- 1Department of Food Science and Engineering, Moutai Institute, Renhuai, China
- 2School of Food Science and Technology, Jiangnan University, Wuxi, China
Traditional Tibetan medicine (TTM) is an ancient healing system that has been practiced for more than 2,000 years and involves the use of various medicinal plants for preventing and treating acute mountain sickness, depression, asthma, etc. Geo-authentic medicinal materials, also known as “Daodi herbs” in Chinese, have heightened efficacy and quality relative to their counterparts sourced from alternative geographic locales. In 2024, eight medicinal materials, typified by Cordyceps sinensis Sacc., were listed as geo-authentic Tibetan medicine under the administration of the local government. However, there is no comprehensive review on these geo-authentic TTMs, especially with respect to their pharmacological benefits to human health. This review aims to document the pharmacological properties, phytochemical components, safety, toxicity, and future developments of the geo-authentic TTMs that play essential roles in promoting health and wellness. Plant-derived molecules (i.e., polysaccharides, flavonoids, glycosides, terpenoids, and alkaloids) in the TTMs show therapeutic potentials for the management of both mental and physical health. Finally, the applications and prospects of TTM plants are discussed to support the use of these species in folk medicine for human wellness and to promote public health in modern societies.
1 Introduction
Geo-authentic medicinal materials, known as “Daodi herbs” in Chinese, are often selected based on extensive use in Chinese medicine clinics; they are cultivated in specific geographic regions and are known for their superior quality, efficacy, and reputation compared to herbs of the same species grown elsewhere (Zhao et al., 2012). This division is based on the natural traits of a topography and geomorphology as well as the essence of the national medical system; this has resulted in the establishment of 15 production regions for geo-authentic medicinal materials in China (Figure 1). The diversity of the biological and medicinal cultures of traditional Tibetan medicines (TTMs) in the Qinghai–Tibet Plateau is amazing; the medicinal materials have been widely used in conventional medicine for thousands of years to treat conditions such as acute mountain sickness, depression, and asthma, among others. Following the prescribed protocols and selection criteria, the Tibetan Pharmaceutical Association has directed its attention toward Tibetan-produced herbs, culminating in the release of the most recent list of eight geo-authentic TTMs in 2024: Cordyceps sinensis Sacc., Rhodiola rosea L., Saussurea involucrate, Onosma hookeri, Dracocephalum tanguticum M., Carum carvi L., Gymnadenia conopsea L., and Brassica rapa L.
The bioactive components in these plants have been studied extensively for their pharmacological effects, and significant advances have been made in this field. Some of these medicinal plants, like R. rosea L., B. rapa L., and S. involucrate, grow at high altitudes. Most of the aforementioned species are famous for their pharmacological potentials to promote health in traditional culinary and medical practices (Chen, 2023). These medicinal plants have been listed as the top-grade drugs in literature on Tibetan medicine and have been used for tonifying qi and deficiencies for over 3,800 years (Hao et al., 2018). Owing to the renewed interest in the reapplication of traditional medicinal plants in recent times, there have been new investigations on the use of natural plants in anti-hypoxia and anti-fatigue treatments (Zhou and Jiang, 2019). Given the similarities between fatigue and hypoxia (i.e., weakness, bradykinesia, and depression), potential interactions have been proposed and are still being actively debated (Degens et al., 2006; Huang et al., 2022).
The therapeutic potentials of Tibetan medicinal plants have led to promising results in managing hypoxia, fatigue, and related symptoms (Hua et al., 2021b; Zhu et al., 2021; Zhu et al., 2022a). However, based on current understanding, there is a paucity of scholarly literature offering a comprehensive overview of the eight newly acknowledged geo-authentic Tibetan medicinal materials for the treatment of hypoxia, fatigue, and related symptoms. This review seeks to analyze a selection of these geo-authentic medicinal materials as well as several other high-altitude species commonly utilized in TTMs for their efficacy in treating common symptoms such as hypoxia and fatigue (Li et al., 2018a; Zhao et al., 2021b), in addition to conditions like depression, asthma, and acute mountain sickness (Ma et al., 2011). Finally, we list the opportunities for and challenges of harnessing the geo-authentic traditional medicinal plants.
2 Pharmacological properties of the geo-authentic Tibetan medicinal plants
2.1 Cordyceps sinensis Sacc.
C. sinensis Sacc. (3,500–4,500 m) is a type of fungus that is widely used in traditional Chinese medicine (TCM) for its medicinal properties; studies have shown that it can improve oxygen utilization and reduce oxidative stress, which are beneficial for individuals suffering from hypoxia- and fatigue-related disorders (Long et al., 2021b). Cordycepin, which is a nucleoside derivative obtained from C. sinensis Sacc. (Figure 2A), has been verified to possess a diverse range of biological activities, including liver protection, antioxidation, immunomodulation, and anti-inflammation (Ashraf et al., 2020). The traditional use of this medicine to improve the body’s hypoxia tolerance and fatigue resistance was recorded as early as the Qing dynasty (1757 AD) (Long et al., 2021a). Additionally, C. sinensis has been found to have positive effects on energy metabolism and physical performance, which may also be helpful for individuals experiencing fatigue (Table 1).
2.2 Rhodiola rosea L.
R. rosea L. is considered as the king of Tibetan medicine and grows at an altitude of 1,800–4,000 m; it has been traditionally used in eastern and northern Europe, Asia, and North America (Cui et al., 2014), while the Qinghai–Tibet Plateau is generally considered as the center of its origin and diversity (Brinckmann et al., 2021). The Rhodiola species has been extensively used in tonics since time immemorial for its medicinal qualities in traditional folk medicines in China, Tibet, and Mongolia (Cunningham et al., 2020). R. rosea L. promotes physical/cognitive vitality as well as recovery from prolonged and minor physical exhaustion (Khanum et al., 2005; Angeli et al., 2018). In addition to the treatment of hypoxia and fatigue, it is applied to treat associated symptoms, such as poor appetite, sleep difficulties, irritability, hypertension, and headaches (Kelly, 2001).
Glycosides are unique secondary metabolites that display rich effects against human diseases and metabolic disorders. The anti-hypoxia and anti-fatigue properties of Rhodiola may be related to salidroside (Figure 2B) and flavones. R. rosea L. extracts (600 mg/day) containing salidroside (C14H20O7, 2.62%) have been shown to significantly increased exercise capacity and ameliorate exhaustive exercise-induced stress damage. Hou et al. (2020) reported that Rhodiola (salidroside, 1.27 mg/mL) ameliorated fatigue via suppression of mitophagy in the skeletal muscles during exhaustive exercise. The protective effects of salidroside against CoCl2-simulated hypoxia injury in PC12 cells have also been reported (Tang et al., 2021).
2.3 Saussurea involucrata
S. involucrata (Kar. et Kir.) is a well-known traditional medicinal plant that grows in high mountains (elevation over 4,000 m) covered by snow (Li et al., 2017a). It grows under harsh climatic conditions and takes more than 8 years to mature before harvesting. Owing to its great pharmacological activities, slow growth, and high demand, S. involucrata is rare and important in various ethnomedical systems, including TCM, Uyghur, Mongolian, and Kazakhstan medicine (Ma et al., 2014; Han et al., 2016). Since the 1990s, there is increasing attention on the anti-hypoxia and anti-fatigue properties of S. involucrata, which can significantly strengthen and invigorate the body, partly owing to its antioxidant capacity (Zheng et al., 1993). Petroleum ether extracts of S. involucrata were found to exhibit high neuroprotective effects and reverse damage to the brain caused by acute mountain sickness (Ma et al., 2014; Jing et al., 2016). Both tissue cultures (Jia and Wu, 2008) and ethanolic extracts (Lee et al., 2011) of S. involucrata have been shown to significantly enhance the endurance capacities of mice, showing their anti-fatigue activities. In traditional Tibetan/Uyghur medicine, it has long been used under the herbal name of “Tianshan Snow Lotus” for preventing and treating a wide spectrum of disorders. It is famous for its outstanding efficacy in removing dampness, eliminating inflammation, and improving blood circulation and has long been employed to treat acute mountain sickness, fatigue, arthritis, dysmenorrhea, and stomachache (Ma et al., 2011).
Mosloflavon in petroleum extract, such as moslosooflavone, negletein, and 5,6-dihydroxy-7,8-dimethoxyflavone, has been associated with the anti-hypoxia ability of S. involucrata (Jing et al., 2016). Dozens of compounds, including flavonoids, phenylpropanoids, and sphingolipids, have also been isolated, characterized, and identified in recent years (Jia et al., 2014; Gong et al., 2020). Rutin (Figure 2C) is a flavonoid and a principal component in S. involucrata that can alleviate injuries to the brain (Celik et al., 2020), heart (Diwan et al., 2017), and skeletal muscles (Kappel et al., 2013). A supplement of rutin (60 mg/kg) was shown to significantly attenuate physical fatigue via upregulation of the proliferator-activated receptor-α coactivator and sirtuin 1 expression (Su et al., 2014).
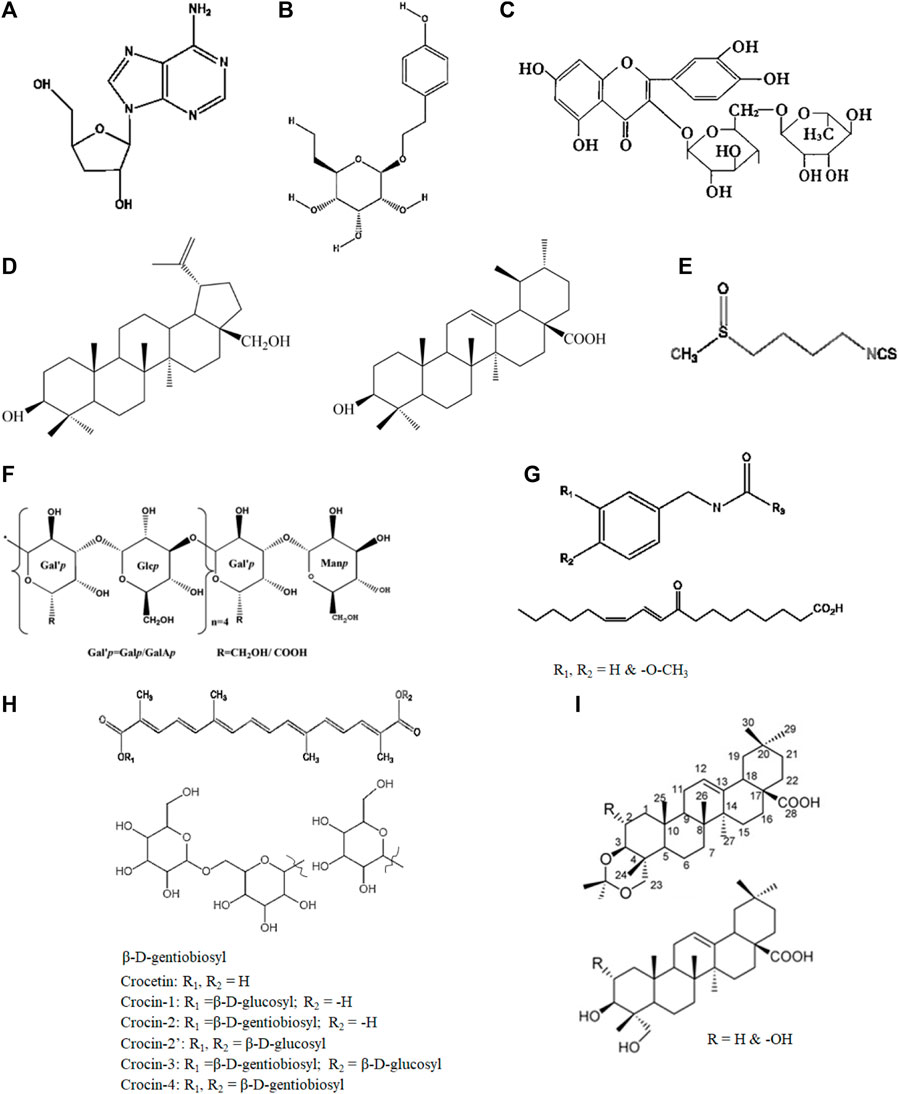
Figure 2. Representative chemical components of the plants used in TTM: (A) cordycepin (Cordyceps sinensis Sacc.), (B) salidroside (Rhodiola rosea L.), (C) rutin (Crocus sativus L.), (D) ursolic acid and botulin (Dracocephalum tanguticum Maxim.), (E) sulforaphane (Brassica rapa L.), (F) Lepidium meyenii Walp. polysaccharides, (G) macamides and macaenes (Lepidium meyenii Walp.), (H) crocin (Crocus sativus L.), (I) triterpenes (Stachyurus himalaicus var. himalaicus Hook. f. et Thoms. ex Benth.).
2.4 Onosma hookeri
The traditional Chinese medicinal herb known as “Zi Cao” has a long history of use as both a medicinal and culinary plant (Wu et al., 2023). However, there is limited documentation on the specific variety of “Zi Cao” sourced from Tibet, specifically O. hookeri, despite its classification as a multisource medicinal material. O. hookeri is the root of the O. hookeri Clarke. var. longiforum Duthie and O. hookeri CB Clarke. varieties that are predominantly found in the eastern and southern regions of Tibet.
The active compounds isolated from O. hookeri include naphthoquinones, alkaloids, flavonoids, and other constituents. Additionally, tigloyl-shikonin, a derivative of shikonin and an acidic polysaccharide with hydrophilic characteristics has been identified in O. hookeri Clarke. var. longiforum Duthie (Wu et al., 2020b; Wu et al., 2023). The acidic polysaccharide derived from O. hookeri Clarke. var. longiforum Duthie, which has shown efficacy in boosting immunity in vitro and in vivo, indicates that the distinct plateau surroundings could influence its metabolic processes and stimulate the synthesis of biologically active substances, ultimately aiding in the identification of polysaccharides with immunomodulatory effects (Wu et al., 2023). Research has also demonstrated that naphthoquinone-enriched ethanolic extracts from the roots of O. hookeri Clarke. var. longiforum Duthie exhibit strong antioxidant properties, suggesting their potential utility as a natural antioxidants (Wu et al., 2020a).
2.5 Carum carvi L.
C. carvi L. is a perennial herbaceous plant belonging to the Umbelliferae family and primarily thrives in grasslands, ravines, riverbanks, and slopes at altitudes exceeding 4,000 m above sea level. This plant is a significant medicinal resource in Tibetan medicine, with historical records in ancient pharmacopoeias and local chronicles documenting the use of its roots and fruits for medicinal purposes.
The main components of C. carvi L. are primarily volatile oils and fatty acids, with minor quantities of flavonoids and phenolic acids (Mahboubi, 2019). The pharmacological properties of C. carvi L. encompass antimicrobial (Kocić-Tanackov et al., 2019), antioxidant (Kamaleeswari and Nalini, 2006), and anti-inflammatory (Keshavarz et al., 2013) effects as well as the potential to enhance gastrointestinal function, mitigate obesity, and demonstrate anticonvulsant properties (Lemhadri et al., 2006; Showraki et al., 2016; Krueger et al., 2020), among other therapeutic benefits. The anti-aflatoxigenic, antioxidant, and antimicrobial effects of C. carvi L. oil along with its reputation as a spice have been used in industries as natural preservatives and antioxidant agents instead of synthetic substances (Mahboubi, 2019).
2.6 Dracocephalum tanguticum Maxim.
D. tanguticum Maxim. is commonly referred to as “Zhiyangge” in Tibetan medicine and is acknowledged as a prominent medicinal plant in Tibet; it has been incorporated into the standard of Tibetan medicine (Volume 1) and is predominantly distributed in the southeastern region of Tibet, eastern region of Qinghai, southwestern region of Gansu, and western region of Sichuan, as documented by the Editorial Committee of the Flora of China in 1997. D. tanguticum Maxim. is a widely used anti-hypoxia medication in Tibetan medicine and is known for its efficacy in enhancing organismal tolerance to hypoxia under both static and locomotor conditions. Studies have shown that this botanical compound can increase blood oxygen levels, elevate ATP concentrations in the brain and heart of hypoxic individuals, and mitigate the depletion of cardiac glycogen reserves. These collective effects contribute to the preservation of normal physiological responses in hypoxic organisms.
At present, the main active components extracted from D. tanguticum Maxim. include triterpenes, flavonoids, alkaloids, phenylpropanoids, sesquiterpenoids, and various other compounds within its phytochemical composition (Hongting et al., 2007; Zhang et al., 2007; Liu et al., 2009; Wang et al., 2009; Wang S. Q. et al., 2010; Zeng et al., 2011; Hu et al., 2020; Wang et al., 2024). Hongting et al. (2007) utilized silica gel column chromatography to investigate the fat-soluble chemical constituents of D. tanguticum Maxim., resulting in the isolation and identification of ursolic acid, botulin, and additional compounds from its supercritical extract (Figure 2D).
2.7 Gymnadenia conopsea (L.) R. Br.
G. conopsea (L.) R. Br. is a perennial herbaceous flowering plant classified within the family Orchidaceae that exhibits a broad distribution across the temperate and subtropical regions of northern Europe and Asia, spanning altitudes ranging from 200 to 4,700 m (Shang et al., 2017). In mainland China, G. conopsea (L.) R. Br. predominantly inhabits western regions, specifically within the provinces of Tibet, Xinjiang, Inner Mongolia, Sichuan, Qinghai, and Gansu (Zi et al., 2008). The botanical species G. conopsea (L.) R. Br. is given the vernacular name “Shou Zhang Shen,” which translates to “palm-like ginseng,” owing to the resemblance of its tuber shape to that of a human hand. The pharmacological properties of G. conopsea (L.) R. Br. have garnered significant attention in academic research, where studies have confirmed its antioxidant, antiviral, and anti-fatigue characteristics, aligning with its historical medicinal applications. Furthermore, recent investigations have revealed additional pharmacological effects, including immunomodulatory, anti-hyperlipidemic, and neuroprotective actions (Meng et al., 2023). It is noteworthy that the anti-fatigue properties of G. conopsea (L.) R. Br. and its derivatives are notably significant in oxygen-deprived environments, making them valuable ingredients in pharmaceuticals, nutraceuticals, and food products.
A total of 170 natural compounds have been identified and characterized from G. conopsea (L.) R. Br., all of which were extracted from its tubers. Notably, 54 of these compounds are benzylester glucosides, which are considered as significant constituents. Furthermore, various other compounds, such as dihydrostilbenes, phenanthrenes, polysaccharides, lignans, alkaloids, triterpenoids, flavonoids, steroids, and phenolic compounds, have been detected (Meng et al., 2023).
2.8 Brassica rapa L.
B. rapa L. (also called Tibetan turnip) is native throughout Europe, Russia, Central Asia, and the Near East; it is commonly known as turnip and is one of the oldest cultivated vegetables, with wide consumption in Asia for thousands of years (Cao et al., 2021). It has been widely cultivated for dietary, feeding, and medical purposes owing to its diuretic and detoxifying properties, which can help alleviate water retention and promote elimination of toxins from the body (Yin et al., 2017). The glucosinolates present in this plant have been found to have protective effects against hypoxia-induced oxidative stress and inflammation. Additionally, the high fiber content of B. rapa L. can help regulate blood glucose levels and promote healthy digestion, both of which may help reduce fatigue and increase overall energy levels. As an edible plant, B. rapa L. is used to achieve anti-hypoxia and anti-fatigue outcomes (Zhao et al., 2021b) as well as for tonic, heat-clearing, detoxification, and neuroprotection applications (Chu et al., 2017; Paul et al., 2019; Hua et al., 2021a).
Polysaccharides are generally recognized as one of the major bioactive substances present mostly in the root parts of plants used in TTM, providing excellent anti-fatigue effects and antioxidant enzyme activities (Li et al., 2018b). B. rapa L. polysaccharides (BRP, 94.61% purity) obtained by hot-water extraction with ethanol precipitation have been verified to have anti-fatigue properties (Tang et al., 2018); BRP was shown to effectively extend the swimming time and average swimming speed of mice by regulating the levels of their antioxidative-related enzyme, such as glutathione peroxidase and lactate dehydrogenase at doses of 100 mg/kg bodyweight (bw) per day. Another subspecies called B. rapa chinensis (L.) (200 and 400 mg/kg) was observed to have analgesic and antidepressant activities in the Swiss albino mice model (Rahman et al., 2015). Glucosinolates are naturally found in Brassicaceae plants, such as B. rapa L. (Paul et al., 2019); in addition to polysaccharides, flavonoids, and phenylpropanoids, which normally exist in most edible plants, other active constituents with turnip characteristics such as sulforaphane (Figure 2E) and p-coumaric acid (Li et al., 2018a) have also been shown to have beneficial effects against hypoxia and fatigue. Sulforaphane is one of the most important isothiocyanates and is present in cruciferous vegetables, including Lepidium meyenii Walp. and B. rapa L.; at a dose of 25 mg/kg, it was shown to upregulate the nuclear factor (erythroid derived 2)-like 2 (Nrf2) and downstream genes, significantly attenuating muscle fatigue and improving exercise performance (Oh et al., 2017). Ikeuchi et al. (2009) found that benzylglucosinolate (0.015 or 0.030 mg/kg) significantly increased mice endurance exercise capacity due to greater utilization of fatty acids as the energy sources.
2.9 Other traditional Tibetan medicinal plants and bioactive components
L, meyenii Walp. (also called Maca) has been utilized for almost 2,000 years across the Peruvian highlands, South American Andes, Tibetan Plateau, and Taipei Plateau (Gonzales, 2012); it has been traditionally employed in the Andean region for its activities against hypoxia- and fatigue-associated symptoms in the highlands, especially those related to sexual behaviors (Cicero et al., 2001). Daily consumption of spray-dried extracts of red and black L. meyenii Walp. (3 g) was shown to improve health-related quality of life (sexual desire, mood, and energy) at low altitudes as well as chronic mountain sickness (CMS) (glycemia, blood pressure, and hemoglobin levels) at high altitudes (Gonzales, 2012). A cross-sectional study suggested that L. meyenii Walp. significantly decreased the testosterone/estradiol ratio and IL-6 levels along with low CMS scores (Gonzales et al., 2013). Li et al. (2017b) isolated and purified two fractions of L. meyenii Walp. polysaccharides from its aqueous extract, which presented dose-dependent positive effects on forced swimming in mice. Other L. meyenii Walp. polysaccharides were isolated from a water extract of L. meyenii Walp. tubers, demonstrating significant anti-fatigue effects (Figure 2F) (Weimin et al., 2017). Remarkably, several studies showed that macamides (Figure 2G), which are a series of characteristic compounds in the root of L. meyenii Walp., had significant anti-fatigue activities (Zhu et al., 2020).
In addition to the aforementioned plants, several others, such as Nitraria tangutorum Bobrov. (1,900–3,500 m) (Suo et al., 2005), Hordeum vulgare L. (4,200–4,500 m) (Kim et al., 2012), and Crocus sativus L., have also been shown to have different degrees of anti-hypoxia and anti-fatigue activities (Table 1). Interestingly, most of these species having considerable anti-hypoxia and anti-fatigue properties grow in plateau regions under extreme cold climates. They have adjusted growths and stresses to adapt to the high altitudes and frigid weather conditions (Reyes et al., 2020). C. sativus L. contains several bioactive compounds, including crocin (Figure 2H), crocetin, and safranal (Akbari et al., 2018), which are responsible for its pharmacological effects, including antioxidant, anti-inflammatory, and neuroprotective properties. Terpenoids are one of the largest classes of chemical components in natural products; they are rich resources for developing pharmaceuticals, fragrances, and biofuels. Additionally, triterpenoids have been identified from Stachyurus himalaicus var. himalaicus Hook. f. et Thoms. ex Benth. (Figure 2I) (Wang Y. S. et al., 2010).
In conclusion, biological multilevel theory describes that plant pharmacology mechanisms may be linked to the habitats of high-altitude medical plants for quantification of the active ingredients and human internal biological processes (Li et al., 2020). The manner in which the plateau environment and climate participate in such regulation of the metabolisms of high-altitude plants and their effects on the pharmacological properties, especially for anti-hypoxia and anti-fatigue activities, may be interesting questions for further exploration.
3 Characteristic ethnomedical uses in modern healthcare practices
Given their specific environmental conditions, the majority of TTMs serve distinct and individualized functions in combating hypoxia and mitigating fatigue (Hua et al., 2021b; Zhu et al., 2021). Additionally, a strong correlation exists between hypoxia and fatigue. Hypoxia adversely affects cellular aerobic respiration, leading to tissue hypoxia and the onset of fatigue (Wirth and Scheibenbogen, 2020). The capacity to withstand hypoxia aids in upholding regular energy metabolism, consequently diminishing fatigue. Hypoxia triggers a cascade of metabolic dysfunctions, and the potential anti-fatigue mechanisms may involve management of these metabolic irregularities to sustain homeostasis (Zhu et al., 2022a).
3.1 Highlands: focus on hypoxia
Hypoxia or fatigue remains one of the major risk factors for human life and health in the highlands, especially in people with underlying conditions. There are approximately over 140 million people who live above altitudes of 2,500 m across the world, and the highest population density is located above 3,500 m (Chu et al., 2017). In recent years, there have also been over 40 million visitors from plains who have been exposed to plateau environments yearly through traveling, altitude training, or military needs, who could be plagued by high-altitude hypoxia and related illnesses (Pena et al., 2022). The Tibetan Plateau, with an average altitude exceeding 4,000 m, is one of the highest regions on Earth (Dong et al., 2020). Residents of the Tibetan Plateau have often suffered the harsh highland environment from birth for thousands of years, thus necessitating the development of TTMs to combat hypoxia, fatigue, and related symptoms (Chu et al., 2017).
Alpine plants have demonstrated multiple pharmacological activities for regulating acute mountain sickness and physical fatigue (Khanum et al., 2005; Larmo et al., 2008; Li et al., 2017b; Akbari-Fakhrabadi et al., 2019a). Most of these high-altitude plants have been used in TCM for over 2,000 years. Interestingly, these medicinal plants, which are commonly used to replenish the kidney and spleen as well as soothe the lung (Chinese Pharmacopoeia Commission, 2010), have long been used to ameliorate human conditions associated with hypoxia, fatigue, and related symptoms (Ma et al., 2011; Qian et al., 2011; Gonzales et al., 2013; Long et al., 2021b; Cao et al., 2021). It is fascinating and awe-inspiring for permanent residents and tourists to live in the highlands, but symptoms of altitude sickness can be unbearable given the low atmospheric pressures and low oxygen levels (León-Velarde et al., 2005). Thin air in the highlands may cause acute and chronic hypoxia, inducing increased right ventricular afterload and pulmonary hypertension (Grimminger et al., 2017). Fortunately, most native foods and traditional medicines in such regions may potentially provide protection to highland dwellers for multisystem regulation (Zhu et al., 2022a). For example, R. rosea L. is widely used by highland inhabitants to ameliorate depression, altitude sickness, and fatigue (Panossian et al., 2010). By enhancing immunity and hypoxia tolerance, these products combine healthcare properties with medicinal value. Thus, these nutraceuticals can be widely used in the highlands to prevent and treat symptoms of altitude hypoxia (Hou et al., 2021).
In humans, muscle fatigue is often defined as an exercise-induced decrease in producing force that relies on contractile mechanisms. On the other hand, impaired circulation or insufficient oxygen supply experienced in varying degrees by patients with chronic fatigue syndrome (CFS) can lead to tissue hypoxia as well as clinical manifestations such as fatigue and dizziness (Wirth and Scheibenbogen, 2020). Therefore, hypoxia-related genes like the hypoxia-inducible factors (HIFs) have been proven to be appropriate targets for fatigue resistance and exercise tolerance via hypoxic adaptation (Dang and Gao, 2008), which is a commonly suggested treatment for fatigue-related disorders in several physiologic and pathologic processes (Halley et al., 2019; Holland et al., 2020). Two ancient Tibetan pharmacopoeias, namely The Four Medical Tantras and Jing Zhu Materia Medica, state that B. rapa L. has effects like “tonic and anti-hypoxia,” “alleviating fatigue,” and “heat-clearing and detoxification,” and has been traditionally exploited for alleviation of plateau hypoxia, fatigue, and associated symptoms (Chu et al., 2017). Chu et al. (2017) also found that consumption a powder made of Tibetan turnips (7.5 g twice daily) for a week could improve oxygen uptake and delivery, body antioxidant capacity, and cardio-pulmonary conditions in healthy humans, based on the Bruce treadmill protocol. A 7-day human self-control and single-blind human feeding trial showed that B. rapa L. improved hypoxia tolerance in healthy humans, characterized by improved pulse oxygen saturation (SpO2) (Chu et al., 2017). Chu et al. (2018) found that B. rapa L. improved the tolerances of athletes to hypoxia, which might be attributed to enhancements of their cardiopulmonary functions and oxygen metabolisms. Because of the bioactivities of anti-hypoxia medications, they can be used in cerebral stroke as inhibitors of reactive oxygen species (ROS) induced by oxygen glucose deprivation/reperfusion (OGD/R) to restore mitochondrial expression via the PI3K/Akt/mTOR pathway (Hua et al., 2021b).
3.2 Flatlands: focus on fatigue
Fatigue is best described as an overwhelming feeling of tiredness and exhaustion; similar to the symptoms of hypoxia, it is characterized by several processes like angiogenesis and energy metabolism disorders (Wang et al., 2019). In general, fatigue can be classified as central or peripheral fatigue at different levels of the motor pathway. The former is commonly caused by changes in the central neurotransmitters and is perceived in the central nervous system (CNS), which can lead to severe neurological damage and dysfunction by hypoxia (Hanyi et al., 2021). It can be accompanied by multiple mental disorders, such as psychological loss of motivation or appetite, sleepiness, and reduced psychological alertness (Kwak et al., 2020). As a progressive, exercise-induced degradation of muscle voluntary activation, it is closely related to peripheral fatigue (Hogan et al., 2020). Specifically, energy consumption through glycogen, adenosine triphosphate (ATP), and metabolic factors like H+, lactate, Pi, and ROS generated by strenuous exercise can influence the contractile functions of skeletal muscles to accelerate fatigue (Wu et al., 2014). In Tibetan medicine, R. rosea L. has been used to relieve high-altitude sickness, anoxia, and mountain malhypoxia (Qian et al., 2011). R. rosea L. has been categorized as an adaptogen by Russian researchers for its pharmacological activity of preventing chemical, biological, and physical stressors. Several anti-fatigue trials have identified multiple pharmacological effects of R. rosea L. extracts (Hung et al., 2011). Ballmann et al. (2019) found that short-term supplementation with R. rosea L. (golden root extract, 1,500 mg/person) enhanced the anaerobic exercise performances of college-age females. Another clinical trial on healthy men showed that chronic R. rosea L. supplementation contributed to better mental and physical performances, with enhanced total antioxidant capacity (Jowko et al., 2018).
Although the partial pressure of oxygen is normal in the flatlands, the rich phytochemical profiles of high-altitude plants have been exploited further with high commercial value (Zhu et al., 2022a). Based on their ethnomedical uses and market demands, a series of products based on the value chain of high-altitude medicinal plants are being developed rapidly. Nowadays, R. rosea L. has been shown to have medicinal and food homology (El-Houri et al., 2014), and L. meyenii Walp., which is a typical example of a superfood, has been certified as a new food resource in China (Zhu et al., 2020). L. meyenii Walp. rose to fame in the last decade for its reputation as “plant Viagra” mainly because of its fertility-related activities, which were shown to improve sexual behaviors (Cicero et al., 2001), litter size (Ruiz-Luna et al., 2005), and spermatogenesis quality (Gonzales et al., 2006). Based on the pharmacological characteristics of S. involucrata, many snow lotus products like cosmeceuticals, food supplements, and medications are now available in the current herbal market. Thus far, international exports of high-altitude plant products in China, mostly from the Qinghai–Tibet Plateau, have become a major driver of commercial trade. For example, R. rosea L. has been formulated into capsules to achieve significant improvements in maximal oxygen uptake (VO2max) and endurance performance among healthy males after dietary supplementation for 7 weeks (Zhang et al., 2009). Medicinal herbs are often used as nutritious and functional foods nowadays, and high-altitude plants have been utilized for several health-associated functions, such as anti-fatigue (Li et al., 2017b), antioxidant (Lee and Chang, 2019), neuroprotective (Yu et al., 2019), prebiotic, and anti-inflammatory (Lee et al., 2020) properties, gradually garnering attention as well as increased consumption worldwide over the past 20 years.
4 Safety and toxicity
Most of the compounds present in high-altitude plants are orally administered, with low or no toxicity. The oral median lethal dose (LD50) of Rhodiola root aqueous extract was observed to be more than 10 g/kg (Gupta et al., 2008), and the LD50 of the L. meyenii Walp. ethanolic extract was greater than 2 g/kg (Abarikwu et al., 2020). It was also observed that a single dose of L. meyenii Walp. extract at 17 g/kg did not result in acute toxicity in rats (Chen et al., 2021), suggesting that the concentrations required for exhibiting bioactivities are lower than their toxic concentrations (Hudson et al., 2018). The acute toxicity profiles of C. carvi L. suggests that the highest non-lethal doses of parsley essential oil and aqueous extract are 400 mg/kg and 3,200 mg/kg, respectively (Showraki et al., 2016). Furthermore, it was found that the LD50 value of C. sativus L. in mice was 4,120 ± 556 mg/kg (Bahmani et al., 2014). Mice and rats were subjected to different doses (1.00, 2.15, 4.64, and 10 g/kg) of G. conopsea (L.) R. Br. tubers, and no statistically significant changes in their behaviors and growths were observed compared to the control group over a 2-week observation period, suggesting that the LD50 of G. conopsea (L.) R. Br. tubers exceeds 10 g/kg (Dian-Qing and Ying, 2007). An acute toxicity experiment of D. tanguticum Maxim. conducted on mice indicated that the LD50 values for intraperitoneal injection and intragastric administration were 18.8 ± 0.6 g/kg and 142.3 ± 4.2 g/kg, respectively (Hai et al., 1995). Moreover, research has shown that laboratory-cultured mycelia powder of C. sinensis Sacc. is safe and non-toxic for dosages up to 2 g/kg (Meena et al., 2013). No fatalities were recorded in mice administered 18.6 g/kg of B. rapa L. polysaccharides (Bakasi et al., 2019).
These scientific studies and centuries of traditional use confirm the acceptability and safety of consumption of high-altitude medicinal products, along with improvements to the mood, energy, and health status, as well as reduced CMS scores (Gonzales-Arimborgo et al., 2016). However, S. involucrata is prohibited for use by pregnant women in the Pharmacopoeia of China (Chinese Pharmacopoeia Commission, 2010) owing to its side effect of abortion at 0.3 mL of intravenous administration; in addition, S. involucrata has been associated with some nervous and cardiovascular disorders, such as arrhythmia, hypotension, and nausea. Contradictorily, S. involucrata injection (Chik et al., 2015) and its culture (Han et al., 2021) have been proven to have no distinct toxicity or adverse effects (Table 1).
5 Challenges and prospects
5.1 Globalization and modernization research on TTMs
Future developments in TTM will likely involve a combination of traditional knowledge and modern scientific approaches (Luo, 2015). Globalization and modernization have become some of the principal targets of most traditional medicine systems, particularly the herbal drugs and prescriptions of Asia, south/west Africa, and Latin America. However, modernization of TTM is still a long way off owing to its complex ingredients and uncertain mechanisms (Yan et al., 2021). Thus, one of the key segments in the modernization research on TTM is clarification of the chemical compositions and transformation of pharmacological research findings on traditional Tibetan plants into products of commercial value. However, the Tibetan medicine industry is plagued by insufficient and overdrawn Tibetan medicinal resources as well as small-scale enterprises, which poses problems on sustainable and rapid developments (Luo, 2015).
5.2 Sustainable development of places growing Tibetan medicinal plants
Places where geo-authentic medicines are produced are termed as “trueborn areas,” and medicinal herbs from trueborn areas are most remarkable in terms of both quality and efficacy, particularly those that grow in the highlands (Tang et al., 2017). However, most of the high-altitude medicinal plants are grown and described with respect to plateau areas, where both economy and productivity are relatively poor. In addition, excessive exploitation of high-altitude plants has resulted in serious regional damage to natural niches owing to the fragile ecological environments on plateaus. Growing and developing high-altitude plants also entails transportation barriers stemming from distance and the lack of intelligence and informatization in the mountains. Based on TCM theory, L. meyenii Walp. has been used as a classic medicinal plant for warming and invigorating the kidney (Yang et al., 2018) as well as for spleen-deficiency syndrome (Fei et al., 2020). Compared with people residing at sea levels, delayed healing rates are observed among wounded dwellers at high altitudes as well as increased wound widths; red L. meyenii Walp. has been shown to promote skin wound healing at both sea level and high altitudes (Nunez et al., 2017). Thus, sustainable development of medicinal origin places and wild collection standards must be prioritized over the next several decades (Brinckmann et al., 2021).
5.3 Adulteration in the Tibetan medicinal materials market
Traditional Tibetan medicinal plants are critically endangered given the increasingly lucrative market for TTM in recent times, especially the “Daodi herbs”. The present discriminations between raw and processed high-altitude medicines are mostly focused on important active components, such as salidroside in R. rosea L. and macamides in L. meyenii Walp. However, there is increasing concern about economically motivated adulteration (EMA) and substitution of inferior species admixtures in the raw high-altitude herbal trade, which can harm consumer faith (Srirama et al., 2017). It is also particularly alarming that the reported adulterants in medicinal plants may have uncertain effects on its safety and efficacy. Thus, it is imperative to enforce universally acceptable pharmacopoeia-specified standards on products to authenticate quality control (QC) of Tibetan medicinal plants.
6 Conclusion
TTM is homologous to the roots of the Chinese traditional systems of medicine, where the concept of homology of medicine and food is a fundamental aspect. Adhering to this holistic therapeutic philosophy, TTM is based on herbal medicines that have natural synergistic effects (multiple compounds with multiple targets and one pathway), emphasizing the essential role of food and herbs in promoting health and preventing illness. In particular, geo-authentic Tibetan medicines carefully chosen through extensive clinical experience by practitioners of TCM exhibit superior quality and therapeutic effectiveness compared to similar herbs from other regions, thus enjoying widespread popularity. In addition, compared to mainstream allopathic medicines, it is generally known natural plants, especially those that are homologous medicine and food, are considered to be safer with fewer side-effects.
The market for geo-authentic Tibetan medicines is witnessing an ever-increasing demand globally as herbal medicines are being preferred by increasing numbers of elderly and health-conscious people. Traditional medicinal plants tend to be potential therapeutic strategies for more tolerant to some chronic disorders in daily life, which may be critical for improving the physical states in subhealth populations. Although further research is necessary to fully understand the mechanisms of action and potential benefits of TTMs, their use in folk medicine serves as a valuable starting point for modern medical research.
Author contributions
NW: Writing–original draft, Conceptualization. HZ: Writing–review and editing, Validation, Formal analysis.
Funding
The author(s) declare that financial support was received for the research, authorship, and/or publication of this article. This study was supported by the Research Start-up Funding Program for High-Level Talents of Moutai Institute (no. mygccrc [2023]012).
Conflict of interest
The authors declare that the research was conducted in the absence of any commercial or financial relationships that could be construed as a potential conflict of interest.
Publisher’s note
All claims expressed in this article are solely those of the authors and do not necessarily represent those of their affiliated organizations or those of the publisher, editors, and reviewers. Any product that may be evaluated in this article or claim that may be made by its manufacturer is not guaranteed or endorsed by the publisher.
References
Abarikwu, S. O., Onuah, C. L., and Singh, S. K. (2020). Plants in the management of male infertility. Andrologia 52 (3), e13509. doi:10.1111/and.13509
Akbari, G., Mard, S. a., and Veisi, A. (2018). A comprehensive review on regulatory effects of crocin on ischemia/reperfusion injury in multiple organs. Biomed. Pharmacother. 99, 664–670. doi:10.1016/j.biopha.2018.01.113
Akbari-Fakhrabadi, M., Najafi, M., Mortazavian, S., Rasouli, M., Memari, A.-H., and Shidfar, F. (2019a). Effect of saffron (Crocus sativus L.) and endurance training on mitochondrial biogenesis, endurance capacity, inflammation, antioxidant, and metabolic biomarkers in Wistar rats. J. Food Biochem. 43 (8), e12946. doi:10.1111/jfbc.12946
Angeli, G., Neto, T. B., and Kirkendall, D. (2018). The effects of Rhodiola rosea supplementation on time to fatigue and recovery after exercise in rats. Med. Sci. Sports Exerc. 50 (5), 722. doi:10.1249/01.mss.0000538379.40307.44
Ashraf, S. A., Elkhalifa, A. E. O., Siddiqui, A. J., Patel, M., Awadelkareem, A. M., Snoussi, M., et al. (2020). Cordycepin for health and wellbeing: a potent bioactive metabolite of an entomopathogenic cordyceps medicinal fungus and its nutraceutical and therapeutic potential. Molecules 25 (12), 2735. doi:10.3390/molecules25122735
Bahmani, M., Rafieian, M., Baradaran, A., Rafieian, S., and Rafieian-Kopaei, M. (2014). Nephrotoxicity and hepatotoxicity evaluation of Crocus sativus stigmas in neonates of nursing mice. J. Nephropathol. 3 (2), 81–85. doi:10.12860/jnp.2014.16
Bakasi, A., Sheng, H., Li, C., Taoerdahong, H., Su-Lin, C., Zhi-Jin, C., et al. (2019). Study on the anti-Lewis lung cancer activity of Brassica rapa L. acid polysaccharide-2 in vivo. J. Food Saf. Qual. 10 (15), 5111–5116.
Ballmann, C. G., Maze, S. B., Wells, A. C., Marshall, M. M., and Rogers, R. R. (2019). Effects of short-term Rhodiola rosea (golden root extract) supplementation on anaerobic exercise performance. J. Sports Sci. 37 (9), 998–1003. doi:10.1080/02640414.2018.1538028
Brinckmann, J. A., Cunningham, A. B., and Harter, D. E. V. (2021). Running out of time to smell the roseroots: reviewing threats and trade in wild Rhodiola rosea L. J. Ethnopharmacol. 269, 113710. doi:10.1016/j.jep.2020.113710
Cao, Q., Wang, G., and Peng, Y. (2021). A critical review on phytochemical profile and biological effects of turnip (Brassica rapa L.). Front. Nutr. 8, 721733. doi:10.3389/fnut.2021.721733
Celik, H., Kandemir, F. M., Caglayan, C., Ozdemir, S., Comakli, S., Kucukler, S., et al. (2020). Neuroprotective effect of rutin against colistin-induced oxidative stress, inflammation and apoptosis in rat brain associated with the CREB/BDNF expressions. Mol. Biol. Rep. 47 (3), 2023–2034. doi:10.1007/s11033-020-05302-z
Chen, J. (2023). Essential role of medicine and food homology in health and wellness. Chin. Herb. Med. 15 (3), 347–348. doi:10.1016/j.chmed.2023.05.001
Chen, R., Wei, J. C., and Gao, Y. M. (2021). A review of the study of active components and their pharmacology value in Lepidium meyenii (Maca). Phytotherapy Res. 35 (12), 6706–6719. doi:10.1002/ptr.7257
Chik, W.-I., Zhu, L., Fan, L.-L., Yi, T., Zhu, G.-Y., Gou, X.-J., et al. (2015). Saussurea involucrata: a review of the botany, phytochemistry and ethnopharmacology of a rare traditional herbal medicine. J. Ethnopharmacol. 172, 44–60. doi:10.1016/j.jep.2015.06.033
Chu, B., Chen, C., Li, J., Chen, X., Li, Y., Tang, W., et al. (2017). Effects of Tibetan turnip (Brassica rapa L.) on promoting hypoxia-tolerance in healthy humans. J. Ethnopharmacol. 195, 246–254. doi:10.1016/j.jep.2016.11.028
Chu, B., Li, Y., Gong, J., Li, J., Wang, N., Chen, X., et al. (2018). Human trials of Tibetan turnip on promoting athletes’ tolerant ability to hypoxia. J. Chin. Inst. Food Sci. Technol. 18 (8), 62–71. doi:10.16429/j.1009-7848.2018.08.009
Cicero, A. F. G., Bandieri, E., and Arletti, R. (2001). Lepidium meyenii Walp. improves sexual behaviour in male rats independently from its action on spontaneous locomotor activity. J. Ethnopharmacol. 75(2-3), 225–229. doi:10.1016/S0378-8741(01)00195-7
Cui, E., Zhi, X., Chen, Y., Gao, Y., Fan, Y., Zhang, W., et al. (2014). Coptis chinensis and myrobalan (Terminalia chebula) can synergistically inhibit inflammatory response in vitro and in vivo. Evidence-based complementary Altern. Med. eCAM 2014, 510157. doi:10.1155/2014/510157
Cunningham, A. B., Li, H. L., Luo, P., Zhao, W. J., Long, X. C., and Brinckmann, J. A. (2020). There "ain’t no mountain high enough"? the drivers, diversity and sustainability of China’s Rhodiola trade. J. Ethnopharmacol. 252, 112379. doi:10.1016/j.jep.2019.112379
Dang, C. V., and Gao, P. (2008). Muscle fatigue from losing your PHD. Cell Metab. 7 (3), 191–192. doi:10.1016/j.cmet.2008.02.007
Degens, H., Horneros, J. M. S., and Hopman, M. T. E. (2006). Acute hypoxia limits endurance but does not affect muscle contractile properties. Muscle & Nerve 33 (4), 532–537. doi:10.1002/mus.20485
Dian-Qing, B., and Ying, Z. (2007). Toxicological evaluation of the safty of Tibetan drug wangla. Chin. J. Comp. Med. 17 (12), 740–742.
Diwan, V., Brown, L., and Gobe, G. C. (2017). The flavonoid rutin improves kidney and heart structure and function in an adenine-induced rat model of chronic kidney disease. J. Funct. Foods 33, 85–93. doi:10.1016/j.jff.2017.03.012
Dong, Y., Dun, B., Dui, P. B. W., Zhuo, L. B., Fang, J., Zong, C. R. Y., et al. (2020). Therapeutic erythrocytapheresis is effective in treating high altitude polycythemia on the Qinghai-Tibet Plateau. Wilderness & Environ. Med. 31 (4), 426–430. doi:10.1016/j.wem.2020.07.006
El-Houri, R. B., Kotowska, D., Olsen, L. C., Bhattacharya, S., Christensen, L. P., Grevsen, K., et al. (2014). Screening for bioactive metabolites in plant extracts modulating glucose uptake and fat accumulation. Evid. Based Complement. Altern. Med. 2014, 156398. doi:10.1155/2014/156398
Fei, W., Hou, Y., Yue, N., Zhou, X., Wang, Y., Wang, L., et al. (2020). The effects of aqueous extract of Maca on energy metabolism and immunoregulation. Eur. J. Med. Res. 25 (1), 24. doi:10.1186/s40001-020-00420-7
Gong, G. W., Huang, J., Yang, Y., Qi, B. H., Han, G. Y., Zheng, Y. Z., et al. (2020). Saussureae involucratae herba (snow Lotus): review of chemical compositions and pharmacological properties. Front. Pharmacol. 10, 1549. doi:10.3389/fphar.2019.01549
Gonzales, C., Rubio, J., Gasco, M., Nieto, J., Yucra, S., and Gonzales, G. F. (2006). Effect of short-term and long-term treatments with three ecotypes of Lepidium meyenii (MACA) on spermatogenesis in rats. J. Ethnopharmacol. 103 (3), 448–454. doi:10.1016/j.jep.2005.08.035
Gonzales, G. F. (2012). Ethnobiology and ethnopharmacology of Lepidium meyenii (maca), a plant from the Peruvian highlands. Evidence-Based Complementary Altern. Med. 2012, 193496. doi:10.1155/2012/193496
Gonzales, G. F., Gasco, M., and Lozada-Requena, I. (2013). Role of maca (Lepidium meyenii) consumption on serum interleukin-6 levels and health status in populations living in the Peruvian central Andes over 4000 m of altitude. Plant Foods Hum. Nutr. 68 (4), 347–351. doi:10.1007/s11130-013-0378-5
Gonzales-Arimborgo, C., Yupanqui, I., Montero, E., Alarcon-Yaquetto, D. E., Zevallos-Concha, A., Caballero, L., et al. (2016). Acceptability, safety, and efficacy of oral administration of extracts of black or red maca (Lepidium meyenii) in adult human subjects: a randomized, double-blind, placebo-controlled study. Pharm. (Basel) 9 (3), 49. doi:10.3390/ph9030049
Grimminger, J., Richter, M., Tello, K., Sommer, N., Gall, H., and Ghofrani, H. A. (2017). Thin air resulting in high pressure: mountain Sickness and hypoxia-induced pulmonary hypertension. Can. Respir. J. 2017, 8381653. doi:10.1155/2017/8381653
Gupta, V., Saggu, S., Tulsawani, R. K., Sawhney, R. C., and Kumar, R. (2008). A dose dependent adaptogenic and safety evaluation of Rhodiola imbricata Edgew, a high altitude rhizome. Food Chem. Toxicol. 46 (5), 1645–1652. doi:10.1016/j.fct.2007.12.027
Hai, P., Ye, Y., Zhou, S., and Quan, K. (1995). THE ANTIHYPOXIC EFFECT OF DRACOCEPHALUM TANGUTICUM MAXIM. J. High Alt. Med. 5 (2), 4.
Hai, P., Zhou, S., Shang, H., and Zhao, G. (1997). Antianoxic effects of Dracocephalum tanguticum on brain of mice. Zhong yao cai = Zhongyaocai = J. Chin. Med. Mater. 20 (4), 198–200.
Halley, S. L., Marshall, P., and Siegler, J. C. (2019). Effect of ischemic preconditioning and changing inspired O-2 fractions on neuromuscular function during intense exercise. J. Appl. Physiology 127 (6), 1688–1697. doi:10.1152/japplphysiol.00539.2019
Han, L., Chen, K., Liu, P., Yang, L., Kang, Y., Gao, Y., et al. (2021). Toxicological evaluation of S. involucrata culture: acute, 90-day subchronic and genotoxicity studies. Regul. Toxicol. Pharmacol. 124, 104980. doi:10.1016/j.yrtph.2021.104980
Han, X., Su, D., Xian, X., Zhou, M., Li, X., Huang, J., et al. (2016). Inhibitory effects of Saussurea involucrata (Kar. et Kir.) Sch -Bip. on adjuvant arthritis in rats. J. Ethnopharmacol. 194, 228–235. doi:10.1016/j.jep.2016.09.008
Hanyi, H., Hongkang, Z., Chang, L., Wenyi, Z., Jiayi, L., Bin, H., et al. (2021). Bioactive compound from the Tibetan turnip (Brassica rapa L.) elicited anti-hypoxia effects in OGD/R-injured HT22 cells by activating the PI3K/AKT pathway. Food & Funct. 12 (7), 2901–2913. doi:10.1039/d0fo03190a
Hao, D. C., Xiao, P. G., and Liu, C. (2018). Traditional Tibetan medicinal plants: a highlighted resource for novel therapeutic compounds. Future Med. Chem. 10 (21), 2537–2555. doi:10.4155/fmc-2018-0235
Hogan, P. S., Chen, S. X., Teh, W. W., and Chib, V. S. (2020). Neural mechanisms underlying the effects of physical fatigue on effort-based choice. Nat. Commun. 11 (1), 4026. doi:10.1038/s41467-020-17855-5
Holland, A. E., Corte, T., Chambers, D. C., Palmer, A. J., Ekstrom, M. P., Glaspole, I., et al. (2020). Ambulatory oxygen for treatment of exertional hypoxaemia in pulmonary fibrosis (PFOX trial): a randomised controlled trial. Bmj Open 10 (12), e040798. doi:10.1136/bmjopen-2020-040798
Hongkang, Z., Wenqian, X., Ning, W., Wenhao, J., Yuliang, C., Yahui, G., et al. (2021). Anti-fatigue effect of Lepidium meyenii Walp. (Maca) on preventing mitochondria-mediated muscle damage and oxidative stress in vivo and vitro. Food & Funct. 12 (7), 3132–3141. doi:10.1039/d1fo00383f
Hongting, Z., Guogang, Z., Yafu, Z., Yinping, J. I. N., Shushan, D. U., and Zhiwei, D. (2007). Liposoluble constituents of Dracocephalum tanguticum maxim. (Ⅰ). Chin. J. Med. Chem. 17 (5), 314–315.
Hou, Y., Tang, Y., Wang, X., Ai, X., Wang, H., Li, X., et al. (2020). Rhodiola Crenulata ameliorates exhaustive exercise-induced fatigue in mice by suppressing mitophagy in skeletal muscle. Exp. Ther. Med. 20 (4), 3161–3173. doi:10.3892/etm.2020.9072
Hou, Y., Wang, X. B., Zhang, Y., Wang, S. H., and Meng, X. L. (2021). Highland mate: edible and functional foods in traditional medicine for the prevention and treatment of hypoxia-related symptoms. Curr. Opin. Pharmacol. 60, 306–314. doi:10.1016/j.coph.2021.07.018
Hu, L.-J., Zeng, L., Ma, E.-G., Yang, C., Wu, H.-Y., and Li, G.-P. (2020). Dratanguticumides G and H, two new glucosides from Dracocephalum tanguticum Maxim relax vessels via NO pathway. Phytochem. Lett. 40, 42–48. doi:10.1016/j.phytol.2020.08.006
Hua, H., Zhang, W., Li, J., Li, J., Liu, C., Guo, Y., et al. (2021a). Neuroprotection against cerebral ischemia/reperfusion by dietary phytochemical extracts from Tibetan turnip (Brassica rapa L.). J. Ethnopharmacol. 265, 113410. doi:10.1016/j.jep.2020.113410
Hua, H., Zhu, H., Liu, C., Zhang, W., Li, J., Hu, B., et al. (2021b). Bioactive compound from the Tibetan turnip (Brassica rapa L.) elicited anti-hypoxia effects in OGD/R-injured HT22 cells by activating the PI3K/AKT pathway. Food & Funct. 12 (7), 2901–2913. doi:10.1039/d0fo03190a
Huang, L., Li, T. Y., Zhou, M., Deng, M. Y., Zhang, L. D., Yi, L., et al. (2022). Hypoxia improves endurance performance by enhancing short chain fatty acids production via gut microbiota remodeling. Front. Microbiol. 12, 820691. doi:10.3389/fmicb.2021.820691
Huarancca Reyes, T., Esparza, E., Crestani, G., Limonchi, F., Cruz, R., Salinas, N., et al. (2020). Physiological responses of maca (Lepidium meyenii Walp.) plants to UV radiation in its high-altitude mountain ecosystem. Sci. Rep. 10 (1), 2654. doi:10.1038/s41598-020-59638-4
Hudson, A., Lopez, E., Almalki, A. J., Roe, A. L., and Calderon, A. I. (2018). A review of the toxicity of compounds found in herbal dietary supplements. Planta Medica 84 (9-10), 613–626. doi:10.1055/a-0605-3786
Hung, S. K., Perry, R., and Ernst, E. (2011). The effectiveness and efficacy of Rhodiola rosea L.: a systematic review of randomized clinical trials. Phytomedicine 18 (4), 235–244. doi:10.1016/j.phymed.2010.08.014
Ikeuchi, M., Koyama, T., Takei, S., Kino, T., and Yazawa, K. (2009). Effects of benzylglucosinolate on endurance capacity in mice. J. Health Sci. 55(2), 178–182. doi:10.1248/jhs.55.178
Ivanova Stojcheva, E., and Quintela, J. C. (2022). The effectiveness of Rhodiola rosea L. Preparations in alleviating various aspects of life-stress symptoms and stress-induced conditions-encouraging clinical evidence. Molecules 27 (12), 3902. doi:10.3390/molecules27123902
Jia, J. M., and Wu, C. F. (2008). Antifatigue activity of tissue culture extracts of Saussurea involucrata. Pharm. Biol. 46 (6), 433–436. doi:10.1080/13880200802055909
Jia, Z., Wu, C., Jin, H., and Zhang, J. (2014). Identification of the chemical components of Saussurea involucrata by high-resolution mass spectrometry and the mass spectral trees similarity filter technique. Rapid Commun. Mass Spectrom. 28 (21), 2237–2251. doi:10.1002/rcm.7014
Jing, L., He, L., Fan, P., Jia, Z., and Ma, H. (2016). Chemical constituents of Saussurea involucrata with anti-hypoxia activity. Chem. Nat. Compd. 52 (3), 487–489. doi:10.1007/s10600-016-1681-9
Jowko, E., Sadowski, J., Dlugolecka, B., Gierczuk, D., Opaszowski, B., and Cieslinski, I. (2018). Effects of Rhodiola rosea supplementation on mental performance, physical capacity, and oxidative stress biomarkers in healthy men. J. Sport Health Sci. 7 (4), 473–480. doi:10.1016/j.jshs.2016.05.005
Kamaleeswari, M., and Nalini, N. (2006). Dose-response efficacy of caraway (Carum carvi L.) on tissue lipid peroxidation and antioxidant profile in rat colon carcinogenesis. J. Pharm. Pharmacol. 58 (8), 1121–1130. doi:10.1211/jpp.58.8.0014
Kanliang, H. (2016). Influence of different processing methods on Gymnadenia R.Br anti-fatigue effect and antioxidant activity. Shaanxi J. Traditional Chin. Med. 37 (6), 754–755.
Kappel, V. D., Zanatta, L., Postal, B. G., and Mena Barreto Silva, F. R. (2013). Rutin potentiates calcium uptake via voltage-dependent calcium channel associated with stimulation of glucose uptake in skeletal muscle. Archives Biochem. Biophysics 532 (2), 55–60. doi:10.1016/j.abb.2013.01.008
Kelly, G. S. (2001). Rhodiola rosea: a possible plant adaptogen. Altern. Med. Rev. a J. Clin. Ther. 6 (3), 293–302.
Keshavarz, A., Minaiyan, M., Ghannadi, A., and Mahzouni, P. (2013). Effects of Carum carvi L. (Caraway) extract and essential oil on TNBS-induced colitis in rats. Res. Pharm. Sci. 8 (1), 1–8.
Khanum, F., Bawa, A. S., and Singh, B. (2005). Rhodiola rosea: a versatile adaptogen. Compr. Rev. Food Sci. Food Saf. 4 (3), 55–62. doi:10.1111/j.1541-4337.2005.tb00073.x
Kim, K. J., Jung, Y. S., You, D. M., Lee, S. H., Lee, G., Kwon, K.-B., et al. (2021). Neuroprotective effects of ethanolic extract from dry Rhodiola rosea L. rhizomes. Food Sci. Biotechnol. 30 (2), 287–297. doi:10.1007/s10068-020-00868-7
Kim, N.-H., Moon, P.-D., Pak, S. C., Kim, H.-M., and Jeong, H.-J. (2012). Anti-fatigue effect of zizania caudiflora (turczaninow) nakai. Am. J. Chin. Med. 40 (1), 111–120. doi:10.1142/s0192415x12500097
Kocić-Tanackov, S., Dimić, G., Jakšić, S., Mojović, L., Djukić-Vuković, A., Mladenović, D., et al. (2019). Effects of caraway and juniper essential oils on aflatoxigenic fungi growth and aflatoxins secretion in polenta. J. Food Process. Preserv. 43 (12). doi:10.1111/jfpp.14224
Krueger, D., Schaeuffele, S., Zeller, F., Demir, I. E., Theisen, J., Michel, K., et al. (2020). Peppermint and caraway oils have muscle inhibitory and pro-secretory activity in the human intestine in vitro. Neurogastroenterol. Motil. 32 (2), e13748. doi:10.1111/nmo.13748
Kwak, J. J., Yook, J. S., and Ha, M. S. (2020). Potential biomarkers of peripheral and central fatigue in high-intensity trained athletes at high-temperature: a pilot study with momordica charantia (bitter melon). J. Immunol. Res. 2020, 4768390. doi:10.1155/2020/4768390
Larmo, P., Alin, J., Salminen, E., Kallio, H., and Tahvonen, R. (2008). Effects of sea buckthorn berries on infections and inflammation: a double-blind, randomized, placebo-controlled trial. Eur. J. Clin. Nutr. 62 (9), 1123–1130. doi:10.1038/sj.ejcn.1602831
Lee, J.-C., Kao, J.-Y., Kuo, D.-H., Liao, C.-F., Huang, C.-H., Fan, L.-L., et al. (2011). Antifatigue and antioxidant activity of alcoholic extract from Saussurea involucrata. J. traditional complementary Med. 1 (1), 64–68. doi:10.1016/s2225-4110(16)30058-x
Lee, Y.-K., and Chang, Y. H. (2019). Physicochemical and antioxidant properties of methanol extract from Maca (Lepidium meyenii Walp.) leaves and roots. Food Sci. Technol. 39, 278–286. doi:10.1590/fst.03818
Lee, Y.-K., Jung, S. K., and Chang, Y. H. (2020). Rheological properties of a neutral polysaccharide extracted from maca (Lepidium meyenii Walp.) roots with prebiotic and anti-inflammatory activities. Int. J. Biol. Macromol. 152, 757–765. doi:10.1016/j.ijbiomac.2020.02.307
Lemhadri, A., Hajji, L., Michel, J. B., and Eddouks, M. (2006). Cholesterol and triglycerides lowering activities of caraway fruits in normal and streptozotocin diabetic rats. J. Ethnopharmacol. 106 (3), 321–326. doi:10.1016/j.jep.2006.01.033
León-Velarde, F., Maggiorini, M., Reeves, J. T., Aldashev, A., Asmus, I., Bernardi, L., et al. (2005). Consensus statement on chronic and subacute high altitude diseases. High Alt. Med. Biol. 6 (2), 147–157. doi:10.1089/ham.2005.6.147
Li, J., Liu, H. L., Xia, W. W., Mu, J. Q., Feng, Y. J., Liu, R. N., et al. (2017a). De novo transcriptome sequencing and the hypothetical cold response mode of Saussurea involucrata in extreme cold environments. Int. J. Mol. Sci. 18 (6), 1155. doi:10.3390/ijms18061155
Li, J., Sun, Q., Meng, Q., Wang, L., Xiong, W., and Zhang, L. (2017b). Anti-fatigue activity of polysaccharide fractions from Lepidium meyenii Walp. (maca). Int. J. Biol. Macromol. 95, 1305–1311. doi:10.1016/j.ijbiomac.2016.11.031
Li, W., Zhang, X., Chen, R., Li, Y., Miao, J., Liu, G., et al. (2020). HPLC fingerprint analysis of Phyllanthus emblica ethanol extract and their antioxidant and anti-inflammatory properties. J. Ethnopharmacol. 254, 112740. doi:10.1016/j.jep.2020.112740
Li, Y., Han, J., Chen, Y., Chen, C., Chu, B., and Zhang, Y. (2018a). p-Coumaric acid as a prophylactic measure against normobaric hypoxia induced pulmonary edema in mice. Life Sci. 211, 215–223. doi:10.1016/j.lfs.2018.09.039
Li, Y., Xu, F., Zheng, M., Xi, X., Cui, X., and Han, C. (2018b). Maca polysaccharides: a review of compositions, isolation, therapeutics and prospects. Int. J. Biol. Macromol. 111, 894–902. doi:10.1016/j.ijbiomac.2018.01.059
Li, Z., Zhu, H., Hua, H., Liu, C., Cheng, Y., Guo, Y., et al. (2022). Anti-fatigue activity of Brassica rapa L. extract and correlation among biochemical changes in forced swimming mice. Food Biosci. 47, 101633. doi:10.1016/j.fbio.2022.101633
Liu, G., Xu, Z., Chen, J., Lang, G., Tian, Q., Shen, Y., et al. (2009). On-line strategies for the identification of unknown flavone glycosides in Dracocephalum tanguticum Maxim. J. Chromatogr. B Anal. Technol. Biomed. Life Sci. 877 (24), 2545–2550. doi:10.1016/j.jchromb.2009.06.040
Long, H., Qiu, X., Cao, L., and Han, R. (2021a). Discovery of the signal pathways and major bioactive compounds responsible for the anti-hypoxia effect of Chinese cordyceps. J. Ethnopharmacol. 277, 114215. doi:10.1016/j.jep.2021.114215
Long, H. L., Qiu, X. H., Cao, L., Han, R. C., and Rao, Z. (2021b). Toxicological safety evaluation of the cultivated Chinese cordyceps. J. Ethnopharmacol. 277, 113600. doi:10.1016/j.jep.2020.113600
Luo, H. (2015). Traditional Tibetan medicine in China: an overview of current status and prospects. Chin. J. Integr. Med. 21 (1), 67–70. doi:10.1007/s11655-014-1893-4
Ma, H.-P., Fan, P.-C., Jing, L.-L., Yao, J., He, X.-R., Yang, Y., et al. (2011). Anti-hypoxic activity at simulated high altitude was isolated in petroleum ether extract of Saussurea involucrata. J. Ethnopharmacol. 137 (3), 1510–1515. doi:10.1016/j.jep.2011.08.037
Ma, H. P., Jiao, Y., Gao, R. M., Yang, Y., Fan, P. C., Jing, L. L., et al. (2014). Effect of ethanol extract from Saussurea involucrata on biochemical indicators of simulated high-altitude hypoxia induced mice. Zhong Yao Cai 37 (1), 99–103.
Mahboubi, M. (2019). Caraway as important medicinal plants in management of diseases. Nat. Prod. Bioprospect 9 (1), 1–11. doi:10.1007/s13659-018-0190-x
Meena, H., Singh, K. P., Negi, P. S., and Ahmed, Z. (2013). Sub-acute toxicity of cultured mycelia of Himalayan entomogenous fungus Cordyceps sinensis (Berk.) Sacc. in rats. Indian J. Exp. Biol. 51 (5), 381–387.
Meng, X. H., Wang, M., and Lin, P. C. (2023). Gymnadenia conopsea (L.) R. Br.: comprehensive review of propagation and breeding, traditional uses, chemical composition, pharmacology, quality control, and processing. J. Ethnopharmacol. 306, 116205. doi:10.1016/j.jep.2023.116205
Nunez, D., Olavegoya, P., Gonzales, G. F., and Gonzales-Castaneda, C. (2017). Red maca (Lepidium meyenii), a plant from the Peruvian highlands, promotes skin wound healing at sea level and at high altitude in adult male mice. High Alt. Med. Biol. 18 (4), 372–383. doi:10.1089/ham.2017.0038
Oh, S., Komine, S., Warabi, E., Akiyama, K., Ishii, A., Ishige, K., et al. (2017). Nuclear factor (erythroid derived 2)-like 2 activation increases exercise endurance capacity via redox modulation in skeletal muscles. Sci. Rep. 7, 12902. doi:10.1038/s41598-017-12926-y
Panossian, A., Wikman, G., and Sarris, J. (2010). Rosenroot (Rhodiola rosea): traditional use, chemical composition, pharmacology and clinical efficacy. Phytomedicine 17 (7), 481–493. doi:10.1016/j.phymed.2010.02.002
Paul, S., Geng, C.-A., Yang, T.-H., Yang, Y.-P., and Chen, J.-J. (2019). Phytochemical and health-beneficial progress of turnip (Brassica rapa). J. Food Sci. 84 (1), 19–30. doi:10.1111/1750-3841.14417
Pena, E., El Alam, S., Siques, P., and Brito, J. (2022). Oxidative stress and diseases associated with high-altitude exposure. Antioxidants 11 (2), 267. doi:10.3390/antiox11020267
Qian, E. W., Ge, D. T., and Kong, S. K. (2011). Salidroside promotes erythropoiesis and protects erythroblasts against oxidative stress by up-regulating glutathione peroxidase and thioredoxin. J. Ethnopharmacol. 133 (2), 308–314. doi:10.1016/j.jep.2010.09.025
Rahman, M. S., Jahan, N., Rahman, S. M. A., and Rashid, M. A. (2015). Analgesic and antidepressant activities of Brassica rapa subspecies chinensis (L.) Hanelt on Swiss-albino mice model. Bangladesh Med. Res. Counc. Bull. 41 (3), 114–120. doi:10.3329/bmrcb.v41i3.29886
Ruiz-Luna, A. C., Salazar, S., Aspajo, N. J., Rubio, J., Gasco, M., and Gonzales, G. F. (2005). Lepidium meyenii (Maca) increases litter size in normal adult female mice. Reproductive Biol. Endocrinol. 3, 16. doi:10.1186/1477-7827-3-16
Shang, X., Guo, X., Liu, Y., Pan, H., Miao, X., and Zhang, J. (2017). Gymnadenia conopsea (L.) R. Br.: a systemic review of the ethnobotany, phytochemistry, and pharmacology of an important asian folk medicine. Front. Pharmacol. 8, 24. doi:10.3389/fphar.2017.00024
Showraki, A., Emamghoreishi, M., and Oftadegan, S. (2016). Anticonvulsant effect of the aqueous extract and essential oil of Carum carvi L. Seeds in a pentylenetetrazol model of seizure in mice. Iran. J. Med. Sci. 41 (3), 200–208.
Srirama, R., Kumar, J. U. S., Seethapathy, G. S., Newmaster, S. G., Ragupathy, S., Ganeshaiah, K. N., et al. (2017). Species adulteration in the herbal trade: causes, consequences and mitigation. Drug Saf. 40 (8), 651–661. doi:10.1007/s40264-017-0527-0
Su, K.-Y., Yu, C. Y., Chen, Y.-W., Huang, Y.-T., Chen, C.-T., Wu, H.-F., et al. (2014). Rutin, a flavonoid and principal component of Saussurea involucrata, attenuates physical fatigue in a forced swimming mouse model. Int. J. Med. Sci. 11 (5), 528–537. doi:10.7150/ijms.8220
Suo, Y., Li, Y., Wang, H., Yu, J., and Chen, G. (2005). Effect of fruit of Nitraria tangutorum bobr. From qaidam basin on immunomodulatory, anti-fatigue and cold-tolerance. Nat. Prod. R D 17 (6), 717–721.
Tang, J.-F., Li, W.-X., Zhang, F., Li, Y.-H., Cao, Y.-J., Zhao, Y., et al. (2017). Discrimination of Radix Polygoni Multiflori from different geographical areas by UPLC-QTOF/MS combined with chemometrics. Chin. Med. 12, 34. doi:10.1186/s13020-017-0155-8
Tang, W., Jin, L., Xie, U., Huan, J., Chu, B., Dai, X., et al. (2018). Purification, structural characterization and anti-fatigue effect in vivo of Tibetan Turnip (Brassica rapa L.) polysaccharide. J. Chin. Inst. Food Sci. Technol. 18 (12), 22–31. doi:10.16429/j.1009-7848.2018.12.003
Tang, Y., Hou, Y., Zeng, Y., Hu, Y., Zhang, Y., Wang, X., et al. (2021). Salidroside attenuates CoCl2-simulated hypoxia injury in PC12 cells partly by mitochondrial protection. Eur. J. Pharmacol. 912, 174617. doi:10.1016/j.ejphar.2021.174617
Wang, K., Xu, C., and Li, Z. (2003). Study on effect and mechanism of Cordyceps sinensis on fatigue in mice induced by exercise. J. Harbin Med. Coll. 37 (4), 311–314.
Wang, S. Q., Han, X. Z., Li, X., Ren, D. M., Wang, X. N., and Lou, H. X. (2010a). Flavonoids from Dracocephalum tanguticum and their cardioprotective effects against doxorubicin-induced toxicity in H9c2 cells. Bioorg Med. Chem. Lett. 20 (22), 6411–6415. doi:10.1016/j.bmcl.2010.09.086
Wang, S. Q., Ren, D. M., Xiang, F., Wang, X. N., Zhu, C. J., Yuan, H. Q., et al. (2009). Dracotanosides A-D, spermidine glycosides from Dracocephalum tanguticum: structure and amide rotational barrier. J. Nat. Prod. 72 (6), 1006–1010. doi:10.1021/np900140s
Wang, T. T., Li, C. P., Wei, Y. S., Dong, Z. Y., Meng, F. C., Chen, M., et al. (2024). Dracotangusions A and B, two new sesquiterpenes from Dracocephalum tanguticum Maxim. with anti-inflammatory activity. Nat. Prod. Res., 1–7. doi:10.1080/14786419.2024.2305655
Wang, X. B., Hou, Y., Li, Q. Y., Li, X. H., Wang, W. X., Ai, X. P., et al. (2019). Rhodiola crenulata attenuates apoptosis and mitochondrial energy metabolism disorder in rats with hypobaric hypoxia-induced brain injury by regulating the HIF-1α/microRNA 210/ISCU1/2(COX10) signaling pathway. J. Ethnopharmacol. 241, 111801. doi:10.1016/j.jep.2019.03.028
Wang, Y. S., Huang, R., Li, N. Z., Xu, H. Y., and Yang, J. H. (2010b). Triterpenes from Stachyurus himalaicus var. himalaicus Hook. f. et Thoms. ex Benth. Molecules 15 (4), 2096–2102. doi:10.3390/molecules15042096
Weimin, T., Lu, J., Lianghua, X., Juqing, H., Nan, W., Bingquan, C., et al. (2017). Structural characterization and antifatigue effect in vivo of maca (Lepidium meyenii Walp) polysaccharide. J. Food Sci. 82 (3), 757–764. doi:10.1111/1750-3841.13619
Wirth, K., and Scheibenbogen, C. (2020). A unifying hypothesis of the pathophysiology of myalgic encephalomyelitis/chronic fatigue syndrome (ME/CFS): recognitions from the finding of autoantibodies against ß2-adrenergic receptors. Autoimmun. Rev. 19 (6), 102527. doi:10.1016/j.autrev.2020.102527
Wu, Q., Er-Bu, A., Liang, X., He, C., Yin, L., Xu, F., et al. (2023). Isolation, structure identification, and immunostimulatory effects in vitro and in vivo of polysaccharides from Onosma hookeri Clarke var. longiforum Duthie. J. Sci. Food Agric. 103 (1), 328–338. doi:10.1002/jsfa.12145
Wu, Q., Er-bu, A., Liang, X., Luan, S., He, C., Yin, L., et al. (2020b). Determination of the main naphthoquinones in Onosma hookeri Clarke. var. longiforum Duthie and its optimization of the ultrasound-assisted extraction using response surface methodology. J. Food Sci. 86 (2), 357–365. doi:10.1111/1750-3841.15460
Wu, Q., Er-Bu, A., Liang, X., Luan, S., Wang, Y., Yin, Z., et al. (2020a). Evaluation of antioxidant and anticancer activities of naphthoquinones-enriched ethanol extracts from the roots of Onosma hookeri Clarke. var. longiforum Duthie. Food Sci. Nutr. 8 (8), 4320–4329. doi:10.1002/fsn3.1729
Wu, R. M., Sun, Y. Y., Zhou, T. T., Zhu, Z. Y., Zhuang, J. J., Tang, X., et al. (2014). Arctigenin enhances swimming endurance of sedentary rats partially by regulation of antioxidant pathways. Acta Pharmacol. Sin. 35 (10), 1274–1284. doi:10.1038/aps.2014.70
Xue, H., and Kang, H. (2020). Rhodiola rosea extract improves the antioxidant capacity of high-intensity treadmill mice. Genomics Appl. Biol. 39 (2), 955–960.
Yan, L. S., Cheng, B. C., Zhang, S. F., Luo, G., Zhang, C., Wang, Q. G., et al. (2021). Tibetan medicine for diabetes mellitus: overview of pharmacological perspectives. Front. Pharmacol. 12, 748500. doi:10.3389/fphar.2021.748500
Yang, S., Liu, H., Huang, X., Zhan, L., Luo, P., Xue, J., et al. (2018). The metabolism and distribution of imidazole alkaloids from Lepidium meyenii (Maca) in mouse by matrix-assisted laser desorption/ionization mass spectrometry imaging. Int. J. Mass Spectrom. 434, 93–99. doi:10.1016/j.ijms.2018.09.014
Yin, X., Wang, Q., Chen, Q., Xiang, N., Yang, Y., and Yang, Y. (2017). Genome-wide identification and functional analysis of the calcineurin B-like protein and calcineurin B-like protein-interacting protein kinase gene families in turnip (Brassica rapa var. rapa). Front. Plant Sci. 8, 1191. doi:10.3389/fpls.2017.01191
Yu, Z., Jin, W., Cui, Y., Ao, M., Liu, H., Xu, H., et al. (2019). Protective effects of macamides from Lepidium meyenii Walp. against corticosterone-induced neurotoxicity in PC12 cells. Rsc Adv. 9 (40), 23096–23108. doi:10.1039/c9ra03268a
Zeng, Q., Chang, R., Qin, J., Cheng, X., Zhang, W., and Jin, H. (2011). New glycosides from Dracocephalum tanguticum maxim. Arch. Pharm. Res. 34 (12), 2015–2020. doi:10.1007/s12272-011-1202-0
Zhang, G., Ling, J., and Cui, Z. (2007). Supercritical CO2 extraction of essential oil from Dracocephalum tanguticum max-im and analysis by GC-MS. J. Liq. Chromatogr. Relat. Technol. 30 (2), 287–292. doi:10.1080/10826070601064607
Zhang, Z. J., Tong, Y., Zou, J., Chen, P. J., and Yu, D. H. (2009). Dietary supplement with a combination of Rhodiola crenulata and ginkgo biloba enhances the endurance performance in healthy volunteers. Chin. J. Integr. Med. 15 (3), 177–183. doi:10.1007/s11655-009-0177-x
Zhao, W. J., Zhang, W. Y., Liu, L., Cheng, Y. L., Guo, Y. H., Yao, W. R., et al. (2021b). Fractionation, characterization and anti-fatigue activity of polysaccharides from Brassica rapa L. Process Biochem. 106, 163–175. doi:10.1016/j.procbio.2021.04.016
Zhao, Z., Guo, P., and Brand, E. (2012). The formation of daodi medicinal materials. J. Ethnopharmacol. 140 (3), 476–481. doi:10.1016/j.jep.2012.01.048
Zheng, R. L., Liu, G. S., Xing, G. X., Jia, Z. J., Du, M., and Tan, L. Q. (1993). FREE-RADICAL SCAVENGING AND ANTIFATIGUE ACTIVITIES OF SAUSSUREA-INVOLUCRATA POLYSACCHARIDES. Acta Pharmacol. Sin. 14, S47–S49.
Zhou, S. S., and Jiang, J. G. (2019). Anti-fatigue effects of active ingredients from traditional Chinese medicine: a review. Curr. Med. Chem. 26 (10), 1833–1848. doi:10.2174/0929867324666170414164607
Zhou, Z., Liu, Y., and Guo, S.Suolangouzhu (2021). Anti-inflammatory effects and mechanism of Onosma hookeri ethanol extract. Chin. Tradit. Pat. Med. 43 (11), 3002–3007.
Zhu, H., Hu, B., Hua, H., Liu, C., Cheng, Y., Guo, Y., et al. (2020). Macamides: a review of structures, isolation, therapeutics and prospects. Food Res. Int. 138 (Pt B), 109819. doi:10.1016/j.foodres.2020.109819
Zhu, H., Liu, C., and Qian, H. (2022a). Pharmaceutical potential of high-altitude plants for fatigue-related disorders: a review. Plants-Basel 11 (15), 2004. doi:10.3390/plants11152004
Zhu, H., Yang, Y., Li, Z., Wang, X., and Qian, H. (2022b). An integrated network pharmacology and metabolomics approach to reveal the immunomodulatory mechanism of Brassica rapa L. (Tibetan Turnip) in fatigue mice. Food & Funct. 13 (21), 11097–11110. doi:10.1039/d2fo02308c
Zhu, H. K., Xu, W. Q., Wang, N., Jiang, W. H., Cheng, Y. L., Guo, Y. H., et al. (2021). Anti-fatigue effect of Lepidium meyenii Walp. (Maca) on preventing mitochondria-mediated muscle damage and oxidative stress in vivo and vitro. Food & Funct. 12 (7), 3132–3141. doi:10.1039/d1fo00383f
Keywords: traditional Tibetan medicine, geo-authentic medicinal materials, pharmacology, human health, medicine and food homology
Citation: Wang N and Zhu H (2024) Geo-authentic Tibetan medicine: a traditional pharmacological resource for promoting human health and wellness. Front. Pharmacol. 15:1432221. doi: 10.3389/fphar.2024.1432221
Received: 13 May 2024; Accepted: 11 June 2024;
Published: 23 July 2024.
Edited by:
Miguel Angel Prieto Lage, University of Vigo, SpainReviewed by:
Paula Barciela, University of Vigo, SpainPauline Donn, University of Yaounde I, Cameroon
Ana Pérez Vázquez, University of Vigo, Spain
Copyright © 2024 Wang and Zhu. This is an open-access article distributed under the terms of the Creative Commons Attribution License (CC BY). The use, distribution or reproduction in other forums is permitted, provided the original author(s) and the copyright owner(s) are credited and that the original publication in this journal is cited, in accordance with accepted academic practice. No use, distribution or reproduction is permitted which does not comply with these terms.
*Correspondence: Hongkang Zhu, NzIxMDExMjExOEBzdHUuamlhbmduYW4uZWR1LmNu