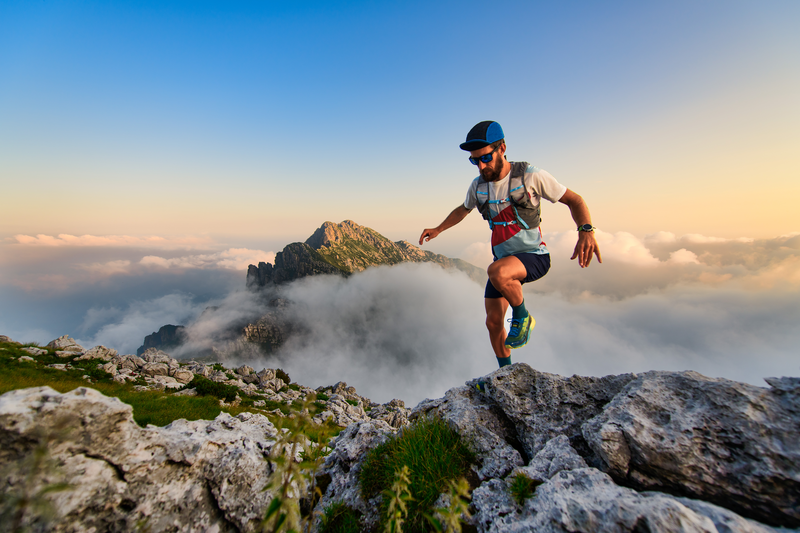
94% of researchers rate our articles as excellent or good
Learn more about the work of our research integrity team to safeguard the quality of each article we publish.
Find out more
REVIEW article
Front. Pharmacol. , 08 November 2024
Sec. Pharmacology of Anti-Cancer Drugs
Volume 15 - 2024 | https://doi.org/10.3389/fphar.2024.1431581
This article is part of the Research Topic Exploring the Role of Exosomes in Cancer Metastasis View all articles
In recent years, the incidence of thyroid cancer has surged globally, posing significant challenges in its diagnosis, treatment, and prognosis. Exosomes, as a class of extracellular vesicles, are secreted by nearly all cell types and encapsulate a variety of nucleic acids and proteins reflective of their cell of origin, thereby facilitating critical intercellular communication. Recent advancements in understanding these exosomes have catalyzed their application in oncology, particularly through uncovering their roles in the pathogenesis, diagnosis, and therapy of cancers. Notably, the latest literature highlights the integral role of exosomes in refining diagnostic techniques, enhancing targeted therapies, optimizing radiotherapy outcomes, and advancing immunotherapeutic approaches in thyroid cancer management. This review provides a current synthesis of the implications of exosomes in thyroid cancer tumorigenesis and progression, as well as their emerging applications in diagnosis and treatment strategies. Furthermore, we discuss the profound clinical potential of exosome-based interventions in managing thyroid cancer, serving as a foundational reference for future therapeutic developments.
In the past decade, thyroid cancer (TC) has emerged as the most prevalent endocrine malignancy, accounting for approximately 3.8% of all newly diagnosed cancers worldwide (Pavlidis et al., 2021). This alarming rise underscores the critical need for innovative management strategies to enhance patient outcomes. Exosomes, nano-sized extracellular vesicles secreted by various cell types, play a fundamental role in intercellular communication by transmitting bioactive molecules, thereby reflecting the molecular signature of their cells of origin. Extensive studies across multiple cancer types, including breast cancer (da Fonseca Alves et al., 2024), lung cancer (Li M. Y. et al., 2021), liver cancer (Wang H. et al., 2019), colorectal cancer (Chen C. et al., 2022), prostate cancer (Lorenc et al., 2020), and pancreatic cancer (Fang et al., 2023), have elucidated the pivotal role of exosomes in tumorigenesis and disease progression. Moreover, recent advancements have demonstrated the significant potential of exosome-based clinical applications, including cancer diagnosis (Zhong et al., 2024), monitoring (Li S. et al., 2023), and therapeutic interventions (Yue et al., 2023; Si et al., 2024). Specifically in thyroid cancer, studies have demonstrated that exosomes derived from tumor cells facilitate both the progression and the metastasis (Feng et al., 2020). Further exploration of exosome-mediated mechanisms may reveal novel insights into thyroid cancer pathophysiology (Wang et al., 2024). Additionally, the non-invasive nature and high specificity of exosome-based diagnostic and therapeutic strategies hold promise for revolutionizing the management of thyroid cancer, potentially ushering in a new era of precision medicine (Figure 1).
Differentiated thyroid cancer (DTC), which originates from thyroid follicular epithelial cells, accounts for over 95% of all cases (Uludağ et al., 2018). This category primarily includes papillary thyroid cancer (PTC) and follicular thyroid cancer (FTC). PTC, the most prevalent subtype, is characterized by its indolent behavior, favorable prognosis, and a propensity for regional lymphatic spread, particularly to the cervical lymph nodes, with less frequent metastasis to distant sites such as the lungs and bones (Schlumberger and Leboulleux, 2021). In contrast, poorly differentiated thyroid cancer (PDTC) and the Hürthle cell variant, which are categorized as high-risk, generally present with poorer outcomes (Cabanillas et al., 2016; Houten et al., 2022). Anaplastic thyroid cancer (ATC), although constituting less than 1% of all thyroid cancers, is the most lethal form, exhibiting an extremely aggressive nature and rapid disease progression. ATC commonly infiltrates adjacent neck structures and metastasizes to distant organs, leading to a median survival of merely 3 months (Lin et al., 2019). Medullary thyroid carcinoma (MTC), which accounts for 1%–2% of all thyroid cancers, originates from parafollicular C cells and is distinguished by its high metastatic potential and poor prognosis, often identified through elevated serum calcitonin levels (Okafor et al., 2021; Kaliszewski et al., 2022).
The primary diagnostic modalities for DTC include ultrasound and fine-needle aspiration (FNA), which are further supported by cytological evaluations (Haugen et al., 2016; Filetti et al., 2019). Additionally, biochemical assays and genetic screenings, such as those detecting BRAF and TERT mutations, play a crucial role in refining diagnosis and prognostication (Houten et al., 2022). ATC, known for its rapid progression, requires swift histopathological evaluation, frequently complemented by immunohistochemistry to confirm diagnoses. The diagnostic approach for MTC integrates imaging modalities, serum biochemical markers, and genetic testing, highlighting the pivotal role of early and accurate detection in enhancing patient outcomes (Wells Jr et al., 2015; Filetti et al., 2019) (Figure 2). Given the non-invasive and convenient nature of peripheral blood assays, they are increasingly recognized as viable options for continuous monitoring of thyroid cancer. Consequently, the investigation of biomarkers in peripheral blood, particularly exosomal markers, is gaining prominence, reflecting their crucial role in both diagnosing TC and monitoring patient progress.
The clinical management of DTC typically involves a combination of surgery, radioactive iodine (RAI) therapy, and TSH suppression therapy to minimize the risk of recurrence (Haugen et al., 2016). In contrast, ATC and MTC, known for their rapid progression and high recurrence rates, necessitate more aggressive treatment strategies. These include extensive surgery, radiation, systemic therapies, and the incorporation of emerging modalities such as immunotherapy (Dierks et al., 2021). Given the resistance and recurrence of ATC and MTC, identifying innovative therapeutic strategies is crucial for improving survival. Exosomes, extracellular vesicles from cells, are central in tumorigenesis, progression, and resistance within the tumor microenvironment. Their role in these processes marks them as key targets for novel therapies, especially as vehicles for targeted drug delivery. In the following sections, we will explore these aspects of exosome function and their therapeutic potential in detail.
Diagnostic procedures for TC encompass a range of modalities, including ultrasonography, CT/MRI imaging, FNA, and circulating blood tests. Traditional approaches to managing TC predominantly involve a combination of surgical intervention, localized radiation using RAI, therapeutic strategies to suppress TSH, chemotherapy, and targeted therapy. US, ultrasonography; FNA, fine-needle aspiration; T3, triiodothyronine; T4, thyroxine; Tg, thyroglobulin; TPO, thyroid peroxidase; TSH, thyroid stimulating hormone; CEA, carcinoembryonic antigen; RAI, radio-active iodine.
Over the past four decades, since their initial identification by Pan and Johnstone (1983), Johnstone et al., (1987), exosomes have been recognized to originate from a diverse array of cell types including reticulocytes (Pan and Johnstone, 1983), immunocytes (Li et al., 2019), neurons (Jiang D. et al., 2020) and tumor cells (Hardin et al., 2018; Dai D. et al., 2020; Wang Y. et al., 2020). These extracellular vesicles can be isolated from various bodily fluids such as serum (Dai W. et al., 2020; Rao et al., 2020), urine (Huang et al., 2020), saliva (Nik Mohamed Kamal et al., 2020), semen (Su et al., 2021), and cerebrospinal fluid (Jia et al., 2019). Characterized by their distinctive cup-like morphology when visualized through transmission electron microscopy, exosomes are nanoparticles typically ranging in diameter from 40 to 150 nm (Zhang et al., 2020; Wang D. et al., 2022). Their identification can be confirmed through Western blot analysis using specific protein markers, such as CD9, CD81, CD63, TSG101, and Alix (Kalluri and LeBleu, 2020). As a subclass of the larger extracellular vesicle family, exosomes are distinguished from other types, including microvesicles, microparticles, and apoptotic bodies, based on their size, biogenesis, and molecular composition (Zhang et al., 2021) (Figure 3).
Figure 3. Schematic illustration of exosome biogenesis, identification, and roles in tumorigenesis, diagnosis, and therapy. This diagram differentiates various types of extracellular vesicles by size and secretion pathways: membrane particles (50–600 nm), microvesicles (100–1,000 nm) originating from the cell surface, and apoptotic bodies (1,000–5,000 nm) from apoptotic cells. Exosomes, derived from multivesicular bodies (MVBs), are depicted as nanoparticles with an average diameter of 40–150 nm. They contain nucleic acids, proteins, lipids, and metabolites, playing crucial roles in intercellular communication. Exosomes influence the tumor microenvironment, aid in tumor formation, and are utilized in blood or urine for early tumor diagnosis. Additionally, they serve as vehicles for targeted cancer therapy.
Exosome biogenesis is initiated through the formation of early-sorting endosomes (ESEs), which arise via endocytic uptake of extracellular substances and involve contributions from the Golgi apparatus and endoplasmic reticulum. These ESEs mature into late-sorting endosomes (LSEs) and subsequently develop into multivesicular bodies (MVBs) containing intraluminal vesicles (ILVs) (Minciacchi et al., 2015; Al-Sowayan et al., 2020). During the maturation process of MVBs, specific proteins, nucleic acids, and lipids are selectively incorporated into ILVs, which are then released into the extracellular space as exosomes upon fusion of MVBs with the plasma membrane. Alternatively, MVBs may be targeted for degradation through lysosomal pathways or recycled back to the plasma membrane, depending on cellular requirements (Figure 3).
Initially considered mere cellular waste, exosomes have since been recognized for their crucial role in cell-to-cell communication (Harding and Stahl, 1983; Zhang et al., 2019). These phospholipid bilayer-enclosed nanoparticles contain a diverse array of biomolecules, including proteins, nucleic acids, RNAs, DNAs, amino acids, and metabolites, all of which can elicit specific regulatory effects on recipient cells (Zhang et al., 2016). Numerous proteins involved in exosome biogenesis also serve as markers for these vesicles (Kalluri and LeBleu, 2020). Recent research has further elucidated the critical role of exosomes in tumorigenesis and their potential to refine diagnostic and therapeutic strategies in oncology (Xu et al., 2020; Glass and Coffey, 2022; Zhou et al., 2022) (Figure 3).
Exosome isolation utilizes a variety of techniques, including ultracentrifugation, immunoaffinity capture, ultrafiltration, and polymer-based precipitation, however, no single method can simultaneously achieve high yield and purity (Yang X. X. et al., 2019). Ultracentrifugation, a conventional method involving prolonged spinning at 100,000 g, is often contaminated and limited in throughput (Herroon et al., 2019). Alternative methods, including polymer-based precipitation and density-gradient ultracentrifugation, face similar challenges in yield and purity (Gardiner et al., 2016; Konoshenko et al., 2018). Despite the availability of alternative methods like polymer-based precipitation, density-gradient ultracentrifugation, and immunoprecipitation, these extraction techniques continue to grapple with diminishing yields and purity challenges. Moreover, distinguishing exosomes from other extracellular vesicle proves challenging due to overlapping properties (Cocozza et al., 2020). Therefore, in this review, we use the term “exosome” interchangeably with “extracellular vesicles” as per International Society for Extracellular Vesicles (ISEV) guidelines (Théry et al., 2018).
Recent research has increasingly focused on the role of exosomes in modulating physiological and pathological processes in cancer, including thyroid cancer (Zhang and Yu, 2019). In TC patients, a significant presence of exosomes in tissues and blood indicates their critical role in carcinogenesis. Originating from thyroid cancer cells, these exosomes facilitate tumor development, progression, metastasis, and radioiodine resistance by transferring their contents to other cells (Li Q. et al., 2021) (Figure 4). Broadly, the exosomal mechanisms underlying TC development encompass several key components, as illustrated in Table 1. Research into exosomes in thyroid cancer development is poised to facilitate the identification of novel therapeutic targets, thereby offering promising avenues for more effective treatments.
Figure 4. Exosomal mechanisms in thyroid cancer development. This illustration delineates how exosomes derived from thyroid cancer cells promote various oncogenic processes. These include orchestrating angiogenesis, facilitating the epithelial-to-mesenchymal transition, and contributing to tumor growth, invasion, and metastasis. Additionally, exosomes play roles in immunosuppression and in the development of resistance to iodine-131 radioiodine treatment.
The epithelial-mesenchymal transition (EMT) plays a critical role in cancer initiation and progression, driving the phenotypic transformation of epithelial cells along the epithelial-mesenchymal spectrum (Zhang and Weinberg, 2018). TC cells release various cytokines into the tumor microenvironment (TME), inducing EMT in thyroid follicular epithelial cells and promoting extrathyroidal extension and distant metastasis (Puppin et al., 2008; Ma et al., 2014; Sponziello et al., 2016; Jarboe et al., 2021). Recent studies highlight exosomes and their cargoes as key mediators in this process, with potential as therapeutic targets to inhibit TC progression by regulating EMT (Kim et al., 2020). Lin et al. discovered that exosomal circ007293 triggers EMT and enhances invasiveness in PTC cells via the miR-653-5p/PAX6 axis (Lin et al., 2021). Similarly, Luo and colleagues linked exosomal proteins like SRC, TLN1, ITGB2, and CAPNS1 to EMT in TC patients with lymph node metastasis (LNM) (Luo et al., 2018). Furthermore, exosomal annexin A1 has been shown to stimulate EMT, proliferation, and invasion, contributing to the malignant transformation of thyroid cells (Li Q. et al., 2021). Additionally, cancer stem-like cells (CSCs), known for their self-renewal and asymmetric division capabilities, have been implicated in tumorigenesis and metastasis (Tang et al., 2016; Babaei et al., 2021). Recently, Wu and colleagues demonstrated that exosomal CDKN2B-AS1 from CSCs influences EMT and TGFβ/Smad signaling in human PTC and ATC cell lines, thereby promoting TC growth and metastasis (Wu et al., 2022). Hardin et al. further revealed that CSC-derived exosomes could induce EMT in non-cancerous thyroid cells via the transfer of linc-ROR, offering insights into potential therapeutic strategies targeting CSCs and their exosomes to combat aggressive TC (Hardin et al., 2018). These findings underscore the promise of exosome-based therapies in mitigating EMT-related alterations and reducing TC invasion and metastasis.
Angiogenesis plays a critical role in oncogenesis by expanding blood vessels that supply nutrients and remove wastes, thus facilitating malignant tumor growth (Rajabi et al., 2019). Studies have shown that exosomes secreted by cancer cells can enhance angiogenesis, supporting the progression of various tumors (Kosaka et al., 2013; Hsu et al., 2017; Du et al., 2020; Shang et al., 2020). Specifically, in thyroid cancer, angiogenesis is crucial for tumor progression, with DTC tissues exhibiting higher vascular densities compared to adjacent non-tumor tissues (Akslen and Livolsi, 2000; Ramsden, 2000; Rajabi et al., 2019). Research by Wu et al. highlighted increased exosomal miR-21-5p levels in the blood of PTC patients, which enhances angiogenesis in human umbilical vein endothelial cells (HUVECs) through the miR-21-5p/TGFβ and miR-21-5p/COL4α pathways under hypoxic conditions (Wu et al., 2019). Likewise, Wang’s research demonstrated that under hypoxia, exosomal miR-181a from PTC cells significantly increases cell proliferation, migration, and angiogenesis in HUVECs, ultimately leading to enhanced angiogenesis and tumor growth in nude mice (Wang et al., 2021). Liu et al. further reported that exosomal FGD5-AS1 influences angiogenesis and vascular permeability by modulating the miR-6838-5p/VAV2 axis in endothelial cells, pushing forward TC development and providing a new target for diagnosis and therapy (Liu et al., 2022). These findings suggest that targeting exosome-mediated angiogenesis might offer effective therapeutic strategies for thyroid cancer.
Over time, tumor cells gain the ability to infiltrate and interact with neighboring tissues, culminating in metastasis (Hardin et al., 2018). Hoshino et al. demonstrated that exosomes from tumors are internalized by organ-specific cells, playing a crucial role in metastasis (Hoshino et al., 2015). Recently, the promotion of TC invasiveness and metastasis by exosomes has been increasingly documented (Feng et al., 2020; Wang Y. et al., 2020).
Studies have demonstrated that the expression level of ANXA1 in exosomes derived from 8305C cells is 3.4 times higher than in exosomes from FTC cells and 3.5 times higher than in exosomes from normal cells (Surman et al., 2024). Moreover, elevated levels of ANXA1 in ATC-derived exosomes have been shown to promote the proliferation and invasion of thyroid follicular epithelial cells, contributing to their malignant transformation (Li Q. et al., 2021). Similarly, exosomal EGFR in PTC is linked to increased tumor aggressiveness (Al-Abdallah et al., 2023), and CSC-derived exosomes containing lncRNA DOCK9-AS2 and CDKN2B-AS1 are implicated in advancing thyroid cancer malignancy (Dai W. et al., 2020; Wu et al., 2023). Functional assays reveal that overexpression of exosomal miRNA423-5p increases PTC cell migration and invasion, while its silencing reduces these processes (Ye et al., 2019). Jiang’s research further identifies miR-146b-5p and miR-222-3p in exosomes as enhancers of PTC cell motility (Jiang K. et al., 2020), whereas Lin et al. found that exosomal circ007293 promotes invasiveness via the miR-653-5p/PAX6 axis (Lin et al., 2021). Conversely, exosomal DIO3OS and miR-655-3p were found to inhibit PTC cell proliferation and motility (Qiao et al., 2022; Wang Y. et al., 2022).
Lymph node metastasis (LNM) is a critical marker of malignancy severity in TC, associated with higher recurrence rates and worse outcomes (Nishino and Jacob, 2020). Exosomes play a key role in lymphangiogenesis and lymphatic network restructuring, facilitating LNM (Sun et al., 2019; Wang S. H. et al., 2019; Zhou et al., 2019). Specific exosomal miRNAs correlate with tumor size and LNM occurrence (Dai D. et al., 2020), and miR-6774-3p and miR-6879-5p serve as potential biomarkers for LNM diagnosis in PTC (Chen W. et al., 2022). Furthermore, Jiang’s investigation encompassed 49 PTC patients with LNM and 15 without LNM, revealing upregulated expression of exosomal miR-222-3p and miR-146b-5p in PTC patients with LNM (Jiang K. et al., 2020). Regarding exosome-derived proteins, Luo et al. postulated that integrin-associated proteins within serum-purified exosomes from PTC patients may facilitate the creation of premetastatic niches and contribute to subsequent LNM (Luo et al., 2018). These findings collectively underscore the significant role of exosomes in mediating the process of LNM in TC.
For ATC, distant metastasis to the lung or brain is common (Abe and Lam, 2021), with exosomes facilitating intercellular communication crucial for poor prognosis. Wu et al. examined the impact of CSCs-derived exosomal CDKN2B-AS1 on TC, utilizing mouse models to establish tumor formation and metastasis scenarios. Notably, observation of metastasis loci in the lung underscored the significant role of exosomal CDKN2B-AS1 in driving TC distant metastasis (Wu et al., 2022). Similarly, Liu et al. reported that exosomal lncRNAs like FGD5-AS1 induce tumor angiogenesis and support both local and distant metastatic niches (Liu et al., 2022). Additionally, research demonstrated that miR-519e-5p, harbored within exosomes from PTC, is transferred to recipient CD8+ T cells and aid in tumor immune escape in distant organs (Li G. et al., 2023).
These insights underline the complex role of exosomes in TC progression and metastasis, highlighting their potential as targets for therapeutic intervention.
While the prognosis for most PTC patients is favorable, recurrence, especially in advanced stages and aggressive phenotypes, remains a challenge (Lundgren et al., 2006; Aragon Han et al., 2015). Exosomes serve as a valuable model for studying tumor recurrence and predicting associated risks (Soeda et al., 2019; Zhu et al., 2021). Regarding exosome-related TC recurrence, Wen et al. reported that among patients with low serum exosomal miR-29a levels, 25 out of 65 experienced PTC recurrence, indicating a higher risk associated with lower miR-29a levels (Wen et al., 2021). Additionally, Lee and colleagues identified overexpression of miR-146b and miR-222 in PTC cell-derived exosomes, proposing them as potential biomarkers for recurrence (Lee et al., 2015).
In addition to TC recurrence, another critical consideration is the emergence of RAIR cases. A subset of cases displays progressive behaviors, ultimately leading to the development of RAIR PTC. Remarkably, Li et al. have documented that the presence of circulating exosomal miR-1296-5p might play a contributory role in the pathogenesis of RAIR PTC by specifically targeting the Na+/I− symporter (Li et al., 2022).
Tumor-derived exosomes can significantly impair anti-tumor immunity, thereby promoting tumor progression. For example, cancer cell-released exosomes have been shown to inhibit the function of NK cells (Liu et al., 2006) and stimulate tumor-promoting responses via interactions with B cells (Pucci et al., 2016). They also facilitate immune evasion by impairing T cell function through exosome-mediated PD-L1 expression (Kim et al., 2019) and promote the expansion of myeloid-derived suppressor cells at both primary and metastatic sites (Chalmin et al., 2010). Among cancers, TC is notably immunogenic (Zhu et al., 2018; Chakladar et al., 2019). Zhu et al. found that stimulating NK cells with IL-15 increases exosome production, enhancing their potential for immunotherapy in various cancers, including thyroid cancer (Zhu et al., 2018). Additionally, Liu demonstrated that miR-374a/b-5p inhibits the growth of PTC cells by blocking the exosome-induced M2 macrophage polarization mediated by ANXA1 (Liu, 2024). Similarly, Qiao et al. showed that exosomal miR-655-3p suppresses cell growth, invasion, and M2 macrophage polarization in PTC by targeting CXCR4 (Qiao et al., 2022). These findings underscore the potential of leveraging exosomes as a novel delivery platform to advance immunotherapy for thyroid carcinoma, highlighting ongoing research efforts to harness this capability.
The primary diagnostic methods for TC include imaging and serum TSH measurement, followed by ultrasound-guided FNA, which, despite its invasiveness, is limited to diagnosis without aiding in therapy monitoring. Additionally, neck ultrasound for LNM detection reveals only half of the nodes identified during surgery. Although tissue biopsies offer high diagnostic accuracy, their invasiveness constrains their further use. Liquid biopsy, incorporating circulating tumor cells (CTCs), tumor DNA (ctDNA), cell-free DNA (cfDNA), and RNA (cfRNA), provides a non-invasive alternative for both diagnosing and monitoring cancer (Khatami and Tavangar, 2018; Rappa et al., 2019). Recent studies underscore the significant potential of exosomes as fluid biopsy tools in various cancers (Hesari et al., 2018; Huang et al., 2019; Yang et al., 2019a). For TC, experimental evidence also increasingly underscores the potential of exosomes as effective fluid biopsy tools (Table 2). The bilayer protection of circulating exosomes offers substantial advantages over CTCs and ctDNA in stability and integrity in the bloodstream (Junqueira-Neto et al., 2019). Furthermore, exosomal microRNAs, circular RNAs, and long non-coding RNAs are increasingly recognized for their critical roles in diagnosing TC, enhancing the accuracy and non-invasiveness of current diagnostic approaches.
Exosomes are increasingly recognized for their stability as RNA carriers, offering a more reliable biomarker source than circulating plasma RNAs for disease diagnosis. They maintain RNA integrity even when stored at −20°C, proving essential for long-term studies (Sanz-Rubio et al., 2018). Recent advances show that exosomal miRNAs are potent diagnostic markers for TC, particularly PTC. For instance, Wen et al. found that low levels of serum exosomal miR-29a correlate with a higher recurrence risk in PTC, suggesting its dual utility in diagnosis and prognosis (Wen et al., 2021). Similarly, Dai et al. and Li et al. have highlighted the diagnostic relevance of miR-485-3p and miR-519e-5p, respectively, with the former aiding in risk stratification among PTC patients (Dai D. et al., 2020; Li G. et al., 2023). Further differentiation between PTC and FTC has been achieved through miRNA profiling, revealing distinct patterns of miR-21-5p, miR-221-3p, and miR-181a, achieving 100% sensitivity and 77% specificity (Samsonov et al., 2016). Boufraqech et al.'s work suggests that decreased exosomal miR-145 could serve as a diagnostic tool for cases with inconclusive FNABs (Boufraqech et al., 2014). More precisely, enhanced diagnostic accuracy is reported with miRNA panels, such as those identified by Liang et al., which differentiate PTC from benign thyroid nodules. Liang et al. identified a panel comprising miR-16-2-3p, miR-223-5p, miR-101-3p, and miR-34c-5p, which proves valuable in distinguishing PTC from thyroid nodules (Liang et al., 2020). Meanwhile, Pan et al. suggested the amalgamation of miR-5189-3p and miR-5010-3p within plasma exosomes as prospective biomarkers for diagnosing thyroid nodules (Pan et al., 2020). Additionally, two other miRNAs enriched in plasma exosomes, miR-146b-5p and miR-21a-5p, exhibited notable differential abundance between patients with PTC and those with benign conditions (Delcorte et al., 2022a). Correspondingly, Wang and colleagues identified the upregulation of miR-346, miR-10a-5p, and miR-34a-5p in PTC plasma exosomes (Wang Z. et al., 2019). These findings underscore the significant clinical potential of miRNA panel for TC diagnosis. For LNM, exosomal liquid biopsy is also advantageous for safety, early diagnosis, and dynamic monitoring. Jiang’s study, for example, demonstrated the potential of exosomal miR-146b-5p and miR-222-3p in predicting LNM in PTC (Jiang K. et al., 2020), while other studies have identified novel miRNAs like miR-6774-3p and miR-6879-5p as potential LNM biomarkers in PTC (Chen W. et al., 2022).
Circular RNAs (circRNAs), a subclass of non-coding RNAs with a closed loop structure, are protected within exosomes and have also been identified as promising biomarkers for thyroid cancer diagnosis (Yao and Zhang, 2022). Peng et al. demonstrated that circTACC2 and circBIRC6 were significantly elevated in the serum exosomes of patients with PTC accompanied by LNM (Peng et al., 2024). Similarly, Yang et al. observed altered expressions of circRNAs in serum exosomes from PTC patients, highlighting three specific circRNAs (circ-007293, circ-031752, and circ-020135), with unique patterns that could distinguish PTC cases (Yang et al., 2019b). Additionally, the levels of hsa_circ_0082002 and hsa_circ_0003863 were found to be elevated in PTC patients, correlating positively with clinical indicators such as LNM and vascular invasion, suggesting their potential to assess disease severity (Dai et al., 2023).
Beyond circRNAs, exosomal long non-coding RNAs (lncRNAs) are crucial in TC intercellular communication. Dai et al. reported the upregulation of lncRNA DOCK9-AS2 in PTC, with significant increases found in patients’ plasma exosomes, indicating its relevance in PTC pathology (Dai W. et al., 2020). Furthermore, Liu et al. suggested that elevated levels of lncRNA FGD5-AS1 in blood-derived exosomes might influence TC prognosis and serve as a new diagnostic reference (Liu et al., 2022).
Exosomal proteins have also shown promise in diagnosing TC. A study by Bavisotto et al. revealed that circulating exosomal proteins such as Hsp27, Hsp60, and Hsp90 can effectively distinguish PTC from benign goiter, underscoring their potential as diagnostic biomarkers (Caruso Bavisotto et al., 2019). Additionally, analyses identified activated integrin signaling-related proteins such as TLN1, ITGB2, SRC, and CAPNS1 in serum-purified exosomes from PTC patients with LNM (Luo et al., 2018). In pediatric TC, elevated levels of PD-1 and PD-L1 on exosomes have been associated with clinical outcomes, indicating their diagnostic value (Wang G. et al., 2020). Interestingly, beyond the existence of exosomal proteins in the bloodstream, urinary exosomal thyroglobulin has emerged as a potential early prognostic biomarker for individuals afflicted with PTC (Huang et al., 2020). This innovative finding led to the publication of a patent in Japan in 2017, with further validation from an ongoing study recruiting a larger cohort. Moreover, Wang et al. also reported that urinary exosomal TIMP and Angiotensin-1 are significant markers linked to LNM, corroborated by pathological reports (Wang C. Y. et al., 2022).
These investigations underscore the growing utility of exosomal proteins in TC diagnostics. With advancements in nanotechnologies and single extracellular vesicle analysis, there is optimism that these methods will enhance the identification and utilization of dynamic biomarkers with high accuracy and sensitivity for TC (Ferguson et al., 2022).
Recent evidence underscores the significant role of exosomes in the development and progression of TC, highlighting the urgency of translating this knowledge into clinical applications (Kamerkar et al., 2017). Thyroid cancer-derived exosomes, marked by unique biomarkers, are used for detecting cancer and monitoring therapy. Targeting these exosomes to curb disease progression is a key therapeutic strategy. Additionally, exploiting their ability to deliver specific cargoes to targeted cells makes exosomes promising vehicles for precision drug delivery or gene therapy. Given the substantial evidence supporting the role of exosomes in the TC tumor microenvironment, they also hold potential as adjunctive agents in radiotherapy and immunotherapy for TC (Table 3).
As previously discussed, exosomes play a pivotal role in orchestrating interactions between TC cells and non-cancer cells, thereby facilitating the progression of TC. Consequently, the strategic targeting of exosome-mediated tumor-initiated intercellular communication has emerged as an appealing avenue for TC therapy. Presently, exosome-targeted therapeutic strategies can be categorized into three principal domains: modulation of exosome function, attenuation of tumor exosome secretion, and interference with the uptake of tumor-exosomes by recipient cells (Wang, 2022).
Initially, direct blockade of exosome cargos, or inhibition of exosome-mediated cell-cell communication, is expected to be more effective when combined with surgical resection or cytotoxic therapies. In a study by Tang et al., it was revealed that exosomal miR-152 possesses the ability to impede the proliferation, migration, and invasion of TC cells through its interaction with Dipeptidyl dipeptidase 4, thus presenting a potential novel target for TC treatment (Tang et al., 2020). Likewise, Ye et al. conducted functional experiments showcasing that the overexpression of miRNA423-5p within exosomes enhances PTC cell migration and invasion, while the silencing of miRNA423-5p in exosomes elicits the converse impact (Ye et al., 2019). These findings suggest that targeted suppression of specific exosomal components could represent a promising therapeutic strategy for combating PTC.
Beyond the direct suppression of exosomes, various chemical compounds have demonstrated the capability to impede exosome secretion. For example, GW4869, a recognized inhibitor of exosome secretion, is frequently used in vivo to reduce exosome release. In a colorectal cancer model, exosomes derived from cancer cells were shown to promote tumor growth by enhancing cell proliferation and inhibiting apoptosis. Conversely, intra-tumoral administration of GW4869 was found to suppress tumor growth (Wang B. et al., 2019). This approach further offers an alternative avenue for exosome-mediated TC therapy. Nevertheless, in the context of future TC treatments, the potential off-target effects of these chemical substances necessitate elucidation. Furthermore, a deeper understanding is imperative to determine the optimal threshold influencing exosome secretion.
In conjunction with these strategies, efforts have been focused on inhibiting the cellular uptake of tumor-derived exosomes to block their impact on target cells (Wang, 2022). A notable example is the use of heparin to impede exosome uptake in a breast cancer mouse model (Wills et al., 2021). Due to its natural origin, heparin possesses an excellent safety profile, making it a promising therapeutic candidate for obstructing the uptake of tumor-derived exosomes. As a result, heparin and its derivatives offer potential avenues for future TC treatment.
Exosomes surpass other lipidic vesicles as superior nanocarriers owing to their enhanced targeting capacity (El Andaloussi et al., 2013) and heightened binding affinity (Kibria et al., 2018). Notably, exosomes derived from cancer cells exhibit specific tropism that can be harnessed for drug delivery into tumor cells (Feng et al., 2020). Therefore, the encapsulation of anticancer drugs within exosomes holds substantial promise for tumor-targeted therapy. In a recent study, exosomes loaded with triptolide exhibited notable encapsulation efficiency and demonstrated the ability to selectively induce apoptosis in ovarian cancer cells (Liu et al., 2019). Srivastava and colleagues additionally formulated and assessed doxorubicin-loaded exosomes for the treatment of lung cancer. The investigators concluded that these exosomes effectively reached the cancer cell niche, inducing a cytotoxic effect specifically on cancer cells (Srivastava et al., 2016). Regarding the impact of drug-loaded exosomes on TC treatment, Gangadaran and co-researchers isolated exosomes from cultured ATC cells to assess the targeting competence of tumor-derived exosomes towards their parental tumor within a mouse model. The exosomes administered to ATC model mice were promptly internalized by ATC tumors, accumulating within the tumor region within 30 min (Gangadaran et al., 2018). Employing this approach, Melzer and co-authors prompted the development of profoundly metastatic breast cancer tumors in NODscid mice. Notably, intravenous administration of taxol-loaded exosomes yielded a notable 60% decrease in subcutaneous tumors alongside a 50% reduction in the count of distant metastases (Melzer et al., 2019). Although these outcomes mirrored those of the control group subjected solely to taxol application, it is noteworthy that the concentration of taxol within exosomes was remarkably lower by a factor of 1,000. These findings suggest that exosomes hold the potential to serve as a viable alternative for cancer treatment, offering the advantage of minimal side effects. In the realm of exosome-mediated drug delivery for TC therapy, Wang et al. innovatively devised a dual antitumor approach that amalgamates internal irradiation and chemotherapy. This strategy employs iRGD-targeted exosomes as delivery vectors, facilitating the transport of Iodine-131 and doxorubicin to ATC cells (Wang C. et al., 2022). Beyond drug cargos, a separate investigation demonstrated that exosomes loaded with SCD-1 siRNA effectively curbed ATC cellular proliferation. The underlying mechanisms potentially involve the modulation of fatty acid metabolism and regulation of ROS levels (Wang C. et al., 2022). These findings suggest that exosomes, whether derived from natural tumor cells or engineered counterparts, could serve as highly effective carriers for future TC therapeutic drug delivery.
Radioactive iodine represents an alternative therapeutic avenue for TC, utilizing radiation to impede TC progression and induce apoptosis in cancer cells. Nonetheless, the tumor microenvironment, marked by specific conditions like hypoxia, can foster radioresistance in cancer cells. Recent investigations have focused on elucidating the involvement of exosomes in conferring radioresistance upon cancer cells. Notably, an experimental study has illuminated the capacity of cancer-associated fibroblasts to release exosomes that foster stemness in colorectal cancer cells, thereby provoking radioresistance (Liu et al., 2020). In another investigation, Rajendran and colleagues proposed a novel utilization of exosomes derived from primary human adipose-derived stem cells. These exosomes have been repurposed as delivery vectors for tyrosine kinase inhibitors due to their capacity for efficient loading and uptake by TC cells refractory to radioactive iodine treatment (Rajendran et al., 2021). In conjunction with these findings, it is plausible to surmise that compounds specifically targeted to exosomes may constitute a viable strategy for addressing refractory Iodine-131 TC therapy.
Exosomes have also been engineered for use in cancer immunotherapies due to their ability to support an immunomodulatory microenvironment. Engineered exosomes, loaded with bioactive agents, are designed to activate various stages of the cancer immunity cycle, culminating in robust cancer-specific immune responses (Nam et al., 2020). Moreover, bioengineered exosomes show enhanced stability in circulation, as they are less susceptible to macrophage-mediated phagocytosis, thereby increasing their biostability. In a separate investigation, Plebanek et al. demonstrated that exosomes from poorly metastatic melanoma cells promote immune surveillance by mobilizing patrolling monocytes, leading to the elimination of cancer cells within the pre-metastatic niche (Plebanek et al., 2017). In the context of exosome-mediated TC immunotherapies, Zhu and collaborators illustrated that exosome mimetics originating from NK cells manifest robust cytotoxic effects against cancer cells, encompassing ATC cells (Zhu et al., 2018). This research group delved deeper into the prospects of NK-cell-derived exosomes for immunotherapy by pre-conditioning with IL-15. The resultant exosomes, subjected to IL-15 treatment, displayed markedly elevated cytolytic activity directed at human thyroid cancer cells. Furthermore, this treatment led to a concomitant augmentation in the expression of molecules linked to NK-cell-mediated cytotoxicity (Zhu et al., 2019).
Collectively, owing to the protective function of their bilayer membranes, exosomes excel as promising drug carriers. Their robust biocompatibility and precise targeting capabilities position them as a promising new approach for treating TC. However, the clinical implementation of exosome-based therapies remains a future prospect, necessitating extensive experimental investigation into additional biomarkers and exosomal targets. Simultaneously, recent advances in engineered exosomes have unveiled innovative avenues for exosome-driven therapies in TC.
This review provides a comprehensive summary of recent discoveries and advancements regarding the involvement of exosomes in the development and diagnosis of TC. Furthermore, we underscore the significant potential of exosomes across various clinical applications, broadening the scope for innovative TC therapies. Unlike traditional approaches, exosomes offer a minimally invasive option for both diagnosis and continuous monitoring of TC, marking a new era in precision oncology. Future research is anticipated to confirm the therapeutic efficacy of exosome-based interventions, potentially extending their benefits to a broader spectrum of TC patients.
Despite significant advancements in exosome research, several challenges persist in translating these findings into clinical practice. One pressing challenge is detecting TC-derived exosomes in patients with small to medium-sized tumors, which requires further large-scale studies to thoroughly assess the accuracy and sensitivity of exosomal biomarkers (Delcorte et al., 2022b). Additionally, the implications of tumor-derived exosomes as therapeutic agents need careful consideration due to their potential dual effects on tumor progression. Optimizing exosome isolation techniques is critical to ensure cost-effective, large-scale production that could facilitate their integration into clinical settings (Grangier et al., 2021). Continued research into exosomes will deepen our understanding of their multifaceted roles in thyroid cancer and support the development of innovative diagnostic and therapeutic strategies. With advancements in nanotechnology and bioengineering, exosomes are poised to revolutionize the approach to thyroid cancer management, promising significant improvements in diagnostic accuracy and treatment efficacy.
SZ: Writing–original draft, Writing–review and editing. YY: Writing–review and editing. DW: Writing–review and editing. XY: Writing–review and editing. YC: Writing–review and editing. CS: Writing–review and editing. RY: Writing–review and editing. WT: Writing–review and editing. CL: Writing–review and editing.
The author(s) declare that financial support was received for the research, authorship, and/or publication of this article. This review was funded by the Natural Science Foundation of Sichuan Province (2023NSFSC1501); the Excellent Youth Project of Sichuan Cancer Hospital (YB2023017); the Chengdu key research and development support plan (2019-YF09-00095-SN).
We acknowledge the use of the ChatGPT-4 (https://chatgpt.com) for assistance in revising the grammar and enhancing the flow of this manuscript. It is important to note that the content was edited and finalized by the author, and no content was generated by generative AI technology.
The authors declare that the research was conducted in the absence of any commercial or financial relationships that could be construed as a potential conflict of interest.
All claims expressed in this article are solely those of the authors and do not necessarily represent those of their affiliated organizations, or those of the publisher, the editors and the reviewers. Any product that may be evaluated in this article, or claim that may be made by its manufacturer, is not guaranteed or endorsed by the publisher.
Abe, I., and Lam, A. K. (2021). Anaplastic thyroid carcinoma: updates on WHO classification, clinicopathological features and staging. Histol. Histopathol. 36, 239–248. doi:10.14670/HH-18-277
Akslen, L. A., and Livolsi, V. A. (2000). Increased angiogenesis in papillary thyroid carcinoma but lack of prognostic importance. Hum. Pathol. 31, 439–442. doi:10.1053/1-ip.2000.6548
Al-Abdallah, A., Jahanbani, I., Ali, R. H., Al-Brahim, N., Prasanth, J., Al-Shammary, B., et al. (2023). A new paradigm for epidermal growth factor receptor expression exists in PTC and NIFTP regulated by microRNAs. Front. Oncol. 13, 1080008. doi:10.3389/fonc.2023.1080008
Al-Sowayan, B. S., Al-Shareeda, A. T., and Alrfaei, B. M. (2020). Cancer stem cell-exosomes, unexposed player in tumorigenicity. Front. Pharmacol. 11, 384. doi:10.3389/fphar.2020.00384
Aragon Han, P., Weng, C. H., Khawaja, H. T., Nagarajan, N., Schneider, E. B., Umbricht, C. B., et al. (2015). MicroRNA expression and association with clinicopathologic features in papillary thyroid cancer: a systematic review. Thyroid 25, 1322–1329. doi:10.1089/thy.2015.0193
Babaei, G., Aziz, S. G., and Jaghi, N. (2021). EMT, cancer stem cells and autophagy; the three main axes of metastasis. Biomed. Pharmacother. 133, 110909. doi:10.1016/j.biopha.2020.110909
Boufraqech, M., Zhang, L., Jain, M., Patel, D., Ellis, R., Xiong, Y., et al. (2014). miR-145 suppresses thyroid cancer growth and metastasis and targets AKT3. Endocr. Relat. Cancer 21, 517–531. doi:10.1530/ERC-14-0077
Cabanillas, M. E., McFadden, D. G., and Durante, C. (2016). Thyroid cancer. Lancet 388, 2783–2795. doi:10.1016/S0140-6736(16)30172-6
Capriglione, F., Verrienti, A., Celano, M., Maggisano, V., Sponziello, M., Pecce, V., et al. (2022). Analysis of serum microRNA in exosomal vehicles of papillary thyroid cancer. Endocrine 75, 185–193. doi:10.1007/s12020-021-02847-2
Caruso Bavisotto, C., Cipolla, C., Graceffa, G., Barone, R., Bucchieri, F., Bulone, D., et al. (2019). Immunomorphological pattern of molecular chaperones in normal and pathological thyroid tissues and circulating exosomes: potential use in clinics. Int. J. Mol. Sci. 20, 4496. doi:10.3390/ijms20184496
Chakladar, J., Li, W. T., Bouvet, M., Chang, E. Y., Wang-Rodriguez, J., and Ongkeko, W. M. (2019). Papillary thyroid carcinoma variants are characterized by co-dysregulation of immune and cancer associated genes. Cancers (Basel) 11, 1179. doi:10.3390/cancers11081179
Chalmin, F., Ladoire, S., Mignot, G., Vincent, J., Bruchard, M., Remy-Martin, J. P., et al. (2010). Membrane-associated Hsp72 from tumor-derived exosomes mediates STAT3-dependent immunosuppressive function of mouse and human myeloid-derived suppressor cells. J. Clin. Invest. 120, 457–471. doi:10.1172/JCI40483
Chen, C., Yu, H., Han, F., Lai, X., Ye, K., Lei, S., et al. (2022). Tumor-suppressive circRHOBTB3 is excreted out of cells via exosome to sustain colorectal cancer cell fitness. Mol. Cancer 21, 46. doi:10.1186/s12943-022-01511-1
Chen, W., Li, G., Li, Z., Zhu, J., Wei, T., and Lei, J. (2022). Evaluation of plasma exosomal miRNAs as potential diagnostic biomarkers of lymph node metastasis in papillary thyroid carcinoma. Endocrine 75, 846–855. doi:10.1007/s12020-021-02949-x
Cocozza, F., Grisard, E., Martin-Jaular, L., Mathieu, M., and Théry, C. (2020). SnapShot: extracellular vesicles. Cell 182, 262–262.e1. doi:10.1016/j.cell.2020.04.054
da Fonseca Alves, R., Pallarès-Rusiñol, A., Rossi, R., Martí, M., Vaz, E. R., de Araújo, T. G., et al. (2024). Peptide-based biosensing approaches for targeting breast cancer-derived exosomes. Biosens. Bioelectron. 255, 116211. doi:10.1016/j.bios.2024.116211
Dai, L., Hu, W., Jiang, H., Wang, Y., Le, Q., Wu, X., et al. (2023). The diagnostic potential of two exosome-derived circRNAs for papillary thyroid cancer. Int. J. Clin. Oncol. 28, 1461–1474. doi:10.1007/s10147-023-02400-3
Dai, D., Tan, Y., Guo, L., Tang, A., and Zhao, Y. (2020). Identification of exosomal miRNA biomarkers for diagnosis of papillary thyroid cancer by small RNA sequencing. Eur. J. Endocrinol. 182, 111–121. doi:10.1530/EJE-19-0524
Dai, W., Jin, X., Han, L., Huang, H., Ji, Z., Xu, X., et al. (2020). Exosomal lncRNA DOCK9-AS2 derived from cancer stem cell-like cells activated Wnt/β-catenin pathway to aggravate stemness, proliferation, migration, and invasion in papillary thyroid carcinoma. Cell Death Dis. 11, 743. doi:10.1038/s41419-020-02827-w
Delcorte, O., Craps, J., Mahibullah, S., Spourquet, C., D'Auria, L., Van Der Smissen, P., et al. (2022a). Two miRNAs enriched in plasma extracellular vesicles are potential biomarkers for thyroid cancer. Endocr. Relat. Cancer 29, 389–401. doi:10.1530/ERC-21-0343
Delcorte, O., Degosserie, J., and Pierreux, C. E. (2022b). Role of extracellular vesicles in thyroid physiology and diseases: implications for diagnosis and treatment. Biomedicines 10, 2585. doi:10.3390/biomedicines10102585
Dierks, C., Seufert, J., Aumann, K., Ruf, J., Klein, C., Kiefer, S., et al. (2021). Combination of lenvatinib and pembrolizumab is an effective treatment option for anaplastic and poorly differentiated thyroid carcinoma. Thyroid 31, 1076–1085. doi:10.1089/thy.2020.0322
Du, J., Liang, Y., Li, J., Zhao, J. M., and Lin, X. Y. (2020). Gastric cancer cell-derived exosomal microRNA-23a promotes angiogenesis by targeting PTEN. Front. Oncol. 10, 326. doi:10.3389/fonc.2020.00326
El Andaloussi, S., Lakhal, S., Mäger, I., and Wood, M. J. (2013). Exosomes for targeted siRNA delivery across biological barriers. Adv. Drug Deliv. Rev. 65, 391–397. doi:10.1016/j.addr.2012.08.008
Fang, X., Lan, H., Jin, K., and Qian, J. (2023). Pancreatic cancer and exosomes: role in progression, diagnosis, monitoring, and treatment. Front. Oncol. 13, 1149551. doi:10.3389/fonc.2023.1149551
Feng, K., Ma, R., Zhang, L., Li, H., Tang, Y., Du, G., et al. (2020). The role of exosomes in thyroid cancer and their potential clinical application. Front. Oncol. 10, 596132. doi:10.3389/fonc.2020.596132
Ferguson, S., Yang, K. S., and Weissleder, R. (2022). Single extracellular vesicle analysis for early cancer detection. Trends Mol. Med. 28, 681–692. doi:10.1016/j.molmed.2022.05.003
Filetti, S., Durante, C., Hartl, D., Leboulleux, S., Locati, L. D., Newbold, K., et al. (2019). Thyroid cancer: ESMO Clinical Practice Guidelines for diagnosis, treatment and follow-up. Ann. Oncol. 30, 1856–1883. doi:10.1093/annonc/mdz400
Gangadaran, P., Li, X. J., Kalimuthu, S. K., Min, O. J., Hong, C. M., Rajendran, R. L., et al. (2018). New optical imaging reporter-labeled anaplastic thyroid cancer-derived extracellular vesicles as a platform for in vivo tumor targeting in a mouse model. Sci. Rep. 8, 13509. doi:10.1038/s41598-018-31998-y
Gardiner, C., Di Vizio, D., Sahoo, S., Théry, C., Witwer, K. W., Wauben, M., et al. (2016). Techniques used for the isolation and characterization of extracellular vesicles: results of a worldwide survey. J. Extracell. Vesicles 5, 32945. doi:10.3402/jev.v5.32945
Glass, S. E., and Coffey, R. J. (2022). Recent advances in the study of extracellular vesicles in colorectal cancer. Gastroenterology 163, 1188–1197. doi:10.1053/j.gastro.2022.06.039
Grangier, A., Branchu, J., Volatron, J., Piffoux, M., Gazeau, F., Wilhelm, C., et al. (2021). Technological advances towards extracellular vesicles mass production. Adv. Drug Deliv. Rev. 176, 113843. doi:10.1016/j.addr.2021.113843
Hardin, H., Helein, H., Meyer, K., Robertson, S., Zhang, R., Zhong, W., et al. (2018). Thyroid cancer stem-like cell exosomes: regulation of EMT via transfer of lncRNAs. Lab. Invest. 98, 1133–1142. doi:10.1038/s41374-018-0065-0
Harding, C., and Stahl, P. (1983). Transferrin recycling in reticulocytes: pH and iron are important determinants of ligand binding and processing. Biochem. Biophys. Res. Commun. 113, 650–658. doi:10.1016/0006-291x(83)91776-x
Haugen, B. R., Alexander, E. K., Bible, K. C., Doherty, G. M., Mandel, S. J., Nikiforov, Y. E., et al. (2016). 2015 American thyroid association management guidelines for adult patients with thyroid nodules and differentiated thyroid cancer: the American thyroid association guidelines task force on thyroid nodules and differentiated thyroid cancer. Thyroid 26, 1–133. doi:10.1089/thy.2015.0020
Herroon, M. K., Diedrich, J. D., Rajagurubandara, E., Martin, C., Maddipati, K. R., Kim, S., et al. (2019). Prostate tumor cell-derived IL1β induces an inflammatory phenotype in bone marrow adipocytes and reduces sensitivity to docetaxel via lipolysis-dependent mechanisms. Mol. Cancer Res. 17, 2508–2521. doi:10.1158/1541-7786.MCR-19-0540
Hesari, A., Golrokh Moghadam, S. A., Siasi, A., Rahmani, M., Behboodi, N., Rastgar-Moghadam, A., et al. (2018). Tumor-derived exosomes: potential biomarker or therapeutic target in breast cancer. J. Cell. Biochem. 119, 4236–4240. doi:10.1002/jcb.26364
Hoshino, A., Costa-Silva, B., Shen, T. L., Rodrigues, G., Hashimoto, A., Tesic Mark, M., et al. (2015). Tumour exosome integrins determine organotropic metastasis. Nature 527, 329–335. doi:10.1038/nature15756
Houten, P. V., Netea-Maier, R. T., and Smit, J. W. (2022). Differentiated thyroid carcinoma: an update. Best. Pract. Res. Clin. Endocrinol. Metab. 101687, 101687. doi:10.1016/j.beem.2022.101687
Hsu, Y. L., Hung, J. Y., Chang, W. A., Lin, Y. S., Pan, Y. C., Tsai, P. H., et al. (2017). Hypoxic lung cancer-secreted exosomal miR-23a increased angiogenesis and vascular permeability by targeting prolyl hydroxylase and tight junction protein ZO-1. Oncogene 36, 4929–4942. doi:10.1038/onc.2017.105
Huang, T., Song, C., Zheng, L., Xia, L., Li, Y., and Zhou, Y. (2019). The roles of extracellular vesicles in gastric cancer development, microenvironment, anti-cancer drug resistance, and therapy. Mol. Cancer 18, 62. doi:10.1186/s12943-019-0967-5
Huang, T. Y., Wang, C. Y., Chen, K. Y., and Huang, L. T. (2020). Urinary exosomal thyroglobulin in thyroid cancer patients with post-ablative therapy: a new biomarker in thyroid cancer. Front. Endocrinol. (Lausanne) 11, 382. doi:10.3389/fendo.2020.00382
Jarboe, T., Tuli, N. Y., Chakraborty, S., Maniyar, R. R., DeSouza, N., Li, X., et al. (2021). Inflammatory components of the thyroid cancer microenvironment: an avenue for identification of novel biomarkers. Adv. Exp. Med. Biol. 1350, 1–31. doi:10.1007/978-3-030-83282-7_1
Jia, L., Qiu, Q., Zhang, H., Chu, L., Du, Y., Zhang, J., et al. (2019). Concordance between the assessment of Aβ42, T-tau, and P-T181-tau in peripheral blood neuronal-derived exosomes and cerebrospinal fluid. Alzheimers Dement. 15, 1071–1080. doi:10.1016/j.jalz.2019.05.002
Jiang, D., Gong, F., Ge, X., Lv, C., Huang, C., Feng, S., et al. (2020). Neuron-derived exosomes-transmitted miR-124-3p protect traumatically injured spinal cord by suppressing the activation of neurotoxic microglia and astrocytes. J. Nanobiotechnology 18, 105. doi:10.1186/s12951-020-00665-8
Jiang, K., Li, G., Chen, W., Song, L., Wei, T., Li, Z., et al. (2020). Plasma exosomal miR-146b-5p and miR-222-3p are potential biomarkers for lymph node metastasis in papillary thyroid carcinomas. Onco Targets Ther. 13, 1311–1319. doi:10.2147/OTT.S231361
Johnstone, R. M., Adam, M., Hammond, J. R., Orr, L., and Turbide, C. (1987). Vesicle formation during reticulocyte maturation. Association of plasma membrane activities with released vesicles (exosomes). J. Biol. Chem. 262, 9412–9420. doi:10.1016/s0021-9258(18)48095-7
Junqueira-Neto, S., Batista, I. A., Costa, J. L., and Melo, S. A. (2019). Liquid biopsy beyond circulating tumor cells and cell-free DNA. Acta Cytol. 63, 479–488. doi:10.1159/000493969
Kaliszewski, K., Ludwig, M., Ludwig, B., Mikuła, A., Greniuk, M., and Rudnicki, J. (2022). Update on the diagnosis and management of medullary thyroid cancer: what has changed in recent years. Cancers (Basel) 14, 3643. doi:10.3390/cancers14153643
Kalluri, R., and LeBleu, V. S. (2020). The biology, function, and biomedical applications of exosomes. Science 367, eaau6977. doi:10.1126/science.aau6977
Kamerkar, S., LeBleu, V. S., Sugimoto, H., Yang, S., Ruivo, C. F., Melo, S. A., et al. (2017). Exosomes facilitate therapeutic targeting of oncogenic KRAS in pancreatic cancer. Nature 546, 498–503. doi:10.1038/nature22341
Khatami, F., and Tavangar, S. M. (2018). Liquid biopsy in thyroid cancer: new insight. Int. J. Hematol. Oncol. Stem Cell Res. 12, 235–248.
Kibria, G., Ramos, E. K., Wan, Y., Gius, D. R., and Liu, H. (2018). Exosomes as a drug delivery system in cancer therapy: potential and challenges. Mol. Pharm. 15, 3625–3633. doi:10.1021/acs.molpharmaceut.8b00277
Kim, D. H., Kim, H., Choi, Y. J., Kim, S. Y., Lee, J. E., Sung, K. J., et al. (2019). Exosomal PD-L1 promotes tumor growth through immune escape in non-small cell lung cancer. Exp. Mol. Med. 51, 1–13. doi:10.1038/s12276-019-0295-2
Kim, H., Lee, S., Shin, E., Seong, K. M., Jin, Y. W., Youn, H., et al. (2020). The emerging roles of exosomes as EMT regulators in cancer. Cells 9, 861. doi:10.3390/cells9040861
Konoshenko, M. Y., Lekchnov, E. A., Vlassov, A. V., and Laktionov, P. P. (2018). Isolation of extracellular vesicles: general methodologies and latest trends. Biomed. Res. Int. 2018, 8545347. doi:10.1155/2018/8545347
Kosaka, N., Iguchi, H., Hagiwara, K., Yoshioka, Y., Takeshita, F., and Ochiya, T. (2013). Neutral sphingomyelinase 2 (nSMase2)-dependent exosomal transfer of angiogenic microRNAs regulate cancer cell metastasis. J. Biol. Chem. 288, 10849–10859. doi:10.1074/jbc.M112.446831
Lee, J. C., Zhao, J. T., Gundara, J., Serpell, J., Bach, L. A., and Sidhu, S. (2015). Papillary thyroid cancer-derived exosomes contain miRNA-146b and miRNA-222. J. Surg. Res. 196, 39–48. doi:10.1016/j.jss.2015.02.027
Li, G., Chen, W., Gong, Y., Wei, T., Gong, R., Zhu, J., et al. (2022). Identification of circulating exosomal microRNAs associated with radioiodine refractory in papillary thyroid carcinoma. J. Pers. Med. 12, 2017. doi:10.3390/jpm12122017
Li, G., Chen, W., Jiang, K., Huang, J., Zhong, J., Liu, X., et al. (2023). Exosome-mediated delivery of miR-519e-5p promotes malignant tumor phenotype and CD8+ T-cell exhaustion in metastatic PTC. J. Clin. Endocrinol. Metab. 109, 1601–1617. doi:10.1210/clinem/dgad725
Li, M. Y., Liu, L. Z., and Dong, M. (2021). Progress on pivotal role and application of exosome in lung cancer carcinogenesis, diagnosis, therapy and prognosis. Mol. Cancer 20, 22. doi:10.1186/s12943-021-01312-y
Li, Q., Liu, W., Wang, Z., Wang, C., and Ai, Z. (2021). Exosomal ANXA1 derived from thyroid cancer cells is associated with malignant transformation of human thyroid follicular epithelial cells by promoting cell proliferation. Int. J. Oncol. 59, 104. doi:10.3892/ijo.2021.5284
Li, Q., Wang, H., Peng, H., Huyan, T., and Cacalano, N. A. (2019). Exosomes: versatile nano mediators of immune regulation. Cancers (Basel) 11, 1557. doi:10.3390/cancers11101557
Li, S., Xin, K., Pan, S., Wang, Y., Zheng, J., Li, Z., et al. (2023). Blood-based liquid biopsy: insights into early detection, prediction, and treatment monitoring of bladder cancer. Cell. Mol. Biol. Lett. 28, 28. doi:10.1186/s11658-023-00442-z
Liang, M., Yu, S., Tang, S., Bai, L., Cheng, J., Gu, Y., et al. (2020). A panel of plasma exosomal miRNAs as potential biomarkers for differential diagnosis of thyroid nodules. Front. Genet. 11, 449. doi:10.3389/fgene.2020.00449
Lin, B., Ma, H., Ma, M., Zhang, Z., Sun, Z., Hsieh, I. Y., et al. (2019). The incidence and survival analysis for anaplastic thyroid cancer: a SEER database analysis. Am. J. Transl. Res. 11, 5888–5896.
Lin, Q., Qi, Q., Hou, S., Chen, Z., Jiang, N., Zhang, L., et al. (2021). Exosomal circular RNA hsa_circ_007293 promotes proliferation, migration, invasion, and epithelial-mesenchymal transition of papillary thyroid carcinoma cells through regulation of the microRNA-653-5p/paired box 6 axis. Bioengineered 12, 10136–10149. doi:10.1080/21655979.2021.2000745
Liu, B., Chen, J., Shang, F., Lian, M., Shen, X., and Fang, J. (2022). Tumor-derived exosome FGD5-AS1 promotes angiogenesis, vascular permeability, and metastasis in thyroid cancer by targeting the miR-6838-5p/VAV2 Axis. J. Oncol. 2022, 4702855. doi:10.1155/2022/4702855
Liu, C., Yu, S., Zinn, K., Wang, J., Zhang, L., Jia, Y., et al. (2006). Murine mammary carcinoma exosomes promote tumor growth by suppression of NK cell function. J. Immunol. 176, 1375–1385. doi:10.4049/jimmunol.176.3.1375
Liu, H., Shen, M., Zhao, D., Ru, D., Duan, Y., Ding, C., et al. (2019). The effect of triptolide-loaded exosomes on the proliferation and apoptosis of human ovarian cancer SKOV3 cells. Biomed. Res. Int. 2019, 2595801. doi:10.1155/2019/2595801
Liu, L., Zhang, Z., Zhou, L., Hu, L., Yin, C., Qing, D., et al. (2020). Cancer associated fibroblasts-derived exosomes contribute to radioresistance through promoting colorectal cancer stem cells phenotype. Exp. Cell Res. 391, 111956. doi:10.1016/j.yexcr.2020.111956
Liu, S. (2024). MiR-374a/b-5p suppresses cell growth in papillary thyroid carcinoma through blocking exosomal ANXA1-induced macrophage M2 polarization. Biochem. Genet. doi:10.1007/s10528-024-10747-z
Lorenc, T., Klimczyk, K., Michalczewska, I., Słomka, M., Kubiak-Tomaszewska, G., and Olejarz, W. (2020). Exosomes in prostate cancer diagnosis, prognosis and therapy. Int. J. Mol. Sci. 21, 2118. doi:10.3390/ijms21062118
Lundgren, C. I., Hall, P., Dickman, P. W., and Zedenius, J. (2006). Clinically significant prognostic factors for differentiated thyroid carcinoma: a population-based, nested case-control study. Cancer 106, 524–531. doi:10.1002/cncr.21653
Luo, D., Zhan, S., Xia, W., Huang, L., Ge, W., and Wang, T. (2018). Proteomics study of serum exosomes from papillary thyroid cancer patients. Endocr. Relat. Cancer 25, 879–891. doi:10.1530/ERC-17-0547
Ma, R., Minsky, N., Morshed, S. A., and Davies, T. F. (2014). Stemness in human thyroid cancers and derived cell lines: the role of asymmetrically dividing cancer stem cells resistant to chemotherapy. J. Clin. Endocrinol. Metab. 99, E400–E409. doi:10.1210/jc.2013-3545
Melzer, C., Rehn, V., Yang, Y., Bähre, H., von der Ohe, J., and Hass, R. (2019). Taxol-loaded MSC-derived exosomes provide a therapeutic vehicle to target metastatic breast cancer and other carcinoma cells. Cancers (Basel) 11, 798. doi:10.3390/cancers11060798
Minciacchi, V. R., Freeman, M. R., and Di Vizio, D. (2015). Extracellular vesicles in cancer: exosomes, microvesicles and the emerging role of large oncosomes. Semin. Cell Dev. Biol. 40, 41–51. doi:10.1016/j.semcdb.2015.02.010
Nam, G. H., Choi, Y., Kim, G. B., Kim, S., Kim, S. A., and Kim, I. S. (2020). Emerging prospects of exosomes for cancer treatment: from conventional therapy to immunotherapy. Adv. Mater. Weinh. 32, e2002440. doi:10.1002/adma.202002440
Nik Mohamed Kamal, N., Awang, R., Mohamad, S., and Shahidan, W. (2020). Plasma- and saliva exosome profile reveals a distinct MicroRNA signature in chronic periodontitis. Front. Physiol. 11, 587381. doi:10.3389/fphys.2020.587381
Nishino, M., and Jacob, J. (2020). Invasion in thyroid cancer: controversies and best practices. Semin. Diagn Pathol. 37, 219–227. doi:10.1053/j.semdp.2020.02.003
Okafor, C., Hogan, J., Raygada, M., Thomas, B. J., Akshintala, S., Glod, J. W., et al. (2021). Update on targeted therapy in medullary thyroid cancer. Front. Endocrinol. (Lausanne) 12, 708949. doi:10.3389/fendo.2021.708949
Pan, B. T., and Johnstone, R. M. (1983). Fate of the transferrin receptor during maturation of sheep reticulocytes in vitro: selective externalization of the receptor. Cell 33, 967–978. doi:10.1016/0092-8674(83)90040-5
Pan, Q., Zhao, J., Li, M., Liu, X., Xu, Y., Li, W., et al. (2020). Exosomal miRNAs are potential diagnostic biomarkers between malignant and benign thyroid nodules based on next-generation sequencing. Carcinogenesis 41, 18–24. doi:10.1093/carcin/bgz160
Pavlidis, E. T., Psarras, K. K., Symeonidis, N. G., Martzivanou, E. K., Nikolaidou, C. C., Stavrati, K. E., et al. (2021). Robot-assisted thyroidectomy versus open thyroidectomy in the treatment of well differentiated thyroid carcinoma. JSLS 25, e2021.00032. doi:10.4293/JSLS.2021.00032
Peng, H., Zhu, Z., Xing, J., Xu, Q., Man, C., Wang, S., et al. (2024). Expression profiling and bioinformatics analysis of serum exosomal circular RNAs in lymph node metastasis of papillary thyroid carcinoma. J. Biomed. Res. 38, 1–15. doi:10.7555/JBR.37.20230304
Plebanek, M. P., Angeloni, N. L., Vinokour, E., Li, J., Henkin, A., Martinez-Marin, D., et al. (2017). Pre-metastatic cancer exosomes induce immune surveillance by patrolling monocytes at the metastatic niche. Nat. Commun. 8, 1319. doi:10.1038/s41467-017-01433-3
Pucci, F., Garris, C., Lai, C. P., Newton, A., Pfirschke, C., Engblom, C., et al. (2016). SCS macrophages suppress melanoma by restricting tumor-derived vesicle-B cell interactions. Science 352, 242–246. doi:10.1126/science.aaf1328
Puppin, C., Fabbro, D., Dima, M., Di Loreto, C., Puxeddu, E., Filetti, S., et al. (2008). High periostin expression correlates with aggressiveness in papillary thyroid carcinomas. J. Endocrinol. 197, 401–408. doi:10.1677/JOE-07-0618
Qiao, L., Dong, C., Jia, W., and Ma, B. (2022). Exosomal miR-655-3p inhibits growth, and invasion and macrophage M2 polarization through targeting CXCR4 in papillary thyroid carcinoma. Acta biochim. Pol. 69, 773–779. doi:10.18388/abp.2020_6027
Rajabi, S., Dehghan, M. H., Dastmalchi, R., Jalali Mashayekhi, F., Salami, S., and Hedayati, M. (2019). The roles and role-players in thyroid cancer angiogenesis. Endocr. J. 66, 277–293. doi:10.1507/endocrj.EJ18-0537
Rajendran, R. L., Paudel, S., Gangadaran, P., Oh, J. M., Oh, E. J., Hong, C. M., et al. (2021). Extracellular vesicles act as nano-transporters of tyrosine kinase inhibitors to revert iodine avidity in thyroid cancer. Pharmaceutics 13, 248. doi:10.3390/pharmaceutics13020248
Ramsden, J. D. (2000). Angiogenesis in the thyroid gland. J. Endocrinol. 166, 475–480. doi:10.1677/joe.0.1660475
Rao, M., Zhu, Y., Qi, L., Hu, F., and Gao, P. (2020). Circular RNA profiling in plasma exosomes from patients with gastric cancer. Oncol. Lett. 20, 2199–2208. doi:10.3892/ol.2020.11800
Rappa, G., Puglisi, C., Santos, M. F., Forte, S., Memeo, L., and Lorico, A. (2019). Extracellular vesicles from thyroid carcinoma: the new frontier of liquid biopsy. Int. J. Mol. Sci. 20, 1114. doi:10.3390/ijms20051114
Samsonov, R., Burdakov, V., Shtam, T., Radzhabovа, Z., Vasilyev, D., Tsyrlina, E., et al. (2016). Plasma exosomal miR-21 and miR-181a differentiates follicular from papillary thyroid cancer. Tumour Biol. 37, 12011–12021. doi:10.1007/s13277-016-5065-3
Sanz-Rubio, D., Martin-Burriel, I., Gil, A., Cubero, P., Forner, M., Khalyfa, A., et al. (2018). Stability of circulating exosomal miRNAs in Healthy subjects. Sci. Rep. 8, 10306. doi:10.1038/s41598-018-28748-5
Schlumberger, M., and Leboulleux, S. (2021). Current practice in patients with differentiated thyroid cancer. Nat. Rev. Endocrinol. 17, 176–188. doi:10.1038/s41574-020-00448-z
Shang, A., Wang, X., Gu, C., Liu, W., Sun, J., Zeng, B., et al. (2020). Exosomal miR-183-5p promotes angiogenesis in colorectal cancer by regulation of FOXO1. Aging (Albany NY) 12, 8352–8371. doi:10.18632/aging.103145
Si, C., Gao, J., and Ma, X. (2024). Natural killer cell-derived exosome-based cancer therapy: from biological roles to clinical significance and implications. Mol. Cancer 23, 134. doi:10.1186/s12943-024-02045-4
Soeda, N., Iinuma, H., Suzuki, Y., Tsukahara, D., Midorikawa, H., Igarashi, Y., et al. (2019). Plasma exosome-encapsulated microRNA-21 and microRNA-92a are promising biomarkers for the prediction of peritoneal recurrence in patients with gastric cancer. Oncol. Lett. 18, 4467–4480. doi:10.3892/ol.2019.10807
Sponziello, M., Rosignolo, F., Celano, M., Maggisano, V., Pecce, V., De Rose, R. F., et al. (2016). Fibronectin-1 expression is increased in aggressive thyroid cancer and favors the migration and invasion of cancer cells. Mol. Cell. Endocrinol. 431, 123–132. doi:10.1016/j.mce.2016.05.007
Srivastava, A., Amreddy, N., Babu, A., Panneerselvam, J., Mehta, M., Muralidharan, R., et al. (2016). Nanosomes carrying doxorubicin exhibit potent anticancer activity against human lung cancer cells. Sci. Rep. 6, 38541. doi:10.1038/srep38541
Su, Q., Zhang, Y., Cui, Z., Chang, S., and Zhao, P. (2021). Semen-derived exosomes mediate immune escape and transmission of reticuloendotheliosis virus. Front. Immunol. 12, 735280. doi:10.3389/fimmu.2021.735280
Sun, B., Zhou, Y., Fang, Y., Li, Z., Gu, X., and Xiang, J. (2019). Colorectal cancer exosomes induce lymphatic network remodeling in lymph nodes. Int. J. Cancer 145, 1648–1659. doi:10.1002/ijc.32196
Surman, M., Wilczak, M., Jankowska, U., Skupień-Rabian, B., and Przybyło, M. (2024). Shotgun proteomics of thyroid carcinoma exosomes - insight into the role of exosomal proteins in carcinogenesis and thyroid homeostasis. Biochim. Biophys. Acta Gen. Subj. 1868, 130672. doi:10.1016/j.bbagen.2024.130672
Tang, K. D., Holzapfel, B. M., Liu, J., Lee, T. K., Ma, S., Jovanovic, L., et al. (2016). Tie-2 regulates the stemness and metastatic properties of prostate cancer cells. Oncotarget 7, 2572–2584. doi:10.18632/oncotarget.3950
Tang, M., Wang, Q., Wang, K., and Wang, F. (2020). Mesenchymal stem cells-originated exosomal microRNA-152 impairs proliferation, invasion and migration of thyroid carcinoma cells by interacting with DPP4. J. Endocrinol. Invest. 43, 1787–1796. doi:10.1007/s40618-020-01406-2
Théry, C., Witwer, K. W., Aikawa, E., Alcaraz, M. J., Anderson, J. D., Andriantsitohaina, R., et al. (2018). Minimal information for studies of extracellular vesicles 2018 (MISEV2018): a position statement of the International Society for Extracellular Vesicles and update of the MISEV2014 guidelines. J. Extracell. Vesicles 7, 1535750. doi:10.1080/20013078.2018.1535750
Uludağ, M., Tanal, M., and İşgör, A. (2018). Standards and definitions in neck dissections of differentiated thyroid cancer. Sisli Etfal Hast. Tip. Bul. 52, 149–163. doi:10.14744/SEMB.2018.14227
Wang, B., Wang, Y., Yan, Z., Sun, Y., and Su, C. (2019). Colorectal cancer cell-derived exosomes promote proliferation and decrease apoptosis by activating the ERK pathway. Int. J. Clin. Exp. Pathol. 12, 2485–2495.
Wang, C., Li, N., Li, Y., Hou, S., Zhang, W., Meng, Z., et al. (2022). Engineering a HEK-293T exosome-based delivery platform for efficient tumor-targeting chemotherapy/internal irradiation combination therapy. J. Nanobiotechnology 20, 247. doi:10.1186/s12951-022-01462-1
Wang, C. Y., Shih, S. R., Chen, K. Y., and Huang, P. J. (2022). Urinary exosomal tissue TIMP and angiopoietin-1 are preoperative novel biomarkers of well-differentiated thyroid cancer. Biomedicines 11, 24. doi:10.3390/biomedicines11010024
Wang, D., Lyu, Y., Yang, Y., Zhang, S., Chen, G., Pan, J., et al. (2022). Schwann cell-derived EVs facilitate dental pulp regeneration through endogenous stem cell recruitment via SDF-1/CXCR4 axis. Acta Biomater. 140, 610–624. doi:10.1016/j.actbio.2021.11.039
Wang, M. H., Ye, Y., Zhang, M., Zhou, B. R., Wang, J. N., Song, Y. N., et al. (2022). Exosome-mediated delivery of SCD-1 siRNA promoted the death of anaplastic thyroid carcinoma cells via regulating ROS level. Clin. Transl. Oncol. 24, 288–296. doi:10.1007/s12094-021-02682-x
Wang, S. E. (2022). Extracellular vesicles in cancer therapy. Semin. Cancer Biol. 86, 296–309. doi:10.1016/j.semcancer.2022.06.001
Wang, S. H., Liou, G. G., Liu, S. H., Chang, J. S., Hsiao, J. R., Yen, Y. C., et al. (2019). Laminin γ2-enriched extracellular vesicles of oral squamous cell carcinoma cells enhance in vitro lymphangiogenesis via integrin α3-dependent uptake by lymphatic endothelial cells. Int. J. Cancer 144, 2795–2810. doi:10.1002/ijc.32027
Wang, Y., Cen, A., Yang, Y., Ye, H., Li, J., Liu, S., et al. (2021). miR-181a, delivered by hypoxic PTC-secreted exosomes, inhibits DACT2 by downregulating MLL3, leading to YAP-VEGF-mediated angiogenesis. Mol. Ther. Nucleic Acids 24, 610–621. doi:10.1016/j.omtn.2021.02.027
Wang, Y., Li, Q., Yang, X., Guo, H., Ren, T., Zhang, T., et al. (2024). Exosome-mediated communication in thyroid cancer: implications for prognosis and therapeutic targets. Biochem. Genet. doi:10.1007/s10528-024-10833-2
Wang, Y., Wang, J., Wang, C., Chen, Y., and Chen, J. (2022). DIO3OS as a potential biomarker of papillary thyroid cancer. Pathol. Res. Pract. 229, 153695. doi:10.1016/j.prp.2021.153695
Wang, Y., Xu, F., Zhong, J. Y., Lin, X., Shan, S. K., Guo, B., et al. (2020). Exosomes as mediators of cell-to-cell communication in thyroid disease. Int. J. Endocrinol. 2020, 4378345. doi:10.1155/2020/4378345
Wang G., G., He, L., Wang, S., Zhang, M., Li, Y., Liu, Q., et al. (2020). EV PD-L1 is correlated with clinical features and contributes to T cell suppression in pediatric thyroid cancer. J. Clin. Endocrinol. Metab. 105, dgaa309. doi:10.1210/clinem/dgaa309
Wang H., H., Lu, Z., and Zhao, X. (2019). Tumorigenesis, diagnosis, and therapeutic potential of exosomes in liver cancer. J. Hematol. Oncol. 12, 133. doi:10.1186/s13045-019-0806-6
Wang Z., Z., Lv, J., Zou, X., Huang, Z., Zhang, H., Liu, Q., et al. (2019). A three plasma microRNA signature for papillary thyroid carcinoma diagnosis in Chinese patients. Gene 693, 37–45. doi:10.1016/j.gene.2019.01.016
Wells, S. A.Jr, Asa, S. L., Dralle, H., Elisei, R., Evans, D. B., Gagel, R. F., et al. (2015). Revised American Thyroid Association guidelines for the management of medullary thyroid carcinoma. Thyroid 25, 567–610. doi:10.1089/thy.2014.0335
Wen, Q., Wang, Y., Li, X., Jin, X., and Wang, G. (2021). Decreased serum exosomal miR-29a expression and its clinical significance in papillary thyroid carcinoma. J. Clin. Lab. Anal. 35, e23560. doi:10.1002/jcla.23560
Wills, C. A., Liu, X., Chen, L., Zhao, Y., Dower, C. M., Sundstrom, J., et al. (2021). Chemotherapy-induced upregulation of small extracellular vesicle-associated PTX3 accelerates breast cancer metastasis. Cancer Res. 81, 452–463. doi:10.1158/0008-5472.CAN-20-1976
Wu, F., Li, F., Lin, X., Xu, F., Cui, R. R., Zhong, J. Y., et al. (2019). Exosomes increased angiogenesis in papillary thyroid cancer microenvironment. Endocr. Relat. Cancer 26, 525–538. doi:10.1530/ERC-19-0008
Wu, Q., He, Y., Liu, X., Luo, F., Jiang, Y., Xiang, M., et al. (2022). Cancer stem cell-like cells-derived exosomal CDKN2B-AS1 stabilizes CDKN2B to promote the growth and metastasis of thyroid cancer via TGF-β1/Smad2/3 signaling. Exp. Cell Res. 419, 113268. doi:10.1016/j.yexcr.2022.113268
Wu, Q., He, Y., Liu, X., Luo, F., Jiang, Y., Xiang, M., et al. (2023). Cancer stem cell-like cells-derived exosomal lncRNA CDKN2B-AS1 promotes biological characteristics in thyroid cancer via miR-122-5p/P4HA1 axis. Regen. Ther. 22, 19–29. doi:10.1016/j.reth.2022.11.005
Xin, Y., Meng, K., Guo, H., Chen, B., Zheng, C., Shou, X., et al. (2022). Exosomal hsa-miR-129-2 and hsa-miR-889 from a 6-microRNA signature might be a potential biomarker for predicting the prognosis of papillary thyroid carcinoma. Comb. Chem. High. Throughput Screen. 25, 819–830. doi:10.2174/1386207324666210125110732
Xu, Z., Zeng, S., Gong, Z., and Yan, Y. (2020). Exosome-based immunotherapy: a promising approach for cancer treatment. Mol. Cancer 19, 160. doi:10.1186/s12943-020-01278-3
Yang, C., Kim, H. S., Song, G., and Lim, W. (2019a). The potential role of exosomes derived from ovarian cancer cells for diagnostic and therapeutic approaches. J. Cell. Physiol. 234, 21493–21503. doi:10.1002/jcp.28905
Yang, C., Wei, Y., Yu, L., and Xiao, Y. (2019b). Identification of altered circular RNA expression in serum exosomes from patients with papillary thyroid carcinoma by high-throughput sequencing. Med. Sci. Monit. 25, 2785–2791. doi:10.12659/MSM.915658
Yang, X. X., Sun, C., Wang, L., and Guo, X. L. (2019c). New insight into isolation, identification techniques and medical applications of exosomes. J. Control Release 308, 119–129. doi:10.1016/j.jconrel.2019.07.021
Yao, X., and Zhang, Q. (2022). Function and clinical significance of circular RNAs in thyroid cancer. Front. Mol. Biosci. 9, 925389. doi:10.3389/fmolb.2022.925389
Ye, W., Deng, X., and Fan, Y. (2019). Exosomal miRNA423-5p mediated oncogene activity in papillary thyroid carcinoma: a potential diagnostic and biological target for cancer therapy. Neoplasma 66, 516–523. doi:10.4149/neo_2018_180824N643
Yue, M., Hu, S., Sun, H., Tuo, B., Jia, B., Chen, C., et al. (2023). Extracellular vesicles remodel tumor environment for cancer immunotherapy. Mol. Cancer 22, 203. doi:10.1186/s12943-023-01898-5
Zabegina, L., Nazarova, I., Knyazeva, M., Nikiforova, N., Slyusarenko, M., Titov, S., et al. (2020). MiRNA let-7 from TPO(+) extracellular vesicles is a potential marker for a differential diagnosis of follicular thyroid nodules. Cells 9, 1917. doi:10.3390/cells9081917
Zhang, L., and Yu, D. (2019). Exosomes in cancer development, metastasis, and immunity. Biochim. Biophys. Acta Rev. Cancer 1871, 455–468. doi:10.1016/j.bbcan.2019.04.004
Zhang, S., Yang, Y., Jia, S., Chen, H., Duan, Y., Li, X., et al. (2020). Exosome-like vesicles derived from Hertwig's epithelial root sheath cells promote the regeneration of dentin-pulp tissue. Theranostics 10, 5914–5931. doi:10.7150/thno.43156
Zhang, Y., Liu, Y., Liu, H., and Tang, W. H. (2019). Exosomes: biogenesis, biologic function and clinical potential. Cell Biosci. 9, 19. doi:10.1186/s13578-019-0282-2
Zhang, Y., and Weinberg, R. A. (2018). Epithelial-to-mesenchymal transition in cancer: complexity and opportunities. Front. Med. 12, 361–373. doi:10.1007/s11684-018-0656-6
Zhang, Y., Yu, M., and Tian, W. (2016). Physiological and pathological impact of exosomes of adipose tissue. Cell Prolif. 49, 3–13. doi:10.1111/cpr.12233
Zhang, Y., Zhang, C., Wang, J., Liu, H., and Wang, M. (2021). Bone-adipose tissue crosstalk: role of adipose tissue derived extracellular vesicles in bone diseases. J. Cell. Physiol. 236, 7874–7886. doi:10.1002/jcp.30414
Zhong, D., Wang, Z., Ye, Z., Wang, Y., and Cai, X. (2024). Cancer-derived exosomes as novel biomarkers in metastatic gastrointestinal cancer. Mol. Cancer 23, 67. doi:10.1186/s12943-024-01948-6
Zhou, C. F., Ma, J., Huang, L., Yi, H. Y., Zhang, Y. M., Wu, X. G., et al. (2019). Cervical squamous cell carcinoma-secreted exosomal miR-221-3p promotes lymphangiogenesis and lymphatic metastasis by targeting VASH1. Oncogene 38, 1256–1268. doi:10.1038/s41388-018-0511-x
Zhou, H., Zhu, L., Song, J., Wang, G., Li, P., Li, W., et al. (2022). Liquid biopsy at the frontier of detection, prognosis and progression monitoring in colorectal cancer. Mol. Cancer 21, 86. doi:10.1186/s12943-022-01556-2
Zhu, J., Tang, B., Gao, Y., Xu, S., Tu, J., Wang, Y., et al. (2021). Predictive models for HCC prognosis, recurrence risk, and immune infiltration based on two exosomal genes: MYL6B and THOC2. J. Inflamm. Res. 14, 4089–4109. doi:10.2147/JIR.S315957
Zhu, L., Gangadaran, P., Kalimuthu, S., Oh, J. M., Baek, S. H., Jeong, S. Y., et al. (2018). Novel alternatives to extracellular vesicle-based immunotherapy - exosome mimetics derived from natural killer cells. Artif. Cells Nanomed Biotechnol. 46, S166-S179–166S179. doi:10.1080/21691401.2018.1489824
Keywords: thyroid cancer, exosome, tumorigenesis, diagnosis, therapy
Citation: Zhang S, Yang Y, Wang D, Yang X, Cai Y, Shui C, Yang R, Tian W and Li C (2024) Exploring exosomes: novel diagnostic and therapeutic frontiers in thyroid cancer. Front. Pharmacol. 15:1431581. doi: 10.3389/fphar.2024.1431581
Received: 12 May 2024; Accepted: 25 October 2024;
Published: 08 November 2024.
Edited by:
Sheema Khan, The University of Texas Rio Grande Valley, United StatesReviewed by:
Jessian Munoz, Texas Children’s Hospital, United StatesCopyright © 2024 Zhang, Yang, Wang, Yang, Cai, Shui, Yang, Tian and Li. This is an open-access article distributed under the terms of the Creative Commons Attribution License (CC BY). The use, distribution or reproduction in other forums is permitted, provided the original author(s) and the copyright owner(s) are credited and that the original publication in this journal is cited, in accordance with accepted academic practice. No use, distribution or reproduction is permitted which does not comply with these terms.
*Correspondence: Chao Li, bGljaGFvQHNjc3pseXkub3JnLmNu
Disclaimer: All claims expressed in this article are solely those of the authors and do not necessarily represent those of their affiliated organizations, or those of the publisher, the editors and the reviewers. Any product that may be evaluated in this article or claim that may be made by its manufacturer is not guaranteed or endorsed by the publisher.
Research integrity at Frontiers
Learn more about the work of our research integrity team to safeguard the quality of each article we publish.