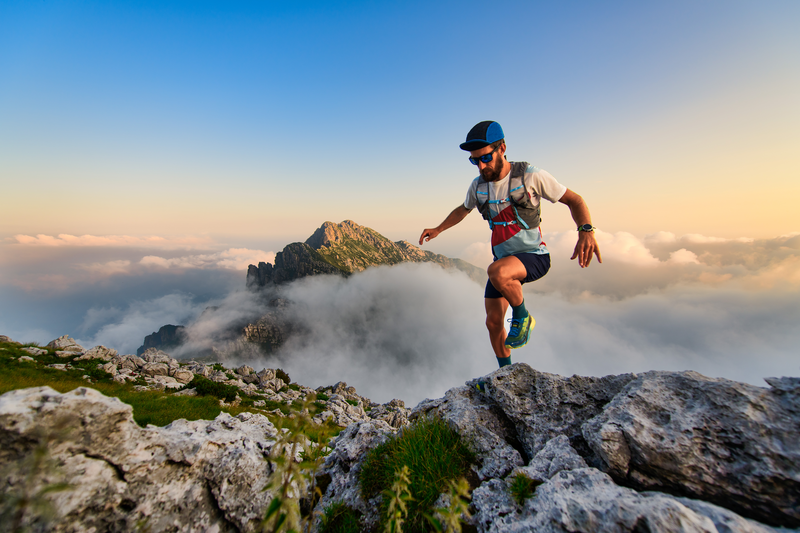
94% of researchers rate our articles as excellent or good
Learn more about the work of our research integrity team to safeguard the quality of each article we publish.
Find out more
REVIEW article
Front. Pharmacol. , 19 December 2024
Sec. Ethnopharmacology
Volume 15 - 2024 | https://doi.org/10.3389/fphar.2024.1454348
This article is part of the Research Topic Efficacy and Mechanism of Herbal Medicines and Their Functional Compounds in Preventing and Treating Cardiovascular Diseases and Cardiovascular Disease Risk Factors - Volume II View all 10 articles
Globally, cardiovascular diseases (CVDs) remain the leading cause of death, and their prevention and treatment continue to face major challenges. Oxylipins, as novel circulating markers of cardiovascular disease, are crucial mediators linking cardiovascular risk factors such as inflammation and platelet activation, and they play an important role in unraveling cardiovascular pathogenesis and therapeutic mechanisms. Chinese herbal medicine plays an important role in the adjuvant treatment of cardiovascular diseases, which has predominantly focused on the key pathways of classic lipids, inflammation, and oxidative stress to elucidate the therapeutic mechanisms of cardiovascular diseases. However,The regulatory effect of traditional Chinese medicine on oxylipins in cardiovascular diseases remains largely unknown. With the increasing number of recent reports on the regulation of oxylipins by Chinese herbal medicine in cardiovascular diseases, it is necessary to comprehensively elucidate the regulatory role of Chinese herbal medicine in cardiovascular diseases from the perspective of oxylipins. This approach not only benefits further research on the therapeutic targets of Chinese herbal medicine, but also brings new perspectives to the treatment of cardiovascular diseases.
The incidence of cardiovascular diseases is increasing worldwide. In 2021, cardiovascular diseases (CVDs) were responsible for the deaths of 20.5 million people globally, accounting for one-third of all global deaths, and standing as the leading cause of human mortality (World Heart Federation, 2023). CVDs remain a major public health issue worldwide, imposing a significant socioeconomic burden. This group of disorders encompasses a variety of heart diseases related to blood flow, circulation, and cardiac function, such as coronary artery disease, heart failure. (Roth et al., 2020). The pathogenesis of CVD involves a complex interplay of genetic, environmental, and lifestyle factors that contribute to the development of key pathological features such as inflammation (Henein et al., 2022), endothelial dysfunction (Xu et al., 2021), and thrombosis (Khodadi, 2020). These pathophysiological processes are mediated by a variety of biochemical and molecular mechanisms, including the dysregulation of lipid mediators (Manke et al., 2022), cytokines (Henein et al., 2022), and cellular adhesion molecules (Troncoso et al., 2021), making them critical targets for therapeutic intervention. As research deepens, researchers have found that cytokines and cellular adhesion molecules are both closely related to lipid mediators, making lipid mediators a key focus in the study of cardiovascular disease mechanisms.
Lipid mediators are important chemical substances in living organisms that can be converted into highly active substances through processes such as fatty acid oxidation. Among them, the oxidation products produced by lipid molecules through endogenous peroxidase and reactive oxygen species pathways are called oxylipins (Nayeem, 2018). These oxylipins play critical roles in modulating various biological processes, including inflammation, vascular endothelial dysfunction, and platelet aggregation (Caligiuri et al., 2017b; Nayeem, 2018). Oxylipins are integral to the regulation of cardiovascular function and pathology, influencing processes such as atherosclerosis (Gleim et al., 2012), hypertension (Gleim et al., 2012), heart failure (Lau et al., 2023) As such, oxylipins represent promising diagnostic tools and target for novel therapeutic approaches aimed at modulating these pathways to improve cardiovascular health (Shearer and Walker, 2018).
Traditional Chinese herbal medicine, which has been utilized for thousands of years in East Asia, is increasingly being integrated into standard biomedical treatments as a complementary or alternative treatment option for CVDs. Interestingly, it has been found that certain herbal medicines, including herbal monomers, extracts, and compound preparations, significantly regulate oxylipin products, their substrates, and the enzymes involved in their pathways (Fu et al., 2018; Zhi et al., 2021). These herbal medicines have also been shown to play an important role in cardioprotection and in slowing the progression of cardiovascular and cerebrovascular diseases in pharmacological studies. This suggests the great potential of Chinese medicines in treating cardiovascular diseases. Therefore, fully elucidating the mechanisms by which Chinese medicines exert cardioprotective effects from the perspective of oxylipins is essential. Such elucidation would facilitate further in-depth studies on the therapeutic mechanisms of Chinese medicines and promote the development of novel therapeutic strategies based on oxylipins, bringing new hope for the treatment of cardiovascular diseases. In this review, we will summarize relevant cellular, animal, and clinical trials, providing a comprehensive overview of the mechanism by which Chinese herbal medicine regulates oxylipins in cardiovascular disease.
Oxylipins are bioactive substances formed by the decomposition of polyunsaturated fatty acids (PUFAs) through spontaneous oxidation or enzymatic processes (Nayeem, 2018). Typically, free PUFAs are oxidized into oxylipins either via auto-oxidation, or through three main enzymatic pathways including lipoxygenase (LOX), cyclooxygenase (COX), and cytochrome P450 (CYP450) pathways. Free PUFAs like arachidonic acid (AA) can be converted into epoxyeicosatrienoic acids (EETs) by the CYP pathway, into hydroxyeicosatetraenoic acids (HETEs) by the CYP or LOX pathway, into leukotrienes (LTs) by the LOX pathway, or into prostanoids by the COX pathway; free PUFAs like linoleic acid (LA) can be metabolized into hydroxyoctadecadienoic acids (HODEs) by the LOX pathway, or into epoxymetabolites (EpoMEs) through the CYP epoxygenase pathway (Nayeem, 2018). (Detailed pathways of oxylipins formation are provided in Figure 1). Oxylipin disorder is commonly present in cardiovascular diseases (see Table 1). Oxylipins play diverse regulatory roles (For select examples of oxylipins with cardiovascular bioactive functions, see Table 2), exerting cardioprotective effects and maintaining vascular homeostasis through direct or indirect actions, such as interactions with oxylipin receptors and modulation of inflammation (Hernandez-Saavedra and Stanford, 2022).
EETs are synthesized in cardiomyocytes and endothelial cells (Campbell et al., 2017) through the metabolism of AA. Cytochrome P450 enzymes, such as CYP2J2, convert AA into four regioisomeric EETs: 5, 6-, 8, 9-, 11, 12-, and 14,15-EET, which are then rapidly hydrolyzed to the less active dihydroxyeicosatrienoic acids (DHETs) in the presence of soluble epoxide hydrolase (sEH) (Lai and Chen, 2021). EETs have a wide range of cardioprotective effects, including inhibiting cardiomyocyte hypertrophy, ameliorating apoptosis, inhibiting platelet adhesion to endothelial cells, and possessing anti-inflammatory properties (Krötz et al., 2004; Spector, 2009; Tacconelli and Patrignani, 2014). DHETs, as stable metabolites of EETs, reflect the concentration of EETs in circulation. These metabolites contribute to improved vascular endothelial function by increasing the expression of nitric oxide, which has been identified as the main chemical compound of endothelial-derived relaxation factors (Zuo et al., 2021).
In CYP2J knockout (KO) rats, plasma EET levels were significantly reduced, exacerbating myocardial inflammation, hypertrophy, and fibrosis. Additionally, myocardial injury became more severe with age in KO rats, corresponding with significant reductions in 11,12-EET and 14,15-EET levels in the plasma (Zhang Y. et al., 2023). Changes in EET or DHET were also observed in animal models of hyperlipidemia and acute myocardial infarction (AMI). Specifically, levels of 14, 15-DHET decreased in hyperlipidemic mice (Chen et al., 2022); and 5, 6-EET, 8, 9-EET, 14, 15-EET, 5, 6-DHET, 11, 12-EET, 14, 15-DHET showed a clear downward trend in post-myocardial infarction rats (Zhi et al., 2021). In large-scale clinical studies, coronary artery disease (CAD) patients exhibit elevated levels of plasma EETs (Theken et al., 2012) and 5, 6-EET is closely associated with blood pressure status (Palmu et al., 2020).
AA produces medium-chain HETEs, including 5-, 8-, 9-, 11-, 12-, and 15-HETEs, through allylic oxidation in the presence of LOX (Nayeem, 2018). In addition, AA is also metabolized by CYP450 enzymes, such as CYP4A and CYP4F, through allylic oxidation to produce terminal HETEs, including 16-, 17-, 18-, 20-HETE, etc. (Shoieb et al., 2019). Multiple cell types in the heart, including cardiomyocytes and fibroblasts, synthesize and respond to HETEs (Escoubet et al., 1986).
Unlike the effects of EETs, most medium-chain HETEs have pro-inflammatory and vasoconstrictive properties, and their formation can increase cardiovascular dysfunction (Nayeem, 2018; ElKhatib et al., 2023). HETEs also block the synthesis of EETs by RL-14 cells and increase the conversion of EETs to DHETs, affecting EET levels (Maayah et al., 2015). Among the medium-chain HETEs, 12- and 15-HETE are closely associated with the cardiovascular system (Breitbart et al., 1996; Huang et al., 2020). Both are produced through CYP in addition to the LOX pathway. 12-HETE has chemotactic properties, can alter vascular tone, and induce VEGF growth (Buchanan et al., 1998). Levels of 12-HETE were also significantly elevated in patients 24–40 h after acute myocardial infarction (Freeman et al., 2015). 15-HETE has been reported to have anti-inflammatory effects and can be converted to LXs, which play a role in reducing inflammation (Buckley et al., 2014). In large-scale clinical studies, patients with coronary artery disease who experienced an acute myocardial ischemic event within 2 years had elevated levels of 8-, 9-, 11-, 12-, and 15-HETE compared to those without a cardiovascular event (Huang et al., 2020). In hypertensive patients, elevated levels of 5-, 8-, 11-, 12-, and 15-HETE have also been observed (Xie et al., 2019; Feugray et al., 2022).
Among the terminal HETEs, 16-HETE, 18-HETE, and 19-HETE and metabolites of 20-HETE can promote vasodilation (Carroll et al., 1996; Caligiuri et al., 2017b). The main functions of 16-HETE include promoting vasodilation and inhibiting inflammation mediated by neutrophil adhesion (Reddy et al., 2003; Shoieb et al., 2019). The precise role of 20-HETE in the cardiovascular system is complex. Studies have shown that 20-HETE produced by CYP4A is an effective vasoconstrictor in mouse aortas and coronary arteries (Hoopes et al., 2015), but there is also research evidence suggesting that 20-HETE can promote the proliferation of endothelial cells through activation of the vascular endothelial growth factor (VEGF) pathway. In clinical trials, among acute coronary syndrome (ACS) patients, the main abnormalities in oxylipins concentrate in terminal HETEs, with higher levels of 19-HETE associated with better ACS prognosis (Solati and Ravandi, 2019). In AMI rats, levels of oxylipins such as 20-HETE increased, while 16-HETE and 18-HETE showed a decreased tendency post-myocardial infarction (Zhi et al., 2021).
However, it is worth noting that HETEs have a relatively short existence time and can be converted into oxoETE under the action of dehydrogenase. Among various oxoETEs, 5-oxoETE has been shown to have the effect of inducing myocardial injury (Lai et al., 2021); And 15-oxoETE has a hypotensive effect (Yu et al., 2023). In cell cultures, 15-oxoETE activates NRF2-regulated antioxidant responses and inhibits NF-κB-mediated pro-inflammatory responses (Snyder et al., 2015).
Leukotrienes (LTs) are formed from AA through the 5-LOX pathway, producing unstable epoxide metabolites LTA4, which are then metabolized by leukotriene A4 hydrolase (LTA4H) and leukotriene C4 synthase (LTC4S) into various metabolites like LTB4, LTC4, LTD4 (Hammarström, 1983). LTs play roles in various acute and chronic inflammations. Especially LTB4, which can induce the release of pro-inflammatory cytokines, recruit and infiltrate leukocytes, and promote inflammation (Das, 2021). LTC4 has been reported to be associated with pro-inflammatory activities, plaque formation, and myocardial ischemia (Nobili et al., 2012). In some animal experiments, researchers also observed changes in LTs. For example, serum LTB4 levels were significantly increased in hyperlipidemic rats (Wang Y. Q. et al., 2020). LTD4 showed high levels in spontaneously hypertensive rats, which may be related to the induction of inflammatory status (Jiang et al., 2015).
Prostaglandins (PGs) and thromboxanes (TXs) are collectively referred to as prostanoids. Prostanoids are a class of lipid mediators produced from AA through enzymatic metabolism. Under various physiological and pathological stimuli, AA is catalyzed by phospholipase A2 (PLA2), released from cell membrane phospholipids, and then converted into prostaglandin intermediates PGG2 and PGH under the activity of COX enzymes, which are then metabolized into various bioactive prostaglandins, including PGD2, PGE2, PGF2α, PGI2, TXA2, and others (Beccacece et al., 2023).
Among the different prostanoids, PGD2 is expressed in cells involved in immunity and inflammation (Beccacece et al., 2023). Both PGD2 and its main degradation product, 15-deoxy-∆-12, 14-Prostaglandin J2 (15d-PGJ2), play roles in inflammation resolution (Yousefnia et al., 2018). PGE2 is one of the most abundant prostaglandins and participates in all processes leading to inflammation (Nakanishi and Rosenberg, 2013). PGF2α is considered a vasoconstrictor of coronary arteries, associated with cardiac dysfunction and hypertrophy (Adams et al., 1998; Kuhn et al., 2015). PGI2 and TXA2 are considered the predominant prostaglandins of the cardiovascular system (Beccacece et al., 2023) produced by vascular endothelial cells and platelets, respectively, exerting opposite effects on vessels and platelets. PGI2 induces vasodilation and inhibits platelet aggregation (Ozen and Norel, 2017), TXA2 induces vasoconstriction and is a potent platelet agonist (Scridon, 2022). The balance between PGI2 and TXA2 is one of the key factors determining the homeostasis of the cardiovascular system. Clinical research shows that for every unit increase in the TXB2 (a metabolite of TXA2)/PGF1α (a downstream metabolite of PGI2) ratio, the likelihood of cardiovascular events increases (Caligiuri et al., 2017a).
Diseases such as thrombosis and atherosclerosis predominantly involve abnormal changes in prostanoids-type oxylipins, closely associated with the activation, adhesion, and aggregation of platelets. Atherosclerosis, a precursor to coronary artery disease and heart failure, involves lipid deposition and thrombus formation, leading to fibrous tissue proliferation and calcification (Tong et al., 2022). These processes thicken and harden the arterial walls, narrow the lumen, induce myocardial ischemia, and ultimately lead to heart failure. Research on oxylipins in atherosclerosis is limited, but increases in TXB2 levels and decreases in 6-keto-PGF1α have been noted (Zhang et al., 2013). Clinical studies have shown that oxylipins, including 11-dehydro-2, 3-dinor-TXB2 is closely associated with blood pressure status (Palmu et al., 2020). In hypertensive patients, elevated levels of TXs (TXA2) and reduced levels of prostanoids have also been observed (Jiang et al., 2015; Xie et al., 2019; Feugray et al., 2022). PGD2/PGE2 and 15d-PGJ2 are inversely correlated with CAD events (Chiang et al., 2022). Compared to healthy volunteers, CAD patients exhibit elevated levels of PGE2 (Theken et al., 2012). Similarly, prostanoids change post-myocardial infarction. Prostanoids like TXB2, TXA2, PGD2, PGE2, PGF2, and 15-Deoxy-PGJ2 rise, while 6-keto-PGF1α and PGI2 decrease (Cao et al., 2016; Li et al., 2016; Jiang et al., 2021; Zhi et al., 2021).
As the terminal stage of various cardiac diseases, heart failure is characterized by structural changes and functional impairments (Snipelisky et al., 2019). Abnormal oxylipins associated with heart failure reflect inflammatory responses, oxidative stress, myocardial fibrosis, and changes in cellular energy metabolism (González et al., 2018; Yin et al., 2019), with notable lipids such as PGE2 mediating inflammation, myocardial fibrosis, and cardiac cell apoptosis. Specifically, in terms of inflammation, prostaglandins like 15R-PGF2α, 11β-DHK-PGF2α, and PGE1 show high relevance to heart failure (Lau et al., 2023). As for myocardial fibrosis, the AA-COX pathway is essential for synthesizing prostanoids (e.g., PGI2, PGD2, PGE2, PGF2, and TXA2), representing the most extensively studied pathway in this area. Studies indicate that in heart failure models in rats, the expression of COX1 and COX2 are significantly upregulated, levels of 13, 14-dihydro-PGF2α, TXB2, PGE2, TXB2/6-keto-PGF1α rise significantly, while 6-keto-PGF1α decreases, concurrently with RAAS system activation, exacerbating the myocardial fibrosis process and inducing heart failure (Wang et al., 2015b).
EpOMEs are oxides generated by LA via the action of CYP cyclooxygenase. Among the family of EpOMEs, 9, 10-EpOMEs have cardiac inhibitory effects (Sugiyama et al., 1987), and 12, 13-EpOMEs have a higher correlation with heart failure (Lau et al., 2023). Both 9, 10-EpOME and 12, 13-EpOME exhibit elevated levels in CAD patients (Theken et al., 2012). sEH converts EpOMEs to DiHOMEs. Both EpOMEs and DiHOMEs are cytotoxic, cardiotoxic, and can mediate inflammation and vasoconstriction (Hildreth et al., 2020). In CYP2C8-Tie2 mice, hearts perfused in the Langendorff mode with 9, 10-DiHOME or 12, 13-DiHOME showed a reduced recovery rate from reperfusion injury and increased coronary artery resistance (Edin et al., 2011; Bannehr et al., 2019). However, there are also studies supporting the beneficial effects of 12, 13-EpOMEs and 12, 13-DiHOME (Bannehr et al., 2019). In one study, cardiac perfusion of rats with LA, 12, 13-EpOMEs, and 12, 13-DiHOME resulted in a moderate increase in cardiac contraction observed within 10–20 min, with the perfusion effect of 12, 13-DiHOME lasting into the washout period. Accordingly, it is suggested that the impact of EpOMEs and DiHOMEs on cardiovascular function is still uncertain, and the specific effects may be related to other factors such as the dose of oxylipins and the environment.
HODEs are secondary oxidation products of LA, mainly formed through hydroxylation by CYP epoxygenases. The role of HODE in cardiovascular disease remains unclear, as the results of current studies are not consistent. Common HODEs include 9-HODE and 13-HODE. Both 9-HODE and 13-HODE can regulate oxidative stress and inflammation (Folcik and Cathcart, 1994; Krämer et al., 1996; Marmol et al., 1999; Belvisi and Mitchell, 2009). 9-HODE mediates alterations in pro-inflammatory markers associated with chronic inflammation. One study showed that 9-HODE had an inflammation-inducing effect and regulated Forkhead box O nuclear levels through the c-Jun N-terminal protein kinases pathway, linking fatty acid homeostasis, inflammation, and insulin resistance (Kwon et al., 2020). In contrast to 9-HODE, 13-HODE has anti-inflammatory effects (Marmol et al., 1999; Belvisi and Mitchell, 2009), with 13-HODE levels negatively correlated with vascular wall adhesiveness, inhibiting thrombus formation on damaged vascular walls and exerting numerous beneficial effects on cardiovascular health (Buchanan et al., 1991).
Dehydrogenation of HODE by dehydrogenase results in the formation of oxo-ODE. In nested case-control studies of CAD patients, 13-oxo-ODE appears to have a harmful effect (Chiang et al., 2022). The metabolic disorders of HODE and oxo-ODE often occur in acute cardiovascular diseases, such as myocardial infarction (MI) and reperfusion injury after myocardial infarction. However, it is worth noting that HODE and oxo-ODE do not have the same metabolic disturbances in the same disease, probably due to the variability of animal experiments and clinical trials. In clinical trials of myocardial infarction and post-myocardial infarction reperfusion injury, the levels of 9-HODE, 13-HODE, 9-oxo-ODE, and 13-oxo-ODE showed varying degrees of decline (Li et al., 2016; Solati et al., 2023). However, in rats with myocardial infarction, the levels of all these oxylipins were increased (Zhi et al., 2021).
In China, several traditional Chinese medicine (TCM) formulations are commonly used as adjunct therapies for cardiovascular diseases, including the Xinyue Capsules and Danqi Tongmai Tablets, which are popular used in clinics (Zhi et al., 2021; Wang et al., 2023). Modern pharmacology has shown that these TCM formulations are beneficial for the treatment and prognosis of cardiovascular diseases. As research continues to deepen, the mechanisms by which TCM regulates oxylipins are being gradually revealed, especially in animal experiments and in vitro cell studies. Currently, the regulation of oxylipins in cardiovascular diseases by TCM mainly involves lipids such as EETs, HETEs, PGs, HODEs, and EpOMEs produced through the LOX, COX, and CYP pathways (refer to Table 3; Figure 2 for details).
Figure 2. Disrupted Oxylipins in Cardiovascular Disease and Oxylipins Targets in Chinese Herbal Medicine. This Sangi diagram is used to demonstrate the oxylipin targets of cardiovascular disease and Chinese herbal medicine, providing a comprehensive overview of the regulation of oxylipins by Chinese herbal medicine in cardiovascular disease.
TCM formulations, including Xinyue Capsules, Danqi Tongmai Tablets, and Erchen Decoction, regulate four abnormal types of EETs involved in cardiovascular diseases. Xinyue Capsules, a TCM used for treating cardiovascular diseases, primarily consist of Panax quinquefolius saponins (PQS) and are widely used as a supplementary antiplatelet therapy. Recent pharmacological studies have shown that PQS, in combination with antiplatelet drugs, have a synergistic protective effect on platelet adhesion to endothelial cells and reduce the bleeding risk associated with antiplatelet drugs (Wang et al., 2023). This effect is likely due to the activation of the AA-CYP-EET pathway, leading to increased EET synthesis, reduced platelet adhesion and aggregation, and elevated levels of 8, 9-DHET and 11, 12-DHET, thereby ameliorating the cardiac dilation observed in MI rats (Wang et al., 2023).
Danqi Tongmai Tablet, a TCM formula for treating blood stasis-type stable angina pectoris, mainly composed of Salvianolic acid extract and Panax notoginseng (Burk.) F. H. Chen. [Araliaceae], can increase the levels of 5, 6-EET, 8, 9-EET, 14, 15-EET, 5, 6-DHET, and 11, 12-DHET in the plasma of AMI rats through the CYP2C/2J pathway and downregulate pro-inflammatory cytokines, significantly improving the inflammatory state of AMI rats. The modulation of 5, 6-EET and 8, 9-EET by Danqi Tongmai Tablet at a concentration of 130 mg/kg was the most pronounced, revealing a dose-dependent regulation of arachidonic acid metabolites EET by Danqi Tongmai Tablet (Zhi et al., 2021).
The Erchen Decoction, composed of Pinellia ternata (Thunb.) Breit. [Araceae], Poria cocos (Schw.)Wolf. [Polyporaceae], Citrus reticulata Blanco. [Rutaceae], etc. (names based on the Chinese Pharmacopoeia and http://mpns.kew.org/mpns-portal/), has the effects of drying dampness and resolving phlegm, regulating qi, and harmonizing the middle. It is often used in the treatment of clinical lipid metabolism disorders (Li S. et al., 2020; Zhang L. et al., 2023). In the formula-disease correspondence group, the concentration of 14, 15-DHET was significantly increased, indicating that Erchen Decoction protected the vascular endothelium of mice with dyslipidemia and stasis syndrome by increasing serum 14, 15- DHET (Chen et al., 2022). Similarly, Qili Qiangxin Capsule, a TCM formula mainly composed of Panax ginseng C. A. Mey. [Araliaceae], Astragalus membranaceus (Fisch.) Bge. var.mongholicus (Bge.) Hsiao or Astragalus membranaceus (Fisch.) Bge. [Fabaceae] (Usually using Astragalus membranaceus (Fisch.) Bge. var.mongholicus (Bge.)Hsiao), Aconitum carmichaelii Debeaux. [Ranunculaceae], etc., could increase levels of 5, 6-DHET through the AA-CYP450 pathway, regulating inflammatory responses induced by heart failure (Fu et al., 2018).
Current research indicates that TCM regulation of HETEs primarily focuses on 5-, 12-, 15-, 16-, 17-, and 20-HETE. As previously mentioned, 5-, 12-, and 15-HETE all have harmful effects on the cardiovascular system. The main functions of 16-HETE include promoting vasodilation and inhibiting inflammation mediated by neutrophil adhesion. In AMI model rats, Danqi Tongmai Tablet can elevate levels of 16-HETE and 17-HETE through the CYP pathway and reduce levels of 5-, 12-, 15-HETE, and 5-, 15-oxoETE through the LOX pathway.
The exact role of 20-HETE in the cardiovascular system needs further exploration. Current research evidence indicates the beneficial effects of 20-HETE but also emphasizes its harm to the cardiovascular system. In dyslipidemic and stasis syndrome model mice (n-5C57BL/6J mice and n-30 apolipoprotein E knockout mice), the serum concentration of 20-HETE was reduced by Erchen Decoction, and the effect of Erchen Decoction on 20-HETE was also related to the prescription-syndrome correspondence. When the prescription-syndrome did not correspond, Erchen Decoction had no significant regulatory effect on 20-HETE compared to the model group (Chen et al., 2022). However, some studies support the beneficial effects of 20-HETE, highlighting its ability to promote endothelial cell proliferation via the VEGF pathway (Chen et al., 2012). Some TCMs, like Shexiang Baoxin Pill, a pill made from Moschus berezovskii Flerov or Moschus sifanicus Przewalski or Moschus moschiferus Linnaeus. [Cervidae] (Usually using M. berezovskii Flerov), Panax ginseng C. A. Mey. [Araliaceae], and Liquidambar orientalis Mill. [Hamamelidaceae R. Br.], etc., commonly used in China for the clinical treatment of angina. Shexiang Baoxin Pill can increase levels of 20-HETE and endothelial progenitor cells (EPCs) in rats with myocardial infarction, along with increasing VEGF expression. Conversely, HET0016 (a 20-HETE synthesis inhibitor) could partially weaken the effects of Shexiang Baoxin Pill on EPCs, VEGF, and angiogenesis. These results strongly suggest that the effects of Shexiang Baoxin Pill on angiogenesis in myocardial infarction are mediated by promoting 20-HETE-induced mobilization of EPCs and VEGF expression (Huang et al., 2017).
Danqi Tongmai Tablet can reduce LTB4 levels through the LOX5 pathway, significantly downregulate pro-inflammatory cytokines, and alleviate the inflammatory status of AMI model rats (Zhi et al., 2021). In spontaneously hypertensive rats, LTD4 levels were elevated, and the Chinese herbal formula Tengfu Jiangya Tablet, main constituents are Uncaria rhynchophylla (Miq.) Miq. ex Havil or Uncaria macrophylla Wall or Uncaria hirsuta Havil or Uncaria sinensis (Oliv.) Havil or Uncaria sessilifructus Roxb. [Rubiaceae] (Usually using Uncaria rhynchophylla (Miq.) Miq. ex Havil) and Raphanus sativus L. [Brassicaceae], with the effects of lowering blood pressure) could lower leukotriene LTD4 levels through AA metabolism, suggesting that the interaction between inflammation and hypertension could be one of the potential mechanisms by which leukotrienes exert cardiovascular protective effects (Jiang et al., 2015).
Various herbal formulas have regulatory effects on PGs/TXs oxylipins by COX pathway. For example, Danqi Tongmai Tablet can restore AA metabolism disorder, downregulate metabolic levels of PGD2, PGE2, PGF2α, 15-d-PGJ2, TXB2, and upregulate levels of 6-keto-PGF1α, PGE1, and PGI1 in AMI rats (Zhi et al., 2021). Danhong Injection, a Chinese herbal injection made from Salvia miltiorrhiza Bunge. [Lamiaceae] and Carthamus tinctorius L. [Asteraceae], is commonly used in the treatment of occlusive cardiovascular diseases (Zhang et al., 2019). It has been shown that Danhong Injection could relax aortic vessels, increase COX mRNA expression, raise 6-keto-PGF1α levels, and promote PGI2 release in vitro. Moreover, in hyperlipidemic model rats, Danhong Injection could increase PGE mRNA and 6-keto-PGF1α expression and decrease TXA2 levels, thereby inhibiting platelet aggregation (Wang et al., 2013; Fan et al., 2018). Salvianolic acid, the main component of traditional Chinese herbal medicine Salvia miltiorrhiza Bunge. [Lamiaceae], can reduce PGE2 levels and inhibit lipid peroxidation through the AA pathway (Li Y. P. et al., 2020).
In TCM formulations, Qishen Yiqi Dripping Pill, a TCM formula for treating blood stasis-type stable angina pectoris, mainly composed of Astragalus membranaceus (Fisch.) Bge. var.mongholicus (Bge.) Hsiao or Astragalus membranaceus (Fisch.) Bge. [Fabaceae] (Usually using Astragalus membranaceus (Fisch.) Bge. var.mongholicus (Bge.) Hsiao, Salvia miltiorrhiza Bunge. [Lamiaceae], Panax notoginseng (Burk.) F. H. Chen. [Araliaceae], etc., can lower NF-κB, COX2, and PGE2 receptor levels, alleviating the inflammatory state (Wang et al., 2015b). Under pathological conditions, inflammation stimulates the production of PGE2 through the COX2/mPGES-1 (membrane-associated prostaglandin E2 synthase 1) pathway, leading to macrophage activation in plaques and matrix metalloproteinase (MMP)2 and MMP9 via the cAMP-dependent pathway. MMPs can degrade the extracellular matrix in the fibrous cap, reducing its components, thinning the fibrous cap, making plaques unstable and prone to rupture, leading to acute ischemic events (Mezzetti, 2005; Sun and Li, 2018). In addition, PGE2 can activate G protein-coupled receptors EP2 and EP4, both of which are associated with vasodilation, stimulation of inflammation, and cardiac hypertrophy (Torres et al., 2015; Sun and Li, 2018; Wang et al., 2019). EP4 is also one of the most important receptors in human inflammation-related diseases (Takayama et al., 2002; Tang et al., 2012), playing a significant role in PGE2-dependent MMP expression. In ankylosing spondylitis patients with concomitant cardiovascular diseases, EP4 expression is much higher than in ankylosing spondylitis patients without clinical manifestations (Cipollone et al., 2005). Thus, it can be inferred that COX2, PGE2, and its receptors EP2, EP4, MMP play significant roles in cardiovascular events. Qishen Yiqi Dripping Pill downregulates AA levels through the AA-COX1/COX2-PGE2 pathway, inhibiting PGE2-mediated apoptosis, regulating PGE2 downstream metabolites EP2 and EP4, reducing MMP2 and MMP9 production, and downregulating p53 and FasL protein. Compared to the model group, the myocardial apoptosis rate in the Qishen Yiqi Dripping Pill group rats was decreased (Wang et al., 2015b; 2015a).
The cardiovascular protective mixture, a TCM formula made from Salvia miltiorrhiza Bunge. [Lamiaceae], Ligusticum chuanxiong Hort. [Apiaceae], and nine other blood-activating and stasis-removing herbs, can activate the proliferation of vascular endothelial cells in vitro, thereby promoting the synthesis and secretion of PGI2 and playing a protective role in the vascular endothelium (Tu et al., 2003). In the MI model established by left coronary artery ligation, the expression level of PGI2 mRNA decreased and TXA2 increased compared to the MI group. Shensong Yangxin Capsule (main constituents: Panax ginseng C. A. Mey. [Araliaceae], Nardostachys jatamansi DC. [Caprifoliaceae], Salvia miltiorrhiza Bunge. [Lamiaceae], etc., with the effects of benefiting qi and nourishing yin, activating blood and dredging collaterals). could increase plasma PGI2 mRNA levels in MI rabbits, reduce TXA2 levels, thereby balancing vasoconstriction and dilation (Jiang et al., 2021). Among the single herbs in Chinese medicine, Callicarpa nudiflora Hook. and Arn. [Lamiaceae], a herb that dispels blood stasis, reduces swelling, and stops bleeding, has an extract, 1,6-di-O-caffeoyl-β-D-glucopyranoside, that has been found to resist P2Y12 and TP2 receptors (prostaglandin-like), thereby inhibiting TXA2 synthesis (Fu et al., 2017). In MI model rats, Panax quinquefolius saponins combined with dual antiplatelet therapy (PQS + DAPT) significantly increased PGI2 synthesis, reduced platelet activation and thrombogenesis agonist TXA2 synthesis and TXB2 levels, while plasma levels of 6-keto-PGF1α increased tenfold, and 6,15-2keto-13,14-2H-PGF1α levels increased threefold. This suggests that the improvement in platelet inhibition by PQS + DAPT may be partly due to the upregulation of AA/PGI2 and the downregulation of AA/TXA2 metabolism (Wang et al., 2023). The downregulation of TXA2 metabolism by PQS + DAPT could be the basis for the enhanced anti-aggregation effect of the combined therapy, possibly helping to reduce cardiovascular events to some extent. Additionally, PGI2 can counteract the thrombotic properties of TXA2, further inhibiting platelet activation. Further analysis found that PGI2 is co-regulated by COX1 and COX2 produced by endothelial cells, which possess vasodilatory properties. In endothelial cells, the use of DAPT alone or in combination with PQS does not affect the expression of COX1 protein; however, it elicits distinct effects on the expression and biological activity of COX2 protein. When DAPT was used alone, COX2 protein expression decreased, and activity declined, whereas PQS + DAPT had no inhibitory effect on COX2 protein expression, and at the same time, COX2 protein activity was enhanced. TXA2 is synthesized in platelets through the COX1 pathway, possessing vasoconstrictive properties. In platelets, compared to the use of DAPT alone, PQS + DAPT showed no significant differences in the regulation of COX1 and COX2 expression and activity. Therefore, compared to the inhibitory effect of the platelet COX1/TXA2 pathway, the regulatory effect of the combined application of PQS with DAPT on the endothelial cell COX2/PGI2 pathway is more optimal (Wang et al., 2023).
Syringa pinnatifolia Hemsl. [Oleaceae] is a Chinese herb mainly produced in Mongolia, China, which has the effect of moving qi and relieving pain and is often made into powder or Chinese medicine formulations for the treatment of myocardial ischemia (Zhang et al., 2022). The peeled extract of Syringa pinnatifolia Hemsl. [Oleaceae] downregulated the expression of COX1 and COX2 expression in myocardial ischemia model mice (C57BL/6 mice). Specifically, the strongest inhibitory effects on COX2 and COX1 were observed when the drug concentrations of the extract of Syringa pinnatifolia Hemsl. [Oleaceae] were at 20–80 mg/kg and 80 mg/kg, respectively. In addition, Syringa pinnatifolia Hemsl. [Oleaceae] pretreatment slightly increased plasma levels and reduced TXB2 production, while the opposite was observed in protein homogenates. Interestingly, the 6-keto-PGF1α/TXB2 ratio in the Syringa pinnatifolia Hemsl. [Oleaceae] pretreatment group was strongly dose-dependent, approaching the observed values in the myocardial ischemia sham surgery group, and was significantly superior to the positive drug pretreatment group (Cao et al., 2016).
The balance between 6-keto-PGF1α and TXB2 maintains the homeostasis of the cardiovascular system. Some blood-activating Chinese medicines like aqueous extract of Crataegus Pinnatifida Bge. var. major N. E. Br. [Rosaceae], Danshensu, Leech powder, the formulas Qishen Granule (main constituents: Astragalus membranaceus (Fisch.) Bge. var.mongholicus (Bge.) Hsiao or Astragalus membranaceus (Fisch.) Bge. [Fabaceae] (Usually using Astragalus membranaceus (Fisch.) Bge. var.mongholicus (Bge.) Hsiao, Salvia miltiorrhiza Bunge. [Lamiaceae], Scrophularia ningpoensis Hemsl. [Scrophulariaceae], etc.), Shexiang Boxin pill, and Danqi Tablet (main constituents: Salvia miltiorrhiza Bunge. [Lamiaceae] and Panax notoginseng (Burk.) F. H. Chen. [Araliaceae]) have mechanisms similar to the peeled extract of Syringa pinnatifolia Hemsl. [Oleaceae]. These extracts are all capable of lowering plasma TXB2 levels, increasing plasma 6-keto-PGF1α levels, maintaining a relatively stable 6-keto-PGF1α/TXB2 ratio, reducing lipid peroxidation levels (Zhang et al., 2013; Wang et al., 2014; Yu et al., 2014; Li et al., 2016; Li J. et al., 2020; Huang et al., 2017; Jiang et al., 2019).
In rats with heart failure, levels of LA-produced 9, 10-EpOME and 12, 13-EpOME were elevated, and Qili Qiangxin Capsule reduced these oxylipin levels while maintaining stable levels of 9, 10-DiHOME, thereby controlling cardiac hypertrophy and inflammation associated with heart failure (Fu et al., 2018).
TCM has been shown to regulate two common types of HODEs. Interestingly, in a study involving Danqi Tongmai Tablets, these tablets significantly reduced the levels of the pro-inflammatory lipid 9-HODE and the anti-inflammatory lipid 13-HODE in rats with myocardial infarction compared to those in acute myocardial infarction and sham operation groups. The levels of their downstream metabolites, 9-OxoODE and 13-OxoODE, were also significantly lowered (Zhi et al., 2021). However, the inflammation status of MI in the Danqi Tongmai Tablet group was still improved, suggesting that Danqi Tongmai Tablet may have a stronger regulatory effect on anti-inflammatory oxylipins, though further research is needed to confirm these results. Similarly, in rats with heart failure, Qili Qiangxin Capsule significantly lowered downstream metabolites of 9-HODE and 13-HODE, such as 9-OxoODE, 13-OxoODE, 12-, 13-DiHODE, and 9-, 10-, 13-TriHOME (Fu et al., 2018), suggesting a regulatory mechanism for HODE metabolites similar to that of Danqi Tongmai Tablet.
Currently, in the research of TCM in treating cardiovascular diseases, some studies have preliminarily revealed the effects of TCM on upstream substrates for oxylipin generation and related enzymes (including PLA2 and COX), such as the formula Xinkeshu Capsules (main constituents: Salvia miltiorrhiza Bunge. [Lamiaceae] and Pueraria lobata (Willd.) Ohwi. [Fabaceae], used for common cardiovascular diseases such as coronary heart disease, angina, hypertension, arrhythmia, and hyperlipidemia), Huanglian Jiedu Tang (main constituents: Coptis chinensis Franch. [Ranunculaceae], Scutellaria baicalensis Georgi. [Lamiaceae], Phellodendron chinense Schneid. [Rutaceae], etc., commonly used for febrile diseases), and Yixin Shu (main constituents: Salvia miltiorrhiza Bunge. [Lamiaceae], Panax ginseng C. A. Mey. [Araliaceae], Ophiopogon japonicus (L.f). Ker Gawl. [Liliaceae], etc., commonly used to treat coronary heart disease).
The mechanism of Xinke Shu Capsules in treating myocardial infarction involves inhibiting fatty acid β-oxidation, specifically manifested as reversing phospholipase A2ⅡA and regulating the levels of LA and AA in myocardial tissue (Sun et al., 2021). Huanglian Jiedu Decoction and Yixin Shu can restore AA levels in rats with heart failure (Xu et al., 2019; Yuan et al., 2020).
The COX/LOX pathway is an important route for LA and AA to be converted into oxylipins. Studies have shown that some Chinese medicinal herbs can regulate COX and LOX in animals with cardiovascular disease models, thereby improving myocardial injury and protecting the heart. Danshen Dripping Pill downregulates AA metabolism through the AT1-mediated PLA2-COX2/5-LOX metabolic pathway, inhibits RAAS system activation and MMPs expression, and thus inhibits myocardial fibrosis in myocardial failure rats (Zhang et al., 2018). In coronary heart disease models, Danqi pill downregulates the expression of PLA2, COX2, NF-κB on the inflammatory pathway, significantly upregulating PPARα levels on both gene and protein expression levels (Chang et al., 2016). Danqi Tongmai Tablet and Sanhuang Xiexin Decoction (A decoction composed of Rheum palmatum L or Rheum tanguticum Maxim. ex Balf or Rheum officinale Baill. [Polygonaceae] (Usually using Rheum officinale Baill), Coptis chinensis Franch. [Ranunculaceae], Scutellaria baicalensis Georgi. [Lamiaceae], and has the effects of purging fire, detoxifying, and relieving heat). Affected the gene and protein expression levels of COX mRNA, but with different effects. In the myocardial cell oxidative-glucose deprivation/reoxygenation model, Danqi Tongmai Tablet can increase COX2 mRNA, and reduce ALOX5 mRNA levels, alleviating oxidative damage in myocardial cells; in the atherosclerosis model, Sanhuang Xiexin Decoction downregulates COX2 gene expression and protein levels (Wang et al., 2011; Zhi et al., 2021).
The CYP pathway is another route for the conversion of polyunsaturated fatty acids into oxylipins, and many studies have demonstrated the regulatory effects of Chinese medicinal herbs on a variety of CYP enzymes, especially the CYP450 metabolic pathway (Gu et al., 2014; Wang et al., 2018). The cardiovascular protective effect of Pueraria lobata (Willd.) Ohwi. [Fabaceae] may be related to the inhibition of CYP2B6, CYP2C9, and CYP3A4 enzymes (Gu et al., 2014). EETs can inhibit tissue factor TF expression and prevent thrombosis, which is related to the activation of the PI3K/AKT pathway to inhibit NF-κB nuclear translocation and target CYP cyclooxygenase (Luo et al., 2023). Salvia miltiorrhiza Bunge. [Lamiaceae], a commonly used TCM for treating cardiovascular diseases with blood-activating and stasis-resolving effects, has Tanshinone IIA as its main component. Data mining revealed its potential therapeutic targets might lie in CYP450 3A4, CYP450 A1, and NF-κB1, which may exert an anti-inflammatory and cardiovascular protective effect (Chen, 2015). Ophiopogon D, the main pharmacologically active component of Shenmai Injection, has been used to prevent and treat cardiovascular diseases. Ophiopogon D can alleviate myocardial hypertrophy and inflammation through the CYP450 2J3-NF-κB pathway (Wang et al., 2018).
Most of the Chinese herbal medicines mentioned above are adjunctive therapeutic drugs for CVDs and have good therapeutic effects. Given the important role of oxylipins in CVDs, it is necessary to analyze the regulatory effect of traditional Chinese herbal medicine on oxylipins in CVDs using oxylipins as a therapeutic target. Our review found that traditional Chinese herbal medicine has regulatory effects on the oxylipins’ substrate, oxylipins, and downstream metabolites of oxylipins, indicating the enormous potential of traditional Chinese herbal medicine in the treatment of cardiovascular diseases. Further exploration is needed to explore the regulatory effects of more Chinese herbal medicines on oxylipins.
From the perspective of Chinese medicine involved in regulating oxylipins, we have conducted a frequency analysis of the involved Chinese medicines and found that the four most frequently mentioned are Salvia miltiorrhiza Bunge. [Lamiaceae] (Danshen in Chinese), Panax notoginseng (Burk.) F. H. Chen. [Araliaceae] (Sanqi in Chinese), Panax ginseng C. A. Mey. [Araliaceae] (Renshen in Chinese), and Astragalus membranaceus (Fisch.) Bge. var.mongholicus (Bge.) Hsiao or Astragalus membranaceus (Fisch.) Bge. [Fabaceae] (Huangqi in Chinese) (details are provided in the Supplementary Appendix. We have previously mentioned that AA can produce oxylipins such as prostanoids through the COX pathway. Prostanoids are closely related to platelet aggregation, endothelial cell adhesion, and vascular permeability. Some studies have shown that AA-COX-prostanoids are one of the most extensively studied pathways in the COX pathway, closely related to myocardial fibrosis. Salvia miltiorrhiza Bunge. [Lamiaceae] is a traditional Chinese herb that promotes blood circulation and improves cardiac fibrosis. In the systematic evaluation of the bioactive components of Salvia miltiorrhiza Bunge. [Lamiaceae] in the treatment of thrombotic diseases, Tanshinone, and Salvianolic acids are considered the main active components, including but not limited to Salvianolic Acid B, Tanshinone IIA, and Danshensu (Wei et al., 2023). From Table 2, we know that current research on Chinese medicine has revealed the regulatory effects of Danshensu and Salvianolic acids on oxylipins. Danshensu and Salvianolic acids target the AA-COX-prostanoids signaling pathway in thrombosis and coronary heart disease, which to some extent explains the anti-fibrotic effect of Salvia miltiorrhiza Bunge. [Lamiaceae]. Moreover, we also found that frequently used Chinese medicines Panax notoginseng (Burk.) F. H. Chen. [Araliaceae] and Panax ginseng C. A. Mey. [Araliaceae] belong to the same genus and have similar active components such as Ginsenoside Rg3 (Wang et al., 2022). Modern pharmacology shows that Ginsenoside Rg3 has antioxidant, anti-inflammatory, antihypertensive, and myocardial ischemia-reperfusion injury preventive effects, which may explain why different Chinese medicines have similar cardiovascular effects in regulating oxylipins (Liu et al., 2020; Wang et al., 2022). Furthermore, this leads us to further speculate that components such as Salvianolic acids and Ginsenoside Rg3, representative of Chinese medicine, may be effective tools for regulating oxylipins, awaiting further research confirmation.
Furthermore, from the current research on TCM, we find that the study of Chinese medicine regulating lipid oxidation not only involves lipid oxidation itself but also its upstream and downstream metabolites. Upstream metabolites of lipid oxidation, such as AA, LA, cPLA, LOX, etc., are closely related to the production of oxylipins and are themselves associated with platelet activation and other cardiovascular risk factors (Yeung et al., 2014), which may play a coordinated role in regulating cardiovascular function with oxylipins. However, it is worth mentioning that the upstream regulatory mechanism of oxylipins usually involves signal cell transduction, and genetic and epigenetic factors in addition to related enzymes and substrates. Various signaling pathways that activate phospholipases can increase the release of free fatty acids from cell membranes, thereby providing substrates for oxylipin synthesis. Hormones, growth factors, and cytokines are examples of signaling molecules that can modulate enzyme expression and activity in oxylipin pathways. Genetic polymorphisms and mutations in genes encoding oxylipin-synthesizing enzymes can significantly affect their expression and function. Additionally, epigenetic modifications such as DNA methylation and histone acetylation can alter gene expression in response to environmental signals. However, there is currently no research on signal cell transduction and genetic correlation in current studies. Looking at the downstream metabolites of oxylipins, they may involve other pathways such as myocardial cell apoptosis, inflammation, and myocardial fibrosis. For example, PGE2 is produced by AA through COX metabolism and has pro-inflammatory and apoptotic effects. The specific mechanism may be related to PGE2-mediated P53 and FasL. TCM has a regulatory effect on the AA-COX-prostanoids-P53/FasL pathway in cardiovascular diseases. For instance, Qishen Yiqi Drop Pills improve myocardial cells by reducing the expression of COX and PGE2 receptors, downregulating PGE2-mediated P53 and FasL proteins; similarly, there is also the PPAR inflammation pathway, where Chinese medicine Danqi Pill can regulate the PPARα/NF-kB signaling pathway related to inflammation, improving the inflammation state mediated by AA. In addition, a large number of oxylipins like 15-HETE, PGJ2, etc., seem to be able to activate PPAR (Chen et al., 2003; Li J. et al., 2019), thereby regulating platelet activation. From the perspective of upstream and downstream metabolites, the mechanism of action of oxylipins in TCM treatment of cardiovascular diseases needs further research to fill the research gap.
Currently, significant progress has been made in the study of the mechanisms by which TCM regulates oxylipins in cardiovascular diseases, yet certain shortcomings persist: (1) Oxylipins have diverse and complex chemical structures, making their detection and analysis methods particularly important. Traditional laboratory methods, such as UV visible and fluorescence spectrophotometry, nuclear magnetic resonance, chemiluminescence analysis, immunoassay, etc., have made some progress in the characterization and quantification of oxidized lipids, but they are very limited (Li L. et al., 2019; Kodali et al., 2020). In recent years, with the advancement of mass spectrometry technology, research on oxylipins in biological samples has been promoted. The combination of mass spectrometry and liquid chromatography can selectively identify individual compounds or compound groups with common characteristics, greatly improving the sensitivity and specificity of detection (Spickett and Pitt, 2015). Thus far, over 100 oxylipins have been identified. Regrettably, most of the experimental techniques employed in the study of TCM regulation of oxylipins still rely on the ELISA method, with only a few studies utilizing lipidomics techniques. Future research in TCM needs to adapt faster to technological developments, selecting new and appropriate lipid oxidation technologies for detection based on the focused lipid oxidation categories, which is conducive to obtaining consistent, reproducible, and reliable lipid oxidation omics results. Additionally, it should be noted that there are still some shortcomings in the current TCM studies using oxidative lipidomics techniques. These studies usually only focus on oxygenases related to inflammation and platelets, rather than the full spectrum. The lipid profiles used in TCM research include up to 71 oxylipins (Fu et al., 2018), while others include 38 oxylipins (Zhi et al., 2021), which is not conducive to a comprehensive revelation of TCM’s regulatory mechanisms and its cardiovascular effects. For instance, current research reveals a predominant focus on the AA-COX-prostanoids pathway in most TCM studies, a focus that may reflect researchers’ subjective bias. Thus, incorporating the full spectrum of oxylipins in TCM research is imperative. (2) Studies on the regulation of oxylipins by TCM primarily focus on metabolites produced by ω-6 PUFA (AA, LA), with less attention to those derived from ω-3 PUFA. The reason might be that the cardiovascular effects of most ω-3 PUFA-derived oxylipins remain unclear, although research has shown that the risk of cardiovascular diseases is reduced after intake of ω-3 PUFAs and their derivatives. Still, the specific cardiovascular effects of these oxylipins require further study to clarify their roles and regulatory mechanisms in cardiovascular diseases. (3) Current studies on the abnormal regulation of oxylipins in cardiovascular diseases by TCM are primarily based on animal experiments, which yield relatively uniform results, but there is a lack of related clinical studies, particularly large-scale clinical evidence. It remains uncertain whether the regulatory effects of Chinese herbal medicine on oxylipins in cardiovascular patients mirror those observed in animal studies. Also, further research is needed to investigate the impact of Chinese herbal monomers or individual herbs on oxylipins in cardiovascular disease. We also urge more researchers to focus on the significant role of oxylipins in cardiovascular disease and to conduct large-scale, long-term, high-quality clinical studies on Chinese herbal medicine. Such efforts would provide clearer insights into the regulatory effects and safety of Chinese herbal medicine on oxylipins, enhance the robustness of the data, and increase the potential for broader clinical application.
MqL: Conceptualization, Methodology, Writing–original draft. MH: Conceptualization, Methodology, Writing–original draft, Funding acquisition. MS: Data curation, Investigation, Writing–review and editing. YL: Resources, Visualization, Writing–review and editing. MyL: Project administration, Writing–review and editing. XJ: Resources, Visualization, Writing–review and editing. YW: Supervision, Writing–review and editing. HW: Funding acquisition, Writing–review and editing.
The author(s) declare that financial support was received for the research, authorship, and/or publication of this article. This study was supported by grants from the National Key R&D Program of China (2022YFC3500705). MH appreciates the support by the Open Scientific Project of Institute of Basic Theory for Chinese Medicine, China Academy of Chinese Medical Sciences (No. YZX-202207).
The authors would like to express their gratitude to Dychart for providing us with an online platform for image production.
Author YL was employed by Changchun Sino-Russian Science and Technology Park Co., Ltd.
The remaining authors declare that the research was conducted in the absence of any commercial or financial relationships that could be construed as a potential conflict of interest.
All claims expressed in this article are solely those of the authors and do not necessarily represent those of their affiliated organizations, or those of the publisher, the editors and the reviewers. Any product that may be evaluated in this article, or claim that may be made by its manufacturer, is not guaranteed or endorsed by the publisher.
The Supplementary Material for this article can be found online at: https://www.frontiersin.org/articles/10.3389/fphar.2024.1454348/full#supplementary-material
Adams, J. W., Sah, V. P., Henderson, S. A., and Brown, J. H. (1998). Tyrosine kinase and c-Jun NH2-terminal kinase mediate hypertrophic responses to prostaglandin F2alpha in cultured neonatal rat ventricular myocytes. Circ. Res. 83, 167–178. doi:10.1161/01.res.83.2.167
Alonso-Galicia, M., Falck, J. R., Reddy, K. M., and Roman, R. J. (1999). 20-HETE agonists and antagonists in the renal circulation. Am. J. Physiol. 277, F790–F796. doi:10.1152/ajprenal.1999.277.5.F790
Bannehr, M., Löhr, L., Gelep, J., Haverkamp, W., Schunck, W.-H., Gollasch, M., et al. (2019). Linoleic acid metabolite DiHOME decreases post-ischemic cardiac recovery in murine hearts. Cardiovasc Toxicol. 19, 365–371. doi:10.1007/s12012-019-09508-x
Beccacece, L., Abondio, P., Bini, C., Pelotti, S., and Luiselli, D. (2023). The link between prostanoids and cardiovascular diseases. Int. J. Mol. Sci. 24, 4193. doi:10.3390/ijms24044193
Belvisi, M. G., and Mitchell, J. A. (2009). Targeting PPAR receptors in the airway for the treatment of inflammatory lung disease. Br. J. Pharmacol. 158, 994–1003. doi:10.1111/j.1476-5381.2009.00373.x
Breitbart, E., Sofer, Y., Shainberg, A., and Grossman, S. (1996). Lipoxygenase activity in heart cells. FEBS Lett. 395, 148–152. doi:10.1016/0014-5793(96)01017-4
Brinkman, H. J., van Buul-Wortelboer, M. F., and van Mourik, J. A. (1990). Involvement of cyclooxygenase- and lipoxygenase-mediated conversion of arachidonic acid in controlling human vascular smooth muscle cell proliferation. Thromb. Haemost. 63, 291–297. doi:10.1055/s-0038-1645212
Buchanan, M. R., Bertomeu, M. C., Brister, S. J., and Haas, T. A. (1991). 13-Hydroxyoctadecadienoic acid (13-HODE) metabolism and endothelial cell adhesion molecule expression: effect on platelet vessel wall adhesion. Wien Klin. Wochenschr 103, 416–421.
Buchanan, M. R., Horsewood, P., and Brister, S. J. (1998). Regulation of endothelial cell and platelet receptor-ligand binding by the 12- and 15-lipoxygenase monohydroxides, 12-15-HETE and 13-HODE. Prostagl. Leukot. Essent. Fat. Acids 58, 339–346. doi:10.1016/s0952-3278(98)90069-2
Buckley, C. D., Gilroy, D. W., and Serhan, C. N. (2014). Proresolving lipid mediators and mechanisms in the resolution of acute inflammation. Immunity 40, 315–327. doi:10.1016/j.immuni.2014.02.009
Caligiuri, S. P. B., Aukema, H. M., Ravandi, A., Lavallée, R., Guzman, R., and Pierce, G. N. (2017a). Specific plasma oxylipins increase the odds of cardiovascular and cerebrovascular events in patients with peripheral artery disease. Can. J. Physiol. Pharmacol. 95, 961–968. doi:10.1139/cjpp-2016-0615
Caligiuri, S. P. B., Parikh, M., Stamenkovic, A., Pierce, G. N., and Aukema, H. M. (2017b). Dietary modulation of oxylipins in cardiovascular disease and aging. Am. J. Physiol. Heart Circ. Physiol. 313, H903–H918. doi:10.1152/ajpheart.00201.2017
Campbell, W. B., Imig, J. D., Schmitz, J. M., and Falck, J. R. (2017). Orally active epoxyeicosatrienoic acid analogs. J. Cardiovasc Pharmacol. 70, 211–224. doi:10.1097/FJC.0000000000000523
Cao, D., Xu, C. C., Xue, Y. Y., Ruan, Q. F., Yang, B., Liu, Z. Q., et al. (2018). The therapeutic effect of Ilex pubescens extract on blood stasis model rats according to serum metabolomics. J. Ethnopharmacol. 227, 18–28. doi:10.1016/j.jep.2018.08.026
Cao, Y., Wang, J., Su, G., Wu, Y., Bai, R., Zhang, Q., et al. (2016). Anti-myocardial ischemia effect of Syringa pinnatifolia Hemsl. by inhibiting expression of cyclooxygenase-1 and -2 in myocardial tissues of mice. J. Ethnopharmacol. 187, 259–268. doi:10.1016/j.jep.2016.04.039
Carroll, M. A., Balazy, M., Margiotta, P., Huang, D. D., Falck, J. R., and McGiff, J. C. (1996). Cytochrome P-450-dependent HETEs: profile of biological activity and stimulation by vasoactive peptides. Am. J. Physiol. 271, R863–R869. doi:10.1152/ajpregu.1996.271.4.R863
Chang, H., Wang, Q., Shi, T., Huo, K., Li, C., Zhang, Q., et al. (2016). Effect of DanQi Pill on PPARα, lipid disorders and arachidonic acid pathway in rat model of coronary heart disease. BMC Complement. Altern. Med. 16, 103. doi:10.1186/s12906-016-1083-3
Chen, G. G., Xu, H., Lee, J. F. Y., Subramaniam, M., Leung, K. L., Wang, S. H., et al. (2003). 15-hydroxy-eicosatetraenoic acid arrests growth of colorectal cancer cells via a peroxisome proliferator-activated receptor gamma-dependent pathway. Int. J. Cancer 107, 837–843. doi:10.1002/ijc.11447
Chen, J., Ye, C., Yang, Z., Li, P., Wu, H., Xu, B., et al. (2022). Effects of erchen decoction on oxidative stress-related cytochrome P450 metabolites of arachidonic acid in dyslipidemic mice with phlegm-dampness retention syndrome: a randomized, controlled trial on the correspondence between prescription and syndrome. Evid. Based Complement. Altern. Med. 2022, 1079803. doi:10.1155/2022/1079803
Chen, L., Ackerman, R., and Guo, A. M. (2012). 20-HETE in neovascularization. Prostagl. Other Lipid Mediat 98, 63–68. doi:10.1016/j.prostaglandins.2011.12.005
Chen, S. J. (2015). Drug-target networks for Tanshinone IIA identified by data mining. Chin. J. Nat. Med. 13, 751–759. doi:10.1016/S1875-5364(15)30075-3
Chiang, K.-M., Chen, J.-F., Yang, C.-A., Xiu, L., Yang, H.-C., Shyur, L.-F., et al. (2022). Identification of serum oxylipins associated with the development of coronary artery disease: a nested case-control study. Metabolites 12, 495. doi:10.3390/metabo12060495
Cipollone, F. (2008). The balance between PGD synthase and PGE synthase is a major determinant of atherosclerotic plaque instability in humans. Arterioscler. Thromb. Vasc. Biol. 28, e1. doi:10.1161/ATVBAHA.107.158881
Cipollone, F., Fazia, M. L., Iezzi, A., Cuccurullo, C., De Cesare, D., Ucchino, S., et al. (2005). Association between prostaglandin E receptor subtype EP4 overexpression and unstable phenotype in atherosclerotic plaques in human. Arterioscler. Thromb. Vasc. Biol. 25, 1925–1931. doi:10.1161/01.ATV.0000177814.41505.41
Dang, X., Miao, J.-J., Chen, A.-Q., Li, P., Chen, L., Liang, J.-R., et al. (2015). The antithrombotic effect of RSNK in blood-stasis model rats. J. Ethnopharmacol. 173, 266–272. doi:10.1016/j.jep.2015.06.030
Das, U. N. (2021). Essential fatty acids and their metabolites in the pathobiology of inflammation and its resolution. Biomolecules 11, 1873. doi:10.3390/biom11121873
Edin, M. L., Wang, Z., Bradbury, J. A., Graves, J. P., Lih, F. B., DeGraff, L. M., et al. (2011). Endothelial expression of human cytochrome P450 epoxygenase CYP2C8 increases susceptibility to ischemia-reperfusion injury in isolated mouse heart. FASEB J. 25, 3436–3447. doi:10.1096/fj.11-188300
ElKhatib, M., Isse, F. A., and El-Kadi, A. (2023). Effect of inflammation on cytochrome P450-mediated arachidonic acid metabolism and the consequences on cardiac hypertrophy. Drug Metab. Rev. 55, 50–74. doi:10.1080/03602532.2022.2162075
El-Sherbeni, A. A., and El-Kadi, A. O. S. (2014). Alterations in cytochrome P450-derived arachidonic acid metabolism during pressure overload-induced cardiac hypertrophy. Biochem. Pharmacol. 87, 456–466. doi:10.1016/j.bcp.2013.11.015
Escoubet, B., Griffaton, G., and Lechat, P. (1986). Verapamil depresses the synthesis of lipoxygenase products by hypoxic cardiac rat fibroblasts in culture. Biochem. Pharmacol. 35, 1879–1882. doi:10.1016/0006-2952(86)90306-0
Fan, H. J., Li, M., Yu, L., Jin, W. F., Yang, J. H., Zhang, Y. Y., et al. (2018). Effects of Danhong Injection on platelet aggregation in hyperlipidemia rats. J. Ethnopharmacol. 212, 67–73. doi:10.1016/j.jep.2017.10.017
Feugray, G., Pereira, T., Iacob, M., Moreau-Grangé, L., Prévost, G., Brunel, V., et al. (2022). Determination of lipoxygenase, CYP450, and non-enzymatic metabolites of arachidonic acid in essential hypertension and type 2 diabetes. Metabolites 12, 859. doi:10.3390/metabo12090859
Folcik, V. A., and Cathcart, M. K. (1994). Predominance of esterified hydroperoxy-linoleic acid in human monocyte-oxidized LDL. J. Lipid Res. 35, 1570–1582. doi:10.1016/s0022-2275(20)41155-1
Freeman, P. M., Moschonas, K. E., Hinz, C., O’Donnell, V. B., Kinnaird, T. D., James, P. E., et al. (2015). Changes in platelet function independent of pharmacotherapy following coronary intervention in non-ST-elevation myocardial infarction patients. Atherosclerosis 243, 320–327. doi:10.1016/j.atherosclerosis.2015.09.024
Fu, J., Chang, L., Harms, A. C., Jia, Z., Wang, H., Wei, C., et al. (2018). A metabolomics study of Qiliqiangxin in a rat model of heart failure: a reverse pharmacology approach. Sci. Rep. 8, 3688. doi:10.1038/s41598-018-22074-6
Fu, J. J., Zhu, X. C., Wang, W., Lu, H., Zhang, Z. M., Liu, T., et al. (2017). 1, 6-di-O-caffeoyl-β-D-glucopyranoside, a natural compound from Callicarpa nudiflora Hook impairs P2Y12 and thromboxane A2 receptor-mediated amplification of platelet activation and aggregation. Phytomedicine 36, 273–282. doi:10.1016/j.phymed.2017.10.012
Gleim, S., Stitham, J., Tang, W. H., Martin, K. A., and Hwa, J. (2012). An eicosanoid-centric view of atherothrombotic risk factors. Cell Mol. Life Sci. 69, 3361–3380. doi:10.1007/s00018-012-0982-9
González, A., Schelbert, E. B., Díez, J., and Butler, J. (2018). Myocardial interstitial fibrosis in heart failure: biological and translational perspectives. J. Am. Coll. Cardiol. 71, 1696–1706. doi:10.1016/j.jacc.2018.02.021
Gu, Y. J., Liang, D. L., Xu, Z. S., and Ye, Q. (2014). In vivo inhibitory effects of puerarin on selected rat cytochrome P450 isoenzymes. Pharmazie 69, 367–370. doi:10.1691/ph.2014.3823
Hammarström, S. (1983). Leukotrienes. Annu. Rev. Biochem. 52, 355–377. doi:10.1146/annurev.bi.52.070183.002035
Henein, M. Y., Vancheri, S., Longo, G., and Vancheri, F. (2022). The role of inflammation in cardiovascular disease. Int. J. Mol. Sci. 23, 12906. doi:10.3390/ijms232112906
Hernandez-Saavedra, D., and Stanford, K. I. (2022). “Lipid mediators in cardiovascular physiology and disease,” in Cardiovascular signaling in health and disease. Editors N. L. Parinandi, and T. J. Hund (Cham (CH): Springer), 235–258. doi:10.1007/978-3-031-08309-9_8
Hildreth, K., Kodani, S. D., Hammock, B. D., and Zhao, L. (2020). Cytochrome P450-derived linoleic acid metabolites EpOMEs and DiHOMEs: a review of recent studies. J. Nutr. Biochem. 86, 108484. doi:10.1016/j.jnutbio.2020.108484
Hoopes, S. L., Garcia, V., Edin, M. L., Schwartzman, M. L., and Zeldin, D. C. (2015). Vascular actions of 20-HETE. Prostagl. Other Lipid Mediat 120, 9–16. doi:10.1016/j.prostaglandins.2015.03.002
Huang, C., Chang, M., Leu, H., Yin, W., Tseng, W., Wu, Y. W., et al. (2020). Association of arachidonic acid-derived lipid mediators with subsequent onset of acute myocardial infarction in patients with coronary artery disease. Sci. Rep. 10, 8105. doi:10.1038/s41598-020-65014-z
Huang, F., Liu, Y., Yang, X., Che, D., Qiu, K., Hammock, B. D., et al. (2017). Shexiang Baoxin pills promotes angiogenesis in myocardial infarction rats via up-regulation of 20-HETE-mediated endothelial progenitor cells mobilization. Atherosclerosis 263, 184–191. doi:10.1016/j.atherosclerosis.2017.06.012
Isse, F. A., Alammari, A. H., El-Sherbeni, A. A., and El-Kadi, A. O. S. (2023). 17-(R/S)-hydroxyeicosatetraenoic acid (HETE) induces cardiac hypertrophy through the CYP1B1 in enantioselective manners. Prostagl. Other Lipid Mediat 168, 106749. doi:10.1016/j.prostaglandins.2023.106749
Jiang, C., Wang, X., Dang, S., Wang, X., Deng, Q., Hu, J., et al. (2021). Chinese medicine shensong yangxin Capsule ameliorates myocardial microcirculation dysfunction in rabbits with chronic myocardial infarction. Chin. J. Integr. Med. 27, 24–30. doi:10.1007/s11655-018-2578-1
Jiang, H., Shen, Z., Chu, Y., Li, Y., Li, J., Wang, X., et al. (2015). Serum metabolomics research of the anti-hypertensive effects of Tengfu Jiangya tablet on spontaneously hypertensive rats. J. Chromatogr. B Anal. Technol. Biomed. Life Sci. 1002, 210–217. doi:10.1016/j.jchromb.2015.08.010
Jiang, Q., Wang, L. N., Hu, J. H., and Zhang, Y. Q. (2019). Oral administration of leeches (Shuizhi): a review of the mechanisms of action on antiplatelet aggregation. J. Ethnopharmacol. 232, 103–109. doi:10.1016/j.jep.2018.12.010
Khodadi, E. (2020). Platelet function in cardiovascular disease: activation of molecules and activation by molecules. Cardiovasc Toxicol. 20, 1–10. doi:10.1007/s12012-019-09555-4
Kodali, S. T., Kauffman, P., Kotha, S. R., Yenigalla, A., Veeraraghavan, R., Pannu, S. R., et al. (2020). “Oxidative lipidomics: analysis of oxidized lipids and lipid peroxidation in biological systems with relevance to health and disease,” in Measuring oxidants and oxidative stress in biological systems. Editors L. J. Berliner, and N. L. Parinandi (Cham (CH): Springer), 61–92. doi:10.1007/978-3-030-47318-1_5
Krämer, H. J., Stevens, J., Grimminger, F., and Seeger, W. (1996). Fish oil fatty acids and human platelets: dose-dependent decrease in dienoic and increase in trienoic thromboxane generation. Biochem. Pharmacol. 52, 1211–1217. doi:10.1016/0006-2952(96)00473-x
Krötz, F., Riexinger, T., Buerkle, M. A., Nithipatikom, K., Gloe, T., Sohn, H. Y., et al. (2004). Membrane-potential-dependent inhibition of platelet adhesion to endothelial cells by epoxyeicosatrienoic acids. Arterioscler. Thromb. Vasc. Biol. 24, 595–600. doi:10.1161/01.ATV.0000116219.09040.8c
Kuhn, H., Banthiya, S., and van Leyen, K. (2015). Mammalian lipoxygenases and their biological relevance. Biochim. Biophys. Acta 1851, 308–330. doi:10.1016/j.bbalip.2014.10.002
Kwon, S. Y., Massey, K., Watson, M. A., Hussain, T., Volpe, G., Buckley, C. D., et al. (2020). Oxidised metabolites of the omega-6 fatty acid linoleic acid activate dFOXO. Life Sci. Alliance 3, e201900356. doi:10.26508/lsa.201900356
Lai, J., and Chen, C. (2021). The role of epoxyeicosatrienoic acids in cardiac remodeling. Front. Physiol. 12, 642470. doi:10.3389/fphys.2021.642470
Lai, Q., Yuan, G., Shen, L., Zhang, L., Fu, F., Liu, Z., et al. (2021). Oxoeicosanoid receptor inhibition alleviates acute myocardial infarction through activation of BCAT1. Basic Res. Cardiol. 116, 3. doi:10.1007/s00395-021-00844-0
Lau, E. S., Roshandelpoor, A., Zarbafian, S., Wang, D., Guseh, J. S., Allen, N., et al. (2023). Eicosanoid and eicosanoid-related inflammatory mediators and exercise intolerance in heart failure with preserved ejection fraction. Nat. Commun. 14, 7557. doi:10.1038/s41467-023-43363-3
Li, C., Wang, J., Wang, Q., Zhang, Y., Zhang, N., Lu, L., et al. (2016). Qishen granules inhibit myocardial inflammation injury through regulating arachidonic acid metabolism. Sci. Rep. 6, 36949. doi:10.1038/srep36949
Li, J., Cao, G. Y., Zhang, X. F., Meng, Z. Q., Gan, L., Li, J. X., et al. (2020a). Chinese medicine she-Xiang-Xin-Tong-Ning, containing Moschus, corydalis and ginseng, protects from myocardial ischemia injury via angiogenesis. Am. J. Chin. Med. 48, 107–126. doi:10.1142/S0192415X20500068
Li, J., Guo, C., and Wu, J. (2019a). 15-Deoxy-∆-(12,14)-Prostaglandin J2 (15d-PGJ2), an endogenous ligand of PPAR-γ: function and mechanism. PPAR Res. 2019, 7242030. doi:10.1155/2019/7242030
Li, L., Zhong, S., Shen, X., Li, Q., Xu, W., Tao, Y., et al. (2019b). Recent development on liquid chromatography-mass spectrometry analysis of oxidized lipids. Free Radic. Biol. Med. 144, 16–34. doi:10.1016/j.freeradbiomed.2019.06.006
Li, S., Xu, Y., Guo, W., Chen, F., Zhang, C., Tan, H. Y., et al. (2020b). The impacts of herbal medicines and natural products on regulating the hepatic lipid metabolism. Front. Pharmacol. 11, 351. doi:10.3389/fphar.2020.00351
Li, Y. P., Wang, C. Y., Shang, H. T., Hu, R. R., Fu, H., and Xiao, X. F. (2020c). A high-throughput and untargeted lipidomics approach reveals new mechanistic insight and the effects of salvianolic acid B on the metabolic profiles in coronary heart disease rats using ultra-performance liquid chromatography with mass spectrometry. RSC Adv. 10, 17101–17113. doi:10.1039/d0ra00049c
Liu, X., Mi, X., Wang, Z., Zhang, M., Hou, J., Jiang, S., et al. (2020). Ginsenoside Rg3 promotes regression from hepatic fibrosis through reducing inflammation-mediated autophagy signaling pathway. Cell Death Dis. 11, 454. doi:10.1038/s41419-020-2597-7
Luo, L., Yang, Y., Fu, M., Luo, J., Li, W., Tu, L., et al. (2023). 11,12-EET suppressed LPS induced TF expression and thrombus formation by accelerating mRNA degradation rate via strengthening PI3K-Akt signaling pathway and inhibiting p38-TTP pathway. Prostagl. Other Lipid Mediat 167, 106740. doi:10.1016/j.prostaglandins.2023.106740
Maayah, Z. H., Abdelhamid, G., and El-Kadi, A. O. (2015). Development of cellular hypertrophy by 8-hydroxyeicosatetraenoic acid in the human ventricular cardiomyocyte, RL-14 cell line, is implicated by MAPK and NF-κB. Cell Biol. Toxicol. 31, 241–259. doi:10.1007/s10565-015-9308-7
Manke, M.-C., Ahrends, R., and Borst, O. (2022). Platelet lipid metabolism in vascular thrombo-inflammation. Pharmacol. Ther. 237, 108258. doi:10.1016/j.pharmthera.2022.108258
Marmol, F., Puig-Parellada, P., Sanchez, J., and Trullas, R. (1999). Influence of aging on thromboxane A2 and prostacyclin levels in rat hippocampal brain slices. Neurobiol. Aging 20, 695–697. doi:10.1016/s0197-4580(99)00069-x
Mezzetti, A. (2005). Pharmacological modulation of plaque instability. Lupus 14, 769–772. doi:10.1191/0961203305lu2218oa
Nakanishi, M., and Rosenberg, D. W. (2013). Multifaceted roles of PGE2 in inflammation and cancer. Semin. Immunopathol. 35, 123–137. doi:10.1007/s00281-012-0342-8
Nayeem, M. A. (2018). Role of oxylipins in cardiovascular diseases. Acta Pharmacol. Sin. 39, 1142–1154. doi:10.1038/aps.2018.24
Nobili, E., Salvado, M. D., Folkersen, L., Castiglioni, L., Kastrup, J., Wetterholm, A., et al. (2012). Cysteinyl leukotriene signaling aggravates myocardial hypoxia in experimental atherosclerotic heart disease. PLoS One 7, e41786. doi:10.1371/journal.pone.0041786
Ozen, G., and Norel, X. (2017). Prostanoids in the pathophysiology of human coronary artery. Prostagl. Other Lipid Mediat 133, 20–28. doi:10.1016/j.prostaglandins.2017.03.003
Palmu, J., Watrous, J. D., Mercader, K., Havulinna, A. S., Lagerborg, K. A., Salosensaari, A., et al. (2020). Eicosanoid inflammatory mediators are robustly associated with blood pressure in the general population. J. Am. Heart Assoc. 9, e017598. doi:10.1161/JAHA.120.017598
Pinckard, K. M., Shettigar, V. K., Wright, K. R., Abay, E., Baer, L. A., Vidal, P., et al. (2021). A novel endocrine role for the BAT-released lipokine 12,13-diHOME to mediate cardiac function. Circulation 143, 145–159. doi:10.1161/CIRCULATIONAHA.120.049813
Qiu, H., Gabrielsen, A., Agardh, H. E., Wan, M., Wetterholm, A., Wong, C.-H., et al. (2006). Expression of 5-lipoxygenase and leukotriene A4 hydrolase in human atherosclerotic lesions correlates with symptoms of plaque instability. Proc. Natl. Acad. Sci. U. S. A. 103, 8161–8166. doi:10.1073/pnas.0602414103
Reddy, Y. K., Reddy, L. M., Capdevila, J. H., and Falck, J. R. (2003). Practical, asymmetric synthesis of 16-hydroxyeicosa-5(Z), 8(Z), 11(Z), 14(Z)-tetraenoic acid (16-HETE), an endogenous inhibitor of neutrophil activity. Bioorg Med. Chem. Lett. 13, 3719–3720. doi:10.1016/j.bmcl.2003.08.005
Rocic, P., and Schwartzman, M. L. (2018). 20-HETE in the regulation of vascular and cardiac function. Pharmacol. Ther. 192, 74–87. doi:10.1016/j.pharmthera.2018.07.004
Roman, R. J., and Fan, F. (2018). 20-HETE: hypertension and beyond. Hypertension 72, 12–18. doi:10.1161/HYPERTENSIONAHA.118.10269
Roth, G. A., Mensah, G. A., Johnson, C. O., Addolorato, G., Ammirati, E., Baddour, L. M., et al. (2020). Global burden of cardiovascular diseases and risk factors, 1990-2019: update from the GBD 2019 study. J. Am. Coll. Cardiol. 76, 2982–3021. doi:10.1016/j.jacc.2020.11.010
Scridon, A. (2022). Platelets and their role in hemostasis and thrombosis-from physiology to pathophysiology and therapeutic implications. Int. J. Mol. Sci. 23, 12772. doi:10.3390/ijms232112772
Shearer, G. C., and Walker, R. E. (2018). An overview of the biologic effects of omega-6 oxylipins in humans. Prostagl. Leukot. Essent. Fat. Acids 137, 26–38. doi:10.1016/j.plefa.2018.06.005
Shoieb, S. M., El-Sherbeni, A. A., and El-Kadi, A. (2019). Subterminal hydroxyeicosatetraenoic acids: crucial lipid mediators in normal physiology and disease states. Chem. Biol. Interact. 299, 140–150. doi:10.1016/j.cbi.2018.12.004
Snipelisky, D., Chaudhry, S.-P., and Stewart, G. C. (2019). The many faces of heart failure. Card. Electrophysiol. Clin. 11, 11–20. doi:10.1016/j.ccep.2018.11.001
Snyder, N. W., Golin-Bisello, F., Gao, Y., Blair, I. A., Freeman, B. A., and Wendell, S. G. (2015). 15-Oxoeicosatetraenoic acid is a 15-hydroxyprostaglandin dehydrogenase-derived electrophilic mediator of inflammatory signaling pathways. Chem. Biol. Interact. 234, 144–153. doi:10.1016/j.cbi.2014.10.029
Solati, Z., and Ravandi, A. (2019). Lipidomics of bioactive lipids in acute coronary syndromes. Int. J. Mol. Sci. 20, 1051. doi:10.3390/ijms20051051
Solati, Z., Surendran, A., Aukema, H. M., and Ravandi, A. (2023). Impact of reperfusion on plasma oxylipins in ST-segment elevation myocardial infarction. Metabolites 14, 19. doi:10.3390/metabo14010019
Spector, A. A. (2009). Arachidonic acid cytochrome P450 epoxygenase pathway. J. Lipid Res. 50 (Suppl. l), S52–S56. doi:10.1194/jlr.R800038-JLR200
Spickett, C. M., and Pitt, A. R. (2015). Oxidative lipidomics coming of age: advances in analysis of oxidized phospholipids in physiology and pathology. Antioxid. Redox Signal 22, 1646–1666. doi:10.1089/ars.2014.6098
Stanke-Labesque, F., Hardy, G., Cracowski, J. L., and Bessard, G. (2002). Leukotrienes and 12-HETE: key mediators of angiotensin II-mediated vascular effects.Rol in hypertension. Therapie 57, 151–156.
Sudhahar, V., Shaw, S., and Imig, J. D. (2010). Epoxyeicosatrienoic acid analogs and vascular function. Curr. Med. Chem. 17, 1181–1190. doi:10.2174/092986710790827843
Sugiyama, S., Hayakawa, M., Nagai, S., Ajioka, M., and Ozawa, T. (1987). Leukotoxin, 9, 10-epoxy-12-octadecenoate, causes cardiac failure in dogs. Life Sci. 40, 225–231. doi:10.1016/0024-3205(87)90336-5
Sun, L., Jia, H., Yu, M., Yang, Y., Li, J., Tian, D., et al. (2021). Salvia miltiorrhiza and Pueraria lobata, two eminent herbs in Xin-Ke-Shu, ameliorate myocardial ischemia partially by modulating the accumulation of free fatty acids in rats. Phytomedicine 89, 153620. doi:10.1016/j.phymed.2021.153620
Sun, X., and Li, Q. (2018). Prostaglandin EP2 receptor: novel therapeutic target for human cancers (Review). Int. J. Mol. Med. 42, 1203–1214. doi:10.3892/ijmm.2018.3744
Tacconelli, S., and Patrignani, P. (2014). Inside epoxyeicosatrienoic acids and cardiovascular disease. Front. Pharmacol. 5, 239. doi:10.3389/fphar.2014.00239
Takayama, K., García-Cardena, G., Sukhova, G. K., Comander, J., Gimbrone, M. J., and Libby, P. (2002). Prostaglandin E2 suppresses chemokine production in human macrophages through the EP4 receptor. J. Biol. Chem. 277, 44147–44154. doi:10.1074/jbc.M204810200
Tang, E. H., Libby, P., Vanhoutte, P. M., and Xu, A. (2012). Anti-inflammation therapy by activation of prostaglandin EP4 receptor in cardiovascular and other inflammatory diseases. J. Cardiovasc Pharmacol. 59, 116–123. doi:10.1097/FJC.0b013e3182244a12
Theken, K. N., Schuck, R. N., Edin, M. L., Tran, B., Ellis, K., Bass, A., et al. (2012). Evaluation of cytochrome P450-derived eicosanoids in humans with stable atherosclerotic cardiovascular disease. Atherosclerosis 222, 530–536. doi:10.1016/j.atherosclerosis.2012.03.022
Tong, K.-L., Tan, K.-E., Lim, Y.-Y., Tien, X.-Y., and Wong, P.-F. (2022). CircRNA-miRNA interactions in atherogenesis. Mol. Cell Biochem. 477, 2703–2733. doi:10.1007/s11010-022-04455-8
Torres, R., Picado, C., and de Mora, F. (2015). The PGE2-EP2-mast cell axis: an antiasthma mechanism. Mol. Immunol. 63, 61–68. doi:10.1016/j.molimm.2014.03.007
Troncoso, M. F., Ortiz-Quintero, J., Garrido-Moreno, V., Sanhueza-Olivares, F., Guerrero-Moncayo, A., Chiong, M., et al. (2021). VCAM-1 as a predictor biomarker in cardiovascular disease. Biochim. Biophys. Acta Mol. Basis Dis. 1867, 166170. doi:10.1016/j.bbadis.2021.166170
Tu, Z. G., Han, X. D., Wang, X. Y., Hou, Y. Y., Shao, B. Y., Wang, X., et al. (2003). Protective effects of CVPM on vascular endothelium in rats fed cholesterol diet. Clin. Chim. Acta 333, 85–90. doi:10.1016/s0009-8981(03)00201-8
Wang, D. D., Fan, G. W., Wang, Y. F., Liu, H. T., Wang, B. Y., Dong, J., et al. (2013). Vascular reactivity screen of Chinese medicine Danhong injection identifies danshensu as a NO-independent but PGI2-mediated relaxation factor. J. Cardiovasc Pharm. 62, 457–465. doi:10.1097/FJC.0b013e3182a29657
Wang, J., Li, C., Cao, Y., Wang, Q., Lu, L., Chang, H., et al. (2015a). Mechanism of QSYQ on anti-apoptosis mediated by different subtypes of cyclooxygenase in AMI induced heart failure rats. BMC Complement. Altern. Med. 15, 352. doi:10.1186/s12906-015-0869-z
Wang, J., Lu, L., Wang, Y., Wu, Y., Han, J., Wang, W., et al. (2015b). Qishenyiqi Dropping Pill attenuates myocardial fibrosis in rats by inhibiting RAAS-mediated arachidonic acid inflammation. J. Ethnopharmacol. 176, 375–384. doi:10.1016/j.jep.2015.11.023
Wang, J., Zeng, L., Zhang, Y., Qi, W., Wang, Z., Tian, L., et al. (2022). Pharmacological properties, molecular mechanisms and therapeutic potential of ginsenoside Rg3 as an antioxidant and anti-inflammatory agent. Front. Pharmacol. 13, 975784. doi:10.3389/fphar.2022.975784
Wang, S. L., Lu, J. W., Guan, Y. F., Zhang, X. Y., and Xu, H. (2019). Role of prostaglandin E2 receptor 4 in cardiovascular diseases. Sheng Li Xue Bao 71, 361–370.
Wang, W., Song, L., Yang, L., Li, C., Ma, Y., Xue, M., et al. (2023). Panax quinquefolius saponins combined with dual antiplatelet therapy enhanced platelet inhibition with alleviated gastric injury via regulating eicosanoids metabolism. BMC Complement. Med. Ther. 23, 289. doi:10.1186/s12906-023-04112-7
Wang, X., Gao, Y., Tian, Y., Liu, X., Zhang, G., Wang, Q., et al. (2020a). Integrative serum metabolomics and network analysis on mechanisms exploration of Ling-Gui-Zhu-Gan Decoction on doxorubicin-induced heart failure mice. J. Ethnopharmacol. 250, 112397. doi:10.1016/j.jep.2019.112397
Wang, Y., Huang, X. Y., Ma, Z. C., Wang, Y. G., Chen, X. M., and Gao, Y. (2018). Ophiopogonin D alleviates cardiac hypertrophy in rat by upregulating CYP2J3 in vitro and suppressing inflammation in vivo. Biochem. Bioph Res. Co. 503, 1011–1019. doi:10.1016/j.bbrc.2018.06.110
Wang, Y., Li, C., Liu, Z. Y., Shi, T. J., Wang, Q. Y., Li, D., et al. (2014). DanQi Pill protects against heart failure through the arachidonic acid metabolism pathway by attenuating different cyclooxygenases and leukotrienes B4. BMC Complem Altern. M. 14, 67. doi:10.1186/1472-6882-14-67
Wang, Y. Q., Li, S. J., Man, Y. H., and Zhuang, G. (2020b). Serum metabonomics coupled with HPLC-LTQ/orbitrap MS and multivariate data analysis on the ameliorative effects of Bidens bipinnata L. in hyperlipidemic rats. J. Ethnopharmacol. 262, 113196. doi:10.1016/j.jep.2020.113196
Wang, Y. S., Lin, R. T., Cheng, H. Y., Yang, S. F., Chou, W. W., and Juo, S. (2011). Anti-atherogenic effect of San-Huang-Xie-Xin-Tang, a traditional Chinese medicine, in cultured human aortic smooth muscle cells. J. Ethnopharmacol. 133, 442–447. doi:10.1016/j.jep.2010.10.018
Wei, B., Sun, C., Wan, H., Shou, Q., Han, B., Sheng, M., et al. (2023). Bioactive components and molecular mechanisms of Salvia miltiorrhiza Bunge in promoting blood circulation to remove blood stasis. J. Ethnopharmacol. 317, 116697. doi:10.1016/j.jep.2023.116697
Wittwer, J., and Hersberger, M. (2007). The two faces of the 15-lipoxygenase in atherosclerosis. Prostagl. Leukot. Essent. Fat. Acids 77, 67–77. doi:10.1016/j.plefa.2007.08.001
World Heart Federation (2023). World heart report 2023: confronting the world’s number one killer. Available at: https://world-heart-federation.org/wp-content/uploads/World-Heart-Report-2023.pdf (Accessed May 27, 2024).
Xie, J., Jiang, H. Q., Li, Y. L., Nie, L., Zhou, H. L., and Yang, W. Q. (2019). Study on the intervention effects of pinggan prescription on spontaneously hypertensive rats based on metabonomic and pharmacodynamic methods. Chin. J. Integr. Med. 25, 348–353. doi:10.1007/s11655-015-2126-1
Xu, H. J., Lu, H., Zhu, X. C., Wang, W., Zhang, Z. M., Fu, H. Z., et al. (2018). Inhibitory effects of luteolin-4′-O-β-D-glucopyranoside on P2Y12 and thromboxane A2 receptor-mediated amplification of platelet activation in vitro. Int. J. Mol. Med. 42, 615–624. doi:10.3892/ijmm.2018.3634
Xu, J., Li, X., Zhang, F., Tang, L., Wei, J., Lei, X., et al. (2019). Integrated UPLC-Q/TOF-MS technique and MALDI-MS to study of the efficacy of YiXinshu Capsules against heart failure in a rat model. Front. Pharmacol. 10, 1474. doi:10.3389/fphar.2019.01474
Xu, S., Ilyas, I., Little, P. J., Li, H., Kamato, D., Zheng, X., et al. (2021). Endothelial dysfunction in atherosclerotic cardiovascular diseases and beyond: from mechanism to pharmacotherapies. Pharmacol. Rev. 73, 924–967. doi:10.1124/pharmrev.120.000096
Yeung, J., Tourdot, B. E., Fernandez-Perez, P., Vesci, J., Ren, J., Smyrniotis, C. J., et al. (2014). Platelet 12-LOX is essential for FcγRIIa-mediated platelet activation. Blood 124, 2271–2279. doi:10.1182/blood-2014-05-575878
Yin, J., Lu, X., Qian, Z., Xu, W., and Zhou, X. (2019). New insights into the pathogenesis and treatment of sarcopenia in chronic heart failure. Theranostics 9, 4019–4029. doi:10.7150/thno.33000
Yousefnia, S., Momenzadeh, S., Seyed, F. F., Ghaedi, K., and Nasr, E. M. (2018). The influence of peroxisome proliferator-activated receptor γ (PPARγ) ligands on cancer cell tumorigenicity. Gene 649, 14–22. doi:10.1016/j.gene.2018.01.018
Yu, C., Qi, D., Lian, W., Li, Q. Z., Li, H. J., and Fan, H. Y. (2014). Effects of danshensu on platelet aggregation and thrombosis: in vivo arteriovenous shunt and venous thrombosis models in rats. PLoS One 9, e110124. doi:10.1371/journal.pone.0110124
Yu, Z., Wang, L., Wu, S., and Zhao, W. (2023). Dissecting the potential mechanism of antihypertensive effects of RVPSL on spontaneously hypertensive rats via widely targeted kidney metabolomics. J. Sci. Food Agric. 103, 428–436. doi:10.1002/jsfa.12157
Yuan, Z., Yang, L., Zhang, X., Ji, P., Hua, Y., and Wei, Y. (2020). Mechanism of Huang-lian-Jie-du decoction and its effective fraction in alleviating acute ulcerative colitis in mice: regulating arachidonic acid metabolism and glycerophospholipid metabolism. J. Ethnopharmacol. 259, 112872. doi:10.1016/j.jep.2020.112872
Yuhki, K., Kojima, F., Kashiwagi, H., Kawabe, J., Fujino, T., Narumiya, S., et al. (2011). Roles of prostanoids in the pathogenesis of cardiovascular diseases: novel insights from knockout mouse studies. Pharmacol. Ther. 129, 195–205. doi:10.1016/j.pharmthera.2010.09.004
Zhang, F., Deng, H., Kemp, R., Singh, H., Gopal, V. R., Falck, J. R., et al. (2005). Decreased levels of cytochrome P450 2E1-derived eicosanoids sensitize renal arteries to constrictor agonists in spontaneously hypertensive rats. Hypertension 45, 103–108. doi:10.1161/01.HYP.0000150782.28485.91
Zhang, J. X., Qi, M. J., Shi, M. Z., Chen, J. J., Zhang, X. Q., Yang, J., et al. (2019). Effects of Danhong injection, a traditional Chinese medicine, on nine cytochrome P450 isoforms in vitro. Biomed. Chromatogr. 33, e4454. doi:10.1002/bmc.4454
Zhang, J. Y., Liang, R. X., Wang, L., Yan, R. Y., Hou, R., Gao, S. R., et al. (2013). Effects of an aqueous extract of Crataegus pinnatifida Bge. var. major NEBr. fruit on experimental atherosclerosis in rats. J. Ethnopharmacol. 148, 563–569. doi:10.1016/j.jep.2013.04.053
Zhang, L., Chen, N., Zhan, L., Bi, T., Zhou, W., Zhang, L., et al. (2023a). Erchen Decoction alleviates obesity-related hepatic steatosis via modulating gut microbiota-drived butyric acid contents and promoting fatty acid β-oxidation. J. Ethnopharmacol. 317, 116811. doi:10.1016/j.jep.2023.116811
Zhang, Y., Guallar, E., Blasco-Colmenares, E., Harms, A. C., Vreeken, R. J., Hankemeier, T., et al. (2016). Serum-based oxylipins are associated with outcomes in primary prevention implantable cardioverter defibrillator patients. PLoS One 11, e0157035. doi:10.1371/journal.pone.0157035
Zhang, Y., Hong, Z., Yuan, Z., Wang, T., Wu, X., Liu, B., et al. (2020). Extract from Rostellularia procumbens (L.) nees inhibits thrombosis and platelet aggregation by regulating integrin β(3) and MAPK pathways. ACS Omega 5, 32123–32130. doi:10.1021/acsomega.0c05227
Zhang, Y., Lu, J., Huang, S., Zhang, Y., Liu, J., Xu, Y., et al. (2023b). CYP2J deficiency leads to cardiac injury and presents dual regulatory effects on cardiac function in rats. Toxicol. Appl. Pharmacol. 473, 116610. doi:10.1016/j.taap.2023.116610
Zhang, Y., Lu, J., Ma, Y., Sun, L., Wang, S., Yue, X., et al. (2022). Establishment of fingerprint and mechanism of anti-myocardial ischemic effect of Syringa pinnatifolia. Biomed. Chromatogr. 36, e5475. doi:10.1002/bmc.5475
Zhang, Y., Wang, Y., Meng, H., Lu, W. J., Wang, Q. X., Zhang, Q., et al. (2018). Mechanisms of Fufang Danshen tablets on treating heart failure via regulating AT1-mediated PLA2-COX2/5-LOX metabolic pathway. Zhongguo Zhong Yao Za Zhi 43, 2593–2599. doi:10.19540/j.cnki.cjcmm.2018.0083
Zhi, H., Zhang, Z., Deng, Y., Yan, B., Li, Z., Wu, W., et al. (2021). Restoring perturbed oxylipins with Danqi Tongmai Tablet attenuates acute myocardial infarction. Phytomedicine 90, 153616. doi:10.1016/j.phymed.2021.153616
Zuo, D., Pi, Q., Shi, Y., Luo, S., and Xia, Y. (2021). Dihydroxyeicosatrienoic acid, a metabolite of epoxyeicosatrienoic acids upregulates endothelial nitric oxide synthase expression through transcription: mechanism of vascular endothelial function protection. Cell Biochem. Biophys. 79, 289–299. doi:10.1007/s12013-021-00978-x
Keywords: herbal medicine, cardiovascular disease, polyunsaturated fatty acids, oxylipins, review
Citation: Li M, He M, Sun M, Li Y, Li M, Jiang X, Wang Y and Wang H (2024) Oxylipins as therapeutic indicators of herbal medicines in cardiovascular diseases: a review. Front. Pharmacol. 15:1454348. doi: 10.3389/fphar.2024.1454348
Received: 25 June 2024; Accepted: 03 December 2024;
Published: 19 December 2024.
Edited by:
Chen Huei Leo, National University of Singapore, SingaporeReviewed by:
Cheng Xue Helena Qin, Monash University, AustraliaCopyright © 2024 Li, He, Sun, Li, Li, Jiang, Wang and Wang. This is an open-access article distributed under the terms of the Creative Commons Attribution License (CC BY). The use, distribution or reproduction in other forums is permitted, provided the original author(s) and the copyright owner(s) are credited and that the original publication in this journal is cited, in accordance with accepted academic practice. No use, distribution or reproduction is permitted which does not comply with these terms.
*Correspondence: Yanxin Wang, d3lhbnhpbjIwMDBAMTI2LmNvbQ==; Hongfeng Wang, Y2N3aGZAMTI2LmNvbQ==
†These authors have contributed equally to this work and share first authorship
Disclaimer: All claims expressed in this article are solely those of the authors and do not necessarily represent those of their affiliated organizations, or those of the publisher, the editors and the reviewers. Any product that may be evaluated in this article or claim that may be made by its manufacturer is not guaranteed or endorsed by the publisher.
Research integrity at Frontiers
Learn more about the work of our research integrity team to safeguard the quality of each article we publish.