- 1Department of Hematology, The First Hospital of Jilin University, Changchun, China
- 2Department of Obstetrics, The Affiliated Taian City Central Hospital of Qingdao University, Taian, China
As an increasingly well-known derivative of coumarin, daphnetin (7,8-dithydroxycoumarin) has demonstrated various pharmacological activities, including anti-inflammation, anti-cancer, anti-autoimmune diseases, antibacterial, organ protection, and neuroprotection properties. Various studies have been conducted to explore the action mechanisms and synthetic methods of daphnetin, given its therapeutic potential in clinical. Despite these initial insights, the precise mechanisms underlying the pharmacological activities of daphnetin remain largely unknown. In order to address this knowledge gap, we explore the molecular effects from the perspectives of signaling pathways, NOD-like receptor protein 3 (NLRP3) inflammasome and inflammatory factors; and try to find out how these mechanisms can be utilized to inform new combined therapeutic strategies.
1 Introduction
Herbal medicines have been used for thousands of years. Even in recent years, not only in developing countries but also in developed countries, including Europe and North America, it is estimated that more than 50% of the population has used herbal medicinal approaches at least once (Bhoi et al., 2023). Furthermore, herbal medicine has captivated the attention of scientists to probe bioactive compounds derived from natural sources for future drug discovery.
Among plant active metabolites, coumarins and their derivatives are prominent paradigms and have been used widely. Coumarin, first isolated from tonka beans and melilot flowers by Vogel in 1820, is considered the most basic heterocyclic compound with fused phenolic benzene and α-pyrone rings (Ando et al., 2018). As secondary metabolites, coumarins have been found in bacteria, fungi, and about 150 species of plants, where more than 1,300 natural-based coumarins are isolated and identified (Iranshahi et al., 2009). Based on their chemical structure, coumarins are classified into six main types: simple coumarins, furanocoumarins, dihydrofuranocoumarins, phenylcoumarins, pyranocoumarins, and biscoumarins (Hassanein et al., 2020). All six types comprise a coumarin moiety and exert diverse medical functions via their distinct structural characteristics. Therefore, coumarins have been widely used in complementary and alternative medicine owing to their potent and comprehensive pharmacological activities, including anti-inflammatory (Min et al., 2023), antibacterial (Zeng et al., 2023), antiviral (Hwu et al., 2022), antioxidant (Sultana et al., 2022), anti-Alzheimer’s Disease (AD) (Liu W. et al., 2022), and antitumor effects (Ahmed et al., 2022). Based on this, a variety of derivative drugs containing a coumarin moiety have been developed and used in clinics, such as esculetin, phenprocoumon, warfarin, acenocoumarol, hymecromone, carbochromen, dicoumarol, and daphnetin (Figure 1). Structural modification of coumarin can derivate new compounds with potent bioactivities. Of all the derivative drugs, warfarin is the most famous. Warfarin has been widely used as an oral anticoagulant medication for prophylaxis and treatment of venous thrombosis and thromboembolic events (Yaghi et al., 2022).
Daphnetin, another well-known derivative of coumarin, was first isolated from plants of the Daphne genus, hence the name (Wróblewska-Łuczka et al., 2023). Like coumarin, daphnetin is a plant secondary metabolite widely distributed in food and medicinal herbs, especially in Chinese medicinal herbs. As a consequence, daphnetin can be extracted from a variety of natural plants, such as D. gnidium, D. giraldii, D. mezereum, D. oleoides, and so on (Han et al., 2020; Khouchlaa et al., 2021). Chemically, daphnetin includes an essential coumarin-like backbone, yet it owns two more hydroxyl groups at C-7 and C-8 compared to coumarin. Thus, daphnetin is also called 7,8-dithydroxycoumarin (Figure 1). Physically, daphnetin exists as an odorless and tasteless powder, dissolving freely in ethanol, methanol and dimethyl-sulfoxide but water slightly (Zhu et al., 2010). As a natural product, daphnetin was mainly extracted from plants at first, which limited its large-scale utilization. Though there is no report suggesting that coumarin can be synthesized via coumarin, daphnetin can be synthesized from pyrogallol, 2,3,4-trihydroxybenzaldehyde, and umbelliferone (7-hydroxycoumarin) as illustrated in Figure 2 (Bizzarri et al., 2017; Wang et al., 2017; Pardo-Castaño et al., 2019). When pyrogallol and propionic acid are heated at 125 C, daphnetin can be synthesized under the catalysis of concentrated sulfuric acid (Pardo-Castaño et al., 2019). Daphnetin is synthesized when 2,3,4-trihydroxybenzaldehyde and ethyl acetate are chemical reaction substrates in the presence of N, N-diethylaniline under a nitrogen atmosphere (Wang et al., 2017).
As a representative derivative of coumarins, the biological activities of daphnetin have drawn much attention among scientists and have been the subject of extensive research (Wang et al., 2020b; Boulebd and Amine Khodja, 2021; Pei et al., 2021). Since the 1980s, daphnetin has been an adjunctive therapy for cardiovascular diseases (Gao et al., 2008). Additionally, daphnetin has been increasingly identified as an essential compound of the Zushima tablet, a traditional Chinese medicine preparation used to treat rheumatoid arthritis (Han et al., 2020). Recently, more pharmacological activities of daphnetin have been reported, including anti-inflammation, anti-cancer, anti-autoimmune diseases, and neuroprotection properties (Figure 3) (Finn et al., 2004; Shu et al., 2014; Lv et al., 2018; Zhi et al., 2019; Yan et al., 2022; Zhang et al., 2022). Although the bioactivities and therapeutic potentials of daphnetin have been well documented (Hang et al., 2022; Javed et al., 2022), the elaborate molecular mechanisms associated with its functions remain largely unknown. Here, we appreciate more attention to the molecular effects underlying the pharmacological activities of daphnetin. We intend to explore how daphnetin performs its pharmacological effects via distinct signaling pathways, NLRP3 inflammasome, and inflammatory factors (Figure 4; Table 1) to provide insights for developing new therapeutic strategies for relevant diseases.
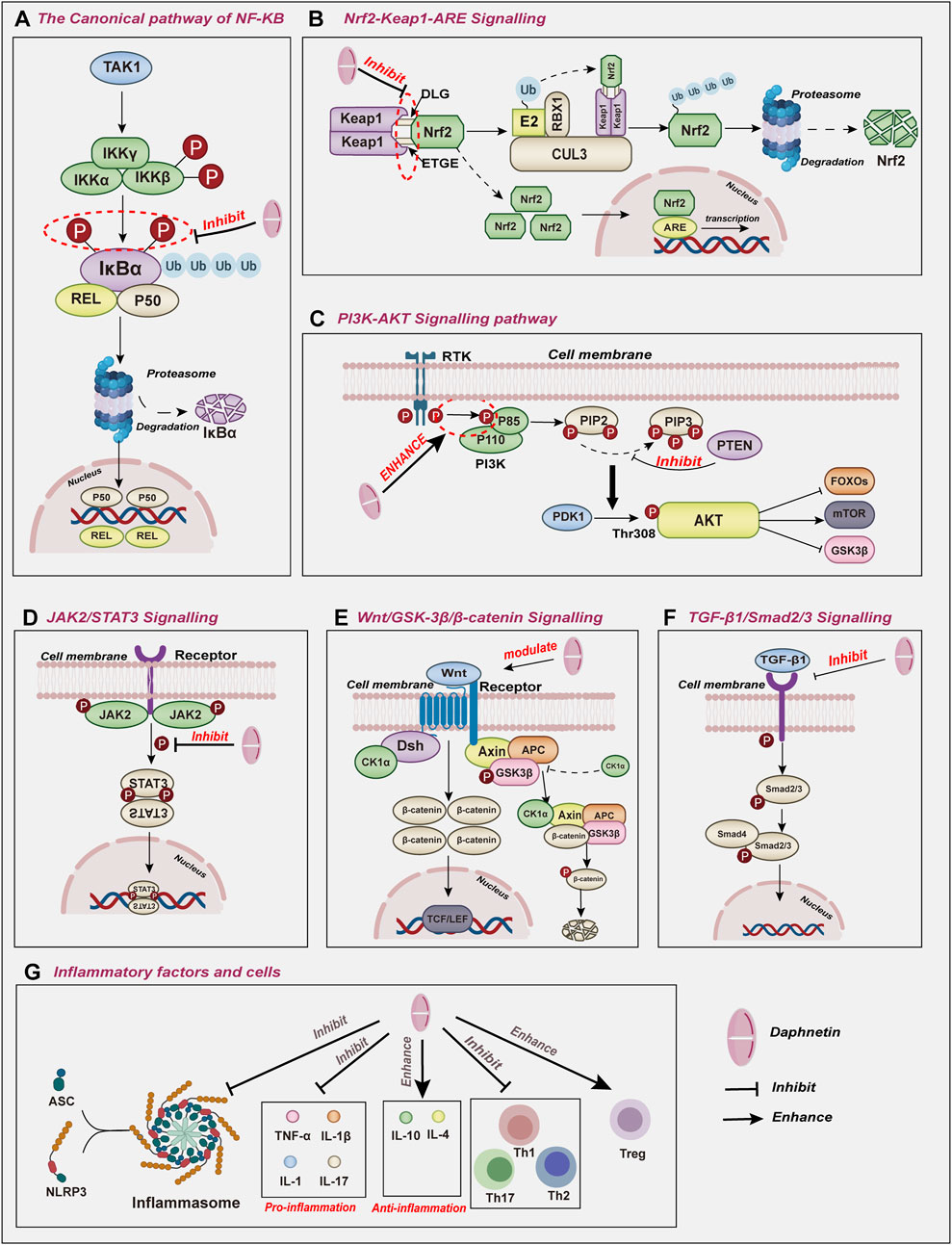
Figure 4. The molecular effects underlying the pharmacological activities of daphnetin include (A) NF-κB, (B) Nrf2, (C) PI3K/AKT, (D) JAK2/STAT3, (E) Wnt/GSK-3β/βcatenin, (F) TGF-β1/Smad2/3signaling pathways and (G) inflammatory factors and cells.
To determine whether elaborate evidence elucidates the molecular effects underlying the pharmacological activities of daphnetin, we conducted a comprehensive search across multiple reputable databases, including MEDLINE, EMBASE, Web of Science, and Google Scholar, to identify relevant articles published. The search strategy used a combination of MeSH terms pertaining to signaling pathways and daphnetin (7,8-dithydroxycoumarin) and Boolean operators to retrieve articles. The inclusion criteria to guide the selection process was as follows: well-controlled intervention studies in cell lines and animal models detecting the changes of signaling pathways in the presence of daphnetin. Studies that did not assign a control group and irrelevant literature were excluded. Two independent investigators conducted an initial screening of the articles based on the inclusion criteria above. Articles that met the predefined inclusion criteria were selected for a full-text assessment. Any discrepancies between investigators were resolved by discussion until a consensus was reached. Subsequently, based on this point, we proposed the possible signaling pathways, inflammation cells and factors linked to the pharmacological activities of daphnetin and promising combined therapeutic strategies.
2 Effects of daphnetin on signaling pathways
2.1 Effects of daphnetin on NF-κB signaling pathway
Nuclear Factor-kappa B (NF-κB), an essential transcription factor, has been reported to exert an increasingly fundamental role in regulating inflammatory and immune responses (Zhang et al., 2017; Yu et al., 2020; Fang et al., 2023). NF-κB family consists of five prominent inducible members: RELA (p65), RELB, c-REL, NF-κB1 (p50), and NF-κB2 (p52). These proteins interact with each other to form distinct homodimers and exert their physiological functions (Ghosh et al., 1998). NF-κB proteins usually are kept in inactive, as bound to the inhibitor of κB (IκB) family. Various inflammatory stimuli can trigger the phosphorylation of IκB, which leads to its degradation and the release of the active NF-κB dimer (Esposito et al., 2016).
NF-κB can be activated through the canonical pathway, which responds to various external stimuli involved in inflammation and immune responses such as cytokines, pathogens, and stress (Lawrence, 2009; Sun, 2017). NF-κB1, RELA, and c-REL are activated and translocated to the nucleus to mediate downstream gene transcription in the canonical pathway (Figure 4A).
Recently, mounting evidence shows that daphnetin possesses multiple bioactivities via regulating the NF-κB signaling pathway in various exogenous inflammatory animal models. Western blot analysis reveals that the NF-κB signaling pathway is over-activated in a rat severe acute pancreatitis model induced by sodium taurocholate. Pretreatment of daphnetin at 4 mg/kg significantly blocks the TLR4/NF-κB signaling pathway by inhibiting the phosphorylation of IκBα and the expression of TLR4, thereby attenuating pancreatic injury in rat severe acute pancreatitis (SAP) model compared to the control group (Liu et al., 2016). Similarly, in a rabbit model of osteoarthritis called the Hulth-Telhag model, Zhang et al. found that daphnetin exerted a fundamentally chondroprotective role in Hulth-Telhag rabbit chondrocytes (Rogart et al., 1999). Furthermore, in vitro assays suggested that daphnetin (12, 24 and 48 ng/mL) markedly suppressed the expression of matrix metalloproteinases MMP-3, MMP-9 and MMP-13 in synovial cells, which is partially due to the inhibition of NF-κB signaling pathways and subsequent downregulation of IL-1β, IL-6, IL-12 (Zhang X. et al., 2020). Daphnetin at 5 and 10 mg/kg also exerts anti-inflammatory and protective properties in a mouse endotoxin-induced lung injury (EILI) model, a severe inflammatory condition caused by bacterial toxins (Yu et al., 2014). Subsequently, both in vitro and in vivo experiments indicated that daphnetin decreased the levels of inflammatory cytokines, reactive oxygen species, and apoptotic markers in EILI by modulating the NF-κB signaling pathways in a dose-dependent manner at a range from 10 to 160 μM, which partly explains the role of daphnetin (Yu et al., 2014). Likewise, in a mouse acute liver failure (ALF) model induced via lipopolysaccharide (LPS)/D-galactosamine (GalN), the NF-κB signaling pathway is involved in the process of inflammation, mediating the occurrence and progression of ALF. Compared with the LPS/GalN-challenged group, daphnetin at 20, 40, and 80 mg/kg effectively and dose-dependently inhibited JNK, ERK, and P38, blocking the phosphorylation and degradation of IκBα. Therefore, daphnetin decreased the translocation of NF-κB (p65), significantly induced autophagy activation and finally prolonged the survival of the treatment group, suggesting that the anti-inflammation property of daphnetin is partly attributed to the inhibition of the NF-κB signaling pathway activation (Lv et al., 2018).
By modulating the NF-κB signaling pathway, daphnetin can also relieve several auto-immune inflammation diseases. Given that autoreactive T-cell has been implicated in the pathogenesis of a variety of autoimmune diseases (Rosetti et al., 2022), researchers have focused on the inhibitory effects of daphnetin(0–64 mg/mL) at 48 h and 24 h on concanavalin A (ConA) induced T-cell proliferation and found that daphnetin significantly suppressed splenocyte proliferation and cell cycle progression by blocking the G0/G1 transition and the NF-κB pathway activation in mouse T-cell, finally mediating immunosuppressive activity on T-cell (Song et al., 2014). As one of the most common chronic disabling neurological diseases, multiple sclerosis (MS) mainly affects young adults and is closely related to aging (Zhang Y. et al., 2020; Graves et al., 2023). In a mice experimental autoimmune encephalomyelitis (EAE) animal model for MS, constant administration of daphnetin(8 mg/kg) for 28 days markedly alleviated the clinical symptoms and demyelination of the mouse by repressing Th1 and Th17 cell responses. Mechanistically, daphnetin repressed the phenotype and function of dendritic cells via modulating NF-κB signaling pathways (Wang et al., 2016). Recently, daphnetin has been used to intervene in systemic lupus erythematosus (SLE), a systemic autoimmune disease with multiple pathogenic factors and organ involvement (Gatto et al., 2013). In an SLE NZB/WF 1 mouse model, administration of daphnetin (at 5 mg/kg) once a day for 12 weeks reduced the organ damage caused by SLE, lowered the serum autoantibody production, and increased the survival rate of mice with SLE via suppressing RELA and NF-κB signaling pathways (Gatto et al., 2013). Furthermore, phosphorylation and degradation of IκBα, the indicators of NF-κB activation, are prevented by daphnetin (Li et al., 2017).
Moreover, daphnetin has been identified to prevent against cancer via modulating NF-κB signaling pathways. In Sprague-Dawley rats with mammary carcinogenesis induced by 7,12-dimethylbenz(a)anthracene (DMBA), constant daphnetin treatment for 28 days at 20, 40, 80 mg/kg dose-dependently corrected these inflammatory changes as well as enhanced the antioxidative protection in these cancer-bearing animals by hindering the expression and nuclear translocation of NF-κB (Kumar et al., 2016). Still, administration of daphnetin at 10, 20, and 30 mg/kg for 16 weeks was verified to ameliorate the invasion of chemically induced hepatocellular carcinoma via reduction of inflammation and oxidative stress in a concentration-dependent manner (Li et al., 2022). Daphnetin suggestively suppressed the tumor incidence and the weight of the liver and spleen in a dose-dependent manner compared with the untreated group. The mechanism of anti-cancer in daphnetin involved the suppression of inflammatory responses via the NF-κB signaling pathway (Li et al., 2022). Furthermore, daphnetin affected the epithelial-mesenchymal transition process in human lung adenocarcinoma cells. Gong et al. (2023) found that daphnetin(10–80 mg/kg) inhibited the proliferation and migration of human lung adenocarcinoma epithelial A549 cells through regulating the NF-κB signaling pathway (Gong et al., 2023).
Consequently, daphnetin exerts its bioactivity via modulating NF-κB pathways, including anti-inflammation and anti-cancer effects.
2.2 Effects of daphnetin on Nrf2 signaling
Nuclear factor erythroid 2-related factor 2 (Nrf2) is known as a pivotal transcription factor that belongs to the Cap’n’Collar (CNC) family of conserved basic leucine zipper (bZIP) transcription factors (Moi et al., 1994). Structurally, Nrf2 is composed of seven conserved Nrf2-ECH homology domains (Neh1–7) with different functions to regulate Nrf 2 transcriptional activity (Hayes and Dinkova-Kostova, 2014). Of all Nehs, Neh1 allows the recognition of antioxidant response elements (ARE) to activate gene transcription. Under normal physiological homeostasis, Neh2 is bound to Kelch-like-ECH-associated protein 1 (Keap1), which stimulates CULLIN3(CUL3) E3 ubiquitin ligase and leads to proteasomal degradation of Nrf2 via the proteasome system. This degradation mechanism maintains Nrf2 dynamic equilibrium at the protein level, ensuring only a tiny fraction of Nrf2 reaches the nucleus to regulate the basal expression of target genes (Torrente and DeNicola, 2022). Once stress occurs, inhibition of Nrf2 directed by Keap1 is abrogated, resulting in Nrf2 stabilization (Dayalan Naidu and Dinkova-Kostova, 2020). Consequently, Nrf2 can liberate and translocate into the nucleus to interact with AREs, activating their transcription and antioxidant characteristics (Figure 4B) (Itoh et al., 1997; Kobayashi et al., 2016). Nrf2 has emerged as a pivotal player in various cellular processes maintaining cell homeostasis (Shaw and Chattopadhyay, 2020). By upregulating the Nrf2 pathway, daphnetin can alter the expression levels of Bcl-2, Bax, and caspase 3, critical apoptosis regulators (programmed cell death) in cells exposed to oxidative stress or injury (Liang et al., 2010).
By modulating the Nrf2 signaling pathway, daphnetin protects cells against oxidative damage and mitochondrial dysfunction. The organic peroxide tert-butyl hydroperoxide (t-BHP), acting as a cellular toxin, promotes oxidative stress and leads to various types of cell damage. In a cell apoptosis model induced by t-BHP, daphnetin (2.5, 5, and 10 μg/mL) significantly guarded RAW 264.7 cells against t-BHP-induced cytotoxicity and cell apoptosis in a concentration-dependent manner (Lv et al., 2017). Furthermore, daphnetin decreased t-BHP-induced ROS generation and inhibited the expression of cytochrome c in RAW 264.7 cell cytoplasm and mitochondria, which preserved the mitochondrial physiological function (Lv et al., 2017). Mechanically, daphnetin activated the Keap1-Nrf2/ARE signaling pathway to exert antioxidant effects and modulate the expression of numerous antioxidant enzymes, including GCLC, GCLM, and HO-1 (de Oliveira et al., 2016). However, daphnetin-mediated cell viability, ROS blockade, and the expression of antioxidative enzymes was almost abolished in Nrf2 knockout RAW 264.7 cells, which verified that the role of daphnetin in preventing mitochondrial dysfunction was largely dependent on the upregulation of the Nrf2 pathway (Niture et al., 2014; Lv et al., 2017).
Similarly, daphnetin treatment significantly alleviated the cellular toxic effects of arsenic on human lung epithelial cells (Lv et al., 2019). As a natural toxicant, arsenic is demonstrated to induce acute and chronic toxicity in lung tissue, including tissue injury and cell apoptosis (Putila and Guo, 2011; Wang et al., 2023). Further research showed that daphnetin (2.5, 5 and 10 μg/mL) enhanced Nrf2 activation and translocation and increased Keap1 degradation in Beas-2B cells, which activated genes downstream of Nrf2 in Beas-2B cells to protect Beas-2B cells from arsenic-induced oxidative stress and cytotoxicity (Lv et al., 2019). Significantly, pretreatment with daphnetin reversed the decrease in the anti-apoptotic factor Bcl-2 induced by arsenic and reduced the increase in the pro-apoptotic factor Bcl-2-associated X protein (Bax), which was considerably attenuated when Nrf2 was depleted in vitro (Lv et al., 2019).
Not only cellular protection, but also organic preservation is remarkably mediated by daphnetin. Through enhancing the Nrf2 antioxidant signaling pathway, daphnetin inhibits inflammatory and oxidative responses, protects against organic injury and toxicity, and sustains the physiological function and homeostasis of organs (Mohamed et al., 2014; Zhang et al., 2018; Lv et al., 2020; Fan et al., 2021a).
Though known as a potent antimicrobial agent against Gram-negative infections, gentamicin (GM) has limited clinical applicability due to its nephrotoxic effects (Lopez-Novoa et al., 2011; Lee et al., 2012). In a GM-induced renal injury mice model, daphnetin at 40 mg/kg is demonstrated to ameliorate GM-induced kidney dysfunction and cell damage in mice. Pretreatment with daphnetin significantly reduced the level of BUN and creatinine and improved renal appearance and histopathological evaluation in mice impaired by GM (Fan et al., 2021a). Mechanism research showed that daphnetin activated the Nrf2/ARE pathway in a dose-dependent manner, attenuated oxidative stress and inflammation, and protected against tubular cell apoptosis induced by GM (Fan et al., 2021a). Meanwhile, daphnetin (40 mg/kg) markedly ameliorated nephrotoxicity and renal dysfunction induced by chemotherapeutic cisplatin via modulating the Nrf2 pathway (Zhang et al., 2018). Daphnetin lessened both cisplatin-induced kidney biochemical parameters disorder and histopathological changes. Subsequent evidence proved that daphnetin improved the kidney’s oxidative stress and inflammatory reaction, reversed the nephrotoxicity caused by cisplatin, and sustained renal normal physiological function (Zhang et al., 2018).
Based on the remarkably protective effects of daphnetin in t-BHP-induced mitochondrial dysfunction, Lv et al. (2020) verified that daphnetin (40 or 80 mg/kg) alleviated t-BHP and acetaminophen (APAP)-induced hepatotoxicity through altering Nrf2 pathway activation. Moreover, the pharmacological effect was improved with the mounting concentration. It is reported that daphnetin attenuated t-BHP-triggered hepatotoxicity as well as mitochondrial dysfunction in HepG2 cells, protected against APAP-induced acute liver failure in mice, and prolonged the survival of mice treated by APAP. The hepatoprotective mechanism of daphnetin against APAP relies on the regulation of the Nrf2 signaling pathway, and these beneficial effects were eliminated in Nrf2-deficient mice. Additionally, daphnetin suppressed JNK and ASK1 phosphorylation, Txnip and NLRP3 expression, and caspase-3 cleavage in WT mice, which were related to oxidative phosphorylation, inflammation, and apoptosis (Cao et al., 2017; Woolbright and Jaeschke, 2017; Lv et al., 2020).
Another previous research suggested that daphnetin administrated for 4 weeks at 4.5 mg/kg effectively protected the liver from CCL4-induced damage, possibly through its antioxidant and anti-inflammatory effects (Mohamed et al., 2014). Daphnetin restored near control levels of the hepatic enzymes ALT and AST and markedly improved the histopathology of the liver in CCL4-treated mice, indicating its improvement in liver function. Moreover, daphnetin reduced the levels of oxidative stress marker malondialdehyde in the liver tissues of CCL4-treated mice, indicating its ability to suppress inflammatory responses. mRNA analysis revealed that the expression of HO-1, which was dependent on the Nrf2 pathway, was induced. Consequently, daphnetin facilitated Nrf2 nuclear translocation to confer hepatoprotection against oxidative injury (Mohamed et al., 2014).
Accordingly, by regulating the Nrf2 pathways, daphnetin mediates antioxidant damage and mitochondrial maintenance at cellular and organic levels.
2.3 Effects of daphnetin on PI3K/AKT signaling
The Phosphatidylinositol 3-kinase (PI3K)/Protein Kinase B (PKB, also named AKT) signaling pathway, is renowned for its pivotal role in regulating various cellular processes, including proliferation, differentiation, and apoptosis (Arcaro and Guerreiro, 2007). Among three PI3Ks (Vanhaesebroeck et al., 2010), Class IA PI3K, a heterodimeric protein, comprises a catalytic (p110) and a regulatory subunit(p85) (Vidal et al., 2022). In normal physiological conditions, catalytic subunits are bonded and inhibited by regulatory proteins. The regulatory proteins bring the catalytic subunits in contact with their lipid substrates at the membranes on cellular activation (Bilanges et al., 2019; Vidal et al., 2022). AKT serine/threonine kinase family plays pivotal roles as key downstream effector molecules in the PI3K signaling pathway (Toulany et al., 2017). When extracellular signals are detected, PI3K is recruited to the plasma membrane and subsequently activated by either receptor tyrosine kinases or G-protein coupled receptors, initiating the conversion of PIP2 into PIP3 (Vanhaesebroeck et al., 2010). Subsequently, AKT and phosphoinositide-dependent kinase 1 (PDK1) are recruited to the inner surface of the plasma membrane. Once at the membrane, PDK1 phosphorylates AKT at Thr308 to initiate AKT activation (Figure 4C) (Toulany et al., 2017).
Subsequent regulatory effects of activated AKT on cellular biological processes are mediated by various downstream target proteins. AKT regulates downstream target proteins through a phosphorylation cascade, including FOXO, mTOR, and GSK3b, to control cell survival, growth, and proliferation (He et al., 2021). Given the essential function of PI3K/Akt signaling including cell proliferation, survival, metabolism and motility, the promising ability of daphnetin to selectively modulate PI3K signaling has garnered increasing attention for its potential development.
Recent studies have identified daphnetin as a natural compound that effectively activates NK cell effector functions. Further research revealed that daphnetin at 10 μM directly improved the cytotoxicity of NK cells and promoted IFN-γ production in the presence of IL-12 (Yao et al., 2021). Subsequent RNA-sequencing analyses demonstrated that the mechanisms of daphnetin in regulating NK cells are dependent on the PI3K-Akt signaling pathway, which is further confirmed by the impact of PI3K-Akt and mTOR inhibitors (such as LY294002 and rapamycin) on daphnetin-mediated NK cell activation (Yao et al., 2021).
Daphnetin (2, 4, and 8 mg/kg) is reported to exert neuroprotective action in Alzheimer’s disease (AD) model mice (Yan et al., 2022). As a progressive neurodegenerative disorder with genetic complexity, AD is clinically characterized by the dysfunction of memory and cognition (Author Anonymous, 2023). Neuropathological features of AD include neurofibrillary tangles, neuroinflammation, and β-amyloid accumulation, which significantly contribute to AD development due to their impact on synaptic function (Author Anonymous, 2023; Jorfi et al., 2023). In AD-linked transgenic model mice, daphnetin alleviated cognitive impairment by reducing β-amyloid deposition compared to the control group. Moreover, daphnetin promotes the dendrite branch density and increases synaptic protein generation via activating PI3K-Akt signaling (Yan et al., 2022). This finding was corroborated by the use of the PI3K inhibitor LY294002, which reversed daphnetin-induced neuroprotective effects.
By targeting the PI3K/AKT/mTOR signaling pathway, Daphnetin(0–60 μg/mL) can inhibit autophagy and relieve inflammation in fibroblast-like synoviocytes (FLS) in rats with collagen-induced arthritis (CIA) induced by TNF-α. Likewise, the pharmacological inhibitive effects increased with the increasing concentration (Deng et al., 2020). In this disease, the PI3K-Akt signaling pathway significantly impacts the migration of FLS and the inhibition of cartilage formation (Su et al., 2019; Weng et al., 2023). Compared to the disease model group, daphnetin reduces the phosphorylation of AKT and mTOR by inhibiting the mRNA expression of AKT and increasing the mRNA expression of the PI3K negative regulatory gene PTEN. Subsequently, PI3K/AKT signaling downstream effector mTOR and BAD, which govern autophagy negatively and apoptosis respectively, are significantly suppressed. Consequently, daphnetin may be a potential therapeutic approach in treating rheumatoid arthritis (Deng et al., 2020).
In addition, daphnetin is believed to possess antitumor potential (Kumar et al., 2018; Deng et al., 2020), and exhibit potent antitumor effects in ovarian cancer by inducing ROS-dependent apoptosis, which relies on the Akt/mTOR pathway (Fan et al., 2021b). Daphnetin inhibited ovarian cancer proliferation and promoted cell apoptosis in vivo at 30 mg/kg and in vitro at 0, 5, 10, 20, and 40 μg/mL in three different cell types, which was mediated via the production of ROS. Moreover, daphnetin treatment accumulated the level of LC3B-II, autophagic vacuoles, and autophagic flux in ovarian cancer, suggesting that cytoprotective autophagy was activated. Notably, once combined with autophagy inhibitor HCQ, the anti-cancer effect of daphnetin on ovarian cancer cells was enhanced. Daphnetin-induced autophagy and apoptosis may depend on the AMPK/Akt/mTOR pathway in ovarian cancer cells (Lei et al., 2013). Furthermore, daphnetin significantly elevated the level of AMPK in A2780 cells, yet the expression levels of p-Akt and p-mTOR were downregulated. APMK inhibitor (Compound C) reversed the expression of p-Akt and p-mTOR in A2780 cells treated with daphnetin and synergically enhanced daphnetin-induced antitumor effects. Briefly, the Akt/mTOR pathway is involved in Daphnetin-induced protective autophagy and apoptosis (Fan et al., 2021b).
Collectively, daphnetin modulates immune reaction and autophagy, as well as exerts anti-inflammation and anti-cancer effects via the PI3K/AKT pathway.
2.4 Effects of daphnetin on JAK2/STAT3 signaling
The JAK2/STAT3 pathway has become a crucial regulator in the initiation and progression of inflammatory and immune responses across a wide range of pathological conditions, thereby exerting significant influence in the pathogenesis of various diseases (Chen et al., 2023; Ott et al., 2023). Janus kinase 2 (JAK2) belongs to the JAK family. These kinases are implicated in immune system regulation, immunocyte differentiation and proliferation, and pro-inflammatory response (Agashe et al., 2022). As a member of the signal transducer and activator of the transcription family, STAT3 acts as a transcription factor, controlling cell cycle progression and apoptotic mechanisms. In addition, STAT3 is also associated with autoimmune and inflammatory diseases (Dong et al., 2021). The JAK2/STAT3 pathway has attracted considerable interest for its distinctive impact on inflammation and lung injury (Figure 4D) (Montero et al., 2021; Liu D. et al., 2022).
Daphnetin has been demonstrated to exert gastrointestinal protective effects, ameliorating the severity of colitis and attenuating the damage to the intestinal structure in DSS-induced ulcerative colitis mice (He et al., 2023). In addition, daphnetin regulated the expression of apoptosis-related proteins in vivo at 16 mg/kg for six consecutive days. Daphnetin treatment significantly decreased the level of pro-apoptotic proteins Bax and cleaved caspase 3, while enhanced the anti-apoptotic protein (BCL-2) expression compared with the control group. Daphnetin substantially suppressed the activity and the levels of inflammatory cytokines, including MDA and SOD, conferring anti-inflammatory effects. Likewise, in vitro assays verified the cytoprotective effects of daphnetin on Caco-2 cells from LPS-stimulated viability impairment, apoptosis, oxidative stress, and inflammation. Furthermore, daphnetin suppressed the activity of JAK2/STAT3 signaling in LPS-induced Caco-2 cells in a REG3A-dependent manner. Meanwhile, JAK2/STAT signaling inhibition synergized with daphnetin in LPS-stimulated Caco-2 cells. Hence, daphnetin inhibited the UC progression primarily through REG3A-mediated JAK2/STAT3 signaling (He et al., 2023).
By suppressing the JAK2/STAT3 pathway, daphnetin is verified to ameliorate acute lung injury in mice with severe acute pancreatitis (Yang et al., 2021). In the L-arginine-induced SAP-associated acute lung injury model, daphnetin at 2–4 mg/kg significantly reduced IL-6 and TNFα concentrations in both serum and lung tissues, serum amylase and myeloperoxidase activities, and macrophage and neutrophil infiltration and cell apoptosis in the lung tissue; finally alleviating SAP-induced pancreatic and lung tissue damage. Notably, immunohistochemical staining assays suggested that daphnetin pretreatment attenuated the levels of p-JAK2 and p-STAT3, which were comparably increased in the SAP group (Yang et al., 2021). These results are consistent with a previous study that found daphnetin reduces endotoxin lethality and improves LPS-induced acute lung injury in mice via suppressing JAK/STATs activation and ROS production (Shen et al., 2017). Moreover, cell viability was not influenced notably during the daphnetin treatment.
In conclusion, these results showed that inhibiting the JAK2/STAT3 pathway is the essential mechanism of daphnetin to mediate antioxidant activity and anti-inflammatory properties.
2.5 Effects of daphnetin on Wnt/GSK-3β/β-catenin signaling
As a highly conserved signaling pathway, the Wnt/β-catenin signaling pathway is pivotal in regulating fundamental physiological and pathological processes, including cell proliferation, survival, differentiation, and migration (Liu J. et al., 2022; Yao et al., 2023). The activation of the canonical Wnt pathway relies on the cooperation between Wnt glycoproteins and several transmembrane receptors (Ma et al., 2023). However, the regulation of β-catenin is influenced by GSK3β, as GSK3β is the upstream molecule of β-catenin. Nonphosphorylated GSK3β can cause the phosphorylation and degradation of β-catenin in the cytoplasm. When GSK3β is inhibited, the phosphorylation of β-catenin will be blocked and cannot be degraded (Figure 4E) (Xia et al., 2019; Cheng et al., 2020).
In a current dexamethasone-induced osteoporosis model, dexamethasone remarkably affected the histological changes, femoral bone mineral content, and femoral microstructure parameters of experimental rats, finally causing osteoporosis (Wang et al., 2020a). However, daphnetin treatment improved bone mineral content and microstructure parameters at 1 and 4 mg/kg, restoring the levels of bone turnover markers (Wang et al., 2020a). Moreover, the Wnt/GSK-3β/β-catenin signaling pathway was stimulated when daphnetin was added, which indicated that daphnetin performed osteoprotective effects via Wnt/GSK-3β/β-catenin signaling pathway. Further study verified this mechanism via XAV939, which inhibits the Wnt/GSK-3β/β-catenin signaling pathway. Once the small-molecule inhibitor XAV939 was added, the transduction of Wnt/GSK-3 β/β-catenin pathway was blocked, which abolished the effect of daphnetin on the differentiation and mineralization of MC3T3-E1 cells, indicating that daphnetin specifically exerted its effects against GIOP via Wnt/GSK-3β/β-catenin pathway (Stakheev et al., 2019; Wang et al., 2020a).
However, daphnetin (25 and 50 mg/kg) is reported to exert antitumor effects by inhibiting the Wnt/β-catenin signaling pathway in hepatocellular carcinoma xenograft models (Liu C. et al., 2022). The roles of daphnetin in apoptosis and G1 phase arrest of hepatocellular carcinoma cells were potently neutralized by activation of the Wnt/β-catenin signaling with SKL2001 treatment, which is an agonist of the Wnt/β-catenin signaling pathway (Liu C. et al., 2022).
Consequently, the effects of daphnetin on Wnt/GSK-3β/β-catenin signaling pathway may vary from the microenvironment of targeting cells; however, daphnetin governs the maintenance of physiological homeostasis by modulating this pathway.
2.6 Effects of daphnetin on TGF-β1/Smad2/3 signaling
Known for its pivotal effects on the progression of organic fibrosis, TGF-β1 signaling is closely related to immune response, inflammation, and matrix synthesis (Su et al., 2020; Saadat et al., 2021; Liu Z. et al., 2022). In addition, TGF-β1 can stimulate the phosphorylation of the pro-fibrotic transcription factors Smad2 and Smad3, further driving the expression of TGF-β-sensitive and pro-fibrotic genes (Figure 4F) (Hu et al., 2020).
Currently, a study by Lee et al. explored the effects of daphnetin on transverse aortic constriction (TAC)-induced cardiac hypertrophy and myocardial fibrosis in mice at 10 and 20 mg/kg and angiotensin II (Ang II)–induced hypertrophy in H9c2 cardiomyoblasts at 10 and 20 μg/mL (Syed et al., 2022). The results showed that daphnetin reduced cardiac remodeling by modulating the TGF-β1/Smad2/3 signaling pathway. In addition, daphnetin decreased ECM overproduction, cardiac fibrotic event, and myofibroblast alterations by inhibiting TGF-β1/Smad2/3 signaling proteins, indicating that daphnetin effectively protected against cardiac hypertrophy and fibrosis.
Therefore, daphnetin may have potential therapeutic benefits for cardiac diseases involving heart enlargement and scarring.
2.7 Effects of daphnetin on autophagy signaling
Triggered by stress or starvation, autophagy evolves as an intracellular conserved catabolic process mediated by lysosome sustains to degrade cellular components (Yamamoto et al., 2023). During this process, targeting proteins and aged or damaged organelles sequestered in double-membrane vesicles are called autophagosomes, which ultimately fuse to lysosomes, leading to the degradation of the sequestered components (Behrends et al., 2010). The stimulation of autophagy is usually beneficial in disease, as it helps to remove toxic proteins and cells. However, autophagy can serve both tumor-suppressive and tumor-promoting roles, which depend on the tumor stage, biology, and the microenvironment in cancer (Debnath et al., 2023). Therefore, autophagy is a complex and dynamic mechanism that interacts with other cellular pathways in tumorigenesis.
As described above, daphnetin at 10 mg/kg performed antitumor effects in the ovarian cancer A2780 xenograft model (Fan et al., 2021b). Meanwhile, daphnetin also induced autophagy due to the accumulation of LC3-II and endogenous LC3, which was verified as cytoprotective autophagy in ovarian cancer. Because an autophagy inhibitor further enhanced the antitumor efficacy of daphnetin, indicating intricate roles of daphnetin in anti-ovarian cancer effects (Zhang et al., 2019).
By inducing an autophagic response, daphnetin prevents methicillin-resistant Staphylococcus aureus and attenuates inflammation (Zhang et al., 2019). A study indicated that daphnetin enhanced microphage bactericidal activity and suppressed inflammatory responses via mTOR-dependent autophagic pathway in C57BL/6 mice. However, once a putative autophagy inhibitor, Bafilomycin A1, was added, the autophagy pathway was blocked, and DAPH-elicited repression of the inflammatory response as well as macrophage antibacterial capability, was abolished (Zhang et al., 2019).
Thus, daphnetin exerts anti-cancer and anti-infection effects by modulating autophagy signaling.
3 Effects of daphnetin on NLRP3 inflammasome
Defined as an inflammasome for its ability to respond to DAMPs or PAMPs, NLRP3 Belongs to the nucleotide-binding domain (NBD)- and leucine-rich repeat (LRR)-containing protein (NLR) family, containing a caspase-recruitment domain (ASC) and Caspase-1 (Fu and Wu, 2023). NLRP3 inflammasome functions as a cytosolic signaling complex to mediate the activation of potent inflammation, particularly responding to aging, physical inactivity, over-nutrition, or environmental factors (Mangan et al., 2018).
In the LPS/GalN induced ALF mice model described above, the levels of NLRP3 inflammasome, as well as its downstream inflammatory proteins ASC, Cleaved-caspase-1 (p20), and Mature-IL-1β (p17), were evidently elevated (Lv et al., 2018). As western blotting analysis showed, LPS/GalN activated NLRP3 and related inflammatory proteins were inhibited when treated with daphnetin at 20, 40, and 80 mg/kg (Lv et al., 2018). Therefore, the inflammatory suppression effects of daphnetin are partly attributed to inhibiting NLRP3 inflammasome activation. In addition, daphnetin inhibited the corneal inflammation and neovascularization induced by alkali burn in vitro and vivo. Moreover, further research showed that alkali burn-induced NLRP3 inflammasome activation was significantly blocked when daphnetin was added, attenuating inflammation and improving wound healing and corneal clarity (Lv et al., 2018; Shimizu et al., 2019).
As mentioned above, the NLRP3 inflammasome exerted a substantial influence on ovarian aging, and high TXNIP protein expression indicates oxidative damage to cells. Daphnetin treatment significantly decreased NLRP3 protein expression compared to the control group, confirming that daphnetin significantly rescued premature ovarian failure (Shimizu et al., 2019).
4 Effects of daphnetin on inflammatory factors
As essential mediators, inflammatory factors play critically modificative roles in immune responses (Wang et al., 2022). It is believed that pro-inflammatory factors, including interleukin-1 (IL-1), IL-6, and tumor necrosis factor (TNFα), are dependent on the type I cytokine receptors. While anti-inflammatory factors mainly consist of IL-4 and IL-10 (Turner et al., 2014). Dysregulation in inflammatory factors may cause immune aberrances, hypercoagulability, and reproductive disorders. Intriguingly, daphnetin seems to regulate inflammatory responses by affecting levels of inflammatory factors (Figure 4G).
In the neuropathic pain rats, daphnetin was demonstrated to suppress the expression of pro-inflammatory factors IL-1β, IL-6, and TNF-α, exerting neuroprotective effects. Meanwhile, daphnetin suppressed the activation of microglia, astrocytes, and neurons, thus reducing the nociceptive sensitization in neuropathic pain rats (Zhang et al., 2023). In addition, in an experimental autoimmune encephalomyelitis (EAE) mice model, daphnetin (2 and 8 mg/kg) treatment significantly decreased lymphocyte infiltration and demyelination, which was attributed to reduction in pro-inflammatory factors, including TNF-α and IL-17 and an increase in anti-inflammatory factors, such as IL-4 and IL-10 (Soltanmohammadi et al., 2022). Evidence showed that daphnetin ameliorated the progress of hepatocellular carcinoma by reducing inflammation (Li et al., 2022). Daphnetin(10, 20, and 30 mg/kg) potently suppressed oxidant and inflammatory reactions by reducing the secretion of inflammatory factors TNF-α, IL-1β, and IL-6, ultimately leading to growth cease of hepatic cancer (Kumar et al., 2017; Li et al., 2022).
In fact, these inflammatory factors are secreted by T helper (Th) cells, which can be divided into Th1/Th2/Th17 and regulatory T cells (Tregs) according to specialized functions and patterns of cytokine secretion (Yin et al., 2021). Therefore, the regulatory effects of daphnetin on Th cells have increasingly received widespread attention. By regulating Th17 cells, daphnetin occurred to inhibit immune responses and exert protective effects in a CIA model. Moreover, the level of Th1/Th2 type inflammatory factors was also reduced after daphnetin treatment at 1 and 4 mg/kg (Tu et al., 2012). By modulating both Th17 differentiation and the TGF-β signaling pathway, daphnetin is expected to be a drug candidate for the treatment of idiopathic pulmonary fibrosis, a chronic and refractory interstitial lung disease (Park et al., 2023). As described above, daphnetin at 8 mg/kg profoundly repressed Th1 and Th17 responses, inhibited the secretion of inflammatory factors, and alleviated the clinical symptoms of EAE mice (Wang et al., 2016). Another previous study demonstrated that daphnetin (1 and 4 mg/kg) improved the clinical symptoms and pathological changes in arthritis joints and the beneficial effects associated with restoring the balance of Th cells, including enhancement of Tregs responses and inhibition of Th1/Th2/Th17 cells (Yao et al., 2011). Similarly, in patients with unexplained recurrent pregnancy loss, daphnetin (20 and 40 μg/mL) may exert a regulatory effect on the balance of Th17 and Tregs via decreasing IL-2 and increasing TGF-β1 and IL-6 levels (Zhang Z. et al., 2020). Collectively, daphnetin effectively modulates Th cells and related inflammatory factors.
5 Conclusion and perspective
In this review, we provide a novel perspective of the essential molecular effects to elucidate the mechanisms of daphnetin’s sophisticated pharmacological activities. Notably, in almost all research mentioned above, the pharmacological activities of daphnetin are highly likely to increase with its concentration. Furthermore, based on the literature reviewed, it has been reported that daphnetin exhibited a remarkable pharmacological profile. On the one hand, daphnetin can exert diverse molecular effects and pharmacological activities via various signaling pathways, NLRP3 inflammasome, inflammatory cells, and cytokines (Table 1); on the other hand, the specific pharmacological effects of daphnetin within a given signaling pathway can be variable, which depends on the physiological or pathological context present (Javed et al., 2022). Thus, the pharmacological activities of daphnetin may vary with different physiological and pathological contexts, which is due to its distinct interactions with various cell types and the different activation stages of signaling pathways. The diversity of interactions is crucial for understanding and determining the pharmacological effects of daphnetin.
As a natural product, daphnetin has been recognized as an inhibitor of protein kinase, which can partially elucidate the mechanisms underlying the functional diversity of daphnetin. Of coumarin and its derivatives, including daphnetin, esculin, 2-OH-coumarin, 4-OH-coumarin and 7-OH-coumarin, only daphnetin was found to inhibit protein kinases potently. Specifically, daphnetin was verified to inhibit tyrosine-specific protein kinase EGFR (IC50 = 7.67 µM) and serine/threonine kinases PKA (IC50 = 9.33 µM) and PKC (IC50 = 25.01 µM) in vitro (Yang et al., 1999). Mechanically, the inhibition of EGF receptor tyrosine kinase by daphnetin was competitive with respect to ATP and non-competitive with respect to the peptide substrate. Moreover, the hydroxylation at the C8 position is likely essential for daphnetin to function as a protein kinase inhibitor when compared to coumarin and its derivatives (Yang et al., 1999).
In addition, the pharmacological activities of daphnetin rely on its effects on various signaling pathways, inflammasomes, inflammatory cells, and cytokines mentioned above. When in the distinct physiological and pathological context, the associated signaling cascades and molecular entities undergo different degrees of dysregulation and disorder. Daphnetin, through its regulatory influence on the equilibrium of these pathways, manifests its therapeutic repertoire, including anti-inflammation, anti-cancer, anti-autoimmune diseases, antibacterial, organic protection, and neuroprotection properties in cell and animal experimental models described above.
Given these underlying mechanisms reviewed, daphnetin is likely to exert a more remarkable pharmacological profile in future research. Hence, we expect daphnetin to be a potential drug candidate for several aberrant disorders, including inflammation-associated diseases, organic injury, cancers, and multidrug-resistant infections. Inspiringly, a clinical trial in which the therapeutic effects of daphnetin on colitis verified its ability to promote the healing of the intestinal mucosa of UC patients and effectively improve the patient’s condition and quality of life (Hu et al., 2021). Moreover, the toxicology studies of daphnetin suggest no morality and other known toxicities.
Still, it is particularly vital to verify the safe dosage range of daphnetin, given its pharmacological activities and pharmacokinetics. Thus, relevant pre-clinical and clinical trials are required for daphnetin’s toxicity assessment and therapeutic application. In addition, the functional effects of daphnetin hinge upon the intricate and interconnected interplay of various mechanisms working in tandem; urgently, there is a lack of detailed mechanisms of daphnetin in epigenetic and metabolic research. Thus, further research is warranted to comprehensively investigate the diverse bioactivities and underlying mechanisms of daphnetin and its derivatives. Additionally, novel combination therapy, including daphnetin and other drugs, needs to be further and extensively investigated.
Author contributions
ZW: Writing–review and editing, Writing–original draft. NW: Writing–review and editing, Supervision. LS: Writing–review and editing. SG: Writing–review and editing.
Funding
The authors declare that financial support was received for the research, authorship, and/or publication of this article. This work was supported by National Natural Science Foundation of China (grant number 81900174, 2020; grant number 82370153, 2024); Bethune Plan Project (grant number 2022B17, 2022); and Technology Development Project of Jilin Province (grant number YDZJ202201ZYTS606, 2022; grant number YDZJ202301ZYTS501, 2023).
Acknowledgments
We would like to thank staffs in the Department of Hematology, the First Hospital of Jilin University for their assistance in this work.
Conflict of interest
The authors declare that the research was conducted in the absence of any commercial or financial relationships that could be construed as a potential conflict of interest.
Publisher’s note
All claims expressed in this article are solely those of the authors and do not necessarily represent those of their affiliated organizations, or those of the publisher, the editors and the reviewers. Any product that may be evaluated in this article, or claim that may be made by its manufacturer, is not guaranteed or endorsed by the publisher.
References
Agashe, R. P., Lippman, S. M., and Kurzrock, R. (2022). JAK: not just another kinase. Mol. Cancer Ther. 21, 1757–1764. doi:10.1158/1535-7163.MCT-22-0323
Ahmed, E. Y., Abdelhafez, O. M., Zaafar, D., Serry, A. M., Ahmed, Y. H., El-Telbany, R. F. A., et al. (2022). Antitumor and multikinase inhibition activities of some synthesized coumarin and benzofuran derivatives. Arch. Pharm. Weinh. 355, e2100327. doi:10.1002/ardp.202100327
Ando, T., Nagumo, M., Ninomiya, M., Tanaka, K., Linhardt, R. J., and Koketsu, M. (2018). Synthesis of coumarin derivatives and their cytoprotective effects on t-BHP-induced oxidative damage in HepG2 cells. Bioorg. Med. Chem. Lett. 28, 2422–2425. doi:10.1016/j.bmcl.2018.06.018
Arcaro, A., and Guerreiro, A. S. (2007). The phosphoinositide 3-kinase pathway in human cancer: genetic alterations and therapeutic implications. Curr. Genomics 8, 271–306. doi:10.2174/138920207782446160
Author Anonymous (2023). 2023 Alzheimer’s disease facts and figures. Alzheimers Dement. 19, 1598–1695. doi:10.1002/alz.13016
Behrends, C., Sowa, M. E., Gygi, S. P., and Harper, J. W. (2010). Network organization of the human autophagy system. Nature 466, 68–76. doi:10.1038/nature09204
Bhoi, A., Dwivedi, S. D., Singh, D., Singh, M. R., and Keshavkant, S. (2023). “Chapter 2 - worldwide health scenario from the perspective of herbal medicine research,” in Phytopharmaceuticals and herbal drugs. Editors M. R. Singh, and D. Singh (Academic Press), 13–32. doi:10.1016/B978-0-323-99125-4.00014-7
Bilanges, B., Posor, Y., and Vanhaesebroeck, B. (2019). PI3K isoforms in cell signalling and vesicle trafficking. Nat. Rev. Mol. Cell Biol. 20, 515–534. doi:10.1038/s41580-019-0129-z
Bizzarri, B. M., Botta, L., Capecchi, E., Celestino, I., Checconi, P., Palamara, A. T., et al. (2017). Regioselective IBX-mediated synthesis of coumarin derivatives with antioxidant and anti-influenza activities. J. Nat. Prod. 80, 3247–3254. doi:10.1021/acs.jnatprod.7b00665
Boulebd, H., and Amine Khodja, I. (2021). A detailed DFT-based study of the free radical scavenging activity and mechanism of daphnetin in physiological environments. Phytochemistry 189, 112831. doi:10.1016/j.phytochem.2021.112831
Cao, Z., Fang, Y., Lu, Y., Tan, D., Du, C., Li, Y., et al. (2017). Melatonin alleviates cadmium-induced liver injury by inhibiting the TXNIP-NLRP3 inflammasome. J. Pineal Res. 62. doi:10.1111/jpi.12389
Chen, B., Ning, K., Sun, M.-L., and Zhang, X.-A. (2023). Regulation and therapy, the role of JAK2/STAT3 signaling pathway in OA: a systematic review. Cell Commun. Signal. CCS 21, 67. doi:10.1186/s12964-023-01094-4
Cheng, C., Huang, Z., Zhou, R., An, H., Cao, G., Ye, J., et al. (2020). Numb negatively regulates the epithelial-to-mesenchymal transition in colorectal cancer through the Wnt signaling pathway. Am. J. Physiol. Gastrointest. Liver Physiol. 318, G841–G853. doi:10.1152/ajpgi.00178.2019
Dayalan Naidu, S., and Dinkova-Kostova, A. T. (2020). KEAP1, a cysteine-based sensor and a drug target for the prevention and treatment of chronic disease. Open Biol. 10, 200105. doi:10.1098/rsob.200105
Debnath, J., Gammoh, N., and Ryan, K. M. (2023). Autophagy and autophagy-related pathways in cancer. Nat. Rev. Mol. Cell Biol. 24, 560–575. doi:10.1038/s41580-023-00585-z
Deng, H., Zheng, M., Hu, Z., Zeng, X., Kuang, N., and Fu, Y. (2020). Effects of daphnetin on the autophagy signaling pathway of fibroblast-like synoviocytes in rats with collagen-induced arthritis (CIA) induced by TNF-α. Cytokine 127, 154952. doi:10.1016/j.cyto.2019.154952
de Oliveira, M. R., Ferreira, G. C., and Schuck, P. F. (2016). Protective effect of carnosic acid against paraquat-induced redox impairment and mitochondrial dysfunction in SH-SY5Y cells: role for PI3K/Akt/Nrf2 pathway. Toxicol. Vitro 32, 41–54. doi:10.1016/j.tiv.2015.12.005
Dong, J., Cheng, X.-D., Zhang, W.-D., and Qin, J.-J. (2021). Recent update on development of small-molecule STAT3 inhibitors for cancer therapy: from phosphorylation inhibition to protein degradation. J. Med. Chem. 64, 8884–8915. doi:10.1021/acs.jmedchem.1c00629
Esposito, E., Napolitano, G., Pescatore, A., Calculli, G., Incoronato, M. R., Leonardi, A., et al. (2016). COMMD7 as a novel NEMO interacting protein involved in the termination of NF-κB signaling. J. Cell. Physiol. 231, 152–161. doi:10.1002/jcp.25066
Fan, X., Gu, W., Gao, Y., Ma, N., Fan, C., and Ci, X. (2021a). Daphnetin ameliorated GM-induced renal injury through the suppression of oxidative stress and apoptosis in mice. Int. Immunopharmacol. 96, 107601. doi:10.1016/j.intimp.2021.107601
Fan, X., Xie, M., Zhao, F., Li, J., Fan, C., Zheng, H., et al. (2021b). Daphnetin triggers ROS-induced cell death and induces cytoprotective autophagy by modulating the AMPK/Akt/mTOR pathway in ovarian cancer. Phytomedicine 82, 153465. doi:10.1016/j.phymed.2021.153465
Fang, Z., Meng, Q., Xu, J., Wang, W., Zhang, B., Liu, J., et al. (2023). Signaling pathways in cancer-associated fibroblasts: recent advances and future perspectives. Cancer Commun. lond. Engl. 43, 3–41. doi:10.1002/cac2.12392
Finn, G. J., Creaven, B. S., and Egan, D. A. (2004). Daphnetin induced differentiation of human renal carcinoma cells and its mediation by p38 mitogen-activated protein kinase. Biochem. Pharmacol. 67, 1779–1788. doi:10.1016/j.bcp.2004.01.014
Fu, J., and Wu, H. (2023). Structural mechanisms of NLRP3 inflammasome assembly and activation. Annu. Rev. Immunol. 41, 301–316. doi:10.1146/annurev-immunol-081022-021207
Gao, Q., Shan, J., Di, L., Jiang, L., and Xu, H. (2008). Therapeutic effects of daphnetin on adjuvant-induced arthritic rats. J. Ethnopharmacol. 120, 259–263. doi:10.1016/j.jep.2008.08.031
Gatto, M., Zen, M., Ghirardello, A., Bettio, S., Bassi, N., Iaccarino, L., et al. (2013). Emerging and critical issues in the pathogenesis of lupus. Autoimmun. Rev. 12, 523–536. doi:10.1016/j.autrev.2012.09.003
Ghosh, S., May, M. J., and Kopp, E. B. (1998). NF-kappa B and Rel proteins: evolutionarily conserved mediators of immune responses. Annu. Rev. Immunol. 16, 225–260. doi:10.1146/annurev.immunol.16.1.225
Gong, S.-Q., Liu, H., Wu, J.-L., and Xu, J.-X. (2023). Effects of daphnetin on the mechanism of epithelial-mesenchymal transition induced by HMGB1 in human lung adenocarcinoma cells (A549 cell line). Biotechnol. Genet. Eng. Rev., 1–22. doi:10.1080/02648725.2023.2194092
Graves, J. S., Krysko, K. M., Hua, L. H., Absinta, M., Franklin, R. J. M., and Segal, B. M. (2023). Ageing and multiple sclerosis. Lancet Neurol. 22, 66–77. doi:10.1016/S1474-4422(22)00184-3
Han, S., Li, L.-Z., and Song, S.-J. (2020). Daphne giraldii Nitsche (Thymelaeaceae): phytochemistry, pharmacology and medicinal uses. Phytochemistry 171, 112231. doi:10.1016/j.phytochem.2019.112231
Hang, S., Wu, W., Wang, Y., Sheng, R., Fang, Y., and Guo, R. (2022). Daphnetin, a coumarin in genus stellera chamaejasme linn: chemistry, bioactivity and therapeutic potential. Chem. Biodivers. 19, e202200261. doi:10.1002/cbdv.202200261
Hassanein, E. H. M., Sayed, A. M., Hussein, O. E., and Mahmoud, A. M. (2020). Coumarins as modulators of the keap1/nrf2/ARE signaling pathway. Oxid. Med. Cell. Longev. 2020, 1675957. doi:10.1155/2020/1675957
Hayes, J. D., and Dinkova-Kostova, A. T. (2014). The Nrf2 regulatory network provides an interface between redox and intermediary metabolism. Trends biochem. Sci. 39, 199–218. doi:10.1016/j.tibs.2014.02.002
He, Y., Sun, M. M., Zhang, G. G., Yang, J., Chen, K. S., Xu, W. W., et al. (2021). Targeting PI3K/Akt signal transduction for cancer therapy. Signal Transduct. Target. Ther. 6, 425. doi:10.1038/s41392-021-00828-5
He, Z., Liu, J., and Liu, Y. (2023). Daphnetin attenuates intestinal inflammation, oxidative stress, and apoptosis in ulcerative colitis via inhibiting REG3A - dependent JAK2/STAT3 signaling pathway. Environ. Toxicol. 38, 2132–2142. doi:10.1002/tox.23837
Hu, H.-F., Zhan, Y.-Z., Ding, J.-N., Guo, L.-Y., Jin, W.-F., and Zhang, X.-H. (2021). Clinical observation and preliminary study of mechanism of daphnetin in improving therapeutic effects for colitis. World Chin. J. Dig. 29, 223–230. doi:10.11569/wcjd.v29.i5.223
Hu, J., Chen, Y., Huang, Y., and Su, Y. (2020). Human umbilical cord mesenchymal stem cell-derived exosomes suppress dermal fibroblasts-myofibroblats transition via inhibiting the TGF-β1/Smad 2/3 signaling pathway. Exp. Mol. Pathol. 115, 104468. doi:10.1016/j.yexmp.2020.104468
Hwu, J. R., Kapoor, M., Gupta, N. K., Tsay, S.-C., Huang, W.-C., Tan, K.-T., et al. (2022). Synthesis and antiviral activities of quinazolinamine-coumarin conjugates toward chikungunya and hepatitis C viruses. Eur. J. Med. Chem. 232, 114164. doi:10.1016/j.ejmech.2022.114164
Iranshahi, M., Askari, M., Sahebkar, A., and Hadjipavlou-Litina, D. (2009). Evaluation of antioxidant, anti-inflammatory and lipoxygenase inhibitory activities of the prenylated coumarin umbelliprenin. DARU J. Pharm. Sci. 17, 99–103.
Itoh, K., Chiba, T., Takahashi, S., Ishii, T., Igarashi, K., Katoh, Y., et al. (1997). An nrf2/small maf heterodimer mediates the induction of phase II detoxifying enzyme genes through antioxidant response elements. Biochem. Biophys. Res. Commun. 236, 313–322. doi:10.1006/bbrc.1997.6943
Javed, M., Saleem, A., Xaveria, A., and Akhtar, M. F. (2022). Daphnetin: a bioactive natural coumarin with diverse therapeutic potentials. Front. Pharmacol. 13, 993562. doi:10.3389/fphar.2022.993562
Jorfi, M., Maaser-Hecker, A., and Tanzi, R. E. (2023). The neuroimmune axis of Alzheimer’s disease. Genome Med. 15, 6. doi:10.1186/s13073-023-01155-w
Khouchlaa, A., El Menyiy, N., Guaouguaou, F.-E., El Baaboua, A., Charfi, S., Lakhdar, F., et al. (2021). Ethnomedicinal use, phytochemistry, pharmacology, and toxicology of Daphne gnidium: a review. J. Ethnopharmacol. 275, 114124. doi:10.1016/j.jep.2021.114124
Kobayashi, E. H., Suzuki, T., Funayama, R., Nagashima, T., Hayashi, M., Sekine, H., et al. (2016). Nrf2 suppresses macrophage inflammatory response by blocking proinflammatory cytokine transcription. Nat. Commun. 7, 11624. doi:10.1038/ncomms11624
Kumar, A., Jha, S., and Pattanayak, S. P. (2016). Daphnetin ameliorates 7,12-dimethylbenz[a]anthracene-induced mammary carcinogenesis through Nrf-2-Keap1 and NF-κB pathways. Biomed. Pharmacother. Biomedecine Pharmacother. 82, 439–448. doi:10.1016/j.biopha.2016.05.028
Kumar, A., Sunita, P., Jha, S., and Pattanayak, S. P. (2018). 7,8-Dihydroxycoumarin exerts antitumor potential on DMBA-induced mammary carcinogenesis by inhibiting ERα, PR, EGFR, and IGF1R: involvement of MAPK1/2-JNK1/2-Akt pathway. J. Physiol. Biochem. 74, 223–234. doi:10.1007/s13105-018-0608-2
Kumar, V., Bhatt, P. C., Rahman, M., Al-Abbasi, F. A., Anwar, F., and Verma, A. (2017). Umbelliferon-α-d-glucopyranosyl-(2I→ 1II)-α-Dglucopyranoside ameliorates Diethylnitrosamine induced precancerous lesion development in liver via regulation of inflammation, hyperproliferation and antioxidant at pre-clinical stage. Biomed. Pharmacother. 94, 834–842. doi:10.1016/j.biopha.2017.07.047
Lawrence, T. (2009). The nuclear factor NF-kappaB pathway in inflammation. Cold Spring Harb. Perspect. Biol. 1, a001651. doi:10.1101/cshperspect.a001651
Lee, I.-C., Kim, S.-H., Lee, S.-M., Baek, H.-S., Moon, C., Kim, S.-H., et al. (2012). Melatonin attenuates gentamicin-induced nephrotoxicity and oxidative stress in rats. Arch. Toxicol. 86, 1527–1536. doi:10.1007/s00204-012-0849-8
Lei, Y., Li, H.-X., Jin, W.-S., Peng, W.-R., Zhang, C.-J., Bu, L.-J., et al. (2013). The radiosensitizing effect of Paeonol on lung adenocarcinoma by augmentation of radiation-induced apoptosis and inhibition of the PI3K/Akt pathway. Int. J. Radiat. Biol. 89, 1079–1086. doi:10.3109/09553002.2013.825058
Li, M., Shi, X., Chen, F., and Hao, F. (2017). Daphnetin inhibits inflammation in the NZB/W F1 systemic lupus erythematosus murine model via inhibition of NF-κB activity. Exp. Ther. Med. 13, 455–460. doi:10.3892/etm.2016.3971
Li, T., Yang, G., Hao, Q., Zhang, X., and Zhang, X. (2022). Daphnetin ameliorates the expansion of chemically induced hepatocellular carcinoma via reduction of inflammation and oxidative stress. J. Oleo Sci. 71, 575–585. doi:10.5650/jos.ess21415
Liang, S.-C., Ge, G.-B., Liu, H.-X., Zhang, Y.-Y., Wang, L.-M., Zhang, J.-W., et al. (2010). Identification and characterization of human UDP-glucuronosyltransferases responsible for the in vitro glucuronidation of daphnetin. Drug Metab. Dispos. 38, 973–980. doi:10.1124/dmd.109.030734
Liu, C., Pan, J., Liu, H., Lin, R., Chen, Y., and Zhang, C. (2022a). Daphnetin inhibits the survival of hepatocellular carcinoma cells through regulating Wnt/β-catenin signaling pathway. Drug Dev. Res. 83, 952–960. doi:10.1002/ddr.21920
Liu, D., Wen, L., Wang, Z., Hai, Y., Yang, D., Zhang, Y., et al. (2022b). The mechanism of lung and intestinal injury in acute pancreatitis: a review. Front. Med. 9, 904078. doi:10.3389/fmed.2022.904078
Liu, J., Xiao, Q., Xiao, J., Niu, C., Li, Y., Zhang, X., et al. (2022c). Wnt/β-catenin signalling: function, biological mechanisms, and therapeutic opportunities. Signal Transduct. Target. Ther. 7, 3. doi:10.1038/s41392-021-00762-6
Liu, W., Wu, L., Liu, W., Tian, L., Chen, H., Wu, Z., et al. (2022d). Design, synthesis and biological evaluation of novel coumarin derivatives as multifunctional ligands for the treatment of Alzheimer’s disease. Eur. J. Med. Chem. 242, 114689. doi:10.1016/j.ejmech.2022.114689
Liu, Z., Liu, J., Zhao, K., Shi, Q., Zuo, T., Wang, G., et al. (2016). Role of daphnetin in rat severe acute pancreatitis through the regulation of TLR4/NF-[Formula: see text]B signaling pathway activation. Am. J. Chin. Med. 44, 149–163. doi:10.1142/S0192415X16500105
Liu, Z., Wang, W., Li, X., Tang, S., Meng, D., Xia, W., et al. (2022e). Capsaicin ameliorates renal fibrosis by inhibiting TGF-β1–Smad2/3 signaling. Phytomedicine 100, 154067. doi:10.1016/j.phymed.2022.154067
Lopez-Novoa, J. M., Quiros, Y., Vicente, L., Morales, A. I., and Lopez-Hernandez, F. J. (2011). New insights into the mechanism of aminoglycoside nephrotoxicity: an integrative point of view. Kidney Int. 79, 33–45. doi:10.1038/ki.2010.337
Lv, H., Fan, X., Wang, L., Feng, H., and Ci, X. (2018). Daphnetin alleviates lipopolysaccharide/d-galactosamine-induced acute liver failure via the inhibition of NLRP3, MAPK and NF-κB, and the induction of autophagy. Int. J. Biol. Macromol. 119, 240–248. doi:10.1016/j.ijbiomac.2018.07.101
Lv, H., Liu, Q., Zhou, J., Tan, G., Deng, X., and Ci, X. (2017). Daphnetin-mediated Nrf2 antioxidant signaling pathways ameliorate tert-butyl hydroperoxide (t-BHP)-induced mitochondrial dysfunction and cell death. Free Radic. Biol. Med. 106, 38–52. doi:10.1016/j.freeradbiomed.2017.02.016
Lv, H., Zhu, C., Wei, W., Lv, X., Yu, Q., Deng, X., et al. (2020). Enhanced Keap1-Nrf2/Trx-1 axis by daphnetin protects against oxidative stress-driven hepatotoxicity via inhibiting ASK1/JNK and Txnip/NLRP3 inflammasome activation. Phytomedicine 71, 153241. doi:10.1016/j.phymed.2020.153241
Lv, X., Li, Y., Xiao, Q., and Li, D. (2019). Daphnetin activates the Nrf2-dependent antioxidant response to prevent arsenic-induced oxidative insult in human lung epithelial cells. Chem. Biol. Interact. 302, 93–100. doi:10.1016/j.cbi.2019.02.001
Ma, Q., Yu, J., Zhang, X., Wu, X., and Deng, G. (2023). Wnt/β-catenin signaling pathway-a versatile player in apoptosis and autophagy. Biochimie 211, 57–67. doi:10.1016/j.biochi.2023.03.001
Mangan, M. S. J., Olhava, E. J., Roush, W. R., Seidel, H. M., Glick, G. D., and Latz, E. (2018). Targeting the NLRP3 inflammasome in inflammatory diseases. Nat. Rev. Drug Discov. 17, 588–606. doi:10.1038/nrd.2018.97
Min, S. J., Lee, H., Shin, M.-S., and Lee, J. W. (2023). Synthesis and biological properties of pyranocoumarin derivatives as potent anti-inflammatory agents. Int. J. Mol. Sci. 24, 10026. doi:10.3390/ijms241210026
Mohamed, M. R., Emam, M. A., Hassan, N. S., and Mogadem, A. I. (2014). Umbelliferone and daphnetin ameliorate carbon tetrachloride-induced hepatotoxicity in rats via nuclear factor erythroid 2-related factor 2-mediated heme oxygenase-1 expression. Environ. Toxicol. Pharmacol. 38, 531–541. doi:10.1016/j.etap.2014.08.004
Moi, P., Chan, K., Asunis, I., Cao, A., and Kan, Y. W. (1994). Isolation of NF-E2-related factor 2 (Nrf2), a NF-E2-like basic leucine zipper transcriptional activator that binds to the tandem NF-E2/AP1 repeat of the beta-globin locus control region. Proc. Natl. Acad. Sci. 91, 9926–9930. doi:10.1073/pnas.91.21.9926
Montero, P., Milara, J., Roger, I., and Cortijo, J. (2021). Role of JAK/STAT in interstitial lung diseases; molecular and cellular mechanisms. Int. J. Mol. Sci. 22, 6211. doi:10.3390/ijms22126211
Niture, S. K., Khatri, R., and Jaiswal, A. K. (2014). Regulation of nrf2—an update. Free Radic. Biol. Med. 66, 36–44. doi:10.1016/j.freeradbiomed.2013.02.008
Ott, N., Faletti, L., Heeg, M., Andreani, V., and Grimbacher, B. (2023). JAKs and STATs from a clinical perspective: loss-of-function mutations, gain-of-function mutations, and their multidimensional consequences. J. Clin. Immunol. 43, 1326–1359. doi:10.1007/s10875-023-01483-x
Pardo-Castaño, C., García, A. C., Benavides, P., and Bolaños, G. (2019). Solubility of collinin and isocollinin in pressurized carbon dioxide: synthesis, solubility parameters, and equilibrium measurements. J. Chem. Eng. Data 64, 3799–3810. doi:10.1021/acs.jced.9b00234
Park, S.-J., Ryu, H. W., Kim, J.-H., Hahn, H.-J., Jang, H.-J., Ko, S.-K., et al. (2023). Daphnetin alleviates bleomycin-induced pulmonary fibrosis through inhibition of epithelial-to-mesenchymal transition and IL-17a. Cells 12, 2795. doi:10.3390/cells12242795
Pei, Q., Hu, P., Zhang, H., Li, H., Yang, T., and Liu, R. (2021). Daphnetin exerts an anticancer effect by attenuating the pro-inflammatory cytokines. J. Biochem. Mol. Toxicol. 35, 1–8. doi:10.1002/jbt.22759
Putila, J. J., and Guo, N. L. (2011). Association of arsenic exposure with lung cancer incidence rates in the United States. PLoS ONE 6, e25886. doi:10.1371/journal.pone.0025886
Rogart, J. N., Barrach, H.-J., and Chichester, C. O. (1999). Articular collagen degradation in the Hulth-Telhag model of osteoarthritis. Osteoarthr. Cartil. 7, 539–547. doi:10.1053/joca.1999.0258
Rosetti, F., Madera-Salcedo, I. K., Rodríguez-Rodríguez, N., and Crispín, J. C. (2022). Regulation of activated T cell survival in rheumatic autoimmune diseases. Nat. Rev. Rheumatol. 18, 232–244. doi:10.1038/s41584-021-00741-9
Saadat, S., Noureddini, M., Mahjoubin-Tehran, M., Nazemi, S., Shojaie, L., Aschner, M., et al. (2021). Pivotal role of TGF-β/smad signaling in cardiac fibrosis: non-coding RNAs as effectual players. Front. Cardiovasc. Med. 7, 588347. doi:10.3389/fcvm.2020.588347
Shaw, P., and Chattopadhyay, A. (2020). Nrf2–ARE signaling in cellular protection: mechanism of action and the regulatory mechanisms. J. Cell. Physiol. 235, 3119–3130. doi:10.1002/jcp.29219
Shen, L., Zhou, T., Wang, J., Sang, X., Lan, L., Luo, L., et al. (2017). Daphnetin reduces endotoxin lethality in mice and decreases LPS-induced inflammation in Raw264.7 cells via suppressing JAK/STATs activation and ROS production. Inflamm. Res. 66, 579–589. doi:10.1007/s00011-017-1039-1
Shimizu, H., Sakimoto, T., and Yamagami, S. (2019). Pro-inflammatory role of NLRP3 inflammasome in experimental sterile corneal inflammation. Sci. Rep. 9, 9596. doi:10.1038/s41598-019-46116-9
Shu, K., Kuang, N., Zhang, Z., Hu, Z., Zhang, Y., Fu, Y., et al. (2014). Therapeutic effect of daphnetin on the autoimmune arthritis through demethylation of proapoptotic genes in synovial cells. J. Transl. Med. 12, 287. doi:10.1186/s12967-014-0287-x
Soltanmohammadi, A., Tavaf, M. J., Zargarani, S., Yazdanpanah, E., Sadighi-Moghaddam, B., Yousefi, B., et al. (2022). Daphnetin alleviates experimental autoimmune encephalomyelitis by suppressing Th1 and Th17 cells and upregulating Th2 and regulatory T cells. Acta Neurobiol. Exp. (Warsz.) 82 (3), 273–283. doi:10.55782/ane-2022-026
Song, B., Wang, Z., Liu, Y., Xu, S., Huang, G., Xiong, Y., et al. (2014). Immunosuppressive activity of daphnetin, one of coumarin derivatives, is mediated through suppression of NF-κB and NFAT signaling pathways in mouse T cells. PloS One 9, e96502. doi:10.1371/journal.pone.0096502
Stakheev, D., Taborska, P., Strizova, Z., Podrazil, M., Bartunkova, J., and Smrz, D. (2019). The WNT/β-catenin signaling inhibitor XAV939 enhances the elimination of LNCaP and PC-3 prostate cancer cells by prostate cancer patient lymphocytes in vitro. Sci. Rep. 9, 4761. doi:10.1038/s41598-019-41182-5
Su, C., Hu, S., Sun, Y., Zhao, J., Dai, C., Wang, L., et al. (2019). Myostatin induces tumor necrosis factor-α expression in rheumatoid arthritis synovial fibroblasts through the PI3K–Akt signaling pathway. J. Cell. Physiol. 234, 9793–9801. doi:10.1002/jcp.27665
Su, J., Morgani, S. M., David, C. J., Wang, Q., Er, E. E., Huang, Y.-H., et al. (2020). TGF-β orchestrates fibrogenic and developmental EMTs via the RAS effector RREB1. Nature 577, 566–571. doi:10.1038/s41586-019-1897-5
Sultana, R., Wang, X., Azeem, M., Hussain, T., Mahmood, A., Fiaz, S., et al. (2022). Coumarin-mediated growth regulations, antioxidant enzyme activities, and photosynthetic efficiency of sorghum bicolor under saline conditions. Front. Plant Sci. 13, 799404. doi:10.3389/fpls.2022.799404
Sun, S.-C. (2017). The non-canonical NF-κB pathway in immunity and inflammation. Nat. Rev. Immunol. 17, 545–558. doi:10.1038/nri.2017.52
Syed, A. M., Kundu, S., Ram, C., Kulhari, U., Kumar, A., Mugale, M. N., et al. (2022). Up-regulation of Nrf2/HO-1 and inhibition of TGF-β1/Smad2/3 signaling axis by daphnetin alleviates transverse aortic constriction-induced cardiac remodeling in mice. Free Radic. Biol. Med. 186, 17–30. doi:10.1016/j.freeradbiomed.2022.04.019
Torrente, L., and DeNicola, G. M. (2022). Targeting NRF2 and its downstream processes: opportunities and challenges. Annu. Rev. Pharmacol. Toxicol. 62, 279–300. doi:10.1146/annurev-pharmtox-052220-104025
Toulany, M., Maier, J., Iida, M., Rebholz, S., Holler, M., Grottke, A., et al. (2017). Akt1 and Akt3 but not Akt2 through interaction with DNA-PKcs stimulate proliferation and post-irradiation cell survival of K-RAS-mutated cancer cells. Cell Death Discov. 3, 17072. doi:10.1038/cddiscovery.2017.72
Tu, L., Li, S., Fu, Y., Yao, R., Zhang, Z., Yang, S., et al. (2012). The therapeutic effects of daphnetin in collagen-induced arthritis involve its regulation of Th17 cells. Int. Immunopharmacol. 13, 417–423. doi:10.1016/j.intimp.2012.04.001
Turner, M. D., Nedjai, B., Hurst, T., and Pennington, D. J. (2014). Cytokines and chemokines: at the crossroads of cell signalling and inflammatory disease. Biochim. Biophys. Acta BBA - Mol. Cell Res. 1843, 2563–2582. doi:10.1016/j.bbamcr.2014.05.014
Vanhaesebroeck, B., Guillermet-Guibert, J., Graupera, M., and Bilanges, B. (2010). The emerging mechanisms of isoform-specific PI3K signalling. Nat. Rev. Mol. Cell Biol. 11, 329–341. doi:10.1038/nrm2882
Vidal, S., Bouzaher, Y. H., El Motiam, A., Seoane, R., and Rivas, C. (2022). Overview of the regulation of the class IA PI3K/AKT pathway by SUMO. Semin. Cell Dev. Biol. 132, 51–61. doi:10.1016/j.semcdb.2021.10.012
Wang, C.-W., Chiou, H.-Y. C., Chen, S.-C., Wu, D.-W., Lin, H.-H., Chen, H.-C., et al. (2023). Arsenic exposure and lung fibrotic changes-evidence from a longitudinal cohort study and experimental models. Front. Immunol. 14, 1225348. doi:10.3389/fimmu.2023.1225348
Wang, D., Lu, Z., Zhang, H., Jin, S.-F., Yang, H., Li, Y.-M., et al. (2016). Daphnetin alleviates experimental autoimmune encephalomyelitis via regulating dendritic cell activity. CNS Neurosci. Ther. 22, 558–567. doi:10.1111/cns.12537
Wang, P., Xia, Y.-L., Zou, L.-W., Qian, X.-K., Dou, T.-Y., Jin, Q., et al. (2017). An optimized two-photon fluorescent probe for biological sensing and imaging of catechol-O-methyltransferase. Chem. Weinh. Bergstr. Ger. 23, 10800–10807. doi:10.1002/chem.201701384
Wang, Q., Sun, Y., Fan, R., Wang, M., Ren, C., Jiang, A., et al. (2022). Role of inflammatory factors in the etiology and treatment of recurrent implantation failure. Reprod. Biol. 22, 100698. doi:10.1016/j.repbio.2022.100698
Wang, Y., Chen, J., Chen, J., Dong, C., Yan, X., Zhu, Z., et al. (2020a). Daphnetin ameliorates glucocorticoid-induced osteoporosis via activation of Wnt/GSK-3β/β-catenin signaling. Toxicol. Appl. Pharmacol. 409, 115333. doi:10.1016/j.taap.2020.115333
Wang, Y., Wang, J., Fu, Z., Sheng, R., Wu, W., Fan, J., et al. (2020b). Syntheses and evaluation of daphnetin derivatives as novel G protein-coupled receptor inhibitors and activators. Bioorg. Chem. 104, 104342. doi:10.1016/j.bioorg.2020.104342
Weng, W., Liu, Y., Hu, Z., Li, Z., Peng, X., Wang, M., et al. (2023). Macrophage extracellular traps promote tumor-like biologic behaviors of fibroblast-like synoviocytes through cGAS-mediated PI3K/Akt signaling pathway in patients with rheumatoid arthritis. J. Leukoc. Biol. 115, 116–129. doi:10.1093/jleuko/qiad102
Woolbright, B. L., and Jaeschke, H. (2017). Role of the inflammasome in acetaminophen-induced liver injury and acute liver failure. J. Hepatol. 66, 836–848. doi:10.1016/j.jhep.2016.11.017
Wróblewska-Łuczka, P., Góralczyk, A., and Łuszczki, J. J. (2023). Daphnetin, a coumarin with anticancer potential against human melanoma: in vitro study of its effective combination with selected cytostatic drugs. Cells 12, 1593. doi:10.3390/cells12121593
Xia, P., Gao, X., Shao, L., Chen, Q., Li, F., Wu, C., et al. (2019). Down-regulation of RAC2 by small interfering RNA restrains the progression of osteosarcoma by suppressing the Wnt signaling pathway. Int. J. Biol. Macromol. 137, 1221–1231. doi:10.1016/j.ijbiomac.2019.07.016
Yaghi, S., Shu, L., Bakradze, E., Salehi Omran, S., Giles, J. A., Amar, J. Y., et al. (2022). Direct oral anticoagulants versus warfarin in the treatment of cerebral venous thrombosis (ACTION-CVT): a multicenter international study. Stroke 53, 728–738. doi:10.1161/STROKEAHA.121.037541
Yamamoto, H., Zhang, S., and Mizushima, N. (2023). Autophagy genes in biology and disease. Nat. Rev. Genet. 24, 382–400. doi:10.1038/s41576-022-00562-w
Yan, L., Jin, Y., Pan, J., He, X., Zhong, S., Zhang, R., et al. (2022). 7,8-Dihydroxycoumarin alleviates synaptic loss by activated PI3K-Akt-CREB-BDNF signaling in Alzheimer’s disease model mice. J. Agric. Food Chem. 70, 7130–7138. doi:10.1021/acs.jafc.2c02140
Yang, E. B., Zhao, Y. N., Zhang, K., and Mack, P. (1999). Daphnetin, one of coumarin derivatives, is a protein kinase inhibitor. Biochem. Biophys. Res. Commun. 260, 682–685. doi:10.1006/bbrc.1999.0958
Yang, S., Song, Y., Wang, Q., Liu, Y., Wu, Z., Duan, X., et al. (2021). Daphnetin ameliorates acute lung injury in mice with severe acute pancreatitis by inhibiting the JAK2–STAT3 pathway. Sci. Rep. 11, 11491. doi:10.1038/s41598-021-91008-6
Yao, B., Yang, Q., Yang, Y., Li, Y., Peng, H., Wu, S., et al. (2021). Screening for active compounds targeting human natural killer cell activation identifying daphnetin as an enhancer for IFN-γ production and direct cytotoxicity. Front. Immunol. 12, 680611. doi:10.3389/fimmu.2021.680611
Yao, Q., Wu, X., Tao, C., Gong, W., Chen, M., Qu, M., et al. (2023). Osteoarthritis: pathogenic signaling pathways and therapeutic targets. Signal Transduct. Target. Ther. 8, 56. doi:10.1038/s41392-023-01330-w
Yao, R., Fu, Y., Li, S., Tu, L., Zeng, X., and Kuang, N. (2011). Regulatory effect of daphnetin, a coumarin extracted from Daphne odora, on the balance of Treg and Th17 in collagen-induced arthritis. Eur. J. Pharmacol. 670, 286–294. doi:10.1016/j.ejphar.2011.08.019
Yin, X., Chen, S., and Eisenbarth, S. C. (2021). Dendritic cell regulation of T helper cells. Annu. Rev. Immunol. 39, 759–790. doi:10.1146/annurev-immunol-101819-025146
Yu, H., Lin, L., Zhang, Z., Zhang, H., and Hu, H. (2020). Targeting NF-κB pathway for the therapy of diseases: mechanism and clinical study. Signal Transduct. Target. Ther. 5, 209. doi:10.1038/s41392-020-00312-6
Yu, W., Lu, Z., Zhang, H., Kang, Y., Mao, Y., Wang, H., et al. (2014). Anti-inflammatory and protective properties of daphnetin in endotoxin-induced lung injury. J. Agric. Food Chem. 62, 12315–12325. doi:10.1021/jf503667v
Zeng, C., Avula, S. R., Meng, J., and Zhou, C. (2023). Synthesis and biological evaluation of piperazine hybridized coumarin indolylcyanoenones with antibacterial potential. Mol. Basel Switz. 28, 2511. doi:10.3390/molecules28062511
Zhang, L., Gu, Y., Li, H., Cao, H., Liu, B., Zhang, H., et al. (2018). Daphnetin protects against cisplatin-induced nephrotoxicity by inhibiting inflammatory and oxidative response. Int. Immunopharmacol. 65, 402–407. doi:10.1016/j.intimp.2018.10.018
Zhang, Q., Lenardo, M. J., and Baltimore, D. (2017). 30 Years of NF-κB: a blossoming of relevance to human pathobiology. Cell 168, 37–57. doi:10.1016/j.cell.2016.12.012
Zhang, T., Liang, W., Zhang, M., Cui, S., Huang, X., Ou, W., et al. (2023). Daphnetin improves neuropathic pain by inhibiting the expression of chemokines and inflammatory factors in the spinal cord and interfering with glial cell polarization. Pharmaceuticals 16, 243. doi:10.3390/ph16020243
Zhang, W., Zhuo, S., He, L., Cheng, C., Zhu, B., Lu, Y., et al. (2019). Daphnetin prevents methicillin-resistant Staphylococcus aureus infection by inducing autophagic response. Int. Immunopharmacol. 72, 195–203. doi:10.1016/j.intimp.2019.04.007
Zhang, X., Yao, J., Wu, Z., Zou, K., Yang, Z., Huang, X., et al. (2020a). Chondroprotective and antiarthritic effects of Daphnetin used in vitro and in vivo osteoarthritis models. Life Sci. 240, 116857. doi:10.1016/j.lfs.2019.116857
Zhang, Y., Qu, L., Sun, Y., Lin, Y., Zeng, J., He, L., et al. (2022). Daphnetin contributes to allergen-induced Th2 cytokine expression and type 2-immune responses in atopic dermatitis and asthma. Food Funct. 13, 12383–12399. doi:10.1039/d2fo02518c
Zhang, Y., Xu, Y., Xu, T., Yin, H., Zhu, Y., Peng, B., et al. (2020b). Prediction of long-term disability in Chinese patients with multiple sclerosis: a prospective cohort study. Mult. Scler. Relat. Disord. 46, 102461. doi:10.1016/j.msard.2020.102461
Zhang, Z., Long, S., Huang, Z., Tan, J., Wu, Q., and Huang, O. (2020c). Regulatory effect of daphnetin on the balance of Th17 and Treg cells in the peripheral blood mononuclear cells from patients with unexplained recurrent pregnancy loss. Cent. Eur. J. Immunol. 45, 403–408. doi:10.5114/ceji.2020.103414
Zhi, J., Duan, B., Pei, J., Wu, S., and Wei, J. (2019). Daphnetin protects hippocampal neurons from oxygen-glucose deprivation-induced injury. J. Cell. Biochem. 120, 4132–4139. doi:10.1002/jcb.27698
Keywords: daphnetin, molecular effects, signaling pathways, NLRP3 inflammasome, inflammatory factors
Citation: Wei Z, Wei N, Su L and Gao S (2024) The molecular effects underlying the pharmacological activities of daphnetin. Front. Pharmacol. 15:1407010. doi: 10.3389/fphar.2024.1407010
Received: 26 March 2024; Accepted: 13 June 2024;
Published: 01 July 2024.
Edited by:
Karim Hosni, Institut National de Recherche et d’Analyse Physico-Chimique (INRAP), TunisiaReviewed by:
Xinghua Gao, China Pharmaceutical University, ChinaYali Ren, Chengdu University of Traditional Chinese Medicine, China
Di Pan, Guizhou Medical University, China
Copyright © 2024 Wei, Wei, Su and Gao. This is an open-access article distributed under the terms of the Creative Commons Attribution License (CC BY). The use, distribution or reproduction in other forums is permitted, provided the original author(s) and the copyright owner(s) are credited and that the original publication in this journal is cited, in accordance with accepted academic practice. No use, distribution or reproduction is permitted which does not comply with these terms.
*Correspondence: Sujun Gao, sjgao@jlu.edu.cn