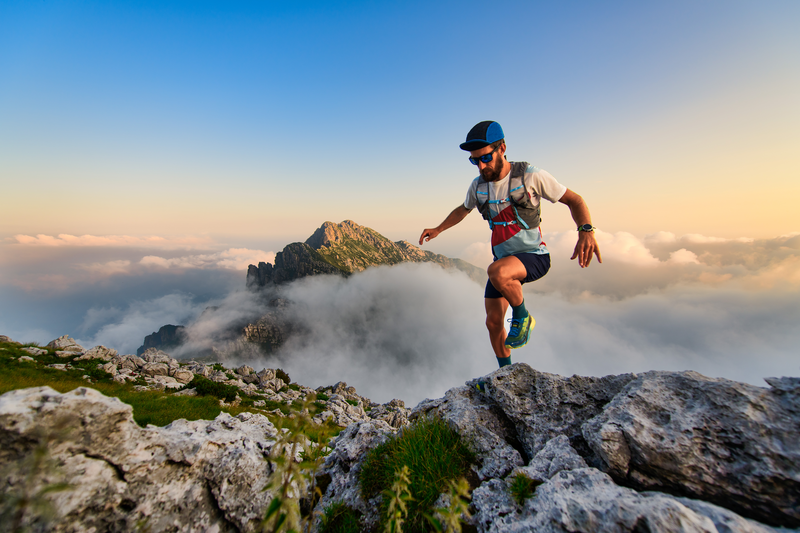
95% of researchers rate our articles as excellent or good
Learn more about the work of our research integrity team to safeguard the quality of each article we publish.
Find out more
REVIEW article
Front. Pharmacol. , 16 July 2024
Sec. Neuropharmacology
Volume 15 - 2024 | https://doi.org/10.3389/fphar.2024.1412420
This article is part of the Research Topic Medicinal Drugs, the Effect on Memories and Beyond View all 7 articles
Fluoxetine, the prototypical selective serotonin reuptake inhibitor (SSRI), is widely used to treat major depressive disorder (MDD) and a variety of other central nervous system conditions, primarily due to its established clinical safety profile. Although its efficacy in treating depression is well-recognized, the impact of fluoxetine on cognitive functions remains inconsistent and elusive. In this review, we first examine the well-substantiated biological mechanisms underlying fluoxetine’s antidepressant effects, which include serotonin reuptake inhibition and activation of TrkB receptors—key to brain-derived neurotrophic factor (BDNF) signaling. Subsequently, we delve into the cognitive side effects observed in both preclinical and clinical studies, affecting domains such as memory, attention, and executive functions. While certain studies indicate cognitive improvements in patients with underlying disorders, there is also evidence of negative effects, influenced by variables like gender, duration of treatment, age, disease pathology, and the specifics of cognitive testing. Significantly, the negative cognitive outcomes reported in preclinical research often involve healthy, non-diseased animals. This review underscores the necessity for heightened caution in fluoxetine prescription and further investigation into its potentially detrimental cognitive effects, even when used prophylactically.
Embarking on a quest to mitigate the burden of major depressive disorder (MDD), a pervasive mental health challenge, scientists heralded a new era in the 1950s with the discovery of imipramine, the archetype of tricyclic antidepressants. This breakthrough paved the way for a novel class of therapeutics. In this vein, fluoxetine emerged from an extensive search to find antidepressants that sidestepped the high affinity for monoaminergic neurotransmitter receptors, a common source of the undesirable side effects linked with their tricyclic counterparts (Beaumont, 1988). From a pool of 55 phenoxyphenylpropylamine derivatives assessed for their ability to inhibit monoamine uptake, one, in particular, stood out by 1972: fluoxetine sodium oxalate distinguished itself as a potent and selective serotonin reuptake inhibitor (SSRI), charting a new course in antidepressant treatment (Wong et al., 1995; Wong and Licinio, 2001; Wong et al., 2005; Lopez-Munoz and Alamo, 2009).
In vivo studies have delineated the role of fluoxetine in modulating synaptic serotonin (5-HT) levels, revealing a modest yet persistent surge in extracellular 5-HT concentrations (Guan and McBride, 1988; Rutter et al., 1994; Invernizzi et al., 1996; Tao et al., 2000; Koch et al., 2002; Wong et al., 2005). Its bioactive metabolite, norfluoxetine, also elevates 5-HT levels post-treatment (Qu et al., 2009). Despite diminished interactions with monoamine receptors, fluoxetine still has interactions with 5-HT2A and 5-HT2C receptors, displaying a spectrum of signaling from agonist at 5-HT1A/2A receptors to antagonist at 5HT2C and 5-HT2A receptors (Ni and Miledi, 1997; Bampalis et al., 2010; Liu L. et al., 2018). Such findings highlight the complex role of serotonin receptor activity in shaping fluoxetine’s pharmacological action. There is a strong imperative for more detailed research into fluoxetine’s genetic and cellular mechanisms and its influence on the dynamics of neuronal networks. A thorough understanding of these processes is crucial for maximizing fluoxetine’s therapeutic benefits and reducing its adverse effects, representing a pivotal challenge in the advancement of antidepressant therapy.
Fluoxetine is known to rapidly increase synaptic 5-HT levels, but its therapeutic effect is mediated by plastic changes within neuronal networks that require weeks or even months to establish (Saarelainen et al., 2003; Wyneken et al., 2006). The neurotrophin Brain-Derived Neurotrophic Factor (BDNF) and its receptor, tropomyosin kinase B (TrkB), are believed to play a crucial role in inducing these plastic adaptations (Nibuya et al., 1995; Nibuya et al., 1996; Saarelainen et al., 2003; Wyneken et al., 2006; Martinowich et al., 2007; Rantamaki et al., 2007; Rantamaki et al., 2011; Mikics et al., 2018). Indeed, BDNF is known to be involved in processes critical to neuronal health, including development, survival, neurogenesis, and synaptic plasticity (Reichardt, 2006; Leal et al., 2015). There is substantial evidence indicating that following several weeks of fluoxetine treatment, as well as other antidepressants, there are significant changes in the BDNF signaling pathway and downstream molecules such as Erk1/2 and, more recently, NF-κB (Castren, 2004; Molteni et al., 2006; Castren et al., 2007; Castren and Rantamaki, 2010; Huang et al., 2013; Yang et al., 2014; Cui et al., 2016; Mikics et al., 2018; Song et al., 2019). An intriguing finding by Casarotto et al. indicates that the antidepressant effect of fluoxetine is reliant on its direct binding to the TrkB receptor, which facilitates synaptic localization of TRKB and its activation by BDNF (Casarotto et al., 2021). Thus, different experimental approaches suggest that enhancements in BDNF/TrkB signaling are fundamental to the activity-dependent synaptic plasticity that leads to mood improvements due to fluoxetine treatment (Stewart and Reid, 2000; Maya Vetencourt et al., 2008; Wang et al., 2008; Guirado et al., 2014; Ruiz-Perera et al., 2015; Mikics et al., 2018). Furthermore, transactivation of the TrkB receptor by external factors such as oxytocin or exercise, possibly acting through this same signaling system, is suggested to augment the therapeutic action of fluoxetine and other antidepressants (Sleiman et al., 2016; Mitre et al., 2022), which may constitute an innovative and complementary approach for depression treatment (Madjid et al., 2023).
BDNF also plays a critical role in adult neurogenesis, particularly within specialized regions of the brain known as adult neurogenic niches. One such niche is the subgranular zone of the hippocampus, where BDNF facilitates the terminal differentiation of new neurons (Chan et al., 2008). The significance of adult neurogenesis in the mechanism of antidepressants has been well established, with numerous studies documenting this relationship (Waterhouse et al., 2012; Quesseveur et al., 2013; Bjorkholm and Monteggia, 2016; Tunc-Ozcan et al., 2019; Malberg et al., 2021). The positive effect on neurogenesis of several antidepressants converges on bone morphogenetic protein (BMP), a central regulator of neural stem cell proliferation, and BDNF/TrkB is proposed to be one of the implied signaling systems (Tunc-Ozcan et al., 2021). Fluoxetine has been shown to stimulate neurogenesis, which is not only associated with its therapeutic effects but also counteracts the neurogenesis decline caused by chronic stress (Santarelli et al., 2003; Tanti et al., 2012). This restorative effect on neurogenesis is linked to increases in BDNF and involves a 5-HT4 receptor-dependent de-maturation of granule cell populations (Imoto et al., 2015). Interestingly, fluoxetine also promotes the neurogenesis of interneurons in the cerebral cortex, a process that is coupled with an inhibitory effect on ischemia-induced apoptosis (Ohira et al., 2013). Currently, the relationship between fluoxetine’s neurogenesis-enhancing effects and its ability to selectively induce structural and synaptic changes that alleviate depressive symptoms is not fully understood and warrants further investigation.
Fluoxetine treatment elicits morphological and synaptic changes in neuronal networks, whose beneficial effects may depend on factors like age, brain region, and physiological status. As such, fluoxetine treatment induces a reduction in dendritic arbor length and complexity in the cortical regions of adult animals (Ampuero et al., 2019), while these parameters are enhanced in the dentate gyrus of young animals, suggesting age and brain region-specific therapeutic window for both parameters (Amellem et al., 2017). At the synaptic level, fluoxetine treatment increases dendritic spine density in the hippocampus and medial prefrontal cortex (Ampuero et al., 2010; Rubio et al., 2013; McAllister et al., 2015; Pedraza et al., 2019; Song et al., 2019). In naïve animals, repetitive fluoxetine treatment is characterized by the development of robust and larger spines, which are enriched with AMPA and GluN2A-type NMDA receptor subunits, known to be prevalent in mature dendritic spines, and associated with enhanced synaptic responses (Popoli et al., 2002; Ampuero et al., 2010; Barbon et al., 2011; Rubio et al., 2013). This type of synaptic maturation, however, is linked to altered or diminished long-term potentiation (LTP) and long-term depression (LTD), both of which are extensively validated models of synaptic plasticity (Stewart and Reid, 2000; Rubio et al., 2013). These changes imply that, under physiological or healthy conditions, repetitive fluoxetine treatment leads to long-term stabilization and decreased plasticity of neuronal networks that might be relevant for learning and cognition. However, the induction of such plastic adaptations may be beneficial in restoring the synaptic balance disrupted during pathological states, including prolonged stress and mood disorders (Kallarackal et al., 2013; Mikics et al., 2018). Furthermore, beneficial effects of fluoxetine’s plasticity-promoting actions may extend to other pathological conditions such as neurodegenerative diseases or injury. In Alzheimer’s disease mice models, for example, fluoxetine treatment has been shown to increase dendritic spine numbers and elevate levels of synaptic proteins such as postsynaptic density 95 (PSD-95) and synapsin-1 in the hippocampus, indicative of a beneficial increase in synapse formation and stability (Ma et al., 2020). Similarly, following medial frontal cortex injury, fluoxetine led to an increase in dendritic spine density and basal dendritic arborization in layer II/III pyramidal neurons (McAllister et al., 2015). The exact role of BDNF or its downstream pathways in mediating these fluoxetine-enhanced plastic changes remains to be fully elucidated.
The long-term implications of fluoxetine’s capacity to promote neural plasticity, beyond its antidepressant effects, remain unclear and under documented. Establishing clear causal links between prolonged fluoxetine use and its potential side effects in relevant domains such as cognitive function is essential. Next, we will describe evidence regarding the detrimental and positive effects of fluoxetine in preclinical models of depression and in humans.
Fluoxetine is primarily used to treat major depressive disorder (MDD), a disease that is often accompanied by impaired cognitive functions, a complex concept globally including memory, executive functions, attention, and psychomotor processing speed (Wagner et al., 2012; Hammar et al., 2022). Moreover, cognitive deficits may persist in treated patients that recover from depressive symptoms, and thus, the contribution of antidepressant treatment to persistence, aggravation or improvement on cognition is difficult to grasp. Regarding cognitive functions, this the first time that preclinical findings and clinical findings are summarized side-by-side in adults receiving fluoxetine. Recent reviews have addressed the most commonly studied side effects (but not cognition) in children and adolescents (an age group that was excluded in the present review) (Locher et al., 2017). We also excluded pre-natal and perinatal fluoxetine treatments. One of the reviews on adults focuses on suicide ideation, which cannot be studied in pre-clinical models (Edinoff et al., 2021). In one recent review, the levels of BDNF in preclinical and clinical studies are analyzed side-by-side. Interestingly, conflicting results were found in animal studies while in humans, the anti-depressant effect of drugs (including fluoxetine) seems not to depend on BDNF (Talaee et al., 2024). We now summarize the available literature in the field focusing first on animal models and second, on studies with patients, and separating detrimental effects (Table 1) from positive effects (Table 2). Excellent reviews have examined preclinical studies, which, to some extent, address the time and ethical constraints inherent in human studies (Monleon et al., 2008; Olivier et al., 2011). The explanation of the cognitive test used are contained in Box 1, and the clinical tests that have a preclinical counterpart are marked * (to see Supplementary material).
Table 1. Detrimental effects of fluoxetine on cognitive function as evaluated in preclinical and clinical studies.
Table 2. Positive effects of fluoxetine on cognitive function as evaluated in preclinical and clinical studies.
In the search for learning and memory outcomes of fluoxetine treatment, both single-dose and sustained administration of this antidepressant (over 2 weeks) have been shown to impair long-term memory in naïve rats across a range of tests for measuring inhibitory avoidance, learning and impulsivity, while short-term memory is not impaired (refer to Table 1 for a detailed breakdown). However, despite rats treated with fluoxetine maintain their ability to learn the location of a submerged platform (Keith et al., 2007; Valluzzi and Chan, 2007; Satvat et al., 2012; Ampuero et al., 2013), their recall might be compromised after an extended period post-training (i.e., tested 17 days after training) (Ampuero et al., 2013), suggesting that fluoxetine elicits an specific impairment in remote memory. Also underscoring the complexity of fluoxetine treatment outcomes on cognition, hippocampal dependent tasks were not impaired during the training period while non-hippocampal tasks, such as novel object recognition were impaired in adult as well as adolescent animals (Valluzzi and Chan, 2007; Ampuero et al., 2013; Sadegzadeh et al., 2020), which may be associated to increased 5-HT2A receptor signaling in the prefrontal cortex (Lalonde and Vikis-Freibergs, 1985; Castane et al., 2015). Also, in the Barnes maze test, fluoxetine was not able to induce a recovery of the spatial memory deficits induced by traumatic brain injury (Wang et al., 2011). By the other hand, the prenatal administration of fluoxetine to pregnant females induces deficits in non-hippocampal memory in the adult rat (Moraga-Amaro et al., 2021). Regarding other relevant domains, chronic fluoxetine treatment induced sleep disruption, facilitated social interaction, but led to impairment of vigilance/sustained attention and enhanced impulsivity in juvenile rhesus monkeys (He et al., 2014; Golub et al., 2018) (To see Table 1).
Considering the cognitive deficits observed in MDD patients, some studies address the outcomes of fluoxetine treatment on this issue (Table 1). In patients with a first diagnose of MDD, it was found that fluoxetine treatment reduces depressive symptoms, but it does not improve a specific memory scale, directional memory, free recall, associative learning, nor face recognition (Luo et al., 2013). Another cohort with MDD and obsessive-compulsive disorder patients, fluoxetine gradually and significantly decreased the score in the Mini-Mental State Examination (MMSE) test over 8 weeks of treatment (Luo et al., 2013; Sayyah et al., 2016; Shehab et al., 2016). In addition, deficits in the Subjective Memory Questionnaire were detected in MDD patients treated with fluoxetine compared to matched controls, both in remitted and non-remitted patients, while in objective memory tests no differences were found (Gorenstein et al., 2006). In aged patients a case report in an 85 years-old depressed patient showed a significant memory loss after the beginning of fluoxetine treatment, and this effect was reversed during drug withdrawal (Joss et al., 2003).This striking case suggest that potential the age-related sensitivity to fluoxetine’s cognitive effects should be spotlighted (To see Table 1).
Sustained attention and vigilance were also negatively affected in healthy adults treated with fluoxetine, underscoring the drug’s broader cognitive footprint beyond its therapeutic use (Ramaekers et al., 1995). Of note, a large number of studies indicate that the use of SSRIs in general and fluoxetine in particular elevate the likelihood of suicide attempts or fatalities, causing a continuous growth of apprehension regarding the use of fluoxetine especially among adolescents and young patients (Zhou et al., 2020; Lagerberg et al., 2022). The collective insights from these studies underscore an intricate tapestry of cognitive effects woven by fluoxetine treatment. It invites a deeper reflection on the balance between managing MDD and preserving cognitive integrity, especially in vulnerable populations.
Several preclinical studies have shown memory improvements after fluoxetine treatments. For instance, fluoxetine administration to naïve mice increases memory retention (Yi et al., 2018; Casarotto et al., 2021) and reverts memory deficits induced by neuropathological conditions such as in the social isolation model of depression (Abu-Elfotuh et al., 2022), Alzheimer disease (Wang et al., 2014; Jin et al., 2017; Ma et al., 2017; Abu-Elfotuh et al., 2022), ischemia (Chang et al., 2006; Lee et al., 2014), Down´s Syndrome (Begenisic et al., 2014), stroke (Li et al., 2009; Vahid-Ansari and Albert, 2018), epilepsy (Barkas et al., 2012), cancer chemotherapy (ElBeltagy et al., 2010; Lyons et al., 2011). These studies strongly suggest that in different brain pathological conditions, fluoxetine treatment positively affects the recovery of cognitive functioning. Conversely, healthy or control animals submitted to fluoxetine treatment are not necessarily benefited in their cognition, as fluoxetine-induced plastic changes might be shifting some neuronal networks out of a functional or homeostatic set point (To see Table 2).
However, the dose and timing of fluoxetine administration are important factors that may influence the impact of fluoxetine in the evaluation of cognitive functions under pathology. For instance, early life exposure of low doses of fluoxetine reduces the deficits in spatial memory induced by ischemic brain injury, while high doses of fluoxetine have no effects (Chang et al., 2006). Moreover, 28 days of fluoxetine treatment reduces the deficits on spatial memory induced after stroke by intraluminal middle cerebral artery occlusion in adult mice (Li et al., 2009). Depending on the animal model and experimental protocol, fluoxetine can exert opposing effects on cognitive functions: only short-term administrations (1 dose-up to 2 weeks) in naïve rats causes improvements in memory tests (Marwari and Dawe, 2018; Yi et al., 2018; Casarotto et al., 2021). Thus, while fluoxetine has shown cognitive benefits in various models of brain pathology, its effects in healthy systems and the optimal therapeutic window for its administration remain areas ripe for further inquiry. These findings not only expand our understanding of fluoxetine’s therapeutic scope but also caution against oversimplified applications, advocating for a tailored approach in its clinical use.
Chronic fluoxetine administration in patients with MDD has been associated with cognitive enhancements. Notably, adults on fluoxetine show improved recall memory and sharper attention and concentration, with benefits persisting even a month post-treatment (Jaykaran et al., 2009) (To see Table 2). Similarly, elderly MDD patients on long-term fluoxetine therapy exhibit cognitive improvements on memory assessments (Cassano et al., 2002; Doraiswamy et al., 2003). In adolescents, cognitive improvement in fluoxetine-treated depressed patients was shown (Maalouf et al., 2018). However, in a different controlled study performed in adolescents, 6 and 12 weeks of fluoxetine treatment improved executive functioning, sustained attention, inhibition, and impulsivity and executive functioning in healthy controls while in depressed patients, cognitive deficits were maintained (Shehab et al., 2016).
Beyond MDD, fluoxetine shows potential in ameliorating cognitive deficits related to other conditions. For example, individuals with traumatic brain injury (TBI) display improved working memory when treated with fluoxetine, as measured by the WAIS-III (Horsfield et al., 2002). Patients with mild cognitive impairment, often considered an early stage of Alzheimer’s disease, also benefit from fluoxetine, exhibiting better outcomes on the MMSE memory test than those on a placebo (Mowla et al., 2007). Moreover, those suffering from vascular dementia have shown cognitive improvements under fluoxetine treatment (Liu et al., 2014; Zhang et al., 2021). Collectively, these findings underscore fluoxetine’s significant role in reversing cognitive dysfunctions across various pathological conditions, bolstering memory, attention, planning, and concentration.
The weak interaction of fluoxetine with other neurotransmitter receptors explains its high tolerability profile. Its most common side effects include nausea and anorexia (Oliva et al., 2021). Beyond the gastrointestinal discomfort, a range of non-gastrointestinal side effects have been documented, such as headache, dizziness, variations in sleep patterns, anxiety, tremor, and sexual dysfunction, painting a comprehensive picture of the drug’s tolerability (Benfield et al., 1986; Ferguson, 2001; Carr and Ensom, 2002; Wernicke, 2004). Behavioral side effects, however, present a more elusive challenge, with detection proving more difficult and fewer studies available for reference. The translation of findings from animal models to human subjects adds an additional layer of complexity to the analysis of cognitive side effects, necessitating careful consideration.
In preclinical settings, specific behavioral impacts of fluoxetine have been more extensively studied. Its influence includes, reducing alcohol preference in rats (Naranjo et al., 1994), inhibiting muricidal behavior (Fuller, 1996), and decreasing the self-administration of substances like D-amphetamine (Yu et al., 1986) or cocaine (Glatz et al., 2002). Interestingly, fluoxetine has also been noted to enhance the analgesic properties of morphine (Barakat et al., 2018), showcasing its diverse array of effects in animal behavior. Specific behavioral effects of fluoxetine have been tested more frequently in preclinical models. This intricate network of fluoxetine’s interactions—spanning the spectrum from common side effects to specific behavioral changes—highlights the need for ongoing research to fully understand and effectively manage the drug’s diverse impacts.
The impact of fluoxetine on multiple cognitive domains are diverse, and there is evidence indicating potential advantages for memory and cognition across different conditions, although detrimental effects cannot be disregarded, especially in young subjects and in prenatal exposure to the drug. A second factor of disparity are variable baseline conditions in these studies, as fluoxetine may negatively affect the cognition of healthy brains (naïve animals), while improving diseased brains (e.g., including models of MDD, Alzheimer´s Disease, Traumatic Brain Injury, Obsessive-Compulsive Disorder, Stroke, Ischemia, Mild Cognitive Impairment, among others). For example, fluoxetine may affect positively especially depressive behavior and motor recovery after stroke, although its positive cognitive effects are still under discussion (Mead et al., 2020; Wu and Qin, 2023).
Special attention should be paid to evidence showing that perinatal fluoxetine administered to the mother may impair cognitive functions in the offspring, revealing a negative fetal programming action (Boulle et al., 2016a; Boulle et al., 2016b; Laureano-Melo et al., 2020; Lan et al., 2023).
It is difficult to predict the impact on human daily life of any of the reported alterations detected in preclinical studies, while complex cognitive skills are still very scarcely evaluated in any study. Considering these and other relevant preclinical and clinical findings from this article, we propose a multidimensional model of fluoxetine’s therapeutic response, which is dependent on the age, dose, and the level and nature of the allostatic load of the recipient organism (Figure 1).
Figure 1. Multidimensional Model of Fluoxetine’s Therapeutic Response. This graphical abstract synthesizes the integrated preclinical and clinical findings, illustrating how the efficacy of fluoxetine therapy is modulated by allostatic load and dosing. The surface’s color gradient delineates the therapeutic optimum, pinpointing where allostatic load and fluoxetine dosage intersect to provide the greatest therapeutic advantage. For instance, comparable allostatic stress levels yield divergent therapeutic outcomes when fluoxetine is administered during different life stages; early life exposure (e.g., prenatal or adolescent phases) may be less beneficial than treatment in adulthood. Moreover, the therapeutic impact of a constant fluoxetine dose varies significantly with allostatic intensity, peaking at moderate levels (e.g., Mood disorders) while diminishing when levels are too low (Neurological/psychiatric homeostasis) or excessively high (Neurodegeneration).
Long-term consequences of fluoxetine have not adequately been addressed (e.g., after at least over 1 year of administration), especially when considering that it can be administered during long time periods. In a pioneering work including RNA-seq and H3K27ac ChIP-seq analysis of 27 brain regions after 6 weeks of fluoxetine treatment, the complexity and heterogeneity of fluoxetine-induced changes were addressed, opening a real possibility that the biological basis of antidepressant efficacy and adverse cognitive effects can be mechanistically explored (Rayan et al., 2022).
More recently, emerging evidence supports the anti-inflammatory role of fluoxetine. Indeed, central and peripheral inflammation may constitute a main etiological factor contributing to MDD (Beurel et al., 2020). In addition, neuroinflammation is consistently associated with cognitive impairment and with diseases that are risk factors for cognitive decline (Ownby, 2010). Interestingly, fluoxetine decreases neuroinflammation associated with various neurological disorders and inflammatory conditions, including MDD (Lee et al., 2012; Zhang et al., 2012; Du et al., 2016; Liu F. Y. et al., 2018). For instance, fluoxetine has anti-inflammatory properties in “healthy” rats subjected to a periodontal disease model (Branco-de-Almeida et al., 2012). Also, the cognitive impairment associated to neuroinflammation after surgery for knee removal of elderly patients can be effectively treated with fluoxetine (Lin et al., 2022). It remains unknown whether cognitive deficits are associated with differential patterns of neuroinflammation during MDD or even MDD subtypes (Lotrich, 2015; Gaspersz et al., 2017). On the other side, while anti-inflammatory drugs have been used successfully as add-on therapies for MDD (Zhang et al., 2016; Kohler-Forsberg et al., 2019), the associated eventually positive cognitive side effects have not yet been evaluated (Kohler et al., 2014).
To refine the therapeutic use of fluoxetine and even bolster our understanding of MDD pathogenic mechanisms, the following may contribute to guide future research:
How do the cognitive side effects of fluoxetine vary across different subtypes of depression, and what implications does this have for personalized treatment approaches?
What is the relationship between the age of patients and the manifestation of cognitive side effects, and how the age-specific treatment protocols would be developed?
How do underlying health conditions, both mental (such as MDD) and physical, interact with fluoxetine’s cognitive side effects, and what does this mean for comprehensive patient care?
Investigating these questions is crucial for tailoring fluoxetine use to individual needs, minimizing its risks, and harnessing its potential benefits. The insights gained from such research could revolutionize our approach to treating cognitive impairments associated with depression and inflammation.
EA: Writing–original draft, Investigation, Supervision. AL: Conceptualization, Writing–review and editing. FF: Formal Analysis, Investigation, Writing–original draft. AS: Writing–original draft. CP: Writing–original draft. VS: Writing–original draft. ME: Writing–original draft. TC: Writing–original draft. UW: Writing–original draft, Writing–review and editing.
The author(s) declare that financial support was received for the research, authorship, and/or publication of this article. This work was funded by ANID projects (Chile) #11180777 (to EA) and # 1200693 and 1240604 (to UW); by Basal funding for Scientific and Technological Center of Excellence, IMPACT, #FB210024 and by Vicerrectoría de Investigación, Desarrollo e Innovación USACH-DYCIT #022243ALL_Asociativo; #022242ALL_Ayudante.
The authors declare that the research was conducted in the absence of any commercial or financial relationships that could be construed as a potential conflict of interest.
All claims expressed in this article are solely those of the authors and do not necessarily represent those of their affiliated organizations, or those of the publisher, the editors and the reviewers. Any product that may be evaluated in this article, or claim that may be made by its manufacturer, is not guaranteed or endorsed by the publisher.
The Supplementary Material for this article can be found online at: https://www.frontiersin.org/articles/10.3389/fphar.2024.1412420/full#supplementary-material
Abu-Elfotuh, K., Al-Najjar, A. H., Mohammed, A. A., Aboutaleb, A. S., and Badawi, G. A. (2022). Fluoxetine ameliorates Alzheimer's disease progression and prevents the exacerbation of cardiovascular dysfunction of socially isolated depressed rats through activation of Nrf2/HO-1 and hindering TLR4/NLRP3 inflammasome signaling pathway. Int. Immunopharmacol. 104, 108488. doi:10.1016/j.intimp.2021.108488
Amellem, I., Suresh, S., Chang, C. C., Tok, S. S. L., and Tashiro, A. (2017). A critical period for antidepressant-induced acceleration of neuronal maturation in adult dentate gyrus. Transl. Psychiatry 7, e1235. doi:10.1038/tp.2017.208
Ampuero, E., Cerda, M., Hartel, S., Rubio, F. J., Massa, S., Cubillos, P., et al. (2019). Chronic fluoxetine treatment induces maturation-compatible changes in the dendritic arbor and in synaptic responses in the auditory cortex. Front. Pharmacol. 10, 804. doi:10.3389/fphar.2019.00804
Ampuero, E., Rubio, F. J., Falcon, R., Sandoval, M., Diaz-Veliz, G., Gonzalez, R. E., et al. (2010). Chronic fluoxetine treatment induces structural plasticity and selective changes in glutamate receptor subunits in the rat cerebral cortex. Neuroscience 169, 98–108. doi:10.1016/j.neuroscience.2010.04.035
Ampuero, E., Stehberg, J., Gonzalez, D., Besser, N., Ferrero, M., Diaz-Veliz, G., et al. (2013). Repetitive fluoxetine treatment affects long-term memories but not learning. Behav. Brain Res. 247, 92–100. doi:10.1016/j.bbr.2013.03.011
Bampalis, V. G., Khandoga, A. L., and Siess, W. (2010). Fluoxetine inhibition of 5-HT-potentiated platelet aggregation in whole blood. Thromb. Haemost. 104, 1272–1274. doi:10.1160/TH10-05-0313
Barakat, A., Hamdy, M. M., and Elbadr, M. M. (2018). Uses of fluoxetine in nociceptive pain management: a literature overview. Eur. J. Pharmacol. 829, 12–25. doi:10.1016/j.ejphar.2018.03.042
Barbon, A., Caracciolo, L., Orlandi, C., Musazzi, L., Mallei, A., La Via, L., et al. (2011). Chronic antidepressant treatments induce a time-dependent up-regulation of AMPA receptor subunit protein levels. Neurochem. Int. 59, 896–905. doi:10.1016/j.neuint.2011.07.013
Barkas, L., Redhead, E., Taylor, M., Shtaya, A., Hamilton, D. A., and Gray, W. P. (2012). Fluoxetine restores spatial learning but not accelerated forgetting in mesial temporal lobe epilepsy. Brain 135, 2358–2374. doi:10.1093/brain/aws176
Beaumont, G. (1988). Adverse effects of tricyclic and non-tricyclic antidepressants. Int. Clin. Psychopharmacol. 3 (Suppl. 2), 55–61.
Begenisic, T., Baroncelli, L., Sansevero, G., Milanese, M., Bonifacino, T., Bonanno, G., et al. (2014). Fluoxetine in adulthood normalizes GABA release and rescues hippocampal synaptic plasticity and spatial memory in a mouse model of Down syndrome. Neurobiol. Dis. 63, 12–19. doi:10.1016/j.nbd.2013.11.010
Ben-Azu, B., Adebesin, A., Moke, G. E., Ojiokor, V. O., Olusegun, A., Jarikre, T. A., et al. (2024). Alcohol exacerbates psychosocial stress-induced neuropsychiatric symptoms: attenuation by geraniol. Neurochem. Int. 177, 105748. doi:10.1016/j.neuint.2024.105748
Benfield, P., Heel, R. C., and Lewis, S. P. (1986). Fluoxetine. A review of its pharmacodynamic and pharmacokinetic properties, and therapeutic efficacy in depressive illness. Drugs 32, 481–508. doi:10.2165/00003495-198632060-00002
Beurel, E., Toups, M., and Nemeroff, C. B. (2020). The bidirectional relationship of depression and inflammation: double trouble. Neuron 107, 234–256. doi:10.1016/j.neuron.2020.06.002
Bjorkholm, C., and Monteggia, L. M. (2016). BDNF - a key transducer of antidepressant effects. Neuropharmacology 102, 72–79. doi:10.1016/j.neuropharm.2015.10.034doi:10.1016/j.neuropharm.2015.10.034
Boulle, F., Pawluski, J. L., Homberg, J. R., Machiels, B., Kroeze, Y., Kumar, N., et al. (2016a). Prenatal stress and early-life exposure to fluoxetine have enduring effects on anxiety and hippocampal BDNF gene expression in adult male offspring. Dev. Psychobiol. 58, 427–438. doi:10.1002/dev.21385
Boulle, F., Pawluski, J. L., Homberg, J. R., Machiels, B., Kroeze, Y., Kumar, N., et al. (2016b). Developmental fluoxetine exposure increases behavioral despair and alters epigenetic regulation of the hippocampal BDNF gene in adult female offspring. Horm. Behav. 80, 47–57. doi:10.1016/j.yhbeh.2016.01.017
Branco-De-Almeida, L. S., Franco, G. C., Castro, M. L., Dos Santos, J. G., Anbinder, A. L., Cortelli, S. C., et al. (2012). Fluoxetine inhibits inflammatory response and bone loss in a rat model of ligature-induced periodontitis. J. Periodontol. 83, 664–671. doi:10.1902/jop.2011.110370
Carr, R. R., and Ensom, M. H. (2002). Fluoxetine in the treatment of premenstrual dysphoric disorder. Ann. Pharmacother. 36, 713–717. doi:10.1345/aph.1A265
Casarotto, P. C., Girych, M., Fred, S. M., Kovaleva, V., Moliner, R., Enkavi, G., et al. (2021). Antidepressant drugs act by directly binding to TRKB neurotrophin receptors. Cell 184, 1299–1313.e19 e1219. doi:10.1016/j.cell.2021.01.034
Cassano, G. B., Puca, F., Scapicchio, P. L., and Trabucchi, M.Italian Study Group on Depression in Elderly Patients (2002). Paroxetine and fluoxetine effects on mood and cognitive functions in depressed nondemented elderly patients. J. Clin. Psychiatry 63, 396–402. doi:10.4088/jcp.v63n0504
Castane, A., Kargieman, L., Celada, P., Bortolozzi, A., and Artigas, F. (2015). 5-HT2A receptors are involved in cognitive but not antidepressant effects of fluoxetine. Eur. Neuropsychopharmacol. 25, 1353–1361. doi:10.1016/j.euroneuro.2015.04.006
Castren, E. (2004). Neurotrophic effects of antidepressant drugs. Curr. Opin. Pharmacol. 4, 58–64. doi:10.1016/j.coph.2003.10.004
Castren, E., and Rantamaki, T. (2010). The role of BDNF and its receptors in depression and antidepressant drug action: reactivation of developmental plasticity. Dev. Neurobiol. 70, 289–297. doi:10.1002/dneu.20758
Castren, E., Voikar, V., and Rantamaki, T. (2007). Role of neurotrophic factors in depression. Curr. Opin. Pharmacol. 7, 18–21. doi:10.1016/j.coph.2006.08.009
Chan, J. P., Cordeira, J., Calderon, G. A., Iyer, L. K., and Rios, M. (2008). Depletion of central BDNF in mice impedes terminal differentiation of new granule neurons in the adult hippocampus. Mol. Cell Neurosci. 39, 372–383. doi:10.1016/j.mcn.2008.07.017
Chang, Y. C., Tzeng, S. F., Yu, L., Huang, A. M., Lee, H. T., Huang, C. C., et al. (2006). Early-life fluoxetine exposure reduced functional deficits after hypoxic-ischemia brain injury in rat pups. Neurobiol. Dis. 24, 101–113. doi:10.1016/j.nbd.2006.06.001
Cui, J., Yang, K., Yu, X., Wang, J. L., Li, J., Zhang, Y., et al. (2016). Chronic fluoxetine treatment upregulates the activity of the ERK1/2-NF-κB signaling pathway in the Hippocampus and prefrontal cortex of rats exposed to forced-swimming stress. Med. Princ. Pract. 25, 539–547. doi:10.1159/000449165
Doraiswamy, P. M., Krishnan, K. R., Oxman, T., Jenkyn, L. R., Coffey, D. J., Burt, T., et al. (2003). Does antidepressant therapy improve cognition in elderly depressed patients? J. Gerontol. A Biol. Sci. Med. Sci. 58, M1137–M1144. doi:10.1093/gerona/58.12.m1137
Du, R. H., Tan, J., Sun, X. Y., Lu, M., Ding, J. H., and Hu, G. (2016). Fluoxetine inhibits NLRP3 inflammasome activation: implication in depression. Int. J. Neuropsychopharmacol. 19, pyw037. doi:10.1093/ijnp/pyw037
Edinoff, A. N., Akuly, H. A., Hanna, T. A., Ochoa, C. O., Patti, S. J., Ghaffar, Y. A., et al. (2021). Selective serotonin reuptake inhibitors and adverse effects: a narrative review. Neurol. Int. 13, 387–401. doi:10.3390/neurolint13030038
Elbeltagy, M., Mustafa, S., Umka, J., Lyons, L., Salman, A., Chur-Yoe, G. T., et al. (2010). Fluoxetine improves the memory deficits caused by the chemotherapy agent 5-fluorouracil. Behav. Brain Res. 208, 112–117. doi:10.1016/j.bbr.2009.11.017
Ferguson, J. M. (2001). SSRI antidepressant medications: adverse effects and tolerability. Prim. Care Companion J. Clin. Psychiatry 3, 22–27. doi:10.4088/pcc.v03n0105
Fuller, R. W. (1996). The influence of fluoxetine on aggressive behavior. Neuropsychopharmacology 14, 77–81. doi:10.1016/0893-133X(95)00110-Y
Gacoin, M., and Ben Hamed, S. (2023). Fluoxetine degrades luminance perceptual thresholds while enhancing motivation and reward sensitivity. Front. Pharmacol. 14, 1103999. doi:10.3389/fphar.2023.1103999
Gaspersz, R., Lamers, F., Wittenberg, G., Beekman, A. T. F., Van Hemert, A. M., Schoevers, R. A., et al. (2017). The role of anxious distress in immune dysregulation in patients with major depressive disorder. Transl. Psychiatry 7, 1268. doi:10.1038/s41398-017-0016-3
Glatz, A. C., Ehrlich, M., Bae, R. S., Clarke, M. J., Quinlan, P. A., Brown, E. C., et al. (2002). Inhibition of cocaine self-administration by fluoxetine or D-fenfluramine combined with phentermine. Pharmacol. Biochem. Behav. 71, 197–204. doi:10.1016/s0091-3057(01)00657-8
Golub, M. S., Hogrefe, C. E., Sherwood, R. J., and Turck, C. W. (2018). Fluoxetine administration in juvenile monkeys: implications for pharmacotherapy in children. Front. Pediatr. 6, 21. doi:10.3389/fped.2018.00021
Gomes, K. S., De Carvalho-Netto, E. F., Monte, K. C., Acco, B., Nogueira, P. J., and Nunes-De-Souza, R. L. (2009). Contrasting effects of acute and chronic treatment with imipramine and fluoxetine on inhibitory avoidance and escape responses in mice exposed to the elevated T-maze. Brain Res. Bull. 78, 323–327. doi:10.1016/j.brainresbull.2008.11.003
Gorenstein, C., De Carvalho, S. C., Artes, R., Moreno, R. A., and Marcourakis, T. (2006). Cognitive performance in depressed patients after chronic use of antidepressants. Psychopharmacol. Berl. 185, 84–92. doi:10.1007/s00213-005-0274-2
Guan, X. M., and Mcbride, W. J. (1988). Fluoxetine increases the extracellular levels of serotonin in the nucleus accumbens. Brain Res. Bull. 21, 43–46. doi:10.1016/0361-9230(88)90118-9
Guirado, R., Perez-Rando, M., Sanchez-Matarredona, D., Castren, E., and Nacher, J. (2014). Chronic fluoxetine treatment alters the structure, connectivity and plasticity of cortical interneurons. Int. J. Neuropsychopharmacol. 17, 1635–1646. doi:10.1017/S1461145714000406
Hammar, A., Ronold, E. H., and Rekkedal, G. A. (2022). Cognitive impairment and neurocognitive profiles in major depression-A clinical perspective. Front. Psychiatry 13, 764374. doi:10.3389/fpsyt.2022.764374
He, Y., Hogrefe, C. E., Grapov, D., Palazoglu, M., Fiehn, O., Turck, C. W., et al. (2014). Identifying individual differences of fluoxetine response in juvenile rhesus monkeys by metabolite profiling. Transl. Psychiatry 4, e478. doi:10.1038/tp.2014.116
Horsfield, S. A., Rosse, R. B., Tomasino, V., Schwartz, B. L., Mastropaolo, J., and Deutsch, S. I. (2002). Fluoxetine's effects on cognitive performance in patients with traumatic brain injury. Int. J. Psychiatry Med. 32, 337–344. doi:10.2190/KQ48-XT0L-2H14-5UMV
Huang, W., Zhao, Y., Zhu, X., Cai, Z., Wang, S., Yao, S., et al. (2013). Fluoxetine upregulates phosphorylated-AKT and phosphorylated-ERK1/2 proteins in neural stem cells: evidence for a crosstalk between AKT and ERK1/2 pathways. J. Mol. Neurosci. 49, 244–249. doi:10.1007/s12031-012-9822-5
Imoto, Y., Kira, T., Sukeno, M., Nishitani, N., Nagayasu, K., Nakagawa, T., et al. (2015). Role of the 5-HT4 receptor in chronic fluoxetine treatment-induced neurogenic activity and granule cell dematuration in the dentate gyrus. Mol. Brain 8, 29. doi:10.1186/s13041-015-0120-3
Invernizzi, R., Bramante, M., and Samanin, R. (1996). Role of 5-HT1A receptors in the effects of acute chronic fluoxetine on extracellular serotonin in the frontal cortex. Pharmacol. Biochem. Behav. 54, 143–147. doi:10.1016/0091-3057(95)02159-0
Jaykaran, C., Bhardwaj, P., Kantharia, N. D., Yadav, P., and Panwar, A. (2009). Effect of fluoxetine on some cognitive functions of patients of depression. Indian J. Psychol. Med. 31, 24–29. doi:10.4103/0253-7176.53311
Jin, L., Gao, L. F., Sun, D. S., Wu, H., Wang, Q., Ke, D., et al. (2017). Long-term ameliorative effects of the antidepressant fluoxetine exposure on cognitive deficits in 3 × TgAD mice. Mol. Neurobiol. 54, 4160–4171. doi:10.1007/s12035-016-9952-9
Joo, B., Xu, S., Park, H., Kim, K., Rah, J. C., and Koo, J. W. (2024). Parietal-frontal pathway controls relapse of fear memory in a novel context. Biol. Psychiatry Glob. Open Sci. 4, 100315. doi:10.1016/j.bpsgos.2024.100315
Joss, J. D., Burton, R. M., and Keller, C. A. (2003). Memory loss in a patient treated with fluoxetine. Ann. Pharmacother. 37, 1800–1803. doi:10.1345/aph.1D154
Kallarackal, A. J., Kvarta, M. D., Cammarata, E., Jaberi, L., Cai, X., Bailey, A. M., et al. (2013). Chronic stress induces a selective decrease in AMPA receptor-mediated synaptic excitation at hippocampal temporoammonic-CA1 synapses. J. Neurosci. 33, 15669–15674. doi:10.1523/JNEUROSCI.2588-13.2013
Keith, J. R., Wu, Y., Epp, J. R., and Sutherland, R. J. (2007). Fluoxetine and the dentate gyrus: memory, recovery of function, and electrophysiology. Behav. Pharmacol. 18, 521–531. doi:10.1097/FBP.0b013e3282d28f83
Koch, S., Perry, K. W., Nelson, D. L., Conway, R. G., Threlkeld, P. G., and Bymaster, F. P. (2002). R-fluoxetine increases extracellular DA, NE, as well as 5-HT in rat prefrontal cortex and hypothalamus: an in vivo microdialysis and receptor binding study. Neuropsychopharmacology 27, 949–959. doi:10.1016/S0893-133X(02)00377-9
Kohler, O., Benros, M. E., Nordentoft, M., Farkouh, M. E., Iyengar, R. L., Mors, O., et al. (2014). Effect of anti-inflammatory treatment on depression, depressive symptoms, and adverse effects: a systematic review and meta-analysis of randomized clinical trials. JAMA Psychiatry 71, 1381–1391. doi:10.1001/jamapsychiatry.2014.1611
Kohler-Forsberg, O., C, N. L., Hjorthoj, C., Nordentoft, M., Mors, O., and Benros, M. E. (2019). Efficacy of anti-inflammatory treatment on major depressive disorder or depressive symptoms: meta-analysis of clinical trials. Acta Psychiatr. Scand. 139, 404–419. doi:10.1111/acps.13016
Lagerberg, T., Fazel, S., Sjolander, A., Hellner, C., Lichtenstein, P., and Chang, Z. (2022). Selective serotonin reuptake inhibitors and suicidal behaviour: a population-based cohort study. Neuropsychopharmacology 47, 817–823. doi:10.1038/s41386-021-01179-z
Lalonde, R., and Vikis-Freibergs, V. (1985). Manipulations of 5-HT activity and memory in the rat. Pharmacol. Biochem. Behav. 22, 377–382. doi:10.1016/0091-3057(85)90035-8
Lamoureux, L., Beverley, J. A., Marinelli, M., and Steiner, H. (2023). Fluoxetine potentiates methylphenidate-induced behavioral responses: enhanced locomotion or stereotypies and facilitated acquisition of cocaine self-administration. Addict. Neurosci. 9, 100131. doi:10.1016/j.addicn.2023.100131
Lan, Z., Tachibana, R. O., and Kanno, K. (2023). Chronic exposure of female mice to selective serotonin reuptake inhibitors during lactation induces vocal behavior deficits in pre-weaned offspring. Pharmacol. Biochem. Behav. 230, 173606. doi:10.1016/j.pbb.2023.173606
Laureano-Melo, R., Dos-Santos, R. C., Da Conceicao, R. R., De Souza, J. S., Da Silva Lau, R., Da Silva Souza Silva, S., et al. (2020). Perinatal fluoxetine treatment promotes long-term behavioral changes in adult mice. Metab. Brain Dis. 35, 1341–1351. doi:10.1007/s11011-020-00606-2
Leal, G., Afonso, P. M., Salazar, I. L., and Duarte, C. B. (2015). Regulation of hippocampal synaptic plasticity by BDNF. Brain Res. 1621, 82–101. doi:10.1016/j.brainres.2014.10.019
Lee, J. Y., Kim, H. S., Choi, H. Y., Oh, T. H., and Yune, T. Y. (2012). Fluoxetine inhibits matrix metalloprotease activation and prevents disruption of blood-spinal cord barrier after spinal cord injury. Brain 135, 2375–2389. doi:10.1093/brain/aws171
Lee, J. Y., Lee, H. E., Kang, S. R., Choi, H. Y., Ryu, J. H., and Yune, T. Y. (2014). Fluoxetine inhibits transient global ischemia-induced hippocampal neuronal death and memory impairment by preventing blood-brain barrier disruption. Neuropharmacology 79, 161–171. doi:10.1016/j.neuropharm.2013.11.011
Lee, K. K. Y., Chattopadhyaya, B., Do Nascimento, A. S. F., Moquin, L., Rosa-Neto, P., Amilhon, B., et al. (2024). Neonatal hypoxia impairs serotonin release and cognitive functions in adult mice. Neurobiol. Dis. 193, 106465. doi:10.1016/j.nbd.2024.106465
Li, J. M., Hu, T., Zhou, X. N., Zhang, T., Guo, J. H., Wang, M. Y., et al. (2023). The involvement of NLRP3 inflammasome in CUMS-induced AD-like pathological changes and related cognitive decline in mice. J. Neuroinflammation 20, 112. doi:10.1186/s12974-023-02791-0
Li, W. L., Cai, H. H., Wang, B., Chen, L., Zhou, Q. G., Luo, C. X., et al. (2009). Chronic fluoxetine treatment improves ischemia-induced spatial cognitive deficits through increasing hippocampal neurogenesis after stroke. J. Neurosci. Res. 87, 112–122. doi:10.1002/jnr.21829
Lin, D., Yu, L., Chen, J., Ye, H., Wu, Y., and Yao, Y. (2022). Fluoxetine for reducing postoperative cognitive dysfunction in elderly patients after total knee replacement: study protocol for a single-centre, double-blind, randomised, parallel-group, superiority, placebo-controlled trial. BMJ Open 12, e057000. doi:10.1136/bmjopen-2021-057000
Liu, F. Y., Cai, J., Wang, C., Ruan, W., Guan, G. P., Pan, H. Z., et al. (2018a). Fluoxetine attenuates neuroinflammation in early brain injury after subarachnoid hemorrhage: a possible role for the regulation of TLR4/MyD88/NF-κB signaling pathway. J. Neuroinflammation 15, 347. doi:10.1186/s12974-018-1388-x
Liu, L., Fu, M., Pei, S., Zhou, L., and Shang, J. (2018b). R-fluoxetine increases melanin synthesis through a 5-ht1a/2A receptor and p38 MAPK signaling pathways. Int. J. Mol. Sci. 20, 80. doi:10.3390/ijms20010080
Liu, Q. R., Shi, C. N., Wang, F., and Tong, J. H. (2023). Neuroinflammation-induced parvalbumin interneuron and oscillation deficits might contribute to neurobehavioral abnormities in a two-hit model of depression. Heliyon 9, e18468. doi:10.1016/j.heliyon.2023.e18468
Liu, X., Zhang, J., Sun, D., Fan, Y., Zhou, H., and Fu, B. (2014). Effects of fluoxetine on brain-derived neurotrophic factor serum concentration and cognition in patients with vascular dementia. Clin. Interv. Aging 9, 411–418. doi:10.2147/CIA.S58830
Locher, C., Koechlin, H., Zion, S. R., Werner, C., Pine, D. S., Kirsch, I., et al. (2017). Efficacy and safety of selective serotonin reuptake inhibitors, serotonin-norepinephrine reuptake inhibitors, and placebo for common psychiatric disorders among children and adolescents: a systematic review and meta-analysis. JAMA Psychiatry 74, 1011–1020. doi:10.1001/jamapsychiatry.2017.2432
Lopez-Munoz, F., and Alamo, C. (2009). Monoaminergic neurotransmission: the history of the discovery of antidepressants from 1950s until today. Curr. Pharm. Des. 15, 1563–1586. doi:10.2174/138161209788168001
Lotrich, F. E. (2015). Inflammatory cytokine-associated depression. Brain Res. 1617, 113–125. doi:10.1016/j.brainres.2014.06.032
Luo, L. L., Chen, X., Chai, Y., Li, J. H., Zhang, M., and Zhang, J. N. (2013). A distinct pattern of memory and attention deficiency in patients with depression. Chin. Med. J. Engl. 126, 1144–1149. doi:10.3760/cma.j.issn.0366-6999.20122636
Lyons, L., Elbeltagy, M., Umka, J., Markwick, R., Startin, C., Bennett, G., et al. (2011). Fluoxetine reverses the memory impairment and reduction in proliferation and survival of hippocampal cells caused by methotrexate chemotherapy. Psychopharmacol. Berl. 215, 105–115. doi:10.1007/s00213-010-2122-2
Ma, J., Gao, Y., Jiang, L., Chao, F. L., Huang, W., Zhou, C. N., et al. (2017). Fluoxetine attenuates the impairment of spatial learning ability and prevents neuron loss in middle-aged APPswe/PSEN1dE9 double transgenic Alzheimer's disease mice. Oncotarget 8, 27676–27692. doi:10.18632/oncotarget.15398
Ma, J., Gao, Y., Tang, W., Huang, W., and Tang, Y. (2020). Fluoxetine protects against dendritic spine loss in middle-aged APPswe/PSEN1dE9 double transgenic Alzheimer's disease mice. Curr. Alzheimer Res. 17, 93–103. doi:10.2174/1567205017666200213095419
Maalouf, F., Bakhti, R., Tamim, H., Shehab, S., and Brent, D. (2018). Neurocognitive predictors of clinical improvement in selective serotonin reuptake inhibitor-treated adolescents with major depressive disorder. J. Child. Adolesc. Psychopharmacol. 28, 387–394. doi:10.1089/cap.2017.0105
Madjid, N., Lidell, V., Nordvall, G., Lindskog, M., Ogren, S. O., Forsell, P., et al. (2023). Antidepressant effects of novel positive allosteric modulators of Trk-receptor mediated signaling - a potential therapeutic concept? Psychopharmacol. Berl. 240, 1789–1804. doi:10.1007/s00213-023-06410-x
Majlessi, N., and Naghdi, N. (2002). Impaired spatial learning in the Morris water maze induced by serotonin reuptake inhibitors in rats. Behav. Pharmacol. 13, 237–242. doi:10.1097/00008877-200205000-00007
Malberg, J. E., Hen, R., and Madsen, T. M. (2021). Adult neurogenesis and antidepressant treatment: the surprise finding by Ron Duman and the field 20 Years later. Biol. Psychiatry 90, 96–101. doi:10.1016/j.biopsych.2021.01.010
Martinowich, K., Manji, H., and Lu, B. (2007). New insights into BDNF function in depression and anxiety. Nat. Neurosci. 10, 1089–1093. doi:10.1038/nn1971doi:10.1038/nn1971
Marwari, S., and Dawe, G. S. (2018). (R)-fluoxetine enhances cognitive flexibility and hippocampal cell proliferation in mice. J. Psychopharmacol. 32, 441–457. doi:10.1177/0269881118754733
Maya Vetencourt, J. F., Sale, A., Viegi, A., Baroncelli, L., De Pasquale, R., O'leary, O. F., et al. (2008). The antidepressant fluoxetine restores plasticity in the adult visual cortex. Science 320, 385–388. doi:10.1126/science.1150516
Mcallister, B. B., Spanswick, S. C., Patel, P. P., Barneto, A. A., and Dyck, R. H. (2015). The effects of chronic fluoxetine treatment following injury of medial frontal cortex in mice. Behav. Brain Res. 290, 102–116. doi:10.1016/j.bbr.2015.04.049
Mead, G. E., Legg, L., Tilney, R., Hsieh, C. F., Wu, S., Lundstrom, E., et al. (2020). Fluoxetine for stroke recovery: meta-analysis of randomized controlled trials. Int. J. Stroke 15, 365–376. doi:10.1177/1747493019879655
Mikics, E., Guirado, R., Umemori, J., Toth, M., Biro, L., Miskolczi, C., et al. (2018). Social learning requires plasticity enhanced by fluoxetine through prefrontal bdnf-TrkB signaling to limit aggression induced by post-weaning social isolation. Neuropsychopharmacology 43, 235–245. doi:10.1038/npp.2017.142
Mitre, M., Saadipour, K., Williams, K., Khatri, L., Froemke, R. C., and Chao, M. V. (2022). Transactivation of TrkB receptors by oxytocin and its G protein-coupled receptor. Front. Mol. Neurosci. 15, 891537. doi:10.3389/fnmol.2022.891537
Molteni, R., Calabrese, F., Bedogni, F., Tongiorgi, E., Fumagalli, F., Racagni, G., et al. (2006). Chronic treatment with fluoxetine up-regulates cellular BDNF mRNA expression in rat dopaminergic regions. Int. J. Neuropsychopharmacol. 9, 307–317. doi:10.1017/S1461145705005766
Monleon, S., Urquiza, A., Carmen Arenas, M., Vinader-Caerols, C., and Parra, A. (2002). Chronic administration of fluoxetine impairs inhibitory avoidance in male but not female mice. Behav. Brain Res. 136, 483–488. doi:10.1016/s0166-4328(02)00194-8
Monleon, S., Vinader-Caerols, C., Arenas, M. C., and Parra, A. (2008). Antidepressant drugs and memory: insights from animal studies. Eur. Neuropsychopharmacol. 18, 235–248. doi:10.1016/j.euroneuro.2007.07.001
Moraga-Amaro, R., Diaz-Galarce, R., Donoso-Ramos, J. P., Ugalde, V., Linsambarth, S., Doorduin, J., et al. (2021). Prenatal fluoxetine impairs non-hippocampal but not hippocampal memory in adult male rat offspring. Neuropharmacology 197, 108751. doi:10.1016/j.neuropharm.2021.108751
Mowla, A., Mosavinasab, M., and Pani, A. (2007). Does fluoxetine have any effect on the cognition of patients with mild cognitive impairment? A double-blind, placebo-controlled, clinical trial. J. Clin. Psychopharmacol. 27, 67–70. doi:10.1097/JCP.0b013e31802e0002
Naranjo, C. A., Poulos, C. X., Bremner, K. E., and Lanctot, K. L. (1994). Fluoxetine attenuates alcohol intake and desire to drink. Int. Clin. Psychopharmacol. 9, 163–172. doi:10.1097/00004850-199409000-00004
Nelson, C. J., Jordan, W. P., and Bohan, R. T. (1997). Daily fluoxetine administration impairs avoidance learning in the rat without altering sensory thresholds. Prog. Neuropsychopharmacol. Biol. Psychiatry 21, 1043–1057. doi:10.1016/s0278-5846(97)00097-3
Ni, Y. G., and Miledi, R. (1997). Blockage of 5HT2C serotonin receptors by fluoxetine (Prozac). Proc. Natl. Acad. Sci. U. S. A. 94, 2036–2040. doi:10.1073/pnas.94.5.2036
Nibuya, M., Morinobu, S., and Duman, R. S. (1995). Regulation of BDNF and trkB mRNA in rat brain by chronic electroconvulsive seizure and antidepressant drug treatments. J. Neurosci. 15, 7539–7547. doi:10.1523/JNEUROSCI.15-11-07539.1995
Nibuya, M., Nestler, E. J., and Duman, R. S. (1996). Chronic antidepressant administration increases the expression of cAMP response element binding protein (CREB) in rat hippocampus. J. Neurosci. 16, 2365–2372. doi:10.1523/JNEUROSCI.16-07-02365.1996
Ohira, K., Takeuchi, R., Shoji, H., and Miyakawa, T. (2013). Fluoxetine-induced cortical adult neurogenesis. Neuropsychopharmacology 38, 909–920. doi:10.1038/npp.2013.2
Oliva, V., Lippi, M., Paci, R., Del Fabro, L., Delvecchio, G., Brambilla, P., et al. (2021). Gastrointestinal side effects associated with antidepressant treatments in patients with major depressive disorder: a systematic review and meta-analysis. Prog. Neuropsychopharmacol. Biol. Psychiatry 109, 110266. doi:10.1016/j.pnpbp.2021.110266
Olivier, J. D., Blom, T., Arentsen, T., and Homberg, J. R. (2011). The age-dependent effects of selective serotonin reuptake inhibitors in humans and rodents: a review. Prog. Neuropsychopharmacol. Biol. Psychiatry 35, 1400–1408. doi:10.1016/j.pnpbp.2010.09.013
Ownby, R. L. (2010). Neuroinflammation and cognitive aging. Curr. Psychiatry Rep. 12, 39–45. doi:10.1007/s11920-009-0082-1
Parra, A., Ferrer-Ano, A., Fuentes, C., Monleon, S., and Vinader-Caerols, C. (2010). Effects of co-administration of amitriptyline and fluoxetine on inhibitory avoidance in mice. Behav. Brain Res. 214, 343–348. doi:10.1016/j.bbr.2010.06.002
Payet, J. M., Stevens, L., Russo, A. M., Jaehne, E. J., Van Den Buuse, M., Kent, S., et al. (2023). The role of dorsal raphe nucleus serotonergic systems in emotional learning and memory in male BALB/c mice. Neuroscience 534, 1–15. doi:10.1016/j.neuroscience.2023.10.003
Pedraza, L. K., Sierra, R. O., Giachero, M., Nunes-Souza, W., Lotz, F. N., and De Oliveira Alvares, L. (2019). Chronic fluoxetine prevents fear memory generalization and enhances subsequent extinction by remodeling hippocampal dendritic spines and slowing down systems consolidation. Transl. Psychiatry 9, 53. doi:10.1038/s41398-019-0371-3
Popoli, M., Gennarelli, M., and Racagni, G. (2002). Modulation of synaptic plasticity by stress and antidepressants. Bipolar Disord. 4, 166–182. doi:10.1034/j.1399-5618.2002.01159.x
Puscian, A., Winiarski, M., Leski, S., Charzewski, L., Nikolaev, T., Borowska, J., et al. (2021). Chronic fluoxetine treatment impairs motivation and reward learning by affecting neuronal plasticity in the central amygdala. Br. J. Pharmacol. 178, 672–688. doi:10.1111/bph.15319
Qu, Y., Aluisio, L., Lord, B., Boggs, J., Hoey, K., Mazur, C., et al. (2009). Pharmacokinetics and pharmacodynamics of norfluoxetine in rats: increasing extracellular serotonin level in the frontal cortex. Pharmacol. Biochem. Behav. 92, 469–473. doi:10.1016/j.pbb.2009.01.023
Quesseveur, G., David, D. J., Gaillard, M. C., Pla, P., Wu, M. V., Nguyen, H. T., et al. (2013). BDNF overexpression in mouse hippocampal astrocytes promotes local neurogenesis and elicits anxiolytic-like activities. Transl. Psychiatry 3, e253. doi:10.1038/tp.2013.30
Ramaekers, J. G., Muntjewerff, N. D., and O'hanlon, J. F. (1995). A comparative study of acute and subchronic effects of dothiepin, fluoxetine and placebo on psychomotor and actual driving performance. Br. J. Clin. Pharmacol. 39, 397–404. doi:10.1111/j.1365-2125.1995.tb04468.x
Rantamaki, T., Hendolin, P., Kankaanpaa, A., Mijatovic, J., Piepponen, P., Domenici, E., et al. (2007). Pharmacologically diverse antidepressants rapidly activate brain-derived neurotrophic factor receptor TrkB and induce phospholipase-Cgamma signaling pathways in mouse brain. Neuropsychopharmacology 32, 2152–2162. doi:10.1038/sj.npp.1301345
Rantamaki, T., Vesa, L., Antila, H., Di Lieto, A., Tammela, P., Schmitt, A., et al. (2011). Antidepressant drugs transactivate TrkB neurotrophin receptors in the adult rodent brain independently of BDNF and monoamine transporter blockade. PLoS One 6, e20567. doi:10.1371/journal.pone.0020567
Rayan, N. A., Kumar, V., Aow, J., Rastegar, N., Lim, M. G. L., O'toole, N., et al. (2022). Integrative multi-omics landscape of fluoxetine action across 27 brain regions reveals global increase in energy metabolism and region-specific chromatin remodelling. Mol. Psychiatry 27, 4510–4525. doi:10.1038/s41380-022-01725-1
Reichardt, L. F. (2006). Neurotrophin-regulated signalling pathways. Philos. Trans. R. Soc. Lond B Biol. Sci. 361, 1545–1564. doi:10.1098/rstb.2006.1894
Rubio, F. J., Ampuero, E., Sandoval, R., Toledo, J., Pancetti, F., and Wyneken, U. (2013). Long-term fluoxetine treatment induces input-specific LTP and LTD impairment and structural plasticity in the CA1 hippocampal subfield. Front. Cell Neurosci. 7, 66. doi:10.3389/fncel.2013.00066
Ruiz-Perera, L., Muniz, M., Vierci, G., Bornia, N., Baroncelli, L., Sale, A., et al. (2015). Fluoxetine increases plasticity and modulates the proteomic profile in the adult mouse visual cortex. Sci. Rep. 5, 12517. doi:10.1038/srep12517
Rutter, J. J., Gundlah, C., and Auerbach, S. B. (1994). Increase in extracellular serotonin produced by uptake inhibitors is enhanced after chronic treatment with fluoxetine. Neurosci. Lett. 171, 183–186. doi:10.1016/0304-3940(94)90635-1
Saarelainen, T., Hendolin, P., Lucas, G., Koponen, E., Sairanen, M., Macdonald, E., et al. (2003). Activation of the TrkB neurotrophin receptor is induced by antidepressant drugs and is required for antidepressant-induced behavioral effects. J. Neurosci. 23, 349–357. doi:10.1523/JNEUROSCI.23-01-00349.2003
Sadegzadeh, F., Sakhaie, N., Dehghany, R., Adak, O., and Saadati, H. (2020). Effects of adolescent administration of fluoxetine on novel object recognition memory, anxiety-like behaviors, and hippocampal brain-derived neurotrophic factor level. Life Sci. 260, 118338. doi:10.1016/j.lfs.2020.118338
Santarelli, L., Saxe, M., Gross, C., Surget, A., Battaglia, F., Dulawa, S., et al. (2003). Requirement of hippocampal neurogenesis for the behavioral effects of antidepressants. Science 301, 805–809. doi:10.1126/science.1083328
Satvat, E., Gheidi, A., Voll, S., Odintsova, I. V., and Marrone, D. F. (2012). Location is everything: neurons born during fluoxetine treatment accumulate in regions that do not support spatial learning. Neuropharmacology 62, 1627–1633. doi:10.1016/j.neuropharm.2011.11.025
Sayyah, M., Eslami, K., Alaishehni, S., and Kouti, L. (2016). Cognitive function before and during treatment with selective serotonin reuptake inhibitors in patients with depression or obsessive-compulsive disorder. Psychiatry J. 2016, 5480391. doi:10.1155/2016/5480391
Shehab, A. A., Brent, D., and Maalouf, F. T. (2016). Neurocognitive changes in selective serotonin reuptake inhibitors-treated adolescents with depression. J. Child. Adolesc. Psychopharmacol. 26, 713–720. doi:10.1089/cap.2015.0190
Sleiman, S. F., Henry, J., Al-Haddad, R., El Hayek, L., Abou Haidar, E., Stringer, T., et al. (2016). Exercise promotes the expression of brain derived neurotrophic factor (BDNF) through the action of the ketone body β-hydroxybutyrate. Elife 5, e15092. doi:10.7554/eLife.15092
Song, T., Wu, H., Li, R., Xu, H., Rao, X., Gao, L., et al. (2019). Repeated fluoxetine treatment induces long-lasting neurotrophic changes in the medial prefrontal cortex of adult rats. Behav. Brain Res. 365, 114–124. doi:10.1016/j.bbr.2019.03.009
Stewart, C. A., and Reid, I. C. (2000). Repeated ECS and fluoxetine administration have equivalent effects on hippocampal synaptic plasticity. Psychopharmacol. Berl. 148, 217–223. doi:10.1007/s002130050045
Talaee, N., Azadvar, S., Khodadadi, S., Abbasi, N., Asli-Pashaki, Z. N., Mirabzadeh, Y., et al. (2024). Comparing the effect of fluoxetine, escitalopram, and sertraline, on the level of BDNF and depression in preclinical and clinical studies: a systematic review. Eur. J. Clin. Pharmacol. 80, 983–1016. doi:10.1007/s00228-024-03680-y
Tanti, A., Rainer, Q., Minier, F., Surget, A., and Belzung, C. (2012). Differential environmental regulation of neurogenesis along the septo-temporal axis of the hippocampus. Neuropharmacology 63, 374–384. doi:10.1016/j.neuropharm.2012.04.022
Tao, R., Ma, Z., and Auerbach, S. B. (2000). Differential effect of local infusion of serotonin reuptake inhibitors in the raphe versus forebrain and the role of depolarization-induced release in increased extracellular serotonin. J. Pharmacol. Exp. Ther. 294, 571–579.
Tunc-Ozcan, E., Brooker, S. M., Bonds, J. A., Tsai, Y. H., Rawat, R., Mcguire, T. L., et al. (2021). Hippocampal BMP signaling as a common pathway for antidepressant action. Cell Mol. Life Sci. 79, 31. doi:10.1007/s00018-021-04026-y
Tunc-Ozcan, E., Peng, C. Y., Zhu, Y., Dunlop, S. R., Contractor, A., and Kessler, J. A. (2019). Activating newborn neurons suppresses depression and anxiety-like behaviors. Nat. Commun. 10, 3768. doi:10.1038/s41467-019-11641-8
Vahid-Ansari, F., and Albert, P. R. (2018). Chronic fluoxetine induces activity changes in recovery from poststroke anxiety, depression, and cognitive impairment. Neurotherapeutics 15, 200–215. doi:10.1007/s13311-017-0590-3
Valluzzi, J. A., and Chan, K. (2007). Effects of fluoxetine on hippocampal-dependent and hippocampal-independent learning tasks. Behav. Pharmacol. 18, 507–513. doi:10.1097/FBP.0b013e3282ee2a91
Vilar-Pereira, G., Gibaldi, D., Castano-Barrios, L., Da Silva, A. A., Resende Pereira, I., Cruz Moreira, O., et al. (2024). The beneficial effect of fluoxetine on behavioral and cognitive changes in chronic experimental Chagas disease unveils the role of serotonin fueling astrocyte infection by Trypanosoma cruzi. PLoS Negl. Trop. Dis. 18, e0012199. doi:10.1371/journal.pntd.0012199
Wagner, S., Doering, B., Helmreich, I., Lieb, K., and Tadic, A. (2012). A meta-analysis of executive dysfunctions in unipolar major depressive disorder without psychotic symptoms and their changes during antidepressant treatment. Acta Psychiatr. Scand. 125, 281–292. doi:10.1111/j.1600-0447.2011.01762.x
Wang, J., Zhang, Y., Xu, H., Zhu, S., Wang, H., He, J., et al. (2014). Fluoxetine improves behavioral performance by suppressing the production of soluble β-amyloid in APP/PS1 mice. Curr. Alzheimer Res. 11, 672–680. doi:10.2174/1567205011666140812114715
Wang, J. W., David, D. J., Monckton, J. E., Battaglia, F., and Hen, R. (2008). Chronic fluoxetine stimulates maturation and synaptic plasticity of adult-born hippocampal granule cells. J. Neurosci. 28, 1374–1384. doi:10.1523/JNEUROSCI.3632-07.2008
Wang, Y., Neumann, M., Hansen, K., Hong, S. M., Kim, S., Noble-Haeusslein, L. J., et al. (2011). Fluoxetine increases hippocampal neurogenesis and induces epigenetic factors but does not improve functional recovery after traumatic brain injury. J. Neurotrauma 28, 259–268. doi:10.1089/neu.2010.1648
Waterhouse, E. G., An, J. J., Orefice, L. L., Baydyuk, M., Liao, G. Y., Zheng, K., et al. (2012). BDNF promotes differentiation and maturation of adult-born neurons through GABAergic transmission. J. Neurosci. 32, 14318–14330. doi:10.1523/JNEUROSCI.0709-12.2012
Wernicke, J. F. (2004). Safety and side effect profile of fluoxetine. Expert Opin. Drug Saf. 3, 495–504. doi:10.1517/14740338.3.5.495
Wong, D. T., Bymaster, F. P., and Engleman, E. A. (1995). Prozac (fluoxetine, Lilly 110140), the first selective serotonin uptake inhibitor and an antidepressant drug: twenty years since its first publication. Life Sci. 57, 411–441. doi:10.1016/0024-3205(95)00209-o
Wong, D. T., Perry, K. W., and Bymaster, F. P. (2005). Case history: the discovery of fluoxetine hydrochloride (Prozac). Nat. Rev. Drug Discov. 4, 764–774. doi:10.1038/nrd1821
Wong, M. L., and Licinio, J. (2001). Research and treatment approaches to depression. Nat. Rev. Neurosci. 2, 343–351. doi:10.1038/35072566
Wu, J., and Qin, G. (2023). The efficacy and safety of fluoxetine versus placebo for stroke recovery: a meta-analysis of randomized controlled trials. Int. J. Clin. Pharm. 45, 839–846. doi:10.1007/s11096-023-01573-1
Wyneken, U., Sandoval, M., Sandoval, S., Jorquera, F., Gonzalez, I., Vargas, F., et al. (2006). Clinically relevant doses of fluoxetine and reboxetine induce changes in the TrkB content of central excitatory synapses. Neuropsychopharmacology 31, 2415–2423. doi:10.1038/sj.npp.1301052
Yang, J. M., Rui, B. B., Chen, C., Chen, H., Xu, T. J., Xu, W. P., et al. (2014). Acetylsalicylic acid enhances the anti-inflammatory effect of fluoxetine through inhibition of NF-κB, p38-MAPK and ERK1/2 activation in lipopolysaccharide-induced BV-2 microglia cells. Neuroscience 275, 296–304. doi:10.1016/j.neuroscience.2014.06.016
Yi, J. H., Zhang, J., Ko, S. Y., Kwon, H., Jeon, S. J., Park, S. J., et al. (2018). Fluoxetine inhibits natural decay of long-term memory via akt/GSK-3β signaling. Mol. Neurobiol. 55, 7453–7462. doi:10.1007/s12035-018-0919-x
Yu, D. S., Smith, F. L., Smith, D. G., and Lyness, W. H. (1986). Fluoxetine-induced attenuation of amphetamine self-administration in rats. Life Sci. 39, 1383–1388. doi:10.1016/0024-3205(86)90337-1
Zabot, G. C., Medeiros, E. B., Macarini, B. M. N., Peruchi, B. B., Keller, G. S., Lidio, A. V., et al. (2024). The involvement of neuroinflammation in an animal model of dementia and depression. Prog. Neuropsychopharmacol. Biol. Psychiatry 133, 110999. doi:10.1016/j.pnpbp.2024.110999
Zhang, C., Tian, F., Peng, J., Wang, X., Li, J., Zhang, L., et al. (2024). Serotonergic neurotransmission mediated cognitive dysfunction in two mouse models of sepsis-associated encephalopathy. CNS Neurosci. Ther. 30, e14655. doi:10.1111/cns.14655
Zhang, F., Zhou, H., Wilson, B. C., Shi, J. S., Hong, J. S., and Gao, H. M. (2012). Fluoxetine protects neurons against microglial activation-mediated neurotoxicity. Park. Relat. Disord. 18 (Suppl. 1), S213–S217. doi:10.1016/S1353-8020(11)70066-9
Zhang, J. C., Yao, W., and Hashimoto, K. (2016). Brain-derived neurotrophic factor (BDNF)-TrkB signaling in inflammation-related depression and potential therapeutic targets. Curr. Neuropharmacol. 14, 721–731. doi:10.2174/1570159x14666160119094646
Zhang, L., Liu, X., Li, T., Xu, B., and Fu, B. (2021). Fluoxetine may enhance VEGF, BDNF and cognition in patients with vascular cognitive impairment No dementia: an open-label randomized clinical study. Neuropsychiatr. Dis. Treat. 17, 3819–3825. doi:10.2147/NDT.S334647
Zhou, X., Teng, T., Zhang, Y., Del Giovane, C., Furukawa, T. A., Weisz, J. R., et al. (2020). Comparative efficacy and acceptability of antidepressants, psychotherapies, and their combination for acute treatment of children and adolescents with depressive disorder: a systematic review and network meta-analysis. Lancet Psychiatry 7, 581–601. doi:10.1016/S2215-0366(20)30137-1
Keywords: antidepressants, SSRIs, side-effects, memory, cognition
Citation: Ampuero E, Luarte A, Flores FS, Soto AI, Pino C, Silva V, Erlandsen M, Concha T and Wyneken U (2024) The multifaceted effects of fluoxetine treatment on cognitive functions. Front. Pharmacol. 15:1412420. doi: 10.3389/fphar.2024.1412420
Received: 04 April 2024; Accepted: 10 June 2024;
Published: 16 July 2024.
Edited by:
Natalija Popovic, University of Murcia, SpainReviewed by:
Yan Li, Apellis Pharmaceuticals, United StatesCopyright © 2024 Ampuero, Luarte, Flores, Soto, Pino, Silva, Erlandsen, Concha and Wyneken. This is an open-access article distributed under the terms of the Creative Commons Attribution License (CC BY). The use, distribution or reproduction in other forums is permitted, provided the original author(s) and the copyright owner(s) are credited and that the original publication in this journal is cited, in accordance with accepted academic practice. No use, distribution or reproduction is permitted which does not comply with these terms.
*Correspondence: Estíbaliz Ampuero, ZXN0aWJhbGl6LmFtcHVlcm9AdXNhY2guY2w=; Ursula Wyneken, dXd5bmVrZW5AdWFuZGVzLmNs
Disclaimer: All claims expressed in this article are solely those of the authors and do not necessarily represent those of their affiliated organizations, or those of the publisher, the editors and the reviewers. Any product that may be evaluated in this article or claim that may be made by its manufacturer is not guaranteed or endorsed by the publisher.
Research integrity at Frontiers
Learn more about the work of our research integrity team to safeguard the quality of each article we publish.