- 1Division of Life Science and Center for Chinese Medicine, The Hong Kong University of Science and Technology, Hong Kong SAR, China
- 2Shenzhen Key Laboratory of Edible and Medicinal Bioresources, HKUST Shenzhen Research Institute, Shenzhen, China
- 3Department of Physics, The Hong Kong University of Science and Technology, Hong Kong SAR, China
- 4Kunming Institute of Botany, Chinese Academy of Sciences, Kunming, Yunnan, China
Background: The development of effective inhibitors that can inhibit amyloid β (Aβ) peptides aggregation and promote neurite outgrowth is crucial for the possible treatment of Alzheimer’s disease (AD). Lobaria (Schreb.) Hoffm., a traditional Chinese medicine used in Himalaya region for inflammatory diseases, contains depsides/depsidones (DEPs) such as gyrophoric acid, norstictic acid, and stictic acid known for their anti-cancer and anti-inflammation properties.
Methods: Lobaria extracts were analyzed using HPLC to identify DEPs and establish standards. The inhibitory effects of Lobaria on Aβ42 fibrillization and depolymerization were assessed using various approaches with biophysical and cellular methods. The neuroprotective activity of Lobaria extracts and its DEPs aganist Aβ-mediated cytotoxicity was also evaluated.
Results: Norstictic and stictic acid were found in the water extract, while norstictic, stictic, and gyrophoric acid were detected in the ethanol extract of Lobaria. Both extracts, and their DEPs effectively inhibited Aβ42 fibrillation and disaggregate mature Aβ42 fibrils. Notably, the ethanol extract showed superior inhibitory effect compared to the water extract, with gyrophoric acid being the most effective DEPs. Additionally, herbal extract-treated Aβ42 aggregation species significantly protected neuronal cells from Aβ42-induced cell damage and promoted neurite outgrowth.
Conclusion: This study is the first to investigate the effect of Lobaria on Aβ42 and neuronal cell in AD. Given that Lobaria is commonly used in ethnic medicine and food with good safety records, our findings propose that Lobaria extracts and DEPs have potential as neuroprotective and therapeutic agents for AD patients.
1 Introduction
Alzheimer’s disease (AD) is the most prevalent form of dementia, which is a complex, multifactorial, progressive neurodegenerative disease primarily affecting the elderly population (Eratne et al., 2018). The complicated pathophysiology of AD is commonly attributed to excessive production and subsequent aggregation of amyloid β (Aβ) oligomers, which is a major cause of a series of neuronal death and tissue damage (Adlard et al., 2014). The peptide Aβ containing 39–43 amino acids is produced from sequential hydrolyzation of a transmembrane protein, the Aβ precursor, by both β- and γ-secretases (Hardy and Selkoe, 2002). Because of different cleavage sites of secretases, Aβ has multiple variants that have already been found in the brains of AD patients. The Aβ monomers can aggregate into insoluble fibrils having a highly ordered β-sheet structure. The Aβ fibril is strongly neurotoxic in human brain (Jia et al., 2018; Linse, 2019; Jia et al., 2020), and which is thus believed to be one of the main driving factors for the occurrence and development of AD (Hardy and Selkoe, 2002). Thus, the inhibition of Aβ fibrillation and disaggregation of mature Aβ fibrils are possible methods to combat AD.
In addition to Aβ fibrils-induced neuronal damage, the natural aging of neurons, characterized by decreased neuronal activities, e.g., neurite outgrowth and neuronal differentiation, presents further challenges in AD treatment. An efficient AD treatment should address not only Aβ fibrillation but also the impaired, or aged, neurons to revoke their full functionality in development and regeneration. Neurotrophic factors, such as nerve growth factor (NGF), play crucial roles in the neuronal activity by promoting processes, like neurite outgrowth (Calabrese, 2008). However, NGF is typically at low level in the brains of AD patients, and due to the blood–brain barrier, direct supplementation of NGF to human brains is not feasible (Bartus, 2000; Budni et al., 2015). Therefore, an effective drug for AD should consist of small molecules that have entry to the brain and inhibit Aβ fibrillation, disassemble mature Aβ fibrils, and promote neurite outgrowth (Chen et al., 2017; Jia et al., 2018; Linse, 2019; Jia et al., 2020). Blockage of Aβ aggregation is one of the several therapeutic strategies for AD treatment, as used in the well-known phytochemical, curcumin, and newly developed drugs aducanumab and lecanemab, in reducing Aβ plaques (Huang et al., 2023).
Natural products are valued for their bioactive compounds with minimal side effects (Pagano et al., 2020). Developing drug that can inhibit Aβ aggregation and break down mature Aβ fibrils is crucial for AD treatment. Lobaria, a genus of lichen, is widely used as food and/or ethnomedicine medicine to treat pneumonia in the Himalayan regions, and importantly, this herb maintains a good safety record (Yang et al., 2021). It is also a rich source of calcium, dietary fiber, and protein with a protein content as high as that in Tremella (Cui and Duan, 2000). Its metabolites, depsides and depsidones (DEPs), have antibacterial, anti-inflammatory, and cytotoxic properties (Hidalgo et al., 1994; Urena-Vacas et al., 2021). Some DEPs being found in Lobaria, e.g., gyrophoric acid, norstictic acid, and stictic acid, have shown effectiveness in treating cancer and inflammation (Ebrahim et al., 2016; Mohammadi et al., 2022; Yang et al., 2023). For the first time, we utilized biochemical and physical techniques to explore Lobaria’s inhibitory effects on Aβ42 fibrillization and depolymerization of mature fibrils. The neuroprotective potential of Lobaria extracts and DEPs against Aβ42-induced cytotoxicity are also investigated, suggesting Lobaria’s potential in AD treatment.
2 Materials and methods
2.1 Raw material preparation
The dried green-algal Lobaria were purchased from Yunnan market (Yunnan, China). The three DEPs, stictic acid (SA), norstictic acid (NA) and gyrophoric acid (GA), all with a purity >95%, were obtained from Cayman Chemical (Ann Arbor, MI). All chemicals and ethanol extracts were dissolved in dimethyl sulfoxide (DMSO) as the stock solutions, and controlled DMSO at 0.1% final concentration was employed during the experiments (Moskot et al., 2019). Synthetic Aβ42 was obtained from GL Biochem (Shanghai, China). Thioflavin T fluorescence (ThT) and 3-(4,5-dimethylthiazol-2-yl)-2,5-diphenyltetrazolium bromide (MTT) were acquired from Sigma-Aldrich (St. Louis, MO). Ultrapure water was prepared from a Milli-Q purification system (Millipore, Molsheim, France). All culture reagents were from Thermo Fisher Scientific (Waltham, MA). All chemicals used were of analytical grade (AR) or HPLC grade.
2.2 Herbal extract preparation
Lobaria powder (10.0 g) was dissolved in 100 mL of 90% ethanol (LEtOH) or distilled water (Lwater) in a 250-mL round-bottomed flask. The solutions were refluxed for 1 h and then filtered through a paper filter (Advantec, Tokyo, Japan). Then, extract was evaporated to dryness using a rotary evaporator.
2.3 HPLC analysis of the Lobaria extracts
The Lobaria extracts (10 mg), LEtOH, and Lwater, were dissolved in ethanol and analyzed by HPLC-DAD with an ACE Excel 5 Super C18 column (4.6 × 250 mm, 5 μm) (Agilent, Santa Clara, CA). The mobile phase was acetonitrile A) with 0.1% phosphoric acid B) using a gradient program of approximately 20%–80% at 0–70 min. The injection volume was 10 μL. The column temperature was at 25°C, and the absorbance was measured at 264 nm.
2.4 Preparation of Aβ fibrils
The synthetic Aβ42 powder was dissolved in 100% hexafluoro-isopropanol at a concentration of 1 mM to disaggregate any Aβ pre-aggregates. After sonication for 20 min at 25°C, the Aβ42 monomer solution was aliquoted and dried in a fume hood overnight for complete removal of hexafluoro-isopropanol and then stored at −20°C. To obtain Aβ42 fibrils, the dried peptide film was first resuspended in 20 μL DMSO and then dissolved in 10 mM HCl aqueous solution to obtain a monomer solution of 100 μM. The peptide film was first resuspended in 20 μL DMSO and then dissolved in 10 mM HCl aqueous solution to obtain a monomer solution of 100 μM. The aggregation kinetics of the Aβ42 monomer (100 μM) incubated at 37°C was monitored using dynamic light scattering. The Aβ42 fibril formation inclined steadily until day 3 and plateaued on day 6 of incubation (Supplementary Figure S1).
2.5 ThT fluorescence assay
To determine the amount of Aβ42 fibrils, the fluorescence intensity of ThT dye was measured after adding it to the protein solution, following the method by Biancalana and Koide (2010). In the inhibitory effect test, Aβ42 monomer (10 μM) was allowed to self-aggregation or co-aggregation with various concentrations of Lobaria extracts and DEPs, then incubated at 37°C for 6 days. For the disassembling effect, Aβ fibrils (10 μM) aged for 6 days were incubated with or without extracts and DEPs at 37°C for 5 days. ThT fluorescent dye was added at a final concentration of 20 μM, and the ThT fluorescence was measured in a 96-well black plate at excitation and emission wavelengths of 435 and 488 nm, respectively.
2.6 AFM measurement of the aggregate morphology
To prepare the sample for atomic force microscopy (AFM) scanning, 10 μL of each protein solution (10 μM) was deposited on a freshly cleaved mica sheet and left still for 3 min. Then, the mica surface was rinsed with about 200 μL deionized water to remove redundant protein solution and let dry for about 2 h. The dried samples were then scanned using tapping mode AFM (MFP-3D, Asylum Research, Santa Barbara, CA).
2.7 DLS measurement of the aggregate size
Dynamic light scattering (DLS) is a standard technique for determining the particle size in a solution, including protein aggregates. While DLS accurately measures the radius of spherical particles, it provides a more complex size measurement for non-spherical particles, like the fibrillar aggregate of Aβ42, influenced by the particle’s three-dimensional geometry. The DLS experiment utilized a 30-mW helium-neon laser (wavelength λ = 633 nm), a photomultiplier tube (Hamamatsu HC120-08), and a correlator (Correlator Flex02-12D/C), with measurements taken at a 90° scattering angle and at room temperature. The sample, consisting of 1 mL of protein solution with less than 10 μL of tested extracts or DEPs, was placed in a square quartz cuvette (length/width 1 mm). Prior to measure the auto-correlation function of the scattered light, the protein solution was gently shaken by hand and allowed to settle for about 1 min to ensure uniform dispersion of aggregates in the solution. Each measurement lasted for 30 s and was repeated 4–5 times to determine the uncertainty in the measured size.
The measured autocorrelation function of the scattered light was fitted to the second-order cumulant,
where the decay rate Γ = Dq2 with q = 4πn sin (θ/2)/λ and D = kBT/6πηR, the scattering angle θ = π/2, the wavelength of laser λ = 633 nm, the thermal energy kBT = 4.07 × 10–21 J (T = 22°C), the refraction index n = 1.33 and the viscosity η = 1.0 × 10−3 Pas. By fitting the measured correlation function to the above equation, one could obtain the aggregate size R.
2.8 Neurite outgrowth assay
Cell differentiation was assessed based on neurite length, with a differentiated cell having a neurite longer than the diameter of the cell body. PC12 cells were seeded at low cell density in a 6-well plate (1 × 104 cells/well) and cultured for 24 h. Following 24 h of serum starvation, the cells were treated with Lobaria extracts and DEPs, with or without a low concentration of NGF at 1.5 ng/mL, for 48 h. A positive control using NGF at 50 ng/mL was included. The morphology of PC12 cells was examined under a phase-contrast microscope (Caarl Zeiss, Oberkochen, Germany, ×10 objective), and images were captured using a digital camera (SPOT basic software, Diagnostic Instruments, MI). The neurite length of approximately 100 cells from five randomly selected visual fields was quantified using the ImageJ software.
2.9 Cytotoxicity assay
Rat pheochromocytoma PC12 cells from rat adrenal medulla was procured from American Type Culture Collection (ATCC 1721, Manassas, VA). These cells were cultured in Dulbecco’s modified eagle medium (DMEM), supplemented with 6% fetal bovine serum (FBS), 6% horse serum, and 1% penicillin/streptomycin (10,000 U/mL and 10,000 μg/mL). The culture was maintained in a humidified incubator with 5% CO2 at 37°C and sub-cultured until passage 20. Cell viability was evaluated using the MTT assay. PC12 cells were seeded in a 96-well plate at a density of 1 × 104 cells/well and incubated for 24 h. Following a 24 h serum starvation period (1% Fetal Bovine Serum (FBS) and 1% horse serum), the cells were co-cultured with varying concentrations of Lobaria extracts and DEPs. To investigate the preventive effect of Lobaria extracts and DEPs on Aβ42 fibril-induced cell death, cells were exposed to Aβ42 aggregates (10 μM) that had been cultured for 6 days with or without Lobaria extracts and DEPs for 24 h in culture. For evaluating the protective role of Lobaria extracts and DEPs against Aβ42 fibril-induced cell toxicity, PC12 cells were pre-treated with the extracts for 24 h before being exposed to Aβ42 fibrils at 10 μM for an additional 24 h. Subsequently, the cells were treated with MTT solution (0.5 mg/mL) for another 2 h. The purple formazan produced in viable cells was dissolved in DMSO, and the absorbance was measured at 570 nm.
2.10 Statistical analysis
Statistical analyses were performed by using data were represented as mean ± SEM. The significance of difference was determined by one-way analysis of variance (ANOVA). The p < 0.05 was considered statistically significant.
3 Results
3.1 Extracts of Lobaria inhibit fibrillogenesis
The obtained Lobaria extracts using water and 90% ethanol were labeled as Lwater and LEtOH, respectively. The extraction yields were 15.3% and for LEtOH and 14.5% for Lwater. Overlay chromatograms of the 12 samples at wavelength 264 nm were obtained (Figures 1A, B). A representative fingerprint of Lobaria, with peaks ranging from 1-11 of LEtOH and 1-9 of Lwater, was utilized as common characteristic peaks for quantitative control. In conclusion, our chemical analysis confirmed the presence of only norstictic and stictic acid in Lwater, while norstictic acid, stictic acid, and gyrophoric acid were found in LEtOH (Figure 1). Consistent with our previous study (Yang et al., 2023), we observed significantly higher levels of DEPs in ethanol extract compared to water extract.
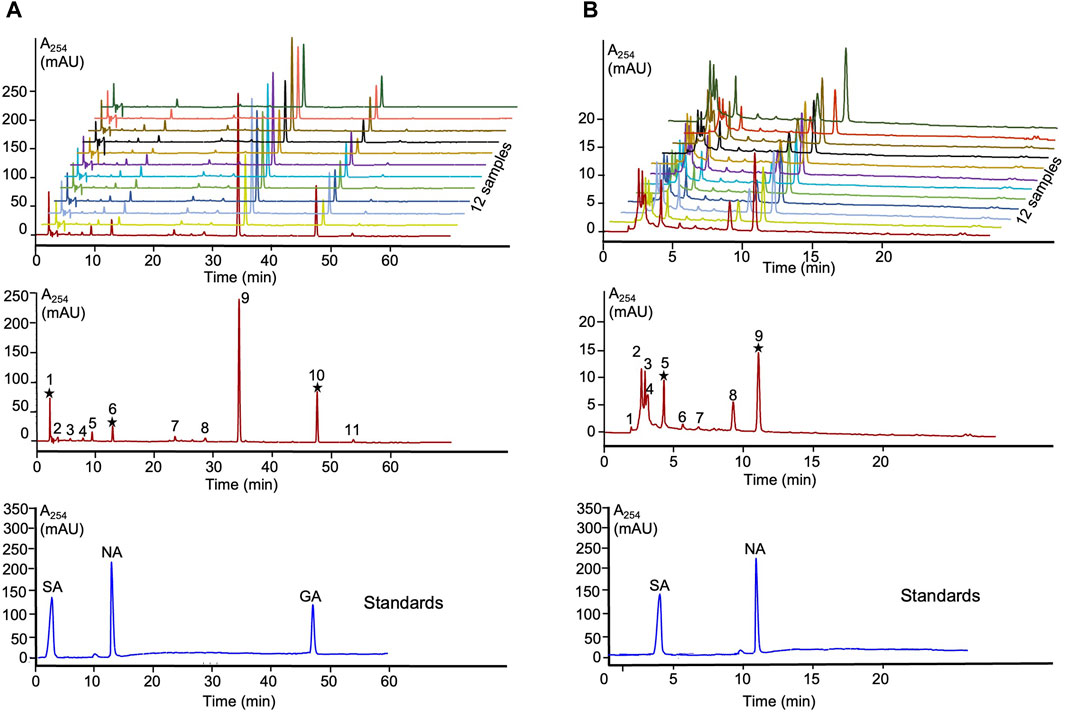
Figure 1. HPLC fingerprints and authentic standards of Lobaria extracts. (A) SA: stictic acid (1), NA: norstictic acid (6) and GA: gyrophoric acid (10) in LEtOH; (B) SA: stictic acid (5) and NA: norstictic acid (9) in Lwater.
To investigate the inhibitory effect of Lobaria extract (Lwater and LEtOH) against Aβ42 fibrillation, the ThT assay was first applied. The results, shown in Figure 2, indicated that both water and ethanol extracts of Lobaria inhibited Aβ42 fibrillation in dose-dependent manner. The half-maximal inhibitory concentration (IC50) for LEtOH was 19.61 ± 1.78 μg/mL (Figure 2A) and for Lwater was 32.53 ± 7.43 μg/mL (Figure 2B). When observing the Aβ42 aggregates using AFM (Figure 2C), it was found that in the presence of 200 μg/mL of either Lwater or LEtOH, only short fibrils or spheroidal species were seen, instead of long fibrils that typically formed. Lwater showed more short fibrils or spheroidal species compared to LEtOH, indicating that LEtOH was more effective in inhibiting Aβ42 aggregation. These results show that Lobaria extracts can prevent the formation of long fibrillar structures during Aβ42 aggregation.
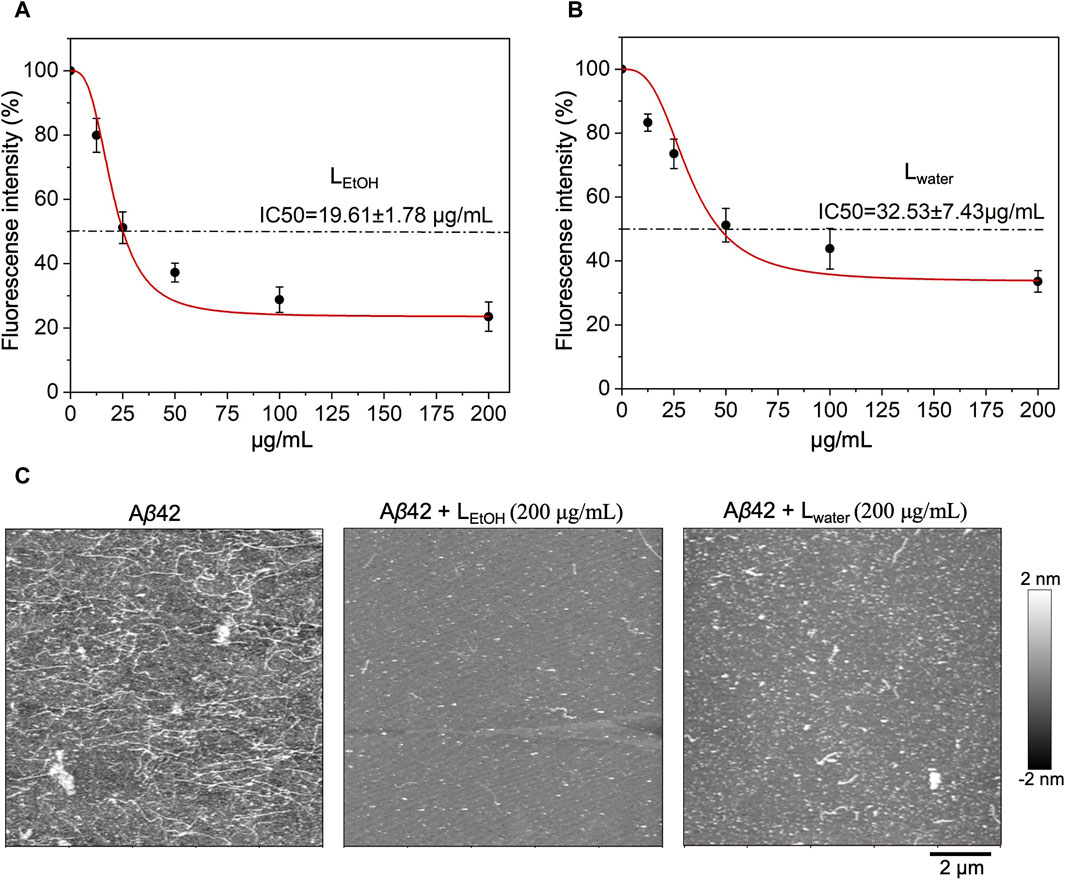
Figure 2. Inhibitory effect of Lobaria extract (Lwater and LEtOH) on Aβ42 fibrillation. The half-maximal inhibitory concentration (IC50) of (A) Lobaria ethanol extract (LEtOH) and (B) water extract (Lwater) as characterized by ThT fluorescence assays. (C) AFM images of Aβ42 species with or without Lwater (200 μg/mL) and LEtOH (200 μg/mL). The representative data of a minimum of four independent experiments are shown in mean ± SEM fold change.
3.2 Lobaria extracts and DEPs reduce the Aβ42-induced cytotoxicity
The neurotoxicity of Aβ42 aggregates plays a crucial role in the development of AD (Kowall et al., 1991). To examine the protective activity of Lobaria extracts (Lwater and LEtOH) on Aβ42-induced cytotoxicity of cultured PC12 cells, MTT assay was performed. Initially, the Lobaria extracts and three DEPs (NA: norstictic acid; SA: stictic acid; GA: gyrophoric acid) on PC12 cells was examined. At low concentration of extracts (12.5 μg/mL) and DEPs, i.e., SA (30 μM), NA (30 μM) and GA (20 μM), no adverse effects on cell survival were observed (Supplementary Figure S2). As the concentration increased, Lwater seemed to enhance cell survival, while LEtOH showed slight toxicity. The cell survival rates in different concentrations of Lwater (25, 50, 100, 200 μg/mL) were 89.5%, 89.5%, 99.7%, and 101.5%, respectively. Meanwhile, the cell survival rates in different concentrations of LEtOH (25, 50, 100, 200 μg/mL) were 97.8%, 91.5%, 91.8%, 85.7%, 80.7%.
We then examined whether the Lobaria extracts and DEPs could improve cell survival when exposed to cytotoxic Aβ42 fibrils. As shown in Figure 3A, when PC12 cells were exposed to Aβ42 fibril for 48 h, cell viability decreased by about 50%, showing the severe toxicity of Aβ42 fibrils to the cells. However, co-treating Aβ42 with Lobaria extracts and DEPs notably reduced the toxicity in dose-dependent manner (Figure 3A). The presence of 30 μM NA, 30 μM SA, and 20 μM GA increased cell viability by 22.3%, 29.3% and 34.8%, respectively. Moreover, the presence of 12.5, 25, 50, 100, 200 μg/mL Lwater increased cell viability by 6.6%, 12%, 21, 6%, 23.5%, and 35.2%, respectively. Similarly, the presence of 12.5, 25, 50, 100, 200 μg/mL LEtOH increased cell viability by and 5.6% 11.9%, 19.5%, 27.7%, and 31.3%, respectively, This result suggested that Lobaria and its DEPs effectively protected PC12 cells from the injury mediated by toxic Aβ42 species.
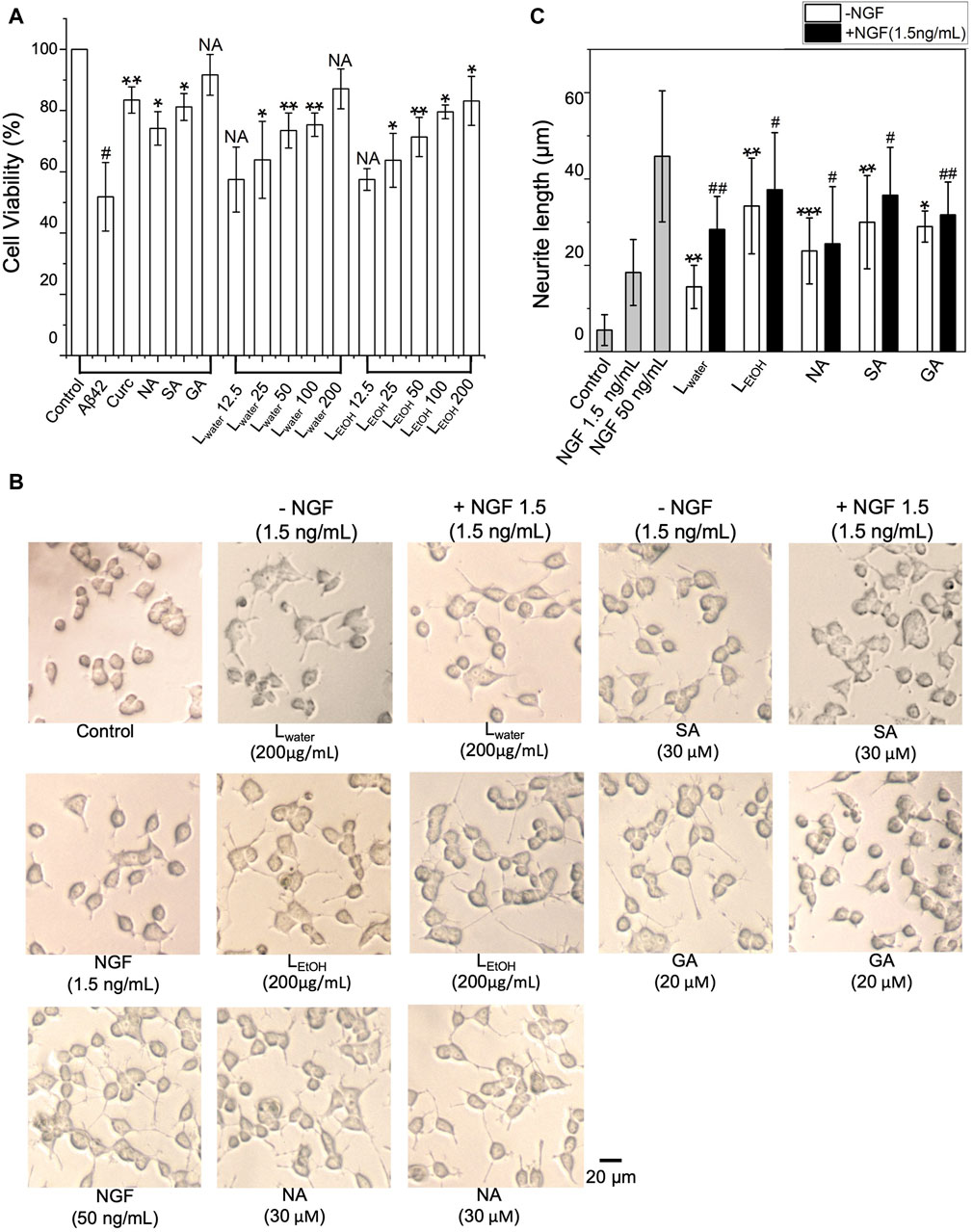
Figure 3. (A) Cytotoxicity of Aβ42 species depolymerized by DEPs (norstictic acid: NA, 30 μM; stictic acid: SA, 30 μM; gyrophoric aicd: GA, 20 µM) and different concentrations of Lobaria extracts (Lwater and LEtOH) determined using the MTT assay. #p < 0.05 compared to the control group treated only with PBS buffer; NS, not significant; *p < 0.05, **p < 0.01, and ***p < 0.001 compared to the samples with Aβ42 only. Values are presented in Mean ± SEM, n = 4. (B) Lobaria extracts and DEPs promote neurite outgrowth. The morphology of PC12 cells was analyzed under a light microscope after 48 h treatment of 200 μg/mL Lwater, 200 μg/mL LEtOH and NA (30 µM), SA (30 µM), GA (30 µM) presence or absence 1.5 ng/mL nerve growth factor (NGF). NGF at a concentration of 50 ng/mL was used as a positive control. (C) The average neurite length was measured after 48 h treatment with extracts and three DEPs in presence or absence of 1.5 ng/mL NGF. Values are presented in mean ± SEM fold change, n = 4. *p < 0.05, **p < 0.01, ***p < 0.001 compared to the control group. #p < 0.05, ##p < 0.01 compared to the group treated only with 1.5 ng/mL NGF.
The impact of Lobaria extracts and DEPs on the neurite outgrowth in cultured PC12 cells was assessed by measuring neurite length. As shown in Figure 3B, without nerve growth factor (NGF), Lobaria extracts or DEPs, PC12 cells had minimal neurites with very short lengths. However, when treated with NGF, Lobaria extracts, DEPs or a combination of NGF with extracts or DEPs, the cells developed long neurites. The comparison of neurite length for different treatments is shown in Figure 3C. The neurite length increased significantly from about 5 μm to above 20 μm when the cells were treated with LEtOH or DEPs. For the extract Lwater, the neurite length was about 15 μm. When the cells were treated with the extracts (or DEPs) and a low dose of NGF (1.5 ng/mL) simultaneously, the neurite outgrowth was further enhanced. In particular, the LEtOH and SA induced neurite outgrowth almost as effectively as the high dose NGF (50 ng/mL) when in the presence of a low dose 1.5 ng/mL NGF. These results indicate that the Lobaria extracts and DEPs can effectively promote neurite outgrowth and thus can be very helpful in treatment of the AD.
3.3 Lobaria extracts and DEPs disassemble Aβ42 fibrils
The deposition of mature Aβ42 fibrils in the brain causes the death of neurons. The toxic oligomers are mainly formed by the secondary nucleation mechanism, catalyzed by the fibers (Cohen et al., 2013). Thus, an excellent inhibitor should not only be able to inhibit Aβ fibrillation but also be able to disaggregate mature Aβ fibrils. For example, brazilin (Du et al., 2015), tanshinone (Ren et al., 2018) and curcumin (Ono et al., 2004) have been reported to be capable of killing the fibrillar structure of Aβ42 (Yang et al., 2005; Hamaguchi et al., 2010). In this work, Lobaria extracts, and its DEPs were tested for their ability to disassemble Aβ42 fibrils.
We used AFM to observe the structure of Aβ42 aggregates with and without treatment of Lobaria extracts (LEtOH and Lwater), and its DEPs (norstictic acid: NA; stictic acid: SA; gyrophoric acid: GA). As shown in Figure 4A, the untreated Aβ42 contained a dense population of mature fibrils with each fiber being several micrometers long. Once the Aβ42 fibrils solution was treated with Lwater (200 μg/mL), LEtOH (200 μg/mL), NA (30 μM), SA (30 μM), GA (20 μM), and curcumin (Curc, 20 μM), the long fibrils disappeared, and the remains were mostly spheroidal species, which could be the oligomers or oligomer clusters. Importantly, it was found that the Aβ42 remains contained a few short fibers, as shown by the insets of Figure 4A. Thus, the Aβ42 fibrils were broken down into oligomers or short fiber fragments by the Lobaria extracts or DEPs. The distributions of the height and length of the treated and untreated Aβ42 aggregates are shown in Figure 4B; Supplementary Figure S3, respectively, which statistically displayed the disaggregation effect. However, due to the significant uncertainties in preparing dried protein samples and the limited imaging area, the relative effectiveness of the extracts and DEPs could not be resolved.
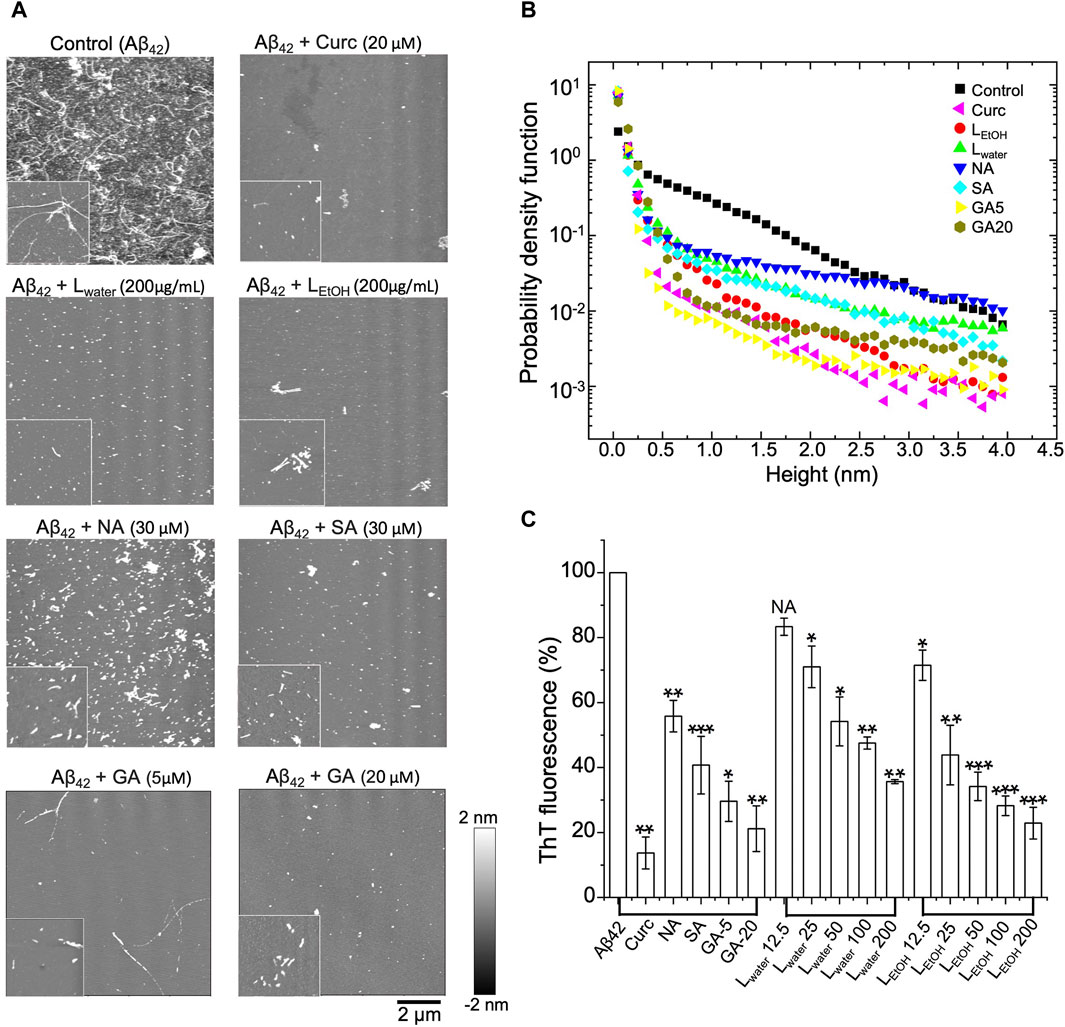
Figure 4. Disaggregation effect of Lobaria extracts (Lwater and LEtOH), and DEPs compounds (norstictic acid: NA; stictic acid: SA; gyrophoric acid: GA), against Aβ42 mature fibrils, Curcumin (Curc, 20 µM) was used as a positive control. (A) AFM images of the aggregates after treatment of the Aβ42 fibrils with the Lobaria extracts or the DEPs (Lwater 200 μg/mL, LEtOH 200 μg/mL, NA 30 μM, SA 30 μM, GA 20 μM, GA 5 µM). The images have a dimension of 10 μm × 10 μm, whereas the inset images have a dimension of 2 μm × 2 µm. The representative data in four independent experiments. (B) Probability distributions of the height of the Aβ42 aggregates after different treatments. (C) The final thioflavin T (ThT) fluorescence signal of Aβ42 mature fibrils incubated with NA, SA, GA and different concentrations of Lwater and LEtOH for 3 days. Values are presented in mean ± SEM fold change. n = 4, NS, not significant; *p < 0.05, **p < 0.01, and ***p < 0.001 compared to the samples with Aβ42 only.
To have a quantitative comparison of the disaggregation effect of different treatments, the ThT assay was applied. In the experiment, the mature Aβ42 fibrils were first co-incubated with Lwater, LEtOH, SA, NA, GA and curcumin, respectively, for 3 days. After that, ThT fluorescence dye was added to the solution, and the fluorescence intensity was measured. The relative intensity for different treatments compared to the control (i.e., PBS) was shown in Figure 4C. It was found that all treatments reduced the ThT fluorescence intensity, which signified a reduction of the β-sheet structure of the aggregates. The two extracts, LEtOH and Lwater, showed an improved efficiency with increasing concentrations, but LEtOH reduced the aggregates more than Lwater at all concentrations. For the three DEPs, the reduced efficiency rank was: GA > SA > NA, given about the same concentration of the DEPs. These results further confirmed the AFM results that the Lobaria extracts, and its DEPs, disassembled the mature Aβ42 fibrils by killing the β-sheet structure.
We conducted DLS assays to measure the size of Aβ42 aggregates in solution over time when treated with Lwater (200 μg/mL), LEtOH (200 μg/mL), NA (30 μM), SA (30 μM), GA (20 μM), and curcumin (5 μM). The results are shown in Figure 5A. In the figure, the data point at time 0 h was obtained right after (within 5 min) addition of the extracts or DEPs, and the data for the aggregate size right before the treatment was shifted to time −2 h for clarity. The measured aggregate size was normalized by the one right before the treatment. The aggregate size showed a remarkable decrease within 2 h of treatment for all tested extracts or DEPs and remained almost unchanged thereafter (Figure 5A). This result suggests that the Lobaria extracts and DEPs disassembled the Aβ42 fibrils into smaller-sized structures over a short period of time (less than 2 h). However, the efficiency in reducing the aggregate size varied. We plotted the relative aggregate size measured at time 2 h for all the treatments in Figure 5B, which showed that the rank of aggregate reduction efficiency was gyrophoric acid > LEtOH > curcumin > stictic acid ≈ norstictic acid ≈ Lwater. Furthermore, we examined the concentration-dependence effect on aggregate reduction for the most effective treatments, LEtOH and GA (Figures 5C–F). The results showed that the aggregate reducing efficiency increased with increasing concentrations of LEtOH (Figures 5C, D) and GA (Figures 5E, F) and saturated at a critical concentration, above which there was no further improvement. The critical concentrations of LEtOH and GA were 25 μg/mL and 5 μM respectively, corresponding to a reduction efficiency of ∼40% and ∼50%.
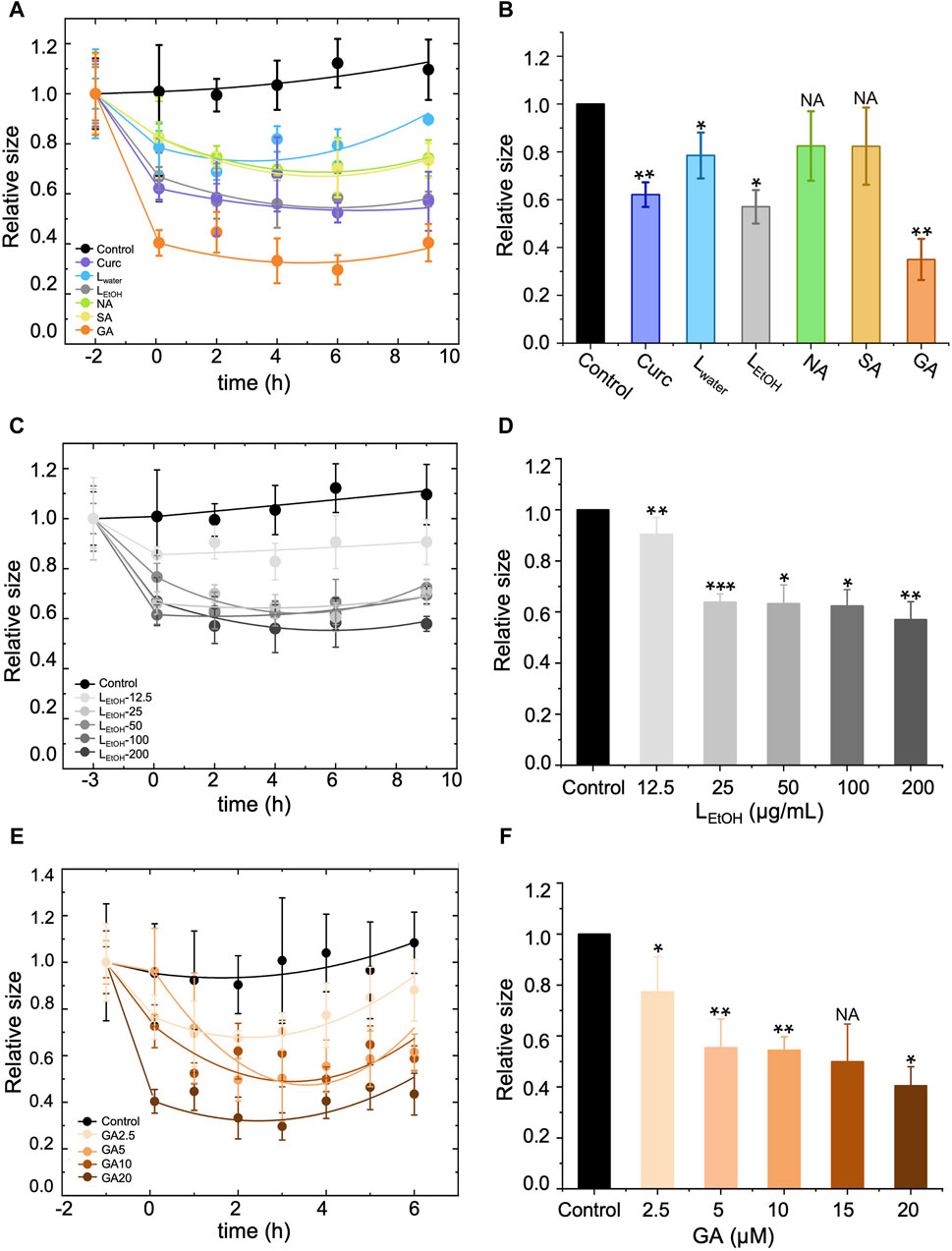
Figure 5. Effect of different treatments, i.e., Lobaria extracts (Lwater and LEtOH), norstictic acid (NA), stictic acid (SA), gyrophoric acid (GA) and curcumin (Curc) on the disassembly of the Aβ42 mature fibrils by dynamic light scattering assay (DLS). Curcumin (Curc, 5 μg/mL) was used a positive control. (A), (C) and (E) show change of the aggregate size with time for treatment of, respectively, (A) different materials (200 μg/mL Lwater, 200 μg/mL LEtOH, 30 μM NA, 30 μM SA, 20 μM GA, 5 μM Curc), (C) different concentrations of LEtOH (12.5, 25, 50, 100, 200 μg/mL), and (E) different concentrations of GA (2.5, 5, 10, 20 μM). The first data point of each curve was measured right before the addition of the extracts or DEPs and thus, represents the original size of the Aβ42 aggregates (mature fibrils). After the treatment, the aggregate size decreased with time and eventually saturated. (B), (D) and (F) show a comparison of the aggregate size at time = 2 h after the treatment of, respectively, (B) different materials (200 μg/mL Lwater, 200 μg/mL LEtOH, 30 μM NA, 30 μM SA, 20 μM GA, 5 μM Curc), (D) different concentrations of LEtOH (12.5, 25, 50, 100, 200 μg/mL), and (F) different concentrations of GA (2.5, 5, 10, 20 μM). Values are presented in mean ± SEM fold change. n = 4, NS, not significant; *p < 0.05, **p < 0.01, and ***p < 0.001 compared to the samples with Aβ42 only of control.
4 Discussion
The inhibitors having properties of inhibiting Aβ aggregation and promoting neurite outgrowth are novel drugs for the treatment of AD. In our study, we discovered that Lobaria water and ethanol extracts (Lwater, LEtOH) exhibit a superior inhibitory effect on Aβ aggregation through systematic biophysical and cellular experiments. The ThT fluorescence assay demonstrated that Lwater and LEtOH effectively delayed the onset of Aβ42 fibril formation and ultimately suppressed the formation of β-sheet-rich Aβ42 aggregates, with IC50 values of 19.61 ± 1.78 μg/mL and 32.53 ± 7.43 μg/mL, respectively (Figure 2). Increasing the concentrations of Lwater and LEtOH to 200 μg/mL resulted in significant inhibition of Aβ fibril formation. Notably, while Lwater exhibited a cell survival rate-promoting effect with increased concentration, LEtOH showed weak toxicity. Therefore, utilizing low concentrations of LEtOH for therapeutic purposes may be beneficial, as it demonstrates good functionality. AFM experiments further proved that Lwater and LEtOH could suppress the formation of long and dense Aβ42 such that only short fibrils and amorphous species of the Aβ aggregate could be formed. At the same time, MTT and neurite outgrowth tests have been performed and showed that these herbal extract-treated Aβ aggregation species have low toxicity and thus significantly protect neuronal cells from Aβ-induced cell damage (Figure 3). Within the experimental concentration range of Lwater and LEtOH, higher Lwater and LEtOH doses showed less toxicity of the Aβ species (Supplementary Figure S2).
What is more interesting is that Aβ42 mature fibrils can be depolymerized into short stick or amorphous off-pathway species with low toxicity in the presence of different amounts of Lwater and LEtOH, along with DEPs (Figure 3A). Among the extracts, LEtOH outperforms Lwater, with a high concentration of LEtOH (200 μg/mL) showing a disaggregating effect similar to that of 5 μM curcumin. Among the DEPs, gyrophoric acid demonstrates the highest efficacy, as 20 μM gyrophoric acid disassembled Aβ42 mature fibrils more effectively than 30 μM stictic acid, 30 μM norstictic acid, and 5 μM curcumin (Figures 5A, B).
The superior effectiveness of LEtOH over Lwater in inhibiting Aβ aggregation, disaggregating Aβ mature fibrils and promoting neurite outgrowth could be attributed to the higher content of DEPs in LEtOH, especially the gyrophoric acid. The symbiotic relationship between fungus and a photosynthetic partner in lichens produces a distinct metabolism that leads to the production of complex secondary metabolites, such as DEPs. Many DEPs have exhibited pharmacological properties, such as antioxidant, antimicrobial, and cytotoxic activities (Urena-Vacas et al., 2021). Most studies on DEPs have been conducted in cell models, with limited in vivo studies and clinical trials. Our study, for the first time, revealed the strong inhibitory effects of three DEPs (NA, SA, GA) on Aβ42 fibrillation and disaggregation of mature fibrils, with GA showing the highest effectiveness (Figures 5A, B). Furthermore, a molecular docking analysis was conducted to investigate the interactions between Aβ peptides and GA. The results showed a binding energy of −15.1 kJ/mol for GA, whereas curcumin had a binding energy of −22.4 kJ/mol (Supplementary Figure S4). According to molecular docking analysis, curcumin was found to bind to Aβ protein by forming a key hydrogen bond with ALA21 residue (Supplementary Figure S4B). On the other hand, GA displayed significant binding affinity to Aβ and bound to the same domain as curcumin. Interestingly, it was observed that GA established three hydrogen bonds with LEU17, PHE19 and ALA21, respectively (Supplementary Figure S4A). This suggests that GA may exhibit strong binding activity at the binding site, potentially leading to higher inhibitory effects on Aβ fibril formation. These findings align with our experimental results demonstrating the positive impact of GA. As a biological polysaccharide, GA has good bioavailability, which has also been proven by previous studies (Sovrlic et al., 2023; Thakur et al., 2023).
While Lobaria extracts and DEPs show promise as effective inhibitors of Aβ aggregation and potential neuroprotective agents for AD treatment, their development into anti-AD drugs poses significant challenges. Lobaria and other DEP-producing lichen species have slow growth rates, limiting DEPs productivity. To overcome limitations, alternative methods, like artificial culturing or fermentation, are being explored to acquire essential active ingredients and decrease dependence on natural resources. In addition, it is important to note that while the cell study provides valuable insights, further validation through a series of in vivo studies is essential for generalization. To overcome the challenges associated with in vivo efficacy and safety testing, the key considerations, such as selecting appropriate animal models, determining optimal dosage for in vivo administration, conducting comprehensive toxicity studies, assessing behavioural effects, should be implemented.
5 Conclusion
In conclusion, Lobaria extracts, and its DEPs demonstrate significant potential as inhibitors of Aβ42 peptides aggregation and neurotoxicity in AD. The study highlights the superior inhibitory effects of Lobaria water and ethanol extracts (Lwater, LEtOH) on Aβ42 fibrillation through comprehensive biophysical and cellular experiments. Notably, the extracts effectively delayed the onset of Aβ42 fibril formation and inhibited the formation of β-sheet-rich Aβ42 aggregates. Furthermore, the DEPs, particularly gyrophoric acid, exhibited strong efficacy in disintegrating mature Aβ fibrils, offering promising neuroprotective effects. The discoveries made here open up possibilities for the future utilization of Lobaria extracts and DEPs in the treatment of AD, offering a diverse range of therapeutic advantages.
Data availability statement
The original contributions presented in the study are included in the article/Supplementary Material, further inquiries can be directed to the corresponding author.
Ethics statement
Ethical approval was not required for the studies on animals in accordance with the local legislation and institutional requirements because only commercially available established cell lines were used.
Author contributions
MY: Conceptualization, Data curation, Formal Analysis, Investigation, Methodology, Visualization, Writing–original draft, Writing–review and editing, Software. CY: Writing–review and editing, Data curation, Methodology, Visualization. DO: Methodology, Writing–review and editing. LW: Investigation, Writing–review and editing. SL: Methodology, Writing–review and editing. WT: Methodology, Writing–review and editing. TD: Investigation, Writing–review and editing. PT: Supervision, Writing–review and editing. QX: Supervision, Writing–review and editing. KT: Supervision, Writing–review and editing, Conceptualization.
Funding
The author(s) declare that financial support was received for the research, authorship, and/or publication of this article. This work is supported by supported by Hong Kong Research Grants Council Hong Kong (GRF 16100921); Zhongshan Municipal Bureau of Science and Technology (2019AG035); Guangzhou Science and Technology Committee Research Grant (GZSTI16SC02; GZSTI17SC02); GBA Institute of Collaborate Innovation (GICI-022); The Key-Area Research and Development Program of Guangdong Province (2020B1111110006); Special project of Foshan University of Science and Technology in 2019 (FSUST19-SRI10); Hong Kong RGC Theme-based Research Scheme (T13-605/18-W); Hong Kong Innovation Technology Fund (ITS/500/18FP; MHP/004/21; GHP/016/21SZ; ITCPD/17-9; ITC-CNERC14SC01); TUYF19SC02, PD18SC01 and HMRF18SC06; HMRF20SC07, AFD20SC01; Shenzhen Science and Technology Innovation Committee (ZDSYS201707281432317).
Conflict of interest
The authors declare that the research was conducted in the absence of any commercial or financial relationships that could be construed as a potential conflict of interest.
Publisher’s note
All claims expressed in this article are solely those of the authors and do not necessarily represent those of their affiliated organizations, or those of the publisher, the editors and the reviewers. Any product that may be evaluated in this article, or claim that may be made by its manufacturer, is not guaranteed or endorsed by the publisher.
Supplementary material
The Supplementary Material for this article can be found online at: https://www.frontiersin.org/articles/10.3389/fphar.2024.1426569/full#supplementary-material
Abbreviations
Aβ, amyloid β peptides; AD, Alzheimer’s disease; DEPs, depsides and depsidones; NGF, nerve growth factor; ThT, thioflavin T fluorescence; AFM, atomic force microscopy; DLS, dynamic light scattering; MTT, 3-(4,5-dimethylthiazol-2-yl)-2,5-diphenyltetrazolium bromide; LEtOH, Lobaria ethanol extract; Lwater, Lobaria water extract; FBS, fetal bovine serum; DMSO, dimethyl sulfoxide; GA, gyrophoric acid; NA, norstictic acid; SA, stictic acid.
References
Adlard, P. A., Tran, B. A., Finkelstein, D. I., Desmond, P. M., Johnston, L. A., Bush, A. I., et al. (2014). A review of β-amyloid neuroimaging in Alzheimer's disease. Front. Neurosci. 8, 327. doi:10.3389/fnins.2014.00327
Bartus, R. T. (2000). On neurodegenerative diseases, models, and treatment strategies: lessons learned and lessons forgotten a generation following the cholinergic hypothesis. Exp. Neurol. 163 (2), 495–529. doi:10.1006/exnr.2000.7397
Biancalana, M., and Koide, S. (2010). Molecular mechanism of Thioflavin-T binding to amyloid fibrils. Biochim. Biophys. Acta - Proteins Proteom. 1804 (7), 1405–1412. doi:10.1016/j.bbapap.2010.04.001
Budni, J., Bellettini-Santos, T., Mina, F., Garcez, M. L., and Zugno, A. I. (2015). The involvement of BDNF, NGF and GDNF in aging and Alzheimer’s disease. Aging Dis. 6 (5), 331–341. doi:10.14336/AD.2015.0825
Calabrese, E. J. (2008). Enhancing and regulating neurite outgrowth. Crit. Rev. Toxicol. 38 (4), 391–418. doi:10.1080/10408440801981981
Chen, L., Huang, J., Yang, L., Zeng, X. A., Zhang, Y., Wang, X., et al. (2017). Sleep deprivation accelerates the progression of Alzheimer’s disease by influencing Aβ-related metabolism. Neurosci. Lett. 650, 146–152. doi:10.1016/j.neulet.2017.04.047
Cohen, S. I. A., Linse, S., Luheshi, L. M., Hellstrand, E., White, D. A., Rajah, L., et al. (2013). Proliferation of amyloid-β42 aggregates occurs through a secondary nucleation mechanism. Proc. Natl. Acad. Sci. 110 (24), 9758–9763. doi:10.1073/pnas.1218402110
Cui, G. Y., and Duan, H. (2000). Study on edible lichens in China. Jiangsu Agric. Res. 21 (3), 59–62. (In Chinese).
Du, W. J., Guo, J. J., Gao, M. T., Hu, S. Q., Dong, X. Y., Han, Y. F., et al. (2015). Brazilin Inhibits amyloid β-protein fibrillogenesis, remodels amyloid fibrils and reduces amyloid cytotoxicity. Sci. Rep. 5, 7992. doi:10.1038/srep07992
Ebrahim, H. Y., Elsayed, H. E., Mohyeldin, M. M., Akl, M. R., Bhattacharjee, J., Egbert, S., et al. (2016). Norstictic acid inhibits breast cancer cell proliferation, migration, invasion, and in vivo invasive growth through targeting C-Met. Phytotherapy Res. 30 (4), 557–566. doi:10.1002/ptr.5551
Eratne, D., Loi, S. M., Farrand, S., Kelso, W., Velakoulis, D., and Looi, J. C. (2018). Alzheimer’s disease: clinical update on epidemiology, pathophysiology and diagnosis. Australas. Psychiatry. 26 (4), 347–357. doi:10.1177/1039856218762308
Hamaguchi, T., Ono, K., and Yamada, M. (2010). Review: curcumin and Alzheimer's disease. CNS Neurosci. and Ther. 16 (5), 285–297. doi:10.1111/j.1755-5949.2010.00147.x
Hardy, J., and Selkoe, D. J. (2002). The amyloid hypothesis of Alzheimer’s disease: progress and problems on the road to therapeutics. Science 297 (5580), 353–356. doi:10.1126/science.1072994
Hidalgo, M. E., Fernandez, E., Quilhot, W., and Lissi, E. (1994). Antioxidant activity of depsides and depsidones. Phytochemistry 37 (6), 1585–1587. doi:10.1016/s0031-9422(00)89571-0
Huang, L. K., Kuan, Y. C., Lin, H. W., and Hu, C. J. (2023). Clinical trials of new drugs for Alzheimer disease: a 2020–2023 update. J. Biomed. Sci. 30 (1), 83. doi:10.1186/s12929-023-00976-6
Jia, L., Wang, W., Yan, Y., Hu, R., Sang, J., Zhao, W., et al. (2020). General aggregation-induced emission probes for amyloid inhibitors with dual inhibition capacity against amyloid β-protein and α-synuclein. ACS Appl. Mater. Interfaces. 12, 31182–31194. doi:10.1021/acsami.0c07745
Jia, L. G., Wang, W. J., Shang, J. Z., Zhao, W. P., Wei, W., Wang, Y., et al. (2018). Highly efficient soluble expression, purification and characterization of recombinant Aβ42 from Escherichia coli. RSC Adv. 8 (33), 18434–18441. doi:10.1039/c8ra00042e
Kowall, N. W., Beal, M. F., Busciglio, J., Duffy, L. K., and Yankner, B. A. (1991). An in vivo model for the neurodegenerative effects of β-amyloid and protection by substance P. Proc. Natl. Acad. Sci. 88 (16), 7247–7251. doi:10.1073/pnas.88.16.7247
Linse, S. (2019). Mechanism of amyloid protein aggregation and the role of inhibitors. Pure Appl. Chem. 91 (2), 211–229. doi:10.1515/pac-2018-1017
Mohammadi, M., Bagheri, L., Badreldin, A., Fatehi, P., Pakzad, L., Suntres, Z., et al. (2022). Biological effects of gyrophoric acid and other lichen derived metabolites, on cell proliferation, apoptosis and cell signaling pathways. Chem. Biol. Interact. 5 (351), 109768. doi:10.1016/j.cbi.2021.109768
Moskot, M., Jakóbkiewicz-Banecka, J., Kloska, A., Piotrowska, E., Narajczyk, M., and Gabig-Cimińska, M. (2019). The role of dimethyl sulfoxide (DMSO) in gene expression modulation and glycosaminoglycan metabolism in lysosomal storage disorders on an example of mucopolysaccharidosis. Int. J. Mol. Sci. 20, 304. doi:10.3390/ijms20020304
Ono, K., Hasegawa, K., Naiki, H., and Yamada, M. (2004). Curcumin Has Potent anti-amyloidogenic effects for Alzheimer’s β-amyloid fibrils in vitro. J. Neuro Sci. Res. 75 (6), 742–750. doi:10.1002/jnr.20025
Pagano, K., Tomaselli, S., Molinari, H., and Ragona, L. (2020). Natural compounds as inhibitors of Aβ peptide aggregation: chemical requirements and molecular mechanisms. Front. Neurosci. 14, 619667. doi:10.3389/fnins.2020.619667
Ren, B. P., Liu, Y. L., Zhang, Y. X., Zhang, M. Z., Sun, Y., Liang, G. Z., et al. (2018). Tanshinones inhibit hIAPP aggregation, disaggregate preformed hIAPP fibrils, and protect cultured cells. J. Mater. Chem. 6 (1), 56–67. doi:10.1039/c7tb02538f
Sovrlic, M., Manojlovic, N., Kosanic, M., Kocovic, A., Tomovic, J., and Vasiljevic, P. (2023). “Lichenochemical analysis and in vitro antioxidant activity of extracts and gyrophoric acid from lichen Umbilicaria grisea,” in 2nd international conference on chemo and BioInformatics (Kragujevac, Serbia: University of Kragujevac, Institute for Information Technologies).
Thakur, M., Bhardwaj, S., Kumar, V., and Rodrigo-Comino, J. (2023). Lichens as effective bioindicators for monitoring environmental changes: a comprehensive review. Total Environ. Adv. 9, 200085. doi:10.1016/j.teadva.2023.200085
Urena-Vacas, I., Gonzalez-Burgos, E., Divakar, P. K., and Gomez-Serranillos, M. P. (2021). Lichen depsidones with biological interest. Planta Medica 88 (11), 855–880. doi:10.1055/a-1482-6381
Yang, F., Lim, G. P., Begum, A. N., Ubeda, O. J., Simmons, M. R., Ambegaokar, S. S., et al. (2005). Curcumin inhibits formation of amyloid beta oligomers and fibrils, binds plaques, and reduces amyloid in vivo. JBC 280 (7), 5892–5901. doi:10.1074/jbc.M404751200
Yang, M. X., Cheng, E. H. C., Ospondpant, D., Tung, K. K., Wang, L. S., Lin, S. Y., et al. (2023). The extracts of Lobaria pindarensis, an edible lichen from Himalayas, suppress inflammatory response and fibrillation of amyloid β-protein in cultured microglial cells: active depsidones, norstictic acid and stictic acid, are responsible for the functions. J. Funct. Foods. 107, 105607. doi:10.1016/j.jff.2023.105607
Keywords: Alzheimer’s disease, Aβ peptides, Lobaria extracts, depsides/depsidones, inhibition, disaggregation, neurite outgrowth
Citation: Yang M, Yan C, Ospondpant D, Wang L, Lin S, Tang WL, Dong TT, Tong P, Xu Q and Tsim KWK (2024) Unveiling the therapeutic potential of Lobaria extract and its depsides/depsidones in combatting Aβ42 peptides aggregation and neurotoxicity in Alzheimer’s disease. Front. Pharmacol. 15:1426569. doi: 10.3389/fphar.2024.1426569
Received: 01 May 2024; Accepted: 29 July 2024;
Published: 13 August 2024.
Edited by:
Venkatesh Katari, University of Toledo, United StatesReviewed by:
Prabhat Upadhyay, Massachusetts General Hospital, United StatesChiranjeevi Tikka, Indiana University School of Medicine, Indianapolis, United States
Santhosh Kumar Pasupuleti, Indiana University School of Medicine, United States
Sadaf Jahan, Majmaah University, Saudi Arabia
Copyright © 2024 Yang, Yan, Ospondpant, Wang, Lin, Tang, Dong, Tong, Xu and Tsim. This is an open-access article distributed under the terms of the Creative Commons Attribution License (CC BY). The use, distribution or reproduction in other forums is permitted, provided the original author(s) and the copyright owner(s) are credited and that the original publication in this journal is cited, in accordance with accepted academic practice. No use, distribution or reproduction is permitted which does not comply with these terms.
*Correspondence: Karl Wah Keung Tsim, botsim@ust.hk