- 1Third Affiliated Hospital of Zunyi Medical University (First People’s Hospital of Zunyi), Zunyi, China
- 2Kweichow Moutai Hospital, Zunyi, China
Chronic prostatitis is a prevalent male urinary system disorder characterized by pelvic discomfort or pain, bladder dysfunction, sexual dysfunction, and infertility. Pain and lower urinary tract symptoms (LUTS) are the most common symptoms, significantly impacting patients’ quality of life and driving them to seek medical attention. Transient receptor potential vanilloid subtype 1 (TRPV1) is a non-selective calcium ion-dependent cation channel in the TRPV channel family that is widely distributed in neural tissue and plays a role in signal transmission. In this review, we provide a comprehensive overview of the current understanding of the role of TRPV1 in chronic prostatitis. The discussion focuses on the connection between TRPV1 and prostatitis pain and LUTS, and highlights the potential for targeting this channel in the development of novel treatment strategies.
1 Introduction
Prostatitis is characterized by an inflammatory response triggered by microbial pathogens infecting the prostate or by certain non-infectious factors. The incidence rate of prostatitis is approximately 4.5%–9%, with a recurrence rate of around 50% in elderly patients (Polackwich and Shoskes, 2016). In 1995, the National Institute of Health (NIH) classified prostatitis into four categories. Among these categories, type III prostatitis, known as chronic prostatitis/chronic pelvic pain syndrome (CP/CPPS), accounts for more than 90% of cases. Its primary clinical manifestations include discomfort or pain in the pelvic area, abnormal urinary function, sexual dysfunction, and infertility. Pain and lower urinary tract symptoms (LUTS) are the most prevalent and impactful symptoms, significantly affecting patients’ quality of life and being the primary reasons for patient visits (Pena et al., 2021). At present, the primary factors contributing to chronic prostatitis are latent infection, autoimmunity, neuroendocrine factors, sex hormone disorders, pelvic floor muscle dysfunction, peripheral and central sensitization, neuroplasticity, and psychosocial disorders (Lai et al., 2015; Bharucha and Lee, 2016). Nevertheless, the precise mechanism underlying chronic prostatitis remains elusive. Cross-organ sensitization has been a popular theory for the past decade, whereby irritation from an affected pelvic organ can be transmitted to nearby healthy organs, causing healthy organ dysfunction. A study found that sensory nerves from the prostate and bladder converge in the lumbosacral dorsal root ganglion (DRG) (Lee et al., 2016; Schwartz et al., 2016; Funahashi et al., 2019). Research by Aydogdu et al. (2021) and Funahashi et al. (2019) suggested that LUTS in prostatitis may be associated with sensitization of the prostate-bladder organ. Clinically, the main treatments for prostatitis include pharmaceutical treatments (antibiotics, alpha-blockers, anti-inflammatory/immunomodulatory drugs, etc.) and non-drug treatments (Franco et al., 2019; Pena et al., 2021). However, in certain cases, although the inflammation of the prostate tissue resolves after treatment, the symptoms of prostate inflammation persist, highlighting the need for the development of new treatment approaches.
In recent years, some studies have indicated that the transient receptor potential vanilloid subtype 1 (TRPV1) channel is significant in the pathogenesis and progression of prostatitis. TRPV1 is a member of the TRPV channel family, responding to various stimuli such as mechanical, thermal, and chemical cues from both intracellular and extracellular environments (Aghazadeh Tabrizi et al., 2017). This channel is found in both neural and non-neural tissues (Bevan et al., 2014). Activation of TRPV1 by different substances can result in nociceptive or neuropathic pain responses. For instance, certain pro-inflammatory molecules can trigger inflammatory pain by acting on TRPV1, leading to increased expression of this channel in neurons and intensifying the pain response (Aghazadeh Tabrizi et al., 2017). Previous research has identified the expression of TRPV1 in both prostate tissue and prostate innervating nerves (Dinis et al., 2005; Zhang et al., 2019; Vanneste et al., 2021). Following prostate inflammation, an upregulation of TRPV1 expression was noted in bladder tissue, prostate tissue, and prostate-bladder co-innervated neurons (Zhang et al., 2019). Therefore, further elucidating the role of TRPV1 in prostatitis can enhance our understanding of the underlying mechanisms of this condition.
This review provides an overview of the TRPV1 channel structure and explores its expression in prostate tissue and innervation, aiming to understand the role of TRPV1 in pain and LUTS associated with prostatitis. The findings have important implications for future research on the pathogenesis and potential treatment of prostatitis.
2 Overview of TRPV1 channels
TRPV1 is a non-selective cation channel that is located on the plasma membrane of cells, enabling the passage of cations such as H+, Na+, Ca2+, and Mg2+ (Caterina et al., 1997). The TRPV1 protein exhibits a homotetrameric structure, with each subunit consisting of three main parts (Maximiano et al., 2024): six transmembrane domains (S1-S6) located on the cell membrane, which form a hydrophobic pore; an intracellular N-terminus that includes six ankyrin repeat domains and multiple phosphorylation sites; and a C-terminus that contains a TRP domain, endogenous substance binding sites, and multiple calmodulin-binding domains (Figure 1).
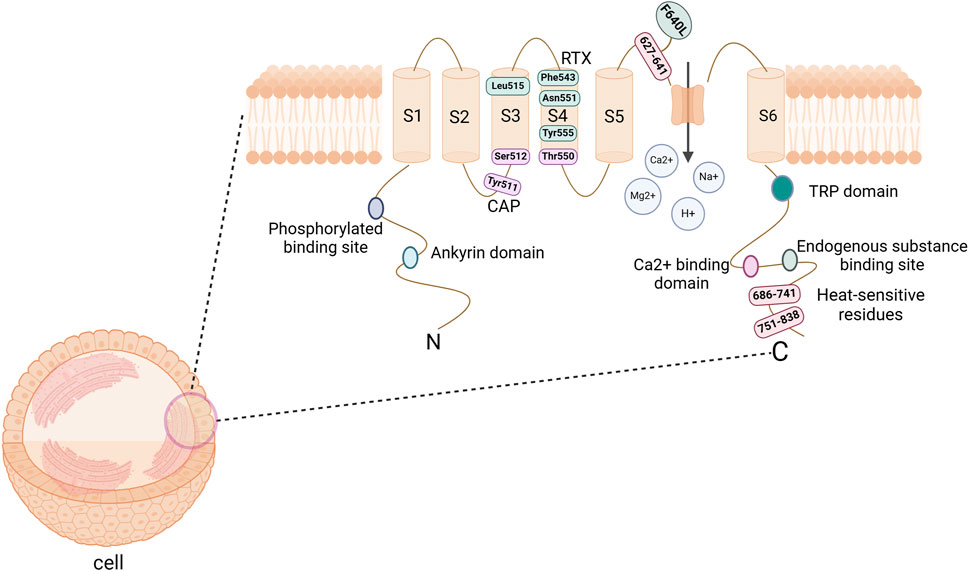
Figure 1. The structure of TRPV1 channel protein. It consists of a tetramer, with each subunit comprising 6 transmembrane domains (S1-S6) on the cell membrane, along with the N-terminus and C-terminus within the cell. The hydrophobic group formed by the transmembrane domains forms a pore that allows cations to pass through. The N-terminal serves as a site for phosphorylation and ankyrin binding, while the C-terminal acts as a binding site for endogenous substances and calcium ions. CAP binding sites are Tyr511, Ser512, and Thr550; RTX binding sites are Leu515, Phe543, Asn551, and Tyr555; Heat-sensitive binding sites are 686–741, 751–838, and F640L. Abbreviations: TRPV1, transient receptor potential vanilloid subtype 1; CAP, Capsaicin; RTX, Resiniferatoxin. Image was created with BioRender.com.
TRPV1 channels are an integration of multiple noxious stimulation molecules and can be activated by various thermal, physical or chemical stimuli (Maximiano et al., 2024). In an environment with a neutral pH, the channel is triggered by harmful heat (>43°C). However, in settings where the pH levels are decreased (like in cases of inflammation or ischemia), the receptor may be activated even at room temperature (Caterina et al., 1997; Frias and Merighi, 2016); In particular, the temperature-sensitive response of TRPV1 is associated with the C-terminal domain, particularly the representative domain sequences 686–741 and 751–838, as well as certain residues in the pore region, such as F640L within the 624–641 sequence (Bevan et al., 2014). In addition, endogenous substances such as cannabinoids, Oleoylethanolamine (OEA), palmitamide ethanol (PEA), bradykinin, and growth factors can modulate the expression of this receptor; Exogenous substances such as capsaicin (CAP), resiniferatoxin (RTX), ethanol, and vanillin have been discovered to stimulate the receptor (Frias and Merighi, 2016; Maximiano et al., 2024). Research has pinpointed the importance of TM3 and TM4 in this channel for interacting with the agonists CAP and RTX (Bevan et al., 2014). Notably, the key binding sites for CAP include Tyr511, Ser512, and Thr550, while the significant binding sites for RTX are Leu515, Phe543, Asn551, and Tyr555 (Andrei et al., 2023). Although the exact binding sites for other agonists remain unclear, there is a hypothesis suggesting potential overlap with the known binding sites (Bevan et al., 2014).
TRPV1 acts as an ion channel that allows high permeability to Ca2+ (Caterina et al., 1997). Activation of this channel leads to the influx of extracellular Ca2+ into the cell, which in turn triggers the release of intracellular Ca2+ stores. This process ultimately results in an increase in intracellular calcium ions, causing cell depolarization and the generation of an action potential. Subsequently, a cascade of intracellular signals is initiated, leading to the release of neurotransmitters such as calcitonin gene peptide, somatostatin, and SP substances. These events play a crucial role in triggering multiple signaling cascades (Caterina et al., 1997; Frias and Merighi, 2016; Satheesh et al., 2016; Maximiano et al., 2024).
TRPV1 is widely expressed in neural and non-neural tissues. The expression of this protein is predominantly found in small to medium-sized neurons within the DRG, trigeminal ganglia, and vagal ganglia. These neurons give rise to unmyelinated C-type sensory nerve fibers or myelinated Aδ-type sensory nerve fibers that innervate various organs and tissues (Caterina et al., 1997; Ho et al., 2012; Bevan et al., 2014; Bujak et al., 2019). Nonneuronal expression of TRPV1 can be found in arteriolar smooth muscle (Phan et al., 2020). Relevant evidence suggests that TRPV1 channels play a role in multiple physiological functions (Szallasi and Blumberg, 1999). Roman et al. (2020) utilized TRPV1 antagonists to mitigate hyperalgesia induced by prostatitis. However, the absence of pelvic allodynia in TRPV1 knockout mice suggests a direct correlation between TRPV1 channels and pain. Therefore, we can infer that The abnormal activation or overactivation of TRPV1 channels is likely associated with the development and pathophysiological progression of the disease. In certain instances, excessive activation of TRPV1 channels can result in heightened pain sensitivity and inflammatory reactions.
3 Expression and function of TRPV1 channel in normal prostate, bladder and DRG
In human prostate tissue, TRPV1- immunoreactive (-IR) fibers are present in the prostate urethral mucosa, seminal fluid, ejaculatory ducts, and periurethral prostate acini. Conversely, TRPV1-IR fibers are not detected in the transitional zone and peripheral zone of the gland (Dinis et al., 2005). In animal models of experimental autoimmune prostatitis, TRPV1 expression was found to be upregulated not only in the prostate but also in the L5-S1 DRG and bladder tissue (Zhang et al., 2019).
Under a microscope, bladder tissue is divided into mucosal, muscular, and adventitial layers. Within the bladder mucosa, the majority of TRPV1-IR fibers are positioned near the basal cells of the bladder transitional epithelium. In the bladder muscle layer, TRPV1-IR fibers exhibit a close association with smooth muscle cells (Lazzeri et al., 2004; Lazzeri et al., 2009). Early pharmacological studies have demonstrated that capsaicin-sensitive afferent fibers (C-fibers) are crucial in the micturition reflex. These fibers have a dual role, acting as sensory afferents in regulating the micturition threshold and vesicourethral pain perception, as well as efferents in controlling nerve excitability, contractility of smooth muscle, and extravasation of plasma proteins (Lazzeri et al., 2009).
In the DRG, TRPV1 is primarily found on the plasma membrane and endoplasmic reticulum of neurons. Activation of TRPV1 leads to the release of Ca2+ from the endoplasmic reticulum, influencing synaptic and presynaptic growth. This process is crucial for signal and synaptic transmission (Wong et al., 2014). These sensory neurons transmit sensory information to various internal organs including the prostate, periurethral area, ejaculatory ducts, skin, and muscles of the genitourinary tract (Light et al., 2008), resulting in a range of biological responses. TRPV1 sensory nerves located in the prostate and perineal skin contribute to heightened pain sensitivity in individuals with chronic prostatitis/chronic pelvic pain syndrome (Lazzeri et al., 2009). Preclinical studies have shown that DRG is the key to this phenomenon (Grundy and Brierley, 2018). Nerve damage, neuropathy, and inflammation in the pelvic organs may cause an upregulation of DRG neurons. This heightened activity can persist as a result of neuroplasticity at the peripheral nerve terminals, DRG neurons, and spinal cord (Grundy and Brierley, 2018; He et al., 2023).
Therefore, the presence of numerous TRPV1 sensory nerves in the prostate and bladder suggests a potential new approach for treating pain and LUTS in patients with chronic prostatitis.
4 Relationship between TRPV1 channels and CP/CPPS
4.1 Association between TRPV1 and autonomic nervous function of CP/CPPS
The autonomic nervous system plays a significant role in the development of chronic pelvic pain syndromes (CP/CPPS). This system encompasses both the peripheral autonomic nervous system, which includes sensory, sympathetic, and parasympathetic nerves, and the central nervous system, comprising the spinal cord and brain (He et al., 2023). During the development of CP/CPPS, alterations in the autonomic nervous system are accompanied by changes in associated substances, receptors, and ion channels. These include substance P (SP), calcitonin gene-related peptide (CGRP), nerve growth factor (NGF), prostaglandin E2 (PGE2), TRPV1 channel, tropomyosin receptor kinase A (TrkA), and protease-activated receptor 2 (PAR2), among others. In this review, we will focus exclusively on the alterations of TRPV1 channels within the autonomic nervous system in the context of CP/CPPS.
The sensory nervous system plays a crucial role in mediating neurogenic inflammation and peripheral sensitization in CP/CPPS. When nociceptive receptors in prostate tissue are repeatedly stimulated by noxious stimuli, C-fibers transmit nociceptive signals to the spinal cord and central nervous system via the DRG. During this process, these sensory nerve fibers activate and release neurogenic peptides, such as SP and CGRP, which mediate neurogenic inflammation and peripheral sensitization (Birder and Kullmann, 2018; He et al., 2023). The upregulation of neurotransmitters and channel receptors within the DRG can activate and sensitize sensory nerves, while also regulating organ cross-sensitization due to the bipartite afferent properties of the DRG (He et al., 2023). TRPV1 plays a crucial role in pain perception within the sensory nervous system (Shiers et al., 2020). The activation of this channel results in an increase in intracellular calcium ion concentration and membrane depolarization, which facilitates the transmission of pain signals as electrical impulses to peripheral nerve fibers, spinal nerves, and cranial nerves (Moore et al., 2017). Consequently, the intrinsic properties of the TRPV1 channel contribute to a lowered pain threshold within the sensory system associated with prostatitis, thereby intensifying the perception of pain. This channel plays a crucial role in mediating the persistent pain experienced in prostatitis.
Sympathetic and parasympathetic nerves originate from the hypogastric plexus. During the onset and progression of prostatitis, alterations in these nerve pathways influence the persistence of pelvic pain and contribute to cross-sensitization between organs (He et al., 2023). The destruction of sympathetic nerves or the release of catecholamines may contribute to the development of chronic prostatitis (Hu et al., 2022; He et al., 2023). Additionally, the imbalance between sympathetic and parasympathetic nerves resulting from chronic prostatitis is a contributing factor to organ cross-sensitization. This sensitization affects various systems, including the cardiovascular, reproductive, and urinary systems. In this review, we will focus exclusively on bladder dysfunction associated with prostatitis. Under normal circumstances, the innervation of the bladder comprises both afferent and efferent nerves. Afferent nerves gather peripheral sensory information and relay it to the spinal cord, while efferent nerves primarily consist of sympathetic and parasympathetic fibers. The interplay between these two types of nerves collaboratively regulates the activity of urination. When the bladder is full, sympathetic nerves release norepinephrine to facilitate smooth muscle relaxation. Conversely, during bladder emptying, parasympathetic nerves release acetylcholine, which induces contraction of the bladder (Yoshimura et al., 2014; Miyazato et al., 2017; Birder and Kullmann, 2018). The sensitization of bladder afferent nerves and the associated material changes induced by CP/CPPS will be discussed in the subsequent chapters. Concerning the efferent nerve activity of the bladder, Lepiarczyk et al. (2017) demonstrated that the number of cholinergic and norepinephrine nerve fibers decreased following the administration of TRPV1 agonists in the bladder. The intraspinal administration of TRPV1 agonists has been shown to decrease sympathetic activity (Wu et al., 2020). These studies have demonstrated a significant relationship between TRPV1 and both sympathetic and parasympathetic nerves. Consequently, during the progression of chronic prostatitis, alterations in TRPV1 may indirectly or directly influence the sympathetic and parasympathetic nervous systems of the prostate and bladder, thereby exacerbating the development and functional changes associated with chronic prostatitis.
The central nervous system, comprising the spinal cord and brain, integrates and processes nociceptive signals. Central sensitization can reduce the pain threshold and heighten the perception of pelvic pain in chronic prostatitis, thereby contributing to the onset and progression of persistent pelvic pain (Birder and Kullmann, 2018; He et al., 2023). Furthermore, the spinal cord segment at the lesion can influence changes in related substances within the adjacent healthy spinal cord segment, indicating that spinal cord sensitization also plays a role in pain sensitization (Javed et al., 2020). In the central nervous system, TRPV1 is predominantly expressed in layers I and II of the dorsal horn of the spinal cord, where it plays a crucial role in regulating the synaptic transmission of nociceptive signals originating from the periphery (Jeffry et al., 2009; Spicarova and Palecek, 2009). Following intraspinal administration of TRPV1 agonists, the connection between TRPV1-expressing neurons and the spinal cord may be permanently disrupted (Iadarola and Gonnella, 2013), resulting in prolonged analgesia (Jeffry et al., 2009).
4.2 TRPV1 and pain caused by CP/CPPS
Activation of TRPV1 in humans triggers nociceptive neurons in the lower urinary tract, causing pain and burning sensations (Maggi et al., 1989). Interestingly, this is the predominant description of pain during urination or ejaculation in patients with CP/CPPS. Dinis et al. (2005) demonstrated that urination, ejaculation, and perineal pain in patients with CP/CPPS are directly related to activation of TRPV1-IR fibers. TRPV1 receptors are thought to be critical for pain perception in prostatitis patients (He et al., 2023). Animal experimental studies have demonstrated that the expression of the nociceptive substance TRPV1 in sensory neurons innervating the prostate increases during prostatic inflammation. Furthermore, the loss of TRPV1 channels has been shown to disrupt the progression of pelvic abnormal pain (Schwartz et al., 2015; Roman et al., 2020).
Prostatic pain exhibits characteristics of visceral organ pain, with pain sensation being transmitted through C-fibers nerves (Lee et al., 2001). The majority of TRPV1 fibers co-express the neuropeptides SP and CGRP, which are commonly associated with pain perception (He et al., 2023; Marek-Jozefowicz et al., 2023). Therefore, inflammatory sensitization of TRPV1 channels on C-fibers nerves plays a crucial role in the development of persistent pain (Malek et al., 2015). First, after prostate inflammation, various cells produce pro-inflammatory factors (TNF-α, IL-8, IFN-γ), anti-inflammatory factors (IL-4, IL-6, IL-10), and regulatory factors (CD4+). Imbalances in these factors contribute to the progression of pain (Wang et al., 2023), with mast cells playing a central role among these cells (Zheng et al., 2017; Li et al., 2023). Following mast cell activation, the cell undergoes degranulation and releases pain-inducing molecules such as NGF and trypsin-β (Done et al., 2012; Roman et al., 2014). NGF, in particular, enhances the expression and transportation of ion channels (e.g., TRPV1) to increase the excitability of nociceptive neurons (Zhang et al., 2005; Stein et al., 2006). Moreover, NGF is involved in regulating the expression of pro-inflammatory molecules like SP and CGRP on nociceptive neurons (Donnerer et al., 1992). Secondly, inflammation triggers the activation of C-fibers nerves, leading to the release of pain transmitters in central terminals. This process also initiates multiple reverse impulses in sensory afferent nerve fibers through local axon reflexes and spinal dorsal root reflexes. Additionally, it involves the release of various neuroactive substances, such as SP and CGRP, resulting in neurogenic inflammation characterized by local vasodilation, congestion, and plasma protein extravasation. Furthermore, some DRG neurons have axon branches connected to neighboring pelvic visceral structures, enabling retrograde propagation of action potentials that may cause neurogenic inflammation in these adjacent pelvic visceral structures, potentially contributing to persistent and referred pain beyond the prostate region (Foreman, 1987; He et al., 2023). And neurogenic substances released from nerve endings can stimulate mast cells to produce additional neuropeptides like NGF, cytokines, and chemokines (Theoharides et al., 2012; Birder and Kullmann, 2018; Lauritano et al., 2023). This process can exacerbate pain sensitization, thereby contributing to the development of a vicious cycle.
In summary, inflammatory changes in pathological prostatitis can trigger the activation of TRPV1 channels in both neural and non-neural tissues, as well as sensitize C-fibers. These sensitized nerve fibers perform a dual role in transmitting pain signals from the periphery to the central nervous system and releasing neuropeptide substances at peripheral nerve endings, potentially intensifying the pain response (Figure 2).
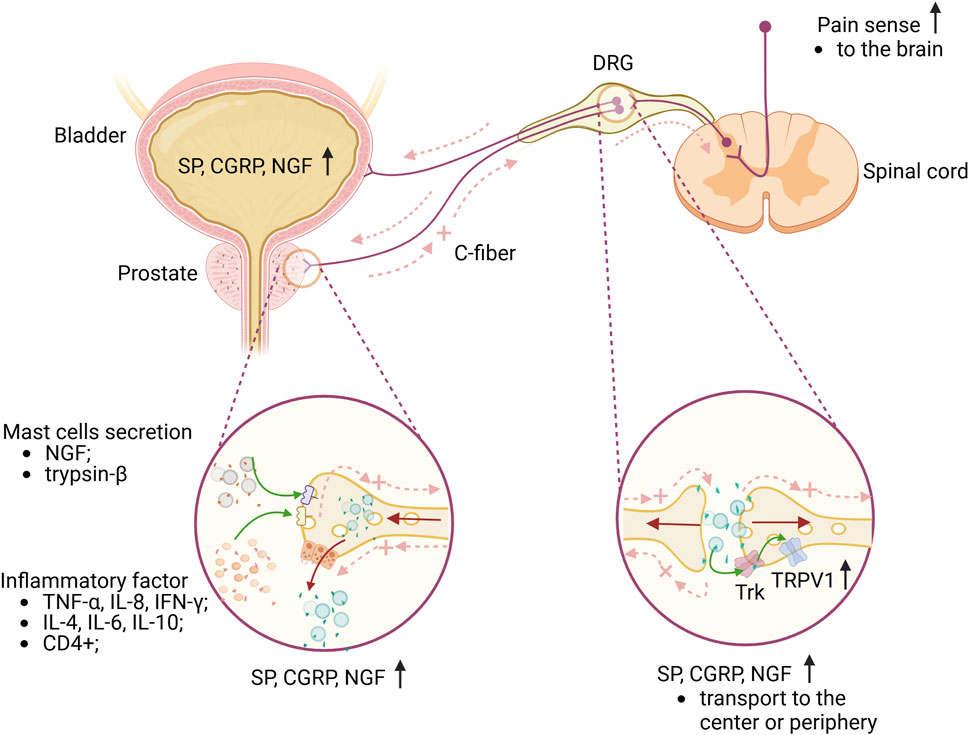
Figure 2. Briefly explain the mechanism of CP/CPPS pain and lower urinary tract. The accumulation of inflammatory factors in the prostate, along with the activation and degranulation of mast cells, results in the sensitization of C-fibers nerve endings in the prostate. This sensitization causes the DRG neurons, which innervate both the prostate and bladder, to express elevated levels of SP, CGRP, and NGF. The release of these neuropeptides into the central nervous system contributes to pain sensitization. Additionally, a significant number of antidromic nerve impulses are generated through dorsal root reflexes or local axonal reflexes, which further release these substances peripherally, leading to a neurogenic inflammatory response in the prostate or bladder. This response can exacerbate pain sensitization or may also contribute to bladder dysfunction. Furthermore, NGF influences sensory nerve endings in the prostate or bladder and can be transported from the periphery to DRG neurons. Upon binding to Trk, NGF enhances neuronal excitability, thereby further sensitizing pain. Green arrows represent the effects of different substances on sensory nerves or receptors; brown arrows represent the transport direction of SP, CGRP and NGF; light red dotted arrows represent the direction of nerve impulse transmission. Abbreviations: TNF-α, tumor necrosis factor-alpha; IL-8, interleukin-8; IFN-γ,interferon-gamma; IL-4, interleukin-4; IL-6, interleukin-6; IL-10, interleukin-10; NGF, nerve growth factor; CGRG, calcitonin gene peptide; SP, substance P; DRG, dorsal root ganglion; Trk, tropomyosin receptor kinase; TRPV1, transient receptor potential vanilloid subtype 1; DRG, dorsal root ganglia; C-fiber, capsaicin-sensitive afferent fiber. Image was created with BioRender.com.
4.3 TRPV1 and LUTS caused by CP/CPPS
In addition to pain, patients with chronic prostatitis may also experience LUTS including frequent urination, urgency, and increased nocturia. Clinical data indicates that approximately 50%–60% of patients with chronic prostatitis are affected by LUTS (Nickel, 2012; Magistro and Nickel, 2019). However, The relationship between histological prostatitis and LUTS remains unclear. Some studies suggest a close association between histological prostatitis and LUTS (Chung et al., 2012; Urkmez et al., 2016; Mizuno et al., 2017) while conflicting findings are presented by Kumsar et al. (2016). Discrepancies in these findings may be attributed to variations in patient inclusion criteria. Although the relationship between prostatitis and LUTS has yielded mixed results in clinical studies, this relationship can be elucidated through cross-organ sensitization observed in animal studies.
Cross-organ sensitization is the core link of LUTS. Funahashi et al. (2019) discovered that in rats with right pelvic nerve transection, formalin injection into the right ventral lobe of the prostate did not trigger bladder overactivity. However, injection into the left prostate lobe resulted in more frequent instances of bladder overactivity. Schwartz et al. (2016) discovered in a chemically induced prostatitis model that sensory nerves (afferent nerves) of the prostate and bladder share common afferent signals in the DRG. They also observed that during prostatic inflammation, bladder afferent nerves become sensitized, leading to a significant increase in micturition frequency. The above research data indicate that chronic prostatitis can result in bladder afferent sensitization, ultimately affecting bladder functionality. The DRG innervating both the prostate and bladder serves as the anatomical foundation for the sensitization of the bladder due to prostatitis. Several studies have identified that certain sensory nerves from the prostate and bladder converge in the DRG (Chen et al., 2010; Lee et al., 2016; Schwartz et al., 2016; Funahashi et al., 2019). The DRG serves as the site where the cell bodies of primary afferent neurons from these two organs come together. In cases where the afferent neurons of one organ are triggered by abnormal stimulation or inflammation, neurogenic substances may be generated within the DRG and then transmitted to another organ, leading to neurogenic inflammation in healthy organs and facilitating cross-sensitization between organs (He et al., 2023). As the cell body of various sensory neurons, the DRG also expresses a range of receptors (such as TRPV1 receptors and NGF receptors) and neuropeptide substances (such as SP and CGRP). The interaction of these receptors and neuropeptide substances can lead to a series of neurophysiological activities that play a significant role in the development of LUTS in chronic prostatitis (Haberberger et al., 2019; He et al., 2023).
An increasing number of animal experimental studies demonstrate that chronic prostatitis can result in alterations in bladder function (Chen et al., 2010; Majima et al., 2015; Schwartz et al., 2016; Park et al., 2018; Ni et al., 2019; Aydogdu et al., 2021). One study discovered a correlation between prostatitis-induced bladder overactivity, alterations in TRPV1 in DRG, and heightened expression of neurogenic substances like NGF in the bladder mucosa with the heightened excitability of bladder afferent nerves triggered by prostatitis (Funahashi et al., 2019). The neurogenic substances produced can impact the excitability of bladder afferent neurons (Shimizu et al., 2023; Ni et al., 2022) and the expression of TRPV1 (Shimizu et al., 2018; Igarashi et al., 2021). Additionally, locally overexpressed NGF can promote neurite growth (Ekman et al., 2017), increase the density of bladder afferent neurons (Schnegelsberg et al., 2010), and facilitate the transportation of NGF to the DRG, ultimately up-regulating TRPV1 expression via the NGF-trk pathway (Zhang et al., 2019). When the TRPV1 gene is knocked out, the presence of NGF and trk will not cause bladder overactivity (Frias et al., 2012). This highlights the importance of TRPV1 as a key target in prostatitis-induced bladder overactivity and as a necessary factor for NGF to drive bladder dysfunction (Figure 2).
5 TRPV1 channel is a potential therapeutic target for CP/CPPS
Due to TRPV1’s distinctive ability to integrate various nociceptive stimuli, it has been extensively researched and utilized as a target for pain management and other medical conditions (Danigo et al., 2013; Gao et al., 2021; Jaffal, 2023). At varying doses and through different administration methods, TRPV1 can induce three distinct effects on nociceptive nerves: excitation, desensitization, and neurotoxicity (Szallasi and Blumberg, 1999). The natural agonists of the TRPV1 channel include irritating plant products like CAP and RTX, as well as animal toxins and venoms (Fischer et al., 2020). CAP and RTX are the two main vanillic acid compounds. Prolonged exposure to both compounds leads to dephosphorylation and homologous desensitization of the channel in a calcium ion-dependent manner (Aghazadeh Tabrizi et al., 2017), resulting in the “defunctionalization” of sensory neurons and depletion of neuropeptide substances (Iftinca et al., 2021). However, the main difference between the two is that RTX causes a slow and long-lasting depolarization after acting on TRPV1, whereas CAP has the opposite effect (Caterina et al., 1997). This mechanism helps prevent the adverse effects of intense neuronal excitation resulting from CAP’s interaction with TRPV1 (Kissin and Szallasi, 2011).
Preclinical data indicate that TRPV1 channels are significantly involved in the pathogenesis and progression of prostatitis. Modulating TRPV1 channels has the potential to alleviate LUTS and pain associated with prostatitis (Table 1.) (Tang et al., 2007; Park et al., 2018; Roman et al., 2020; Lao et al., 2024; Piao et al., 2024). These studies are currently limited to animal models and have not progressed to clinical research. This could be attributed to a lack of understanding regarding the pathogenesis of prostatitis and the potential side effects of TRPV1. Future research should focus on more extensive zoological and pharmacological investigations to enhance our understanding of prostatitis pathogenesis and to develop new TRPV1 derivatives.
In clinical trials, TRPV1 agonists, antagonists, and their derivatives have been extensively utilized across various medical disciplines (Danigo et al., 2013). For instance, 8% capsaicin skin patches have shown efficacy in treating neuropathic pain, liquid capsaicin has been utilized for osteoarthritis management, and Intrathecal administration of RTX has demonstrated effectiveness in alleviating cancer pain (Iftinca et al., 2021). Due to the distribution of TRPV1 channels in various systems of the body (Jaffal, 2023), systemic medication may result in unpredictable adverse reactions. As a result, current medication methods primarily focus on local administration (Iftinca et al., 2021). In most Phase I clinical trials, while TRPV1-related drugs provide pain relief for various patients, they often come with the side effect of elevated body temperature (Bamps et al., 2021). Brown et al. (2017) recently conducted a phase I study to evaluate the safety and pharmacokinetics of oral NEO6860, a mode-selective TRPV1 antagonist, in healthy volunteers. The study found no clinically significant increase in temperature or heat pain threshold/tolerance. Round et al. (2011) investigated the safety of repeated administration of the novel XEN-D0501, a TRPV1 antagonist currently used to treat overactive bladder syndrome, in healthy subjects. They found that the 5 mg dose was safe and well-tolerated, exhibiting good performance with no serious adverse reactions. In comparison to other commonly used TRPV1 antagonists, SB-366791 demonstrates efficient affinity inhibition along with safe and effective selective effects (Gunthorpe et al., 2004). Neuberger et al. (2023) further elucidated the mechanism of interaction between SB-366791 and human TRPV1 following binding and the subsequent conformational changes, thereby advancing the research and application of TRPV1-targeted therapies. Therefore, these clinical studies demonstrate that the systemic application of novel TRPV1 antagonists can significantly mitigate drug-induced adverse reactions, thereby facilitating the advancement of clinical research on TRPV1 medications. Secondly, in terms of topical administration, Turini et al. (2006) found that the topical application of CAP to the perineal skin in patients with CP/CPPS resulted in a significant reduction in the Chronic Prostatitis Symptom Index score and an improvement in pain symptoms. Apostolidis et al. (2006) conducted a follow-up study on patients experiencing refractory urinary urgency and frequency. They found that a single intravesical infusion of RTX could improve the patients’ LUTS, urethral mechanical parameters, and overall quality of life for up to 6 months. Clinical trial studies have demonstrated that intravesical instillation of either RTX or CAP is effective for treating bladder dysfunction resulting from various diseases (Toktanis et al., 2018). Recent studies have demonstrated that several new TRPV1 antagonists have shown promise in the treatment of pain. Notably, Stasi et al. (2022) conducted a study on the topical administration of SAF312, a potent and highly selective non-competitive TRPV1 inhibitor, in healthy subjects. Their findings indicate that this method of administration is both safe and well-tolerated. Furthermore, SAF312 has proven effective in alleviating postoperative pain (Thompson et al., 2023). A randomized, double-blind, controlled study conducted by Segerdahl et al. (2024) demonstrated that the topical application of the novel ACD440 gel, a TRPV1 receptor antagonist, effectively reduced evoked pain responses in healthy volunteers. These clinical studies have demonstrated the role of TRPV1-targeted drugs in the treatment of pain and overactive bladder. Consequently, by integrating the pathogenesis of chronic prostatitis with the function of TRPV1 in the sensitization of internal organs, it is crucial to develop TRPV1-targeted drugs that are safe, efficient, and have minimal side effects for the management of prostatitis in the future.
6 Conclusion
This article outlines the mechanism through which prostatitis induces pain and LUTS, with a specific focus on the role and potential therapeutic targets of TRPV1 in prostatitis. Patients with CP/CPPS experience long-lasting pain and increased activity in the bladder, which is caused by cross-sensitization between the prostate and bladder. This cross-sensitization involves the regulation and/or heightened expression of TRPV1 ion channels. Therefore, targeting TRPV1 may be beneficial in controlling CP/CPPS. However, many uncertainties remain regarding the exact mechanism of action and potential therapeutic targets of TRPV1 channels in prostate disease. Further research and clinical trials are needed to improve our understanding and utilization of the potential of TRPV1 channels to treat prostate disease. In summary, the role of TRPV1 channels in prostate diseases holds significant research value and potential therapeutic applications. However, further in-depth research is necessary to elucidate the precise mechanism of TRPV1 channels in prostate diseases and to formulate appropriate treatment strategies.
Author contributions
ZJ: Conceptualization, Methodology, Writing–original draft, Writing–review and editing. WL: Writing–review and editing. ZL: Writing–review and editing. JC: Writing–review and editing.
Funding
The author(s) declare that no financial support was received for the research, authorship, and/or publication of this article.
Acknowledgments
All images were created with BioRender.com.
Conflict of interest
The authors declare that the research was conducted in the absence of any commercial or financial relationships that could be construed as a potential conflict of interest.
Publisher’s note
All claims expressed in this article are solely those of the authors and do not necessarily represent those of their affiliated organizations, or those of the publisher, the editors and the reviewers. Any product that may be evaluated in this article, or claim that may be made by its manufacturer, is not guaranteed or endorsed by the publisher.
References
Aghazadeh Tabrizi, M., Baraldi, P. G., Baraldi, S., Gessi, S., Merighi, S., and Borea, P. A. (2017). Medicinal chemistry, pharmacology, and clinical implications of TRPV1 receptor antagonists. Med. Res. Rev. 37 (4), 936–983. doi:10.1002/med.21427
Andrei, C., Zanfirescu, A., Nițulescu, G. M., Olaru, O. T., and Negreș, S. (2023). Natural active ingredients and TRPV1 modulation: focus on key chemical moieties involved in ligand–target interaction. Plants 12 (2), 339. doi:10.3390/plants12020339
Apostolidis, A., Gonzales, G. E., and Fowler, C. J. (2006). Effect of intravesical resiniferatoxin (RTX) on lower urinary tract symptoms, urodynamic parameters, and quality of life of patients with urodynamic increased bladder sensation. Eur. Urol. 50 (6), 1299–1305. doi:10.1016/j.eururo.2006.04.006
Aydogdu, O., Gocun, P. U., Aronsson, P., Carlsson, T., and Winder, M. (2021). Prostate-to-bladder cross-sensitization in a model of zymosan-induced chronic pelvic pain syndrome in rats. Prostate 81 (4), 252–260. doi:10.1002/pros.24101
Bamps, D., Vriens, J., Hoon, J. d., and Voets, T. (2021). TRP channel cooperation for nociception: therapeutic opportunities. Annu. Rev. Pharmacol. Toxicol. 61, 655–677. doi:10.1146/annurev-pharmtox-010919-023238
Bevan, S., Quallo, T., and Andersson, D. A. (2014). TRPV1. Handb. Exp. Pharmacol. 222, 207–245. doi:10.1007/978-3-642-54215-2_9
Bharucha, A. E., and Lee, T. H. (2016). Anorectal and pelvic pain. Mayo Clin. Proc. 91 (10), 1471–1486. doi:10.1016/j.mayocp.2016.08.011
Birder, L. A., and Kullmann, F. A. (2018). Role of neurogenic inflammation in local communication in the visceral mucosa. Seminars Immunopathol. 40 (3), 261–279. doi:10.1007/s00281-018-0674-0
Brown, W., Leff, R. L., Griffin, A., Hossack, S., Aubray, R., Walker, P., et al. (2017). Safety, pharmacokinetics, and pharmacodynamics study in healthy subjects of oral NEO6860, a modality selective transient receptor potential vanilloid subtype 1 antagonist. J. Pain 18 (6), 726–738. doi:10.1016/j.jpain.2017.01.009
Bujak, J. K., Kosmala, D., Szopa, I. M., Majchrzak, K., and Bednarczyk, P. (2019). Inflammation, cancer and immunity-implication of TRPV1 channel. Front. Oncol. 9, 1087. doi:10.3389/fonc.2019.01087
Caterina, M. J., Schumacher, M. A., Tominaga, M., Rosen, T. A., Levine, J. D., and Julius, D. (1997). The capsaicin receptor: a heat-activated ion channel in the pain pathway. Nature 389 (6653), 816–824. doi:10.1038/39807
Chen, Y., Wu, X., Liu, J., Tang, W., Zhao, T., and Zhang, J. (2010). Distribution of convergent afferents innervating bladder and prostate at dorsal root Ganglia in rats. Urology 76 (3), 764.e1–e6. doi:10.1016/j.urology.2010.04.002
Chung, J. H., Yu, J. H., Sung, L. H., and Noh, C. H. (2012). Effect of prostatitis on lower urinary tract symptoms: retrospective analysis of prostate biopsy tissue. Korean J. Urology 53 (2), 109–113. doi:10.4111/kju.2012.53.2.109
Danigo, A., Magy, L., and Demiot, C. (2013). TRPV1 in neuropathic pain: from animal models to therapeutical prospects. Med. Sci. Paris. 29 (6–7), 597–606. doi:10.1051/medsci/2013296012
Dinis, P., Charrua, A., Avelino, A., Nagy, I., Quintas, J., Ribau, U., et al. (2005). The distribution of sensory fibers immunoreactive for the TRPV1 (capsaicin) receptor in the human prostate. Eur. Urol. 48 (1), 162–167. doi:10.1016/j.eururo.2005.01.009
Done, J. D., Rudick, C. N., Quick, M. L., Schaeffer, A. J., and Thumbikat, P. (2012). Role of mast cells in male chronic pelvic pain. J. Urology 187 (4), 1473–1482. doi:10.1016/j.juro.2011.11.116
Donnerer, J., Schuligoi, R., and Stein, C. (1992). Increased content and transport of substance P and calcitonin gene-related peptide in sensory nerves innervating inflamed tissue: evidence for a regulatory function of nerve growth factor in vivo. Neuroscience 49 (3), 693–698. doi:10.1016/0306-4522(92)90237-v
Ekman, M., Zhu, B., Swärd, K., and Uvelius, B. (2017). Neurite outgrowth in cultured mouse pelvic ganglia - effects of neurotrophins and bladder tissue. Aut. Neurosci. basic and Clin. 205, 41–49. doi:10.1016/j.autneu.2017.03.004
Fischer, M. J. M., Ciotu, C. I., and Szallasi, A. (2020). The mysteries of capsaicin-sensitive afferents. Front. Physiology 11, 554195. doi:10.3389/fphys.2020.554195
Foreman, J. C. (1987). Peptides and neurogenic inflammation. Br. Med. Bull. 43 (2), 386–400. doi:10.1093/oxfordjournals.bmb.a072189
Franco, J. V., Turk, T., Jung, J. H., Xiao, Y. T., Iakhno, S., Tirapegui, F. I., et al. (2019). Pharmacological interventions for treating chronic prostatitis/chronic pelvic pain syndrome. Cochrane Database Syst. Rev. 2019 (10), CD012552. doi:10.1002/14651858.CD012552.pub2
Frias, B., Charrua, A., Avelino, A., Michel, M. C., Cruz, F., and Cruz, C. D. (2012). Transient receptor potential vanilloid 1 mediates nerve growth factor-induced bladder hyperactivity and noxious input. BJU Int. 110 (8 Pt B), E422–E428. doi:10.1111/j.1464-410X.2012.11187.x
Frias, B., and Merighi, A. (2016). Capsaicin, nociception and pain. Molecules 21 (6), 797. doi:10.3390/molecules21060797
Funahashi, Y., Takahashi, R., Mizoguchi, S., Suzuki, T., Takaoka, E., Ni, J., et al. (2019). Bladder overactivity and afferent hyperexcitability induced by prostate-to-bladder cross-sensitization in rats with prostatic inflammation. J. Physiology 597 (7), 2063–2078. doi:10.1113/JP277452
Gao, M., Wang, Y., Liu, L., Qiao, Z., and Yan, L. (2021). A patent review of transient receptor potential vanilloid type 1 modulators (2014-present). Expert Opin. Ther. Pat. 31 (2), 169–187. doi:10.1080/13543776.2021.1854225
Grundy, L., and Brierley, S. M. (2018). Cross-organ sensitization between the colon and bladder: to pee or not to pee? Am. J. Physiology. Gastrointest. Liver Physiology 314 (3), G301–G308. doi:10.1152/ajpgi.00272.2017
Gunthorpe, M. J., Rami, H. K., Jerman, J. C., Smart, D., Gill, C. H., Soffin, E. M., et al. (2004). Identification and characterisation of SB-366791, a potent and selective vanilloid receptor (VR1/TRPV1) antagonist. Neuropharmacology 46 (1), 133–149. doi:10.1016/s0028-3908(03)00305-8
Haberberger, R. V., Barry, C., Dominguez, N., and Matusica, D. (2019). Human dorsal root ganglia. Front. Cell. Neurosci. 13, 271. doi:10.3389/fncel.2019.00271
He, H., Luo, H., Qian, B., Xu, H., Zhang, G., Zou, X., et al. (2023). Autonomic nervous system dysfunction is related to chronic prostatitis/chronic pelvic pain syndrome. World J. Men’s Health 41, 1–28. doi:10.5534/wjmh.220248
Ho, K. W., Ward, N. J., and Calkins, D. J. (2012). TRPV1: a stress response protein in the central nervous system. Am. J. Neurodegener. Dis. 1 (1), 1–14.
Hu, H., Cui, Y., Yang, J., and Cao, Y. (2022). Loss of the sympathetic signal produces sterile inflammation of the prostate. Front. Mol. Neurosci. 15, 855376. doi:10.3389/fnmol.2022.855376
Iadarola, M. J., and Gonnella, G. L. (2013). Resiniferatoxin for pain treatment: an interventional approach to personalized pain medicine. open pain J. 6, 95–107. doi:10.2174/1876386301306010095
Iftinca, M., Defaye, M., and Altier, C. (2021). TRPV1-Targeted drugs in development for human pain conditions. Drugs 81 (1), 7–27. doi:10.1007/s40265-020-01429-2
Igarashi, T., Tyagi, P., Mizoguchi, S., Saito, T., Furuta, A., Suzuki, Y., et al. (2021). Therapeutic effects of nerve growth factor-targeting therapy on bladder overactivity in rats with prostatic inflammation. Prostate 81 (16), 1303–1309. doi:10.1002/pros.24227
Jaffal, S. M. (2023). Role of transient receptor potential vanilloid 1 in Health and disease. J. Explor. Res. Pharmacol. 8 (4), 348–361. doi:10.14218/JERP.2023.00013
Javed, H., Rehmathulla, S., Tariq, S., Emerald, B. S., Ljubisavljevic, M., and Shehab, S. (2020). Perineural application of resiniferatoxin on uninjured L3 and L4 nerves completely alleviates thermal and mechanical hypersensitivity following L5 nerve injury in rats. J. Comp. Neurology 528 (13), 2195–2217. doi:10.1002/cne.24884
Jeffry, J. A., Yu, S. Q., Sikand, P., Parihar, A., Evans, M. S., and Premkumar, L. S. (2009). Selective targeting of TRPV1 expressing sensory nerve terminals in the spinal cord for long lasting analgesia. PloS One 4 (9), e7021. doi:10.1371/journal.pone.0007021
Kissin, I., and Szallasi, A. (2011). Therapeutic targeting of TRPV1 by resiniferatoxin, from preclinical studies to clinical trials. Curr. Top. Med. Chem. 11 (17), 2159–2170. doi:10.2174/156802611796904924
Kumsar, S., Kose, O., Aydemir, H., Halis, F., Gokce, A., Adsan, O., et al. (2016). The relationship between histological prostatitis and lower urinary tract symptoms and sexual function. Int. Braz J Urol Official J. Braz. Soc. Urology 42 (3), 540–545. doi:10.1590/S1677-5538.IBJU.2015.0254
Lai, H., Gereau, R. W., Luo, Y., O'Donnell, M., Rudick, C. N., Pontari, M., et al. (2015). Animal models of urologic chronic pelvic pain syndromes: findings from the multidisciplinary approach to the study of chronic pelvic pain research network. Urology 85 (6), 1454–1465. doi:10.1016/j.urology.2015.03.007
Lao, Y., Bai, Y., Guan, X., Wang, J., Wang, Y., Li, R., et al. (2024). Sertraline alleviates chronic prostatitis by regulating the TRPV1 channel. J. Inflamm. Res. 17, 4257–4275. doi:10.2147/JIR.S458132
Lauritano, D., Mastrangelo, F., D'Ovidio, C., Ronconi, G., Caraffa, A., Gallenga, C. E., et al. (2023). Activation of mast cells by neuropeptides: the role of pro-inflammatory and anti-inflammatory cytokines. Int. J. Mol. Sci. 24 (5), 4811. doi:10.3390/ijms24054811
Lazzeri, M., Costantini, E., and Porena, M. (2009). TRP family proteins in the lower urinary tract: translating basic science into new clinical prospective. Ther. Adv. Urology 1 (1), 33–42. doi:10.1177/1756287209103922
Lazzeri, M., Vannucchi, M. G., Zardo, C., Spinelli, M., Beneforti, P., Turini, D., et al. (2004). Immunohistochemical evidence of vanilloid receptor 1 in normal human urinary bladder. Eur. Urol. 46 (6), 792–798. doi:10.1016/j.eururo.2004.08.007
Lee, J. C., Yang, C. C., Kromm, B. G., and Berger, R. E. (2001). Neurophysiologic testing in chronic pelvic pain syndrome: a pilot study. Urology 58 (2), 246–250. doi:10.1016/s0090-4295(01)01143-8
Lee, S., Yang, G., Xiang, W., and Bushman, W. (2016). Retrograde double-labeling demonstrates convergent afferent innervation of the prostate and bladder. Prostate 76 (8), 767–775. doi:10.1002/pros.23170
Lepiarczyk, E., Bossowska, A., Kaleczyc, J., Skowrońska, A., Majewska, M., Majewski, M., et al. (2017). The influence of resiniferatoxin (RTX) and tetrodotoxin (TTX) on the distribution, relative frequency, and chemical coding of noradrenergic and cholinergic nerve fibers supplying the porcine urinary bladder wall. Toxins 9 (10), 310. doi:10.3390/toxins9100310
Li, Z.-H., Zhao, X. D., Ming, S. R., Li, X., Hu, J. W., and Chen, Y. L. (2023). Effect of electroacupuncture on the morphology of prostate and degranulation of mast cells in rats with chronic nonbacterial prostatitis. Zhen Ci Yan Jiu = Acupunct. Res. 48 (1), 56–62. doi:10.13702/j.1000-0607.20211148
Light, A. R., Hughen, R. W., Zhang, J., Rainier, J., Liu, Z., and Lee, J. (2008). Dorsal root ganglion neurons innervating skeletal muscle respond to physiological combinations of protons, ATP, and lactate mediated by ASIC, P2X, and TRPV1. J. Neurophysiology 100 (3), 1184–1201. doi:10.1152/jn.01344.2007
Maggi, C. A., Barbanti, G., Santicioli, P., Beneforti, P., Misuri, D., Meli, A., et al. (1989). Cystometric evidence that capsaicin-sensitive nerves modulate the afferent branch of micturition reflex in humans. J. Urology 142 (1), 150–154. doi:10.1016/s0022-5347(17)38701-3
Magistro, G., and Nickel, J. C. (2019). Evaluation of chronic prostatitis/chronic pelvic pain syndrome. Ger. Med. Sci. 0 (0), 0. doi:10.5680/lhuii000014
Majima, T., Funahashi, Y., Kadekawa, K., Gotoh, M., and Yoshimura, N. (2015). Mp25-05 enhanced bladder pain sensitivity due to the prostate-to-bladder afferent cross sensitization in A rat model of prostatitis. J. Urology 193. doi:10.1016/j.juro.2015.02.1208
Malek, N., Pajak, A., Kolosowska, N., Kucharczyk, M., and Starowicz, K. (2015). The importance of TRPV1-sensitisation factors for the development of neuropathic pain. Mol. Cell. Neurosci. 65, 1–10. doi:10.1016/j.mcn.2015.02.001
Marek-Jozefowicz, L., Nedoszytko, B., Grochocka, M., Żmijewski, M. A., Czajkowski, R., Cubała, W. J., et al. (2023). Molecular mechanisms of neurogenic inflammation of the skin. Int. J. Mol. Sci. 24 (5), 5001. doi:10.3390/ijms24055001
Maximiano, T. K. E., Carneiro, J. A., Fattori, V., and Verri, W. A. (2024). TRPV1: receptor structure, activation, modulation and role in neuro-immune interactions and pain. Cell Calcium 119, 102870. doi:10.1016/j.ceca.2024.102870
Miyazato, M., Kadekawa, K., Kitta, T., Wada, N., Shimizu, N., de Groat, W. C., et al. (2017). New frontiers of basic science research in neurogenic lower urinary tract dysfunction. Urologic Clin. N. Am. 44 (3), 491–505. doi:10.1016/j.ucl.2017.04.014
Mizuno, T., Hiramatsu, I., Aoki, Y., Shimoyama, H., Nozaki, T., Shirai, M., et al. (2017). Relation between histological prostatitis and lower urinary tract symptoms and erectile function. Prostate Int. 5 (3), 119–123. doi:10.1016/j.prnil.2017.04.001
Moore, C., Gupta, R., Jordt, S. E., Chen, Y., and Liedtke, W. B. (2017). Regulation of pain and itch by TRP channels. Neurosci. Bull. 34 (1), 120–142. doi:10.1007/s12264-017-0200-8
Neuberger, A., Oda, M., Nikolaev, Y. A., Nadezhdin, K. D., Gracheva, E. O., Bagriantsev, S. N., et al. (2023). Human TRPV1 structure and inhibition by the analgesic SB-366791. Nat. Commun. 14 (1), 2451. doi:10.1038/s41467-023-38162-9
Ni, J., Mizoguchi, S., Bernardi, K., Suzuki, T., Kurobe, M., Takaoka, E., et al. (2019). Long-lasting bladder overactivity and bladder afferent hyperexcitability in rats with chemically-induced prostatic inflammation. Prostate 79 (8), 872–879. doi:10.1002/pros.23794
Ni, J., Suzuki, T., Karnup, S. V., Gu, B., and Yoshimura, N. (2022). Nerve growth factor-mediated Na+ channel plasticity of bladder afferent neurons in mice with spinal cord injury. Life Sci. 298, 120524. doi:10.1016/j.lfs.2022.120524
Nickel, J. C. (2012). Lower urinary tract symptoms associated with prostatitis. Can. Urological Assoc. J. 6 (5 Suppl. 2), S133–S135. doi:10.5489/cuaj.12201
Park, J. S., Jin, M. H., and Hong, C. H. (2018). Neurologic mechanisms underlying voiding dysfunction due to prostatitis in a rat model of nonbacterial prostatic inflammation. Int. Neurourol. J. 22 (2), 90–98. doi:10.5213/inj.1836124.062
Pena, V. N., Engel, N., Gabrielson, A. T., Rabinowitz, M. J., and Herati, A. S. (2021). Diagnostic and management strategies for patients with chronic prostatitis and chronic pelvic pain syndrome. Drugs and Aging 38 (10), 845–886. doi:10.1007/s40266-021-00890-2
Phan, T. X., Ton, H. T., Gulyás, H., Pórszász, R., Tóth, A., Russo, R., et al. (2020). TRPV1 expressed throughout the arterial circulation regulates vasoconstriction and blood pressure. J. physiology 598 (24), 5639–5659. doi:10.1113/JP279909
Piao, J. J., Kim, S., Shin, D., Lee, H. J., Jeon, K. H., Tian, W. J., et al. (2024). Cannabidiol alleviates chronic prostatitis and chronic pelvic pain syndrome via CB2 receptor activation and TRPV1 desensitization. World J. Men’s Health 42. doi:10.5534/wjmh.230352
Polackwich, A. S., and Shoskes, D. A. (2016). Chronic prostatitis/chronic pelvic pain syndrome: a review of evaluation and therapy. Prostate Cancer Prostatic Dis. 19 (2), 132–138. doi:10.1038/pcan.2016.8
Roman, K., Done, J. D., Schaeffer, A. J., Murphy, S. F., and Thumbikat, P. (2014). Tryptase-PAR2 axis in experimental autoimmune prostatitis, a model for chronic pelvic pain syndrome. Pain 155 (7), 1328–1338. doi:10.1016/j.pain.2014.04.009
Roman, K., Hall, C., Schaeffer, A. J., and Thumbikat, P. (2020). TRPV1 in experimental autoimmune prostatitis. Prostate 80 (1), 28–37. doi:10.1002/pros.23913
Round, P., Priestley, A., and Robinson, J. (2011). An investigation of the safety and pharmacokinetics of the novel TRPV1 antagonist XEN-D0501 in healthy subjects. Br. J. Clin. Pharmacol. 72 (6), 921–931. doi:10.1111/j.1365-2125.2011.04040.x
Satheesh, N. J., Uehara, Y., Fedotova, J., Pohanka, M., Büsselberg, D., and Kruzliak, P. (2016). TRPV currents and their role in the nociception and neuroplasticity. Neuropeptides 57, 1–8. doi:10.1016/j.npep.2016.01.003
Schnegelsberg, B., Sun, T. T., Cain, G., Bhattacharya, A., Nunn, P. A., Ford, A. P. D. W., et al. (2010). Overexpression of NGF in mouse urothelium leads to neuronal hyperinnervation, pelvic sensitivity, and changes in urinary bladder function. Am. J. physiology. Regul. Integr. Comp. physiology 298 (3), R534–R547. doi:10.1152/ajpregu.00367.2009
Schwartz, E. S., La, J. H., Young, E. E., Feng, B., Joyce, S., and Gebhart, G. F. (2016). Chronic prostatitis induces bladder hypersensitivity and sensitizes bladder afferents in the mouse. J. Urology 196 (3), 892–901. doi:10.1016/j.juro.2016.03.077
Schwartz, E. S., Xie, A., La, J. H., and Gebhart, G. F. (2015). Nociceptive and inflammatory mediator upregulation in a mouse model of chronic prostatitis. Pain 156 (8), 1537–1544. doi:10.1097/j.pain.0000000000000201
Segerdahl, M., Rother, M., Halldin, M. M., Popescu, T., and Schaffler, K. (2024). Topically applied novel TRPV1 receptor antagonist, ACD440 Gel, reduces evoked pain in healthy volunteers, a randomized, double-blind, placebo-controlled, crossover study. Eur. J. Pain. doi:10.1002/ejp.2299
Shiers, S., Klein, R. M., and Price, T. J. (2020). Quantitative differences in neuronal subpopulations between mouse and human dorsal root ganglia demonstrated with RNAscope in situ hybridization. Pain 161 (10), 2410–2424. doi:10.1097/j.pain.0000000000001973
Shimizu, N., Saito, T., Wada, N., Hashimoto, M., Shimizu, T., Kwon, J., et al. (2023). Molecular mechanisms of neurogenic lower urinary tract dysfunction after spinal cord injury. Int. J. Mol. Sci. 24 (9), 7885. doi:10.3390/ijms24097885
Shimizu, N., Wada, N., Shimizu, T., Suzuki, T., Takaoka, E. I., Kanai, A. J., et al. (2018). Effects of nerve growth factor neutralization on TRP channel expression in laser-captured bladder afferent neurons in mice with spinal cord injury. Neurosci. Lett. 683, 100–103. doi:10.1016/j.neulet.2018.06.049
Spicarova, D., and Palecek, J. (2009). The role of the TRPV1 endogenous agonist N-Oleoyldopamine in modulation of nociceptive signaling at the spinal cord level. J. Neurophysiology 102 (1), 234–243. doi:10.1152/jn.00024.2009
Stasi, K., Alshare, Q., Jain, M., Wald, M., and Li, Y. (2022). Topical ocular TRPV1 antagonist SAF312 (libvatrep) demonstrates safety, low systemic exposure, and No anesthetic effect in healthy participants. Transl. Vis. Sci. and Technol. 11 (11), 15. doi:10.1167/tvst.11.11.15
Stein, A. T., Ufret-Vincenty, C. A., Hua, L., Santana, L. F., and Gordon, S. E. (2006). Phosphoinositide 3-kinase binds to TRPV1 and mediates NGF-stimulated TRPV1 trafficking to the plasma membrane. J. General Physiology 128 (5), 509–522. doi:10.1085/jgp.200609576
Szallasi, A., and Blumberg, P. M. (1999). Vanilloid (Capsaicin) receptors and mechanisms. Pharmacol. Rev. 51 (2), 159–212.
Tang, W., Song, B., Zhou, Z. S., and Lu, G. S. (2007). Intrathecal administration of resiniferatoxin produces analgesia against prostatodynia in rats. Chin. Med. J. 120 (18), 1616–1621. doi:10.1097/00029330-200709020-00013
Theoharides, T. C., Alysandratos, K. D., Angelidou, A., Delivanis, D. A., Sismanopoulos, N., Zhang, B., et al. (2012). Mast cells and inflammation. Biochimica Biophysica Acta 1822 (1), 21–33. doi:10.1016/j.bbadis.2010.12.014
Thompson, V., Moshirfar, M., Clinch, T., Scoper, S., Linn, S. H., McIntosh, A., et al. (2023). Topical ocular TRPV1 antagonist SAF312 (Libvatrep) for postoperative pain after photorefractive keratectomy. Transl. Vis. Sci. and Technol. 12 (3), 7. doi:10.1167/tvst.12.3.7
Toktanis, G., Kaya-Sezginer, E., Yilmaz-Oral, D., and Gur, S. (2018). Potential therapeutic value of transient receptor potential channels in male urogenital system. Pflugers Archiv Eur. J. physiology 470 (11), 1583–1596. doi:10.1007/s00424-018-2188-y
Turini, D., Beneforti, P., Spinelli, M., Malagutti, S., and Lazzeri, M. (2006). Heat/burning sensation induced by topical application of capsaicin on perineal cutaneous area: new approach in diagnosis and treatment of chronic prostatitis/chronic pelvic pain syndrome? Urology 67 (5), 910–913. doi:10.1016/j.urology.2005.11.028
Urkmez, A., Yuksel, O. H., Uruc, F., Akan, S., Yildirim, C., Sahin, A., et al. (2016). The effect of asymptomatic histological prostatitis on sexual function and lower urinary tract symptoms. Arch. Espanoles De. Urol. 69 (4), 185–191.
Vanneste, M., Segal, A., Voets, T., and Everaerts, W. (2021). Transient receptor potential channels in sensory mechanisms of the lower urinary tract. Nat. Rev. Urol. 18 (3), 139–159. doi:10.1038/s41585-021-00428-6
Wang, H., Zhao, M., Zhang, J., Yan, B., Gao, Q., and Guo, J. (2023). Traditional Chinese medicine regulates inflammatory factors in chronic prostatitis/chronic pelvic pain syndrome: a review. Integr. Med. Nephrol. Androl. 10 (1), e00001. doi:10.1097/IMNA-D-22-00001
Wong, C.-O., Chen, K., Lin, Y. Q., Chao, Y., Duraine, L., Lu, Z., et al. (2014). A TRPV channel in Drosophila motor neurons regulates presynaptic resting Ca2+ levels, synapse growth, and synaptic transmission. Neuron 84 (4), 764–777. doi:10.1016/j.neuron.2014.09.030
Wu, Y., Hu, Z., Wang, D., Lv, K., and Hu, N. (2020). Resiniferatoxin reduces ventricular arrhythmias in heart failure via selectively blunting cardiac sympathetic afferent projection into spinal cord in rats. Eur. J. Pharmacol. 867, 172836. doi:10.1016/j.ejphar.2019.172836
Yoshimura, N., Ogawa, T., Miyazato, M., Kitta, T., Furuta, A., Chancellor, M. B., et al. (2014). Neural mechanisms underlying lower urinary tract dysfunction. Korean J. Urology 55 (2), 81–90. doi:10.4111/kju.2014.55.2.81
Zhang, J., Yi, Q. T., Gong, M., Zhang, Y. Q., Liu, D., and Zhu, R. J. (2019). Upregulation of TRPV1 in spinal dorsal root ganglion by activating NGF-TrkA pathway contributes to pelvic organ cross-sensitisation in rats with experimental autoimmune prostatitis. Andrologia 51 (8), e13302. doi:10.1111/and.13302
Zhang, X., Huang, J., and McNaughton, P. A. (2005). NGF rapidly increases membrane expression of TRPV1 heat-gated ion channels. EMBO J. 24 (24), 4211–4223. doi:10.1038/sj.emboj.7600893
Keywords: TRPV1 channel, prostatitis, pain, lower urinary tract symptoms, therapeutic target
Citation: Jiang Z, Luo W, Long Z and Chen J (2024) The role of TRPV1 in chronic prostatitis: a review. Front. Pharmacol. 15:1459683. doi: 10.3389/fphar.2024.1459683
Received: 04 July 2024; Accepted: 10 September 2024;
Published: 19 September 2024.
Edited by:
Lingyun Wang, University of Alabama at Birmingham, United StatesReviewed by:
Bin-Nan Wu, Kaohsiung Medical University, TaiwanManigandan Venkatesan, The University of Texas Health Science Center at San Antonio, United States
Copyright © 2024 Jiang, Luo, Long and Chen. This is an open-access article distributed under the terms of the Creative Commons Attribution License (CC BY). The use, distribution or reproduction in other forums is permitted, provided the original author(s) and the copyright owner(s) are credited and that the original publication in this journal is cited, in accordance with accepted academic practice. No use, distribution or reproduction is permitted which does not comply with these terms.
*Correspondence: Wen Luo, d2VuLmx1by44OThAZ21haWwuY29t