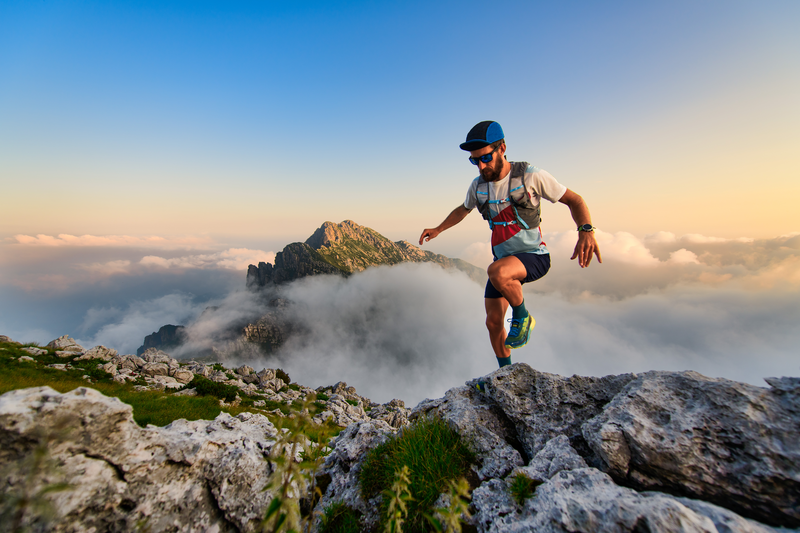
95% of researchers rate our articles as excellent or good
Learn more about the work of our research integrity team to safeguard the quality of each article we publish.
Find out more
REVIEW article
Front. Pharmacol. , 26 August 2024
Sec. Ethnopharmacology
Volume 15 - 2024 | https://doi.org/10.3389/fphar.2024.1426769
This article is part of the Research Topic Drug Discovery Derived from Herbal Medicine/Polypeptide for Neurological Diseases View all 14 articles
Depression, a mood disorder characterized by a persistent low mood and lack of enjoyment, is considered the leading cause of non-fatal health losses worldwide. Neuroplasticity refers to the brain’s ability to adapt to external or internal stimuli, resulting in functional and structural changes. This process plays a crucial role in the development of depression. Traditional Chinese Medicine (TCM) shows significant potential as a complementary and alternative therapy for neurological diseases, including depression. However, there has been no systematic summary of the role of neuroplasticity in the pathological development of depression and TCM Interventions currently. This review systematically summarized recent literature on changes in neuroplasticity in depression and analyzed the regulatory mechanisms of active metabolites in TCM and TCM formulas on neuroplasticity in antidepressant treatment. Additionally, this review discussed the limitations of current research and the application prospects of TCM in regulating neuroplasticity in antidepressant research.
Depression is a mood illness marked by enduring feelings of sadness and lack of enjoyment. The global average incidence rate is about 4.4%. By 2030, depression is expected to become the leading cause of disease burden worldwide, being the primary contributor to non-fatal health loss globally (Rehm and Shield, 2019; Bayes et al., 2020). Selective serotonin reuptake inhibitors (SSRIs) and other Western medicine therapies are the mainstays of treatment; however, most medications have delayed effects, high rates of non-responsiveness, and significant side effects such as headaches, nausea, weight gain, and chronic dysfunction (Wang et al., 2019; Qu et al., 2021; Wei et al., 2022).
Therefore, developing more effective and safer antidepressant drugs has become an urgent problem to be solved. Traditional Chinese Medicine (TCM) has a long history of understanding and treating depression. TCM is known for its multi metabolite, multi target, multi link, and multi pathway characteristics, which can act on multiple aspects of the disease and have high efficacy and low toxicity. This highlights the advantages and good prospects of TCM in treating depression. Importantly, compared to Western medicine, they have the advantages of easy use, good therapeutic effects, minimal dosage, and fewer side effects. Due to the shortcomings of existing antidepressants and the urgent market demand, research on the antidepressant mechanism of TCM has attracted much attention (Zhuang et al., 2023).
Neuroplasticity refers to the brain’s ability to respond to external or internal stimuli from the environment or organs, resulting in functional and structural changes (Vints et al., 2022). Neuroplasticity is closely related to depression (Tartt et al., 2022), and is a significant focus for the development of future antidepressant drugs (Duman et al., 2016). However, there remains a notable lack of a systematic overview regarding the role of neuroplasticity in the pathological development of depression and the intervention of TCM.
Based on the above findings, this review systematically summarized the changes in neuroplasticity observed in clinical and preclinical studies of depression by searching relevant literature from recent years. Furthermore, it explored into the pharmacological mechanisms through which TCM modulated neuroplasticity to treat depression, providing scientific basis for subsequent basic research and clinical applications.
To investigate how TCM exerted antidepressant effects by regulating neuroplasticity, we conducted a comprehensive search of articles in PubMed, Embase, Web of Science, and ScienceDirect databases. The search keywords included “Traditional Chinese Medicine,” “Chinese herbal medicine,” “herb,” “Traditional Chinese Medicine formulas,” “Traditional Chinese Medicine metabolites,” “depression,” “major depressive disorder,” “syntactic plasticity,” and “neuroplasticity.” The retrieved articles were reviewed by two independent reviewers based on their titles, abstracts, and full texts, adhering to specific inclusion and exclusion criteria. The inclusion criteria were: 1) Original articles written in English; 2) Articles that examined the relevant mechanisms of TCM in regulating neuroplasticity for the treatment of depression. Exclusion criteria were as follows: 1) Articles written in any language other than English; 2) Gray literature; 3) Editorials; 4) Review articles; 5) Duplicate publications.
Neuroplasticity is a crucial concept in life sciences, describing how the brain changes and adapts to environmental changes by continually forming new neural connections (Price and Duman, 2020). It represents the adaptability of the nervous system, enabling it to adjust to learning, memory, environmental changes, and rehabilitation following brain injury. The main mechanisms include the regulation of synaptic strength, structural remodeling, and the regulation of intrinsic neuronal properties. These processes are dynamic, involving changes in the number of brain nuclei and structures, various functions, and numerous interactions (Xing and Bai, 2020; Dzyubenko and Hermann, 2023). Neuroplasticity is essential for understanding brain development, learning, and the regulation of homeostasis in the central nervous system (CNS).
Neuroplasticity includes two primary types: structural plasticity and functional plasticity. Structural plasticity refers to changes in mechanisms that promote neurogenesis, the formation of dendritic spines, and the growth and repair of axons. It includes changes in the number and connectivity of synapses, the density of dendritic spines, and modifications in neural processes like axons and dendrites, as well as variations in the number of neuronal cells (De Paola et al., 2006; Knott et al., 2006). On the other hand, functional plasticity involves synaptic changes between neurons without modifying their physical structure, such as long-term potentiation (LTP) and long-term depression (LTD) effects (Castillo, 2012; Marsden, 2013; Diering and Huganir, 2018). LTP and LTD are crucial mechanisms that affect cognitive and emotional functions in depression patients. Intense and sustained stimulation leads to an increase in neuronal discharge, which in turn enhances the strength of synapses. This process facilitates learning and memory, thereby promoting LTP. In contrast, LTD is characterized by a decrease in the efficacy and connectivity of neuronal synapses (Figure 1 showed a schematic diagram of neurogenesis).
Neuroplasticity is regulated by several key mechanisms, one of which is the brain-derived neurotrophic factor (BDNF)/tyrosine kinase receptor B (TrkB) signaling pathway. The synthesis of BDNF is triggered by the activation of cyclic adenosine monophosphate (cAMP) responsive element binding protein (CREB). CREB is pivotal in facilitating LTP and synaptic plasticity. When BDNF binds to TrkB receptors, it triggers various signaling cascades, such as the mitogen-activated protein kinase/extracellular signal-regulated kinase (MAPK/ERK), phosphoinositide 3-kinase (PI3K), and mammalian target of rapamycin (mTOR) pathways, which are responsible for spine enlargement and increased glutamate sensitivity (Figure 2 showed the regulatory mechanism) (Bourtchuladze et al., 1994; Tanaka et al., 2008; Tejeda and Díaz-Guerra, 2017).
Meta-analysis is a prominent method for evaluating the effectiveness of public health interventions (Tanner-Smith and Grant, 2018). In the pathophysiology of depression, impaired neuroplasticity plays a crucial role, as indicated by a meta-analysis conducted on the cerebrospinal fluid of individuals with unipolar depression (Mousten et al., 2022). Studies have shown that the increase in motor evoked potential amplitude, induced by paired associative stimulation, weakens during severe depressive episodes and normalizes during remission. It suggests the presence of LTP deficits in individuals with depression (Player et al., 2013; Kuhn et al., 2016). Furthermore, compared to healthy subjects, patients with depression, particularly those with refractory depression, exhibit impaired neuroplasticity in the dorsolateral prefrontal cortex. Female patients with depression also demonstrate persistent LTD-like plasticity deficits (Noda et al., 2018; Yu et al., 2020; Kaneko et al., 2024). Abnormal changes in neuroplasticity-related proteins have been observed in depression patients (Hidese et al., 2020). The ratio of BDNF to leptin levels has been associated with treatment responses in depression and may also be related to the neuroplasticity of depression, as evidenced by a 12-week follow-up study (An et al., 2019).
Stress is recognized as a normal physiological and psychological response to both positive and negative situations. Chronic stress, in particular, plays a key role in the development of mental illnesses such as depression (Ray et al., 2017; Beurel et al., 2020; Monroe and Harkness, 2022). Prolonged exposure to chronic stress exacerbates the phagocytosis of synaptic elements and results in defects in neuroplasticity (Kokkosis et al., 2024). Synaptic pruning, as a developmental process, is closely related to synaptic plasticity. In models of depression induced by chronic unpredictable mild stress (CUMS), excessive activation of microglia leads to exaggerated synaptic pruning (Zhang et al., 2022a), accompanied by impairments in synaptic plasticity (Li et al., 2021a; Yan et al., 2021). Early-life stress increases susceptibility to depression in adolescent mice by regulating the miR-34c-5p/synaptotagmin-1 (SYT1) axis and disrupting hippocampal neuroplasticity (Yu et al., 2024). In a combined model, adult female rats subjected to maternal-infant separation (MS) and CUMS exhibited more severe depressive and anxiety-like behaviors, potentially linked to compromised synaptic plasticity (Huang et al., 2021). In a depression model where insomnia was induced by CUMS combined with sleep deprivation, dendritic spines in the hippocampal dentate gyrus (DG) region were damaged, neural networks were disrupted, and neuroplasticity was inhibited (Li et al., 2022). Studies have also demonstrated that the absence of bombesin receptor-activated protein homologous protein affects hippocampal synaptic plasticity and exacerbates CUMS-mediated behavioral changes (Yao et al., 2023). Mechanistic research has revealed that CUMS alters synaptic plasticity in the nucleus accumbens (NAc) by influencing Kv4.2 channels through glycogen synthase kinase 3β (GSK3β)-dependent mechanisms (Aceto et al., 2020). Additionally, CUMS can disrupt the synaptic plasticity of regenerating neurons in the hippocampus of ischemic rats via astrocytic glutamate transporter-1 (Yu et al., 2019).
Social isolation can induce fatigue, behavioral changes, substance abuse, and various mental illnesses. These effects can be sustained and irreversible, impacting both humans and animals and increasing the risk of developing mental illness (Jaremka et al., 2014; Hueston et al., 2017). Neuroplasticity-related signals play a crucial role in the impact of induced isolation on sexual and neurological behavioral deficits (Liu et al., 2020). Animals subjected to chronic social isolation (CSIS) displayed depressive-like behavior (Perić et al., 2021), accompanied by proteomic findings showing dysregulated expression of synaptic plasticity-related proteins (Filipović et al., 2023). Moreover, animals raised in isolation exhibited immature dendritic spines that appear small and thin, with impaired neuroplasticity observed through LTP testing (Medendorp et al., 2018). However, treatment with fluoxetine has been shown to alleviate depressive-like behavior induced by CSIS and regulate neuroplasticity-related proteins (Filipović et al., 2022).
The hypothalamic-pituitary-adrenal (HPA) axis is a vital metabolite of the neuroendocrine system. When active, the anterior pituitary gland releases adrenocorticotropin (ACTH) into the bloodstream. This signal is received by the paraventricular nucleus of the hypothalamus, which then produces corticotropin-releasing hormone. ACTH, in turn, stimulates the adrenal cortex to release cortisol (CORT) (Frankiensztajn et al., 2020). Excessive activation of the HPA axis correlates significantly with sustained elevation of CORT levels and depression. Elevated CORT levels observed in individuals with depression closely correlate with the severity of depressive symptoms and poor treatment outcomes (Karin et al., 2020). Chronic exposure to CORT reduces the structural plasticity of astrocytes in the hippocampus of mice, leading to hippocampal atrophy (Zhang et al., 2015). Mice treated with CORT exhibit depressive-like behavior accompanied by changes in synaptic plasticity (Crupi et al., 2013; Freitas et al., 2016). Mechanistic studies have shown that CORT reduces synaptic density and vesicle recycling by downregulating BNIP3 like (BNIP3L)/NIX, thereby inhibiting mitochondrial autophagy (Choi et al., 2021).
LPS can be found in the outer wall of Gram-negative bacterial cells, consisting of lipids and polysaccharides. Mouse models induced with LPS to mimic depression-like symptoms are commonly used to study the mechanisms of inflammation-related depression and the therapeutic effects of various drugs (Yin et al., 2023). Early reports indicated that LPS administration could induce LTP and depression in the hippocampal CA1 area (Jo et al., 2001). Recent studies have found that LPS mediates depressive-like behavior by promoting neuroinflammation in the basolateral amygdala (BLA), enhancing glutamatergic synaptic transmission, and increasing the intrinsic excitability of BLA projection neurons (Zheng et al., 2021). Wu et al. (2019), through a combination of proteomics and metabolomics, found that LPS intervention in mice disrupts glutamatergic transmission and Ephrin receptor signaling, potentially leading to impaired hypothalamic synaptic plasticity and depressive-like behavior.
Fluoxetine, a widely used SSRI in clinical practice, exerts its antidepressant effects by enhancing synaptic plasticity (Qian et al., 2024). It alos modified mood behaviors and hippocampal neuroplasticity by disrupting the nNOS-CAPON interaction that links postsynaptic 5-HT1AR activation (Shi et al., 2022). Additionally, fluoxetine enhances hippocampal neuroplasticity by promoting axonal formation induced by growth-associated protein 43 (GAP-43) (Zavvari et al., 2020). Pre-treatment with fluoxetine has been shown to prevent stress-induced LTD and spatial memory deficits in the hippocampus of rats (Han et al., 2015). Citalopram, another SSRI, is composed of two enantiomers, R-citalopram and S-citalopram, which inhibit serotonin (5-HT) reuptake in the brain, thereby exerting an antidepressant effect (Yan et al., 2023a). When combined with Punica granatum, citalopram can alleviate damage to dendritic spines in the hippocampal DG region (Vega-Rivera et al., 2023).
Agomelatine, a synthetic analogue of melatonin, exerts its antidepressant effects by stimulating melatonin receptors (MT1 and MT2) and antagonizing 5-HT2C receptors (Maddukuri et al., 2021). Research indicates that agomelatine improves pathological behavior in stressed mice by modulating BDNF signaling, synaptic plasticity, and epigenetic remodeling (Martin et al., 2017). It also demonstrates beneficial effects in mitigating stress-induced brain damage, as it restores the activity of hippocampal neurons affected by stress and promotes adult hippocampal neurogenesis (Dagyte et al., 2010).
Ketamine, a non-competitive N-methyl-D-aspartate receptor (NMDAR) antagonist, specifically inhibits GluN2B-containing NMDARs on inhibitory GABAergic interneurons (Sato et al., 2022). Its antidepressant mechanism involves modulating neuroplasticity (Clarke et al., 2017), and low concentrations of ketamine (20 μM) can induce postsynaptic enhancement in the hippocampal CA1 (Kim and Monteggia, 2020). Its antidepressant effects are mediated by increased neuroplasticity, including synaptic actions (Kopelman et al., 2023), and it can “reset the system” by participating in synaptic plasticity processes to reverse stress-induced loss of key neural circuit connections (Aleksandrova et al., 2020). In animal models with chronic pain and depression, TIAM1-mediated synaptic plasticity is a crucial factor in the antidepressant effect of ketamine (Ru et al., 2022). Ketamine may also exert rapid antidepressant effects by enhancing neuroplasticity, triggering autophagy, and preventing ferroptosis in the nucleus (Zhang et al., 2022b). Studies have found that ketamine-induced hippocampal synaptic plasticity during antidepressant treatment depends on 4E binding proteins (Aguilar-Valles et al., 2021).
Metformin is the first-line treatment for type 2 diabetes, primarily acting by reducing liver gluconeogenesis and enhancing glucose metabolism. It also exhibits pleiotropic effects (LaMoia and Shulman, 2021). Beyond its antidiabetic role, metformin has been investigated for its potential in treating depression. Studies indicate that compared to other oral hypoglycemic drugs, metformin is associated with a lower risk of depression and demonstrates pleiotropic effects in depression management (Yu et al., 2022). Additionally, metformin can upregulate the expression of plasticity markers such as synapsin, sirtuin-1, AMP-activated protein kinase, and BDNF (Muñoz-Arenas et al., 2020). When combined with fluoxetine, metformin enhances the survival of NeuN-positive cells in the hippocampus and increases the number of BDNF-positive cells stimulated by fluoxetine, thereby enhancing its impact on neuroplasticity (Mendonça et al., 2022). Furthermore, metformin has been shown to mitigate synaptic plasticity damage induced by LPS in rats (Zhou et al., 2021) and improve the expression of synaptic plasticity markers [anti-microtubule-associated protein 2, synaptophysin (SYP), postsynaptic density protein 95], thereby alleviating depressive-like behavior in mice with allergic rhinitis (AR) (Lv et al., 2023). Hydrogen sulfide (H2S) is recognized as the third endogenous gas transmitter and can be produced in mammals through four enzyme pathways (Wu et al., 2018). H2S has been found to improve hippocampal synaptic plasticity in a Warburg-dependent manner, alleviating depression related to Parkinson’s disease (PD) (Liu et al., 2024) (Table 1).
In summary, neuroplasticity undergoes alterations in patients with depression and is also impaired in stress-induced, social isolation-induced, CORT-induced, and LPS-induced depression models. Substances like fluoxetine, ketamine, and metformin can mitigate depressive symptoms by modulating neuroplasticity, suggesting that targeted manipulation of neuroplasticity offers potential for treating depression. However, fluoxetine carries specific adverse effects in therapeutic contexts, including the potential for hallucinations, hepatotoxicity, neurotoxicity, and addiction, which may restrict its clinical application.
At present, the treatment of depression is a major issue in the medical field, and neuroplasticity is closely related to depression. Regulation based on neuroplasticity is one of the potential important measures for the treatment of depression. However, the current Western medicine for treating depression is mainly developed based on the “monoamine neurotransmitter hypothesis” of depression, but drug dependence and withdrawal reactions are common. Therefore, the development of new antidepressant drugs has become a hot topic at present. TCM has unique advantages in preventing and treating depression, including its overall concept, syndrome differentiation, and treatment methods, as well as the specific characteristics of its multiple components and targets, which are beneficial to the overall internal environment while treating depression (Zhuang et al., 2023). Numerous studies have shown that the active metabolites and herbal formulas in TCM are involved in regulating neuroplasticity during the process of antidepressant treatment.
Engeletin, a flavonoid metabolite initially extracted from the leaves of Astragalus mongholicus Bunge (Huang et al., 2011), is a potent natural metabolite with antioxidant and anti-inflammatory properties (Fang et al., 2023). Recent research has shown that Engeletin exerts antidepressant effects by activating the BDNF/TrkB/mTORC1 signaling pathway and enhancing synaptic plasticity in the prefrontal cortex (Xu et al., 2023). Baicalein, an important flavonoid found in the roots Scutellaria baicalensis Georgi, is frequently used in Chinese medicine and herbal tea preparations to promote wellbeing (Chandrashekar and Pandi, 2022). In preclinical studies of antidepressant effects, baicalein has been found to activate the BDNF/TrkB/CREB signaling pathway and protect against synaptic plasticity damage in mice with depression related to PD (Zhao et al., 2021). It also increases the ratio of mature BDNF (mBDNF) to proBDNF, regulates neuronal survival and synaptic plasticity, and suppresses neuroinflammation, effectively alleviating LPS-induced depressive symptoms in mice (Liu et al., 2022). Baicalin, extracted from S. baicalensis Georgi, has significant biological activity, including anti-inflammatory properties (Guo et al., 2019). Its antidepressant effect involves regulating the expression of synaptophysin (SYP), PSD95, BDNF, and TrkB, activating the Rac1-cofilin pathway, and enhancing synaptic plasticity (Lu et al., 2019).
Quercitrin, a naturally occurring flavonoid found in various fruits and vegetables, is commonly used as a dietary metabolite and supplement (Chen et al., 2022a). In mice with LPS-induced depression, quercitrin intervention could improve hippocampal damage, restore the abnormal expression of the pCREB/BDNF/PSD95/Synapsin1 pathway, regulates the PI3K/AKT/NF-κB signaling pathway, and enhances neuroplasticity (Sun et al., 2021). Luteolin, another natural flavonoid found in plants such as Chrysanthemum indicum L., Capsicum annuum L., and Perilla frutescens (L.) Britton has been studied for its pharmacological mechanism in treating late-onset depression, involving the regulation of neuroplasticity-related proteins (Li et al., 2021b; Liu et al., 2023; Rauf et al., 2024).
Soy isoflavones (SI), essential metabolites of Glycine max (L.) Merr., have different biological functions. SI can upregulate the expression of phosphorylated SYP (p-SYP) and PSD95 in the hippocampus of mice, inhibit neuroinflammation, regulate tryptophan metabolism, and reverse LPS-induced depressive behavior (Lu et al., 2022). Additionally, S-equol, a metabolite of dietary soy isoflavones, has demonstrated antidepressant effects by increasing synaptic plasticity proteins and inhibiting neuroinflammation (Lu et al., 2021). Silibinin, a polymorphic flavonoid extracted from milk thistle [Silybum marianum (L.) Gaertn.] (Ma et al., 2023), exerts its antidepressant effects by improving neuroplasticity and increasing neurotransmitter levels (Yan et al., 2015).
Polyphenols, metabolites widely distributed in a variety of plants, have garnered significant interest for their potential pharmacological actions, particularly their immune-stimulating and anticancer activities (Wang et al., 2022a). These metabolites have been found to enhance brain function by directly influencing cells and processes in the CNS (Grabska-Kobyłecka et al., 2023). Curcumin,a primary bioactive polyphenolic metabolite extracted from the rhizomes of Curcuma longa L., has been extensively studied for its therapeutic properties. In an ovariectomy-induced depression model, Curcumin was found to be a safe and effective regulator of 5-HT, similar to fluoxetine and neurotrophic E2, and was involved in regulating neuroplasticity (Abd-Rabo et al., 2019; Zia et al., 2021). Additionally, in the CUMS model, Curcumin was found to improve depressive-like behavior in animals by regulating the expression of synaptic plasticity proteins (Zhang et al., 2014).
The defining characteristic uniting the diverse class of chemicals known as alkaloids is the presence of a nitrogen atom in a heterocyclic ring (Ziegler and Facchini, 2008). Berberine, an isoquinoline alkaloid derived from the Chinese botanical drug Coptis chinensis Franch. and related Berberis species, possesses a broad variety of pharmacological effects (Song et al., 2020). Studies have shown that Berberine effectively treats depression by inhibiting neuroinflammation and improving gut microbiota (Zhu et al., 2017; Yang et al., 2023a). Berberine has multi-target and multi-pathway antidepressant characteristics (Gao et al., 2024). Recent research has emphasized the impact of Berberine on neuroplasticity in the context of depression. In mouse models of depression treated with Berberine, an increase in neuronal and synaptic plasticity has been observed. Berberine targets enzymes such as tryptophan 5-hydroxylase one and indoleamine 2,3-dioxygenase one involved in tryptophan metabolism, thereby improving depressive symptoms in CUMS stimulated mice (Ge et al., 2023). Additionally, Berberine’s antidepressant effect is accompanied by a reduction in neuroinflammatory responses through the inhibition of NLRP3 inflammasome activation, promoting plasticity and neurogenesis to alleviate neuronal damage (Qin et al., 2023).
Saponins, naturally occurring substances found in a wide range of plants, have garnered interest for their potential pharmacological properties (Zhang et al., 2023). Saikosaponin C, a metabolite purified from the traditional Chinese botanical drug Bupleurum chinense DC., has been studied for its effects on depression. Recent reports indicate that saikosaponin C reduces IL6 levels by inhibiting DNA methyltransferase one protein, leading to a decrease in IL6 expression. This metabolite promotes synaptic plasticity and alleviates depression-like behavior induced by chronic social defeat stress (Pan et al., 2019; Bai et al., 2023).
Ginsenoside Rb1, one of the main ginsenosides found in Panax ginseng C.A.Mey., is known for its neuroprotective properties (Ni et al., 2022). Research has shown that ginsenoside Rb1 can alleviate depressive symptoms induced by CUMS by modulating hippocampal synaptic plasticity through the miR-134-mediated BDNF signaling pathway (Wang et al., 2022b). Additionally, ginsenoside Rb1 regulates mitochondrial autophagy and the NF-κB pathway to inhibit astrocyte apoptosis, thereby reducing neuroinflammation and enhancing synaptic plasticity to maintain nervous system homeostasis (Li et al., 2023a). Ginsenoside Rg1, another key metabolite of P. ginseng C.A.Mey., has gained attention for its potential in preventing neurological diseases, especially dementia and depression (Yang et al., 2023b). It has been found to synergize with exercise in treating depression by reducing inflammation and improving neuroplasticity (Wang et al., 2023a).
The largest class of natural products is terpenoids, offering a plethora of potential therapeutic candidates (Huang et al., 2012). Gardenia jasminoides J. Ellis contains a type of iridoid glycoside called geniposide, which has various of biological benefits, including anti-neurodegenerative effects (Shen et al., 2020a). In a mouse model of postpartum depression, researchers observed excessive activation of the HPA axis and abnormal expression of proteins related to synaptic plasticity. Treatment with geniposide can alleviate these pathological phenomena and improve depressive-like behavior in mice (Ma et al., 2024). Xia et al. (2021) found that iridoids from Gardeniae fructus exerted antidepressant-like effects by stimulating AMPAR/mTOR signaling to enhance synaptic plasticity. For over a millennium, Paeonia lactiflora Pall. has been used in TCM to address ailments related to pain, inflammation, and the immune system (Zhang and Wei, 2020). Paeonia lactiflora Pall. produces a water-soluble monoterpene glycoside known as paeoniflorin (Cao et al., 2023), effectively reversing LTP damage induced by CUMS in the hippocampal CA1 region. Additionally, it can prevent CUMS-induced changes in dendritic spine density in the mouse hippocampus and downregulate BDNF and postsynaptic density protein 95 (PSD95) expression (Liu et al., 2019).
The pentacyclic triterpenoid chemical oleanolic acid (OA) is a naturally occurring substance extracted from various plants, including Olea europaea L. (Luo et al., 2024). Ursolic acid (UA) is another naturally occurring pentacyclic triterpenoid found in plants (Li et al., 2023b). Kong et al. (2023) conducted a study comparing the antidepressant effects of OA and UA, and found that in a depression model induced by CMS, OA was more effective than UA at reversing the depressive-like behavior induced by MS. In their mechanistic study, it was found that both OA and UA treatments reversed the decrease in synapsin expression levels caused by MS, but only OA upregulated the expression level of PSD-95 (Kong et al., 2023).
Polysaccharides are carbohydrate polymers composed of at least ten monosaccharides linked by glycosidic linkages (Yi et al., 2020). They are found in plants, microbes, bacteria, fungi, and seaweed all contain polysaccharides, playing crucial roles in various physiological processes (Chen et al., 2017). Post-traumatic stress disorder (PTSD) is a type of depression syndrome, and Xie et al. (2024) found that polysaccharides from Polygonatum cyrtonema Hua can improve PTSD-induced behavioral abnormalities and synaptic damage in mice by reducing oxidative stress and neuroinflammation, and by acting on the Nrf2/HO-1 signaling pathway. Inulin, a non-digestible fructan-type carbohydrate, was originally isolated from the roots of Inula helenium L. (Illippangama et al., 2022). Studies suggest that inulin improves neurogenesis and synaptic plasticity by enhancing CREB/BDNF signaling, prevents CUMS-induced reduction in blood-brain barrier permeability, reduces neuroinflammation, preserves intestinal barrier integrity, and promotes the production of short-chain fatty acids (SCFAs) (Wang et al., 2023b). Schisandra chinensis (Turcz.) Baill., belonging to the Magnoliaceae family and has been widely used as a medicinal plant in China for centuries. Modern pharmacological research has revealed the anti-inflammatory and anti-aging properties of S. chinensis and its active metabolites (Bian et al., 2022). Notably, studies have shown that the polysaccharide-rich fraction from S. chinensis (Turcz.) Baill. exhibits antidepressant effects in olfactory bulbectomized mice by enhancing abnormal synaptic plasticity (upregulating PSD95 expression), suppressing excessive activity of the HPA axis, and regulating gut microbiota (Zhu et al., 2024).
Botanical drugs extracts are considered valuable for their comprehensive active properties, driven by complex biochemical interactions and synergistic effects among their natural metabolites (Pace and Martinelli, 2022). Saffron (Crocus sativus L.), a well-known natural product, has long been used to prevent and treat different disorders (Ghaffari and Roshanravan, 2019). In rats exposed to chronic mild stress (CMS), repeated administration of doses of 100 mg/kg and 200 mg/kg doses of Saffron Extract (Affron®) effectively normalized HPA axis dysregulation. Moreover, hypothalamic neuroplasticity showed a significant dose-dependent increase following treatment with Saffron Extract (Affron®) (Kim et al., 2023). Blueberry (Vaccinium spp.), a member of the Vaccinium genus, is recognized as one of the top five nutritious foods for humans and is often referred to as the “king of fruits.” This reputation has fueled considerable interest in the market for plant-based prebiotics (Duan et al., 2022). Blueberry Extract has demonstrated efficacy in alleviating depression-like behavior in LPS-induced mice. It also mitigates the increase in acetylcholinesterase (AChE) activity in the hippocampus induced by LPS and inhibits the mRNA expression of TNF-α, IL-1β, and IL-10 in the cerebral cortex following LPS administration, indicating a potential protective effect on neuroplasticity (Spohr et al., 2023).
Honokiol is a versatile lignan metabolites naturally occurring in plants like Magnolia grandiflora L., known for its anti-inflammatory and neuroprotective effects (Rauf et al., 2021; Hu et al., 2023). Fan et al. (2022) found that the antidepressant mechanism of Honokiol involved the activation of the HIF-1α/VEGF signaling pathway and the upregulation of synaptic protein one and PSD 95 expression levels. Salidroside, an active metabolites found in Rhodiola rosea L. used in TCM, has various pharmacological effects (Xue et al., 2019). It enhances BDNF expression, improve synaptic plasticity, and inhibits pyroptosis mediated by the P2X7/NF-κB/NLRP3 signaling pathway, thereby providing a treatment for depression (Chai et al., 2022).
Crocin is a hydrophilic carotenoid produced in the blooms of C. sativus L., has been associated with promoting new nerve cell generation in the adult hippocampus and exerting antidepressant effects by activating the Wnt/β-catenin signaling pathway (Boozari and Hosseinzadeh, 2022; Tao et al., 2023a), Neurogenesis plays a key role in the physiological mechanism of structural neuroplasticity. Wu et al. (2020) also found that crocin rapidly and persistently induced antidepressant effects in mice subjected to Prenatal stress (PNS), acting through the GHSR-PI3K signaling pathway and modulating the expression of hippocampal synaptic plasticity-related proteins. Panaxynol, commonly found in plants of the P. ginseng C.A.Mey., can alleviate HPA axis overactivity induced by CUMS, promote the release of 5-HT and dopamine (DA), enhance hippocampal synaptic plasticity, and improve neurotransmitter effectiveness (Table 2 showed the active metabolites of TCM information) (Sun et al., 2020).
Zhi-Zi-Chi-Tang (ZZCT) is a potent traditional Chinese herbal remedy with a historical record in the “Shanghan Lun.” It consists of the dehydrated mature fruits of G. jasminoides J. Ellis and G. max (L.) Merr. In a rat depression model induced by CUMS, ZZCT enhances neuroplasticity through the 14–3–3ζ/GSK-3β/CREB/BDNF signaling pathway. It restores the expression of synaptic plasticity-related proteins like MAP2 and PSD95 in the hippocampal CA1 region, enhances LTP induction, and improves neuronal damage caused by CUMS (Tao et al., 2023b).
Zi-Shui-Qing-Gan-Yin (ZSQGY) is another traditional Chinese herbal remedy commonly used in China for depression symptoms. ZSQGY consists of 12 botanical drugs, including P. ginseng C.A.Mey et al. In a study conducted both in vivo and in vitro by Zhu et al. (2023), it was found that ZSQGY significantly improved depression-like behavior induced by monosodium glutamate (MSG) in rats. Further investigations revealed that ZSQGY improved synaptic ultrastructure by upregulating PGC-1α, regulating mitochondrial function, and inhibiting the expression of pro-inflammatory cytokines (Zhu et al., 2023).
The traditional remedy Danggui-Buxue Decoction (DBD), is taken from Li Dongyuan’s work on differentiating endogenous and exogenous diseases in the Jin and Yuan Dynasties (Shi et al., 2019). Studies suggest that DBD protects and reshapes hippocampal neurons by regulating the CREB/BDNF/TrkB pathway. It shows promise as a potential metabolite for preventing diabetes mellitus with depression (DD), with ferric acid potentially playing a crucial role in its effects (Wang et al., 2021). The Erzhi formula, composed of Ligustrum lucidum W.T.Aiton and Eclipta prostrata (L.) L., represents a TCM treatment (Peng et al., 2022). In an in vitro depression model, the Erzhi formula revealed the capacity to diminish dexamethasone-induced apoptosis in primary cultured cortical neurons and repair synaptic damage. Its neuroprotective effects were linked to the 11β-hydroxysteroid dehydrogenase 1 (HSD1)-glucocorticoids (GC)/glucocorticoid receptor (GR) signaling pathway (Han et al., 2023).
Xiaoyaosan, a TCM formula first introduced in the book “Prescriptions of the Bureau of Taiping People’s Welfare Pharmacy,” has a historical use in treating mental disorders, such as depression (Jiao et al., 2024). The ancient Chinese medicine pharmacopoeia also mentions Jiawei-Xiaoyao pill (JWX), a traditional Chinese medication, for the treatment of a variety of illnesses, including mood disorders. JWX consists of nine botanical drugs, including G. jasminoides J. Ellis et al. Studies have shown that JWX stimulates CaMKII signaling, leading to the activation of the mTOR/BDNF signaling pathway, Furthermore, it also enhances hippocampal neuroplasticity and triggering rapid antidepressant effects (Zhang et al., 2024a).
For more precise administration in patients with depression, Gao et al. (2018) introduced an empirical prescription called modified Xiaoshan (MXYS) based on Xiaoshan consisting of B. chinense DC et al. In a depression model induced by CUMS, MXYS was found to promote hippocampal neurogenesis and improve brain blood oxygen level-dependent signaling, indicating its potential therapeutic benefits for depression (Gao et al., 2018).
SiNiSan (SNS) is a TCM formula. Originally mentioned in the Treatise on Febrile Diseases for controlling liver qi (Cao et al., 2024), SNS has been shown to regulate neuroplasticity by activating the Calcium sensitive receptor (CaSR)-protein kinase C (PKC)-ERK signaling pathway. It also helps in regulating mitochondrial function and improving neuroplasticity (Shen et al., 2020b; Deng et al., 2022). Suanzaoren Decoction (SZRD), a TCM combination with a history of insomnia treatment (Dong et al., 2021; Yan et al., 2023b). Research by Du et al. (2024) using in vivo and in vitro experiments demonstrated that SZRD increases the expression levels of BDNF, SYP, and PSD95. It also inhibits the activation of the TLR4/MyD88/NF-κB and Wnt/β-catenin pathways, showing antidepressant effects, and SZRD could also adjust the CaMK signal system (Zhang et al., 2024b; Du et al., 2024).
Zhi-Zi Hou-Po Decoction (ZZHP), a TCM formula widely used in depression treatment (Feng et al., 2022). Studies suggest that ZZHP effectively reverses the decrease of monoamine neurotransmitters in the hippocampus, maintains their homeostasis, activates the BDNF/TrkB/CREB pathway, protects neuronal synaptic plasticity, promotes hippocampal neurogenesis, and alleviates depression-like symptoms in mice caused by CUMS (Ye et al., 2024). Kaiyu Zhishen Decoction (KZD) is composed of botanical drugs such as P. lactiflora Pall. Chen et al. (2024) found through network pharmacology and experimental verification that the antidepressant effect of KZD involves regulating the ERK-CREB-BDNF signaling pathway and promoting neuronal repair, potentially regulating neuroplasticity (Figure 3 showed the mechanism of TCM action and Table 3 showed the TCM formulas information).
Figure 3. The pharmacological mechanism of TCM regulation of neuroplasticity in the treatment of depression. The red arrow indicates changes caused by stress, while the green arrow indicates changes caused by TCM.
Depression is a common long-lasting mental disorder marked by enduring feelings of sadness, low self-esteem, and potentially dangerous suicidal ideation. Understanding the pathogenesis of depression remains a challenge in modern medicine, and there is a deficiency of therapeutic strategies that may effectively prevent or entirely reverse depression (Chen et al., 2022b; Xia et al., 2023). At now, great progress has been achieved in the study of depression, both at the preclinical level and at the fundamental research level. Multiple chemicals with antidepressant effects have been developed in some clinical treatments, but there are still certain side effects and insufficient efficacy. In addition, there is a lack of suitable and appropriate depression prediction tools in clinical practice. Currently, finding antidepressant drugs with multiple targets, high safety, good efficacy, and minimal adverse reactions is a major task.
In recent years, TCM has received attention and promotion, and has been vigorously developed in various aspects. In the research of antidepressants, TCM has gradually become the focus and hotspot of research. In the treatment of depression, it is crucial to explore how TCM can complement Western medicine approaches, leveraging the strengths of TCM’s multi-target effects and individualized treatments. Research in multi-target antidepressant therapies is essential to achieve outcomes comparable to modern medical “cocktail therapy.” TCM offers multiple advantages, including its emphasis on multiple targets and individualized treatment in line with the principles of precision medicine. Active metabolites in TCM, such as flavonoids, polyphenols, alkaloids, saponins, terpenes, polysaccharides, and TCM extracts, along with TCM formulas such as ZZCT, ZSQGY, DBD, Erzhi formula, JWX, MXYS, SNS, SZRD, KZD and ZZHP, play a role in regulating neuroplasticity through various targets and pathways when exerting antidepressant effects.
However, the causes and mechanisms of depression have not been fully elucidated, and there is a lack of unified and relatively authoritative methods for evaluating depression symptoms in clinical practice. There is no clear standard for the specific indicators of depression. More importantly, current research mostly focuses on the in vivo or in vitro levels, lacking high-quality clinical research on active metabolites and TCM formulas. Most studies only explore the mechanism of drug action, and the connection between TCM theory and neuroplasticity has not been thoroughly investigated. Furthermore, compared to the active metabolites of TCM, research on TCM formulas is relatively weak, and the diversity and depth of neuroplasticity-related signaling pathways explored are insufficient. There is no active substance in the world that not only exerts its pharmacological effects but also has non-specific off-target effects on normal tissues of the body (Guo et al., 2023). In current research on antidepressants, there has been insufficient exploration of the toxicology and side effects of TCM.
In addition, some Chinese herbal medicines lack clear quality control standards, compromising the stability and consistency of their chemical metabolites, limiting their clinical application and complicating the study of their pharmacological mechanisms. Furthermore, certain active metabolites of TCM face challenges such as poor stability, solubility issues, and difficulty in crossing the blood-brain barrier, which need further investigation to ascertain their efficacy in targeting CNS organs. The mechanism of neuroplasticity is complex, involving multiple signaling pathways and cell coordination. While TCM possesses the advantage of targeting multiple pathways, current research predominantly focuses on single signaling pathways with limited detection indicators. This approach fails to comprehensively elucidate the synergistic mechanisms underlying TCM’s multi-target and multi-pathway regulation of neuroplasticity.
Therefore, in future research, multicenter, large-sample clinical randomized controlled trials guided by TCM theory should be conducted to explore the efficacy and safety of TCM in treating depression, as well as the regulatory mechanisms of neuroplasticity, aiming to provide deeper insights into how TCM works in antidepressant treatment. Simultaneously, it is essential to enhance the quality control standards for TCM and strengthen the exploration of targeted delivery systems for TCM to increase the concentration and duration of TCM in target organs, thereby improving the therapeutic outcomes. Furthermore, focusing on cutting-edge technologies such as combined single-cell sequencing and spatial transcriptomics is necessary to further reveal the key regulatory targets of TCM and the regulatory mechanisms of neuroplasticity at different time points and cell types. This review systematically elucidated the role of neuroplasticity in the pathological development of depression and the regulatory role of TCM. In conclusion, substantial research efforts are still needed to fully explore the potential of TCM in modulating neuroplasticity for the prevention and treatment of depression.
SL: Writing–original draft. NY: Writing–review and editing. YL: Writing–review and editing. GZ: Writing–review and editing. XZ: Writing–review and editing. YC: Writing–review and editing. YH: Writing–review and editing. JT: Writing–review and editing. YS: Writing–review and editing.
The author(s) declare that financial support was received for the research, authorship, and/or publication of this article. This work was supported by the Shandong Province Special Disease Prevention Project of Integrated Traditional Chinese and Western Medicine (YXH2019ZXY006) and Academic Project for College Students of Shandong Province Youth Education Science Planning Project (24BSH467).
The authors declare that the research was conducted in the absence of any commercial or financial relationships that could be construed as a potential conflict of interest.
All claims expressed in this article are solely those of the authors and do not necessarily represent those of their affiliated organizations, or those of the publisher, the editors and the reviewers. Any product that may be evaluated in this article, or claim that may be made by its manufacturer, is not guaranteed or endorsed by the publisher.
Abd-Rabo, M. M., Georgy, G. S., Saied, N. M., and Hassan, W. A. (2019). Involvement of the serotonergic system and neuroplasticity in the antidepressant effect of curcumin in ovariectomized rats: comparison with oestradiol and fluoxetine. Phytother. Res. 33 (2), 387–396. doi:10.1002/ptr.6232
Aceto, G., Colussi, C., Leone, L., Fusco, S., Rinaudo, M., Scala, F., et al. (2020). Chronic mild stress alters synaptic plasticity in the nucleus accumbens through GSK3β-dependent modulation of Kv4.2 channels. Proc. Natl. Acad. Sci. U. S. A. 117 (14), 8143–8153. doi:10.1073/pnas.1917423117
Aguilar-Valles, A., De Gregorio, D., Matta-Camacho, E., Eslamizade, M. J., Khlaifia, A., Skaleka, A., et al. (2021). Antidepressant actions of ketamine engage cell-specific translation via eIF4E. Nature 590 (7845), 315–319. doi:10.1038/s41586-020-03047-0
Aleksandrova, L. R., Wang, Y. T., and Phillips, A. G. (2020). Ketamine and its metabolite, (2R,6R)-HNK, restore hippocampal LTP and long-term spatial memory in the Wistar-Kyoto rat model of depression. Mol. Brain. 13 (1), 92. doi:10.1186/s13041-020-00627-z
An, J. H., Jang, E. H., Kim, A. Y., Fava, M., Mischoulon, D., Papakostas, G. I., et al. (2019). Ratio of plasma BDNF to leptin levels are associated with treatment response in major depressive disorder but not in panic disorder: a 12-week follow-up study. J. Affect. Disord. 259, 349–354. doi:10.1016/j.jad.2019.08.021
Bai, Z., Gao, T., Zhang, R., Lu, Y., Tian, J., Wang, T., et al. (2023). Inhibition of IL-6 methylation by Saikosaponin C regulates neuroinflammation to alleviate depression. Int. Immunopharmacol. 118, 110043. doi:10.1016/j.intimp.2023.110043
Bayes, J., Schloss, J., and Sibbritt, D. (2020). Effects of polyphenols in a mediterranean diet on symptoms of depression: a systematic literature review. Adv. Nutr. 11 (3), 602–615. doi:10.1093/advances/nmz117
Beurel, E., Toups, M., and Nemeroff, C. B. (2020). The bidirectional relationship of depression and inflammation: double trouble. Neuron 107 (2), 234–256. doi:10.1016/j.neuron.2020.06.002
Bian, Z., Qin, Y., Li, L., Su, L., Fei, C., Li, Y., et al. (2022). Schisandra chinensis (Turcz.) Baill. Protects against DSS-induced colitis in mice: involvement of TLR4/NF-κB/NLRP3 inflammasome pathway and gut microbiota. J. Ethnopharmacol. 298, 115570. doi:10.1016/j.jep.2022.115570
Boozari, M., and Hosseinzadeh, H. (2022). Crocin molecular signaling pathways at a glance: a comprehensive review. Phytother. Res. 36 (10), 3859–3884. doi:10.1002/ptr.7583
Bourtchuladze, R., Frenguelli, B., Blendy, J., Cioffi, D., Schutz, G., and Silva, A. J. (1994). Deficient long-term memory in mice with a targeted mutation of the cAMP-responsive element-binding protein. Cell. 79 (1), 59–68. doi:10.1016/0092-8674(94)90400-6
Cao, K., Zhong, J., Wang, S., Shi, Y., Bai, S., Zhao, J., et al. (2024). SiNiSan exerts antidepressant effects by modulating serotonergic/GABAergic neuron activity in the dorsal raphe nucleus region through NMDA receptor in the adolescent depression mouse model. J. Ethnopharmacol. 328, 118040. doi:10.1016/j.jep.2024.118040
Cao, Y., Xiong, J., Guan, X., Yin, S., Chen, J., Yuan, S., et al. (2023). Paeoniflorin suppresses kidney inflammation by regulating macrophage polarization via KLF4-mediated mitophagy. Phytomedicine 116, 154901. doi:10.1016/j.phymed.2023.154901
Castillo, P. E. (2012). Presynaptic LTP and LTD of excitatory and inhibitory synapses. Cold. Spring. Harb. Perspect. Biol. 4 (2), a005728. doi:10.1101/cshperspect.a005728
Chai, Y., Cai, Y., Fu, Y., Wang, Y., Zhang, Y., Zhang, X., et al. (2022). Salidroside ameliorates depression by suppressing NLRP3-mediated pyroptosis via P2X7/NF-κB/NLRP3 signaling pathway. Front. Pharmacol. 13, 812362. doi:10.3389/fphar.2022.812362
Chandrashekar, N., and Pandi, A. (2022). Baicalein: a review on its anti-cancer effects and mechanisms in lung carcinoma. J. Food. Biochem. 46 (9), e14230. doi:10.1111/jfbc.14230
Chen, J., Lei, C., Li, X., Wu, Q., Liu, C., Ma, Q., et al. (2022b). Research progress on classical traditional Chinese medicine formula xiaoyaosan in the treatment of depression. Front. Pharmacol. 13, 925514. doi:10.3389/fphar.2022.925514
Chen, J., Li, G., Sun, C., Peng, F., Yu, L., Chen, Y., et al. (2022a). Chemistry, pharmacokinetics, pharmacological activities, and toxicity of Quercitrin. Phytother. Res. 36, 1545–1575. doi:10.1002/ptr.7397
Chen, Q., Shao, X., Ling, P., Liu, F., Han, G., and Wang, F. (2017). Recent advances in polysaccharides for osteoarthritis therapy. Eur. J. Med. Chem. 139, 926–935. doi:10.1016/j.ejmech.2017.08.048
Chen, Y., Chen, X., Zhang, J., Wang, D., Lu, N., et al. (2024). Network pharmacology and experimental evidence: ERK/CREB/BDNF signaling pathway is involved in the antidepressive roles of Kaiyu Zhishen decoction. J. Ethnopharmacol. 329, 118098. doi:10.1016/j.jep.2024.118098
Choi, G. E., Lee, H. J., Chae, C. W., Cho, J. H., Jung, Y. H., Kim, J. S., et al. (2021). BNIP3L/NIX-mediated mitophagy protects against glucocorticoid-induced synapse defects. Nat. Commun. 12 (1), 487. doi:10.1038/s41467-020-20679-y
Clarke, M., Razmjou, S., Prowse, N., Dwyer, Z., Litteljohn, D., Pentz, R., et al. (2017). Ketamine modulates hippocampal neurogenesis and pro-inflammatory cytokines but not stressor induced neurochemical changes. Neuropharmacology 112 (Pt A), 210–220. doi:10.1016/j.neuropharm.2016.04.021
Crupi, R., Paterniti, I., Ahmad, A., Campolo, M., Esposito, E., and Cuzzocrea, S. (2013). Effects of palmitoylethanolamide and luteolin in an animal model of anxiety/depression. CNS. Neurol. Disord. Drug. Targets. 12 (7), 989–1001. doi:10.2174/18715273113129990084
Dagyte, G., Trentani, A., Postema, F., Luiten, P. G., Den Boer, J. A., Gabriel, C., et al. (2010). The novel antidepressant agomelatine normalizes hippocampal neuronal activity and promotes neurogenesis in chronically stressed rats. CNS. Neurosci. Ther. 16 (4), 195–207. doi:10.1111/j.1755-5949.2009.00125.x
Deng, D., Cui, Y., Gan, S., Xie, Z., Cui, S., Cao, K., et al. (2022). Sinisan alleviates depression-like behaviors by regulating mitochondrial function and synaptic plasticity in maternal separation rats. Phytomedicine 106, 154395. doi:10.1016/j.phymed.2022.154395
De Paola, V., Holtmaat, A., Knott, G., Song, S., Wilbrecht, L., Caroni, P., et al. (2006). Cell type-specific structural plasticity of axonal branches and boutons in the adult neocortex. Neuron 49 (6), 861–875. doi:10.1016/j.neuron.2006.02.017
Diering, G. H., and Huganir, R. L. (2018). The AMPA receptor code of synaptic plasticity. Neuron 100 (2), 314–329. doi:10.1016/j.neuron.2018.10.018
Dong, Y. J., Jiang, N. H., Zhan, L. H., Teng, X., Fang, X., Lin, M. Q., et al. (2021). Soporific effect of modified Suanzaoren Decoction on mice models of insomnia by regulating Orexin-A and HPA axis homeostasis. Biomed. Pharmacother. 143, 112141. doi:10.1016/j.biopha.2021.112141
Du, Y., Yan, T., Wu, B., He, B., and Jia, Y. (2024). Research on the mechanism of antidepressive effect of Suanzaoren Decoction through TLR4/MyD88/NF-κB pathway and Wnt/β-catenin pathway. J. Ethnopharmacol. 319 (Pt 1), 117190. doi:10.1016/j.jep.2023.117190
Duan, Y., Tarafdar, A., Chaurasia, D., Singh, A., Bhargava, P. C., Yang, J., et al. (2022). Blueberry fruit valorization and valuable constituents: a review. Int. J. Food. Microbiol. 381, 109890. doi:10.1016/j.ijfoodmicro.2022.109890
Duman, R. S., Aghajanian, G. K., Sanacora, G., and Krystal, J. H. (2016). Synaptic plasticity and depression: new insights from stress and rapid-acting antidepressants. Nat. Med. 22 (3), 238–249. doi:10.1038/nm.4050
Dzyubenko, E., and Hermann, D. M. (2023). Role of glia and extracellular matrix in controlling neuroplasticity in the central nervous system. Semin. Immunopatho. 45 (3), 377–387. doi:10.1007/s00281-023-00989-1
Fan, X. X., Sun, W. Y., Li, Y., Tang, Q., Li, L. N., Yu, X., et al. (2022). Honokiol improves depression-like behaviors in rats by HIF-1α- VEGF signaling pathway activation. Front. Pharmacol. 13, 968124. doi:10.3389/fphar.2022.968124
Fang, Z., Liu, Z., Tao, B., and Jiang, X. (2023). Engeletin mediates antiarrhythmic effects in mice with isoproterenol-induced cardiac remodeling. Biomed. Pharmacother. 161, 114439. doi:10.1016/j.biopha.2023.114439
Feng, X., Bi, Y., Wang, J., Li, T., Yu, G., Zhang, T., et al. (2022). Discovery of the potential novel pharmacodynamic substances from zhi-zi-hou-Po decoction based on the concept of Co-decoction reaction and analysis strategy. Front. Pharmacol. 12, 830558. doi:10.3389/fphar.2021.830558
Filipović, D., Novak, B., Xiao, J., Yan, Y., Bernardi, R. E., and Turck, C. W. (2023). Chronic fluoxetine treatment in socially-isolated rats modulates the prefrontal cortex synaptoproteome. J. Proteomics. 282, 104925. doi:10.1016/j.jprot.2023.104925
Filipović, D., Novak, B., Xiao, J., Yan, Y., Yeoh, K., and Turck, C. W. (2022). Chronic fluoxetine treatment of socially isolated rats modulates prefrontal cortex proteome. Neuroscience 501, 52–71. doi:10.1016/j.neuroscience.2022.08.011
Frankiensztajn, L. M., Elliott, E., and Koren, O. (2020). The microbiota and the hypothalamus-pituitary-adrenocortical (HPA) axis, implications for anxiety and stress disorders. Curr. Opin. Neurobiol. 62, 76–82. doi:10.1016/j.conb.2019.12.003
Freitas, A. E., Egea, J., Buendia, I., Gómez-Rangel, V., Parada, E., Navarro, E., et al. (2016). Agmatine, by improving neuroplasticity markers and inducing Nrf2, prevents corticosterone-induced depressive-like behavior in mice. Mol. Neurobiol. 53 (5), 3030–3045. doi:10.1007/s12035-015-9182-6
Gao, L., Huang, P., Dong, Z., Gao, T., Huang, S., Zhou, C., et al. (2018). Modified xiaoyaosan (MXYS) exerts anti-depressive effects by rectifying the brain blood oxygen level-dependent fMRI signals and improving hippocampal neurogenesis in mice. Front. Pharmacol. 9, 1098. doi:10.3389/fphar.2018.01098
Gao, Y., Nie, K., Wang, H., Dong, H., and Tang, Y. (2024). Research progress on antidepressant effects and mechanisms of berberine. Front. Pharmacol. 15, 1331440. doi:10.3389/fphar.2024.1331440
Ge, P. Y., Qu, S. Y., Ni, S. J., Yao, Z. Y., Qi, Y. Y., Zhao, X., et al. (2023). Berberine ameliorates depression-like behavior in CUMS mice by activating TPH1 and inhibiting Ido1-associated with tryptophan metabolism. Phytother. Res. 37 (1), 342–357. doi:10.1002/ptr.7616
Ghaffari, S., and Roshanravan, N. (2019). Saffron; an updated review on biological properties with special focus on cardiovascular effects. Biomed. Pharmacother. 109, 21–27. doi:10.1016/j.biopha.2018.10.031
Grabska-Kobyłecka, I., Szpakowski, P., Król, A., Książek-Winiarek, D., Kobyłecki, A., Głąbiński, A., et al. (2023). Polyphenols and their impact on the prevention of neurodegenerative diseases and development. Nutrients 15 (15), 3454. doi:10.3390/nu15153454
Guo, C., Huang, Q., Wang, Y., Yao, Y., Li, J., Chen, J., et al. (2023). Therapeutic application of natural products: NAD+ metabolism as potential target. Phytomedicine 114, 154768. doi:10.1016/j.phymed.2023.154768
Guo, L. T., Wang, S. Q., Su, J., Xu, L. X., Ji, Z. Y., Zhang, R. Y., et al. (2019). Baicalin ameliorates neuroinflammation-induced depressive-like behavior through inhibition of toll-like receptor 4 expression via the PI3K/AKT/FoxO1 pathway. J. Neuroinflammation 16 (1), 95. doi:10.1186/s12974-019-1474-8
Han, H., Dai, C., and Dong, Z. (2015). Single fluoxetine treatment before but not after stress prevents stress-induced hippocampal long-term depression and spatial memory retrieval impairment in rats. Sci. Rep. 5, 12667. doi:10.1038/srep12667
Han, R., Han, G., Yan, Y., Han, L., Li, L., and Zhang, H. (2023). Protective effects and mechanisms of the Erzhi formula on glucocorticoid induced primary cortical neuron injury. Front. Pharmacol. 14, 1038492. doi:10.3389/fphar.2023.1038492
Hidese, S., Hattori, K., Sasayama, D., Tsumagari, T., Miyakawa, T., Matsumura, R., et al. (2020). Cerebrospinal fluid neuroplasticity-associated protein levels in patients with psychiatric disorders: a multiplex immunoassay study. Transl. Psychiatry. 10 (1), 161. doi:10.1038/s41398-020-0843-5
Hu, M., Jiang, W., Ye, C., Hu, T., Yu, Q., Meng, M., et al. (2023). Honokiol attenuates high glucose-induced peripheral neuropathy via inhibiting ferroptosis and activating AMPK/SIRT1/PGC-1α pathway in Schwann cells. Phytother. Res. 37 (12), 5787–5802. doi:10.1002/ptr.7984
Huang, H., Cheng, Z., Shi, H., Xin, W., Wang, T. T., and Yu, L. L. (2011). Isolation and characterization of two flavonoids, engeletin and astilbin, from the leaves of Engelhardia roxburghiana and their potential anti-inflammatory properties. J. Agric. Food. Chem. 59 (9), 4562–4569. doi:10.1021/jf2002969
Huang, J., Shen, C., Ye, R., Shi, Y., and Li, W. (2021). The effect of early maternal separation combined with adolescent chronic unpredictable mild stress on behavior and synaptic plasticity in adult female rats. Front. Psychiatry. 12, 539299. doi:10.3389/fpsyt.2021.539299
Huang, M., Lu, J. J., Huang, M. Q., Bao, J. L., Chen, X. P., and Wang, Y. T. (2012). Terpenoids: natural products for cancer therapy. Expert. Opin. Investig. Drugs. 21 (12), 1801–1818. doi:10.1517/13543784.2012.727395
Hueston, C. M., Cryan, J. F., and Nolan, Y. M. (2017). Adolescent social isolation stress unmasks the combined effects of adolescent exercise and adult inflammation on hippocampal neurogenesis and behavior. Neuroscience 365, 226–236. doi:10.1016/j.neuroscience.2017.09.020
Illippangama, A. U., Jayasena, D. D., Jo, C., and Mudannayake, D. C. (2022). Inulin as a functional ingredient and their applications in meat products. Carbohydr. Polym. 275, 118706. doi:10.1016/j.carbpol.2021.118706
Jaremka, L. M., Andridge, R. R., Fagundes, C. P., Alfano, C. M., Povoski, S. P., Lipari, A. M., et al. (2014). Pain, depression, and fatigue: loneliness as a longitudinal risk factor. Health. Psychol. 33 (9), 948–957. doi:10.1037/a0034012
Jiao, H., Fan, Y., Gong, A., Li, T., Fu, X., and Yan, Z. (2024). Xiaoyaosan ameliorates CUMS-induced depressive-like and anorexia behaviors in mice via necroptosis related cellular senescence in hypothalamus. J. Ethnopharmacol. 318 (Pt A), 116938. doi:10.1016/j.jep.2023.116938
Jo, J. H., Park, E. J., Lee, J. K., Jung, M. W., and Lee, C. J. (2001). Lipopolysaccharide inhibits induction of long-term potentiation and depression in the rat hippocampal CA1 area. Eur. J. Pharmacol. 422 (1-3), 69–76. doi:10.1016/s0014-2999(01)01075-5
Kaneko, N., Wada, M., Nakajima, S., Takano, M., Taniguchi, K., Honda, S., et al. (2024). Neuroplasticity of the left dorsolateral prefrontal cortex in patients with treatment-resistant depression as indexed with paired associative stimulation: a TMS-EEG study. Cereb. Cortex 34 (2), bhad515. doi:10.1093/cercor/bhad515
Karin, O., Raz, M., Tendler, A., Bar, A., Korem Kohanim, Y., Milo, T., et al. (2020). A new model for the HPA axis explains dysregulation of stress hormones on the timescale of weeks. Mol. Syst. Biol. 16 (7), e9510. doi:10.15252/msb.20209510
Kim, C. Y., Ko, K., Choi, S. H., Jo, M., Kim, J., Yoon, S., et al. (2023). Effects of Saffron extract (Affron®) with 100 mg/kg and 200 mg/kg on hypothalamic-pituitary-adrenal Axis and stress resilience in chronic mild stress-induced depression in wistar rats. Nutrients 15 (23), 4855. doi:10.3390/nu15234855
Kim, J. W., and Monteggia, L. M. (2020). Increasing doses of ketamine curtail antidepressant responses and suppress associated synaptic signaling pathways. Behav. Brain. Res. 380, 112378. doi:10.1016/j.bbr.2019.112378
Knott, G. W., Holtmaat, A., Wilbrecht, L., Welker, E., and Svoboda, K. (2006). Spine growth precedes synapse formation in the adult neocortex in vivo. Nat. Neurosci. 9 (9), 1117–1124. doi:10.1038/nn1747
Kokkosis, A. G., Madeira, M. M., Hage, Z., Valais, K., Koliatsis, D., Resutov, E., et al. (2024). Chronic psychosocial stress triggers microglial-/macrophage-induced inflammatory responses leading to neuronal dysfunction and depressive-related behavior. Glia 72 (1), 111–132. doi:10.1002/glia.24464
Kong, C. H., Park, K., Kim, D. Y., Kim, J. Y., Kang, W. C., Jeon, M., et al. (2023). Effects of oleanolic acid and ursolic acid on depression-like behaviors induced by maternal separation in mice. Eur. J. Pharmacol. 956, 175954. doi:10.1016/j.ejphar.2023.175954
Kopelman, J., Keller, T. A., Panny, B., Griffo, A., Degutis, M., Spotts, C., et al. (2023). Rapid neuroplasticity changes and response to intravenous ketamine: a randomized controlled trial in treatment-resistant depression. Transl. Psychiatry. 13 (1), 159. doi:10.1038/s41398-023-02451-0
Kuhn, M., Mainberger, F., Feige, B., Maier, J. G., Wirminghaus, M., Limbach, L., et al. (2016). State-dependent partial occlusion of cortical LTP-like plasticity in major depression. Neuropsychopharmacology 41 (6), 1521–1529. doi:10.1038/npp.2015.310
LaMoia, T. E., and Shulman, G. I. (2021). Cellular and molecular mechanisms of metformin action. Endocr. Rev. 42 (1), 77–96. doi:10.1210/endrev/bnaa023
Li, B., Du, P., Du, Y., Zhao, D., Cai, Y., Yang, Q., et al. (2021b). Luteolin alleviates inflammation and modulates gut microbiota in ulcerative colitis rats. Life. Sci. 269, 119008. doi:10.1016/j.lfs.2020.119008
Li, J., Tian, S., Wang, H., Wang, Y., Du, C., Fang, J., et al. (2021a). Protection of hUC-MSCs against neuronal complement C3a receptor-mediated NLRP3 activation in CUMS-induced mice. Neurosci. Lett. 741, 135485. doi:10.1016/j.neulet.2020.135485
Li, Y., Li, J., Yang, L., Ren, F., Dong, K., Zhao, Z., et al. (2023a). Ginsenoside Rb1 protects hippocampal neurons in depressed rats based on mitophagy-regulated astrocytic pyroptosis. Phytomedicine 121, 155083. doi:10.1016/j.phymed.2023.155083
Li, Y., Zhao, L., Zhao, Q., Zhou, Y., Zhou, L., Song, P., et al. (2023b). Ursolic acid nanoparticles for glioblastoma therapy. Nanomedicine 50, 102684. doi:10.1016/j.nano.2023.102684
Li, Z. R., Liu, D. G., Xie, S., Wang, Y. H., Han, Y. S., Li, C. Y., et al. (2022). Sleep deprivation leads to further impairment of hippocampal synaptic plasticity by suppressing melatonin secretion in the pineal gland of chronically unpredictable stress rats. Eur. J. Pharmacol. 930, 175149. doi:10.1016/j.ejphar.2022.175149
Liu, F., Tian, Q., Tang, H. L., Cheng, X., Zou, W., and Zhang, P. (2024). Hydrogen sulfide attenuates depression-like behaviours in Parkinson's disease model rats by improving synaptic plasticity in a hippocampal Warburg effect-dependent manner. Pharmacol. Biochem. Behav. 234, 173677. doi:10.1016/j.pbb.2023.173677
Liu, H. T., Lin, Y. N., Tsai, M. C., Wu, Y. C., and Lee, M. C. (2022). Baicalein exerts therapeutic effects against endotoxin-induced depression-like behavior in mice by decreasing inflammatory cytokines and increasing brain-derived neurotrophic factor levels. Antioxidants (Basel) 11 (5), 947. doi:10.3390/antiox11050947
Liu, K., Li, H., Zeng, N., Li, B., Yao, G., Wu, X., et al. (2023). Exploration of the core pathways and potential targets of luteolin treatment on late-onset depression based on cerebrospinal fluid proteomics. Int. J. Mol. Sci. 24 (4), 3485. doi:10.3390/ijms24043485
Liu, N., Wang, Y., An, A. Y., Banker, C., Qian, Y. H., and O'Donnell, J. M. (2020). Single housing-induced effects on cognitive impairment and depression-like behavior in male and female mice involve neuroplasticity-related signaling. Eur. J. Neurosci. 52 (1), 2694–2704. doi:10.1111/ejn.14565
Liu, S. C., Hu, W. Y., Zhang, W. Y., Yang, L., Li, Y., Xiao, Z. C., et al. (2019). Paeoniflorin attenuates impairment of spatial learning and hippocampal long-term potentiation in mice subjected to chronic unpredictable mild stress. Psychopharmacol. Berl. 236 (9), 2823–2834. doi:10.1007/s00213-019-05257-5
Lu, C., Gao, R., Zhang, Y., Jiang, N., Chen, Y., Sun, J., et al. (2021). S-equol, a metabolite of dietary soy isoflavones, alleviates lipopolysaccharide-induced depressive-like behavior in mice by inhibiting neuroinflammation and enhancing synaptic plasticity. Food. Funct. 12 (13), 5770–5778. doi:10.1039/d1fo00547b
Lu, C., Wei, Z., Wang, Y., Li, S., Tong, L., Liu, X., et al. (2022). Soy isoflavones alleviate lipopolysaccharide-induced depressive-like behavior by suppressing neuroinflammation, mediating tryptophan metabolism and promoting synaptic plasticity. Food. Funct. 13 (18), 9513–9522. doi:10.1039/d2fo01437h
Lu, Y., Sun, G., Yang, F., Guan, Z., Zhang, Z., Zhao, J., et al. (2019). Baicalin regulates depression behavior in mice exposed to chronic mild stress via the Rac/LIMK/cofilin pathway. Biomed. Pharmacother. 116, 109054. doi:10.1016/j.biopha.2019.109054
Luo, Q., Wei, Y., Lv, X., Chen, W., Yang, D., and Tuo, Q. (2024). The effect and mechanism of oleanolic acid in the treatment of metabolic syndrome and related cardiovascular diseases. Molecules 29 (4), 758. doi:10.3390/molecules29040758
Lv, H., Gao, Z., Wang, Y., Chen, S., Liu, P., Xie, Y., et al. (2023). Metformin improves comorbid depressive symptoms in mice with allergic rhinitis by reducing olfactory bulb damage. Neurochem. Res. 48 (12), 3639–3651. doi:10.1007/s11064-023-04012-9
Ma, X., Xie, Y., Gong, Y., Hu, C., Qiu, K., Yang, Y., et al. (2023). Silibinin prevents tgfβ-induced EMT of RPE in proliferative vitreoretinopathy by inhibiting Stat3 and Smad3 phosphorylation. Invest. Ophthalmol. Vis. Sci. 64 (13), 47. doi:10.1167/iovs.64.13.47
Ma, Y., Li, S. X., Zhou, R. Y., Deng, L. J., le He, W., Guo, L. L., et al. (2024). Geniposide improves depression-like behavior in prenatal stress male offspring through restoring HPA axis- and glucocorticoid receptor-associated dysfunction. Life. Sci. 340, 122434. doi:10.1016/j.lfs.2024.122434
Maddukuri, R. K., Hema, C., Sri Tejaswi, K., Venkata Mounika, M., and Vegesana, B. P. (2021). Antidepressant efficacy of Agomelatine: meta-analysis of placebo controlled and active comparator studies. Asian. J. Psychiatr. 65, 102866. doi:10.1016/j.ajp.2021.102866
Marsden, W. N. (2013). Synaptic plasticity in depression: molecular, cellular and functional correlates. Prog. Neuropsychopharmacol. Biol. Psychiatry 43, 168–184. doi:10.1016/j.pnpbp.2012.12.012
Martin, V., Allaïli, N., Euvrard, M., Marday, T., Riffaud, A., Franc, B., et al. (2017). Effect of agomelatine on memory deficits and hippocampal gene expression induced by chronic social defeat stress in mice. Sci. Rep. 8, 45907. doi:10.1038/srep45907
Medendorp, W. E., Petersen, E. D., Pal, A., Wagner, L. M., Myers, A. R., Hochgeschwender, U., et al. (2018). Altered behavior in mice socially isolated during adolescence corresponds with immature dendritic spine morphology and impaired plasticity in the prefrontal cortex. Front. Behav. Neurosci. 12, 87. doi:10.3389/fnbeh.2018.00087
Mendonça, I. P., Paiva, I. H. R., Duarte-Silva, E. P., Melo, M. G., Silva, R. S. D., Oliveira, W. H., et al. (2022). Metformin and fluoxetine improve depressive-like behavior in a murine model of Parkinsońs disease through the modulation of neuroinflammation, neurogenesis and neuroplasticity. Int. Immunopharmacol. 102, 108415. doi:10.1016/j.intimp.2021.108415
Monroe, S. M., and Harkness, K. L. (2022). Major depression and its recurrences: life course matters. Annu. Rev. Clin. Psychol. 18, 329–357. doi:10.1146/annurev-clinpsy-072220-021440
Mousten, I. V., Sørensen, N. V., Christensen, R. H. B., and Benros, M. E. (2022). Cerebrospinal fluid biomarkers in patients with unipolar depression compared with healthy control individuals: a systematic review and meta-analysis. JAMA. Psychiatry. 79 (6), 571–581. doi:10.1001/jamapsychiatry.2022.0645
Muñoz-Arenas, G., Pulido, G., Treviño, S., Vázquez-Roque, R., Flores, G., Moran, C., et al. (2020). Effects of metformin on recognition memory and hippocampal neuroplasticity in rats with metabolic syndrome. Synapse 74 (9), e22153. doi:10.1002/syn.22153
Ni, X. C., Wang, H. F., Cai, Y. Y., Yang, D., Alolga, R. N., Liu, B., et al. (2022). Ginsenoside Rb1 inhibits astrocyte activation and promotes transfer of astrocytic mitochondria to neurons against ischemic stroke. Redox. Biol. 54, 102363. doi:10.1016/j.redox.2022.102363
Noda, Y., Zomorrodi, R., Vila-Rodriguez, F., Downar, J., Farzan, F., Cash, R. F. H., et al. (2018). Impaired neuroplasticity in the prefrontal cortex in depression indexed through paired associative stimulation. Depress. Anxiety. 35 (5), 448–456. doi:10.1002/da.22738
Pace, R., and Martinelli, E. M. (2022). The phytoequivalence of herbal extracts: a critical evaluation. Fitoterapia 162, 105262. doi:10.1016/j.fitote.2022.105262
Pan, Y., Ke, Z., Ye, H., Sun, L., Ding, X., Shen, Y., et al. (2019). Saikosaponin C exerts anti-HBV effects by attenuating HNF1α and HNF4α expression to suppress HBV pgRNA synthesis. Inflamm. Res. 68 (12), 1025–1034. doi:10.1007/s00011-019-01284-2
Peng, M., Xia, T., Zhong, Y., Zhao, M., Yue, Y., Liang, L., et al. (2022). Integrative pharmacology reveals the mechanisms of Erzhi Pill, a traditional Chinese formulation, against diabetic cardiomyopathy. J. Ethnopharmacol. 296, 115474. doi:10.1016/j.jep.2022.115474
Perić, I., Costina, V., Djordjević, S., Gass, P., Findeisen, P., Inta, D., et al. (2021). Tianeptine modulates synaptic vesicle dynamics and favors synaptic mitochondria processes in socially isolated rats. Sci. Rep. 11 (1), 17747. doi:10.1038/s41598-021-97186-7
Player, M. J., Taylor, J. L., Weickert, C. S., Alonzo, A., Sachdev, P., Martin, D., et al. (2013). Neuroplasticity in depressed individuals compared with healthy controls. Neuropsychopharmacology 38 (11), 2101–2108. doi:10.1038/npp.2013.126
Price, R. B., and Duman, R. (2020). Neuroplasticity in cognitive and psychological mechanisms of depression: an integrative model. Mol. Psychiatry. 25 (3), 530–543. doi:10.1038/s41380-019-0615-x
Qian, X., Zhong, Z. D., Zhang, Y., Qiu, L. Q., and Tan, H. J. (2024). Fluoxetine mitigates depressive-like behaviors in mice via anti-inflammation and enhancing neuroplasticity. Brain. Res. 1825, 148723. doi:10.1016/j.brainres.2023.148723
Qin, Z., Shi, D. D., Li, W., Cheng, D., Zhang, Y. D., Zhang, S., et al. (2023). Berberine ameliorates depression-like behaviors in mice via inhibiting NLRP3 inflammasome-mediated neuroinflammation and preventing neuroplasticity disruption. J. Neuroinflammation 20 (1), 54. doi:10.1186/s12974-023-02744-7
Qu, S. Y., Li, X. Y., Heng, X., Qi, Y. Y., Ge, P. Y., Ni, S. J., et al. (2021). Analysis of antidepressant activity of huang-lianie Jie-du decoction through network pharmacology and metabolomics. Front. Pharmacol. 12, 619288. doi:10.3389/fphar.2021.619288
Rauf, A., Olatunde, A., Imran, M., Alhumaydhi, F. A., Aljohani, A. S. M., Khan, S. A., et al. (2021). Honokiol: a review of its pharmacological potential and therapeutic insights. Phytomedicine 90, 153647. doi:10.1016/j.phymed.2021.153647
Rauf, A., Wilairatana, P., Joshi, P. B., Ahmad, Z., Olatunde, A., Hafeez, N., et al. (2024). Revisiting luteolin: an updated review on its anticancer potential. Heliyon 10 (5), e26701. doi:10.1016/j.heliyon.2024.e26701
Ray, A., Gulati, K., and Rai, N. (2017). Stress, anxiety, and immunomodulation: a pharmacological analysis. Vitam. Horm. 103, 1–25. doi:10.1016/bs.vh.2016.09.007
Rehm, J., and Shield, K. D. (2019). Global burden of disease and the impact of mental and addictive disorders. Curr. Psychiatry. Rep. 21 (2), 10. doi:10.1007/s11920-019-0997-0
Ru, Q., Lu, Y., Saifullah, A. B., Blanco, F. A., Yao, C., Cata, J. P., et al. (2022). TIAM1-mediated synaptic plasticity underlies comorbid depression-like and ketamine antidepressant-like actions in chronic pain. J. Clin. Invest. 132 (24), e158545. doi:10.1172/JCI158545
Sato, S., Bunney, B., Mendoza-Viveros, L., Bunney, W., Borrelli, E., Sassone-Corsi, P., et al. (2022). Rapid-acting antidepressants and the circadian clock. Neuropsychopharmacology 47 (4), 805–816. doi:10.1038/s41386-021-01241-w
Shen, B., Feng, H., Cheng, J., Li, Z., Jin, M., Zhao, L., et al. (2020a). Geniposide alleviates non-alcohol fatty liver disease via regulating Nrf2/AMPK/mTOR signalling pathways. J. Cell. Mol. Med. 24 (9), 5097–5108. doi:10.1111/jcmm.15139
Shen, C., Cao, K., Cui, S., Cui, Y., Mo, H., Wen, W., et al. (2020b). SiNiSan ameliorates depression-like behavior in rats by enhancing synaptic plasticity via the CaSR-PKC-ERK signaling pathway. Biomed. Pharmacother. 124, 109787. doi:10.1016/j.biopha.2019.109787
Shi, H. J., Wu, D. L., Chen, R., Li, N., and Zhu, L. J. (2022). Requirement of hippocampal DG nNOS-CAPON dissociation for the anxiolytic and antidepressant effects of fluoxetine. Theranostics 12 (8), 3656–3675. doi:10.7150/thno.70370
Shi, X. Q., Yue, S. J., Tang, Y. P., Chen, Y. Y., Zhou, G. S., Zhang, J., et al. (2019). A network pharmacology approach to investigate the blood enriching mechanism of Danggui buxue Decoction. J. Ethnopharmacol. 235, 227–242. doi:10.1016/j.jep.2019.01.027
Song, D., Hao, J., and Fan, D. (2020). Biological properties and clinical applications of berberine. Front. Med. 14 (5), 564–582. doi:10.1007/s11684-019-0724-6
Spohr, L., de Aguiar, M. S. S., Bona, N. P., Luduvico, K. P., Alves, A. G., Domingues, W. B., et al. (2023). Blueberry extract modulates brain enzymes activities and reduces neuroinflammation: promising effect on lipopolysaccharide-induced depressive-like behavior. Neurochem. Res. 48 (3), 846–861. doi:10.1007/s11064-022-03813-8
Sun, X., Zhang, T., Zhao, Y., Cai, E., Zhu, H., and Liu, S. (2020). Panaxynol attenuates CUMS-induced anxiety and depressive-like behaviors via regulating neurotransmitters, synapses and the HPA axis in mice. Food. Funct. 11 (2), 1235–1244. doi:10.1039/c9fo03104a
Sun, Y., Zhang, H., Wu, Z., Yu, X., Yin, Y., Qian, S., et al. (2021). Quercitrin rapidly alleviated depression-like behaviors in lipopolysaccharide-treated mice: the involvement of PI3K/AKT/NF-κB signaling suppression and CREB/BDNF signaling restoration in the Hippocampus. Acs. Chem. Neurosci. 12 (18), 3387–3396. doi:10.1021/acschemneuro.1c00371
Tanaka, J., Horiike, Y., Matsuzaki, M., Miyazaki, T., Ellis-Davies, G. C., and Kasai, H. (2008). Protein synthesis and neurotrophin-dependent structural plasticity of single dendritic spines. Science 319 (5870), 1683–1687. doi:10.1126/science.1152864
Tanner-Smith, E. E., and Grant, S. (2018). Meta-analysis of complex interventions. Annu. Rev. Public. Health. 39, 135–151. doi:10.1146/annurev-publhealth-040617-014112
Tao, W., Ruan, J., Wu, R., Zhao, M., Zhao, T., Qi, M., et al. (2023a). A natural carotenoid crocin exerts antidepressant action by promoting adult hippocampal neurogenesis through Wnt/β-catenin signaling. J. Adv. Res. 43, 219–231. doi:10.1016/j.jare.2022.02.015
Tao, W., Yao, G., Yue, Q., Xu, C., Hu, Y., Cheng, X., et al. (2023b). 14-3-3ζ Plays a key role in the modulation of neuroplasticity underlying the antidepressant-like effects of Zhi-Zi-Chi-Tang. Phytomedicine 116, 154888. doi:10.1016/j.phymed.2023.154888
Tartt, A. N., Mariani, M. B., Hen, R., Mann, J. J., and Boldrini, M. (2022). Dysregulation of adult hippocampal neuroplasticity in major depression: pathogenesis and therapeutic implications. Mol. Psychiatry. 27 (6), 2689–2699. doi:10.1038/s41380-022-01520-y
Tejeda, G. S., and Díaz-Guerra, M. (2017). Integral characterization of defective BDNF/TrkB signalling in neurological and psychiatric disorders leads the way to new therapies. Int. J. Mol. Sci. 18 (2), 268. doi:10.3390/ijms18020268
Vega-Rivera, N. M., González-Trujano, M. E., Luna-Angula, A., Sánchez-Chapul, L., and Estrada-Camarena, E. (2023). Antidepressant-like effects of the Punica granatum and citalopram combination are associated with structural changes in dendritic spines of granule cells in the dentate gyrus of rats. Front. Pharmacol. 14, 1211663. doi:10.3389/fphar.2023.1211663
Vints, W. A. J., Levin, O., Fujiyama, H., Verbunt, J., and Masiulis, N. (2022). Exerkines and long-term synaptic potentiation: mechanisms of exercise-induced neuroplasticity. Front. Neuroendocrinol. 66, 100993. doi:10.1016/j.yfrne.2022.100993
Wang, G., An, T., Lei, C., Zhu, X., Yang, L., Zhang, L., et al. (2022b). Antidepressant-like effect of ginsenoside Rb1 on potentiating synaptic plasticity via the miR-134-mediated BDNF signaling pathway in a mouse model of chronic stress-induced depression. J. Ginseng. Res. 46 (3), 376–386. doi:10.1016/j.jgr.2021.03.005
Wang, L., Wang, Z., Lan, Y., Tuo, Y., Ma, S., and Liu, X. (2023b). Inulin attenuates blood-brain barrier permeability and alleviates behavioral disorders by modulating the TLR4/MyD88/NF-κB pathway in mice with chronic stress. J. Agric. Food. Chem. 71 (36), 13325–13337. doi:10.1021/acs.jafc.3c03568
Wang, Q., Yang, B., Wang, N., and Gu, J. (2022a). Tumor immunomodulatory effects of polyphenols. Front. Immunol. 13, 1041138. doi:10.3389/fimmu.2022.1041138
Wang, W., Wang, L., Wang, L., Li, Y., Lan, T., Wang, C., et al. (2023a). Ginsenoside-Rg1 synergized with voluntary running exercise protects against glial activation and dysregulation of neuronal plasticity in depression. Food. Funct. 14 (15), 7222–7239. doi:10.1039/d3fo00496a
Wang, W. K., Zhou, Y., Fan, L., Sun, Y., Ge, F., and Xue, M. (2021). The antidepressant-like effects of Danggui Buxue Decoction in GK rats by activating CREB/BDNF/TrkB signaling pathway. Phytomedicine 89, 153600. doi:10.1016/j.phymed.2021.153600
Wang, Y. S., Shen, C. Y., and Jiang, J. G. (2019). Antidepressant active ingredients from herbs and nutraceuticals used in TCM: pharmacological mechanisms and prospects for drug discovery. Pharmacol. Res. 150, 104520. doi:10.1016/j.phrs.2019.104520
Wei, Y., Chang, L., and Hashimoto, K. (2022). Molecular mechanisms underlying the antidepressant actions of arketamine: beyond the NMDA receptor. Mol. Psychiatry. 27 (1), 559–573. doi:10.1038/s41380-021-01121-1
Wu, D., Wang, H., Teng, T., Duan, S., Ji, A., and Li, Y. (2018). Hydrogen sulfide and autophagy: a double edged sword. Pharmacol. Res. 131, 120–127. doi:10.1016/j.phrs.2018.03.002
Wu, R., Xiao, D., Shan, X., Dong, Y., and Tao, W. W. (2020). Rapid and prolonged antidepressant-like effect of crocin is associated with GHSR-mediated hippocampal plasticity-related proteins in mice exposed to prenatal stress. Acs. Chem. Neurosci. 11 (8), 1159–1170. doi:10.1021/acschemneuro.0c00022
Wu, Y., Wei, Z., Li, Y., Wei, C., Li, Y., Cheng, P., et al. (2019). Perturbation of Ephrin receptor signaling and glutamatergic transmission in the hypothalamus in depression using proteomics integrated with metabolomics. Front. Neurosci. 13, 1359. doi:10.3389/fnins.2019.01359
Xia, B., Huang, X., Sun, G., and Tao, W. (2021). Iridoids from Gardeniae fructus ameliorates depression by enhancing synaptic plasticity via AMPA receptor-mTOR signaling. J. Ethnopharmacol. 268, 113665. doi:10.1016/j.jep.2020.113665
Xia, C. Y., Guo, Y. X., Lian, W. W., Yan, Y., Ma, B. Z., Cheng, Y. C., et al. (2023). The NLRP3 inflammasome in depression: potential mechanisms and therapies. Pharmacol. Res. 187, 106625. doi:10.1016/j.phrs.2022.106625
Xie, P., Chen, L., Wang, J., Wang, X., Yang, S., and Zhu, G. (2024). Polysaccharides from Polygonatum cyrtonema Hua prevent post-traumatic stress disorder behaviors in mice: mechanisms from the perspective of synaptic injury, oxidative stress, and neuroinflammation. J. Ethnopharmacol. 319 (Pt 1), 117165. doi:10.1016/j.jep.2023.117165
Xing, Y., and Bai, Y. (2020). A review of exercise-induced neuroplasticity in ischemic stroke: pathology and mechanisms. Mol. Neurobiol. 57 (10), 4218–4231. doi:10.1007/s12035-020-02021-1
Xu, Y., Zhang, J., Yu, L., Zhang, W., Zhang, Y., Shi, Y., et al. (2023). Engeletin alleviates depression-like phenotype by increasing synaptic plasticity via the BDNF-TrkB-mTORC1 signalling pathway. J. Cell. Mol. Med. 27 (23), 3928–3938. doi:10.1111/jcmm.17975
Xue, H., Li, P., Luo, Y., Wu, C., Liu, Y., Qin, X., et al. (2019). Salidroside stimulates the Sirt1/PGC-1α axis and ameliorates diabetic nephropathy in mice. Phytomedicine 54, 240–247. doi:10.1016/j.phymed.2018.10.031
Yan, L., Ai, Y., Xing, Y., Wang, B., Gao, A., Xu, Q., et al. (2023a). Citalopram in the treatment of elderly chronic heart failure combined with depression: a systematic review and meta-analysis. Front. Cardiovasc. Med. 10, 1107672. doi:10.3389/fcvm.2023.1107672
Yan, W. J., Tan, Y. C., Xu, J. C., Tang, X. P., Zhang, C., Zhang, P. B., et al. (2015). Protective effects of silibinin and its possible mechanism of action in mice exposed to chronic unpredictable mild stress. Biomol. Ther. Seoul. 23 (3), 245–250. doi:10.4062/biomolther.2014.138
Yan, Y., Li, J., Zhang, Y., Wang, H., Qin, X., Zhai, K., et al. (2023b). Screening the effective components of Suanzaoren decoction on the treatment of chronic restraint stress induced anxiety-like mice by integrated chinmedomics and network pharmacology. Phytomedicine 115, 154853. doi:10.1016/j.phymed.2023.154853
Yan, Y., Xu, X., Chen, R., Wu, S., Yang, Z., Wang, H., et al. (2021). Down-regulation of MST1 in hippocampus protects against stress-induced depression-like behaviours and synaptic plasticity impairments. Brain. Behav. Immun. 94, 196–209. doi:10.1016/j.bbi.2021.02.007
Yang, L., Huang, Y., Chen, F., Wang, Y., Su, K., Zhao, M., et al. (2023a). Berberine attenuates depression-like behavior by modulating the hippocampal NLRP3 ubiquitination signaling pathway through Trim65. Int. Immunopharmacol. 123, 110808. doi:10.1016/j.intimp.2023.110808
Yang, S. J., Wang, J. J., Cheng, P., Chen, L. X., Hu, J. M., and Zhu, G. Q. (2023b). Ginsenoside Rg1 in neurological diseases: from bench to bedside. Acta. Pharmacol. Sin. 44 (5), 913–930. doi:10.1038/s41401-022-01022-1
Yao, X., Qin, X., Wang, H., Zheng, J., Peng, Z., Wang, J., et al. (2023). Lack of bombesin receptor-activated protein homologous protein impairs hippocampal synaptic plasticity and promotes chronic unpredictable mild stress induced behavioral changes in mice. Stress 26 (1), 1–14. doi:10.1080/10253890.2022.2155513
Ye, Z., Wang, J., Fang, F., Wang, Y., Liu, Z., Shen, C., et al. (2024). Zhi-Zi-Hou-Po decoction alleviates depressive-like behavior and promotes hippocampal neurogenesis in chronic unpredictable mild stress induced mice via activating the BDNF/TrkB/CREB pathway. J. Ethnopharmacol. 319 (Pt 3), 117355. doi:10.1016/j.jep.2023.117355
Yi, Y., Xu, W., Wang, H. X., Huang, F., and Wang, L. M. (2020). Natural polysaccharides experience physiochemical and functional changes during preparation: a review. Carbohydr. Polym. 234, 115896. doi:10.1016/j.carbpol.2020.115896
Yin, R., Zhang, K., Li, Y., Tang, Z., Zheng, R., Ma, Y., et al. (2023). Lipopolysaccharide-induced depression-like model in mice: meta-analysis and systematic evaluation. Front. Immunol. 14, 1181973. doi:10.3389/fimmu.2023.1181973
Yu, C., Li, A., Li, X., Chen, Z., Wang, P., Dong, Z., et al. (2020). Impaired LTD-like motor cortical plasticity in female patients with major depression disorder. Neuropharmacology 179, 108268. doi:10.1016/j.neuropharm.2020.108268
Yu, D., Cheng, Z., Ali, A. I., Wang, J., Le, K., Chibaatar, E., et al. (2019). Chronic unexpected mild stress destroys synaptic plasticity of neurons through a glutamate transporter, GLT-1, of astrocytes in the ischemic stroke rat. Neural. Plast. 2019, 1615925. doi:10.1155/2019/1615925
Yu, H., Yang, R., Wu, J., Wang, S., Qin, X., Wu, T., et al. (2022). Association of metformin and depression in patients with type 2 diabetes. J. Affect. Disord. 318, 380–385. doi:10.1016/j.jad.2022.09.015
Yu, S., Zhao, Y., Luo, Q., Gu, B., Wang, X., Cheng, J., et al. (2024). Early life stress enhances the susceptibility to depression and interferes with neuroplasticity in the hippocampus of adolescent mice via regulating miR-34c-5p/SYT1 axis. J. Psychiatr. Res. 170, 262–276. doi:10.1016/j.jpsychires.2023.12.030
Zavvari, F., Nahavandi, A., and Goudarzi, M. (2020). Fluoxetine attenuates stress-induced depressive-like behavior through modulation of hippocampal GAP43 and neurogenesis in male rats. Jj. Chem. Neuroanat. 103, 101711. doi:10.1016/j.jchemneu.2019.101711
Zhang, H., Sun, Y., Huang, Z., Wu, Z., Ying, Y., Liu, R., et al. (2024a). Jiawei-Xiaoyao pill elicits a rapid antidepressant effect, dependent on activating CaMKII/mTOR/BDNF signaling pathway in the hippocampus. J. Ethnopharmacol. 318 (Pt B), 117016. doi:10.1016/j.jep.2023.117016
Zhang, H., Zhao, Y., and Wang, Z. (2015). Chronic corticosterone exposure reduces hippocampal astrocyte structural plasticity and induces hippocampal atrophy in mice. Neurosci. Lett. 592, 76–81. doi:10.1016/j.neulet.2015.03.006
Zhang, L., Luo, J., Zhang, M., Yao, W., Ma, X., and Yu, S. Y. (2014). Effects of curcumin on chronic, unpredictable, mild, stress-induced depressive-like behaviour and structural plasticity in the lateral amygdala of rats. Int. J. Neuropsychopharmacol. 17 (5), 793–806. doi:10.1017/S1461145713001661
Zhang, L., and Wei, W. (2020). Anti-inflammatory and immunoregulatory effects of paeoniflorin and total glucosides of paeony. Pharmacol. Ther. 207, 107452. doi:10.1016/j.pharmthera.2019.107452
Zhang, M., Lyu, D., Wang, F., Shi, S., Wang, M., Yang, W., et al. (2022b). Ketamine may exert rapid antidepressant effects through modulation of neuroplasticity, autophagy, and ferroptosis in the habenular nucleus. Neuroscience 506, 29–37. doi:10.1016/j.neuroscience.2022.10.015
Zhang, M. M., Huo, G. M., Cheng, J., Zhang, Q. P., Li, N. Z., Guo, M. X., et al. (2022a). Gypenoside XVII, an active ingredient from gynostemma pentaphyllum, inhibits C3aR-associated synaptic pruning in stressed mice. Nutrients 14 (12), 2418. doi:10.3390/nu14122418
Zhang, R., Zeng, M., Zhang, X., Zheng, Y., Lv, N., Wang, L., et al. (2023). Therapeutic candidates for alzheimer's disease: saponins. Int. J. Mol. Sci. 24 (13), 10505. doi:10.3390/ijms241310505
Zhang, X., Guo, J., Zhang, C., Wang, W., Du, S., and Tian, X. (2024b). Suanzaoren decoction exerts its antidepressant effect via the CaMK signaling pathway. Transl. Neurosci. 15 (1), 20220341. doi:10.1515/tnsci-2022-0341
Zhao, X., Kong, D., Zhou, Q., Wei, G., Song, J., Liang, Y., et al. (2021). Baicalein alleviates depression-like behavior in rotenone-induced Parkinson's disease model in mice through activating the BDNF/TrkB/CREB pathway. Biomed. Pharmacother. 140, 111556. doi:10.1016/j.biopha.2021.111556
Zheng, Z. H., Tu, J. L., Li, X. H., Hua, Q., Liu, W. Z., Liu, Y., et al. (2021). Neuroinflammation induces anxiety- and depressive-like behavior by modulating neuronal plasticity in the basolateral amygdala. Brain. Behav. Immun. 91, 505–518. doi:10.1016/j.bbi.2020.11.007
Zhou, C., Peng, B., Qin, Z., Zhu, W., and Guo, C. (2021). Metformin attenuates LPS-induced neuronal injury and cognitive impairments by blocking NF-κB pathway. Bmc. Neurosci. 22 (1), 73. doi:10.1186/s12868-021-00678-5
Zhu, S. M., Luo, F. Y., Peng, J., Luo, L. Y., Xue, R., Yang, Y., et al. (2024). The physicochemical characteristics and antidepressant-like effects of a polysaccharide-rich fraction from Schisandra chinensis (Turcz.) Baill in behavioral despair mice and olfactory bulbectomy-induced depression-like mice. J. Ethnopharmacol. 320, 117464. doi:10.1016/j.jep.2023.117464
Zhu, W., Zhang, W., Yang, F., Cai, M., Li, X., Xiang, Y., et al. (2023). Role of PGC-1α mediated synaptic plasticity, mitochondrial function, and neuroinflammation in the antidepressant effect of Zi-Shui-Qing-Gan-Yin. Front. Neurol. 14, 1108494. doi:10.3389/fneur.2023.1108494
Zhu, X., Sun, Y., Zhang, C., and Liu, H. (2017). Effects of berberine on a rat model of chronic stress and depression via gastrointestinal tract pathology and gastrointestinal flora profile assays. Mol. Med. Rep. 15 (5), 3161–3171. doi:10.3892/mmr.2017.6353
Zhuang, W., Liu, S. L., Xi, S. Y., Feng, Y. N., Wang, K., Abduwali, T., et al. (2023). Traditional Chinese medicine decoctions and Chinese patent medicines for the treatment of depression: efficacies and mechanisms. J. Ethnopharmacol. 307, 116272. doi:10.1016/j.jep.2023.116272
Zia, A., Farkhondeh, T., Pourbagher-Shahri, A. M., and Samarghandian, S. (2021). The role of curcumin in aging and senescence: molecular mechanisms. Biomed. Pharmacother. 134, 111119. doi:10.1016/j.biopha.2020.111119
Ziegler, J., and Facchini, P. J. (2008). Alkaloid biosynthesis: metabolism and trafficking. Annu. Rev. Plant. Biol. 59, 735–769. doi:10.1146/annurev.arplant.59.032607.092730
Keywords: depression, major depressive disorder, antidepressant, traditional Chinese medicine, neuroplasticity
Citation: Lv S, Yang N, Lu Y, Zhang G, Zhong X, Cui Y, Huang Y, Teng J and Sai Y (2024) The therapeutic potential of traditional Chinese medicine in depression: focused on the modulation of neuroplasticity. Front. Pharmacol. 15:1426769. doi: 10.3389/fphar.2024.1426769
Received: 03 May 2024; Accepted: 06 August 2024;
Published: 26 August 2024.
Edited by:
Qi Liang, Air Force Medical University, ChinaCopyright © 2024 Lv, Yang, Lu, Zhang, Zhong, Cui, Huang, Teng and Sai. This is an open-access article distributed under the terms of the Creative Commons Attribution License (CC BY). The use, distribution or reproduction in other forums is permitted, provided the original author(s) and the copyright owner(s) are credited and that the original publication in this journal is cited, in accordance with accepted academic practice. No use, distribution or reproduction is permitted which does not comply with these terms.
*Correspondence: Yanyan Sai, NTAwNDE0NTBAcXEuY29t
Disclaimer: All claims expressed in this article are solely those of the authors and do not necessarily represent those of their affiliated organizations, or those of the publisher, the editors and the reviewers. Any product that may be evaluated in this article or claim that may be made by its manufacturer is not guaranteed or endorsed by the publisher.
Research integrity at Frontiers
Learn more about the work of our research integrity team to safeguard the quality of each article we publish.