- 1Department of Gastroenterology, Heilongjiang Academy of Traditional Chinese Medicine, Harbin, China
- 2First Clinical Medical College, Heilongjiang University of Chinese Medicine, Harbin, China
- 3School of Medicine and Allied Health Sciences, University of The Gambia, Banjul, Gambia
Despite the advanced technologies and global attention on cancer treatment strategies, cancer continues to claim lives and adversely affects socio-economic development. Although combination therapies were anticipated to eradicate this disease, the resilient and restorative nature of cancers allows them to proliferate at the expense of host immune cells energetically. This proliferation is driven by metabolic profiles specific to the cancer type and the patient. An emerging field is exploring the metabolic reprogramming (MR) of cancers to predict effective treatments. This mini-review discusses the recent advancements in cancer MR that have contributed to predictive, preventive, and precision medicine. Current perspectives on the mechanisms of various cancer types and prospects for MR and personalized cancer medicine are essential for optimizing metabolic outputs necessary for personalized treatments.
Introduction
Cancer is a multifaceted genetic disease that arises from elaborate changes to the genome. Metabolic reprogramming (MR) is considered to be one of the emerging hallmarkers of cancer, which promotes cell survival and infinite proliferation of malignant cells through changes in the characteristics of metabolic enzymes, upstream regulatory molecules and downstream metabolites (Ciardiello et al., 2022). Owing to the high metabolic plasticity, tumor cells exhibit complex metabolic patterns, with glucose metabolism, amino acid metabolism and lipid metabolism being dominant (Xu X. et al., 2023). Glucose metabolism includes glycolysis and glucose oxidative phosphorylation (OXPHOS), lipid metabolism mainly consists of fatty acid oxidation, fatty acid synthesis and cholesterol esterification, and amino acid metabolism includes pentose phosphate pathway (PPP) and serine/glycine pathway (An et al., 2024; Liu X. et al., 2024; Yang K. et al., 2023). Figure 1. In addition, there is metabolic crosstalk among glucose, lipid and amino acid metabolism. Furthermore, MR also elucidates the inherent fragility of cancer therapeutics, and the foundation of precision medicine for cancers based on MR requires detailed insights into both tumorigenesis and progression.
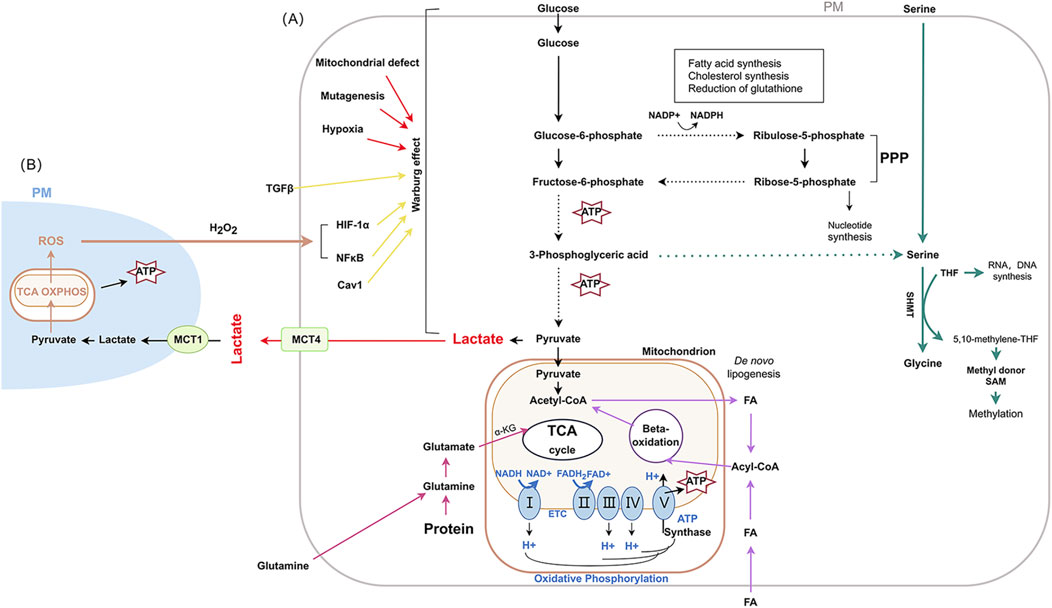
Figure 1. The main metabolic pathways and changes in cancer Abbreviation used: PM: plasma membrane; ROS: reactive oxygen species; TCA: tricarboxylic acid; OXPHOS: oxidative phosphorylation; ATP: adenosine triphosphate; MCT1: monocarboxylate transporter-1; TGF-β:Transforming growth factor-β; MCT4: monocarboxylate transporters 4; HIF-1α: hypoxia-inducible factor-1 α; NF-κB: nuclear factor κB; Cav1: Caveolin-1; PPP: pentose phosphate pathway; SHMT: serine hydroxy methyltransferase; THF: tetrahydrofolate; SAM: S-adenosylmethionine; α-KG: α-ketoglutarate; NADH: nicotinamide adenine dinucleotide; FA: fatty acid. FAD: flavin adenine dinucleotide; FADH2: reduced flavin adenine dinucleotide; I: NADH-coenzyme Q reductase; II: succinate coenzyme Q reductase; III: coenzyme Q cytochrome c reductase; IV: cytochrome c oxidase; V: ATP synthase.
The challenges of drug resistance and immune evasion remain major, persistent, unsolved issues in conventional cancer treatments, and are closely related to MR and epigenetics factors (Sun et al., 2022). Epigenetic modifications play a crucial role in maintaining normal physiological functions of the body, including covalent modification of bases in DNA, post-translational modification of the terminal amino acids of histones, chromatin remodeling, and modification and regulation of non-coding RNA. A large number of studies have shown that abnormal epigenetic modifications can affect the occurrence and development of cancer by regulating cancer MR (Wang et al., 2022; Yang J. et al., 2023; Yu et al., 2018). The complex interactions between genes and the environment as epigenetic factors make it impossible to adopt one-size-fits-all approach, thus necessitating precision medicine interventions for cancer (Rulten et al., 2023). MR and dysregulation are fundamental processes that result in phenotypic alterations through molecular mechanisms, which are critical for precision therapeutic interventions for cancer. Therefore, targeting cancer metabolic pathways is a promising approach to combat drug resistance. Moreover, metabolic, epigenetic, and transcriptional regulation involving immune cell plasticity in cancers are crucial for personalized treatment (Yuan et al., 2024). Considering the diverse MR, immune evasion strategies, and drug resistance mechanisms exhibited by different cancers, it is crucial to identify a range of cancer-specific genes for biomarker screening (Wamsley et al., 2023). Therefore, this mini-review collects evidence on energy rewiring mechanisms and the prospects of personalized medicine for various cancers.
Metabolic reprogramming and tumor microenvironment
The secondary objective of cancer MR is to reconfigure the tumor microenvironment (TME), which is primarily characterized by insufficient nutrient supply, hypoxia, and acidosis (Safi et al., 2023). The TME comprises intense interactions among tumor cells, stroma, and immune cells, which are crucial in tumorigenesis, metastasis, and drug resistance. The heterogeneity of TME not only provides survival and adaptability advantages for tumor cells, but also endows tumor cells with different reactivity and resistance to chemotherapy and targeted therapy. Immune cells also undergo MR in TME to produce metabolic adaptations associated with tolerance phenotypes, thereby exerting anti-tumor and gaining the ability to evade immune surveillance (Liu C. et al., 2024). Immunoediting intricately shapes the immunogenic profile of tumor cells and effectively undermines host anti-tumor responses throughout the process of tumorigenesis. Although intrinsic factors and metabolites regulate these processes within the TME, the interaction of immune cells, metabolites, and their niche is essential for effective treatment regimens (Buck et al., 2017). Recent findings have correlated deregulated bioenergetics programs with immunoediting, creating a counterbalance between infiltrating T cells and tumor in the TME (Tsai et al., 2023). Given the wide heterogeneity and adaptability of tumors, the TME also provide potential mechanistic targets, including oncometabolites and epigenetic modifiers (Das et al., 2024).
Various metabolic mechanisms on cancer precision medicine
The dissemination and metastasis of cancer are driven by epithelial-to-mesenchymal transition (EMT) through invasion and migration. This process is intimately linked to MR arising from the rewiring of cellular states and signaling pathways for survival in the context of dietary deprivation. The binding of activating transcription factor 4 (ATF4) to enhancers of mesenchymal factors and amino acid deprivation-responsive genes promotes the loss of epithelial characteristics and the acquisition of transforming growth factor β (TGF-β)-signaling-associated mesenchymal traits, further enhancing lung cancer cell metastasis (Lin et al., 2024). Hence, the specific epithelial enhancers regulation of EMT through MR represents a promising intervention for precision medicine. Hypoxia profoundly impacts the TME, leading to therapy resistance. It is practicable to employ hypoxia biomarkers as predictive and prognostic indicators for solid tumors treatment (Bigos et al., 2024). Lipid metabolism holds potential in targeting isocitrate dehydrogenase 1 (IDH1) and IDH2 for metabolic interventions. Distinct fatty acid metabolism is detected between IDH1 and IDH2, making these mutated genes a potential focus for precision medicine (Thomas et al., 2023). In the targeted therapy of cancer, regulators such as hexokinase 2 (HK2), pyruvate kinase M2 (PKM2), enolase 1 (ENO1), and lactate dehydrogenase A (LDHA) are crucial for the glycolytic pathway. At the transcriptional level, glycolysis is regulated by p53, c-Myc, hypoxia-inducible factor 1 (HIF1), and sine oculis homeobox homolog 1 (SIX1). Additionally, post-translational modifications such as methylation, phosphorylation, ubiquitination, and acetylation also exert significant roles in signal transduction and MR within the glycolytic pathway (Ni et al., 2024).
The response of individual patients to cisplatin treatment have revealed metabolic changes as factors influencing sensitivity and resistance, highlighting the need for personalized medicine for cisplatin resistance and other cancer resistant therapies (Yu et al., 2023). Combination therapy is essential for glioblastoma, and epidermal growth factor receptor (EGFR)-activated MR holds great potential. Temozolomide’s antitumor effects, targeting the mevalonate and EGFR/AKT pathways, demonstrate significant clinical management of glioblastoma (Cui et al., 2023). In glioblastoma multiforme, the uptake of phospholipids and fatty acid synthesis is increased, while glycerolipid and glycerophospholipid metabolism is aberrant, disclosing its distinctive metabolic characteristics (Miao et al., 2023). A significant breakthrough has been achieved in the application of predictive medicine for gliomas, where features related to mitochondrial genome composition can predict its sensitivity to chemotherapy drugs, thereby exerting a positive impact on the prognosis of patients (Wu J. et al., 2023).
Luminal B breast cancer (LBBC) has a complex molecular landscape, and a comprehensive muti-platform analysis offers valuable targets and signaling pathways for the examination of differences between the two subtypes to attain more precise treatment of LBBC (Wang H. et al., 2023). Phosphoinositide 3-kinase (PI3K) inhibitors combined with autophagy have a strong rationale for breast cancer treatment. For instance, the metabolism of breast cancer is influenced by mitochondrial translation dysregulation and loss of core binding factor subunit β (CBFB) function (Malik et al., 2023). A metabolic switch involving triple-negative breast cancer (TNBC) shifts from glycolysis to fatty acid β-oxidation (FAO) through the inhibition of PKM2. Impaired histone methyltransferase, enhancer of zeste homolog 2 (EZH2), can be recruited to solute carrier family 16 member 9 (SLC16A9), a carnitine transporter, coordinated by the direct interaction of PKM2, thus epigenetically influencing tumor progression (Zhang Y. et al., 2024). In cervical squamous cell carcinoma (CESC), lipid metabolism-related genes (LMRGs) signature plays a significant role in advancing precision medicine strategies for the management of patients with cervical cancer by enhancing CESC prognostication (Wang and Zhang, 2024). The depletion of aconitate decarboxylase 1 (ACOD1) has been shown to reduce the levels of the immune metabolite itaconate, while simultaneously driving macrophages to polarize strongly and persistently towards a pro-inflammatory state, demonstrating an enhanced tumour-inhibiting ability and thereby improving ovarian cancer (OC) survival (Wang X. et al., 2023).
Overexpression of mucin 1 (MUC1) can promote cancer cell proliferation by regulating cell metabolism, and tumor-related MUC1 exhibiting loss of apical localization and aberrant glycosylation in kidney cancer, especially in renal cell carcinoma (RCC) (Milella et al., 2024). Fatty acid metabolism presents the potential clinical application value of PD-1/PD-L1 in the TME of clear cell renal cell carcinoma (ccRCC), thereby providing a predictive treatment response for personalized medicine (Lin et al., 2023). Regulating glycolytic metabolism through targeting the c-Myc oncoprotein is demonstrated in the ubiquitin specific peptidase 43 (USP43) enzyme in bladder cancer. The degradation of c-Myc is achieved through interference with f-box and WD repeat domain containing 7 (FBXW7) by USP43 upregulation (Li M. et al., 2024).
In liver cancer, the identification and characterization of novel invasive cancer types will enhance the understanding of invasion and metastasis, leading to the development of novel precision therapies (Wu L. et al., 2023). In hepatocellular carcinoma (HCC), solute carrier family 25 member 15 (SLC25A15) is hypoxia-responsive with low glutamine reprogramming, facilitating anti-PD-L1 therapy (Zhang Q. et al., 2024). The loss of NADPH oxidase 4 (NOX4) in HCC induces MR in a nuclear factor erythroid 2-related factor 2 (Nrf2)/Myc-dependent manner, promoting tumor progression and making this tumor suppressor function a targeted therapy (Penuelas-Haro et al., 2023). Recently, nanoparticles-mediated co-delivery of cofilin 1 (CFL1) silencing with sorafenib, a chemotherapeutic agent, showed elevated inhibitory properties for HCC tumor growth without exhibiting significant toxicity (Li S. et al., 2023).
For Lung cancer, solute carrier family 3 member 2 (SLC3A2) acts as a metabolic switch factor in tumor-associated macrophages (TAM), suggesting that lung adenocarcinoma (LUAD) phenotyping reprogramming occurs via arachidonic acid (Li Z. et al., 2023). Oncogenic mutations are likely to facilitate MR within cancer cells for sustaining their energy and biomass demands. Results obtained from non-small cell lung cancer (NSCLC) cells with varying EGFR and kristen rat sarcoma (KRAS) statuses indicated that NSCLC cell lines possess a heterogeneous metabolic profile, which could facilitate metabolically targeted therapy for NSCLC patients through identification and stratification (Mendes et al., 2023).
Acute myeloid leukemia (AML) demonstrates a resistance to immunosurveillance and chemotherapy in leukemia stem cells (LSCs). For instance, inhibition of the Src homology region 2 (SH-2) domain-containing phosphatase 1 (Shp1) can alter the energy mechanisms of LSCs to confer sensitivity to chemotherapeutic drugs and presenting a promising prospect for surmounting resistance in AML (Xu et al., 2024). Through the AKT-β-catenin pathway, the expression of phosphofructokinase platelet (PFKP) is upregulated, which increased the metabolic activities and promote the degradation of Myc, thereby reducing the abilities of LSCs to evade the immune system and enhance the sensitivity to chemotherapy. Despite the emergence of drug resistance, precision treatment with a combined synergistic effect of mTOR and fibroblast growth factor receptor 1 (FGFR1) inhibitors is promising for T-cell acute lymphoblastic leukemia (T-ALL). This is practically achievable through MR, leading to the reversal of FGFR1 inhibitor resistance (Zhang et al., 2023). In osteosarcoma, clustering of lactic acid metabolism could identify the NADH dehydrogenase (ubiquinone) complex I assembly factor 6 (NDUFAF6, also known as C8ORF38) gene, which is a lactic acid metabolism-related gene and a prognostic marker, thus making this gene a functional target for individuals with this cancer type (Wang et al., 2024a).
While promising for metabolism-based therapies, advanced feature analysis through single-cell, multi-omics and spatial technologies, as well as accurate tracking of dynamic changes in metabolic adaptation, will promote the application of precision metabolic therapy in cancer treatment (Wu Z. et al., 2023). Trans-omics, as part of the quest for multiple biomarkers, provides reliability and accuracy for cancer diagnosis. With growing interest in lung cancer and MR, specific metabolites such as lipids can offer a comprehensive analysis of lung cancer. To some extent, precision therapy for lung cancer depends on this novel trans-omics network approach (Yan et al., 2024). Considering the impact of MR on inter-patient heterogeneity, a model pipeline has been developed for ensemble learning, providing insights for the treatment of LUAD (Sun et al., 2024). Formyl peptide receptor 3 (FPR3) can hinder the nuclear translocation of the nuclear factor of activated T cells 1 (NFATc1) by blocking cytoplasmic calcium influx and deactivating the NFATc1-binding neurogenic locus notch homolog protein 3 (NOTCH3) promoter. This process leads to the downregulation of glycolysis through the blocking of the AKT/mTORC1 signaling pathway and NOTCH3 expression. Gastric cancer precision therapy has proven the role of FPR3 in a calcium-dependent manner, thus providing insights into other precision therapy options (Wang et al., 2024b). Cancer-associated fibroblasts (CAF) have metabotropic subtypes with promising precision therapy applications, especially in liver metastases of colorectal cancer (CRC). The interaction of different immune cells with various communication strategies determines the sensitivity of chemotherapeutic drugs (Wu C. et al., 2023). Phosphoglycerate dehydrogenase (PHGDH) can modulate aryl hydrocarbon receptor (AhR) signaling and the redox-dependent autophagy pathway in CRC. Consequently, combination therapy that includes inhibition of both AhR and PHGDH is promising for this type of cancer (Li HM. et al., 2024). Additionally, a notable correlation exists between the expression level of PD-L1 and the individualized treatment regimen, as demonstrated by the mitochondrial pyruvate carrier 3 (MPC3) energy type in the treatment of esophageal squamous cell carcinoma (ESCC) (Wang Z. et al., 2024). Figure 2.
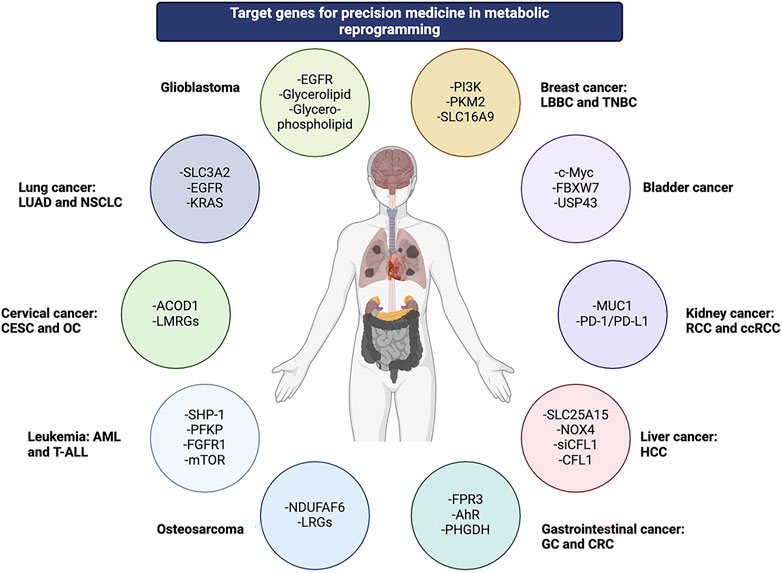
Figure 2. Various genes across multiple cancer types are involved in metabolic reprogramming as potential targets for precision medicine.
Prospects on metabolic reprogramming and cancer personalized medicine
A model derived from machine learning could potentially identify the metabolic landscape of gastric cancer for early detection (Chen et al., 2024). Another promising approach involves ultrasound-mediated cancer diagnosis using noninvasive mass spectrometry imaging (MSI), particularly effective for breast cancers, offering detailed “fingerprints” of elasticity and histopathology metabolism (Zhou et al., 2024). MR can impact T cell exhaustion via R-loop score patterns present tailored treatment avenues, crucial as R-loop regulators drive tumor progression (Zhang S. et al., 2024). Moreover, MR modulates tumor cell survival under hypoxia, influencing tumor proliferation and genomic stability (Abou Khouzam et al., 2023). The hypoxic TME also enhances chimeric antigen receptor (CAR)-T cell efficacy as a durable antitumor strategy (Zhu et al., 2024).
The risk of cancer, notably metabolic cancers linked to obesity, affects the phenotype and metabolism of immune cells. MR studies focusing on metabolic disorder-induced cancers may uncover biomarkers crucial for immunotherapy (Rosario et al., 2023). Oxidative stress and metabolism-related genes serve as reliable predictors for personalized medicine, aiding in targeted drug therapies and early diagnosis of patients at high risk for stomach adenocarcinoma (STAD) (Dong et al., 2023). In melanoma, targeted therapies exploiting metabolic phenotypes have shown promise with immune checkpoint inhibitors (ICI) (Carlino et al., 2021). Additionally, the study of platelet-derived microparticles in chronic lymphocytic leukemia (CLL) has revealed potential for precision medicine by highlighting immunologically dysfunctional B-lymphocytes (Gharib et al., 2023). A347D mutant p53 variant is implicated in cancer susceptibility among individuals with Li-Fraumeni syndrome (Choe et al., 2023). Mitochondrial OXPHOS adversely impacts antitumor immunity by reducing tumor-infiltrating T cells (Zhao et al., 2022). Future research should explore tumor heterogeneity within the TME and its implications for immune responses in precision medicine (Xu W. et al., 2023).
Improving cancer treatment outcomes and enhancing precision and predictive medicine require MR to reveal treatment vulnerabilities. As one of the newest approaches, some metabolic molecules have already progressed from the preclinical stage to the later stage of clinical trials. For example, AG-120 (ivosidenib), an inhibitor of the IDH1 mutant enzyme, has an acceptable safety profile and clinical activity, according to preliminary data from a Phase I clinical trial recruiting cancer patients with the IDH1 mutation (Popovici-Muller et al., 2018). Inhibition of fatty acid synthase (FASN) revealed a distinct tumor response, which were exacerbated by proliferative potential and mitochondrial respiration. The differences in expression patterns exhibited by FASN in pancreatic ductal adenocarcinoma (PDAC) illuminate the hallmarks of lipid metabolism (Chianese et al., 2023). TVB-2640, a FASN inhibitor, was found to be a well-tolerated oral agent in a Phase II study of recurrent high-grade astrocytoma and could be safely combined with bevacizumab to improve progression-free survival (PFS) (Kelly et al., 2023). Preclinical and clinical trial data suggest that the combination of CB-839, a glutaminase inhibitor, and capecitabine can be an effective treatment for PIK3CA-mutated CRC (Grkovski et al., 2020). CPI-613 is a novel anticancer agent that selectively targets altered forms of mitochondrial energy metabolism in tumor cells, causing changes in mitochondrial enzyme activity and REDOX status, which lead to apoptosis, necrosis, and autophagy in tumor cells. The use of CPI-613 in combination with modified FOLFIRINOX in patients with metastatic pancreatic cancer requires validation in a phase 2 trial (Alistar et al., 2017). However, despite the outstanding results of basic research in tumor MR, many metabolic enzymes have been targeted as tumor therapy, but the vulnerability of specific tumor types to specific inhibitors remains to be further investigated, whether it is a single drug or combination chemotherapy, radiotherapy, targeted therapy or immunotherapy.
In summary, the concept of modifying cancer cell metabolism to slow disease progression while enhancing immune cell function represents a groundbreaking approach in personalized MR intervention. Essential to this endeavor are enzyme inhibitors, metabolic enzyme modifications, pathway interactions, MR drug delivery targets, and methodical study designs (Wang Q. et al., 2024). The efficacy of MR is contingent upon a comprehensive understanding of the diverse metabolic environments present within cancers, which influence DNA repair mechanisms and therapeutic resistance, thus elucidating the broader metabolic landscape in cancer (Das et al., 2023). A novel therapeutic strategy that targets the interplay between cancer epigenetics, metabolism, and DNA repair pathways holds promise (Xing et al., 2023). Recent advances in molecular subtyping, including proteomic, genomic, transcriptomic, and phosphoproteomic profiling, along with assessments of microenvironment dysregulation, genetic alterations, and kinase-substrate regulatory networks, are poised to yield distinct therapeutic responses.
Conclusion
Personalized medicine in cancer therapy is patient-dependent and largely influenced by gene profile. Cancer cells survive within the TME through mechanisms of energy reprogramming. Given the genetic predisposition to various metabolic disorders, research into personalized pharmacotherapy will enhance the long-term efficacy of anti-cancer agents and mitigate drug resistance. Cancer precision medicine based on MR to suppress cancer growth while enhancing immunity is part of the hallmark of cancer research. While this mini review gives some examples of the forms of cancer with distinct energy requirements, obstructing the sources of these energies to rewire metabolic output will give essential requirements for personalized medicine.
Author contributions
TG: Writing–original draft, Conceptualization. LY: Writing–review and editing. YZ: Writing–review and editing. OB: Writing–review and editing. XY: Visualization, Writing–original draft.
Funding
The author(s) declare that financial support was received for the research, authorship, and/or publication of this article. Excellent Youth Project of Natural Science Foundation of Heilongjiang Province (No: YQ2022H015).
Conflict of interest
The authors declare that the research was conducted in the absence of any commercial or financial relationships that could be construed as a potential conflict of interest.
Publisher’s note
All claims expressed in this article are solely those of the authors and do not necessarily represent those of their affiliated organizations, or those of the publisher, the editors and the reviewers. Any product that may be evaluated in this article, or claim that may be made by its manufacturer, is not guaranteed or endorsed by the publisher.
References
Abou Khouzam, R., Sharda, M., Rao, S. P., Kyerewah-Kersi, S. M., Zeinelabdin, N. A., Mahmood, A. S., et al. (2023). Chronic hypoxia is associated with transcriptomic reprogramming and increased genomic instability in cancer cells. Front. Cell Dev. Biol. 11, 1095419. doi:10.3389/fcell.2023.1095419
Alistar, A., Morris, B. B., Desnoyer, R., Klepin, H. D., Hosseinzadeh, K., Clark, C., et al. (2017). Safety and tolerability of the first-in-class agent CPI-613 in combination with modified FOLFIRINOX in patients with metastatic pancreatic cancer: a single-centre, open-label, dose-escalation, phase 1 trial. Lancet Oncol. 18 (6), 770–778. doi:10.1016/S1470-2045(17)30314-5
An, F., Chang, W., Song, J., Zhang, J., Li, Z., Gao, P., et al. (2024). Reprogramming of glucose metabolism: metabolic alterations in the progression of osteosarcoma. J. Bone Oncol. 44, 100521. doi:10.1016/j.jbo.2024.100521
Bigos, K. J., Quiles, C. G., Lunj, S., Smith, D. J., Krause, M., Troost, E. G., et al. (2024). Tumour response to hypoxia: understanding the hypoxic tumour microenvironment to improve treatment outcome in solid tumours. Front. Oncol. 14, 1331355. doi:10.3389/fonc.2024.1331355
Buck, M. D., Sowell, R. T., Kaech, S. M., and Pearce, E. L. (2017). Metabolic instruction of immunity. Cell 169 (4), 570–586. doi:10.1016/j.cell.2017.04.004
Carlino, M. S., Larkin, J., and Long, G. V. (2021). Immune checkpoint inhibitors in melanoma. Lancet 398 (10304), 1002–1014. doi:10.1016/S0140-6736(21)01206-X
Chen, Y., Wang, B., Zhao, Y., Shao, X., Wang, M., Ma, F., et al. (2024). Metabolomic machine learning predictor for diagnosis and prognosis of gastric cancer. Nat. Commun. 15 (1), 1657. doi:10.1038/s41467-024-46043-y
Chianese, U., Papulino, C., Ali, A., Ciardiello, F., Cappabianca, S., Altucci, L., et al. (2023). FASN multi-omic characterization reveals metabolic heterogeneity in pancreatic and prostate adenocarcinoma. J. Transl. Med. 21 (1), 32. doi:10.1186/s12967-023-03874-5
Choe, J. H., Kawase, T., Xu, A., Guzman, A., Obradovic, A. Z., Low-Calle, A. M., et al. (2023). Li-fraumeni syndrome-associated dimer-forming mutant p53 promotes transactivation-independent mitochondrial cell death. Cancer Discov. 13 (5), 1250–1273. doi:10.1158/2159-8290.CD-22-0882
Ciardiello, F., Ciardiello, D., Martini, G., Napolitano, S., Tabernero, J., and Cervantes, A. (2022). Clinical management of metastatic colorectal cancer in the era of precision medicine. CA Cancer J. Clin. 72 (4), 372–401. doi:10.3322/caac.21728
Cui, X., Zhao, J., Li, G., Yang, C., Yang, S., Zhan, Q., et al. (2023). Blockage of EGFR/AKT and mevalonate pathways synergize the antitumor effect of temozolomide by reprogramming energy metabolism in glioblastoma. Cancer Commun. (Lond) 43 (12), 1326–1353. doi:10.1002/cac2.12502
Das, C., Adhikari, S., Bhattacharya, A., Chakraborty, S., Mondal, P., Yadav, S. S., et al. (2023). Epigenetic-metabolic interplay in the DNA damage response and therapeutic resistance of breast cancer. Cancer Res. 83 (5), 657–666. doi:10.1158/0008-5472.CAN-22-3015
Das, C., Bhattacharya, A., Adhikari, S., Mondal, A., Mondal, P., Adhikary, S., et al. (2024). A prismatic view of the epigenetic-metabolic regulatory axis in breast cancer therapy resistance. Oncogene 43 (23), 1727–1741. doi:10.1038/s41388-024-03054-9
Dong, Y., Yuan, Q., Ren, J., Li, H., Guo, H., Guan, H., et al. (2023). Identification and characterization of a novel molecular classification incorporating oxidative stress and metabolism-related genes for stomach adenocarcinoma in the framework of predictive, preventive, and personalized medicine. Front. Endocrinol. (Lausanne) 14, 1090906. doi:10.3389/fendo.2023.1090906
Gharib, E., Veilleux, V., Boudreau, L. H., Pichaud, N., and Robichaud, G. A. (2023). Platelet-derived microparticles provoke chronic lymphocytic leukemia malignancy through metabolic reprogramming. Front. Immunol. 14, 1207631. doi:10.3389/fimmu.2023.1207631
Grkovski, M., Goel, R., Krebs, S., Staton, K. D., Harding, J. J., Mellinghoff, I. K., et al. (2020). Pharmacokinetic assessment of (18)F-(2S,4R)-4-Fluoroglutamine in patients with cancer. J. Nucl. Med. 61 (3), 357–366. doi:10.2967/jnumed.119.229740
Kelly, W., Diaz Duque, A. E., Michalek, J., Konkel, B., Caflisch, L., Chen, Y., et al. (2023). Phase II investigation of TVB-2640 (denifanstat) with bevacizumab in patients with first relapse high-grade astrocytoma. Clin. Cancer Res. 29 (13), 2419–2425. doi:10.1158/1078-0432.CCR-22-2807
Li, H. M., Li, X., Xia, R., Zhang, X., Jin, T. Z., and Zhang, H. S. (2024b). PHGDH knockdown increases sensitivity to SR1, an aryl hydrocarbon receptor antagonist, in colorectal cancer by activating the autophagy pathway. FEBS J. 291 (8), 1780–1794. doi:10.1111/febs.17080
Li, M., Yu, J., Ju, L., Wang, Y., Jin, W., Zhang, R., et al. (2024a). USP43 stabilizes c-Myc to promote glycolysis and metastasis in bladder cancer. Cell Death Dis. 15 (1), 44. doi:10.1038/s41419-024-06446-7
Li, S., Xu, L., Wu, G., Huang, Z., Huang, L., Zhang, F., et al. (2023a). Remodeling serine synthesis and metabolism via nanoparticles (NPs)-Mediated CFL1 silencing to enhance the sensitivity of hepatocellular carcinoma to sorafenib. Adv. Sci. (Weinh) 10 (19), e2207118. doi:10.1002/advs.202207118
Li, Z., Chen, S., He, X., Gong, S., Sun, L., and Weng, L. (2023b). SLC3A2 promotes tumor-associated macrophage polarization through metabolic reprogramming in lung cancer. Cancer Sci. 114 (6), 2306–2317. doi:10.1111/cas.15760
Lin, H., Fu, L., Li, P., Zhu, J., Xu, Q., Wang, Y., et al. (2023). Fatty acids metabolism affects the therapeutic effect of anti-PD-1/PD-L1 in tumor immune microenvironment in clear cell renal cell carcinoma. J. Transl. Med. 21 (1), 343. doi:10.1186/s12967-023-04161-z
Lin, J., Hou, L., Zhao, X., Zhong, J., Lv, Y., Jiang, X., et al. (2024). Switch of ELF3 and ATF4 transcriptional axis programs the amino acid insufficiency-linked epithelial-to-mesenchymal transition. Mol. Ther. 32 (6), 1956–1969. doi:10.1016/j.ymthe.2024.04.025
Liu, C., Yang, L., Gao, T., Yuan, X., Bajinka, O., and Wang, K. (2024b). A mini-review-cancer energy reprogramming on drug resistance and immune response. Transl. Oncol. 49, 102099. doi:10.1016/j.tranon.2024.102099
Liu, X., Ren, B., Ren, J., Gu, M., You, L., and Zhao, Y. (2024a). The significant role of amino acid metabolic reprogramming in cancer. Cell Commun. Signal 22 (1), 380. doi:10.1186/s12964-024-01760-1
Malik, N., Kim, Y. I., Yan, H., Tseng, Y. C., du Bois, W., Ayaz, G., et al. (2023). Dysregulation of mitochondrial translation caused by CBFB deficiency cooperates with mutant PIK3CA and is a vulnerability in breast cancer. Cancer Res. 83 (8), 1280–1298. doi:10.1158/0008-5472.CAN-22-2525
Mendes, C., Lemos, I., Francisco, I., Almodovar, T., Cunha, F., Albuquerque, C., et al. (2023). NSCLC presents metabolic heterogeneity, and there is still some leeway for EGF stimuli in EGFR-mutated NSCLC. Lung Cancer 182, 107283. doi:10.1016/j.lungcan.2023.107283
Miao, Y. Z., Wang, J., Hao, S. Y., Deng, Y. X., Zhang, Z., Jin, Z. P., et al. (2023). The inhibition of Aurora A kinase regulates phospholipid remodeling by upregulating LPCAT1 in glioblastoma. Neoplasma 70 (2), 260–271. doi:10.4149/neo_2023_221126N1140
Milella, M., Rutigliano, M., Lasorsa, F., Ferro, M., Bianchi, R., Fallara, G., et al. (2024). The role of MUC1 in renal cell carcinoma. Biomolecules 14 (3), 315. doi:10.3390/biom14030315
Ni, X., Lu, C. P., Xu, G. Q., and Ma, J. J. (2024). Transcriptional regulation and post-translational modifications in the glycolytic pathway for targeted cancer therapy. Acta Pharmacol. Sin. 45, 1533–1555. doi:10.1038/s41401-024-01264-1
Penuelas-Haro, I., Espinosa-Sotelo, R., Crosas-Molist, E., Herranz-Iturbide, M., Caballero-Diaz, D., Alay, A., et al. (2023). The NADPH oxidase NOX4 regulates redox and metabolic homeostasis preventing HCC progression. Hepatology 78 (2), 416–433. doi:10.1002/hep.32702
Popovici-Muller, J., Lemieux, R. M., Artin, E., Saunders, J. O., Salituro, F. G., Travins, J., et al. (2018). Discovery of AG-120 (ivosidenib): a first-in-class mutant IDH1 inhibitor for the treatment of IDH1 mutant cancers. ACS Med. Chem. Lett. 9 (4), 300–305. doi:10.1021/acsmedchemlett.7b00421
Rosario, S. R., Dong, B., Zhang, Y., Hsiao, H. H., Isenhart, E., Wang, J., et al. (2023). Metabolic dysregulation explains the diverse impacts of obesity in males and females with gastrointestinal cancers. Int. J. Mol. Sci. 24 (13), 10847. doi:10.3390/ijms241310847
Rulten, S. L., Grose, R. P., Gatz, S. A., Jones, J. L., and Cameron, A. J. M. (2023). The future of precision oncology. Int. J. Mol. Sci. 24 (16), 12613. doi:10.3390/ijms241612613
Safi, A., Saberiyan, M., Sanaei, M. J., Adelian, S., Davarani Asl, F., Zeinaly, M., et al. (2023). The role of noncoding RNAs in metabolic reprogramming of cancer cells. Cell Mol. Biol. Lett. 28 (1), 37. doi:10.1186/s11658-023-00447-8
Sun, L., Zhang, H., and Gao, P. (2022). Metabolic reprogramming and epigenetic modifications on the path to cancer. Protein Cell 13 (12), 877–919. doi:10.1007/s13238-021-00846-7
Sun, X., Nong, M., Meng, F., Sun, X., Jiang, L., Li, Z., et al. (2024). Architecting the metabolic reprogramming survival risk framework in LUAD through single-cell landscape analysis: three-stage ensemble learning with genetic algorithm optimization. J. Transl. Med. 22 (1), 353. doi:10.1186/s12967-024-05138-2
Thomas, D., Wu, M., Nakauchi, Y., Zheng, M., Thompson-Peach, C. A. L., Lim, K., et al. (2023). Dysregulated lipid synthesis by oncogenic IDH1 mutation is a targetable synthetic lethal vulnerability. Cancer Discov. 13 (2), 496–515. doi:10.1158/2159-8290.CD-21-0218
Tsai, C. H., Chuang, Y. M., Li, X., Yu, Y. R., Tzeng, S. F., Teoh, S. T., et al. (2023). Immunoediting instructs tumor metabolic reprogramming to support immune evasion. Cell Metab. 35 (1), 118–133.e7. doi:10.1016/j.cmet.2022.12.003
Wamsley, N. T., Wilkerson, E. M., Guan, L., LaPak, K. M., Schrank, T. P., Holmes, B. J., et al. (2023). Targeted proteomic quantitation of NRF2 signaling and predictive biomarkers in HNSCC. Mol. Cell Proteomics 22 (11), 100647. doi:10.1016/j.mcpro.2023.100647
Wang, H., Liu, B., Long, J., Yu, J., Ji, X., Li, J., et al. (2023a). Integrative analysis identifies two molecular and clinical subsets in Luminal B breast cancer. iScience 26 (9), 107466. doi:10.1016/j.isci.2023.107466
Wang, L., Dou, X., Xie, L., Zhou, X., Liu, Y., Liu, J., et al. (2024a). Metabolic landscape of osteosarcoma: reprogramming of lactic acid metabolism and metabolic communication. Front. Biosci. Landmark Ed. 29 (2), 83. doi:10.31083/j.fbl2902083
Wang, L., Mao, X., Yu, X., Su, J., Li, Z., Chen, Z., et al. (2024b). FPR3 reprograms glycolytic metabolism and stemness in gastric cancer via calcium-NFATc1 pathway. Cancer Lett. 593, 216841. doi:10.1016/j.canlet.2024.216841
Wang, N., Wang, W., Wang, X., Mang, G., Chen, J., Yan, X., et al. (2022). Histone lactylation boosts reparative gene activation post-myocardial infarction. Circ. Res. 131 (11), 893–908. doi:10.1161/CIRCRESAHA.122.320488
Wang, Q., Liu, J., Chen, Z., Zheng, J., Wang, Y., and Dong, J. (2024d). Targeting metabolic reprogramming in hepatocellular carcinoma to overcome therapeutic resistance: a comprehensive review. Biomed. Pharmacother. 170, 116021. doi:10.1016/j.biopha.2023.116021
Wang, S., and Zhang, S. (2024). A novel eight-gene signature for lipid metabolism predicts the progression of cervical squamous cell carcinoma and endocervical adenocarcinoma. Reprod. Sci. 31 (2), 514–531. doi:10.1007/s43032-023-01364-z
Wang, X., Su, S., Zhu, Y., Cheng, X., Cheng, C., Chen, L., et al. (2023b). Metabolic Reprogramming via ACOD1 depletion enhances function of human induced pluripotent stem cell-derived CAR-macrophages in solid tumors. Nat. Commun. 14 (1), 5778. doi:10.1038/s41467-023-41470-9
Wang, Z., Zhang, Y., Yang, X., Zhang, T., Li, Z., Zhong, Y., et al. (2024c). Genetic and molecular characterization of metabolic pathway-based clusters in esophageal squamous cell carcinoma. Sci. Rep. 14 (1), 6200. doi:10.1038/s41598-024-56391-w
Wu, C., Yu, S., Wang, Y., Gao, Y., Xie, X., and Zhang, J. (2023d). Metabolic-suppressed cancer-associated fibroblasts limit the immune environment and survival in colorectal cancer with liver metastasis. Front. Pharmacol. 14, 1212420. doi:10.3389/fphar.2023.1212420
Wu, J., Zhou, J., Chai, Y., Qin, C., Cai, Y., Xu, D., et al. (2023a). Novel prognostic features and personalized treatment strategies for mitochondria-related genes in glioma patients. Front. Endocrinol. (Lausanne) 14, 1172182. doi:10.3389/fendo.2023.1172182
Wu, L., Yan, J., Bai, Y., Chen, F., Zou, X., Xu, J., et al. (2023b). An invasive zone in human liver cancer identified by Stereo-seq promotes hepatocyte-tumor cell crosstalk, local immunosuppression and tumor progression. Cell Res. 33 (8), 585–603. doi:10.1038/s41422-023-00831-1
Wu, Z., Li, X., Gu, Z., Xia, X., and Yang, J. (2023c). Pyrimidine metabolism regulator-mediated molecular subtypes display tumor microenvironmental hallmarks and assist precision treatment in bladder cancer. Front. Oncol. 13, 1102518. doi:10.3389/fonc.2023.1102518
Xing, X., Hu, E., Ouyang, J., Zhong, X., Wang, F., Liu, K., et al. (2023). Integrated omics landscape of hepatocellular carcinoma suggests proteomic subtypes for precision therapy. Cell Rep. Med. 4 (12), 101315. doi:10.1016/j.xcrm.2023.101315
Xu, W., Lu, J., Liu, W. R., Anwaier, A., Wu, Y., Tian, X., et al. (2023b). Heterogeneity in tertiary lymphoid structures predicts distinct prognosis and immune microenvironment characterizations of clear cell renal cell carcinoma. J. Immunother. Cancer 11 (12), e006667. doi:10.1136/jitc-2023-006667
Xu, X., Peng, Q., Jiang, X., Tan, S., Yang, Y., Yang, W., et al. (2023a). Metabolic reprogramming and epigenetic modifications in cancer: from the impacts and mechanisms to the treatment potential. Exp. Mol. Med. 55 (7), 1357–1370. doi:10.1038/s12276-023-01020-1
Xu, X., Yu, Y., Zhang, W., Ma, W., He, C., Qiu, G., et al. (2024). SHP-1 inhibition targets leukaemia stem cells to restore immunosurveillance and enhance chemosensitivity by metabolic reprogramming. Nat. Cell Biol. 26 (3), 464–477. doi:10.1038/s41556-024-01349-3
Yan, F., Liu, C., Song, D., Zeng, Y., Zhan, Y., Zhuang, X., et al. (2024). Integration of clinical phenoms and metabolomics facilitates precision medicine for lung cancer. Cell Biol. Toxicol. 40 (1), 25. doi:10.1007/s10565-024-09861-w
Yang, J., Ren, B., Ren, J., Yang, G., Fang, Y., Wang, X., et al. (2023b). Epigenetic reprogramming-induced guanidinoacetic acid synthesis promotes pancreatic cancer metastasis and transcription-activating histone modifications. J. Exp. Clin. Cancer Res. 42 (1), 155. doi:10.1186/s13046-023-02698-x
Yang, K., Wang, X., Song, C., He, Z., Wang, R., Xu, Y., et al. (2023a). The role of lipid metabolic reprogramming in tumor microenvironment. Theranostics 13 (6), 1774–1808. doi:10.7150/thno.82920
Yu, W., Chen, Y., Putluri, N., Osman, A., Coarfa, C., Putluri, V., et al. (2023). Evolution of cisplatin resistance through coordinated metabolic reprogramming of the cellular reductive state. Br. J. Cancer 128 (11), 2013–2024. doi:10.1038/s41416-023-02253-7
Yu, X., Ma, R., Wu, Y., Zhai, Y., and Li, S. (2018). Reciprocal regulation of metabolic reprogramming and epigenetic modifications in cancer. Front. Genet. 9, 394. doi:10.3389/fgene.2018.00394
Yuan, X., Ouedraogo, S. Y., Trawally, M., Tan, Y., and Bajinka, O. (2024). Cancer energy reprogramming and the immune responses. Cytokine 177, 156561. doi:10.1016/j.cyto.2024.156561
Zhang, Q., Wei, T., Jin, W., Yan, L., Shi, L., Zhu, S., et al. (2024b). Deficiency in SLC25A15, a hypoxia-responsive gene, promotes hepatocellular carcinoma by reprogramming glutamine metabolism. J. Hepatol. 80 (2), 293–308. doi:10.1016/j.jhep.2023.10.024
Zhang, S., Liu, Y., Sun, Y., Liu, Q., Gu, Y., Huang, Y., et al. (2024c). Aberrant R-loop-mediated immune evasion, cellular communication, and metabolic reprogramming affect cancer progression: a single-cell analysis. Mol. Cancer 23 (1), 11. doi:10.1186/s12943-023-01924-6
Zhang, Y., Wu, M. J., Lu, W. C., Li, Y. C., Chang, C. J., and Yang, J. Y. (2024a). Metabolic switch regulates lineage plasticity and induces synthetic lethality in triple-negative breast cancer. Cell Metab. 36 (1), 193–208.e8. doi:10.1016/j.cmet.2023.12.003
Zhang, Z. J., Wu, Q. F., Ren, A. Q., Chen, Q., Shi, J. Z., Li, J. P., et al. (2023). ATF4 renders human T-cell acute lymphoblastic leukemia cell resistance to FGFR1 inhibitors through amino acid metabolic reprogramming. Acta Pharmacol. Sin. 44 (11), 2282–2295. doi:10.1038/s41401-023-01108-4
Zhao, Z., Mei, Y., Wang, Z., and He, W. (2022). The effect of oxidative phosphorylation on cancer drug resistance. Cancers (Basel) 15 (1), 62. doi:10.3390/cancers15010062
Zhou, P., Xiao, Y., Zhou, X., Fang, J., Zhang, J., Liu, J., et al. (2024). Mapping spatiotemporal heterogeneity in multifocal breast tumor progression by noninvasive ultrasound elastography-guided mass spectrometry imaging strategy. JACS Au 4 (2), 465–475. doi:10.1021/jacsau.3c00589
Zhu, X., Chen, J., Li, W., Xu, Y., Shan, J., Hong, J., et al. (2024). Hypoxia-responsive CAR-T cells exhibit reduced exhaustion and enhanced efficacy in solid tumors. Cancer Res. 84 (1), 84–100. doi:10.1158/0008-5472.CAN-23-1038
Glossary
ACOD1 Aconitate decarboxylase 1
AhR Aryl hydrocarbon receptor
AML Acute myeloid leukemia
ATF4 Activating transcription factor 4
CAF Cancer-associated fibroblasts
CAR Chimeric antigen receptor
CBFB Core binding factor subunit β
ccRCC Clear cell renal cell carcinoma
CESC Cervical squamous cell carcinoma
CFL1 Co-delivery of cofilin 1
CLL Chronic lymphocytic leukemia
CRC Colorectal cancer
EGFR Epidermal growth factor receptor
EMT Epithelial-to-mesenchymal transition
ENO1 Enolase 1
ESCC Esophageal squamous cell carcinoma
EZH2 Enhancer of zeste homolog 2
FASN Fatty acid synthase
FAO Fatty acid β-oxidation
FBXW7 F-box and WD repeat domain containing 7
FGFR1 Fibroblast growth factor receptor 1
FPR3 Formyl peptide receptor 3
HCC Hepatocellular carcinoma
HIF1 Hypoxia-inducible factor 1
HK2 Hexokinase 2
IDH1 Isocitrate dehydrogenase 1
KRAS Kristen rat sarcoma
LBBC Luminal B breast cancer
LDHA Lactate dehydrogenase A
LMRGs Lipid metabolism-related genes
LSCs Leukemia stem cells
LUAD Lung adenocarcinoma
MPC3 Mitochondrial pyruvate carrier 3
MR Metabolic reprogramming
MSI Mass spectrometry imaging
MUC1 Mucin 1
NFATc1 Nuclear factor of activated T cells 1
NOX4 NADPH oxidase 4
NOTCH3 Notch homolog protein 3
NDUFAF6 NADH dehydrogenase (ubiquinone) complex I assembly factor 6
Nrf2 Nuclear factor erythroid 2-related factor 2
NSCLC Non-small cell lung cancer
OXPHOS Oxidative phosphorylation
OC Ovarian cancer
PDAC Pancreatic ductal adenocarcinoma
PHGDH Phosphoglycerate dehydrogenase
PKM2 Pyruvate kinase M2
PPP Pentose phosphate pathway
PI3K Phosphoinositide 3-kinase
PFS Progression-free survival
PFKP Phosphofructokinase platelet
RCC Renal cell carcinoma
SH-2 Src homology region 2
Shp1 Phosphatase 1
SIX1 Sine oculis homeobox homolog 1
SLC3A2 Solute carrier family 3 member 2
SLC25A15 Solute carrier family 25 member 15
SLC16A9 Solute carrier family 16 member 9
STAD Stomach adenocarcinoma
TAM Tumor-associated macrophages
T-ALL T-cell acute lymphoblastic leukemia
TGF-β Transforming growth factor β
TME Tumor microenvironment
TNBC Triple-negative breast cancer
MPC3 Mitochondrial pyruvate carrier 3
USP43 Ubiquitin specific peptidase 43
Keywords: metabolic reprogramming, precision medicine, treatment strategies, immune cell, mechanism
Citation: Gao T, Yang L, Zhang Y, Bajinka O and Yuan X (2024) Cancer metabolic reprogramming and precision medicine-current perspective. Front. Pharmacol. 15:1450441. doi: 10.3389/fphar.2024.1450441
Received: 17 June 2024; Accepted: 04 October 2024;
Published: 17 October 2024.
Edited by:
Elisa Giovannetti, VU Medical Center, NetherlandsReviewed by:
Chiara Papulino, University of Campania Luigi Vanvitelli, ItalyCopyright © 2024 Gao, Yang, Zhang, Bajinka and Yuan. This is an open-access article distributed under the terms of the Creative Commons Attribution License (CC BY). The use, distribution or reproduction in other forums is permitted, provided the original author(s) and the copyright owner(s) are credited and that the original publication in this journal is cited, in accordance with accepted academic practice. No use, distribution or reproduction is permitted which does not comply with these terms.
*Correspondence: Xingxing Yuan, eXVhbnhpbmd4aW5nQGhsanVjbS5lZHUuY24=