- 1School of Health Preservation and Rehabilitation, Chengdu University of Traditional Chinese Medicine, Chengdu, Sichuan, China
- 2Institute of Traditional Chinese Medicine Health Industry, China Academy of Chinese Medical Sciences, Nanchang, China
- 3Jiangxi Province Key Laboratory of Traditional Chinese Medicine Pharmacology, Nanchang, China
- 4College of Material Science and Chemical Engineering, Southwest Forestry University, Kunming, Yunnan, China
- 5Center for Quality Evaluation and Research in Higher Education, Nanjing University of Chinese Medicine, Nanjing, China
- 6Department of Pharmacological and Pharmaceutical Sciences, College of Pharmacy, University of Houston, Houston, TX, United States
Vascular smooth muscle cells (VSMCs) are integral to the pathophysiology of cardiovascular diseases (CVDs). Enhancer of zeste homolog 2 (EZH2), a histone methyltransferase, plays a crucial role in epigenetic regulation of VSMCs gene expression. Emerging researches suggest that EZH2 has a dual role in VSMCs, contingent on the pathological context of specific CVDs. This mini-review synthesizes the current knowledge on the mechanisms by which EZH2 regulates VSMC proliferation, migration and survival in the context of CVDs. The goal is to underscore the potential of EZH2 as a therapeutic target for CVDs treatment. Modulating EZH2 and its associated epigenetic pathways in VSMCs could potentially ameliorate vascular remodeling, a key factor in the progression of many CVDs. Despite the promising outlook, further investigation is warranted to elucidate the epigenetic mechanisms mediated by EZH2 in VSMCs, which may pave the way for novel epigenetic therapies for conditions such as atherosclerosis and hypertension.
1 Introduction
Cardiovascular diseases (CVDs) have long posed a significant global health challenge, characterized by for high incidence, disability, and mortality rates. According to the World Health Statistics Report published by the World Health Organization in 2017, CVDs accounted for a staggering 17.7 million deaths globally in 2015, representing 44% of all deaths worldwide. This figure includes more than half of total deaths in European region (Machado et al., 2020). Despite advancements in existing treatments that have improved patient quality of life, the morbidity and mortality associated with CVDs continue to rise in correlation with societal progress and changes in human lifestyles. Thus, the pursuit of new therapeutic targets is both urgent and critical.
Vascular smooth muscle cells (VSMCs) are predominantly located within the vessel wall and are essential for regulating vascular contraction, thus maintaining vascular homeostasis. In response to vascular injury or stimulation by bioactive substances such as nitric oxide products, angiotensin II (Ang II), and platelet-derived growth factor, VSMCs proliferate and migrate. This process is a key mechanism underlying blood vessel wall thickening, lumen narrowing, and vascular remodeling (Ren et al., 2017; Zhang et al., 2018; Wang et al., 2019; Wang et al., 2019; Yuan et al., 2020). VSMCs can also undergo phenotypic changes in various environmental contexts, which significantly impact the development of atherosclerosis (AS) (Basatemur et al., 2019). During vascular injury and inflammation, VSMCs proliferate and migrate to form a fibrous cap, eventually invading the core of the plaque, thereby advancing AS (Misra et al., 2018). Consequently, the phenotype of VSMCs, influenced by the immune milieu, plays a crucial role in AS etiology and subsequent CVDs (Ramel et al., 2019). The apoptosis of VSMCs, contributing to fibrous cap and plaque instability, activation of calcification (Proudfoot et al., 2000) and immune system (Schrijvers et al., 2005), further exacerbate AS development. Additionally, abnormal VSMC proliferation, migration, and apoptosis are pivotal in other CVDs, including hypertension, aortic dissection (AD), aortic aneurysm (AA), and coronary heart disease (CHD) (Wang and Chen, 2020). These insights underscore the critical role of VSMCs in the pathophysiology of CVDs and provide new perspectives for understanding and treating these diseases.
Enhancer of zeste homolog 2 (EZH2) is a methyltransferase from the Polycomb gene family, significantly impacting VSMC proliferation and differentiation. EZH2 regulates these processes by modifying histone methylation on microRNAs and the N-terminal tail of the 27th amino acid, lysine, on core histone H3 (H3K27me3) (Zhang et al., 2021; Li et al., 2022; Zhong et al., 2022). Furthermore, EZH2 influences VSMC contraction and relaxation by modulating the expression of critical genes such as those encoding calcium channels and actin. Inhibitors of EZH2 have shown considerable potential in enhancing vascular function and treating CVDs, including hypertension and AS. Therefore, a comprehensive understanding of the mechanisms underlying EZH2-targeted regulation of VSMC function in CVDs is imperative for developing novel therapeutic strategies. This mini-review aims to summarize the research progress on EZH2-mediated epigenetic modifications in VSMCs in the context of CVDs, providing a reference for the development of EZH2-targeted therapies.
2 CVDs from the EZH2 perspective
2.1 Structure and function of EZH2
EZH2 is a key component of the polycomb repressive complex 2 (PRC2) (Heng et al., 2023). It interacts with several proteins, including suppressor of zeste 12 (SUZ12), embryonic ectoderm development protein (EED), retinoblastoma-binding protein 4/7 (RBBP4/7), and adipocyte enhancer-binding protein 2 (AEBP2), forming PRC2 through domains WD-40, I, and II. This complex plays a critical role in initiating transcriptional repression. The human EZH2 gene is located in the q35 region of chromosome 7 and consists of 20 exons encoding 747 amino acids (Pingping et al., 2022). EZH2 comprises five primary domains: 1) WD-40 binding domain, which mediates EED binding; 2) Domains I and II, in which domain I binds to PHF1 and domain Ⅱ binds to SUZ12; 3) two SANT domains, which involve in recruiting other chromatin remodeling factors for histone binding; 4) The cysteine-rich domain (CXC), which influences HMTase activity and participates in EZH2 stability and subcellular localization; 5) The SET domain, which is a conserved catalytic domain at the C-terminal, catalyzing the production of mono-, di-, and trimethylated H3K27 (H3K27me1, H3K27me2, and H3K27me3) (Pingping et al., 2022; Heng et al., 2023) (Figure 1).
Initially, studies focused on EZH2-mediated gene silencing through its histone methyltransferase activity in a PRC2-dependent manner (Heng et al., 2023). By trimethylating the lysine 27 site of histone H3 to form the H3K27me3, EZH2 regulates various physiological and pathological processes, including cell proliferation, differentiation, aging and tumorigenesis. Recent research has unveiled that EZH2 is a multifunctional molecule. At transcriptional level, EZH2 can modify non-histone proteins via methylation, thereby altering their functions. Additionally, EZH2 can act as a protein scaffold, recruiting different transcription factors to form large complexes that influence their transcriptional activity (Pingping et al., 2022). Beyond transcriptional regulation, EZH2 also regulates cell migration, activates signaling pathways (Adamik et al., 2020), and interacts with RNA (Kaneko et al., 2010), independently of transcriptional functions. Today, EZH2 is recognized for its significant regulatory roles in various biological events, including cardiovascular development, tumorigenesis and metastasis, and degenerative aging (Mahara et al., 2016; Yuan et al., 2021; Chao et al., 2022; Pingping et al., 2022).
2.2 Development of atherosclerosis from the EZH2 perspective
Atherosclerosis (AS) is a typical CVDs characterized by chronic inflammatory vascular lesion (Herrington et al., 2016). The proliferation, migration, apoptosis, and phenotypic transformation of VSMCs are the critical processes driving AS development (Bennett et al., 2016; Wu et al., 2017; Wang et al., 2019). The histone methyltransferase EZH2 in VSMCs plays a significant role in the pathogenesis of AS by affecting epigenetic regulation. Investigating EZH2 in VSMCs offers new perspectives on the pathophysiology and treatment of AS.
During AS formation, vascular injury is a crucial factor in initiating lesion development. Studies have shown that vascular injury increases the expression of EZH2 and H3K27me3 in smooth muscle cells of carotid arteries. Growth medium and platelet-derived growth factor BB also enhance the expression of EZH2 and H3K27me3 in cultured VSMCs (Liang et al., 2019). This process inhibits the expression of VSMCs-specific marker smooth muscle protein 22-α (SM-22α) (Liang et al., 2019) affecting the maintenance of VSMCs phenotype and resulting in proliferative and migratory phenotypes. This phenotype switch promotes neointima formation and exacerbates AS development (Liu et al., 2016). SM-22α knockout mice, which lack this key phenotype maintenance factor, are more prone to phenotypic switching, leading to an increased incidence of AS (Shen et al., 2010). These findings suggest that EZH2 upregulation is involved in injury-triggered VSMCs phenotypic transformation promoting AS development by affecting the expression of H3K27me3 and SM-22α.
Inflammatory responses are another key factor in AS formation. EZH2-regulated signaling pathways play an important role in this process. Specifically, expression of EZH2 is upregulated in inflammatory environments, leading to increased H3K27me3 levels, which suppress the expression of SM-22α. Conversely, the anti-inflammatory effect of the histone deacetylase Silent Information Regulator 1 (SIRT1) is dependent on the presence of SM-22α. Thus, the upregulation of EZH2 inhibits the anti-inflammatory function of SIRT1. In turn, SIRT1 can remove the acetyl modification of SM-22α by EZH2 through deacetylation, thereby increasing SM-22α expression and exerting anti-inflammatory effects (Shu et al., 2017). This EZH2-SIRT1-SM-22α regulatory loop is crucial in the onset and progression of AS. Overall, EZH2 in VSMCs promotes AS development by influencing cell proliferation, migration, apoptosis, and phenotypic transformation during vascular injury and inflammation.
The microRNA miR-139-5p is a highly conserved small non-coding RNA that mediates the response of mRNA transcripts to various signaling pathways in cells. Its direct target gene, signal transducer and activator of transcription (STAT1), can reduce angiogenesis and thus inhibit AS development (Zheng et al., 2021). In VSMCs, EZH2 can bind to H3K27me3, the promoter of miR-139-5p, down-regulating miR-139-5p expression and increasing STAT1 signaling expression, as verified in arterial tissues of AS patients (Zheng et al., 2021). Thus, EZH2 can influence AS development by regulating miR-139-5p/STAT1 signaling pathway.
Moreover, proprotein convertase subtilisin/kexin type 9 (PCSK9), a serine protease produced by the liver, has been shown to be involved in the development of dyslipidemia and CVDs caused by AS (Ragusa et al., 2021; Katsuki et al., 2022). PCSK9 inhibitors are used for the treatment of AS or other CVDs. Small nucleolar RNA Host Gene 16 (SNHG16), a downstream effector of PCSK9, promotes VSMC proliferation, migration, and foam cell formation, and this effect can be reversed by TNF receptor associated factor 5 (TRAF5). SNHG16 inhibits the reversal pathway of TRAF5 by recruiting EZH2, which promotes AS development (Liu et al., 2023). Therefore, EZH2 plays a crucial role in SNHG16-mediated TRAF5-regulated VSMC proliferation, migration and foam cell formation pathway, further influencing AS progression.
In advanced AS and vascular inflammation, the pro-fibrotic molecule cellular communication network factor 2 (CCN2) is highly expressed, and its expression is further upregulated by EZH2 under hypoxic conditions. In contrast, the polymerase delta-interacting protein 2 (Poldip2) inhibits CCN2 expression, but itself is inhibited by EZH2 under hypoxic conditions (Paredes et al., 2023). Thus, EZH2 can inhibit Poldip2 expression, increase CCN2 expression, and exacerbate fibrosis caused by AS under hypoxic conditions. Inhibition of EZH2 can mitigate AS development by ensuring the normal expression of Poldip2 and its downstream CCN2.
Additionally, the circHECTD1 gene promotes VSMC proliferation and migration (Feng et al., 2023). Silencing EZH2 in VSMCs can reverse the proliferation enhancement effect of circHECTD1 on VSMCs. As an important histone modification regulator, EZH2 can be specifically targeted to inhibit circHECTD1 and reduce atherosclerotic plaque formation (Feng et al., 2023). Conversely, mechanical stretch (Zhong et al., 2022) and miR-630 overexpression (Miao et al., 2022) can inhibit EZH2 expression in VSMCs, reducing atherosclerotic lesions (Figure 2). Overall, EZH2 is involved in the pathogenesis of AS through multiple upstream and downstream pathways. Focusing on EZH2 targets in VSMCs holds promising prospects for exploring new directions for AS treatment.
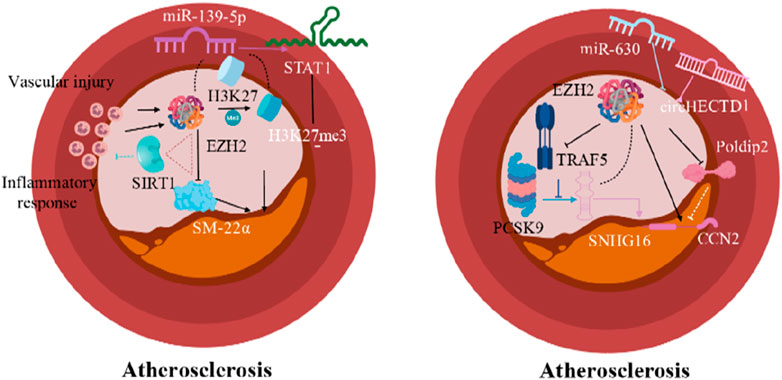
Figure 2. Epigenetic modification mechanisms of EZH2 in AS (Arrows indicate facilitation of this molecular mechanism, and horizontal lines indicate inhibition of this molecular mechanism).
2.3 Development of hypertension from the EZH2 perspective
Hypertension is a chronic diseases characterized by endothelial dysfunction, increased vasoconstriction, abnormal proliferation and migration of VSMCs and vascular remodeling (Intengan and Schiffrin, 2000; Savoia et al., 2011; Montezano et al., 2015). Vascular remodeling is persistent throughout the course of hypertension (Hayashi and Naiki, 2009) and serve as an important indicator of the hypertensive condition (Bakker et al., 2005). Dysfunction of VSMCs plays a key role in vascular remodeling. EZH2 influences the development of hypertension by regulating VSMC proliferation, migration and phenotypic transition. Therefore, an in-depth study of the mechanism of EZH2 in VSMCs dysfunction is necessary for better understand and treat hypertension.
MicroRNAs are known to regulate VSMC proliferation, migration and differentiation, processes highly correlated with vascular remodeling (Chen et al., 2018; Chen et al., 2018; Wang and Atanasov, 2019; Wang et al., 2019). For example, miR-26a can regulate EZH2 expression and play a protective role in vascular health (Zhang et al., 2021). Targeting EZH2 via modulating miR-26a expression can reduce VSMC proliferation and inhibit vascular remodeling in hypertension.
The vascular remodeling caused by abnormal VSMC proliferation and migration is also a critical event in the process in the onset and progression of pulmonary arterial hypertension (PAH) (Archer et al., 2010; Gladwin and Ghofrani, 2010), leading to vascular obstruction and destruction that adversely affect lung and heart function. Experimental studies have shown that EZH2 overexpression enhances proliferation, migration and apoptosis resistance of pulmonary artery VSMCs in the mice with hypoxia-mediated PAH (Aljubran et al., 2012; Saco et al., 2014). This suggests that EZH2 is an effective regulator of pulmonary artery VSMC homeostasis, with disruption in its expression influencing PAH development. Moreover, EZH2 and its downstream effectors could serve as therapeutic targets for combating pulmonary vascular remodeling (Habbout et al., 2021). Superoxide dismutase 2 (SOD2) deficiency, linked to PAH through redox homeostasis disruption and VSMC phenotypic transformation, is also influenced by EZH2 (Archer et al., 2010; Wang et al., 2021). Upregulation of EZH2 exacerbates PAH by promoting the transformation of pulmonary VSMC into a proliferative phenotype, thereby worsening vascular remodeling and hemodynamic changes in SOD2 deficiency mice (Wang et al., 2021). This implies a connection between EZH2’s role in VSMC phenotype transition and redox signaling (Wang et al., 2021).
Bone morphogenetic protein receptor type 2 (BMPR2) belongs to a family of genes involved in cell growth and differentiation (Soon et al., 2015; Orriols et al., 2017). Loss of BMPR2 function exacerbates PAH (Liu et al., 2017) with most PAH patients exhibiting significantly reduced BMPR2 expression (Atkinson et al., 2002; Morrell, 2010; Rabinovitch, 2012). Soon et al. demonstrated that BMPR2 deficiency promotes PAH development by decreasing superoxide dismutase 3 (SOD3) expression and enhancing inflammatory responses. Recent findings indicate that EZH2 interacts with switch-independent 3a (SIN3a) to regulate BMPR2 in human pulmonary artery VSMCs, impacting PAH development. SIN3a deficiency promotes proliferation and migration of pulmonary artery VSMCs, while its overexpression counteracts these effects. Specifically, overexpression of SIN3a reduces methylation of the BMPR2 promoter region in human pulmonary artery VSMCs, activating BMPR2 transcription (Soon et al., 2015). This suggests that SIN3a overexpression can inhibit EZH2-mediated proliferation of human pulmonary artery VSMCs. Since EZH2 suppresses target gene expression via H3K27me3 catalysis, SIN3a antagonizes EZH2’s function. Thus, the epigenetic regulation between SIN3a and EZH2 represent a critical node in controlling pulmonary artery VSMCs’s phenotype switch and growth. SIN3a modulates BMPR2 DNA methylation and expression by regulating EZH2 levels and decreasing H3K27me3 content. While EZH2 inhibits BMPR2 expression through H3K27me3, SIN3a opposes this function, upregulates BMPR2, and mitigates PAH (Bisserier et al., 2021). Therefore, targeting the combination of SIN3a and EZH2 could represent a novel therapeutic strategy for PAH.
2.4 Development of aortic dissection from the EZH2 perspective
Aortic dissection (AD) is a life-threatening cardiovascular emergency caused by a tear in the aorta’s lining or bleeding within the aortic wall, leading to the separation of its layers (Nienaber et al., 2016; Gawinecka et al., 2017). The key pathological features in AD patients include media degeneration, characterized by elastic fibers breakage and loss, VSMCs loss, and accumulation of mucopolysaccharides (Larson and Edwards, 1984; Zhu et al., 2006; Jiang et al., 2016). VSMC cycle arrest, apoptosis, necrosis and autophagic cell death are potential causes of VSMC loss in the aortic wall (Huang et al., 2015; Jia et al., 2015; Wang et al., 2016). Epigenetic regulatory factors present in VSMCs can effectively manage these processes.
Studies have shown that alkylation repair homologous protein 5 (ALKBH5), a key m6A demethylase, is highly expressed in the aortic tissue of AD patients, suggesting m6A modification’s involvement in AD progression (Wang et al., 2021). ALKBH5 exacerbates Ang-II-induced inflammatory responses and apoptosis in human aortic VSMCs. Long non-coding RNA (lncRNA)-TMPO-AS1, a downstream target of ALKBH5, affects AD progression partly by binding with EZH2 to epigenetically regulate interleukin-1 receptor-associated kinase 4 (IRAK4). EZH2 downregulates IRAK4, reducing ALKBH5 expression and alleviating AD disease (Wang et al., 2021). DNA methylome analysis reveals hypomethylation of EZH2 targets and overexpression of retinoic acid receptor alpha gene in AD patients (Pan et al., 2017). Additionally, cytosolic DNA through stimulator of interferon response CGAMP interactor 1 (STING-1) and interferon regulatory factor 3 (IRF3) signaling recruits EZH2, inducing H3K27me3 modification and driving VSMCs from a contractile to an inflammatory phenotype during AD formation (Chakraborty et al., 2023). Therefore, targeting EZH2 in the regulatory network for AD progression is promising for AD intervention.
Moderate autophagy is crucial for VSMC proliferation, migration, apoptosis and vascular remodeling (Grootaert et al., 2018), but excessive autophagy leads to VSMC loss. Inhibition or knockdown of EZH2 induces cell cycle arrest and autophagic cell death via MEK-ERK1/2 signaling pathway, contributing to VSMC loss and AD development, while EZH2 overexpression promotes proliferation and reduces autophagic cell death. Thus, EZH2 affects AD development by regulating VSMC autophagy (Li et al., 2018). Therefore, enhancing EZH2 function could be explored to reduce AD occurrence.
2.5 Development of AAA and TAA from the EZH2 perspective
EZH2 is also closely related to the occurrence and development of abdominal aortic aneurysm (AAA) and thoracic aortic aneurysm (TAA). Both AAA and TAA involve structural and functional disorders of the aorta, increasing the risk of aortic wall dilation and rupture. VSMCs dysfunction and pathological processes such as inflammation, immune response and extracellular matrix remodeling contribute to AAA formation (Wortmann et al., 2019). lncRNAs regulate VSMC proliferation, migration, and apoptosis, affecting AAA development (Wu et al., 2020). For example, lncRNAs like GAS5, H19, LCC-HLTF-5, and HIF1-α-AS1 are implicated in AAA development (Kumar et al., 2019). Retinal acid-induced gene I (RIG-I) gain-of-function variation leads to aortic and coronary artery calcification (Jang et al., 2015). EZH2 inhibits VSMC apoptosis by suppressing RIG-I signaling pathway independent of methylation, while GAS5 may promote VSMC apoptosis by inhibiting EZH2 expression (Le et al., 2021). Therefore, GAS5 may regulate AAA development through the EZH2/RIG-I axis, promoting apoptosis and accelerating the AAA development by inhibiting EZH2 expression.
Contractile proteins are crucial for VSMC cytoskeleton maintenance. Defects in contractile protein expression are identified TAA development (Cardenas et al., 2018). SM-22α, specifically expressed in contractile VSMCs, is essential for maintaining the VSMC contractile phenotype. SM-22α expression negatively correlates with aneurysm size, and EZH2 inhibition can enhance SM-22α expression. Targeting EZH2 with the small molecule inhibitor like GSK343 improves aortic function in Fbn1C1039G/+ mice by restoring contractile protein expression (Cardenas et al., 2018). Thus, VSMC contractile proteins play a key role in the maintaining aortic homeostasis and are potential targets for epigenetic modification in thoracic aortic disease (Cardenas et al., 2018). EZH2 maintains VSMC lineage and properties in TAA (Wang et al., 2010). Inhibiting EZH2 more effectively expresses VSMCs contractile proteins (Figure 3), presenting new therapeutic pathways for TAA and insights into clinical epigenetic events. Overall, EZH2 plays a significant role in AAA and TAA development, offering potential research and clinical applications as a therapeutic target.
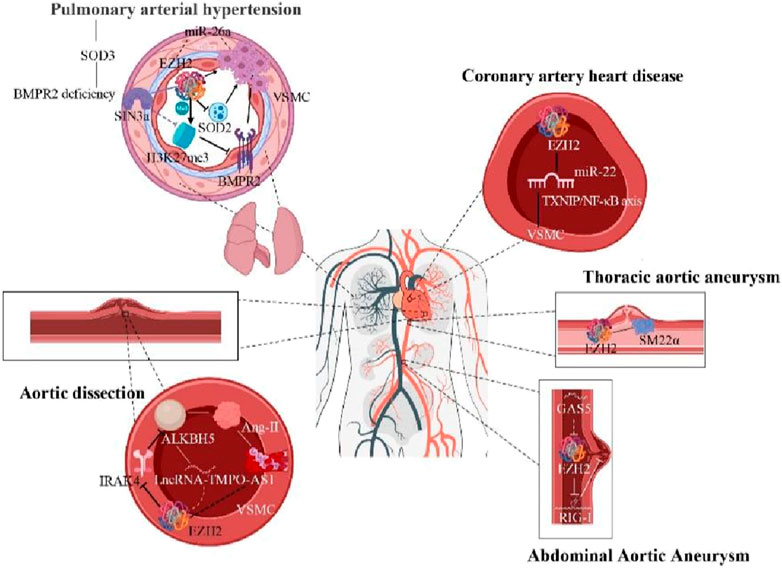
Figure 3. Epigenetic modification mechanisms of EZH2 in hypertension, AD, CHD, AAA, and TAA (Arrows indicate facilitation of this molecular mechanism, and horizontal lines indicate inhibition of this molecular mechanism).
2.6 Development of other CVDs from the EZH2 perspective
Coronary heart disease (CHD), or ischemic heart disease, results from myocardial ischemia and hypoxia due to coronary atherosclerosis. VSMC dysfunction significantly contributes to CHD pathogenesis. Downregulation of miR-22 in peripheral blood mononuclear cells corelates with CHD in patients (Chen et al., 2016). Overexpression of miR-22 reduces VSMC proliferation and migration, thereby inhibiting CHD development (Liu et al., 2020). EZH2 inhibits miR-22 transcription, leading to an imbalance in the TXNIP/NF-κB axis, a classical pathway contributes to CHD development (Liu et al., 2020). Thus, EZH2 can affect TXNIP/NF-κB signaling pathway by regulating miR-22 expression, offering a new potential therapeutic target for CHD treatment.
3 Discussion
EZH2 has garnered significant attention as a therapeutic target in epigenetics for various diseases. However, its regulatory role in VSMCs in CVDs is often overlooked. As an epigenetic regulator, EZH2 in VSMCs is crucial for maintaining the balance of several signaling pathways in CVDs.
This review discusses the recent advances in understanding the role of EZH2 in preventing and treating CVDs by modulating the proliferation, migration and apoptosis of VSMCs. From an epigenetic perspective, EZH2 emerges as a potential target for CVDs therapy. Our analysis indicates that EZH2 exerts a bidirectional regulatory effect on the pathogenesis and progression of CVDs by affecting the signaling pathways within VSMCs.
Despite the varied drivers of VSMCs dysfunction, abnormal proliferation and migration processes are strongly linked to EZH2 expression. We propose that EZH2 inhibitor could serve as therapeutic strategies for conditions like restenosis after angioplasty, atherosclerosis, and vein graft intimal thickening by inhibiting post-surgical VSMC proliferation and migration, thereby reducing restenosis incidence. Preclinical studies have shown that the EZH2 inhibitor UNC1999 significantly suppresses PDGF-BB-induced VSMC proliferation and neointimal hyperplasia (Liang et al., 2019). VSMCs’ ability to dedifferentiate and switch phenotypes in response to environmental stimuli is critical hypertension pathophysiology (Liu et al., 2015). This phenotypic switch can be regulated by epigenetic modifications (Liu et al., 2015; Levy et al., 2017). Increased EZH2 expression promotes hyperproliferation and an anti-apoptotic phenotype in PAH-PASMC through both classical and non-classical mechanisms (Habbout et al., 2021). Another preclinical study demonstrated that the EZH2 inhibitor EPZ005687 ameliorates PAH and improves cardiovascular function in mice (Shi et al., 2018). Hence, targeting EZH2 in VSMCs maybe a promising approach for treating diseases like AS and hypertension characterized by abnormal VSMC proliferation, migration and vascular remodeling.
Conversely, EZH2 is also vital for VSMCs survival. The pathophysiological of AD is complex with VSMC dysfunction playing a crucial role (Liu et al., 2022). Studies have shown that EZH2 reduces autophagic cell death in aortic VSMCs by inhibiting autophagosomes formation through ATG5 and ATG7 repression, thereby supporting VSMC survival during AD (Li et al., 2018). Epigenetic modification-mediated VSMC dysfunction and extracellular matrix degradation are common in TAA and AAA (Michel et al., 2018). EZH2 overexpression promotes PASMC proliferation and migration while reducing apoptosis (Aljubran et al., 2012). EZH2 knockdown leads to VSMC loss (Li et al., 2018), which can accelerate AAA development (Siasos et al., 2015). Moreover, EZH2 overexpression can mitigate AAA progression by increasing H3K27me3 levels at annexin A6 (ANXA6) promoter, reducing ROS levels and VSMC senescence induced by Ang II (Li et al., 2022). Multiple gene products, particularly the VSMC contractile protein SM-22α, are deficient human and mouse TAA samples, and its loss is a directly linked to aneurysm progression and AD in vivo. EZH2 inhibition can improve SM-22α expression, potentially benefiting TAA conditions (Cardenas et al., 2018). Additionally, the activation of the STING-IRF3-EZH2 axis drives VSMCs from a contractile to an inflammatory phenotype during AD formation (Chakraborty et al., 2023). Thus, enhancing EZH2 function in VSMCs could be explored as a treatment for vascular diseases characterized by VSMC loss, such as AD, TAA and AAA.
Reprogramming somatic cells into induced cardiomyocytes (iCMs) is a promising regenerative medicine strategy for CVDs. Studies have shown that EZH2 acts as an epigenetic barrier during human iCMs reprogramming, with its inhibition leading to decreased H3K27me3 occupancy and activation of cardiac genes. However, this inhibition also reduces human iCMs incidence, conflicting with heart reprogramming goals (Tang et al., 2021). EZH2 is essential for epicardial cell migration by suppressing tissue inhibitor of metalloproteinase 3 (TIMP3), playing a critical role in cardiac development (Jiang et al., 2023).
While EZH2 in VSMCs is potential target for CVDs treatment, its mechanism and clinical applications require further investigation. Most studies have focused on animal models, with few using human cells. There is lack of experiments with EZH2-specific knock-out or knock-in in smooth muscle tissue to verify its role in disease. Consequently, significant challenges remain before clinical application.
As a single epigenetic regulator influencing multiple genes and pathways, EZH2 is an attractive target for complex diseases. However, many epigenetic modifying enzymes involved in diseases development remain unexplored, with unclear mechanisms. A better understanding of EZH2’s specific functions and regulatory mechanisms in cardiovascular system is necessary. Current research has primarily focused on EZH2’s role in VSMCs, with limited investigation in other cardiovascular cell types. Studies on the effects of EZH2-targeted therapy on different cell types and tissues will enhance our understanding of its role in CVDs and mitigate adverse effects from global EZH2 inhibition. For instance, EZH2 ablation in murine hearts causes various cardiovascular malformations and perinatal death, highlighting its crucial role in cardiovascular development (Chen et al., 2012). Additionally, EZH2 inhibition can affect hematopoietic and muscle stem cells, causing issues like blood disorder and impaired muscle repair (Juan et al., 2011; Mochizuki-Kashio et al., 2015). EZH2 inhibitor GSK-343 enhances human mononuclear cell reparative function post-myocardial infarction, thereby preventing infarct size expansion and cardiac dysfunction, by resolving H3K27 methylation and promoting inflammation resolution (Rondeaux et al., 2023). However, EZH2 inhibitor GSK126 increases vascular stiffness and elastin degradation, highlighting potential adverse cardiovascular effects (Ibarrola et al., 2024). Therefore, specific targeted inhibitors should be chosen for EZH2-targeted CVD therapy to minimize adverse effects.
Developing novel EZH2 inhibitors is crucial for improving therapy efficacy. Inhibition of EZH2 by UNC1999 significantly suppressed PDGF-BB-induced VSMC proliferation and neointima formation (Liu et al., 2020). In another study, PCSK9 inhibitor PEP2-8 trifluoracetate attenuates AS by regulating SNHG16/EZH2/TRAF5-mediated VSMC proliferation, migration and foam cell formation (Liu et al., 2023). Some EZH2 inhibitors, like DS-3201b, are in phase 2 clinical trials for solid tumors (Izutsu et al., 2023). Traditional Chinese medicines have demonstrated unique advantages in managing CVDs, and screening for EZH2 inhibitor among them is a feasible strategy (Li et al., 2020; Zhao et al., 2020; Wang et al., 2021; Li et al., 2022; Wu et al., 2022; Hou et al., 2024; Qi et al., 2024; Wang et al., 2024; Zhao et al., 2024). In personalized medicine, EZH2 can serve as a breakthrough point for individualized CVD treatment, enabling accurate diagnosis and treatment based on related biomarkers. Therefore, more preclinical studies and clinical trials are necessary to evaluate the potential application of EZH2 inhibitors CVDs. Current EZH2 inhibitors exhibit varying degrees of side effects and drug resistance (Duan et al., 2020; Adema and Colla, 2022). Consequently, further research on the three-dimensional structure and function of EZH2, as well as the screening for more selective and effective EZH2 inhibitors, is essential to provide better options for clinical application.
In conclusion, EZH2 plays a significant role in VSMCs proliferation, migration and survival. Despite its potential as a target for CVD treatment, many unresolved issues require further research. Future work should focus on elucidating EZH2’s mechanism, developing individualized treatment strategies, and creating novel inhibitors to advance CVD treatment and prevention. This will enhance our understanding and therapeutic capabilities, improving patient quality of life and reducing the global burden of CVDs.
Author contributions
HL: Writing–original draft. YL: Funding acquisition, Writing–original draft. HS: Formal Analysis, Writing–original draft. KZ: Formal Analysis, Supervision, Writing–review and editing. WL: Formal Analysis, Investigation, Writing–review and editing. HH: Formal Analysis, Investigation, Writing–review and editing. Y-TW: Conceptualization, Writing–review and editing. LQ: Conceptualization, Funding acquisition, Writing–review and editing. YZ: Funding acquisition, Writing–review and editing.
Funding
The author(s) declare that financial support was received for the research, authorship, and/or publication of this article. This work was supported by Construction of standardization of traditional Chinese medicine diet therapy for diabetes mellitus (2022B13, ZZ16-ND-12), Jiangxi Province Key Laboratory of Traditional Chinese Medicine Pharmacology (2024S5Y07113), the “Xinglin Scholars” Discipline Talent Research Enhancement Plan of Chengdu University of TCM (030058080), and the grant from National Institutes of Health (R01HL122937).
Conflict of interest
The authors declare that the research was conducted in the absence of any commercial or financial relationships that could be construed as a potential conflict of interest.
Publisher’s note
All claims expressed in this article are solely those of the authors and do not necessarily represent those of their affiliated organizations, or those of the publisher, the editors and the reviewers. Any product that may be evaluated in this article, or claim that may be made by its manufacturer, is not guaranteed or endorsed by the publisher.
References
Adamik, J., Pulugulla, S. H., Zhang, P., Sun, Q., Lontos, K., Macar, D. A., et al. (2020). EZH2 supports osteoclast differentiation and bone resorption via epigenetic and cytoplasmic targets. J. Bone Mineral Res. Official J. Am. Soc. Bone Mineral Res. 35 (1), 181–195. doi:10.1002/jbmr.3863
Adema, V., and Colla, S. (2022). EZH2 inhibitors: the unpacking revolution. Cancer Res. 82 (3), 359–361. doi:10.1158/0008-5472.CAN-21-4311
Aljubran, S. A., Cox, R., Tamarapu Parthasarathy, P., Kollongod Ramanathan, G., Rajanbabu, V., Bao, H., et al. (2012). Enhancer of zeste homolog 2 induces pulmonary artery smooth muscle cell proliferation. PLoS One 7 (5), e37712. doi:10.1371/journal.pone.0037712
Archer, S. L., Marsboom, G., Kim, G. H., Zhang, H. J., Toth, P. T., Svensson, E. C., et al. (2010). Epigenetic attenuation of mitochondrial superoxide dismutase 2 in pulmonary arterial hypertension: a basis for excessive cell proliferation and a new therapeutic target. Circulation 121 (24), 2661–2671. doi:10.1161/CIRCULATIONAHA.109.916098
Archer, S. L., Weir, E. K., and Wilkins, M. R. (2010). Basic science of pulmonary arterial hypertension for clinicians: new concepts and experimental therapies. Circulation 121 (18), 2045–2066. doi:10.1161/CIRCULATIONAHA.108.847707
Atkinson, C., Stewart, S., Upton, P. D., Machado, R., Thomson, J. R., Trembath, R. C., et al. (2002). Primary pulmonary hypertension is associated with reduced pulmonary vascular expression of type II bone morphogenetic protein receptor. Circulation 105 (14), 1672–1678. doi:10.1161/01.cir.0000012754.72951.3d
Bakker, E. N., Buus, C. L., Spaan, J. A., Perree, J., Ganga, A., Rolf, T. M., et al. (2005). Small artery remodeling depends on tissue-type transglutaminase. Circulation Res. 96 (1), 119–126. doi:10.1161/01.RES.0000151333.56089.66
Basatemur, G. L., Jørgensen, H. F., Clarke, M. C., Bennett, M. R., and Mallat, Z. (2019). Vascular smooth muscle cells in atherosclerosis. Nat. Rev. Cardiol. 16 (12), 727–744. doi:10.1038/s41569-019-0227-9
Bennett, M. R., Sinha, S., and Owens, G. K. (2016). Vascular smooth muscle cells in atherosclerosis. Circulation Res. 118 (4), 692–702. doi:10.1161/CIRCRESAHA.115.306361
Bisserier, M., Mathiyalagan, P., Zhang, S., Elmastour, F., Dorfmüller, P., Humbert, M., et al. (2021). Regulation of the methylation and expression levels of the BMPR2 gene by SIN3a as a novel therapeutic mechanism in pulmonary arterial hypertension. Circulation 144 (1), 52–73. doi:10.1161/CIRCULATIONAHA.120.047978
Cardenas, C. L. L., Kessinger, C. W., MacDonald, C., Jassar, A. S., Isselbacher, E. M., Jaffer, F. A., et al. (2018). Inhibition of the methyltranferase EZH2 improves aortic performance in experimental thoracic aortic aneurysm. JCI Insight 3 (5), e97493. doi:10.1172/jci.insight.97493
Chakraborty, A., Li, Y., Zhang, C., Li, Y., Rebello, K. R., Li, S., et al. (2023). Epigenetic induction of smooth muscle cell phenotypic alterations in aortic aneurysms and dissections. Circulation 148 (12), 959–977. doi:10.1161/CIRCULATIONAHA.123.063332
Chao, L., Xuan, Z., and Yu, R. (2022). Research progress on enhancer of zeste homolog 2 and tumor metastasis. Chin. J. Clin. Oncol. 49 (3), 4. doi:10.12354/j.issn.1000-8179.2022.20210861
Chen, B., Luo, L., Zhu, W., Wei, X., Li, S., Huang, Y., et al. (2016). miR-22 contributes to the pathogenesis of patients with coronary artery disease by targeting MCP-1: an observational study. Medicine 95 (33), e4418. doi:10.1097/MD.0000000000004418
Chen, L., Ma, Y., Kim, E. Y., Yu, W., Schwartz, R. J., Qian, L., et al. (2012). Conditional ablation of Ezh2 in murine hearts reveals its essential roles in endocardial cushion formation, cardiomyocyte proliferation and survival. PLoS One 7 (2), e31005. doi:10.1371/journal.pone.0031005
Chen, W.-J., Chen, Y.-H., Hsu, Y.-J., Lin, K.-H., and Yeh, Y.-H. (2018). MicroRNA-132 targeting PTEN contributes to cilostazol-promoted vascular smooth muscle cell differentiation. Atherosclerosis 274, 1–7. doi:10.1016/j.atherosclerosis.2018.04.030
Chen, Z., Wang, M., Huang, K., He, Q., Li, H., and Chang, G. (2018). MicroRNA-125b affects vascular smooth muscle cell function by targeting serum response factor. Cell. Physiology Biochem. 46 (4), 1566–1580. doi:10.1159/000489203
Duan, R., Du, W., and Guo, W. (2020). EZH2: a novel target for cancer treatment. J. Hematol. Oncol. 13 (1), 104. doi:10.1186/s13045-020-00937-8
Feng, M., Tu, W., Zhou, Q., Du, Y., Xu, K., and Wang, Y. (2023). circHECTD1 promotes the proliferation and migration of human brain vascular smooth muscle cells via interacting with KHDRBS3 to stabilize EZH2 mRNA expression. J. Inflamm. Res. 16, 1311–1323. doi:10.2147/JIR.S398199
Gawinecka, J., Schönrath, F., and von Eckardstein, A. (2017). Acute aortic dissection: pathogenesis, risk factors and diagnosis. Swiss Med. Wkly. 147 (3334), w14489. doi:10.4414/smw.2017.14489
Gladwin, M. T., and Ghofrani, H.-A. (2010). Update on pulmonary hypertension 2009. Am. J. Respir. Crit. Care Med. 181 (10), 1020–1026. doi:10.1164/rccm.201002-0235UP
Grootaert, M. O. J., Moulis, M., Roth, L., Martinet, W., Vindis, C., Bennett, M. R., et al. (2018). Vascular smooth muscle cell death, autophagy and senescence in atherosclerosis. Cardiovasc. Res. 114 (4), 622–634. doi:10.1093/cvr/cvy007
Habbout, K., Omura, J., Awada, C., Bourgeois, A., Grobs, Y., Krishna, V., et al. (2021). Implication of EZH2 in the pro-proliferative and apoptosis-resistant phenotype of pulmonary artery smooth muscle cells in pah: a transcriptomic and proteomic approach. Int. J. Mol. Sci. 22 (6), 2957. doi:10.3390/ijms22062957
Hayashi, K., and Naiki, T. (2009). Adaptation and remodeling of vascular wall; biomechanical response to hypertension. J. Mech. Behav. Biomed. Mater. 2 (1), 3–19. doi:10.1016/j.jmbbm.2008.05.002
Heng, Y., Xiao, X., Liujuan, W., Xinrui, N., Haopeng, W., and Tao, L. (2023). Role of EZH2 in cardiac and vascular development. Chin. J. Histochem. Cytochem. 32 (1), 81–88. doi:10.16705/j.cnki.1004-1850.2023.01.012
Herrington, W., Lacey, B., Sherliker, P., Armitage, J., and Lewington, S. (2016). Epidemiology of atherosclerosis and the potential to reduce the global burden of atherothrombotic disease. Circulation Res. 118 (4), 535–546. doi:10.1161/CIRCRESAHA.115.307611
Hou, Y. F., Fan, F., Xie, N., Zhang, Y., Wang, X., and Meng, X. (2024). Rhodiola crenulata alleviates hypobaric hypoxia-induced brain injury by maintaining BBB integrity and balancing energy metabolism dysfunction. Phytomedicine 128, 155529. doi:10.1016/j.phymed.2024.155529
Huang, J., Wang, T., Wright, A. C., Yang, J., Zhou, S., Li, L., et al. (2015). Myocardin is required for maintenance of vascular and visceral smooth muscle homeostasis during postnatal development. Proc. Natl. Acad. Sci. 112 (14), 4447–4452. doi:10.1073/pnas.1420363112
Ibarrola, J., Xiang, R. R., Sun, Z., Lu, Q., Hill, M. A., and Jaffe, I. Z. (2024). Inhibition of the histone methyltransferase EZH2 induces vascular stiffness. Clin. Sci. 138 (5), 251–268. doi:10.1042/CS20231478
Intengan, H. D., and Schiffrin, E. L. (2000). Structure and mechanical properties of resistance arteries in hypertension: role of adhesion molecules and extracellular matrix determinants. Hypertension 36 (3), 312–318. doi:10.1161/01.hyp.36.3.312
Izutsu, K., Makita, S., Nosaka, K., Yoshimitsu, M., Utsunomiya, A., Kusumoto, S., et al. (2023). An open-label, single-arm phase 2 trial of valemetostat for relapsed or refractory adult T-cell leukemia/lymphoma. Blood 141 (10), 1159–1168. doi:10.1182/blood.2022016862
Jang, M.-A., Kim, E. K., Nguyen, N. T., Kim, W.-J., Yoo, J.-Y., Lee, J., et al. (2015). Mutations in DDX58, which encodes RIG-I, cause atypical Singleton-Merten syndrome. Am. J. Hum. Genet. 96 (2), 266–274. doi:10.1016/j.ajhg.2014.11.019
Jia, L.-X., Zhang, W.-M., Zhang, H.-J., Li, T.-T., Wang, Y.-L., Qin, Y.-W., et al. (2015). Mechanical stretch-induced endoplasmic reticulum stress, apoptosis and inflammation contribute to thoracic aortic aneurysm and dissection. J. Pathology 236 (3), 373–383. doi:10.1002/path.4534
Jiang, D.-S., Yi, X., Zhu, X.-H., and Wei, X. (2016). Experimental in vivo and ex vivo models for the study of human aortic dissection: promises and challenges. Am. J. Transl. Res. 8 (12), 5125–5140.
Jiang, H., Bai, L., Song, S., Yin, Q., Shi, A., Zhou, B., et al. (2023). EZH2 controls epicardial cell migration during heart development. Life Sci. Alliance 6 (6), e202201765. doi:10.26508/lsa.202201765
Juan, A. H., Derfoul, A., Feng, X., Ryall, J. G., Dell'Orso, S., Pasut, A., et al. (2011). Polycomb EZH2 controls self-renewal and safeguards the transcriptional identity of skeletal muscle stem cells. Genes and Dev. 25 (8), 789–794. doi:10.1101/gad.2027911
Kaneko, S., Li, G., Son, J., Xu, C.-F., Margueron, R., Neubert, T. A., et al. (2010). Phosphorylation of the PRC2 component Ezh2 is cell cycle-regulated and up-regulates its binding to ncRNA. Genes and Dev. 24 (23), 2615–2620. doi:10.1101/gad.1983810
Katsuki, S., Jha, P. K., Lupieri, A., Nakano, T., Passos, L. S., Rogers, M. A., et al. (2022). Proprotein convertase subtilisin/kexin 9 (PCSK9) promotes macrophage activation via LDL receptor-independent mechanisms. Circulation Res. 131 (11), 873–889. doi:10.1161/CIRCRESAHA.121.320056
Kumar, S., Boon, R. A., Maegdefessel, L., Dimmeler, S., and Jo, H. (2019). Role of noncoding RNAs in the pathogenesis of abdominal aortic aneurysm: possible therapeutic targets? Circulation Res. 124 (4), 619–630. doi:10.1161/CIRCRESAHA.118.312438
Larson, E. W., and Edwards, W. D. (1984). Risk factors for aortic dissection: a necropsy study of 161 cases. Am. J. Cardiol. 53 (6), 849–855. doi:10.1016/0002-9149(84)90418-1
Le, T., He, X., Huang, J., Liu, S., Bai, Y., and Wu, K. (2021). Knockdown of long noncoding RNA GAS5 reduces vascular smooth muscle cell apoptosis by inactivating EZH2-mediated RIG-I signaling pathway in abdominal aortic aneurysm. J. Transl. Med. 19 (1), 466–512. doi:10.1186/s12967-021-03023-w
Levy, E., Spahis, S., Bigras, J.-L., Delvin, E., and Borys, J.-M. (2017). The epigenetic machinery in vascular dysfunction and hypertension. Curr. Hypertens. Rep. 19 (6), 52. doi:10.1007/s11906-017-0745-y
Li, Q., Qi, L., Zhao, K., Ke, W., Li, T., and Xia, L. (2022). Integrative quantitative and qualitative analysis for the quality evaluation and monitoring of Danshen medicines from different sources using HPLC-DAD and NIR combined with chemometrics. Front. Plant Sci. 13, 932855. doi:10.3389/fpls.2022.932855
Li, Q., Yan, X. J., Zhao, K., Li, L., Peng, S. G., Luo, X., et al. (2020). Fast inspection of saffron on the spot based on cloud-connected portable near-infrared technology. Spectrosc. Spectr. Analysis 40 (10), 3029–3037. doi:10.3964/j.issn.1000-0593(2020)10-3029-09
Li, R., Yi, X., Wei, X., Huo, B., Guo, X., Cheng, C., et al. (2018). EZH2 inhibits autophagic cell death of aortic vascular smooth muscle cells to affect aortic dissection. Cell Death Dis. 9 (2), 180. doi:10.1038/s41419-017-0213-2
Li, Y., Guo, S., Zhao, Y., Li, R., Li, Y., Qiu, C., et al. (2022). EZH2 regulates ANXA6 expression via H3K27me3 and is involved in angiotensin II-induced vascular smooth muscle cell senescence. Oxidative Med. Cell. Longev. 2022, e4838760. doi:10.1155/2022/4838760
Liang, J., Li, Q., Cai, W., Zhang, X., Yang, B., Li, X., et al. (2019). Inhibition of polycomb repressor complex 2 ameliorates neointimal hyperplasia by suppressing trimethylation of H3K27 in vascular smooth muscle cells. Br. J. Pharmacol. 176 (17), 3206–3219. doi:10.1111/bph.14754
Liu, D., Yan, Y., Chen, J.-W., Yuan, P., Wang, X.-J., Jiang, R., et al. (2017). Hypermethylation of BMPR2 promoter occurs in patients with heritable pulmonary arterial hypertension and inhibits BMPR2 expression. Am. J. Respir. Crit. Care Med. 196 (7), 925–928. doi:10.1164/rccm.201611-2273LE
Liu, R., Bauer, A. J., and Martin, K. A. (2016). A new editor of smooth muscle phenotype. Am. Heart Assoc. 119, 401–403. doi:10.1161/CIRCRESAHA.116.309218
Liu, R., Huang, S.-S., Shi, H., Chang, S., and Ge, J. (2022). Alpha-lipoic acid protects against aortic aneurysm and dissection by improving vascular smooth muscle cell function. Life Sci. 311 (Pt A), 121159. doi:10.1016/j.lfs.2022.121159
Liu, R., Leslie, K. L., and Martin, K. A. (2015). Epigenetic regulation of smooth muscle cell plasticity. Biochimica Biophysica Acta 1849 (4), 448–453. doi:10.1016/j.bbagrm.2014.06.004
Liu, Y., Dai, C., Lei, Y., Wu, W., and Liu, W. (2020). Inhibition of EZH2 attenuates coronary heart disease by interacting with microRNA-22 to regulate the TXNIP/nuclear factor-κB pathway. Exp. Physiol. 105 (12), 2038–2050. doi:10.1113/EP088881
Liu, Y., Zhao, Y., Feng, P., and Jiang, H. (2023). PCSK9 inhibitor attenuates atherosclerosis by regulating SNHG16/EZH2/TRAF5-mediated VSMC proliferation, migration, and foam cell formation. Cell Biol. Int. 47, 1267–1280. doi:10.1002/cbin.12018
Machado, D. B., Pescarini, J. M., Ramos, D., Teixeira, R., Lozano, R., Pereira, V. O. M., et al. (2020). Monitoring the progress of health-related sustainable development goals (SDGs) in Brazilian states using the Global Burden of Disease indicators. Popul. Health Metrics 18 (Suppl. 1), 7. doi:10.1186/s12963-020-00207-2
Mahara, S., Lee, P. L., Feng, M., Tergaonkar, V., Chng, W. J., and Yu, Q. (2016). HIFI-α activation underlies a functional switch in the paradoxical role of Ezh2/PRC2 in breast cancer. Proc. Natl. Acad. Sci. 113 (26), E3735–E3744. doi:10.1073/pnas.1602079113
Miao, R., Qi, C., Fu, Y., Wang, Y., Lang, Y., Liu, W., et al. (2022). Silencing of circARHGAP12 inhibits the progression of atherosclerosis via miR-630/EZH2/TIMP2 signal axis. J. Cell. Physiology 237 (1), 1057–1069. doi:10.1002/jcp.30598
Michel, J.-B., Jondeau, G., and Milewicz, D. M. (2018). From genetics to response to injury: vascular smooth muscle cells in aneurysms and dissections of the ascending aorta. Cardiovasc. Res. 114 (4), 578–589. doi:10.1093/cvr/cvy006
Misra, A., Feng, Z., Chandran, R. R., Kabir, I., Rotllan, N., Aryal, B., et al. (2018). Integrin beta3 regulates clonality and fate of smooth muscle-derived atherosclerotic plaque cells. Nat. Commun. 9 (1), 2073. doi:10.1038/s41467-018-04447-7
Mochizuki-Kashio, M., Aoyama, K., Sashida, G., Oshima, M., Tomioka, T., Muto, T., et al. (2015). Ezh2 loss in hematopoietic stem cells predisposes mice to develop heterogeneous malignancies in an Ezh1-dependent manner. Blood 126 (10), 1172–1183. doi:10.1182/blood-2015-03-634428
Montezano, A. C., Tsiropoulou, S., Dulak-Lis, M., Harvey, A., Camargo, L. D. L., and Touyz, R. M. (2015). Redox signaling, Nox5 and vascular remodeling in hypertension. Curr. Opin. Nephrol. Hypertens. 24 (5), 425–433. doi:10.1097/MNH.0000000000000153
Morrell, N. W. (2010). Role of bone morphogenetic protein receptors in the development of pulmonary arterial hypertension. Membr. Recept. Channels Transp. Pulm. Circulation 661, 251–264. doi:10.1007/978-1-60761-500-2_16
Nienaber, C. A., Clough, R. E., Sakalihasan, N., Suzuki, T., Gibbs, R., Mussa, F., et al. (2016). Aortic dissection. Nat. Rev. Dis. Prim. 2 (1), 16053–16118. doi:10.1038/nrdp.2016.53
Orriols, M., Gomez-Puerto, M. C., and Ten Dijke, P. (2017). BMP type II receptor as a therapeutic target in pulmonary arterial hypertension. Cell. Mol. Life Sci. 74, 2979–2995. doi:10.1007/s00018-017-2510-4
Pan, S., Lai, H., Shen, Y., Breeze, C., Beck, S., Hong, T., et al. (2017). DNA methylome analysis reveals distinct epigenetic patterns of ascending aortic dissection and bicuspid aortic valve. Cardiovasc. Res. 113 (6), 692–704. doi:10.1093/cvr/cvx050
Paredes, F., Williams, H. C., Suster, I., Tejos, M., Fuentealba, R., Bogan, B., et al. (2023). Metabolic regulation of the proteasome under hypoxia by Poldip2 controls fibrotic signaling in vascular smooth muscle cells. Free Radic. Biol. Med. 195, 283–297. doi:10.1016/j.freeradbiomed.2022.12.098
Pingping, W., Xiaofei, W., Ruolan, W., Guanchang, C., and Tao, L. (2022). Noncanonical role of histone methylase EZH2. Chem. Life 002, 042. doi:10.13488/j.smhx.20210575
Proudfoot, D., Skepper, J. N., Hegyi, L., Bennett, M. R., Shanahan, C. M., and Weissberg, P. L. (2000). Apoptosis regulates human vascular calcification in vitro: evidence for initiation of vascular calcification by apoptotic bodies. Circulation Res. 87 (11), 1055–1062. doi:10.1161/01.res.87.11.1055
Qi, L., Wu, S., Liu, N., Zhang, X., Ping, L., and Xia, L. (2024). Salvia miltiorrhiza bunge extract improves the Th17/Treg imbalance and modulates gut microbiota of hypertensive rats induced by high-salt diet. J. Funct. Foods 117, 106211. doi:10.1016/j.jff.2024.106211
Rabinovitch, M. (2012). Molecular pathogenesis of pulmonary arterial hypertension. J. Clin. Investigation 122 (12), 4306–4313. doi:10.1172/JCI60658
Ragusa, R., Basta, G., Neglia, D., De Caterina, R., Del Turco, S., and Caselli, C. (2021). PCSK9 and atherosclerosis: looking beyond LDL regulation. Eur. J. Clin. Investigation 51 (4), e13459. doi:10.1111/eci.13459
Ramel, D., Gayral, S., Sarthou, M.-K., Augé, N., Nègre-Salvayre, A., and Laffargue, M. (2019). Immune and smooth muscle cells interactions in atherosclerosis: how to target a breaking bad dialogue? Front. Pharmacol. 10, 1276. doi:10.3389/fphar.2019.01276
Ren, X. S., Tong, Y., Ling, L., Chen, D., Sun, H. J., Zhou, H., et al. (2017). NLRP3 gene deletion attenuates angiotensin II-induced phenotypic transformation of vascular smooth muscle cells and vascular remodeling. Cell. Physiology Biochem. 44 (6), 2269–2280. doi:10.1159/000486061
Rondeaux, J., Groussard, D., Renet, S., Tardif, V., Dumesnil, A., Chu, A., et al. (2023). Ezh2 emerges as an epigenetic checkpoint regulator during monocyte differentiation limiting cardiac dysfunction post-MI. Nat. Commun. 14 (1), 4461. doi:10.1038/s41467-023-40186-0
Saco, T. V., Parthasarathy, P. T., Cho, Y., Lockey, R. F., and Kolliputi, N. (2014). Role of epigenetics in pulmonary hypertension. Am. J. Physiology-Cell Physiology 306 (12), C1101–C1105. doi:10.1152/ajpcell.00314.2013
Savoia, C., Burger, D., Nishigaki, N., Montezano, A., and Touyz, R. M. (2011). Angiotensin II and the vascular phenotype in hypertension. Expert Rev. Mol. Med. 13, e11. doi:10.1017/S1462399411001815
Schrijvers, D. M., De Meyer, G. R., Kockx, M. M., Herman, A. G., and Martinet, W. (2005). Phagocytosis of apoptotic cells by macrophages is impaired in atherosclerosis. Arteriosclerosis, Thrombosis, Vasc. Biol. 25 (6), 1256–1261. doi:10.1161/01.ATV.0000166517.18801.a7
Shen, J., Yang, M., Ju, D., Jiang, H., Zheng, J.-P., Xu, Z., et al. (2010). Disruption of SM22 promotes inflammation after artery injury via nuclear factor kappaB activation. Circulation Res. 106 (8), 1351–1362. doi:10.1161/CIRCRESAHA.109.213900
Shi, Z.-L., Fang, K., Li, Z.-H., Ren, D.-H., Zhang, J.-Y., and Sun, J. (2018). EZH2 inhibition ameliorates transverse aortic constriction-induced pulmonary arterial hypertension in mice. Can. Respir. J. 2018, 9174926. doi:10.1155/2018/9174926
Shu, Y.-N., Dong, L.-H., Li, H., Pei, Q.-Q., Miao, S.-B., Zhang, F., et al. (2017). CKII-SIRT1-SM22α loop evokes a self-limited inflammatory response in vascular smooth muscle cells. Cardiovasc. Res. 113 (10), 1198–1207. doi:10.1093/cvr/cvx048
Siasos, G., Mourouzis, K., Oikonomou, E., Tsalamandris, S., Tsigkou, V., Vlasis, K., et al. (2015). The role of endothelial dysfunction in aortic aneurysms. Curr. Pharm. Des. 21 (28), 4016–4034. doi:10.2174/1381612821666150826094156
Soon, E., Crosby, A., Southwood, M., Yang, P., Tajsic, T., Toshner, M., et al. (2015). Bone morphogenetic protein receptor type II deficiency and increased inflammatory cytokine production. A gateway to pulmonary arterial hypertension. Am. J. Respir. Crit. Care Med. 192 (7), 859–872. doi:10.1164/rccm.201408-1509OC
Tang, Y., Zhao, L., Yu, X., Zhang, J., Qian, L., Jin, J., et al. (2021). Inhibition of EZH2 primes the cardiac gene activation via removal of epigenetic repression during human direct cardiac reprogramming. Stem Cell Res. 53, 102365. doi:10.1016/j.scr.2021.102365
Wang, D., and Atanasov, A. G. (2019). The microRNAs regulating vascular smooth muscle cell proliferation: a minireview. Int. J. Mol. Sci. 20 (2), 324. doi:10.3390/ijms20020324
Wang, F., and Chen, H.-Z. (2020). Histone deacetylase SIRT1, smooth muscle cell function, and vascular diseases. Front. Pharmacol. 11, 537519. doi:10.3389/fphar.2020.537519
Wang, J., An, G., Peng, X., Zhong, F., Zhao, K., Qi, L., et al. (2024). Effects of three Huanglian-derived polysaccharides on the gut microbiome and fecal metabolome of high-fat diet/streptozocin-induced type 2 diabetes mice. Int. J. Biol. Macromol. 133060. doi:10.1016/j.ijbiomac.2024.133060
Wang, J., Zhang, C., Li, C., Zhao, D., Li, S., Ma, L., et al. (2019). MicroRNA-92a promotes vascular smooth muscle cell proliferation and migration through the ROCK/MLCK signalling pathway. J. Cell. Mol. Med. 23 (5), 3696–3710. doi:10.1111/jcmm.14274
Wang, K., Yan, Z. Y., Ma, Y., Li, B., Wang, W., Qi, L., et al. (2021). A mathematical model for characterizing the biomass and the physiological/biochemical indicators of salvia miltiorrhiza based on growth-defense tradeoff. Front. Plant Sci. 12, 793574. doi:10.3389/fpls.2021.793574
Wang, L., Jin, Q., Lee, J.-E., Su, I.-h., and Ge, K. (2010). Histone H3K27 methyltransferase Ezh2 represses Wnt genes to facilitate adipogenesis. Proc. Natl. Acad. Sci. 107 (16), 7317–7322. doi:10.1073/pnas.1000031107
Wang, P., Zhang, M., Wang, Z., Wu, Q., Shi, F., and Yuan, S. (2021). ALKBH5 exacerbates aortic dissection by promoting inflammatory response and apoptosis of aortic smooth muscle cells via regulating lnc-TMPO-AS1/EZH2/IRAK4 signals in an m6A modification manner. Oxidative Med. Cell. Longev. 2021, 1–24. doi:10.1155/2021/5513966
Wang, Y., Huang, X.-X., Leng, D., Li, J.-F., Liang, Y., and Jiang, T. (2021). Effect of EZH2 on pulmonary artery smooth muscle cell migration in pulmonary hypertension. Mol. Med. Rep. 23 (2), 129. doi:10.3892/mmr.2020.11768
Wang, Y., Zhao, Z.-M., Zhang, G.-X., Yang, F., Yan, Y., Liu, S.-X., et al. (2016). Dynamic autophagic activity affected the development of thoracic aortic dissection by regulating functional properties of smooth muscle cells. Biochem. Biophysical Res. Commun. 479 (2), 358–364. doi:10.1016/j.bbrc.2016.09.080
Wang, Y.-T., Chen, J., Li, X., Umetani, M., Chen, Y., Li, P.-L., et al. (2019). Contribution of transcription factor EB to adipoRon-induced inhibition of arterial smooth muscle cell proliferation and migration. Am. J. Physiology. Cell Physiology 317 (5), C1034–C1047. doi:10.1152/ajpcell.00294.2019
Wang, Y.-T., Li, X., Chen, J., McConnell, B. K., Chen, L., Li, P.-L., et al. (2019). Activation of TFEB ameliorates dedifferentiation of arterial smooth muscle cells and neointima formation in mice with high-fat diet. Cell Death Dis. 10 (9), 676. doi:10.1038/s41419-019-1931-4
Wang, Z., Liu, B., Zhu, J., Wang, D., and Wang, Y. (2019). Nicotine-mediated autophagy of vascular smooth muscle cell accelerates atherosclerosis via nAChRs/ROS/NF-κB signaling pathway. Atherosclerosis 284, 1–10. doi:10.1016/j.atherosclerosis.2019.02.008
Wortmann, M., Skorubskaya, E., Peters, A. S., Hakimi, M., Böckler, D., and Dihlmann, S. (2019). Necrotic cell debris induces a NF-κB-driven inflammasome response in vascular smooth muscle cells derived from abdominal aortic aneurysms (AAA-SMC). Biochem. Biophysical Res. Commun. 511 (2), 343–349. doi:10.1016/j.bbrc.2019.02.051
Wu, M.-Y., Li, C.-J., Hou, M.-F., and Chu, P.-Y. (2017). New insights into the role of inflammation in the pathogenesis of atherosclerosis. Int. J. Mol. Sci. 18 (10), 2034. doi:10.3390/ijms18102034
Wu, S., Zhao, K., Wang, J., Liu, N., Nie, K., Qi, L., et al. (2022). Recent advances of tanshinone in regulating autophagy for medicinal research. Front. Pharmacol. 13, 1059360. doi:10.3389/fphar.2022.1059360
Wu, Z.-y., Trenner, M., Boon, R. A., Spin, J. M., and Maegdefessel, L. (2020). Long noncoding RNAs in key cellular processes involved in aortic aneurysms. Atherosclerosis 292, 112–118. doi:10.1016/j.atherosclerosis.2019.11.013
Yuan, J.-L., Yin, C.-Y., Li, Y.-Z., Song, S., Fang, G.-J., and Wang, Q.-S. (2021). EZH2 as an epigenetic regulator of cardiovascular development and diseases. J. Cardiovasc. Pharmacol. 78 (2), 192–201. doi:10.1097/FJC.0000000000001062
Yuan, X., Bhat, O. M., Lohner, H., Zhang, Y., and Li, P. L. (2020). Downregulation of lysosomal acid ceramidase mediates HMGB1-induced migration and proliferation of mouse coronary arterial myocytes. Front. Cell Dev. Biol. 8, 111. doi:10.3389/fcell.2020.00111
Zhang, P., Guan, Y., Chen, J., Li, X., McConnell, B. K., Zhou, W., et al. (2018). Contribution of p62/SQSTM1 to PDGF-BB-induced myofibroblast-like phenotypic transition in vascular smooth muscle cells lacking Smpd1 gene. Cell Death Dis. 9 (12), 1145. doi:10.1038/s41419-018-1197-2
Zhang, W., Wang, Q., Xing, X., Yang, L., Xu, M., Cao, C., et al. (2021). The antagonistic effects and mechanisms of microRNA-26a action in hypertensive vascular remodelling. Br. J. Pharmacol. 178 (5), 1037–1054. doi:10.1111/bph.15337
Zhao, K. C., Qian, L., Qi, Q., Li, C., Zhao, J., Zhang, G., et al. (2024). Modified acid polysaccharide derived from Salvia przewalskii with excellent wound healing and enhanced bioactivity. Int. J. Biol. Macromol. 263(Pt 2). doi:10.1016/j.ijbiomac.2024.129803
Zhao, K., Li, B., He, D., Zhao, C., Shi, Z., Dong, B., et al. (2020). Chemical characteristic and bioactivity of hemicellulose-based polysaccharides isolated from Salvia miltiorrhiza. Int. J. Biol. Macromol. 165 (Pt B), 2475–2483. doi:10.1016/j.ijbiomac.2020.10.113
Zheng, X., Zhao, X., Han, Z., and Chen, K. (2021). Enhancer of zeste homolog 2 participates in the process of atherosclerosis by modulating microRNA-139-5p methylation and signal transducer and activator of transcription 1 expression. IUBMB Life 73 (1), 238–251. doi:10.1002/iub.2423
Zhong, H.-y., Yuan, C., Liu, X.-l., Wang, Q.-q., Li, X., Zhao, Y.-c., et al. (2022). Mechanical stretch aggravates vascular smooth muscle cell apoptosis and vascular remodeling by downregulating EZH2. Int. J. Biochem. Cell Biol. 151, 106278. doi:10.1016/j.biocel.2022.106278
Keywords: epigenetic modification, EZH2, vascular smooth muscle cells, cardiovascular disease, atherosclerosis, hypertension
Citation: Luo H, Li Y, Song H, Zhao K, Li W, Hong H, Wang Y-T, Qi L and Zhang Y (2024) Role of EZH2-mediated epigenetic modification on vascular smooth muscle in cardiovascular diseases: A mini-review. Front. Pharmacol. 15:1416992. doi: 10.3389/fphar.2024.1416992
Received: 13 April 2024; Accepted: 03 June 2024;
Published: 27 June 2024.
Edited by:
Xiangxiang Wei, Fudan University, ChinaReviewed by:
Shivani Srivastava, Yale University, United StatesPan Jiang, Zhongshan Hospital, Fudan University, China
Copyright © 2024 Luo, Li, Song, Zhao, Li, Hong, Wang, Qi and Zhang. This is an open-access article distributed under the terms of the Creative Commons Attribution License (CC BY). The use, distribution or reproduction in other forums is permitted, provided the original author(s) and the copyright owner(s) are credited and that the original publication in this journal is cited, in accordance with accepted academic practice. No use, distribution or reproduction is permitted which does not comply with these terms.
*Correspondence: Yun-Ting Wang, ywang264@central.uh.edu; Luming Qi, lmqi_tcm@126.com; Yang Zhang, yzhan219@central.uh.edu
†These authors have contributed equally to this work