- 1Department of Pharmacy, The Affiliated Suqian First People’s Hospital of Nanjing Medical University, Suqian, China
- 2Department of Pharmaceutics, State Key Laboratory of Natural Medicines, China Pharmaceutical University, Nanjing, Jiangsu, China
- 3Department of Rheumatology and Immunology, The Affiliated Suqian First People’s Hospital of Nanjing Medical University, Suqian, China
Background: Delivery systems based on albumin nanoparticles (NPs) have recently garnered substantial interest in anti-tumor drug development. However, systematic bibliometric analyses in this field remain lacking. This study aimed to analyze the current research status, hotspots, and frontiers in the application of albumin NPs in the field of oncology from a bibliometric perspective.
Methods: Using the Web of Science Core Collection (WOSCC) as the data source, retrieved articles were analyzed using software, such as VOSviewer 1.6.18 and CiteSpace 6.1.6, and the relevant visualization maps were plotted.
Results: From 1 January 2000, to 15 April 2024, 2,262 institutions from 67 countries/regions published 1,624 articles related to the application of albumin NPs in the field of oncology. The USA was a leader in this field and held a formidable academic reputation. The most productive institution was the Chinese Academy of Sciences. The most productive author was Youn YS, whereas Kratz F was the most frequently co-cited author. The most productive journal was the International Journal of Nanomedicine, whereas the Journal of Controlled Release was the most co-cited journal. Future research hotspots and frontiers included “rapid and convenient synthesis methods predominated by self-assembly,” “surface modification,” “construction of multifunctional NPs for theranostics,” “research on natural active ingredients mainly based on phenolic compounds,” “combination therapy,” and “clinical applications.”
Conclusion: Based on our bibliometric analysis and summary, we obtained an overview of the research on albumin NPs in the field of oncology, identified the most influential countries, institutions, authors, journals, and citations, and discussed the current research hotspots and frontiers in this field. Our study may serve as an important reference for future research in this field.
1 Introduction
Despite the considerable progress in the development of anti-tumor drugs, the mortality rate of cancer remains high owing to the low water solubility, poor bioavailability, major adverse reactions, and weak targeting of most anti-tumor drugs. Furthermore, tumor resistance and hyperprogression during drug treatment limit the effective use of these drugs. Therefore, developing more effective cancer treatment strategies is imperative. Currently, drug delivery systems are employed in clinical applications owing to their advantages in improving drug solubility, targeting, and half-life, as well as reducing systemic side effects.
Nano-delivery systems based on albumin have garnered widespread interest among researchers (Hong et al., 2020; Martínez-López et al., 2020). Albumin, the most abundant protein in plasma, accounting for approximately 50% of total plasma protein, is endogenous and multifunctional. In the physiological environment, it acts as a natural transport carrier in the blood and can reversibly bind to various endogenous and exogenous molecules, including long-chain fatty acids, bilirubin, iron, calcium, taxanes, sulfonamides, and other drugs, which are then transported to various tissues (Kouchakzadeh et al., 2015). Since Peters first described the structure, molecular genetics, and in vivo metabolism of albumin in 1985, subsequent research has revealed its numerous advantages, including biodegradability, good biocompatibility, non-immunogenicity, and a long half-life (Peters, 1985). Consequently, albumin-based drug delivery systems have gained attention in the field of oncology.
Albumin and albumin-drug complexes mainly enhance their accumulation in tumor tissues through the following pathways:
1) Dysopsonins. Opsonins, proteins found in serum, can enhance phagocytosis by phagocytes. They can be adsorbed to the surface of nanoparticles (NPs), making them more vulnerable to phagocytosis and ultimately shortening their half-life. Dysopsonins, however, are a class of proteins that can extend the half-life of NPs within the body. Dysopsonins exhibit a relatively low affinity for the cell surface. They inhibit the further adsorption of opsonins or block the adsorption sites of opsonins, reducing the immunogenicity of NPs and exhibiting a stealth effect. Encapsulating drugs within albumin, which is a type of dysopsonin, reduces the adsorption of opsonins and inhibits phagocytosis by phagocytes, extending the half-life of drugs in vivo (Wang and Zhang, 2022).
2) Enhanced permeability and retention (EPR) effect. Tumor tissue blood vessels have large gaps, poor structural integrity, and lack lymphatic vessels, allowing albumin to accumulate in tumor tissues without being removed by the return of lymph fluid. Therefore, albumin and albumin–drug complexes can passively target tumor tissues based on the EPR effect (Bertrand et al., 2014).
3) Macropinocytosis. Tumor cells actively take up extracellular albumin through macropinocytosis to support the supply of amino acids and energy required for their growth (Commisso et al., 2013).
4) Albumin receptors. Tumor cells can also transport albumin to certain tumor tissues through albumin-mediated transendothelial cell transport (Hoogenboezem and Duvall, 2018).
The above pathways facilitate the accumulation and uptake of albumin and albumin–drug complexes at tumor sites.
Albumin binds to drugs through four main methods: human serum albumin (HSA)–drug NPs, HSA–drug conjugates, HSA-binding prodrugs, and HSA-based recombinant fusion proteins (Tao et al., 2021). The latter three methods have limited applicability and low drug-loading efficiency, as their drug-loading capacities are significantly constrained by the physiochemical properties of the drug. However, encapsulating drugs in albumin to form albumin NPs can circumvent these drawbacks, achieving a drug-loading method with wide applicability. This method is suitable for various therapeutic molecules that cannot be noncovalently or covalently linked with albumin or undergo gene fusion. It also allows for the simultaneous encapsulation of multiple drugs and imaging agents to implement combination therapy. In addition, albumin NPs can be modified to increase their accumulation at specific sites.
Currently, two anti-tumor drugs—Abraxane® (Bristol-Myers Squibb, Princeton, NJ, USA) and Fyarro® (AADI Bioscience, Pacific Palisades, CA, USA)—are delivered using albumin NPs. On 7 January 2005, the US Food and Drug Administration (FDA) approved Abraxane®, a type of albumin-bound paclitaxel NPs, to treat metastatic breast cancer after failed combination chemotherapy. Abraxane® was later approved in 2012 and 2013 for the first-line treatment of non-small cell lung cancer and metastatic adenocarcinoma of the pancreas, respectively. Abraxane® was the first successful case of albumin nanoparticle (NP) drug delivery technology, leading to increased clinical research on other albumin NP-based drug delivery formulations in the field of oncology (Meng et al., 2023). On 23 November 2021, the FDA approved Fyarro®, an albumin-bound NP formulation of sirolimus, to treat locally advanced unresectable or metastatic malignant perivascular epithelioid cell tumor (PEComa). This is the first FDA-approved drug for treating advanced malignant PEComa in adults and the second anti-tumor product developed and marketed based on albumin technology.
The growing number of studies on albumin NPs as carriers of anti-tumor drugs has led to the development of various methods for drug–albumin conjugation and discovery of many new drugs that can be conjugated with albumin to form NPs, enhancing their anti-tumor activity and targeting. This has laid a solid theoretical foundation for basic research and the rational development and utilization of albumin NPs as targeted carriers of anti-tumor drugs. Several reviews exist on albumin-based nanodrugs, covering aspects such as preparation techniques, surface modifications, construction of multifunctional NPs, and therapeutic applications. These reviews summarize albumin-based nanomedicine and have promoted the rapid technological development of this field. However, studies presenting intuitive analysis and summary of the development trends, publishing institutions, research authors, research hotspots, and frontiers in the oncological applications of albumin NPs remain lacking.
The information age has facilitated the rapid development of bibliometrics, a field that focuses on mining, analyzing, and summarizing the course and structure of knowledge development over time and space. Recently, the fastest-growing area of bibliometric research has been the quantitative and qualitative analysis of literature related to a specific research direction, to reveal research trends, hotspot distribution, and status (Pei et al., 2022; Gu et al., 2023; Ling et al., 2023; Zhiguo et al., 2023). This study aimed to conduct bibliometric research on the use of albumin NPs in oncology within English-language literature using software, including CiteSpace (v6.1.6; https://citespace.podia.com/) and VOSviewer (v1.6.18; https://www.vosviewer.com/). The literature retrieved was used to create knowledge maps, such as co-authorship networks, institutional collaboration networks, co-citations, co-words, and keyword clustering. These maps were then analyzed visually to explore current research hotspots and frontiers (Figure 1). This research may help experts and novices intuitively determine the breadth of their fields, find new topics of interest, gain intimate knowledge of clinical application, and formulate future research plans, thereby providing great convenience for researchers.
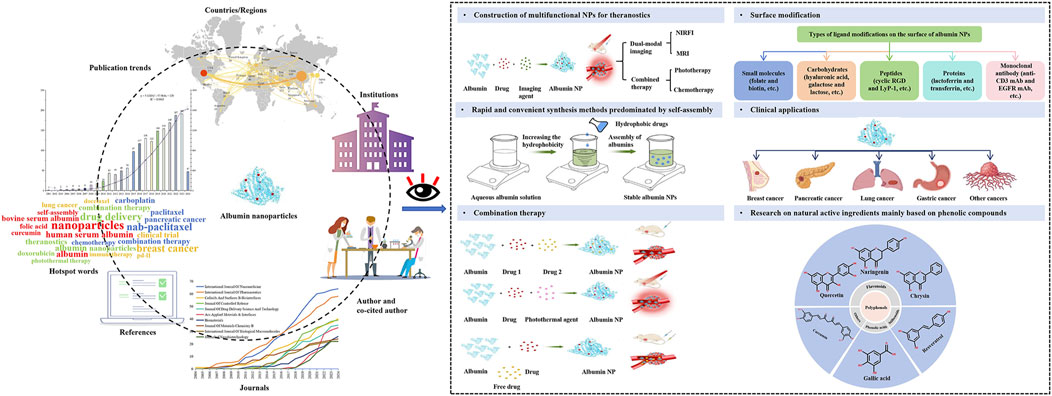
Figure 1. A bibliometric analysis of the research progress and prospects of albumin nanoparticles in oncology.
2 Materials and methods
2.1 Data source
All studies were retrieved and downloaded from the Web of Science Core Collection (WOSCC) database. The WOSCC is one of the most authoritative citation databases in the scientific community. It contains a curated collection of over 21,000 globally recognized, high-impact academic journals, over 200,000 conference proceedings, and the bibliographic abstracts of over 120,000 scientific and technical books, with topics spanning the natural sciences, engineering technology, biomedicine, social sciences, arts and humanities, and other fields. Articles in the WOSCC represent the position of medical science, and numerous existing articles on bibliometric analyses and visualization of scientific literature have used the WOSCC as their data source (Pei et al., 2022; Xu et al., 2023; Cao et al., 2024). Therefore, we selected the WOSCC as the data source for this study. The search queries were as follows: TS = “nanoparticle albumin” OR “albumin-based nanoparticle” OR “albumin-based nanoparticles” OR “albumin based nanoparticle” OR “albumin based nanoparticles” OR “(BSA) nanoparticle” OR “(BSA) nanoparticles” OR “BSA NP” OR “BSA NPs” OR “BSA nanoparticle” OR “BSA nanoparticles” OR “albumin NPs” OR “albumin nanoparticles” OR “albumin nanoparticle” OR “ALB nanoparticle” OR “ALB nanoparticles” OR “HSA nanoparticle” OR “HSA nanoparticles” OR “(HSA) nanoparticle” OR “(HSA) nanoparticles” OR “HSA NP” OR “HSA NPs” or “albumin-bound nanoparticle” OR “albumin-bound nanoparticles” OR “albumin-stabilized nanoparticle” OR “albumin-stabilized nanoparticles” OR “albumin-stabilized nap” OR “albumin-stabilized naps” AND TS = “tumor” OR “neoplasm” OR “tumors” OR “neoplasia” OR “neoplasias” OR “cancer” OR “cancers” OR “anticancer” OR “anti-cancer” OR “antitumor” OR “anti-tumor”. The search date range was between 1 January 2000, and 15 April 2024.
2.2 Screening of the literature
Following the initial data search, two researchers independently reviewed the title, abstract, keywords, author information, and main text of the articles to ensure their relevance to the topic of our study. Only original research articles and review papers were included in this study, and letters, briefings, and book reviews were excluded. In cases of disagreement among the selections, other group members were consulted. Notably, 1,624 articles related to the topic were selected (Supplementary Figure S1).
2.3 Methods of visualization
Bibliometric methods were used to perform visualization analyses on the authors, institutions, countries, journals, co-citations, and co-words of the retrieved literature using the VOSviewer 1.6.18, CiteSpace 6.1.6, Pajek (http://mrvar.fdv.uni-lj.si/pajek/), and Scimago Graphica (https://www.graphica.app/) software. The resulting visualization maps were analyzed to determine the status, hotspots, and trends of research.
3 Results
3.1 Publication trends
Statistical analysis was performed on the retrieved articles to reflect changes in the number of articles published on albumin NPs in the field of oncology (Supplementary Figure S2). From 1 January 2000, to 15 April 2024, 1,624 articles were published on this topic (as no articles were published in 2000, those data were not included in the figure). The average number of articles published per year was 68.57 (2001–2023), with an average annual growth rate of 26.96 (2001–2023). The overall development trends can be roughly divided into three phases, as described in the following paragraphs.
During Phase 1 (2001–2007), fewer than three articles were published per year. Notably, on 7 January 2005, the FDA approved Abraxane®, the first drug synthesized with albumin NP drug delivery technology, for second-line treatment of metastatic breast cancer (Shi et al., 2017).
During Phase 2 (2008–2014), Abraxane® achieved great success and contributed to the growing body of research on albumin NPs as anti-tumor drug carriers. The number of articles published on this topic increased compared with that of the previous phase, although the overall number of publications remained relatively low, with an average of 34.57 articles per year, which may primarily be attributed to the fact that albumin nanodrugs fall under the category of nano-preparations, which presents technical barriers and research challenges.
During Phase 3 (2015–2023), there was a trend of high-level growth and fluctuations, with an average of 146.11 articles published per year, a significant increase from the previous phase. Furthermore, a new albumin NP—sirolimus albumin-bound NP (Fyarro®) was introduced on 23 November 2021, for the treatment of locally advanced unresectable or metastatic malignant PEComa. The launch of this drug succeeded and provided a boost to the field of albumin NP drug delivery technology.
An exponential function was created to represent the annual publication trend: y = 5.1243x2 − 57.964x + 129 (R2 = 0.9943, where x is the year and y is the number of publications per year). This function showed good agreement with the growth of annual publications. Based on this curve, we predicted that the number of articles published each year will steadily increase, indicating a growing interest among researchers in albumin NPs as anti-tumor drug carriers.
3.2 Distribution of countries/regions
Articles related to the application of albumin NPs in the field of oncology were published in 67 countries. China was the most productive country, with 649 articles, followed by the USA with 273 articles. These two countries accounted for 56.77% of the articles published in this field. In Table 1, China ranked first in terms of H-index of 64, followed by the USA of 60, India of 35, Germany of 34, and South Korea of 33. In terms of the average number of citations per article, the top five countries were Germany with 247.27, Australia with 180.44, Spain with 101.93, South Korea with 90.68, and the USA with 75.62. The USA had a high number of citations, H-index, and average citations per article, indicating its positive impact and importance in this field. As shown in Table 1, China had the highest number of publications and the second highest number of total citations among the top 10 countries. However, it ranked only eighth in terms of the average number of citations per article. In contrast, Germany had a much lower number of published articles, only 9.71% of China’s, but had the highest average number of citations per article, seven times that of China, and ranked first among the top 10 countries. These findings suggest that although China has published numerous articles, the quality of those articles was inadequate. In contrast, Germany published high-quality articles that served as valuable references.
The single country publications (SCP) value refers to the number of articles co-written by authors of the same nationality, whereas multiple country publications (MCP) value refers to the number of articles co-written by authors from different countries. The MCP ratio represents the level of global collaboration. China and the USA exhibited the highest MCP values, indicating a high level of collaboration among the top 10 countries with the most publications. However, the MCP ratios of all countries were relatively low, suggesting limited collaboration among different countries.
Visualization analysis was conducted for the publication regions (Supplementary Figure S3). The analysis revealed that the USA had the highest total intensity of cooperation. Further analysis indicated that the USA and China had the closest cooperation. Furthermore, according to the citation burst map (Supplementary Figure S4), Saudi Arabia, Turkey, and Iraq had publication bursts in the last 3 years, suggesting that these countries were relatively active in this field during that period with a concentrated number of articles published.
3.3 Analysis of the research institution network
The retrieved articles in this study were published by 2,262 institutions. The Chinese Academy of Sciences had the highest number of publications (52) and engaged in active cooperation and communication with other institutions, making it one of the most impactful institutions in the field (Table 2). Other institutions included Fudan University (42), Sichuan University (30), Nanjing University (28), and Sungkyunkwan University (28). The total link strength (TLS) indicates the number of co-occurrences of each institution with other institutions, reflecting their collaboration and communication relationships (Pei et al., 2022). Among all institutions, Sungkyunkwan University in South Korea had the highest TLS score of 63, indicating a strong desire to collaborate with other institutions, followed by the Chinese Academy of Sciences (58), Catholic University Korea (57), and Hanyang University in South Korea (55). Apart from these four institutions, cooperation among other institutions was relatively limited (Supplementary Figure S5), which is unfavorable for the development of this field. The top five institutions, ranked by the average citation per paper, were Seoul National University in South Korea (176.06), the University of Texas MD Anderson Cancer Center (81) in the USA, Nanjing University (63.32), Soochow University (62.63) in China, and Sungkyunkwan University (55.14) in South Korea (Table 2). The Chinese Academy of Sciences, Nanjing University, and Sungkyunkwan University are leaders in this field, as evidenced by their relatively high number of publications and average citations per paper.
3.4 Author and co-cited author analysis
The quantity of publications can indicate an author’s level of contribution. The 10 most innovative authors among all authors who published articles related to albumin NPs in the field of oncology are listed in Table 3. Youn YS was the most productive author, with 22 published articles, followed by Choi HG and Lee ES, each with 20 articles.
The co-authorship network map (Supplementary Figure S6A) shows that among the top 10 most productive authors, Youn YS collaborated the most with other authors, mainly with Choi HG, Lee ES, and Oh KT, forming one team. Dinarvand R and Atyabi F collaborated closely and formed another team. Hu Y and Wu J collaborated closely and formed a third team. Among the three teams, the Youn YS team published the highest number of articles and had the highest H-index, making it an important driving force for research in this field. The co-authorship network map also revealed a lack of collaboration and communication among the teams.
The co-cited author network map is shown in Supplementary Figure S6B. A higher number of co-citations suggests that the author is more recognized in the field. The three authors with the highest number of co-citations are Kratz F (300 citations), Elzoghby AO (288 citations), and Desai N (269 citations), which indicates these three authors had the greatest academic impact on the application of albumin NPs in oncology (Table 4). Notably, Desai N was involved in the development and application of Abraxane® and Fyarro®, which are the only two albumin-bound anti-tumor drugs used in clinic (Ibrahim et al., 2005; Wagner et al., 2021). Therefore, Desai N is a pioneer in the application of albumin-based anti-tumor nanomedicine to clinical practice.
3.5 Journal publication analysis
An analysis of publication journals can assist researchers in quickly and accurately identifying current authoritative journals in the field. Table 5 shows the 10 most productive journals that published 363 articles, accounting for 22.35% of all articles published in this field. The International Journal of Nanomedicine published the highest number of articles in this field (64 articles) and had an impact factor (IF) of 8.0 in 2022. It was followed by the International Journal of Pharmaceutics (58 articles) with an IF of 5.8 and Journal of Controlled Release (40 articles) with an IF of 10.8. Among the 10 most productive journals, Biomaterials had the highest IF in 2022 (14.0), the International Journal of Pharmaceutics had the highest H-index (30), and the Journal of Controlled Release had the highest number of citations (5,762 citations). Furthermore, four of the top 10 journals were ranked Q1 (30%), whereas five were ranked Q2 (50%), indicating that the research content in this field is of relatively high quality.
As shown in Table 6, 50% (5/10) of the top 10 co-cited journals were from the USA, and the other 50% (5/10) were from the Netherlands. The Journal of Controlled Release had the most co-citations (3,326 citations), with an IF of 10.8 in 2022. The second and third highest co-citations were found in the Journal of Clinical Oncology (2,423 citations, IF 45.3) and Biomaterials (2,360 citations, IF 14.0), respectively. The Journal of Clinical Oncology had the highest IF (45.3) among the top 10 co-cited journals.
The trends in the annual publication volume of the top 10 journals on the application of albumin NPs in oncology from 1 January 2000, to 15 April 2024 are shown in Supplementary Figure S7A. The number of publications in the top 10 journals continues to increase. The International Journal of Nanomedicine and International Journal of Pharmaceutics were the most productive compared with other journals, indicating a greater focus on publishing articles related to this field.
The dual-map overlays of the journals is shown in Supplementary Figure S7B. The figure is divided into two parts: the left side shows the citing journals, and the right side shows the cited journals. The results indicate the position of albumin NPs research in oncology relative to other major research disciplines. Each point on the map represents a journal, and the lines connecting the left and right sides of the map represent citation links. The trajectories of these links provide an understanding of the interdisciplinary relationships within the field and offer a complete picture of the citations. Four main citation paths were notable, indicating that the research on albumin NPs in oncology mainly focused on journals in the fields of Physics, Materials, Chemistry, Molecular, Biology, and Immunology, whereas the cited articles were mainly from journals in the fields of Chemistry, Materials, Physics, Molecular, Biology, and Genetics.
3.6 Citation analysis
The top 10 most cited and co-cited articles on the use of albumin NPs in oncology are listed in Tables 7, 8. The article by Schmid P et al. had the highest number of citations (2,675 citations) and ranked first in the average number of citations per year (445.83). As the total number of citations tends to increase with time, earlier publications are more likely to be cited. Therefore, we calculated the average number of citations per year. The article by Schmid P et al. ranked first in both the number of total citations and average citations per year, indicating that the research on immunotherapy in the field of albumin NPs in oncology was an important topic for researchers. This study demonstrated that atezolizumab plus nab-paclitaxel combination therapy prolonged progression-free survival among patients with metastatic triple-negative breast cancer (TNBC) in both the intention-to-treat population and the PD-L1-positive subgroup, and it exhibited good safety (Schmid et al., 2018). The article by De Jong WH et al. ranked second in the total number of citations (2,593) and third in the average number of citations per year (162.06). The article provided a review on the application and potential toxicity of drug-loaded NP technology, emphasizing the need for researchers to consider not only the benefits of nano-drug delivery preparations, such as reducing drug toxicity and side effects and improving therapeutic efficacy, but also how to conduct safety assessments on these preparations (De Jong and Borm, 2008). The study conducted by Paz-Ares L et al. ranked third in the total number of citations (2,353) but ranked second in the average number of citations per year (392.17). The article demonstrated that the addition of pembrolizumab to either the carboplatin-plus-paclitaxel or nab-paclitaxel combination chemotherapy regimen resulted in significantly longer overall survival and progression-free survival (Paz-Ares et al., 2018). This article shows that research on clinical applications is more likely to attract the attention of scholars.
CiteSpace was used to perform co-citation analysis (Figure 2A), involving various data mining tools to analyze the cited articles. The results can provide researchers with multifaceted information, including the ability to identify articles with far-reaching impact in each field. As shown in Figure 2A, the most influential co-cited article was that of Elzoghby AO et al., with 92 co-citations (Table 8). The article provided a detailed discussion of the various types of albumin NPs, their preparation methods, and the main in vivo and in vitro research findings. Furthermore, it highlighted the potential of albumin NPs in targeted drug delivery to specific tumor sites (Elzoghby et al., 2012).
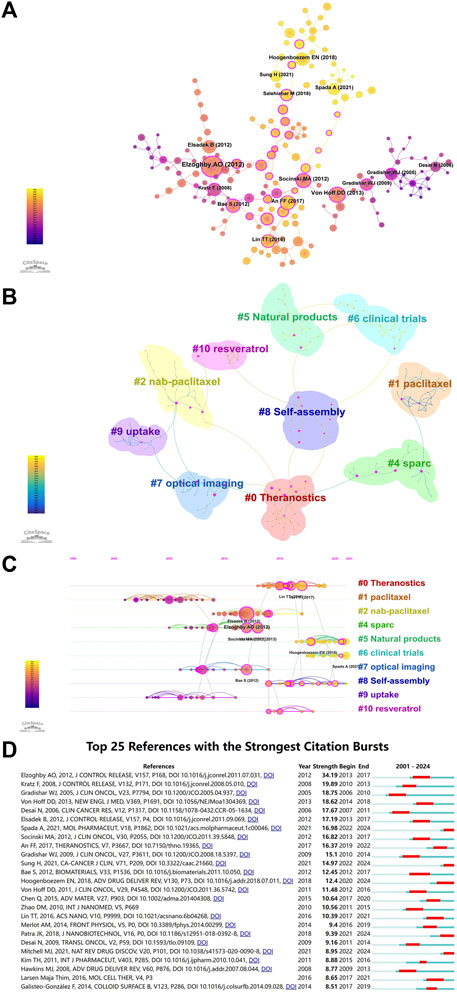
Figure 2. (A) Co-citation network of references. The size of the circle is positively correlated with the number of citations. Purple indicates earlier citations and yellow indicates more recent citations. The overlapping colors indicate that the article was cited in all corresponding years. The lines connecting the circles represent co-cited articles. The rose red nodes are the key nodes. (B) Cluster analysis of co-cited references. The different colors mean different clusters. (C) Timeline distribution of co-cited references. The size of the circle is proportional to the number of co-citations. The line between the articles represents co-citation, i.e., co-cited articles. Purple indicates earlier co-citations, and yellow indicates more recent co-citations. Overlapping colors indicate the article was co-cited in all the corresponding years. Nodes in rose red are nodes with relatively strong centrality (i.e., occupies a central position and serves as a hub). Co-cited articles in the same cluster are positioned along the same horizontal line. The timeline for the occurrence of co-cited articles is placed at the top of the visualization map, with the right representing more recent periods. (D) The top 25 References with the Strongest Citation Bursts.
Next, cluster co-cited analysis was performed based on co-citation analysis (Figure 2B). CiteSpace provides modularity (Q value) and mean silhouette (S value) to assess the clarity and reasonableness of the network structure and clusters. A Q value > 0.3 indicates a significant cluster structure, whereas an S value > 0.5 indicates reasonable clustering. The Q value of Figure 2B is 0.8469 (>0.3), and the S value is 0.961 (>0.5), indicating significant cluster structures and meaningful clustering of the keywords in the sample. Figure 2B also displays that the co-cited articles formed 10 clusters (few nodes in #3 are of no research significance and have been automatically filtered out by CiteSpace), with the top three being: #0 theranostics, #1 paclitaxel, and #2 nab-paclitaxel. The number of articles in each cluster is negatively correlated with the cluster number. For example, cluster #0 contains the highest number of articles. We performed a timeline analysis of the co-cited articles based on their clustering to elucidate the changes in research hotspots over time (Figure 2C). The vertical axis in the figure represents the cluster labels (10 clusters), and the horizontal axis represents the time at which the key reference nodes appeared. The figure illustrates that paclitaxel, uptake, and SPARC (secreted protein acidic and rich in cysteine) were the earliest hotspots (#1, #9, and #4, respectively), followed by theranostics (#0), natural products (#5), clinical trials (#6), and self-assembly (#8), which appeared later, first occurring in 2013, 2017, 2018, and 2014, respectively. However, these fields have received growing interest in recent years and are now considered research hotspots.
The application of albumin NPs in oncology from 1 January 2000, to 15 April 2024 was analyzed using CiteSpace to identify the top 25 citation bursts. A citation burst is defined as a surge in the citations of a given article within a specific period. The article by Elzoghby AO et al. had the strongest citation burst, with the burst period occurring in 2013–2017 (Figure 2D). This article was also the most influential co-cited article (Table 8). The first article to undergo a citation burst (in 2005) was by Gradishar WJ et al., which reported the results of a phase III clinical trial of Abraxane®, demonstrating greater efficacy and a better safety profile than standard paclitaxel based on polyethylated castor oil.
Recent highly cited articles (2021–2024) undergoing bursts were published by Spada A et al., Sung H et al., Hoogenboezem EN et al., Patra JK et al., and Mitchell MJ et al. (Figure 2D). The article by Spada A et al. described the advantages of endogenous substances such as albumin as drug delivery carriers, along with the importance of drug carriers to drug properties in biological systems and the possible future application trends of drug delivery systems (Spada et al., 2021). The article by Sung H et al. provided an update on the global cancer burden using the GLOBOCAN 2020 estimates of cancer incidence and mortality projected by the International Agency for Research on Cancer (Sung et al., 2021). Hoogenboezem EN et al. systematically reviewed the advantages of albumin as an anti-tumor drug carrier, drug–albumin binding methods, loadable drugs, and the results of in vivo and in vitro studies. In addition, the paper also highlighted that characterizing how different classes of cargo and types of albumin engagement affect systemic and intracellular trafficking will be critical for optimizing future therapeutic candidates (Hoogenboezem and Duvall, 2018). The review by Patra JK et al. summarized the recent progress and development prospects in the field of nanomedicines and nano-based drug delivery systems and highlighted the opportunities and challenges of developing nanomedicines from synthetic and natural sources and their clinical applications (Patra et al., 2018). Mitchell MJ et al. demonstrated advances in NP design that overcome heterogeneous barriers to delivery, arguing that intelligent NP design can improve overall patient outcomes by improving efficacy in general delivery applications while enabling tailored designs for precision applications (Mitchell et al., 2021).
3.7 Hotspot word frequency analysis
Keywords are a brief expression of an article’s primary theme and content. Related keywords that appear in the same article exhibit keyword co-occurrence, and the number of co-occurrences can indicate their proximity. A keyword co-occurrence network represents the research focus and connections within a field. By analyzing the keywords, we can clarify the hotspots and frontiers of the research field and predict future developments.
Based on the top 20 most frequently used keywords (Table 9), the most investigated drugs in this field were nab-paclitaxel, paclitaxel, doxorubicin, curcumin, and gemcitabine. The most investigated tumors were breast cancer and pancreatic cancer, and the most investigated carrier protein was HSA. However, HSA has a limited yield and is costly to produce owing to its derivation from human blood. Therefore, BSA has also been widely investigated because of its similar amino acid composition, molecular weight, and spatial structure to those of HSA. Furthermore, it is more widely available and cost-effective than HSA. However, BSA is an exogenous macromolecule that can induce allergic reactions in humans, limiting its use mainly to animal studies (Voltolini et al., 2013). In contrast, HSA is non-immunogenic as it is derived from humans. Therefore, it is extensively used in clinical trials as a material in the final product. The main treatment method adopted in the studies was chemotherapy. Another focus of research in this field was photothermal therapy based on albumin NPs.
Using VOSviewer, co-occurrence analysis was conducted on the article keywords, with a minimum of three occurrences for each keyword. The analysis resulted in four clusters (Figure 3A), which can be summarized as the following four themes: “Comprehensive Evaluation,” “Multifunctional NPs and Combination Therapy,” “Nab-paclitaxel,” and “Clinical Application.” The red cluster represents comprehensive evaluation, which involves constructing, characterizing, and evaluating drug-loaded albumin NPs in vivo and in vitro. The keywords in this cluster included “HSA,” “BSA,” “self-assembly,” “curcumin,” and “folic acid.” The green cluster represents the study on combination therapy based on albumin nanotechnology and the construction of multifunctional albumin NPs. The keywords in this cluster included “photothermal therapy,” “theranostics,” “photodynamic therapy,” “combination therapy,” and “doxorubicin.” The blue cluster represents the topic “nab-paclitaxel,” which involves the study of the application of nab-paclitaxel monotherapy and combination therapy in different types of tumors. The keywords included in this cluster included “nab-paclitaxel,” “non-small cell lung cancer,” “carboplatin,” “pancreatic cancer,” and “gemcitabine.” The yellow cluster represents studies on clinical application, focusing on the clinical research of various anti-tumor treatment options based on albumin NPs. The keywords in this cluster included “breast cancer,” “docetaxel,” “immunotherapy,” “lung cancer,” and “clinical trial.”; Keywords were clustered by CiteSpace, and a total of 11 clusters were obtained (Figure 3B). The Q value of Figure 3B was 0.8256 (>0.3), and the S value was 0.9643 (>0.5), indicating significant cluster structures and meaningful clustering of the keywords in the sample. As shown in Figure 3B, these keywords formed 11 clusters: #0 drug delivery, #1 human serum albumin, #5 human serum albumin nanoparticles, and #6 bovine serum albumin nanoparticles can be classified under comprehensive evaluation; #2 breast cancer, #3 lung cancer, #4 cancer therapy, #8 pancreatic cancer, and #9 esophageal squamous cell carcinoma can be classified under clinical application; and #7 photodynamic therapy and #10 multimodal therapy can be classified under multifunctional NPs and combination therapy. These classifications were generally consistent with those of VOSviewer.
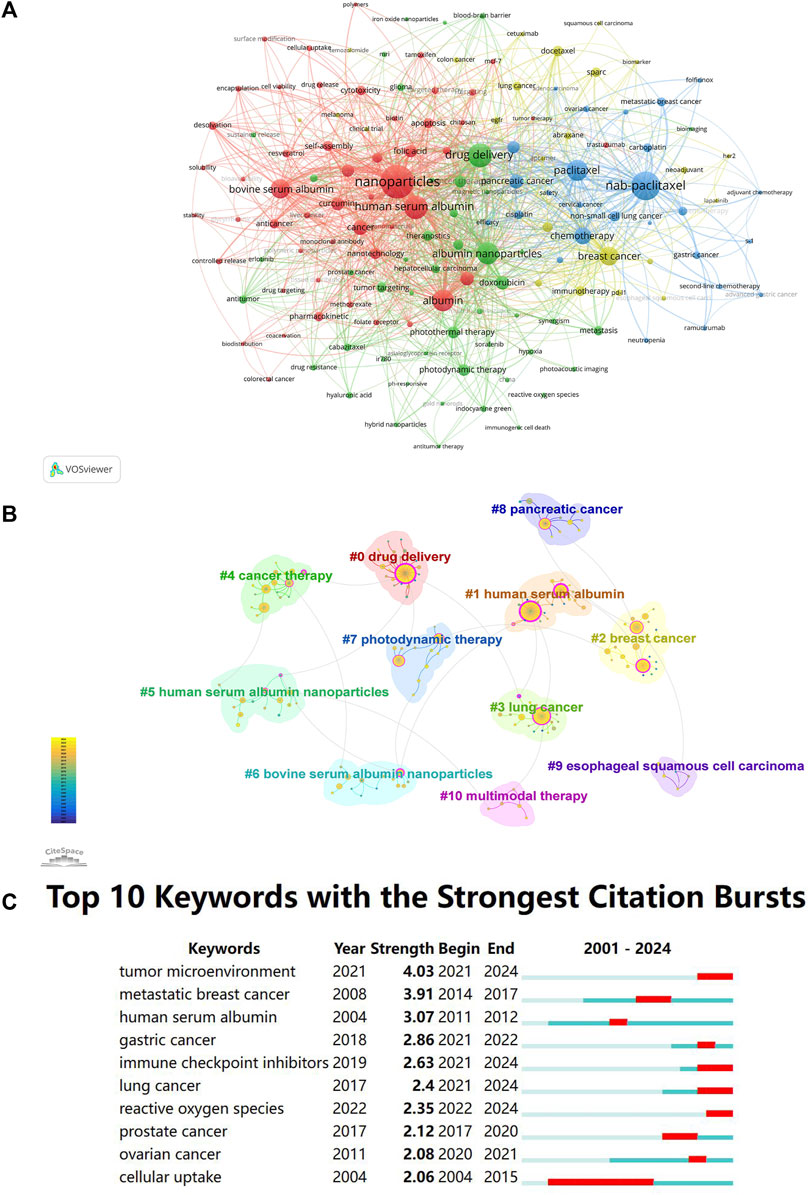
Figure 3. (A) Clustering network of keywords by VOSviewer. Each circle and label form one node. The size of the circle is positively correlated with the frequency of keywords. The thickness of the line connecting the circles indicates the strength of the positive correlation between keywords. Nodes of different colors form different clusters, and different colors represent different research directions. (B) Clustering network of keywords by CiteSpace. (C) The top 25 keywords with the strongest citation bursts.
The term “burst map” refers to the sudden increase in the frequency of a particular keyword within a specific time frame. The top 10 keywords with the strongest bursts between 1 January 2000, and 15 April 2024 in the field of albumin NPs in oncology are shown in Figure 3C. The red area in the figure indicates the burst period for each keyword. The most popular keywords from 2022 to 2024 were “tumor microenvironment,” “gastric cancer,” “immune checkpoint inhibitors,” “lung cancer,” and “reactive oxygen species.” The burst of tumor microenvironment (TME) and immune checkpoint inhibitors suggests that albumin NP-based tumor immunotherapy is a current hotspot. Tumor immunotherapy is an emerging treatment method that activates the immune system to fight tumors and exhibits the advantages of long-lasting efficacy, few side effects, and wide application. Conversely, the traditional drug delivery method has poor targeting and a short residence time in TME, which limit the therapeutic effect. The albumin NPs effectively penetrate the TME and specifically deliver immunotherapy drugs to the TME for a certain period, which improves the therapeutic effect (Yang et al., 2021). Reactive oxygen species (ROS) refers to a class of oxygen-containing substances that are directly or indirectly converted from oxygen molecules and have more active chemical reactivity than oxygen molecules. They have multiple functions such as killing tumors, inhibiting chemoresistance, and inducing systemic immunity (Bhushan and Gopinath, 2015). The burst of the keyword “reactive oxygen species” indicates that these ROS-based anti-tumor therapies (such as chemodynamic, photodynamic, and sonodynamic therapies) are hot topics in the field of albumin NPs in oncology. Similarly, bursts of the keywords “gastric cancer” and “lung cancer” indicate that these tumors have attracted much attention from researchers in recent years.
4 Discussion
4.1 Overview of research
The research indicates a growing trend in the use of albumin NPs as anti-tumor drug carriers over the past 20 years, with a notable increase in publications after 2015 suggesting a continued interest in this topic. Considering the numerous advantages of albumin NPs as drug carriers, including biodegradability, biocompatibility, non-immunogenicity, and long half-life, as well as the successful launch of Abraxane® and Fyarro®, albumin NPs hold great promise in the targeted delivery of anti-tumor drugs. After analyzing research regions, we found that China and the USA published a significantly higher number of articles than other countries/regions and these were the two most productive countries in this field. However, the average citation frequency of Chinese articles was low, ranking eighth among the top 10 countries. These findings suggest that, despite the large number of articles published in China, the quality of their articles is relatively low. Therefore, researchers should be guided and encouraged to conduct innovative research. Quantity alone is not meaningful without high-quality research results. Furthermore, when evaluating scientific research performance, quantitative evaluation indicators cannot replace research evaluation. Instead, a quality-oriented evaluation system should be actively explored to promote the high-quality development of scientific research. The USA has a dominant presence in this field, as evidenced by high citations, H-index, average citations per paper, and TLS. These objective metrics indicate the impact of the articles from the United States.
Eleven of the 20 most productive institutions in this field were from China, which ranked first in productivity. This indicates that Chinese researchers prioritize research in this field and have invested substantial research effort, resulting in China having the highest number of publications. Researcher network analysis revealed that four authors from four South Korean institutions (Youn YS, Choi HG, Lee ES, and Oh KT) formed one team and published the highest number of articles with the highest H-index, making them an important driving force for research in this field. Kratz F, Elzoghby AO, and Desai N were identified as co-cited authors with considerable academic influence in this field. Desai N was involved in the development and application of Abraxane® and Fyarro® and is a pioneer in the application of albumin-bound anti-tumor drugs to clinical practice.
The International Journal of Nanomedicine and the International Journal of Pharmaceutics had the highest number of publications in this field, indicating that these two journals focus on the publication of articles in this field. Therefore, researchers in this field can be recommended to contribute to these journals. The Journal of Controlled Release had the highest number of co-citations, whereas the Journal of Clinical Oncology had the highest IF among the co-cited journals. For researchers to gain a better understanding of research advances in this field, they should read articles published by the authors or journals mentioned above. Furthermore, these high-impact authors may serve as potential collaborators. However, collaboration among different countries, institutions, and teams is limited, particularly among those from different countries, which hinders this aspect of research. Therefore, we recommend that researchers improve collaboration, mutual learning, and communication.
4.2 Research hotspots and frontiers
Co-citation analysis is the use of data mining tools to analyze cited articles, authors, and journals. The results provide researchers with multifaceted information, including the identification of authors with far-reaching impact within the field, research teams who are active within the academic community, and trends in research topics, thereby facilitating better recognition of important research directions and future research hotspots.
Based on the citation analysis, we found that in addition to review articles, three articles by Schmid, P et al., Paz-Ares et al., and Von Hoff DD et al. were heavily cited or co-cited. These three articles were all clinical studies on the combination therapy of nab-paclitaxel, which indicates that this topic is of great concern to researchers. The combination therapy regimens discussed in these articles were all related to nab-paclitaxel plus immune checkpoint inhibition, indicating that research on immune checkpoint inhibitors is also a hot research direction in this field. Cluster analysis was used to identify content in the knowledge map network and facilitate the clear extraction of popular topics within the research field. The cluster timeline of co-cited articles revealed that theranostics, natural products, clinical trials, and self-assembly have received growing attention in recent years. Through the analysis of keyword co-occurrence, we found that research on albumin NPs mainly focuses on comprehensive evaluation, multifunctional NPs, combination therapy, nab-paclitaxel, and clinical application. The analysis of high-frequency keywords revealed that the most investigated drugs were paclitaxel, doxorubicin, curcumin, and gemcitabine. The most investigated tumors were breast cancer and pancreatic cancer. The most widely investigated carrier proteins were HSA and BSA. Citation and keyword burst maps reflect research frontiers, hotspots, and growing trends within a field. Notably, according to citation and keyword burst maps, articles on TME, gastric cancer, immune checkpoint inhibitors, lung cancer, and ROS have been extensively cited in recent years, and these topics may become research hotspots.
Based on the results of our analysis of article co-citation, clusters, bursts, keyword co-citation, high-frequency keywords, cluster labels, and our cognition in this field, we suggest that the six categories mentioned in the following text may become future research hotspots and frontiers.
4.2.1 Rapid and convenient synthesis methods predominated by self-assembly
Various methods have been developed for preparing albumin NPs, including thermal gelation (Qi et al., 2010), nano-spray drying (Arpagaus et al., 2018), desolvation (Jahanban-Esfahlan et al., 2016), emulsification (Zu et al., 2013), NabTM technology (Wan et al., 2015), and self-assembly (Safavi et al., 2017) (Table 10). Thermal gelation is a simple and high-yield method that produces NPs with good uniformity. However, it requires a high preparation temperature that is unsuitable for encapsulating unstable drugs. Nano-spray drying enables the production of NPs with high uniformity and encapsulation efficiency, making them capable of encapsulating hydrophilic drugs. However, this method has strict preparation requirements, long preparation times and produces NPs with a relatively large particle size. Desolvation is a commonly used method for preparing albumin nanocarriers owing to its simplicity and rapidity, despite the need for the addition of organic solvents or cross-linking agents, which can be relatively toxic (Loureiro et al., 2016). Another simple and cost-effective method is emulsification. However, the emulsification process can disrupt the stability of albumin, making it difficult to control the microscopic morphology of the NPs and resulting in poor reproducibility and high batch-to-batch variation. Furthermore, like desolvation, the preparation process involves the use of organic solvents or cross-linking agents, which can be relatively toxic.
NabTM technology uses a self-cross-linking method, eliminating the need for cross-linking agents. The process involves disrupting the disulfide bonds in albumin molecules through heating, reductants, and high pressure to induce homogeneous unfolding. This is followed by the resynthesis of disulfide bonds within and between albumin molecules, resulting in the cross-linking of albumin to form NPs. Notably, only two albumin-bound anti-tumor drugs are currently available worldwide—Abraxane® and Fyarro®—both of which are prepared using NabTM technology. However, the preparation process still involves the addition of organic solvents, which may lead to issues with solvent residues (Ding et al., 2014). In addition, NabTM technology requires relatively high equipment standards. Furthermore, albumin aggregation during the homogenization process may cause difficulties in dispersing the product after freeze-drying. Each method listed above has its own advantages and disadvantages, and the appropriate method should be selected based on the properties of the drugs when preparing NPs. Moreover, new preparation technologies are actively being explored in addition to the aforementioned methods. Self-assembly preparation method has gained increasing attention in recent years (Safavi et al., 2017). Similar to NabTM technology, self-assembly uses the self-cross-linking approach without requiring the highly specialized equipments and organic solvent residues associated with NabTM technology. Furthermore, self-assembled NPs have small particle sizes and good flexibility, enabling effective penetration of physiological barriers and efficient drug delivery to target tissues or organs. Self-assembly has become a research hotspot in recent years because of its advantages and has been extensively studied and applied (Lin et al., 2016; Liu et al., 2023; Lai et al., 2023). However, this method also has limitations, including the requirement of the use of reductants such as β-mercaptoethanol, dithiothreitol, and cysteine, which pose certain safety risks during preparation. Therefore, further research is necessary to optimize this method.
4.2.2 Surface modification
Owing to the EPR effect and macropinocytosis, albumin can evade the recognition and phagocytosis of the mononuclear phagocyte system and passively target the liver, kidney, bone marrow, and other organs. However, the EPR effect demonstrates great heterogeneity in solid tumors, varying among different tumor types, sizes, sites, patients, and stages of development (Subhan et al., 2023). Therefore, the EPR effect does not guarantee the efficacy and reliability of targeted therapy. To enhance the delivery of chemotherapy drugs to specific tumor sites, researchers usually modify the surface of albumin NPs to achieve active targeting of albumin NPs in vivo. The surface-modified albumin NPs enter the blood circulation and the tumor cells by recognizing the highly expressed receptors on the membranes of tumor cells. Compared with the unmodified albumin NPs, surface-modified albumin NPs exhibit a more specific targeting effect and further increase the accumulation of NPs at the tumor site. In tumor tissues, the tight junctions of cells disappear and receptors on the tumor cells are exposed, which mediates the entry of surface-modified NPs into cells. Conversely, the vascular epidermal junctions on the surface of some normal cells are tightly connected, and receptors are not directly exposed to the blood circulation, preventing the entry of the surface-modified NPs in these cells. Common methods for surface modification of albumin NPs include covalent coupling modification, conjugated ligand modification, and antibody modification. Among them, ligand-modified albumin NPs have been more frequently studied. The types of modified ligands currently studied include small molecules (such as folic acid and biotin), carbohydrates (such as hyaluronic acid, galactose, and lactose), peptides (such as cyclic RGD and LyP-1), proteins (such as lactoferrin and transferrin) and monoclonal antibody (Monoclonal antibody (anti-CD3 mAb and EGFR mAb) (Tao et al., 2021) (Figure 4).
Folic acid (FA) is one of the most widely used ligands owing to its high efficiency and low cost. Its receptor is overexpressed on the surface of many human tumors, such as ovarian, lung, breast, and endometrial cancers. The upregulation of folate receptors in tumor tissue leads to lower local toxicity and greater anti-tumor efficacy of folate-related therapeutic drugs compared with those of non-targeted drugs. Therefore, the use of FA to modify albumin nanomedicine increases specific tumor targeting (Bardania et al., 2023). Firouzabadi K et al. used desolvation to synthesize polyethylene glycol-modified albumin NPs loaded with naringenin and bound FA to the NP surface and reported that these NPs exhibited dramatic anti-breast cancer effects (Firouzabadi et al., 2023).
Hyaluronic acid (HA) is another widely used ligand. It has the broadest application prospects and is also a current research hotspot. Albumin and HA have rich functional groups and can be easily combined together. The negatively charged HA in the physiological environment reverses the positive charge on the surface of albumin, avoiding the non-specific adsorption caused by the positive charge and prolonging the half-life of NPs in vivo. HA can specifically bind to various receptors that are highly expressed in tumor cells, such as cell surface receptor CD44, in a variety of cancers, including breast, lung, and gastric cancers. In addition, HA, like albumin, is biocompatible, biodegradable, and non-immunogenic, and their combination can better adapt to the in vivo environment (Lei et al., 2021; Subhan et al., 2023). Based on these advantages, the combination of HA and albumin has irreplaceable advantages as a nano-carrier for chemotherapy drugs, and some studies have demonstrated their potential and development prospects. Ye M et al. encapsulated the fluorescent tracer boron drug BS-CyP in albumin NPs and modified the NPs with HA to develop a novel tumor-targeting boron NP. Notably, the NPs effectively delayed the release of drugs in tumors, enhanced the accumulation of drugs in tumors, and exhibited good safety with no notable toxicity to cells and mice.
Studies on modified ligands such as glycyrrhizin, lactose, Arg–Gly–Asp peptide, aptamer, and transferrin are extensive and show an increasing trend year over year (Saleh et al., 2019; Stecanella et al., 2021; Zheng et al., 2023). Owing to the role of surface modification in improving the targeting of albumin nanodrugs, prolonging the circulation time in vivo and increasing the selective accumulation of drugs at tumor sites may result in broad application prospects in the field of albumin NPs in oncology.
4.2.3 Construction of multifunctional NPs for theranostics
Although numerous diagnosis and treatment methods have been developed, cancer still poses a global threat to human health. The mortality rate of cancer continues to increase each year. Therefore, more effective cancer treatment strategies are needed to achieve more sensitive diagnosis methods and efficacious treatment (Siddique and Chow, 2022). Theranostics based on multifunctional nanomedicine is a powerful strategy that combines diagnostics with therapeutic regimens to overcome the shortcomings of current conventional clinical treatments for cancer (Hoogenboezem and Duvall, 2018). However, a single diagnostic and treatment method is usually insufficient because of the insidiousness and drug resistance of tumors. Therefore, the long-term goal has been to develop multifunctional NPs that combine multiple diagnostic and therapeutic modalities. Albumin NPs have been widely employed in numerous fields, including drug delivery, bioimaging, diagnostics, and therapeutics, based on their unique physical, chemical, and biological properties (An and Zhang, 2017). Albumin NPs have a large specific surface area and numerous groups for chemical modification, making them an excellent platform for designing and constructing multifunctional NP systems for theranostics. Multifunctional albumin NPs can combine magnetic resonance imaging (MRI) with near-infrared fluorescence imaging (NIRFI), enabling the high spatial resolution of MRI to complement the high sensitivity of NIRFI (Liu et al., 2023). In addition, photothermal therapy (PTT) can be combined with photodynamic therapy (PDT) during imaging to overcome the heat shock effect of PTT and the hypoxic TME of PDT, thereby achieving the combination of multiple diagnostic and therapeutic modalities to improve treatment outcomes (Sahu et al., 2016; Liu et al., 2023).
Heptamethine cyanines with near infrared (NIR) light responsiveness are the most widely studied reagents for bioimaging and diagnosis. Their encapsulation in nanomaterials enables high accumulation at tumor sites, allowing tumor visualization via NIR fluorescence and photoacoustic imaging. After interacting with NIR light, these heptamethine cyanine-incorporating nanomaterials produce photothermal/photodynamic effects with high spatio–temporal resolution and minimal side effects, thereby improving therapeutic outcomes (Leitão et al., 2020). Indocyanine green (ICG) is a star member of the heptamethine cyanine family, as it is one of the few organic dyes approved by the FDA for medical imaging and diagnosis. Besides its bioimaging and diagnostic functions, ICG serves as a multifunctional drug to generate heat or ROS in response to NIR in PTT or PDT (Pham et al., 2020). Loading ICG into albumin NPs can overcome the limitations of free ICG, such as poor stability, a short half-life, and a lack of tissue targeting. Furthermore, it allows for simultaneous application with other co-loaded reagents to achieve imaging-guided combination therapy with multiple therapeutic modalities, including PTT, PDT, thermotherapy, and chemo-PTT, thereby greatly enhancing treatment outcomes (Liu et al., 2018; Kim et al., 2021; Zhong et al., 2021) (Figure 5). However, ICG has low photostability and low fluorescence quantum yield, stimulating the development of other members of the heptamethine cyanine family. IR780 is another heptamethine cyanine reagent that has shown great potential for biomedical applications and drug delivery, and it has been widely used in PTT and PDT because of its high selective uptake by tumor cells and phototoxicity to tumor cells under NIR light irradiation. However, its strong hydrophobicity, poor photostability, poor tolerance, and high toxicity in vivo limit its clinical application. The use of albumin NPs as IR780 nanocarriers is an effective strategy to overcome this. Lian et al. co-loaded IR780 and docetaxel (DTX) in albumin NPs for targeted imaging and for PTT/PDT with chemotherapy for castration-resistant prostate cancer treatment. Notably, HSA–IR780–DTX NPs demonstrated significant targeting and therapeutic potential for castration-resistant prostate cancer treatment (Lian et al., 2017).
The construction of multifunctional NPs for theranostics in which albumin NPs act as carriers of magnetic resonance contrast agents is a current research hotspot. Gadolinium-based contrast agents (GBCAs) are mostly used in clinically enhanced MRI. However, GBCAs lack tumor specificity and can cause chronic gadolinium poisoning, limiting their clinical application. Encapsulation of GBCAs in albumin NPs may overcome these challenges (Du et al., 2016). Xuejiao Song et al. used polypyrrole (PPy), Gd (3+)-labeled photosensitizer chlorine e6 (Ce6), and BSA to construct PPy@BSA–Ce6 NPs that exhibited strong tumor aggregation and improved the synergistic therapeutic effects of PDT and PTT (Song et al., 2015). In addition, some metal particles (such as Fe3+) can be used as MRI contrast agents and may induce tumor cell apoptosis. Qi Xie et al. used BSA, Fe3+-based metal–phenolic networks, and bleomycin (BLM) to construct an all-in-one therapeutic nanoplatform (BFE@BSA NPs) for TNBC with synergistic photothermal and chemodynamic therapy guided by MRI. After entering the body, under laser irradiation, BFE@BSA NPs converted light into heat to ablate tumors. The elevated local temperature accelerated the Fenton reaction initiated by Fe2+ (from Fe3+ reduction by glutathione) and BLM, further killing tumor cells. Moreover, owing to the presence of Fe3+, BFE@BSA NPs can be used as T1-weighted MRI contrast agents to provide diagnosis and treatment monitoring for individualized precise therapy (Xie et al., 2022).
Furthermore, in addition to NIR and MRI imaging, computer X-ray tomography and photoacoustic image-guided PDT and PTT therapy for cancer using albumin NPs have also been widely studied in recent years, which may catch the attention of future researchers (Wu et al., 2020).
4.2.4 Research on natural active ingredients mainly based on phenolic compounds
Natural products with anti-inflammatory, anticancer, and antioxidant properties have been another focus of research. Encapsulation of these compounds using various delivery methods enhances their stability and bioavailability in vivo, reduces adverse reactions, and improves targeting, which enhances their anti-tumor effects. One example of an extensively studied and encapsulated natural active ingredient in the application of albumin NPs to oncology is paclitaxel (Chavda et al., 2022). Phenolic compounds, which are widely found in natural plants, are the most studied and encapsulated natural active ingredients in the albumin NPs for oncological application owing to their anti-tumor, anti-inflammatory, and anti-microbial capabilities (Wang et al., 2022). Phenolic compounds can be divided into three main categories: flavonoids, stilbenoids, and phenolic acids. Flavonoids are the largest category of phenolic compounds and are the primary focus of research (Guo et al., 2021), including quercetin (Desale et al., 2018), naringenin (Firouzabadi et al., 2023), diosgenin (Ayatollahi et al., 2022), and chrysin (Ferrado et al., 2019). Furthermore, stilbenoids (mainly resveratrol (Geng et al., 2017; Long et al., 2020; Zhao et al., 2020)) and curcuminoids (mainly curcumin (Kim et al., 2017; Salehiabar et al., 2018; Matloubi and Hassan, 2020)) are the most studied non-flavonoid phenolic compounds in this context, whereas phenolic acids have been studied less frequently. Figure 6 presents the primary classifications of phenolic compounds and their representative compounds in the albumin NPs for oncological application.
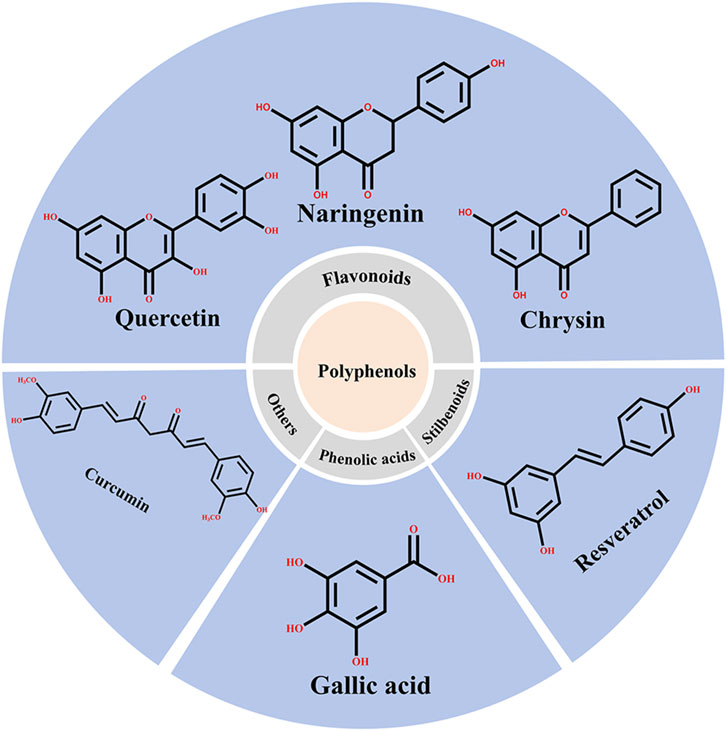
Figure 6. Classification of polyphenolic compounds and their representative compounds in the application of albumin NPs to cancer research.
The prominent studies on phenolic compounds in the application of albumin NPs to oncology are listed in Table 11. Curcumin is the most investigated phenolic compound, with BSA being the most widely used carrier and self-assembly being the most common preparation method. Curcumin, a natural fatty acid synthase inhibitor, inhibits the proliferation of tumor cells, induces apoptosis of tumor cells, and is widely used in the treatment of breast cancer, lung cancer, pancreatic cancer, and other tumors (Ma et al., 2022). However, the hydrophobicity and instability of curcumin have led to its limited clinical application. Therefore, the development of nano-drug delivery systems for curcumin is particularly important to improve its therapeutic efficiency. In addition, the pharmacological activity of curcumin reduces the side effects of paclitaxel. Accordingly, the co-loading of paclitaxel and curcumin in albumin NPs may exert their combined anti-tumor effect and reduce the side effects of paclitaxel (Ashrafizadeh et al., 2020; Gao et al., 2024). Consequently, curcumin is the most studied phenolic compound in the field of anti-tumor albumin NPs in oncology. In addition, Table 11 shows that although phenolic compounds have been widely used in anti-tumor research, studies on this type of drugs in this field are limited.
Phenolic compounds have gained attention owing to their diverse biological activities and pharmacological effects, such as antioxidant, anti-inflammatory, anticancer, and anti-viral activities. However, the clinical use of certain phenolic compounds is limited due to their instability, low solubility, short half-life, and high toxicity. Drug-loaded albumin NPs enhance the stability, solubility, and half-life of these drugs while also facilitating their targeted delivery. The drugs can be locally released, thereby reducing systemic toxicity, improving anticancer efficacy, and making them more promising for clinical use. However, compared with research on other types of nanocarriers, the anti-tumor research on phenolic compounds based on albumin NPs is lacking. In line with the good performance of phenolic compounds and the brilliant achievements of this class of drugs in the field of anti-tumor nanocarriers, including albumin NPs, the research on incorporating phenolic compounds into albumin NPs to improve their efficacy and safety or exerting a synergistic anti-tumor effect with other drugs could be a research hotspot in the future.
4.2.5 Combination therapy
Achieving the desired anti-tumor effect solely through a single treatment method is difficult because of the considerable heterogeneity and genetic instability of tumors, as well as their complex pathogenic mechanisms. Based on in-depth research on the interaction mechanisms among different treatment methods, combination therapy has gained considerable momentum in recent years. This approach involves combining targeted therapy, cytotoxic chemotherapy, and immunotherapy. Combination therapy can include the combination of different therapeutic drugs or modalities. Multifunctional NPs are commonly used for combination therapy. Albumin NPs are useful owing to their large specific surface area and the availability of multiple groups for chemical modification. They can be used to co-load different therapeutic molecules (Liu et al., 2018; Hao et al., 2019), such as paclitaxel and resveratrol, enabling the synergistic effect of the two drugs and overcoming resistance to paclitaxel (Zhao et al., 2020) (Figure 7A). Besides therapeutic drugs, photosensitive molecules and photothermal agents can also be loaded onto albumin NPs. These substances are typically hydrophobic and require organic solvents for dissolution, which can be toxic. Furthermore, they lack tumor-targeted accumulation and are easily cleared from the body (Li et al., 2020). These deficiencies have limited the potential for clinical translation of these substances. However, these limitations can be resolved by loading them onto albumin NPs.
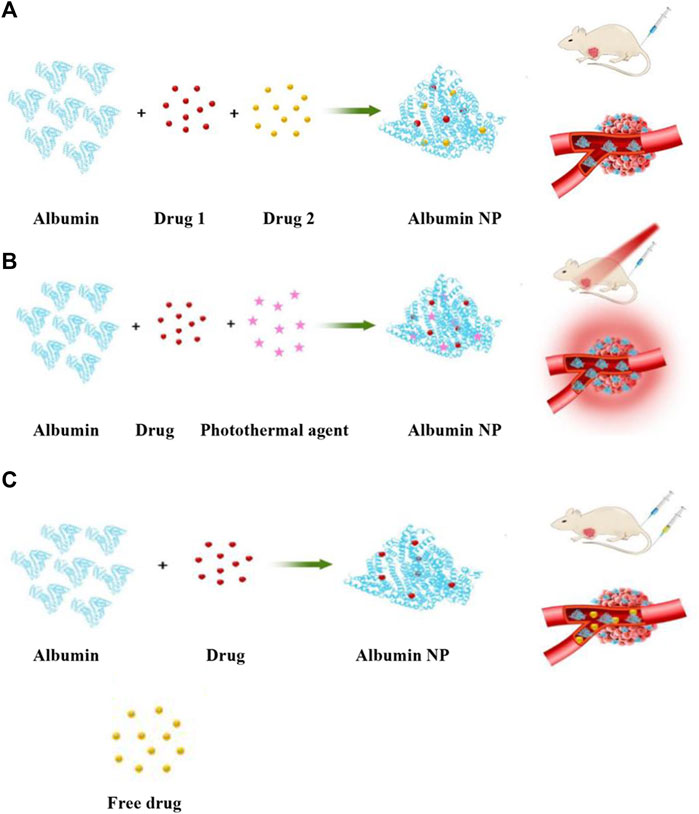
Figure 7. (A) Co-loading of different therapeutic molecules into the same albumin NP. (B) Co-loading of substances such as photosensitive molecules or photothermal agents with therapeutic molecules into the same albumin NP. (C) Combination of albumin NP drugs with another free drug.
Unlike free drugs, chemotherapy drugs, immune adjuvants, and photosensitizers can be loaded into the same NP through the albumin multifunctional nanoplatform and enter the tumor tissue at the same time, enabling a variety of treatments to be performed simultaneously or in sequence. This development allows for complementary relationships between various therapies that overcome each other’s deficiencies and improve the overall therapeutic effect. Therefore, albumin NP drug-loading technology has sparked the development of novel anticancer technologies, such as phototherapy (including PDT and PTT), thermotherapy, and sonodynamic therapy. Consequently, multifunctional NPs can be used to achieve combination therapy with novel anticancer technologies or conventional chemotherapy (Battogtokh and Ko, 2017; Pham et al., 2020; Chen et al., 2022; Ye et al., 2023) (Figure 7B). For instance, PDT is a non-invasive cancer treatment method that selectively treats malignant lesions through photodynamic responses. Under the irradiation of a specific wavelength of laser, a photosensitizer produces a large number of ROS at the tumor site to kill tumor cells and inhibit tumor growth. Furthermore, ROS prevents the chemical resistance caused by the scavenging of ROS by the thioredoxin system and increases the effect of chemotherapy. Xu et al. prepared multifunctional albumin NPs by co-carrying porphyrin IX and paclitaxel in albumin NPs to prevent chemoresistance and increase the effect of chemotherapy while performing PDT. Notably, these NPs improved the efficacy of chemotherapy and PDT and inhibited chemotherapy resistance (Xu et al., 2023).
In addition, the production of ROS in PDT intensifies local hypoxia in the hypoxic TME, offering the possibility of releasing hypoxia-activated prodrugs (HAPs). HAPs can be activated into highly cytotoxic substances under hypoxia conditions to selectively kill hypoxic cells in solid tumors and have low cytotoxicity to normal tissues. However, owing to the uneven distribution of hypoxic levels among tumors, the release efficiency of HAPs is usually limited by the degree of hypoxia, which leads to unsatisfactory therapeutic effects. The increased hypoxia of tumors caused by PDT can improve the efficiency of HAP release, and the combination of the two therapies can also effectively improve the treatment effect (Yuan et al., 2022; Xu et al., 2024). In addition, PDT can induce a systemic anti-tumor immune response through immunogenic cell death (ICD) to further strengthen the anti-tumor effect of PDT. However, this immune response is easily tolerated by tumor cells through a variety of molecular and cellular mechanisms, including the immune checkpoint pathway. Therefore, immune checkpoint inhibitors can simply overcome this deficiency, providing a scientific basis for the combination of immune checkpoint blockade therapy (ICBT) and PDT. Wang et al. constructed an albumin-based nanoplatform for co-delivery of IR780, NLG919 dimer, and a hypoxia-activated prodrug tirapazamine (TPZ) as the dual enhancer for synergistic cancer therapy. IR780 generates 1O2 under NIR irradiation for PDT and triggers the release of TPZ, activating its chemotherapy via exacerbated tumor hypoxia. The study also found that TPZ-mediated chemotherapy boosts PDT-induced tumor ICD, inducing stronger anti-tumor immunity. In addition, the enriched intratumoral glutathione triggered the activation of NLG919 to mitigate the immunosuppressive TME by specifically inhibiting indoleamine 2 and 3-dioxygenase 1 (IDO-1). This promoted the intratumoral infiltration of cytotoxic T lymphocytes and the suppression of primary and distant tumors, whereas the generated memory T cells suppressed tumor recurrence and metastasis by approximately 100%. In summary, the developed albumin multifunctional nanoplatform enabled dual enhanced photodynamic immunotherapy for breast cancer via hypoxia-activated chemotherapy (Wang et al., 2023).
Similarly, combination therapy may involve combining drug-loaded albumin NPs with another free drug (Chen et al., 2022; Schaal et al., 2022) (Figure 7C). The most commonly used albumin nanodrug in this combination modality is nab-paclitaxel, as it is the first and currently most widely used albumin nanodrug in oncology. This combination therapy is still a research hotspot, involving the study of the efficacy and safety of platinum, fluorouracil, immune checkpoint inhibitors, and other antineoplastic drugs combined with nap-paclitaxel in the treatment of various refractory tumors to provide a basis for clinical medication (Paz-Ares et al., 2018; Yin et al., 2020; Kase et al., 2023). Jiang et al. used torpalimab plus nab-paclitaxel for metastatic or recurrent TNBC in a randomized phase Ⅲ trial and found that this combination improved progression-free survival with an acceptable safety profile for PD-L1-positive patients with metastatic or recurrent TNBC (Jiang et al., 2024).
In summary, combination therapy is a super-additive approach, facilitating the mutual promotion and complementation of multiple treatment methods or drugs, resulting in greater efficacy than each individual treatment method/drug alone. This approach also helps prevent multidrug resistance, improves the body’s tolerance, and ultimately contributes to an improvement in therapeutic indices (Chou, 2010; Hatami et al., 2022).
4.2.6 Clinical applications
Albumin has been widely used as a carrier for drug delivery in oncological research. Abraxane® is a prime example of clinical success among all FDA-approved cancer nanomedicines (Esim and Hascicek, 2021; Meng et al., 2023). On 23 November 2021, the FDA approved Fyarro®, the second product developed and marketed using albumin-based technology, to treat adults with locally advanced unresectable or metastatic malignant PEComa. Furthermore, various chemotherapy drugs that use albumin NPs, such as albumin-bound docetaxel, SYHX 2011 (modified albumin-bound paclitaxel), and AR60 (Abraxane/Rituximab 160 nm NP), are currently undergoing clinical trials and have not yet been released on the market (Table 12). Notably, only two albumin-bound anti-tumor drugs have been launched since the introduction of Abraxane® in 2005 (generic versions of Abraxane® from different countries are not included). Most research on the use of albumin NPs in oncology is limited to animal experiments, with a low rate of clinical translation. Moreover, although albumin-based nanomedicines have shown greater efficacy than free drugs, they still encounter the same obstacles as traditional drugs, such as drug resistance and inadequate drug accumulation in tumors. Notably, owing to the EPR heterogeneity, albumin-based nanomedicines exhibit differences in treatment outcomes across various tumors, patients, or even different times in the same patient (Kunde and Wairkar, 2022a; Kong et al., 2023). Therefore, albumin–drug loading technology is still underdeveloped and requires significant investment in scientific research for further improvement.
In current clinical research on albumin NPs for anti-tumor purposes, breast, pancreatic, lung, and gastric cancers are the most widely investigated tumors (Table 9; Figure 3B). Breast cancer is a highly malignant tumor that poses a serious threat to women’s health. Notably, the number of patients with breast cancer worldwide is predicted to reach 3.04 million by 2040. Breast cancer is a highly heterogeneous disease, with different phenotypes showing varying sensitivities to drug treatment, resulting in different prognoses in patients in the same stage receiving the same treatment regimen. Genetic and molecular subtyping of breast cancer is closely associated with the prognosis. Therefore, new treatment options are needed to implement precision medicine and maximize treatment benefits for the various molecular subtypes (Alamdari et al., 2022; Kunde and Wairkar, 2022b). The ongoing clinical trials of albumin NPs for breast cancer in this field are listed in Table 13. The table indicates that current breast cancer clinical trials are focused on the therapeutic effects of nab-paclitaxel combined with new drugs with different mechanisms for treating breast cancer with different molecular types, such as the tyrosine kinase inhibitor pyrotinib and the anti-PD-L1 monoclonal antibody SHR-1316.
Pancreatic cancer is a highly aggressive malignant disease commonly referred to as the “king of cancer” owing to its rapid progression, poor treatment response, and short survival. The most common type of pancreatic cancer is pancreatic ductal adenocarcinoma (PDAC), accounting for 90% of all cases. It is predicted to be the second leading cause of cancer death in the next 20 years (Elsayed and Abdelrahim, 2021; Sung et al., 2021). Pancreatic cancer has a high KRAS mutation rate, with nearly 90% of cases being RAS-dependent. Research suggests that this mutation is a major driver of PDAC onset and progression (Spaargaren et al., 1995; Scheffzek et al., 1997). Furthermore, albumin and albumin-related drugs accumulate in KRAS-mutated pancreatic cancer cells and tissues. Therefore, nano-delivery systems based on serum albumin are widely used in pancreatic cancer treatment (Kratz, 2008). Abraxane® is currently the only albumin-bound drug approved to treat metastatic pancreatic cancer. However, new albumin-based nanomedicines for pancreatic cancer are also in development. Table 14 lists ongoing clinical trials of albumin NPs for pancreatic cancer in this field, which currently focus on the development of new drugs such as albumin-bound docetaxel and combination treatment regimens based on nab-paclitaxel.
Lung cancer is one of the most common cancers and the leading cause of death in males in >90 countries worldwide. Although multiple treatment options are available, satisfactory results in lung cancer treatment are difficult to achieve because of high proliferation rate, easy early metastasis, heterogeneity, and poor prognosis. Abraxane® was approved as a first-line treatment for non-small cell lung cancer in 2012. However, the use of nab-paclitaxel alone in the treatment of tumors has failed to elicit the desired outcomes (Ghosh et al., 2021). Therefore, the combination therapy based on nab-paclitaxel has been widely explored. Notably, clinical studies have focused on this combination therapy for various refractory or drug-resistant lung cancers to provide a basis for clinical drug use. Table 15 lists ongoing clinical trials of albumin NPs for lung cancer in this field.
Gastric cancer is a leading cause of cancer-related death worldwide, particularly in East Asia. Although many combination treatment regimens are available, the prognosis for patients with advanced gastric cancer remains unsatisfactory. Nab-paclitaxel is currently used to treat advanced gastric cancer, but it is reserved for second-line treatment. Furthermore, its use has only been approved in a few countries owing to the limited number of studies conducted (He et al., 2018). Moreover, the CSPC Pharmaceutical Group has developed a new drug called docetaxel for injection (albumin-bound), which was designated as an orphan drug by the FDA in 2022. This drug is indicated for gastric cancer, including gastroesophageal junction cancer. However, clinical trials for advanced gastric cancer in this field are still ongoing (Table 15). Current clinical research is primarily focused on developing new drugs, such as albumin-bound docetaxel, and studying the efficacy and safety of nab-paclitaxel-based monotherapy or combination therapy as a first- or second-line treatment for advanced gastric cancer. This research may provide more evidence for the clinical use of these treatments (Bando et al., 2018; Ju et al., 2019).
5 Limitations
The study has some limitations. First, the data was only retrieved from a single database, which may have resulted in the exclusion of articles published in other sources such as PubMed and Scopus. Second, our analysis only included articles published in English, which may have led to the exclusion of some articles. Third, new papers published after the search date were not included in the study because the database was kept open (Rousseau and Rousseau, 2021). Finally, although we have defined as many search terms as possible, some articles may have been missed.
6 Conclusion
In this study, we used bibliometric analysis to obtain an overview of the research on albumin NPs in the field of oncology. We demonstrated the trends in publications in this field since 2000, identified the most influential countries, institutions, authors, journals, and citations, and discussed the current research hotspots and frontiers in this field, including “rapid and convenient synthesis methods predominated by self-assembly,” “surface modification,” “construction of multifunctional NPs for theranostics,” “research on natural active ingredients mainly based on phenolic compounds,” “combination therapy,” and “clinical applications.” Furthermore, we discussed the challenges in the clinical application of albumin NPs in the field of oncology. However, we believe that significant continuous research efforts and investigations will facilitate the promising application of albumin NP drug-loading technology in the field of oncology in the future.
Data availability statement
The original contributions presented in the study are included in the article/Supplementary Material, further inquiries can be directed to the corresponding authors.
Author contributions
YeL: Conceptualization, Data curation, Formal analysis, Writing–review and editing. YiL: Writing–original draft, Methodology, Software. WS: Writing–review and editing, Validation. ML: Writing–review and editing, Investigation, Methodology. WW: Writing–review and editing, Data curation, Formal analysis. XJ: Project administration, Supervision, Writing–review and editing, Funding acquisition.
Funding
The author(s) declare that financial support was received for the research, authorship, and/or publication of this article. This research was financially supported by the Medical Research Project of Jiangsu Provincial Health Commission (No.: H2019096), Suqian Science and Technology Program (No.: KY202206), and Jiangsu Pharmaceutical Association Program (No.: A202344).
Conflict of interest
The authors declare that the research was conducted in the absence of any commercial or financial relationships that could be construed as a potential conflict of interest.
Publisher’s note
All claims expressed in this article are solely those of the authors and do not necessarily represent those of their affiliated organizations, or those of the publisher, the editors and the reviewers. Any product that may be evaluated in this article, or claim that may be made by its manufacturer, is not guaranteed or endorsed by the publisher.
Supplementary material
The Supplementary Material for this article can be found online at: https://www.frontiersin.org/articles/10.3389/fphar.2024.1409163/full#supplementary-material
Abbreviations
ALA, α-Lactalbumin; BSA, Bovine serum albumin; BLM, Bleomycin; CSA, Camel serum albumin; Ce6, Chlorine e6; EPR, Enhanced permeability and retention; FDA, Food and Drug Administration; FA, Folic acid; GBCA, Gadolinium-based contrast agents; HSA, Human serum albumin; HA, Hyaluronic acid; HAPs, Hypoxia activated prodrugs; ICG, Indocyanine green; ICD, Immunogenic cell death; ICBT, Immune checkpoint blockade therapy; IDO-1, Indoleamine 2, 3-dioxygenase 1; MRI, Magnetic resonance imaging; NIRFI, Near-infrared fluorescence imaging; NPs, Nanoparticles; PDAC, Pancreatic ductal adenocarcinoma; PDT, Photodynamic therapy; PEComa, Perivascular epithelioid cell tumor; PPy, Polypyrrole; PTT, Photothermal therapy; ROS, Reactive oxygen species; SPARC, Secreted protein acidic and rich in cysteine; TNBC, Triple-negative breast cancer; TME, Tumor microenvironment; WOSCC, Web of Science Core Collection.
References
Abolhassani, H., Safavi, M. S., Handali, S., Nosrati, M., and Shojaosadati, S. A. (2020). Synergistic effect of self-assembled curcumin and piperine Co-loaded human serum albumin nanoparticles on suppressing cancer cells. Drug Dev. Ind. Pharm. 46, 1647–1655. doi:10.1080/03639045.2020.1820032
Abolhassani, H., Zaer, M., Shojaosadati, S. A., and Hashemi-Najafabadi, S. (2023). Rapid generation of homogenous tumor spheroid microtissues in a scaffold-free platform for high-throughput screening of a novel combination nanomedicine. PLoS One 18, e0282064. doi:10.1371/journal.pone.0282064
Alamdari, S. G., Amini, M., Jalilzadeh, N., Baradaran, B., Mohammadzadeh, R., Mokhtarzadeh, A., et al. (2022). Recent advances in nanoparticle-based photothermal therapy for breast cancer. J. Control Release 349, 269–303. doi:10.1016/j.jconrel.2022.06.050
Aljabali, A. A. A., Bakshi, H. A., Hakkim, F. L., Haggag, Y. A., Al-Batanyeh, K. M., Al, Z. M. S., et al. (2020). Albumin nano-encapsulation of piceatannol enhances its anticancer potential in colon cancer via downregulation of nuclear p65 and HIF-1α. Cancers (Basel) 12, 113. doi:10.3390/cancers12010113
Al-Salih, M. Y. A., Pouresmaeil, V., Davoodi-Dehaghani, F., Haghighi, H. N., and Tabrizi, M. H. (2023). Study the anticancer properties of thymol-loaded PEGylated bovine serum albumin nanoparticles conjugated with folic acid. Chem. Biodivers. 20, e202301122. doi:10.1002/cbdv.202301122
Amiri, F., Mahmazi, S., and Danafar, H. (2020). Effect of biotin-targeted protein-based nanoparticles contain of curcumin on the expression of apoptotic index bax and Bcl2 proteins. J. Polym. Environ. 28, 2939–2946. doi:10.1007/s10924-020-01831-7
An, F. F., and Zhang, X. H. (2017). Strategies for preparing albumin-based nanoparticles for multifunctional bioimaging and drug delivery. Theranostics 7, 3667–3689. doi:10.7150/thno.19365
An, L., Yan, C., Mu, X., Tao, C., Tian, Q., Lin, J., et al. (2018). Paclitaxel-induced ultrasmall gallic acid-Fe@BSA self-assembly with enhanced MRI performance and tumor accumulation for cancer theranostics. ACS Appl. Mater Interfaces 10, 28483–28493. doi:10.1021/acsami.8b10625
Arpagaus, C., Collenberg, A., Rütti, D., Assadpour, E., and Jafari, S. M. (2018). Nano spray drying for encapsulation of pharmaceuticals. Int. J. Pharm. 546, 194–214. doi:10.1016/j.ijpharm.2018.05.037
Ashrafizadeh, M., Zarrabi, A., Hashemi, F., Moghadam, E. R., Hashemi, F., Entezari, M., et al. (2020). Curcumin in cancer therapy: a novel adjunct for combination chemotherapy with paclitaxel and alleviation of its adverse effects. Life Sci. 256, 117984. doi:10.1016/j.lfs.2020.117984
Ayatollahi, S., Karimi, E., and Tabrizi, M. H. (2022). Fabrication of bovine serum albumin coated ZnO nanoparticles loaded disogenin conjugated folate to improve the anti-cancer potential. J. Polym. Environ. 30, 5049–5056. doi:10.1007/s10924-022-02540-z
Bae, S., Ma, K., Kim, T. H., Lee, E. S., Oh, K. T., Park, E. S., et al. (2012). Doxorubicin-loaded human serum albumin nanoparticles surface-modified with TNF-related apoptosis-inducing ligand and transferrin for targeting multiple tumor types. Biomaterials 33, 1536–1546. doi:10.1016/j.biomaterials.2011.10.050
Bando, H., Shimodaira, H., Fujitani, K., Takashima, A., Yamaguchi, K., Nakayama, N., et al. (2018). A phase II study of nab-paclitaxel in combination with ramucirumab in patients with previously treated advanced gastric cancer. Eur. J. Cancer 91, 86–91. doi:10.1016/j.ejca.2017.11.032
Bardania, H., Jafari, F., Baneshi, M., Mahmoudi, R., Ardakani, M. T., Safari, F., et al. (2023). Folic acid-functionalized albumin/graphene oxide nanocomposite to simultaneously deliver curcumin and 5-fluorouracil into human colorectal cancer cells: an in vitro study. Biomed. Res. Int. 2023, 8334102. doi:10.1155/2023/8334102
Battogtokh, G., and Ko, Y. T. (2017). Mitochondrial-targeted photosensitizer-loaded folate-albumin nanoparticle for photodynamic therapy of cancer. Nanomedicine 13, 733–743. doi:10.1016/j.nano.2016.10.014
Bertrand, N., Wu, J., Xu, X., Kamaly, N., and Farokhzad, O. C. (2014). Cancer nanotechnology: the impact of passive and active targeting in the era of modern cancer biology. Adv. Drug Deliv. Rev. 66, 2–25. doi:10.1016/j.addr.2013.11.009
Bhushan, B., and Gopinath, P. (2015). Antioxidant nanozyme: a facile synthesis and evaluation of the reactive oxygen species scavenging potential of nanoceria encapsulated albumin nanoparticles. J. Mater Chem. B 3, 4843–4852. doi:10.1039/c5tb00572h
Cao, W., Jin, M., Zhou, W., Yang, K., Cheng, Y., Chen, J., et al. (2024). Forefronts and hotspots evolution of the nanomaterial application in anti-tumor immunotherapy: a scientometric analysis. J. Nanobiotechnology 22, 30. doi:10.1186/s12951-023-02278-3
Chavda, V. P., Patel, A. B., Mistry, K. J., Suthar, S. F., Wu, Z. X., Chen, Z. S., et al. (2022). Nano-drug delivery systems entrapping natural bioactive compounds for cancer: recent progress and future challenges. Front. Oncol. 12, 867655. doi:10.3389/fonc.2022.867655
Chen, X., Cheng, D., Ding, M., Yu, N., Liu, J., Li, J., et al. (2022). Tumor-targeting biomimetic sonosensitizer-conjugated iron oxide nanocatalysts for combinational chemodynamic-sonodynamic therapy of colorectal cancer. J. Mater Chem. B 10, 4595–4604. doi:10.1039/d2tb00872f
Chou, T. C. (2010). Drug combination studies and their synergy quantification using the Chou-Talalay method. Cancer Res. 70, 440–446. doi:10.1158/0008-5472.Can-09-1947
Commisso, C., Davidson, S. M., Soydaner-Azeloglu, R. G., Parker, S. J., Kamphorst, J. J., Hackett, S., et al. (2013). Macropinocytosis of protein is an amino acid supply route in Ras-transformed cells. Nature 497, 633–637. doi:10.1038/nature12138
De Jong, W. H., and Borm, P. J. (2008). Drug delivery and nanoparticles:applications and hazards. Int. J. Nanomedicine 3, 133–149. doi:10.2147/ijn.s596
Desale, J. P., Swami, R., Kushwah, V., Katiyar, S. S., and Jain, S. (2018). Chemosensitizer and docetaxel-loaded albumin nanoparticle: overcoming drug resistance and improving therapeutic efficacy. Nanomedicine (Lond) 13, 2759–2776. doi:10.2217/nnm-2018-0206
Diao, M., Liang, Y., Zhao, J., Zhao, C., Zhang, J., and Zhang, T. (2021). Enhanced cytotoxicity and antioxidant capacity of kaempferol complexed with α-lactalbumin. Food Chem. Toxicol. 153, 112265. doi:10.1016/j.fct.2021.112265
Ding, D., Tang, X., Cao, X., Wu, J., Yuan, A., Qiao, Q., et al. (2014). Novel self-assembly endows human serum albumin nanoparticles with an enhanced antitumor efficacy. AAPS PharmSciTech 15, 213–222. doi:10.1208/s12249-013-0041-3
Du, H., Yu, J., Guo, D., Yang, W., Wang, J., and Zhang, B. (2016). Improving the MR imaging sensitivity of upconversion nanoparticles by an internal and external incorporation of the Gd(3+) strategy for in vivo tumor-targeted imaging. Langmuir 32, 1155–1165. doi:10.1021/acs.langmuir.5b04186
E, A. A. C., B, H. K., A, B. U., B, Z. K., and C, G. O. I. A. B. (2022). Self-assembled albumin nanoparticles for redox responsive release of curcumin. J. Drug Deliv. Sci. Technol. 76, 103831. doi:10.1016/j.jddst.2022.103831
Elsadek, B., and Kratz, F. (2012). Impact of albumin on drug delivery--new applications on the horizon. J. Control Release 157, 4–28. doi:10.1016/j.jconrel.2011.09.069
Elsayed, M., and Abdelrahim, M. (2021). The latest advancement in pancreatic ductal adenocarcinoma therapy: a review article for the latest guidelines and novel therapies. Biomedicines 9, 389. doi:10.3390/biomedicines9040389
Elzoghby, A. O., Samy, W. M., and Elgindy, N. A. (2012). Albumin-based nanoparticles as potential controlled release drug delivery systems. J. Control Release 157, 168–182. doi:10.1016/j.jconrel.2011.07.031
Esim, O., and Hascicek, C. (2021). Albumin-based nanoparticles as promising drug delivery systems for cancer treatment. Curr. Pharm. Anal. 17, 346–359. doi:10.2174/1573412916999200421142008
Ferrado, J. B., Perez, A. A., Baravalle, M. E., Renna, M. S., Ortega, H. H., and Santiago, L. G. (2021). Genistein loaded in self-assembled bovine serum albumin nanovehicles and their effects on mouse mammary adenocarcinoma cells. Colloids Surf. B Biointerfaces 204, 111777. doi:10.1016/j.colsurfb.2021.111777
Ferrado, J. B., Perez, A. A., Menegon, M., Vaillard, V. A., Gasser, F., Baravalle, M. E., et al. (2023). PEGylation of genistein-loaded bovine serum albumin nanoparticles and its effect on in vitro cell viability and genotoxicity properties. Colloids Surf. B Biointerfaces 222, 113082. doi:10.1016/j.colsurfb.2022.113082
Ferrado, J. B., Perez, A. A., Ruiz, M. C., León, I. E., and Santiago, L. G. (2020). Chrysin-loaded bovine serum albumin particles as bioactive nanosupplements. Food Funct. 11, 6007–6019. doi:10.1039/d0fo00299b
Ferrado, J. B., Perez, A. A., Visentini, F. F., Islan, G. A., Castro, G. R., and Santiago, L. G. (2019). Formation and characterization of self-assembled bovine serum albumin nanoparticles as chrysin delivery systems. Colloids Surf. B Biointerfaces 173, 43–51. doi:10.1016/j.colsurfb.2018.09.046
Firouzabadi, K., Karimi, E., and Tabrizi, M. H. (2023). Fabrication of bovine serum albumin-polyethylene glycol nanoparticle conjugated-folic acid loaded-naringenin as an efficient carrier biomacromolecule for suppression of cancer cells. Biotechnol. Appl. Biochem. 70, 790–797. doi:10.1002/bab.2399
Gao, G., Zhou, W., Jiang, X., and Ma, J. (2024). Bovine serum albumin and folic acid-modified aurum nanoparticles loaded with paclitaxel and curcumin enhance radiotherapy sensitization for esophageal cancer. Int. J. Radiat. Biol. 100, 411–419. doi:10.1080/09553002.2023.2281524
Geng, T., Zhao, X., Ma, M., Zhu, G., and Yin, L. (2017). Resveratrol-loaded albumin nanoparticles with prolonged blood circulation and improved biocompatibility for highly effective targeted pancreatic tumor therapy. Nanoscale Res. Lett. 12, 437. doi:10.1186/s11671-017-2206-6
Ghosh, S., Javia, A., Shetty, S., Bardoliwala, D., Maiti, K., Banerjee, S., et al. (2021). Triple negative breast cancer and non-small cell lung cancer: clinical challenges and nano-formulation approaches. J. Control Release 337, 27–58. doi:10.1016/j.jconrel.2021.07.014
Gradishar, W. J., Tjulandin, S., Davidson, N., Shaw, H., Desai, N., Bhar, P., et al. (2005). Phase III trial of nanoparticle albumin-bound paclitaxel compared with polyethylated castor oil-based paclitaxel in women with breast cancer. J. Clin. Oncol. 23, 7794–7803. doi:10.1200/jco.2005.04.937
Gu, C., Wang, Z., Pan, Y., Zhu, S., and Gu, Z. (2023). Tungsten-based nanomaterials in the biomedical field: a bibliometric analysis of research progress and prospects. Adv. Mater 35, e2204397. doi:10.1002/adma.202204397
Guo, Y., Sun, Q., Wu, F. G., Dai, Y., and Chen, X. (2021). Polyphenol-containing nanoparticles: synthesis, properties, and therapeutic delivery. Adv. Mater 33, e2007356. doi:10.1002/adma.202007356
Hao, T., Chen, Q., Qi, Y., Sun, P., Chen, D., Jiang, W., et al. (2019). Biomineralized Gd(2) O(3) @HSA nanoparticles as a versatile platform for dual-modal imaging and chemo-phototherapy-synergized tumor ablation. Adv. Healthc. Mater 8, e1901005. doi:10.1002/adhm.201901005
Hasanpoor, Z., Mostafaie, A., Nikokar, I., and Hassan, Z. M. (2020). Curcumin-human serum albumin nanoparticles decorated with PDL1 binding peptide for targeting PDL1-expressing breast cancer cells. Int. J. Biol. Macromol. 159, 137–153. doi:10.1016/j.ijbiomac.2020.04.130
Hatami, E., Nagesh, P. K. B., Chauhan, N., Jaggi, M., Chauhan, S. C., and Yallapu, M. M. (2022). In situ nanoparticle self-assembly for combination delivery of therapeutics to non-small cell lung cancer. ACS Appl. Bio Mater 5, 1104–1119. doi:10.1021/acsabm.1c01158
He, M. M., Wang, F., Jin, Y., Yuan, S. Q., Ren, C., Luo, H. Y., et al. (2018). Phase II clinical trial of S-1 plus nanoparticle albumin-bound paclitaxel in untreated patients with metastatic gastric cancer. Cancer Sci. 109, 3575–3582. doi:10.1111/cas.13813
Hong, S., Choi, D. W., Kim, H. N., Park, C. G., Lee, W., and Park, H. H. (2020). Protein-based nanoparticles as drug delivery systems. Pharmaceutics 12, 604. doi:10.3390/pharmaceutics12070604
Hoogenboezem, E. N., and Duvall, C. L. (2018). Harnessing albumin as a carrier for cancer therapies. Adv. Drug Deliv. Rev. 130, 73–89. doi:10.1016/j.addr.2018.07.011
Huang, Y., Deng, S., Luo, X., Liu, Y., Xu, W., Pan, J., et al. (2019). Evaluation of intestinal absorption mechanism and pharmacokinetics of curcumin-loaded galactosylated albumin nanoparticles. Int. J. Nanomedicine 14, 9721–9730. doi:10.2147/ijn.S229992
Ibrahim, N. K., Samuels, B., Page, R., Doval, D., Patel, K. M., Rao, S. C., et al. (2005). Multicenter phase II trial of ABI-007, an albumin-bound paclitaxel, in women with metastatic breast cancer. J. Clin. Oncol. 23, 6019–6026. doi:10.1200/jco.2005.11.013
Jahanban-Esfahlan, A., Dastmalchi, S., and Davaran, S. (2016). A simple improved desolvation method for the rapid preparation of albumin nanoparticles. Int. J. Biol. Macromol. 91, 703–709. doi:10.1016/j.ijbiomac.2016.05.032
Jiang, Z., Ouyang, Q., Sun, T., Zhang, Q., Teng, Y., Cui, J., et al. (2024). Toripalimab plus nab-paclitaxel in metastatic or recurrent triple-negative breast cancer: a randomized phase 3 trial. Nat. Med. 30, 249–256. doi:10.1038/s41591-023-02677-x
Ju, C., Wen, Y., Zhang, L., Wang, Q., Xue, L., Shen, J., et al. (2019). Neoadjuvant chemotherapy based on abraxane/human neutrophils cytopharmaceuticals with radiotherapy for gastric cancer. Small 15, e1804191. doi:10.1002/smll.201804191
Kamisawa, T., Wood, L. D., Itoi, T., and Takaori, K. (2016). Pancreatic cancer. Lancet 388, 73–85. doi:10.1016/s0140-6736(16)00141-0
Kase, A. M., Azzouqa, A. G., Kochuveettil, S., and Colon-Otero, G. (2023). Efficacy of gemcitabine in combination with nanoparticle albumin-bound paclitaxel in the treatment of recurrent ovarian cancer: a retrospective single institution review. Cancer Med. 12, 9434–9438. doi:10.1002/cam4.5705
Khella, K. F., Abd El Maksoud, A. I., Hassan, A., Abdel-Ghany, S. E., Elsanhoty, R. M., Aladhadh, M. A., et al. (2022). Carnosic acid encapsulated in albumin nanoparticles induces apoptosis in breast and colorectal cancer cells. Molecules 27, 4102. doi:10.3390/molecules27134102
Kim, B., Seo, B., Park, S., Lee, C., Kim, J. O., Oh, K. T., et al. (2017). Albumin nanoparticles with synergistic antitumor efficacy against metastatic lung cancers. Colloids Surf. B Biointerfaces 158, 157–166. doi:10.1016/j.colsurfb.2017.06.039
Kim, S. S., Kim, H. K., Kim, H., Lee, W. T., Lee, E. S., Oh, K. T., et al. (2021). Hyperthermal paclitaxel-bound albumin nanoparticles co-loaded with indocyanine green and hyaluronidase for treating pancreatic cancers. Arch. Pharm. Res. 44, 182–193. doi:10.1007/s12272-020-01264-9
Kong, X., Qi, Y., Wang, X., Jiang, R., Wang, J., Fang, Y., et al. (2023). Nanoparticle drug delivery systems and their applications as targeted therapies for triple negative breast cancer. Prog. Mater. Sci. 134, 101070. doi:10.1016/j.pmatsci.2023.101070
Kouchakzadeh, H., Safavi, M. S., and Shojaosadati, S. A. (2015). Efficient delivery of therapeutic agents by using targeted albumin nanoparticles. Adv. Protein Chem. Struct. Biol. 98, 121–143. doi:10.1016/bs.apcsb.2014.11.002
Kratz, F. (2008). Albumin as a drug carrier: design of prodrugs, drug conjugates and nanoparticles. J. Control Release 132, 171–183. doi:10.1016/j.jconrel.2008.05.010
Kumari, P., Paul, M., Bobde, Y., Soniya, K., Kiran Rompicharla, S. V., Ghosh, B., et al. (2020). Albumin-based lipoprotein nanoparticles for improved delivery and anticancer activity of curcumin for cancer treatment. Nanomedicine (Lond) 15, 2851–2869. doi:10.2217/nnm-2020-0232
Kunde, S. S., and Wairkar, S. (2022a). Targeted delivery of albumin nanoparticles for breast cancer: a review. Colloids Surfaces B Biointerfaces 213, 112422. doi:10.1016/j.colsurfb.2022.112422
Kunde, S. S., and Wairkar, S. (2022b). Targeted delivery of albumin nanoparticles for breast cancer: a review. Colloids Surf. B Biointerfaces 213, 112422. doi:10.1016/j.colsurfb.2022.112422
Kunjiappan, S., Govindaraj, S., Parasuraman, P., Sankaranarayanan, M., Arunachalam, S., Palanisamy, P., et al. (2020). Design, in silico modelling and functionality theory of folate-receptor-targeted myricetin-loaded bovine serum albumin nanoparticle formulation for cancer treatment. Nanotechnology 31, 155102. doi:10.1088/1361-6528/ab5c56
Lai, X., Yao, F., An, Y., Li, X., and Yang, X. D. (2023). Novel nanotherapeutics for cancer immunotherapy by PD-L1-aptamer-functionalized and fexofenadine-loaded albumin nanoparticles. Molecules 28, 2556. doi:10.3390/molecules28062556
Lee, S. H., Heng, D., Ng, W. K., Chan, H. K., and Tan, R. B. (2011). Nano spray drying: a novel method for preparing protein nanoparticles for protein therapy. Int. J. Pharm. 403, 192–200. doi:10.1016/j.ijpharm.2010.10.012
Lei, C., Liu, X. R., Chen, Q. B., Li, Y., Zhou, J. L., Zhou, L. Y., et al. (2021). Hyaluronic acid and albumin based nanoparticles for drug delivery. J. Control Release 331, 416–433. doi:10.1016/j.jconrel.2021.01.033
Leitão, M. M., de Melo-Diogo, D., Alves, C. G., Lima-Sousa, R., and Correia, I. J. (2020). Prototypic heptamethine cyanine incorporating nanomaterials for cancer phototheragnostic. Adv. Healthc. Mater 9, e1901665. doi:10.1002/adhm.201901665
Li, C., Wang, X., Song, H., Deng, S., Li, W., Li, J., et al. (2020). Current multifunctional albumin-based nanoplatforms for cancer multi-mode therapy. Asian J. Pharm. Sci. 15, 1–12. doi:10.1016/j.ajps.2018.12.006
Lian, B., Wu, M., Feng, Z., Deng, Y., Zhong, C., and Zhao, X. (2019). Folate-conjugated human serum albumin-encapsulated resveratrol nanoparticles: preparation, characterization, bioavailability and targeting of liver tumors. Artif. Cells Nanomed Biotechnol. 47, 154–165. doi:10.1080/21691401.2018.1548468
Lian, H., Wu, J., Hu, Y., and Guo, H. (2017). Self-assembled albumin nanoparticles for combination therapy in prostate cancer. Int. J. Nanomedicine 12, 7777–7787. doi:10.2147/ijn.S144634
Lin, T., Zhao, P., Jiang, Y., Tang, Y., Jin, H., Pan, Z., et al. (2016). Blood-brain-barrier-penetrating albumin nanoparticles for biomimetic drug delivery via albumin-binding protein pathways for antiglioma therapy. ACS Nano 10, 9999–10012. doi:10.1021/acsnano.6b04268
Ling, L. X., Ouyang, Y., and Hu, Y. (2023). Research trends on nanomaterials in gastric cancer: a bibliometric analysis from 2004 to 2023. J. Nanobiotechnology 21, 248. doi:10.1186/s12951-023-02033-8
Liu, G., Wen, Z., Liu, F., Xu, Y., Li, H., and Sun, S. (2023a). Multisubcellular organelle-targeting nanoparticle for synergistic chemotherapy and photodynamic/photothermal tumor therapy. Nanomedicine (Lond) 18, 613–631. doi:10.2217/nnm-2023-0021
Liu, R., Xiao, W., Hu, C., Xie, R., and Gao, H. (2018). Theranostic size-reducible and no donor conjugated gold nanocluster fabricated hyaluronic acid nanoparticle with optimal size for combinational treatment of breast cancer and lung metastasis. J. Control Release 278, 127–139. doi:10.1016/j.jconrel.2018.04.005
Liu, W. L., Zhang, Y. Q., Luo, X. J., Zhu, Y. Y., Song, L., Ming, Z. H., et al. (2023b). Novel dual-mode NIR-II/MRI nanoprobe targeting PD-L1 accurately evaluates the efficacy of immunotherapy for triple-negative breast cancer. Int. J. Nanomedicine 18, 5141–5157. doi:10.2147/ijn.S417944
Livney, Y. D. (2010). Milk proteins as vehicles for bioactives. Curr. Opin. Colloid and Interface Sci. 15, 73–83. doi:10.1016/j.cocis.2009.11.002
Long, Q., Zhu, W., Guo, L., and Pu, L. (2020). RGD-conjugated resveratrol HSA nanoparticles as a novel delivery system in ovarian cancer therapy. Drug Des. Devel Ther. 14, 5747–5756. doi:10.2147/dddt.S248950
Loureiro, A., Azoia, N. G., Gomes, A. C., and Cavaco-Paulo, A. (2016). Albumin-based nanodevices as drug carriers. Curr. Pharm. Des. 22, 1371–1390. doi:10.2174/1381612822666160125114900
Ma, T., Jiang, J. L., Qi, W. X., Chen, J. Y., and Xu, H. P. (2022). A novel delivery system of RGD-HSA loaded GEM/CUR nanoparticles for the treatment of pancreatic cancer therapy. Drug Des. Devel Ther. 16, 2395–2406. doi:10.2147/dddt.S366558
Malam, Y., Loizidou, M., and Seifalian, A. M. (2009). Liposomes and nanoparticles: nanosized vehicles for drug delivery in cancer. Trends Pharmacol. Sci. 30, 592–599. doi:10.1016/j.tips.2009.08.004
Martínez-López, A. L., Pangua, C., Reboredo, C., Campión, R., Morales-Gracia, J., and Irache, J. M. (2020). Protein-based nanoparticles for drug delivery purposes. Int. J. Pharm. 581, 119289. doi:10.1016/j.ijpharm.2020.119289
Matloubi, Z., and Hassan, Z. (2020). HSA-curcumin nanoparticles: a promising substitution for Curcumin as a Cancer chemoprevention and therapy. Daru 28, 209–219. doi:10.1007/s40199-020-00331-2
Meng, R., Zhu, H., Deng, P., Li, M., Ji, Q., He, H., et al. (2023). Research progress on albumin-based hydrogels: properties, preparation methods, types and its application for antitumor-drug delivery and tissue engineering. Front. Bioeng. Biotechnol. 11, 1137145. doi:10.3389/fbioe.2023.1137145
Mitchell, M. J., Billingsley, M. M., Haley, R. M., Wechsler, M. E., Peppas, N. A., and Langer, R. (2021). Engineering precision nanoparticles for drug delivery. Nat. Rev. Drug Discov. 20, 101–124. doi:10.1038/s41573-020-0090-8
Mohammad-Beigi, H., Shojaosadati, S. A., Morshedi, D., Arpanaei, A., and Marvian, A. T. (2015). Preparation and in vitro characterization of gallic acid-loaded human serum albumin nanoparticles. J. Nanoparticle Res. 17, 167–216. doi:10.1007/s11051-015-2978-5
Noori, T., Kashanian, S., Rafipour, R., Mansouri, K., and Nazari, M. (2021). Dual-targeted drug delivery system based on dopamine functionalized human serum albumin nanoparticles as a carrier for methyltestosterone drug. Nanomedicine J. 8, 147–155. doi:10.22038/NMJ.2021.08.008
Nosrati, H., Abhari, F., Charmi, J., Davaran, S., and Danafar, H. (2019). Multifunctional nanoparticles from albumin for stimuli-responsive efficient dual drug delivery. Bioorg Chem. 88, 102959. doi:10.1016/j.bioorg.2019.102959
Patra, J. K., Das, G., Fraceto, L. F., Campos, E. V. R., Rodriguez-Torres, M. D. P., Acosta-Torres, L. S., et al. (2018). Nano based drug delivery systems: recent developments and future prospects. J. Nanobiotechnology 16, 71. doi:10.1186/s12951-018-0392-8
Paz-Ares, L., Luft, A., Vicente, D., Tafreshi, A., Gümüş, M., Mazières, J., et al. (2018). Pembrolizumab plus chemotherapy for squamous non-small-cell lung cancer. N. Engl. J. Med. 379, 2040–2051. doi:10.1056/NEJMoa1810865
Pei, Z., Chen, S., Ding, L., Liu, J., Cui, X., Li, F., et al. (2022). Current perspectives and trend of nanomedicine in cancer: a review and bibliometric analysis. J. Control Release 352, 211–241. doi:10.1016/j.jconrel.2022.10.023
Pham, P. T. T., Le, X. T., Kim, H., Kim, H. K., Lee, E. S., Oh, K. T., et al. (2020). Indocyanine green and curcumin Co-loaded nano-fireball-like albumin nanoparticles based on near-infrared-induced hyperthermia for tumor ablation. Int. J. Nanomedicine 15, 6469–6484. doi:10.2147/ijn.S262690
Qi, J., Yao, P., He, F., Yu, C., and Huang, C. (2010). Nanoparticles with dextran/chitosan shell and BSA/chitosan core--doxorubicin loading and delivery. Int. J. Pharm. 393, 176–184. doi:10.1016/j.ijpharm.2010.03.063
Qin, Y., Xu, L., Guan, Y. Y., and Liu, H. (2020). Multifunctionalized protein-based drug delivery system for inhibition of tumor growth and progression. ACS Appl. Bio Mater 3, 3196–3202. doi:10.1021/acsabm.0c00190
Rousseau, S., and Rousseau, R. (2021). Bibliometric techniques and their use in business and economics research. J. Econ. Surv. 35, 1428–1451. doi:10.1111/joes.12415
Safavi, M. S., Shojaosadati, S. A., Yang, H. G., Kim, Y., Park, E. J., Lee, K. C., et al. (2017). Reducing agent-free synthesis of curcumin-loaded albumin nanoparticles by self-assembly at room temperature. Int. J. Pharm. 529, 303–309. doi:10.1016/j.ijpharm.2017.06.087
Sahu, A., Lee, J. H., Lee, H. G., Jeong, Y. Y., and Tae, G. (2016). Prussian blue/serum albumin/indocyanine green as a multifunctional nanotheranostic agent for bimodal imaging guided laser mediated combinatorial phototherapy. J. Control Release 236, 90–99. doi:10.1016/j.jconrel.2016.06.031
Saleh, T., Soudi, T., and Shojaosadati, S. A. (2019). Aptamer functionalized curcumin-loaded human serum albumin (HSA) nanoparticles for targeted delivery to HER-2 positive breast cancer cells. Int. J. Biol. Macromol. 130, 109–116. doi:10.1016/j.ijbiomac.2019.02.129
Salehiabar, M., Nosrati, H., Javani, E., Aliakbarzadeh, F., Kheiri Manjili, H., Davaran, S., et al. (2018). Production of biological nanoparticles from bovine serum albumin as controlled release carrier for curcumin delivery. Int. J. Biol. Macromol. 115, 83–89. doi:10.1016/j.ijbiomac.2018.04.043
Schaal, J. L., Bhattacharyya, J., Brownstein, J., Strickland, K. C., Kelly, G., Saha, S., et al. (2022). Brachytherapy via a depot of biopolymer-bound (131)I synergizes with nanoparticle paclitaxel in therapy-resistant pancreatic tumours. Nat. Biomed. Eng. 6, 1148–1166. doi:10.1038/s41551-022-00949-4
Scheffzek, K., Ahmadian, M. R., Kabsch, W., Wiesmüller, L., Lautwein, A., Schmitz, F., et al. (1997). The Ras-RasGAP complex: structural basis for GTPase activation and its loss in oncogenic Ras mutants. Science 277, 333–338. doi:10.1126/science.277.5324.333
Schmid, P., Adams, S., Rugo, H. S., Schneeweiss, A., Barrios, C. H., Iwata, H., et al. (2018). Atezolizumab and nab-paclitaxel in advanced triple-negative breast cancer. N. Engl. J. Med. 379, 2108–2121. doi:10.1056/NEJMoa1809615
Shi, J., Kantoff, P. W., Wooster, R., and Farokhzad, O. C. (2017). Cancer nanomedicine: progress, challenges and opportunities. Nat. Rev. Cancer 17, 20–37. doi:10.1038/nrc.2016.108
Siddique, S., and Chow, J. C. L. (2022). Recent advances in functionalized nanoparticles in cancer theranostics. Nanomater. (Basel) 12, 2826. doi:10.3390/nano12162826
Socinski, M. A., Bondarenko, I., Karaseva, N. A., Makhson, A. M., Vynnychenko, I., Okamoto, I., et al. (2012). Weekly nab-paclitaxel in combination with carboplatin versus solvent-based paclitaxel plus carboplatin as first-line therapy in patients with advanced non-small-cell lung cancer: final results of a phase III trial. J. Clin. Oncol. 30, 2055–2062. doi:10.1200/jco.2011.39.5848
Song, X., Liang, C., Gong, H., Chen, Q., Wang, C., and Liu, Z. (2015). Photosensitizer-conjugated albumin-polypyrrole nanoparticles for imaging-guided in vivo photodynamic/photothermal therapy. Small 11, 3932–3941. doi:10.1002/smll.201500550
Spaargaren, M., Bischoff, J. R., and McCormick, F. (1995). Signal transduction by Ras-like GTPases: a potential target for anticancer drugs. Gene Expr. 4, 345–356. doi:10.1007/BF02818528
Spada, A., Emami, J., Tuszynski, J. A., and Lavasanifar, A. (2021). The uniqueness of albumin as a carrier in nanodrug delivery. Mol. Pharm. 18, 1862–1894. doi:10.1021/acs.molpharmaceut.1c00046
Stecanella, L. A., Bitencourt, A. P. R., Vaz, G. R., Quarta, E., Silva Júnior, J. O. C., and Rossi, A. (2021). Glycyrrhizic acid and its hydrolyzed metabolite 18β-glycyrrhetinic acid as specific ligands for targeting nanosystems in the treatment of liver cancer. Pharmaceutics 13, 1792. doi:10.3390/pharmaceutics13111792
Subhan, M. A., Parveen, F., Filipczak, N., Yalamarty, S. S. K., and Torchilin, V. P. (2023). Approaches to improve EPR-based drug delivery for cancer therapy and diagnosis. J. Pers. Med. 13, 389. doi:10.3390/jpm13030389
Sung, H., Ferlay, J., Siegel, R. L., Laversanne, M., Soerjomataram, I., Jemal, A., et al. (2021). Global cancer statistics 2020: GLOBOCAN estimates of incidence and mortality worldwide for 36 cancers in 185 countries. CA Cancer J. Clin. 71, 209–249. doi:10.3322/caac.21660
Tao, H. Y., Wang, R. Q., Sheng, W. J., and Zhen, Y. S. (2021). The development of human serum albumin-based drugs and relevant fusion proteins for cancer therapy. Int. J. Biol. Macromol. 187, 24–34. doi:10.1016/j.ijbiomac.2021.07.080
Voltolini, S., Spigno, F., Cioè, A., Cagnati, P., Bignardi, D., and Minale, P. (2013). Bovine Serum Albumin: a double allergy risk. Eur. Ann. Allergy Clin. Immunol. 45, 144–147.
Von Hoff, D. D., Ervin, T., Arena, F. P., Chiorean, E. G., Infante, J., Moore, M., et al. (2013). Increased survival in pancreatic cancer with nab-paclitaxel plus gemcitabine. N. Engl. J. Med. 369, 1691–1703. doi:10.1056/NEJMoa1304369
Wagner, A. J., Ravi, V., Riedel, R. F., Ganjoo, K., Van Tine, B. A., Chugh, R., et al. (2021). Nab-sirolimus for patients with malignant perivascular epithelioid cell tumors. J. Clin. Oncol. 39, 3660–3670. doi:10.1200/jco.21.01728
Wan, X., Zheng, X., Pang, X., Zhang, Z., Jing, T., Xu, W., et al. (2015). The potential use of lapatinib-loaded human serum albumin nanoparticles in the treatment of triple-negative breast cancer. Int. J. Pharm. 484, 16–28. doi:10.1016/j.ijpharm.2015.02.037
Wang, M., He, M., Zhang, M., Xue, S., Xu, T., Zhao, Y., et al. (2023). Controllable hypoxia-activated chemotherapy as a dual enhancer for synergistic cancer photodynamic immunotherapy. Biomaterials 301, 122257. doi:10.1016/j.biomaterials.2023.122257
Wang, X., Fan, Y., Yan, J., and Yang, M. (2022). Engineering polyphenol-based polymeric nanoparticles for drug delivery and bioimaging. Chem. Eng. J. 439, 135661. doi:10.1016/j.cej.2022.135661
Wang, X., and Zhang, W. (2022). The Janus of Protein Corona on nanoparticles for tumor targeting, immunotherapy and diagnosis. J. Control Release 345, 832–850. doi:10.1016/j.jconrel.2022.03.056
Wu, J., Williams, G. R., Niu, S., Yang, Y., Li, Y., Zhang, X., et al. (2020). Biomineralized bimetallic oxide nanotheranostics for multimodal imaging-guided combination therapy. Theranostics 10, 841–855. doi:10.7150/thno.40715
Xie, Q., Li, S., Feng, X., Shi, J., Li, Y., Yuan, G., et al. (2022). All-in-one approaches for triple-negative breast cancer therapy: metal-phenolic nanoplatform for MR imaging-guided combinational therapy. J. Nanobiotechnology 20, 226. doi:10.1186/s12951-022-01416-7
Xu, Q., Zhou, Y., Zhang, H., Li, H., Qin, H., and Wang, H. (2023a). Bibliometric analysis of hotspots and frontiers of immunotherapy in pancreatic cancer. Healthc. (Basel) 11, 304. doi:10.3390/healthcare11030304
Xu, X., Wang, C., Guan, W., Wang, F., Li, X., Yuan, J., et al. (2023b). Protoporphyrin IX-loaded albumin nanoparticles reverse cancer chemoresistance by enhancing intracellular reactive oxygen species. Nanomedicine 51, 102688. doi:10.1016/j.nano.2023.102688
Xu, Y., Lv, J., Kong, C., Liu, Y., Wang, K., Tang, Z., et al. (2024). Introducing urea into tirapazamine derivatives to enhance anticancer therapy. Natl. Sci. Rev. 11, nwae038. doi:10.1093/nsr/nwae038
Yang, M., Li, J., Gu, P., and Fan, X. (2021). The application of nanoparticles in cancer immunotherapy: targeting tumor microenvironment. Bioact. Mater 6, 1973–1987. doi:10.1016/j.bioactmat.2020.12.010
Ye, M., Li, B., Shi, W., Liu, H., Wang, Y., Chen, W., et al. (2023). Preparation and tumor-targeting evaluation of BS-CyP albumin nanoparticles modified with hyaluronic acid based on boron neutron capture therapy. J. Biomed. Mater Res. A 111, 1176–1184. doi:10.1002/jbm.a.37506
Yin, Y., Li, W., Zha, X., and Wang, J. (2020). 105P Lower-dose apatinib combined with nanoparticle albumin-bound paclitaxel and carboplatin as a neoadjuvant regimen for triple negative breast cancer: a prospective, single-arm, phase II study. Ann. Oncol. 31, S52. doi:10.1016/j.annonc.2020.03.044
Yu, X., Xieripu, A., Xu, Q., Zulipikaer, A., Song, Y., Cai, L., et al. (2019). GSH-responsive curcumin/doxorubicin encapsulated Bactrian camel serum albumin nanocomposites with synergistic effect against lung cancer cells. J. Biomed. Res. 34, 54–66. doi:10.7555/jbr.33.20190036
Yuan, J., Zhou, Q. H., Xu, S., Zuo, Q. P., Li, W., Zhang, X. X., et al. (2022). Enhancing the release efficiency of a molecular chemotherapeutic prodrug by photodynamic therapy. Angew. Chem. Int. Ed. Engl. 61, e202206169. doi:10.1002/anie.202206169
Zhang, Y. N., Poon, W., Tavares, A. J., McGilvray, I. D., and Chan, W. C. W. (2016). Nanoparticle-liver interactions: cellular uptake and hepatobiliary elimination. J. Control Release 240, 332–348. doi:10.1016/j.jconrel.2016.01.020
Zhao, Y., Cai, C., Liu, M., Zhao, Y., Wu, Y., Fan, Z., et al. (2020). Drug-binding albumins forming stabilized nanoparticles for co-delivery of paclitaxel and resveratrol: in vitro/in vivo evaluation and binding properties investigation. Int. J. Biol. Macromol. 153, 873–882. doi:10.1016/j.ijbiomac.2020.03.060
Zheng, S., Zhang, X., Pang, Z., Liu, J., Liu, S., and Sheng, R. (2023). Anti-pan-rspo chimeric protein-conjugated albumin nanoparticle provides promising opportunities in cancer targeted therapy. Adv. Healthc. Mater 12, e2301441. doi:10.1002/adhm.202301441
Zhiguo, F., Ji, W., Shenyuan, C., Guoyou, Z., Chen, K., Hui, Q., et al. (2023). A swift expanding trend of extracellular vesicles in spinal cord injury research: a bibliometric analysis. J. Nanobiotechnology 21, 289. doi:10.1186/s12951-023-02051-6
Zhong, L., Chen, B., He, J., He, M., Zhao, Q., Wang, B., et al. (2021). Focused ultrasound-augmented cancer phototheranostics using albumin-indocyanine green nanoparticles. Ultrasound Med. Biol. 47, 1801–1813. doi:10.1016/j.ultrasmedbio.2021.02.026
Keywords: albumin nanoparticles, anti-tumor, bibliometric research, hotspots, clinical applications
Citation: Liu Y, Li Y, Shen W, Li M, Wang W and Jin X (2024) Trend of albumin nanoparticles in oncology: a bibliometric analysis of research progress and prospects. Front. Pharmacol. 15:1409163. doi: 10.3389/fphar.2024.1409163
Received: 29 March 2024; Accepted: 12 June 2024;
Published: 12 July 2024.
Edited by:
P. S. Rajinikanth, Babasaheb Bhimrao Ambedkar University, IndiaReviewed by:
Priya Singh, Gandhi Institute of Technology and Management (GITAM), IndiaHuali Chen, Chongqing Medical University, China
Copyright © 2024 Liu, Li, Shen, Li, Wang and Jin. This is an open-access article distributed under the terms of the Creative Commons Attribution License (CC BY). The use, distribution or reproduction in other forums is permitted, provided the original author(s) and the copyright owner(s) are credited and that the original publication in this journal is cited, in accordance with accepted academic practice. No use, distribution or reproduction is permitted which does not comply with these terms.
*Correspondence: Wen Wang, OTgxNzY5ODUwQHFxLmNvbQ==; Xin Jin, amlueGluODcxMjExQDE2My5jb20=