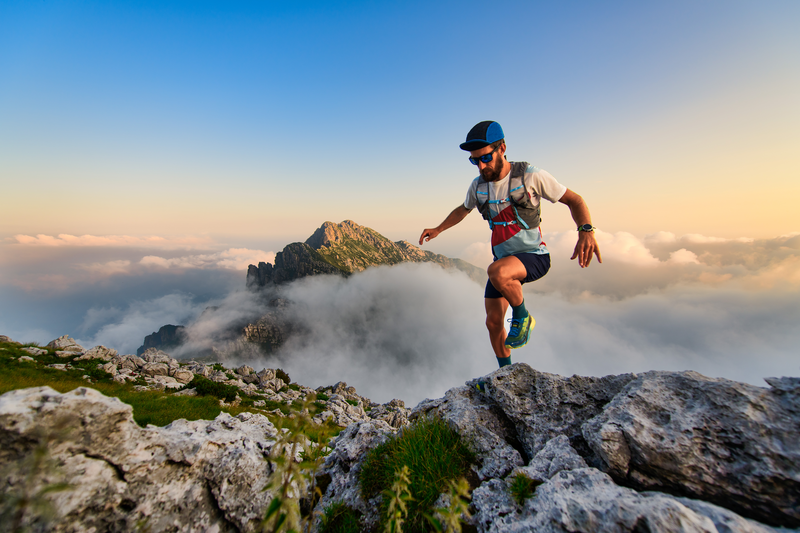
94% of researchers rate our articles as excellent or good
Learn more about the work of our research integrity team to safeguard the quality of each article we publish.
Find out more
DATA REPORT article
Front. Pharmacol. , 23 September 2024
Sec. Pharmacology of Infectious Diseases
Volume 15 - 2024 | https://doi.org/10.3389/fphar.2024.1459408
This article is part of the Research Topic Emerging and Reemerging Neglected Tropical Diseases: Epidemiology, Transmission, Mitigation, and Vaccines and Chemotherapy Advancements View all 9 articles
A correction has been applied to this article in:
Corrigendum: ArboItaly: leveraging open data for enhanced arbovirus surveillance in Italy
Arboviruses, or arthropod-borne viruses, constitute a significant group of pathogens that frequently induce diseases in diverse global regions (Troppens, 2024). These viruses are predominantly transmitted through biological interactions between infected hematophagous arthropods and susceptible vertebrate hosts. Notably, the rapid and concerning reemergence of these viruses in the 21st century is attributed to several factors, including viral adaptation to new vectors, global population growth, urbanization of forest areas, climate change, globalization, and increased population mobility (Troppens, 2024; Weaver, 2013). The mosquito species Aedes aegypti and Aedes albopictus (Kraemer et al., 2019; Nakase et al., 2023) are among the most prominent vectors of arboviruses, transmitting major pathogens such as Zika (Giovanetti et al., 2020), dengue and chikungunya (Mohapatra et al., 2024a), yellow fever (YFV) (Giovanetti et al., 2023). These viruses impose significant health and economic burdens globally. The widespread distribution of Aedes spp. Mosquitoes now extends across all continents, including North America and Europe, a geographical expansion facilitated by globalization and anthropogenic environmental changes, particularly those associated with climate change. Furthermore, Culex spp. Mosquitoes serve as vectors for medically significant arboviruses to animals, including humans, such as West Nile Virus (WNV) (Mingione et al., 2023), Japanese encephalitis virus (Unni et al., 2011), St. Louis encephalitis virus (Kopp et al., 2013), Usutu virus (Roesch et al., 2019), and Murray Valley encephalitis virus (Knox et al., 2012). These and other viruses of the Japanese encephalitis serocomplex are typically spread via infected migratory birds that act as amplifying hosts, influencing the geographical dispersion patterns of these arboviruses. The term “arbovirus” is not a taxonomic classification but encompasses viruses with diverse characteristics from various viral families. Over 500 species of arboviruses are known, and this number continues to grow with advances in DNA sequencing technologies facilitating the genetic characterization of viruses. It is estimated that only about 1% of the world’s total arbovirus diversity is currently identified.
The emergence of infectious diseases poses significant public health challenges globally, with recent outbreaks highlighting the urgent need for effective surveillance and response strategies. In India, the recent surge of scrub typhus (Mohapatra et al., 2024b), particularly in the Sundergarh district of Odisha, has raised alarms as it coincides with outbreaks of other infectious diseases such as leptospirosis. Scrub typhus, a rickettsial infection transmitted by chiggers, manifests with symptoms that can lead to severe complications if not diagnosed and treated promptly. The increasing incidence of scrub typhus, alongside other vector-borne diseases, underscores the impact of environmental changes, urbanization, and population mobility on disease transmission dynamics.
With the rapid global dissemination of these viruses, there is a critical need for robust public data and genomic surveillance systems. These systems are vital for monitoring these diseases and facilitating effective public health responses to prevent large-scale epidemics. The emergence and dispersion of arboviruses are thus significant public health concerns, necessitating comprehensive strategies for surveillance and control. Climate change is leading to an increase in extreme weather events, such as severe droughts and floods, across various continents. With ongoing global warming, these pathogens are progressively moving northward. Since 2007, several European countries, including France, Spain, and Croatia, have faced arboviral epidemics (Zatta et al., 2023; Herrero-Martínez et al., 2023; Pem-Novosel et al., 2015). This underscores the urgent need for the European Union to proactively address the evolving threat posed by these diseases as they extend further into traditionally non-endemic regions.
Such tropical diseases are expected to become more prevalent in Europe and North America soon. Therefore, it is crucial to enhance our understanding of the genetic variants of pathogens with epidemic and pandemic potential to ensure their rapid identification and containment. Factors such as increased connectivity between endemic regions, urbanization, population growth, and climate change are likely to amplify the potential for sustained outbreaks, yet substantial evidence is still required.
In recent years, Italy has faced an increasing number of arboviral disease outbreaks, especially concerning WNV. Despite this growing threat, the fragmented nature of data, often scattered across different sources and formats, has limited the ability to conduct timely analyses, hampering public health efforts to respond quickly to outbreaks. To fill these critical gaps, this paper presents ArboItaly, the first comprehensive and centralized repository dedicated to arboviral disease surveillance in Italy, which not only consolidates epidemiological data from different official sources, but also standardizes and integrates information on different hosts (humans, mosquitoes, equids, and birds) as in the case of West Nile, facilitating the identification of spatiotemporal trends in the spread of a given arbovirus and enabling public health authorities to target control measures more efficiently and allocate resources to high-risk areas.
A paradigm shift in the approach to the growing threat of arboviral diseases is needed, emphasizing the need for greater investment in public health infrastructure. Enhanced surveillance systems, such as ArboItaly, should be supplemented with genomic data to better understand the evolution of arboviruses and their adaptation to new environmental conditions. As arboviral diseases continue to expand into previously unaffected regions, international cooperation and information sharing will be key to mitigating their global impact. Strengthening global surveillance networks will ensure that countries such as Italy, which face similar challenges, can learn from each other’s experiences and best practices, promoting a collaborative approach to disease prevention and control. Integrating environmental monitoring with health data analysis is particularly crucial in the context of climate change, which is changing the geographic range of mosquito vectors and increasing the likelihood of arboviral outbreaks in temperate regions. By using predictive models that incorporate climate data along with epidemiological and genomic information, public health systems can anticipate epidemics and implement preventive measures before they occur. This proactive approach is essential for managing the evolving threat posed by arboviruses, as traditional strategies, such as vector control through insecticides and education and awareness campaigns, are often insufficient in the face of rapid ecological changes and the spread of disease. Through the active and strategic promotion of international collaboration, we can build more resilient and adaptable public health systems that can promptly and competently address emerging challenges and growing risks related to arboviruses, ensuring a coordinated and effective global response.
Our goal was to develop a centralized dataset that stores key information about arboviruses in Italy. Figure 1 summarized the data production process covering from the digitalization to the release of ArboItaly.
The first step (i.e., Collection of bulletins) was to systematically collect the publications from the Italian National Institute of Health (ISS) available on the EpiCentro webpage (https://www.epicentro.iss.it/arbovirosi/bollettini). To do so, we reviewed a total of 192 reports (https://www.epicentro.iss.it/westnile/bollettino) published in PDF format regarding WNV from 27 September 2012, to 4 September 2024. For dengue and chikungunya, we utilized data shared through a dashboard from 2015 to 6 August 2024 (https://www.epicentro.iss.it/arbovirosi/dashboard).
Once the bulletins were collected, the next step (i.e., Classification of information) was to classify the information according to the type of arbovirus being treated. This process involved extracting and categorizing key information, such as demographic information about the age and gender of cases, geographic information at the highest resolution available down to the province level, and other relevant epidemiological information (e.g., type of infection, species affected). We adopted a mixed system combining automatic scripts and manual extraction to transform the data into a structured format that could be easily used for analysis. Automatic scripts, including Tabula (https://tabula.technology/) and the online services such as WebPlotDigitizer (https://automeris.io/) and Wayback Machine (https://wayback-api.archive.org/) were used to extract tabular information from PDFs of WNV bulletins, digitize data from dashboard charts, and reconstruct the history of shared dengue-related data, respectively. However, bulletins also contain sections of unstructured text that cannot be easily extracted with automated tools. Therefore, we supplemented our automated process with manual extraction of these text sections to ensure that important information is not overlooked, and that all data are collected accurately.
The third and final step in the process before releasing the dataset publicly was the pre-processing of the digitalized data. We addressed various challenges related to data quality and consistency, implementing rigorous quality controls to ensure the accuracy of the final dataset. This included cleaning the data to remove any errors or inconsistencies. For example, due to reporting delays and possible measurement errors, weekly WNV cases may have negative values, and this is not correct because cumulative counts must meet a monotonicity constraint. As a result, we chose to set as “Not Available” (“NA”) all negative values of weekly cases (“new_cases”) whenever the cumulative number of cases for the current week (“total_cases”) was lower than that of the previous week. A standardized procedure was also adopted to code geographic information following ISTAT nomenclature, which involved several steps: (i) coding all geographic data using official ISTAT codes for regions and provinces to ensure exact correspondence with official administrative divisions; (ii) adding geographic coordinates (i.e., longitude and latitude) for each unit to facilitate spatial analysis and mapping; (iii) managing geographic ambiguities by using unique identifiers for localities with the same name; and (iv) implementing a consistent schema with standardized prefixes and suffixes to improve readability and integration with other datasets.
To accurately track epidemiological trends of WNV infections in Italy at regional and provincial levels, we organized the collected data according to the different hosts monitored, i.e., humans, mosquitoes, equids, wild and targets birds. In the case of humans, we acquired and analyzed detailed data, including the age of patients and the type of infection (e.g., neuroinvasive, fever, blood donor), allowing a thorough understanding of the spread and impact of the virus in local communities. Mosquitoes, the main vectors of WNV, were monitored through regular collection and testing to identify new cases and outbreaks. In addition, monitoring of equids and birds (both target and wild), has been crucial, focusing on specific species and geographic areas most at risk to identify outbreaks and understand the dynamics of WNV transmission in wildlife. Monitoring dengue and chikungunya infections, on the other hand, involves weekly surveillance of reported cases, with a focus on new cases and related deaths to identify trends in incidence and spread over time. In addition, we subdivided the data according to several key parameters, such as gender, age, and type of infection (autochthonous or imported), to obtain a comprehensive view of the demographic profile of infections. Finally, the subdivision of region cases allows for precise mapping of the most affected areas to develop effective policies in the highest risk areas.
Table 1 provides a concise overview of the structure and main contents of the dataset. The README file of the GitHub repository includes detailed code snippets that describe how to import and manipulate the data. Brief instructions to assist other researchers with reuse of the data are available in Supplementary Material.
The spread of arboviruses is significantly influenced by various environmental and socioeconomic factors, the understanding of which is critical to developing effective strategies for epidemic control and prevention. Temperature and rainfall are two critical seasonal factors that directly influence the life cycle and population dynamics of vectors such as mosquitoes (Ciota and Keyel, 2019; Pascoe et al., 2022). For example, transmission of Zika, dengue, and yellow fever viruses is significantly higher during hot and humid seasons in tropical regions (Mordecai et al., 2017; Aliaga-Samanez et al., 2024). Similarly, abundant rainfall creates more breeding sites by filling containers, ditches and other natural watersheds, facilitating mosquito proliferation (Benedum et al., 2018). Migratory birds contribute significantly to the spread of arboviruses, serving as both reservoirs and vectors. These birds can carry viruses over long distances during their migratory journeys, introducing pathogens to new regions where they can infect local mosquito populations. The study by Ferraguti et al. (Ferraguti et al., 2024) showed that bird migration patterns are aligned with the spread of WNV in Europe. The work, conducted in southwestern Spain, revealed that migratory birds are more likely to be exposed to WNV than native and exotic species. As these birds move between breeding and wintering areas, they can bridge geographic gaps and carry virus across international borders, complicating control efforts. International borders can hinder or facilitate the spread of arboviruses, depending on the effectiveness and coordination of health management and control policies. Well-monitored borders with effective vector control measures can limit the spread of viruses. However, in regions where neighboring countries have divergent resources and policies, the virus can easily cross borders, leading to outbreaks (O'Connor et al., 2021). For example, differences in health infrastructure and disease surveillance between countries can result in undetected transmission, especially when people travel across borders for trade, tourism, or work. Population movements, driven by factors such as seasonal labor migration, displacement due to conflict or environmental changes, can also alter the distribution of arboviruses. These movements can introduce viruses into new areas and increase the frequency and intensity of outbreaks. Refugee camps and temporary settlements often lack adequate vector controls and sanitation facilities, making them hotspots for arbovirus transmission (Kolimenakis et al., 2022). In addition, urbanization and deforestation can change the habitats of vectors, putting them in closer contact with human populations and increasing transmission risks (Tazerji et al., 2022).
Arboviruses epidemics require an integrated and multifactorial approach for effective management (Wallau et al., 2023). Strategies adopted focus on several key aspects, including strengthening epidemiological, entomological, and genomic surveillance, vector control, health system preparedness, public awareness, and advancing scientific research. Epidemiological surveillance is critical for the early identification of human cases of infection, enabling timely interventions to contain the spread of disease. In parallel, entomological surveillance monitors the presence and density of vectors, such as mosquitoes, that are responsible for the transmission of these infections. Genomic information is critical for the early detection of viral outbreaks because it helps to assess the epidemic potential of existing and newly introduced viral strains, to identify the spread of new strains in populations lacking immunity, and to detect the emergence of more virulent variants or those resistant to interventions such as vaccines, antiviral drugs, and various vector control strategies. Vector control is achieved through chemical, biological, and environmental methods (Achee et al., 2019; Huang et al., 2017) aimed at reducing mosquito populations and, consequently, the risk of arboviruses transmission. Health system preparedness is another essential pillar and involves the ongoing training of medical personnel, updating diagnosis and treatment protocols, and adjusting hospital resources to address any outbreaks. Education and public awareness play a crucial role in informing the population about preventive measures and disease symptoms, promoting behaviors that reduce the risk of transmission. In Europe, the approach to arboviruses has had to take into account regional specificities, considering both the species of vectors present and the patterns of disease transmission. The European Union, through the European Centre for Disease Prevention and Control (ECDC), plays a key role in coordinating transnational efforts by providing technical guidelines (https://www.ecdc.europa.eu/sites/default/files/media/en/publications/Publications/TER-Mosquito-surveillance-guidelines.pdf), facilitating information exchange between countries (https://www.ecdc.europa.eu/sites/default/files/documents/Organisation-vector-surveillance-control-Europe_0.pdf), and supporting national and regional preparedness (https://www.ecdc.europa.eu/sites/default/files/documents/ECDC-country-preparedness-2013-2017.pdf). Several European countries have implemented tailored strategies to address specific threats from arboviruses. In Italy, the Ministry of Health has developed a National Plan for Prevention, Surveillance and Response to Arboviruses (https://www.salute.gov.it/imgs/C_17_pubblicazioni_2947_allegato.pdf). This plan focuses on viruses such as Zika, dengue, and chikungunya and includes the following aspects: (i) an early warning system that uses epidemiological and climatic data to predict outbreaks and alert health authorities; (ii) guidelines for diagnosis and treatment of patients, developed in collaboration with infectious disease experts; (iii) strategies for reducing mosquito populations, such as pest control and elimination of breeding sites. France has strengthened its mosquito surveillance network through the use of advanced technologies to monitor mosquito presence in real-time (Calba et al., 2015) and specific interventions in high-risk areas to reduce mosquito populations and prevent outbreaks. Spain has highlighted the need to improve diagnostic capacity by creating standardized procedures for dengue case management. Fernández-Martínez et al. (Fernández-Martínez et al., 2023) conducted a study to assess the risk of local transmission of dengue, focusing on the invasive mosquito A. albopictus. They developed a risk assessment tool that combines environmental, entomological, epidemiological, demographic and travel data to map mosquito distribution and potential areas of spread. The study introduced the Index of Travelers from Endemic Areas (IDVZE) to assess the impact of travelers from dengue-prone regions. Some countries have also adopted innovative approaches. For example, Bellini et al. (Bellini et al., 2013) presented a comprehensive study on the application of the sterile insect technique (SIT) (Lees et al., 2015) for controlling A. albopictus populations in Italy. The research highlights the importance of weather variables, such as temperature and rainfall, in influencing egg sterility and mosquito density. The findings demonstrate that adjusting gamma ray doses during the sterilization process significantly impacts male mortality rates, ultimately enhancing the efficacy of the SIT program. The collaboration with local authorities and the proactive communication with citizens underscores the community’s support for the initiative. Germany has implemented a surveillance system based on citizen volunteers to monitor the presence of invasive mosquitoes (Pernat et al., 2022). The study found that increased media reporting correlated positively with the number of mosquito samples sent, suggesting that effective communication through mass media significantly increases participant activation. The work identified key factors influencing participation, such as project awareness, attention economy and individual characteristics of potential citizen scientists. The results underscored the importance of strategic media communication in promoting public involvement in scientific research, particularly during periods of heightened mosquito-related public health concern. Significant investment has been devoted to genomic research in the United Kingdom to improve understanding of the spread and evolution of vector-borne viruses. The One Health Vector-Borne Diseases Hub has received £1.5 million in funding, which will facilitate the sharing of data and research findings on vector-borne diseases among researchers and policymakers in the UK (https://www.imperial.ac.uk/news/250073/new-gather-data-diseases-spread-mosquitoes/).
At the research and development level, a notable advancement is Valneva’s Ixchiq vaccine for chikungunya, approved under the FDA’s accelerated approval pathway (Branda et al., 2023a). This vaccine represents a significant advancement for individuals over the age of 18 who are at risk. However, for WNV, there is currently no approved vaccine for human use (Cendejas and Goodman, 2024). Although numerous vaccine candidates have entered clinical trials, none have progressed beyond phase II of the FDA’s four-phase licensure process. One prominent candidate, developed by the Vaccine Research Center (VRC), expresses pre-membrane (prM) and envelope (E) glycoproteins and has reached phase I clinical trials, showing neutralizing antibodies in over 90% of young and elderly subjects after three doses. Additionally, a recombinant yellow fever vaccine that also expresses prM and E glycoproteins has demonstrated the ability to generate neutralizing antibodies in 90% of subjects after a single dose. Despite these promising results, challenges remain in developing a human vaccine against WNV. The sporadic nature of WNV outbreaks and high regional variability complicates the logistics of conducting large-scale phase III clinical trials, which require thousands of participants. Moreover, since most individuals infected with WNV are asymptomatic, it is challenging to establish a clear correlation between neutralizing antibody levels and protection. For dengue fever, two vaccines have been licensed: Dengvaxia® (CYD-TDV), developed by Sanofi Pasteur, and Qdenga® (TAK-003), developed by Takeda. Another dengue vaccine, developed by the Laboratory of Infectious Diseases at the National Institutes of Allergy and Infectious Diseases (NIAID) in the United States, is in the late stages of clinical development (https://www.who.int/news-room/questions-and-answers/item/dengue-vaccines).
The presence of competent mosquito vectors such as A. albopictus in Italy has raised concerns about the potential for more widespread transmission. Similar to other Southern European countries, Italy faces a growing threat from arboviruses, exacerbated by climate change and global travel. However, the specific patterns of disease spread, and the effectiveness of control measures can vary significantly. For instance, the 2017 outbreak of chikungunya in Italy, driven by a strain with the E1 226A mutation, reflects a unique adaptation that has not been as prominent in other European outbreaks. The systematic collection and classification of data from ISS have allowed us to create a comprehensive and accessible dataset for the major arboviruses present in Italy, i.e., West Nile, dengue and chikungunya, the details of which are available in the Supplementary Material, that will serve as a necessary tool for public health surveillance and research. The data indicate that WNV has established a significant presence in Italy, with a notable increase in cases over the years (Mingione et al., 2023). Both dengue and chikungunya have shown sporadic outbreaks, with data suggesting a correlation with increased travel and climate variability (Branda et al., 2023b). This trend underscores the need for enhanced vector control measures and public health interventions, particularly in regions with high case numbers.
Despite the comprehensive nature of our dataset, some limitations need to be recognized. First, surveillance coverage may vary across regions, leading to potential under-reporting of cases, particularly in less accessible or resource-limited areas. In addition, the sporadic nature of outbreaks, particularly for chikungunya, can make it difficult to establish clear transmission patterns and predict future outbreaks. Another limitation is the reliance on existing public health infrastructure, which may not be fully equipped to deal with rapid changes in disease patterns due to climate change. In addition, the genetic variability of arboviruses and their vectors presents a challenge for developing targeted interventions that remain effective over time.
Future research should focus on improving understanding of how climate change affects arbovirus transmission and vector behavior. Detailed genomic analyses are critical to identify mutations that impact virus transmission, virulence, and resistance to control methods. In addition, advanced climate modeling is needed to predict changes in vector habitats, enabling more proactive interventions. There is also a need to develop integrated surveillance platforms that combine health, environmental, and genomic data. These platforms can improve real-time monitoring and response capabilities, especially in regions with rapidly changing vector dynamics. Given the continuing threat of arboviruses, future strategies should prioritize strengthening early detection systems that integrate epidemiological, environmental, and genomic data for faster response to outbreaks. This requires not only national efforts but also greater international cooperation, as sharing data and best practices can significantly improve global preparedness. Another important area for future research is the development of new vector control technologies and sustainable methods, such as genetic approaches to reduce mosquito populations or the development of more efficient biocontrol methods.
Climate change poses a growing challenge in the fight against arboviruses as warming temperatures and altered rainfall patterns create environments more conducive to mosquito proliferation. To address these climate-related problems, health policies must be adapted to anticipate long-term changes in disease transmission patterns. Governments should invest in building climate-resilient health systems that can withstand more frequent and intense arbovirus outbreaks. This includes increasing funding for public health infrastructure, training health workers, and improving vector control measures, particularly in high-risk areas. Mitigation efforts should also focus on public education, encouraging communities to reduce mosquito breeding sites and adopt personal protective measures to avoid mosquito bites. The use of advanced technologies, such as climate monitoring systems and predictive modeling tools, can help predict outbreaks and plan early interventions. Finally, continued international collaboration will be essential to manage the cross-border spread of arboviruses, especially as climate-driven migration increases the risk of introducing new diseases to previously unaffected regions.
The datasets presented in this study can be found in online repositories. The names of the repository/repositories and accession number(s) can be found in the article/Supplementary Material.
FB: Conceptualization; Formal analysis; Investigation; Data Curation; Resources; Visualization; Writing–original draft; Writing–review and editing. MG: Investigation; Writing - Original Draft; Writing - Review & Editing. GC: Writing - Original Draft; Writing - Review & Editing. MC: Validation; Supervision; Writing - Original Draft; Writing - Review & Editing. FS: Writing - Original Draft; Writing - Review & Editing.
The author(s) declare that financial support was received for the research, authorship, and/or publication of this article. We acknowledge financial support under the National Recovery and Resilience Plan (NRRP), Mission 4, Component 2, Investment 1.1, Call for tender No. 104 published on 2.2.2022 by the Italian Ministry of University and Research (MUR), funded by the European Union – NextGenerationEU–Project Title “New targets for innovative strategies to reduce SARS-CoV-2 infectivity and identify susceptibility condition in SARS-CoV-2 infected subjects and in Long-COVID patients” – CUP C53D23002260008- Grant Assignment Decree No. 970 adopted on 30/06/2023 by the Italian Ministry of Ministry of University and Research (MUR).
The authors declare that the research was conducted in the absence of any commercial or financial relationships that could be construed as a potential conflict of interest.
All claims expressed in this article are solely those of the authors and do not necessarily represent those of their affiliated organizations, or those of the publisher, the editors and the reviewers. Any product that may be evaluated in this article, or claim that may be made by its manufacturer, is not guaranteed or endorsed by the publisher.
The Supplementary Material for this article can be found online at: https://www.frontiersin.org/articles/10.3389/fphar.2024.1459408/full#supplementary-material
Achee, N. L., Grieco, J. P., Vatandoost, H., Seixas, G., Pinto, J., Ching-Ng, L., et al. (2019). Alternative strategies for mosquito-borne arbovirus control. PLoS neglected Trop. Dis. 13 (1), e0006822. doi:10.1371/journal.pntd.0006822
Aliaga-Samanez, A., Romero, D., Murray, K., Cobos-Mayo, M., Segura, M., Real, R., et al. (2024). Climate change is aggravating dengue and yellow fever transmission risk. Ecography, e06942. doi:10.1111/ecog.06942
Bellini, R., Medici, A., Puggioli, A., Balestrino, F., and Carrieri, M. (2013). Pilot field trials with Aedes albopictus irradiated sterile males in Italian urban areas. J. Med. entomology 50 (2), 317–325. doi:10.1603/me12048
Benedum, C. M., Seidahmed, O. M., Eltahir, E. A., and Markuzon, N. (2018). Statistical modeling of the effect of rainfall flushing on dengue transmission in Singapore. PLoS neglected Trop. Dis. 12 (12), e0006935. doi:10.1371/journal.pntd.0006935
Branda, F., Nakase, T., Maruotti, A., Scarpa, F., Ciccozzi, A., Romano, C., et al. (2023b). “Dengue virus transmission in Italy: surveillance and epidemiological trends up to 2023,” in medRxiv–12. doi:10.1101/2023.12.19.23300208
Branda, F., Scarpa, F., Romano, C., Ciccozzi, A., Maruotti, A., Giovanetti, M., et al. (2023a). Chikungunya vaccine: is it time for it? J. Med. Virology 95 (12), e29341. doi:10.1002/jmv.29341
Calba, C., Goutard, F. L., Hoinville, L., Hendrikx, P., Lindberg, A., Saegerman, C., et al. (2015). Surveillance systems evaluation: a systematic review of the existing approaches. BMC public health 15, 448–453. doi:10.1186/s12889-015-1791-5
Cendejas, P. M., and Goodman, A. G. (2024). Vaccination and control methods of West Nile virus infection in equids and humans. Vaccines 12 (5), 485. doi:10.3390/vaccines12050485
Ciota, A. T., and Keyel, A. C. (2019). The role of temperature in transmission of zoonotic arboviruses. Viruses 11 (11), 1013. doi:10.3390/v11111013
Fernández-Martínez, B., Pampaka, D., Suárez-Sánchez, P., Figuerola, J., Sierra, M. J., León-Gomez, I., et al. (2023). Spatial analysis for risk assessment of dengue in Spain. Enfermedades Infecc. Microbiol. clinica (English doi:10.1016/j.eimce.2023.06.010
Ferraguti, M., Magallanes, S., Mora-Rubio, C., Bravo-Barriga, D., Marzal, A., Hernandez-Caballero, I., et al. (2024). Implications of migratory and exotic birds and the mosquito community on West Nile virus transmission. Infect. Dis. 56 (3), 206–219. doi:10.1080/23744235.2023.2288614
Giovanetti, M., Faria, N. R., Lourenço, J., de Jesus, J. G., Xavier, J., Claro, I. M., et al. (2020). Genomic and epidemiological surveillance of Zika virus in the Amazon region. Cell Rep. 30 (7), 2275–2283. doi:10.1016/j.celrep.2020.01.085
Giovanetti, M., Pinotti, F., Zanluca, C., Fonseca, V., Nakase, T., Koishi, A. C., et al. (2023). Genomic epidemiology unveils the dynamics and spatial corridor behind the Yellow Fever virus outbreak in Southern Brazil. Sci. Adv. 9 (35), eadg9204. doi:10.1126/sciadv.adg9204
Herrero-Martínez, J. M., Sánchez-Ledesma, M., and Ramos-Rincón, J. M. (2023). Imported and autochthonous dengue in Spain. Rev. Clínica Española Engl. Ed. 223 (8), 510–519. doi:10.1016/j.rceng.2023.07.007
Huang, Y. J., Higgs, S., and Vanlandingham, D. L. (2017). Biological control strategies for mosquito vectors of arboviruses. Insects 8 (1), 21. doi:10.3390/insects8010021
Knox, J., Cowan, R., Doyle, J., Ligtermoet, M., Archer, J., Burrow, J., et al. (2012). Murray Valley encephalitis: a review of clinical features, diagnosis and treatment. Med. J. Aust. 196, 322–326. doi:10.5694/mja11.11026
Kolimenakis, A., Tsesmelis, D., Richardson, C., Balatsos, G., Milonas, P. G., Stefopoulou, A., et al. (2022). Knowledge, attitudes and perception of mosquito control in different citizenship regimes within and surrounding the Malakasa open accommodation refugee camp in Athens, Greece. Int. J. Environ. Res. public health 19 (24), 16900. doi:10.3390/ijerph192416900
Kopp, A., Gillespie, T. R., Hobelsberger, D., Estrada, A., Harper, J. M., Miller, R. A., et al. (2013). Provenance and geographic spread of St. Louis encephalitis virus. MBio 4 (3), e00322–e00313. doi:10.1128/mBio.00322-13
Kraemer, M. U., Reiner, Jr R. C., Brady, O. J., Messina, J. P., Gilbert, M., Pigott, D. M., et al. (2019). Past and future spread of the arbovirus vectors Aedes aegypti and Aedes albopictus. Nat. Microbiol. 4 (5), 854–863. doi:10.1038/s41564-019-0376-y
Lees, R. S., Gilles, J. R., Hendrichs, J., Vreysen, M. J., and Bourtzis, K. (2015). Back to the future: the sterile insect technique against mosquito disease vectors. Curr. Opin. Insect Sci. 10, 156–162. doi:10.1016/j.cois.2015.05.011
Mingione, M., Branda, F., Maruotti, A., Ciccozzi, M., and Mazzoli, S. (2023). Monitoring the West Nile virus outbreaks in Italy using open access data. Sci. Data 10 (1), 777. doi:10.1038/s41597-023-02676-0
Mohapatra, R. K., Bhattacharjee, P., Desai, D. N., Kandi, V., Sarangi, A. K., Mishra, S., et al. (2024a). Global health concern on the rising dengue and chikungunya cases in the American regions: countermeasures and preparedness. Health Sci. Rep. 7 (1), e1831. doi:10.1002/hsr2.1831
Mohapatra, R. K., Mishra, S., Tuglo, L. S., Kandi, V., Mohanty, A., and Sah, R. (2024b). Health emergency with recent surge of scrub typhus alongside other infectious diseases in India with Odisha in focus: suggested mitigation measures and preparedness of public healthcare infrastructure. New Microbes New Infect. 56, 101216. doi:10.1016/j.nmni.2023.101216
Mordecai, E. A., Cohen, J. M., Evans, M. V., Gudapati, P., Johnson, L. R., Lippi, C. A., et al. (2017). Detecting the impact of temperature on transmission of Zika, dengue, and chikungunya using mechanistic models. PLoS neglected Trop. Dis. 11 (4), e0005568. doi:10.1371/journal.pntd.0005568
Nakase, T., Giovanetti, M., Obolski, U., and Lourenço, J. (2023). Global transmission suitability maps for dengue virus transmitted by Aedes aegypti from 1981 to 2019. Sci. Data 10 (1), 275. doi:10.1038/s41597-023-02170-7
O'Connor, C., O'Connell, N., Burke, E., Dempster, M., Graham, C. D., Scally, G., et al. (2021). Bordering on crisis: a qualitative analysis of focus group, social media, and news media perspectives on the Republic of Ireland-Northern Ireland border during the ‘first wave’of the COVID-19 pandemic. Soc. Sci. and Med. 282, 114111. doi:10.1016/j.socscimed.2021.114111
Pascoe, L., Clemen, T., Bradshaw, K., and Nyambo, D. (2022). Review of importance of weather and environmental variables in agent-based arbovirus models. Int. J. Environ. Res. public health 19 (23), 15578. doi:10.3390/ijerph192315578
Pem-Novosel, I., Vilibic-Cavlek, T., Gjenero-Margan, I., Kaic, B., Babic-Erceg, A., Merdic, E., et al. (2015). Dengue virus infection in Croatia: seroprevalence and entomological study. New Microbiol. 38 (1), 97–100.
Pernat, N., Zscheischler, J., Kampen, H., Ostermann-Miyashita, E. F., Jeschke, J. M., and Werner, D. (2022). How media presence triggers participation in citizen science—the case of the mosquito monitoring project ‘Mückenatlas. PLoS One 17 (2), e0262850. doi:10.1371/journal.pone.0262850
Roesch, F., Fajardo, A., Moratorio, G., and Vignuzzi, M. (2019). Usutu virus: an arbovirus on the rise. Viruses 11 (7), 640. doi:10.3390/v11070640
Tazerji, S. S., Nardini, R., Safdar, M., Shehata, A. A., and Duarte, P. M. (2022). An overview of anthropogenic actions as drivers for emerging and re-emerging zoonotic diseases. Pathogens 11 (11), 1376. doi:10.3390/pathogens11111376
Unni, S. K., Růžek, D., Chhatbar, C., Mishra, R., Johri, M. K., and Singh, S. K. (2011). Japanese encephalitis virus: from genome to infectome. Microbes Infect. 13 (4), 312–321. doi:10.1016/j.micinf.2011.01.002
Wallau, G. L., Abanda, N. N., Abbud, A., Abdella, S., Abera, A., Ahuka-Mundeke, S., et al. (2023). Arbovirus researchers unite: expanding genomic surveillance for an urgent global need. Lancet Glob. health 11 (10), e1501–e1502. doi:10.1016/S2214-109X(23)00325-X
Weaver, S. C. (2013). Urbanization and geographic expansion of zoonotic arboviral diseases: mechanisms and potential strategies for prevention. Trends Microbiol. 21 (8), 360–363. doi:10.1016/j.tim.2013.03.003
Keywords: arboviruses, Italy, open data, west nile, chikungunya, dengue, public health, surveillance
Citation: Branda F, Giovanetti M, Ceccarelli G, Ciccozzi M and Scarpa F (2024) ArboItaly: Leveraging open data for enhanced arbovirus surveillance in Italy. Front. Pharmacol. 15:1459408. doi: 10.3389/fphar.2024.1459408
Received: 04 July 2024; Accepted: 11 September 2024;
Published: 23 September 2024.
Edited by:
Chandra Sekhar Sirka, All India Institute of Medical Sciences Bhubaneswar, IndiaReviewed by:
Manoj Pradhan, National Institute of Science and Technology, IndiaCopyright © 2024 Branda, Giovanetti, Ceccarelli, Ciccozzi and Scarpa. This is an open-access article distributed under the terms of the Creative Commons Attribution License (CC BY). The use, distribution or reproduction in other forums is permitted, provided the original author(s) and the copyright owner(s) are credited and that the original publication in this journal is cited, in accordance with accepted academic practice. No use, distribution or reproduction is permitted which does not comply with these terms.
*Correspondence: Francesco Branda, Zi5icmFuZGFAdW5pY2FtcHVzLml0
Disclaimer: All claims expressed in this article are solely those of the authors and do not necessarily represent those of their affiliated organizations, or those of the publisher, the editors and the reviewers. Any product that may be evaluated in this article or claim that may be made by its manufacturer is not guaranteed or endorsed by the publisher.
Research integrity at Frontiers
Learn more about the work of our research integrity team to safeguard the quality of each article we publish.