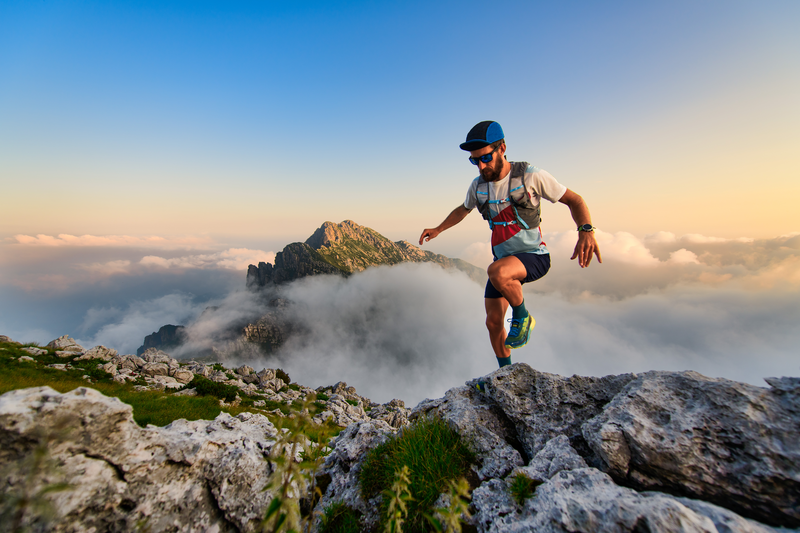
95% of researchers rate our articles as excellent or good
Learn more about the work of our research integrity team to safeguard the quality of each article we publish.
Find out more
ORIGINAL RESEARCH article
Front. Pharmacol. , 23 August 2024
Sec. Drugs Outcomes Research and Policies
Volume 15 - 2024 | https://doi.org/10.3389/fphar.2024.1419196
Objective: Using the FDA adverse event reporting system (FAERS) database to analyze the safety profile of Dexmedetomidine and provide guidance for clinical application.
Methods: Data from the FAERS database from the first quarter of 2004 to the third quarter of 2023 were collected. Reporting odds ratio (ROR), the proportional reporting ratio (PRR), and the Bayesian confidence propagation neural network (BCPNN) were employed to detect and assess adverse events associated with Dexmedetomidine.
Results: A total of 1910 reports of Dexmedetomidine as the primary suspect drug were obtained. After screening, 892 preferred terms were obtained, including 52 new preferred terms not mentioned in the drug insert. The common adverse events of Dexmedetomidine include bradycardia, cardiac arrest, hypotension, diabetes insipidus, arteriospasm coronary and agitation. Notably, cardiac disorders exhibited the highest number of reports and the highest signal intensity in the system organ class. Among the new preferred terms, those with high signal intensity include transcranial electrical motor evoked potential monitoring abnormal, acute motor axonal neuropathy, trigemino-cardiac reflex, glossoptosis, floppy iris syndrome, phaeochromocytoma crisis, postresuscitation encephalopathy and diabetes insipidus.
Conclusion: This study mined and evaluated adverse events associated with Dexmedetomidine and also identified new adverse events. This could help alert clinicians to new adverse events not mentioned in the drug inserts, reducing the risk of drug.
Dexmedetomidine is a sedative medication. It is a very specific agonist for
The FAERS database is composed of AEs for drugs submitted by consumers, pharmacists, healthcare industry practitioners, and others. It is an open, spontaneous reporting system that records AEs occurring after a drug has been marketed and used by a wide range of people. The aim of this study was to analyze AEs related to Dexmedetomidine in the FAERS database and provide guidance for its clinical application.
This study aims to assess the safety of Dexmedetomidine in real-world postmarketing setting. We collected adverse event reports with Dexmedetomidine as the primary suspect (PS) drug from the FAERS database, covering the period from the first quarter of 2004 to the third quarter of 2023. Data collection and cleaning were performed using R studio. The FAERS database generated 20,214,432 reports, and 32,502 duplicate reports were removed following FDA guidelines. Removal of duplicate reports refers to selecting the latest FDA_DT when CASEID and FDA_DT are the same in the DEMO table. Otherwise, choose the PRIMARYID with the greater value (Wang et al., 2023). All AEs in FAERS were categorized using preferred term (PT) and system organ class (SOC) using the Medical Dictionary for Regulatory Activities 20.0 (MedDRA 20.0) (Zhang et al., 2023). A total of 1913 adverse event reports associated with Dexmedetomidine were identified and 892 preferred terms (PTs) related to Dexmedetomidine were obtained in this study. Figure 1 illustrates the flowchart for selecting Dexmedetomidine-related AEs based on the FAERS database.
In our study, we used the reporting odds ratio (ROR), the proportional reporting ratio (PRR), and the Bayesian confidence propagation neural network (BCPNN) to assess the significant association of Dexmedetomidine with AEs (Sakaeda et al., 2013). The ROR and PRR algorithms are frequentist (non-Bayesian) algorithms. Non-Bayesian algorithms generally exhibit high sensitivity but relatively low specificity. The possibility of false positives increases, especially when the number of AEs is low. The BCPNN algorithms are Bayesian algorithms, known for their stability and higher specificity, especially in situations with a small reporting volume, which could reduce the likelihood of false positives (Zou et al., 2023). In this study, the ROR algorithm, PRR algorithm, and BCPNN algorithm are combined to leverage the strengths of multiple algorithms and reduce the result bias that may arise from relying on a single algorithm. Before calculating these equations, the values of four variables (a, b, c, and d) need to be determined. Refer to a two-by-two contingency table (Table 1), where a represents the number of target AE to the target drug, b represents the number of other AEs to the target drug, c represents the number of target AE to other drugs, and d represents the number of other AEs to other drugs. The specific formulas for the three algorithms are provided below.
If
If
If
The time to event onset of Dexmedetomidine was calculated as the gap between EVENT_DT (date of onset of AEs) and START_DT (date of Dexmedetomidine use initiation). In addition, inaccurate dates, missing dates or input error reports were excluded. R software (version 4.3.2) and R Studio were utilized for data processing and statistical analysis.
Between the first quarter of 2004 and the third quarter of 2023, the FAERS database received a total of 20,214,432 reports. After deduplication, there were 1,913 cases in which Dexmedetomidine was the PS drug. Table 2 demonstrates the basic population characteristics with Dexmedetomidine-related AEs. The majority of patients were male (48.8%), with only 28.7% being female, while the gender of 22.4% of patients was unknown. The weight of the patients was predominantly distributed between 50 and 100 kg (17.1%). Regarding age, AEs were more prevalent among patients aged 18–6 years (31.2%) than in those over 65 years (19.2%). Most of the adverse event cases were submitted by Physician (30.8%), Health professional (25.3%), Other health-professional (24.2%), Pharmacist (14.4%) and only 2.3% of the cases were submitted by consumers.
Between the first quarter of 2004 and the third quarter of 2023, a total of 176 reports documenting information on the timing of AEs were collected. The median time to the onset of AEs was 2 days, with an interquartile range of 1–6 days. In Figure 2, it was found that the majority of Dexmedetomidine AEs occurred within 5 days (n = 125, 73%) after drug administration. AEs rarely occurred within the time intervals of 6–10 days, 11–15 days, 16–20 days, and >20 days. Among these, there were 16 cases reported in the 6–10 days, accounting for 9% of the total, and 10 cases each in the remaining three categories, each representing 6% of the total. Because Dexmedetomidine is administered intravenously, concentrating its effects over a short period of time and dissipating quickly upon discontinuation. This means that adverse reactions usually occur shortly after administration. It also emphasizes the significance of early detection of AEs in patients shortly after drug administration.
Figure 3 demonstrates the signal strength of Dexmedetomidine at the SOC level in FAERS database. AEs related to Dexmedetomidine were observed in 26 organ systems. Among them, Dexmedetomidine was most frequently reported in cardiac disorders (n = 984) and exhibited the strongest signal [ROR (95%CI) = 8.55 (7.97–9.16)]. Several other SOCs have also shown strong signals, including Endocrine disorders [n = 79, ROR (95%CI) = 6.33 (5.07–7.91)], Vascular disorders [n = 288, ROR (95%CI) = 2.67 (2.37–3.01)], Respiratory, thoracic and mediastinal disorders [n = 458, ROR (95%CI) = 1.97 (1.79–2.16)], Injury, poisoning and procedural complications [n = 776, ROR (95%CI) = 1.59 (1.47–1.71)], Investigations [n = 462, ROR (95%CI) = 1.48 (1.35–1.63)], Congenital, familial and genetic disorders [n = 20, ROR (95%CI) = 1.23 (0.79–1.91)] and Pregnancy, puerperium and perinatal conditions [n = 27, ROR (95%CI) = 1.19 (0.81–1.74)]. However, Congenital, familial and genetic disorders and Pregnancy, puerperium and perinatal conditions did not have statistical significance due to the lower limit of ROR (95%CI) < 1.
Figure 3. Signal strength of Dexmedetomidine at the system organ class (SOC) level in FAERS database. SOC, system organ class; ROR, reporting odds ratio.
Because cardiac disorders were reported most frequently and with the highest signal intensity in the SOC, we chose cardiac disorders as the outcome variable for subgroup analyses and further analyzed the effects of age and sex on Dexmedetomidine AEs (Table 3). According to the results of univariate and multivariate logistic regression analyses, age is an influential factor in the occurrence of cardiac disorders. For those aged 18–64 years (OR = 1.09, P = 0.510), the risk of cardiac disorders was slightly increased relative to those under 18 years of age, but not statistically significant. However, for those over 65 years of age (OR = 1.69, P < 0.001), the risk of cardiac disorders was significantly higher than for those under 18 years of age. Regarding gender, for men (OR = 1.22, P = 0.068), there was a slight increase in the risk of cardiac disorders relative to women, approaching a level of statistical significance that may be clinically significant but has not yet reached significance.
Our study employed three algorithms to analyze drug responses and assess whether they met various screening criteria. A total of 201 positive signals were detected (Supplementary Table S1). The signals not related to the AEs of the drug itself, such as product problems, social environment, off-label use, drug interaction, drug ineffective and overdose were excluded. The signals were ranked by case reports (Figure 4) and ROR (Figure 5) respectively, and the PTs within the top 30 rankings were selected for presentation. PTs seen to occur with higher frequency include Bradycardia, Cardiac Arrest, Hypotension, Diabetes Insipidus, Arteriospasm Coronary, Agitation, Hyperthermia, Tachycardia, Oxygen Saturation Decreased and Withdrawal Syndrome (Figure 4). As shown in Figure 5, some PTs with high ROR signals were observed, such as Transcranial Electrical Motor Evoked Potential Monitoring Abnormal, Acute Motor, Axonal Neuropathy, Trigemino-Cardiac Reflex, Glossoptosis, Central Sleep, Apnoea Syndrome, Floppy Iris Syndrome, Phaeochromocytoma Crisis, Upper Airway Obstruction, Postresuscitation Encephalopathy and Diabetes Insipidus.
Figure 4. The top 30 adverse events of Dexmedetomidine at the preferred terms level in FAERS database. PT, preferred term of the Medical Dictionary for Regulatory Activities; ROR, reporting odds ratio; *indicates new signals of Dexmedetomidine adverse events from FAERS Database.
Figure 5. The top 30 signal strength of AEs of Dexmedetomidine ranked by ROR at the preferred terms level in the FAERS database. PT, preferred term of the Medical Dictionary for Regulatory Activities; ROR, reporting odds ratio; *indicates new signals of Dexmedetomidine adverse events from FAERS Database.
In our study, 57 new AEs of Dexmedetomidine not mentioned in the drug insert were identified, and these new signs were ranked by ROR (Supplementary Table S2). Among these, the top 10 new AEs are illustrated in Figure 6, including Transcranial Electrical Motor Evoked Potential Monitoring Abnormal [ROR (95%CI) = 2,723.16 (1,010.65–7,337.50)], Acute Motor Axonal Neuropathy [ROR (95%CI) = 1,509.67 (775.35–2,939.47)], Trigemino-Cardiac Reflex [ROR (95%CI) = 1,204.39 (549.10–2,641.72)], Glossoptosis [ROR (95%CI) = 1,079.59 (671.73–1735.12)], Floppy Iris Syndrome [ROR (95%CI) = 623.90 (401.04–970.58)], Phaeochromocytoma Crisis [ROR (95%CI) = 524.05 (244.87–1,121.49)], Postresuscitation Encephalopathy [ROR (95%CI) = 498.28 (156.15–1,590.03)], Diabetes Insipidus [ROR (95%CI) = 389.00 (308.35–490.74)], Recurrence Of Neuromuscular Blockade [ROR (95%CI) =379.98 (155.45–928.79)] and Intestinal Pseudo-Obstruction [ROR (95%CI =347.47 (239.69–503.72)]. The new AEs data provides us with more comprehensive information, aiding in a deeper understanding of the safety profile and potential risks associated with Dexmedetomidine. By analyzing these data, we can assess a wide range of reactions that the drug may exhibit in real-world applications, offering crucial insights for clinicians and researchers.
Figure 6. The top 10 new AEs of Dexmedetomidine ranked by ROR at the preferred terms level in the FAERS database. PT, preferred term of the Medical Dictionary for Regulatory Activities; ROR, reporting odds ratio.
Dexmedetomidine acts as a highly selective agonist for
By analyzing the data, we found that the most common SOCs for Dexmedetomidine was cardiac disorders, which also had the strongest signal strength. And the next most common SOCs was vascular disorders. The most common AEs for cardiac disorders and vascular disorders are bradycardia and hypotension, respectively. The reason for this is that Dexmedetomidine, at high plasma concentrations, activates receptors in
Based on the data analysis, except from cardiac and vascular disorders, we also observed a significant correlation between Dexmedetomidine and respiratory, thoracic, and mediastinal disorders. Common AEs associated with respiratory, thoracic, and mediastinal disorders were respiratory depression, upper airway obstruction, respiratory failure, and hypoxia. While previous studies have suggested that Dexmedetomidine has minimal respiratory depression, our real-world data indicate that respiratory-related AEs associated with Dexmedetomidine are still relatively common. This observation may be attributed to the role of
Our study also found that the most common AEs at the PT level included bradycardia, cardiac arrest, hypotension, diabetes insipidus, arteriospasm coronary, agitation. AEs to be aware of include arteriospasm coronary, and most studies have now concluded that the application of Dexmedetomidine during cardiac surgery improves the prognosis of patients (Ji et al., 2013). However, studies have also revealed that Dexmedetomidine may result in a reduction in coronary artery diameter (Kundra et al., 2016). In addition, Sato et al. (2022) published a case report describing transient coronary artery spasm in a patient who received postoperative sedation with Dexmedetomidine. This phenomenon is thought to be related to the ability of Dexmedetomidine to activate
Based on our study data, we also observed AEs of drug withdrawal syndrome associated with Dexmedetomidine. The drug withdrawal syndrome of Dexmedetomidine is characterized by tension, agitation, headache, elevated blood pressure, and increased catecholamine concentrations following abrupt discontinuation of an infusion of Dexmedetomidine (Infusion time
In our study, several new signals were identified. Particularly, Transcranial Electrical Motor Evoked Potential Monitoring Abnormal exhibited the highest signal intensity. The underlying mechanism by which Dexmedetomidine induces this phenomenon may be similar to that of clonidine. It may act by suppressing electrical responses in specific spinal neurons, inhibiting the activity of adrenergic neurons in the locus coeruleus, and decreasing activity in serotonergic neurons in the dorsal raphe nucleus (Wolff et al., 2007; Calderón et al., 2018). In addition, Mahmoud et al. (2010) also found that Dexmedetomidine significantly attenuated the amplitude of transcranial electrical motor evoked potential at plasma concentrations range from 0.6 to 0.8 ng/mL during spinal surgery. Our study adds to the evidence on real-world use, but a more in-depth exploration of the specific mechanisms requires further investigation through additional studies.
Secondly, diabetes insipidus as the most common PT in new signals, Van Decar et al. (2022) found that Dexmedetomidine was significantly associated with perioperative diabetes insipidus. In dog and rat studies, it was shown that the mechanism is related to the reduction of central arginine vasopressin (AVP) release by Dexmedetomidine, as well as the reduction of renal response to AVP (Van Decar et al., 2022). However, the specific mechanism in humans is not clear, and further studies on Dexmedetomidine-induced diabetes insipidus in humans are still needed to elucidate the underlying mechanisms in the future.
An interesting finding is that we observed a significant association between Floppy Iris Syndrome and Dexmedetomidine. As of now, there are no reported cases in the literature of Dexmedetomidine causing Floppy Iris Syndrome. The pathogenesis of this condition remains incompletely understood at present, and it may be associated with the impact on the sympathetic nervous system by
Overall, based on our findings, Dexmedetomidine can cause bradycardia and hypotension and should therefore be avoided in patients with severe heart block or bradycardia. As well as in patients with severe hypotension or poorly controlled blood pressure, Dexmedetomidine should be used with caution to avoid exacerbating symptoms of hypotension. Although Dexmedetomidine is less inhibitory to the respiratory system, AEs associated with respiratory depression, upper airway obstruction, and hypoxemia have been observed. Therefore, respiratory function should be monitored when Dexmedetomidine is administered, especially in patients with respiratory disease. In addition, our study suggests that Dexmedetomidine may cause diabetes insipidus by a mechanism that may involve an effect on antidiuretic hormone release. Therefore, the water-electrolyte balance should be monitored when Dexmedetomidine is administered. When using Dexmedetomidine in clinical practice, we recommend that the dose be adjusted according to the patient’s age, condition, and drug interactions. In particular, lower doses should be used and gradually adjusted for elderly patients. And continuous monitoring of heart rate, blood pressure, and respiratory function is required during use for the timely detection and management of AEs. In addition, after prolonged use, the medication should be discontinued by gradually reducing the dose to minimize or avoid withdrawal syndrome. If withdrawal syndrome occurs, drugs such as clonidine may be considered to alleviate withdrawal symptoms.
Certainly, this study has several limitations. Firstly, the FAERS database is a spontaneous reporting system with a diverse range of reporting sources. Therefore, there may be omissions, delayed reporting, missing information and misreporting, which could impact the analysis of results. Secondly, we were unable to calculate the incidence of AEs due to the unavailability of the overall number of people on medication. Thirdly, the three calculations we employed (ROR, PRR, and BCPNN) can only reflect the statistical correlation between drugs and AEs, but cannot determine the causal relationship between drugs and AEs. Therefore, more research experiments are still needed to establish the relationship between them. In spite of these limitations, the FAERS database still provides a substantial volume of data samples and relevant information on drugs in real-world applications. This helps researchers and clinicians identify potential safety issues following the release of a medication on the market, thereby reducing the risk associated with clinical drug use.
In summary, this study analyzed the real-world safety of Dexmedetomidine through the FAERS database. The common AEs include bradycardia, cardiac arrest, hypotension, diabetes insipidus, arteriospasm coronary and agitation. In addition, this study identified 57 new signals not mentioned in the drug insert. Among them, transcranial electrical motor evoked potential monitoring abnormal, acute motor axonal neuropathy, trigemino-cardiac reflex, glossoptosis, floppy iris syndrome, phaeochromocytoma crisis, postresuscitation encephalopathy, and diabetes insipidus showed a significant correlation with Dexmedetomidine. Therefore, physicians should closely monitor these common AEs and new AEs with high signal intensity during clinical use.
The original contributions presented in the study are included in the article/Supplementary Material, further inquiries can be directed to the corresponding authors.
Ethical approval was not required for the study involving humans in accordance with the local legislation and institutional requirements. Written informed consent to participate in this study was not required from the participants or the participants’ legal guardians/next of kin in accordance with the national legislation and the institutional requirements.
YS: Data curation, Formal Analysis, Investigation, Methodology, Software, Validation, Visualization, Writing–original draft, Writing–review and editing. ZC: Conceptualization, Data curation, Methodology, Software, Validation, Writing–original draft, Writing–review and editing. QW: Data curation, Formal Analysis, Investigation, Software, Validation, Visualization, Writing–review and editing. JW: Conceptualization, Investigation, Project administration, Supervision, Validation, Writing–review and editing. XW: Conceptualization, Funding acquisition, Investigation, Methodology, Project administration, Resources, Software, Supervision, Validation, Writing–review and editing.
The author(s) declare that financial support was received for the research, authorship, and/or publication of this article. This research is funded by the Natural Science Foundation of Hunan Province (2024JJ5504 to XW).
The authors declare that the research was conducted in the absence of any commercial or financial relationships that could be construed as a potential conflict of interest.
All claims expressed in this article are solely those of the authors and do not necessarily represent those of their affiliated organizations, or those of the publisher, the editors and the reviewers. Any product that may be evaluated in this article, or claim that may be made by its manufacturer, is not guaranteed or endorsed by the publisher.
The Supplementary Material for this article can be found online at: https://www.frontiersin.org/articles/10.3389/fphar.2024.1419196/full#supplementary-material
Afonso, J., and Reis, F. (2012). Dexmedetomidine: current role in anesthesia and intensive care. Braz. J. Anesthesiol. 62, 118–133. doi:10.1016/S0034-7094(12)70110-1
Calderón, P., Deltenre, P., Stany, I., Kaleeta Maalu, J.-P., Stevens, M., Lamoureux, J., et al. (2018). Clonidine administration during intraoperative monitoring for pediatric scoliosis surgery: effects on central and peripheral motor responses. Neurophysiol. Clin. 48, 93–102. doi:10.1016/j.neucli.2017.11.001
Chrysostomou, C., and Schmitt, C. G. (2008). Dexmedetomidine: sedation, analgesia and beyond. Expert Opin. Drug Metabolism and Toxicol. 4, 619–627. doi:10.1517/17425255.4.5.619
Fowler, R. A., Sabur, N., Li, P., Juurlink, D. N., Pinto, R., Hladunewich, M. A., et al. (2007). Sex-and age-based differences in the delivery and outcomes of critical care. Can. Med. Assoc. J. 177, 1513–1519. doi:10.1503/cmaj.071112
Gerlach, A. T., Dasta, J. F., Steinberg, S., Martin, L. C., and Cook, C. H. (2009). A new dosing protocol reduces dexmedetomidine-associated hypotension in critically ill surgical patients. J. Crit. Care 24, 568–574. doi:10.1016/j.jcrc.2009.05.015
Grape, S., Kirkham, K. R., Frauenknecht, J., and Albrecht, E. (2019). Intra-operative analgesia with remifentanil vs. dexmedetomidine: a systematic review and meta-analysis with trial sequential analysis. Anaesthesia 74, 793–800. doi:10.1111/anae.14657
Heybati, K., Zhou, F., Ali, S., Deng, J., Mohananey, D., Villablanca, P., et al. (2022). Outcomes of dexmedetomidine versus propofol sedation in critically ill adults requiring mechanical ventilation: a systematic review and meta-analysis of randomised controlled trials. Br. J. Anaesth. 129, 515–526. doi:10.1016/j.bja.2022.06.020
Hsu, Y.-W., Cortinez, L. I., Robertson, K. M., Keifer, J. C., Sum-Ping, S. T., Moretti, E. W., et al. (2004). Dexmedetomidine pharmacodynamics: part I: crossover comparison of the respiratory effects of dexmedetomidine and remifentanil in healthy volunteers. Anesthesiology 101, 1066–1076. doi:10.1097/00000542-200411000-00005
Hung, T.-Y., Lin, Y.-C., Wang, Y.-L., and Lin, M.-C. (2023). Efficacy and safety of intravenous dexmedetomidine as an adjuvant to general anesthesia in gynecological surgeries: a systematic review and meta-analysis of randomized controlled trials. Taiwan. J. Obstetrics Gynecol. 62, 239–251. doi:10.1016/j.tjog.2022.11.010
Ji, F., Li, Z., Nguyen, H., Young, N., Shi, P., Fleming, N., et al. (2013). Perioperative dexmedetomidine improves outcomes of cardiac surgery. Circulation 127, 1576–1584. doi:10.1161/CIRCULATIONAHA.112.000936
Keating, G. M. (2015). Dexmedetomidine: a review of its use for sedation in the intensive care setting. Drugs 75, 1119–1130. doi:10.1007/s40265-015-0419-5
Knapp, T., DiLeonardo, O., Maul, T., Hochwald, A., Li, Z., Hossain, J., et al. (2024). Dexmedetomidine withdrawal syndrome in children in the PICU: systematic review and meta-analysis. Pediatr. Crit. Care Med. 25, 62–71. doi:10.1097/PCC.0000000000003376
Ko, K.-H., Jun, I.-J., Lee, S., Lim, Y., Yoo, B., and Kim, K.-M. (2015). Effective dose of dexmedetomidine to induce adequate sedation in elderly patients under spinal anesthesia. Korean J. Anesthesiol. 68, 575–580. doi:10.4097/kjae.2015.68.6.575
Kukoyi, A., Coker, S., Lewis, L., and Nierenberg, D. (2013). Two cases of acute dexmedetomidine withdrawal syndrome following prolonged infusion in the intensive care unit: report of cases and review of the literature. Hum. Exp. Toxicol. 32, 107–110. doi:10.1177/0960327112454896
Kundra, T., Nagaraja, P., Singh, N., Dhananjaya, M., Sathish, N., and Manjunatha, N. (2016). Effect of dexmedetomidine on diseased coronary vessel diameter and myocardial protection in percutaneous coronary interventional patients. Ann. Card. Anaesth. 19, 394–398. doi:10.4103/0971-9784.185517
Lewis, K., Alshamsi, F., Carayannopoulos, K. L., Granholm, A., Piticaru, J., Al Duhailib, Z., et al. (2022). Dexmedetomidine vs other sedatives in critically ill mechanically ventilated adults: a systematic review and meta-analysis of randomized trials. Intensive Care Med. 48, 811–840. doi:10.1007/s00134-022-06712-2
Li, Y., Meng, L., Peng, Y., Qiao, H., Guo, L., Han, R., et al. (2015). Effects of Dexmedetomidine on motor- and somatosensory-evoked potentials in patients with thoracic spinal cord tumor: a randomized controlled trial. BMC Anesthesiol. 16, 51. doi:10.1186/s12871-016-0217-y
Mahmoud, M., Sadhasivam, S., Salisbury, S., Nick, T. G., Schnell, B., Sestokas, A. K., et al. (2010). Susceptibility of transcranial electric motor-evoked potentials to varying targeted blood levels of dexmedetomidine during spine surgery. Anesthesiology 112, 1364–1373. doi:10.1097/ALN.0b013e3181d74f55
Mantz, J., Josserand, J., and Hamada, S. (2011). Dexmedetomidine: new insights. Eur. J. Anaesthesiol. 28, 3–6. doi:10.1097/EJA.0b013e32833e266d
Modra, L. J., Higgins, A. M., Abeygunawardana, V. S., Vithanage, R. N., Bailey, M. J., and Bellomo, R. (2022). Sex differences in treatment of adult intensive care patients: a systematic review and meta-analysis. Crit. Care Med. 50, 913–923. doi:10.1097/CCM.0000000000005469
Sakaeda, T., Tamon, A., Kadoyama, K., and Okuno, Y. (2013). Data mining of the public version of the FDA adverse event reporting system. Int. J. Med. Sci. 10, 796–803. doi:10.7150/ijms.6048
Sato, Y., Matsumura, T., Abe, Y., Kutsumizu, C., and Maeda, S. (2022). Coronary spasm during postoperative sedation with dexmedetomidine. Anesth. Prog. 69, 20–24. doi:10.2344/anpr-69-01-02
Van Decar, L. M., Reynolds, E. G., Sharpe, E. E., Harbell, M. W., Kosiorek, H. E., and Kraus, M. B. (2022). Perioperative diabetes insipidus caused by anesthetic medications: a review of the literature. Anesth. and Analgesia 134, 82–89. doi:10.1213/ANE.0000000000005344
Venn, R., Newman, P., and Grounds, R. (2003). A phase II study to evaluate the efficacy of dexmedetomidine for sedation in the medical intensive care unit. Intensive Care Med. 29, 201–207. doi:10.1007/s00134-002-1579-9
Wang, Y., Zhao, B., Yang, H., and Wan, Z. (2023). A real-world pharmacovigilance study of FDA adverse event reporting system events for sildenafil. Andrology 12, 785–792. doi:10.1111/andr.13533
Weerink, M. A. S., Struys, M. M. R. F., Hannivoort, L. N., Barends, C. R. M., Absalom, A. R., and Colin, P. (2017). Clinical pharmacokinetics and pharmacodynamics of dexmedetomidine. Clin. Pharmacokinet. 56, 893–913. doi:10.1007/s40262-017-0507-7
Wolff, M., Heugel, P., Hempelmann, G., Scholz, A., Mühling, J., and Olschewski, A. (2007). Clonidine reduces the excitability of spinal dorsal horn neurones. Br. J. Anaesth. 98, 353–361. doi:10.1093/bja/ael379
Wolfran, L., Debiage, R. R., Lopes, D. M., and Fukushima, F. B. (2022). Ophthalmic effects of dexmedetomidine, methadone and dexmedetomidine–methadone in healthy cats and their reversal with atipamezole. J. Feline Med. Surg. 24, 1253–1259. doi:10.1177/1098612X221077023
Zhang, Q., Ding, Y., Shu, Y., and Chen, J. (2023). A real-world disproportionality analysis of rucaparib: post-marketing pharmacovigilance data. BMC Cancer 23, 745. doi:10.1186/s12885-023-11201-w
Keywords: adverse event, Dexmedetomidine, FDA adverse event reporting system, real-world, safety
Citation: Shuai Y, Chen Z, Wan Q, Wu J and Wang X (2024) Dexmedetomidine: a real-world safety analysis based on FDA adverse event reporting system database. Front. Pharmacol. 15:1419196. doi: 10.3389/fphar.2024.1419196
Received: 17 April 2024; Accepted: 12 August 2024;
Published: 23 August 2024.
Edited by:
Federico Bilotta, Sapienza University of Rome, ItalyReviewed by:
Somchai Amornyotin, Mahidol University, ThailandCopyright © 2024 Shuai, Chen, Wan, Wu and Wang. This is an open-access article distributed under the terms of the Creative Commons Attribution License (CC BY). The use, distribution or reproduction in other forums is permitted, provided the original author(s) and the copyright owner(s) are credited and that the original publication in this journal is cited, in accordance with accepted academic practice. No use, distribution or reproduction is permitted which does not comply with these terms.
*Correspondence: Xin Wang, d2FuZ3hpbjY2ODhAY3N1LmVkdS5jbg==
†These authors have contributed equally to this work and share first authorship
Disclaimer: All claims expressed in this article are solely those of the authors and do not necessarily represent those of their affiliated organizations, or those of the publisher, the editors and the reviewers. Any product that may be evaluated in this article or claim that may be made by its manufacturer is not guaranteed or endorsed by the publisher.
Research integrity at Frontiers
Learn more about the work of our research integrity team to safeguard the quality of each article we publish.