- Department of Anesthesiology, The First Affiliated Hospital of Sun Yat-sen University, Guangzhou, China
Renal dysfunction, including acute renal failure (ARF) and chronic kidney disease (CKD), continues to present significant health challenges, with renal ischemia-reperfusion injury (IRI) being a pivotal factor in their development and progression. This condition, notably impacting kidney transplantation outcomes, underscores the urgent need for innovative therapeutic interventions. The role of opioid agonists in this context, however, remains a subject of considerable debate. Current reviews tend to offer limited perspectives, focusing predominantly on either the protective or detrimental effects of opioids in isolation. Our review addresses this gap through a thorough and comprehensive evaluation of the existing literature, providing a balanced examination of the dualistic nature of opioids’ influence on renal health. We delve into both the nephroprotective and nephrotoxic aspects of opioids, dissecting the complex interactions and paradoxical effects that embody the “two sides of the same coin” phenomenon. This comprehensive analysis is vital for understanding the intricate roles of opioids in renal pathophysiology, potentially informing the development of novel therapeutic strategies for preventing or treating hypoxic kidney injury.
Highlights
1. Current research shows that while some opioids may help prevent damage from reduced blood flow and restoration to the kidneys, others may lead to negative effects such as increased cell death or disruption of kidney function.
2. Opioids may have a complex and dual role in kidney health, where they can both protect and harm the kidneys, depending on various factors such as dosage and specific opioid receptors targeted.
3. There is a need for more detailed and standardized research to fully understand how different opioids affect kidney health, which could lead to better and safer treatments for kidney-related diseases.
1 Introduction
Renal dysfunction, encompassing both acute renal failure (ARF) and chronic kidney disease (CKD), continues to be a significant health burden, with morbidity and mortality rates that have shown little improvement over the past 3 decades (Star, 1998; Imai et al., 2009). A pivotal factor in the development and progression of both acute and chronic renal dysfunction is renal ischemia-reperfusion injury (IRI), which is recognized as a leading cause and a common pathological pathway in these conditions (Liu et al., 2018).
Kidney transplantation remains the definitive treatment for end-stage renal disease, offering the best outcomes in terms of quality of life and longevity. However, the success of transplantation is frequently compromised by IRI, which adversely affects both short-term and long-term graft survival (Chatterjee, 2007; Wu et al., 2007). This highlights a critical gap in current medical practice, as effective strategies to mitigate renal IRI are notably lacking. The inadequacy of existing approaches underscores the urgent need for innovative therapeutic interventions to address this formidable challenge in renal transplantation (Wever et al., 2013).
Opioid receptors (ORs) and their ligands—encompassing both endogenous opioid peptides and exogenous opiate alkaloids—are known to affect a myriad of physiological functions, including sensory processes, neuroendocrine regulation, food consumption, and autonomic control (Mutoh et al., 2013). The identification of three classical OR subtypes—mu (MOR), delta (DOR), and kappa (KOR)—along with the less well-characterized nociceptin/orphanin FQ peptide (NOP; also referred to as opioid receptor-like 1 peptide) receptor, has significantly advanced our understanding of opioid pharmacology over recent decades (Mansour et al., 1987; Kapusta et al., 2005). Endogenous opioid peptides (EOPs), which are naturally occurring peptides within the body, include enkephalins, endorphins, and dynorphins. These peptides specifically bind to DOR, MOR, and KOR, and play a crucial role in modulating these physiological functions. For instance, enkephalins primarily interact with delta receptors, while beta-endorphins have high affinity for both mu and delta receptors, and dynorphins are more selective for kappa receptors. In contrast, exogenous opiate alkaloids, such as morphine, codeine, and oxycodone, are external substances introduced into the body. These alkaloids bind to ORs and can affect a broad range of physiological functions (Cox, 1982; Lefebvre, 1986). The widespread use of opioid agonists for pain management, particularly in the perioperative period of procedures such as kidney transplantation, and in treating pain associated with end-stage renal disease (Franco-Acevedo et al., 2020), underscores the importance of elucidating their effects on renal pathophysiology. However, this aspect remains a subject of considerable debate within current scientific literature. Some studies suggest a protective role of opioids against renal IRI (Habibey et al., 2010; Luo et al., 2020), akin to their reported benefits in other organs such as the heart and brain (He et al., 2013; Maslov et al., 2016). In contrast, other evidence indicates that activation of ORs may be detrimental to kidney health (Yoo et al., 2018; Dasari et al., 2021). This apparent contradiction may be attributed to the complex interplay of OR activation and the subsequent biological responses. For instance, opioid-induced sympathetic nerve excitation can contribute to AKI in the context of IRI (Mutoh et al., 2013), while, on the other hand, opioids might provide protective effects by mimicking ischemic preconditioning to alleviate renal IRI (Lee and Emala, 2001).
Existing literature reviews addressing the impact of opioids on renal diseases often present a limited perspective, tending to focus on either the protective or detrimental effects in isolation (Mercadante and Arcuri, 2004; Palomino et al., 2019; Mallappallil et al., 2021). In an effort to bridge this gap, our review aims to deliver a more balanced and comprehensive examination of the dualistic nature of opioids’ influence on renal health. We will delve into both the nephroprotective and nephrotoxic aspects of opioids, endeavoring to unravel the paradoxical “two sides of the same coin” phenomenon, drawing upon a wealth of literature evidence accumulated over recent decades. By dissecting these complex interactions, this review aspires to shed light on potential pathways that could inform the development of novel therapeutic strategies, aimed at either preventing or treating hypoxic kidney injury.
2 Expression of ORs in kidney
Understanding the localization of ORs is fundamental to comprehending how they exert their effects on the kidney. Historically, these receptors were primarily associated with the central nervous system (CNS), recognized for their role in mediating anti-nociception (Millan, 1986). Subsequent research expanded this view, revealing the presence of ORs in peripheral tissues, including the kidneys (Peng et al., 2012; Weber et al., 2013).
While the existence of ORs in renal tissues is now well-established, the exact distribution and prevalence of their subtypes continue to be debated. Table 1 collates findings from various studies that have explored the expression of ORs in the kidney across different species. Despite the diversity of methodologies and species studied, and the resulting discrepancies in outcomes, there is a general agreement on the consistent expression of KOR in the kidneys.
Variations in the reported expression levels of MOR and DOR are not unexpected, considering the differences in experimental animal species, techniques used, and experimental conditions. To converge on a more coherent understanding of OR distribution in the kidney, future research endeavors would benefit from a concerted effort to standardize experimental protocols and methodologies across varied animal models. This approach would facilitate a more consistent and reliable interpretation of OR expression and their subsequent impact on renal physiology.
3 Functions of opioids on the kidney
The functional impact of ORs on renal physiology and pathology presents a more intricate and debatable landscape than the mere presence of these receptors in kidney tissue. In the ensuing sections, this review will undertake a comprehensive exploration of various common opioids along with specific agonists for each recognized subtype of ORs. The aim is to systematically dissect their individual as well as synergistic influences on renal function and pathology. This analysis is critical for unraveling the nuanced roles that these receptors and their ligands play in renal health and disease, thereby contributing to a more profound understanding of renal pharmacology and therapeutics.
3.1 Morphine
Morphine is recognized primarily as a potent MOR agonist (Ricarte et al., 2021); however, it also exhibits binding affinity to KOR and DOR (Chen et al., 1993). Although morphine stands as the most extensively studied opioid agonist within the corpus of opioid-related research, there remains a considerable lacuna in the literature regarding the specific OR subtypes implicated in its pharmacodynamic effects. The prevailing investigations into morphine’s action do not consistently delineate the particular receptor subtype—MOR, KOR, or DOR—to which its therapeutic and adverse effects can be attributed. Yet, a significant gap persists in discerning the effects attributable to specific OR subtypes. Historically, the scientific exploration into morphine’s effects initially prioritized understanding its influence on renal physiology. Kapusta et al. (1991) discerned a marked diminution in both urinary flow rate and sodium excretion subsequent to morphine administration in anesthetized rodents. On this basis, Flores et al. posited a dose- and temporally contingent paradigm, wherein lower morphine dosages may induce a transient augmentation of urine output within the initial hour following administration (Flores et al., 1997).
Pathological implications of morphine on renal health are subject to considerable debate. A number of investigations have postulated detrimental effects on the healthy kidney (Singhal et al., 1997; Atici et al., 2005; Weber et al., 2008; Lan et al., 2013; Luo et al., 2013; Jalili et al., 2017), with mechanisms encompassing apoptosis induction via mitochondria-dependent pathways (Luo et al., 2013), perturbation of the glomerular filtration apparatus (Lan et al., 2013), incitement of glomerulopathy (Weber et al., 2008), and facilitation of mesangial and medullary interstitial cell proliferation (Singhal et al., 1997; Weber et al., 2008). Notably, Weber et al. and Lan et al. have implicated KOR in mediating these harmful outcomes, rather than MOR, thus challenging conventional receptor-associated paradigms (Singhal et al., 1997; Lan et al., 2013).
Conversely, other scholarly works advocate for a renoprotective role of morphine in mitigating renal IRI (Habibey and Pazoki-Toroudi, 2008; Habibey et al., 2010; Pazoki-Toroudi et al., 2010; Bellini et al., 2016; Franco-Acevedo et al., 2020), with proposed mechanisms including the facilitation of vascular repair and angiogenic responses (Franco-Acevedo et al., 2020), enhancement of cell viability coupled with caspase modulation (Bellini et al., 2016), and the upregulation of nitric oxide production alongside inducible nitric oxide synthase expression (Habibey et al., 2010). The majority of these studies are rooted in animal experimentation, with a select few employing cellular models (Lan et al., 2013; Bellini et al., 2016).
Despite the dichotomous conclusions drawn by Bellini et al. and Lan et al., both research endeavors intriguingly implicate KOR as the pivotal site of morphine’s action within opossum kidney proximal tubular cells and human podocytes, respectively (Lan et al., 2013; Bellini et al., 2016). This denotes a compelling avenue for further inquiry, as the elucidation of receptor-specific pathways may afford critical insights into the nuanced roles of opioids in renal pathophysiology.
3.2 Fentanyl and its analogues
Fentanyl and its analogues represent a class of potent MOR agonists, exhibiting a higher affinity for MOR compared to morphine. Frequently, fentanyl is examined in conjunction with morphine due to their analogous effects on physiological processes, albeit with varying potencies (Bellini et al., 2016; Franco-Acevedo et al., 2020). Notably, remifentanil, an ultra-short-acting analogue of fentanyl, has garnered increasing attention in recent scientific inquiries. Xiong et al. demonstrated that pre-treatment with remifentanil ameliorates renal IRI in rodent models (Xiong et al., 2012). Corroborating these findings, Vianna et al. reported protective outcomes following the administration of remifentanil in isoflurane-anesthetized rats (Vianna et al., 2009). These observations from animal models received clinical substantiation through a retrospective study by Terashi et al., 2013, which indicated a temporary amelioration of renal function in perioperative adult CKD patients during orthopedic procedures.
Despite these insights, the consensus on the renoprotective effects of remifentanil remains tentative, with a pressing need for further empirical validation through meticulously structured experiments and clinical trials. The exploration into other fentanyl analogues, such as 4-fluoro-isobutyrylfentanyl, 4-chloroisobutyrylfentanyl, and isobutyrylfentanyl, is comparatively sparse. Ono et al. (2023) have delineated the nephrotoxic potential of these substances, positing respiratory depression mediated by MOP receptors as the underlying mechanism of renal impairment.
3.3 Tramadol
Tramadol, a less potent opioid, demonstrates a modest affinity for the MOR and weaker interactions with DOR and KOR (Raffa et al., 1992). The impact of tramadol on renal function is currently a subject of extensive debate. Sen et al. and Oliveira et al. observed that short-term administration of tramadol can decrease reactive oxygen species, thereby alleviating oxidative stress in animal models, suggesting a protective effect on the kidneys (de Oliveira et al., 2017; Sen et al., 2020). Contrarily, studies by Kizilkaya et al., 2002; Buhari et al., 2012 reported that tramadol’s impact on renal health in various animal models was neither beneficial nor harmful, indicating a neutral effect.
Further complicating the understanding of tramadol’s renal implications, Abdullah et al. and Elnagar et al. presented findings that suggest potential renal damage following prolonged use (exceeding 1 month) (Abdullah, 2018; Elnagar et al., 2022). This perspective is echoed in several clinical studies examining long-term opioid prescriptions, including tramadol, which have indicated potential harm to both healthy and transplanted kidneys during extended use (Barbosa-Leiker et al., 2016; Abbott et al., 2018; Novick et al., 2019). Although there is comparatively substantial evidence suggesting that long-term tramadol usage could be detrimental to kidney health, the implications of short-term use remain less clear and warrant further investigation.
3.4 Agonists with high selectivity of classical ORs
To delineate the distinct functionalities of various OR subtypes, researchers have employed highly selective agonists. This approach facilitates a more precise understanding of receptor-specific actions, particularly in renal physiology. A notable focus has been placed on KORs, with U-50488H, Asimadoline, and Niravoline serving as prototypical selective KOR agonists. Investigations utilizing these compounds have yielded consensus findings on the renal effects of KOR activation. Predominantly, activation of KOR has been associated with diuresis, antinatriuresis, and an increase in renal sympathetic nerve activity (Ashton et al., 1990; Kapusta and Obih, 1993; Moreau et al., 1996; Kramer et al., 2000; Gottlieb et al., 2005). Liu et al. (2018) conducted a notable animal study that observed a protective effect against renal IRI, purportedly mediated via the phosphatidylinositol 3 kinase (PI3K)/protein kinase B (AKT) signaling pathway. However, the necessity for additional research to corroborate these findings remains paramount.
In contrast, the understanding of MOR subtype functions has been informed by studies using highly selective MOR agonists, such as Dermorphin, cyclo [N-epsilon,N-beta-carbonyl-D-Lys (2) Dap (5)]enkephalinamide (cUENK6), and TAPP (H-Tyr-d-Ala-Phe-Phe-NH2). These studies consistently report diuretic effects following MOR activation (Kapusta et al., 1993; Gutkowska and Schiller, 1996; Gutkowska et al., 2004). However, there exists a notable divergence in findings related to sodium excretion. This discrepancy underscores the complexity inherent in MOR-mediated renal responses and highlights the need for further investigative efforts to elucidate these mechanisms more clearly.
Turning to the DOR, research has employed highly selective agonists such as UFP-512 (H-Dmt-Tic-NH-CH(CH2-COOH)-Bid) and DADLE ([D-Ala2, D-Leu5]-Enkephalin) to probe its renal implications. These studies have consistently observed protective effects on renal function, both in rat kidney epithelial cell models and in rat models of kidney disease (Habibey et al., 2010; Lian-Jin et al., 2010; Luo et al., 2020). While these observations regarding DOR agonists suggest potential avenues for therapeutic applications in renal pathologies, it is important to approach these conclusions with caution. The current body of research, although promising, requires further validation to ascertain the robustness and applicability of these findings. This is particularly crucial given the varying quality and scope of studies in this domain, which may impact the reliability of these preliminary conclusions.
Despite these advancements, a significant limitation of current research is the predominant reliance on animal models. This raises the question of how accurately these findings can be extrapolated to human physiology. Moreover, there exists a need to differentiate the effects mediated by central OR from those associated with receptors located directly in the kidney. While most studies have tended to overlook this aspect, recognizing and addressing this distinction is crucial for developing a more nuanced and comprehensive understanding of OR functions in renal physiology.
3.5 EOPs
EOPs are a fascinating class of molecules that have been found to influence various physiological systems in the body, including the kidneys. However, the literature on EOPs is relatively sparse, as very few studies directly use endogenous peptides to investigate opioid receptors, especially in the field of kidney research. This is largely due to the availability and convenience of using exogenous opioids in experiments. Despite this limitation, there is still some evidence that demonstrates the role of EOPs in renal function.
One of the key roles of EOPs in renal function is their contribution to renal adaptation to sodium restriction (Dibona and Jones, 1994). In normotensive rats, the inhibition of endogenous peripheral opioid mechanisms impaired the normal renal adaptive response to dietary sodium restriction. This suggests that EOPs are important for helping the kidneys adjust to changes in sodium intake.
EOPs also play a role in the regulation of renal responses during stress (Kapusta et al., 1989). Studies have shown that EOPs contribute to the antinatriuretic response (reduced sodium excretion) to environmental stress, which is mediated through the central nervous system. This indicates that EOPs are involved in helping the body conserve sodium during times of stress.
Another interesting effect of EOPs on renal function is their ability to stimulate renal growth (Haddox and Russell, 1979). The opioid peptide β-endorphin has been shown to increase the activity of renal ornithine decarboxylase, a marker of tissue growth response. This suggests that EOPs may be involved in regulating the growth and development of the kidneys.
Furthermore, Recent studies have identified proenkephalin (PENK), a stable surrogate marker for endogenous enkephalins, as a promising biomarker for kidney function. PENK is freely filtered by the glomerulus and has been shown to strongly correlate with glomerular filtration rate (GFR) (Ng et al., 2017; Schulz et al., 2017; Khorashadi et al., 2020). Moreover, elevated PENK concentrations have been linked to both acute kidney injury (AKI) and chronic kidney disease (CKD), as well as long-term kidney outcomes and mortality in various clinical settings, such as heart failure and acute myocardial infarction (Ng et al., 2014; Arbit et al., 2016; Matsue et al., 2017).
While the current evidence provides valuable insights into the role of EOPs, particularly enkephalins, in renal function, the specific mechanisms by which endogenous opioid peptides act on the kidneys remain unclear. The growing body of research on PENK as a novel biomarker for kidney function and its association with clinical outcomes highlights the potential importance of the opioid system in renal physiology. However, more research is needed to elucidate the precise pathways and signaling cascades involved in EOP-mediated regulation of renal function. Future studies should focus on using endogenous opioid peptides to investigate their effects on the kidneys and to unravel the complex interplay between the opioid system and renal physiology.
3.6 NOP
NOP, a 17-amino acid opioid-like peptide, is identified as the endogenous ligand for the NOP receptor, also known as opioid receptor-like 1 (Meunier et al., 1995). Although primarily located in the CNS, its presence in the kidney is less understood, with few studies indicating renal expression (Osinski et al., 1999). The role of NOP in renal physiology, particularly its diuretic and antinatriuretic effects, has been notably explored by Prof. Kapusta (Kapusta et al., 1997; 1999). These findings imply that NOP’s primary renal effects may be exerted indirectly, possibly outside the kidney.
The mechanisms by which the NOP receptor influences renal function, specifically in water and sodium balance, remain largely speculative. Calo et al. (2000) have suggested that these effects could be mediated through the inhibition of vasopressin secretion from supraoptic nucleus neurons. Research specifically addressing the impact of the NOP receptor on renal pathology is exceedingly scarce. Given the potential implications of NOP receptor modulation in renal function and its pathological states, there is a pressing need for further research in this area. Studies exploring both the molecular mechanisms of NOP receptor activity and its physiological effects on the kidney are essential to fully understand the role of this receptor system in renal health and disease.
3.7 Other opioids
The landscape of research on the renal effects of opioids beyond the commonly studied agonists is comparatively sparse. Buprenorphine and Biphalin, characterized by their broad affinity across all OR subtypes (Lukowiak et al., 2009; Davis et al., 2018), are two of the few examples in this field that have been studied. In a study conducted by Deng et al., 2000, it was observed that short-term administration of Buprenorphine did not significantly alter renal function in rat models. This finding suggests a potential renal safety profile for Buprenorphine, at least in the context of acute administration. Biphalin, a synthetic opioid with non-addictive properties, has also been the subject of renal studies. Notably, one study reported that Biphalin administration could maintain renal blood flow and reduce mean arterial pressure in spontaneously hypertensive rats, indicating potential therapeutic implications in hypertensive renal pathologies (Bądzyńska et al., 2016).
Naloxone, a non-selective opioid antagonist, has been observed in several studies to potentially benefit diseased kidneys in rat models (Mutoh et al., 2013; Takhtfooladi et al., 2016). The proposed mechanism for this beneficial effect might involve the inhibition of renal sympathoexcitation (Mutoh et al., 2013). Furthermore, the selective delta opioid antagonist 7-benzylindanylnaltrexone has shown promise in improving renal function in kidney-transplanted rats (Linner et al., 1998).
3.8 Sex differences in opioid effects on renal health
Despite the growing body of literature on the renal effects of opioids, there is a paucity of research specifically addressing sex differences in this context. While studies have demonstrated that sex can influence the analgesic effects of opioids, with some of these differences attributed to variations in pharmacokinetics and hormone levels between males and females (Craft, 2003; Fillingim and Gear, 2004), the potential sex-specific impact of opioids on renal function remains largely unexplored.
Animal studies have yielded inconsistent findings regarding sex differences in opioid-induced antinociception, with some reports suggesting greater sensitivity in males and others indicating no significant differences or even enhanced effects in females, depending on the specific opioid, dose, and pain model employed (Bartok and Craft, 1997; Craft, 2003). These discrepancies underscore the complexity of sex-related factors in modulating opioid responses and highlight the need for a more comprehensive examination of these differences across various experimental paradigms.
In the clinical setting, a systematic review and meta-analysis by Pisanu et al., 2019 found that while men and women may differ in their response to opioids for pain relief, these differences are significantly influenced by factors such as age and comorbid mental disorders. However, the role of these factors is not routinely considered in the prescription of opioids for pain management. The authors emphasize the urgent need for clinical trials that provide sex-specific data and account for potential confounding variables.
Given the established sex differences in opioid analgesia, which are partially mediated by pharmacokinetic factors and hormone levels (Craft, 2003; Huhn et al., 2018), it is plausible to hypothesize that sex may also play a role in the way opioids influence renal health. However, the current body of evidence is insufficient to draw definitive conclusions about these potential sex-specific effects. Future research should focus on elucidating the sex-based differences in the impact of opioids on renal function, with studies designed to investigate the pharmacokinetic and pharmacodynamic properties of opioids in the context of renal health, and a specific emphasis on sex as a variable. Additionally, clinical studies examining the long-term effects of opioid use on renal outcomes in both males and females would provide valuable insights into any potential sex-specific risks or protective factors.
The divergent effects of these opioid agonists and antagonists on the kidney highlight the complexity of OR subtypes and their varied influences on renal physiology. This complexity is compounded by the fact that the direction of these effects does not appear to be uniform across different agents, thereby challenging the establishment of a consensus. In the subsequent sections, we endeavor to dissect the underlying causes of this variability and explore the differential roles of OR subtypes in renal function.
4 What makes the coin’s two sides?
4.1 Central or peripheral
First, the pharmacokinetic properties of opioids, including their ability to cross the blood-brain barrier (BBB) and their distribution within different tissues, may play a significant role in modulating their renal effects. ORs, predominantly expressed in the CNS, play a pivotal role in mediating the systemic effects of most opioid drugs. A significant aspect of opioid pharmacodynamics is the ability of these drugs to traverse the BBB, thereby exerting central effects that can indirectly influence renal function. This interaction suggests that renal effects of opioids may not be solely attributed to their direct action on renal tissue but could also be a consequence of altered systemic hemodynamics and renal sympathetic nerve activity (RSNA) (Figure 1).
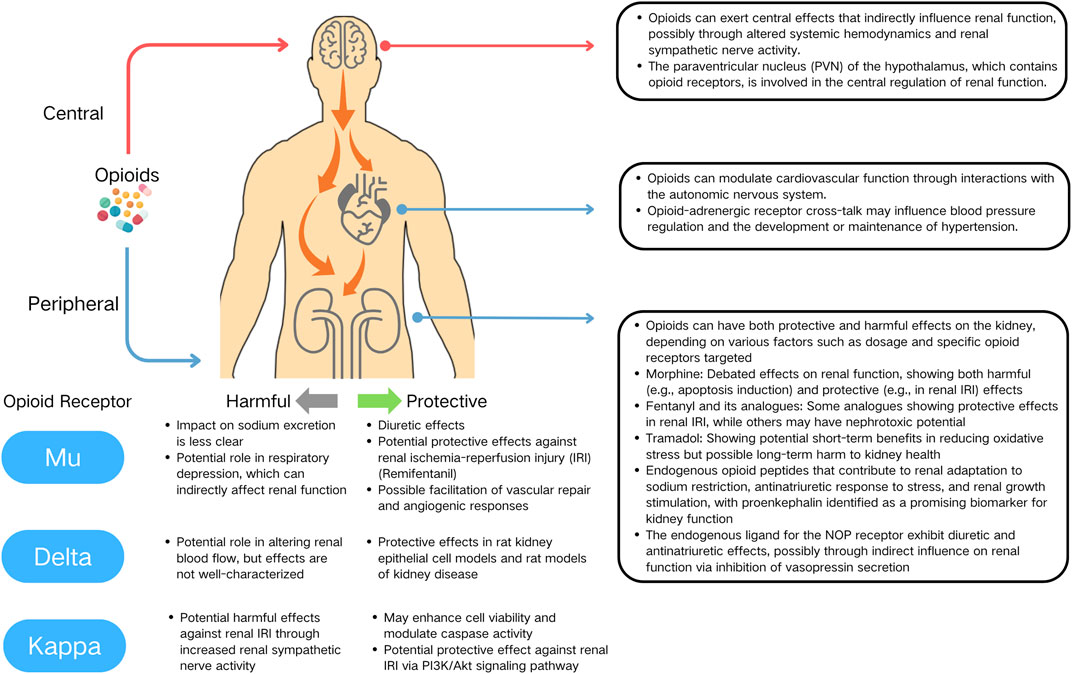
Figure 1. Multifaceted actions of opioids on the kidney, cardiovascular system, and central nervous system.
This hypothesis gains support from experimental findings by Prof. Kapusta and colleagues. They demonstrated divergent renal responses to opioids depending on the route of administration. Specifically, local administration of Dermorphine into the renal artery induced diuresis, whereas intracerebroventricular (icv.) administration resulted in antidiuresis (Kapusta et al., 1991; 1993). Further disparities were observed in experiments involving NOP receptor partial agonists. While icv. administration led to diuresis, intravenous administration did not produce a similar change in urinary flow (Kapusta et al., 2005). These results imply that the site of OR activation—central or peripheral—can distinctly modulate renal physiology.
4.1.1 Where’s opioids’ central hub for kidney
Emerging evidence has begun to shed light on the specific brain regions that may play a crucial role in mediating the renal effects of opioids. The hypothalamus, particularly the paraventricular nucleus (PVN), has been identified as a critical region influencing RSNA (Katsurada et al., 2021). The PVN integrates neural and hormonal signals to regulate kidney function, with various neuropeptides and neurotransmitters modulating RSNA. Opioid receptors, such as KOR and MOR, are widely distributed in the hypothalamus, including the PVN (Gottlieb et al., 2005; Zhang et al., 2019). These receptors are involved in pain modulation and autonomic regulation, including sympathetic outflow (Zhang et al., 2019). The presence of KORs in the PVN raises the question of how opioids might regulate renal function through central mechanisms. A study by Gottlieb et al. (2005) provides valuable insights into this question. They demonstrated that microinjection of the KOR agonist U-50488H into specific subregions of the PVN produced differential cardiovascular and renal responses in anesthetized rats (Gottlieb et al., 2005). This finding suggests that KORs in the PVN are involved in the central regulation of renal function, possibly through modulation of RSNA. Undoubtedly, more detailed and in-depth research is eagerly needed to elucidate the brain regions and neural circuits involved in the central mechanisms by which opioids influence renal function. Future studies should focus on exploring the distribution of opioid receptors in the hypothalamus and other brain regions, their signaling pathways, and the regulatory mechanisms of renal sympathetic nerve activity.
4.1.2 The crosstalk between OR and adrenergic receptors in the periphery
Opioids’ peripheral actions add further complexity through their interactions with other receptor systems, such as adrenergic receptors. Extensive evidence demonstrates functional interactions between the opioid and adrenergic receptor systems. This crosstalk involves second messenger systems, the formation of receptor heterodimers, and the presence of allosteric binding regions (Root-Bernstein and Churchill, 2021).
Several studies have shed light on the molecular mechanisms underlying opioid-adrenergic receptor interactions. For instance, Pepe et al. (1997) demonstrated that stimulation of δ-OR inhibits β1-adrenergic receptor (β1-AR)-induced positive inotropic effects and cAMP increases in rat heart (Pepe et al., 1997). This inhibitory crosstalk was found to be mediated by a pertussis toxin-sensitive G (i/o) protein involved in adenylyl cyclase inhibition. Similarly, Xiao et al. (1997) showed that the δ-OR agonist leucine-enkephalin markedly inhibited β1-AR-stimulated cAMP production and contractility in isolated rat heart (Xiao et al., 1997).
In the context of cardiovascular function and hypertension, Yu et al. (1999) investigated the crosstalk between cardiac κ-ORs and β-AR in developing hypertensive rats (Yu et al., 1999). They found that the inhibitory effect of κ-OR stimulation on β-adrenergic signaling was attenuated in the ventricular myocytes of 13-week-old spontaneously hypertensive rats with established hypertension, but not in younger rats before hypertension was fully developed, indicating that the blunted crosstalk between κ-ORs and β-ARs may contribute to the maintenance but not the initiation of hypertension in this model.
Given the presence of both opioid and adrenergic receptors in the kidney, it is plausible that similar interactions may occur in this organ, potentially influencing renal function and blood pressure regulation. The kidney plays a crucial role in maintaining fluid and electrolyte balance, as well as regulating blood pressure through various mechanisms, including the renin-angiotensin-aldosterone system and sympathetic nervous system. Opioid-adrenergic receptor crosstalk in the kidney may modulate these processes, thereby contributing to the development or maintenance of hypertension.
4.1.3 Future directions
Despite these insights, there remains a gap in understanding how these differing receptor sites might influence the progression of renal diseases, such as IRI or renal functional failure. Most opioids, capable of freely crossing the BBB, could produce mixed and complex effects in physiological and pathological states, unlike the more controlled experimental conditions. Future advancements in this field may depend on the development of new pharmaceutical agents, designed to be impermeable to the BBB, such as Loperamide (Proskuryakova et al., 2009). Such drugs could offer more precise insights into the peripheral versus central actions of opioids on renal function, thereby enhancing our understanding of opioid-related renal pathophysiology.
4.2 Study design issues
Secondly, it is imperative to consider the impact of study design, particularly the distinction between clinical studies and animal experiments, on the outcomes of opioid-related renal research. Variations in experimental conditions, such as the choice of model organism and the duration of opioid exposure, are critical factors that likely contribute to the divergent findings observed in the literature.
Clinical studies, in particular, have tended to report deleterious effects of opioids on renal function (Barbosa-Leiker et al., 2016; Novick et al., 2016; Babak et al., 2017; Abbott et al., 2018; Fassio et al., 2018; Dasari et al., 2021). Notably, these studies often involve participants who have been exposed to opioids over extended periods. Furthermore, the opioids used in these clinical contexts are typically a combination of various types, rather than a single, specific agent. This polypharmacy aspect could potentially confound the attribution of renal harm to any one opioid compound, despite the well-established evidence that long-term use of traditional opioids is linked to adverse renal outcomes.
In light of these considerations, future research might beneficially focus on novel OR agonists that demonstrate minimal side effects and exhibit high affinity for specific receptor subtypes. An encouraging trend in this direction is evident in the research surrounding Remifentanil (Vianna et al., 2009; Xiong et al., 2012; Terashi et al., 2013). Studies on this agent have consistently reported protective effects on renal function, suggesting a potentially safer profile compared to traditional opioids. Such findings provide a compelling rationale for further exploration into selective OR agonists as a means to mitigate the renal risks associated with opioid therapy.
4.3 Heterogeneity
Finally, individual genetic variations among subjects, which can affect receptor expression and opioid metabolism, might also contribute to the variability in response to opioid agonists.
These considerations underscore the need for a more nuanced approach to studying opioid effects on the kidney, taking into account the multifaceted nature of opioid-receptor interactions and the diverse physiological contexts in which they operate.
5 Conclusion
In conclusion, this review highlights the multifaceted impact of OR agonists on renal function, illustrating a complex interplay of protective and harmful effects. It underscores the necessity for a deeper understanding of the central and peripheral actions of these agonists and calls for standardized research methodologies. Such insights are crucial for advancing therapeutic strategies in managing renal pathologies, ultimately contributing to better patient outcomes in renal health and disease management.
Author contributions
SG: Conceptualization, Data curation, Formal Analysis, Funding acquisition, Investigation, Methodology, Project administration, Resources, Software, Supervision, Validation, Visualization, Writing–original draft, Writing–review and editing. QH: Writing–original draft, Writing–review and editing.
Funding
The authors declare that financial support was received for the research, authorship, and/or publication of this article. This research was funded by Guangdong Provincial Regional Joint Young Scientists Fund for Natural Sciences, grant number “2021A1515110580.”
Conflict of interest
The authors declare that the research was conducted in the absence of any commercial or financial relationships that could be construed as a potential conflict of interest.
Publisher’s note
All claims expressed in this article are solely those of the authors and do not necessarily represent those of their affiliated organizations, or those of the publisher, the editors and the reviewers. Any product that may be evaluated in this article, or claim that may be made by its manufacturer, is not guaranteed or endorsed by the publisher.
References
Abbott, K. C., Fwu, C.-W., Eggers, P. W., Eggers, A. W., Kline, P. P., and Kimmel, P. L. (2018). Opioid prescription, morbidity, and mortality in US transplant recipients. Transplantation 102, 994–1004. doi:10.1097/TP.0000000000002057
Abdullah, B. (2018). Histological and molecular studies of the effects of tramadol on brain, liver and kidney of adult rabbits. Iraqi J. Agric. Sci. 49, 1083–1089. doi:10.36103/ijas.v49i6.159
Arbit, B., Marston, N., Shah, K., Lee, E., Aramin, H., Clopton, P., et al. (2016). Prognostic usefulness of proenkephalin in stable ambulatory patients with heart failure. Am. J. Cardiol. 117 (8), 1310–1314. doi:10.1016/j.amjcard.2016.01.042
Ashton, N., Balment, R. J., and Blackburn, T. P. (1990). Kappa-opioid-receptor agonists modulate the renal excretion of water and electrolytes in anaesthetized rats. Br. J. Pharmacol. 99, 181–185. doi:10.1111/j.1476-5381.1990.tb14674.x
Atici, S., Cinel, I., Cinel, L., Doruk, N., Eskandari, G., and Oral, U. (2005). Liver and kidney toxicity in chronic use of opioids: an experimental long term treatment model. J. Biosci. 30, 245–252. doi:10.1007/BF02703705
Babak, K., Mohammad, A., Mazaher, G., Samaneh, A., and Fatemeh, T. (2017). Clinical and laboratory findings of rhabdomyolysis in opioid overdose patients in the intensive care unit of a poisoning center in 2014 in Iran. Epidemiol. Health 39, e2017050. doi:10.4178/epih.e2017050
Bądzyńska, B., Lipkowski, A. W., and Sadowski, J. (2016). An antihypertensive opioid: Biphalin, a synthetic non-addictive enkephalin analog decreases blood pressure in spontaneously hypertensive rats. Pharmacol. Rep. 68, 51–55. doi:10.1016/j.pharep.2015.06.006
Barbosa-Leiker, C., McPherson, S., Daratha, K., Alicic, R., Short, R., Dieter, B., et al. (2016). Association between prescription opioid use and biomarkers of kidney disease in US adults. KIDNEY & BLOOD Press. Res. 41, 365–373. doi:10.1159/000443436
Bartok, R. E., and Craft, R. M. (1997). Sex differences in opioid antinociception. J. Pharmacol. Exp. Ther. 282, 769–778.
Bellini, L., Vadori, M., De Benedictis, G. M., and Busetto, R. (2016). Effects of opioids on proximal renal tubular cells undergoing ATP depletion. J. Pharmacol. Sci. 131, 288–291. doi:10.1016/j.jphs.2016.08.002
Buhari, S., Kalthum, H., Goh, Y., Noordin, M., Chen, H., and Gan, S. (2012). Effects of tramadol on liver and renal biochemistry and histopathology in dogs undergoing surgery under pentobarbitone anesthesia. J. ANIMAL VETERINARY Adv. 11, 1188–1194. doi:10.3923/javaa.2012.1188.1194
Cai, X., Huang, H., Kuzirian, M. S., Snyder, L. M., Matsushita, M., Lee, M. C., et al. (2016). Generation of a KOR-Cre knockin mouse strain to study cells involved in kappa opioid signaling. GENESIS 54, 29–37. doi:10.1002/dvg.22910
Calo, G., Guerrini, R., Rizzi, A., Salvadori, S., and Regoli, D. (2000). Pharmacology of nociceptin and its receptor: a novel therapeutic target. Br. J. Pharmacol. 129, 1261–1283. doi:10.1038/sj.bjp.0703219
Chatterjee, P. K. (2007). Novel pharmacological approaches to the treatment of renal ischemia-reperfusion injury: a comprehensive review. Naunyn Schmiedeb. Arch. Pharmacol. 376, 1–43. doi:10.1007/s00210-007-0183-5
Chen, J. C., Smith, E. R., Cahill, M., Cohen, R., and Fishman, J. B. (1993). The opioid receptor binding of dezocine, morphine, fentanyl, butorphanol and nalbuphine. Life Sci. 52, 389–396. doi:10.1016/0024-3205(93)90152-s
Cox, B. M. (1982). Endogenous opioid peptides: a guide to structures and terminology. Life Sci. 31, 1645–1658. doi:10.1016/0024-3205(82)90179-5
Craft, R. M. (2003). Sex differences in opioid analgesia: “from mouse to man.”. Clin. J. Pain 19, 175–186. doi:10.1097/00002508-200305000-00005
Dasari, T., Shah, R., Spinner, J., and Walther, C. (2021). Abstract 27: opioid use strongly increases incidence of acute kidney injury in patients with acute ischemic stroke: an analysis of 3.4 million admissions. STROKE 52. doi:10.1161/str.52.suppl_1.27
Davis, M. P., Pasternak, G., and Behm, B. (2018). Treating chronic pain: an overview of clinical studies centered on the buprenorphine option. Drugs 78, 1211–1228. doi:10.1007/s40265-018-0953-z
Deng, J., St Clair, M., Everett, C., Reitman, M., and Star, R. A. (2000). Buprenorphine given after surgery does not alter renal ischemia/reperfusion injury. Comp. Med. 50, 628–632.
de Oliveira, R., Brito, M., Ribeiro, R., Oliveira, L., Monteiro, A., Brandao, F., et al. (2017). Influence of remote ischemic conditioning and tramadol hydrochloride on oxidative stress in kidney ischemia/reperfusion injury in rats. ACTA CIR. BRAS. 32, 229–235. doi:10.1590/S0102-865020170030000007
Dibona, G., and Jones, S. (1994). Role of endogenous peripheral opioid mechanisms in renal function. J. Am. Soc. Nephrol. JASN 4, 1792–1797. doi:10.1681/ASN.V4101792
Elnagar, G., Elseweidy, M., Mahmoud, Y., Elkomy, N., Althafar, Z., Alnomasy, S., et al. (2022). 10-Dehydrogingerdione attenuates tramadol-induced nephrotoxicity by modulating renal oxidative stress, inflammation and apoptosis in experimental rats: role of HO-1 activation and TLR4/NF-kappa B/ERK inhibition. Int. J. Mol. Sci. 23, 1384. doi:10.3390/ijms23031384
Fassio, V., Aspinall, S. L., Zhao, X., Miller, D. R., Singh, J. A., Good, C. B., et al. (2018). Trends in opioid and nonsteroidal anti-inflammatory use and adverse events. Am. J. Manag. Care 24, e61–e72.
Fillingim, R. B., and Gear, R. W. (2004). Sex differences in opioid analgesia: clinical and experimental findings. Eur. J. Pain 8, 413–425. doi:10.1016/j.ejpain.2004.01.007
Flores, O., Camera, L. A., Hergueta, A., Gallego, B., Pérez Barriocanal, F., Gutkowska, J., et al. (1997). Role of atrial natriuretic factor, hemodynamic changes and renal nerves in the renal effects of intraperitoneal morphine in conscious rats. Kidney Blood Press Res. 20, 18–24. doi:10.1159/000174106
Franco-Acevedo, A., Echavarria, R., Moreno-Carranza, B., Ortiz, C., Garcia, D., Gonzalez-Gonzalez, R., et al. (2020). Opioid preconditioning modulates repair responses to prevent renal ischemia-reperfusion injury. PHARMACEUTICALS 13, 387. doi:10.3390/ph13110387
Gottlieb, H. B., Varner, K. J., Kenigs, V. A., Cabral, A. M., and Kapusta, D. R. (2005). Differential cardiovascular and renal responses produced by microinjection of the κ-opioid U-50488H [(trans -3,4-Dichloro- N -methyl- N -[2-(1-pyrrolidinyl)cyclohexyl]-benzene-acetamide) methane sulfonate] into subregions of the paraventricular nucleus. J. Pharmacol. Exp. Ther. 312, 678–685. doi:10.1124/jpet.104.076828
Gutkowska, J., Jankowski, M., Pawlak, D., Mukaddam-Daher, S., and Izdebski, J. (2004). The cardiovascular and renal effects of a highly potent mu-opioid receptor agonist, cyclo[N-epsilon,N-beta-carbonyl-D-Lys(2),Dap (5)]enkephalinamide. Eur. J. Pharmacol. 496, 167–174. doi:10.1016/j.ejphar.2004.06.007
Gutkowska, J., and Schiller, P. W. (1996). Renal effects of TAPP, a highly selective mu-opioid agonist. Br. J. Pharmacol. 119, 239–244. doi:10.1111/j.1476-5381.1996.tb15977.x
Habibey, R., Ajami, M., Ebrahimi, S., Hesami, A., Babakoohi, S., and Pazoki-Toroudi, H. (2010). Nitric oxide and renal protection in morphine-dependent rats. FREE Radic. Biol. Med. 49, 1109–1118. doi:10.1016/j.freeradbiomed.2010.06.024
Habibey, R., and Pazoki-Toroudi, H. (2008). Morphine dependence protects rat kidney against ischaemia-reperfusion injury. Clin. Exp. Pharmacol. PHYSIOLOGY 35, 1209–1214. doi:10.1111/j.1440-1681.2008.04986.x
Haddox, M. K., and Russell, D. H. (1979). beta-Endorphin is a kidney trophic hormone. Life Sci. 25, 615–620. doi:10.1016/0024-3205(79)90556-3
He, X., Sandhu, H. K., Yang, Y., Hua, F., Belser, N., Kim, D. H., et al. (2013). Neuroprotection against hypoxia/ischemia: delta-opioid receptor-mediated cellular/molecular events. Cell. Mol. LIFE Sci. 70, 2291–2303. doi:10.1007/s00018-012-1167-2
Huhn, A. S., Berry, M. S., and Dunn, K. E. (2018). Systematic review of sex-based differences in opioid-based effects. Int. Rev. Psychiatry 30, 107–116. doi:10.1080/09540261.2018.1514295
Imai, E., Horio, M., Watanabe, T., Iseki, K., Yamagata, K., Hara, S., et al. (2009). Prevalence of chronic kidney disease in the Japanese general population. Clin. Exp. Nephrol. 13, 621–630. doi:10.1007/s10157-009-0199-x
Jalili, C., Makalani, F., Roshankhah, S., Sohrabi, K., and Salahshoor, M. (2017). Protective effect of resveratrol against morphine damage to kidneys of mice. Int. J. Morphol. 35, 1409–1415. doi:10.4067/S0717-95022017000401409
Kapusta, D., Burmeister, M., Calo, G., Guerrini, R., Gottlieb, H., and Kenigs, V. (2005). Functional selectivity of nociceptin/orphanin FQ peptide receptor partial agonists on cardiovascular and renal function. J. Pharmacol. Exp. Ther. 314, 643–651. doi:10.1124/jpet.104.082768
Kapusta, D., Chang, J., and Kenigs, V. (1999). Central administration of [Phe(1)Psi(CH2-NH)Gly(2)]nociceptin(1-13)-NH2 and orphanin FQ/nociceptin (OFQ/N) produce similar cardiovascular and renal responses in conscious rats. J. Pharmacol. Exp. Ther. 289, 173–180.
Kapusta, D., Jones, S. Y., and Dibona, G. (1989). Opioids in the systemic hemodynamic and renal responses to stress in spontaneously hypertensive rats. Hypertension 13, 808–816. doi:10.1161/01.hyp.13.6.808
Kapusta, D. R., Jones, S. Y., and DiBona, G. F. (1991). Renal mu opioid receptor mechanisms in regulation of renal function in rats. J. Pharmacol. Exp. Ther. 258, 111–117.
Kapusta, D. R., and Obih, J. C. (1993). Central kappa opioid receptor-evoked changes in renal function in conscious rats: participation of renal nerves. J. Pharmacol. Exp. Ther. 267, 197–204.
Kapusta, D. R., Obih, J. C., and Dibona, G. F. (1993). Central mu opioid receptor-mediated changes in renal function in conscious rats. J. Pharmacol. Exp. Ther. 265, 134–143.
Kapusta, D. R., Sezen, S. F., Chang, J. K., Lippton, H., and Kenigs, V. A. (1997). Diuretic and antinatriuretic responses produced by the endogenous opioid-like peptide, nociceptin (orphanin FQ). Life Sci. 60, PL15–21. doi:10.1016/s0024-3205(96)00593-0
Katsurada, K., Ogoyama, Y., Imai, Y., Patel, K. P., and Kario, K. (2021). Renal denervation based on experimental rationale. Hypertens. Res. 44, 1385–1394. doi:10.1038/s41440-021-00746-7
Khorashadi, M., Beunders, R., Pickkers, P., and Legrand, M. (2020). Proenkephalin: a new biomarker for glomerular filtration rate and acute kidney injury. Nephron 144, 655–661. doi:10.1159/000509352
Kizilkaya, M., Dogan, N., Kursad, H., Erdogan, F., and Yildirim, O. (2002). in The renal effects of tramadol after isoflurane anesthesia. Editor G. Varrassi, 251–255.
Kramer, H. J., Uhl, W., Ladstetter, B., and Bäcker, A. (2000). Influence of asimadoline, a new kappa-opioid receptor agonist, on tubular water absorption and vasopressin secretion in man. Br. J. Clin. Pharmacol. 50, 227–235. doi:10.1046/j.1365-2125.2000.00256.x
Lan, X., Rai, P., Chandel, N., Cheng, K., Lederman, R., Saleem, M. A., et al. (2013). Morphine induces albuminuria by compromising podocyte integrity. PLoS One 8, e55748. doi:10.1371/journal.pone.0055748
Lee, H., and Emala, C. (2001). Protein kinase C and G(i/o) proteins are involved in adenosine- and ischemic preconditioning-mediated renal protection. J. Am. Soc. Nephrol. 12, 233–240. doi:10.1681/ASN.V122233
Lefebvre, R. A. (1986). “Opioid peptides and their receptors,” in Comparative veterinary pharmacology, toxicology and theraphy: proceedings of the 3rd congress of the European association for veterinary pharmacology and toxicology, august 25–29 1985, ghent, Belgium Part II, invited lectures. Editors A. S. J. P. A. M. Van Miert, M. G. Bogaert, and M. Debackere (Dordrecht: Springer Netherlands), 447–453. doi:10.1007/978-94-009-4153-3_42
Lian-Jin, Q., Wen-Ming, F., Ying, B., Mao-Yun, F., Yao, W., and Cheng-Wu, T. (2010). Effects of delta-opioid receptor agonist DADLE on renal function in rats with sepsis. Chin. J. Emerg. Med. 19, 140–144. doi:10.3760/cma.j.issn.1671-0282.2010.02.008
Linner, K. M., Stickney, B. J., Quist, H. E., Sharp, B. M., and Portoghese, P. S. (1998). The delta1-opioid receptor antagonist, 7-(benzospiroindanyl)naltrexone [correction of 7-benzylspiroindanylnaltrexone], prolongs renal allograft survival in a rat model. Eur. J. Pharmacol. 354, R3–R5. doi:10.1016/S0014-2999(98)00477-4
Liu, L., Yu, J., and Xu, X. (2018). Kappa-opioid receptor agonist U50448H protects against renal ischemia-reperfusion injury in rats via activating the PI3K/Akt signaling pathway. ACTA Pharmacol. SIN. 39, 97–106. doi:10.1038/aps.2017.51
Lukowiak, M., Kosson, P., Hennink, W., and Lipkowski, A. (2009). Synthesis and pharmacological properties of a new fluorescent opioid peptide analog. Pharmacol. Rep. P. R. 61 (4), 727–731. doi:10.1016/S1734-1140(09)70126-4
Luo, F., Xu, R., Song, G., Lu, H., He, X., and Ying, X. (2020). The delta-opioid receptor differentially regulates MAPKs and anti-inflammatory cytokines in rat kidney epithelial cells under hypoxia. Front. PHYSIOLOGY 10, 1572. doi:10.3389/fphys.2019.01572
Luo, F., Zhao, L., Deng, J., Liang, M., Zeng, X., Liu, H., et al. (2013). Geranylgeranylacetone protects against morphine-induced hepatic and renal damage in mice. Mol. Med. Rep. 7, 694–700. doi:10.3892/mmr.2012.1217
Mallappallil, M., Bajracharya, S., Salifu, M., and Yap, E. (2021). Opioids and acute kidney injury. SEMINARS Nephrol. 41, 11–18. doi:10.1016/j.semnephrol.2021.02.002
Mansour, A., Khachaturian, H., Lewis, M. E., Akil, H., and Watson, S. J. (1987). Autoradiographic differentiation of mu, delta, and kappa opioid receptors in the rat forebrain and midbrain. J. Neurosci. 7, 2445–2464.
Maslov, L. N., Khaliulin, I., Oeltgen, P. R., Naryzhnaya, N. V., Pei, J.-M., Brown, S. A., et al. (2016). Prospects for creation of cardioprotective and antiarrhythmic drugs based on opioid receptor agonists. Med. Res. Rev. 36, 871–923. doi:10.1002/med.21395
Matsue, Y., Maaten, J. M., Struck, J., Metra, M., O’connor, C., Ponikowski, P., et al. (2017). Clinical correlates and prognostic value of proenkephalin in acute and chronic heart failure. J. cardiac Fail. 23 (3), 231–239. doi:10.1016/j.cardfail.2016.09.007
Mercadante, S., and Arcuri, E. (2004). Opioids and renal function. J. PAIN 5, 2–19. doi:10.1016/j.jpain.2003.09.007
Meunier, J.-C., Mollereau, C., Toll, L., Suaudeau, C., Moisand, C., Alvinerie, P., et al. (1995). Isolation and structure of the endogenous agonist of opioid receptor-like ORL1 receptor. Nature 377, 532–535. doi:10.1038/377532a0
Millan, M. J. (1986). Multiple opioid systems and pain. Pain 27, 303–347. doi:10.1016/0304-3959(86)90158-2
Moreau, R., Cailmail, S., Hamon, G., and Lebrec, D. (1996). Renal and haemodynamic responses to a novel kappa opioid receptor agonist, niravoline (RU 51,599), in rats with cirrhosis. J. Gastroenterology Hepatology 11, 857–863. doi:10.1111/j.1440-1746.1996.tb00093.x
Mutoh, J., Ohsawa, M., and Hisa, H. (2013). Effect of naloxone on ischemic acute kidney injury in the mouse. Neuropharmacology 71, 10–18. doi:10.1016/j.neuropharm.2013.03.001
Ng, L., Sandhu, J., Narayan, H., Quinn, P., Squire, I., Davies, J., et al. (2014). Proenkephalin and prognosis after acute myocardial infarction. J. Am. Coll. Cardiol. 63 (3), 280–289. doi:10.1016/j.jacc.2013.09.037
Ng, L., Squire, I., Jones, D. J. L., Cao, T., Chan, D., Sandhu, J., et al. (2017). Proenkephalin, renal dysfunction, and prognosis in patients with acute heart failure: a great network study. J. Am. Coll. Cardiol. 69 (1), 56–69. doi:10.1016/j.jacc.2016.10.038
Novick, T., Liu, Y., Alvanzo, A., Zonderman, A., Evans, M., and Crews, D. (2016). Lifetime cocaine and opiate use and chronic kidney disease. Am. J. Nephrol. 44, 447–453. doi:10.1159/000452348
Novick, T., Surapaneni, A., Ballew, S., Shin, J., Alexander, C., Inker, L., et al. (2019). Abstract MP12: the risk of adverse outcomes associated with opioid prescriptions in people with chronic kidney disease. CIRCULATION 139. doi:10.1161/circ.139.suppl_1.MP12
Ono, Y., Sakamoto, M., Makino, K., Tayama, K., Tada, Y., Nakagawa, Y., et al. (2023). Hepatic and renal toxicities and metabolism of fentanyl analogues in rats. NAUNYN-SCHMIEDEBERGS ARCHIVES Pharmacol. 396, 149–159. doi:10.1007/s00210-022-02301-8
Osinski, M. A., Pampusch, M. S., Murtaugh, M. P., and Brown, D. R. (1999). Cloning, expression and functional role of a nociceptin/orphanin FQ receptor in the porcine gastrointestinal tract. Eur. J. Pharmacol. 365, 281–289. doi:10.1016/s0014-2999(98)00869-3
Palomino, J., Echavarria, R., Franco-Acevedo, A., Moreno-Carranza, B., and Melo, Z. (2019). Opioids preconditioning upon renal function and ischemia-reperfusion injury: a narrative review. MEDICINA-LITHUANIA 55, 522. doi:10.3390/medicina55090522
Pazoki-Toroudi, H., Ajami, M., and Habibey, R. (2010). Pre-medication and renal pre-conditioning: a role for alprazolam, atropine, morphine and promethazine. Fundam. Clin. Pharmacol. 24, 189–198. doi:10.1111/j.1472-8206.2009.00743.x
Peng, J., Sarkar, S., and Chang, S. (2012). Opioid receptor expression in human brain and peripheral tissues using absolute quantitative real-time RT-PCR. DRUG ALCOHOL DEPENDENCE 124, 223–228. doi:10.1016/j.drugalcdep.2012.01.013
Pepe, S., Xiao, R., Hohl, C., Altschuld, R., and Lakatta, E. (1997). “Cross talk” between opioid peptide and adrenergic receptor signaling in isolated rat heart. Circulation 95 (8), 2122–2129. doi:10.1161/01.CIR.95.8.2122
Pisanu, C., Franconi, F., Gessa, G. L., Mameli, S., Pisanu, G. M., Campesi, I., et al. (2019). Sex differences in the response to opioids for pain relief: a systematic review and meta-analysis. Pharmacol. Res. 148, 104447. doi:10.1016/j.phrs.2019.104447
Proskuryakova, T., Shokhonova, V., Chumakova, Y. A., Bashkatova, V., and Sudakov, S. (2009). Modulation of peripheral opioid receptors affects the concentration of μ-opioid receptors in rat brain. Bull. Exp. Biol. Med. 148, 357–359. doi:10.1007/s10517-010-0711-z
Quirion, R., Finkel, M. S., Mendelsohn, F. A., and Zamir, N. (1983). Localization of opiate binding sites in kidney and adrenal gland of the rat. Life Sci. 33 (Suppl. 1), 299–302. doi:10.1016/0024-3205(83)90502-7
Raffa, R. B., Friderichs, E., Reimann, W., Shank, R. P., Codd, E. E., and Vaught, J. L. (1992). Opioid and nonopioid components independently contribute to the mechanism of action of tramadol, an “atypical” opioid analgesic. J. Pharmacol. Exp. Ther. 260, 275–285.
Ricarte, A., Dalton, J. A. R., and Giraldo, J. (2021). Structural assessment of agonist efficacy in the μ-opioid receptor: morphine and fentanyl elicit different activation patterns. J. Chem. Inf. Model. 61, 1251–1274. doi:10.1021/acs.jcim.0c00890
Root-Bernstein, R., and Churchill, B. (2021). Co-evolution of opioid and adrenergic ligands and receptors: shared, complementary modules explain evolution of functional interactions and suggest novel engineering possibilities. Life 11, 1217. doi:10.3390/life11111217
Schmitt, S., Delamare, J., Tirel, O., Fillesoye, F., Dhilly, M., and Perrio, C. (2017). N-[18F]-FluoropropylJDTic for κ-opioid receptor PET imaging: radiosynthesis, pre-clinical evaluation, and metabolic investigation in comparison with parent JDTic. Nucl. Med. Biol. 44, 50–61. doi:10.1016/j.nucmedbio.2016.09.005
Schulz, C.-A., Christensson, A., Ericson, U., Almgren, P., Hindy, G., Nilsson, P., et al. (2017). High level of fasting plasma proenkephalin-A predicts deterioration of kidney function and incidence of CKD. J. Am. Soc. Nephrol. JASN 28 (1), 291–303. doi:10.1681/ASN.2015101177
Sen, E., Kaplan, D., Bozdag, Z., Orkmez, M., Mizrak, A., Sen, H., et al. (2020). The effect of tramadol on oxidative stress total antioxidant levels in rats with renal ischemia-reperfusion injury. TURKISH J. UROLOGY 46, 388–392. doi:10.5152/tud.2020.20227
Singhal, P. C., Sharma, P., Gibbons, N., Franki, N., Kapasi, A., and Wagner, J. D. (1997). Effect of morphine on renomedullary interstitial cell proliferation and matrix accumulation. Nephron 77, 225–234. doi:10.1159/000190277
Slizgi, G. R., and Ludens, J. H. (1985). Displacement of 3H-EKC binding by opioids in rat kidney: a correlate to diuretic activity. Life Sci. 36, 2189–2193. doi:10.1016/0024-3205(85)90328-5
Star, R. A. (1998). Treatment of acute renal failure. Kidney Int. 54, 1817–1831. doi:10.1046/j.1523-1755.1998.00210.x
Takhtfooladi, M., Shahzamani, M., Asghari, A., and Fakouri, A. (2016). Naloxone pretreatment prevents kidney injury after liver ischemia reperfusion injury. Int. UROLOGY Nephrol. 48, 1113–1120. doi:10.1007/s11255-016-1280-5
Terashi, T., Takehara, A., Kuniyoshi, T., Matsunaga, A., Kawasaki, K., and Kanmura, Y. (2013). Remifentanil temporarily improves renal function in adult patients with chronic kidney disease undergoing orthopedic surgery. J. Anesth. 27, 340–345. doi:10.1007/s00540-012-1545-9
Vianna, P., Castiglia, Y., Braz, J., Viero, R., Beier, S., Vianna, P., et al. (2009). Remifentanil, isoflurane, and preconditioning attenuate renal ischemia/reperfusion injury in rats. Transplant. Proc. 41, 4080–4082. doi:10.1016/j.transproceed.2009.09.078
Weber, M., Chen, C., Li, Y., Farooqui, M., Nguyen, J., Poonawala, T., et al. (2013). Morphine stimulates platelet-derived growth factor receptor-β signalling in mesangial cells in vitro and transgenic sickle mouse kidney in vivo. Br. J. Anaesth. 111, 1004–1012. doi:10.1093/bja/aet221
Weber, M. L., Farooqui, M., Nguyen, J., Ansonoff, M., Pintar, J. E., Hebbel, R. P., et al. (2008). Morphine induces mesangial cell proliferation and glomerulopathy via kappa-opioid receptors. Am. J. Physiol. Ren. Physiol. 294, F1388–F1397. doi:10.1152/ajprenal.00389.2007
Wever, K. E., Masereeuw, R., Wagener, F. A., Verweij, V. G. M., Peters, J. G. P., Pertijs, J. C. L. M., et al. (2013). Humoral signalling compounds in remote ischaemic preconditioning of the kidney, a role for the opioid receptor. Nephrol. Dial. Transplant. 28, 1721–1732. doi:10.1093/ndt/gfs601
Wittert, G., Hope, P., and Pyle, D. (1996). Tissue distribution of opioid receptor gene expression in the rat. Biochem. Biophys. Res. Commun. 218, 877–881. doi:10.1006/bbrc.1996.0156
Wu, H., Chen, G., Wyburn, K. R., Yin, J., Bertolino, P., Eris, J. M., et al. (2007). TLR4 activation mediates kidney ischemia/reperfusion injury. J. Clin. Invest. 117, 2847–2859. doi:10.1172/JCI31008
Xiao, R.-P., Pepe, S., Spurgeon, H., Capogrossi, M. C., and Lakatta, E. (1997). Opioid peptide receptor stimulation reverses beta-adrenergic effects in rat heart cells. Am. J. physiology 272 (2 Pt 2), H797–H805. doi:10.1152/AJPHEART.1997.272.2.H797
Xiong, Y., Jin, X., Ye, M., Yanxia, L. U., and Xiuli, W. (2012). Role of opioid receptors in remifentanil-induced attenuation of renal ischemia/reperfusion injury in rats. Chin. J. Anesthesiol. 32, 877–879.
Yoo, S., Nam, K., and Kim, W. (2018). Association between remifentanil and acute kidney injury. J. Anesth. 32, 306. doi:10.1007/s00540-018-2450-7
Yu, X., Wang, H., Zhang, W., and Wong, T. M. (1999). Cross-talk between cardiac kappa-opioid and beta-adrenergic receptors in developing hypertensive rats. J. Mol. Cell. Cardiol. 31, 597–605. doi:10.1006/jmcc.1998.0896
Keywords: opioid receptor, kidney, ischemia-reperfusion injury, renal function, acute renal failure, chronic kidney disease
Citation: Gao S and He Q (2024) Opioids and the kidney: two sides of the same coin. Front. Pharmacol. 15:1421248. doi: 10.3389/fphar.2024.1421248
Received: 22 April 2024; Accepted: 03 July 2024;
Published: 25 July 2024.
Edited by:
Edgar Jaimes, Memorial Sloan Kettering Cancer Center, United StatesReviewed by:
Ewa Krystyna Szczepanska-Sadowska, Medical University of Warsaw, PolandAnalia S. Loria, University Press of Kentucky, United States
Copyright © 2024 Gao and He. This is an open-access article distributed under the terms of the Creative Commons Attribution License (CC BY). The use, distribution or reproduction in other forums is permitted, provided the original author(s) and the copyright owner(s) are credited and that the original publication in this journal is cited, in accordance with accepted academic practice. No use, distribution or reproduction is permitted which does not comply with these terms.
*Correspondence: Shaowei Gao, Z2Fvc2hhb3dAbWFpbDMuc3lzdS5lZHUuY24=