- Department of Integrative Biology, School of Bio Sciences and Technology (SBST), Vellore Institute of Technology (VIT), Vellore, Tamil Nadu, India
Cancer remains a multifactorial disease with an increased mortality rate around the world for the past several decades. Despite advancements in treatment strategies, lower survival rates, drug-associated side effects, and drug resistance create a need for novel anticancer agents. Ample evidence shows that imbalances in the gut microbiota are associated with the formation of cancer and its progression. Altering the gut microbiota via probiotics and their metabolites has gained attention among the research community as an alternative therapy to treat cancer. Probiotics exhibit health benefits as well as modulate the immunological and cellular responses in the host. Apart from probiotics, their secreted products like bacteriocins, exopolysaccharides, short-chain fatty acids, conjugated linoleic acid, peptidoglycan, and other metabolites are found to possess anticancer activity. The beneficiary role of these postbiotic compounds is widely studied for characterizing their mechanism and mode of action that reduces cancer growth. The present review mainly focuses on the postbiotic components that are employed against cancer with their reported mechanism of action. It also describes recent research works carried out so far with specific strain and anticancer activity of derived compounds both in vitro and in vivo, validating that the probiotic approach would pave an alternative way to reduce the burden of cancer.
1 Introduction
Cancer remains one of the main causes of mortality and creates despair in the human community across the globe (Sung et al., 2021). Along with the growth of population and aging, cancer continues to exist as the leading determinant of mortality compared to heart diseases (Bray et al., 2018). Cancer denotes the uncontrolled growth of cells following the spread to distant organs by metastasis (Ghosh and George, 2023). It is mainly characterized by altered cell signaling and metabolism leading to countless proliferation (Upadhyay, 2020). International Classification of Diseases for Oncology has classified cancer into six main groups based on tissue types, namely, carcinoma, lymphoma, sarcoma, myeloma, leukemia, and mixed type. Even though there are more than 100 types of cancer, the most common cancer type includes breast cancer with an incidence rate of 11.7%, followed by lung cancer at 11.4%, colon cancer at 10%, prostate cancer at 10%, and finally the stomach cancer with 5.5% (Sathishkumar et al., 2022). Compared to other diseases, there are very few treatments for cancer including surgery, chemotherapy, immunotherapy, and radiation therapy (Debela et al., 2021). Despite advances in diagnostics and therapeutics, the number of cancer cases has been increasing in the past 2 decades (Falzone et al., 2018). Reduction in cancer mortality mainly relies on continuous progress in pharmacological fields and the introduction of effective drugs and therapies (Miller et al., 2016).
Human gut harbors trillions of microbes like bacteria, fungi, and yeast that execute favorable tasks to the host (Thursby and Juge, 2017). The microbiota favors the host through a wide range of functions like strengthening and shaping the intestinal epithelium, acting against harmful pathogens, regulating host immunity, and also a part in energy scavenging (Natividad and Verdu, 2013; Gensollen et al., 2016). Gut microbiota has been considered an important organ, due to its communicational axis with the rest of the host’s organs through humoral, endocrine, neural, and metabolic pathways (Ahlawat and Sharma, 2021). Gut microbiota depends on several factors like human lifestyle, age, environmental factors, and diet as it can modulate the microbiome (Afzaal et al., 2022). However, dysbiosis occurs when intestinal bacterial growth and related metabolism are disintegrated. Dysbiosis is the disproportion of the microbial composition that results in the alteration of bacterial metabolic activities in the human gut (DeGruttola et al., 2016). Dysbiosis can result in developing inflammation in the gastrointestinal tract (GIT), diarrhea, neurodegenerative disorders, and even cancer (Asseri et al., 2023). Numerous studies have concluded that dysbiotic microbiome and its derivatives are associated with the cause of inflammatory diseases like IBDs (inflammatory bowel diseases), CVDs (cardiovascular diseases), CKDs (chronic kidney diseases), NAFLD (non-alcoholic fatty liver diseases), and cancer (Afzaal et al., 2022). Therefore, the role of homeostatic gut microbiota and their metabolites play a significant role in human health which has directed researchers to investigate the connections of microbes in metabolism (Cardona and Roman, 2022). The prokaryotic members in a healthy gut are generally friendly and beneficial to health and are probiotics.
Probiotics are beneficial microorganisms that utilize dietary fibres, prebiotics, and secrete metabolites known as postbiotics (Kim S. et al., 2021. Generally, probiotics can be consumed by integrating them into foods like dairy products or non-dairy food forms as additional supplements (Latif et al., 2023). Fermented foods that are consumed comprise active microbes closely similar to the strains regarded as probiotics. These fermented foods enhance their nutritional value by converting substrates into bioactive metabolites (Marco et al., 2017). There are expanding shreds of evidence favoring the beneficial effects of probiotic consumption, including maintenance of gut health, improved immune response, and cancer prevention (Kechagia et al., 2013). Probiotics are widely known as a potential therapeutic agent against many diseases like necrotizing enterocolitis (NEC), acute infectious diarrhea, antibiotic-associated diarrhea (AAD), upper respiratory infections, irritable bowel syndrome (IBS), gastroenteritis, vaginal candidiasis, ulcerative colitis, traveler’s diarrhea, and various allergic diseases (Hawrelak, 2003; Wang et al., 2019; Kumar et al., 2024). Apart from probiotics, postbiotics have been reported to possess health benefits to hosts with several properties including infection control and prevention, induction to apoptosis, mitigation of inflammation, immunomodulation, and reinstating of eubiosis. Postbiotics refer to the bioactive molecules that are released from probiotics as a result of fermentation and cell lysis (Kim Y. J. et al., 2021). Recent research findings have validated the effect of postbiotic components against several life-threatening diseases and disorders.
Current management of cancer treatment involves standard drugs that not only act against cancer cells but also affect normal cells developing resistance towards them and likely related therapies remain under challenge (Raguz and Yagüe, 2008). Side effects are the common outcome of cancer treatment (chemo- and radiotherapy) which reduces the quality of patients’ lives and increases agonies. Probiotics and prebiotics-mediated therapeutics showed promising results in mitigating such unwanted side effects. A study analyzed 20 published clinical trials with probiotics where 17 trials experienced beneficial impact in reducing side effects and three did not show visible results (Rodriguez-Arrastia et al., 2021). Due to chemo- and radio-therapy, non-cancerous normal cells are also induced to malfunction physiologically which may lead to bleeding, anemia, loss of taste, nausea, diarrhea, inflamed mucus, dysbiosis, and many more discomforts (Akbarali et al., 2022). To overcome such situations, there is continuous research to use probiotics as an adjuvant if not directly for cancer therapy. Research outcome shows acceptable information in several clinical trials [Renzis et al., 2007,]. The use of probiotics in mitigating side-effects developed in patients due to cancer treatment is enlisted in Table 1, demonstrating the type of treatment offered to patients with different cancer types and the relative improvement of patient’s quality of life. On the other hand, there is a long list of probiotics that are mostly used as a dietary supplement to maintain good health from different conditions (gas, constipation, diarrhea, oral thrush, IBS, urinary tract infection, vaginal pH imbalance, etc.) though none of the listed drugs is FDA approved but approved by similar other organizations across countries, like World Organisation of Gastroenterology, Therapeutic Goods Administration (TGA), Ministry of Health Malaysia, and Chinese regulatory authority, the State Food and Drug Administration (SFDA) and commercially viable (Table 1) [https://www.drugs.com/drug-class/probiotics.html].
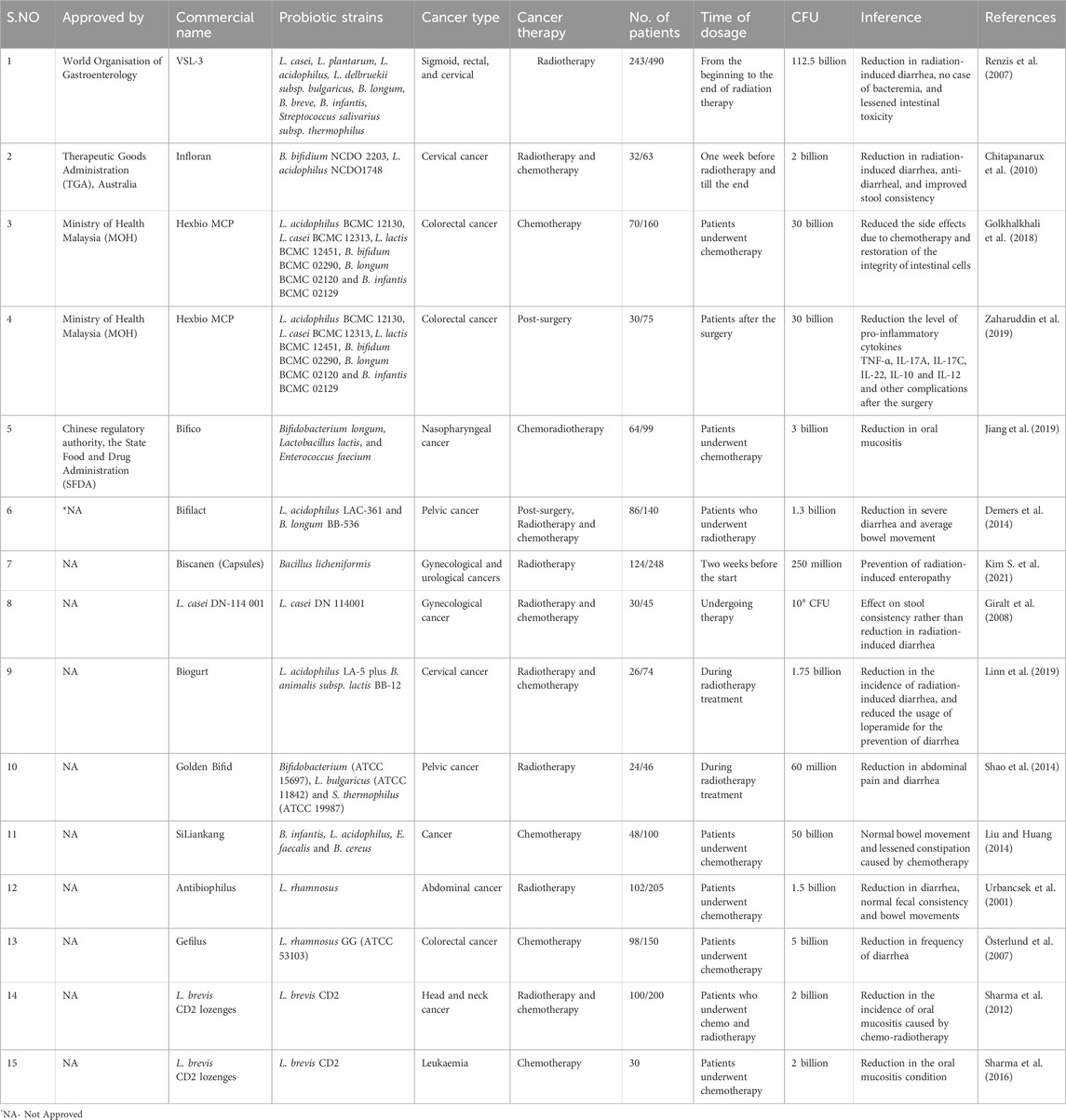
Table 1. List of approved and non-approved commercial probiotics employed as adjuvants to cancer therapies.
In the act of preventing and treating cancer, probiotics are employed due to their pivotal role in host interactions and conferring health benefits (Legesse Bedada et al., 2020). Since the last centennial, probiotics and their derived metabolites (components of postbiotics) have set up the cornerstone of research against all types of cancer (Nazir et al., 2018). Most reviews have mainly focused on the role of probiotics against colorectal cancer (CRC) however the current discussion reviews exclusively on postbiotics with a general view of probiotics, prebiotics, postbiotics, next-gen probiotics and their preventive roles on different cancers with plausible explanations of underlying mechanisms of action and future directions.
2 Probiotics, prebiotics, postbiotics, and synbiotics
According to the Food and Agriculture Organization of the United Nations (FAO) and the World Health Organization (WHO) in 2001, “Probiotics are live microorganisms which, when administered in adequate amounts, confer a health benefit on the host” (Hill et al., 2014; Indian Council of Medical Research Task ForceCo-ordinating Unit ICMRCo-ordinating Unit DBT, 2011). Bacterial strains in the genera of Lactobacillus, Lactococcus, Bacillus, Enterococcus, Pediococcus, Streptococcus, and Propionibacterium are considered to be potential probiotic microbes (Hamad et al., 2022). Among them, lactic acid-producing bacteria [LAB] and Bifidobacteria have been explored for a wide range of applications (Figure 1) (Bron et al., 2011). Probiotics produce various products like antimicrobial substances, exopolysaccharides (EPS), short-chain fatty acids (SCFAs), conjugated linoleic acids (CLA), and other metabolites during metabolism, which are directly involved in the benefit of human health (Marco et al., 2017). Probiotics affect the immune responses that are intervened by various immune cells like B and T lymphocytes, dendritic cells, macrophages, and natural killer (NK) cells (Kerry et al., 2018). The innate immune system of the host has been studied against its link to probiotics and revealed that expression of cytokines presented by antigen-presenting cells, augmenting type 1 helper T cell response, and finally activation of natural killer cells (Ashraf and Shah, 2014). Additionally, these probiotic bacteria can have the ability to influence the nervous system by communicating via the gut-brain axis (Morkl et al., 2020). Probiotics are thus considered functional foods with scientific proofs which validate beneficial properties by producing bioactive metabolites for modulating gut microbiota, and immunomodulation (Lin, 2003). Apart from health benefits, the widespread usage of live probiotics is associated with some unwanted health effects among children and adults (Doron and Snydman, 2015). Another concern about using live probiotics is that they might get transported into blood vessels and neighbouring tissues resulting in bacteremia in immunocompromised individuals (Kataria et al., 2009). Similarly, other issues with live probiotics may include the transfer of antibiotic-resistant genes in the human gastrointestinal tract (GIT) (Mater et al., 2008). However, the health-beneficial realms of probiotics and their derivatives are so big and effective, that these demerits are negligible.
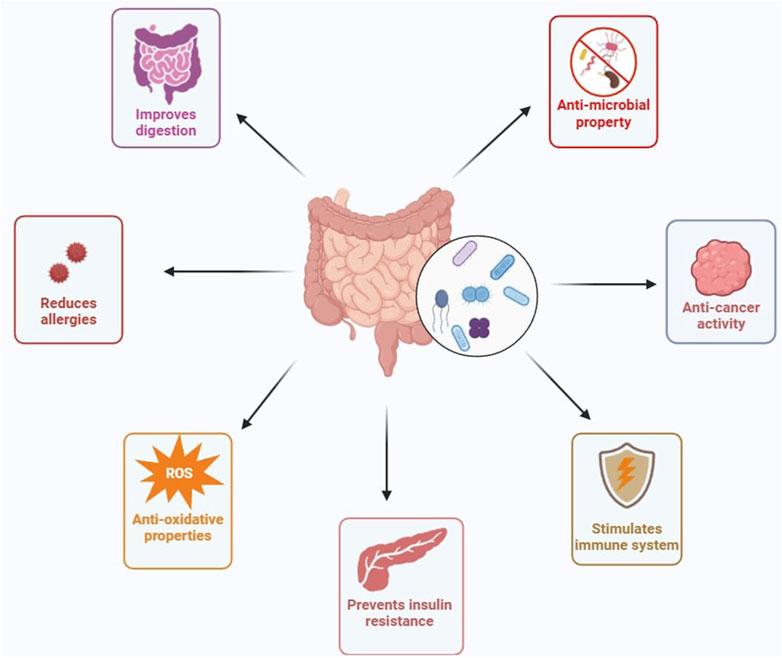
Figure 1. Various effects of probiotics on host’s health. (Figures were generated using BioRender.com)
For the past 2 decades, postbiotics have acquired more attention from researchers to explore their potential applications in medicine (Ali et al., 2023). Postbiotics are defined as the preparation of inanimate probiotics (para probiotics, ghost probiotics), their fermented metabolites, and structural components that confer health benefits on the host (Salminen et al., 2021). The International Scientific Association of Probiotics and Prebiotics (ISAPP) defined postbiotics are “preparations of inanimate microorganisms and/or their components that confer health benefit on the host.” Postbiotic preparations contain probiotic-derived components such as cell lysates, metabolites, peptides, enzymes, vitamins, proteins, exopolysaccharides, and extracellular vesicles (Deshpande et al., 2018). Studies on health beneficial properties of postbiotics reveal that postbiotics possess characteristics and are more advantageous than live probiotics. Probiotics need support for assured shelf life while postbiotics need not. However, probiotics can colonize, and antagonize pathogens by interacting with the host system. On the other hand, postbiotics can pass through the mucous layer quickly, with no risk of infection in immunocompromised individuals, no possibility of antibiotic resistance gene transfer, and are convenient to standardize transport and storage (De Marco et al., 2018). Postbiotics therefore own better choices of their applications in developing several functional foods than probiotics (Vinderola et al., 2022).
On the other hand, prebiotics is another term used for food ingredients like non-digestible, resistant starch, and fibers in human GIT and are good for the growth of gut microbiota. Prebiotics are defined as a “selectively fermented ingredient that results in changes in the composition and activity of gastrointestinal microbiota, conferring benefits upon host health” (Gibson et al., 2017). These ingredients are not digested by humans but become the source of energy harvest, growth, and metabolite production by gut microbiota. Thus, it can modify the gut microbiome and influence the host’s health condition (Rastall and Gibson, 2015). Most of the prebiotics belong to the class of carbohydrates that are present naturally in the human diet (Slavin, 2013). Common prebiotics include oligofructose, inulin, fructo-oligosaccharides (FOS), galactose-oligosaccharides (GOS), and xylose-oligosaccharides (XOS) (Hutkins et al., 2016). These are obtained from natural resources like fruits, vegetables, and grains that are commonly used in day-to-day life. Prebiotics have been reported to reduce the prevalence of diarrhea, irritable bowel syndrome, and even colon cancer (Peña, 2007). Despite this, prebiotics were identified to enhance the bioavailability and uptake of nutrition, and suppression of risk factors of cardiovascular diseases (Pokusaeva et al., 2011). These components are stable, temperature resistant, and can thrive in stomach acids, but may lead to gastrointestinal discomfort (Marteau and Seksik, 2004). Considering differences among probiotics, prebiotics, and postbiotics, all three have functional relationships for the promotion of health benefits of the host (Ji et al., 2023).
Synbiotics refers to the complex mixture of both prebiotics and probiotics formulation to improve human health (Markowiak and Śliżewska, 2017). According to ISAPP, synbiotics are of two types: complementary and synergistic. Synergistic synbiotics consist of a substrate that is specifically utilized by a co-administered microbe, whereas in complementary synbiotics both probiotics as well as prebiotics together confer health benefits independently (Swanson et al., 2020). Several studies have reported that synbiotics stimulate health and nutrition in the host (Yadav et al., 2022). Synbiotics were found to reduce the risk of CVDs and insulin resistance in aged individuals (Cicero et al., 2021).
3 Postbiotics-an endowing anticancer agent
The concept of postbiotics mounted during this decade however terms like postbiotics, paraprobiotics, and fermented infant formulas (FIFs) came into existence in 1986 with increasing growth of research and development, as reviewed elsewhere (Wegh et al., 2019). The postbiotic is a pool of functional components that include cell-free supernatants (CFS), short-chain fatty acids (SCFA), peptides, bacteriocins, exopolysaccharides (EPS), biosurfactants, conjugated linoleic acid (CLA) and peptidoglycans (PG) (Figure 2).
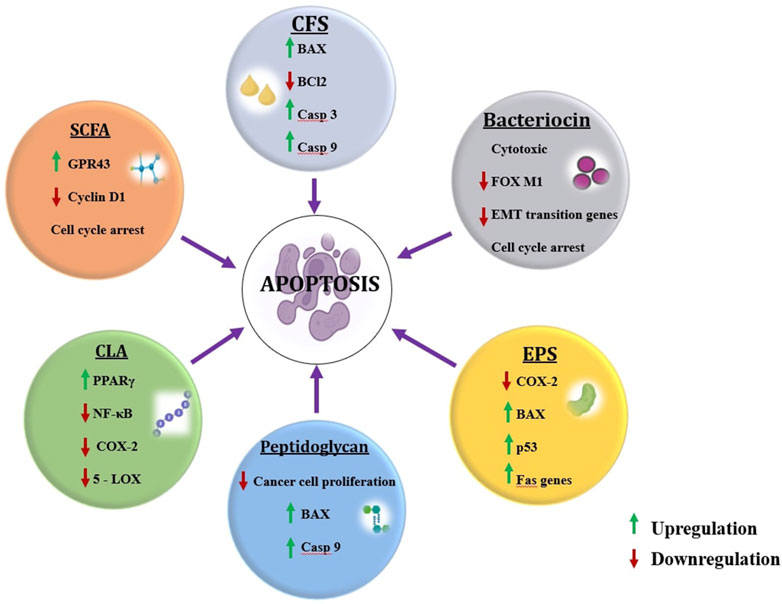
Figure 2. Postbiotic components and their roles in mitigating cancer. (Figures were generated using BioRender.com)
3.1 Cell-free supernatants
Cell-free supernatant (CFS) of probiotic strains has driven interest among researchers in finding bioactive molecules targeting various health problems. Generally, CFS is a fluid that consists of nutrients utilized in the growth medium and metabolites released as a result of microbial development (Lee et al., 2022). CFS of probiotics contains low molecular weight compounds like hydrogen peroxide, and organic acids and high molecular weight compounds like bacteriocins (Kapustian et al., 2018). These components are obtained from centrifugation of the grown cultures directly followed by filtration. The composition of CFS may vary with the supplementation in the base culture medium like MRSB (de Man Rogosa, and Sharpe broth). CFS has been explored widely for its anti-microbial, anti-biofilm, anti-inflammatory, and anti-cancer studies. The results obtained through numerous studies report that CFS of probiotic bacteria is one of the promising biotherapeutic agents to treat a wide range of diseases including cancer (Żółkiewicz et al., 2020).
As CFS of probiotic bacteria contains a wide range of metabolites, they are employed in studying many types of cancer. There are several studies carried out with the involvement of CFS of Lactobacillus spp., Bifidobacterium spp., Bacillus spp., Enterococcus spp., Pediococcus spp., Lactiplantibacillus spp., and Saccharomyces spp. using different cancer cell lines and induced animal models against cancers such as colon, breast, gastric, cervical, skin, and lung cancer (Table 2). Results obtained from these in vitro and in vivo studies suggest that CFS bears therapeutic potential in reducing tumor growth and inducing apoptosis. However, the proper mechanism of action of CFS against cancers and further phase trials need to be elucidated.
3.2 Bacteriocins
Bacteriocins are cationic peptide molecules that are produced by all classes of bacteria. These bioactive components are found to be heat-stable, proteases-sensitive, synthesized ribosomally, and have different modes of action (Darbandi et al., 2022). Bacteriocins have been classified into three classes I, II, and III by their structural and physio-chemical properties (Zacharof and Lovitt, 2012). Class I bacteriocins are known as lantibiotics and are small molecular weight peptides ranging from <5 KDa. Apart from lower molecular weight class I are heat stable and contains amino acids like lanthionine, and methyllanthionine. Further classification of class I bacteriocins is based on the charge associated and the mode of action. Class I lantibiotics are classified into two types, Type A lantibiotics (Ex: nisin, positively charged, causes pore formation in cell membrane on the target species) and type B lantibiotics (Ex: Mersacidin, negative charge, interferes in cell wall synthesis of target species) (Kaur and Kaur, 2015). Class II bacteriocins are <10 kDa, heat stable, and are commonly known as non-lanthionine peptides. Further classification of class II bacteriocins is subclass IIa (monomeric), IIb (Contains two segments), and IIc (circular bacteriocins) (Cotter et al., 2005). Class III are the high molecular weight heat liable proteins ranging around >30 kDa like colicins and enterolysin (Kaur and Kaur, 2015).
Several studies have carried out trials on bacteriocins against cancer. Factors that selectively act against cancer cells have been observed in numerous studies however, no proper mechanism of action could be proposed. Cancer cells are characterized by a lack of asymmetry in phospholipid types, and possess a negative charge due to the presence of anionic phosphatidylserine, glycosylated mucins, heparin, and gangliosides (Riedl et al., 2011). Bacteriocins are cationic peptides that can bind to the negatively charged membrane of cancer cells and act against them (Hoskin and Ramamoorthy, 2008). Here we have listed the studies where bacteriocins derived from probiotic bacterial strains were employed against types of cancer (Table 3).
3.3 Exopolysaccharides
Exopolysaccharides are biopolymers that microorganisms synthesize during their growth and metabolism (Welman and Maddox, 2003). Production of EPS can be varied from microorganism based on the monosaccharide composition and with the degree of branching. EPS is classified into homo-polysaccharide (containing the same monosaccharide units like dextran and cellulose) and hetero-polysaccharide with varying monosaccharides (xanthan) (Zhou et al., 2019). Synthesis of EPS is distinctly a strain-specific behavior and relies on several factors like the media composition, pH, and temperature (Behare et al., 2013). EPS is widely used in food industries as a stabilizing, emulsifying, and water-binding agent (Singh and Saini, 2017). The EPS of probiotic bacteria is found to exhibit anti-oxidative, anti-aging, anti-biofilm, and immunomodulatory effects as well as anti-tumor activity at in vitro and in vivo conditions (Di et al., 2017; Wang et al., 2019).
EPS has gained importance in scientific research due to its diverse properties like adherence towards intestinal epithelium and inhibition of pathogenic microbes in the gastrointestinal environment (Jurášková et al., 2022). Apart from anti-microbial, anti-biofilm, and anti-inflammatory, EPS derived from probiotic strains have been evaluated for their anti-cancer activity in various in vitro and in vivo conditions as shown in Table 4.
3.4 Short-chain fatty acids (SCFAs)
SCFAs belong to the metabolite produced by the probiotic bacteria as a result of metabolism and they are aliphatic compounds with 1-6 carbons. The gut microbiome has the potential to generate large amounts of SCFA from the available fermented carbohydrates and non-digestible components present in the gastrointestinal environment (Mirzaei et al., 2021). SCFAs are absorbed by the process of simple diffusion and active transport by transporters present over the membranes of all tissues and cells including the immune cells (Kim et al., 2014). SCFAs that are not taken up by the colonocytes are transported over the basolateral membrane enter the blood circulation and affect other cells directly (den Besten et al., 2013). Lack of SCFA production may lead to the pathogenicity of several diseases like asthma, neurological disease, and cancer (Tan et al., 2014). The most abundant SCFAs are acetate, propionate and butyrate, produced by Clostridium, Propionibacterium, and Lactobacillus species. In recent days, SCFAs have been developed and employed as immunomodulatory therapeutics as it has several advantages compared to the microbe-based methods (Feitelson et al., 2023). SCFAs interplay between the gut and different organs through systemic circulation (Tsvetikova and Koshel, 2020). Mainly SCFA-related effects are associated with two pathways: activation of GPCR (G-protein coupled receptors) and suppression of histone deacetylases (Carretta et al., 2021). SCFA, especially butyrate has been widely studied against cancer as it is believed to be involved in anti-cancer activity by altering cellular responses to the metabolism and oxidative stress (Vrzáčková et al., 2021). Several studies report that SCFA induces apoptosis in cancer cells by disrupting membrane potential, enhancing the expression of GPCR molecules, and mitochondrial depolarization (Table 5).
3.5 Conjugated linoleic acids (CLA)
Probiotics are capable of hydrogenation of long-chain fatty acids. In the process of hydrogenation, the free fatty acid is converted into its conjugate form (Dubey et al., 2012). Probiotic strains belonging to Bifidobacteria and Lactobacillus species are the predominant CLA producers that are widely used in several functional foods (Ghosh and George, 2023). Apart from these main groups of probiotics, species of Propionibacterium, Streptococcus, and Enterococcus present in the intestinal gut flora also produce fewer amounts of CLA (Palla et al., 2021). CLA production was also identified in the Pediococcus strain apart from well-known probiotics strains (Dubey et al., 2012). CLA has been shown to possess numerous health benefits like anti-diabetic, anti-inflammatory, anti-atherogenic, and anti-carcinogenic properties in both in vitro and in vivo studies (Ewaschuk et al., 2006). Scientific evidence depicts that CLA can inhibit the proliferation and growth of cancer cells and induce apoptosis (Table 6).
3.6 Peptidoglycan and other metabolites
Excepting the major postbiotic components, some structural compounds present in the probiotic bacteria also play a vital role in contributing to host health. Peptidoglycan, commonly known as murein is one of the major bacterial cell wall components which maintain the morphology of cells (Dramsi et al., 2008). Some researchers reported that these molecules possess anticancer activity by altering apoptotic gene expressions and inhibiting cell growth (Table 6). However, PG as a postbiotic component may augment inflammation. PG, being a part of PAMP (pathogen-associated molecular pattern)/or DAMP (damage-associated molecular pattern) may induce components of host-PRR (pattern recognition receptors), mainly via TLR-2, TLR-4 (toll-like recptors) to induce inflammation by innate immune cells, macrophages, neutrophils, dendritic cells to sustain inflammation at the local tissue microenvironments which may cause host-tissue damages. It may induce, and activate immune cells to release of proinflammatory cytokines like IL-1β, IL- 6, IL-8, and TNF α. Thus, it bears immunological limitations in its use for benefitting host health. Considering the wide range of applications of extracellular vesicles (EV), EVs from probiotic bacteria were employed against cancer cell lines. EVs are membrane-bound components that are spherical, consist of a lipid bilayer, and transfer genetic materials through the process of horizontal gene transfer (Ghosh, 2024; Ahmadi Badi et al., 2017). EVs contain proteins, DNA, RNA, glycolipids, polysaccharides, enzymes, and some endotoxins (Chelakkot et al., 2018). EVs were found to block the cell cycle and suppress cell proliferation (Table 7).
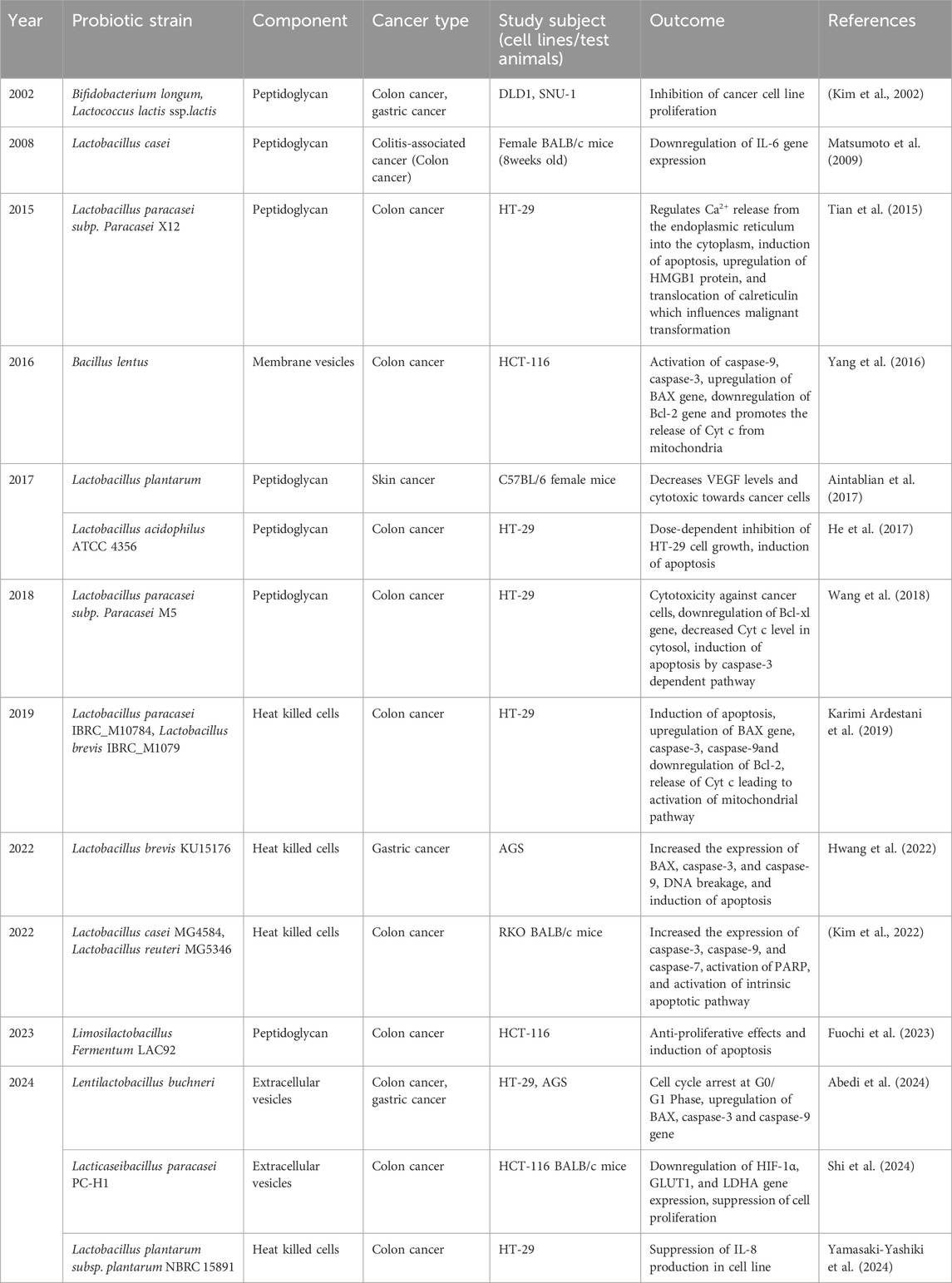
Table 7. Effects of heat-killed cells, peptidoglycan, and other cellular components of probiotics against cancer.
Apart from above mentioned bioactive postbiotics components, heat-killed (HK) probiotic cells have created experimental data in the field of medicine. HK cells exist in inactive form achieved by incomplete autoclaving and by cell freezing technique (Taverniti and Guglielmetti, 2011). These heat-killed cells showcased competency for adhesion sites against pathogens in a Caco-2 cell line model (Singh and Saini, 2017). Additionally, several findings validate that heat-killed cells have the potential to modulate host health and as a competing anti-cancer agent (Table 7)
4 Mechanism of action of postbiotics against cancer
4.1 CFS - Mechanism of action
CFS is a result of simple preparation by cultivating live probiotics in media, centrifugation, and filter sterilization which exhibits multiple probiotic characteristics. Study with CFSs of probiotics (Bifidobacterium adolescentis SPM0212, Lactobacillus rhamnosus GG, Lactobacillus delbrueckii, Bacillus polyfermenticus KU3, Lactobacillus fermentum NCIMB5221, Lactobacillus reuteri NCIMB 701359; Lactobacillus pentosus B281, Lactoplantibacillus plantarum B282; Lactobacillus casei (SR1,SR2), Lactobacillus paracasei (SR4); Lactobacillus salivarius and many other strains) using different cancer cell lines (e.g., Caco-2, HCT-116, HT-29, HeLa, LoVo, SW480, SW620, AGS, and MCF-7, CRL-1831and other cell lines related to respective cancer) demonstrated pathophysiological, cell biological and immunological impact to abrogate the cancer progression, metastasis by induction of apoptosis and inhibition of cancer cell proliferation; downregulation of cyclin D1 (cell cycle arrest in G1-phase), cyclooxygenase-2, protein kinase B and NF- κB activation; downregulation of Bcl-2 gene, cleavage of caspase-9, caspase-3, and PARP; downregulation of MMP2, MMP9, and suppression of the VEGF pathway; and upregulation of BAX, BAD genes (Table 2). CFAs of probiotic strains demonstrate a complete anti-cancer interaction with studied cell lines. Similarly, results obtained from animal studies using the C57BL/6 mouse (Liu et al., 2021), and MCF-7 xenograft mouse (Behzadi et al., 2021) model reveal that probiotic CFSs have the demonstrable potential for inhibition of cell proliferation and reduction in weight of tumor; and inhibition of epithelial-mesenchymal transition (EMT) by regulating Wnt/β-catenin pathway.
4.2 Bacteriocins - Mechanism of action
Bacteriocins were identified to induce apoptosis in cancer cells through cancer signaling pathways. As bacteriocins possess a cationic, amphiphilic, and hydrophobic nature, they target tumor cells resulting in apoptosis (Wang et al., 2024). Nisin, the class I lantibiotics, induced apoptosis in cancer cells by regulating the intrinsic pathway, intervened by mitochondria. Also, BCL-2 (B-cell lymphoma 2) family proteins such as Bcl-2 and BAX gene expression were altered in colon cancer cell lines (Ahmadi Badi et al., 2017). Normally, Bcl-2 protein expression is observed to be higher in cancer cells compared to that of normal cells. Apart from that, Bcl-2 family proteins act as an obstacle to apoptosis, develop resistance to the therapy, and in tumor development (Campbell and Tait, 2018). Generally, cancer cells are found to be resistant to apoptosis, on that note a study reported that, mechanisms that induce apoptosis begin with the release of cytochrome c (Cyt c) from the mitochondria and persuade ER to produce calcium. Both of these molecules play a vital role in apoptosome formation, activating cell surface death receptors and initiating caspase-dependent pathways (Joo et al., 2012). Cyt c mainly functions as an electron carrier during the mitochondrial respiratory chain, interacts with Apaf-1 (apoptotic protease activating factor-1) that exists in the cytosol, and enables it to form apoptosomes leading to activation of caspase-9 and caspase-3 that implements programmed cell death (Figure 3A) (Elena-Real et al., 2018). In another study, nisin treatment in human colon cancer cell lines showcased the altered expression of CEA (Carcinoembryonic antigen) and matrix metalloproteinase (MMP) genes (Norouzi et al., 2018). These MMPs are found to be potential modulators in the development of cancer, which can directly involve cancer signaling pathways and control apoptosis (Kessenbrock et al., 2015).
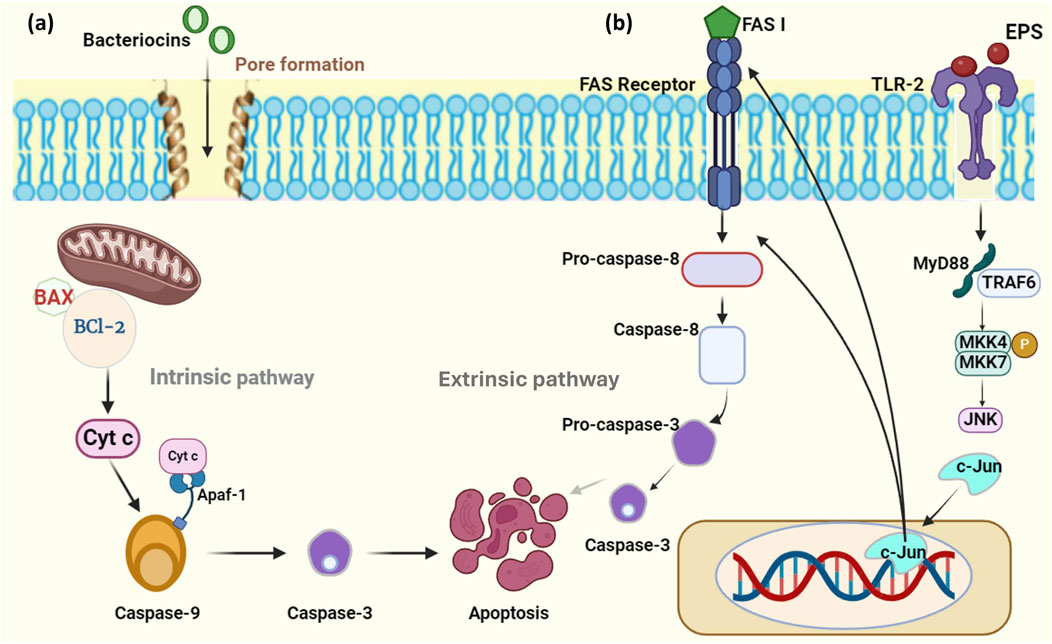
Figure 3. Mechanism of action of bacteriocin and EPS on cancer. (A) Bacteriocins downregulate the expression of the BCl-2 gene, upregulate the BAX gene, and induce mitochondria to release Cyt c which activates the intrinsic pathway resulting in apoptosis. (B) EPS binds to the TLR and activates c-Jun which helps in the activation of the caspase-8-mediated extrinsic apoptotic pathway. (Figures were generated using BioRender.com).
Some of the studies pointed out that enterocin resulted in cell cycle arrest on human cancer cell lines (Anakiah et al., 2017; Al-Fakharany et al., 2018; Al-Madboly et al., 2020). Cell cycle arrest is considered the emerging approach against cancer, as this mechanism supports tumor cells to restore their damaged DNA. Thus, negating cell cycle checkpoints before the DNA repair mechanism can lead to the activation of apoptotic cascade pathways that result in cell death would be a promising approach in cancer therapy (Schwartz et al., 2005).
4.3 Exopolysaccharides - Mechanism of action
Exopolysaccharides comprise proteins, extracellular DNA, lipids, and a major number of polysaccharides which enables them a wide range of health-benefiting properties (Di Martino, 2018). Several studies report that probiotic exopolysaccharides inhibit cancer cells without affecting normal cells, compared to synthetic drugs. There are various possible mechanisms of EPS to act upon cancer cells like induction of apoptosis, prevention of cell proliferation, and improvement of the host immune system (Angelin and Kavitha, 2020). Apoptosis is associated with two major caspase-dependent pathways known as intrinsic and extrinsic pathways. The differences in these two depend on the genes and proteins that are involved in driving the pathway. In intrinsic pathways, caspase-3, caspase-9, BAX, and, BCl-2 genes are expressed, whereas the extrinsic pathway involves caspase-8 and caspase-10 expression (Jan and Chaudhry, 2019). Activation of caspase-3 is a sign indicating that the cancer cells have undergone cell shrinkage, chromatin condensation, and nuclear fragmentation effectively (Jung et al., 2001). Previously it was found that EPS from L. gasseri was able to inhibit the proliferation of HeLa cells by upregulation of BAX and caspase three gene expression which leads to activation of apoptosis (Sungur et al., 2017). EPS derived from L. plantarum NCU116 witnessed an increase in the expression of pro-apoptotic genes like Fas, FasL, and c-Jun along with TLR-2 in mouse intestinal cells (Figure 3B) (Zhou et al., 2017). These Fas genes known as the first apoptosis signal along with its receptor mainly trigger the extrinsic pathway of apoptosis that is responsible for the suppression of tumors, so upregulation of the genes can induce apoptosis (Peter et al., 2015). EPS of L. delbrueckii ssp. Bulgaris exhibited upregulation of the p53 gene along with other caspase genes that are involved in inducing apoptosis (Khalil et al., 2022). p53 acts as a tumor suppressor gene, involved in inducing cell cycle arrest and a nuclear transcription factor possessing pro-apoptotic function. This gene is also found in high levels in patients suffering from cancer with mutant types of p53 (Ozaki and Nakagawara, 2011). Hence due to their disparate mechanisms listed in Table 3 in treating cancer cells, these can be employed in the treatment of cancer with evidence of phase trials.
4.4 Conjugated linoleic acid – mechanism of action
Even though CLA has been well known for its wide range of applications, there are fewer studies involving CLA derived from probiotics against cancer. A study found that CLA extracted from L. plantarum exhibited anti-cancer activity in mammalian breast cancer cell lines by suppressing the NF- κB pathway and then by upregulation of the BAX gene leading to an apoptotic pathway (Kadirareddy et al., 2016). Apart from this, CLA produced by P. pentosaceus GS4 possesses anti-cancer activity in colon cancer cell line (HCT-116) by downregulation of NF- κB and inducing apoptosis (Dubey et al., 2016). This NF- κB pathway is mainly involved in the development and progression of tumors, cellular immunity, inflammation, and regulation of cell differentiation. NF- κB promotes the expression of genes of the Bcl-2 family, caspase-8 inhibitor proteins, and other apoptosis-inhibiting proteins which primarily function by preventing apoptosis of a cell (Figure 4A) (Xia et al., 2018). Moreover, the NF- κB signaling pathway was identified as contributing to metastasis and also preventing the process of epithelial-to-mesenchymal transition (EMT) (Hoesel and Schmid, 2013).
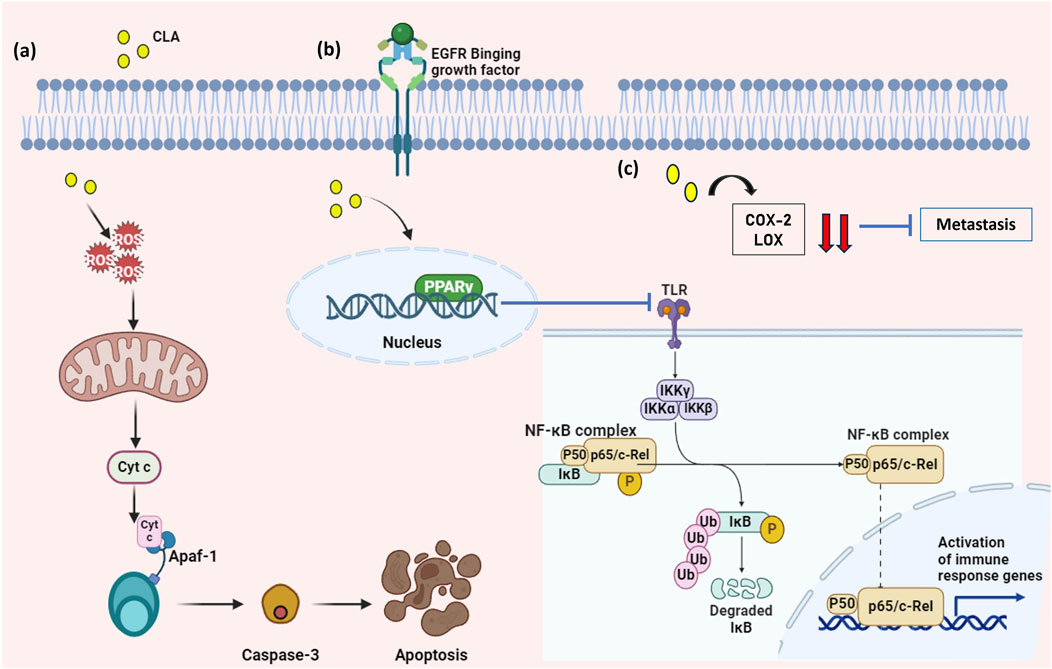
Figure 4. Mechanism of action of CLA against cancer. (A) CLAs produce ROS that stimulates the production of Cyt c which activates the caspase-cascade pathway leading to apoptosis. (B) CLAs upregulate the expression of PPAR γ in the nucleus which inhibits the NF- κB signaling pathway and (C) CLA downregulate the expression of COX and LOX genes that are involved in metastasis. (Figures were generated using BioRender.com).
Apart from these pathways, genes like COX-2 and LOX are identified and reported that they induce metastasis as well as cancer-supporting mechanisms. COX-2 gene is produced by fibroblasts that are associated with cancer and also by type 2 macrophage cells, which mainly promotes proliferation, apoptotic resistance, invasion, and metastasis in cancer cells (Hashemi Goradel et al., 2019). Along with the COX-2 gene, the LOX gene plays a vital role in inflating cancer cell proliferation, angiogenesis, and metastasis. CLA from P. pentosaceus GS4 has been reported to downregulate both the COX-2 and 5-LOX gene expression in the colon cancer cell line (HCT-116) (Figure 4C). Also, the upregulation of PPAR γ, a nuclear receptor that acts as a regulator of cell metabolism and functions as an inhibitor of cancer cell growth. PPAR γ causes oxidative stress and the flow of electrons that promote apoptotic cascades and some effects on mitochondria-mediated cell metabolism (Figure 4B). (Ghosh and George, 2023). CLA that is produced by the P. pentosaceus GS4 possesses biohydrogenation ability which modulates cancer by modulation of PPAR γ concerning anti-proliferative ability (Dubey et al., 2023).
4.5 Short-chain fatty acids – mechanism of action
SCFAs that are produced from probiotics possess anti-proliferative, apoptotic, and cell cycle arrest properties over cancer cells as well as contribute to prohibiting carcinogenesis in the gut (Tripathy et al., 2021). Harnessing SCFAs against cancer can pave the way to getting rid of the crisis because of its impact on the expression of multiple genes and their related pathways that are relevant to cancer. It is found that GPR43 suppresses tumor growth by modifying the gut flora (Kong et al., 2022). SCFAs also function as ligands for G-protein coupled receptors (GPCR). There are receptors like GPR43 which specifically have a higher affinity for propionate and GPR109a for butyrate (Feitelson et al., 2023). SCFAs are found to upregulate the expression of β-catenin and regulate Wnt which may promote the differentiation of cancer cells as well as induce intestinal homeostasis (Jiang et al., 2019). Some studies suggest that these molecules can interfere with cancer pathogenesis before tumor formation by regulating Wnt and inhibiting proliferation (Feitelson et al., 2023). Butyrate from Clostridium butyricum was found to suppress the development of tumors by interfering with the Wnt/β catenin pathway and also by increasing the gene expression of GPR43 and GPR109A (Figure 5A). (Chen et al., 2020). Similarly, butyrate from Butyricicoccus pullicaecorum employed against urinary bladder cancer upregulated the expression of GPR43 resulting in the mitigation of cancer (Wang et al., 2021). Butyrate from L. plantarum S2T10D was found to suppress the expression of the cyclin D1 gene and also arrest the cell cycle at the G2/M phase (Figure 5B). (Botta et al., 2022). Cyclin D1 is one of the key regulators that performs a central role in the pathogenicity of cancer determining the cell proliferation and overexpressed in cases of cancer, whereas they are properly regulated in normal cells. Targeting this cyclin D1 could be a promising strategy to prevent tumor development (Montalto and De Amicis, 2020).
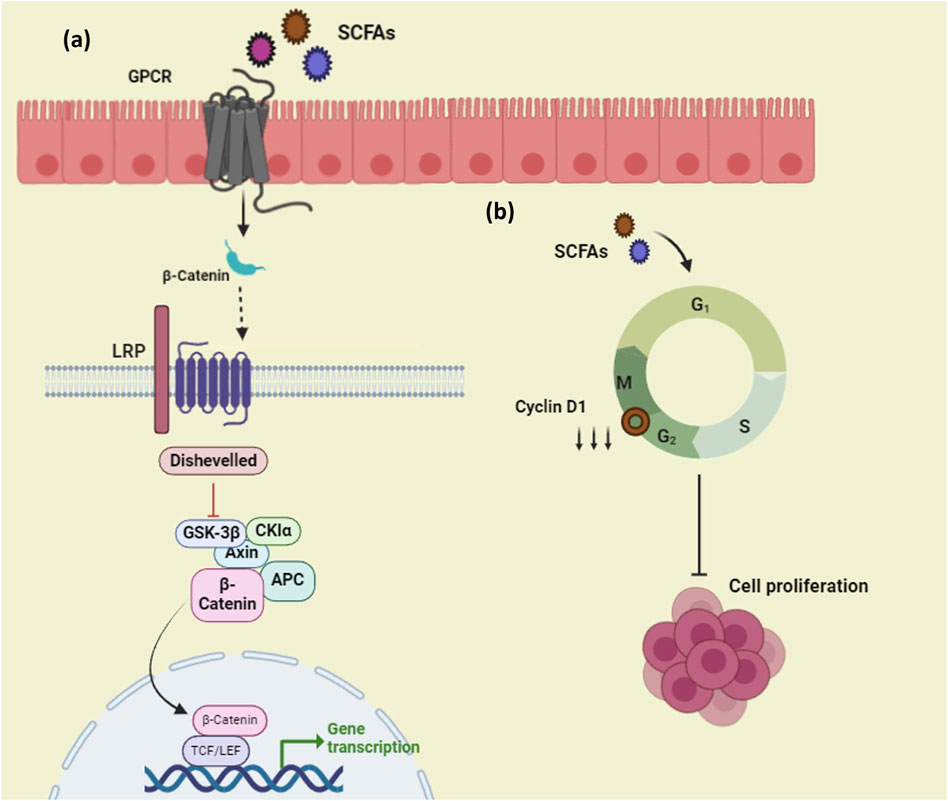
Figure 5. Mechanism of action of SCFAs on cancer, (A) SCFAs act as ligands to the GPCR present at the membrane layer and modulate the Wnt- β catenin pathway, (B)SCFAs downregulate the expression of CDK genes and inhibit cell proliferation of cancer cells. (Figures were generated using BioRender.com).
5 Future directions and conclusion
Cancer treatment in the modern era involves a dual approach based on the type of cancer and its associated characteristics like progression, area of localization, and metastasis (Ghosh and George 2023). Along with chemotherapy, other treatments are being developed with higher effectiveness and minimizing side effects to the host (Mármol et al., 2017). Probiotics and postbiotics have showcased their efficacy against various diseases and cancers with their role in vital processes like apoptosis induction, downregulation of tumor-inducing genes, suppression of cell proliferation, and prevention of metastasis (Sankarapandian et al., 2022). Growing shreds of evidence state that a combination of probiotics and postbiotics can be practiced as an adjuvant for patients undergoing chemotherapy (Lu et al., 2021). Significant research is in progress for employing probiotics and their bioactive metabolites (postbiotics) against cancer. Despite the wide usage of probiotics in treating different diseases, some side effects are caused in a small proportion of the population due to their uptake. In 2002, WHO-FAO released a report (http://www.fda.gov/ohrms/dockets/dockets/95s0316/95s-0316-rpt0282-tab-03-ref-19-joint-faowho-vol219.pdf) stating that “probiotics may be responsible for mainly four types of side effects” including systemic infections, deleterious metabolic activities, excessive immune stimulation in susceptible individuals, and gene transfer. Apart from these major effects, minor gastrointestinal symptoms like diarrhea, and other digestive problems are also reported after the uptake (Doron and Snydman, 2015). Probiotics have been reported to induce bacteremia, fungemia, localized infection, liver abscess, and endocarditis (Liu et al., 2024). Rather than live cells, these postbiotic components have created a cornerstone among researchers all over the globe due to their broad range of applications. In that case, bioactive compounds with anticancer properties, such as bacteriocins, EPS, SCFAs, and CLAs can be utilized as anticancer agents (Liu et al., 2021). Probiotics have been reported to induce bacteremia, fungemia, localized infection, liver abscess, and endocarditis (Liu et al., 2024). Rather than live cells, these postbiotic components have created a cornerstone among researchers all over the globe due to their broad range of applications. In that case, bioactive compounds with anticancer properties, such as bacteriocins, EPS, SCFAs, and CLAs can be utilized as anticancer agents (Liu et al., 2021). Despite their numerous properties, they possess some limitations like decreased bioavailability, and susceptibility against proteolytic enzymes in the GIT when they are orally administered. To overcome this lag, various strategies like encapsulation technology involving semi-synthetic techniques can improve their biological activity, stability, and also physiochemical activities (Xu et al., 2024). Even though there are numerous research articles, review articles, and products based on probiotics, there is countable proper evidence with clinical studies provided for the usage of probiotics with or without postbiotics for cancer prevention.
The present review highlights the involvement of postbiotic components from the potential probiotic strains employed against various types of cancer in both in vitro and in vivo studies along with their reported mechanism of action. From the above-reported studies, it is evident that different postbiotic components can be introduced in treating cancer as adjuvants that aid in decreasing the side effects caused by regular treatments. The rising trends of outcomes from the research are growing significantly with well-grounded data before recommending probiotics and postbiotics as alternative therapies for treating and preventing various forms of cancer. However, extensive research is needed to explore the anticancer efficacy of the specific or combined postbiotic-bioactive components as an alternative treatment strategy for preventing and controlling cancers.
Author contributions
AS: Writing–review and editing, Writing–original draft, Visualization, Formal Analysis, Data curation. AG: Writing–review and editing, Writing–original draft, Visualization, Validation, Supervision, Formal Analysis, Conceptualization.
Funding
The author(s) declare that no financial support was received for the research, authorship, and/or publication of this article.
Acknowledgments
The authors would like to express gratitude to the Vellore Institute of Technology, Vellore for pursuing research work.
Conflict of interest
The authors declare that the research was conducted in the absence of any commercial or financial relationships that could be construed as a potential conflict of interest.
Publisher’s note
All claims expressed in this article are solely those of the authors and do not necessarily represent those of their affiliated organizations, or those of the publisher, the editors and the reviewers. Any product that may be evaluated in this article, or claim that may be made by its manufacturer, is not guaranteed or endorsed by the publisher.
Abbreviations
BAX, Bcl-2 associated protein X; BAK, Bcl-2 homologous antagonist/killer; GLUT-1, Glucose transporter 1; LDHA, Lactate dehydrogenase A; Cyt c, Cytochrome c; VEGF, Vascular endothelial growth factor; IL, Interleukin; COX-2, Cyclooxygenase 2; 5-LOX, 5-lipoxygenase; NF- κB, Nuclear factor kappa B; PPAR, Peroxisome proliferator-activated receptors; GPR, G-Protein coupled receptor; ROS, Reactive oxygen species; Fas, First apoptosis signal; FOX M1, Forkhead Box Protein M1; CEA, Carcinoembryonic antigen; EMT, Epithelial-mesenchymal transition; TNF, Tumor necrosis factor; MMP, Matrix Metalloproteinases.
References
Abbasi, A., Rad, A. H., Maleki, L. A., Kafil, H. S., and Baghbanzadeh, A. (2023). Cytotoxic potentials of cell-free supernatant derived from lactobacillus casei CRL431 on HCT-116 and HT-29 human colon cancer cell lines. Biointerface Res. Appl. Chem. 13 (5). doi:10.33263/BRIAC135.476
Abdelnasser, S. M., Yahya, S. M. M., Mohamed, W. F., Asker, M. M. S., Abu Shady, H. M., Mahmoud, M. G., et al. (2017). Antitumor exopolysaccharides derived from novel marine Bacillus: isolation, characterization aspect and biological activity. Asian pac. J. Cancer Prev. 18 (7), 1847–1854. doi:10.22034/APJCP.2017.18.7.1847
Abedi, A., Tafvizi, F., Akbari, N., and Jafari, P. (2023). Cell-free supernatant of L. Buchneri probiotic bacteria enhancing apoptosis activity in AGS gastric cancer cells. Iran. J. Sci. 47 (4), 1071–1079. doi:10.1007/s40995-023-01495-7
Abedi, A., Tafvizi, F., Jafari, P., and Akbari, N. (2024). The inhibition effects of lentilactobacillus buchneri-derived membrane vesicles on AGS and HT-29 cancer cells by inducing cell apoptosis. Sci. Rep. 14 (1), 3100. doi:10.1038/s41598-024-53773-y
Adiyoga, R., Arief, I. I., Budiman, C., and Abidin, Z. (2022). In vitro anticancer potentials of Lactobacillus plantarum IIA-1A5 and Lactobacillus acidophilus IIA-2B4 extracts against WiDr human colon cancer cell line. Food Sci. Technol. braz. 42. doi:10.1590/fst.87221
Adumuah, N. N., Quarshie, J. T., Danwonno, H., Aikins, A. R., and Ametefe, E. N. (2024). Exploring anti-breast cancer effects of live Pediococcus acidilactici and its cell-free supernatant isolated from human breast milk. Int. J. Breast Cancer 2024, 1841909. doi:10.1155/2024/1841909
Afzaal, M., Saeed, F., Shah, Y. A., Hussain, M., Rabail, R., Socol, C. T., et al. (2022). Human gut microbiota in health and disease: unveiling the relationship. Front. Microbiol. 13, 999001. doi:10.3389/fmicb.2022.999001
Ahlawat, S., Asha, , and Sharma, K. K. (2021). Gut-organ axis: a microbial outreach and networking. Lett. Appl. Microbiol. 72 (6), 636–668. doi:10.1111/lam.13333
Ahmadi, S., Ghollasi, M., and Hosseini, H. M. (2017). The apoptotic impact of nisin as a potent bacteriocin on the colon cancer cells. Microb. Pathog. 111, 193–197. doi:10.1016/j.micpath.2017.08.037
Ahmadi Badi, S., Moshiri, A., Fateh, A., Rahimi Jamnani, F., Sarshar, M., Vaziri, F., et al. (2017). Microbiota-derived extracellular vesicles as new systemic regulators. Front. Microbiol. 8, 1610. doi:10.3389/fmicb.2017.01610
Aintablian, A., Jaber, D. F., Jallad, M. A., and Abdelnoor, A. M. (2017). The effect of lactobacillus plantarum and bacterial peptidoglycan on the growth of mouse tumors in vivo and in vitro. Am. J. Immunol. 13 (3), 201–208. doi:10.3844/ajisp.2017.201.208
Akbarali, H. I., Muchhala, K. H., Jessup, D. K., and Cheatham, S. (2022). Chemotherapy induced gastrointestinal toxicities. Adv. cancer Res. 155, 131–166. doi:10.1016/bs.acr.2022.02.007
Al-Fakharany, O. M., Aziz, A. A., El-Banna, T. E. S., and Sonbol, F. I. (2018). Immunomodulatory and anticancer activities of enterocin Oe-342 produced by Enterococcus feacalis isolated from stool. J. Clin. Cell. Immunol 9, 1000558.
Ali, M. S., Lee, E. B., Hsu, W. H., Suk, K., Sayem, S. A. J., Ullah, H. M. A., et al. (2023). Probiotics and postbiotics as an alternative to antibiotics: an emphasis on pigs. Pathog. Basel, Switz. 12 (7), 874. doi:10.3390/pathogens12070874
Al-Madboly, L. A., El-Deeb, N. M., Kabbash, A., Nael, M. A., Kenawy, A. M., and Ragab, A. E. (2020). Purification, characterization, identification, and anticancer activity of a circular bacteriocin from Enterococcus thailandicus. Front. Bioeng. Biotechnol. 8, 450. doi:10.3389/fbioe.2020.00450
Amin, M., Navidifar, T., Saeb, S., Barzegari, E., and Jamalan, M. (2023). Tumor-targeted induction of intrinsic apoptosis in colon cancer cells by Lactobacillus plantarum and Lactobacillus rhamnosus strains. Mol. Biol. Rep. 50 (6), 5345–5354. doi:10.1007/s11033-023-08445-x
Angelin, J., and Kavitha, M. (2020). Exopolysaccharides from probiotic bacteria and their health potential. Int. J. Biol. Macromol. 162, 853–865. doi:10.1016/j.ijbiomac.2020.06.190
Ankaiah, D., Esakkiraj, P., Perumal, V., Ayyanna, R., and Venkatesan, A. (2017). Probiotic characterization of enterococcus faecium por1: Cloning, over expression of enterocin-A and evaluation of antibacterial, anti-cancer properties. J. Funct. Foods 38, 280–292. doi:10.1016/j.jff.2017.09.034
Ankaiah, D., Palanichamy, E., Antonyraj, C. B., Ayyanna, R., Perumal, V., Ahamed, S. I. B., et al. (2018). Cloning, overexpression, purification of bacteriocin enterocin-B and structural analysis, interaction determination of enterocin-A, B against pathogenic bacteria and human cancer cells. Int. J. Biol. Macromol. 116, 502–512. doi:10.1016/j.ijbiomac.2018.05.002
Ashraf, R., and Shah, N. P. (2014). Immune system stimulation by probiotic microorganisms. Crit. Rev. food Sci. Nutr. 54 (7), 938–956. doi:10.1080/10408398.2011.619671
Asseri, A. H., Bakhsh, T., Abuzahrah, S. S., Ali, S., and Rather, I. A. (2023). The gut dysbiosis-cancer axis: illuminating novel insights and implications for clinical practice. Front. Pharmacol. 14, 1208044. doi:10.3389/fphar.2023.1208044
Baghbani-Arani, F., Asgary, V., and Hashemi, A. (2020). Cell-free extracts of Lactobacillus acidophilus and Lactobacillus delbrueckii display antiproliferative and antioxidant activities against HT-29 cell line. Nutr. Cancer 72 (8), 1390–1399. doi:10.1080/01635581.2019.1685674
Baindara, P., Gautam, A., Raghava, G. P. S., and Korpole, S. (2017). Anticancer properties of a defensin like class IId bacteriocin Laterosporulin10. Sci. Rep. 7, 46541. doi:10.1038/srep46541
Balcik-Ercin, P., and Sever, B. (2022). An investigation of bacteriocin nisin anti-cancer effects and FZD7 protein interactions in liver cancer cells. Chemico-Biological Interact. 366, 110152. doi:10.1016/j.cbi.2022.110152
Barigela, A., and Bhukya, B. (2021). Probiotic Pediococcus acidilactici strain from tomato pickle displays anti-cancer activity and alleviates gut inflammation in-vitro. 3 Biotech. 11 (1), 23. doi:10.1007/s13205-020-02570-1
Behare, P. V., Singh, R., Nagpal, R., and Rao, K. H. (2013). Exopolysaccharides producing Lactobacillus fermentum strain for enhancing rheological and sensory attributes of low-fat dahi. J. food Sci. Technol. 50 (6), 1228–1232. doi:10.1007/s13197-013-0999-6
Behzadi, R., Hormati, A., Eivaziatashbeik, K., Ahmadpour, S., Khodadust, F., and Seidi, K. (2021). Evaluation of anti-tumor potential of Lactobacillus acidophilus ATCC4356 culture supernatants in MCF-7 breast cancer. Anticancer Agents Med Chem. (Formerly Current Medicinal Chemistry-Anti-Cancer Agents) 21 (14), 1861–1870.
Botta, C., Spyridopoulou, K., Bertolino, M., Rantsiou, K., Chlichlia, K., and Cocolin, L. (2022). Lactiplantibacillus plantarum inhibits colon cancer cell proliferation as function of its butyrogenic capability. Biomed. Pharmacother. 149, 112755. doi:10.1016/j.biopha.2022.112755
Bray, F., Ferlay, J., Soerjomataram, I., Siegel, R. L., Torre, L. A., and Jemal, A. (2018). Global cancer statistics 2018: GLOBOCAN estimates of incidence and mortality worldwide for 36 cancers in 185 countries. CA a cancer J. Clin. 68 (6), 394–424. doi:10.3322/caac.21492
Bron, P. A., van Baarlen, P., and Kleerebezem, M. (2011). Emerging molecular insights into the interaction between probiotics and the host intestinal mucosa. Nat. Rev. Microbiol. 10 (1), 66–78. doi:10.1038/nrmicro2690
Broughton, L. J., Giuntini, F., Savoie, H., Bryden, F., Boyle, R. W., Maraveyas, A., et al. (2016). Duramycin-porphyrin conjugates for targeting of tumour cells using photodynamic therapy. J Photochem Photobiol B. 163, 374–384.
Budu, O., Mioc, A., Soica, C., Caruntu, F., Milan, A., Oprean, C., et al. (2024). Lactiplantibacillus plantarum induces apoptosis in melanoma and breast cancer cells. Microorganisms 12 (1), 182. doi:10.3390/microorganisms12010182
Campbell, K. J., and Tait, S. W. G. (2018). Targeting BCL-2 regulated apoptosis in cancer. Open Biol. 8 (5), 180002. doi:10.1098/rsob.180002
Cardona, D., and Roman, P. (2022). New perspectives in health: gut microbiota. Int. J. Environ. Res. public health 19 (10), 5828. doi:10.3390/ijerph19105828
Carretta, M. D., Quiroga, J., López, R., Hidalgo, M. A., and Burgos, R. A. (2021). Participation of short-chain fatty acids and their receptors in gut inflammation and colon cancer. Front. Physiol. 12, 662739. doi:10.3389/fphys.2021.662739
Casanova, M. R., Azevedo-Silva, J., Rodrigues, L. R., and Preto, A. (2018). Colorectal cancer cells increase the production of short chain fatty acids by Propionibacterium freudenreichii impacting on cancer cells survival. Front. Nutr. 5, 44. doi:10.3389/fnut.2018.00044
Chang, S. C., Shen, M. H., Liu, C. Y., Pu, C. M., Hu, J. M., and Huang, C. J. (2020). A gut butyrate producing bacterium Butyricicoccus pullicaecorum regulates short chain fatty acid transporter and receptor to reduce the progression of 1,2 dimethylhydrazine associated colorectal cancer. Oncol. Lett. 20 (6), 327. doi:10.3892/OL.2020.12190
Chelakkot, C., Choi, Y., Kim, D. K., Park, H. T., Ghim, J., Kwon, Y., et al. (2018). Akkermansia muciniphila-derived extracellular vesicles influence gut permeability through the regulation of tight junctions. Exp. and Mol. Med. 50 (2), e450. doi:10.1038/emm.2017.282
Chen, D., Jin, D., Huang, S., Wu, J., Xu, M., Liu, T., et al. (2020). Clostridium butyricum, a butyrate-producing probiotic, inhibits intestinal tumor development through modulating Wnt signaling and gut microbiota. Cancer Lett. 469, 456–467. doi:10.1016/j.canlet.2019.11.019
Chen, Y. T., Yuan, Q., Shan, L. T., Lin, M. A., Cheng, D. Q., and Li, C. Y. (2013). Antitumor activity of bacterial exopolysaccharides from the endophyte bacillus amyloliquefaciens sp.isolated from ophiopogon japonicus. Oncol. Lett. 5 (6), 1787–1792. doi:10.3892/ol.2013.1284
Chiang, C. J., and Hong, Y. H. (2021). In situ delivery of biobutyrate by probiotic Escherichia coli for cancer therapy. Sci. Rep. 11 (1), 18172. doi:10.1038/s41598-021-97457-3
Chitapanarux, I., Chitapanarux, T., Traisathit, P., Kudumpee, S., Tharavichitkul, E., and Lorvidhaya, V. (2010). Randomized controlled trial of live lactobacillus acidophilus plus bifidobacterium bifidum in prophylaxis of diarrhea during radiotherapy in cervical cancer patients. Radiat. Oncol. 5 (1), 31. doi:10.1186/1748-717X-5-31
Cicero, A. F. G., Fogacci, F., Bove, M., Giovannini, M., and Borghi, C. (2021). Impact of a short-term synbiotic supplementation on metabolic syndrome and systemic inflammation in elderly patients: a randomized placebo-controlled clinical trial. Eur. J. Nutr. 60 (2), 655–663. doi:10.1007/s00394-020-02271-8
Coakley, M., Banni, S., Johnson, M. C., Mills, S., Devery, R., Fitzgerald, G., et al. (2009). Inhibitory effect of conjugated alpha-linolenic acid from bifidobacteria of intestinal origin on SW480 cancer cells. Lipids 44 (3), 249–256. doi:10.1007/s11745-008-3269-z
Coakley, M., Johnson, M. C., McGrath, E., Rahman, S., Ross, R. P., Fitzgerald, G. F., et al. (2006). Intestinal bifidobacteria that produce trans-9, trans-11 conjugated linoleic acid: a fatty acid with antiproliferative activity against human colon SW480 and HT-29 cancer cells. Nutr. Cancer 56 (1), 95–102. doi:10.1207/s15327914nc5601_13
Cotter, P. D., Hill, C., and Ross, R. P. (2005). Bacteriocins: developing innate immunity for food. Nat. Rev. Microbiol. 3 (10), 777–788. doi:10.1038/nrmicro1273
Darbandi, A., Asadi, A., Mahdizade Ari, M., Ohadi, E., Talebi, M., Halaj Zadeh, M., et al. (2022). Bacteriocins: properties and potential use as antimicrobials. J. Clin. Lab. Anal. 36 (1), e24093. doi:10.1002/jcla.24093
Debela, D. T., Muzazu, S. G., Heraro, K. D., Ndalama, M. T., Mesele, B. W., Haile, D. C., et al. (2021). New approaches and procedures for cancer treatment: current perspectives, SAGE Open Med. 9, 20503121211034366. doi:10.1177/20503121211034366
Deepak, V., Ramachandran, S., Balahmar, R. M., Pandian, S. R. K., Sivasubramaniam, S. D., Nellaiah, H., et al. (2016). In vitro evaluation of anticancer properties of exopolysaccharides from Lactobacillus acidophilus in colon cancer cell lines. Vitro Cell. Dev. Biol. - Anim. 52 (2), 163–173. doi:10.1007/s11626-015-9970-3
DeGruttola, A. K., Low, D., Mizoguchi, A., and Mizoguchi, E. (2016). Current understanding of dysbiosis in disease in human and animal models. Inflamm. bowel Dis. 22 (5), 1137–1150. doi:10.1097/MIB.0000000000000750
De Marco, S., Sichetti, M., Muradyan, D., Piccioni, M., Traina, G., Pagiotti, R., et al. (2018). Probiotic cell-free supernatants exhibited anti-inflammatory and antioxidant activity on human gut epithelial cells and macrophages stimulated with LPS. Evidence-based Complement. Altern. Med. eCAM 2018, 1756308. doi:10.1155/2018/1756308
Demers, M., Dagnault, A., and Desjardins, J. (2014). A randomized double-blind controlled trial: impact of probiotics on diarrhea in patients treated with pelvic radiation. Clin. Nutr. 33 (5), 761–767. doi:10.1016/j.clnu.2013.10.015
den Besten, G., van Eunen, K., Groen, A. K., Venema, K., Reijngoud, D. J., and Bakker, B. M. (2013). The role of short-chain fatty acids in the interplay between diet, gut microbiota, and host energy metabolism. J. lipid Res. 54 (9), 2325–2340. doi:10.1194/jlr.R036012
Deshpande, G., Athalye-Jape, G., and Patole, S. (2018). Para-probiotics for preterm neonates-the next frontier. Nutrients 10 (7), 871. doi:10.3390/nu10070871
Di, W., Zhang, L., Wang, S., Yi, H., Han, X., Fan, R., et al. (2017). Physicochemical characterization and antitumour activity of exopolysaccharides produced by Lactobacillus casei SB27 from yak milk. Carbohydr. Polym. 171, 307–315. doi:10.1016/j.carbpol.2017.03.018
Dikeocha, I. J., Al-Kabsi, A. M., Chiu, H. T., and Alshawsh, M. A. (2022). Faecalibacterium prausnitzii Ameliorates colorectal tumorigenesis and suppresses proliferation of HCT116 colorectal cancer cells. Biomedicines 10 (5), 1128. doi:10.3390/biomedicines10051128
Di Martino, P. (2018). Extracellular polymeric substances, a key element in understanding biofilm phenotype. AIMS Microbiol. 4 (2), 274–288. doi:10.3934/microbiol.2018.2.274
Dong, Y., Zhu, J., Zhang, M., Ge, S., and Zhao, L. (2020). Probiotic Lactobacillus salivarius Ren prevent dimethylhydrazine-induced colorectal cancer through protein kinase B inhibition. Appl. Microb. CELL Physiol. 104, 7377–7389. doi:10.1007/s00253-020-10775-w
Doron, S., and Snydman, D. R. (2015). Risk and safety of probiotics. Clin. Infect. Dis. official Publ. Infect. Dis. Soc. Am. 60 (Suppl. 2), S129–S134. doi:10.1093/cid/civ085
Dramsi, S., Magnet, S., Davison, S., and Arthur, M. (2008). Covalent attachment of proteins to peptidoglycan. FEMS Microbiol. Rev. 32 (2), 307–320. doi:10.1111/j.1574-6976.2008.00102.x
Dubey, V., Ghosh, A. R., Bishayee, K., and Khuda-Bukhsh, A. R. (2016). Appraisal of the anti-cancer potential of probiotic Pediococcus pentosaceus GS4 against colon cancer: in vitro and in vivo approaches. J. Funct. Foods 23, 66–79. doi:10.1016/j.jff.2016.02.032
Dubey, V., Ghosh, A. R., and Mandal, B. K. (2012). Appraisal of conjugated linoleic acid production by probiotic potential of Pediococcus spp. GS4. Appl. Biochem. Biotechnol. 168 (5), 1265–1276. doi:10.1007/s12010-012-9855-9
Dubey, V., Mishra, A. K., and Ghosh, A. R. (2023). Appraisal of the possible role of PPAR γ upregulation by CLA of probiotic Pediococcus pentosaceus GS4 in colon cancer mitigation. PPAR Research 2023, 9458308. doi:10.1155/2023/9458308
El-Deeb, N. M., Yassin, A. M., Al-Madboly, L. A., and El-Hawiet, A. (2018). A novel purified Lactobacillus acidophilus 20079 exopolysaccharide, LA-EPS-20079, molecularly regulates both apoptotic and NF-ΚB inflammatory pathways in human colon cancer. Microb. Cell Factories 17 (1), 29. doi:10.1186/s12934-018-0877-z
Elena-Real, C. A., Díaz-Quintana, A., González-Arzola, K., Velázquez-Campoy, A., Orzáez, M., López-Rivas, A., et al. (2018). Cytochrome c speeds up caspase cascade activation by blocking 14-3-3ε-dependent Apaf-1 inhibition. Cell death and Dis. 9 (3), 365. doi:10.1038/s41419-018-0408-1
El-Newary, S. A., Ibrahim, A. Y., Asker, M. S., Mahmoud, M. G., and El Awady, M. E. (2017). Production, characterization and biological activities of acidic exopolysaccharide from marine Bacillus amyloliquefaciens 3MS 2017. Asian pac. J. Trop. Med. 10 (7), 652–662. doi:10.1016/j.apjtm.2017.07.005
Escamilla, J., Lane, M. A., and Maitin, V. (2012). Cell-free supernatants from probiotic lactobacillus casei and lactobacillus rhamnosus GG decrease colon cancer cell invasion in vitro. Nutr. Cancer 64 (6), 871–878. doi:10.1080/01635581.2012.700758
Ewaschuk, J. B., Walker, J. W., Diaz, H., and Madsen, K. L. (2006). Bioproduction of conjugated linoleic acid by probiotic bacteria occurs in vitro and in vivo in mice. J. Nutr. 136, 1483–1487. doi:10.1093/jn/136.6.1483
Falzone, L., Salomone, S., and Libra, M. (2018). Evolution of cancer pharmacological treatments at the turn of the third millennium. Front. Pharmacol. 9, 1300. doi:10.3389/fphar.2018.01300
Feitelson, M. A., Arzumanyan, A., Medhat, A., and Spector, I. (2023). Short-chain fatty acids in cancer pathogenesis. Cancer metastasis Rev. 42 (3), 677–698. doi:10.1007/s10555-023-10117-y
Fuochi, V., Spampinato, M., Distefano, A., Palmigiano, A., Garozzo, D., Zagni, C., et al. (2023). Soluble peptidoglycan fragments produced by Limosilactobacillus fermentum with antiproliferative activity are suitable for potential therapeutic development: a preliminary report. Front. Mol. Biosci. 10, 1082526. doi:10.3389/fmolb.2023.1082526
Furusawa, Y., Obata, Y., Fukuda, S., Endo, T. A., Nakato, G., Takahashi, D., et al. (2013). Commensal microbe-derived butyrate induces the differentiation of colonic regulatory T cells. Nature 504 (7480), 446–450. doi:10.1038/nature12721
Gensollen, T., Iyer, S. S., Kasper, D. L., and Blumberg, R. S. (2016). How colonization by microbiota in early life shapes the immune system. Sci. (New York, N.Y.) 352 (6285), 539–544. doi:10.1126/science.aad9378
Ghosh, A. R. (2024). “Probiotics-associated extracellular vesicles in health and diseases,” in Extracellular vesicles in human health and diseases. Editor A. R. Ghosh (Singapore: Springer). doi:10.1007/978-981-97-2494-9_7
Ghosh, A. R., and George, J. (2023). Advances in cancer therapeutics: potential probiotics on cancer therapy. 1, 34. doi:10.1007/978-981-99-2196-6_46-1
Gibson, G., Hutkins, R., Sanders, M., Prescott, S. L., Reimer, R. A., Salminen, S. J., et al. (2017). Expert consensus document: the International Scientific Association for Probiotics and Prebiotics (ISAPP) consensus statement on the definition and scope of prebiotics. Nat. Rev. Gastroenterol. Hepatol. 14, 491–502. doi:10.1038/nrgastro.2017.75
Giralt, J., Regadera, J. P., Verges, R., Romero, J., de la Fuente, I., Biete, A., et al. (2008). Effects of probiotic lactobacillus casei DN-114 001 in prevention of radiation-induced diarrhea: results from Multicenter, randomized, placebo-controlled nutritional trial. Int. J. Radiat. Oncol. Biol. Phys. 71, 1213–1219. doi:10.1016/j.ijrobp.2007.11.009
Golkhalkhali, B., Rajandram, R., Paliany, A. S., Ho, G. F., Wan Ishak, W. Z., Johari, C. S., et al. (2018). Strain-specific probiotic (microbial cell preparation) and omega-3 fatty acid in modulating quality of life and inflammatory markers in colorectal cancer patients: a randomized controlled trial. Asia-Pacific J. Clin. Oncol. 14 (3), 179–191. doi:10.1111/ajco.12758
Guo, Y., Zhang, T., Gao, J., Jiang, X., Tao, M., Zeng, X., et al. (2020). Lactobacillus acidophilus CICC 6074 inhibits growth and induces apoptosis in colorectal cancer cells in vitro and in HT-29 cells induced-mouse model. J. Funct. Foods 75, 104290. doi:10.1016/j.jff.2020.104290
Hamad, G., Ombarak, R. A., Eskander, M., Mehany, T., Anees, F. R., Elfayoumy, R. A., et al. (2022). Detection and inhibition of Clostridium botulinum in some Egyptian fish products by probiotics cell-free supernatants as bio-preservation agents. LWT 163 (2022), 113603. doi:10.1016/j.lwt.2022.113603
Haroun, B. M., Refaat, B. M., El-Menoufy, H. A., Amin, H. A., and El-Waseif, A. A. (2013). Original articles structure analysis and antitumor activity of the exopolysaccharide from probiotic lactobacillus plantarum NRRL B-4496 in vitro and in vivo. J. Appl. Sci. Res. 9 (Issue 1).
Hashemi Goradel, N., Najafi, M., Salehi, E., Farhood, B., and Mortezaee, K. (2019). Cyclooxygenase-2 in cancer: a review. J. Cell. Physiol. 234 (5), 5683–5699. doi:10.1002/jcp.27411
Hawrelak, J. (2003). Probiotics: choosing the right one for your needs. J. Aust. Traditional-Med. Soc. 9 (2), 67–75.
He, J., Wu, Z., Pan, D., Guo, Y., and Zeng, X. (2017). Effect of selenylation modification on antitumor activity of peptidoglycan from Lactobacillus acidophilus. Carbohydr. Polym. 165, 344–350. doi:10.1016/j.carbpol.2017.02.031
Hennessy, A. A., Ross, P., Devery, R., and Stanton, C. (2016). Bifidobacterially produced, C18:3 and C18:4 conjugated fatty acids exhibit in vitro anti-carcinogenic and anti-microbial activity. Eur. J. Lipid Sci. Technol. 118 (11), 1743–1758. doi:10.1002/ejlt.201500424
Hill, C., Guarner, F., Reid, G., Gibson, G. R., Merenstein, D. J., Pot, B., et al. (2014). Expert consensus document. The International Scientific Association for Probiotics and Prebiotics consensus statement on the scope and appropriate use of the term probiotic. Nat. Rev. Gastroenterology and hepatology 11 (8), 506–514. doi:10.1038/nrgastro.2014.66
Hoesel, B., and Schmid, J. A. (2013). The complexity of NF-κB signaling in inflammation and cancer. Mol. Cancer 12, 86. doi:10.1186/1476-4598-12-86
Hoskin, D. W., and Ramamoorthy, A. (2008). Studies on anticancer activities of antimicrobial peptides. Biochim. Biophys. acta 1778 (2), 357–375. doi:10.1016/j.bbamem.2007.11.008
Hutkins, R. W., Krumbeck, J. A., Bindels, L. B., Cani, P. D., Fahey, G., Goh, Y. J., et al. (2016). Prebiotics: why definitions matter. Curr. Opin. Biotechnol. 37, 1–7. doi:10.1016/j.copbio.2015.09.001
Hwang, C. H., Lee, N. K., and Paik, H. D. (2022). The anti-cancer potential of heat-killed lactobacillus brevis KU15176 upon AGS cell lines through intrinsic apoptosis pathway. Int. J. Mol. Sci. 23 (8), 4073. doi:10.3390/ijms23084073
Ibrahim, A. Y., Youness, E. R., Mahmoud, M. G., Asker, M. S., and El-Newary, S. A. (2020). Acidic exopolysaccharide produced from marine Bacillus amyloliquefaciens 3MS 2017 for the protection and treatment of breast cancer. Breast Cancer Basic Clin. Res. 14, 1178223420902075. doi:10.1177/1178223420902075
Indian Council of Medical Research Task Force, Co-ordinating Unit ICMR, Co-ordinating Unit DBT (2011). ICMR-DBT guidelines for evaluation of probiotics in food. Indian J. Med. Res. 134 (1), 22–25.
Jan, G., Belzacq, A.-S., Haouzi, D., Rouault, A., Métivier, D., Kroemer, G., et al. (2002). Propionibacteria induce apoptosis of colorectal carcinoma cells via short-chain fatty acids acting on mitochondria. Cell Death Differ. 9 (2), 179–188. doi:10.1038/sj/cdd/4400935
Jan, R., and Chaudhry, G. E. (2019). Understanding apoptosis and apoptotic pathways targeted cancer therapeutics. Adv. Pharm. Bull. 9 (2), 205–218. doi:10.15171/apb.2019.024
Ji, J., Jin, W., Liu, S. J., Jiao, Z., and Li, X. (2023). Probiotics, prebiotics, and postbiotics in health and disease. MedComm 4 (6), e420. doi:10.1002/mco2.420
Jiang, C., Wang, H., Xia, C., Dong, Q., Chen, E., Qiu, Y., et al. (2019). A randomized, double-blind, placebo-controlled trial of probiotics to reduce the severity of oral mucositis induced by chemoradiotherapy for patients with nasopharyngeal carcinoma. Cancer 125 (7), 1081–1090. doi:10.1002/cncr.31907
Joo, N. E., Ritchie, K., Kamarajan, P., Miao, D., and Kapila, Y. L. (2012). Nisin, an apoptogenic bacteriocin and food preservative, attenuates HNSCC tumorigenesis via CHAC1. Cancer Med. 1 (3), 295–305. doi:10.1002/cam4.35
Jung, M. Y., Kang, H. J., and Moon, A. (2001). Capsaicin-induced apoptosis in SK-Hep-1 hepatocarcinoma cells involves Bcl-2 downregulation and caspase-3 activation. Cancer Lett. 165 (2), 139–145. doi:10.1016/s0304-3835(01)00426-8
Jurášková, D., Ribeiro, S. C., and Silva, C. C. G. (2022). Exopolysaccharides produced by lactic acid bacteria: from biosynthesis to health-promoting properties. Foods Basel, Switz. 11 (2), 156. doi:10.3390/foods11020156
Kadirareddy, R. H., Vemuri, S. G., and Palempalli, U. M. (2016). Probiotic conjugated linoleic acid mediated apoptosis in breast cancer cells by downregulation of NFκB. Asian pac. J. cancer Prev. APJCP 17 (7), 3395–3403.
Kahouli, I., and Handiri, N. R. (2016). Characterization of L. Reuteri NCIMB 701359 probiotic features for potential use as a colorectal cancer biotherapeutic by identifying fatty acid profile and anti-proliferative action against colorectal cancer cells. Drug Des. Open Access 5 (2). doi:10.4172/2169-0138.1000131
Kahouli, I., Malhotra, M., Tomaro-Duchesneau, C., Sonia Rodes, L., Alaoui-Jamali, M. A., Prakash, S., et al. (2015). Identification of Lactobacillus Fermentum Strains with Potential against Colorectal Cancer by Characterizing Short Chain Fatty Acids Production, Anti-Proliferative Activity and Survival in an Intestinal Fluid: in vitro Analysis A Alaoui-Jamali, et al. Identification of Lactobacillus Fermentum Strains with Potential against Colorectal Cancer by Characterizing Short Chain Fatty Acids Production, Anti-Proliferative Activity and Survival in an Intestinal. J. Bioanal. and Biomed. 7 (4), 4. doi:10.4172/1948-593x.1000132ï
Kamarajan, P., Hayami, T., Matte, B., Liu, Y., Danciu, T., Ramamoorthy, A., et al. (2015). Nisin ZP, a bacteriocin and food preservative, inhibits head and neck cancer tumorigenesis and prolongs survival. PLoS ONE 10 (7), e0131008. doi:10.1371/journal.pone.0131008
Kapustian, A., Cherno, N., Kovalenko, A., Naumenko, K., and Kushnir, I. (2018). Products of metabolism and processing of lactic acid bacteria as functional ingredients. Food Sci. Appl. Biotechnol. 1, 47. doi:10.30721/fsab2018.v1.i1.13
Karimi Ardestani, S., Tafvizi, F., and Tajabadi Ebrahimi, M. (2019). Heat-killed probiotic bacteria induce apoptosis of HT-29 human colon adenocarcinoma cell line via the regulation of Bax/Bcl2 and caspases pathway. Hum. Exp. Toxicol. 38 (9), 1069–1081. doi:10.1177/0960327119851255
Karuppiah, P., Vignesh, V., and Ramasamy, T. (2014). Characterization and in vitro studies on anticancer activity of exopolymer of Bacillus thuringiensis S13. Afr. J. Biotechnol. 13 (21), 2137–2144. doi:10.5897/ajb2014.13741
Kataria, J., Li, N., Wynn, J. L., and Neu, J. (2009). Probiotic microbes: do they need to be alive to be beneficial? Nutr. Rev. 67 (9), 546–550. doi:10.1111/j.1753-4887.2009.00226.x
Kaur, B., Balgir, P. P., Mittu, B., Kumar, B., and Garg, N. (2013). Biomedical applications of fermenticin HV6b isolated from lactobacillus fermentum HV6b MTCC10770. Biomed. Res. Int. 2013, 168438. doi:10.1155/2013/168438
Kaur, S., and Kaur, S. (2015). Bacteriocins as potential anticancer agents. Front. Pharmacol. 6, 272. doi:10.3389/fphar.2015.00272
Kechagia, M., Basoulis, D., Konstantopoulou, S., Dimitriadi, D., Gyftopoulou, K., Skarmoutsou, N., et al. (2013). Health benefits of probiotics: a review. ISRN Nutr. 2013, 481651. doi:10.5402/2013/481651
Kerry, R. G., Patra, J. K., Gouda, S., Park, Y., Shin, H. S., and Das, G. (2018). Benefaction of probiotics for human health: a review. J. food drug Anal. 26 (3), 927–939. doi:10.1016/j.jfda.2018.01.002
Kessenbrock, K., Wang, C. Y., and Werb, Z. (2015). Matrix metalloproteinases in stem cell regulation and cancer. Matrix Biol. J. Int. Soc. Matrix Biol. 44-46, 184–190. doi:10.1016/j.matbio.2015.01.022
Khalil, M. A., Sonbol, F. I., Al-Madboly, L. A., Aboshady, T. A., Alqurashi, A. S., and Ali, S. S. (2022). Exploring the therapeutic potentials of exopolysaccharides derived from lactic acid bacteria and bifidobacteria: antioxidant, antitumor, and periodontal regeneration. Front. Microbiol. 13, 803688. doi:10.3389/fmicb.2022.803688
Kim, C. H., Park, J., and Kim, M. (2014). Gut microbiota-derived short-chain Fatty acids, T cells, and inflammation. Immune Netw. 14 (6), 277–288. doi:10.4110/in.2014.14.6.277
Kim, J. Y., Woo, H. J., Kim, Y.-S., and Lee, H. J. (2002). Screening for antiproliferative effects of cellular components from lactic acid bacteria against human cancer cell lines. Biotechnol. Lett. 24, 1431–1436. doi:10.1023/a:1019875204323
Kim, S., Kim, G. H., and Cho, H. (2021a). Postbiotics for cancer prevention and treatment. Microbiol. Soc. Korea 57 (3), 142–153.
Kim, S. J., Kang, C. H., Kim, G. H., and Cho, H. (2022). Anti-tumor effects of heat-killed L. Reuteri MG5346 and L. Casei MG4584 against human colorectal carcinoma through caspase-9-dependent apoptosis in xenograft model. Microorganisms 10 (3), 533. doi:10.3390/microorganisms10030533
Kim, Y., Lee, D., Kim, D., Cho, J., Yang, J., Chung, M., et al. (2008). Inhibition of proliferation in colon cancer cell lines and harmful enzyme activity of colon bacteria by Bifidobacterium adolescentis SPM0212. Arch. Pharmacal Res. 31 (4), 468–473. doi:10.1007/s12272-001-1180-y
Kim, Y., Oh, S., Yun, H. S., Oh, S., and Kim, S. H. (2010). Cell-bound exopolysaccharide from probiotic bacteria induces autophagic cell death of tumour cells. Lett. Appl. Microbiol. 51 (2), 123–130. doi:10.1111/j.1472-765X.2010.02859.x
Kim, Y. J., Yu, J., Park, S. P., Lee, S. H., and Kim, Y. S. (2021b). Prevention of radiotherapy induced enteropathy by probiotics (PREP): protocol for a double-blind randomized placebo-controlled trial. BMC Cancer 21 (1), 1032. doi:10.1186/s12885-021-08757-w
Kong, L., Hoshi, N., Sui, Y., Yamada, Y., Yoshida, R., Ooi, M., et al. (2022). GPR43 suppresses intestinal tumor growth by modification of the mammalian target of rapamycin complex 1 activity in ApcMin/+ mice. Medical principles and practice: international journal of the Kuwait university. Health Sci. Cent. 31 (1), 39–46. doi:10.1159/000518621
Kongsema, M., Wongkhieo, S., Khongkow, M., Lam, E. W. F., Boonnoy, P., Vongsangnak, W., et al. (2019). Molecular mechanism of Forkhead box M1 inhibition by thiostrepton in breast cancer cells. Oncol. Rep. 42 (3), 953–962. doi:10.3892/or.2019.7225
Kumar, B. (2012). In vitro cytotoxicity of native and rec-pediocin CP2 against cancer cell lines: a comparative study. Pharm. Anal. Acta 03 (08). doi:10.4172/2153-2435.1000183
Kumar, S. A. S., Krishnan, D., Jothipandiyan, S., Durai, R., Hari, B. N. V., and Nithyanand, P. (2024). Cell-free supernatants of probiotic consortia impede hyphal formation and disperse biofilms of vulvovaginal candidiasis causing Candida in an ex-vivo model. Ant. Leeuwenhoek 117 (1), 37. doi:10.1007/s10482-024-01929-1
Kwok, J. M. M., Myatt, S. S., Marson, C. M., Coombes, R. C., Constantinidou, D., and Lam, E. W. F. (2008). Thiostrepton selectively targets breast cancer cells through inhibition of forkhead box M1 expression. Mol. Cancer Ther. 7 (7), 2022–2032. doi:10.1158/1535-7163.MCT-08-0188
Lan, A., Lagadic-Gossmann, D., Lemaire, C., Brenner, C., and Jan, G. (2007). Acidic extracellular pH shifts colorectal cancer cell death from apoptosis to necrosis upon exposure to propionate and acetate, major end-products of the human probiotic propionibacteria. Apoptosis 12 (3), 573–591. doi:10.1007/s10495-006-0010-3
Latif, A., Shehzad, A., Niazi, S., Zahid, A., Ashraf, W., Iqbal, M. W., et al. (2023). Probiotics: mechanism of action, health benefits and their application in food industries. Front. Microbiol. 14, 1216674. doi:10.3389/fmicb.2023.1216674
Lee, Ji Y., Kim, Y. G., Kim, J.-I., Lee, H.-Y., Moon, G.-S., and Kang, C.-Ho (2022). Improvements in human Keratinocytes and antimicrobial effect mediated by cell-free supernatants derived from probiotics. Fermentation 8 (7), 332. doi:10.3390/fermentation8070332
Lee, J., Lee, J. E., Kim, S., Kang, D., and Yoo, H. M. (2020). Evaluating cell death using cell-free supernatant of probiotics in three-dimensional spheroid cultures of colorectal cancer cells. J. Vis. Exp. 2020 (160), 1–17. doi:10.3791/61285
Lee, N. K., Son, S. H., Jeon, E. B., Jung, G. H., Lee, J. Y., and Paik, H. D. (2015). The prophylactic effect of probiotic Bacillus polyfermenticus KU3 against cancer cells. J. Funct. Foods 14, 513–518. doi:10.1016/j.jff.2015.02.019
Legesse Bedada, T., Feto, T. K., Awoke, K. S., Garedew, A. D., Yifat, F. T., and Birri, D. J. (2020). Probiotics for cancer alternative prevention and treatment. Biomed. and Pharmacother. = Biomedecine and Pharmacother. 129, 110409. doi:10.1016/j.biopha.2020.110409
Lewies, A., Wentzel, J. F., Miller, H. C., and Du Plessis, L. H. (2018). The antimicrobial peptide nisin Z induces selective toxicity and apoptotic cell death in cultured melanoma cells. Biochimie 144, 28–40. doi:10.1016/j.biochi.2017.10.009
Li, W., Ji, J., Tang, W., Rui, X., Chen, X., Jiang, M., et al. (2014). Characterization of an antiproliferative exopolysaccharide (LHEPS-2) from Lactobacillus helveticus MB2-1. Carbohydr. Polym. 105 (1), 334–340. doi:10.1016/j.carbpol.2014.01.093
Lin, D. C. (2003). Probiotics as functional foods. Nutr. Clin. Pract. official Publ. Am. Soc. Parenter. Enter. Nutr. 18 (6), 497–506. doi:10.1177/0115426503018006497
Linn, Y. H., Thu, K. K., and Win, N. H. H. (2019). Effect of probiotics for the prevention of acute radiation-induced diarrhoea among cervical cancer patients: a randomized double-blind placebo-controlled study. Probiotics Antimicrob. Proteins 11 (2), 638–647. doi:10.1007/s12602-018-9408-9
Liu, C., Zheng, J., Ou, X., and Han, Y. (2021). Anti-cancer substances and safety of lactic acid bacteria in clinical treatment. Front. Microbiol. 12, 722052. doi:10.3389/fmicb.2021.722052
Liu, C. T., Chu, F. J., Chou, C. C., and Yu, R. C. (2011). Antiproliferative and anticytotoxic effects of cell fractions and exopolysaccharides from Lactobacillus casei 01. Mutat. Res. - Genet. Toxicol. Environ. Mutagen. 721 (2), 157–162. doi:10.1016/j.mrgentox.2011.01.005
Liu, X., Zhao, H., and Wong, A. (2024). Accounting for the health risk of probiotics. Heliyon 10 (6), e27908. doi:10.1016/j.heliyon.2024.e27908
Liu, J., Chen, X., Zhou, X., Yi, R., Yang, Z., and Zhao, X. (2021b). Lactobacillus fermentum ZS09 mediates epithelial– mesenchymal transition (EMT) by regulating the transcriptional activity of the Wnt/β-catenin Signalling pathway to inhibit colon cancer activity. J. Inflamm. Res. 14, 7281–7293. doi:10.2147/JIR.S344564
Liu, J., and Huang, X. E. (2014). Efficacy of Bifidobacterium tetragenous viable bacteria tablets for cancer patients with functional constipation. Asian pac. J. Cancer Prev. 15 (23), 10241–10244. doi:10.7314/APJCP.2014.15.23.10241
Lu, K., Dong, S., Wu, X., Jin, R., and Chen, H. (2021). Probiotics in cancer. Front. Oncol. 11, 638148. doi:10.3389/fonc.2021.638148
Ma, E. L., Choi, Y. J., Choi, J., Pothoulakis, C., Rhee, S. H., and Im, E. (2010). The anticancer effect of probiotic bacillus polyfermenticus on human colon cancer cells is mediated through ErbB2 and ErbB3 inhibition. Int. J. Cancer 127 (4), 780–790. doi:10.1002/ijc.25011
Ma, F., Song, Y., Sun, M., Wang, A., Jiang, S., Mu, G., et al. (2021). Exopolysaccharide produced by lactiplantibacillus plantarum-12 alleviates intestinal inflammation and colon cancer symptoms by modulating the gut microbiome and metabolites of C57BL/6 mice treated by azoxymethane/dextran sulfate sodium salt. Foods 10 (12), 3060. doi:10.3390/foods10123060
Madempudi, R. S., and Kalle, A. M. (2017). Antiproliferative effects of Bacillus coagulans Unique IS2 in colon cancer cells. Nutr. Cancer 69 (7), 1062–1068. doi:10.1080/01635581.2017.1359317
Maghsood, F., Johari, B., Rohani, M., Madanchi, H., Saltanatpour, Z., and Kadivar, M. (2020). Anti-proliferative and anti-metastatic potential of high molecular weight secretory molecules from probiotic lactobacillus reuteri cell-free supernatant against human colon cancer stem-like cells (HT29-ShE). Int. J. Pept. Res. Ther. 26 (4), 2619–2631. doi:10.1007/s10989-020-10049-z
Mahgoub, A. M., Mahmoud, M. G., Selim, M. S., and El Awady, M. E. (2018). Exopolysaccharide from marine Bacillus velezensis MHM3 induces apoptosis of human breast cancer MCF-7 cells through a mitochondrial pathway. Asian pac. J. Cancer Prev. 19 (7), 1957–1963. doi:10.22034/APJCP.2018.19.7.1957
Marco, M. L., Heeney, D., Binda, S., Cifelli, C. J., Cotter, P. D., Foligné, B., et al. (2017). Health benefits of fermented foods: microbiota and beyond. Curr. Opin. Biotechnol. 44, 94–102. doi:10.1016/j.copbio.2016.11.010
Markowiak, P., and Śliżewska, K. (2017). Effects of probiotics, prebiotics, and synbiotics on human health. Nutrients 9 (9), 1021. doi:10.3390/nu9091021
Mármol, I., Sánchez-de-Diego, C., Pradilla Dieste, A., Cerrada, E., and Rodriguez Yoldi, M. J. (2017). Colorectal carcinoma: a general overview and future perspectives in colorectal cancer. Int. J. Mol. Sci. 18 (1), 197. doi:10.3390/ijms18010197
Marteau, P., and Seksik, P. (2004). Tolerance of probiotics and prebiotics. J. Clin. Gastroenterol. 38 (6 Suppl. l), S67–S69. doi:10.1097/01.mcg.0000128929.37156.a7
Mater, D. D., Langella, P., Corthier, G., and Flores, M. J. (2008). A probiotic Lactobacillus strain can acquire vancomycin resistance during digestive transit in mice. J. Mol. Microbiol. Biotechnol. 14 (1-3), 123–127. doi:10.1159/000106091
Matsumoto, S., Hara, T., Nagaoka, M., Mike, A., Mitsuyama, K., Sako, T., et al. (2009). A component of polysaccharide peptidoglycan complex on Lactobacillus induced an improvement of murine model of inflammatory bowel disease and colitis-associated cancer. Immunology 128 (1 PART 2), e170–e180. doi:10.1111/j.1365-2567.2008.02942.x
Meenakshi, I. K. (2015). In-Vitro characterization of the anti-cancer activity of the probiotic bacterium lactobacillus fermentum NCIMB 5221 and potential against colorectal cancer. J. Cancer Sci. and Ther. 07 (07). doi:10.4172/1948-5956.1000354
Mehmandar-Oskuie, A., Tohidfar, M., Hajikhani, B., and Karimi, F. (2023). Anticancer effects of cell-free culture supernatant of Escherichia coli in bladder cancer cell line: new insight into the regulation of inflammation. Gene 889, 147795. doi:10.1016/j.gene.2023.147795
Miller, K. D., Siegel, R. L., Lin, C. C., Mariotto, A. B., Kramer, J. L., Rowland, J. H., et al. (2016). Cancer treatment and survivorship statistics, 2016. CA a cancer J. Clin. 66 (4), 271–289. doi:10.3322/caac.21349
Mirzaei, R., Afaghi, A., Babakhani, S., Sohrabi, M. R., Hosseini-Fard, S. R., Babolhavaeji, K., et al. (2021). Role of microbiota-derived short-chain fatty acids in cancer development and prevention. Biomed. and Pharmacother. = Biomedecine and Pharmacother. 139, 111619. doi:10.1016/j.biopha.2021.111619
Mohamed, S. S., Ibrahim, A. Y., Asker, M. S., Mahmoud, M. G., and El-Newary, S. A. (2021). Production, structural and biochemical characterization relevant to antitumor property of acidic exopolysaccharide produced from Bacillus sp. NRC5. Arch. Microbiol. 203 (7), 4337–4350. doi:10.1007/s00203-021-02422-3
Mojibi, P., Tafvizi, F., and Torbati, M. B. (2019). Cell-bound exopolysaccharide extract from indigenous probiotic bacteria induce apoptosis in HT-29 cell-line. Iran. J. Pathol. 14 (1), 41–51. doi:10.30699/IJP.14.1.41
Montalto, F. I., and De Amicis, F. (2020). Cyclin D1 in cancer: a molecular connection for cell cycle control, adhesion and invasion in tumor and stroma. Cells 9 (12), 2648. doi:10.3390/cells9122648
Mörkl, S., Butler, M. I., Holl, A., Cryan, J. F., and Dinan, T. G. (2020). Probiotics and the microbiota-gut-brain axis: focus on psychiatry. Curr. Nutr. Rep. 9 (3), 171–182. doi:10.1007/s13668-020-00313-5
Nakkarach, A., Foo, H. L., Song, A. A. L., Mutalib, N. E. A., Nitisinprasert, S., and Withayagiat, U. (2021). Anti-cancer and anti-inflammatory effects elicited by short chain fatty acids produced by Escherichia coli isolated from healthy human gut microbiota. Microb. Cell Factories 20 (1), 36. doi:10.1186/s12934-020-01477-z
Nami, Y., Haghshenas, B., Haghshenas, M., Abdullah, N., and Khosroushahi, A. Y. (2015). The Prophylactic effect of probiotic Enterococcus lactis IW5 against different human cancer cells. Front. Microbiol. 6 (NOV), 1317. doi:10.3389/fmicb.2015.01317
Natividad, J. M., and Verdu, E. F. (2013). Modulation of intestinal barrier by intestinal microbiota: pathological and therapeutic implications. Pharmacol. Res. 69 (1), 42–51. doi:10.1016/j.phrs.2012.10.007
Nazir, Y., Hussain, S. A., Abdul Hamid, A., and Song, Y. (2018). Probiotics and their potential preventive and therapeutic role for cancer, high serum cholesterol, and allergic and HIV diseases. Biomed. Res. Int. 2018, 3428437. doi:10.1155/2018/3428437
Nguyen, M. R., Ma, E., Wyatt, D., Knight, K. L., and Osipo, C. (2023). The effect of an exopolysaccharide probiotic molecule from Bacillus subtilis on breast cancer cells. Front. Oncol. 13, 1292635. doi:10.3389/fonc.2023.1292635
Norouzi, Z., Salimi, A., Halabian, R., and Fahimi, H. (2018). Nisin, a potent bacteriocin and anti-bacterial peptide, attenuates expression of metastatic genes in colorectal cancer cell lines. Microb. Pathog. 123, 183–189. doi:10.1016/j.micpath.2018.07.006
Nowak, A., Zakłos-Szyda, M., Rosicka-Kaczmarek, J., and Motyl, I. (2022). Anticancer potential of post-fermentation media and cell extracts of probiotic strains: an in vitro study. Cancers 14 (7), 1853. doi:10.3390/cancers14071853
Oh, B. S., Choi, W. J., Kim, J. S., Ryu, S. W., Yu, S. Y., Lee, J. S., et al. (2021). Cell-free supernatant of odoribacter splanchnicus isolated from human feces exhibits anti-colorectal cancer activity. Front. Microbiol. 12, 736343. doi:10.3389/fmicb.2021.736343
Ohkawara, S., Furuya, H., Nagashima, K., Asanuma, N., and Hino, T. (2005) Nutrition and cancer oral administration of butyrivibrio fibrisolvens, a butyrate-producing bacterium, decreases the formation of aberrant crypt foci in the colon and rectum of mice, 1, 2. Available at: www.nutrition.org.
Österlund, P., Ruotsalainen, T., Korpela, R., Saxelin, M., Ollus, A., Valta, P., et al. (2007). Lactobacillus supplementation for diarrhoea related to chemotherapy of colorectal cancer: a randomised study. Br. J. Cancer 97 (8), 1028–1034. doi:10.1038/sj.bjc.6603990
Ozaki, T., and Nakagawara, A. (2011). Role of p53 in cell death and human cancers. Cancers 3 (1), 994–1013. doi:10.3390/cancers3010994
Pahumunto, N., and Teanpaisan, R. (2023). Anti-cancer properties of potential probiotics and their cell-free supernatants for the prevention of colorectal cancer: an in vitro study. Probiotics Antimicrob. Proteins 15 (5), 1137–1150. doi:10.1007/s12602-022-09972-y
Pakbin, B., Allahyari, S., Dibazar, S. P., Zolghadr, L., Chermahini, N. K., Brück, W. M., et al. (2023). Effects of probiotic Saccharomyces boulardii supernatant on viability, nano-mechanical properties of cytoplasmic membrane and pro-inflammatory gene expression in human gastric cancer AGS cells. Int. J. Mol. Sci. 24 (9), 7945. doi:10.3390/ijms24097945
Palla, M., Conte, G., Grassi, A., Esin, S., Serra, A., Mele, M., et al. (2021). Novel yeasts producing high levels of conjugated linoleic acid and organic acids in fermented doughs. Foods Basel, Switz. 10 (9), 2087. doi:10.3390/foods10092087
Park, J., Kwon, M., Lee, J., Park, S., Seo, J., and Roh, S. (2020). Anti-cancer effects of lactobacillus plantarum l-14 cell-free extract on human malignant melanoma A375 cells. Molecules 25 (17), 3895. doi:10.3390/molecules25173895
Peña, A. S. (2007). Intestinal flora, probiotics, prebiotics, symbiotics and novel foods. Rev. espanola enfermedades Dig. 99 (11), 653–658. doi:10.4321/s1130-01082007001100006
Peter, M. E., Hadji, A., Murmann, A. E., Brockway, S., Putzbach, W., Pattanayak, A., et al. (2015). The role of CD95 and CD95 ligand in cancer. Cell death Differ. 22 (4), 549–559. doi:10.1038/cdd.2015.3
Pokusaeva, K., Fitzgerald, G. F., and van Sinderen, D. (2011). Carbohydrate metabolism in bifidobacteria. Genes and Nutr. 6 (3), 285–306. doi:10.1007/s12263-010-0206-6
Preet, S., Bharati, S., Panjeta, A., Tewari, R., and Rishi, P. (2015). Effect of nisin and doxorubicin on DMBA-induced skin carcinogenesis—a possible adjunct therapy. Tumor Biol. 36 (11), 8301–8308. doi:10.1007/s13277-015-3571-3
Prince, A., Tiwari, A., Ror, P., Sandhu, P., Roy, J., Jha, S., et al. (2019). Attenuation of neuroblastoma cell growth by nisin is mediated by modulation of phase behavior and enhanced cell membrane fluidity. Phys. Chem. Chem. Phys. 21 (4), 1980–1987. doi:10.1039/c8cp06378h
Raguz, S., and Yagüe, E. (2008). Resistance to chemotherapy: new treatments and novel insights into an old problem. Br. J. cancer 99 (3), 387–391. doi:10.1038/sj.bjc.6604510
Rastall, R. A., and Gibson, G. R. (2015). Recent developments in prebiotics to selectively impact beneficial microbes and promote intestinal health. Curr. Opin. Biotechnol. 32, 42–46. doi:10.1016/j.copbio.2014.11.002
Renzis, D., Famularo, G., Famularo, G., Frosina, P., Messina, G., De Renzis, C., et al. (2007). Use of probiotics for prevention of radiation-induced diarrhea. World J. Gastroenterol. 13 (6), 912–915. doi:10.3748/wjg.v13.i6.912
Riaz Rajoka, M. S., Mehwish, H. M., Fang, H., Padhiar, A. A., Zeng, X., Khurshid, M., et al. (2019). Characterization and anti-tumor activity of exopolysaccharide produced by Lactobacillus kefiri isolated from Chinese kefir grains. J. Funct. Foods 63, 103588. doi:10.1016/j.jff.2019.103588
Riaz Rajoka, M. S., Zhao, H., Lu, Y., Lian, Z., Li, N., Hussain, N., et al. (2018). Anticancer potential against cervix cancer (HeLa) cell line of probiotic: lactobacillus casei and Lactobacillus paracasei strains isolated from human breast milk. Food Funct. 9 (5), 2705–2715. doi:10.1039/c8fo00547h
Riedl, S., Rinner, B., Asslaber, M., Schaider, H., Walzer, S., Novak, A., et al. (2011). In search of a novel target - phosphatidylserine exposed by non-apoptotic tumor cells and metastases of malignancies with poor treatment efficacy. Biochim. Biophys. acta 1808 (11), 2638–2645. doi:10.1016/j.bbamem.2011.07.026
Rodriguez-Arrastia, M., Martinez-Ortigosa, A., Rueda-Ruzafa, L., Folch Ayora, A., and Ropero-Padilla, C. (2021). Probiotic supplements on Oncology patients' treatment-related side effects: a Systematic review of randomized controlled trials. Int. J. Environ. Res. public health 18 (8), 4265. doi:10.3390/ijerph18084265
Rosberg-Cody, E., Johnson, M. C., Fitzgerald, G. F., Ross, P. R., and Stanton, C. (2007). Heterologous expression of linoleic acid isomerase from Propionibacterium acnes and anti-proliferative activity of recombinant trans-10, cis-12 conjugated linoleic acid. Microbiology 153 (8), 2483–2490. doi:10.1099/mic.0.2006/001966-0
Salek, F., Mirzaei, H., Khandaghi, J., Javadi, A., and Nami, Y. (2023). Apoptosis induction in cancer cell lines and anti-inflammatory and anti-pathogenic properties of proteinaceous metabolites secreted from potential probiotic Enterococcus faecalis KUMS-T48. Sci. Rep. 13 (1), 7813. doi:10.1038/s41598-023-34894-2
Salemi, R., Vivarelli, S., Ricci, D., Scillato, M., Santagati, M., Gattuso, G., et al. (2023). Lactobacillus rhamnosus GG cell-free supernatant as a novel anti-cancer adjuvant. J. Transl. Med. 21 (1), 195. doi:10.1186/s12967-023-123904036-3
Salminen, S., Collado, M. C., Endo, A., Hill, C., Lebeer, S., Quigley, E. M. M., et al. (2021). The International Scientific Association of Probiotics and Prebiotics (ISAPP) consensus statement on the definition and scope of postbiotics. Nat. Rev. Gastroenterology and hepatology 18 (9), 649–667. doi:10.1038/s41575-021-00440-6
Sankarapandian, V., Venmathi Maran, B. A., Rajendran, R. L., Jogalekar, M. P., Gurunagarajan, S., Krishnamoorthy, R., et al. (2022). An update on the effectiveness of probiotics in the prevention and treatment of cancer. Life Basel, Switz. 12 (1), 59. doi:10.3390/life12010059
Sathishkumar, K., Chaturvedi, M., Das, P., Stephen, S., and Mathur, P. (2022). Cancer incidence estimates for 2022 and projection for 2025: result from national cancer registry programme, India. Indian J. Med. Res. 156 (4and5), 598–607. doi:10.4103/ijmr.ijmr_1821_22
Saxami, G., Karapetsas, A., Lamprianidou, E., Kotsianidis, I., Chlichlia, A., Tassou, C., et al. (2016). Two potential probiotic lactobacillus strains isolated from olive microbiota exhibit adhesion and anti-proliferative effects in cancer cell lines. J. Funct. Foods 24, 461–471. doi:10.1016/j.jff.2016.04.036
Schwartz, G. K., and Shah, M. A. (2005). Targeting the cell cycle: a new approach to cancer therapy. J. Clin. Oncol. Off. J. Am. Soc. Clin. Oncol. 23 (36), 9408–9421. doi:10.1200/JCO.2005.01.5594
shao, F., Xin, F., Yang, C., Yang, D., Mi, Y., Yu, J., et al. (2014). The impact of microbial immune enteral nutrition on the patients with acute radiation enteritis in bowel function and immune status. Cell biochem. Biophys. 69 (2), 357–361. doi:10.1007/s12013-013-9807-1
Sharma, A., Rath, G. K., Chaudhary, S. P., Thakar, A., Mohanti, B. K., and Bahadur, S. (2012). Lactobacillus brevis CD2 lozenges reduce radiation- and chemotherapy-induced mucositis in patients with head and neck cancer: a randomized double-blind placebo-controlled study. Eur. J. Cancer 48 (6), 875–881. doi:10.1016/j.ejca.2011.06.010
Sharma, A., Tilak, T., Bakhshi, S., Raina, V., Kumar, L., Chaudhary, S. P., et al. (2016). Lactobacillus brevis CD2 lozenges prevent oral mucositis in patients undergoing high dose chemotherapy followed by haematopoietic stem cell transplantation. ESMO Open 1 (6), e000138. doi:10.1136/esmoopen-2016-000138
Sharma, P., Kaur, S., Chadha, B. S., Kaur, R., Kaur, M., and Kaur, S. (2021). Anticancer and antimicrobial potential of enterocin 12a from Enterococcus faecium. BMC Microbiol. 21 (1), 39. doi:10.1186/s12866-021-02086-5
Sheng, S., Fu, Y., Pan, N., Zhang, H., Xiu, L., Liang, Y., et al. (2022). Novel exopolysaccharide derived from probiotic Lactobacillus pantheris TCP102 strain with immune-enhancing and anticancer activities. Front. Microbiol. 13, 1015270. doi:10.3389/fmicb.2022.1015270
Shi, Y., Zhang, C., Cao, W., Li, L., Liu, K., Zhu, H., et al. (2024). Extracellular vesicles from Lacticaseibacillus paracasei PC-H1 inhibit HIF-1α-mediated glycolysis of colon cancer. Future Microbiol. 19, 227–239. doi:10.2217/fmb-2023-0144
Singh, P., and Saini, P. (2017). Food and health potentials of exopolysaccharides derived from lactobacilli. Microbiol. Res. J. Int. 22, 1–14. doi:10.9734/MRJI/2017/36935
Slavin, J. (2013). Fiber and prebiotics: mechanisms and health benefits. Nutrients 5 (4), 1417–1435. doi:10.3390/nu5041417
Sun, N., Liu, H., Liu, S., Zhang, X., Chen, P., Li, W., et al. (2018). Purification, preliminary structure and antitumor activity of exopolysaccharide produced by Streptococcus thermophilus CH9. Molecules 23 (11), 2898. doi:10.3390/molecules23112898
Sung, H., Ferlay, J., Siegel, R. L., Laversanne, M., Soerjomataram, I., Jemal, A., et al. (2021). Global cancer statistics 2020: GLOBOCAN estimates of incidence and mortality worldwide for 36 cancers in 185 countries. CA a cancer J. Clin. 71 (3), 209–249. doi:10.3322/caac.21660
Sungur, T., Aslim, B., Karaaslan, C., and Aktas, B. (2017). Impact of Exopolysaccharides (EPSs) of Lactobacillus gasseri strains isolated from human vagina on cervical tumor cells (HeLa). Anaerobe 47, 137–144. doi:10.1016/j.anaerobe.2017.05.013
Swanson, K. S., Gibson, G. R., Hutkins, R., Reimer, R. A., Reid, G., Verbeke, K., et al. (2020). The international scientific association for probiotics and prebiotics (ISAPP) consensus statement on the definition and scope of synbiotics. Nat. Rev. Gastroenterol. Hepatol. 17 (11), 687–701. doi:10.1038/s41575-020-0344-2
Tan, J., McKenzie, C., Potamitis, M., Thorburn, A. N., Mackay, C. R., and Macia, L. (2014). The role of short-chain fatty acids in health and disease. Adv. Immunol. 121, 91–119. doi:10.1016/B978-0-12-800100-4.00003-9
Taverniti, V., and Guglielmetti, S. (2011). The immunomodulatory properties of probiotic microorganisms beyond their viability (ghost probiotics: proposal of paraprobiotic concept). Genes. Nutr. 6 (3), 261–274. doi:10.1007/s12263-011-0218-x
Tegopoulos, K., Stergiou, O. S., Kiousi, D. E., Tsifintaris, M., Koletsou, E., Papageorgiou, A. C., et al. (2021). Genomic and phylogenetic analysis of lactiplantibacillus plantarum L125, and evaluation of its anti-proliferative and cytotoxic activity in cancer cells. Biomedicines 9 (11), 1718. doi:10.3390/biomedicines9111718
Thananimit, S., Pahumunto, N., and Teanpaisan, R. (2022). Characterization of short chain fatty acids produced by selected potential probiotic lactobacillus strains. Biomolecules 12 (12), 1829. doi:10.3390/biom12121829
Thirabunyanon, M., and Hongwittayakorn, P. (2013). Potential probiotic lactic acid bacteria of human origin induce antiproliferation of colon cancer cells via synergic actions in adhesion to cancer cells and short-chain fatty acid bioproduction. Appl. Biochem. Biotechnol. 169 (2), 511–525. doi:10.1007/s12010-012-9995-y
Thursby, E., and Juge, N. (2017). Introduction to the human gut microbiota. Biochem. J. 474 (11), 1823–1836. doi:10.1042/BCJ20160510
Tian, P. J., Li, B. L., Shan, Y. J., Zhang, J. N., Chen, J. Y., Yu, M., et al. (2015). Extraction of peptidoglycan from L. Paracasei subp. Paracasei X12 and its preliminary mechanisms of inducing Immunogenic cell death in HT-29 cells. Int. J. Mol. Sci. 16 (8), 20033–20049. doi:10.3390/ijms160820033
Tiptiri-Kourpeti, A., Spyridopoulou, K., Santarmaki, V., Aindelis, G., Tompoulidou, E., Lamprianidou, E. E., et al. (2016). Lactobacillus casei exerts anti-proliferative effects accompanied by apoptotic cell death and up-regulation of TRAIL in colon carcinoma cells. PLoS ONE 11 (2), e0147960. doi:10.1371/journal.pone.0147960
Tripathy, A., Dash, J., Kancharla, S., Kolli, P., Mahajan, D., Senapati, S., et al. (2021). Probiotics: a promising candidate for management of colorectal cancer. Cancers 13 (13), 3178. doi:10.3390/cancers13133178
Tsvetikova, S. A., and Koshel, E. I. (2020). Microbiota and cancer: host cellular mechanisms activated by gut microbial metabolites. Int. J. Med. Microbiol. 310 (4), 151425. doi:10.1016/j.ijmm.2020.151425
Tukenmez, U., Aktas, B., Aslim, B., and Yavuz, S. (2019). The relationship between the structural characteristics of lactobacilli-EPS and its ability to induce apoptosis in colon cancer cells in vitro. Sci. Rep. 9 (1), 8268. doi:10.1038/s41598-019-44753-8
Upadhyay, A. (2020). Cancer: an unknown territory; rethinking before going ahead. Genes Dis. 8 (5), 655–661. doi:10.1016/j.gendis.2020.09.002
Urbancsek, H., Kazar, T., Mezes, I., and Neumann, K. (2001). Results of a double-blind, randomized study to evaluate the efficacy and safety of antibiophilus in patients with radiation-induced diarrhoea. Eur. J. Gastroenterology and Hepatology 13, 391–396. doi:10.1097/00042737-200104000-00015
Vallino, L., Garavaglia, B., Visciglia, A., Amoruso, A., Pane, M., Ferraresi, A., et al. (2023). Cell-free lactiplantibacillus plantarum OC01 supernatant suppresses IL-6-induced proliferation and invasion of human colorectal cancer cells: effect on β-Catenin degradation and induction of autophagy. J. Tradit. Complement. Med. 13 (2), 193–206. doi:10.1016/j.jtcme.2023.02.001
Villarante, K. I., Elegado, F. B., Iwatani, S., Zendo, T., Sonomoto, K., and de Guzman, E. E. (2011). Purification, characterization and in vitro cytotoxicity of the bacteriocin from Pediococcus acidilactici K2a2-3 against human colon adenocarcinoma (HT29) and human cervical carcinoma (HeLa) cells. World J. Microbiol. Biotechnol. 27 (4), 975–980. doi:10.1007/s11274-010-0541-1
Vinderola, G., Sanders, M. E., Salminen, S., and Szajewska, H. (2022). Postbiotics: the concept and their use in healthy populations. Front. Nutr. 9, 1002213. doi:10.3389/fnut.2022.1002213
Vrzáčková, N., Ruml, T., and Zelenka, J. (2021). Postbiotics, metabolic signaling, and cancer. Mol. Basel, Switz. 26 (6), 1528. doi:10.3390/molecules26061528
Wan, Y., Xin, Y., Zhang, C., Wu, D., Ding, D., Tang, L., et al. (2014). Fermentation supernatants of lactobacillus delbrueckii inhibit growth of human colon cancer cells and induce apoptosis through a caspase 3-dependent pathway. Oncol. Lett. 7 (5), 1738–1742. doi:10.3892/ol.2014.1959
Wang, H., Jin, J., Pang, X., Bian, Z., Zhu, J., Hao, Y., et al. (2023). Plantaricin BM-1 decreases viability of SW480 human colorectal cancer cells by inducing caspase-dependent apoptosis. Front. Microbiol. 13, 1103600. doi:10.3389/fmicb.2022.1103600
Wang, K., Li, W., Rui, X., Chen, X., Jiang, M., and Dong, M. (2014). Characterization of a novel exopolysaccharide with antitumor activity from Lactobacillus plantarum 70810. Int. J. Biol. Macromol. 63, 133–139. doi:10.1016/j.ijbiomac.2013.10.036
Wang, L., Wang, Y., Li, Q., Tian, K., Xu, L., Liu, G., et al. (2019). Exopolysaccharide, isolated from a novel strain bifidobacterium breve lw01 possess an anticancer effect on head and neck cancer - genetic and biochemical evidences. Front. Microbiol. 10 (MAY), 1044. doi:10.3389/fmicb.2019.01044
Wang, S., Han, X., Zhang, L., Zhang, Y., Li, H., and Jiao, Y. (2018). Whole peptidoglycan extracts from the lactobacillus paracasei subsp. paracasei m5 strain exert anticancer activity in vitro. Biomed. Res. Int. 2018, 2871710. doi:10.1155/2018/2871710
Wang, Y., Wang, Y., Sun, T., and Xu, J. (2024). Bacteriocins in cancer treatment: mechanisms and clinical potentials. Biomolecules 14 (7), 831. doi:10.3390/biom14070831
Wang, Y. C., Ku, W. C., Liu, C. Y., Cheng, Y. C., Chien, C. C., Chang, K. W., et al. (2021). Supplementation of probiotic Butyricicoccus pullicaecorum mediates anticancer effect on bladder urothelial cells by regulating butyrate-responsive molecular signatures. Diagnostics 11 (12), 2270. doi:10.3390/diagnostics11122270
Wegh, C. A. M., Geerlings, S. Y., Knol, J., Roeselers, G., and Belzer, C. (2019). Postbiotics and their potential applications in early life nutrition and beyond. Int. J. Mol. Sci. 20 (19), 4673. doi:10.3390/ijms20194673
Wei, Y., Li, F., Li, L., Huang, L., and Li, Q. (2019). Genetic and biochemical characterization of an exopolysaccharide with in vitro Antitumoral activity produced by lactobacillus fermentum YL-11. Front. Microbiol. 10, 2898. doi:10.3389/fmicb.2019.02898
Welman, A. D., and Maddox, I. S. (2003). Exopolysaccharides from lactic acid bacteria: perspectives and challenges. Trends Biotechnol. 21 (6), 269–274. doi:10.1016/S0167-7799(03)00107-0
Xia, L., Tan, S., Zhou, Y., Lin, J., Wang, H., Oyang, L., et al. (2018). Role of the NFκB-signaling pathway in cancer. OncoTargets Ther. 11, 2063–2073. doi:10.2147/OTT.S161109
Xu, Y., Yan, X., Zheng, H., Li, J., Wu, X., Xu, J., et al. (2024). The application of encapsulation technology in the food Industry: classifications, recent Advances, and perspectives. Food Chem. X 21, 101240. doi:10.1016/j.fochx.2024.101240
Yadav, M. K., Kumari, I., Singh, B., Sharma, K. K., and Tiwari, S. K. (2022). Probiotics, prebiotics and synbiotics: safe options for next-generation therapeutics. Appl. Microbiol. Biotechnol. 106 (2), 505–521. doi:10.1007/s00253-021-11646-8
Yamasaki-Yashiki, S., Kawashima, F., Saika, A., Hosomi, R., Kunisawa, J., and Katakura, Y. (2024). RNA-based anti-inflammatory effects of membrane vesicles derived from lactiplantibacillus plantarum. Foods 13 (6), 967. doi:10.3390/foods13060967
Yang, B., Huang, X., Li, W., Mouli, S., Lewandowski, R., and Larson, A. (2021). Duramycin radiosensitization of MCA-RH 7777 hepatoma cells through the elevation of reactive oxygen species. J. Cancer Res. Ther. 17 (2), 543–546. doi:10.4103/jcrt.JCRT_284_18
Yang, M., Jang, I. T., Kim, H. J., and Park, J. K. (2016). Bacillus spp. or Bacillus spp.-derived membrane vesicles induce the intrinsic pathways of apoptosis of human colon cancer cell lines. J. Bacteriol. Virol. 46 (2), 84–92. doi:10.4167/jbv.2016.46.2.84
Yue, Y. C., Yang, B. Y., Lu, J., Zhang, S. W., Liu, L., Nassar, K., et al. (2020). Metabolite secretions of Lactobacillus plantarum YYC-3 may inhibit colon cancer cell metastasis by suppressing the VEGF-MMP2/9 signaling pathway. Microb. Cell Factories 19 (1), 213. doi:10.1186/s12934-020-01466-2
Zacharof, M.-P., and Lovitt, R. B. (2012). Bacteriocins produced by lactic acid bacteria A review article. APCBEE Procedia 2, 50–56. doi:10.1016/j.apcbee.2012.06.010
Zaharuddin, L., Mokhtar, N. M., Muhammad Nawawi, K. N., and Raja Ali, R. A. (2019). A randomized double-blind placebo-controlled trial of probiotics in post-surgical colorectal cancer. BMC Gastroenterol. 19 (1), 131. doi:10.1186/s12876-019-1047-4
Zainodini, N., Hassanshahi, G., Hajizadeh, M., Falahati-Pour, S. K., Mahmoodi, M., and Mirzaei, M. R. (2018). Nisin induces cytotoxicity and apoptosis in human asterocytoma cell line (SW1088). Asian pac. J. Cancer Prev. 19 (8), 2217–2222. doi:10.22034/APJCP.2018.19.8.2217
Zhang, R., Zhou, Z., Ma, Y., Du, K., Sun, M., Zhang, H., et al. (2023). Exopolysaccharide from lactiplantibacillus plantarum YT013 and its apoptotic activity on gastric cancer AGS cells. Fermentation 9 (6), 539. doi:10.3390/fermentation9060539
Zhou, X., Hong, T., Yu, Q., Nie, S., Gong, D., Xiong, T., et al. (2017). Exopolysaccharides from Lactobacillus plantarum NCU116 induce c-Jun dependent Fas/Fasl-mediated apoptosis via TLR2 in mouse intestinal epithelial cancer cells. Sci. Rep. 7 (1), 14247. doi:10.1038/s41598-017-14178-2
Zhou, Y., Cui, Y., and Qu, X. (2019). Exopolysaccharides of lactic acid bacteria: structure, bioactivity and associations: a review. Carbohydr. Polym. 207, 317–332. doi:10.1016/j.carbpol.2018.11.093
Keywords: cancer, probiotics, postbiotics, gut microbiota, cell-free supernatants, bacteriocins, conjugated linoleic acid, exopolysaccharides ∗ NA-not approved
Citation: Sudaarsan ASK and Ghosh AR (2024) Appraisal of postbiotics in cancer therapy. Front. Pharmacol. 15:1436021. doi: 10.3389/fphar.2024.1436021
Received: 21 May 2024; Accepted: 05 September 2024;
Published: 20 September 2024.
Edited by:
Pourya Gholizadeh, Ardabil University of Medical Sciences, IranReviewed by:
Mohsen Arzanlou, Ardabil University of Medical Sciences, IranMaría Chávarri, Tecnalia Research and Innovation, Spain
Copyright © 2024 Sudaarsan and Ghosh. This is an open-access article distributed under the terms of the Creative Commons Attribution License (CC BY). The use, distribution or reproduction in other forums is permitted, provided the original author(s) and the copyright owner(s) are credited and that the original publication in this journal is cited, in accordance with accepted academic practice. No use, distribution or reproduction is permitted which does not comply with these terms.
*Correspondence: Asit Ranjan Ghosh, YXNpdHJhbmphbmdob3NoQHZpdC5hYy5pbg==
†ORCID: Aruna Senthil Kumar Sudaarsan, orcid.org/0009-0004-7384-9096; Asit Ranjan Ghosh, orcid.org/0000-0002-7922-3308