a potential conflict of interest.
Publisher’s note
All claims expressed in this article are solely those of the authors and do not necessarily represent those of their affiliated organizations, or those of the publisher, the editors and the reviewers. Any product that may be evaluated in this article, or claim that may be made by its manufacturer, is not guaranteed or endorsed by the publisher.
References
Adam, A. Z., Lee, S. Y., and Mohamed, R. (2017). Pharmacological properties of agarwood tea derived from Aquilaria (Thymelaeaceae) leaves: an emerging contemporary herbal drink. J. Herb. Med. 10, 37–44. doi:10.1016/j.hermed.2017.06.002
Ahn, S., Ma, C. T., Choi, J. M., An, S., Lee, M., Le, T. H. V., et al. (2019). Adiponectin-secretion-promoting phenylethylchromones from the agarwood of Aquilaria malaccensis. J. Nat. Prod. 82, 259–264. doi:10.1021/acs.jnatprod.8b00635
Alamil, J. M. R., Paudel, K. R., Chan, Y., Xenaki, D., Panneerselvam, J., Singh, S. K., et al. (2022). Rediscovering the therapeutic potential of agarwood in the management of chronic inflammatory diseases. Molecules 27, 3038. doi:10.3390/molecules27093038
Alamil, J. M. R., Xenaki, D., Manandhar, B., Paudel, K. R., Hansbro, P. M., Oliver, B. G., et al. (2023). Agarwood oil nanoemulsion attenuates production of lipopolysaccharide (LPS)-induced proinflammatory cytokines, IL-6 and IL-8 in human bronchial epithelial cells. EXCLI J. 22, 681–685. doi:10.17179/excli2023-6282
Azren, P. D., Lee, S. Y., Emang, D., and Mohamed, R. (2018). History and perspectives of induction technology for agarwood production from cultivated Aquilaria in Asia: a review. J. For. Res. 30, 1–11. doi:10.1007/s11676-018-0627-4
Bahrani, H., Mohamad, J., Paydar, M. J., and Rothan, H. A. (2014). Isolation and characterisation of acetylcholinesterase inhibitors from Aquilaria subintegra for the treatment of Alzheimer's disease (AD). Curr. Alzheimer Res. 11, 206–214. doi:10.2174/1567205011666140130151344
Cai, Z., Du, S., Zhao, N., Huang, N., Yang, K., and Qi, L. (2024). Periodontitis promotes the progression of diabetes mellitus by enhancing autophagy. Heliyon 10, e24366. doi:10.1016/j.heliyon.2024.e24366
Castro, K. P., and Ito, M. (2021). Individual and combined inhalational sedative effects in mice of low molecular weight aromatic compounds found in agarwood aroma. Molecules 26, 1320. doi:10.3390/molecules26051320
Cekici, A., Kantarci, A., Hasturk, H., and Van Dyke, T. E. (2014). Inflammatory and immune pathways in the pathogenesis of periodontal disease. Periodontol. 2000 64, 57–80. doi:10.1111/prd.12002
Chang, P. C., Chien, L. Y., Yeo, J. F., Wang, Y. P., Chung, M. C., Chong, L. Y., et al. (2013). Progression of periodontal destruction and the roles of advanced glycation end products in experimental diabetes. J. Periodontol. 84, 379–388. doi:10.1902/jop.2012.120076
Chapple, I. L., and Genco, R.working group 2 of the joint EFP/AAP workshop (2013). Diabetes and periodontal diseases: consensus report of the joint EFP/AAP workshop on periodontitis and systemic diseases. J. Clin. Periodontol. 40 (Suppl. 14), S106–S112. doi:10.1902/jop.2013.1340011
Chen, H., Yang, Y., Xue, J., Wei, J., Zhang, Z., and Chen, H. (2011). Comparison of compositions and antimicrobial activities of essential oils from chemically stimulated agarwood, wild agarwood and healthy Aquilaria sinensis (Lour.) gilg trees. Molecules 16, 4884–4896. doi:10.3390/molecules16064884
Chen, H. Q., Wei, J. H., Yang, J. S., Zhang, Z., Yang, Y., Gao, Z. H., et al. (2012). Chemical constituents of agarwood originating from the endemic genus Aquilaria plants. Chem. Biodivers. 9, 236–250. doi:10.1002/cbdv.201100077
Chen, L., Chen, H., Cai, C., Yuan, J., Gai, C., Liu, S., et al. (2023). Seven new 2-(2-phenethyl)chromone derivatives from agarwood of Aquilaria walla. Fitoterapia 165, 105421. doi:10.1016/j.fitote.2022.105421
Chen, L., Liu, Y., Li, Y., Yin, W., and Cheng, Y. (2022a). Anti-cancer effect of sesquiterpene and triterpenoids from agarwood of Aquilaria sinensis. Molecules 27, 5350. doi:10.3390/molecules27165350
Chen, X., Sui, C., Liu, Y., Yang, Y., Liu, P., Zhang, Z., et al. (2017). Agarwood Formation induced by fermentation liquid of Lasiodiplodia theobromae, the dominating fungus in wounded wood of Aquilaria sinensis. Curr. Microbiol. 74, 460–468. doi:10.1007/s00284-016-1193-7
Chen, X., Wang, C., Feng, J., Chen, D., Wei, J., and Liu, Y. (2022b). Comparative analysis of chemical constituents and anti-oxidant and antiinflammatory activities of six representative agarwood essential oils. Chin. Traditional Herb. Drugs 53, 5720–5730.
Dahham, S. S., Hassan, L. E. A., Ahamed, M. B. K., Majid, A. S. A., Majid, A. M. S. A., and Zulkepli, N. N. (2016). In vivo toxicity and antitumor activity of essential oils extract from agarwood (Aquilaria crassna). BMC Complementary Altern. Med. 16, 236. doi:10.1186/s12906-016-1210-1
Dahham, S. S., Tabana, Y. M., Iqbal, M. A., Ahamed, M. B., Ezzat, M. O., Majid, A. S., et al. (2015). The anticancer, antioxidant and antimicrobial properties of the sesquiterpene β-caryophyllene from the essential oil of Aquilaria crassna. Molecules 20, 11808–11829. doi:10.3390/molecules200711808
De Rubis, G., Paudel, K. R., Manandhar, B., Singh, S. K., Gupta, G., Malik, R., et al. (2023). Agarwood oil nanoemulsion attenuates cigarette smoke-induced inflammation and oxidative stress markers in BCi-ns1.1 airway epithelial cells. Nutrients 15, 1019. doi:10.3390/nu15041019
Deshmukh, R., Sharma, B., Harwansh, R. K., and Dewangan, B. (2021). Nanoemulsion: a snapshot on development, characterization, applications, patents and clinical trials. Internat. J. Pharm. Sci. Res. 12. doi:10.13040/IJPSR.0975-8232.12(4).1978-88
Eissa, M. A., Hashim, Y. Z. H., Abdul Azziz, S. S. S., Salleh, H. M., Isa, M. L. M., Abd Warif, N. M., et al. (2022). Phytochemical constituents of Aquilaria malaccensis leaf extract and their anti-inflammatory activity against LPS/IFN-γ-Stimulated RAW 264.7 cell line. ACS Omega 7, 15637–15646. doi:10.1021/acsomega.2c00439
Faizal, A., Esyanti, R. R., Adn'ain, N., Rahmani, S., Azar, A. W. P., Iriawati and Turjaman, M., et al. (2021). Methyl jasmonate and crude extracts of Fusarium solani elicit agarwood compounds in shoot culture of Aquilaria malaccensis Lamk. Heliyon 7, e06725. doi:10.1016/j.heliyon.2021.e06725
Feng, J., Yang, X. W., and Wang, R. F. (2011). Bio-assay guided isolation and identification of α-glucosidase inhibitors from the leaves of Aquilaria sinensis. Phytochemistry 72, 242–247. doi:10.1016/j.phytochem.2010.11.025
Fischer, V., and Haffner-Luntzer, M. (2022). Interaction between bone and immune cells: implications for postmenopausal osteoporosis. Semin. Cell Dev. Biol. 123, 14–21. doi:10.1016/j.semcdb.2021.05.014
Fretwurst, T., Garaicoa-Pazmino, C., Nelson, K., Giannobile, W. V., Squarize, C. H., Larsson, L., et al. (2020). Characterization of macrophages infiltrating peri-implantitis lesions. Clin. Oral Implants Res. 31, 274–281. doi:10.1111/clr.13568
Gao, X., Zhang, Q., Huo, H., Zhu, Z., Zhao, Y., Tu, P., et al. (2019). Anti-inflammatory effect of Chinese agarwood essential oil via inhibiting p-STAT3 and IL-1beta/IL-6. Chin. Pharm. J. 54, 1951–1957.
Garama, D. J., Harris, T. J., White, C. L., Rossello, F. J., Abdul-Hay, M., Gough, D. J., et al. (2015). A synthetic lethal interaction between glutathione synthesis and mitochondrial reactive oxygen species provides a tumor-specific vulnerability dependent on STAT3. Mol. Cell Biol. 35, 3646–3656. doi:10.1128/MCB.00541-15
Guo, R., Li, J., Gu, Y., Li, Y., Li, S., Gao, X., et al. (2019). GYF-21, an epoxide 2-(2-phenethyl)-chromone derivative, suppresses dysfunction of B cells mainly via inhibiting BAFF activated signaling pathways. Int. Immunopharmacol. 67, 473–482. doi:10.1016/j.intimp.2018.12.048
Guo, R., Zhao, Y. F., Li, J., Gu, Y. F., Huo, H. X., Li, S. S., et al. (2017). GYF-21, an epoxide 2-(2-phenethyl)-chromone derivative, suppresses innate and adaptive immunity via inhibiting STAT1/3 and NF-κB signaling pathways. Front. Pharmacol. 8, 281. doi:10.3389/fphar.2017.00281
Han, M., Zhang, H., Hu, M., Sun, W., Li, Z., Cao, G., et al. (2021). Inhalation administration of agarwood incense rescues scopolamine-induced learning and memory impairment in mice. Front. Pharmacol. 12, 821356. doi:10.3389/fphar.2021.821356
Han, S., Zhuang, H., Lee, P. Y., Li, M., Yang, L., Nigrovic, P. A., et al. (2020). Differential responsiveness of monocyte and macrophage subsets to interferon. Arthritis Rheumatol. 72, 100–113. doi:10.1002/art.41072
Hendra, H., Moeljopawiro, S., and Nuringtyas, T. R. (2016a). “Antioxidant and antibacterial activities of agarwood (Aquilaria malaccensis Lamk.) leaves,” in Advances Of Science And Technology For Society: Proceedings of the 1st International Conference on Science and Technology 2015, Yogyakarta, Indonesia, 11-13 November 2015.
Hendra, H., Moeljopawiro, S., and Nuringtyas, T. R. (2016b). Antioxidant and antibacterial activities of agarwood leaves. Adv. Sci. Technol. Soc.
Hu, B., Ling, S. J., Liu, X., Huang, J. B., Cui, H., and Zhao, Z. X. (2024). Two new 2-(2-phenylethyl)chromone derivatives and two sesquiterpenes from agarwood of Aquilaria sinensis with anti-inflammatory activity. Fitoterapia 173, 105824. doi:10.1016/j.fitote.2024.105824
Hu, Z., Chen, Y., Yan, T., and Li, G. (2023). Differences between qi-nan agarwood and ordinary agarwood in secretion properties and antibacterial activity. Sci. Silvae Sin. 59, 136–144.
Huang, M., Ma, S., Qiao, M., Fu, Y., and Li, Y. (2023a). Quality similarity between induced agarwood by fungus and wild agarwood. J. Agric. Food Chem. 71, 15620–15631. doi:10.1021/acs.jafc.3c04322
Huang, X. L., Cai, D., Gao, P., Wang, J. G., and Cheng, Y. X. (2023b). Aquilariperoxide A, a sesquiterpene dimer from agarwood of Aquilaria sinensis with dual antitumor and antimalarial effects. J. Org. Chem. 88, 8352–8359. doi:10.1021/acs.joc.3c00372
Huang, X. L., Zhou, Y. T., Yan, Y. M., and Cheng, Y. X. (2022). Sesquiterpenoid-chromone heterohybrids from agarwood of Aquilaria sinensis as potent specific Smad3 phosphorylation inhibitors. J. Org. Chem. 87, 7643–7648. doi:10.1021/acs.joc.2c00145
Ibrahim, A. H., Al-Rawi, S. S., Majid, A. M. S. A., Rahman, N. N. A., Salah, K. M. A., and Kadir, M. O. A. (2011). Separation and fractionation of Aquilaria malaccensis oil using supercritical fluid extraction and tThe cytotoxic properties of the extracted oil. Procedia Food Sci. 1, 1953–1959. doi:10.1016/j.profoo.2011.09.287
Ito, H., and Ito, M. (2022). Comparison of phenolic compounds contained in Aquilaria leaves of different species. J. Nat. Med. 76, 693–702. doi:10.1007/s11418-022-01608-3
Jamir, Y., Bhushan, M., Sanjukta, R., and Robindro Singh, L. (2024). Plant-based essential oil encapsulated in nanoemulsions and their enhanced therapeutic applications: an overview. Biotechnol. Bioeng. 121, 415–433. doi:10.1002/bit.28590
Jiao, J., Jing, W., Si, Y., Feng, X., Tai, B., Hu, D., et al. (2021). The prevalence and severity of periodontal disease in mainland China: data from the fourth national oral health survey (2015-2016). J. Clin. Periodontol. 48, 168–179. doi:10.1111/jcpe.13396
Kamonwannasit, S., Nantapong, N., Kumkrai, P., Luecha, P., Kupittayanant, S., and Chudapongse, N. (2013). Antibacterial activity of Aquilaria crassna leaf extract against Staphylococcus epidermidis by disruption of cell wall. Ann. Clin. Microbiol. Antimicrob. 12, 20. doi:10.1186/1476-0711-12-20
Kassebaum, N. J., Bernabe, E., Dahiya, M., Bhandari, B., Murray, C. J., and Marcenes, W. (2014). Global burden of severe periodontitis in 1990-2010: a systematic review and meta-regression. J. Dent. Res. 93, 1045–1053. doi:10.1177/0022034514552491
Kim, S., Koga, T., Isobe, M., Kern, B. E., Yokochi, T., Chin, Y. E., et al. (2003). Stat1 functions as a cytoplasmic attenuator of Runx2 in the transcriptional program of osteoblast differentiation. Genes Dev. 17, 1979–1991. doi:10.1101/gad.1119303
Kohlert, C., Van Rensen, I., März, R., Schindler, G., Graefe, E. U., and Veit, M. (2000). Bioavailability and pharmacokinetics of natural volatile terpenes in animals and humans. Planta Med. 66, 495–505. doi:10.1055/s-2000-8616
Lee, Y. S., and Mohamed, R. (2016). “The origin and domestication of Aquilaria, an important agarwood-producing genus,” in Agarwood. Tropical forestry. Editor R. Mohamed (Singapore: Springer).
Li, C., Xu, M. M., Wang, K., Adler, A. J., Vella, A. T., and Zhou, B. (2018). Macrophage polarization and meta-inflammation. Transl. Res. 191, 29–44. doi:10.1016/j.trsl.2017.10.004
Li, H., Li, Y., Zhang, X., Ren, G., Wang, L., Li, J., et al. (2021a). The combination of Aquilaria sinensis (lour.) gilg and Aucklandia costus Falc. Volatile oils exerts antidepressant effects in a CUMS-induced rat model by regulating the HPA Axis and levels of neurotransmitters. Front. Pharmacol. 11. doi:10.3389/fphar.2020.614413
Li, J., Li, L., Wang, X., and Xiao, L. (2020). Porphyromonas gingivalis inhibition of MicroRNA-205-5p expression modulates proinflammatory cytokines in gingival epithelial cells. Biochem. Genet. 58, 566–579. doi:10.1007/s10528-020-09957-y
Li, Q., Wei, P., Li, Y., and Fu, Y. (2024). Optimization of extraction process and analysis of biological activity of flavonoids from leaves of cultivated 'qi-nan' agarwood. Molecules 29, 1828. doi:10.3390/molecules29081828
Li, W., Chen, H. Q., Wang, H., Mei, W. L., and Dai, H. F. (2021b). Natural products in agarwood and Aquilaria plants: chemistry, biological activities and biosynthesis. Nat. Prod. Rep. 38, 528–565. doi:10.1039/d0np00042f
Li, W., Liao, G., Wang, H., Dong, W. H., Yang, L., Kong, F. D., et al. (2019). Five new epoxy-5,6,7,8-tetrahydro-2-(2-phenylethyl)chromones from Chinese agarwood by artificial holing. Fitoterapia 134, 182–187. doi:10.1016/j.fitote.2019.02.031
Liang, D., Feng, B., Li, N., Su, L., Wang, Z., Kong, F., et al. (2022). Preparation, characterization, and biological activity of Cinnamomum cassia essential oil nano-emulsion. Ultrason. Sonochem 86, 106009. doi:10.1016/j.ultsonch.2022.106009
Liao, G., Mei, W. L., Kong, F. D., Li, W., Yuan, J. Z., and Dai, H. F. (2017). 5,6,7,8-Tetrahydro-2-(2-phenylethyl)chromones from artificial agarwood of Aquilaria sinensis and their inhibitory activity against acetylcholinesterase. Phytochemistry 139, 98–108. doi:10.1016/j.phytochem.2017.04.011
Lin, R., Xu, B., Ye, Z., Gao, Y., Fang, H., Song, J., et al. (2023). Metformin attenuates diabetes-induced osteopenia in rats is associated with down-regulation of the RAGE-JAK2-STAT1 signal axis. J. Orthop. Transl. 40, 37–48. doi:10.1016/j.jot.2023.05.002
Linden, G. J., Lyons, A., and Scannapieco, F. A. (2013). Periodontal systemic associations: review of the evidence. J. Periodontol. 84, S8–S19. doi:10.1111/jcpe.12064
Liu, J., Li, T., Chen, T., Gao, J., Zhang, X., Jiang, C., et al. (2022). Integrating multiple omics identifies phaeoacremonium rubrigenum acting as Aquilaria sinensis marker fungus to promote agarwood sesquiterpene accumulation by inducing plant host phosphorylation. Microbiol. Spectr. 10, e0272221. doi:10.1128/spectrum.02722-21
Liu, J., Xu, Y., Zhang, Z., and Wei, J. (2015). Hydrogen peroxide promotes programmed cell death and salicylic acid accumulation during the induced production of sesquiterpenes in cultured cell suspensions of Aquilaria sinensis. Funct. Plant Biol. 42, 337–346. doi:10.1071/FP14189
Liu, M., Liu, Y., Ge, Y., Zhong, Z., Wang, Z., Wu, T., et al. (2020a). Solubility, antioxidation, and oral bioavailability improvement of mangiferin microparticles prepared using the supercritical antisolvent method. Pharmaceutics 12, 90. doi:10.3390/pharmaceutics12020090
Liu, Y. Y., Chen, D. L., Yu, Z. X., Can-Hong, W., Feng, J., Meng, Y., et al. (2020b). New 2-(2-phenylethyl)chromone derivatives from agarwood and their inhibitory effects on tumor cells. Nat. Prod. Res. 34, 1721–1727. doi:10.1080/14786419.2018.1528591
Lu, W., Ding, Z., Liu, F., Shan, W., Cheng, C., Xu, J., et al. (2019). Dopamine delays articular cartilage degradation in osteoarthritis by negative regulation of the NF-κB and JAK2/STAT3 signaling pathways. Biomed. Pharmacother. 119, 109419. doi:10.1016/j.biopha.2019.109419
Luchian, I., Goriuc, A., Sandu, D., and Covasa, M. (2022). The role of matrix metalloproteinases (MMP-8, MMP-9, MMP-13) in periodontal and peri-implant pathological processes. Int. J. Mol. Sci. 23, 1806. doi:10.3390/ijms23031806
Lv, F., Li, S., Feng, J., Liu, P., Gao, Z., Yang, Y., et al. (2019). Hydrogen peroxide burst triggers accumulation of jasmonates and salicylic acid inducing sesquiterpene biosynthesis in wounded Aquilaria sinesis. J. Plant Physiol. 234-235, 167–175. doi:10.1016/j.jplph.2019.02.006
Ma, J., Huo, H., Zhang, H., Wang, L., Meng, Y., Jin, F., et al. (2022). 2-(2-phenylethyl)chromone-enriched extract of the resinous heartwood of Chinese agarwood (Aquilaria sinensis) protects against taurocholic acid-induced gastric epithelial cells apoptosis through Perk/eIF2α/CHOP pathway. Phytomedicine 98, 153935. doi:10.1016/j.phymed.2022.153935
Ma, J., Wang, L., Zhao, Y., Gao, Y., Yin, Z., Zhao, M., et al. (2024). 2-(2-Phenylethyl)chromone-enriched extract of Chinese agarwood (Aquilaria sinensis) inhibits atherosclerosis progression through endoplasmic reticulum stress-mediated CD36 expression in macrophages. J. Ethnopharmacol. 320, 117411. doi:10.1016/j.jep.2023.117411
Malik, R., Paudel, K. R., Manandhar, B., De Rubis, G., Shen, J., Mujwar, S., et al. (2023). Agarwood oil nanoemulsion counteracts LPS-induced inflammation and oxidative stress in RAW264.7 mouse macrophages. Pathol. Res. Pract. 251, 154895. doi:10.1016/j.prp.2023.154895
Marcenes, W., Kassebaum, N. J., Bernabe, E., Flaxman, A., Naghavi, M., Lopez, A., et al. (2013). Global burden of oral conditions in 1990-2010: a systematic analysis. J. Dent. Res. 92, 592–597. doi:10.1177/0022034513490168
Ma, S., Huang, M., Fu, Y., Qiao, M., and Li, Y. (2023). How closely Does induced Agarwood's biological activity Resemble that of wild agarwood? Molecules 28, 2922. doi:10.3390/molecules28072922
Mi, C. N., Mei, W. L., Wang, H., Yang, L., Dong, W. H., Gai, C. J., et al. (2019). Four new guaiane sesquiterpenoids from agarwood of Aquilaria filaria. Fitoterapia 135, 79–84. doi:10.1016/j.fitote.2019.04.007
Mi, C. N., Yuan, J. Z., Zhu, M. M., Yang, L., Wei, Y. M., Wang, H., et al. (2021). 2-(2-Phenylethyl)chromone derivatives: promising α-glucosidase inhibitors in agarwood from Aquilaria filaria. Phytochemistry 181, 112578. doi:10.1016/j.phytochem.2020.112578
Muluke, M., Gold, T., Kiefhaber, K., Al-Sahli, A., Celenti, R., Jiang, H., et al. (2016). Diet-induced obesity and its differential impact on periodontal bone loss. J. Dent. Res. 95, 223–229. doi:10.1177/0022034515609882
Naziz, P. S., Das, R., and Sen, S. (2019). The scent of stress: evidence from the unique fragrance of agarwood. Front. Plant Sci. 10, 840. doi:10.3389/fpls.2019.00840
Nędzi-Góra, M., Górska, R., and Górski, B. (2021). The utility of gingival crevicular fluid matrix metalloproteinase-8 provides site-specific diagnostic value for periodontal grading. Cent. Eur. J. Immunol. 46, 236–243. doi:10.5114/ceji.2021.107031
Nurminah, M., Batubara, R., Ismanelly, T., and Albert, (2021). Taking essential oil by water distillation and antibacterial activity test of refined water from agarwood plant parts (Aquilaria malaccensis Lamk). IOP Conf. Ser. Earth Environ. Sci. 782, 032076. doi:10.1088/1755-1315/782/3/032076
Oka, H., Miyauchi, M., Sakamoto, K., Moriwaki, S., Niida, S., Noguchi, K., et al. (2007). PGE2 activates cementoclastogenesis by cementoblasts via EP4. J. Dent. Res. 86, 974–979. doi:10.1177/154405910708601011
Pang, K. L., Chin, K. Y., and Nirwana, S. I. (2023). Immunomodulatory effects of agarwood leaf extract on RAW264.7 murine macrophages. Endocr. Metab. Immune Disord. Drug Targets 23, 964–976. doi:10.2174/1871530323666230103153134
Pang, Y., Yu, W., Liang, W., Gao, Y., Yang, F., Zhu, Y., et al. (2024). Solid-phase microextraction/gas chromatography-time-of-flight mass spectrometry approach combined with network pharmacology analysis to evaluate the quality of agarwood from different regions against anxiety disorder. Molecules 29, 468. doi:10.3390/molecules29020468
Pattarachotanant, N., Rangsinth, P., Warayanon, W., Leung, G. P., Chuchawankul, S., Prasansuklab, A., et al. (2023). Protective effect of Aquilaria crassna leaf extract against benzo[a]pyrene-induced toxicity in neuronal cells and Caenorhabditis elegans: possible active constituent includes Clionasterol. Nutrients 15, 3985. doi:10.3390/nu15183985
Pattarachotanant, N., Sornkaew, N., Warayanon, W., Rangsinth, P., Sillapachaiyaporn, C., Vongthip, W., et al. (2022). Aquilaria crassna leaf extract ameliorates glucose-induced neurotoxicity in vitro and improves lifespan in Caenorhabditis elegans. Nutrients 14, 3668. doi:10.3390/nu14173668
Pavlic, V., Peric, D., Kalezic, I. S., Madi, M., Bhat, S. G., Brkic, Z., et al. (2021). Identification of periopathogens in atheromatous plaques obtained from carotid and coronary arteries. Biomed. Res. Int. 2021, 9986375. doi:10.1155/2021/9986375
Qi, J., Lu, J. J., Liu, J. H., and Yu, B. Y. (2009). Flavonoid and a rare benzophenone glycoside from the leaves of Aquilaria sinensis. Chem. Pharm. Bull. (Tokyo) 57, 134–137. doi:10.1248/cpb.57.134
Reinhardt, R. A., Bolton, R. W., Mcdonald, T. L., Dubois, L. M., and Kaldahl, W. B. (1988). In situ lymphocyte subpopulations from active versus stable periodontal sites. J. Periodontol. 59, 656–670. doi:10.1902/jop.1988.59.10.656
Saini, R., Saini, S., and Sugandha, R. (2011). Periodontal disease: the sixth complication of diabetes. J. Fam. Community Med. 18, 31. doi:10.4103/1319-1683.78636
Samadi, M., Zainal Abidin, Z., Yoshida, H., Yunus, R., and Awang Biak, D. R. (2020). Towards higher oil yield and quality of essential oil extracted from Aquilaria malaccensis wood via the subcritical technique. Molecules 25, 3872. doi:10.3390/molecules25173872
Sangareswari Nagajothi, M., Thangamuthu Parthiban, K., Umesh Kanna, S., Karthiba, L., and Saravanakumar, D. (2016). Fungal microbes associated with Agarwood Formation. Am. J. Plant Sci. 07, 1445–1452. doi:10.4236/ajps.2016.710138
Sanz, M., Marco Del Castillo, A., Jepsen, S., Gonzalez-Juanatey, J. R., D'aiuto, F., Bouchard, P., et al. (2020). Periodontitis and cardiovascular diseases: consensus report. J. Clin. Periodontol. 47, 268–288. doi:10.1111/jcpe.13189
Sharma, P., Dietrich, T., Ferro, C. J., Cockwell, P., and Chapple, I. L. (2016). Association between periodontitis and mortality in stages 3-5 chronic kidney disease: NHANES III and linked mortality study. J. Clin. Periodontol. 43, 104–113. doi:10.1111/jcpe.12502
Shetta, A., Ali, I. H., Sharaf, N. S., and Mamdouh, W. (2024). Review of strategic methods for encapsulating essential oils into chitosan nanosystems and their applications. Int. J. Biol. Macromol. 259, 129212. doi:10.1016/j.ijbiomac.2024.129212
Silva, G. L., Luft, C., Lunardelli, A., Amaral, R. H., Melo, D. A., Donadio, M. V., et al. (2015). Antioxidant, analgesic and anti-inflammatory effects of lavender essential oil. Acad Bras Cienc 87, 1397–1408. doi:10.1590/0001-3765201520150056
Singh, Y., Meher, J. G., Raval, K., Khan, F. A., Chaurasia, M., Jain, N. K., et al. (2017). Nanoemulsion: concepts, development and applications in drug delivery. J. Control Release 252, 28–49. doi:10.1016/j.jconrel.2017.03.008
Smiley, C. J., Tracy, S. L., Abt, E., Michalowicz, B. S., John, M. T., Gunsolley, J., et al. (2015). Systematic review and meta-analysis on the nonsurgical treatment of chronic periodontitis by means of scaling and root planing with or without adjuncts. J. Am. Dent. Assoc. 146, 508–524. doi:10.1016/j.adaj.2015.01.028
Song, H. K., Noh, E. M., Kim, J. M., You, Y. O., Kwon, K. B., and Lee, Y. R. (2021). Evodiae fructus extract inhibits interleukin-1β-induced MMP-1, MMP-3, and inflammatory cytokine expression by suppressing the activation of MAPK and STAT-3 in human gingival fibroblasts in vitro. Evid. Based Complement. Altern. Med. 2021, 5858393. doi:10.1155/2021/5858393
Sun, P. W., Xu, Y. H., Yu, C. C., Lv, F. F., Tang, X. L., Gao, Z. H., et al. (2020a). WRKY44 represses expression of the wound-induced sesquiterpene biosynthetic gene ASS1 in Aquilaria sinensis. J. Exp. Bot. 71, 1128–1138. doi:10.1093/jxb/erz469
Sun, S., Zhang, D., Wu, Y., Yan, L., Liu, J., Pan, C., et al. (2020b). The expression of inducible nitric oxide synthase in the gingiva of rats with periodontitis and diabetes mellitus. Arch. Oral Biol. 112, 104652. doi:10.1016/j.archoralbio.2020.104652
Sun, S. C. (2011). Non-canonical NF-κB signaling pathway. Cell Res. 21, 71–85. doi:10.1038/cr.2010.177
Takamatsu, S., and Ito, M. (2018). Agarotetrol: a source compound for low molecular weight aromatic compounds from agarwood heating. J. Nat. Med. 72, 537–541. doi:10.1007/s11418-018-1185-y
Tan, C. S., Isa, N. M., Ismail, I., and Zainal, Z. (2019). Agarwood induction: current developments and future perspectives. Front. Plant Sci. 10, 122. doi:10.3389/fpls.2019.00122
Tay, P. Y., Tan, C. P., Abas, F., Yim, H. S., and Ho, C. W. (2014). Assessment of extraction parameters on antioxidant capacity, polyphenol content, epigallocatechin gallate (EGCG), epicatechin gallate (ECG) and iriflophenone 3-C-β-glucoside of agarwood (Aquilaria crassna) young leaves. Molecules 19, 12304–12319. doi:10.3390/molecules190812304
Tian, C. P., Song, Y. L., Xu, H. T., Niu, S. Q., Wu, Z. H., and Shen, L. Q. (2019). Composition analysis,antioxidative and antibacterial activities comparison of agarwood oils extracted by supercritical and steam distillation. Zhongguo Zhong Yao Za Zhi 44, 4000–4008. doi:10.19540/j.cnki.cjcmm.20190629.302
Tonetti, M. S., and Sanz, M. (2019). Implementation of the new classification of periodontal diseases: decision-making algorithms for clinical practice and education. J. Clin. Periodontol. 46, 398–405. doi:10.1111/jcpe.13104
Tongnuanchan, P., and Benjakul, S. (2014). Essential oils: extraction, bioactivities, and their uses for food preservation. J. Food Sci. 79, R1231–R1249. doi:10.1111/1750-3841.12492
Usui, M., Onizuka, S., Sato, T., Kokabu, S., Ariyoshi, W., and Nakashima, K. (2021). Mechanism of alveolar bone destruction in periodontitis - periodontal bacteria and inflammation. Jpn. Dent. Sci. Rev. 57, 201–208. doi:10.1016/j.jdsr.2021.09.005
Van Dyke, T. E., and Van Winkelhoff, A. J. (2013). Infection and inflammatory mechanisms. J. Clin. Periodontol. 40 (Suppl. 14), S1–S7. doi:10.1111/jcpe.12088
Veldhoen, M. (2017). Interleukin 17 is a chief orchestrator of immunity. Nat. Immunol. 18, 612–621. doi:10.1038/ni.3742
Wang, C., Gong, B., Peng, D., Liu, Y., Wu, Y., and Wei, J. (2023). Agarwood extract mitigates alcoholic fatty liver in C57 mice via anti-oxidation and anti-inflammation. Mol. Med. Rep. 28, 210. doi:10.3892/mmr.2023.13097
Wang, C., Peng, D., Liu, Y., Wu, Y., Guo, P., and Wei, J. (2021a). Agarwood alcohol extract protects against gastric ulcer by inhibiting oxidation and inflammation. Evid. Based Complement. Altern. Med. 2021, 9944685. doi:10.1155/2021/9944685
Wang, C., Peng, D., Liu, Y., Yu, Z., Guo, P., and Wei, J. (2020). Agarwood alcohol extract ameliorates isoproterenol-induced myocardial ischemia by inhibiting oxidation and apoptosis. Cardiol. Res. Pract. 2020, 3640815. doi:10.1155/2020/3640815
Wang, C., Wang, Y., Gong, B., Wu, Y., Chen, X., Liu, Y., et al. (2022a). Effective components and molecular mechanism of agarwood essential oil inhalation and the sedative and hypnotic effects based on GC-MS-qtof and molecular docking. Molecules 27, 3483. doi:10.3390/molecules27113483
Wang, J. S., Lv, Q., Hen, C. Q., Chen, B. C., Chang, X. Y., and Wen, Y. Z. (2014). The comparative extraction process of volatile oil from leaves of Aquilaria sinensis. Food Industry 35, 70–73.
Wang, S., Wang, C., Peng, D., Liu, X., Wu, C., Guo, P., et al. (2017). Agarwood essential oil displays sedative-hypnotic effects through the GABAergic system. Molecules 22, 2190. doi:10.3390/molecules22122190
Wang, S., Wang, C., Yu, Z., Wu, C., Peng, D., Liu, X., et al. (2018a). Agarwood essential oil ameliorates restrain stress-induced anxiety and depression by inhibiting HPA Axis hyperactivity. Int. J. Mol. Sci. 19, 3468. doi:10.3390/ijms19113468
Wang, S. L., Tsai, Y. C., Fu, S. L., Cheng, M. J., Chung, M. I., and Chen, J. J. (2018b). 2-(2-Phenylethyl)-4H-chromen-4-one derivatives from the resinous wood of Aquilaria sinensis with anti-inflammatory effects in LPS-induced macrophages. Molecules 23, 289. doi:10.3390/molecules23020289
Wang, W., Zheng, C., Yang, J., and Li, B. (2021b). Intersection between macrophages and periodontal pathogens in periodontitis. J. Leukoc. Biol. 110, 577–583. doi:10.1002/JLB.4MR0421-756R
Wang, Y., Hou, W., Wei, J., and Liu, Y. (2022b). Research progress on sesquiterpenoids in Aquilariae Lignum Resinatum and their biological activities and predictive analysis on quality marker. Chin. Traditional Herb. Drugs 53, 1191–1209.
Wisutthathum, S., Kamkaew, N., Inchan, A., Chatturong, U., Paracha, T. U., Ingkaninan, K., et al. (2019). Extract of Aquilaria crassna leaves and mangiferin are vasodilators while showing no cytotoxicity. J. Tradit. Complement. Med. 9, 237–242. doi:10.1016/j.jtcme.2018.09.002
Wu, W., Sun, X., Wilson, I., Jiang, L., Jiang, X., Shao, F., et al. (2024). Effects of ferrous sulfate and methyl jasmonate treatment on the content of 2-(2-phenethyl)chromones in Aquilaria sinensis. Plant Cell, Tissue Organ Cult. (PCTOC) 156, 51. doi:10.1007/s11240-024-02683-y
Wu, Z., and Yu, L. (2023). Characteristic quality analysis for biologically induced agarwood columns in Aquilaria sinensis. Environ. Res. 235, 116633. doi:10.1016/j.envres.2023.116633
Xia, L., Li, W., Wang, H., Chen, H., Cai, C., Yang, L., et al. (2019). LC-MS guided identification of dimeric 2-(2-phenylethyl)chromones and sesquiterpene-2-(2-phenylethyl)chromone conjugates from agarwood of Aquilaria crassna and their cytotoxicity. Fitoterapia 138, 104349. doi:10.1016/j.fitote.2019.104349
Xie, Y., Song, L., Li, C., Yang, Y., Zhang, S., Xu, H., et al. (2021). Eudesmane-type and agarospirane-type sesquiterpenes from agarwood of Aquilaria agallocha. Phytochemistry 192, 112920. doi:10.1016/j.phytochem.2021.112920
Xu, J., Du, R., Wang, Y., and Chen, J. (2023). Wound-induced temporal reprogramming of gene expression during Agarwood Formation in Aquilaria sinensis. Plants (Basel) 12, 2901. doi:10.3390/plants12162901
Xu, Y. H., Liao, Y. C., Zhang, Z., Liu, J., Sun, P. W., Gao, Z. H., et al. (2016). Jasmonic acid is a crucial signal transducer in heat shock induced sesquiterpene formation in Aquilaria sinensis. Sci. Rep. 6, 21843. doi:10.1038/srep21843
Yan, T., Yang, S., Chen, Y., Wang, Q., and Li, G. (2019). Chemical profiles of cultivated agarwood induced by different techniques. Molecules 24, 1990. doi:10.3390/molecules24101990
Yang, D. L., Wang, H., Guo, Z. K., Dong, W. H., Mei, W. L., and Dai, H. F. (2014). A new 2-(2-phenylethyl)chromone derivative in Chinese agarwood 'Qi-Nan' from Aquilaria sinensis. J. Asian Nat. Prod. Res. 16, 770–776. doi:10.1080/10286020.2014.896342
Yang, L., Yang, Y. L., Dong, W. H., Li, W., Wang, P., Cao, X., et al. (2019). Sesquiterpenoids and 2-(2-phenylethyl)chromones respectively acting as α-glucosidase and tyrosinase inhibitors from agarwood of an Aquilaria plant. J. Enzyme Inhib. Med. Chem. 34, 853–862. doi:10.1080/14756366.2019.1576657
Yang, X. B., Feng, J., Yang, X. W., Zhao, B., and Liu, J. X. (2012). Aquisiflavoside, a new nitric oxide production inhibitor from the leaves of Aquilaria sinensis. J. Asian Nat. Prod. Res. 14, 867–872. doi:10.1080/10286020.2012.701209
Yang, Y., Chen, H. Q., Kong, F. D., Zhou, L. M., Li, W., Dong, W. H., et al. (2018). Dimeric sesquiterpenoid-4H-chromone derivatives from agarwood of Aquilaria crassna and their cytotoxicity. Phytochemistry 145, 207–213. doi:10.1016/j.phytochem.2017.08.007
Yang, Y., Mei, W. L., Kong, F. D., Chen, H. Q., Li, W., Chen, Z. B., et al. (2017). Four new bi-2-(2-phenylethyl)chromone derivatives of agarwood from Aquilaria crassna. Fitoterapia 119, 20–25. doi:10.1016/j.fitote.2017.03.008
Yang, Y. L., Sun, H. D., Yang, J., Liu, C. Z., Kang, C. Z., Liu, J., et al. (2024). Tandem mass spectrometry (MS/MS) molecular networking guided profiling of small molecules from Aquilaria sinensis (Lour.) Gilg leaves and their bioactivity evaluation. Phytochem. Anal. 35, 135–145. doi:10.1002/pca.3280
Yang, Z. H., Fang, H. B., Tao, C. T., Jiao, Y. B., and Cheng, Y. X. (2023). Eight new 2-(2-phenylethyl)chromone derivatives from agarwood of Aquilaria sinensis with anti-inflammatory activity. Fitoterapia 169, 105564. doi:10.1016/j.fitote.2023.105564
Yu, J. J., Ruddy, M. J., Wong, G. C., Sfintescu, C., Baker, P. J., Smith, J. B., et al. (2007). An essential role for IL-17 in preventing pathogen-initiated bone destruction: recruitment of neutrophils to inflamed bone requires IL-17 receptor-dependent signals. Blood 109, 3794–3802. doi:10.1182/blood-2005-09-010116
Yu, Z., Wang, C., Zheng, W., Chen, D., Liu, Y., Yang, Y., et al. (2020). Anti-inflammatory 5,6,7,8-tetrahydro-2-(2-phenylethyl)chromones from agarwood of Aquilaria sinensis. Bioorg Chem. 99, 103789. doi:10.1016/j.bioorg.2020.103789
Zhang, H., Ma, J. L., Chang, C., Ta, H., Zhao, Y. F., Shi, S. P., et al. (2023). Gastroprotective 2-(2-phenylethyl)chromone-sesquiterpene hybrids from the resinous wood of Aquilaria sinensis (Lour.) Gilg. Bioorg Chem. 133, 106396. doi:10.1016/j.bioorg.2023.106396
Zhang, Y. H. (2014). Study on leaves of Aquilaria sinensis (Lour) Gilg anti-inflammatory substance foundation and the quality.
Zhao, H., Peng, Q., Han, Z., Yang, L., and Wang, Z. (2016). Three new sesquiterpenoids and one new sesquiterpenoid derivative from Chinese eaglewood. Molecules 21, 281. doi:10.3390/molecules21030281
Zheng, H., Gao, J., Man, S., Zhang, J., Jin, Z., and Gao, W. (2019). The protective effects of Aquilariae Lignum Resinatum extract on 5-Fuorouracil-induced intestinal mucositis in mice. Phytomedicine 54, 308–317. doi:10.1016/j.phymed.2018.07.006
Zheng, J., Chen, S., Albiero, M. L., Vieira, G. H. A., Wang, J., Feng, J. Q., et al. (2018). Diabetes activates periodontal ligament fibroblasts via NF-κB in vivo. J. Dent. Res. 97, 580–588. doi:10.1177/0022034518755697
Zhou, M., Wang, H., Suolangjiba, , Kou, J., and Yu, B. (2008). Antinociceptive and anti-inflammatory activities of Aquilaria sinensis (Lour.) Gilg. Leaves extract. J. Ethnopharmacol. 117, 345–350. doi:10.1016/j.jep.2008.02.005
Zhu, Z., Gu, Y., Zhao, Y., Song, Y., Li, J., and Tu, P. (2016a). GYF-17, a chloride substituted 2-(2-phenethyl)-chromone, suppresses LPS-induced inflammatory mediator production in RAW264.7 cells by inhibiting STAT1/3 and ERK1/2 signaling pathways. Int. Immunopharmacol. 35, 185–192. doi:10.1016/j.intimp.2016.03.044
Zhu, Z., Zhao, Y., Huo, H., Gao, X., Zheng, J., Li, J., et al. (2016b). HHX-5, a derivative of sesquiterpene from Chinese agarwood, suppresses innate and adaptive immunity via inhibiting STAT signaling pathways. Eur. J. Pharmacol. 791, 412–423. doi:10.1016/j.ejphar.2016.09.023
Zou, J., Zeng, Z., Xie, W., and Zeng, Z. (2022). Immunotherapy with regulatory T and B cells in periodontitis. Int. Immunopharmacol. 109, 108797. doi:10.1016/j.intimp.2022.108797
Nomenclature
Resource identification initiative
To take part in the Resource Identification Initiative, please use the corresponding catalog number and RRID in your current manuscript. For more information about the project and for steps on how to search for an RRID, please click here.
Life science identifiers
Life Science Identifiers (LSIDs) for ZOOBANK registered names or nomenclatural acts should be listed in the manuscript before the keywords with the following format:
urn:lsid:<Authority>:<Namespace>:<ObjectID>[:<Version>].
Keywords: agarwood, agarwood tree leaves, pharmacological action, mechanism, periodontitis, nanoemulsions
Citation: Xie C, Dong J-Z, Lu B-S, Yan P-Y, Zhao Y-S, Ding X-Y, Lv C-E and Zheng X (2024) Pharmacology and therapeutic potential of agarwood and agarwood tree leaves in periodontitis. Front. Pharmacol. 15:1468393. doi: 10.3389/fphar.2024.1468393
Received: 22 July 2024; Accepted: 21 August 2024;
Published: 11 September 2024.
Edited by:
Zhuo Luo, Guangxi Medical University, ChinaReviewed by:
Yi Mu, ClinChoice Inc., United StatesJiayan Zhang, University of California, Los Angeles, United States
Aihui Wang, University of California, Los Angeles, United States
Copyright © 2024 Xie, Dong, Lu, Yan, Zhao, Ding, Lv and Zheng. This is an open-access article distributed under the terms of the Creative Commons Attribution License (CC BY). The use, distribution or reproduction in other forums is permitted, provided the original author(s) and the copyright owner(s) are credited and that the original publication in this journal is cited, in accordance with accepted academic practice. No use, distribution or reproduction is permitted which does not comply with these terms.
*Correspondence: Xu Zheng, aHkwMjEwMDIyQGhhaW5tYy5lZHUuY24=
†These authors have contributed equally to this work and share first authorship