- 1College of Chinese Medicine, Shandong University of Traditional Chinese Medicine, Jinan, China
- 2First Clinical Medical College, Shandong University of Traditional Chinese Medicine, Jinan, China
The incidence of common gynecological malignancies remains high, with current treatments facing multiple limitations and adverse effects. Thus, continuing the search for safe and effective oncologic treatment strategies continues. Resveratrol (RES), a natural non-flavonoid polyphenolic compound, is widely found in various plants and fruits, such as grapes, Reynoutria japonica Houtt., peanuts, and berries. RES possesses diverse biological properties, including neuroprotective, antitumor, anti-inflammatory, and osteoporosis inhibition effects. Notably, RES is broadly applicable in antitumor therapy, particularly for treating gynecological tumors (cervical, endometrial, and ovarian carcinomas). RES exerts antitumor effects by promoting tumor cell apoptosis, inhibiting cell proliferation, invasion, and metastasis, regulating tumor cell autophagy, and enhancing the efficacy of antitumor drugs while minimizing their toxic side effects. However, comprehensive reviews on the role of RES in combating gynecological tumors and its mechanisms of action are lacking. This review aims to fill this gap by examining the RES antitumor mechanisms of action in gynecological tumors, providing valuable insights for clinical treatment.
1 Introduction
The incidence of gynecological malignant tumors, a major cause of female mortality worldwide, remains high and poses a significant threat to women’s health (Sung et al., 2021). The most common gynecological tumors include cervical carcinoma (CC), endometrial carcinoma (EC), and ovarian carcinoma (OC). Studies have shown that both genetic mutations and epigenetic alterations are critical factors in promoting cancer development and progression (Tomasetti et al., 2017; Yang et al., 2021). However, the pathogenesis of these cancers is not yet fully understood, resulting in a lack of effective therapeutic strategies. Despite significant advances in treating common gynecological tumors, current first-line regimens, including radiotherapy, chemotherapy, and surgery, have multiple limitations and adverse effects. Chemotherapeutic drugs, although broadly effective against a wide range of tumors, often harm normal tissues due to their inability to precisely target tumor cells, leading to significant side effects. In recent years, tumor immunotherapy and targeted therapy have shown great potential (Jin et al., 2019; Xiang et al., 2021; Zhao et al., 2021; Wang et al., 2022). Although these therapies have yielded some positive results compared to conventional treatments, they have not fundamentally improved the problems of adverse effects and patient quality of life. Furthermore, advanced gynecological tumors are prone to metastasis and recurrence, rendering prevention and treatment challenging. Therefore, exploring safe and effective tumor treatment strategies remains a critical priority in oncology research.
Natural botanicals show significant potential in preventing and treating various types of cancer. These botanicals not only have synergistic anti-tumor effects but also improve patients’ quality of life by reducing side effects during radiation and chemotherapy (Moody et al., 2020). Notably, they have demonstrated therapeutic potential for a wide range of cancer types, primarily due to their ability to modulate pathways involved in cancer onset and progression (Hsu and Hwang, 2019; Wang et al., 2020a; Saggam et al., 2020). Resveratrol (RES), a natural non-flavonoid polyphenolic compound, is widely found in various plants and fruits, particularly in P. suffruticosa Andr. Var. Papaveracea (Andr.) Kerner and Reynoutria japonica Houtt. (Tian and Liu, 2020). RES is a major component in the roots of the medicinal plant R. japonica Houtt. (Hou et al., 2024). Furthermore, the Compendium of Materia Medica records that R. japonica Houtt. Has a variety of effects, such as invigorating blood circulation, removing blood stasis, clearing heat, and draining pus (Li, 2014). RES (trans-3, 4, 5-trihydroxystilbene) (Figure 1), with the molecular formula C14H12O3, appears as a white to yellowish powder in its pure form. Due to the presence of two phenolic rings interconnected by a bis-styrene bond, RES exists in both trans- and cis-isomeric forms. Among these, the cis isomer is not suitable for commercial applications due to its instability. However, the trans isomer of RES exhibits higher stability and greater biological activity than the cis isomer (Wenzel and Somoza, 2005).
RES exhibits a wide range of biological activities, including antioxidant (Iuga et al., 2012; Brenjian et al., 2020), anti-inflammatory (Alrafas et al., 2020; He et al., 2021), antitumor (Khusbu et al., 2020), cardiovascular protection (Gal et al., 2021), neuroprotection (Abd Aziz et al., 2020), hypoglycemic (Joycharat et al., 2018), and antiviral effects. (Zhao et al., 2018). These multi-faceted biological activities render RES useful in the treatment and prevention of diseases, such as cardiovascular disease, Alzheimer’s disease, diabetes, and cancer. RES influences all stages of carcinogenesis (tumor initiation and promotion) and progression (angiogenesis and metastasis) (Ren et al., 2021). Notably, RES demonstrates a broad spectrum of antitumor properties and exhibits significant inhibitory effects on various cancer cell types, including lung (Li et al., 2020), renal cell carcinoma (Tian et al., 2020), hepatocellular carcinoma (Zhang et al., 2020), and ovarian cancer (Vergara et al., 2012). The mechanisms of action of RES involve inducing tumor cell apoptosis via multiple pathways, inhibiting proliferation, migration, and invasion, and enhancing the drug efficacy while reducing toxicity when used in combination with chemotherapy or targeted drugs. RES exerts its anticancer functions by regulating signaling pathways, such as Wnt/β-catenin, Hedgehog, mitogen-activated protein kinase (MAPK), and nuclear factor kappa B (NF-κB) (Xie et al., 2023; Ye et al., 2023). In this review, we examine the anticancer mechanisms and targets of RES in common gynecological tumors, aiming to provide a new theoretical basis for treatment.
2 RES background, absorption, and metabolism
RES was first discovered and isolated in 1940 from the roots of Veratrum grandiflorum (Cione et al., 2019). Subsequent studies revealed that RES is widely found in various common medicinal plants, such as R. japonica Houtt., C. tora L., M. alba L., and F. multiflora (Thunb.) Harald. Furthermore, RES has been isolated from crops, such as grapes and peanuts (Tian and Liu, 2020). RES plays a crucial defense role in plants as an antitoxin; for example, its synthesis significantly increases when plants are attacked by pathogenic bacteria or are placed in unfavorable environments (Malaguarnera, 2019). Moreover, RES is regarded as a phytoestrogen (Gehm et al., 1997). Since the anticancer effects of RES were first reported in 1997, its mechanism of action against a wide range of cancer cells has been extensively studied.
The clinical application of RES is limited due to its poor water solubility, short half-life, rapid absorption and metabolism by the body, and low bioavailability (Huang et al., 2019). The oral absorption of RES is approximately 75% (Walle, 2011). After oral ingestion, RES can be absorbed in large quantities by intestinal cells via passive diffusion or carrier-mediated transport across the apical cell membrane. It is then rapidly converted by phase II enzymes in the liver, forming mainly glucuronide or sulfate, which is subsequently excreted by the body through the urine (Lançon et al., 2004; Ratz-Łyko and Arct, 2019). Consequently, relatively low levels of unmetabolized RES are available in the systemic circulation after oral ingestion (Boocock et al., 2007a). Furthermore, RES exhibits a high volume of distribution in the human body due to its lipophilic nature (Boocock et al., 2007b; la Porte et al., 2010). Animal experiments have shown that upon ingestion, RES is concentrated in organs with abundant blood flow, such as the liver, heart, kidneys, and brain (Liang et al., 2013; Menet et al., 2017). However, due to the difficulty in obtaining biopsy samples, little is known about the detailed distribution of RES in different human tissues of the human body, and relevant research information is lacking (Juan et al., 2010). Human oral metabolism assays for RES have indicated that the peak drug mass concentration of the RES prototype was <10 ng/mL, and the plasma concentration of RES with total metabolites was only 400 ng/mL at 0.5–2.0 h following a single oral dose of 25 mg (Goldberg et al., 2003; Walle et al., 2004). Additionally, Walle et al. observed a significant second peak in total plasma radioactivity levels 6 h after oral RES administration (25 mg dose) (Walle et al., 2004), hypothesizing that this may be due to the reabsorption of the conjugated metabolite into the intestine, resulting in an intestinal recirculation effect. In a similar study by Brown et al., the dose-dependent pharmacokinetics of multiple administrations were evaluated over a 29-d period, showing a significant elevation in RES blood concentrations in participants after continuous administration of 5 g doses (Brown et al., 2010).
3 Role of RES in inducing tumor cell apoptosis
Apoptosis is a key component in maintaining tissue homeostasis (Morana et al., 2022). As apoptosis inhibition is a characteristic of tumor cells (Pistritto et al., 2016), inducing apoptosis is an important strategy in tumor therapy.
3.1 B-cell lymphoma-2 (Bcl-2) family of molecules
The Bcl-2 family coordinates apoptosis and regulates mitochondrial outer membrane permeability. Bcl-2 proteins block apoptosis in tumor cells (Fitzsimmons et al., 2020), whereas Bcl-2-associated X protein (Bax) promotes apoptosis (Jeng et al., 2018). Li et al. (2018) reported that RES treatment increases Bax expression and decreases Bcl-2 and B-cell lymphoma-extra-large (Bcl-XL) expression, leading to increased apoptosis in CC (HeLa) cells. RES induces apoptosis in OC A2780 cells by increasing Bax protein expression and activating caspase-3 in normoxic environments (Synowiec et al., 2023). Yao et al. (2021) found that RES treatment effectively accelerates apoptosis in OC cells (SKOV-3 and OV-90) by increasing miR-34a levels and decreasing Bcl-2 levels, suggesting that its pro-apoptotic mechanism might involve the miR-34a/Bcl-2 axis. Tang et al. (2015) reported that RES induces apoptosis in OC cells by upregulating Bax and caspase-3 expression and downregulating Bcl-2 expression in the human OC cell line, SKOV-3.
3.2 p53 oncogenes
p53 is a critical oncogene in the human body, and the loss of its function is a prerequisite for cancer development (Zhang et al., 2020). RES promotes apoptosis in CC cell lines (HeLa and CaSki) (Sun et al., 2021) by inhibiting the expression of the human papillomavirus E6 and E7 proteins (E6/E7) and significantly increasing p53 and Bax levels, which promote G1/S phase blocking. This finding is consistent with that of Chatterjee et al. (2018), who reported that RES treatment suppresses E6 expression in CC cells (HeLa), significantly enhancing p53-mediated apoptosis. Moreover, Flores-Pérez and Elizondo (2018) also found that RES promotes apoptosis in HeLa cells. Specifically, RES promotes G1/S phase cell blockade and induces apoptosis by increasing p53 expression and inducing G1/S phase cell blockade.
3.3 Endoplasmic reticulum (ER) stress
Proper ER function, particularly in protein synthesis, folding, and modification, is vital for cell survival (Schwarz and Blower, 2016). Disrupting homeostasis triggers the accumulation of unfolded or misfolded proteins in the ER, inducing an unfolded protein response (UPR) (Di Conza and Ho, 2020). A mild UPR maintains cellular homeostasis, whereas a sustained, overly strong UPR induces apoptosis (Koksal et al., 2021). Gwak et al. (2016) studied OC cells and showed that by inhibiting protein kinase B (AKT) activation, RES triggers glycogen synthase kinase-3β (GSK3β) activation and downregulates ectonucleoside triphosphate diphosphohydrolase 5 (ENTPD5) expression, ultimately interfering with N-linked protein glycosylation and inducing ER stress-mediated apoptosis.
3.4 Other mechanisms of RES-induced apoptosis in tumor cells
The sodium/lithium/calcium exchanger (NCLX) is a key protein involved in maintaining intracellular calcium homeostasis (Takeuchi et al., 2020; Britti et al., 2021). Devi et al. (2021) reported that NCLX mRNA levels were significantly upregulated in HeLa cells after RES treatment, leading to increased intracytoplasmic calcium ions and ultimately triggering calcium homeostasis disruption-mediated apoptosis. In another study, RES treatment inhibited extracellular signal-regulated kinase (ERK) and Forkhead box O (FOXO) 3a phosphorylation while increasing FOXO3a and Bim expression and promoting FOXO3a nuclear translocation, which induced apoptosis in HeLa cells (Liu et al., 2020). Reactive oxygen species (ROS) are highly reactive, short-lived chemically active molecules. Tumor cell proliferation often coincides with elevated ROS production (Hayes et al., 2020). ROS induces apoptosis in tumor cells by mediating oxidative stress (Moloney and Cotter, 2018; Srinivas et al., 2019). According to Kim et al. (Kim et al., 2019), RES treatment stimulates ROS generation in cells, which inhibits neurogenic locus notch homolog protein 1 (Notch1) signaling, ultimately resulting in decreased p-AKT expression and increased phosphatase and tensin homolog (PTEN) expression. Therefore, RES induces apoptosis in OC cells via the ROS/Notch1/PTEN/AKT signaling pathway (Table 1; Figure 2).
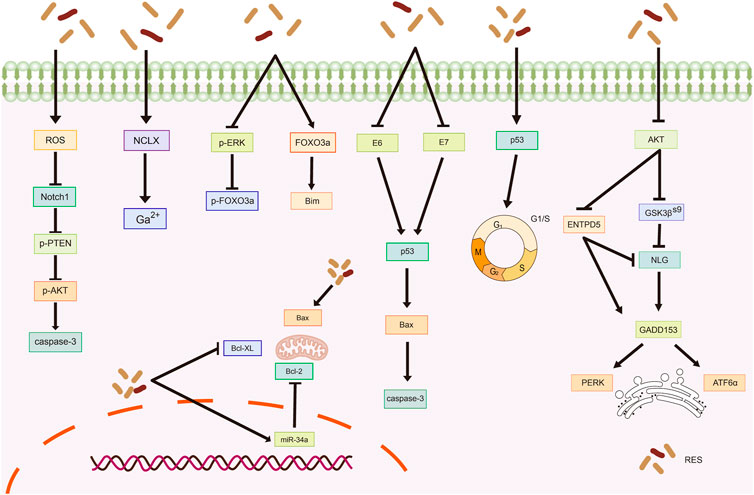
Figure 2. Mechanism of resveratrol inducing apoptosis in common gynecological tumors. RES, resveratrol; ROS, reactive oxygen species; Notch1, Neurogenic locus notch homolog protein 1; p-PTEN, phospho- Phosphatase and tensin homolog; p-AKT, phospho-Protein kinase B; NCLX, sodium/lithium/calcium exchanger; FOXO3a, Forkhead box O3; Bim, Bim Protein; E6, Human papillomavirus E6 protein; E7, Human papillomavirus E7 protein; Bcl-2, B-cell lymphoma-2; Bax, Bcl-2-associated X; Bovine B-cell lymphoma-XL; GSK3β(S9), glycogen synthase kinase-3β(Ser9); ENTPD5, ER UDPase, ectonucleoside triphosphate diphosphohydrolase 5; NLG, N-linked glycosylation; GADD153, rowth arrest/DNA damage-inducible protein153; PERK, Protein Kinase RNA-like Endoplasmic Reticulum Kinase; ATF6α, Recombinant Activating Transcription Factor 6-α.
4 Role of RES in inhibiting tumor cell proliferation
Tumorigenesis is closely related to malignant tumor cell proliferation (Hanahan and Weinberg, 2011); therefore, inhibiting tumor cell proliferation presents an effective means of treating tumors.
4.1 Proliferating cell nuclear antigen (PCNA)
PCNA is a common marker of cell proliferation and plays a crucial role in processes, such as DNA replication, repair, and recombination (Cardano et al., 2020). In recent years, PCNA has emerged as a potential non-carcinogenic antitumor target, and its inhibition may control the abnormal proliferation of tumor cells (Kowalska et al., 2018; Horsfall et al., 2020). An in vivo experiment confirmed that by inhibiting PCNA protein expression, RES can block the cell cycle and inhibit proliferation, thereby significantly reducing the size of CC tumors (Chatterjee et al., 2019). Additionally, RES downregulated E6 and vascular endothelial growth factor expression. Zhang et al. (2020) reported that RES effectively inhibited NUTU-19 (OC cell line)-transplanted tumor growth in rats. Specifically, RES treatment significantly decreased G1/S-specific cyclin (Cyclin)-D1 and PCNA expression; notably increased PTEN, Fas ligand (FasL), and p21 expression; and inhibited the hepatocyte growth factor (HGF)/cellular-mesenchymal epithelial transition factor (c-Met) pathway.
4.2 Wnt signaling pathway
Wnt factors are secreted glycoproteins that play important roles in various biological processes involved in tumor growth and development (Cruciat and Niehrs, 2013). Targeting the Wnt signaling pathway can affect pathophysiological processes, such as cancer cell proliferation, apoptosis, and differentiation. Notably, the Wnt signaling pathway is crucial in ovarian carcinogenesis, development, invasion, and drug resistance (Nguyen et al., 2019). According to Wang and Shi (2019), RES modulates silent mating type information regulation two homolog (SIRT)-1 to inhibit OC cell proliferation and promote apoptosis through a mechanism likely related to the inactivation of the Wnt signaling pathway. Hou et al. (2020) demonstrated that RES significantly inhibits OC cell proliferation in a dose-dependent manner, an effect associated with Wnt signaling pathway activation suppression. Specifically, RES treatment decreased the mRNA expression levels of myelocytomatosis viral oncogene homolog (c-Myc), cyclin A, cyclin D1, N-cadherin, vimentin, p21, and E-cadherin as well as the protein expression levels of β-catenin and GSK3β.
4.3 Other mechanisms of RES-mediated inhibition of tumor cell proliferation
Ma et al. (2021) demonstrated that RES inhibited the integrin-linked kinase/β-catenin pathway activity in A2780 cells, affecting the expression of the downstream target gene associated with cyclin D1, resulting in the inhibition of A2780 cell growth and proliferation. An in vitro experiment on CC revealed that telomerase activity was effectively inhibited by RES treatment in HeLa cells, with a concentration-dependent reduction in human telomerase reverse transcriptase (hTERT) expression levels being observed (He et al., 2019). This finding suggests that RES inhibits the telomerase activity of HeLa cells by regulating telomerase transcription, thereby inhibiting their proliferation. Furthermore, Zhao et al. (2019) reported that RES effectively inhibited CC cell proliferation by downregulating phospholipid scramblase one expression (Table 2; Figure 3).
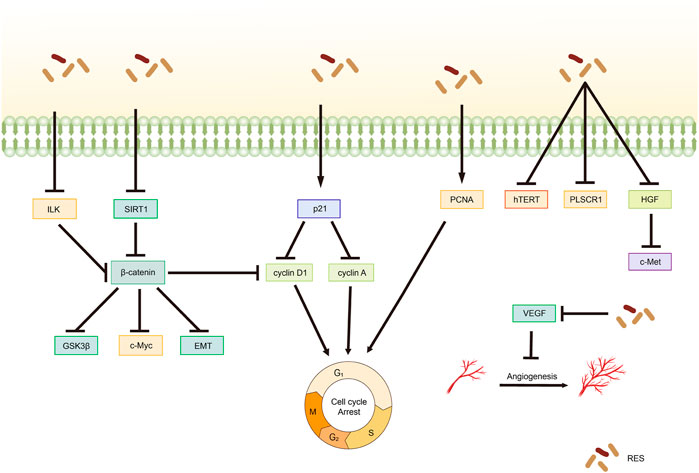
Figure 3. Mechanism of resveratrol suppressing proliferation in common gynecological tumors. RES, resveratrol; ILK, integrin-linked kinase; GSK3β, glycogen synthase kinase-3β; SIRT1, Silent mating type information regulation 2 homolog-1; c-Met, cellular-Mesenchymal epithelial transition factor; EMT, Epithelial–Mesenchymal transition; PCNA, proliferating cell nuclear antigen; hTERT, human telomerase reverse transcriptase; PLSCR1, Phospholipid scramblase 1; HGF, hepatocyte growth factor; c-Met, cellular-Mesenchymal epithelial transition factor; VEGF, Vascular endothelial growth factor.
5 Role of RES in inhibiting metastasis and tumor cell invasion
Migration and invasion of cancer cells are crucial factors in accelerating cancer progression, closely related to poor prognosis and causing death in patients with cancer (Padmanaban et al., 2019). Inhibition of tumor metastasis is an important measure for delaying tumor progression (Paul et al., 2017).
5.1 Epithelial–mesenchymal transition (EMT)
EMT is a critical step in the process of tumor migration and invasion. EMT can be induced or regulated by various growth and differentiation factors. Moreover, EMT is associated with the progression, invasion, and metastasis of a wide range of tumors (Kalluri, 2009; Koren et al., 2015; Wang et al., 2020b; Taki et al., 2021). Sun et al. (2020) demonstrated that RES suppresses the metastatic potential of CC in vitro and in vivo by inhibiting the phosphorylation of signal transduction and transcriptional activator 3 (STAT3) at the Tyr705 site. Specifically, RES downregulated the intracellular expression levels of N-cadherin, vimentin, matrix metalloproteinase (MMP)-3, and MMP-9 and increased the expression of E-cadherin, likely through EMT inhibition and extracellular matrix degradation. Li et al. (2020b) showed that RES effectively inhibited the invasion and migration of SKOV3 cells. After RES treatment with RES, the phosphorylation levels of interleukin (IL)-6, phospho-Janus kinase (JAK) 2, and STAT3 phosphorylation levels significantly decreased, suppressing the EMT process. This suggests that RES inhibits the invasive and migratory abilities of OC cells by downregulating the JAK2/STAT3 signaling pathway. Additionally, Kim et al. (2016) revealed that RES inhibits norepinephrine (NE)-induced OC cell invasiveness by inhibiting tyrosine protein kinase (Src) phosphorylation and hypoxia-inducible factor (HIF)-1α expression, thereby reducing NE-induced hTERT expression, inhibiting Slug expression, and ultimately blocking the NE-induced EMT process.
5.2 Other mechanisms of RES in suppressing tumor metastasis and invasion
Jeong et al. (2013) showed that RES attenuates lysophosphatidic acid-induced OC invasion by inhibiting epidermal growth factor receptor activation. According to Chen et al. (2023), RES effectively curtails the migration and metastasis of CC cells and improves survival rates in murine models. Specifically, by directly binding to fatty acid binding protein 5 (FABP5), RES competitively inhibits fatty acid binding to FABP5, consequently inhibiting MMP-2 and MMP-9 expression. Additionally, Li et al. (2019) showed that in vitro, RES inhibited the migration and invasion of SKOV3 cells, likely related to the inhibition of the Wnt3a/β-catenin axis. The disintegrin and metalloproteinase (ADAM) family is associated with various biological processes, including angiogenesis, cell–cell interactions, and migration (Schumacher et al., 2020). A recent study demonstrated that by inhibiting ADAM9 protein expression, RES reduces ROS production, significantly inhibiting the migratory ability of CC cells (Nan et al., 2021) (Table 3; Figure 4).
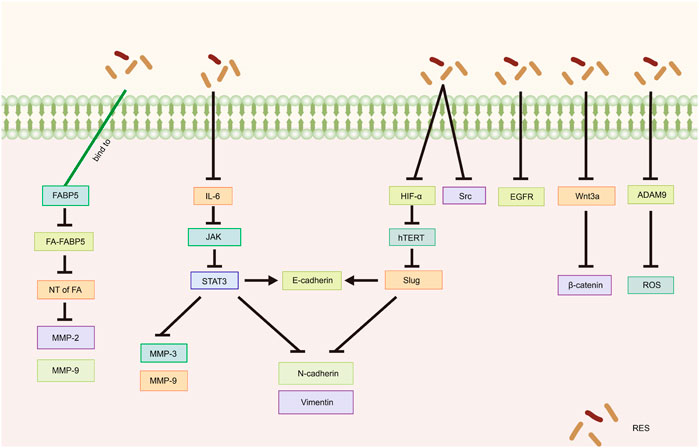
Figure 4. Mechanism of resveratrol suppressing metastasis and invasion in common gynecological tumors. RES, resveratrol; FABP5, Fatty Acid Binding Protein 5; FA, fatty acids; NT of FA, Nuclear transfer of fatty acids; MMP-2, Matrix metalloproteinase-2; MMP-9, Matrix metalloproteinase-9; IL-6, Interleukin-6; JAK, Janus kinase; STAT3, Signal transducer of activators of transcription 3; MMP-3, Matrix metalloproteinase-3; N-cadherin, Neural-cadherin; HIF-1α, Hypoxia Inducible Factor-1α; hTERT, human telomerase reverse transcriptase; Slug, SNAI2; Src, Tyrosine protein kinase; EGFR, Epidermal growth factor receptor; ADAM9, A disintegrin and metalloproteinase 9; ROS, reactive oxygen species.
6 Role of RES in autophagy regulation in tumor cells
Cellular autophagy is an intracellular self-degradation process that maintains normal cellular homeostasis by lysosomal hydrolysis of excessive or damaged organelles and pathogens (Li et al., 2020c). Autophagy can exhibit a dual role, acting either via an antitumor or a protumor mechanism, depending on the stage of cancer development (Zhang Y. et al., 2020).
Fan et al. (2022) observed increased expression of autophagy-related gene six homolog 1 (Beclin1) and microtubule-associated protein one light chain 3 (LC3)-Ⅱ, as well as a rise in the number of autophagic vesicles in cells following RES treatment. Further studies have shown that RES promotes autophagy in Ishikawa cells by inhibiting the phosphatidylinositol 3 kinase (PI3K)/AKT signaling pathway, thereby inhibiting the malignant proliferation of EC (Fan et al., 2022). Aplysia ras homolog I (ARH-I) regulates cellular autophagy (Yan et al., 2019) and is expressed at low levels in OC cells (Washington et al., 2015). In a 3D sphere culture model of OC cell lines (OVCAR3, OAW42, and KURAMOCHI), Esposito et al. (2022) found that IL-6 inhibited autophagy by downregulating ARH-I expression via miR-1305. In contrast, ARH-Ⅰ and LC3-Ⅱ increased, p62 levels decreased, and autophagy was activated in tumor spheroids following RES treatment. Moreover, according to Wang et al. (2019), RES treatment resulted in increased expression levels of the autophagy-related proteins LC3-Ⅱ, Beclin-1, and vacuole membrane protein-1 in SKOV-3 cells, highlighting its potential for inducing autophagy in tumor cells. In another study, Sun X. D. et al. (2021) showed that RES significantly promoted LC3B and Beclin-1 expression and inhibited P62 expression in tumor tissues in vivo and in vitro, hypothesizing that RES inhibits CC by promoting autophagic death in HeLa cells. Sun et al. (2018) also reported similar results in an in vitro assay using HeLa cells (Table 4; Figure 5).
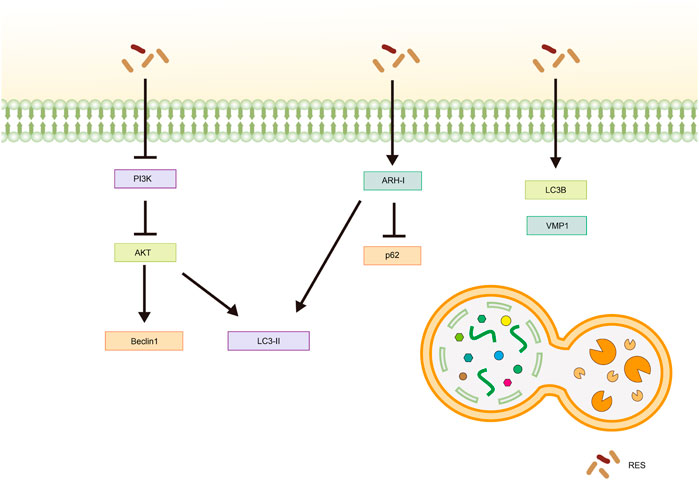
Figure 5. Mechanism of resveratrol inducing autophagy in common gynecological tumors. RES, resveratrol; PI3K, phosphatidylinositol 3-kinase; AKT, Protein Kinase B; LC3-Ⅱ, Microtubule-Associated Protein 1 Light Chain 3-Ⅱ; ARH-I, Aplysia Ras Homolog-I; LC3B, Microtubule-Associated Protein 1 Light Chain 3B; VMP1, vacuole membrane protein-1.
7 Combined with antitumor drugs, RES increases efficacy and reduces toxic side effects
Chemotherapy remains a cornerstone in tumor treatment despite the initial responsiveness of patients with tumors. However, most patients develop acquired resistance after long-term treatment, which is one of the main reasons for unsatisfactory tumor treatment and disease recurrence (Zhang et al., 2022). Consequently, identifying chemotherapeutic sensitizers to prevent and reverse multidrug resistance in tumor cells has become a pressing priority. RES acts synergistically with existing antitumor drugs by enhancing the sensitivity of cancer cells to chemotherapeutic drugs, effectively reversing multidrug resistance and reducing the toxic side effects during chemotherapy. The synergistic effect of RES and other anticancer drugs involves the regulation of multiple signaling pathways and cellular processes, including activation of p53, reduction of the expression of P-glycoprotein (P-gp), NF-κB, and TNF-α expression, and inhibition of the PI3K/AKT/mammalian rapamycin target protein (mTOR) axis. With these synergistic effects, RES can significantly enhance the impact of anticancer drugs, providing new strategies for cancer treatment.
7.1 Potentiating effect of RES on antitumor drugs
7.1.1 Cisplatin (CDDP)
CDDP is considered a first-line therapeutic agent due to its broad-spectrum antitumor effects and significant results in clinical results. However, most patients develop inherent or acquired drug resistance to CDDP (Rocha et al., 2018). RES treatment significantly enhances the sensitivity of SKOV-3 cells to CDDP (Hankittichai et al., 2023). Specifically, the combination of RES and CDDP activates caspase-3, caspase-9, and poly (ADP-ribose) polymerase (PARP); inhibits cyclin A2 and cyclin B1 expression; and induces cell cycle arrest in the S phase, ultimately inhibiting cell proliferation and promoting apoptosis. Further experiments revealed that RES strongly activates p38 phosphorylation and inhibits AKT activation (Hankittichai et al., 2023). Yue et al. (2019) showed that RES enhances the sensitivity of HeLa cells to CDDP by inducing G1 phase blockage and apoptosis. Mechanistically, p21, Bax, caspase-3, and caspase-9 expression increased, whereas cyclin-dependent kinase 2 (CDK2), cyclin D1, and Bcl-2 expression decreased in cells treated with the combination treatment group compared to those treated with CDDP alone. Muhanmode et al. (2022) showed that RES and curcumin reversed OC-acquired CDDP resistance in vitro by significantly inhibiting the PI3K/AKT/mTOR axis. Jiang et al. (2022) demonstrated that RES treatment significantly enhances the sensitivity of SiHa cells to CDDP by activating SIRT3. Specifically, combination treatment increases the levels of hydrogen peroxide (H2O2) and manganese superoxide dismutase (MnSOD) in the cells and significantly reduces mitochondrial membrane potential (ΔΨm) and ROS levels. P-gp, a membrane transporter crucial for recognizing and expelling chemotherapeutic agents, reduces their intracellular accumulation and efficacy (Robey et al., 2018; Afonso de Lima et al., 2020). Wang et al. (2021) showed that RES reduces P-gp expression and reversed CDDP resistance in OC (SKOV-3) cells by downregulating multidrug resistance protein (MDR)-1 and Bcl-2 mRNA expression.
Hypoxia is a major hallmark of any solid tumor. Synowiec et al. (2023) demonstrated that the combination of RES and CDDP was ineffective in a hypoxic environment and that RES counteracted the pharmacological effects of CDDP in OC (A2780 cells). This highlights the need to consider factors, such as hypoxic status, when evaluating the impact of RES on CDDP chemosensitization and conducting assays under conditions mimicking the tumor microenvironment (TME).
7.1.2 Additional potentiation of RES
Docetaxel is a paclitaxel-based antitumor drug with significant effects on advanced malignant tumors. The experimental results of Dou et al. (2022) in OC rats with loaded tumors showed that RES enhanced the inhibitory effect of docetaxel on the growth of xenograft tumor growth, suggesting that the mechanism may be related to an increase in Bax and caspase-3 expression and inhibition of Bcl-2 expression. Doxorubicin (DOX) is widely used as a chemotherapeutic agent for treating various hematological and solid cancers, including osteosarcoma, cervical cancer, and breast cancer. A study on OC stem cells confirmed that RES enhances the sensitivity of SKOV3 cells to DOX by activating the mitochondrial apoptotic pathway and inhibiting MDR-1 and multidrug resistance-associated protein-1 expression (Pouyafar et al., 2019) (Table 5).
7.2 RES attenuates damage to the reproductive system caused by antitumor drugs
Chemotherapeutic agents can damage the female reproductive system, with ovaries being one of the most commonly affected organs (Morgan et al., 2012).
7.2.1 CDDP
CDDP use may result in ovarian hypoplasia or exhaustion (Meirow, 2000; Rossi et al., 2017). Ciplak et al. (2023) showed that RES prevents and attenuates CDDP toxicity in ovarian follicles by increasing superoxide dismutase and catalase levels and decreasing malondialdehyde levels in OC tissue. Similar results were obtained by Chinwe et al. (2018), who hypothesized that RES promotes follicle-stimulating hormone expression. Said et al. (2019) noted that RES, by decreasing PARP1 expression, reversed the CDDP treatment-induced increase in the expression of the inflammatory factors cytochrome c and caspase-3. Another study showed that RES coadministration ameliorated CDDP-mediated ovarian and uterine damage in rats by decreasing the expression of inflammatory factors (NF-κB, tumor necrosis factor-α, cyclooxygenase-2), oxidative stress markers, and increasing cellular estradiol, progesterone, and prolactin levels (Ibrahim et al., 2021). However, in this study, the coadministration of RES and CDDP downregulated follicle-stimulating hormone expression.
7.2.2 Other attenuative effects of RES
Wu et al. (2019) reported that RES effectively alleviates chemotherapy-induced ovarian stress and attenuates oogonial stem cell damage via SIRT1/FOXO1 axis activation. H2O2 was further used to establish an oxidative stress injury model. The results showed that nuclear factor erythroid-2 related factor (Nrf) 2 and superoxide dismutase 2 (SOD2) levels increased in RES-treated cells, and H2O2-induced apoptosis was significantly reduced. Herrero et al. (2023) found that RES effectively attenuated DOX-induced ovarian injury in a murine model, with the mechanism related to the protection of primordial follicular cells, promotion of follicular cell proliferation, and inhibition of DOX-mediated changes in endogenous apoptosis (Table 6).
8 RES nanoparticles for improved bioavailability
Due to the poor water solubility, rapid metabolism, and limited bioavailability of RES, establishing suitable carriers to enhance its delivery is imperative (Asensi et al., 2002; Walle, 2011; Sharifi-Rad et al., 2021). A nanodelivery system can compensate for these shortcomings of the drug itself without altering the active ingredient, improving bioavailability and stability, and achieving targeted drug delivery (Cheng et al., 2008; Pelaz et al., 2017; Li et al., 2020). Long et al. (Long et al., 2020) used RES, arginine-glycine aspartic acid, and human serum albumin to prepare a novel nanocomplex, which exerted an inhibitory effect on SKOV3 cells in a time- and dose-dependent manner in vitro. Moreover, RES significantly inhibited tumor growth in OC model mice in vivo (Long et al., 2020). Tomoaia et al. (2015) developed gold nanoparticles (GNPs) modified with RES that were used to construct nanocomplexes of DOX-GNPs. These GNPs demonstrated a strong accumulation effect and inhibited CC progression through apoptosis induction, showing greater effectiveness in CaSki cells than in HeLa cells.
9 Metabolic, hormonal, and immunological effects of RES in cancer
9.1 Glycolysis
Metabolic reprogramming is a key indicator of cancer development and progression. Glycometabolism is a major cellular energy production pathway. Glycolysis, a basic process of gluconeogenesis, plays a crucial role in cellular metabolism, and its enhancement is a prominent feature of cancer metabolic processes (Liberti and Locasale, 2016; Zhong et al., 2018; Wu et al., 2020). Therefore, inhibiting the glycolytic pathway inhibition should be considered in tumor treatment. Vidoni et al. (2023) demonstrated that RES treatment significantly inhibits glucose uptake and glycolysis, decreases ROS expression, promotes cellular autophagy, and inhibits cancer cell metastasis in OC cells (OVCAR3 and OAW42). Tan et al. (2016) experimentally demonstrated that RES treatment inhibited glycolysis in A2780 cells and suppressed the growth of murine xenograft tumors. Another study confirmed that the anti-OC effects of RES were achieved by inhibiting glycolysis. Moreover, RES induced activation of the AMPK signaling pathway, inhibited the expression and activation of mTOR, increased the apoptosis level, and inhibited the proliferation, migration, and invasion of A2780 and SKOV3 cells, exerting its antitumor effects (Liu et al., 2018). Further in vivo investigations revealed that RES treatment effectively inhibited the growth and metastasis of murine xenograft tumors. Gwak et al. (2015) demonstrated that via AKT activity, RES suppressed the translocation of glucose transporter one and further reduced the glucose uptake capacity of OC cells (PA-1, OVCAR3, MDAH2774, and SKOV3), thus exerting antitumor effects.
9.2 Estrogenic effects
EC is a common gynecological malignancy closely related to estrogen levels (Rodriguez et al., 2019). When estrogen binds to estrogen receptors on the surface of tumor cells, it initiates various signaling pathways that play important roles in the formation, infiltration, and metastasis of EC (Hawsawi et al., 2013; Medhin et al., 2020). Various estrogen metabolites may cause genotoxic damage (Cavalieri et al., 2006). For example, 4-hydroxyestradiol (4-OHE2) and 2-methoxyestradiol (2-MeOE2) are major carcinogens among estrogen metabolites and are considered tumor markers in patients with EC (Zhao et al., 2015). These metabolites bind to DNA via a non-receptor-mediated pathway to form adducts that trigger DNA-coding errors, induce DNA damage, and promote cancer development (Saeed et al., 2009). Therefore, the occurrence and development of EC are closely associated with the abnormal accumulation of estrogen and its toxic metabolites.
Notably, RES acts as a phytoestrogen that can bind to estrogen receptors and exert estrogen-like effects (Gehm et al., 1997; Sato et al., 2003). RES-induced apoptosis in breast cancer MCF-7 cells can be inhibited by E2 (Zhang et al., 2004). A recent study revealed that RES modulated estrogenic homeostasis in mice with endometrial cancer (Yin et al., 2019). Using a murine model of EC induced by N-methyl- N′-nitro-N-nitrosoguanidine, Yin et al. (2019) found that RES effectively decreased 4-OHE2 levels and increased 2-MeOE2 levels, thereby inhibiting EC development and progression.
9.3 Regulation of immunity
Immune surveillance plays a crucial role in recognizing and destroying cancer cells. In the TME, the function of immune cells is significantly suppressed, providing a protective mechanism for tumor cells to successfully achieve immune escape. Notably, the suppressive effect of immune cells in the TME is a critical step in the initiation of tumor metastasis before its occurrence (Liu and Cao, 2016). Therefore, enhancing immunity has been established as a core strategy for cancer prevention and treatment. According to Chen et al. (2022), RES reduces pyruvate kinase isozyme type M2 (PKM2) and glucose transporter (GLUT) one expression, inhibiting the glycolytic process and reducing lactate production in OC cells, thereby reversing T-regulatory cell-mediated immunosuppression. Zhang et al. (2019) found that RES exerted antitumor effects on OC in vitro and in vivo by inducing immunogenic cell death. Further studies showed that in loaded mice, RES improved the TME, promoted IL12p7 and IFN-γ production, inhibited transforming growth factor (TGF)-β expression, and significantly increased the number of CD80+, CD86+, and CD8 cells. Additionally, the experimental results of Chen et al. (2022) and Zhang et al. (2019) demonstrated that RES, in combination with PD-1 antibody, significantly inhibited xenograft tumor growth.
10 Challenges, limitations, and prospects
RES exhibits promising antitumor activity in both in vitro and in vivo experiments; however, it still faces many challenges in practical applications. Due to the complexity and large number of cellular processes involved in carcinogenesis, the specific mechanism of the role of RES in the intricate cell signaling network requires further exploration. Additionally, the lipophilic nature and rapid metabolism of RES result in low bioavailability (Boocock et al., 2007a; Ren et al., 2021). Improving the bioavailability of RES in the human body is essential. Moreover, previous clinical studies have shown certain side effects, such as gastrointestinal reactions (including nausea, flatulence, and diarrhea) and kidney injury (la Porte et al., 2010; Popat et al., 2013). Therefore, an in-depth investigation of the possible side effects and related safety issues associated with the long-term use of RES is required.
While RES demonstrates inhibitory effects on tumor cells under laboratory conditions, clinical trials remain integral to any drug development process (Patel et al., 2010). However, studies on the anticancer mechanisms of RES in common gynecological tumors have focused on rodent models and in vitro cellular experiments, with a paucity of clinical trial data. Although nearly 200 clinical studies have been conducted on RES, studies focusing on cancer treatment remain in the minority. Notably, no clinical trials have been documented on RES in the treatment of EC, CC, and OC (Brown et al., 2024). Accordingly, our understanding of the efficacy and safety of RES in actual patient populations remains limited. The doses of RES used in different studies vary considerably, and the lack of a uniform standardized dose increases the uncertainty of its clinical application. Furthermore, combining RES with nanocarriers can achieve precise targeted drug delivery, significantly enhancing drug bioavailability and reducing side effects. However, there is still a paucity of current research on RES nanoformulations specifically targeting common gynecological tumors.
Despite the many challenges and limitations, RES remains promising as a natural antitumor drug candidate. Future studies should focus on i) clinically validating the therapeutic efficacy of RES in common gynecological tumors through large-sample, high-quality randomized controlled double-blind trials to ensure the certainty of its efficacy; ii) developing nanoformulations of RES to enhance the bioavailability; and iii) using modern technical means to analyze and explore the anti-gynecological tumor mechanisms and mechanisms of action, providing more credible data to support for the development of new clinical drugs.
11 Conclusion
Gynecological cancers, which represent a significant health threat to women, have limited therapeutic options and present challenges in radical treatment. Due to the characteristics of malignant tumors, such as recurrence, distant metastasis, and chemotherapeutic drug resistance, the prognosis of such tumors is often poor, rendering the treatment process complicated and challenging. Therefore, there is an urgent need for comprehensive molecular-level investigations into their pathogenesis, and the active pursuit of efficient and low-toxicity therapeutic targets is urgently needed.
RES is a naturally occurring compound that exerts potent pharmacological effects, particularly in antitumor therapy. RES exhibits broad-spectrum antitumor effects and is effective against various cancer types, such as breast (Sun et al., 2019), pancreatic (Xiao et al., 2020), lung (Gao and Ren, 2021), gastric (Yin et al., 2020), and prostate cancers (Hsieh and Wu, 2020). RES exerts significant antitumor effects on common gynecological tumors via multiple mechanisms. Specifically, RES can induce apoptosis of tumor cells, inhibit cell proliferation, invasion, and metastasis, and regulate tumor cell autophagy. Additionally, RES improves the effectiveness, reduces the toxic side effects of anticancer drugs, and effectively inhibits the glycolytic process of tumor cells. RES can also regulate the immune microenvironment, which is closely related to tumors, and affect estrogen levels. At the molecular level, RES can promote cancer cell death by regulating the Bax/Bcl-2 ratio and the expression of key proteins, such as GSK3β and p53. Furthermore, RES further exerts its antitumor effects by regulating cell signaling pathways, such as miR-34a/Bcl-2, HGF/c-Met, ILK/β-catenin, and PI3K/AKT/mTOR.
In summary, RES, especially the use of RES-modified nanoparticles, shows remarkable potential for application in the treatment of common gynecological tumors. Further scientific and rational experimental studies and clinical validation are needed to explore the specific targets of RES and elucidate its mechanisms of action.
Author contributions
QY: Visualization, Writing–original draft, Writing–review and editing. DM: Writing–review and editing. QZ: Writing–review and editing. JW: Supervision, Writing–original draft, Writing–review and editing.
Funding
The author(s) declare that financial support was received for the research, authorship, and/or publication of this article. Ding Yuanqing National Famous Traditional Chinese Medicine Expert Inheritance Workshop.
Conflict of interest
The authors declare that the research was conducted in the absence of any commercial or financial relationships that could be construed as a potential conflict of interest.
Publisher’s note
All claims expressed in this article are solely those of the authors and do not necessarily represent those of their affiliated organizations, or those of the publisher, the editors and the reviewers. Any product that may be evaluated in this article, or claim that may be made by its manufacturer, is not guaranteed or endorsed by the publisher.
References
Abd Aziz, N. A. W., Iezhitsa, I., Agarwal, R., Abdul Kadir, R. F., Abd Latiff, A., and Ismail, N. M. (2020). Neuroprotection by trans-resveratrol against collagenase-induced neurological and neurobehavioural deficits in rats involves adenosine A1 receptors. Neurol. Res. 42 (3), 189–208. doi:10.1080/01616412.2020.1716470
Afonso de Lima, C., de Souza Bueno, I. L., Nunes Siqueira Vasconcelos, S., Sciani, J. M., Ruiz, A., Foglio, M. A., et al. (2020). Reversal of ovarian cancer cell lines multidrug resistance phenotype by the association of apiole with chemotherapies. Pharm. (Basel) 13 (10), 327. doi:10.3390/ph13100327
Alrafas, H. R., Busbee, P. B., Nagarkatti, M., and Nagarkatti, P. S. (2020). Resveratrol downregulates miR-31 to promote T regulatory cells during prevention of TNBS-induced colitis. Mol. Nutr. Food Res. 64 (1), e1900633. doi:10.1002/mnfr.201900633
Asensi, M., Medina, I., Ortega, A., Carretero, J., Baño, M. C., Obrador, E., et al. (2002). Inhibition of cancer growth by resveratrol is related to its low bioavailability. Free Radic. Biol. Med. 33 (3), 387–398. doi:10.1016/s0891-5849(02)00911-5
Boocock, D. J., Faust, G. E., Patel, K. R., Schinas, A. M., Brown, V. A., Ducharme, M. P., et al. (2007a). Phase I dose escalation pharmacokinetic study in healthy volunteers of resveratrol, a potential cancer chemopreventive agent. Cancer. Epidemiol. biomarkers. Prev. 16 (6), 1246–1252. doi:10.1158/1055-9965.Epi-07-0022
Boocock, D. J., Patel, K. R., Faust, G. E., Normolle, D. P., Marczylo, T. H., Crowell, J. A., et al. (2007b). Quantitation of trans-resveratrol and detection of its metabolites in human plasma and urine by high performance liquid chromatography. J. Chromatogr. B Anal. Technol. Biomed. Life Sci. 848 (2), 182–187. doi:10.1016/j.jchromb.2006.10.017
Brenjian, S., Moini, A., Yamini, N., Kashani, L., Faridmojtahedi, M., Bahramrezaie, M., et al. (2020). Resveratrol treatment in patients with polycystic ovary syndrome decreased pro-inflammatory and endoplasmic reticulum stress markers. Am. J. Reprod. Immunol. 83 (1), e13186. doi:10.1111/aji.13186
Britti, E., Delaspre, F., Tamarit, J., and Ros, J. (2021). Calpain-Inhibitors protect frataxin-deficient dorsal root ganglia neurons from loss of mitochondrial Na(+)/Ca(2+) exchanger, NCLX, and apoptosis. Neurochem. Res. 46 (1), 108–119. doi:10.1007/s11064-020-03020-3
Brown, K., Theofanous, D., Britton, R. G., Aburido, G., Pepper, C., Sri Undru, S., et al. (2024). Resveratrol for the management of human health: how far have we come? A systematic review of resveratrol clinical trials to highlight gaps and opportunities. Int. J. Mol. Sci. 25 (2), 747. doi:10.3390/ijms25020747
Brown, V. A., Patel, K. R., Viskaduraki, M., Crowell, J. A., Perloff, M., Booth, T. D., et al. (2010). Repeat dose study of the cancer chemopreventive agent resveratrol in healthy volunteers: safety, pharmacokinetics, and effect on the insulin-like growth factor axis. Cancer Res. 70 (22), 9003–9011. doi:10.1158/0008-5472.Can-10-2364
Cardano, M., Tribioli, C., and Prosperi, E. (2020). Targeting proliferating cell nuclear antigen (PCNA) as an effective strategy to inhibit tumor cell proliferation. Curr. Cancer Drug Targets 20 (4), 240–252. doi:10.2174/1568009620666200115162814
Cavalieri, E., Chakravarti, D., Guttenplan, J., Hart, E., Ingle, J., Jankowiak, R., et al. (2006). Catechol estrogen quinones as initiators of breast and other human cancers: implications for biomarkers of susceptibility and cancer prevention. Biochim. Biophys. Acta 1766 (1), 63–78. doi:10.1016/j.bbcan.2006.03.001
Chatterjee, K., AlSharif, D., Mazza, C., Syar, P., Al Sharif, M., and Fata, J. E. (2018). Resveratrol and pterostilbene exhibit anticancer properties involving the downregulation of HPV oncoprotein E6 in cervical cancer cells. Nutrients 10 (2), 243. doi:10.3390/nu10020243
Chatterjee, K., Mukherjee, S., Vanmanen, J., Banerjee, P., and Fata, J. E. (2019). Dietary polyphenols, resveratrol and pterostilbene exhibit antitumor activity on an HPV E6-positive cervical cancer model: an in vitro and in vivo analysis. Front. Oncol. 9, 352. doi:10.3389/fonc.2019.00352
Chen, J., Huang, S. T., Chen, J. G., He, J. H., Lin, W. M., Huang, Z. H., et al. (2022). Resveratrol reduces lactate production and modifies the ovarian cancer immune microenvironment. Neoplasma 69 (5), 1129–1137. doi:10.4149/neo_2022_220414N410
Chen, X., Tian, J., Zhao, C., Wu, Y., Li, J., Ji, Z., et al. (2023). Resveratrol, a novel inhibitor of fatty acid binding protein 5, inhibits cervical cancer metastasis by suppressing fatty acid transport into nucleus and downstream pathways. Br. J. Pharmacol. 181, 1614–1634. doi:10.1111/bph.16308
Cheng, L., Anglin, E., Cunin, F., Kim, D., Sailor, M. J., Falkenstein, I., et al. (2008). Intravitreal properties of porous silicon photonic crystals: a potential self-reporting intraocular drug-delivery vehicle. Br. J. Ophthalmol. 92 (5), 705–711. doi:10.1136/bjo.2007.133587
Chinwe, G. S., Azuka, O. I., and Adaeze, N. C. (2018). Resveratrol supplementation rescues pool of growing follicles and ovarian stroma from Cisplatin-induced toxicity on the ovary in Sprague-Dawley rats: an experimental study. Int. J. Reprod. Biomed. 16 (1), 19–30. doi:10.29252/ijrm.16.1.19
Cione, E., La Torre, C., Cannataro, R., Caroleo, M. C., Plastina, P., and Gallelli, L. (2019). Quercetin, epigallocatechin gallate, curcumin, and resveratrol: from dietary sources to human MicroRNA modulation. Molecules 25 (1), 63. doi:10.3390/molecules25010063
Ciplak, B., Turmus, E. G., Kara, O., Daglioglu, G., Altindag, M. M., Simsek, Y., et al. (2023). Does resveratrol reduce cisplatin-induced ovarian damage? Rev. Assoc. Med. Bras. 69 (8), e20230314. doi:10.1590/1806-9282.20230314
Cruciat, C. M., and Niehrs, C. (2013). Secreted and transmembrane wnt inhibitors and activators. Cold Spring Harb. Perspect. Biol. 5 (3), a015081. doi:10.1101/cshperspect.a015081
Devi, R. V., Raj, D., and Doble, M. (2021). Lockdown of mitochondrial Ca(2+) extrusion and subsequent resveratrol treatment kill HeLa cells by Ca(2+) overload. Int. J. Biochem. Cell Biol. 139, 106071. doi:10.1016/j.biocel.2021.106071
Di Conza, G., and Ho, P. C. (2020). ER stress responses: an emerging modulator for innate immunity. Cells 9 (3), 695. doi:10.3390/cells9030695
Dou, S. Y., Yue, X. D., Yang, J. L., Gao, L. L., and Ji, H. H. (2022). Research of resveratrol enhances the inhibitory effect of docetaxel on the growth of transplanted ovarian cancer in rats. Chin. J. Clin. Pharmacol. 38 (05), 413–417. doi:10.13699/j.cnki.1001-6821.2022.05.007
Esposito, A., Ferraresi, A., Salwa, A., Vidoni, C., Dhanasekaran, D. N., and Isidoro, C. (2022). Resveratrol contrasts IL-6 pro-growth effects and promotes autophagy-mediated cancer cell dormancy in 3D ovarian cancer: role of miR-1305 and of its target ARH-I. Cancers (Basel) 14 (9), 2142. doi:10.3390/cancers14092142
Fan, Y. J., Shi, Y. C., Li, J., Dong, Z., and Zhan, L. (2022). Resveratrol induces autophagy in endometrial cancer Ishikawa cells by inhibiting the PI3K/AKT pathway. Acta Univ. Med. Anhui 57 (03), 361–365+373. doi:10.19405/j.cnki.issn1000-1492.2022.03.005
Fitzsimmons, L., Cartlidge, R., Chang, C., Sejic, N., Galbraith, L. C. A., Suraweera, C. D., et al. (2020). EBV BCL-2 homologue BHRF1 drives chemoresistance and lymphomagenesis by inhibiting multiple cellular pro-apoptotic proteins. Cell Death Differ. 27 (5), 1554–1568. doi:10.1038/s41418-019-0435-1
Flores-Pérez, A., and Elizondo, G. (2018). Apoptosis induction and inhibition of HeLa cell proliferation by alpha-naphthoflavone and resveratrol are aryl hydrocarbon receptor-independent. Chem.-Biol. Interact. 281, 98–105. doi:10.1016/j.cbi.2017.12.029
Gal, R., Deres, L., Toth, K., Halmosi, R., and Habon, T. (2021). The effect of resveratrol on the cardiovascular system from molecular mechanisms to clinical results. Int. J. Mol. Sci. 22 (18), 10152. doi:10.3390/ijms221810152
Gao, P., and Ren, G. (2021). Identification of potential target genes of non-small cell lung cancer in response to resveratrol treatment by bioinformatics analysis. Aging 13 (19), 23245–23261. doi:10.18632/aging.203616
Gehm, B. D., McAndrews, J. M., Chien, P. Y., and Jameson, J. L. (1997). Resveratrol, a polyphenolic compound found in grapes and wine, is an agonist for the estrogen receptor. Proc. Natl. Acad. Sci. U. S. A. 94 (25), 14138–14143. doi:10.1073/pnas.94.25.14138
Goldberg, D. M., Yan, J., and Soleas, G. J. (2003). Absorption of three wine-related polyphenols in three different matrices by healthy subjects. Clin. Biochem. 36 (1), 79–87. doi:10.1016/s0009-9120(02)00397-1
Gwak, H., Haegeman, G., Tsang, B. K., and Song, Y. S. (2015). Cancer-specific interruption of glucose metabolism by resveratrol is mediated through inhibition of Akt/GLUT1 axis in ovarian cancer cells. Mol. Carcinog. 54 (12), 1529–1540. doi:10.1002/mc.22227
Gwak, H., Kim, S., Dhanasekaran, D. N., and Song, Y. S. (2016). Resveratrol triggers ER stress-mediated apoptosis by disrupting N-linked glycosylation of proteins in ovarian cancer cells. Cancer Lett. 371 (2), 347–353. doi:10.1016/j.canlet.2015.11.032
Hanahan, D., and Weinberg, R. A. (2011). Hallmarks of cancer: the next generation. Cell 144 (5), 646–674. doi:10.1016/j.cell.2011.02.013
Hankittichai, P., Thaklaewphan, P., Wikan, N., Ruttanapattanakul, J., Potikanond, S., Smith, D. R., et al. (2023). Resveratrol enhances cytotoxic effects of cisplatin by inducing cell cycle arrest and apoptosis in ovarian adenocarcinoma SKOV-3 cells through activating the p38 MAPK and suppressing AKT. Pharm. (Basel) 16 (5), 755. doi:10.3390/ph16050755
Hawsawi, Y., El-Gendy, R., Twelves, C., Speirs, V., and Beattie, J. (2013). Insulin-like growth factor - oestradiol crosstalk and mammary gland tumourigenesis. Biochim. Biophys. Acta 1836 (2), 345–353. doi:10.1016/j.bbcan.2013.10.005
Hayes, J. D., Dinkova-Kostova, A. T., and Tew, K. D. (2020). Oxidative stress in cancer. Cancer Cell 38 (2), 167–197. doi:10.1016/j.ccell.2020.06.001
He, J. M., Yang, S. Y., Lu, J., Han, S. S., Liu, R., and Lian, F. Z. (2019). Effects of resveratrol on telomerase activity and gene expression in human cervical cancer HeLa cells. J. Hyg. Res. 48 (06), 1001–1003. doi:10.19813/j.cnki.weishengyanjiu.2019.06.024
He, Y., Lu, X., Chen, T., Yang, Y., Zheng, J., Chen, C., et al. (2021). Resveratrol protects against myocardial ischemic injury via the inhibition of NF-κB-dependent inflammation and the enhancement of antioxidant defenses. Int. J. Mol. Med. 47 (3), 29. doi:10.3892/ijmm.2021.4862
Herrero, Y., Velázquez, C., Pascuali, N., May, M., Abramovich, D., Scotti, L., et al. (2023). Resveratrol alleviates doxorubicin-induced damage in mice ovary. Chem.-Biol. Interact. 376, 110431. doi:10.1016/j.cbi.2023.110431
Horsfall, A. J., Abell, A. D., and Bruning, J. B. (2020). Targeting PCNA with peptide mimetics for therapeutic purposes. Chembiochem 21 (4), 442–450. doi:10.1002/cbic.201900275
Hou, Q., Zhang, L., Hao, Y., Xu, J., and Zhang, T. (2024). Progress and Q-marker prediction analysis of traditional Chinese medicine polygonum cuspidatum. Mod. Chin. Med., 1–17. doi:10.13313/j.issn.1673-4890.20240131001
Hou, Z. Y., Gao, J., Wu, Y., Sun, X. N., and Yang, X. C. (2020). Effects of resveratrol on proliferation activity, expression of proliferation gene and Wnt signaling pathway in ovarian cancer. Chin. J. Surg. Oncol. 12 (01), 63–66. doi:10.3969/j.issn.1674-4136.2020.01.015
Hsieh, T. C., and Wu, J. M. (2020). Resveratrol suppresses prostate cancer epithelial cell scatter/invasion by targeting inhibition of hepatocyte growth factor (HGF) secretion by prostate stromal cells and upregulation of E-cadherin by prostate cancer epithelial cells. Int. J. Mol. Sci. 21 (5), 1760. doi:10.3390/ijms21051760
Hsu, H. Y., and Hwang, P. A. (2019). Clinical applications of fucoidan in translational medicine for adjuvant cancer therapy. Clin. Transl. Med. 8 (1), 15. doi:10.1186/s40169-019-0234-9
Huang, X. T., Li, X., Xie, M. L., Huang, Z., Huang, Y. X., Wu, G. X., et al. (2019). Resveratrol: review on its discovery, anti-leukemia effects and pharmacokinetics. Chem. Biol. Interact. 306, 29–38. doi:10.1016/j.cbi.2019.04.001
Ibrahim, M. A., Albahlol, I. A., Wani, F. A., Abd-Eltawab Tammam, A., Kelleni, M. T., Sayeed, M. U., et al. (2021). Resveratrol protects against cisplatin-induced ovarian and uterine toxicity in female rats by attenuating oxidative stress, inflammation and apoptosis. Chem.-Biol. Interact. 338, 109402. doi:10.1016/j.cbi.2021.109402
Iuga, C., Alvarez-Idaboy, J. R., and Russo, N. (2012). Antioxidant activity of trans-resveratrol toward hydroxyl and hydroperoxyl radicals: a quantum chemical and computational kinetics study. J. Org. Chem. 77 (8), 3868–3877. doi:10.1021/jo3002134
Jeng, P. S., Inoue-Yamauchi, A., Hsieh, J. J., and Cheng, E. H. (2018). BH3-Dependent and independent activation of BAX and BAK in mitochondrial apoptosis. Curr. Opin. Physiol. 3, 71–81. doi:10.1016/j.cophys.2018.03.005
Jeong, K. J., Cho, K. H., Panupinthu, N., Kim, H., Kang, J., Park, C. G., et al. (2013). EGFR mediates LPA-induced proteolytic enzyme expression and ovarian cancer invasion: inhibition by resveratrol. Mol. Oncol. 7 (1), 121–129. doi:10.1016/j.molonc.2012.10.001
Jiang, B., Tian, Q., Shu, C., Zhao, J., Xue, M., and Zhu, S. (2022). Resveratrol enhances the anti-cancer effects of cis-platinum on human cervical cancer cell lines by activating the SIRT3 relative anti-oxidative pathway. Front. Pharmacol. 13, 916876. doi:10.3389/fphar.2022.916876
Jin, J., Wu, X., Yin, J., Li, M., Shen, J., Li, J., et al. (2019). Identification of genetic mutations in cancer: challenge and opportunity in the new era of targeted therapy. Front. Oncol. 9, 263. doi:10.3389/fonc.2019.00263
Joycharat, N., Issarachote, P., Sontimuang, C., and Voravuthikunchai, S. P. (2018). Alpha-glucosidase inhibitory activity of ethanol extract, fractions and purified compounds from the wood of Albizia myriophylla. Nat. Prod. Res. 32 (11), 1291–1294. doi:10.1080/14786419.2017.1333990
Juan, M. E., Maijó, M., and Planas, J. M. (2010). Quantification of trans-resveratrol and its metabolites in rat plasma and tissues by HPLC. J. Pharm. Biomed. Anal. 51 (2), 391–398. doi:10.1016/j.jpba.2009.03.026
Kalluri, R. (2009). EMT: when epithelial cells decide to become mesenchymal-like cells. J. Clin. Invest. 119 (6), 1417–1419. doi:10.1172/jci39675
Khusbu, F. Y., Zhou, X., Roy, M., Chen, F. Z., Cao, Q., and Chen, H. C. (2020). Resveratrol induces depletion of TRAF6 and suppresses prostate cancer cell proliferation and migration. Int. J. Biochem. Cell Biol. 118, 105644. doi:10.1016/j.biocel.2019.105644
Kim, S. H., Cho, K. H., Kim, Y. N., Jeong, B. Y., Park, C. G., Hur, G. M., et al. (2016). Resveratrol attenuates norepinephrine-induced ovarian cancer invasiveness through downregulating hTERT expression. Arch. Pharmacal Res. 39 (2), 240–248. doi:10.1007/s12272-015-0666-8
Kim, T. H., Park, J. H., and Woo, J. S. (2019). Resveratrol induces cell death through ROS-dependent downregulation of Notch1/PTEN/Akt signaling in ovarian cancer cells. Mol. Med. Rep. 19 (4), 3353–3360. doi:10.3892/mmr.2019.9962
Koksal, A. R., Verne, G. N., and Zhou, Q. (2021). Endoplasmic reticulum stress in biological processing and disease. J. Investig. Med. 69 (2), 309–315. doi:10.1136/jim-2020-001570
Koren, S., Reavie, L., Couto, J. P., De Silva, D., Stadler, M. B., Roloff, T., et al. (2015). PIK3CA(H1047R) induces multipotency and multi-lineage mammary tumours. Nature 525 (7567), 114–118. doi:10.1038/nature14669
Kowalska, E., Bartnicki, F., Fujisawa, R., Bonarek, P., Hermanowicz, P., Tsurimoto, T., et al. (2018). Inhibition of DNA replication by an anti-PCNA aptamer/PCNA complex. Nucleic Acids Res. 46 (1), 25–41. doi:10.1093/nar/gkx1184
Lançon, A., Delmas, D., Osman, H., Thénot, J. P., Jannin, B., and Latruffe, N. (2004). Human hepatic cell uptake of resveratrol: involvement of both passive diffusion and carrier-mediated process. Biochem. Biophys. Res. Commun. 316 (4), 1132–1137. doi:10.1016/j.bbrc.2004.02.164
la Porte, C., Voduc, N., Zhang, G., Seguin, I., Tardiff, D., Singhal, N., et al. (2010). Steady-State pharmacokinetics and tolerability of trans-resveratrol 2000 mg twice daily with food, quercetin and alcohol (ethanol) in healthy human subjects. Clin. Pharmacokinet. 49 (7), 449–454. doi:10.2165/11531820-000000000-00000
Li, G., Liu, J., Xie, J., Yu, F., and Hu, Y. (2020d). Effects of resveratrol on proliferation, apoptosis, migration and invasion of ovarian cancer SKOV-3 cells by regulating IL-6/JAK2/STAT3 signaling pathway. Acta Univ. Med. Anhui 55 (05), 706–711. doi:10.19405/j.cnki.issn1000-1492.2020.05.011
Li, L., Qiu, R. L., Lin, Y., Cai, Y., Bian, Y., Fan, Y., et al. (2018). Resveratrol suppresses human cervical carcinoma cell proliferation and elevates apoptosis via the mitochondrial and p53 signaling pathways. Oncol. Lett. 15 (6), 9845–9851. doi:10.3892/ol.2018.8571
Li, P. F., Shi, X. Y., and Zhou, T. (2019). Mechanism of resveratrol inhibiting proliferation and migration of human ovarian cancer cells by inhibiting Wnt pathway. J. Clin. Exp. Med. 18 (19), 2056–2060. doi:10.3969/j.issn.1671-4695.2019.19.012
Li, W., Li, C., Ma, L., and Jin, F. (2020a). Resveratrol inhibits viability and induces apoptosis in the small-cell lung cancer H446 cell line via the PI3K/Akt/c-Myc pathway. Oncol. Rep. 44 (5), 1821–1830. doi:10.3892/or.2020.7747
Li, X., He, S., and Ma, B. (2020b). Autophagy and autophagy-related proteins in cancer. Mol. Cancer 19 (1), 12. doi:10.1186/s12943-020-1138-4
Li, X., Yu, C., Meng, X., Hou, Y., Cui, Y., Zhu, T., et al. (2020c). Study of double-targeting nanoparticles loaded with MCL-1 siRNA and dexamethasone for adjuvant-induced arthritis therapy. Eur. J. Pharm. Biopharm. 154, 136–143. doi:10.1016/j.ejpb.2020.07.009
Liang, L., Liu, X., Wang, Q., Cheng, S., Zhang, S., and Zhang, M. (2013). Pharmacokinetics, tissue distribution and excretion study of resveratrol and its prodrug 3,5,4'-tri-O-acetylresveratrol in rats. Phytomedicine 20 (6), 558–563. doi:10.1016/j.phymed.2012.12.012
Liberti, M. V., and Locasale, J. W. (2016). The warburg effect: how does it benefit cancer cells? Trends biochem. Sci. 41 (3), 211–218. doi:10.1016/j.tibs.2015.12.001
Liu, Y., and Cao, X. (2016). Characteristics and significance of the pre-metastatic niche. Cancer Cell 30 (5), 668–681. doi:10.1016/j.ccell.2016.09.011
Liu, Y., Tong, L., Luo, Y., Li, X., Chen, G., and Wang, Y. (2018). Resveratrol inhibits the proliferation and induces the apoptosis in ovarian cancer cells via inhibiting glycolysis and targeting AMPK/mTOR signaling pathway. J. Cell. Biochem. 119 (7), 6162–6172. doi:10.1002/jcb.26822
Liu, Z., Li, Y., She, G., Zheng, X., Shao, L., Wang, P., et al. (2020). Resveratrol induces cervical cancer HeLa cell apoptosis through the activation and nuclear translocation promotion of FOXO3a. Pharmazie 75 (6), 250–254. doi:10.1691/ph.2020.0386
Long, Q., Zhu, W., Guo, L., and Pu, L. (2020). RGD-conjugated resveratrol HSA nanoparticles as a novel delivery system in ovarian cancer therapy. Drug Des. Dev. Ther. 14, 5747–5756. doi:10.2147/dddt.S248950
Ma, Z., Hu, J., Deng, Y., Xing, G. B., and Wang, L. L. (2021). Inhibitory effect of resveratrol on proliferation of ovarian cancer cells by down-regulating ILK/β-catenin signaling pathway. J. Chongqing. Med. Univ. 46 (02), 212–216. doi:10.13406/j.cnki.cyxb.002752
Malaguarnera, L. (2019). Influence of resveratrol on the immune response. Nutrients 11 (5), 946. doi:10.3390/nu11050946
Medhin, L. B., Tekle, L. A., Achila, O. O., and Said, S. (2020). Incidence of cervical, ovarian and uterine cancer in Eritrea: data from the national health laboratory, 2011-2017. Sci. Rep. 10 (1), 9099. doi:10.1038/s41598-020-66096-5
Meirow, D. (2000). Reproduction post-chemotherapy in young cancer patients. Mol. Cell. Endocrinol. 169 (1-2), 123–131. doi:10.1016/s0303-7207(00)00365-8
Menet, M. C., Baron, S., Taghi, M., Diestra, R., Dargère, D., Laprévote, O., et al. (2017). Distribution of trans-resveratrol and its metabolites after acute or sustained administration in mouse heart, brain, and liver. Mol. Nutr. Food Res. 61 (8). doi:10.1002/mnfr.201600686
Moloney, J. N., and Cotter, T. G. (2018). ROS signalling in the biology of cancer. Semin. Cell Dev. Biol. 80, 50–64. doi:10.1016/j.semcdb.2017.05.023
Moody, R., Wilson, K., Jaworowski, A., and Plebanski, M. (2020). Natural compounds with potential to modulate cancer therapies and self-reactive immune cells. Cancers (Basel) 12 (3), 673. doi:10.3390/cancers12030673
Morana, O., Wood, W., and Gregory, C. D. (2022). The apoptosis paradox in cancer. Int. J. Mol. Sci. 23 (3), 1328. doi:10.3390/ijms23031328
Morgan, S., Anderson, R. A., Gourley, C., Wallace, W. H., and Spears, N. (2012). How do chemotherapeutic agents damage the ovary? Hum. Reprod. Update 18 (5), 525–535. doi:10.1093/humupd/dms022
Muhanmode, Y., Wen, M. K., Maitinuri, A., and Shen, G. (2022). Curcumin and resveratrol inhibit chemoresistance in cisplatin-resistant epithelial ovarian cancer cells via targeting P13K pathway. Hum. Exp. Toxicol. 41, 9603271221095929. doi:10.1177/09603271221095929
Nan, W. Q., Fan, X. F., Song, T., Wang, Q. X., Fang, C. X., and Fu, W. D. (2021). The effect and mechanism of resveratrol on the inhibition of proliferation and migration of cervical cancer cells by regulation of ADAM9. J. Guangxi. Med. Univ. 38 (11), 2140–2145. doi:10.16190/j.cnki.45-1211/r.2021.11.020
Nguyen, V. H. L., Hough, R., Bernaudo, S., and Peng, C. (2019). Wnt/β-catenin signalling in ovarian cancer: insights into its hyperactivation and function in tumorigenesis. J. Ovarian Res. 12 (1), 122. doi:10.1186/s13048-019-0596-z
Padmanaban, V., Krol, I., Suhail, Y., Szczerba, B. M., Aceto, N., Bader, J. S., et al. (2019). E-cadherin is required for metastasis in multiple models of breast cancer. Nature 573 (7774), 439–444. doi:10.1038/s41586-019-1526-3
Patel, K. R., Brown, V. A., Jones, D. J., Britton, R. G., Hemingway, D., Miller, A. S., et al. (2010). Clinical pharmacology of resveratrol and its metabolites in colorectal cancer patients. Cancer Res. 70 (19), 7392–7399. doi:10.1158/0008-5472.Can-10-2027
Paul, C. D., Mistriotis, P., and Konstantopoulos, K. (2017). Cancer cell motility: lessons from migration in confined spaces. Nat. Rev. Cancer 17 (2), 131–140. doi:10.1038/nrc.2016.123
Pelaz, B., Alexiou, C., Alvarez-Puebla, R. A., Alves, F., Andrews, A. M., Ashraf, S., et al. (2017). Diverse applications of nanomedicine. ACS Nano 11 (3), 2313–2381. doi:10.1021/acsnano.6b06040
Pistritto, G., Trisciuoglio, D., Ceci, C., Garufi, A., and D'Orazi, G. (2016). Apoptosis as anticancer mechanism: function and dysfunction of its modulators and targeted therapeutic strategies. Aging 8 (4), 603–619. doi:10.18632/aging.100934
Popat, R., Plesner, T., Davies, F., Cook, G., Cook, M., Elliott, P., et al. (2013). A phase 2 study of SRT501 (resveratrol) with bortezomib for patients with relapsed and or refractory multiple myeloma. Br. J. Haematol. 160 (5), 714–717. doi:10.1111/bjh.12154
Pouyafar, A., Zadi Heydarabad, M., Aghdam, S. B., Khaksar, M., Azimi, A., Rahbarghazi, R., et al. (2019). Resveratrol potentially increased the tumoricidal effect of doxorubicin on SKOV3 cancer stem cells in vitro. J. Cell. Biochem. 120 (5), 8430–8437. doi:10.1002/jcb.28129
Ratz-Łyko, A., and Arct, J. (2019). Resveratrol as an active ingredient for cosmetic and dermatological applications: a review. J. Cosmet. Laser Ther. 21 (2), 84–90. doi:10.1080/14764172.2018.1469767
Ren, B., Kwah, M. X., Liu, C., Ma, Z., Shanmugam, M. K., Ding, L., et al. (2021). Resveratrol for cancer therapy: challenges and future perspectives. Cancer Lett. 515, 63–72. doi:10.1016/j.canlet.2021.05.001
Robey, R. W., Pluchino, K. M., Hall, M. D., Fojo, A. T., Bates, S. E., and Gottesman, M. M. (2018). Revisiting the role of ABC transporters in multidrug-resistant cancer. Nat. Rev. Cancer 18 (7), 452–464. doi:10.1038/s41568-018-0005-8
Rocha, C. R. R., Silva, M. M., Quinet, A., Cabral-Neto, J. B., and Menck, C. F. M. (2018). DNA repair pathways and cisplatin resistance: an intimate relationship. Clinics 73 (Suppl. 1), e478s. doi:10.6061/clinics/2018/e478s
Rodriguez, A. C., Blanchard, Z., Maurer, K. A., and Gertz, J. (2019). Estrogen signaling in endometrial cancer: a key oncogenic pathway with several open questions. Horm. Cancer 10 (2-3), 51–63. doi:10.1007/s12672-019-0358-9
Rossi, V., Lispi, M., Longobardi, S., Mattei, M., Di Rella, F., Salustri, A., et al. (2017). LH prevents cisplatin-induced apoptosis in oocytes and preserves female fertility in mouse. Cell Death Differ. 24 (1), 72–82. doi:10.1038/cdd.2016.97
Saeed, M., Higginbotham, S., Gaikwad, N., Chakravarti, D., Rogan, E., and Cavalieri, E. (2009). Depurinating naphthalene-DNA adducts in mouse skin related to cancer initiation. Free Radic. Biol. Med. 47 (7), 1075–1081. doi:10.1016/j.freeradbiomed.2009.07.020
Saggam, A., Tillu, G., Dixit, S., Chavan-Gautam, P., Borse, S., Joshi, K., et al. (2020). Withania somnifera (L.) Dunal: a potential therapeutic adjuvant in cancer. J. Ethnopharmacol. 255, 112759. doi:10.1016/j.jep.2020.112759
Said, R. S., Mantawy, E. M., and El-Demerdash, E. (2019). Mechanistic perspective of protective effects of resveratrol against cisplatin-induced ovarian injury in rats: emphasis on anti-inflammatory and anti-apoptotic effects. Naunyn Schmiedeb. Arch. Pharmacol. 392 (10), 1225–1238. doi:10.1007/s00210-019-01662-x
Sato, M., Pei, R. J., Yuri, T., Danbara, N., Nakane, Y., and Tsubura, A. (2003). Prepubertal resveratrol exposure accelerates N-methyl-N-nitrosourea-induced mammary carcinoma in female Sprague-Dawley rats. Cancer Lett. 202 (2), 137–145. doi:10.1016/j.canlet.2003.08.016
Schumacher, N., Rose-John, S., and Schmidt-Arras, D. (2020). ADAM-mediated signalling pathways in gastrointestinal cancer formation. Int. J. Mol. Sci. 21 (14), 5133. doi:10.3390/ijms21145133
Schwarz, D. S., and Blower, M. D. (2016). The endoplasmic reticulum: structure, function and response to cellular signaling. Cell. Mol. Life Sci. 73 (1), 79–94. doi:10.1007/s00018-015-2052-6
Sharifi-Rad, J., Quispe, C., Mukazhanova, Z., Knut, E., Turgumbayeva, A., Kipchakbayeva, A., et al. (2021). Resveratrol-based nanoformulations as an emerging therapeutic strategy for cancer. Front. Mol. Biosci. 8, 649395. doi:10.3389/fmolb.2021.649395
Srinivas, U. S., Tan, B. W. Q., Vellayappan, B. A., and Jeyasekharan, A. D. (2019). ROS and the DNA damage response in cancer. Redox Biol. 25, 101084. doi:10.1016/j.redox.2018.101084
Sun, C. M., Fang, W. X., Zhou, R. S., Wang, T. R., Zhao, W. D., and Chen, X. Y. (2018). Effect of resveratrol inhibits the proliferation of cervical cancer cells with autophagy in vitro. Acta Univ. Med. Anhui 53 (05), 664–667+675. doi:10.19405/j.cnki.issn1000-1492.2018.05.002
Sun, X., Fu, P., Xie, L., Chai, S., Xu, Q., Zeng, L., et al. (2021a). Resveratrol inhibits the progression of cervical cancer by suppressing the transcription and expression of HPV E6 and E7 genes. Int. J. Mol. Med. 47 (1), 335–345. doi:10.3892/ijmm.2020.4789
Sun, X., Xu, Q., Zeng, L., Xie, L., Zhao, Q., Xu, H., et al. (2020). Resveratrol suppresses the growth and metastatic potential of cervical cancer by inhibiting STAT3(Tyr705) phosphorylation. Cancer Med. 9 (22), 8685–8700. doi:10.1002/cam4.3510
Sun, X. D., Zhou, Z., Zeng, L., Xie, L. X., Du, K. L., and Sang, M. (2021b). Resveratrol induces autophagic death of cervical cancer cells to exert antitumor effect. Nat. Prod. Res. Dev. 33 (02), 208–215. doi:10.16333/j.1001-6880.2021.2.004
Sun, Y., Zhou, Q. M., Lu, Y. Y., Zhang, H., Chen, Q. L., Zhao, M., et al. (2019). Resveratrol inhibits the migration and metastasis of MDA-MB-231 human breast cancer by reversing TGF-β1-induced epithelial-mesenchymal transition. Molecules 24 (6), 1131. doi:10.3390/molecules24061131
Sung, H., Ferlay, J., Siegel, R. L., Laversanne, M., Soerjomataram, I., Jemal, A., et al. (2021). Global cancer statistics 2020: GLOBOCAN estimates of incidence and mortality worldwide for 36 cancers in 185 countries. Ca-Cancer J. Clin. 71 (3), 209–249. doi:10.3322/caac.21660
Synowiec, A., Brodaczewska, K., Wcisło, G., Majewska, A., Borkowska, A., Filipiak-Duliban, A., et al. (2023). Hypoxia, but not normoxia, reduces effects of resveratrol on cisplatin treatment in A2780 ovarian cancer cells: a challenge for resveratrol use in anticancer adjuvant cisplatin therapy. Int. J. Mol. Sci. 24 (6), 5715. doi:10.3390/ijms24065715
Takeuchi, A., Kim, B., and Matsuoka, S. (2020). Physiological functions of mitochondrial Na(+)-Ca(2+) exchanger, NCLX, in lymphocytes. Cell Calcium 85, 102114. doi:10.1016/j.ceca.2019.102114
Taki, M., Abiko, K., Ukita, M., Murakami, R., Yamanoi, K., Yamaguchi, K., et al. (2021). Tumor immune microenvironment during epithelial-mesenchymal transition. Clin. Cancer Res. 27 (17), 4669–4679. doi:10.1158/1078-0432.Ccr-20-4459
Tan, L., Wang, W., He, G., Kuick, R. D., Gossner, G., Kueck, A. S., et al. (2016). Resveratrol inhibits ovarian tumor growth in an in vivo mouse model. Cancer 122 (5), 722–729. doi:10.1002/cncr.29793
Tang, R. X., Zhang, W., and Zhao, X. Y. (2015). Resveratrol induces apoptosis in ovarian cancer cells SKOV-3. Chin. Tradit. Pat. Med. 37 (10), 2293–2296. doi:10.3969/j.issn.1001-1528.2015.10.044
Tian, B., and Liu, J. (2020). Resveratrol: a review of plant sources, synthesis, stability, modification and food application. J. Sci. Food Agric. 100 (4), 1392–1404. doi:10.1002/jsfa.10152
Tian, X., Zhang, S., Zhang, Q., Kang, L., Ma, C., Feng, L., et al. (2020). Resveratrol inhibits tumor progression by down-regulation of NLRP3 in renal cell carcinoma. J. Nutr. Biochem. 85, 108489. doi:10.1016/j.jnutbio.2020.108489
Tomasetti, C., Li, L., and Vogelstein, B. (2017). Stem cell divisions, somatic mutations, cancer etiology, and cancer prevention. Science 355 (6331), 1330–1334. doi:10.1126/science.aaf9011
Tomoaia, G., Horovitz, O., Mocanu, A., Nita, A., Avram, A., Racz, C. P., et al. (2015). Effects of doxorubicin mediated by gold nanoparticles and resveratrol in two human cervical tumor cell lines. Colloids Surf. B Biointerfaces 135, 726–734. doi:10.1016/j.colsurfb.2015.08.036
Vergara, D., Simeone, P., Toraldo, D., Del Boccio, P., Vergaro, V., Leporatti, S., et al. (2012). Resveratrol downregulates Akt/GSK and ERK signalling pathways in OVCAR-3 ovarian cancer cells. Mol. Biosyst. 8 (4), 1078–1087. doi:10.1039/c2mb05486h
Vidoni, C., Ferraresi, A., Vallino, L., Salwa, A., Ha, J. H., Seca, C., et al. (2023). Glycolysis inhibition of autophagy drives malignancy in ovarian cancer: exacerbation by IL-6 and attenuation by resveratrol. Int. J. Mol. Sci. 24 (2), 1723. doi:10.3390/ijms24021723
Walle, T. (2011). Bioavailability of resveratrol. Ann. N. Y. Acad. Sci. 1215, 9–15. doi:10.1111/j.1749-6632.2010.05842.x
Walle, T., Hsieh, F., DeLegge, M. H., Oatis, J. E., and Walle, U. K. (2004). High absorption but very low bioavailability of oral resveratrol in humans. Drug Metab. Dispos. 32 (12), 1377–1382. doi:10.1124/dmd.104.000885
Wang, F., Zheng, A., Zhang, D., Zou, T., Xiao, M., Chen, J., et al. (2022). Molecular profiling of core immune-escape genes highlights LCK as an immune-related prognostic biomarker in melanoma. Front. Immunol. 13, 1024931. doi:10.3389/fimmu.2022.1024931
Wang, H., Peng, Y., Wang, J., Gu, A., Li, Q., Mao, D., et al. (2019). Effect of autophagy on the resveratrol-induced apoptosis of ovarian cancer SKOV3 cells. J. Cell. Biochem. 120 (5), 7788–7793. doi:10.1002/jcb.28053
Wang, H., Wang, S. S., Li, L. N., Wang, J., He, Y., Ma, C. X., et al. (2021). Preliminary study on reversal of drug resistance of resveratrol on human ovarian cancer cell line SKOV3/DDP. Sichuan Med. J. 42 (07), 664–668. doi:10.16252/j.cnki.issn1004-0501-2021.07.003
Wang, J., Jiang, C., Li, N., Wang, F., Xu, Y., Shen, Z., et al. (2020a). The circEPSTI1/mir-942-5p/LTBP2 axis regulates the progression of OSCC in the background of OSF via EMT and the PI3K/Akt/mTOR pathway. Cell Death Dis. 11 (8), 682. doi:10.1038/s41419-020-02851-w
Wang, J., Qi, F., Wang, Z., Zhang, Z., Pan, N., Huai, L., et al. (2020b). A review of traditional Chinese medicine for treatment of glioblastoma. Biosci. Trends 13 (6), 476–487. doi:10.5582/bst.2019.01323
Wang, L. J., and Shi, H. R. (2019). Resveratrol inhibits ovarian cancer cell growth and Wnt signaling pathway by regulating SIRT1. Chin. Tradit. Herb. Drugs 50 (03), 675–680. doi:10.7501/j.issn.0253-2670.2019.03.021
Washington, M. N., Suh, G., Orozco, A. F., Sutton, M. N., Yang, H., Wang, Y., et al. (2015). ARHI (DIRAS3)-mediated autophagy-associated cell death enhances chemosensitivity to cisplatin in ovarian cancer cell lines and xenografts. Cell Death Dis. 6 (8), e1836. doi:10.1038/cddis.2015.208
Wenzel, E., and Somoza, V. (2005). Metabolism and bioavailability of trans-resveratrol. Mol. Nutr. Food Res. 49 (5), 472–481. doi:10.1002/mnfr.200500010
Wu, M., Ma, L., Xue, L., Ye, W., Lu, Z., Li, X., et al. (2019). Resveratrol alleviates chemotherapy-induced oogonial stem cell apoptosis and ovarian aging in mice. Aging 11 (3), 1030–1044. doi:10.18632/aging.101808
Wu, Z., Wu, J., Zhao, Q., Fu, S., and Jin, J. (2020). Emerging roles of aerobic glycolysis in breast cancer. Clin. Transl. Oncol. 22 (5), 631–646. doi:10.1007/s12094-019-02187-8
Xiang, S., Li, J., Shen, J., Zhao, Y., Wu, X., Li, M., et al. (2021). Identification of prognostic genes in the tumor microenvironment of hepatocellular carcinoma. Front. Immunol. 12, 653836. doi:10.3389/fimmu.2021.653836
Xiao, Y., Qin, T., Sun, L., Qian, W., Li, J., Duan, W., et al. (2020). Resveratrol ameliorates the malignant progression of pancreatic cancer by inhibiting hypoxia-induced pancreatic stellate cell activation. Cell Transpl. 29, 963689720929987. doi:10.1177/0963689720929987
Xie, C., Liang, C., Wang, R., Yi, K., Zhou, X., Li, X., et al. (2023). Resveratrol suppresses lung cancer by targeting cancer stem-like cells and regulating tumor microenvironment. J. Nutr. Biochem. 112, 109211. doi:10.1016/j.jnutbio.2022.109211
Yan, X., Zhou, R., and Ma, Z. (2019). Autophagy-cell survival and death. Adv. Exp. Med. Biol. 1206, 667–696. doi:10.1007/978-981-15-0602-4_29
Yang, Q., Chen, Y., Guo, R., Dai, Y., Tang, L., Zhao, Y., et al. (2021). Interaction of ncRNA and epigenetic modifications in gastric cancer: focus on histone modification. Front. Oncol. 11, 822745. doi:10.3389/fonc.2021.822745
Yao, S., Gao, M., Wang, Z., Wang, W., Zhan, L., and Wei, B. (2021). Upregulation of MicroRNA-34a sensitizes ovarian cancer cells to resveratrol by targeting bcl-2. Yonsei Med. J. 62 (8), 691–701. doi:10.3349/ymj.2021.62.8.691
Ye, Z., Hu, J., Wang, J., Liu, Y. N., Hu, G. X., and Xu, R. A. (2023). The effect of Resveratrol on the pharmacokinetic profile of tofacitinib and the underlying mechanism. Chem. Biol. Interact. 374, 110398. doi:10.1016/j.cbi.2023.110398
Yin, D., Chen, C. X., Min, M. J., Zhao, F., Du, L., Yin, X. X., et al. (2019). Inhibitory effect of resveratrol on MNNG-induced endometrial carcinogenesis based on estrogen metabolism regulation. Chin. Pharmacol. Bull. 35 (07), 922–928. doi:10.3969/j.issn.1001-1978.2019.07.008
Yin, L., Zhang, R., Hu, Y., Li, W., Wang, M., Liang, Z., et al. (2020). Gastric-cancer-derived mesenchymal stem cells: a promising target for resveratrol in the suppression of gastric cancer metastasis. Hum. Cell 33 (3), 652–662. doi:10.1007/s13577-020-00339-5
Yue, X. D., Li, X. J., Liu, Q., and Yang, J. L. (2019). Resveratrol elevates the chemosensitivity of cervical cancer cels by inducing cel cycle arest and apoptosis. J. Xi'an Jiaot. Univ. Med. Sci. 40 (05), 736–741. doi:10.7652/jdyxb201905014
Zhang, A., Miao, K., Sun, H., and Deng, C. X. (2022). Tumor heterogeneity reshapes the tumor microenvironment to influence drug resistance. Int. J. Biol. Sci. 18 (7), 3019–3033. doi:10.7150/ijbs.72534
Zhang, C., Liu, J., Xu, D., Zhang, T., Hu, W., and Feng, Z. (2020a). Gain-of-function mutant p53 in cancer progression and therapy. J. Mol. Cell Biol. 12 (9), 674–687. doi:10.1093/jmcb/mjaa040
Zhang, Q., Huang, H., Zheng, F., Liu, H., Qiu, F., Chen, Y., et al. (2020b). Resveratrol exerts antitumor effects by downregulating CD8(+)CD122(+) Tregs in murine hepatocellular carcinoma. Oncoimmunology 9 (1), 1829346. doi:10.1080/2162402x.2020.1829346
Zhang, S., Cao, H. J., Davis, F. B., Tang, H. Y., Davis, P. J., and Lin, H. Y. (2004). Oestrogen inhibits resveratrol-induced post-translational modification of p53 and apoptosis in breast cancer cells. Br. J. Cancer 91 (1), 178–185. doi:10.1038/sj.bjc.6601902
Zhang, S. Y., Song, X. Y., Li, Y., Ye, L. L., Zhou, Q., and Yang, W. B. (2020c). Tumor-associated macrophages: a promising target for a cancer immunotherapeutic strategy. Pharmacol. Res. 161, 105111. doi:10.1016/j.phrs.2020.105111
Zhang, Y., Ma, Y. L., Fan, L., Wang, R. X., Li, Z. Y., and Yue, Y. (2020d). Effects of resveratrol on tumor growth, oncogene and HGF/c - met expression in ovarian cancer - bearing rats. J. Clin. Exp. Med. 19 (12), 1275–1278. doi:10.3969/j.issn.1671-4695.2020.12.013
Zhang, Y., Yang, S., Yang, Y., and Liu, T. (2019). Resveratrol induces immunogenic cell death of human and murine ovarian carcinoma cells. Infect. Agents Cancer 14, 27. doi:10.1186/s13027-019-0247-4
Zhao, H., Jiang, Y., Liu, Y., Yun, C., and Li, L. (2015). Endogenous estrogen metabolites as biomarkers for endometrial cancer via a novel method of liquid chromatography-mass spectrometry with hollow fiber liquid-phase microextraction. Horm. Metab. Res. 47 (2), 158–164. doi:10.1055/s-0034-1371865
Zhao, Q., Jiang, Y., Xiang, S., Kaboli, P. J., Shen, J., Zhao, Y., et al. (2021). Engineered TCR-T cell immunotherapy in anticancer precision medicine: pros and cons. Front. Immunol. 12, 658753. doi:10.3389/fimmu.2021.658753
Zhao, X., Tong, W., Song, X., Jia, R., Li, L., Zou, Y., et al. (2018). Antiviral effect of resveratrol in piglets infected with virulent pseudorabies virus. Viruses 10 (9), 457. doi:10.3390/v10090457
Zhao, Y., Yuan, X., Li, X., and Zhang, Y. (2019). Resveratrol significantly inhibits the occurrence and development of cervical cancer by regulating phospholipid scramblase 1. J. Cell. Biochem. 120 (2), 1527–1531. doi:10.1002/jcb.27335
Zhong, X. Y., Yuan, X. M., Xu, Y. Y., Yin, M., Yan, W. W., Zou, S. W., et al. (2018). CARM1 methylates GAPDH to regulate glucose metabolism and is suppressed in liver cancer. Cell Rep. 24 (12), 3207–3223. doi:10.1016/j.celrep.2018.08.066
Glossary
Keywords: resveratrol, gynecological tumors, malignant tumors, antitumor mechanisms, apoptosis
Citation: Yang Q, Meng D, Zhang Q and Wang J (2024) Advances in the role of resveratrol and its mechanism of action in common gynecological tumors. Front. Pharmacol. 15:1417532. doi: 10.3389/fphar.2024.1417532
Received: 15 April 2024; Accepted: 26 June 2024;
Published: 17 July 2024.
Edited by:
Antonella D'Anneo, University of Palermo, ItalyReviewed by:
Debasish Bandyopadhyay, The University of Texas Rio Grande Valley, United StatesTao Yi, Hong Kong Baptist University, Hong Kong, SAR China
Maulana Yusuf Alkandahri, Universitas Buana Perjuangan Karawang, Indonesia
Copyright © 2024 Yang, Meng, Zhang and Wang. This is an open-access article distributed under the terms of the Creative Commons Attribution License (CC BY). The use, distribution or reproduction in other forums is permitted, provided the original author(s) and the copyright owner(s) are credited and that the original publication in this journal is cited, in accordance with accepted academic practice. No use, distribution or reproduction is permitted which does not comply with these terms.
*Correspondence: Jin Wang, zywangjin@163.com