- 1Chengdu University of Traditional Chinese Medicine, Chengdu, China
- 2Chengdu Medical College, Chengdu, China
- 3Department of Nephrology, First Affiliated Hospital of Chengdu Medical College, Chengdu, China
- 4TCM Preventative Treatment Research Center of Chengdu Medical College, Chengdu, China
Gut microecology,the complex community consisting of microorganisms and their microenvironments in the gastrointestinal tract, plays a vital role in maintaining overall health and regulating various physiological and pathological processes. Recent studies have highlighted the significant impact of gut microecology on the regulation of uric acid metabolism. Natural products, including monomers, extracts, and traditional Chinese medicine formulations derived from natural sources such as plants, animals, and microorganisms, have also been investigated for their potential role in modulating uric acid metabolism. According to research, The stability of gut microecology is a crucial link for natural products to maintain healthy uric acid metabolism and reduce hyperuricemia-related diseases. Herein, we review the recent advanced evidence revealing the bidirectional regulation between gut microecology and uric acid metabolism. And separately summarize the key evidence of natural extracts and herbal formulations in regulating both aspects. In addition,we elucidated the important mechanisms of natural products in regulating uric acid metabolism and secondary diseases through gut microecology, especially by modulating the composition of gut microbiota, gut mucosal barrier, inflammatory response, purine catalyzation, and associated transporters. This review may offer a novel insight into uric acid and its associated disorders management and highlight a perspective for exploring its potential therapeutic drugs from natural products.
1 Introduction
In recent years, there has been plenty of research interest in the role of gut microecology in regulating various physiological and pathological processes (Shen et al., 2023). The human gut microbiota, composed of trillions of microorganisms residing in the gastrointestinal tract, is crucial in maintaining overall health and metabolism (Li et al., 2024), and recent studies indicated that it is closely involved in regulating uric acid (UA) metabolism (Dang et al., 2023). For instance, certain gut microorganisms play a beneficial role in UA metabolism, facilitating the breakdown of purine and promoting the excretion of UA through the intestinal tract (Wang J. et al., 2022; Kasahara et al., 2023). On the contrary, many investigations implicated that an altered gut microecology could produce enzymes that contribute to the conversion of purine into UA or impair the excretion of UA, resulting in its accumulation and the development of hyperuricemia (HUA) (Wang Z. et al., 2022; Yin et al., 2022). These inconsistent data indicated the bidirectional effect of gut macroecology on UA metabolism, implying that a favorable interaction between gut microecological homeostasis and UA metabolism balance may be a candidate biomarker for health monitoring and drug target screening.
Natural products, including monomers, extracts, and herbal formulations derived from various sources such as plants, animals, and microorganisms, have been shown as potential drugs to modulate gut microecology and UA metabolism for body health (Guo et al., 2017; Yang L. et al., 2022). It testified that some plant extracts contain bioactive compounds such as polyphenols (Ye et al., 2022), flavonoids (Oteiza et al., 2018), which can selectively promote beneficial bacteria growth while inhibiting harmful ones. In addition, Chinese medicine herbal formulation compounds such as puerarin, glycyrrhizin, berberine, and baicalin significantly repair the gut barrier and reduce pro-inflammatory cytokine expression in the gastrointestinal tract (Wu et al., 2019). Besides regulating gut macroecology, certain extracts and herbal formulations possess UA-lowering properties, which can inhibit the production via diminishing xanthine oxidase (XO) activity or enhance the excretion of UA by adjusting UA transporters, thereby maintaining its physiological levels in the body (Xu et al., 2024). This viewpoint of natural products targeting gut microecology to modulate UA metabolism has recently been accepted theoretically. Considering the interwinding effect on gut microbiota and UA interactively, researchers reasoned that natural products could adjust UA balance via modulating gut microbiota composition, typically engaging an augmentation of beneficial bacteria and a diminution of harmful bacteria (Wang Z. et al., 2022). In recent years, many advanced studies have testified that natural products not only modulate gastrointestinal flora composition but also enhance gut barrier function, attenuate inflammatory responses, modulate purine metabolism, and regulate transporter activity (Bian et al., 2020; Mehmood et al., 2024). In summary, natural products showed promising potential for modulating the interwinding effect between gut microecology and UA metabolism. Therefore, we review the bidirectional regulating relationship between gut microbiota and UA metabolism and summarize natural products targeting gut microecology to modulate UA metabolism. It may provide an innovative avenue for developing natural products interventions that can effectively modulate UA metabolism and mitigate the risk of associated diseases.
2 Overview of uric acid metabolism and its functions
UA is a nitrogenous product resulting from the breakdown of purine nucleotides. It plays a significant role in the human body, acting as an antioxidant and participating in various physiological and pathological processes. The liver, gut, kidneys, and vascular endothelium are the primary tissues for UA metabolism. Endogenous UA production commences with the catabolism of nucleic acids, followed by conversion to xanthine via a series of enzymes, ultimately yielding UA through the action of XO (El Ridi and Tallima, 2017). Differently, exogenous UA synthesis is primarily derived from ingesting of purine-rich foods. Approximately one-third of UA excretion occurs in the gastrointestinal tract, with the remainder excreted from urine in the kidney via transporters (Yin et al., 2022) (Figure 1). Studies demonstrated that UA had a bidirectional role in regulating the body’s health, and a U-shaped correlation has been established between UA levels and total mortality (Crawley et al., 2022). A literature displayed that UA is a potent antioxidant, rivaling the antioxidant capacity of ascorbic acid, and possesses multifaceted functions, including blood pressure maintenance, anti-aging, and neuroprotection (Wen et al., 2024). However, elevated UA levels of HUA are associated with a spectrum of diseases, such as gout, kidney stones, chronic renal disease, hypertension, and metabolic syndrome (So and Thorens, 2010; Alvarez-Lario and Macarron-Vicente, 2011; Yanai et al., 2021). HUA is defined as blood UA levels exceeding 7.0 mg/dL for males or 5.7 mg/dL for females due to UA production surpassing its elimination (Yang B. et al., 2022). Of note, young males currently exhibit an increasing prevalence of HUA, and its incidence rate among Chinese adults was up to 24.4% (Zhang et al., 2021b). Thus, we should pay serious attention to UA metabolism and its homeostasis modulation for human health.
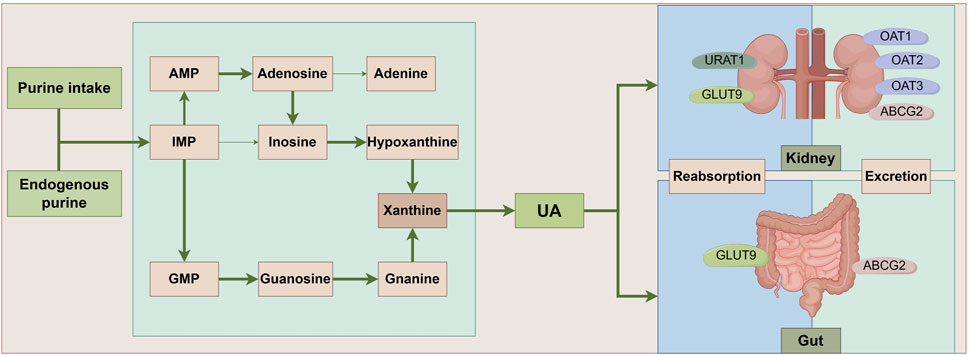
Figure 1. UA metabolic pathways. Ingested and endogenously produced purines impose a significant strain on the body’s purine nucleotide synthesis processes, culminating in the accumulation of intermediates such as IMP, AMP, and GMP. This accumulation triggers an elevated conversion of xanthine, facilitated by xanthine oxidase, ultimately leading to the generation of UA. Notably, approximately one-third of UA undergoes metabolism within the intestinal tract, with UA-associated transporter proteins playing pivotal roles in both the reabsorption and excretion of UA. ABCG2: ATP-Binding Cassette Subfamily G Member 2; AMP: Adenosine Monophosphate; GLUT9: Glucose Transporter 9; GMP: Guanosine Monophosphate; IMP:Inosine Monophosphate; OAT1: Organic Anion Transporter 1; OAT2: Organic Anion Transporter 2; OAT3: Organic Anion Transporter 3; UA: uric acid; URAT1: Uric Acid Transporter 1.
3 Gut microecology and uric acid metabolism
3.1 Mutual favorable effect between gut microbiota and uric acid metabolism
The gastrointestinal tract harbors a diverse ecosystem of microorganisms known as the gut microbiota. These microorganisms maintain a symbiotic relationship with the human host and play a vital role in various aspects of health, including immune regulation, nutrient absorption, and metabolism. Emerging evidence suggests that the gut microbiota significantly influences UA metabolism (Wang J. et al., 2022). To maintain the homeostasis of UA, the gut bacteria adapt to the host’s microenvironment, serving as a crucial regulator of UA production, metabolism, and excretion pathways. The purine degradation clusters have been identified in diverse gut bacterial groups, including Bacillota, Fusobacteriota and Pseudomonadota (Kasahara et al., 2023). Besides, changes in metabolizing gene clusters facilitate UA’s metabolic conversion into hypoxanthine or short-chain fatty acids (SCFAs) (Liu et al., 2023b). Hypoxanthine plays a pivotal role in energy metabolism and safeguards the gut barrier integrity mediated by gut epithelial cells (Lee et al., 2018). SCFAs are involved in gut endocrine and immune modulation, contributing to the treatment and recovery of various metabolic diseases (van der Hee and Wells, 2021). In addition, an investigation implied that an increase in the abundance of beneficial gut bacteria correlates with the upregulation of UA metabolism-related excretory genes, such as ATP-binding cassette sub-family G member 2 (ABCG2) and Organic Anion Transporter 1 (OAT1), and the downregulation of absorption genes, including Urate Transporter 1 (URAT1) and Glucose Transporter 9 (GLUT9) (Li et al., 2021b). For instance, Lactobacillus, a member of the gut flora, exhibits inhibitory effects on the XO and purine nucleoside phosphorylase while promoting the activity of nucleoside hydrolase RihA-C to curtail urate synthesis, and it upregulates ABCG2, enhancing UA excretion as well (Li M. et al., 2023). These studies indicated that the gut microbiota and its metabolites regulate gut microecological functions and facilitate normal UA metabolism (Figure 2).
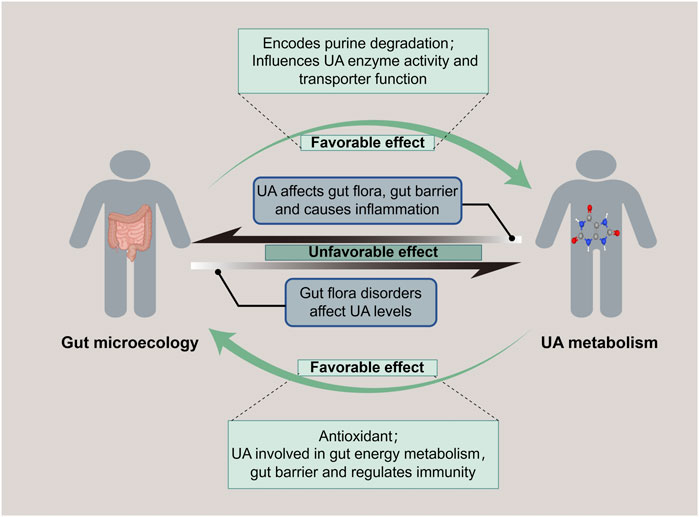
Figure 2. Bidirectional regulation of gut microecology and UA metabolism. Gut microecology promotes both purine degradation to reduce UA level and UA synthesis to increase UA level. While UA is related to gut energy metabolism and maintenance of gut barrier, it also affects the gut flora, destroys the mucosal barrier, and causes an imbalance of gut microecology.
Conversely, UA plays a pivotal role in reverse-modulating the microbiota of the gastrointestinal tract, in addition to the usual consideration of safeguarding cardiac, vascular, and neuronal cells from oxidative stress as a physiological antioxidant. A report evidenced that appropriate levels of UA have conferred an evolutionary advantage on humans by elevating antioxidant markers and fostering the diversity of gut flora (Wada et al., 2022). Moreover, UA is indispensable for free radical scavenging and offers a fundamental tissue repair mechanism that benefits gut microbiota (El Ridi and Tallima, 2017). Purine, a source of carbon and energy for gut bacteria, is well-known as the precursor of UA synthesis, which is also frequently implicated in the auto-regulation of gut microecology (Kasahara et al., 2023). Notably, in healthy individuals with normal levels of UA, butyrate-producing bacteria are more abundant, and other beneficial bacteria, such as Bifidobacterium and Clostridium sensustricto1, are significantly enriched. In turn, these dominant microflora collectively maintain the integrity of the gut barrier, exert immunomodulatory effects, and possess anti-inflammatory properties for favoring UA metabolism (Mendez-Salazar et al., 2021). It has indicated that an appropriate level of UA is crucial for maintaining gut ecological flora diversity to modulate metabolic homeostasis favorably.
3.2 Reciprocal unfavorable effect between dysregulated gut microbiota and uric acid metabolism
A diminution of beneficial bacteria and a proliferation of harmful bacteria within the gut microbiota can incite microecological imbalance, which perturbs UA metabolism, consequently enhancing UA production and diminishing excretion, ultimately resulting in HUA. A Mendelian randomization study has implicated thirty distinct gut microbiota species in modulating UA levels, with five specific types exhibiting a notable influence on blood UA concentrations (Wang et al., 2023). Various gut bacteria exert diverse effects on UA metabolism. Studies have shown that elevated yeast proliferation and decreased bifidobacteria abundance facilitate purine metabolism and augment UA production (Chiaro et al., 2017; Gong et al., 2022). Furthermore, the disruption of gut microbiota induced by various causal agents can also significantly impact UA levels. Recent literature demonstrated that antibiotic-induced alteration in gut microbiota resembled that observed in animals with HUA, with a reduced abundance of purine salvage proteins expression in the gut microbiota, increasing the risk of HUA (Liu et al., 2023). In addition, exposure to the heavy metal nickel suppressed beneficial gut bacteria such as Lactobacillus and Lachnospiraceae, while promoting the proliferation of harmful bacteria like Parabacteriodes and Escherichia-Shigella (Yang et al., 2023). This impairment in purine degradation elevates UA levels and systemic inflammatory response. In this case, gout can be triggered by the disruption of the gut barrier and the subsequent release of inflammatory factors, stemming from a decrease in SCFAs, a metabolite of the gut microbiota, and an increase in lipopolysaccharides (Liu et al., 2022). These indicated that disorder modulation of UA resulting from an imbalance of gut microecology is a common cause for various diseases.
On the contrary, elevated levels of UA have a significant effect on gut microecology reciprocally. A study unveiled that distinctive miRNAs associated with the onset and remission of UA in Apostichopus japonicus hydrolysate. These miRNAs exhibited a strong correlation with the metabolism of tryptophan, bile acid, and SCFAs, suggesting a bidirectional interplay between UA metabolism, gut bacteria and their metabolites (Fan et al., 2022). A further discovery of distinct gut bacterial flora in patients with varying UA levels. In particular, the abundance of enterobacteria capable of producing SCFAs, such as Ruminococcus, was decreased, while the abundance of Proteobacteria and Bacteroides was increased in patients with HUA (Liang et al., 2022). These findings support the hypothesis that gut flora and microbial markers could be predictive models for HUA. Studies have shown that with escalated UA levels, the bacterial flora diversity was diminished, which led to a downregulation of tight junction proteins Occludin and Claudin-1, compromising the gut barrier, enhancing permeability and promoting an imbalance between Th17 and Treg cells in the gut (Lv et al., 2020; Wang P. et al., 2022). The downregulated of UA-secreted transporter proteins ABCG2, OAT1, and OAT3, while upregulated of the UA reabsorption transporter protein URAT1, which contributed to HUA and its continuous secondary disadvantage of the gut microecology (Wu et al., 2022). Notably, cultivating beneficial bacteria, such as lactobacilli, can produce SCFAs and mitigate the severity of HUA and its associated secondary damage (Wan et al., 2020). In a word, abnormally elevated UA can disrupt gut flora’s composition, compromise the gut barrier, and subsequently trigger imbalanced gut microecology modulation.
4 Natural products are innovative modulators of gut microecology
Natural products are compounds derived from natural sources, such as plants, animals, or microorganisms, encompassing a plenty of substances, including monomers, extracts, and herb formulations. In recent years, many investigations have revealed that natural products were innovative drugs with the potential to modulate gut microecology and subsequently regulate UA metabolism. Because more research is interested in the role of extracts and herb formulations modulating the gut microbiota balance, thus we enumerate their action below.
4.1 Natural extracts engage in modulating gut microecology
The advent of liquid chromatography-mass spectrometry (LC-MS) has ushered in a new era of precision in the effective employment of natural products, enabling the accurate determination of phytochemical extract profiles and predictive assessment of plasma absorption capacity based on the molecular weight and lipophilicity of individual chemical components (Selby-Pham et al., 2018). Under such conditions, numerous natural products, particularly these extracts in managing gut microecology, have garnered extensive attention. It has been evidenced that extracts such as Rhodiola crenulata extract (Wang et al., 2021), Ginkgo biloba leaves extract (Wang Y. et al., 2022), Centella asiatica ethanol extract (Li H. et al., 2021), have exhibited promising potential in alleviating gut inflammation by reparative actions on the gut mucosal barrier and modulation of gut flora composition. It was noteworthy that individual natural product preparations exhibited significant therapeutic value. For instance, the Wuzhi capsule, derived from Schisandra chinensis extract, is a clinically recognized synergistic and detoxifying formulation, that can mitigate gut microecological dysbiosis induced by mycophenolate through the inhibition of oxidative stress, inflammation, and apoptosis (Zhang et al., 2022c). In the realm of natural products, our investigation revealed that polysaccharides and polyphenols emerge as potent material foundations for meticulously managing gut microecology (Lin et al., 2019; Pascuta et al., 2022). Diverse polysaccharide fractions, encompassing those derived from Lycium barbarum polysaccharides (Gao et al., 2021), marine algal polysaccharides (Yu et al., 2023), alongside polyphenolic fractions such as blueberry polyphenols (Polewski et al., 2020), tea polyphenols (Ye et al., 2022), exhibit remarkable capability of regulating bacterial flora composition, whereby benefit for bolstering the gut mucosal barrier, mitigating oxidative stress, and gut inflammation. Meanwhile, a nuanced analysis of the intricate relationships between dietary polyphenols and the gut microbiota further verified that polyphenols can modulate gut microecology (Koudoufio et al., 2020). Interestingly, many innovative natural antimicrobials have been developed with the rapid progress in drug discovery based on natural extracts modulating gut microecology in recent years (Guglielmi et al., 2020). It represents a cornerstone of screening natural products for combating human health threats of gut microbiota-related disease and paves a new avenue for natural products to be used in clinical applications.
4.2 Herbal formulations and their ingredients based on clinical efficacy modulate gut microecology
Natural products constitute a pivotal source of herbal remedies in traditional Chinese medicine. Herbal formulations contain massive natural ingredients owning multifaceted pharmaceutical targets and diverse medical therapeutic efficacy. Due to their pharmaceutical activity, these formulations are applied in clinical treatment as pharmaceutical prescriptions in China. Studies have testified that many herbal formulations and their active ingredients exhibited a role in sustaining gut microecological stability with an intricate reciprocal complementary mechanism (Zheng et al., 2022). However, pieces of literature implied that ingredients within herbal formulations displayed vast heterogeneity. For instance, in a mouse model of spleen deficiency syndrome, gut barrier impairment, and disrupted microbiota metabolism were observed. It evidenced that the active polysaccharide component S-3 in Sijunzi decoction primarily enhanced gut immune function and gut microbiomes, whereas its non-polysaccharide component was chiefly engaged in ameliorating gut motility disorders (Ma et al., 2021). It evidenced that metabolic pathways of the gut microbiota play a crucial role in regulating gut microecological balance, especially carbohydrates, SCFAs, and amine metabolites, which are associated with the gut flora. A recent study implied that these metabolites are critical targets of Zengye decoction by modulating gut microecology in constipated rats mode (Liu et al., 2019). Besides, another classic prescription Shenling Baizhu San remarkably alleviated gut microecological disorders induced by indigestion, thereby modulating microflora energy metabolism, amino acid metabolism, and other related pathways (Zhang et al., 2020). In conclusion, evidence suggests that the multi-component and multi-target effects feature of herbal formulations is well-suited to modulating the complexity of gut microecology, and this afford them effectively regulate gut microecological homeostasis. Whereas, despite the clinical efficacy of herbal formulations and their ingredients, many researchers still reckon that there will be a long way and substantial challenging work needs to be done for screening feasible clinical natural products drugs (Zhou et al., 2016).
5 Natural products regulate uric acid metabolism
Several natural products have shown promising effects in modulating UA metabolism, such as resveratrol and quercetin, have been reported to regulate UA metabolism through the inhibition of XO, a key enzyme in UA production. In general mechanism, studies speculated that natural products mainly switch the activity of key enzymes involved in the production process of UA or target critical transporters of its excretion, thereby orchestrating the balance of UA metabolism.
5.1 Natural extracts engage in modulating uric acid metabolism
In recent years, many researchers have manifested that the utilization of natural extracts could effectively regulate the synthesis and excretion metabolism of UA (Zhang X. et al., 2022). XO is a pivotal enzyme for UA production, and its activity can be modulated by administering natural inhibitors (Sun et al., 2024). Compounds of natural extracts such as flavonoids, anthraquinones, and xanthones have been evaluated for their potential inhibitory effect of XO, employing techniques like 3-D QSAR analysis and molecular docking of natural XO inhibitors (Malik et al., 2019). Consistently, flavonoid extracts derived from saffron floral bio-residues have consistently exhibited antagonistic effects against HUA by modifying gut flora associated with host metabolism, inhibiting XO activity, and subsequently reducing UA synthesis (Chen et al., 2022). Intriguingly, in tandem with compound structure-based virtual screening for UA-producing inhibition, isopentenyl dihydro flavones have been identified from a natural herb database, which were as potential activated scaffolds as human urate transporter 1 (hURAT1) inhibitors for the treatment of gout (Chen et al., 2021). These finding emphasized the capability of flavonoids as a primary component for potentially controlling UA metabolism. In addition, tea polyphenols (Zhang G. et al., 2022), apple polyphenols (Cicero et al., 2017), and numerous other polyphenolic compounds also inhibited the activity of XO. Recent studies revealed that many other natural extracts, including the ethanol extract of Amomum villosum Lour (Dong et al., 2023), the active ingredient of Lagotis brachystachya (Zhu et al., 2021) and numerous others, not only inhibited XO but also regulated urate transporter proteins, thereby reducing UA production and promoting excretion. However, the underlying mechanisms of natural extracts involving UA metabolism are rather complicated, which may be influenced by numerous factors, including genetics, gender, health status, etc. Thus, it elicits challenges of natural extracts exerting the modulation of UA metabolism directly. Therefore, screening natural extracts targeting purine degradation, UA transporters, and gut microbiotas or their associated enzymes involved in the UA metabolism process becomes a new highlighting orientation to develop novel therapeutic agents for UA-associated disease treatment (Rullo et al., 2023).
5.2 Herbal formulations based on clinical efficacy modulate uric acid metabolism
With the gradually increased prevalence rates of UA dysmetabolism and its associated disorders in recent years, many researchers have oriented to exploring therapeutic natural products for HUA, particularly focusing on herbal formulations in China. Strikingly, it has testified that several herbal formulations and their ingredients exhibited robust clinical efficacy in modulating UA metabolism and mitigating symptoms of UA-related diseases. For instance, classic formulations such as Wuling San (Huang et al., 2023), Siwu decoction (Wang et al., 2016), Fuling-Zexie formula (Lu et al., 2024), and Simiao San (Zhang Y. et al., 2023) have exhibited modulatory effects on UA metabolism, encompassing inhibition of XO activity, modulation of UA transporter protein, regulation of inflammatory signaling pathways, such as NLRP3 complex signaling activity. Additionally, in a clinical trial of an herbal drug treating gouty arthritis, investigators revealed that the ingredients of Huzhang Granule, a traditional Chinese herbal compound, exerted a better capability of analgesic and anti-inflammatory effects, acquired a lower level of UA, even with less incidence of adverse effects, comparing with that of the etoricoxib control group (Wang et al., 2024). Furthermore, it has been reported that the Shuang-Qi gout capsule, a patented pharmaceutical prescription currently utilized in clinical application, exhibited its ability to benefit UA metabolism effectively in a dose-dependent manner, consequently treating gout-related tissue edema and pain (Kodithuwakku et al., 2013). In conclusion, numerous herbal formulations exerted significant efficacy in modulating UA metabolism. However, plenty of challenging work need to be done to clarify the complexity of their ingredient constitution and decipher their underlying mechanisms of UA metabolism modulation.
6 Complex mechanism of natural products modulating uric acid metabolism via its targeted gut microecology
As aforementioned, natural products, including various extracts and herbal formulas, can modulate gut microbiota and UA metabolism. It inspired researchers to speculate that an interwinding effect existed between gut microecology and UA metabolism, and natural products could modify gut microecology to orchestrate UA metabolism balance. Recent mechanistic studies indicated that natural products could target gut microbiota to orchestrate an intricate network of modifying its composition, gut mucosal barrier, inflammatory response, purine catalyzation, and associated transporters. Understanding these intriguing mechanisms of these natural products modulating UA metabolism balance may propose new strategies for managing HUA and its associated diseases. (Table 1; Table 2; Figure 3).
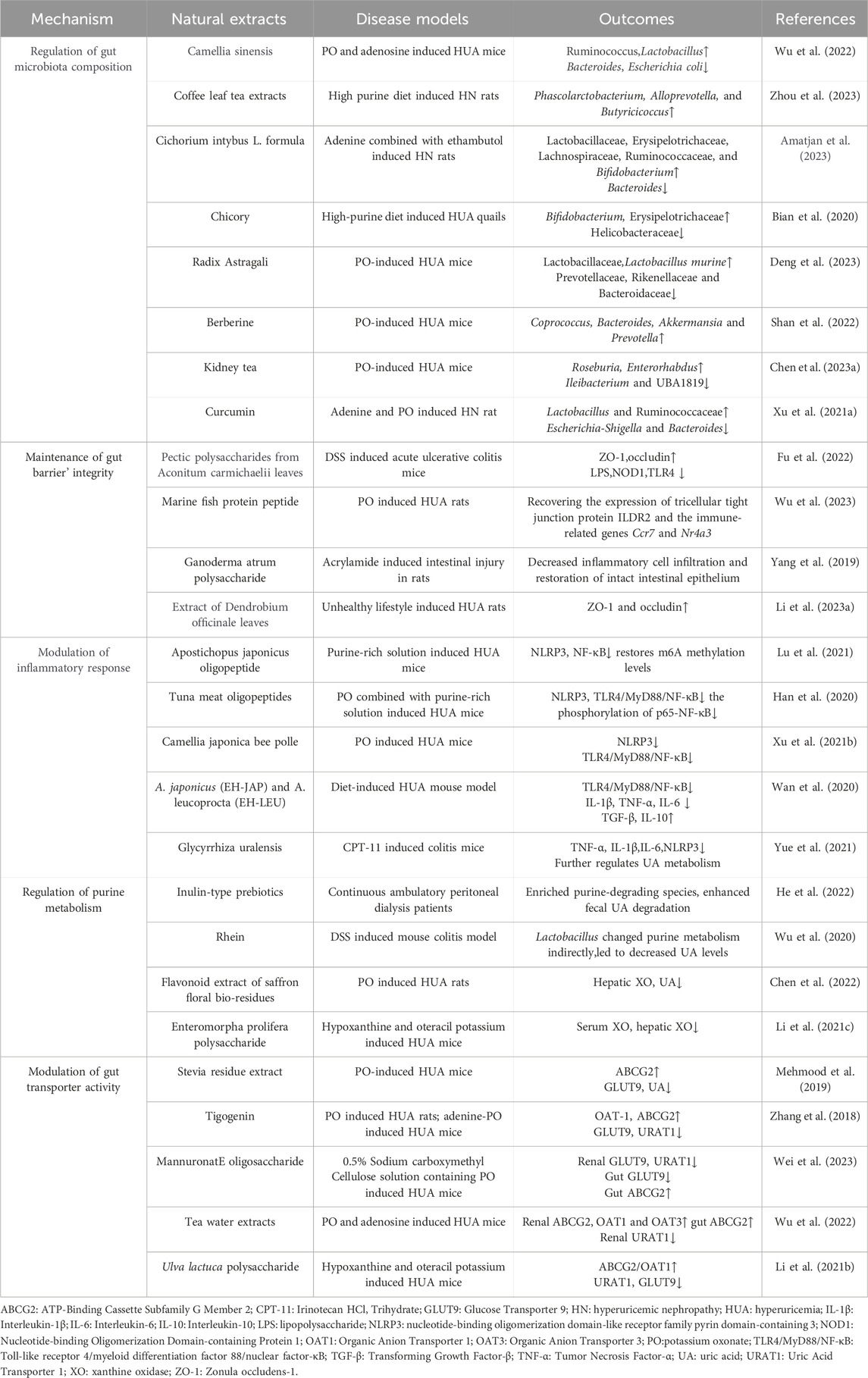
Table 1. Natural extracts target gut microbiome to regulate UA metabolism and antagonize associated disorders.
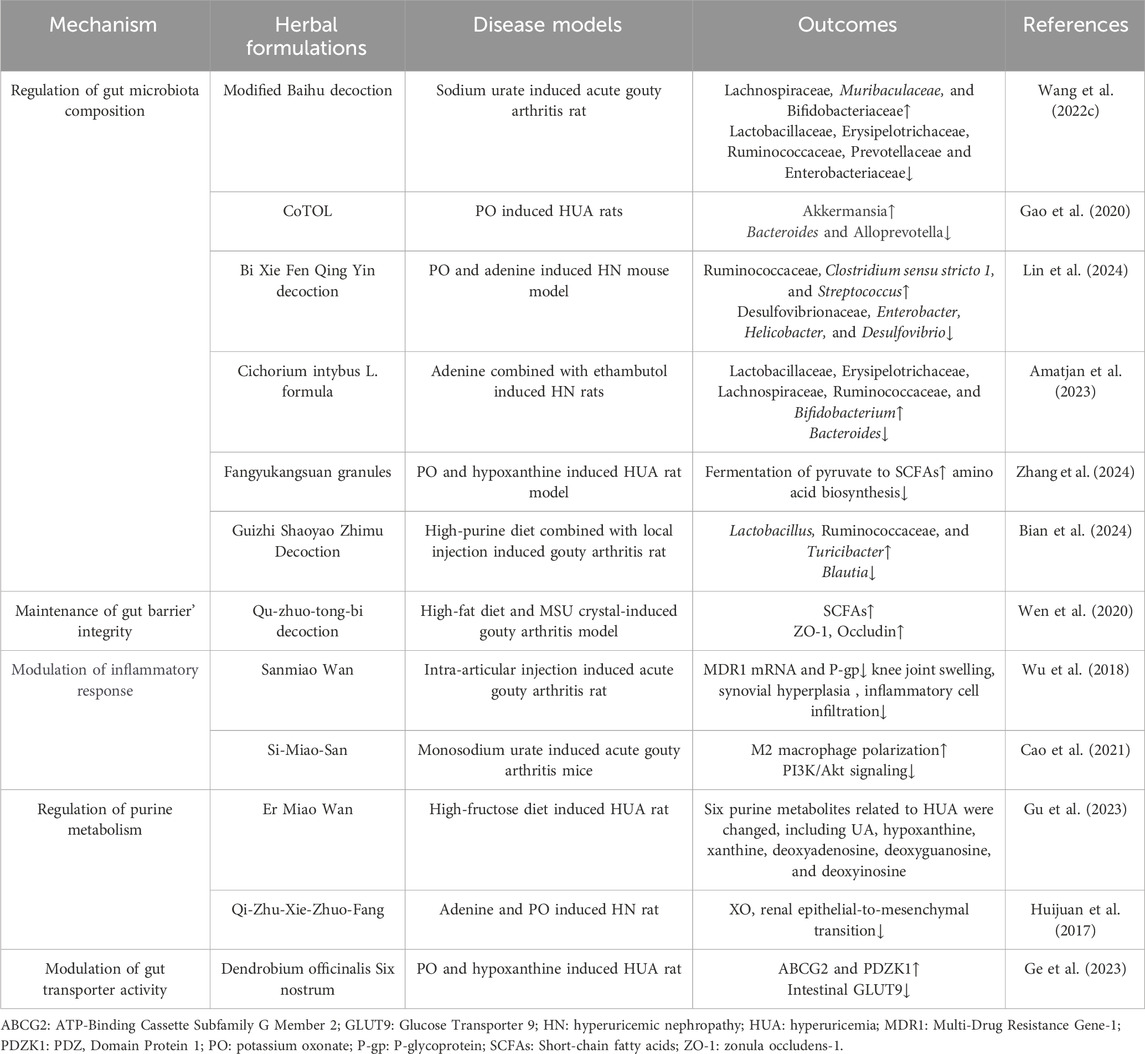
Table 2. Herbal formulations target gut microbiome to regulate UA metabolism and antagonize associated disorders.
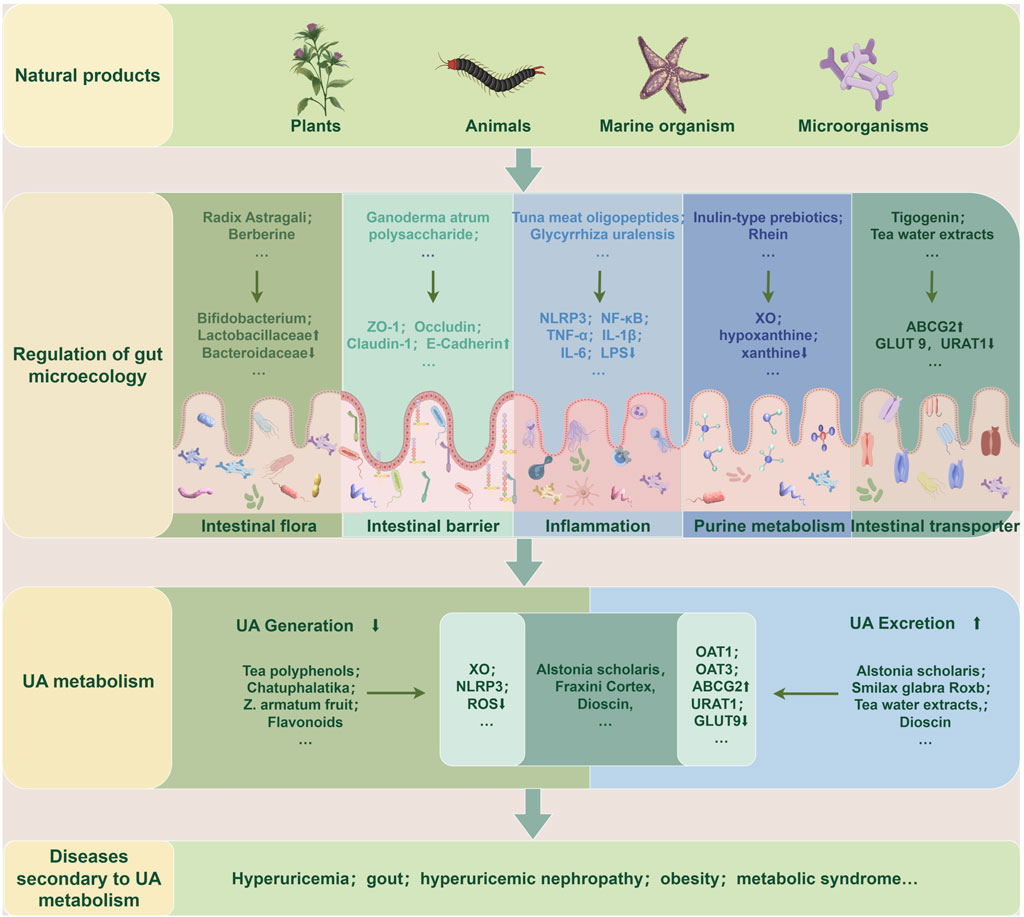
Figure 3. Natural products target gut microecology to regulate UA metabolism and secondary diseases. Extracts or preparations from natural products such as plants, animals, marine organisms, and microorganisms can repair gut microecological imbalance, resulting in reduced UA production or increased excretion, thereby improving UA metabolism and related secondary diseases. The specific ways are to restore the gut flora structure, repair gut barrier function, reduce the inflammatory state, and regulate purine metabolism and transporter activity. ABCG2: ATP-Binding Cassette Subfamily G Member 2; GLUT9: Glucose Transporter 9; IL-1β: Interleukin-1β; IL-6: Interleukin-6; LPS: Lipopolysaccharide; NF-κB: nuclear factor-κB; NLRP3: nucleotide-binding oligomerization domain-like receptor family pyrin domain-containing 3; OAT1: Organic Anion Transporter 1; OAT3: Organic Anion Transporter 3; ROS: Reactive Oxygen Species; TNF-α: Tumor Necrosis Factor-α; UA: uric acid; URAT1: Uric Acid Transporter 1; XO: xanthine oxidase; ZO-1: zonula occludens-1.
6.1 Regulation of gut microbiota composition
The intricate equilibrium of gut microbiota composition is strongly intertwined with UA metabolism and several pathophysiological conditions. Natural products showed a promising role in enhancing UA metabolism and alleviating associated secondary disorders through regulating gut microbiota composition, typically exerting an augmentation of beneficial bacteria and a diminution of harmful bacteria (Chen et al., 2023b). Generally, to resist detrimental Bacteroidaceae, bacterial genera such as Bifidobacterium, Lactobacillaceae, and Lachnospiraceae, which represent the beneficial bacterial spectrum, usually grow superiorly and can be strengthened by natural products (Amatjan et al., 2023). For instance, studies have documented that rhein can upregulate Lactobacillus, thereby enhancing purine metabolism and mitigating secondary manifestations of HUA (Wu et al., 2020; Cao et al., 2023). Moreover, supplementation with Radix Astragali and berberine in HUA model mice could reduce the abundance of Prevotellaceae, Rikenellaceae, and Bacteroidaceae, while augmenting Lactobacillaceae, Coprococcus, Bacteroides, Akkermansia, and Prevotella, which concurrently assist of regulating XO’ activation and renal function, and safeguarding mice against HUA induced damage (Shan et al., 2022; Deng et al., 2023). In a clinical trial, patients with renal failure were administered inulin-type prebiotics, and results showed that it could elevate the ratio of Firmicutes to Bacteroidetes, with the majority of bacteria enriched in their fecal matter correlated with UA degradation (He et al., 2022). Numerous studies have consistently demonstrated that an augmentation in SCFAs production is frequently associated with increased abundance of beneficial bacteria involved in UA metabolism, confirming the beneficial effect of SCFAs on UA metabolism (Ni et al., 2021). It has reported that both coffee leaf tea (Zhou et al., 2023) and chlorogenic acid (Zhou et al., 2021) exhibited therapeutic effects on hyperuricemic nephropathy (HN) by improving the abundance of SCFAs-produced bacteria, indicating that SCFAs-produced gut bacteria may serve as pivotal targets of natural products to ameliorate diseases associated with dysmetabolism of UA. In conclusion, numerous evidences suggest that the optimization of gut microbiota composition serves as a pivotal step in the regulation of UA metabolic imbalance by natural products.
6.2 Maintenance of gut barrier’ integrity
The physiological gut barrier is comprised of physical structure, microflora, and immunological microenvironment, which regulates various physiological activities, including UA metabolism. The physical barrier consists of an epithelial layer rich in tight junction proteins and a mucus layer composed of abundant mucins, and the immunological microenvironment is constituted by immunological tissues and cells (Sylvestre et al., 2023). Recent studies manifested that high levels of UA could impair the gut’s physical barrier and microenvironment (Lv et al., 2020). Interestingly, a report illustrated that natural products could affect UA metabolism by restoring these gut barriers. Administration of the extract of Dendrobium officinale leaves and herbal formulation Qu-zhuo-tong-bi decoction can repair the intestinal barrier damage caused by HUA, manifested by upregulation of ZO-1 and occludin expression, thereby enhances gut mucosal thickness and facilitates the normal excretion of UA (Wen et al., 2020; Li L. Z. et al., 2023). Upon disrupting the gut barrier, inflammatory mediators can readily disseminate into the systemic circulation and exacerbate simultaneous symptoms induced by HUA. Natural extract tuna meat oligopeptide was considered as a potential inhibitor of the TLR4/MyD88/NF-κB signaling, and a study testified that it could suppress TLR4 signaling cascade and NLRP3 inflammasome subsequently, thereby orchestrating the restoration of the gut barrier integrity (Han et al., 2020). Regarding immunological microenvironment coordinates gut immune barrier repairment, a report has manifested that the extract of Aconitum carmichaelii root not only modulated UA metabolsim but also regulated immune-associated siganling proteins, including TLR4 and NOD1, consequently promoting the transcription of tight junction protein in colitis model mice (Fu et al., 2022). Furthermore, a profound interplay between gut microbiota and gut barrier function was exsisted, research elucidated that augmention of SCFAs-generating microflora strains could significantly fortifies gut barrier integrity, and that could be amplified by curcumin supplementation in HN rats (Xu X. et al., 2021). In summary, numerous natural products exhibit potent reparative effects on the intestinal barrier, thereby facilitating the recovery from HUA.
6.3 Modulation of inflammatory response
The inflammatory status of the gut interacts with inflammation related to UA metabolism, while natural products with anti-inflammatory properties can suppress inflammatory signal pathways involved in UA metabolism, potentially reducing the risk of HUA and related diseases (El-Tantawy, 2021). Crucial mediators that regulate the intricate interaction between inflammation and UA metabolism are the NLRP3 inflammasome and the NF-κB signaling molecules. A study has shown that A. japonicus oligopeptide significantly inhibited the NF-κB signaling pathway and NLRP3 inflammatory vesicle, thereby alleviating HUA-induced inflammation in HUA model mice (Lee et al., 2022). Moreover, recent studies have elucidated that Tuna meat oligopeptides (TMOP) (Han et al., 2020), Camellia japonica bee pollen (Xu Y. et al., 2021), A. japonicus (EH-JAP) and Apostichopus leucoprocta (EH-LEU) (Wan et al., 2020) commonly exhibited inhibitory effects on the TLR4/MyD88/NF-κB signaling pathway. Individually, TMOP could modulate the UA metabolic pathway by inhibiting the phosphorylation of p65-NF-κB also. Meanwhile, C. japonica bee pollen could regulate specific gut transport proteins and gut microflora composition. Besides, both EH-JAP and EH-LEU could reduce the abundance of pathogens in the gut tract while exhibiting antagonistic effects on HUA and its induced renal inflammation. Gut inflammation intricately intertwined with various factors underpinning the UA metabolism process. IL-1β and IL-18 are the most pivotal cytokines among these inflammatory molecules, contributing to several pathological processes (Keenan, 2020). UA was considered as a crucial exogenous ligand for the NLRP3 inflammasome, and it reasonably speculated that HUA intensified the inflammatory response processes (Yue et al., 2021). An observation verified that IL-1β and IL-6 were evildoers of initiating systemic inflammation, while chlorogenic acid inhibited their detrimental action via NLRP3 signaling in HUA model mice (Zhou et al., 2021). In summary, natural products emerge as promising candidates for preventing gut tract inflammation and mitigating disorders associated with UA dysmetabolism.
6.4 Regulation of purine metabolism
Purine metabolism comprises de novo synthesis, nucleotide degradation, and salvage processes, requiring a series of synthetic and catabolic enzymes such as phosphoribosyltransferase, xanthine dehydrogenase, XO, and uricase. UA is the end product of human purine metabolism and is generated directly from the purine metabolite xanthine (Furuhashi, 2020). XO is the crucial rate-limiting enzyme involved in this metabolic pathway, which is generated from the liver and plays a pivotal role in orchestrating purine metabolism and concerting gut microenvironment (Bortolotti et al., 2021). Recent studies indicated that natural products could target XO as inhibitors and effectively reduce UA production, providing a potential therapeutic strategy for HUA (Sun et al., 2024). In addition, other scholars have revealed an intricate interaction between gut flora and XO activation, which could be modulated sophisticatedly by several inherent factors in the gut microecology system (Liu et al., 2022; Shan et al., 2022). Perturbation of gut flora profoundly affects purine metabolism, particularly the presence of Lactobacillus, which is closely correlated with the purine catabolic process. These above findings were testified by the flavonoid extract derived from saffron floral bio-residues, which antagonizes HUA by modulating both XO activity and gut flora composition (Chen et al., 2022). Total flavonoids isolated from Glycyrrhiza uralensis and rhein ameliorated the disorder of purine metabolism and reduced UA levels in the feces with dysbacteriosis in colitis mice (Wu et al., 2020; Yue et al., 2021). This definite efficacy of natural compounds to improve purine metabolism. Furthermore, HUA-triggered renal epithelial-mesenchymal transition is linked to altered XO activity, and Qi-Zhu-Xie-Zhuo decoction has the potential to reverse this process (Huijuan et al., 2017). Moreover, the classic herbal prescription Ermiao Wan is usually applied for the therapeutic management of HUA. A report indicate that this formula enhances purine degradation, modulates key metabolites like hypoxanthine, xanthine, and UA, thereby combating HUA (Gu et al., 2023). The above studies indicated that natural products could effectively ameliorate purine metabolism in coordination with microbiota to reduce UA production, which provides a potential therapeutic strategy for HUA-induced disorders.
6.5 Modulation of gut transporter activity
In recent years, studies have illustrated several transporters located in the gastrointestinal tract epithelium that have significantly contributed to modulating UA metabolism through the reabsorption or excretion of UA in the gut microenvironment. For instance, GLUT9 primarily exhibits the regulation of UA reabsorption into enterocytes, while ABCG2 is responsible for the excretion of UA from enterocytes into the gut lumen. Interestingly, URAT1 exerts both reabsorption and excretion of UA, corresponding to environmental changes in gut microecology (Dalbeth et al., 2021). Notably, recent findings have indicated that natural products may modulate the activity of UA transporters in the gut tract, thus influencing UA excretion and preventing its accumulation (Zhang M. Q. et al., 2023). Supplementation with the extracts from Stevia in HUA model mice could change UA levels by suppressing GLUT9 and enhancing ABCG2 expression, resulting in reduced UA levels in the gut tract and serum, and mitigated HUA-induced damage (Mehmood et al., 2019). Coffee-leaf tea has also be testified as an effective intervention to lower serum UA levels, prevent HUA and kidney damage, through modulating the activities of GLUT9, OAT3, and ABCG2 in the HN model (Zhou et al., 2023). In addition, an in vitro study also showed that Dioscin exerted a dual modulatory effect by inhibiting URAT1 expression level while stimulating the activity of ABCG2 to transport UA concurrently (Zhang et al., 2018). Furthermore, utilizing network pharmacology and molecular docking methods revealed that the natural product isobavachin facilitated the reduction of UA by activating ABCG2-mediated bile acid secretion (Luo et al., 2023). These findings provide novel insights into how natural products regulate UA transporters and their intricate interaction to modulate UA metabolism.
7 Conclusion and perspective
Despite the remarkable emergence of natural products as a vital resource for drug development in recent decades, the utilization of natural products in clinical intervention for diseases still needs improvement in quantity and diversity. As the third stage in ingesting food or drugs, the gut tract holds a pivotal role in maintaining fluid homeostasis, metabolizing nutrients, and eliminating waste products. And the stability of the gut microecology is imperative for the efficient absorption and utilization of dietary and medicinal compounds (Lu et al., 2020). UA is closely related to diet and subsequent changes in gut microecology, and is directly or indirectly involved in a variety of pathological injuries (Zeng et al., 2019). With the rapid progression of multi-omics technology fosters the establishment of virtual and physical screening databases grounded in natural products (Wilson et al., 2020), the elucidation of gut physiological and pathological microecological environments (Shalon et al., 2023), and the refinement of mathematical model predictions based on gut microecology’s temporal and spatial dynamics (Geng et al., 2021), these advancements have bolstered confidence in targeting gut microecology to modulate UA metabolism. However, constructing a sophisticated natural product screening platform aimed at gut microecology for medicinal advancements to intervene in UA metabolism poses a feasible yet formidable challenge.
Current investigations into natural products confront several significant hurdles. Natural products constitute a mass of compounds derived from plants, animals, microorganisms, and other sources. Screening for potential natural candidates targeting gut microecology can be arduous, as current methodologies often hinge solely on the product’s active component, thereby restricting its broad development and utilization. Additionally, acquiring a comprehensive understanding of the intricate modes of action and diverse targets of natural products, along with their variable curative potentials across disease types, poses a considerable challenge. Therefore, we espouse a balanced approach that incorporates the pursuit of novel natural products with exploring established natural compounds and their underlying mechanisms. This strategy will facilitate the establishment of a comprehensive network encompassing natural products, individual extracts, complex preparations, and disease mechanisms, along with a comprehensive database serving as a repository of fundamental data to cater to the diverse needs of researchers. In addition, aligned with the complexities inherent in natural product discovery, the precise modulation of gut microecology also encounters significant challenges. Gut microecology represents a dynamic and intricately balanced system characterized by the diverse composition of gut microbiota, where even strains within the same genus perform distinct roles. The precise elucidation of the mechanisms of gut microbiota and the factors that shape gut microecology concurrently poses a formidable challenge, given the intricate interplay of numerous regulatory mechanisms influenced by environmental factors, dietary patterns, multi-systemic diseases, and various pathological aspects. In recognition of this complexity, we advocate for the extensive utilization of multi-omics technology to elucidate the diverse functionalities of the same microbiota in various disease models and the distinct functionalities of different microbiota within a single disease model. Furthermore, we support a comprehensive examination of the spectrum of gut microecological alterations across different pathological stages of a given disease, encompassing alterations in microbiota, gut barriers, inflammatory states, and other contributing factors.
Furthermore, the preponderance of research about UA metabolism primarily focuses on its synthesis and elimination, specifically the activity of XO and the expression of UA transporters. Nonetheless, in the absence of clinical manifestations, patients often ignore HUA. As a result, to augment our comprehension of the dynamic role of UA in pathological processes, it is crucial to conduct research that concurrently examines UA as both a consequence of lesions and an etiological factor.
In conclusion, Delving into potential targets for modulating UA metabolism within the intricate gut microecology utilizing natural products could offer valuable insights into natural product-based drug development, intricate equilibrium maintenance of gut microbiota, and the intricate regulatory mechanisms underlying UA metabolism. Our comprehensive review demonstrates that extracts of natural products and herbal compounds not only have the potential to regulate gut microecology and UA metabolism but can also indirectly improve UA metabolism by modulating the gut microecology. Specifically, they can regulate gut microbiota composition, restore intestinal barrier integrity, alleviate inflammatory responses, modulate purine metabolism, and influence intestinal transporter function, thereby alleviating HUA and related complications. Our review provides conceptual frameworks and a foundation for more comprehensive and precise investigations into natural product-oriented gut microecology interventions in UA metabolism and associated disorders.
Author contributions
HW: Conceptualization, Data curation, Investigation, Writing–original draft, Writing–review and editing. YZ: Conceptualization, Data curation, Writing–original draft, Writing–review and editing. MY: Conceptualization, Writing–original draft, Writing–review and editing. Lu Wang: Conceptualization, Writing–review and editing. YX: Conceptualization, Writing–review and editing. SY: Conceptualization, Writing–review and editing. NM: Data curation, Funding acquisition, Writing–review and editing. JF: Data curation, Funding acquisition, Project administration, Writing–review and editing. SR: Data curation, Funding acquisition, Project administration, Writing–review and editing.
Funding
The author(s) declare that financial support was received for the research, authorship, and/or publication of this article. This work is supported by the Natural Science Foundation of Sichuan Province (2024NSFSC0687), the General Project of Sichuan Provincial Administration of Traditional Chinese Medicine (2023MS203); the Key Project of Chengdu Medical College (CYZZD22-01); the High-level Talent Project of Chengdu Medical College (CYFY-GQ38); Key Special Project of the First Affiliated Hospital of Chengdu Medical College (CYFY2021ZD01); Young Talent’ Program of Chengdu Medical College (2024qnGzn02); and Project of Sichuan Provincial Science and Technology Department (3001030201).
Acknowledgments
The authors are profoundly grateful to the “Figdraw” platform for providing drawing support.
Conflict of interest
The authors declare that the research was conducted in the absence of any commercial or financial relationships that could be construed as a potential conflict of interest.
Publisher’s note
All claims expressed in this article are solely those of the authors and do not necessarily represent those of their affiliated organizations, or those of the publisher, the editors and the reviewers. Any product that may be evaluated in this article, or claim that may be made by its manufacturer, is not guaranteed or endorsed by the publisher.
References
Alvarez-Lario, B., and Macarron-Vicente, J. (2011). Is there anything good in uric acid? QJM 104 (12), 1015–1024. doi:10.1093/qjmed/hcr159
Amatjan, M., Li, N., He, P., Zhang, B., Mai, X., Jiang, Q., et al. (2023). A novel approach based on gut microbiota analysis and network pharmacology to explain the mechanisms of action of cichorium intybus L. Formula in the improvement of hyperuricemic nephropathy in rats. Drug Des. Devel Ther. 17, 107–128. doi:10.2147/dddt.S389811
Bian, M., Wang, J., Wang, Y., Nie, A., Zhu, C., Sun, Z., et al. (2020). Chicory ameliorates hyperuricemia via modulating gut microbiota and alleviating LPS/TLR4 axis in quail. Biomed. Pharmacother. 131, 110719. doi:10.1016/j.biopha.2020.110719
Bian, M., Zhu, C., Nie, A., and Zhou, Z. (2024). Guizhi Shaoyao Zhimu Decoction ameliorates gouty arthritis in rats via altering gut microbiota and improving metabolic profile. Phytomedicine 131, 155800. doi:10.1016/j.phymed.2024.155800
Bortolotti, M., Polito, L., Battelli, M. G., and Bolognesi, A. (2021). Xanthine oxidoreductase: one enzyme for multiple physiological tasks. Redox Biol. 41, 101882. doi:10.1016/j.redox.2021.101882
Cao, J., Wang, T., Liu, Y., Zhou, W., Hao, H., Liu, Q., et al. (2023). Lactobacillus fermentum F40-4 ameliorates hyperuricemia by modulating the gut microbiota and alleviating inflammation in mice. Food Funct. 14 (7), 3259–3268. doi:10.1039/d2fo03701g
Cao, L., Zhao, T., Xue, Y., Xue, L., Chen, Y., Quan, F., et al. (2021). The anti-inflammatory and uric acid lowering effects of Si-Miao-San on gout. Front. Immunol. 12, 777522. doi:10.3389/fimmu.2021.777522
Chen, N., Wang, R., Li, H., Wang, W., Wang, L., Yin, X., et al. (2022). Flavonoid extract of saffron by-product alleviates hyperuricemia via inhibiting xanthine oxidase and modulating gut microbiota. Phytother. Res. 36 (12), 4604–4619. doi:10.1002/ptr.7579
Chen, X., Zhao, Z., Luo, J., Wu, T., Shen, Y., Chang, S., et al. (2021). Novel natural scaffold as hURAT1 inhibitor identified by 3D-shape-based, docking-based virtual screening approach and biological evaluation. Bioorg Chem. 117, 105444. doi:10.1016/j.bioorg.2021.105444
Chen, Y., Pei, C., Chen, Y., Xiao, X., Zhang, X., Cai, K., et al. (2023a). Kidney tea ameliorates hyperuricemia in mice via altering gut microbiota and restoring metabolic profile. Chem. Biol. Interact. 376, 110449. doi:10.1016/j.cbi.2023.110449
Chen, Y., Yang, J., Rao, Q., Wang, C., Chen, X., Zhang, Y., et al. (2023b). Understanding hyperuricemia: pathogenesis, potential therapeutic role of bioactive peptides, and assessing bioactive peptide advantages and challenges. Foods 12 (24), 4465. doi:10.3390/foods12244465
Chiaro, T. R., Soto, R., Zac Stephens, W., Kubinak, J. L., Petersen, C., Gogokhia, L., et al. (2017). A member of the gut mycobiota modulates host purine metabolism exacerbating colitis in mice. Sci. Transl. Med. 9 (380), eaaf9044. doi:10.1126/scitranslmed.aaf9044
Cicero, A. F. G., Caliceti, C., Fogacci, F., Giovannini, M., Calabria, D., Colletti, A., et al. (2017). Effect of apple polyphenols on vascular oxidative stress and endothelium function: a translational study. Mol. Nutr. Food Res. 61 (11). doi:10.1002/mnfr.201700373
Dalbeth, N., Gosling, A. L., Gaffo, A., and Abhishek, A. (2021). Gout. Lancet 397 (10287), 1843–1855. doi:10.1016/s0140-6736(21)00569-9
Dang, K., Zhang, N., Gao, H., Wang, G., Liang, H., and Xue, M. (2023). Influence of intestinal microecology in the development of gout or hyperuricemia and the potential therapeutic targets. Int. J. Rheum. Dis. 26 (10), 1911–1922. doi:10.1111/1756-185x.14888
Deng, S., Cai, K., Pei, C., Zhang, X., Xiao, X., Chen, Y., et al. (2023). 16S rRNA and metagenomics combined with UPLC-Q/TOF-MS metabolomics analysis reveals the potential mechanism of radix Astragali against hyperuricemia in mice. Drug Des. Devel Ther. 17, 1371–1386. doi:10.2147/dddt.S407983
Dong, L., Zhang, S., Chen, L., Lu, J., Zhao, F., Long, T., et al. (2023). In vivo anti-hyperuricemia and anti-gouty arthritis effects of the ethanol extract from Amomumvillosum Lour. Biomed. Pharmacother. 161, 114532. doi:10.1016/j.biopha.2023.114532
El Ridi, R., and Tallima, H. (2017). Physiological functions and pathogenic potential of uric acid: a review. J. Adv. Res. 8 (5), 487–493. doi:10.1016/j.jare.2017.03.003
El-Tantawy, W. H. (2021). Natural products for the management of hyperuricaemia and gout: a review. Arch. Physiol. Biochem. 127 (1), 61–72. doi:10.1080/13813455.2019.1610779
Fan, S., Huang, Y., Lu, G., Sun, N., Wang, R., Lu, C., et al. (2022). Novel anti-hyperuricemic hexapeptides derived from Apostichopus japonicus hydrolysate and their modulation effects on the gut microbiota and host microRNA profile. Food Funct. 13 (7), 3865–3878. doi:10.1039/d1fo03981d
Fu, Y. P., Li, C. Y., Peng, X., Wangensteen, H., Inngjerdingen, K. T., and Zou, Y. F. (2022). Pectic polysaccharides from Aconitum carmichaelii leaves protects against DSS-induced ulcerative colitis in mice through modulations of metabolism and microbiota composition. Biomed. Pharmacother. 155, 113767. doi:10.1016/j.biopha.2022.113767
Furuhashi, M. (2020). New insights into purine metabolism in metabolic diseases: role of xanthine oxidoreductase activity. Am. J. Physiol. Endocrinol. Metab. 319 (5), E827-E834–e834. doi:10.1152/ajpendo.00378.2020
Gao, L. L., Ma, J. M., Fan, Y. N., Zhang, Y. N., Ge, R., Tao, X. J., et al. (2021). Lycium barbarum polysaccharide combined with aerobic exercise ameliorated nonalcoholic fatty liver disease through restoring gut microbiota, intestinal barrier and inhibiting hepatic inflammation. Int. J. Biol. Macromol. 183, 1379–1392. doi:10.1016/j.ijbiomac.2021.05.066
Gao, Y., Sun, J., Zhang, Y., Shao, T., Li, H., Wang, M., et al. (2020). Effect of a traditional Chinese medicine formula (CoTOL) on serum uric acid and intestinal flora in obese hyperuricemic mice inoculated with intestinal bacteria. Evid. Based Complement. Altern. Med. 2020, 8831937. doi:10.1155/2020/8831937
Ge, H., Jiang, Z., Li, B., Xu, P., Wu, H., He, X., et al. (2023). Dendrobium officinalis six nostrum promotes intestinal urate underexcretion via regulations of urate transporter proteins in hyperuricemic rats. Comb. Chem. High. Throughput Screen 26 (4), 848–861. doi:10.2174/1386207325666220830141531
Geng, J., Ji, B., Li, G., López-Isunza, F., and Nielsen, J. (2021). CODY enables quantitatively spatiotemporal predictions on in vivo gut microbial variability induced by diet intervention. Proc. Natl. Acad. Sci. U S A 118 (13), e2019336118. doi:10.1073/pnas.2019336118
Gong, H., Gao, H., Ren, Q., and He, J. (2022). The abundance of bifidobacterium in relation to visceral obesity and serum uric acid. Sci. Rep. 12 (1), 13073. doi:10.1038/s41598-022-17417-3
Gu, C., Hu, X., Shan, B., Wu, X., and Chen, J. (2023). Targeted and non-targeted metabolomics uncovering the effects of Er-Miao-Wan formula on rats with hyperuricemia. J. Pharm. Biomed. Anal. 226, 115246. doi:10.1016/j.jpba.2023.115246
Guo, B. J., Bian, Z. X., Qiu, H. C., Wang, Y. T., and Wang, Y. (2017). Biological and clinical implications of herbal medicine and natural products for the treatment of inflammatory bowel disease. Ann. N Y Acad. Sci. 1401 (1), 37–48. doi:10.1111/nyas.13414
Han, J., Wang, X., Tang, S., Lu, C., Wan, H., Zhou, J., et al. (2020). Protective effects of tuna meat oligopeptides (TMOP) supplementation on hyperuricemia and associated renal inflammation mediated by gut microbiota. Faseb J. 34 (4), 5061–5076. doi:10.1096/fj.201902597RR
He, S., Xiong, Q., Tian, C., Li, L., Zhao, J., Lin, X., et al. (2022). Inulin-type prebiotics reduce serum uric acid levels via gut microbiota modulation: a randomized, controlled crossover trial in peritoneal dialysis patients. Eur. J. Nutr. 61 (2), 665–677. doi:10.1007/s00394-021-02669-y
Huang, J., Lin, Z., Wang, Y., Ding, X., and Zhang, B. (2023). Wuling san based on network pharmacology and in vivo evidence against hyperuricemia via improving oxidative stress and inhibiting inflammation. Drug Des. Devel Ther. 17, 675–690. doi:10.2147/dddt.S398625
Huijuan, W., Xiaoxu, C., Rui, S., Xinghui, L., Beibei, T., and Jianchun, M. (2017). Qi-Zhu-Xie-Zhuo-Fang reduces serum uric acid levels and ameliorates renal fibrosis in hyperuricemic nephropathy rats. Biomed. Pharmacother. 91, 358–365. doi:10.1016/j.biopha.2017.04.031
Kasahara, K., Kerby, R. L., Zhang, Q., Pradhan, M., Mehrabian, M., Lusis, A. J., et al. (2023). Gut bacterial metabolism contributes to host global purine homeostasis. Cell Host Microbe 31 (6), 1038–1053.e10. doi:10.1016/j.chom.2023.05.011
Keenan, R. T. (2020). The biology of urate. Semin. Arthritis Rheum. 50 (3s), S2-S10–s10. doi:10.1016/j.semarthrit.2020.04.007
Kodithuwakku, N. D., Pan, M., Zhu, Y. L., Zhang, Y. Y., Feng, Y. D., Fang, W. R., et al. (2013). Anti-inflammatory and antinociceptive effects of Chinese medicine SQ gout capsules and its modulation of pro-inflammatory cytokines focusing on gout arthritis. J. Ethnopharmacol. 150 (3), 1071–1079. doi:10.1016/j.jep.2013.10.016
Koudoufio, M., Desjardins, Y., Feldman, F., Spahis, S., Delvin, E., and Levy, E. (2020). Insight into polyphenol and gut microbiota crosstalk: are their metabolites the key to understand protective effects against metabolic disorders? Antioxidants (Basel) 9 (10), 982. doi:10.3390/antiox9100982
Lee, J. H., Kim, H. S., Lee, J. H., Yang, G., and Kim, H. J. (2022). Natural products as a novel therapeutic strategy for NLRP3 inflammasome-mediated gout. Front. Pharmacol. 13, 861399. doi:10.3389/fphar.2022.861399
Lee, J. S., Wang, R. X., Alexeev, E. E., Lanis, J. M., Battista, K. D., Glover, L. E., et al. (2018). Hypoxanthine is a checkpoint stress metabolite in colonic epithelial energy modulation and barrier function. J. Biol. Chem. 293 (16), 6039–6051. doi:10.1074/jbc.RA117.000269
Li, H., Chen, X., Liu, J., Chen, M., Huang, M., Huang, G., et al. (2021a). Ethanol extract of Centella asiatica alleviated dextran sulfate sodium-induced colitis: restoration on mucosa barrier and gut microbiota homeostasis. J. Ethnopharmacol. 267, 113445. doi:10.1016/j.jep.2020.113445
Li, L. Z., Wang, X. M., Feng, X. J., Liu, K., Li, B., Zhu, L. J., et al. (2023a). Effects of a macroporous resin extract of Dendrobium officinale leaves in rats with hyperuricemia induced by anthropomorphic unhealthy lifestyle. Evid. Based Complement. Altern. Med. 2023, 9990843. doi:10.1155/2023/9990843
Li, M., Wu, X., Guo, Z., Gao, R., Ni, Z., Cui, H., et al. (2023b). Lactiplantibacillus plantarum enables blood urate control in mice through degradation of nucleosides in gastrointestinal tract. Microbiome 11 (1), 153. doi:10.1186/s40168-023-01605-y
Li, N., Zhao, C., Zhang, P., Wu, S., Dou, X., Xu, S., et al. (2024). The role of gut microbiota associated metabolites in digestive disorders. Eng. Regen. 5 (2), 228–246. doi:10.1016/j.engreg.2024.04.003
Li, X., Chen, Y., Gao, X., Wu, Y., El-Seedi, H. R., Cao, Y., et al. (2021b). Antihyperuricemic effect of green alga ulva lactuca ulvan through regulating urate transporters. J. Agric. Food Chem. 69 (38), 11225–11235. doi:10.1021/acs.jafc.1c03607
Li, X., Gao, X., Zhang, H., Liu, Y., Sarker, M. M. R., Wu, Y., et al. (2021c). The anti-hyperuricemic effects of green alga Enteromorpha prolifera polysaccharide via regulation of the uric acid transporters in vivo. Food Chem. Toxicol. 158, 112630. doi:10.1016/j.fct.2021.112630
Liang, M., Liu, J., Chen, W., He, Y., Kahaer, M., Li, R., et al. (2022). Diagnostic model for predicting hyperuricemia based on alterations of the gut microbiome in individuals with different serum uric acid levels. Front. Endocrinol. (Lausanne) 13, 925119. doi:10.3389/fendo.2022.925119
Lin, S., Wang, Z., Lam, K. L., Zeng, S., Tan, B. K., and Hu, J. (2019). Role of intestinal microecology in the regulation of energy metabolism by dietary polyphenols and their metabolites. Food Nutr. Res. 63. doi:10.29219/fnr.v63.1518
Lin, X., Zou, X., Hu, B., Sheng, D., Zhu, T., Yin, M., et al. (2024). Bi Xie Fen Qing Yin decoction alleviates potassium oxonate and adenine induced-hyperuricemic nephropathy in mice by modulating gut microbiota and intestinal metabolites. Biomed. Pharmacother. 170, 116022. doi:10.1016/j.biopha.2023.116022
Liu, D., Lin, L., Lin, Y., Zhong, Y., Zhang, S., Liu, W., et al. (2019). Zengye decoction induces alterations to metabolically active gut microbiota in aged constipated rats. Biomed. Pharmacother. 109, 1361–1371. doi:10.1016/j.biopha.2018.11.013
Liu, X., Ke, L., Lei, K., Yu, Q., Zhang, W., Li, C., et al. (2023). Antibiotic-induced gut microbiota dysbiosis has a functional impact on purine metabolism. BMC Microbiol. 23 (1), 187. doi:10.1186/s12866-023-02932-8
Liu, Z. Q., Sun, X., Liu, Z. B., Zhang, T., Zhang, L. L., and Wu, C. J. (2022). Phytochemicals in traditional Chinese medicine can treat gout by regulating intestinal flora through inactivating NLRP3 and inhibiting XOD activity. J. Pharm. Pharmacol. 74 (7), 919–929. doi:10.1093/jpp/rgac024
Lu, C., Tang, S., Han, J., Fan, S., Huang, Y., Zhang, Z., et al. (2021). Apostichopus japonicus oligopeptide induced heterogeneity in the gastrointestinal tract microbiota and alleviated hyperuricemia in a microbiota-dependent manner. Mol. Nutr. Food Res. 65 (14), e2100147. doi:10.1002/mnfr.202100147
Lu, D., Huang, Y., Kong, Y., Tao, T., and Zhu, X. (2020). Gut microecology: why our microbes could be key to our health. Biomed. Pharmacother. 131, 110784. doi:10.1016/j.biopha.2020.110784
Lu, M., Yin, J., Xu, T., Dai, X., Liu, T., Zhang, Y., et al. (2024). Fuling-Zexie formula attenuates hyperuricemia-induced nephropathy and inhibits JAK2/STAT3 signaling and NLRP3 inflammasome activation in mice. J. Ethnopharmacol. 319 (Pt 2), 117262. doi:10.1016/j.jep.2023.117262
Luo, J. J., Chen, X. H., Liang, P. Y., Zhao, Z., Wu, T., Li, Z. H., et al. (2023). Mechanism of anti-hyperuricemia of isobavachin based on network pharmacology and molecular docking. Comput. Biol. Med. 155, 106637. doi:10.1016/j.compbiomed.2023.106637
Lv, Q., Xu, D., Zhang, X., Yang, X., Zhao, P., Cui, X., et al. (2020). Association of hyperuricemia with immune disorders and intestinal barrier dysfunction. Front. Physiol. 11, 524236. doi:10.3389/fphys.2020.524236
Ma, P., Peng, Y., Zhao, L., Liu, F., and Li, X. (2021). Differential effect of polysaccharide and nonpolysaccharide components in Sijunzi decoction on spleen deficiency syndrome and their mechanisms. Phytomedicine 93, 153790. doi:10.1016/j.phymed.2021.153790
Malik, N., Dhiman, P., and Khatkar, A. (2019). In silico and 3D QSAR studies of natural based derivatives as xanthine oxidase inhibitors. Curr. Top. Med. Chem. 19 (2), 123–138. doi:10.2174/1568026619666190206122640
Mehmood, A., Iftikhar, A., and Chen, X. (2024). Food-derived bioactive peptides with anti-hyperuricemic activity: a comprehensive review. Food Chem. 451, 139444. doi:10.1016/j.foodchem.2024.139444
Mehmood, A., Zhao, L., Wang, C., Hossen, I., Raka, R. N., and Zhang, H. (2019). Stevia residue extract increases intestinal uric acid excretion via interactions with intestinal urate transporters in hyperuricemic mice. Food Funct. 10 (12), 7900–7912. doi:10.1039/c9fo02032b
Mendez-Salazar, E. O., Vazquez-Mellado, J., Casimiro-Soriguer, C. S., Dopazo, J., Cubuk, C., Zamudio-Cuevas, Y., et al. (2021). Taxonomic variations in the gut microbiome of gout patients with and without tophi might have a functional impact on urate metabolism. Mol. Med. 27 (1), 50. doi:10.1186/s10020-021-00311-5
Ni, C., Li, X., Wang, L., Li, X., Zhao, J., Zhang, H., et al. (2021). Lactic acid bacteria strains relieve hyperuricaemia by suppressing xanthine oxidase activity via a short-chain fatty acid-dependent mechanism. Food Funct. 12 (15), 7054–7067. doi:10.1039/d1fo00198a
Oteiza, P. I., Fraga, C. G., Mills, D. A., and Taft, D. H. (2018). Flavonoids and the gastrointestinal tract: local and systemic effects. Mol. Asp. Med. 61, 41–49. doi:10.1016/j.mam.2018.01.001
Pascuta, M. S., Varvara, R. A., Teleky, B. E., Szabo, K., Plamada, D., Nemeş, S. A., et al. (2022). Polysaccharide-based edible gels as functional ingredients: characterization, applicability, and human health benefits. Gels 8 (8), 524. doi:10.3390/gels8080524
Polewski, M. A., Esquivel-Alvarado, D., Wedde, N. S., Kruger, C. G., and Reed, J. D. (2020). Isolation and characterization of blueberry polyphenolic components and their effects on gut barrier dysfunction. J. Agric. Food Chem. 68 (10), 2940–2947. doi:10.1021/acs.jafc.9b01689
Rullo, R., Cerchia, C., Nasso, R., Romanelli, V., Vendittis, E., Masullo, M., et al. (2023). Novel reversible inhibitors of xanthine oxidase targeting the active site of the enzyme. Antioxidants (Basel) 12 (4), 825. doi:10.3390/antiox12040825
Selby-Pham, S. N. B., Howell, K. S., Dunshea, F. R., Ludbey, J., Lutz, A., and Bennett, L. (2018). Statistical modelling coupled with LC-MS analysis to predict human upper intestinal absorption of phytochemical mixtures. Food Chem. 245, 353–363. doi:10.1016/j.foodchem.2017.10.102
Shalon, D., Culver, R. N., Grembi, J. A., Folz, J., Treit, P. V., Shi, H., et al. (2023). Profiling the human intestinal environment under physiological conditions. Nature 617 (7961), 581–591. doi:10.1038/s41586-023-05989-7
Shan, B., Wu, M., Chen, T., Tang, W., Li, P., and Chen, J. (2022). Berberine attenuates hyperuricemia by regulating urate transporters and gut microbiota. Am. J. Chin. Med. 50 (8), 2199–2221. doi:10.1142/s0192415x22500951
Shen, Y.-H., Zhu, H., Zhou, L., Zheng, Y.-Q., Zhang, Z., Xie, Y., et al. (2023). In inflammatory bowel disease and extraintestinal manifestations: what role does microbiome play? Eng. Regen. 4 (4), 337–348. doi:10.1016/j.engreg.2023.04.005
So, A., and Thorens, B. (2010). Uric acid transport and disease. J. Clin. Invest 120 (6), 1791–1799. doi:10.1172/JCI42344
Sun, Z. G., Wu, K. X., Ullah, I., and Zhu, H. L. (2024). Recent advances in xanthine oxidase inhibitors. Mini Rev. Med. Chem. 24 (12), 1177–1186. doi:10.2174/1389557523666230913091558
Sylvestre, M., Di Carlo, S. E., and Peduto, L. (2023). Stromal regulation of the intestinal barrier. Mucosal Immunol. 16 (2), 221–231. doi:10.1016/j.mucimm.2023.01.006
van der Hee, B., and Wells, J. M. (2021). Microbial regulation of host physiology by short-chain fatty acids. Trends Microbiol. 29 (8), 700–712. doi:10.1016/j.tim.2021.02.001
Wada, A., Higashiyama, M., Kurihara, C., Ito, S., Tanemoto, R., Mizoguchi, A., et al. (2022). Protective effect of luminal uric acid against indomethacin-induced enteropathy: role of antioxidant effect and gut microbiota. Dig. Dis. Sci. 67 (1), 121–133. doi:10.1007/s10620-021-06848-z
Wan, H., Han, J., Tang, S., Bao, W., Lu, C., Zhou, J., et al. (2020). Comparisons of protective effects between two sea cucumber hydrolysates against diet induced hyperuricemia and renal inflammation in mice. Food Funct. 11 (1), 1074–1086. doi:10.1039/c9fo02425e
Wang, H., Chen, S. T., Ding, X. J., Kuai, L., Hua, L., Li, X., et al. (2024). Efficacy and safety of Huzhang Granule, a compound Chinese herbal medicine, for acute gouty arthritis: a double-blind, randomized controlled trial. J. Integr. Med. 22, 270–278. doi:10.1016/j.joim.2024.03.008
Wang, J., Chen, Y., Zhong, H., Chen, F., Regenstein, J., Hu, X., et al. (2022a). The gut microbiota as a target to control hyperuricemia pathogenesis: potential mechanisms and therapeutic strategies. Crit. Rev. Food Sci. Nutr. 62 (14), 3979–3989. doi:10.1080/10408398.2021.1874287
Wang, P., Zhang, X., Zheng, X., Gao, J., Shang, M., Xu, J., et al. (2022b). Folic acid protects against hyperuricemia in C57bl/6J mice via ameliorating gut-kidney Axis dysfunction. J. Agric. Food Chem. 70 (50), 15787–15803. doi:10.1021/acs.jafc.2c06297
Wang, R., Ma, C. H., Zhou, F., and Kong, L. D. (2016). Siwu decoction attenuates oxonate-induced hyperuricemia and kidney inflammation in mice. Chin. J. Nat. Med. 14 (7), 499–507. doi:10.1016/s1875-5364(16)30059-0
Wang, X., Long, H., Chen, M., Zhou, Z., Wu, Q., Xu, S., et al. (2022c). Modified Baihu decoction therapeutically remodels gut microbiota to inhibit acute gouty arthritis. Front. Physiol. 13, 1023453. doi:10.3389/fphys.2022.1023453
Wang, Y., Tao, H., Huang, H., Xiao, Y., Wu, X., Li, M., et al. (2021). The dietary supplement Rhodiola crenulata extract alleviates dextran sulfate sodium-induced colitis in mice through anti-inflammation, mediating gut barrier integrity and reshaping the gut microbiome. Food Funct. 12 (7), 3142–3158. doi:10.1039/d0fo03061a
Wang, Y., Xu, Y., Xu, X., Wang, H., Wang, D., Yan, W., et al. (2022d). Ginkgo biloba extract ameliorates atherosclerosis via rebalancing gut flora and microbial metabolism. Phytother. Res. 36 (6), 2463–2480. doi:10.1002/ptr.7439
Wang, Z., Li, Y., Liao, W., Huang, J., Liu, Y., Li, Z., et al. (2022e). Gut microbiota remodeling: a promising therapeutic strategy to confront hyperuricemia and gout. Front. Cell Infect. Microbiol. 12, 935723. doi:10.3389/fcimb.2022.935723
Wei, B., Ren, P., Yang, R., Gao, Y., Tang, Q., Xue, C., et al. (2023). Ameliorative effect of mannuronate oligosaccharides on hyperuricemic mice via promoting uric acid excretion and modulating gut microbiota. Nutrients 15 (2), 417. doi:10.3390/nu15020417
Wen, X., Lou, Y., Song, S., He, Z., Chen, J., Xie, Z., et al. (2020). Qu-Zhuo-Tong-Bi decoction alleviates gouty arthritis by regulating butyrate-producing bacteria in mice. Front. Pharmacol. 11, 610556. doi:10.3389/fphar.2020.610556
Wilson, B. A. P., Thornburg, C. C., Henrich, C. J., Grkovic, T., and O'Keefe, B. R. (2020). Creating and screening natural product libraries. Nat. Prod. Rep. 37 (7), 893–918. doi:10.1039/c9np00068b
Wu, C., Hu, Q., Peng, X., Luo, J., and Zhang, G. (2023). Marine fish protein peptide regulating potassium oxonate-induced intestinal dysfunction in hyperuricemia rats helps alleviate kidney inflammation. J. Agric. Food Chem. 71 (1), 320–330. doi:10.1021/acs.jafc.2c04017
Wu, D., Chen, R., Li, Q., Lai, X., Sun, L., Zhang, Z., et al. (2022). Tea (Camellia sinensis) ameliorates hyperuricemia via uric acid metabolic pathways and gut microbiota. Nutrients 14 (13), 2666. doi:10.3390/nu14132666
Wu, J., Li, J., Li, W., Sun, B., Xie, J., Cheng, W., et al. (2018). Achyranthis bidentatae radix enhanced articular distribution and anti-inflammatory effect of berberine in Sanmiao Wan using an acute gouty arthritis rat model. J. Ethnopharmacol. 221, 100–108. doi:10.1016/j.jep.2018.04.025
Wu, J., Wei, Z., Cheng, P., Qian, C., Xu, F., Yang, Y., et al. (2020). Rhein modulates host purine metabolism in intestine through gut microbiota and ameliorates experimental colitis. Theranostics 10 (23), 10665–10679. doi:10.7150/thno.43528
Xu, G., Wu, L., Yang, H., Liu, T., Tong, Y., Wan, J., et al. (2024). Eupatilin inhibits xanthine oxidase in vitro and attenuates hyperuricemia and renal injury in vivo. Food Chem. Toxicol. 183, 114307. doi:10.1016/j.fct.2023.114307
Xu, X., Wang, H., Guo, D., Man, X., Liu, J., Li, J., et al. (2021a). Curcumin modulates gut microbiota and improves renal function in rats with uric acid nephropathy. Ren. Fail 43 (1), 1063–1075. doi:10.1080/0886022x.2021.1944875
Xu, Y., Cao, X., Zhao, H., Yang, E., Wang, Y., Cheng, N., et al. (2021b). Impact of Camellia japonica bee pollen polyphenols on hyperuricemia and gut microbiota in potassium oxonate-induced mice. Nutrients 13 (8), 2665. doi:10.3390/nu13082665
Yanai, H., Adachi, H., Hakoshima, M., and Katsuyama, H. (2021). Molecular biological and clinical understanding of the pathophysiology and treatments of hyperuricemia and its association with metabolic syndrome, cardiovascular diseases and chronic kidney disease. Int. J. Mol. Sci. 22 (17), 9221. doi:10.3390/ijms22179221
Yang, B., Xin, M., Liang, S., Xu, X., Cai, T., Dong, L., et al. (2022a). New insight into the management of renal excretion and hyperuricemia: potential therapeutic strategies with natural bioactive compounds. Front. Pharmacol. 13, 1026246. doi:10.3389/fphar.2022.1026246
Yang, J., Feng, P., Ling, Z., Khan, A., Wang, X., Chen, Y., et al. (2023). Nickel exposure induces gut microbiome disorder and serum uric acid elevation. Environ. Pollut. 324, 121349. doi:10.1016/j.envpol.2023.121349
Yang, L., Wang, B., Ma, L., and Fu, P. (2022b). Traditional Chinese herbs and natural products in hyperuricemia-induced chronic kidney disease. Front. Pharmacol. 13, 971032. doi:10.3389/fphar.2022.971032
Yang, Y., Zhang, L., Jiang, G., Lei, A., Yu, Q., Xie, J., et al. (2019). Evaluation of the protective effects of Ganoderma atrum polysaccharide on acrylamide-induced injury in small intestine tissue of rats. Food Funct. 10 (9), 5863–5872. doi:10.1039/c9fo01452g
Ye, Y., Warusawitharana, H., Zhao, H., Liu, Z., Li, B., Wu, Y., et al. (2022). Tea polyphenols attenuates inflammation via reducing lipopolysaccharides level and inhibiting TLR4/NF-κB pathway in obese mice. Plant Foods Hum. Nutr. 77 (1), 105–111. doi:10.1007/s11130-021-00937-0
Yin, H., Liu, N., and Chen, J. (2022). The role of the intestine in the development of hyperuricemia. Front. Immunol. 13, 845684. doi:10.3389/fimmu.2022.845684
Yu, B., Wang, M., Teng, B., Veeraperumal, S., Cheung, P. C., Zhong, S., et al. (2023). Partially acid-hydrolyzed porphyran improved dextran sulfate sodium-induced acute colitis by modulation of gut microbiota and enhancing the mucosal barrier. J. Agric. Food Chem. 71 (19), 7299–7311. doi:10.1021/acs.jafc.2c08564
Yue, S. J., Qin, Y. F., Kang, A., Tao, H. J., Zhou, G. S., Chen, Y. Y., et al. (2021). Total flavonoids of Glycyrrhiza uralensis alleviates irinotecan-induced colitis via modification of gut microbiota and fecal metabolism. Front. Immunol. 12, 628358. doi:10.3389/fimmu.2021.628358
Zeng, Q., Li, D., He, Y., Li, Y., Yang, Z., Zhao, X., et al. (2019). Discrepant gut microbiota markers for the classification of obesity-related metabolic abnormalities. Sci. Rep. 9 (1), 13424. doi:10.1038/s41598-019-49462-w
Zhang, G., Zhu, M., Liao, Y., Gong, D., and Hu, X. (2022a). Action mechanisms of two key xanthine oxidase inhibitors in tea polyphenols and their combined effect with allopurinol. J. Sci. Food Agric. 102 (15), 7195–7208. doi:10.1002/jsfa.12085
Zhang, M. Q., Sun, K. X., Guo, X., Chen, Y. Y., Feng, C. Y., Chen, J. S., et al. (2023a). The antihyperuricemia activity of Astragali Radix through regulating the expression of uric acid transporters via PI3K/Akt signalling pathway. J. Ethnopharmacol. 317, 116770. doi:10.1016/j.jep.2023.116770
Zhang, Q. Z., Zhang, J. R., Li, X., Yin, J. L., Jin, L. M., Xun, Z. R., et al. (2024). Fangyukangsuan granules ameliorate hyperuricemia and modulate gut microbiota in rats. Front. Immunol. 15, 1362642. doi:10.3389/fimmu.2024.1362642
Zhang, S., Xu, Z., Cao, X., Xie, Y., Lin, L., Zhang, X., et al. (2020). Shenling Baizhu San improves functional dyspepsia in rats as revealed by (1)H-NMR based metabolomics. Anal. Methods 12 (18), 2363–2375. doi:10.1039/d0ay00580k
Zhang, X., Cui, J., Hou, J., and Wang, W. (2022b). Research progress of natural active substances with uric-acid-reducing activity. J. Agric. Food Chem. 70 (50), 15647–15664. doi:10.1021/acs.jafc.2c06554
Zhang, Y., Jin, L., Liu, J., Wang, W., Yu, H., Li, J., et al. (2018). Effect and mechanism of dioscin from Dioscorea spongiosa on uric acid excretion in animal model of hyperuricemia. J. Ethnopharmacol. 214, 29–36. doi:10.1016/j.jep.2017.12.004
Zhang, Y., Wang, S., Dai, X., Liu, T., Liu, Y., Shi, H., et al. (2023b). Simiao San alleviates hyperuricemia and kidney inflammation by inhibiting NLRP3 inflammasome and JAK2/STAT3 signaling in hyperuricemia mice. J. Ethnopharmacol. 312, 116530. doi:10.1016/j.jep.2023.116530
Zheng, S., Xue, T., Wang, B., Guo, H., and Liu, Q. (2022). Chinese medicine in the treatment of ulcerative colitis: the mechanisms of signaling pathway regulations. Am. J. Chin. Med. 50 (7), 1781–1798. doi:10.1142/s0192415x22500756
Zhou, X., Seto, S. W., Chang, D., Kiat, H., Razmovski-Naumovski, V., Chan, K., et al. (2016). Synergistic effects of Chinese herbal medicine: a comprehensive review of methodology and current research. Front. Pharmacol. 7, 201. doi:10.3389/fphar.2016.00201
Zhou, X., Zhang, B., Zhao, X., Lin, Y., Wang, J., Wang, X., et al. (2021). Chlorogenic acid supplementation ameliorates hyperuricemia, relieves renal inflammation, and modulates intestinal homeostasis. Food Funct. 12 (12), 5637–5649. doi:10.1039/d0fo03199b
Zhou, X., Zhang, B., Zhao, X., Zhang, P., Guo, J., Zhuang, Y., et al. (2023). Coffee leaf tea extracts improve hyperuricemia nephropathy and its associated negative effect in gut microbiota and amino acid metabolism in rats. J. Agric. Food Chem. 71, 17775–17787. doi:10.1021/acs.jafc.3c02797
Keywords: natural products, gut microecology, gut flora, gut barrier, uric acid, hyperuricemia
Citation: Wang H, Zheng Y, Yang M, Wang L, Xu Y, You S, Mao N, Fan J and Ren S (2024) Gut microecology: effective targets for natural products to modulate uric acid metabolism. Front. Pharmacol. 15:1446776. doi: 10.3389/fphar.2024.1446776
Received: 10 June 2024; Accepted: 19 August 2024;
Published: 28 August 2024.
Edited by:
Barbara De Filippis, University of Studies G d'Annunzio Chieti and Pescara, ItalyReviewed by:
Guoshun Shan, Liaoning University of Traditional Chinese Medicine, ChinaWenguo Cui, Shanghai Jiao Tong University, China
Copyright © 2024 Wang, Zheng, Yang, Wang, Xu, You, Mao, Fan and Ren. This is an open-access article distributed under the terms of the Creative Commons Attribution License (CC BY). The use, distribution or reproduction in other forums is permitted, provided the original author(s) and the copyright owner(s) are credited and that the original publication in this journal is cited, in accordance with accepted academic practice. No use, distribution or reproduction is permitted which does not comply with these terms.
*Correspondence: Junming Fan, anVubWluZ2ZhbkAxNjMuY29t; Sichong Ren, U2ljaG9uZ3JlbkAxNjMuY29t
†These authors have contributed equally to this work and share first authorship