- 1Andalusian Center of Developmental Biology, CSIC, University of Pablo de Olavide - Seville, Seville, Spain
- 2Centro de Investigaciones Biológicas Margarita Salas (CIB-CSIC), Madrid, Spain
- 3Department of Molecular Biology and Biochemical Engineering, University of Pablo de Olavide, Seville, Spain
Our aim is to identify new small molecules with antimicrobial potential, especially against colistin-resistant (Col-R) Acinetobacter baumannii and Escherichia coli. After initial hits identification by fingerprint similarity, MIC of 24 heterocyclic derivatives for A. baumannii and E. coli reference strains, and bactericidal activity of selected thiophenes against Col-R strains were determined. We analyzed changes in bacterial membrane permeability and the OMPs profile. Additionally, we determined bacterial adherence to host cells and performed molecular docking studies to assess their binding to bacterial targets. The compounds’ MICs ranged from 4 to >64 mg/L. Thiophene derivatives 4, 5 and 8 exhibited MIC50 values between 16 and 32 mg/L for Col-R A. baumannii and 8 and 32 mg/L for Col-R E. coli. The time-kill curve assay demonstrated that thiophenes 4 and 8 had bactericidal effects against Col-R A. baumannii and E. coli. Furthermore, treatment with them resulted in increased membrane permeabilization and reduced adherence of these isolates to host cells. Finally, the docking studies showed a stronger binding affinity to CarO1 and Omp33 of A. baumannii and OmpW and OmpC of E. coli. These findings indicate that thiophene derivatives possess antibacterial activity against Col-R A. baumannii and E. coli, suggesting that they may enhance the repertoire of drug treatments against bacteria.
1 Introduction
Gram-negative bacilli (GNB) are highly efficient in acquiring antimicrobial resistance through genomic, transcriptomic, and proteomic changes (Kung et al., 2010; Yeline et al., 2018). Compounding the problem of antimicrobial resistance is the immediate threat of a reduction in the discovery and development of new antibiotics, as highlighted by the World Health Organization (WHO) (Tacconelli et al., 2018) and other European institutions (O’Neil, 2015; Årdal et al., 2017). Consequently, a perfect storm is converging with regard to these infections: increasing antimicrobial resistance with a decreased development of new drugs (O’Neil, 2015). This context is likely the best example of the purported “Post-Antibiotic Era,” which has relevance even in non-specialized media (Bagley, 2017). It is clear that effective solutions are urgently needed, as stressed by various institutions. Therefore, the development of new strategic antimicrobial therapeutic approaches, such as the use of non-antibiotic substances and/or drug repurposing to be used as monotherapies or in combination with one of the scarce but clinically relevant antibiotics, has become an urgent need.
In this environment, “repurposing” (defined as investigating new uses for existing drugs) has gained renewed interest (Gil et al., 2021a). A variety of drug families have been considered, including anticancer, anthelmintic, anti-inflammatory/immunomodulatory, antipsychotic and antidepressant, statins, and iron-storage drugs (Miró-Canturri et al., 2019; Canturri and Smani, 2023). It has been reported that anticancer drugs like tamoxifen and raloxifene can modify the immune response by regulating cytokine release (Flores et al., 2016; Canturri and Smani, 2023). Previously, we showed in vitro and in vivo that tamoxifen metabolites have bactericidal activity by reducing bacterial loads in vitro and in tissues and fluid of mice (Miró-Canturri et al., 2021a; Miró-Canturri et al., 2021b).
This study was designed to identify new chemical entities with antimicrobial potential. With this aim, we carried out a computational approach combined with experimental confirmation. Initially, a ligand-based virtual screening (LBVS) was performed based on the concept that similar compounds usually show similar biological effects (Maggiora et al., 2014; Muegge et al., 2016). We used two anticancer drugs (4-hydroxytamoxifen and raloxifene) as chemical templates due to their antimicrobial activities previously reported (Ho Sui et al., 2012; Flores et al., 2016; Miró-Canturri et al., 2021c). Using topological fingerprint, we selected 12 chemically diverse compounds from the Medicinal and Biological Chemistry (MBC) library (Ginex et al., 2023) to be experimentally checked as antimicrobials. From the initial set, two thiophene derivatives named AGR1.229 (1) and AGR1.230 (2), showed interesting activities against reference strains of Acinetobacter baumannii and Escherichia coli. It is well-documented that many synthetic strategies have allowed the thiophene ring to be present in numerous pharmacologically important compounds (Mabkhot et al., 2016). Various thiophene-based agents have shown antimicrobial activity, such as thiophene-substituted heterocycles (pyridines, azoles, diazines, and azepines, among others), aminothiophenes, compounds based on thiophene carboxylic acids and derivatives, compounds derived from thiophene-2-carboxaldehydes, and metal complexes based on thiophene-containing ligands (for reference, see review by Roman, 2022).
To confirm the results of the two thiophene derivatives AGR1.229 (1) and AGR1.230 (2), we evaluated a focus library of 24 derivatives chemically related to the initial hits, resulting in the identification of thiophenes 4, 5 and 8 with promising antibacterial activity against A. baumannii and E. coli resistant to colistin. Preliminar experiments to decipher the mechanism of action of this new class of antibacterials are also presented.
2 Materials and methods
2.1 Ligand-based virtual screening
The MBC library (Ginex et al., 2023) has been in silico screened to identify new compounds potentially active against A. baumannii, Pseudomonas aeruginosa or E. coli. The 4 well-known 2D similarity metrics, Buser, Cosine, Kulczynski and Tanimoto have been applied for the prioritization of the potential hits, according to the linear fingerprint method implemented in the similarity-based virtual screening protocol of Schrödinger (Schrödinger Release 2021-1, 2021). The MolPrint2D fingerprints, which encodes atom environment descriptors based on their molecular connectivity table, were used as 2D similarity descriptors (Bender et al., 2004). Two anticancer drugs, 4-hydroxytamoxifen and raloxifene, also reported to have antibacterial effect (Ho Sui et al., 2012; Miró-Canturri et al., 2021b) were selected as reference structures for 2D similarity search (Figure 1). After ranking the compounds of the MBC library based on the 2D similarity metrics mentioned above with tamoxifene and raloxifene, mean values were calculated for each compound considering each metric. Once the compounds were ranked according to mean values, the available ones were selected for evaluation. A total of 12 compounds from similarity-based virtual screening has been selected for further in vitro studies. Their similarity indexes with respect to 4-hydroxytamoxifen and raloxifene are reported in Supplementary Table S1 of Supplementary Material.
2.2 Compounds studied
All the compounds tested in this work were prepared in the Centro de Investigaciones Biológicas Margarita Salas (CIB-CSIC) following previously described procedures (Corral et al., 1978; Narender et al., 2007; Palomo et al., 2012; Redondo et al., 2012; Gil et al., 2021b; Gil et al., 2023) and are collected in the MBC library (Ginex et al., 2023). Purity has been checked by HPLC (≥95%) and chromatograms are included in Supplementary Figure S1 of Supported Information.
2.3 Bacterial strains
A total of eight clinical isolates of A. baumannii, 13 clinical isolates of E. coli resistant to colistin and two reference strains of A. baumannii ATCC 17978 and E. coli ATCC 25922 were used in this study. The A. baumannii and E. coli strains were isolated from the University Hospital of Virgen del Rocío in Seville, Spain and confirmed their species using DNA and MALDI-TOF based confirmation (Valencia et al., 2009; López-Rojas et al., 2011; Gálvez Benítez et al., 2021). The A. baumannii ATCC 17978 and E. coli MG1655 deficient in Omp33 and OmpC were also used (Smani et al., 2013; Gálvez Benítez et al., 2023).
2.4 In vitro susceptibility testing
The MICs of thiophene derivatives against reference and colistin-resistant A. baumannii and E. coli, and MIC of thiophene derivative 4 against A. baumannii ATCC 17978 and E. coli MG1655 and its isogenic Omp33 and OmpC-deficient strains, respectively, were determined in two independent experiments using the broth microdilution method, in accordance with the standard guidelines of the European Committee on Antimicrobial Susceptibility Testing (EUCAST) (EUCAST, 2022). A 5 × 105 CFU/mL inoculum of each strain was cultured in Luria Bertani (LB) and added to U-bottom microtiter plates (Deltlab, Spain) containing the studied compound. The plates were then incubated for 18 h at 37°C. The MIC50, which represent the concentrations that were effective against ≥50% of the isolates tested, were determined.
2.5 Time-kill kinetic assays
To determine the bactericidal activity, duplicate time-kill curves were performed for A. baumannii Ab11 and Ab21, and E. coli MCR1+ and R6 MCR1 strains, as previously described (Miró-Canturri et al., 2020). An initial inoculum of 5 × 105 CFU/mL was added to LB in the presence of 1xMIC, 2xMIC and 4xMIC of thiophene derivatives. A drug-free broth was evaluated in parallel as a control. Tubes of each condition were incubated at 37°C with shaking, and viable counts were determined by serial dilution at 0, 2, 4, 8, and 24 h. Viable counts were determined by plating 100 µL of the control, test cultures, or the respective dilutions at the indicated times onto sheep blood agar plates (ThermoFisher, Spain). Plates were incubated for 24 h at 37°C, and after colony counts, the log10 of viable cells (CFU/mL) was determined. Bactericidal activity was defined as a reduction of ≥3 log10 CFU/mL from the initial inoculum.
2.6 Human cell culture
HeLa cells were grown in Dulbecco’s Modified Eagle Medium (DMEM) rich medium containing 10% heat-inactivated fetal bovine serum (FBS), vancomycin (50 mg/L), gentamicin (20 mg/L), and amphotericin B (0.25 mg/L) (Invitrogen, Spain), and 1% HEPES in a humidified incubator with 5% CO2 at 37°C. The HeLa cells were routinely passaged every 3 or 4 days. Following this, a 24-well plate was prepared by seeding HeLa cells with a concentration of 1 × 105 cells/mL and incubated it for 24 h in the DMEM rich medium. Immediately before infection, HeLa cells seeded in 24-well plate were washed three times with prewarmed phosphate buffered saline (PBS) and further incubated in DMEM without FBS, vancomycin, gentamicin and amphotericin B (Parra-Millán et al., 2018).
2.7 Adhesion assays
HeLa cells were infected with A. baumannii Ab11 and Ab21 strains and E. coli MCR1+ and R6 MCR1 strains at a concentration of 1 × 108 CFU/mL, in the absence and presence of 1xMIC of thiophene derivatives at a multiplicity of infection (MOI) of 100. The bacteria were treated with thiophene derivatives separately for 30 min at 1xMIC before being introduced into the experimental setup. The infection was carried out for 2 h with 5% CO2 at 37°C. After that, the infected HeLa cells were washed five times with pre-warmed PBS and lysed with 0.5% Triton X-100. One hundred microliters of the diluted lysates were plated onto LB agar and incubated at 37°C for 24 h to enumerate the developed colonies and determine the number of bacteria that had attached to the HeLa cells (Parra-Millán et al., 2018).
2.8 Membrane permeability assays
The bacterial cells were grown in LB broth and incubated in the absence or presence of 0.5xMIC of thiophene derivatives for 3 h. The pellet was harvested by ultracentrifugation at 4600g for 15 min. The bacterial cells were washed with PBS 1x, and after centrifugation in the same condition described before, the pellet was resuspended in 100 µL of PBS 1x containing 10 μL of Ethidium Homodimer-1 (EthD-1) (Invitrogen, United States). After 10 min of incubation, (i) 10 μL of the pellet was placed on a slide to visualize it using a Zeiss fluorescence microscope (Zeiss MicroImaging GmbH, Germany), and (ii) 100 μL was placed into a 96-well plate to measure fluorescence for 300 min using a Typhoon FLA 9000 laser scanner (GE Healthcare Life Sciences, United States) and quantified using ImageQuant TL software (GE Healthcare Life Sciences, United States) (Miró-Canturri et al., 2020).
2.9 Analysis of outer membrane proteins
To assess the effect of thiophene derivatives on outer membrane proteins (OMPs), bacterial cells of colistin-resistant A. baumannii Ab11 and Ab21 strains, and colistin-resistant E. coli MCR1+ and R6 MCR1 strains were grown in LB broth to logarithmic phase, followed by treatment with 0.5xMIC of the compounds for 24 h. The cells were then lysed by sonication, and OMPs were extracted with sodium lauryl sarcosinate (Sigma, Spain) and recovered by ultracentrifugation, as described previously (Miró-Canturri et al., 2021b). The extracted OMPs were separated by sodium dodecyl sulfate polyacrylamide gel electrophoresis (SDS-PAGE) using 10% SDS gels, and 6 μg of OMP protein was loaded per lane. The gels were stained with SimplyBlue SafeStain (Invitrogen, Spain) to visualize the OMP profiles.
2.10 Docking and molecular modelling
All chemical structures were drawn and optimized by ChemOffice Suite released in 2022 (Rakshit et al., 2023). All molecular docking calculations and preparation of protein and ligand systems were done with the Schrödinger software package (Friesner et al., 2006; Gentile et al., 2022). Protein structures of the transmembrane domain of A. baumannii OmpA and OmpW were obtained by homology modeling using I-TASSER online server (http://zhanglab.ccmb.med.umich.edu/I-TASSER/) (Yang et al., 2015). The amino acid sequences of OmpA and OmpW of A. baumannii were taken from Genbank (ID: AXV53527.1 and SUU48067.1, respectively), and OmpA and OmpW crystal structures of E. coli (PDB IDs: 1bxw and 2f1v, respectively) were applied as template (Hong et al., 2006; Vila-Farrés et al., 2017). The percentage of identity between OmpA of E. coli and A. baumannii, and OmpW of E. coli and A. baumannii obtained by I-TASSER was 26% and 32%, respectively.
The crystal structures for the rest of the OMPs were taken from the Protein Data Bank (PDB) (PDB ID: OMPs of A. baumannii; CarO1: 4rl9, CarO2: 4rlb, Omp33: 6gie, and OMPs of E. coli; OmpC: 2j1n, OmpF: 4gcq) (Baslé et al., 2006; Ziervogel et al., 2013; Zahn et al., 2015; Abellón-Ruiz et al., 2018). All PDB entries (4rl9, 4rlb, 6gie, 2j1n, 4gcq) obtained from X-Ray crystals were used in all docking studies. All models were prepared with Protein Preparation Wizard, minimized, and a post-docking minimization was performed to further improve the accuracy of the results (Schrödinger Release 2013-3, 2013). The SiteMap module of the Schrödinger Suite identified a potentially druggable area for ligand binding in the border of the opening of the transmembrane domain of OMPs and flexible extracellular loops (Schrödinger Release 2013-3, 2013). The same area was used for constructing docking grids for Glide. The chemical structure of selected thiophene derivative 4 was built, and optimized by LigPrep (Schrödinger Release 2013-3, 2013), and additional MacroModel conformational search techniques were applied to this compound. The best and most stable pose was submitted for ligand-protein docking simulations. The ligand was docked using Glide XP extra precision and furthermore, the OPLS4 force field through sampling flexible ligand structures as well as ring conformations and nitrogen inversions were applied.
2.11 Statistical analysis
Group data are presented as means ± standard errors of the means (SEM). The Student’s t-test was used to determine differences between means using the GraphPad Prism 9. A p-value < 0.05 was considered significant.
3 Results
3.1 Ligand-based virtual screening using topological indexes
Due to their reported antibacterial properties (Ho Sui et al., 2012; Miró-Canturri et al., 2021b), 4-hydroxytamoxifen and raloxifene were selected as reference structures for 2D similarity search to identify new antibacterial compounds. With this aim, we selected the MBC library to be screened in silico. MBC library is a suitable reservoir of hits for drug discovery due to its high quality in terms of structural diversity and drug-like properties. Moreover, physicochemical and pharmaceutical properties have been calculated and compared with those of other well-known publicly available libraries corroborating this fact (Ginex et al., 2023). For the prioritization of compounds, four well-known 2D similarity metrics, Buser, Cosine, Kulczynski and Tanimoto indexes were used (Bender et al., 2004). A total of 12 heterocyclic compounds from LBVS were selected for further in vitro studies (Supplementary Table S1). Their similarity indexes with respect to 4-hydroxytamoxifen and raloxifene are reported in Supplementary Table S2 of Supporting Information.
3.2 In vitro activity of thiophene derivatives in Acinetobacter baumannii and Escherichia coli reference strains
Compounds initially selected were screened using a microdilution assay against A. baumannii ATCC 17978 and E. coli ATCC 25922 strains to determine the MIC. Two of them, derivatives AGR1.229 (1) and AGR1.230 (2) showed MICs ranging from 16 to 64 mg/L (Supplementary Table S1). Interestingly, both have a central thiophene scaffold disubstituted with a benzamide group and a phenyl ring attached at positions 2 and 5 respectively. A piperidine linked by an oxygen atom in ortho and meta position respectively is also present in the molecules. These interesting activities motivated us to evaluate different structurally related thiophenes in the same initial screening (Figure 1). Such is the case of 12 new structurally related thiophenes, compounds 4-15, with different substituents into the amide group linked to the thiophene ring and with the piperidin-4-yloxy group in ortho, meta and para position. Additionally, 12 more compounds where the heterocyclic central scaffold was changed to furan or thiazole moieties were also evaluated, compounds 16-23 and 24-27 respectively, in order to see the influence of the main heterocycle in the antibacterial activity. In total 24 new compounds can be found in Table 1 together with their antimicrobial activities. From them, 14 derivatives exhibited MICs ranging from 4 to 64 mg/L (Table 1).
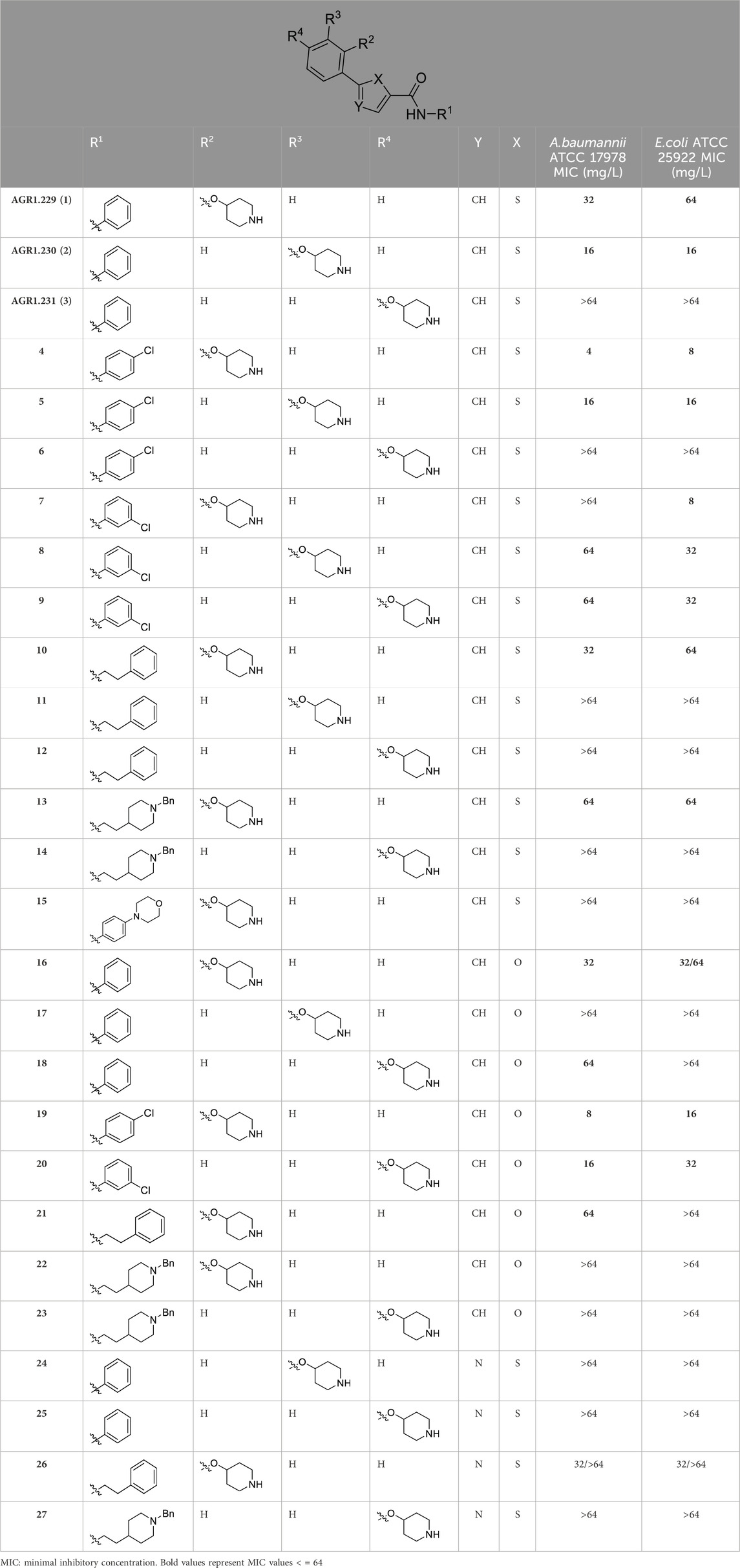
Table 1. Antibacterial activity of new heterocyclic compounds in Acinetobacter baumannii and Escherichia coli reference strains.
Regarding initial evaluated thiophene derivatives (AGR1.229 (1), AGR1.230 (2) and AGR1.231 (3)), the three of them have a benzamide in position 2 and piperidin-4-yloxy group in ortho, meta and para respectively. When this substituent is in ortho position (thiophene 1), the MIC in A. baumannii and E. coli was reduced with respect to the MIC of thiophene 3 with the substituent in para to 32 and 64 mg/L. A more potent reduction was observed when the substituent was in meta (thiophene 2) reaching a MIC level of 16 mg/L against both pathogens.
Substitution in the amide of position 2 of the thiophene ring with a 4-chlorophenyl substituent led to a more potent compound in the case of o-derivatives (4 vs. 1), but in the case of m-derivatives (5 vs. 2) did not improve the MIC, which remained at 16 mg/L against both pathogens. Finally, p-derivatives (6 vs. 3) remained inactive.
When thiophenes were substituted with a 3-chlorophenyl moiety the o-derivative 7 showed a MIC in E. coli of 8 mg/L but a complete lack of activity in A. baumannii whilst the m-derivative 8 did not show an improved MIC. Lastly, p-derivative 9 showed a reduced MIC in both pathogens with respect to inactive analogs 3 and 6.
Furthermore, the change of the phenyl substituent of the amide by phenetyl substituent led to inactive compounds in the case of m- and p-derivatives 11 and 12, respectively, while the o-derivative 10 did not change the MIC with respect to the parent compound (thiophene 1), which remained at 32 and 64 mg/L against A. baumannii and E. coli, respectively.
Introduction of substituents in the amide like 2-(1-benzylpiperidin-4-yl)ethyl or morpholinophenyl (thiophenes 13, 14 and 15) did not seem to be critical for potentiating the antibacterial activity, as the MIC values were >64 mg/L in all the cases.
Moreover, when thiophene ring was substituted by furan and thiazole core no significant improvement was observed with respect to the parent thiophene hit (e.g., furan 16 vs. thiophene 1, furan 19 vs. thiophene 4 or furan 20 vs. thiophene 9).
As a next step, those compounds with a MIC ≤64 mg/L for both pathogens were evaluated against 16 clinical strains of Col-R A. baumannii and E. coli to extend their antibacterial effect to antimicrobial resistant strains. Of note, the MIC50 values for colistin are 128 mg/L and 8 mg/L for Col-R A. baumannii and E. coli, respectively. As shown in Table 2, compounds 4, 5 and 8 exhibited stronger antibacterial activity against Col-R A. baumannii, with an MIC50 (minimum concentrations that inhibit 50% of the screened strains) value of 16, 16 and 32 mg/L, respectively, and against Col-R E. coli, with MIC50 values of 8, 32 and 32 mg/L, respectively. However, compounds 1, 2, 9, 10, 13, 16, 19 and 20 showed higher MIC50 values ranging from 64 to >64 mg/L to both pathogens.
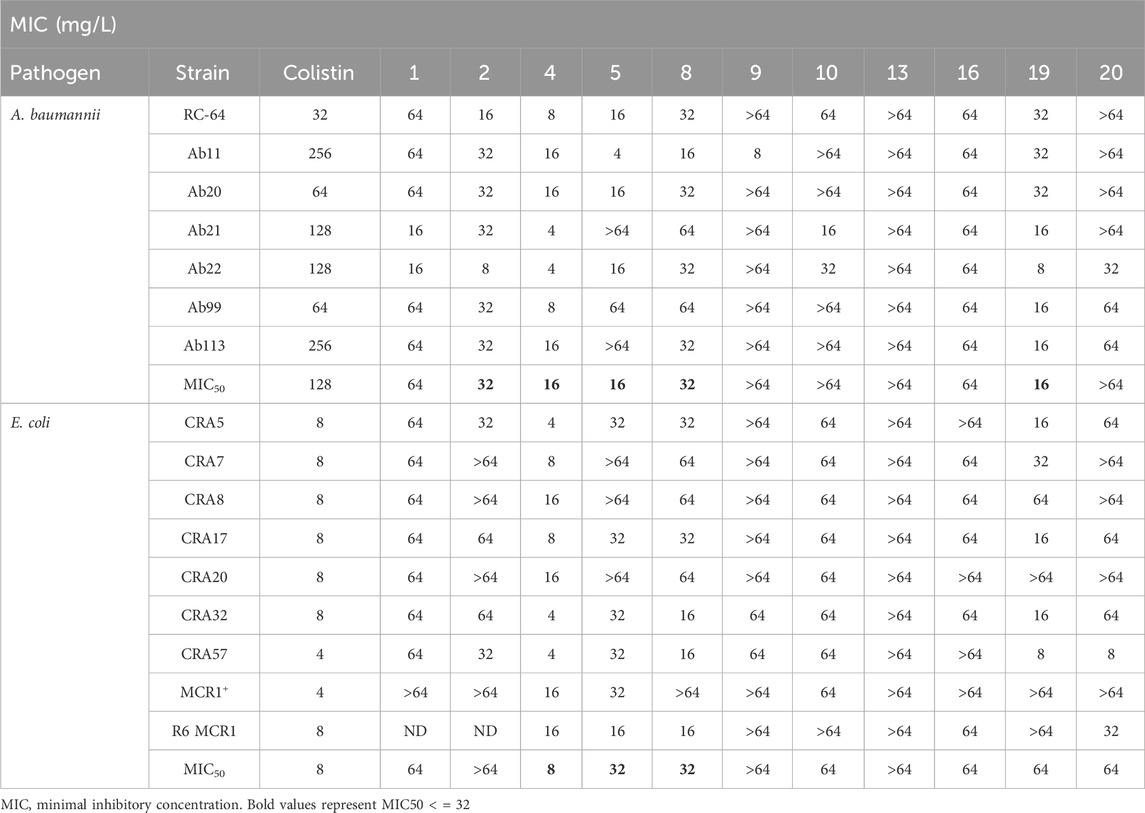
Table 2. MIC of selected thiophene derivatives and colistin for clinical colistin-resistant Acinetobacter baumannii and Escherichia coli.
3.3 Time-kill curves
Using time-course assays, we evaluated the bactericidal and bacteriostatic activities of the most potent thiophene derivatives (4, 5 and 8, Figure 1) against A. baumannii Ab11 and Ab21 strains, and E. coli MCR1+ and R6 MCR1 strains. Figure 2 illustrates that thiophene 4 at 2xMIC and 4xMIC exhibited bactericidal effects after 8 h treatment, reducing the bacterial count of the Ab21 strain by over 5.5 log10 CFU/mL. For the MCR1+ strain, thiophene 4 at 2xMIC and 4xMIC presented bactericidal activity after 24 h, reducing the bacterial count by over 3 and 5.5 log10 CFU/mL, respectively.
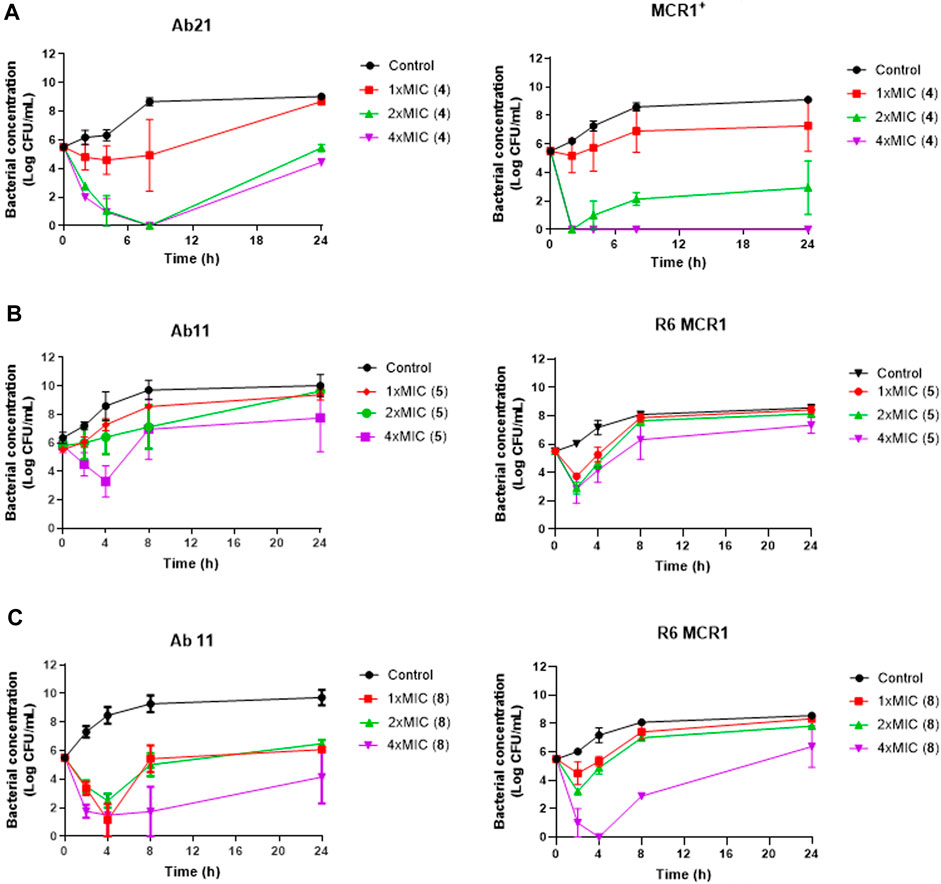
Figure 2. Antibacterial activity of thiophene derivatives 4, 5 and 8 at different concentrations against clinical colistin-resistant A. baumannii and E. coli. (A) Time-kill curves of Acinetobacter baumannii Ab21 and Escherichia coli MCR1+ strains in the presence of 1x and 1x, 2x and 4xMIC thiophene 4 for 24 h. The thiophene 4 MIC for Ab21 and MCR1+ strains is 4 and 16 mg/L. (B, C) Time-kill curves of Acinetobacter baumannii Ab11 and Escherichia coli R6 MCR1 strains in the presence of 1x and 1x, 2x and 4xMIC thiophene 5 and 8, respectively, for 24 h. The thiophene 5 MIC for Ab11 and R6 MCR1 strains is 4 and 16 mg/L, and thiophene 8 MIC for Ab11 and R6 MCR1 strains is 16 mg/L, and Data are represented as mean from two independent experiments.
In the case of thiophene 5, no bactericidal activity against Ab11 and R6 MCR1 strains was found at 1xMIC, 2xMIC, and 4xMIC during 24 h (Figure 2).
For thiophene 8, bactericidal activity against Ab11 and R6 MCR1 strains was found at 4xMIC at 8 h post-treatment. In contrast, this compound at 1x and 2xMIC did not show bactericidal activity during 24 h (Figure 2). Of note, and an exception for thiophene 4 at 4xMIC against MCR1+ strain, the complete inhibition of bacterial growth was not achieved by any of the compounds.
3.4 Effect of thiophene derivatives on the bacterial cell membrane
In order to determine the mode of action of the thiophenes 4, 5 and 8, we examined their effect on the membrane permeability of A. baumannii Ab11 and Ab21 strains, and E. coli MCR1+ and R6 MCR1 strains, by incubating them with ethidium homodimer-1, a DNA stain that is unable to cross intact membranes. Fluorescence microscopy analysis showed an increase in cellular fluorescence of the Ab21 and MCR1+ strains in the presence of 0.5xMIC of compound 4, and the Ab11 and R6 MCR1 strains in the presence of 0.5xMIC of compounds 5 or 8, with a higher effect observed in the case of treatment with compound 4 (Figure 3A).
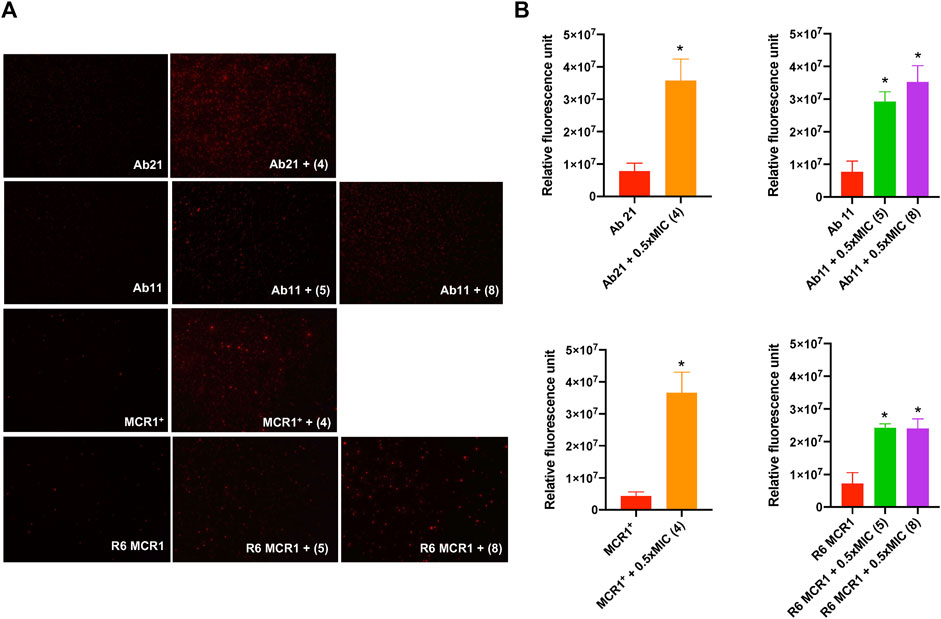
Figure 3. Effect of thiophene derivatives 4, 5 and 8 on the bacterial permeability against clinical colistin-resistant A. baumannii and (E) coli. (A) The membrane permeabilization of colistin-resistant Acinetobacter baumannii and Escherichia coli strains in the absence and presence of 0.5xMIC of thiophenes 4, 5 and 8, using fluorescence microscopy. (B) The membrane permeabilization of colistin-resistant Acinetobacter baumannii and Escherichia coli strains in the absence and presence of 0.5xMIC of thiophenes 4, 5 and 8, incubated for 10 min, was quantified by Typhon Scanner. Data are represented as mean ± SEM from three independent experiments. Acinetobacter baumannii Ab21 and Escherichia coli MCR1+ strains were treated with thiophene 4. Acinetobacter baumannii Ab11 and Escherichia coli R6 MCR1 strains were treated with thiophenes 5 and 8. The thiophene 4 MIC for Ab21 and MCR1+ strains is 4 and 16 mg/L, the thiophene 5 MIC for Ab11 and R6 MCR1 strains is 4 and 16 mg/L, and the thiophene 8 MIC for Ab11 and R6 MCR1 strains is 16 mg/L *p < 0.05 untreated cells compared with thiophene derivative treatment.
Moreover, fluorescence monitoring using a Typhoon FLA scanner for 3 h confirmed the results obtained by fluorescence microscopy, indicating that the three thiophene derivatives significantly increase the membrane permeability of these strains (Figure 3B). Basal levels of fluorescence were observed in the control. These data suggest that thiophene 4, 5 and 8 affect the integrity of the bacterial cell wall of A. baumannii and E. coli.
3.5 Effect of thiophene derivatives on the OMP profiles
The OMP profiles of the A. baumannii strains Ab11 and Ab21, and E. coli strains MCR1+ and R6 MCR1, did not show significant changes between the control and treatment conditions after incubation for 24 h with 0.5xMIC of thiophenes 4, 5 and 8 (Supplementary Figure S2). These results suggest that the thiophene derivatives did not affect the expression of OMPs.
3.6 Effect of thiophene derivatives on the bacterial adherence to host cells
To evaluate the effect of selected thiophenes on A. baumannii and E. coli interaction with host cells, we studied the adherence of Ab11, Ab21, MCR1+, and R6 strains to HeLa cells for 2 h in the presence of the compounds. Treatment with thiophene 4 at 1xMIC reduced the adherence of Ab21 and MCR1+ strains to HeLa cells by 47% (p < 0.05) and 51% (p < 0.05), respectively. Notably, a lesser significant reduction was observed with thiophenes 5 or 8, where both compounds reduced the adherence of Ab11 strain by 38% (p < 0.05) and 37% (p < 0.05), respectively, and the adherence of R6 MCR1 strain by 48% (p < 0.05) and 42% (p < 0.05), respectively (Figure 4).
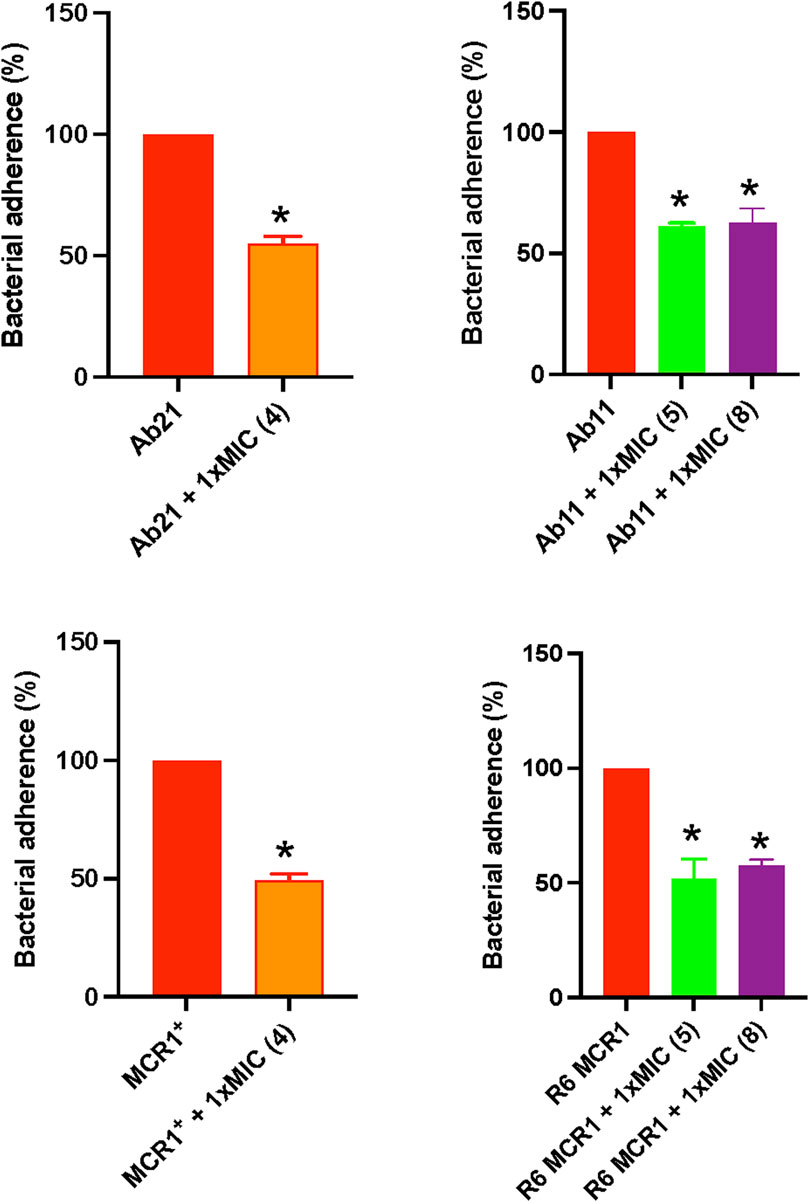
Figure 4. Effect of thiophene derivatives 4, 5 and 8 on clinical colistin-resistant Acinetobacter baumannii and Escherichia coli interaction with host cells. Bacteria were treated with thiophene 4 (1xMIC), 5 (1xMIC) and 8 (1xMIC) for 30 min before to infect HeLa cells. The assay of Acinetobacter baumannii and Escherichia coli adherence to HeLa cells for 2 h was performed as described under “Materials and Methods section”. The thiophene 4 MIC for Ab21 and MCR1+ strains is 4 and 16 mg/L, the thiophene 5 MIC for Ab11 and R6 MCR1 strains is 4 and 16 mg/L, and the thiophene 8 MIC for Ab11 and R6 MCR1 strains is 16 mg/L. Data are represented as mean ± SEM from three independent experiments. *, p < 0.05 untreated cells compared with thiophene derivative treatment.
3.7 Docking and molecular modelling
As thiophene 4 has shown better antimicrobial resistant activity in terms of MIC50, time-kill curve, cell permeability, and bacterial adherence to host, we next selected this compound for the following studies. To throw some light inside its mechanism of action, compound 4 was subsequently docked in the transmembrane (TM) domain of different outer membrane proteins such as OmpA, Omp33, OmpW, and CarO1 of A. baumannii and ranked on the basis of the scoring function (see “Methods” section). As no three-dimensional structures of the TM domain of A. baumannii OmpA and OmpW were found in the PDB, a homology model using the I-TASSER server was built using the amino acid sequence for OmpA and OmpW of A. baumannii (GenBank: AXV53527.1, and ADX03096.1 respectively) using as templates the known 3D structures of OmpA and OmpW of E. coli (PDB IDs: 1qjp, and 2f1v respectively). Similar docking studies were performed for the OmpA, OmpW, OmpC, and OmpF of E. coli.
Thiophene 4 showed better docking score in A. baumannii CarO1and Omp33 and E. coli OmpW and OmpC compared to two other porins (Supplementary Table S3). The most stable pose shows that thiophene 4 binds to Tyr108 of CarO1, and has a powerful interactions with Phe43 of OmpW through π-π stacking bonds with the thiophene ring (Figure 5). The activity of thiophene 4 against A. baumannii ATCC 17978 and E. coli MG1655 is lower when both strains are deficient in Omp33 and OmpC, by increasing MIC 2 and 4 times, respectively (Table 3).
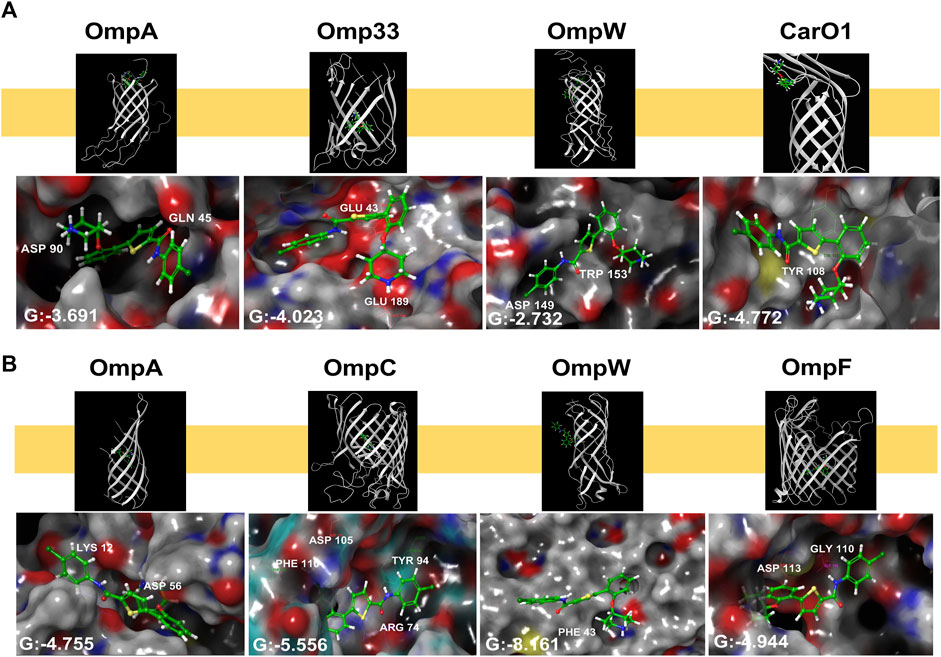
Figure 5. Structural models generated by docking of thiophene derivative 4 into the transmembrane domain of Acinetobacter baumannii Omp33 and CarO1 (A) and Escherichia coli OmpA, OmpC, OmpW and OmpF (B), and into the homology model of Acinetobacter baumannii OmpA and OmpW generated as described under “Methods”. Thiophene 4 is displayed as sticks. G: Glide score.
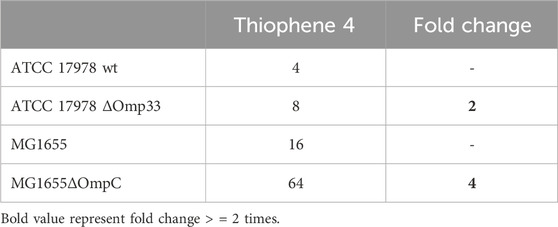
Table 3. MIC of thiophene derivative 4 for Acinetobacter baumannii ATCC 17978 and Escherichia coli MG1655 and its isogenic Omp33 and OmpC-deficient strains, respectively.
4 Discussion
The emergence of multidrug-resistant GNB (MDR-GNB) has prompted the use of colistin as a last resort in the treatment of severe infections caused by these pathogens. Although uncommon, colistin resistance is increasing, and its spread is considered a global health threat (Giamarellou, 2016).
Due to the antibacterial effect of tamoxifen and its metabolites in A. baumannii and E. coli (Miró-Canturri et al., 2021b; Miró-Canturri et al., 2021c), we hypothesized that thiophene derivatives identified in this study after a similarity search based on fingerprint analysis, may exhibit good antibacterial activity against A. baumannii and E. coli resistant to colistin. From initial experimental confirmation of thiophenes AGR1.229 (1) and AGR1.230 (2), a total of new 24 compounds including thiophene and related furan and thiazole derivatives were initially screened against A. baumannii ATCC 17978 and E. coli ATCC 25922 strains (Table 1). Some of these derivatives showed MICs ranging from 4 to 64 mg/L. It is noteworthy that thiophene derivatives did not show antibacterial activity against P. aeruginosa (data not shown), consistent with previously published data on the antibacterial activity of the anticancer drug family tamoxifen and tamoxifen metabolites used as starting points of this work (Miró-Canturri et al., 2021b; Miró-Canturri et al., 2021c).
The MIC50 values for thiophenes 4, 5 and 8 against Col-R A. baumannii were 16, 16 and 32 mg/L, respectively, while for Col-R E. coli, were 8, 32, and 32 mg/L, respectively (Table 2), which overall fell within the range of other known antibiotics such as amikacin, amoxicillin-clavulanic, ceftazidime-avibactam, fosfomycin, among others (EUCAST, 2024). However, the MIC50 values for the other thiophene derivatives were higher than 32 mg/L. Reasons for this difference could be related to the chemical structure differences between these derivatives. The three thiophene derivatives selected for further studies have a substituent with chlorine (Figure 1).
The antibacterial activity of the thiophene 4 at 2x and 4xMIC against Col-R strains of A. baumannii and E. coli is higher than that of thiophenes 5 and 8 (Figure 2). This result may be related to the position of the piperidin-4-yloxy group in the structure. While in thiophene 4, it is located in ortho position of the phenyl group attached to the 5-position of the thiophene, in derivatives 5 and 8, is attached in para.
Moreover, the piperidin-4-yloxy group of the thiophenes 5 and 8 is located in the same position para; however, the thiophene 8 at 4xMIC showed more antibacterial activity in the first 6 h of bacterial growth than thiophene 5. This could be related to the chlorophenyl position in the R1.
The antimicrobial activity of the selected derivatives identified in this work hints at a promising potential that deserves to be further explored in vivo after determining their pharmacokinetic parameters. However, in vitro bacterial growth showed a progressive regrowth of the Col-R A. baumannii and Col-R E. coli strains after treatment with thiophene 4 at 2xMIC and 4xMIC, suggesting that the A. baumannii and E. coli strains have acquired resistance to this derivative. Nevertheless, it should be noted that the MICs of thiophene 4 against for Ab21 and MCR1+ strains in this time-kill assays condition were 64 and 32 mg/L, respectively, which are below the 4x MIC of the thiophene 4. Further investigations, including the determination of its concentration during the time-kill assay, are necessary to better understand the regrowth of these strains in the presence of this compound.
Additionally, OMP profiles of A. baumannii and E. coli strains were not modified after incubation with the three selected thiophene derivatives, indicating that changes in OMP expression could not be related to the mechanism of action of these compounds. Miro Canturri et al. reported similar findings, where tamoxifen metabolites did not alter the expression of OMPs of A. baumannii and E. coli MDR strains (Miró-Canturri et al., 2021b). However, we demonstrated that these thiophene derivatives increased the membrane permeability of A. baumannii and E. coli strains (Figure 3). Similar results were observed in studies on tamoxifen metabolites (Miró-Canturri et al., 2021a; Miró-Canturri et al., 2021b). It is known that the mechanism of action of the anticancer drug tamoxifen in fungi is related to its binding to calmodulin (Dolan et al., 2009; Butts et al., 2015). Additionally, Scott et al. showed that 4-hydroxytamoxifen, a tamoxifen metabolite, might inhibit bacterial phospholipase D (Scott et al., 2015). Further studies on the mechanism of action of thiophene derivatives against A. baumannii and E. coli and their therapeutic efficacy in animal experimental models of infection would be of interest.
It is widely known that OMPs are among the most relevant bacterial targets for antibacterial compounds (Rosas and Lithgow, 2021). Tamoxifen and its metabolites, used here as a chemical template to search similar compound based on topological indexes, are widely associated with the inhibition of bacterial cell membrane function (Luxo et al., 2003; El Arbi et al., 2014; Feng et al., 2015). This is primarily due to the hydrophobic nature of tamoxifen, stemming from the presence of alkyl groups attached to the amino group in its structure (Levinson, 2017). Additionally, a recent study from our group showed that 4-hydroxytamoxifen, a major tamoxifen metabolite, binds rigorously to OmpW of A. baumannii (Vila Dominguez et al., 2022).
For these reasons, we decided to carry out a preliminary computational study to determine if thiophene 4 potentially bind to OMPs. Eight porins were considered, four from A. baumannii (OmpA, Omp33, OmpW and CarO1) and four from E. coli (OmpA, OmpC, OmpW and OmpF). As a result, this compound exhibited a better docking score in CarO1 and Omp33 of A. baumannii and OmpW and OmpC of E. coli compared to other porins of A. baumannii and E. coli. The results revealed a potent π-π stacking interaction between Tyr108 of CarO1 and Phe43 of OmpW and the thiophene ring. For Omp33, thiophene 4 has an affinity for the structure of glutamic acids (Glu189 and Glu43) through its piperidinyl ring. However, for OmpC, this compound shows a strong tendency to bind to Arg74, Phe110, Asp105, and Tyr94 through its carbonyl group, piperidinyl ring, piperidinyl ring, and carbonyl group, respectively. These interactions are so potent in a way that they lead to much greater stability of ligand 4 in the binding pocket to these OMPs. Omp33 and OmpC constitute the main OMPs in A. baumannii and E. coli that are necessary for drug transport across cellular membranes (Smani et al., 2013; Rumbo et al., 2014; Bafna et al., 2020). Biological confirmatory assays showed that their deficiencies in Acinetobacter bauamnnii and E. coli, respectively, allowed both pathogens to be less susceptible to thiophene 4.
The findings of this study showed that the existence of a thiophene ring, an amide group, piperidinyl and carbonyl groups plays a crucial role in the chemical structure of the ligand to interact with OMPs and subsequently to show antimicrobial activity. Thus, it is plausible that thiophene 4 have more than one OMP target in A. baumannii which may explain the higher antibacterial activity observed against A. baumannii ATCC 17978 and E. coli ATCC 25922.
5 Conclusion
The results of this study provide new insights into the use of thiophene derivatives against Col-R A. baumannii and E. coli, where therapeutic options are limited. Despite the potential showed by this new family of antibacterial compounds, further studies are necessary to elucidate the in vivo mechanism of action of this chemical class and their implications for the host, as well as to determine an optimal dosage that achieves therapeutic efficacy in the treatment of severe infections caused by MDR-GNB.
Data availability statement
The data supporting the findings of this study are available from the corresponding author upon reasonable request.
Ethics statement
Ethical approval was not required for the studies on humans in accordance with the local legislation and institutional requirements because only commercially available established cell lines were used.
Author contributions
IM-P: Writing–original draft, Methodology, Investigation, Formal Analysis. MM-T: Writing–review and editing, Methodology, Investigation. AG-R: Writing–review and editing, Methodology, Investigation. TG: Writing–review and editing, Methodology, Investigation. KE: Writing–review and editing, Methodology, Investigation. AM: Writing–review and editing, Supervision, Funding acquisition, Conceptualization. CG: Writing–review and editing, Supervision, Funding acquisition, Conceptualization. YS: Writing–review and editing, Writing–original draft, Supervision, Funding acquisition, Formal Analysis, Conceptualization.
Funding
The author(s) declare that financial support was received for the research, authorship, and/or publication of this article. This work was funded by the Ministerio de Ciencia e Innovación, Agencia Estatal de Investigación, Fondo Europeo de Desarrollo Regional, MCIN/AEI/10.13039/501100011033/FEDER, UE (grant PID2022-136357OB-I00), the Consejería de Universidad, Investigación e Innovación de la Junta de Andalucía (grant ProyExcel_00116), and the Instituto de Salud Carlos III, Subdirección General de Redes y Centros de Investigación Cooperativa, Ministerio de Economía, Industria y Competitividad (grant PI19/01009), cofinanced by the European Development Regional Fund (A way to achieve Europe, Operative Program Intelligent Growth, 2014–2020). This research work was also funded by the European Commission–NextGenerationEU (Regulation EU 2020/2094), through CSIC’s Global Health Platform (PTI Salud Global). MM-T holds a pre-doctoral FPU grant (FPU18/03493) from MICINN.
Acknowledgments
We thank Andrea Vila Domínguez and the Microscopy facility of the Andalusian Center of Developmental Biology for their technical help.
Conflict of interest
The authors declare that the research was conducted in the absence of any commercial or financial relationships that could be construed as a potential conflict of interest.
The author(s) declared that they were an editorial board member of Frontiers, at the time of submission. This had no impact on the peer review process and the final decision.
Publisher’s note
All claims expressed in this article are solely those of the authors and do not necessarily represent those of their affiliated organizations, or those of the publisher, the editors and the reviewers. Any product that may be evaluated in this article, or claim that may be made by its manufacturer, is not guaranteed or endorsed by the publisher.
Supplementary material
The Supplementary Material for this article can be found online at: https://www.frontiersin.org/articles/10.3389/fphar.2024.1412797/full#supplementary-material
References
Abellón-Ruiz, J., Zahn, M., Baslé, A., and van den Berg, B. (2018). Crystal structure of the Acinetobacter baumannii outer membrane protein Omp33. Acta Cryst. 74 (9), 852–860. doi:10.1107/S205979831800904X
Årdal, C., Baraldi, E., Ciabuschi, F., Outterson, K., Rex, J. H., Piddock, L. J. V., et al. (2017). To the G20: incentivising antibacterial research and development. Lancet Infect. Dis. 17, 799–801. doi:10.1016/S1473-3099(17)30404-8
Bafna, J. A., Sans-Serramitjana, E., Acosta-Gutiérrez, S., Bodrenko, I. V., Hörömpöli, D., Berscheid, A., et al. (2020). Kanamycin uptake into Escherichia coli is facilitated by OmpF and OmpC porin channels located in the outer membrane. ACS Infect. Dis. 6, 1855–1865. doi:10.1021/acsinfecdis.0c00102
Bagley, N., and Outterson, K. (2017). We will miss antibiotics when they're Gone. Available at: www.nytimes.com/2017/01/18/opinion/how-to-avoid-a-post-antibiotic-world.html (Accessed April 15, 2021).
Baslé, A., Rummel, G., Storici, P., Rosenbusch, J. P., and Schirmer, T. (2006). Crystal structure of osmoporin OmpC from E. coli at 2.0 Å. J. Mol. Biol. 362 (5), 933–942. doi:10.1016/j.jmb.2006.08.002
Bender, A., Mussa, H. Y., Glen, R. C., and Reiling, S. (2004). Similarity searching of chemical databases using atom environment descriptors (MOLPRINT 2D): evaluation of performance. J. Chem. Infor. Comp. Sci. 44, 1708–1718. doi:10.1021/ci0498719
Butts, A., Martin, J. A., DiDone, L., Bradley, E. K., Mutz, M., and Krysan, J. (2015). Structure-activity relationships for the antifungal activity of selective estrogen receptor antagonists related to tamoxifen. PLoS One 10, e0125927. doi:10.1371/journal.pone.0125927
Canturri, A. M., and Smani, Y. (2022). Anthelmintic drugs for repurposing against Gram-negative bacilli infections. Curr. Med. Chem. 30 (1), 59–71. doi:10.2174/0929867329666220714092916
Corral, C., Madroñero, R., and Ulecia, N. (1978). Bischler and friedlaender reactions with 2-amino-3-aroylthiophenes. Afinidad 35, 129–133.
Dolan, K., Montgomery, S., Buchheit, B., DiDone, L., Wellington, M., and Krysan, D. J. (2009). Antifungal activity of tamoxifen: in vitro and in vivo activities and mechanistic characterization. Antimicrob. Agents Chemother. 53, 3337–3346. doi:10.1128/AAC.01564-08
Ei Arbi, M., Théolier, J., Pigeon, P., Jellali, K., Trigui, F., Top, S., et al. (2014). Antibacterial properties and mode of action of new triaryl butene citrate compounds. Eur. J. Med. Chem. 76, 408–413. doi:10.1016/j.ejmech.2014.02.037
European Committee on Antimicrobial Susceptibility Testing (2022). European antimicrobial breakpoints. Basel: eucast (Accessed January 15, 2022).
European Committee on Antimicrobial Susceptibility Testing (2024). European antimicrobial breakpoints. Basel: EUCAST (Accessed June 4, 2024).
Feng, X., Zhu, W., Schurig-Briccio, L. A., Lindert, S., Shoen, C., Hitchings, R., et al. (2015). Antiinfectives targeting enzymes and the proton motive force. Proc. Natl. Acad. Sci. USA. 112, E7073–E7082. doi:10.1073/pnas.1521988112
Flores, R., Döhrmann, S., Schaal, C., Hakkim, A., Nizet, V., and Corriden, R. (2016). The selective estrogen receptor modulator raloxifene inhibits neutrophil extracellular trap formation. Front. Immunol. 7, 566. doi:10.3389/fimmu.2016.00566
Friesner, R. A., Murphy, R. B., Repasky, M. P., Frye, L. L., Greenwood, J. R., Halgren, T. A., et al. (2006). Extra Precision Glide: docking and scoring incorporating a model of hydrophobic enclosure for protein−ligand complexes. J. Med. Chem. 49, 6177–6196. doi:10.1021/jm051256o
Gálvez-Benítez, L., de la Rosa, J. M. O., Rodriguez-Villodres, A., Casimiro-Soriguer, C. S., Molina-Panadero, I., Alvarez-Marin, R., et al. (2023). Role of blaTEM and OmpC in the piperacillin-tazobactam resistance evolution by E. coli in patients with complicated intra-abdominal infection. J. Infect. 87 (3), 220–229. doi:10.1016/j.jinf.2023.07.005
Gálvez-Benítez, L., Rodríguez-Villodres, Á., Álvarez-Marín, R., Jiménez-Rodríguez, R., Lepe-Jiménez, J. A., Pachón, J., et al. (2021). Impact of the COVID-19 pandemic on survival in the patients with the intra-abdominal infections. Front. Med. 8, 687415. doi:10.3389/fmed.2021.687415
Gentile, F., Yaacoub, J. C., Gleave, J., Fernández, M., Ton, A. T., Ban, F., et al. (2022). Artificial intelligence–enabled virtual screening of ultra-large chemical libraries with deep docking. Nat. Prot. 17, 672–697. doi:10.1038/s41596-021-00659-2
Giamarellou, H. (2016). Epidemiology of infections caused by polymyxin-resistant pathogens. Int. J. Antimicrob. Agents 48, 614–621. doi:10.1016/j.ijantimicag.2016.09.025
Gil, C., and Martinez, A. (2021a). Is drug re-purposing really the future of drug discovery or is new innovation truly the way forward? Expert Opin. Drug Discov. 16, 829–831. doi:10.1080/17460441.2021.1912733
Gil, C., Martínez, A., Morales-Tenorio, M., Garcia-Rubia, A., and Smani, Y. (2023). Nuevos derivados útiles como agentes antibacterianos (P202330628). Priority date: 24/07/2023.
Ginex, T., Madruga, E., Martinez, A., and Gil, C. (2023). MBC and ECBL libraries: outstanding tools for drug discovery. Fronnt. Pharmacol. 14, 1244317. doi:10.3389/fphar.2023.1244317
Hong, H., Patel, D. R., Tamm, L. K., and van den Berg, B. (2006). The outer membrane protein OmpW forms an eight-stranded beta-barrel with a hydrophobic channel. J. Biol. Chem. 281 (11), 7568–7577. doi:10.1074/jbc.M512365200
Ho Sui, S. J., Lo, R., Fernandes, A. R., Caulfield, M. D. G., Lerman, J. A., Xie, L., et al. (2012). Raloxifene attenuates Pseudomonas aeruginosa pyocyanin production and virulence. Int. J. Antimicrob. Agents 40, 246–251. doi:10.1016/j.ijantimicag.2012.05.009
Kung, V. L., Ozer, E. A., and Hauser, A. R. (2010). The accessory genome of Pseudomonas aeruginosa. Microbiol. Mol. Biol. Rev. 74, 621–641. doi:10.1128/MMBR.00027-10
Levinson, N. S. (2017) “Towards the elucidation of the mechanism of the antibiotic activity of tamoxifen,” in MS dissertation in Chemistry, college of science. Atlanta, United States: Georgia Institute of Technology. Available at: https://smartech.gatech.edu/bitstream/handle/1853/58251/LEVINSON-THESIS-2017.pdf.
López-Rojas, R., Jiménez-Mejías, M. E., Lepe, J. A., and Pachón, J. (2011). Acinetobacter baumannii resistant to colistin alters its antibiotic resistance profile: a case report from Spain. J. Infect. Dis. 204, 1147–1148. doi:10.1093/infdis/jir476
Luxo, C., Jurado, A. S., Madeira, V. M., and Silva, M. T. (2003). Tamoxifen induces ultrastructural alterations in membranes of Bacillus stearothermophilus. Toxicol. Vitro. 17, 623–628. doi:10.1016/s0887-2333(03)00113-9
Mabkhot, N. Y., Alatibi, F., El-Sayed, N. N. E., Al-Showiman, S., Kheder, N. A., Wadood, A., et al. (2016). Antimicrobial activity of some novel armed thiophene derivatives and Petra/Osiris/Molinspiration (POM) analyses. Molecules 21 (2), 222. doi:10.3390/molecules21020222
Maggiora, G., Vogt, M., Stumpfe, D., and Bajorath, J. (2014). Molecular similarity in medicinal chemistry. J. Med. Chem. 57, 3186–3204. doi:10.1021/jm401411z
Miró-Canturri, A., Ayerbe-Algaba, R., Del Toro, R., Mejías, M. E., Pachón, J., and Smani, Y. (2021c). Potential tamoxifen repurposing to combat infections by multidrug-resistant Gram-negative bacilli. Pharmaceuticals 14, 507. doi:10.3390/ph14060507
Miró-Canturri, A., Ayerbe-Algaba, R., and Smani, Y. (2019). Drug repurposing for the treatment of bacterial and fungal infections. Front. Microbiol. 10, 41. doi:10.3389/fmicb.2019.00041
Miró-Canturri, A., Ayerbe-Algaba, R., Vila-Domínguez, A., Jiménez-Mejías, M. E., Pachón, J., and Smani, Y. (2021b). Repurposing of the tamoxifen metabolites to combat infections by multidrug-resistant gram-negative bacilli. Antibiotics 10, 336. doi:10.3390/antibiotics10030336
Miró-Canturri, A., Ayerbe-Algaba, R., Villodres, Á. R., Pachón, J., and Smani, Y. (2020). Repositioning rafoxanide to treat Gram-negative bacilli infections. J. Antimicrob. Chemother. 75, 1895–1905. doi:10.1093/jac/dkaa103
Miró-Canturri, A., Vila-Domínguez, A., Caretero-Ledesma, M., Ayerber-Algaba, R., Pachón, J., Jiménez-Mejías, M. E., et al. (2021a). Repurposing of the tamoxifen metabolites to treat methicillin-resistant Staphylococcus epidermidis and vancomycin-resistant Enterococcus faecalis infections. Microbiol. Spectr. 9, e0040321. doi:10.1128/Spectrum.00403-21
Muegge, I., and Mukherjee, P. (2016). An overview of molecular fingerprint similarity search in virtual screening. Expert Opin. Drug Discov. 11, 137–148. doi:10.1517/17460441.2016.1117070
Narender, T., and Papi Reddy, K. (2007). A simple and highly efficient method for the synthesis of chalcones by using borontrifluoride-etherate. Tetrahedron Lett. 48, 3177–3180. doi:10.1016/j.tetlet.2007.03.054
O'Neill, J. (2015). Tackling drug-resistant infections globally: final report and recommendations. Available at: https://amrreview.org/sites/default/files/160525_Final_paper_with_cover.pdf (Accessed April 01, 2015).
Palomo, V., Pérez, D. I., Pérez, C., Morales-García, J. A., Soteras, I., Alonso-Gil, S., et al. (2012). 5-Imino-1,2,4-thiadiazoles: first small molecules as substrate competitive inhibitors of glycogen synthase kinase 3. J. Med. Chem. 55, 1645–1661. doi:10.1021/jm201463v
Parra-Millán, R., Guerrero-Gómez, D., Ayerbe-Algaba, R., Pachón-Ibáñez, M. E., Miranda-Vizuete, A., Pachón, J., et al. (2018). Intracellular trafficking and persistence of Acinetobacter baumannii requires Transcription Factor EB. mSphere 3, e00106–e00118. doi:10.1128/mSphere.00106-18
Rakshit, G., and Jayaprakash, V. (2023). Tuberculosis and HIV responses threatened by nCOVID-19: a situation prompting an in silico investigation of reported MbtA inhibitors for combined inhibition of SARS-CoV-2 and HIV-TB co-infection. Struct. Chem. 34, 655–679. doi:10.1007/s11224-022-02013-y
Redondo, M., Brea, J., Pérez, D. I., Soteras, I., Val, C., Pérez, C., et al. (2012). Effect of phosphodiesterase 7 (PDE7) inhibitors in experimental autoimmune encephalomyelitis mice. Discovery of a new chemically diverse family of compounds. J. Med. Chem. 55, 3274–3284. doi:10.1021/jm201720d
Roman, G. (2022). Thiophene-containing compounds with antimicrobial activity. Arch. Pharm. 355, e2100462. doi:10.1002/ardp.202100462
Rosas, N. C., and Lithgow, T. (2021). Targeting bacterial outer-membrane remodelling to impact antimicrobial drug resistance. Trends Microbiol. 30, 544–552. doi:10.1016/j.tim.2021.11.002
Rumbo, C., Tomás, M., Fernández Moreira, E., Cruz Soares, N., Carvajal, M., Santillana, E., et al. (2014). The Acinetobacter baumannii Omp33-36 porin is a virulence factor that induces apoptosis and modulates autophagy in human cells. Infect. Immun. 82 (11), 4666–4680. doi:10.1128/IAI.02034-14
Schrödinger Release 2013-3 (2013). Schrödinger suite 2013 protein preparation wizard; epik version 2.6, schrödinger, LLC, New York, NY, 2013; impact version 6.1, schrödinger, LLC, New York, NY, 2013; SiteMap, version 2.9, schrödinger, LLC, New York, NY, 2013; MacroModel version 9.9; LigPrep, version 2.8, schrödinger, LLC, New York, NY, 2013; Glide, version 6.1. New York, NY: Schrödinger, LLC.
Schrödinger Release 2021-1 (2021) CanvasFPGen and canvasFPMatrix utilities, Schrödinger, LLC, New York, NY.
Scott, S. A., Spencer, C. T., O’Reilly, M. C., Brown, K. A., Lavieri, R. R., Cho, C. H., et al. (2015). Discovery of desketoraloxifene analogues as inhibitors of mammalian, Pseudomonas aeruginosa, and nape phospholipase d enzymes. ACS Chem. Biol. 10, 421–432. doi:10.1021/cb500828m
Smani, Y., Dominguez-Herrera, J., and Pachón, J. (2013). Association of the outer membrane protein Omp33 with fitness and virulence of Acinetobacter baumannii. J. Infect. Dis. 208 (10), 1561–1570. doi:10.1093/infdis/jit386
Tacconelli, E., Carrara, E., Savoldi, A., Harbarth, S., Mendelson, M., Monnet, D. L., et al. (2018). Discovery, research, and development of new antibiotics: the WHO priority list of antibiotic-resistant bacteria and tuberculosis. Lancet Infect. Dis. 18, 318–327. doi:10.1016/S1473-3099(17)30753-3
Valencia, R., Arroyo, L. A., Conde, M., Aldana, J. M., Torres, M. J., Fernández-Cuenca, F., et al. (2009). Nosocomial outbreak of infection with pan-drug-resistant Acinetobacter baumannii in a tertiary care university hospital. Infect. Control Hosp. Epidemiol. 30, 257–263. doi:10.1086/595977
Vila Domínguez, A., Molino Panadero, I., Pachón, J., Jiménez Mejías, M. E., and Smani, Y. (2022). Identification of the outer membrane protein W (OmpW) as the potential target of tamoxifen metabolites in Acinetobacter baumannii. Poster #2, AcinetoVibes Congr. USA.
Vila-Farrés, X., Parra-Millán, R., Sánchez-Encinales, V., Varese, M., Ayerbe-Algaba, R., Bayó, N., et al. (2017). Combating virulence of Gram-negative bacilli by OmpA inhibition. Sci. Rep. 7 (1), 14683. doi:10.1038/s41598-017-14972-y
Yang, J., Yan, R., Roy, A., Xu, D., Poisson, J., and Zhang, Y. (2015). The I-TASSER Suite: protein structure and function prediction. Nat. Methods 12, 7–8. doi:10.1038/nmeth.3213
Yelin, I., and Kishony, R. (2018). Antibiotic resistance. Cell 172, 1136. doi:10.1016/j.cell.2018.02.018
Zahn, M., D’Agostino, T., Eren, E., Baslé, A., Ceccarelli, M., and van den Berg, B. (2015). Small-molecule transport by CarO, an abundant eight-stranded β-barrel outer membrane protein from acinetobacter baumannii. J. Mol. Biol. 427 (14), 2329–2339. doi:10.1016/j.jmb.2015.03.016
Keywords: new thiophene derivatives, antimicrobial resistant drugs, treatment, bacteria, infection, gram-negative
Citation: Molina-Panadero I, Morales-Tenorio M, García-Rubia A, Ginex T, Eskandari K, Martinez A, Gil C and Smani Y (2024) Discovery of new antimicrobial thiophene derivatives with activity against drug-resistant Gram negative-bacteria. Front. Pharmacol. 15:1412797. doi: 10.3389/fphar.2024.1412797
Received: 05 April 2024; Accepted: 03 July 2024;
Published: 20 August 2024.
Edited by:
Tushar Dhanani, Florida Agricultural and Mechanical University, United StatesReviewed by:
Michal Letek, University of León, SpainNadezhda A. German, Texas Tech University Health Sciences Center, United States
Tripti Nair, University of Southern California, United States
Jayanti Makasana, Marwadi University, India
Copyright © 2024 Molina-Panadero, Morales-Tenorio, García-Rubia, Ginex, Eskandari, Martinez, Gil and Smani. This is an open-access article distributed under the terms of the Creative Commons Attribution License (CC BY). The use, distribution or reproduction in other forums is permitted, provided the original author(s) and the copyright owner(s) are credited and that the original publication in this journal is cited, in accordance with accepted academic practice. No use, distribution or reproduction is permitted which does not comply with these terms.
*Correspondence: Younes Smani, eXNtYUB1cG8uZXM=